Chapter19 Integrating cells into tissues---PPT (1)
Chapter 3 -Li- Cell surface
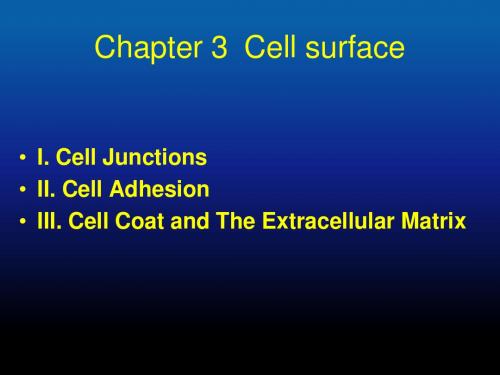
3. Communicating junctions a. gap junctions b. chemical synapses c. plasmodesmata (plants only)
Figure 19-1 Simplified drawing of a cross-section through part of the wall of the Small intestine. This long, tubelike organ is
conventional (C) electron micrographs. Note that the cells are oriented with their apical ends down. In (B) the plane of the micrograph is parallel to the plane of the membrane, and the tight junction appears as a beltlike band of anastomosing sealing strands that encircle each cell in the sheet. The sealing strands are seen as ridges of intramembrane particles on the cytoplasmic fracture face of the membrane (the P face) or as complementary grooves on the external face of the membrane (the E face) (see Figure 3-5). In (C) the junction is seen as a series of focal connections between the outer leaflets of the two interacting plasma membranes, each connection corresponding to a sealing strand in cross-section. (B and C, from N.B. Gilula, in Cell Communication [R.P. Cox,
最新ICH指导原则
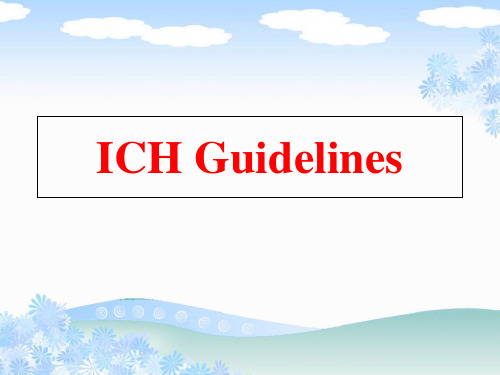
ICH Guidelines
说明
ICH的论题主要分为四类,因此ICH根据论题的类别不同而进行相应的编 码分类:
1. “Q”类论题:Q代表QUALITY,指那些与化工和医药,质量保证方面 的相关的论题。
2. “S”类论题:S代表SAFETY,指那些与实验室和动物实验,临床前研 究方面的相关的论题。
Q7 Good Manufacturing Practice Guide for Active Pharmaceutical Ingredients活性药 物成份的GMP指南
Q7 Q&As Questions and Answers: Good Manufacturing Practice Guide for Active Pharmaceutical Ingredients
Changes in their Manufacturing Process 基于不同生产工艺的生物技术产品/生物产品的可比较性
Q6A- Q6B Specifications规格
Q6A Specifications : Test Procedures and Acceptance Criteria for New Drug Substances and New Drug Products: Chemical Substances质量规格:新原料 药和新制剂的检验程序和可接收标准:化学物质( 包括决定过程)
Q4B Annex 4CR1 Microbiological Examination of NonSterile Products: Acceptance Criteria for Pharmaceutical Preparations and Substances for Pharmaceutical Use General Chapter
B淋巴细胞活化
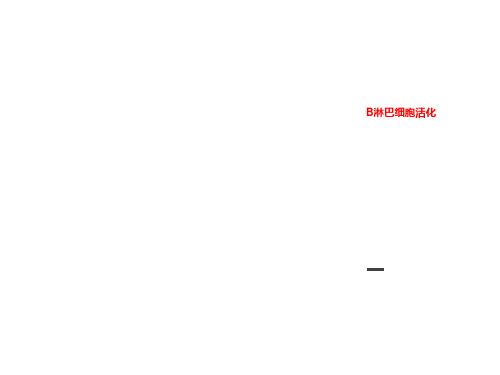
记忆性细胞
somatic hypermutation & Affinity Maturation
Five BCR: • all specific for • different affinities
BB
B B
TB
B
3. T cells proliferate
BB BT T
B TTT BB
4. B cells migrate through
HEV - most pass through the B paracortex and primary follicle.
formation
T
DC
T
T
2. T cells migrate through HEV and are trapped by antigen on DC
• 初次应答与再次应答的比较:
初次应答
再次应答
抗原
TD或TI抗原 TD抗原
潜伏期
长
短
对数期
升高较慢
升高较快
平台期
时间短,低 维持时间长,高
下降期
较短
较长
抗体类型
IgM为主
IgG为主
抗体亲和力 低
高
二、再次应答的机制
• 潜伏期短
– 记忆细胞数量远大于初始细胞 – 记忆细胞已经致敏 – 记忆T表达高水平黏附分子,更易被APC激活
NEUTRALISATION
Bacterium
Toxin
Adhesion to host cells blocked
Invitrogen Neon
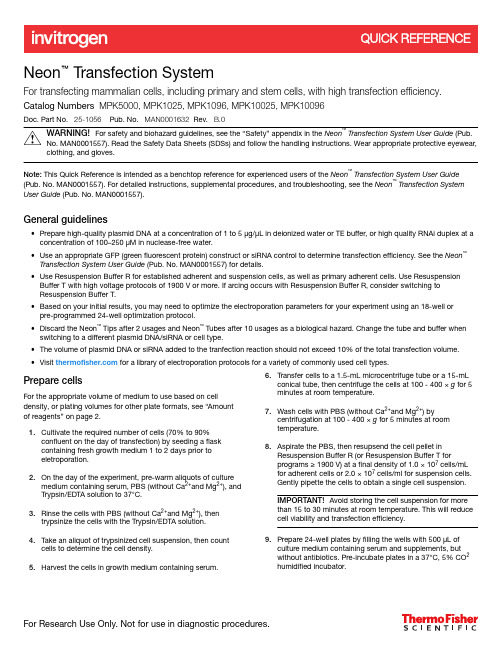
Neon™ Transfection SystemFor transfecting mammalian cells, including primary and stem cells, with high transfection efficiency. Catalog Numbers MPK5000, MPK1025, MPK1096, MPK10025, MPK10096Doc. Part No. 25-1056 Pub. No. MAN0001632 Rev.B.0WARNING! For safety and biohazard guidelines, see the “Safety” appendix in the Neon™ Transfection System User Guide (Pub.No. MAN0001557). Read the Safety Data Sheets (SDSs) and follow the handling instructions. Wear appropriate protective eyewear, clothing, and gloves.Note: This Quick Reference is intended as a benchtop reference for experienced users of the Neon™ Transfection System User Guide (Pub. No. MAN0001557). For detailed instructions, supplemental procedures, and troubleshooting, see the Neon™ Transfection System User Guide (Pub. No. MAN0001557).General guidelines•Prepare high-quality plasmid DNA at a concentration of 1 to 5 μg/μL in deionized water or TE buffer, or high quality RNAi duplex at a concentration of 100–250 μM in nuclease-free water.•Use an appropriate GFP (green fluorescent protein) construct or siRNA control to determine transfection efficiency. See the Neon™Transfection System User Guide (Pub. No. MAN0001557) for details.•Use Resuspension Buffer R for established adherent and suspension cells, as well as primary adherent cells. Use Resuspension Buffer T with high voltage protocols of 1900 V or more. If arcing occurs with Resuspension Buffer R, consider switching toResuspension Buffer T.•Based on your initial results, you may need to optimize the electroporation parameters for your experiment using an 18-well or pre-programmed 24-well optimization protocol.•Discard the Neon™ Tips after 2 usages and Neon™ Tubes after 10 usages as a biological hazard. Change the tube and buffer when switching to a different plasmid DNA/siRNA or cell type.•The volume of plasmid DNA or siRNA added to the tranfection reaction should not exceed 10% of the total transfection volume.•Visit for a library of electroporation protocols for a variety of commonly used cell types.Prepare cellsFor the appropriate volume of medium to use based on cell density, or plating volumes for other plate formats, see “Amount of reagents” on page 2.1.Cultivate the required number of cells (70% to 90%confluent on the day of transfection) by seeding a flaskcontaining fresh growth medium 1 to 2 days prior toeletroporation.2.On the day of the experiment, pre-warm aliquots of culturemedium containing serum, PBS (without Ca2+and Mg2+), and Trypsin/EDTA solution to 37°C.3.Rinse the cells with PBS (without Ca2+and Mg2+), thentrypsinize the cells with the Trypsin/EDTA solution.4.Take an aliquot of trypsinized cell suspension, then countcells to determine the cell density.5.Harvest the cells in growth medium containing serum.6.Transfer cells to a 1.5-mL microcentrifuge tube or a 15-mLconical tube, then centrifuge the cells at 100 - 400 × g for 5 minutes at room temperature.7.Wash cells with PBS (without Ca2+and Mg2+) bycentrifugation at 100 - 400 × g for 5 minutes at roomtemperature.8.Aspirate the PBS, then resupsend the cell pellet inResuspension Buffer R (or Resuspension Buffer T forprograms ≥ 1900 V) at a final density of 1.0 × 107 cells/mL for adherent cells or 2.0 × 107 cells/ml for suspension cells.Gently pipette the cells to obtain a single cell suspension.IMPORTANT! Avoid storing the cell suspension for more than 15 to 30 minutes at room temperature. This will reduce cell viability and transfection efficiency.9.Prepare 24-well plates by filling the wells with 500 μL ofculture medium containing serum and supplements, butwithout antibiotics. Pre-incubate plates in a 37°C, 5% CO2 humidified incubator.Amount of reagentsFor each electroporation sample, the amount of plasmid DNA/siRNA, cell number, and volume of plating medium per well are listed in the following table. Use Resuspension Buffer T for cell types that require high voltage protocols of 1900 V or more. For all other cell types, use Resuspension Buffer R.[1]Use Resuspension Buffer T for primary suspension blood cells.Using the Neon ™Transfection SystemFor details on setting up the Neon ™device and Neon ™PipetteStation, see the Neon ™Transfection System User Guide (Pub. No.MAN0001557).1.Select the appropriate protocol for your cell type. Use one ofthe following options:•Input the electroporation parameters in the Input window if you already have the electroporation parameters for your cell type.•Tap Database , then select the cell-specificelectroporation parameters that you have added for various cell types.•Tap Optimization to perform the optimization protocol for your cell type.2.Fill the Neon ™Tube with 3 mL of Electrolytic Buffer (useBuffer E for the 10 μL Neon ™Tip and Buffer E2 for the 100μL Neon ™Tip).Note: Make sure that the electrode on the side of the tube is completely immersed in buffer. 