Adsorption of Carbon Dioxide onto Activated Carbon and Nitrogen-Enriched Activated Carbon
二氧化碳捕集和原位转化英文
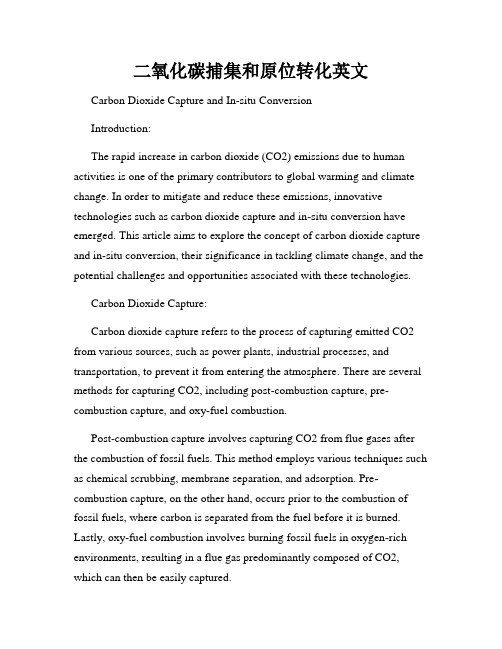
二氧化碳捕集和原位转化英文Carbon Dioxide Capture and In-situ ConversionIntroduction:The rapid increase in carbon dioxide (CO2) emissions due to human activities is one of the primary contributors to global warming and climate change. In order to mitigate and reduce these emissions, innovative technologies such as carbon dioxide capture and in-situ conversion have emerged. This article aims to explore the concept of carbon dioxide capture and in-situ conversion, their significance in tackling climate change, and the potential challenges and opportunities associated with these technologies.Carbon Dioxide Capture:Carbon dioxide capture refers to the process of capturing emitted CO2 from various sources, such as power plants, industrial processes, and transportation, to prevent it from entering the atmosphere. There are several methods for capturing CO2, including post-combustion capture, pre-combustion capture, and oxy-fuel combustion.Post-combustion capture involves capturing CO2 from flue gases after the combustion of fossil fuels. This method employs various techniques such as chemical scrubbing, membrane separation, and adsorption. Pre-combustion capture, on the other hand, occurs prior to the combustion of fossil fuels, where carbon is separated from the fuel before it is burned. Lastly, oxy-fuel combustion involves burning fossil fuels in oxygen-rich environments, resulting in a flue gas predominantly composed of CO2, which can then be easily captured.In-situ Conversion:In-situ conversion of carbon dioxide refers to the process of converting captured CO2 into valuable products or energy sources. This approach aims to utilize the captured CO2 instead of simply storing it underground. In-situ conversion can be achieved through various methods, such as chemical conversion, biological conversion, and electrochemical conversion.Chemical conversion involves the transformation of CO2 into useful chemicals or materials through chemical reactions. This method often requires catalysts to facilitate the conversion process. Biological conversion, on the other hand, utilizes microorganisms or plants to convert CO2 into biofuels or other organic compounds. Electrochemical conversion utilizes electrical energy to convert CO2 into products such as carbon monoxide or formic acid.Significance and Benefits:The development and implementation of carbon dioxide capture and in-situ conversion technologies hold significant potential in mitigating climate change and achieving sustainable development goals. These technologies can significantly reduce CO2 emissions, thereby minimizing the impact on the Earth's atmosphere and climate. Furthermore, in-situ conversion offers the opportunity to transform captured CO2 into valuable resources, promoting a circular economy and reducing dependence on fossil fuels.Challenges and Opportunities:While carbon dioxide capture and in-situ conversion technologies show promise, there are several challenges and opportunities associated with theirimplementation. One of the major challenges is the high cost of capturing and converting CO2. The development of cost-effective technologies is necessary to ensure widespread adoption and scalability. Additionally, the availability of suitable storage sites for captured CO2 and the environmental impact of these storage sites need to be carefully considered.However, opportunities exist to overcome these challenges. Continued research and development efforts can lead to technological advancements, making carbon dioxide capture and in-situ conversion more efficient and affordable. Collaboration between governments, industries, and research institutions is essential to drive innovation and create a supportive policy and regulatory framework. Moreover, the development of carbon markets and incentives can encourage investment and accelerate the adoption of these technologies.Conclusion:Carbon dioxide capture and in-situ conversion technologies offer a promising approach in addressing the challenges posed by rising CO2 emissions. The capture of CO2 prevents its release into the atmosphere, while in-situ conversion transforms it into valuable resources. With proper implementation and support, these technologies can contribute significantly to mitigating climate change and promoting sustainable development. However, continued research and development efforts, as well as collaboration between various stakeholders, are crucial in realizing their full potential and bringing about a greener and more sustainable future.。
Catalytic Conversion of Carbon Dioxide into Fuel
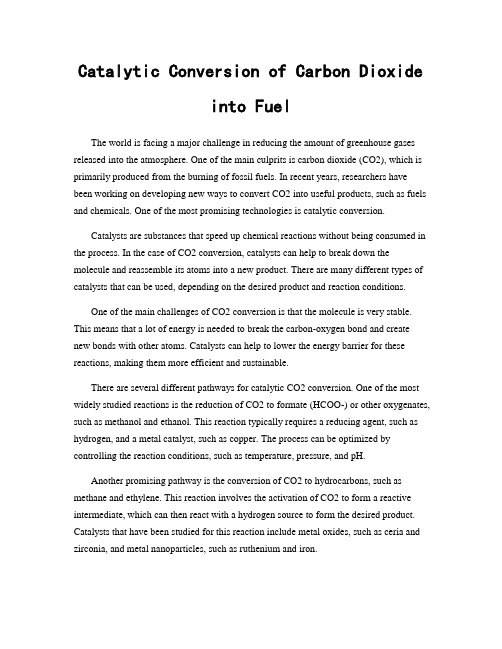
Catalytic Conversion of Carbon Dioxideinto FuelThe world is facing a major challenge in reducing the amount of greenhouse gases released into the atmosphere. One of the main culprits is carbon dioxide (CO2), which is primarily produced from the burning of fossil fuels. In recent years, researchers have been working on developing new ways to convert CO2 into useful products, such as fuels and chemicals. One of the most promising technologies is catalytic conversion.Catalysts are substances that speed up chemical reactions without being consumed in the process. In the case of CO2 conversion, catalysts can help to break down the molecule and reassemble its atoms into a new product. There are many different types of catalysts that can be used, depending on the desired product and reaction conditions.One of the main challenges of CO2 conversion is that the molecule is very stable. This means that a lot of energy is needed to break the carbon-oxygen bond and create new bonds with other atoms. Catalysts can help to lower the energy barrier for these reactions, making them more efficient and sustainable.There are several different pathways for catalytic CO2 conversion. One of the most widely studied reactions is the reduction of CO2 to formate (HCOO-) or other oxygenates, such as methanol and ethanol. This reaction typically requires a reducing agent, such as hydrogen, and a metal catalyst, such as copper. The process can be optimized by controlling the reaction conditions, such as temperature, pressure, and pH.Another promising pathway is the conversion of CO2 to hydrocarbons, such as methane and ethylene. This reaction involves the activation of CO2 to form a reactive intermediate, which can then react with a hydrogen source to form the desired product. Catalysts that have been studied for this reaction include metal oxides, such as ceria and zirconia, and metal nanoparticles, such as ruthenium and iron.One of the key advantages of catalytic CO2 conversion is that it can be integrated with renewable energy sources, such as solar and wind power. In this scenario, excess energy can be used to drive the reactions and produce fuels that can be stored and used when needed. This can help to improve the overall efficiency and sustainability of the energy system.Another advantage is that catalytic CO2 conversion can help to reduce the carbon footprint of industry. By capturing CO2 emissions and converting them into useful products, companies can reduce their reliance on fossil fuels and minimize their environmental impact. This can also help to create new business opportunities and drive innovation in the field.Despite the many benefits, there are still some challenges that need to be addressed when it comes to catalytic CO2 conversion. One of the main issues is scalability. Many of the reactions that have been studied are still in the laboratory stage and have not been scaled up to industrial levels. This requires significant investment in terms of equipment, infrastructure, and supply chain management.Another challenge is the cost of the catalysts. Many of the metals and other materials that are used as catalysts can be expensive and difficult to obtain. This can affect the overall economics of the process and make it less competitive compared to other carbon capture and storage (CCS) technologies.In conclusion, catalytic conversion of CO2 into fuel and other valuable products has the potential to be a game-changer in the fight against climate change. By using catalysts to accelerate reactions and lower energy requirements, the process can be made more efficient and sustainable. With further research and investment, we can unlock the full potential of this technology and create a cleaner, greener future.。
臭氧应用英文文章21
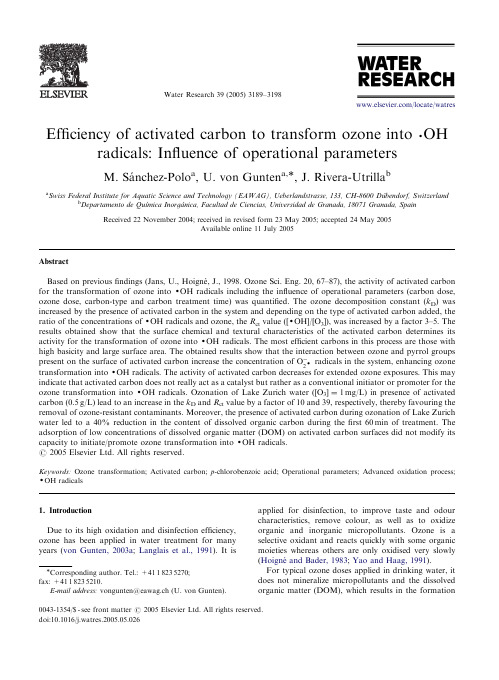
/locate/watres
Efficiency of activated carbon to transform ozone into dOH radicals: Influence of operational parameters
Some recent studies (Jans and Hoigne´ , 1998; Beltra´ n et al., 2002; Ma et al., 2004) have reported that activated carbon can accelerate ozone decomposition resulting in the formation of dOH radicals. It was demonstrated that incorporated metal centres, electrons of the graphenic layers (basal plane electrons) and basic surface groups of the activated carbon, are the main factors responsible for the decomposition of ozone at the activated carbon surface (Rivera-Utrilla et al., 2002; Rivera-Utrilla and Sa´ nchez-Polo, 2002, 2004). In addition, it was also observed that the presence of activated carbon during ozonation of organic micropollutants leads to a reduction in the concentration of DOM increasing the applicability of this treatment system (Rivera-Utrilla et al., 2002; Rivera-Utrilla and Sa´ nchezPolo, 2002, 2004).
