Characterizingtheeffectofheavymetalcontaminationonmarinemusselsusingm.
利用作物生长模型和时序信号甄别水稻镉胁迫

第37卷第4期农业工程学报 V ol.37 No.42021年2月Transactions of the Chinese Society of Agricultural Engineering Feb. 2021 249 利用作物生长模型和时序信号甄别水稻镉胁迫孔丽,刘美玲※,刘湘南,邹信裕(中国地质大学(北京)信息工程学院,北京 100083)摘要:在自然农田生态系统中,农作物的生长通常受到各类环境胁迫(如重金属胁迫、病虫害、水分、营养)的影响,如何区分重金属胁迫与其他胁迫有待进一步研究。
该研究选取了湖南省株洲为试验区,收集2017—2019年的Sentinel-2卫星影像数据,结合野外实测数据,开展水稻重金属镉(Cd)胁迫识别研究。
首先,利用作物生长模型World Food Studies (WOFOST)同化时序遥感数据获取每年的叶面积指数(Leaf Area Index,LAI)时间序列曲线;然后运用集合经验模态分解(Ensemble Empirical Mode Decomposition,EEMD)方法对LAI时间序列进行多尺度分解,得到不同的时序信号分量(Intrinsic Mode Function,IMF);最后使用动态时间规整(Dynamic Time Warping,DTW)方法计算受胁迫水稻分解后的时间序列与健康水稻分解后的时间序列之间的DTW距离,即归一化胁迫指数。
结果表明:归一化胁迫指数是水稻重金属胁迫敏感的参数,与土壤重金属含量的相关系数为0.851,水稻受到的胁迫程度越高,归一化胁迫指数值越大,反之越低;在试验区中,水稻重度重金属胁迫的分布面积比例相对较低,且主要集中在西部、东北部以及偏东南地区。
融合集合经验模态分解和动态时间规整方法能有效地甄别并定量分析水稻重金属胁迫状况,从而为作物重金属污染胁迫监测提供重要参考。
关键词:遥感;模型;重金属;镉胁迫;时序信号分解;WOFOSTdoi:10.11975/j.issn.1002-6819.2021.04.030中图分类号:S127 文献标志码:A 文章编号:1002-6819(2021)-04-0249-08孔丽,刘美玲,刘湘南,等. 利用作物生长模型和时序信号甄别水稻镉胁迫[J]. 农业工程学报,2021,37(4):249-256.doi:10.11975/j.issn.1002-6819.2021.04.030 Kong Li, Liu Meiling, Liu Xiangnan, et al. Identifying heavy metal (Cd) stress in rice using time-series signals and crop growth model[J]. Transactions of the Chinese Society of Agricultural Engineering (Transactions of the CSAE), 2021, 37(4): 249-256. (in Chinese with English abstract) doi:10.11975/j.issn.1002-6819.2021.04.030 0 引 言随着经济的不断发展,工业化不断推进,土壤重金属污染成为当今世界面临的重大生态环境问题之一[1]。
usp31nf26s2_Lead 251
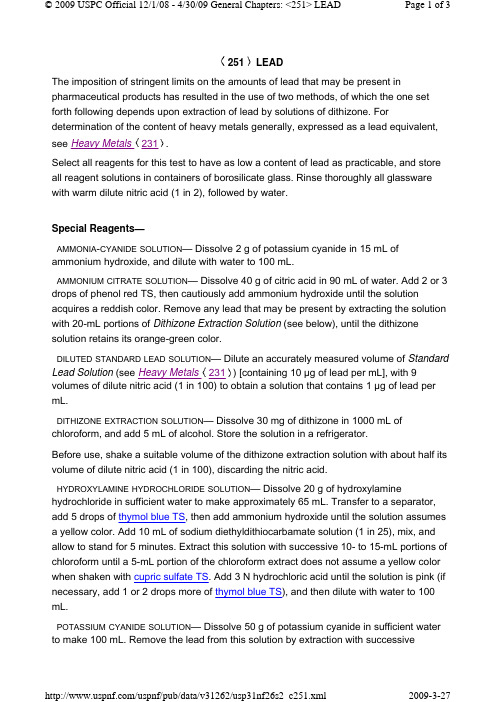
251 LEADThe imposition of stringent limits on the amounts of lead that may be present in pharmaceutical products has resulted in the use of two methods, of which the one set forth following depends upon extraction of lead by solutions of dithizone. For determination of the content of heavy metals generally, expressed as a lead equivalent, see Heavy Metals 231.Select all reagents for this test to have as low a content of lead as practicable, and store all reagent solutions in containers of borosilicate glass. Rinse thoroughly all glassware with warm dilute nitric acid (1 in 2), followed by water.Special Reagents—AMMONIA-CYANIDE SOLUTION— Dissolve 2 g of potassium cyanide in 15 mL of ammonium hydroxide, and dilute with water to 100 mL.AMMONIUM CITRATE SOLUTION— Dissolve 40 g of citric acid in 90 mL of water. Add 2 or 3 drops of phenol red TS, then cautiously add ammonium hydroxide until the solution acquires a reddish color. Remove any lead that may be present by extracting the solution with 20-mL portions of Dithizone Extraction Solution (see below), until the dithizone solution retains its orange-green color.DILUTED STANDARD LEAD SOLUTION— Dilute an accurately measured volume of StandardLead Solution (see Heavy Metals 231) [containing 10 µg of lead per mL], with 9 volumes of dilute nitric acid (1 in 100) to obtain a solution that contains 1 µg of lead per mL.DITHIZONE EXTRACTION SOLUTION— Dissolve 30 mg of dithizone in 1000 mL of chloroform, and add 5 mL of alcohol. Store the solution in a refrigerator.Before use, shake a suitable volume of the dithizone extraction solution with about half its volume of dilute nitric acid (1 in 100), discarding the nitric acid.HYDROXYLAMINE HYDROCHLORIDE SOLUTION— Dissolve 20 g of hydroxylamine hydrochloride in sufficient water to make approximately 