3.Insert the Neon ™ Tube into the Neon ™Pipette Station untilyou hear a click sound (Figure 1).Figure 1 Schematic of Neon ™ Tube and Neon ™ Pipette Station.4.Transfer the appropriate amount of plasmid DNA/siRNA intoa sterile, 1.5 mL microcentrifuge tube.5.Add cells to the tube containing plasmid DNA/siRNA, thengently mix. See “Amount of reagents” on page 2 for cell number, DNA/siRNA amount, and plating volumes to use.6.To insert a Neon ™Tip into the Neon ™Pipette, press thepush-button on the pipette to the second stop to open the clamp.7.Insert the top-head of the Neon ™Pipette into the Neon ™Tipuntil the clamp fully picks up the mount stem of the piston (Figure 2).Figure 2 Schematic of Neon ™ Pipette and Neon ™Tip.8.Gently release the push-button, continuing to apply adownward pressure on the pipette, ensuring that the tip is sealed onto the pipette without any gaps.9.Press the push-button on the Neon ™Pipette to the first stopand immerse the Neon ™Tip into the cell-DNA/siRNA mixture.Slowly release the push-button on the pipette to aspirate thecell-DNA/siRNA mixture into the Neon ™Tip (Figure 3).Figure 3 Schematic of Neon ™Tip.Note: Avoid air bubbles during pipetting as air bubbles cause arcing during electroporation leading to lowered or failed transfection. If you notice air bubbles in the tip,discard the sample, then carefully aspirate the fresh sample into the tip again without any air bubbles.10.Insert the Neon ™Pipette with the sample vertically into theNeon ™ Tube placed in the Neon ™Pipette Station until youhear a click sound (Figure 4).Figure 4 Schematic of Neon ™ Tube and Neon ™ Pipette Station.Note: Ensure that the metal head of the Neon ™pipette projection is inserted into the groove of the pipette station.11.Ensure that you have selected the appropriateelectroporation protocol, then press Start on the touchscreen.12.The Neon ™device automatically checks for the properinsertion of the Neon ™ Tube and Neon ™Pipette before delivering the electric pulse.13.After delivering the electric pulse, Complete is displayed onthe touchscreen to indicate that electroporation is complete.14.Slowly remove the Neon ™Pipette from the Neon ™PipetteStation. Immediately transfer the samples from the Neon ™Tip by pressing the push-button on the pipette to the first stop into the prepared culture plate containing prewarmed medium with serum and supplements but without antibiotics.Note: Discard the Neon ™ Tip into an appropriate biologicalhazardous waste container. To discard the Neon ™Tip, press the push-button to the second stop into an appropriate biological hazardous waste container.15.Repeat step 6 to step 14 for the remaining samples.Note: Be sure to change the Neon ™Tips after using it twiceand Neon ™ Tubes after 10 usages. Use a new Neon ™Tip andNeon ™Tube for each new plasmid DNA sample.16.Gently rock the plate to ensure even distribution of the cells.Incubate the plate at 37℃ in a humidified CO 2 incubator.17.If you are not using the Neon ™device, turn the power switchon the rear to OFF .18.Assay samples to determine the transfection efficiency(e.g., fluorescence microscopy or functional assay) or geneknockdown (for siRNA).19.Based on your initial results, you may need to optimizedthe electroporation parameters for your cell type. For more information, see the Neon™ Transfection System User Guide (Pub. No. MAN0001557).Life Technologies Corporation | 5781 Van Allen Way | Carlsbad, California 92008 USAFor descriptions of symbols on product labels or product documents, go to /symbols-definition.The information in this guide is subject to change without notice.DISCLAIMER: TO THE EXTENT ALLOWED BY LAW, THERMO FISHER SCIENTIFIC INC. AND/OR ITS AFFILIATE(S) WILL NOT BE LIABLE FOR SPECIAL, INCIDENTAL, INDIRECT, PUNITIVE, MULTIPLE, OR CONSEQUENTIAL DAMAGES IN CONNECTION WITH OR ARISING FROM THIS DOCUMENT, INCLUDING YOUR USE OF IT.Important Licensing Information: These products may be covered by one or more Limited Use Label Licenses. By use of these products, you accept the terms and conditions of all applicable Limited Use Label Licenses.©2021 Thermo Fisher Scientific Inc. All rights reserved. All trademarks are the property of Thermo Fisher Scientific and its subsidiaries unless otherwise specified./support | /askaquestion。
Regulation of epithelial cell organization by tuning cell–substrate adhesion
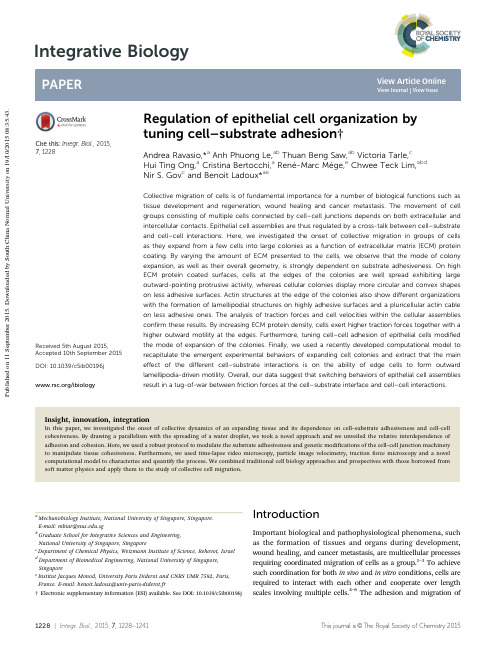
Cite this:Integr.Biol.,2015,7,1228Regulation of epithelial cell organization by tuning cell–substrate adhesion †Andrea Ravasio,*a Anh Phuong Le,ab Thuan Beng Saw,ab Victoria Tarle,cHui Ting Ong,a Cristina Bertocchi,a Rene ´-Marc Me `ge,e Chwee Teck Lim,abdNir c and Benoit Ladoux*aeCollective migration of cells is of fundamental importance for a number of biological functions such as tissue development and regeneration,wound healing and cancer metastasis.The movement of cell groups consisting of multiple cells connected by cell–cell junctions depends on both extracellular and intercellular contacts.Epithelial cell assemblies are thus regulated by a cross-talk between cell–substrate and cell–cell interactions.Here,we investigated the onset of collective migration in groups of cells as they expand from a few cells into large colonies as a function of extracellular matrix (ECM)protein coating.By varying the amount of ECM presented to the cells,we observe that the mode of colony expansion,as well as their overall geometry,is strongly dependent on substrate adhesiveness.On high ECM protein coated surfaces,cells at the edges of the colonies are well spread exhibiting large outward-pointing protrusive activity,whereas cellular colonies display more circular and convex shapes on less adhesive surfaces.Actin structures at the edge of the colonies also show different organizations with the formation of lamellipodial structures on highly adhesive surfaces and a pluricellular actin cable on less adhesive ones.The analysis of traction forces and cell velocities within the cellular assemblies confirm these results.By increasing ECM protein density,cells exert higher traction forces together with a higher outward motility at the edges.Furthermore,tuning cell–cell adhesion of epithelial cells modifiedthe mode of expansion of the colonies.Finally,we used a recently developed computational model to recapitulate the emergent experimental behaviors of expanding cell colonies and extract that the main effect of the different cell–substrate interactions is on the ability of edge cells to form outward lamellipodia-driven motility.Overall,our data suggest that switching behaviors of epithelial cell assemblies result in a tug-of-war between friction forces at the cell–substrate interface and cell–cell interactions.IntroductionImportant biological and pathophysiological phenomena,such as the formation of tissues and organs during development,wound healing,and cancer metastasis,are multicellular processes requiring coordinated migration of cells as a group.1–3To achieve such coordination for both in vivo and in vitro conditions,cells are required to interact with each other and cooperate over length scales involving multiple cells.4–6The adhesion and migrationofaMechanobiology Institute,National University of Singapore,Singapore.E-mail:mbiar@.sg bGraduate School for Integrative Sciences and Engineering,National University of Singapore,Singapore cDepartment of Chemical Physics,Weizmann Institute of Science,Rehovot,Israel dDepartment of Biomedical Engineering,National University of Singapore,Singapore eInstitut Jacques Monod,University Paris Diderot and CNRS UMR 7592,Paris,France.E-mail:doux@univ-paris-diderot.fr†Electronic supplementary information (ESI)available.See DOI:10.1039/c5ib00196jReceived 5th August 2015,Accepted 10th September 2015DOI:10.1039/c5ib00196j/ibiologyIntegrative BiologyP u b l i s h e d o n 11 S e p t e m b e r 2015. D o w n l o a d e d b y S o u t h C h i n a N o r m a l U n i v e r s i t y o n 19/10/2015 08:35:43.single cells on 2D substrates rich in ECM require heterophilic adhesion to the substrate at the site of specialized protein complexes.7This allows cells to stabilize their protrusions,such as lamellipodia,and exert forces onto the substrate to propel forward in a mechanism termed cell crawling.This motility mode involves the protrusion of lamellipodia at the leading edge and adhesion to ECM proteins such as fibronectin (FN)by transmembrane proteins (e.g.integrins).Based on this model,single cell adhesion and migration have been shown to depend on cell–matrix interactions including ECM adhesiveness and ligand density.8,9Besides this well-established mode of migration,one additional major mechanism is relevant to cell translocation within tissues:the movement of cell groups,sheets,or strands consisting of multiple cells connected by cell–cell junctions.10The growth and migration of cell clusters over 2D surfaces also display similar types of protrusions at the edges,their cohesiveness being maintained by cell–cell junctions (CCJs).11This cooperation thus relies on different types of interactions at the cell–matrix and cell–cell interfaces.Indeed,the organization of multicellular assemblies in 2D and 3D environments has been shown to depend on the relative strength of these interactions,which may be explained by a cross-talk between cell–matrix and cell–cell adhesions.12–15In epithelial cells,adherens junctions through homophilic interactions between E-cadherin proteins have been shown to be crucial to understand forces transmitted from one cell to its neighbors and consequently tissue cohesion.16–19During tissue migration and growth,CCJs experience various intercellular stresses that include compressive,tensile and shear stresses.20–23Various experimental and theoretical models have been described to explain multicellular movements including contact inhibition of locomotion,24agent-based models 25–27and continuum multicellular approaches.28–30These models include the description of bulk cellular motions through cell–substrate interactions and intercellular tension