从水中去除重金属离子和染料的农业固体废物吸附剂综述说明书
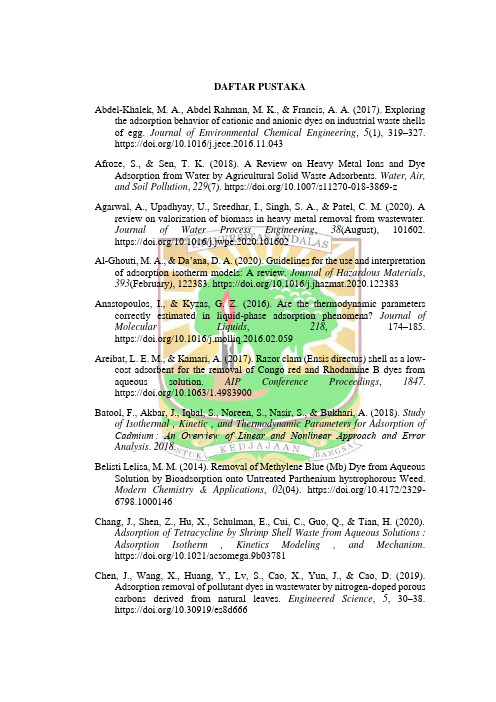
DAFTAR PUSTAKAAbdel-Khalek, M. A., Abdel Rahman, M. K., & Francis, A. A. (2017). Exploring the adsorption behavior of cationic and anionic dyes on industrial waste shells of egg. Journal of Environmental Chemical Engineering, 5(1), 319–327.https:///10.1016/j.jece.2016.11.043Afroze, S., & Sen, T. K. (2018). A Review on Heavy Metal Ions and Dye Adsorption from Water by Agricultural Solid Waste Adsorbents. Water, Air, and Soil Pollution, 229(7). https:///10.1007/s11270-018-3869-z Agarwal, A., Upadhyay, U., Sreedhar, I., Singh, S. A., & Patel, C. M. (2020). A review on valorization of biomass in heavy metal removal from wastewater.Journal of Water Process Engineering, 38(August), 101602.https:///10.1016/j.jwpe.2020.101602Al-Ghouti, M. A., & Da’ana, D. A. (2020). Guidelines for the use and interpretation of adsorption isotherm models: A review. Journal of Hazardous Materials, 393(February), 122383. https:///10.1016/j.jhazmat.2020.122383 Anastopoulos, I., & Kyzas, G. Z. (2016). Are the thermodynamic parameters correctly estimated in liquid-phase adsorption phenomena? Journal of Molecular Liquids, 218, 174–185.https:///10.1016/j.molliq.2016.02.059Areibat, L. E. M., & Kamari, A. (2017). Razor clam (Ensis directus) shell as a low-cost adsorbent for the removal of Congo red and Rhodamine B dyes from aqueous solution. AIP Conference Proceedings, 1847.https:///10.1063/1.4983900Batool, F., Akbar, J., Iqbal, S., Noreen, S., Nasir, S., & Bukhari, A. (2018). Study of Isothermal , Kinetic , and Thermodynamic Parameters for Adsorption of Cadmium : An Overview of Linear and Nonlinear Approach and Error Analysis. 2018.Belisti Lelisa, M. M. (2014). Removal of Methylene Blue (Mb) Dye from Aqueous Solution by Bioadsorption onto Untreated Parthenium hystrophorous Weed.Modern Chemistry & Applications, 02(04). https:///10.4172/2329-6798.1000146Chang, J., Shen, Z., Hu, X., Schulman, E., Cui, C., Guo, Q., & Tian, H. (2020).Adsorption of Tetracycline by Shrimp Shell Waste from Aqueous Solutions : Adsorption Isotherm , Kinetics Modeling , and Mechanism.https:///10.1021/acsomega.9b03781Chen, J., Wang, X., Huang, Y., Lv, S., Cao, X., Yun, J., & Cao, D. (2019).Adsorption removal of pollutant dyes in wastewater by nitrogen-doped porous carbons derived from natural leaves. Engineered Science, 5, 30–38.https:///10.30919/es8d666Dai, L., Zhu, W., He, L., Tan, F., Zhu, N., Zhou, Q., He, M., & Hu, G. (2018).Calcium-rich biochar from crab shell: An unexpected super adsorbent for dye removal. Bioresource Technology, 267(June), 510–516.https:///10.1016/j.biortech.2018.07.090d e Rezende, M. L. R., Coesta, P. T. G., de Oliveira, R. C., Salmeron, S., Sant’Ana,A. C. P., Damante, C. A., Greghi, S. L. A., & Consolaro, A. (2015). BoneDemineralization With Citric Acid Enhances Adhesion and Spreading of Preosteoblasts. Journal of Periodontology, 86(1), 146–154.https:///10.1902/jop.2014.130657Derakhshan, Z., Baghapour, M. A., Ranjbar, M., & Faramarzian, M. (2013).Adsorption of Methylene Blue Dye from Aqueous Solutions by Modified Pumice Stone: Kinetics and Equilibrium Studies. Health Scope, 2(3), 136–144. https:///10.17795/jhealthscope-12492Djelloul, C., & Hamdaoui, O. (2014). Removal of cationic dye from aqueous solution using melon peel as nonconventional low-cost sorbent. Desalination and Water Treatment, 52(40–42), 7701–7710.https:///10.1080/19443994.2013.833555Eletta, O. A. A., Adeniyi, A. G., Ighalo, J. O., Onifade, D. V., & Ayandele, F. O.(2020). Valorisation of Cocoa (Theobroma cacao) pod husk as precursors for the production of adsorbents for water treatment. Environmental Technology Reviews, 9(1), 20–36. https:///10.1080/21622515.2020.1730983Eljiedi, A. A. A., & Kamari, A. (2017). Removal of methyl orange and methylene blue dyes from aqueous solution using lala clam (Orbicularia orbiculata) shell.AIP Conference Proceedings, 1847. https:///10.1063/1.4983899Elwakeel, K. Z., Elgarahy, A. M., & Mohammad, S. H. (2017). Use of beach bivalve shells located at Port Said coast (Egypt) as a green approach for methylene blue removal. Journal of Environmental Chemical Engineering, 5(1), 578–587. https:///10.1016/j.jece.2016.12.032Faisal, A. A. H., Khalid, Z., Al-ansari, N., & Sharma, G. (2021). Chemosphere Precipitation of ( Mg / Fe-CTAB ) - Layered double hydroxide nanoparticles onto sewage sludge for producing novel sorbent to remove Congo red and methylene blue dyes from aqueous environment. Chemosphere, September, 132693. https:///10.1016/j.chemosphere.2021.132693Fajarwati, F. I., Ika Yandini, N., Anugrahwati, M., & Setyawati, A. (2020).Adsorption Study of Methylene Blue and Methyl Orange Using Green Shell (Perna Viridis). EKSAKTA: Journal of Sciences and Data Analysis, 1(1), 92–97. https:///10.20885/eksakta.vol1.iss1.art14Fosso-Kankeu, E., Webster, A., Ntwampe, I. O., & Waanders, F. B. (2017).Coagulation/Flocculation Potential of Polyaluminium Chloride and Bentonite Clay Tested in the Removal of Methyl Red and Crystal Violet. Arabian Journal for Science and Engineering, 42(4), 1389–1397.https:///10.1007/s13369-016-2244-xGhaedi, M., Hajjati, S., Mahmudi, Z., Tyagi, I., Agarwal, S., Maity, A., & Gupta, V. K. (2015). Modeling of competitive ultrasonic assisted removal of the dyes - Methylene blue and Safranin-O using Fe3O4 nanoparticles. Chemical Engineering Journal, 268, 28–37. https:///10.1016/j.cej.2014.12.090 Hevira, L., Rahmi, A., Zein, R., Zilfa, Z., & Rahmayeni, R. (2020). The fast and of low-cost-adsorbent to the removal of cationic and anionic dye using chicken eggshell with its membrane. Mediterranean Journal of Chemistry, 10(3), 294–301. https:///10.13171/mjc020********lhHevira, L., Zilfa, Rahmayeni, Ighalo, J. O., Aziz, H., & Zein, R. (2021). Terminalia catappa shell as low-cost biosorbent for the removal of methylene blue from aqueous solutions. Journal of Industrial and Engineering Chemistry, 97, 188–199. https:///10.1016/j.jiec.2021.01.028Hevira, L., Zilfa, Rahmayeni, Ighalo, J. O., & Zein, R. (2020). Biosorption of indigo carmine from aqueous solution by Terminalia Catappa shell. Journal of Environmental Chemical Engineering, 8(5), 104290.https:///10.1016/j.jece.2020.104290Hubbe, M. A., Azizian, S., & Douven, S. (2019). Implications of apparent pseudo-second-order adsorption kinetics onto cellulosic materials: A review.BioResources, 14(3), 7582–7626. https:///10.15376/biores.14.3.7582-7626Ighalo, J. O., & Adeniyi, A. G. (2020). A mini-review of the morphological properties of biosorbents derived from plant leaves. SN Applied Sciences, 2(3).https:///10.1007/s42452-020-2335-xIgwegbe, C. A., Mohmmadi, L., Ahmadi, S., Rahdar, A., Khadkhodaiy, D., Dehghani, R., & Rahdar, S. (2019). Modeling of adsorption of Methylene Blue dye on Ho-CaWO4 nanoparticles using Response Surface Methodology (RSM) and Artificial Neural Network (ANN) techniques. MethodsX, 6, 1779–1797. https:///10.1016/j.mex.2019.07.016Jafari, M., Vanoppen, M., van Agtmaal, J. M. C., Cornelissen, E. R., Vrouwenvelder, J. S., Verliefde, A., van Loosdrecht, M. C. M., & Picioreanu,C. (2021). Cost of fouling in full-scale reverse osmosis and nanofiltrationinstallations in the Netherlands. Desalination, 500(December 2020), 114865.https:///10.1016/j.desal.2020.114865Jawad, A. H., Rashid, R. A., Ishak, M. A. M., & Ismail, K. (2018). Adsorptive removal of methylene blue by chemically treated cellulosic waste banana ( Musa sapientum ) peels . Journal of Taibah University for Science, 12(6), 809–819. https:///10.1080/16583655.2018.1519893Jawad, A. H., Rashid, R. A., Ishak, M. A. M., & Wilson, L. D. (2016). Adsorption of methylene blue onto activated carbon developed from biomass waste by H2SO4 activation: kinetic, equilibrium and thermodynamic studies.Desalination and Water Treatment, 57(52), 25194–25206.https:///10.1080/19443994.2016.1144534Katneni, V. K., Shekhar, M. S., Jangam, A. K., Prabhudas, S. K., Krishnan, K., Kaikkolante, N., Paran, B. C., Baghel, D. S., Koyadan, V. K., Jena, J., & Mohapatra, T. (2020). Novel Isoform Sequencing Based Full-Length Transcriptome Resource for Indian White Shrimp, Penaeus indicus. Frontiers in Marine Science, 7(December), 1–4.https:///10.3389/fmars.2020.605098Kusumawardani, R., Rismawati, A., Retnowati, R., So, H., & Variabel, C. (2018).MODIFIKASI BIJI PEPAYA SEBAGAI BIOSORBEN ZAT WARNA TEKSTIL MORDANT BLACK 11 MODIFICATION OF PAPAYA SEEDS AS BIOSORBENT COLORS OF MORDANT BLACK 11 TEXTILES biosorben biji pepaya untuk mengadsorpsi zat warna tekstil mordant black 11 . Prinsip kerja dari mor. 1(2), 92–95.Li, W. (2011). Quantitative Analysis of the Reaction between Gliadin and Citric Acid under Weak Acidic and Weak Alkaline Conditions. Thesis.Liu, L., Fan, S., & Li, Y. (2018). Removal behavior of methylene blue from aqueous solution by tea waste: Kinetics, isotherms and mechanism. International Journal of Environmental Research and Public Health, 15(7).https:///10.3390/ijerph15071321Makeswari, M., Santhi, T., & Ezhilarasi, M. R. (2016). Adsorption of methylene blue dye by citric acid modified leaves of Ricinus communis from aqueous solutions. 