65 mL. Transfer to a separator, add 5 drops of thymol blue TS, then add ammonium hydroxide until the solution assumes a yellow color. Add 10 mL of sodium diethyldithiocarbamate solution (1 in 25), mix, and allow to stand for 5 minutes. Extract this solution with successive 10- to 15-mL portions of chloroform until a 5-mL portion of the chloroform extract does not assume a yellow color when shaken with cupric sulfate TS. Add 3 N hydrochloric acid until the solution is pink (if necessary, add 1 or 2 drops more of thymol blue TS), and then dilute with water to 100 mL.POTASSIUM CYANIDE SOLUTION— Dissolve 50 g of potassium cyanide in sufficient water to make 100 mL. Remove the lead from this solution by extraction with successiveportions of Dithizone Extraction Solution, as described under Ammonium Citrate Solution above, then extract any dithizone remaining in the cyanide solution by shaking with chloroform. Finally dilute the cyanide solution with sufficient water so that each 100 mL contains 10 g of potassium cyanide.STANDARD DITHIZONE SOLUTION— Dissolve 10 mg of dithizone in 1000 mL of chloroform. Keep the solution in a glass-stoppered, lead-free bottle, suitably wrapped to protect it from light, and store in a refrigerator.Test Preparation— [NOTE—If, in the following preparation, the substance under test reacts too rapidly and begins charring with 5 mL of sulfuric acid before heating, use instead 10 mL of cooled dilute sulfuric acid (1 in 2), and add a few drops of the hydrogen peroxide before heating.] Where the monograph does not specify preparation of a solution, prepare a Test Preparation as follows. [Caution—Exercise safety precautions in this procedure, as some substances may react with explosive violence when digested with hydrogen peroxide. ] Transfer 1.0 g of the substance under test to a suitable flask, add 5 mL of sulfuric acid and a few glass beads, and digest on a hot plate in a hood until charring begins. Other suitable means of heating may be substituted. (Add additional sulfuric acid, if necessary, to wet the substance completely, but do not add more than a total of 10 mL.) Add, dropwise and with caution, 30 percent hydrogen peroxide, allowing the reaction to subside and again heating between drops. Add the first few drops very slowly, mix carefully to prevent a rapid reaction, and discontinue heating if foaming becomes excessive. Swirl the solution in the flask to prevent unreacted substance from caking on the walls of the flask. [NOTE—Add peroxide whenever the mixture turns brown or darkens.] Continue the digestion until the substance is completely destroyed, copious fumes of sulfur trioxide are evolved, and the solution is colorless. Cool, cautiously add 10 mL of water, evaporate until sulfur trioxide again is evolved, and cool. Repeat this procedure with another 10 mL of water to remove any traces of hydrogen peroxide. Cautiously dilute with 10 mL of water, and cool.Procedure— Transfer the Test Preparation, rinsing with 10 mL of water, or the volume of the prepared sample specified in the monograph to a separator, and, unless otherwise directed in the monograph, add 6 mL of Ammonium Citrate Solution and 2 mL of Hydroxylamine Hydrochloride Solution. (For the determination of lead in iron salts use 10 mL of Ammonium Citrate Solution.) Add 2 drops of phenol red TS, and make the solution