for a continuous mono-layer.29,31,32However,in many cases including wound healing,gap closure and also morphogenetic movements,epithelial tissues present discontinuities.In such situations,cellular move-ments and tension at the free edge,together with bulk cellular rearrangements,largely contribute to tissue dynamics.4,27,29,33–38In this scenario that includes either epithelial expansion or gap closure into cell-free areas,collective migration is aided by the protrusive activity of leading cells.21,39Such cells are able to pull adjacent cells along and form finger-like structures.As such,the colonies’edge presents fingering patterns 40with protrusive activity and actomyosin cables at the rear.Interestingly,such contractile actomyosin cables play a major role during epithelial gap closure 34–36,41,42and are prominent in concave regions during tissue migration or wound closure.The assembly of actomyosin contractile cables plays an opposite role at convex regions where it limits the expansion of the tissue.34,36,43Taken together,the above considerations draw a complex picture of collective cell dynamics during epithelial gap closure,wound healing or tissue expansion driven by cell proliferation and migration,that strongly depend on both biochemical and physical environmental factors.5,34,44–47Cohesive forces acting at the CCJ,19,48as well as the tension exerted by supracellular actomyosincables at the cell edge,36play a role in the maintenance of colony cohesion.Meanwhile,adhesiveness to the substrate and traction forces acting onto it through focal adhesions (FAs)seems to work in the opposite way to modify cell polarity and expand the tissue.39,49This process is somewhat reminiscent of substrate wetting phenomena by liquid droplets that can be described by the relative contributions of adhesive properties of the liquids versus cohesive properties.50Such an analogy has indeed been used to describe the spreading of cell aggregates.51Even though single cell responses,including their signaling and mechanical properties,have been shown to be tightly coupled to the geometrical,chemical and physical interactions with the underlying substrate,52–55little is known about the coupling between substrate properties and regulation of colony shape,tissue expansion and migration for multicellular assemblies.Here,we investigate the emerging behaviors of expanding epithelial Madin–Darby canine kidney (MDCK)colonies in response to various substrate ECM coatings to vary cell–substrate interactions and thus determine their impact on tissue organization.Results and discussionVarying the amount of FN substrate coating leads to different degrees of spreading of epithelial cells colonies,in analogy to the partial wetting of water dropletsPrevious studies reported that spreading of cell aggregates 56as well as the coalescence of cells 57on varying ECM over short time scales (o 10h)can be described by two parameters,i.e.the cohesive (W cohesion ;W c )and adhesive (W adhesion ;W a )energy of the cells.In order to alter the adhesive properties of the cells on the substrate,thus changing W a ,we coated glass-bottom petri dishes (borosilicate)with varying amounts of FN.Fluorescence images revealed that 80m g ml À1provided for ample surface coating,whereas 5m g ml À1showed only limited covering and 0m g ml À1no coating (Fig.1A).In all cases,we observed an overall homogenous protein coating of the substrates with inhomogeneities being much smaller than the cell size (Fig.S1,ESI †).Importantly,surface coating did not markedly change after being submerged in culture medium for 5days and in the presence of cells.Furthermore,the cells did not significantly change the amount or the homogeneity of the protein coating.Under these conditions,we observed that small colonies of epithelial cells originating from a few cells (o 2)and grown for three days adopted diverse shapes in response to different amounts of FN coating (Fig.1B).In particular,the overall aspect ratio of the cell colonies varied in all dimensions.Colonies on high FN (80m g ml À1)grew into large,flat and anisotropic patches,whereas those on low FN (5m g ml À1)were rather round,small and tall.Even more dramatically,colonies tended to form three-dimensional spheroids in the absence of FN (0m g ml À1).Interestingly,the contact angle between the cell patches and the substrate also changes as a function of the FN coating (Fig.1B,x /z images),in a manner highly reminiscent to the partial wetting of droplets on surfaces with varying affinities for the fluid.In the case of the droplet,the contact angle (y )is a direct measure for thePaper Integrative BiologyP u b l i s h e d o n 11 S e p t e m b e r 2015. D o w n l o a d e d b y S o u t h C h i n a N o r m a l U n i v e r s i t y o n 19/10/2015 08:35:43.wettability parameter,S =W a ÀW c ;with W a o W c and S o 0for partial wetting.The more negative S is,the less the droplet spreads,thus giving a larger y .In our experiments,small y values were measured for colonies provided with high adhesion (80m g ml À1)and large y values (>901)for those deprived of it (0m g ml À1)(Fig.1C).This indicates that increased FN concen-tration increases W a and results in a less negative S (smaller y ).Under this condition,colonies spread into patches of larger area.Furthermore,colonies with higher W a show less isotropic shapes (low roundness)indicating higher crawling activity.The dynamics of expansion of cell colonies depend on FN surface coatingDespite previously mentioned analogies,the spreading of a tissue colony is much more complex than a simple spreading of a water droplet as it is driven far from equilibrium due to cellular activities.Further,the localization of activity (mellipodia,actin cable)is variable depending on the FN concentration and probably also time.To investigate these complexities,we visua-lized the expansion of colonies for 5days over different ECM coatings (Fig.2A and Movies 1–3,ESI †).Interestingly,colonies on different concentrations of FN looked alike for the first two days when the number of cells was limited and clear differences appeared only after the second day.Potentially this was due to a very high number of cells per unit of area,which may favor high W c .After the second day,the appearance of the colonies started to differ between the different FN coatings.This was reflected by an exponential increase in colony area (Fig.2B).A rise in the area correlated with the amount of FN absorbed onto the surfaces,with cells on high FN being able to spread into large patches,whereas colonies on 5m g ml À1had less pronounced enlargement.Conversely,cells with low adhesion (0m g ml À1)never really expanded onto the substrate,but rather aggregated into small three dimensional spheroids and remained round till the end of the process.In parallel to the area changes,the roundness of colonies provided with ECM declined after the third day (Fig.2C).This decline in round-ness,which reflected more anisotropic shapes,was also corre-lated to FN concentrations.In particular,colonies on higher concentrations of FN coating showed more anisotropic shapes (lower roundness)then those on lower FN concentrations.Differences in colonies’area and shape could potentially result from differences in proliferation in the two different conditions.58,59The contact angle of the cell colonies with the substrate,was also subject to change over time (Fig.2D).One day after seeding,the colonies had the highest contact angle during the first day and only colonies at 80m g ml À1FN showed the ability to effectively spread onto the substrate.In thefollowingFig.1Surface FN coating changes the aspect ratio of epithelial cell colonies.(A)Top:physisorption of FN on the glass substrate was measured by detection of fluorescently labeled FN (Cy5-FN).Middle:line profiles across the images show the fluorescence intensity values of the Cy5-FN.Bottom:FN coating after 5days in culture with cells.FN coating was only marginally decreased after 5days in culture.The presence of cells (arrows)only caused minor variations in the fluorescence intensity (scale bar =50m m).For direct visual comparison,acquisition and display settings are equal for all fluorescence images in A.(B)Exemplary fluorescence images of colonies grown on different FN coatings for 3days.Colonies originated from a few (o 2)cells stably transfected with GFP-actin are shown.x /y (top)and x /z (side)views of cell colonies are shown.Top (x /y )views were used to measure the area spread (A )and roundness (r –see text)of the colony.Side (x /z )views were used to measure the contact angle (y ).Actual values for the colonies shown are reported in brackets.