8(7), 452–462.Mohan, C. (2003). A guide for the preparation and use of buffers in biological systems.Naghizadeh, A., & Ghafouri, M. (2017). Synthesis and performance evaluation of chitosan prepared from Persian gulf shrimp shell in removal of reactive blue29 dye from aqueous solution (Isotherm, thermodynamic and kinetic study).Iranian Journal of Chemistry and Chemical Engineering, 36(3), 25–36. Pang, Y. L., Tan, J. H., Lim, S., & Chong, W. C. (2021). A state-of-the-art review on biowaste derived chitosan biomaterials for biosorption of organic dyes: Parameter studies, kinetics, isotherms and thermodynamics. Polymers, 13(17).https:///10.3390/polym13173009Pathak, P. D., Mandavgane, S. A., & Kulkarni, B. D. (2016). Characterizing fruit and vegetable peels as bioadsorbents. Current Science, 110(11), 2114–2123.https:///10.18520/cs/v110/i11/2114-2123Ponnusami, V., Vikram, S., & Srivastava, S. N. (2008). Guava (Psidium guajava) leaf powder: Novel adsorbent for removal of methylene blue from aqueous solutions. Journal of Hazardous Materials, 152(1), 276–286.https:///10.1016/j.jhazmat.2007.06.107Pratiwi, R. (2018). ASPEK BIOLOGI DAN ABLASI MATA PADA UDANG WINDU Penaeus monodon SUKU PENAEIDAE (DECAPODA: MALACOSTRACA). Oseana, 43(2), 34–47.https:///10.14203/oseana.2018.vol.43no.2.19Purnomo, J. S. (2022). PENINGKATAN KAPASITAS ADSORPSI METHYLENE BLUE SECARA BATCH MENGGUNAKAN AMPAS DAUN SERAI WANGI (Cymbopogon nardus L. Rendle) YANG DIMODIFIKASI DENGAN ASAM SITRAT. In Universitas Andalas (Issue8.5.2017).Qin, L., Zhou, Z., Dai, J., Ma, P., Zhao, H., He, J., Xie, A., Li, C., & Yan, Y. (2016).Novel N-doped hierarchically porous carbons derived from sustainable shrimp shell for high-performance removal of sulfamethazine and chloramphenicol.Journal of the Taiwan Institute of Chemical Engineers, 62, 228–238.https:///10.1016/j.jtice.2016.02.009Rafatullah, M., Sulaiman, O., Hashim, R., & Ahmad, A. (2010). Adsorption of methylene blue on low-cost adsorbents: A review. Journal of Hazardous Materials, 177(1–3), 70–80. https:///10.1016/j.jhazmat.2009.12.047 Rahmayeni, R., Oktavia, Y., Stiadi, Y., Arief, S., & Zulhadjri, Z. (2021). Spinel ferrite of MnFe2O4 synthesized in Piper betle Linn extract media and its application as photocatalysts and antibacterial. Journal of Dispersion Science and Technology, 42(3), 465–474.https:///10.1080/01932691.2020.1721011Rajumon, R., Anand, J. C., Ealias, A. M., Desai, D. S., George, G., & Saravanakumar, M. P. (2019). Adsorption of textile dyes with ultrasonic assistance using green reduced graphene oxide: An in-depth investigation on sonochemical factors. Journal of Environmental Chemical Engineering, 7(6), 103479. https:///10.1016/j.jece.2019.103479Ramadhani, P., Chaidir, Z., Zilfa, Tomi, Z. B., Rahmiarti, D., & Zein, R. (2020).Shrimp shell (Metapenaeus monoceros) waste as a low-cost adsorbent for metanil yellow dye removal in aqueous solution. Desalination and Water Treatment, 197, 413–423. https:///10.5004/dwt.2020.25963 Ramrakhiani, L., Ghosh, S., & Majumdar, S. (2016). Surface Modification of Naturally Available Biomass for Enhancement of Heavy Metal Removal Efficiency, Upscaling Prospects, and Management Aspects of Spent Biosorbents: A Review. Applied Biochemistry and Biotechnology, 180(1), 41–78. https:///10.1007/s12010-016-2083-yRápó, E., & Tonk, S. (2021). Factors affecting synthetic dye adsorption; desorption studies: A review of results from the last five years (2017–2021). Molecules, 26(17). https:///10.3390/molecules26175419Ravi, & Pandey, L. M. (2019). Enhanced adsorption capacity of designed bentonite and alginate beads for the effective removal of methylene blue. Applied Clay Science, 169(October 2018), 102–111.https:///10.1016/j.clay.2018.12.019Ribeiro, C., Scheufele, F. B., Espinoza-Quiñones, F. R., Módenes, A. N., da Silva,M. G. C., Vieira, M. G. A., & Borba, C. E. (2015). Characterization of Oreochromis niloticus fish scales and assessment of their potential on the adsorption of reactive blue 5G dye. Colloids and Surfaces A: Physicochemical and Engineering Aspects, 482, 693–701.https:///10.1016/j.colsurfa.2015.05.057Sadaf, S., & Bhatti, H. N. (2014). Batch and fixed bed column studies for the removal of Indosol Yellow BG dye by peanut husk. Journal of the Taiwan Institute of Chemical Engineers, 45(2), 541–553.https:///10.1016/j.jtice.2013.05.004Safa, Y., & Bhatti, H. N. (2011). Adsorptive removal of direct dyes by low cost rice husk : Effect of treatments and modifications. 10(16), 3128–3142.https:///10.5897/AJB10.1302Sandip, Q. (2015). Journal of Industrial and Engineering Chemistry Efficient adsorption and photocatalytic degradation of Rhodamine B dye over Bi 2 O 3 -bentonite nanocomposites : A kinetic study. 1–8.Sawasdee, S., & Watcharabundit, P. (2016). Effect of temperature on brilliant green adsorption by shrimp shell: Equilibrium and kinetics. Chiang Mai University Journal of Natural Sciences, 15(3), 221–236.https:///10.12982/cmujns.2016.0017Senthil Kumar, P., Janet Joshiba, G., Femina, C. C., Varshini, P., Priyadharshini, S., Arun Karthick, M. S., & Jothirani, R. (2019). A critical review on recent developments in the low-cost adsorption of dyes from wastewater.Desalination and Water Treatment, 172, 395–416.https:///10.5004/dwt.2019.24613Shakoor, S., & Nasar, A. (2016). Removal of methylene blue dye from artificially contaminated water using citrus limetta peel waste as a very low cost adsorbent. Journal of the Taiwan Institute of Chemical Engineers, 66, 154–163. https:///10.1016/j.jtice.2016.06.009Sun, C., Qiu, J., Zhang, Z., Marhaba, T. F., Zhang, Y., & Zhang, W. (2016).Characterization of Citric Acid-Modified Clam Shells and Application for Aqueous Lead (II) Removal. Water, Air, and Soil Pollution, 227(9).https:///10.1007/s11270-016-2975-zSuryawanshi, N., Jujjavarapu, S. E., & Ayothiraman, S. (2019). Marine shell industrial wastes–an abundant source of chitin and its derivatives: constituents, pretreatment, fermentation, and pleiotropic applications-a revisit.International Journal of Environmental Science and Technology, 16(7), 3877–3898. https:///10.1007/s13762-018-02204-3Taylor, P., Wang, P., Ma, Q., Hu, D., & Wang, L. (2015). Desalination and Water Treatment Adsorption of methylene blue by a low-cost biosorbent : citric acid modified peanut shell. May, 37–41.https:///10.1080/19443994.2015.1033651Taylor, P., Zhou, Y., Zhang, R., Gu, X., Lu, J., Zhou, Y., Zhang, R., Gu, X., & Lu, J. (2015). Separation Science and Technology Adsorption of Divalent Heavy Metal Ions from Aqueous Solution by Citric Acid Modified Pine Sawdust Adsorption of Divalent Heavy Metal Ions from Aqueous Solution by Citric Acid Modified Pine Sawdust. January, 37–41.https:///10.1080/01496395.2014.956223Thamaraiselvan, C., Lerman, S., Weinfeld-Cohen, K., & Dosoretz, C. G. (2018).Characterization of a support-free carbon nanotube-microporous membrane for water and wastewater filtration. Separation and Purification Technology, 202(March), 1–8. https:///10.1016/j.seppur.2018.03.038Xu, Y., Liu, Y., Liu, S., Tan, X., Zeng, G., Zeng, W., Ding, Y., Cao, W., & Zheng,B. (2016). Enhanced adsorption of methylene blue by citric acid modificationof biochar derived from water hyacinth (Eichornia crassipes). Environmental Science and Pollution Research, 23(23), 23606–23618.https:///10.1007/s11356-016-7572-6Zein, R. (2019). Pensi shell (Corbicula moltkiana)as a biosorbent for metanil yellow dyes removal: pH and equilibrium model evaluation. Jurnal Litbang Industri, 15–22.Zein, R., Chaidir, Z., Fauzia, S., & Ramadhani, P. (2022). Isotherm and Kinetic Studies on the Adsorption Behavior of Metanil Yellow Dyes onto Modified Shrimp Shell-Polyethylenimine ( SS-PEI ). 8(May), 10–22.https:///10.15408/jkv.v8i1.22566Zein, R., Purnomo, J. S., Ramadhani, P., Alif, M. F., & Safni, S. (2022).Lemongrass ( Cymbopogon nardus ) leaves biowaste as an effective and low-cost adsorbent for methylene blue dyes removal: isotherms, kinetics, and thermodynamics studies . Separation Science and Technology, 00(00), 1–17.https:///10.1080/01496395.2022.2058549Zein, R., Tomi, Z. B., Fauzia, S., & Zilfa, Z. (2020). Modification of rice husk silica with bovine serum albumin (BSA) for improvement in adsorption of metanil yellow dye. Journal of the Iranian Chemical Society, 17(10), 2599–2612.https:///10.1007/s13738-020-01955-6Zhou, Y., Ge, L., Fan, N., & Xia, M. (2018). Adsorption of Congo red from aqueous solution onto shrimp shell powder. Adsorption Science and Technology, 36(5–6), 1310–1330. https:///10.1177/0263617418768945。