just alkaline (red in color) by the addition of ammonium hydroxide. Cool the solution if necessary, and add 2 mL of Potassium Cyanide Solution. Immediately extract the solution with 5-mL portions of Dithizone Extraction Solution, draining off each extract into another separator, until the dithizone solution retains its green color. Shake the combined dithizone solutions for 30 seconds with 20 mL of dilute nitric acid (1 in 100), and discardthe chloroform layer. Add to the acid solution 5.0 mL of Standard Dithizone Solution and 4 mL of Ammonia-Cyanide Solution , and shake for 30 seconds: the color of the chloroform layer is of no deeper shade of violet than that of a control made with a volume of Diluted Standard Lead Solution equivalent to the amount of lead permitted in the sample under examination, and the same quantities of the same reagents and in the same manner as in the test with the sample.Auxiliary Information— Before contacting USP, have you checked for your question in the FAQs ?USP31–NF26 Page 135 Topic/Question Contact Expert Committee Monograph Kahkashan Zaidi, Ph.D.Senior Scientist1-301-816-8269(GC05) General Chapters 05。
环境化学6-1
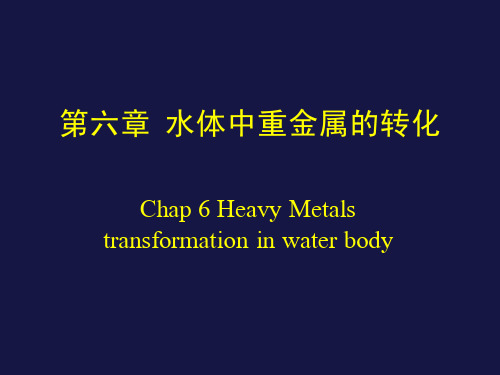
2.金属水合氧化物
hydrous oxide of metals
Al 在水中的主要形态是Al3+、Al(OH)2+、Al2(OH)24+ 、 Al(OH)2+、 Al(OH)3、 Al(OH)4-等无机高分子 (inorganic polymer) 。 Fe 在水中的主要形态是 Fe3+、Fe(OH)2+、 Fe2(OH)24+、 Al(OH)2+、 Fe(OH)3、 FeOOH等无机 高分子。 H 4SiO4 聚合 (polymerizing)成无机高分子: SinO2n- m(OH)2m。 实际上在一定条件下,铁、铝等均能聚合成多核 配合物(polynuclear complexation) ,或称为无机 高分子溶胶(colloidal sols )。
第六章 水体中重金属的转化
Chap 6 Heavy Metals transformation in water body
无机污染物,特别是重金属( heavy metals)和准金 属( metalliod)等污染物,一旦进入水环境,均不能 被生物降解(biodegradation) ,主要通过沉淀-溶解( precipitation-dissolution)、氧化-还原( reductionoxidation)、配合作用( complextion)、胶体形成 (colloid forming) 、吸附-解吸(adsorptiondesorption) 等一系列物理化学作用进行迁移转化 ,参与和干扰各种环境化学过程和物质循环过程 。
(二)颗粒物的吸附作用
Adsorption of Particles in Water
1.吸附作用的种类
Types of adsorption
Assessment_of_Heavy_Metals_Content_in_Sewage_Disch

Journal of Pharmacy and Pharmacology 10 (2022) 190-194doi: 10.17265/2328-2150/2022.06.002Assessment of Heavy Metals Content in Sewage Discharges from Health Structure in MauritaniaMohamed Bouna Ammar1,2,6, Brahim Ahmed Dick2,3, Yahya Maham Ould Sidi1,2, Hanan Deih1,2, Hana Youssef Learoussy3,4, Med Abderrahmane Sanhoury5, Vadly Sadegh6 and Mohamed Fekhaoui11. Geo-biodiversity and Natural Patrimony Laboratory, Scientific Institute, Mohamed V University, Rabat, Morocco2. Research Unit of the Water, Pollution, Environment, FST, University of Nouakchott Al-Aasrya, Mauritania3. National Laboratory for the Quality Control of Medicines (LNCQM), Nouakchott, Mauritania4. Laboratory of Bioactive Molecules, FST, Sidi Mohamed Ben Abdellah University, Fez, Morocco5. Materials Chemistry Research Unit, Department of Chemistry, FST, University of Nouakchott Al-Aasrya, Mauritania6. Central Purchasing Center for Medicines, Medical Equipment and ConsumablesAbstract: This study was carried to assess the quality of liquid waste produced by the Nouakchott Friendship Hospital in Mauritania, the aim is to quantify different heavy metals obtained from discharge of the hospital waste six heavy metals (arsenic, lead, cobalt, chromium, cadmium and copper) were object of evaluation. Analysis was carried using inductively coupled plasma - optical emission spectrometry (ICP-OES) method