(C)Schematic of contact angle as a function of FN concentration.Integrative Biology PaperP u b l i s h e d o n 11 S e p t e m b e r 2015. D o w n l o a d e d b y S o u t h C h i n a N o r m a l U n i v e r s i t y o n 19/10/2015 08:35:43.days,y sensibly declined for colonies at 5and 80m g ml À1FN but not for 0,which remained rather high throughout the whole time of sampling.Contact angle of the cell colonies with the substrate,was also subject to changes over time (Fig.2D).One day after seeding,the colonies had the highest contact angle during the first day and only colonies at 80m g ml À1FN showed to be able to effectively spread onto the substrate.In the following days y sensibly declined for colonies at 5and 80m g ml À1FN but not for 0m g ml À1,for which y remained rather high throughout the whole sampling time.Despite these time-dependent changes,the cell numbers grew exponentially by the same rate at both,5and 80m g ml À1FN (Fig.2E).This was reflectedby a nearly identical doubling time (time taken by the popula-tion to duplicate in number)of approximately 15h in both cases (Fig.2F).This could be due to the fact that cell prolifera-tion was at its maximum speed at this low confluence.Despite having the same proliferation rates,colonies on different amounts of FN had marked differences in cell density (Fig.2G),during the process of expansion.In both cases,for low and high concentrations of FN,cell density increased between day 1and 2.Thereafter,the density of the cells continued climbing at a lower rate for colonies cultured at 5m g ml À1FN,but it declined for those at 80m g ml À1FN,resulting in colonies with large,flat cells.Taken together,theseFig.2The growth of MDCK colonies depends on FN concentrations.(A)A time lapse showing the evolution of cell colonies over 5days as a function of the FN coating concentration.Colonies were derived from 1or 2cells seeded at time 0.Perimeters of the colonies at different time points are shown in the last column.For all images,the horizontal scale bar =100m m and the vertical scale bar =a color-code of the time points.Variation of colony area (B)and roundness (C)as a function of FN coating are plotted over time.The inset in B is a plot of area over time in a semi-logarithmic scale.The geometrical descriptor termed roundness is independent of the size of the patch and it would be 1for a perfect circle and would tend to 0for a highly scattered,anisotropic shape.(D)Contact angle of colonies (y )as a function of FN coating is plotted over time.4to 10colonies per condition have been analyzed.8different positions per each colony have been used to measure y .(E)A plot of cell number in a semi-logarithmic scale shows an exponential increase of the cell population over time.At 0m g ml À1FN,the number of cells cannot be resolved due to very high density within the spheroid.(F)Doubling time of cell population as a function of FN coating was calculated from E.(G)Cell density as a function of time.For B,C,E,F and G,n =3–7.For all images,error bars =standard error of the mean.Data are considered significantly different for p o 0.05(unpaired Student’s t -test).Paper Integrative BiologyP u b l i s h e d o n 11 S e p t e m b e r 2015. D o w n l o a d e d b y S o u t h C h i n a N o r m a l U n i v e r s i t y o n 19/10/2015 08:35:43.results suggest that active expansion dominates the development of colonies at high W a leading to higher colony spreading (S ).Cell motility and mode of collective migration within cell colonies depends on FN surface concentrationCell proliferation fails to explain the different spreading behaviors at different FN coatings.Thus,we further investigated the effect of cell motility and collective behavior on the colony expansion process.Increasing amounts of ECM coating and adhesion of cells to the substrate proved to cause increased cell motility of single cells 60as well as in tissues.38,61Also in our system,MDCK cells overexpressing Snail transcription factor,which down-regulates E-cadherin 62and induces single cell migration,showed increased motility at higher concentrations of FN coating (Fig.S2,ESI †).To explore this aspect in our system,we analyzed the motility within the colonies by particle image velocimetry (PIV;Fig.3and Movies 4and 5,ESI †).At early times,when the number of cells and the patch size was still small (second day),cells had a clear tendency to rotate for both FN conditions (Fig.3A)in a similar fashion as shown on confined micro-patterned disks.63–65After the second day,this rotation continued in colonies on low FN coating concentrations,but it was replaced by a predominantly outward movement for the higher FN concentration (Fig.3B–E).Besides the case of no FN coating,where movement remains slow at all times (average speed =5.21Æ1.72m m h À1),the average speed was low for the duration of this whole initial period for both FN coating concentrations (day 1to 3,average speed =7.48Æ2.04m m h À1for low FN and 7.11Æ1.99m m h À1for high FN)and it increased only thereafter (day 4and 5,average speed =7.95Æ2.83m m h À1for 5m g ml À1and 8.38Æ1.10m m h À1for 80m g ml À1)(Fig.3F and G).During the fifth day,colonies on low FN continued to show a rotational movement (Fig.3C and D).Meanwhile,a radial outward motion became more visible for colonies on high FN (Fig.3C and E).This picture was confirmed by quantitative analysis of move-ment directionality (Fig.3H–J).Rotational movement during the second and third day was a common characteristic of all conditions (orthoradial/radial ratio >1).After the second day,this indicator for rotational movement declined in both cases but the decline was more pronounced for the high concen-tration of FN coating.Despite this decline,the rotation remained prominent for colonies on low FN for the entire duration of the experiment (with the orthoradial component higher than the radial one).Conversely,movement became more radially oriented (orthoradial/radial ratio o 1)at high FN,indicating that an outward collective movement was driving the overall expansion of the tissue through a cell crawling mechanism.Similarly,analysis of the orientation of the vectors shows that the majority of vectors are orthoradial for 0and 5m g ml À1FN and radial for 80m g ml À1.In our interpretation,rotational movement would be induced by high cohesion (high W c )between the cells and/or a lack of sufficient adhesion to the substrate (low W a ),and this would prevent outward cell migration and mark the onset of rotation of the colony.In contrast,on higher FN,higher cell–surface friction would counterbalance the strong cell–cell cohesion.Therefore,cellswere able to move and widen the relative distance separating them from each other,which allowed the colony to spread over the substrate.This expansion was further enhanced by the appearance of leader cells pulling neighbors into finger-like structures (Fig.3E,bottom).Interestingly,in rare cases leader cells moved too fast for their followers and detached from the colony (Fig.S3A and Movie 6,ESI †)in a process that reflects the consequences of an extreme imbalance between cohesion and adhesion (with W a >W c ).These results suggest that at a low FN concentration,cells have a limited capability for expansion due to high cohesion between cells (W a preponderant over W c ).Thus,their motion is frustrated into a rotation,63,65which further suggests a self-confining mechanism leading to high cell density and roundness.Thus,in the absence of outward migration,expansion of colonies on low FN can mostly be attributed to cell proliferation rather than cell motility.On the other hand,cells within colonies on high FN showed high motility and the freedom to collectively move outward (W c preponderant over W a ).In the process,cells become flat and well spread out.Furthermore,the appearance of leader cells seems to be the reason for the highly anisotropic shapes of colonies on a high FN concentration.Actomyosin cables provide cohesiveness to the cell colony,whereas lamellipodia are responsible for adhesiveness and substrate wettingTo investigate the nature of cohesive and adhesive mechanisms,we visualized actin structures at different FN coating concentrations.Previous studies have shown that epithelial cell migration in various situations can be regulated by actomyosin contractility through a purse-string mechanism and/or cell crawling activity.35,38,66We looked for actomyosin cable and lamellipodia distribution at the three different FN concentrations (Fig.4).As previously mentioned,at 0m g ml À1FN,cells did not spread but formed 3D spheroids.At the base,these aggregates where confined by a thick actomyosin cable (Fig.4A and B bottom).However,contact with the substrate is very limited in this case and images taken at the equatorial plane show that actin is mostly engaged at the cell–cell junction (Fig.4B bottom).For the case of 5and 80m g ml À1,the most obvious difference was the presence of large stress fibers at the basal plane of the colonies on high FN,that were virtually absent at lower FN coating concentrations (Fig.4A–D).The actomyosin cable,which pro-vides a strong barrier at the outer perimeter of the colony and inward forces at concave edges,thus improving the cohesive-ness of rounder colonies,38appeared to be continuous over multiple cells at 5m g ml À1FN (Fig.4A and C top).In contrast,the cable was frequently interrupted by the protrusive activity of small and large lamellipodia in colonies on high FN (Fig.4A and D top).Lamellipodia and leader cells were clearly favored at the high FN coating concentration,whereas they appeared to be small at low FN (Fig.4A,C and D bottom).This scenario was confirmed by quantitative analysis of edge occupancy by the actomyosin cable (Fig.4E)and lamellipodia (Fig.4F).The edge of colonies at 0and 5m g ml À1was mostly limited by the actomyosin cable,whereas those at 80m g ml À1had higherIntegrative Biology PaperP u b l i s h e d o n 11 S e p t e m b e r 2015. D o w n l o a d e d b y S o u t h C h i n a N o r m a l U n i v e r s i t y o n 19/10/2015 08:35:43.Fig.3Particle image velocimetry (PIV)analysis of the collective motion of cells.