活性炭吸附的工艺流程
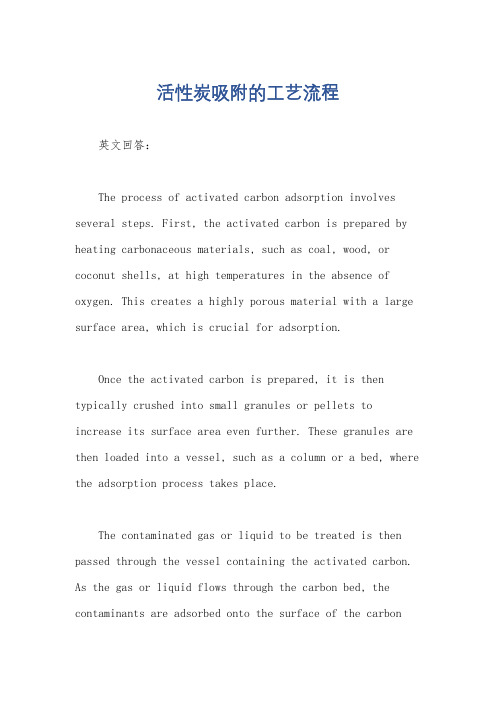
活性炭吸附的工艺流程英文回答:The process of activated carbon adsorption involves several steps. First, the activated carbon is prepared by heating carbonaceous materials, such as coal, wood, or coconut shells, at high temperatures in the absence of oxygen. This creates a highly porous material with a large surface area, which is crucial for adsorption.Once the activated carbon is prepared, it is then typically crushed into small granules or pellets to increase its surface area even further. These granules are then loaded into a vessel, such as a column or a bed, where the adsorption process takes place.The contaminated gas or liquid to be treated is then passed through the vessel containing the activated carbon. As the gas or liquid flows through the carbon bed, the contaminants are adsorbed onto the surface of the carbonparticles. This is due to the attractive forces between the contaminants and the carbon surface.The adsorption capacity of activated carbon isinfluenced by various factors, such as the type and concentration of contaminants, the contact time between the carbon and the contaminants, and the temperature and pressure conditions. For example, higher concentrations of contaminants or longer contact times can lead to higher adsorption capacities.Once the activated carbon is saturated with contaminants, it needs to be regenerated or replaced. Regeneration involves heating the carbon to a high temperature in the presence of steam or other gases to remove the adsorbed contaminants. This restores the adsorption capacity of the carbon, allowing it to be reused.In some cases, the activated carbon may need to be replaced if regeneration is not possible or cost-effective. The spent carbon can then be disposed of or sent forfurther treatment, such as incineration or landfilling.Overall, the process of activated carbon adsorption is a highly effective and widely used method for removing contaminants from gases and liquids. Its versatility, high adsorption capacity, and ease of regeneration make it a popular choice in various industries, including water and wastewater treatment, air purification, and gas separation.中文回答:活性炭吸附的工艺流程包括几个步骤。
响应面法优化浓香型白酒降度除浊工艺研究
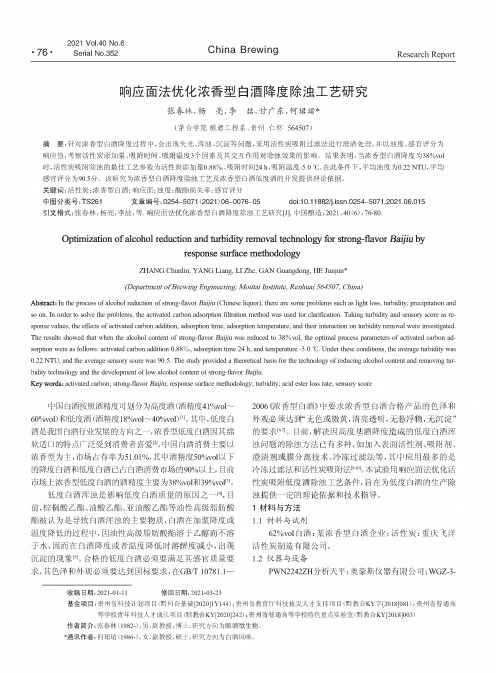
2021 Vol.40 No.6• 76 •Serial No.352China BrewingResearch Report响应面法优化浓香型白酒降度除浊工艺研究张春林,杨亮-李!,甘广东-何(茅台学院酿酒工程系+贵州仁怀564507)摘 要:针对浓香型白酒降度过程中,会出现失光、浑浊、沉淀等问题,采用活性炭吸附过滤法进行澄清处理,并以浊度、感官评分为响应值,考察活性炭添加量、 间、 温度3个因素及其交互作对浊效果的影响。
结果 ,当浓香型白酒降度为3*%$ol 收稿日期*2021-01-11修回日期*2021-03-23基金项0*贵州省科技计划项目(黔科合基础[2020/1Y144)贵州省教育厅科技拔尖人才支持项目(黔教合KY ([2018/081)贵州省普通高等学校青年科技人才成长项目((合KY [2020/242)普通高等学校特色重点实验室(黔教合KY.2018/003)作者简介*张春林(1982-),男,副教授,博士,研究方向为酿酒 物。
*通讯作者*何>>(1986-),女,副教授,硕士,研究方向为白酒风味。
时,活性炭吸附除浊的最佳工艺参数为活性炭添加量0、吸附时间24h 、吸附温度<5=0!。
在此条件下,平均浊度为0.22 NTU ,平均 感评分为30.5分。
该研究为浓香型白酒降度浊 及浓香型白酒低度酒的开发。
关键词:活性炭;浓香型白酒;响应面;浊度;酸酯损失率;感官评分中图分类号:TS261 文章编号 *0254-5071(2021)06-0076-05 doi:10.11882/j.issn.0254-5071.2021.06.015引文格式:张春林,杨亮,李詰,等•响 浓香型白酒降度除浊工艺研究[J ].中国酿造,2021,40(6):76-80.Optimization of alcohol reduction and turbidity removal technology for strong-flavor Baijiu byresponse surface methodologyZHANG Chunlin, YANG Liang, LI Zhe, GAN Guangdong, HE Junjun *(Departoent of B rewing Engineering, Moutai Institute, Renhuai 564507, China)Abstract : In the process of alcohol reduction of strong-flavor Baijiu (Chinese liquor), there are some problems such as light loss, turbidity, precipitation andso on. In order to solve the problems, the activated carbon adsorption filtration method was used for clarification. Taking turbidity and sensory score as re sponse values, the effects of activated carbon addition, adsorption time, adsorption temperature, and their interaction on turbidity removal gere investigated.The results showed that when the alcohol content of strong-flavor Baijiu was reduced to 38%vol, the optimal process parameters of activated carbon ad sorption were as follows: activated carbon addition 0.88", adsorption time 24 h, and temperature -5.0 !. Under these conditions, the average turbidity was 0.22 NTU, and the average sensory score was 90.5. The study provided a theoretical basis for the technology of reducing alcohol content and removing tur bidity technology and the development of low alcohol content of strong-flavor Baijiu .Key words : activated carbon; strong-flavor Baijiu ; response surface methodology; turbidity; acid ester loss rate; sensory score中国白酒按照酒精度可划分为高度酒(酒精度41%vol 〜60%voD 和低度酒(酒精度18%vol 〜40%vol )m 。
活性炭表面物理化学性质对溴酸盐吸附的影响
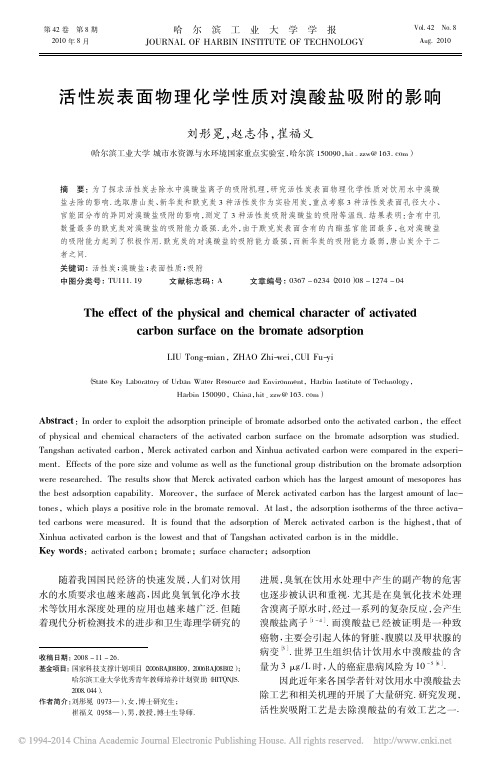
第42卷第8期2010年8月哈尔滨工业大学学报JOURNAL OF HARBIN INSTITUTE OF TECHNOLOGYVol.42No.8Aug.2010活性炭表面物理化学性质对溴酸盐吸附的影响刘彤冕,赵志伟,崔福义(哈尔滨工业大学城市水资源与水环境国家重点实验室,哈尔滨150090,hit -zzw@163.com )摘要:为了探求活性炭去除水中溴酸盐离子的吸附机理,研究活性炭表面物理化学性质对饮用水中溴酸盐去除的影响.选取唐山炭、新华炭和默克炭3种活性炭作为实验用炭,重点考察3种活性炭表面孔径大小、官能团分布的异同对溴酸盐吸附的影响,测定了3种活性炭吸附溴酸盐的吸附等温线.结果表明:含有中孔数量最多的默克炭对溴酸盐的吸附能力最强.此外,由于默克炭表面含有的内酯基官能团最多,也对溴酸盐的吸附能力起到了积极作用.默克炭的对溴酸盐的吸附能力最强,而新华炭的吸附能力最弱,唐山炭介于二者之间.关键词:活性炭;溴酸盐;表面性质;吸附中图分类号:TU111.19文献标志码:A文章编号:0367-6234(2010)08-1274-04The effect of the physical and chemical character of activatedcarbon surface on the bromate adsorptionLIU Tong-mian ,ZHAO Zhi-wei ,CUI Fu-yi(State Key Laboratory of Urban Water Resource and Environment ,Harbin Institute of Technology ,Harbin 150090,China ,hit -zzw@163.com )Abstract :In order to exploit the adsorption principle of bromate adsorbed onto the activated carbon ,the effect of physical and chemical characters of the activated carbon surface on the bromate adsorption was studied.Tangshan activated carbon ,Merck activated carbon and Xinhua activated carbon were compared in the experi-ment.Effects of the pore size and volume as well as the functional group distribution on the bromate adsorption were researched.The results show that Merck activated carbon which has the largest amount of mesopores has the best adsorption capability.Moreover ,the surface of Merck activated carbon has the largest amount of lac-tones ,which plays a positive role in the bromate removal.At last ,the adsorption isotherms of the three activa-ted carbons were measured.It is found that the adsorption of Merck activated carbon is the highest ,that of Xinhua activated carbon is the lowest and that of Tangshan activated carbon is in the middle.Key words :activated carbon ;bromate ;surface character ;adsorption 收稿日期:2008-11-26.基金项目:国家科技支撑计划项目(2006BAJ08B09,2006BAJ08B02);哈尔滨工业大学优秀青年教师培养计划资助(HITQNJS.2008.044).作者简介:刘彤冕(1973—),女,博士研究生;崔福义(1958—),男,教授,博士生导师.随着我国国民经济的快速发展,人们对饮用水的水质要求也越来越高,因此臭氧氧化净水技术等饮用水深度处理的应用也越来越广泛.但随着现代分析检测技术的进步和卫生毒理学研究的进展,臭氧在饮用水处理中产生的副产物的危害也逐步被认识和重视.尤其是在臭氧化技术处理含溴离子原水时,经过一系列的复杂反应,会产生溴酸盐离子[1-4].而溴酸盐已经被证明是一种致癌物,主要会引起人体的肾脏、腹膜以及甲状腺的病变[5].世界卫生组织估计饮用水中溴酸盐的含量为3μg /L 时,人的癌症患病风险为10-5[6].因此近年来各国学者针对饮用水中溴酸盐去除工艺和相关机理的开展了大量研究.研究发现,活性炭吸附工艺是去除溴酸盐的有效工艺之一.而且针对活性炭去除溴酸盐离子的反应机理做了相关研究.研究发现,活性炭去除溴酸盐离子的能力与活性炭本身的性质和原水水质有关[7-8].此外还发现,活性炭表面是否有金属离子的存在不会对溴酸盐离子的吸附产生影响[9].关于活性炭表面的化学性质对溴酸盐吸附性能的影响的研究也已经开展[10].本文针对活性炭表面的物理化学性质对活性炭去除溴酸盐性能的影响进行深入研究.包括活性炭表面孔径大小和分布情况对溴酸盐吸附性能的影响;各种官能组成的不同对溴酸盐吸附性能的影响.为活性炭吸附溴酸盐工艺的实际应用提供理论基础.1实验1.1材料BrO3-混合溶液的配制采用分析纯KBrO3试剂,按照适宜的比例在蒸馏水中按特定质量浓度进行配置.实验中选取3种不同类型活性炭,分别为德国默克产品(AC m),唐山产活性炭(AC t)和新华公司产活性炭(AC x).各种炭的物理性质见表1.表1实验用3种活性炭的物理性质炭种平均粒径/mm比表面积/(m2·g-1)填充密度/(g·mL-1)硬度/(%min)碘值/(mg·g-1)磨损值/(%min)灰分/%水分/%AC m 1.211970.42 0.6397127562<3 1.3 AC t 1.59270.44 0.689397384 4.5 1.6 AC x 1.49630.38 0.5991981906 2.5实验之前,先将活性炭进行筛分,确保实验用的活性炭的平均粒度在230 330目之内.然后用去离子水进行清洗.清洗完毕后在175ħ温度下烘烤1周,去除活性炭表面的挥发性物质;然后放入105ħ干燥箱内.用之前放入干燥器内冷却至室温.1.2方法活性炭表面物理化学性质的测定.活性炭表面的孔径分布,大孔、中孔和微孔的数量均根据N2在77K条件下的吸附、脱附等温线测定.实验中使用Dollimore-Heal法测定活性炭表面的孔径分布和中孔的数量[11];使用T-点法测定微孔的数量[12].活性炭的表面化学性质根据Boehm滴定法测定.溴酸盐检测方法.溴酸盐采用离子色谱检测法检测.离子色谱:ICS-3000.IonpacAS19色谱柱,淋洗液为30mmol/L的KOH溶液,流速为0.9mL/min,电导检测器,检测池温度为30ħ.实验装置.实验中,精确称取50mg磨细的并烘干的颗粒活性炭,依次放入10个150mL的细口瓶中,加入100mL配好的原水(B r O3-质量浓度为500μg/L),盖好盖子,在数显恒温水浴振荡器中振荡,实验温度为25ħ,振荡方式采用的是往复式振荡.每间隔一段时间取出一个细口瓶,过滤后测定溴酸盐.取样时间分别为15、30、45min 及l、1.5、2、2.5、3、4h和5h.2结果与讨论2.1活性炭表面的孔体积容量及官能团的种类表2是3种活性炭表面孔体积容量和官能团的分布情况.从表中可以看出,默克炭表面含有的羧基和内酯基最多,分别为0.05mmol/g和0.075mmol/g,但是含有的碱性基团最少,只有0.425mmol/g.而新华炭含有的碱性基团最多,达到了0.925mmol/g,其他官能团则具有最少的含有量.唐山炭介于二者之间.表2活性炭表面的孔体积容量与官能团的种类及含量炭种含量/(mmol·g-1)羧基内酯基酚羟基碱性基团孔体积容量/(cm3·g-1)中孔微孔唐山炭00.0250.150.7250.7840.231新华炭0.0250.0250.10.9250.7210.344默克炭0.050.0750.1250.4250.8530.192另外,默克炭表面含有的中孔数量最多,达到了0.853cm3/g,但是含有的微孔最少,只有0.192cm3/g.而新华炭含有的微孔最多,中孔则具有最少的含有量.唐山炭介于二者之间.2.2溴酸盐吸附性能曲线图1、图2分别为活性炭吸附量和溶液含量的比值随吸附时间的变化曲线和溶液中溴酸盐质量浓度随吸附时间的变化曲线.从图1和图2可以看出,不同种类的活性炭对溴酸盐的吸附速度和去除效果是有所不同的.唐山炭对溴酸盐的总的去除率为63.4%,吸附后水中的溴酸盐为183μg/L,在对溴酸盐的吸附过·5721·第8期刘彤冕,等:活性炭表面物理化学性质对溴酸盐吸附的影响程中可以发现,吸附30min 后,C /C 0(活性炭吸附量/溶液中溴酸盐质量浓度)为0.53,GAC 对水中的溴酸盐的去除率为34.6%,在随后的2h ,对其去除率都是小幅度的上升,到吸附3h ,去除率达到40.5%,基本达到稳定状态.30min 内的去除率占总去除率的85.4%.在吸附的3h 内,去除率占总去除率的93.7%.可见,该活性炭吸附溴酸盐时,达到吸附饱和所需要的时间为3h.