and the standards used are those of the WHO. The average content of heavy metals in different samples is different: Arsenic (4.625 μg/L), Lead (3.800 μg/L), Cyanide (0.05μg/L), Chromium (0.013μg/L), Cadmium (< LD (0.000000012 μg/L) and Copper (60 μg/L).Results showed that the samples of liquid waste from the Nouakchott Friendship Hospital were very loaded with pollutants, this may constitute a threat to the environment and a potential health risk for human, since the continuous introduction of these heavy metals into aquatic medium could be harmful through bioaccumulation and biomagnifications.Key words: Heavy metals, waste, ICP-OES, pollutants, health risk.1. IntroductionIn view of the nature and importance of specific substances (drug residues, chemical reagents, antiseptics, detergents, developers and x-ray fixators, etc.) contained in the waste generated by hospital activities, as well as its evacuation (like conventional urban waste, to the municipal sanitation network without prior treatment), it may cause potential hazards to humans and their environment [1].In recent years, the health establishments produce waste in large quantities, and of very diverse nature. In developed countries, the majority of wastewater (domestic, hospital and industrial) is passed throughCorresponding author: Mohamed Bouna Ammar, research fields: engineering and environment. Email: sanitation networks and collected and then treated in wastewater treatment plants before being released into the natural environment. This process thus makes it possible to limit the excessive inputs of organic matter and mineral pollutants into the natural environment [2].The management of medical waste in general is seen as a serious problem in some countries especially developing countries. A study conducted by the World Health Organization (WHO) in 2002 among 22 developing countries showed that 18 to 64% of health establishments do not properly dispose of their health care waste. This showed that the current management of medical waste poses a real public health and environmental problem in developing countries. In these countries, an additional danger is added, that of excavation of landfills and manual sorting ofwasteAssessment of Heavy Metals Content in Sewage Discharges from Health Structure in Mauritania 191collected at the exit of health care establishments without discrimination [3], which further complicates this problem and takes it on a different scale [4].The relative problems of risky substances are thus one of the major current concerns [5-7]. In this context, the issue of discharges of hospital waste is becoming increasingly important. Indeed, these establishments generate substantial volumes of liquid waste which contain specific xenobiotics (metals, chemical reagents, disinfectants, detergents, radiographic developers and fixers, etc.) which will thus be disseminated in the environment [8].Indeed, as occurs in all chains of human activity, medical care generates solid waste, liquid discharges and gaseous waste, and therefore causes transfers of pollutants to natural environments that can compromise the biological balance of ecosystems, aquatic life and the health of living beings [9]. Heavy metals like copper can disrupt in high doses the proper functioning of the enzymatic system of humans [10]. These heavy metals therefore