(A–E)Merged PIV quiver plots and phase contrast images show patterns of cell movement at three consecutive time points (D t =30min)during the 2nd (A),3rd (B),and 5th (C)day after seeding.For each image sequence,the top row shows the migration patterns of a colony growing over a substrate coated with 5m g ml À1FN and the bottom row shows those with 80m g ml À1FN.The direction and magnitude of movement are represented by the orientation and length of the vectors.(D and E)Enlarged views from ROI in C.For all images from A to E,the vectors are color-coded according to the angle respective to the center of mass of the colony.901angles are vectors radially oriented to the center of mass (expanding tissue),whereas 01angles are vectors perpendicular to the radial direction (orthoradial –rotational movement of the tissue).(F)A bar plot of the speed magnitude distribution of colonies grown at different FN coating concentrations.The average speed of 3independent colonies over the 4days of observation is shown.(G)Evolution of the speed magnitude over time.The average of 3independent colonies is shown.(H)Ratio between orthoradial and radial components of velocities within the whole colony plotted over time.For ratios above 1,the tissue is mostly rotating;below 1the tissue is mostly expanding.(I)Plot of orthoradial/radial components within 20m m from the edge of the colonies.(J)Frequency (%)of the angles of the velocity vectors as compared to the tissue center of mass.For H–J,the average of 2independent colonies is shown.For all panels,901is radial,01is orthoradial.Error bars =standard error of the mean.When not visible,the SEM bar is smaller than the symbol.Data are considered significantly different for p o 0.05(unpaired Student’s t -test).Paper Integrative BiologyP u b l i s h e d o n 11 S e p t e m b e r 2015. D o w n l o a d e d b y S o u t h C h i n a N o r m a l U n i v e r s i t y o n 19/10/2015 08:35:43.lamellipodia occupancy.Altogether,these results confirmed that the relative contributions of the pluricellular actin cable and the formation of lamellipodia-based protrusions could be tuned by cell–substrate adhesion and were important to control epithelial tissue organization.Tissue traction forces on different FN coatingsNext,we measured traction forces by traction force microscopy (TFM)67on soft silicon gels coated with 5and 80m g ml À1FN,while monitoring actin structures to verify their contribution to adhesive and cohesive mechanisms.Despite differences in substrate rigidity and potentially in FN physisorption,colonies of cells grown on the soft gels demonstrated similar phenotypes as those grown on glass coverslips.Indeed,colonies of cells at low FN concentrations were more circular,with more actomyosin cables and less lamellipodia compared to those grown on high FN (Fig.5A and B,left and Movies 7and 8,ESI †).Compatible with the crawling mechanism,large inward pulling forces were observed at the site of lamellipodia (Fig.5A and B,top right).Importantly,larger forces and larger lamellipodia were found at the edge of colonies grown at 80m g ml À1FN.On the other hand,converging force dipoles (Fig.5A,bottom right)were found at the site of contractile actin cables acting tangentially to the tissue edges.This was particularly obvious at the low FN coating concentration.In contrast,at the high FN concentration,large converging force dipoles appeared within the tissue (Fig.5B,bottomright).Fig.4Lamellipodia and actomyosin cable organization.(A)GFP-actin staining at the basal plane (top)and from the side (bottom)show actin structure organization in colonies grown on different FN coating concentrations.Cells were grown on substrates coated with 0,5or 80m g ml À1FN for 3days.(B–D)High resolution exemplary images of F-actin structures (phalloidin–TRITC,red)and nuclei (DAPI,blue)at different FN coating concentrations.(B)Basal plane (top)and equatorial plane (bottom)of a cell spheroid grown at 0m g ml À1FN.(C and D)Basal plane of cell colonies grown for 3days at 5and 80m g ml À1FN,respectively.In C and D,the top image shows a region with a prominent actomyosin cable.The bottom image shows a cell with lamellipodia with characteristics of a leader cell.In all panels,arrowheads indicate actomyosin cables,and arrows indicate lamellipodia.(E and F)Plots show the percentage of the colonies’edges occupied by actomyosin cables (E)and lamellipodia (F).n =2for 0m g ml À1and 3for 5and 80m g ml À1.Bars =standard error of the mean.When not visible,the SEM bar is smaller than the symbol.Data are considered significantly different for p o 0.05(unpaired Student’s t -test).Integrative Biology PaperP u b l i s h e d o n 11 S e p t e m b e r 2015. D o w n l o a d e d b y S o u t h C h i n a N o r m a l U n i v e r s i t y o n 19/10/2015 08:35:43.。
the expanding universe of T cell subsets_Th1, Th2 and more
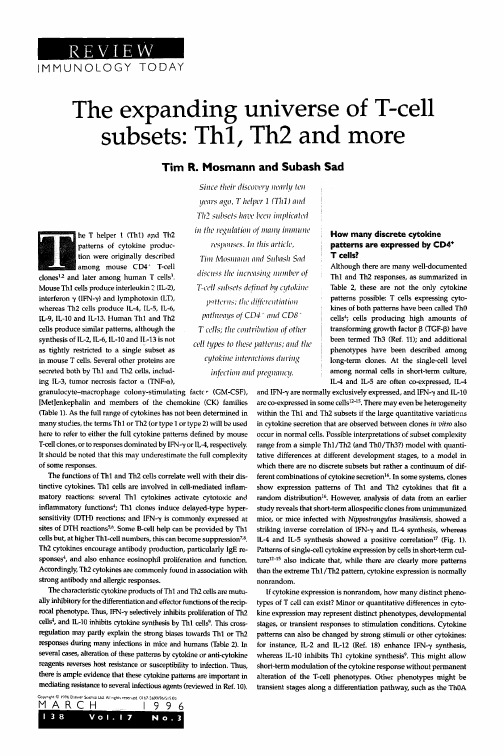
patterns can also be changed by strong stimuli or other cytokines: IL-2 and IL-12 (Ref. 18) enhance 1lW-y synthesis, whereas IL-10 inhibits Thl cytokine synthesis9. This might allow short-term modulation alteration of the cytokine response without permanent Other phenotypes might be pathway, such as the ThOA of the T-cell phenotypes.
IMMUNOLOGY
TODAY
The expanding universe of T-cell subsets: Thl, Th2 and more
Tim R. Mosmann and Subash Sad
he T helper
1 (Thl) and Th2
How many discrete cytokine patterns T cells? Although there are many well-documented Thl and Th2 responses, patterns possible: as summarized in Table 2, these are not the only cytokine T cells expressing cytoof kines of both patterns have been called ThO cells”; ceils producing high amounts transforming growth factor 8 (TGF-B) have been termed Th3 (Ref. 11); and additional phenotypes long-term have been described among level IL-4 culture, clones. At the single-cell are expressed by C
细胞内叠氮化物反应探针的英文
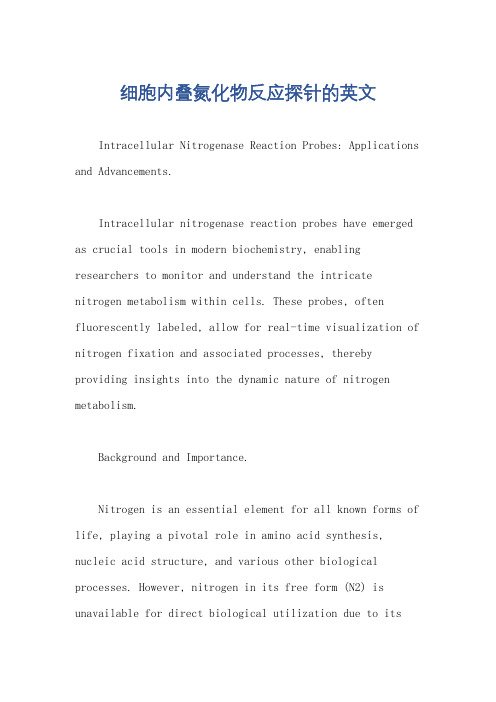
细胞内叠氮化物反应探针的英文Intracellular Nitrogenase Reaction Probes: Applications and Advancements.Intracellular nitrogenase reaction probes have emerged as crucial tools in modern biochemistry, enabling researchers to monitor and understand the intricate nitrogen metabolism within cells. These probes, often fluorescently labeled, allow for real-time visualization of nitrogen fixation and associated processes, thereby providing insights into the dynamic nature of nitrogen metabolism.Background and Importance.Nitrogen is an essential element for all known forms of life, playing a pivotal role in amino acid synthesis, nucleic acid structure, and various other biological processes. However, nitrogen in its free form (N2) is unavailable for direct biological utilization due to itsinertness. Therefore, organisms rely on nitrogenases, a class of enzymes that catalyze the conversion of N2 into ammonia (NH3), a biologically usable form of nitrogen.Within cells, nitrogenase enzymes are often embedded within complex systems, involving multiple cofactors and electron transport chains. Monitoring these reactionswithin the cellular milieu is challenging due to the dynamic nature of the cellular environment and the often-subtle changes in substrate concentration. Intracellular nitrogenase reaction probes have been developed to overcome these challenges, providing a window into the intracellular world of nitrogen metabolism.Types of Intracellular Nitrogenase Reaction Probes.1. Fluorescent Probes: These probes are labeled with fluorescent molecules that change their