在30min 内,活性炭对溴酸盐的平均吸附速度为0.47mg /(g ·h ),5h 的平均吸附速度分别为0.63mg /(g ·h ).C /C 0t /min图1活性炭吸附量和溶液含量的比值与吸附时间的关系时间/min溴酸盐质量浓度/(滋g /L -1)图2溶液中溴酸盐质量浓度与吸附时间的关系新华炭对水中溴酸盐的总去除率为31.6%.吸附后的溴酸盐为342μg /L.在对溴酸盐的吸附过程中,15min 时,新华炭对溴酸盐的去除率达到31.6%.平均吸附速度为0.35mg /(g ·h ).吸附的前15min 内平均吸附速度为0.63mg /(g ·h ).最大吸附速度出现在0 15min.默克炭对溴酸盐的总的去除率为82.8%,吸附后的溴酸盐为86μg /L.在对溴酸盐的吸附过程中,45min 时,默克炭对溴酸盐的去除率达到74.4%,占总去除率的89.8%.平均吸附速度为0.83mg /(g ·h ),吸附的前45min 内的平均吸附速度为0.496mg /(g ·h ).因此该活性炭对溴酸盐的吸附效果要好于其他炭,并且在短时间内能达到良好的吸附效果.默克炭对溴酸盐的吸附速度最快,30min 内对溴酸盐的去除率为72%.在以后的4h 内,对其去除率都是小幅度上升,吸附4h ,去除率达到82.4%,基本达到稳定状态.可见,该活性炭吸附溴酸盐时,达到吸附饱和所需要的时间为4h.在30min 内,活性炭对溴酸盐的平均吸附速度为0.72mg /(g ·h ).从实验结果可以看出,默克炭对溴酸盐的吸附能力最强,而新华炭的吸附能力最弱.而这种实验结果与活性炭表面的性质是密不可分的.国外学者经过实验已经证明,活性炭吸附溴酸盐的最大吸附量是和中孔数量呈正相关性,而本研究再次证明了这个观点.通过检测发现,默克炭含有的中孔数量最多,实验结果发现默克炭对溴酸盐的吸附能力也最强.而含有中孔数量最少的新华炭则具有最弱的吸附能力.一般来说,活性炭表面含氧官能团中酸性化合物越丰富的活性炭在吸附极性化合物时应具有较高的效率,而碱性化合物较多的活性炭易吸附极性较弱或非极性物质.默克炭的酸性基团中羧基和内酯基都要高于另两种炭,而碱性基团要少于其他炭,故在吸附溴酸盐极性物质时,具有较高的效率.2.3溴酸盐吸附等温线本文用Freundlich 公式来表征活性炭吸附溴酸盐的吸附等温线.lg q e =lg k f +1()n lg C e.其中:q e 为平衡吸附容量,mg /g ;k f 为Freundlich 系数,(mg /g )/(mg /L )1/n ;1n 为Freundlich 指数;C e 为溶液平衡浓度,mg /L.图3—图5分别为默克炭、新华炭和唐山炭的吸附等温线.各炭的Freundlich 吸附等温式参数如表3所示,由表3可知默克炭吸附等温线的斜率1/n 最小,为0.1773,次之为唐山炭,1/n 为3.624,而新华炭1/n 最大,达到6.9129.由前述各活性炭对溴酸盐吸附性能比较可知默克炭对溴酸盐吸附性能最好,唐山炭次之,新华炭最差.可见,1/n 可以反应活性炭对溴酸盐吸附性能,1/n 越小,吸附性能越好.反之,吸附性能越差.32.92.82.72.62.52.42.42.422.442.462.482.52.522.54lg C el g q图3唐山炭的吸附等温线·6721·哈尔滨工业大学学报第42卷22.57 2.582.592.62.61 2.622.632.64lg C el g q图4新华炭的吸附等温线3.23.132.92.82.70.51 1.522.5lg C el g q图5默克炭的吸附等温线表3溴酸盐离子在活性炭上吸附平衡参数炭种log k f 1/n R 2默克炭 2.93530.17730.978新华炭-15.68 6.91290.9674唐山炭-6.20263.6240.93773结论1)在活性炭吸附溴酸盐过程中,活性炭表面中孔的数量起到关键的作用.经过检测,默克炭表面的中孔数量最多,唐山炭次之,新华炭最低.实验结果发现,默克炭对溴酸盐的吸附能力最强,唐山炭次之,新华炭则最弱.2)默克炭的酸性基团中羧基和内酯基都要高于其他两种炭,而碱性基团要少于其他炭,故在吸附溴酸盐极性物质时,具有较高的效率,也是默克炭对溴酸盐离子吸附能力强的一个原因.3)通过对默克炭、新华炭和唐山炭的Freun-dlich 吸附等温线比较发现,默克炭附等温线的斜率1/n 最小,而新华炭的1/n 最大,唐山炭介于二者之间.1/n 越小,活性炭对溴酸盐的吸附性能越好.参考文献:[1]VON GUNTEN U ,HOIGN J.Bromate formation duringozonation of bromide containing waters :interaction of o-zone and hydroxyl radical reactions [J ].Environ Sci Technol ,1994,28:1234-1242.[2]GLAZE W H ,WEINBERG H S ,CAVANAGH J E.E-valuating the formation of brominated DBPs during ozo-nation [J ].J Am Water Works Assoc ,1993,85:96-103.[3]KRASNER S W.Formation and control of BrO 3-duringozonation of waters containing bromide [J ].J Am Water Works Assoc ,1993,85:73-80.[4]SIDDIQUI M S ,AMY G L.Factors affecting DBP forma-tion during ozone bromate reactions [J ].J Am Water Works Assoc ,1994,86:63-72.[5]DELKER D G.Molecular biomarkers of oxidative stressassociated with bromate carcinogenicity [J ].Toxicolo-gy ,2006,221:158-165.[6]WHO.Bromate in drinking water :background documentfor of WHO guidelines for drinking-water quality [R ].Veneva :WHO ,2005:4-10.[7]KIRISITS M J ,SNOEYINK V L ,KRUITHOF J C.Thereduction of bromate by granular activated carbon [J ].Water Res ,2000,34:4250-4260.[8]BAO M L.Removal of bromate ion from water usinggranular activated carbon [J ].Water Res ,1999,33:2959-2970.[9]MILLER J ,SNOEYINK V L ,HARRELL S.The effectof granular activated surface chemistry on bromate re-duction [C ]//MINEAR R A ,AMY G L.Disinfection by-products in drinking water treatment :the chemistry of their formation and control.Boca Raton :CRC Press ,1996:293-309.[10]SIDDIQUI M S ,ZHAI W ,AMY G L ,et al.Bromateion removal by activated carbon [J ].Water Res ,1996,30:1651-1660.[11]DOLLIMORE D ,HEAL G R.An improved method forthe calculation of pore size distribution from adsorption data [J ].J Appl Chem ,1964,14:109-114.[12]LIPPENS B C ,DE BOER J H.Studies on pore systemin catalysts V :the t -method [J ].J Catal ,1965,4:319-323.(编辑魏希柱)·7721·第8期刘彤冕,等:活性炭表面物理化学性质对溴酸盐吸附的影响。
co2的end on和side on吸附

co2的end on和side on吸附Title: CO2 Adsorption: End-On and Side-On PerspectivesIntroduction:Carbon dioxide (CO2) adsorption is a crucial topic in the context of climate change and environmental sustainability. In this article, we will explore the fascinating mechanisms of CO2 adsorption from both the end-on and side-on perspectives. By understanding these processes, we can gain insights into the factors influencing CO2 capture and develop effective strategies to mitigate its impact on the environment.1. The End-On Adsorption Perspective:When CO2 molecules approach the surface of an adsorbent material, such as activated carbon or zeolites, they can undergo end-on adsorption. In this process, the CO2 molecule interacts with the surface through its oxygen atom, forming weak chemical bonds. This adsorption mechanism is influenced by factors such as temperature, pressure, and the nature of the adsorbent material.End-on adsorption offers several advantages. Firstly, it allows for a higher adsorption capacity, as multiple CO2 molecules can bind to the same adsorption site. Additionally, end-on adsorption provides enhanced selectivity for CO2 over other gases, making it a promisingtechnique for carbon capture and storage applications. However, the efficiency of end-on adsorption depends on the availability of suitable adsorbent materials and the optimization of operating conditions.2. The Side-On Adsorption Perspective:In contrast to the end-on adsorption, CO2 molecules can also undergo side-on adsorption. In this mode, the CO2 molecule interacts with the adsorbent surface through one of its carbon atoms. Side-on adsorption occurs when the surface functional groups of the adsorbent material can effectively interact with the CO2 molecule, leading to the formation of weak bonds.Side-on adsorption offers unique benefits in terms of selectivity and stability. The interaction between the adsorbent surface and the CO2 molecule in this mode is typically stronger, resulting in a higher adsorption energy. Moreover, side-on adsorption can facilitate the conversion of CO2 into value-added products, such as carbonates or other chemicals, thereby contributing to the development of sustainable technologies for CO2 utilization.Conclusion:CO2 adsorption plays a vital role in addressing the challenges posed by climate change. By exploring the end-on and side-on perspectives of CO2 adsorption, we have gained insights into the mechanisms andpotential applications of this process. Both end-on and side-on adsorption offer unique advantages, and their efficiency depends on various factors. Further research and development efforts are required to optimize the adsorption capacity, selectivity, and stability, ultimately contributing to the development of efficient carbon capture and utilization technologies. Let us embrace these perspectives and work towards a sustainable future.。
- 1、下载文档前请自行甄别文档内容的完整性,平台不提供额外的编辑、内容补充、找答案等附加服务。
- 2、"仅部分预览"的文档,不可在线预览部分如存在完整性等问题,可反馈申请退款(可完整预览的文档不适用该条件!)。
- 3、如文档侵犯您的权益,请联系客服反馈,我们会尽快为您处理(人工客服工作时间:9:00-18:30)。
This article was downloaded by: [Southwest University]On: 13 May 2013, At: 02:30Publisher: Taylor & FrancisInforma Ltd Registered in England and Wales Registered Number: 1072954 Registered office: Mortimer House, 37-41 Mortimer Street, London W1T 3JH, UKSeparation Science and TechnologyPublication details, including instructions for authors and subscription information:/loi/lsst20Adsorption of Carbon Dioxide onto Activated Carbonand Nitrogen-Enriched Activated Carbon: SurfaceChanges, Equilibrium, and Modeling of Fixed-BedAdsorptionTirzhá L. P. Dantas a c , Suélen M. Amorim a , Francisco Murilo T. Luna b , Ivanildo J. Silva Jr.b , Diana C. S. de Azevedo b , Alírio E. Rodriguesc & Regina F. P. M. Moreira aa Department of Chemical and Food Engineering, Federal University of Santa Catarina,Campus Universitário, Trindade, Florianópolis, Brazilb Department of Chemical Engineering, Federal University of Ceará, Fortaleza, CE, Brazilc Department of Chemical Engineering, Faculty of Engineering, University of Porto, Porto,PortugalPublished online: 07 Jan 2010.PLEASE SCROLL DOWN FOR ARTICLEAdsorption of Carbon Dioxide onto Activated Carbon and Nitrogen-Enriched Activated Carbon:Surface Changes,Equilibrium,and Modeling of Fixed-Bed AdsorptionTirzh aL.P.Dantas,1,3Sue ´len M.Amorim,1Francisco Murilo T.Luna,2Ivanildo J.Silva Jr.,2Diana C.S.de Azevedo,2Alı´rio E.Rodrigues,3and Regina F.P.M.Moreira 11Department of Chemical and Food Engineering,Federal University of Santa Catarina,Campus Universit a rio,Trindade,Florian o polis,Brazil 2Department of Chemical Engineering,Federal University of Cear a ,Fortaleza,CE,Brazil 3Department of Chemical Engineering,Faculty of Engineering,University of Porto,Porto,PortugalIt has been reported that the CO 2adsorption capacity of the N-enriched activated carbon can increase or decrease.In this study a commercial activated carbon was functionalized with 3-chloropro-pylamine hydrochloride and its adsorption characteristics in a fixed-bed column were investigated.The N-enriched activated carbon presented lower BET surface area