represent a danger of water pollution since they can modify the physicochemical characteristics of the water and adversely affect the proper functioning of the treatment plant by destroying its purification flora [11].In Mauritania, management of medical waste is a major challenge for national hospitals. Despite the large quantities produced at these hospitals, there is a poor management of effluents, which is mainly due to the lack of an establishment specialized in the treatment and disposal of hospital effluents.In Nouakchott, capital of Mauritania, out of 13,650 m3 of wastewater collected per day including hospital liquid effluents, only 4,250 m3 are treated. Thus, 9,400 m3 of wastewater (68.86%) is untreated and discharged directly into the sea through a public collection network. In view of this observation, it is necessary to implement planned integrated management systems for hospital waste. Mauritania’s environmental and sanitation codes stipulate that producing establishments, namely hospitals and other health facilities are responsible for the biomedical waste they discharge [12].The objective of the study is to evaluate the discharges of waste water at the Friendship Hospital by the quantification of heavy metals by ICP-OES method.2. Material and Methods2.1 Study LocationThe Friendship Hospital was chosen as the study site because it is not directly linked to the city’s sewerage network. The hospital has 9 departments, including surgery, internal medicine, pediatrics, obstetrics and gynecology, emergency, ENT, ophthalmology, anesthesia, resuscitation/hemodialysis/ outpatient, and medical analysis laboratory, with 91 beds. The friendship hospital has a unit responsible for public hygiene, which deals only with the cleaning of the premises and not with the treatment of waste from the various departments.2.2 SamplingSamples of liquid waste were collected from the internal sewage network of the Nouakchott Friendship Hospital. Four samples were collected in sterile bottles from August to September 2019, with an interval of 10 days.The trace metal analysis was conducted using ICP-OES, which limit of detection (LOD) is 0.001 mg/kg. Hg was analyzed using Direct Mercury Analyzer (LOD = 0.003 mg/kg) according to the method [13].3. Results and DiscussionThe liquid waste produced by the Friendship Hospital of Nouakchott has a great variability in characteristics and this variability depends upon the rejection of services. The results show that the concentrations of heavy metals in liquid waste varied at the Friendship Hospital of Nouakchott during the study (Figures 1-5).Assessment of Heavy Metals Content in Sewage Discharges from Health Structure in Mauritania192Fig. 1 Arsenic variation in samples.Fig. 2 Lead variation in samples.Fig. 3 Cyanide variation in samples.Fig. 4Fig. 5 Copper variation in samples.2468Sample 1Sample 2Sample 3Sample 4Arsenic content in µg/L0.020.040.060.080.1Sample 1Sample 2Sample 3Sample 4Cyanide content in µg/LAssessment of Heavy Metals Content in Sewage Discharges from Health Structure in Mauritania 193The results obtained from the analysis of heavy metals in samples of liquid waste from Nouakchott Friendship Hospital are presented in Table 1. The results revealed a variation in contents of different heavy metals. The averages of the heavy metal concentrations of for samples