emission properties upon interacting with nitrogenase or its intermediates. For example, fluorophores such as fluorescein or rhodamine can be conjugated to specific substrates or inhibitors of nitrogenase, allowing for the detection of enzymaticactivity through fluorescence microscopy or flow cytometry.2. Bioluminescent Probes: These probes emit light through a chemical reaction triggered by nitrogenase activity. Bioluminescent probes offer the advantage of being self-luminous, eliminating the need for external excitation sources.3. Radiolabeled Probes: Radiolabeled probes incorporate radioactive atoms (e.g., carbon-14 or tritium) into substrates or inhibitors of nitrogenase. The subsequent detection of radiolabeled products provides quantitative information about enzyme activity. However, the use of radiolabeled probes is limited due to safety concerns and the need for specialized equipment.Applications of Intracellular Nitrogenase Reaction Probes.1. Studying Nitrogen Fixation Pathways: By monitoring the activity of nitrogenase within cells, probes can reveal the preferred nitrogen fixation pathway utilized bydifferent organisms. This information is crucial for understanding the adaptability of microorganisms to varying nitrogen sources and environmental conditions.2. Analyzing Nitrogen Metabolism in Response to External Stimuli: Intracellular probes can be used to study how nitrogen metabolism is affected by external factors such as changes in nutrient availability, pH, or temperature. Such studies can provide insights into the mechanisms underlying cellular responses to environmental perturbations.3. Drug Discovery and Therapeutics: Nitrogenase inhibitors have been explored as potential therapeutics for treating diseases associated with abnormal nitrogen metabolism, such as cancer or certain infectious diseases. Intracellular probes can aid in the identification of effective inhibitors by allowing for high-throughput screening of candidate compounds.Future Directions.With the continuous advancement of biotechnology and imaging techniques, intracellular nitrogenase reaction probes are poised to make significant contributions to our understanding of nitrogen metabolism. Future research may focus on developing probes with improved sensitivity and specificity, enabling the detection of nitrogenase activity in single cells or even subcellular compartments. Additionally, the integration of probes with other omics technologies (e.g., genomics, proteomics, or metabolomics) could provide a comprehensive picture of nitrogen metabolism within cells, leading to new insights and potential therapeutic targets.In conclusion, intracellular nitrogenase reaction probes have emerged as invaluable tools for studying nitrogen metabolism within cells. Their ability to monitor enzymatic activity in real-time, combined with their versatility and sensitivity, makes them critical for advancing our understanding of nitrogen metabolism and its role in health and disease. As technology continues to evolve, these probes will play increasingly important roles in fundamental and applied research.。
Thermo Scientific
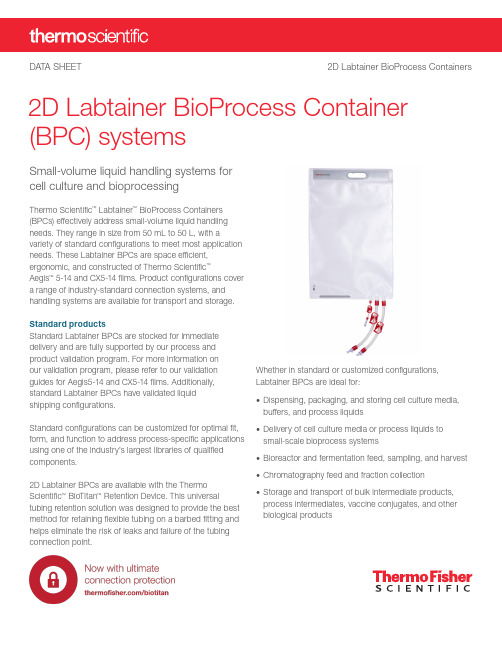
DATA SHEET 2D Labtainer BioProcess Containers2D Labtainer BioProcess Container (BPC) systemsWhether in standard or customized configurations, Labtainer BPCs are ideal for:• Dispensing, packaging, and storing cell culture media, buffers, and process liquids• Delivery of cell culture media or process liquids to small-scale bioprocess systems• Bioreactor and fermentation feed, sampling, and harvest • Chromatography feed and fraction collection• Storage and transport of bulk intermediate products, process intermediates, vaccine conjugates, and other biological productsSmall-volume liquid handling systems for cell culture and bioprocessingThermo Scientific ™ Labtainer ™ BioProcess Containers (BPCs) effectively address small-volume liquid handling needs. They range in size from 50 mL to 50 L, with avariety of standard configurations to meet most application needs. These Labtainer BPCs are space efficient, ergonomic, and constructed of Thermo Scientific ™Aegis ™ 5-14 and CX5-14 films. Product configurations cover a range of industry-standard connection systems, and handling systems are available for transport and storage.Standard productsStandard Labtainer BPCs are stocked for immediate delivery and are fully supported by our process and product validation program. For more information on our validation program, please refer to our validation guides for Aegis5-14 and CX5-14 films. Additionally, standard Labtainer BPCs have validated liquid shipping configurations.Standard configurations can be customized for optimal fit, form, and function to address process-specific applications using one of the industry’s largest libraries of qualified components.2D Labtainer BPCs are available with the Thermo Scientific ™ BioTitan ™ Retention Device. This universaltubing retention solution was designed to provide the best method for retaining flexible tubing on a barbed fitting and helps eliminate the risk of leaks and failure of the tubingconnection point.Table 1. Chamber information.50 mL–2 L, 2-port Labtainer BPC 2 L–50 L, 3-port Labtainer BPC2 L–50 L: Polyethylene ports are welded into the BPC seam: one 1/4 in. ID and two 3/8 in. ID ports on standard chamber.Table 2. Custom BPC options.Tubing type C-Flex™ (animal origin–free), silicone, PharMed™, or AdvantaFlex™Tubing size Specific lengths of 3.18–25.4 mm ID (1/8–1 in.); specific length depends on type of tubing chosenConnectors • Luer: 3.18–6.35 mm (1/8–1/4 in.) ID• CPC quick-connect: 6.35–19 mm (1/4–3/4 in.) ID• Steam-in-place connector: 6.35–19 mm (1/4–3/4 in.) ID• Tri-clamp: 3.18–25.4 mm (1/8–1 in.) ID• Mini tri-clamp: 3.18–12.7 mm (1/8–1/2 in.) ID• Aseptic connection and aseptic disconnection devices: all available sizes of Colder AseptiQuik™, Pall™ Kleenpak™, Cytiva ReadyMate™ DACOthers • Needle-free sample port (SmartSite™ or Clave™ products)• Filter capsule (Millipore™, Pall™, Sartorius™, Parker Bioscience™, Meissner™ products)Table 3. Presentation (as dry BPC systems).Outer packaging Supplied “flat-packed”—two polyethylene outer layersLabel • Description• Product code• Lot number• Expiration date on outer packaging and shipping containerSterilization Irradiation (25–40 kGy) inner side of outer packaging Shipping container Durable cardboard cartonDocumentation • Certificate of Analysis provided with each batch for each delivery • Certificate of Irradiation2 portsPack of 10Line 1Luer lock body connection, polypropyleneTubing: C-Flex; 30 cm (12 in.) lengthID x OD: 3.18 x 6.35 mm (0.125 x 0.25 in.)Line 2Luer lock insert connection, polypropyleneTubing: C-Flex; 30 cm (12 in.) lengthID x OD: 3.18 x 6.35 mm (0.125 x 0.25 in.) Line 1Luer lock body connection, polypropyleneTubing: C-Flex; 8 cm (3 in.) lengthID x OD: 6.35 x 10.92 mm (0.25 x 0.43 in.)Line 2Luer lock insert connection, polypropyleneTubing: C-Flex; 8 cm (3 in.) lengthID x OD: 6.35 x 10.92 mm (0.25 x 0.43 in.)Line 1Luer lock body connection, polypropyleneTubing: C-Flex; 30 cm (12 in.) lengthID x OD: 3.18 x 6.35 mm (0.125 x 0.25 in.)Line 2MPC insert, polycarbonateTubing: C-Flex; 8 cm (3 in.) lengthID x OD: 3.18 x 6.35 mm (0.125 x 0.25 in.)2 portsPack of 10Pack of 10Line 1Luer lock insert connection, polypropyleneTubing: C-Flex; 30 cm (12 in.) length ID x OD: 6.35 x 9.7 mm (0.25 x 0.38 in.)Line 2Luer lock body connection, polypropylene Tubing: C-Flex; 30 cm (12 in.) length ID x OD: 6.35 x 9.7 mm (0.25 x 0.38 in.)Line 3Luer lock body connection, polypropylene Tubing: C-Flex; 30 cm (12 in.) lengthID x OD: 3.18 x 6.35 mm (0.125 x 0.25 in.)Line 1MPC insert, polycarbonateTubing: C-Flex; 61 cm (24 in.) length ID x OD: 9.7 x 15.9 mm (0.38 x 0.63 in.)Line 2MPC body, polycarbonateTubing: C-Flex; 61 cm (24 in.) length ID x OD: 9.7 x 15.9 mm (0.38 x 0.63 in.)Line 3Luer lock body connection, polypropylene Tubing: C-Flex; 61 cm (24 in.) length ID x OD: 3.18 x 6.35 mm (0.125 x 0.25 in.)Line 1MPC insert, polycarbonateTubing: C-Flex; 30 cm (12 in.) length ID x OD: 9.7 x 12.7 mm (0.38 x 0.5 in.)Line 2MPC insert, polycarbonateTubing: C-Flex; 30 cm (12 in.) length ID x OD: 9.7 x 12.7 mm (0.38 x 0.5 in.)Line 3End plug, polypropyleneTubing: C-Flex; 8 cm (3 in.) lengthID x OD: 6.35 x 10.92 mm (0.25 x 0.43 in.)3 portsSingle pack3 portsSingle packSingle pack—edge portsLine 1MPC insert, polycarbonateTubing: C-Flex; 8 cm (3 in.) lengthID x OD: 9.6 x 12.7 mm (0.378 x 0.50 in.)Line 2MPC insert, polycarbonateTubing: C-Flex; 8 cm (3 in.) lengthID x OD: 9.6 x 12.7 mm (0.378 x 0.50 in.)Line 3Injection portTubing: C-Flex; 8 cm (3 in.) lengthID x OD: 6.35 x 9.53 mm (0.25 x 0.375 in.)Note: Aegis5-14 film equivalents for this product areavailable as custom configurations.Line 1MPC insert, polycarbonateTubing: C-Flex; 46 cm (18 in.) lengthID x OD: 3.18 x 6.35 mm (0.125 x 0.25 in.)Line 2MPC body, polypropyleneTubing: C-Flex; 61 cm (24 in.) lengthID x OD: 9.53 x 15.875 mm (0.375 x 0.625 in.)Line 3Luer lock body connection, polypropyleneTubing: C-Flex; 61 cm (24 in.) lengthID x OD: 9.53 x 15.875 mm (0.375 x 0.625 in.)3 portsSingle pack—pillow design withpanel portsFind out more at /bpcFor Research Use or Further Manufacturing. Not for diagnostic use or direct administration into humans or animals.