than the original activated carbon suggesting that the nitrogen incorporation partially blocks the access of N 2to the small pores.Although the surface basicity has increased it is not accomplished by an increase of the capacity of the adsorption of the N-enriched activated carbon.The breakthrough curves in a fixed bed column were obtained at different temperatures (301K,323K,373K,and 423K)and a total pressure of 1.01bar using CO 2diluted in helium at two feed concentrations —10%and 20%(v/v).A model based on the Linear Driving Force (LDF)model for mass transfer was used to estimate the overall mass transfer coefficient and repro-duced the breakthrough curves satisfactorily.Keywords adsorption;carbondioxide;activatedcarbon;functionalizationINTRODUCTIONAccording to the IPCC (Intergovernmental Panel on Climate Change),some direct and indirect measurements have confirmed that the carbon dioxide concentration in the atmosphere has grown globally by around 100ppm (36%)in the last 250years:from 275–285ppm in the pre-industrial age to around 380ppm in 2005.Most of the CO 2released into the atmosphere results from fossil fuel burning and it is identified as one of themajor contributors to global warming and climate changes currently observed around the world.There are 3(three)possibilities available for reducing carbon dioxide emission (1).i.reducing the energy demand;ii.exchanging the current energy matrices for renewable energy,clean fuels,or nuclear energy and iii.carbon dioxide capture.A reduction in the energy demand is impracticable since industrial development has left us highly dependent on energy and fossil fuels represent approximately 85%of the current global energy consumption.The increase in the CO 2concentration in the atmosphere could be con-trolled with alternative energy sources;however,it is known that it will take a certain amount of time for tech-nologies that use renewable energies and clean fuels to be consolidated and for the production costs to fall.The pathways of CO 2separation through pre-combustion decarbonation (that is,CO 2capture after the gasification process and before the combustion stage),O 2=CO 2recycle combustion (using O 2instead of air com-bustor),or post-combustion separation (CO 2capture from the exhaustion gases)can be readily carried out using known technologies and processes.However,existing technologies still present a series of problems related to application in post-combustion separa-tion,including the great volume of the exhaustion gases and the cost-benefit ratio (2).Thus,the goal of reducing carbon dioxide emissions on an industrial scale requires the development of low-cost capture methods.Adsorption is an excellent method of post-combustion separation and has been shown to be an efficient alternative to separation since adsorbents have a high adsorption capacity,high selectivity,good mechanical properties,ease of regeneration Received 9October 2008;accepted 7August 2009.Address correspondence to Alı´rio E.Rodrigues,Laboratory of Separation and Reaction Engineering (LSRE),Department of Chemical Engineering,Faculty of Engineering,University of Porto,Rua Dr.Roberto Frias s/n,Porto 4200-465,Portugal.Tel.:+351225081671;;Fax:+351225081674E-mail:arodrig@fe.up.ptSeparation Science and Technology ,45:73–84,2010Copyright #Taylor &Francis Group,LLC ISSN:0149-6395print =1520-5754online DOI:10.1080/0149639090340176273D o w n l o a d e d b y [S o u t h w e s t U n i v e r s i t y ] a t 02:30 13 M a y 2013and they remain stable over repeated adsorption =desorption cycles (3).Activated carbon is a suitable adsorbent and its CO 2adsorption characteristics are dependent on its surface area and chemical surface characteristics.The surface chemistry of activated carbon is determined by the amount and type of heteroatom,for example oxygen,nitrogen,etc.These heteroatoms exist in the acid,basic,or neutral form of organic functional groups (4).Therefore,the adsorption capacity of activated carbon for carbon dioxide is a func-tion of its pore structure and the properties of the surface chemistry (5).Nitrogen in a carbonaceous matrix can cause an increase in the basic group number and therefore modify the load distribution at the surface of the solid.Some authors have reported the positive effect of nitrogen incor-poration on the activated carbon adsorption of H 2S (6,7)and also SO x and NO x (8).Recently,some authors have investigated the modification of CO 2adsorption capacity on activated carbon (5,9,10,11,12)or other supports (13,14,15)through the enrichment of the surface with nitro-gen functionalities.Immobilized amines have demonstrated characteristics similar to those currently used typical absorption processes in liquid phase,with the advantage that the solids can be handled easily and reduce the problem of corrosion in the equipment (2).In this study,an amine was evaluated as a potential source of basic sites for CO 2capture.A commercial activated carbon was enriched with nitrogen by addition of 3-chloropropylamine hydrochloride (C 3H 8ClN.HCl)and its CO 2adsorption capacity and other characteristics were investigated.The solids adsorbents (commercial acti-vated carbon and nitrogen-enriched activated carbon)were characterized in terms of the apparent surface area,micro-pore volume,and pore size distribution.The surface changes caused by amine enrichment were investigated by TGA and FTIR analysis.The adsorption of carbon dioxide on the adsorbents packed in a fixed-bed was studied.The experimental breakthrough curves were obtained using two different fractions of carbon dioxide diluted in an inert gas (helium)at four different temperatures:301K,323K,373K,and 423K.A model based on the Linear Driving Force (LDF)approximation for mass transfer,taking into account the energy balance,was used to describe the adsorption kinetics of carbon dioxide.EXPERIMENTAL SECTIONThe gases used in this studied were provided by Air Liquide S.A (Portugal)or by White Martins Ltda (Brazil).Carbon Dioxide N48was provided by Air Liquide S.A (Portugal).This gas was used in the determination of the carbon dioxide adsorption equilibrium on activated carbon.Helium (99.995%),carbon dioxide (99.998%),and stan-dard mixtures (10%and 20%CO 2=He v =v)were provided by White Martins Ltd.Nitrogen-Enriched Activated Carbon PreparationThe commercial activated carbon used was Norit R2030(Norit,Netherlands)which was selected due to its high adsorption capacity for CO 2(16).The nitrogen-enriched activated carbon,denoted as CPHCl,was prepared in a way similar way to that as described by Gray et al.(13),mixing 10g of activated carbon with 500mL of 10À1M 3-chloropropylamine hydrochloride solution (melting point:421K–423K).The mixture was kept under constant stirring,at ambient temperature for 5hours.The CPHCl adsorbent was then left to dry for 12hours in an oven at 378K.Characterization of the AdsorbentsThe elemental analysis was carried out using CHNS EA1100equipment (CE Instruments,Italy).The textural characterization was performed by the nitrogen sorption measurements at 77K,using an auto-matic sorptometer,Autosorb 1C (Quantachome,USA).The N 2surface area obtained from the BET equation within the 0.001–0.2relative pressure range was calculated because,despite its controversial interpretation for acti-vated carbons,this parameter provides a value that can be useful when comparing the characteristics of different activated carbons.Thermogravimetric experiments were carried out with a TGA-50thermogravimetric analyzer (Shimadzu,Japan)in the temperature range of 303–1173,at a heating rate of 10K =min under nitrogen flow.Fourier transform infrared (FTIR)spectroscopy was used to qualitatively identify the chemical functionality of activated carbon.To obtain the observable adsorption spectra,the solids were grounded to an average diameter of ca.0.5m m.The transmission spectra of the samples were recorded using KBr pellets containing 0.1%of carbon.The pellets were 12.7mm in diameter and ca.1mm thick and were prepared in a manual hydraulic press set at 10ton.The spectra were measured from 4000to 400cm À1and recorded on a 16PC FTIR spectrometer (Perkin Elmer,USA).Adsorption Equilibrium IsothermsThe equilibrium of CO 2adsorption on commercial acti-vated carbon was measured at different temperatures of 301K,323K,373K,and 423K using the static method in a Rubotherm magnetic suspension microbalance (Bochum,Germany)up to approximately 5bar.The equi-librium of CO 2adsorption on CPHCl was measured at different temperatures of 298K,323K,373K,and 423K by the volumetric method,in an automatic sorptometer,74T.L.P.DANTAS ET AL.D o w n l o a d e d b y [S o u t h w e s t U n i v e r s i t y ] a t 02:30 13 M a y 2013Autosorb 1C (Quantachome,USA),up to approximately 1bar.Before the CO 2adsorption