from the liquid waste collection point of the Friendship Hospital are respectively presented in Table 2.Table 1 Overview of heavy metals content in different samples.Element (µg/L) Sample 1 Sample 2 Sample 3 Sample 4 Arsenic 6 5.8 4.1 2.6 Lead 6.4 5.2 2.5 2.1 Cyanide 0.03 0.03 0.05 0.09 Chromium 0.011 0.006 0.028 0.005 Cadmium < LD < LD < LD < LD Copper 30 30 140 40LD = limit of detection (0.000000012 μg/L).Table 2 Comparison results with different countries standards.Element (µg/L) Range Mean ± SDStandardsMorocco Senegal Benin South Africa OMSArsenic as As 2.6-6 4.625 ± 1.597 1,000 1,000 500 500 - Lead as Pb 2.1-5.4 3.800 ± 1.742 5,000 500 1,000 100 500 Cyanide as Cn 0.03-0.09 0.050 ± 0.028 1,000 - 1,000 - - Chromium as Cr 0.005-0.028 0.013 ± 0.011 100 - 2,500 500 - Cadmium as Cd < LD - - - 1,000 50 -One of the main environmental problems posed by liquid hospital waste is its discharge, like conventional urban effluents, to municipal sanitation networks without prior treatment [14]. Indeed, the use of measuring devices and solutions based on metals in hospitals in developing countries facilitates the presence of these metals in the liquid discharges of the various medical services [15].The sewage discharge from services of the Friendship Hospital of Nouakchott does not reveal a high concentration of heavy metals (Arsenic, Lead, Cyanide, Chromium, Cadmium and Copper). Our results showed a variation of different heavy metals content in period of sampling. Arsenic and lead contents (Figures 1 & 2) decreased in the period of the study, while cyanide (Figure 3) increased. However, the contents of chromium and copper (Figures 4 & 5) had a variation during the study.In Mauritania, there is no regulation about the standards for heavy metals in hospital sewage discharge. In our study, we compared our results with those of the WHO standards and those of other countries standards in Africa (Table 2). In our study, the content of heavy metals found in liquid waste from Friendship Hospital is not significant and are below the sewage discharge standards, except for copper which in Sample 3 (140 µg/L) was above the limit of copper by the Moroccan regulation. Arsenic concentrations varis from 2.6-6 µg/L, the lead content varied between 2.1-5.4 µg/L, and the cyanide, chromium and copper contents varied between 0.03-0.09 µg/L, 0.005-0.028 µg/L, 30-140 µg/L, respectively. The cadmium concentration was lower than the detection limit of 0.000000012 μg/L, which was consistent with the determination result [16]. Other studies have shown that there are low concentrations of heavy metals in hospital liquid wastes, such as arsenic, cadmium, lead, copper andAssessment of Heavy Metals Content in Sewage Discharges from Health Structure in Mauritania 194chromium [16-18].4. ConclusionsThe study is the first to measure heavy metal emissions from health facilities in Mauritania. The concentration of heavy metals in liquid wastes provides valuable information; the toxicity of these metals should be taken into account when comparing the potential impact of these metallic elements on the environment and on aquatic animals. This preliminary study highlights the presence of heavy metals in the liquid wastes from the Nouakchott Friendship Hospital, which are toxic even at very low concentrations.The evaluation of metal pollution degree in this study shows that metal pollution can be seen in all samples (arsenic, lead, cyanide, chromium, cadmium and copper). In order to reduce the risk of these toxic elements in hospital emissions, it is necessary to treat this wastewater.References[1]Darsy, C., et al. 2002. Effluents des établissementshospitaliers et la maîtrise de la diffusion des bactéries multi résistantes: teneur en microorganismes pathogènes, risques san itaires, procédures particulières d’épuration et gestion des boues. pp. 10.