© 2021 Thermo Fisher Scientific Inc. All rights reserved. All trademarks are the property of Thermo Fisher Scientific and its subsidiaries unlessotherwise specified. C-Flex and PharMed are trademarks of Saint-Gobain Performance Plastics. AdvantaFlex is a trademark of NewAge Industries, Inc. AseptiQuik is a trademark of Colder Products Company. Pall and Kleenpak are trademarks of Pall Corporation. ReadyMate is a trademark of Cytiva. SmartSite is a trademark of Becton, Dickinson and Company. Clave is a trademark of Victus Inc. Millipore is a trademark of Merck KGaA, Darmstadt, Germany and/or its affiliates. Meissner is a trademark of Meissner Filtration Products. Parker Bioscience is a trademark of Parker Hannifin Corp. Rubbermaid is a trademark of Rubbermaid Incorporated. Sartorius is a trademark of Sartorius AG. Specifications, terms, and pricing are subject toIndustry-standard Rubbermaid ™ totes with corresponding lids are available. They can be used to protect Labtainer BPCs up to 20 L in size during use, transport, and storage. Use standard 50 L drums for 50 L Labtainer BPCs.Tray with lidFlat-bottom, linear low-density polyethylene (LLDPE) drum with lid。
- 1、下载文档前请自行甄别文档内容的完整性,平台不提供额外的编辑、内容补充、找答案等附加服务。
- 2、"仅部分预览"的文档,不可在线预览部分如存在完整性等问题,可反馈申请退款(可完整预览的文档不适用该条件!)。
- 3、如文档侵犯您的权益,请联系客服反馈,我们会尽快为您处理(人工客服工作时间:9:00-18:30)。
MOLECULAR CELL BIOLOGY19.1 Cell-cell and cell-matrix adhesion: an overview19.2 Cell-cell and cell-ECM junctions and their adhesion molecules 19.3 The ECM I: the basal lamina19.4 The ECM II: connective and other tissues19.5 Adhesive interactions in motile and nonmotile cells19.1 Cell-cell and cell-matrix adhesion: an overview⏹Cells in tissues can adhere directly to one another (cell-cell adhesion) through specialized membrane protein cell-adhesion molecules (CAMs) that often cluster into specialized cell junctions;⏹Cells in animal tissues adhere indirectly (cell-matrix adhesion) through the binding of adhesion receptors in the plasma membrane to components of the surrounding extracellular matrix (ECM), a complex interdigitating meshwork of proteins and polysaccharides secreted by cells into the space between them;19.1 Cell-cell and cell-matrix adhesion: an overview19.1 Cell-cell and cell-matrix adhesion: an overview⏹CAMs: major classes☐The cadherins;☐Immunoglobulin superfamily;☐Integrins;☐Selectins;19.1 Cell-cell and cell-matrix adhesion: an overview⏹CAMs: kind of links☐ Homotypic adhesion: adhere between same type of cells;☐ Heterotypic adhesion: adhere between different type of cells; ☐ Homophilic binding: bind between same type of CAM;☐ Heterophilic binding: bind between different type of CAM;19.1 Cell-cell and cell-matrix adhesion: an overview⏹CAMs: kinds of adhesive interactions☐ cis interaction: CAMs on one cell associate laterally through their extracellular domains, cytosolic domains, or both into homodimers of higher-order oligomers in the plane of the cell`s plasma membrane;☐Trans interaction: CAM oligomers on one cell bind to the same or different CAMs on an adjacent cell;19.1 Cell-cell and cell-matrix adhesion: an overview CAMs: kinds of adhesive interactions19.1 Cell-cell and cell-matrix adhesion: an overview⏹ECM components☐Proteoglycans: a unique type of glycoprotein, such as perlecan; ☐Collagens: proteins that often form fibers;☐Multiadhesive matrix proteins: These are long, flexible molecules that contain multiple domains responsible for binding various types of collagen, other matrix protein, polysaccharides, cell-surface adhesion receptors, and extracellular signaling molecules; Fibronectin and laminin are major kind of multiadhesive matrix proteins;19.2 Cell-cell and cell-ECM junctions and their adhesion molecules Epithelial cells have distinct different surface structures☐The apical surface;☐The lateral surface;☐The basal surfacue;19.2 Cell-cell and cell-ECM junctions and their adhesion molecules Three major types of junctions in animal cellsJunction Adhesiontype CAMS orreceptorsCytoskeletalattachmentFunctionAnchoring - Cell-cellCell-cellCell-matrixCadherinsCadherinsIntegrinActin filamentsIntermediate filamentsIntermediate filamentsShape, tension,strength, durabilityShape, rigidityTight - Cell-cell Occludin,Claudin, JAMs Actin filaments Control solute flow,signalingGap - Cell-cell ConnexinsInnexinsPannexins adapters Communication,transport19.2 Cell-cell and cell-ECM junctions and their adhesion molecules Three major types of junctions in animal cellsAnchoring junctionsTight junctionsGap junctions19.2 Cell-cell and cell-ECM junctions and their adhesion molecules⏹Anchoring junction: there are 3 types of anchoring junctions☐Adhesion junctions:It participate in cell-cell adhesion;☐Desmosomes:It participate in cell-cell adhesion and found on smooth muscle and heart cells;☐Hemidesmosomes:It participate in cell-ECM adhesion and found on the basal surface of epithelial cells;19.2 Cell-cell and cell-ECM junctions and their adhesion molecules CAMs in anchoring junctions:The primary CAMs in adherens junction and desmosomes belong to the cadherin family;(1) C lassical cadherins and (2) Desmosomal cadherins are 2 major classes of the cadherin family;The primary EAM recepter in hemidesmosomes belong to the (3) integrin family;19.2 Cell-cell and cell-ECM junctions and their adhesion molecules (1) Classical cadherins:It include E-, N-, and P-cadherins;The adhesiveness of cadherins depends on the presence of extracellular Ca2+;Each classical cadherin contains a single transmembrane domain, a relatively short C-terminal cytosolic domain, and 5 extracellular cadherin domains;The extracellular cadherin domains are necessary for Ca2+ binding and cadherin-mediated cell-cell adhesion;19.2 Cell-cell and cell-ECM junctions and their adhesion molecules (1) Classical cadherins:A single transmembrane domainA relatively short C-terminal cytosolic domain5 extracellular cadherin domains19.2 Cell-cell and cell-ECM junctions and their adhesion molecules (1) Classical cadherins:The C-terminal cytosolic domain is linked to the actin cytoskeleton by adapter proteins;The C-terminal cytosolic domain also interact with intracellular signaling molecules such as β–catenin and p120-catenin;19.2 Cell-cell and cell-ECM junctions and their adhesion molecules (1) Classical cadherins:19.2 Cell-cell and cell-ECM junctions and their adhesion molecules (2) Desmosomal cadherins:Desmosomes contain 2 specialized cadherin proteins, desmoglein and desmocollin, whose cytosolic domains are distinct from those in classical cadherins;The cytosolic domains of desmosomal cadherins interact with adapters proteins such as plakoglobin, plakophilins and desmoplakin;The adapters, which form the thick cytoplasmic plaques characteristic of desmosomes, in turn interact with IFs;19.2 Cell-cell and cell-ECM junctions and their adhesion molecules(2) Desmosomal cadherins:Cadherin proteins: Desmogleinand DesmocollinThe adapters: Thick cytoplasmicplaques---IFs19.2 Cell-cell and cell-ECM junctions and their adhesion molecules (3) Integrins:The principal ECM adhesion receptor in hemidesmosomes is integrin α6β4, a member of the integrin family of proteins;Integrins function as adhesion receptors and CAMs in wide variety of epithelial and nonepithelial cells, mediating many cell-matrix and cell-cell interactions;19.2 Cell-cell and cell-ECM junctions and their adhesion molecules (3) Integrins:In vertebrates, at least 24 integrin heterodimers, composed of 18 types of α subunits and 8 types β subunits in various combinations;A single β chain of integrin can interact with any one of multiple αchains, forming integrins that bind differet ligands;This allows a relatively small number of components to serve a large number of distinct functions;These is called the combinatorial diversity of Integrins;19.2 Cell-cell and cell-ECM junctions and their adhesion molecules (3) Integrins:19.2 Cell-cell and cell-ECM junctions and their adhesion molecules Tight junctions:Tight junctions seal off body cavities and restrict diffusion of membrane components;Tight junctions are composed of thin bands of plasma-membrane proteins that completely encircle the cell and are in contact with similar thin bands on adjacent cells;Tight junction