measurements,the solid samples were pre-treated for 12hours at 423K under vacuum.This temperature ensures that the amine is homo-geneously tethered to the solid surface without devolatilize or decompose it.Fixed Bed CO 2Adsorption Dynamics–Breakthrough CurvesThe experimental breakthrough curves were obtained by passing the gas mixture of CO 2diluted in helium through the packed column with the adsorbent:commercial acti-vated carbon or CPHCl.The solid adsorbent was pre-treated by passing helium at a flow rate of 30mL =min min and at 423K for 2hours.These breakthrough curves were obtained at 301K,323K,373K,and 423K.The total gas flow rate was 30mL =min at 1bar total pressure.Two different fractions,y F ,of CO 2diluted in helium were used:0.1and 0.2(v =v).The physical properties of the fixed-bed are given in Table 1.The reversibility of the adsorption was studied in deso-rption experiments by passing pure helium through the packed column at a total flow rate of 30mL =min.The column was located inside a furnace with controlled temperature.The flow of gas was controlled by a mass flow unit (Matheson,USA).A gas chromatographic modelCG35(CG Instrumentos Cientı´ficos,Brazil)equipped with a Porapak-N packed column (Cromacon,Brazil)and with a thermal conductivity detector (TCD)was used to moni-tor the carbon dioxide concentration at the bed exit,using helium as the reference gas.At regular time’s interval,1mL of the gas at the end of the column was taken,using a gas tight syringe and immediately analyzed by gas chromography.The experimental system (column and furnace)was con-sidered adiabatic because it was isolated with a layer of 0.10m of fiber glass and with a refractory material.DESCRIPTION OF MODEL FOR CO 2ADSORPTION IN THE FIXED-BEDThe model used to describe the fixed-bed dynamics is derived from the mass balance,taking into account theenergy balance,with the axially dispersed plug flow model and the mass transfer rate represented by a Linear Driving Force (LDF)model.The LDF model is frequently used for this purpose because it is analytically simple and physically consistent.Farooq and Ruthven (17)reported a comparison between one and two-dimensional models for the adsorp-tion in a fixed bed,providing a simple approximate means of estimating the spread to be expected in the break-through curve for a given degree of nonisothermality,expressed in terms of a dimensionless radial conducti-vity parameter.They concluded that in adiabatic condi-tions,the radial temperature profile becomes flat and the one-dimensional model is suitable to describe the dynamics of adsorption in a fixed bed (17).The global mass balance was described by the axial dispersed plug flow model Eq.(1)(18).ÀD L @2C @z 2þ@ðvC Þ@z þ@C @t þ1Àe e@ q@t¼0ð1Þwhere D L is the axial dispersion,e is the voidage of the adsor-bent bed and v is the interstitial velocity.The feed consists ofa small concentration of a single adsorbable component and the frictional pressure drop through the bed is negligible,thus,the linear velocity may be considered constant.The rate of mass transfer to the particle was described by the linear driving force (Eq.2).According to this approxi-mation,the partial differential mass balance equation in the particle is replaced by a much simpler ordinary differen-tial equation,which states that the uptake rate of CO 2is proportional to the difference between the surface concen-tration and the average concentration within the particle.@ q@t¼K L ðq À q Þð2Þwhere K L is the LDF global mass transfer coefficient and q is the adsorbed equilibrium concentration,i.e.,q ¼f(C)given by the adsorption equilibrium isotherm.The energy balance in the fluid phase gives the variation of the gas temperature with the time (1).The heat balance was described by Eq.(3)(18).Àk :@2T g @z þC g @ðvT g Þ@z þC g @T g @t þ1Àe eC s@T s @t¼1Àe e ðÀD H Þ@ q @t À4h w e d cðT g ÀT w Þð3Þwhere k is the thermal axial dispersion coefficient,C g andC s are the specific heat values for gas and solid phases,respectively,(ÀD H )is the isosteric heat adsorption,h w is the internal convective heat coefficient between the gas and the wall,and d c is the bed diameter.TABLE 1Physical properties of the fixed-bedBed Lengh L,m 0.171Bed Diameter d C ,m 0.022Bed Weight W,kg0.0351Voidage adsorbent bed,e0.52Column wall specific heat,C p ,w 440J kg À1K À1Wall density,q w7828kg m À3CO 2ADSORPTION OF CARBON DIOXIDE75D o w n l o a d e d b y [S o u t h w e s t U n i v e r s i t y ] a t 02:30 13 M a y 2013The rate of heat transfer to the particle may be written as:C s@T s @t ¼6h f d pðT g ÀT s ÞþðÀD H Þ@ q@t ð4Þwhere h f is the film heat transfer coefficient,and d p is theparticle diameter.For the column wall,the energy balance for an adiabatic system can be expressed by:q w C p ;w@T w@t¼a w h w ðT g ÀT w Þð5Þwhere q w is the column wall density,C p ,w is the column wall specific heat,and a w is the ratio of the internal surface area to the volume of the column wall (19).The value of the gas phase viscosity was estimated using the Wilke equation and the molecular diffusivities were calculated with the Chapman-Enskong equation (20).The LDF global mass transfer coefficient considers all of the resistances to the mass transfer,i.e.,intra-and extra-particle resistances.The value of the LDF global mass transfer coefficient was estimated using the expression pro-posed by Farooq and Ruthven (21)which considers film resistance,and macropore and micropore resistances:1K L ¼r p q o 3k f C o þr 2p q o15e p D e C o þr 2c 15D cð6Þwhere r p is the particle radius,k f the external mass transfer coefficient,q 0the value of q at equilibrium with C o (adsor-bate concentration in the feed at feed temperature T o and expressed in suitable units),e P the particle porosity,r c the radius of activated carbon crystal,and D c is the micropore diffusivity.The micropore diffusivity values were those reported by Cavenati and coworkers (19)since the micro-pore distribution of the adsorbents are similar to those of carbon molecular sieves (19,22).The mass and heat transport parameters were estimated according to correlations reported in the literature (18,20,23,24,25).The correlations used to evaluate the mass and heat transport parameters are summarized in Table 2.The value of the mean pore radius (r o )was 1.23nm for commercial activated carbon and 1.54nm for CPHCl,estimated through the nitrogen adsorption isotherm.Tortuosity factor (s )values of 2.2and 1.8for activated carbon and CPHCl,respectively,were assumed and the particle porosity of activated carbon and CPHCl are 0.47and 0.37.The mathematical model was solved using the commer-cial software gPROMS (Process System Enterprise Limited,UK)which uses the orthogonal collocation method on finite elements for resolution.The bed wasdivided into six sections with three collocation points for each element of the adsorption bed.RESULTS AND DISCUSSIONCharacterization of the AdsorbentThe nitrogen sorption isotherms for the commercial activated carbon and for the CPHCl are shown in Fig.1.The hysteresis loop is narrow,the adsorption and deso-rption branches are almost the same,indicating that both adsorbents are microporous.This kind of loop is desig-nated type H4hysteresis in the IUPAC classification and is usually found for solids consisting of aggregates or agglomerates of particles forming slit-shaped pores (26).TABLE 2Correlations used for estimation of mass andheat parametersBosanquet Equation 1D e ¼s 1D m þ1D KKnudsen diffusion D K ¼9700r o ffiffiffiffiffiffiTMw q Axial mass dispersionPe ¼0:508Re 0:020Lp ;Pe ¼uL L;Re ¼q g ud pgAxial heat dispersion k k g¼10þ0:5Pr Re ;Pr ¼C g l g k gInternal convective heat coefficient h w d c k g¼12:5þ0:048ReFilm mass transfer Sh ¼1:09Re 0:27Sc 1=3;Sc ¼l g q gD m;Sh ¼k f d pD m Film heat transferNu ¼2:0þ1:1Re 0:6Pr 1=3;Nu ¼h f d pkgFIG.1.Nitrogen sorption isotherms at 77K:&commercial activatedcarbon and 4CPHCl.76T.L.P.DANTAS ET AL.D o w n l o a d e d b y [S o u t h w e s t U n i v e r s i t y ] a t 02:30 13 M a y 2013Table 3summarizes the physical characterization obtained from the nitrogen sorption isotherm and mercury intrusion.The apparent surface area (S BET )was evaluated using the BET equation (27),micropore area (S micro )was calculated according to the DR method (28)and the micro-pore volume (V micro )was determined by the t-method (29).Modifications with nitrogen-containing species may also result in changes in the porous structure (11),as can be observed in the N 2adsorption isotherms shown in Fig.1.The CPHCl had a lower BET area when compared to the commercial activated carbon.The micropores volume of the CPHCl decreases considerably compared with the com-mercial activated carbon,suggesting that the nitrogen incor-poration partially blocks the access of N 2to the