is formed by a double row of protein particles, one row donated by each cell;19.2 Cell-cell and cell-ECM junctions and their adhesion molecules Tight junctions:The 2 principal integral-membrane proteins found in tight junctions are occludin and claudin, each of these proteins has 4 membrane-spanning α helices;A group of junction adhesionmolecule (JAM s) have been foundto contribute to homophilicadhesion of tight junctions;19.2 Cell-cell and cell-ECM junctions and their adhesion molecules Tight junctions:The extracellular domains ofrows of occludin, claudin, andJAM in the plasma membraneof one cell apparently tight linkswith similar rows of the sameproteinsin an adjacent cell,creating a tight seal;19.2 Cell-cell and cell-ECM junctions and their adhesion molecules Gap junctions:The gap junction refers that a well-difined set of cylindrical particles that cross the gap and compose pores connecting the cytoplasms of adjacent cells;Gap junctions between mammalian cells permit the passage of molecules as large as 1.2nm in diameter;Generally speaking, molecules smaller than 1200 Da pass freely and those larger than 2000 Da do not pass;19.2 Cell-cell and cell-ECM junctions and their adhesion molecules Gap junctions:Thus ions, low-molecular-weight molecules, small intracellular signaling molecule (second messengers), can pass from cell to cell through gap junction;The proteins composing Gap junctions:✓Connexins are just found in vertebrate gap junctions;✓Innexins are just found in invertebrate gap junctions;✓Pannexins are found in vertebrate and invertebrate gap junctions;19.2 Cell-cell and cell-ECM junctions and their adhesion molecules Gap junctions:Each vertebrate hexagonal particle consists of 12 noncovalently associated connexin molecules: 6 form a cylindrical connexin hemichannel in one plasma membrane that is joined to a connexin hemichannel in the adjacent cell membrane, forming the continuous aqueous channel between the cells;Each individual connexin molecule spans the plama membrane 4 times;19.2 Cell-cell and cell-ECM junctions and their adhesion molecules Gap junctions:19.2 Cell-cell and cell-ECM junctions and their adhesion molecules Gap junctions:The permeability of gap junctions can be regulated by changes in the intracellular pH and Ca2+ concentration and phosphorylation of connexin;Assembly of connexins, their trafficking within cells, and formation of functional gap junctions depend on N-cadherin and its associated junctional proteins as well as desmosomal proteins;19.2 Cell-cell and cell-ECM junctions and their adhesion molecules Tight junctionsAnchoring junctions Gap junctions19.3 The ECM I: the basal laminaThree types of molecules in ECM, that bind to adhesion receptors: Proteoglycans: a group of glycoproteins that cushion cells and bind a wide variety of ECMs;Collagen fibers: provide structural integrity and mechanical strength and resilience;Soluble multiadhesive matrix proteins: such as laminin and fibronectin, which bind to and cross-link cell-surface adhesion receptors and other ECM components;19.3 The ECM I: the basal laminaThe basal lamina: brief descriptionThe specialized extracellular matrix sheet that plays a particularly important role in determining the overall architecture and function of epithelial tissue;These sheetlike meshwork of ECM components usually no more than 60-120nm thick;The basal lamina is structured differently in different tissues;Basal laminae play important roles in regeneration after tissue damage and in embryonic development;19.3 The ECM I: the basal laminaThe main components of basal laminae: (1)-(4);(1) Type IV collagen: trimeric molecules with both rodlike and globular domains that form a 2-D network;(2) Laminin: a family of multiadhesive, cross-shaped proteins that form a fibrous 2-D network with type IV collagen and that also bind to integrins and other adhesion receptors;(3) Perlecan: a large multidomain proteoglycan that binds to and cross-links many ECM components and cell-surface molecules;19.3 The ECM I: the basal laminaThe main components of basal laminae: (1)-(4);(4) Nidogen (Entactin): a rodlike molecule that cross-links type IV collegan, perlecan, and laminin and helps incorporate other components into the ECM;19.3 The ECM I: the basal lamina Laminin:✓It is a heterotrimeric,cross-shaped protein with a total MW of 820,000;✓The C-terminus of αsubunit mediate –dependent binding to specific carbohydrates on cellsurface;19.3 The ECM I: the basal laminaType IV collagen:✓It is a trimeric protein, made from identical or different 3 α chain; ✓A trimeric collagen molecule contains one or more three-stranded segments, each with a similar triple-helical structure;✓Each strand contributed by one of the α chains is twisted into a left-handed helix, and three such strands from the three α chains wrap around each other to form a right-handed triple helix;19.3 The ECM I: the basal lamina Type IV collagen:✓The collagen triple helixis composed from the characteristic repeatingmotif Gly-X-Y, where Xand Y can be any aminoacid but often proline and hydroxyproline;19.3 The ECM I: the basal laminaType IV collagen:✓All subtypes of type IV collagen form a 400 nm long triple helix that in interrupted about 24 times with nonhelical segments and flanked by large globular domains at the C-termini of the chains and smaller globular domains at the N-termini;✓Tyoe IV collagen molecules assemble into a branching, irregular 2-D fibrous network that forms the lattice on which the basal lamina is built;19.3 The ECM I: the basal lamina Type IV collagen:19.3 The ECM I: the basal laminaPerlecan:✓The the major secreted proteoglycan in basal laminae, consists of a large multidomain core protein made up of a repeats of five distinct domains, including laminin-like LG domains and Ig domains; ✓Perlecan contains 3 types of covalent polysaccharide chains: N-linked, O-linked and glycosaminoglycans (GAGs), which are long polymers of repeating disaccharides;19.4 The ECM II: connective and other tissues Connective tissue: tendon and cartilage✓The matrix of connective tissue ispacked with insoluble protein fibers and contains proteoglycans, various multiadhesive proteins and hyaluronan (a specialized glycosaminoglycan);✓The most abundant fibrous protein in connective tissue is collagen;19.4 The ECM II: connective and other tissuesFibrillar collagens✓About 80-90% of the collagen in the body consists of fibrillar collagens (types I, II, III), located primarily in connective tissues; ✓Type I fibrillar collagen is a long, thin triple helix consisting of 2 α1(I) chains and 1 α2(I) chain, each 1050 amino acids in length;✓The triple-stranded molecules associate into higher-order polymers called collagen fibrils, which in turn often aggregate into larger bundles called collagen fibers;19.4 The ECM II: connective and other tissues Fibrillar collagens✓They are secreted proteins, produced primarily by fibroblast in the ECM;✓The collagen α chains aresynthesized as longer precursor, pro- αchains, and undergo a series ofcovalent modifications and fold intotriple-helical procollagen;19.4 The ECM II: connective and other tissuesType I and II collagens associate with nonfibrillar collagens to form diverse structures✓Type I collagen forms long fibers, whereas networks of type II collagen are more meshlike;✓Most bones are about 70% mineral and 30% protein, the vast majority of which is type I collagen;✓In skeletal muscle, type VI collagen and proteoglycans are noncovalently bound to the sides of type I fibrils and may bind the fibrils together to form thicker collagen fibers;19.4 The ECM II: connective and other tissuesType I and II collagens associate with nonfibrillar collagens to form diverse structures✓The fibrils of type II collagen, the major collagen in cartilage, are smaller in diameter than type I fibrils and are oriented randomly in a various proteoglycan matrix;✓Type II fibrils are cross-linked to matrix proteoglycans by type IX collagen;19.4 The ECM II: connective and other tissuesType I and II collagens associate with nonfibrillar collagens to form diverse structures19.4 The ECM II: connective and other tissuesProteoglycans and their constituent GAGs✓Proteoglycans are a subset of secreted or cell-surface-attached glycoproteins containing covalently linked specialized polysaccharide chains called glycosaminoglycans (GAG);✓GAGs are long linear polymers of specific repeating disaccharides;✓One sugar is either a uronic acid or D-galactose; the other sugar is N-acetylglucosamine or N-acetylgalactosamine;19.4 The ECM II: connective and other tissuesProteoglycans and their constituent GAGs✓One or both of the sugars contain at leasr one anionic group (carboxylate or sulfate), thus each GAG chains bears many negative charges;。