small pores.The hysteresis loop of the N 2adsorption isotherm occurs,for both adsorbents,at relative pressure closes to P =Po ¼0.4.Below this pressure the pore filling mechanism is the so-called micropore filling,for which no hysteresis occurs.When the nitrogen pressures exceeds P =Po ¼0.4,the mesopores are filled by means of capillary condensation (29).The micropore capacity,in terms of N 2,for the two adsorbents was taken from the hysteresis pressure (30,31),as seen in Table 4.For the calculations,the densityTABLE 3Textural properties of the adsorbents studiedAdsorbentCommercial activated carbon CPHCl S BET,m 2=g 1053.0664.6S micro,m 2=g 1343.0753.0V micro,cm 3=g0.09720.0388N 2Micropore Capacity,kg =kg300155N 2Total Capacity,kg =kg 370260Particle density q p .103,kg =m 31.14Particle diameter d p 103,m 3.8Adsorbent specific heat,J =kg.K880TABLE 4Chemical characteristics of the adsorbents studied Adsorbent Commercial activatedcarbonCPHCl C 86.270.2H 1.3 2.0N0.9 1.4N =C.10212FIG.2.Cumulative nitrogen adsorbed as a function of pore diameter calculated with the HK method from the N 2sorption isotherms at 77K:&commercial activated carbon and 4CPHCl.FIG.3.TGA and DrTGA curves for commercial activatedcarbon.FIG.4.TGA and DrTGA curves for CPHCl.CO 2ADSORPTION OF CARBON DIOXIDE77D o w n l o a d e d b y [S o u t h w e s t U n i v e r s i t y ] a t 02:30 13 M a y 2013of adsorbed nitrogen was approximated with its liquid density at 77K (0.808kg =m 3).The effective micropore size distribution,for both adsorbents,was calculated using the HK method (32).The accumulated amount of N 2adsorbed as a function of pore size is shown in Fig.2for the commercially acti-vated carbon and for the CPHCl.From Fig.2,it is evident that the great majority of the pores shown for the two adsorbents are micropores.Micropores have a diameter of less then 2nm.However,the great microporosity of the activated carbon is evidenced by the fact that about 70%of its total capacity,in terms of N 2,is found to be inthe micropores.However,for the CPHCl,approximately 52%of its total capacity is present in the micropores.The chemical characteristics of the adsorbents are given in Table 4.As expected,the adsorbent CPHCl has the greater nitrogen content and an N =C atomic ratio which is twice that of the commercial activated carbon.Figures 3and 4show the weight loss (TG)and the deri-vative thermogravimetric (DrTG)curves obtained for the commercial activated carbon and for the nitrogen-enriched activated carbon ÀCPHCL.For the commercial activated carbon,the first range of thermal decomposition presents a little weight losswhichFIG.5.FTIR spectra of (a)commercial activated carbon;(b)CPHCl;(c)CPHCl after pre-treatment and CO 2adsorption at 301K and (d)CPHCl after pre-treatment and CO 2adsorption at 423K.78T.L.P.DANTAS ET AL.D o w n l o a d e d b y [S o u t h w e s t U n i v e r s i t y ] a t 02:30 13 M a y 2013is due to release of the moisture.Further mass loss at temperature above 490K is due to the release of the vola-tile matter which is consistent with the volatile matter content of the activated carbon,as measured by the ultimate analysis (33).The CPHCl adsorbent had a mass loss at 303–428K which is typical for phase changes,due to the melting of the amine and due to release of the moisture.The subse-quent temperatures give the same total weight loss due to the volatiles.The FTIR spectra of commercial activated carbon and CPHCl are shown in Fig.5.In the FTIR spectra the band of O-H stretching vibra-tions (3600–3100cm À1)was due to surface hydroxyl groups and chemisorbed water.The asymmetry of this band at lower wave numbers indicates the presence of strong hydrogen bonds.It has been suggested that primary amine can react with the activated carbon surface,forming surface complexes with the presence of NH 2surface groups (13).Bands were presence at 3365and 1607cm À1,ascribed to asymmetric stretching (n NH 2)and NH 2deformation,respectively,and at 3303cm À1.However,the CPHCl spectrum shows that these bands may be overlapped by the OH stretching band (3600–3100cm À1)and by the aromatic ring bands and double bond (C =C)vibrations (1650–1500cm À1)(34).The same pattern is observed for CPHCl after CO 2adsorption at 301K and 423K,indicating that there is no difference in the adsorption behavior.Carbon Dioxide Adsorption Equilibrium IsothermsThe CO 2adsorption equilibrium isotherms for com-mercial activated carbon and CPHCl are shown in the Figs.6and 7,respectively.The CO 2adsorption equilibrium for commercial activated carbon was described using the Toth model (35)Eq.(7).q ¼q m K eq P ½1þðK eq P Þð7Þwhere q m is the maximum adsorbed concentration,i.e.,the monolayer capacity;K eq is the equilibrium adsorption con-stant and n is the heterogeneity parameter of the solid.The CO 2adsorption equilibrium for CPHCl was described according to the linear model (Eq.8).The depen-dence of K eq or K p on the temperature was described according to the Van’t Hoff equation.q ¼K p Pð8Þwhere K p is the Henry’s Law constant for the adsorption equilibrium.Table 5gives the parameters used in this study for Toth fitting.It is worth mentioning that the commercial activated carbon used in this studied has a high CO 2adsorption capacity in comparison with other adsorbents reported in literature (36,37).Table 6gives the Henry’s Law constants for the adsorp-tion equilibrium on CPHCl,at the different temperatures studied,the pre-exponencial factor and heat of adsorption.Table 7shows the Henry’s constants for the adsorption equilibrium on commercial activated carbon that was fitted at low pressure.It should be noted,however,that the commercial activated carbon has higher Henry’s Law constant indicating that this solid has a greater carbon dioxide adsorption capacity.The nature of the N functionality is very important because it can affect the basicity of the solid surface(38).FIG. 6.Adsorption equilibrium isotherms for carbon dioxide on commercial activate carbon at different temperatures:&301K;4323K;&,373K and ~423K.Solid lines:Toth Model.Bold line:linearmodel.FIG.7.Adsorption equilibrium isotherms for carbon dioxide on CPHCl at different temperatures:&298K;4323K;&,373K and ~423K.Solid lines:Linear Model.CO 2ADSORPTION OF CARBON DIOXIDE79D o w n l o a d e d b y [S o u t h w e s t U n i v e r s i t y ] a t 02:30 13 M a y 2013Comparing a primary amine with a secondary amine of the same carbon number,the basic character increases due to the increase in the inductive effect caused by the alkyl groups (39).Some authors have reported that although there is a reduction in the BET superficial area which is caused by the partial blockage of the lesser pores,as also observed in this paper,the enrichment of the carbonaceous materials with nitrogen tends to increase the adsorption capacity for CO 2(11).However,there is no consensusabout this issue because sorbents with the high amounts of nitrogen do not have the high CO 2adsorption capacity reported in recent publications by Arenillas and coworkers (11)and Pevida et al.(40).In the present study,we show a decrease in the CO 2adsorption capacity of CPHCl in comparison with non-functionalized activated carbon.The decrease in the CO 2adsorption capacity is not related to the destruction of basic sites in the CPHCl,as shown in the FTIR studies (Fig.5).In fact,Drage et al.(12)have reported that only an activation temperature higher than 873K can destroy the basic sites in the adsorbents.Modeling the Breakthrough CurvesAs previously mentioned,a set of experiments was performed changing the temperature and the feed concentra-tion of the carbon dioxide to determine the breakthrough curves.The experimental conditions and the LDF globalTABLE 5Parameters of adsorption isotherm for carbon dioxide on different adsorbents and parameters used for fittingof the Toth model q m ,mol =kg n K o 105,bar À1ÀD H,kJ =mol10.050.6787.6221.84TABLE 6Henry’s Law constants for the adsorption equilibrium on commercial activated carbon and CPHCl atdifferent temperaturesT,K Commercial activated carbonCPHClK p ,moles =kg.barK p ,moles =kg.barÀD H,kJ =mol 301–298 2.89 2.1620.25323 1.86 1.553730.620.324230.290.11TABLE 7Experimental Conditions and LDF global mass transfer coefficient for CO 2adsorption on the commercialactivated carbon and CPHClRun y F T,K Pe K L ,s À1Commercial activated carbonCPHCl 10.130121.750.00270.0041232321.690.00430.0063337321.590.01250.025*******.490.02590.071950.230121.910.00270.0041632321.850.00430.0063737321.750.01250.0259842321.650.02590.0719FIG.8.Breakthrough curves for the CO 2adsorption on commercial activated carbon.Symbols:experimental data;Lines:LDF model.(a)Runs 1to 4and (b)runs 5to 8.80T.L.P.DANTAS ET AL.D o w n l o a d e d b y [S o u t h w e s t U n i v e r s i t y ] a t 02:30 13 M a y 2013。