[2]Code de la santépublique. Available on:https://www.legifrance.gouv.fr/codes/article_lc/LEGIARTI000032908788.[3]Kissi, L., et al. 2012. Management of Dental CareActivity Waste. (Article in French). Available on: https:///dossiers-du-mois/gestion-des-dechets-des-activites-de-soins-en-odontologie-etude-bibliographique.html.[4]Fihri, A. F. 2016. Medical and Pharmaceutical Waste inMorocco: Towards a Collection and Treatment Project for Health Establishments in the City of Fez. (Article in French). Available on: https://herbrooke.ca/bitstream/handle/11143/8390/Fassi_Fihri_Ahmed_MEnv_2016.pdf.[5]Ayscough, N. J., Fawell, J., Franklin, G, & Young, Y.2000. R & D Technical Report P390. UK Environment Agency, Bristol.[6]Schulman, L. J., Sargent, E. V., Naumann, B. D., et al.2002. “A Human Health Risk Assessment ofPharmaceuticals in the Aquatic Environment.” Human and Ecological Risk Assessment 8 (4): 657-680.[7]Garric, J., & Ferrari, B. 2005. “Phamaceuticals in AquaticEcosystems. Levels of Exposure and Biological Effects:A Review.”Journal of Water Science 18 (3): 307-330.[8]Verlicchi, P., Galletti, A., Petrovic, M., & Barcelo, D.2010. “Hospital Effluents as a Source of Emerging Pollutants: An Overview of Micropollutants and Sustainable Treatment Options.” Journal of Hydrology389 (3-4): 416-428.[9]Hisseien, A. T., Kamga, R., & Mahamat, T. N. 2015.“Physico-chemical Analysis of Logone River Water at Moundou City in Southern Chad.”Int. J. Biol. Chem. Sci9 (3): 1654-1664.[10]Roussel, A. M., & Hininger-Favier, I. 2009.“Éléments-trace Essentiels en Nutrition Humaine: Chrome, Sélénium, Zinc et Fer.”Endocrinologie-Nutrition Doi:10.1016/S1155-1941(09)49501-5.[11]Dan-Badjo, A. T., Tidjani , D. A., Idder, T., et al. 2014.“Diagnostic de la contamination des eaux par les éléments traces métalliques dans la zone aurifère de Komabangou–Tillabéri, Niger.” Int. J. Biol. Chem. Sci. 8(6): 2849-2857.[12]Plan National de la Gestion des Dechets Biomedicaux2017-2021.Available on: https://.mr/?wpfb_dl=189.[13]Ministère du Développement durable, del’Environnement, de la Faune et des Parcs du Québec et Environnement Canada. 2013. Available on: https://www.planstlaurent.qc.ca/fileadmin/site_documents/documents/guide_ecotoxicologique_fr.pdf.[14]Deblonde, T., Dreyer, M., & Hartemann, P. 2012.“Résidus Médicamenteux et eau Destinée àla Consommation Humaine.” Hegel 3: 12-20.[15]Jones, O. A. H., Voulvoulis, N., & Lester, J. N. 2002.“Aquatic Environmental Assessment of the Top 25 English Prescription Pharmaceuticals.” Water Res 36 (20): 5013-22.[16]Toure, A., Garat, A., Diop, C., et al. 2016. “Presence ofHeavy Metals and Drug Residues in Healthcare Facility Effluents in Dakar (Senegal).” (Articl e in French) Int. J.Biol. Chem. Sci. 10 (3): 1422-1432.[17]Adanlokonon, E. A. S., Chabi, B. C., Kanhounnon, W. G.,et al. 2019. “Heavy Metal Content (Hg. Pb. Cd and Fe) of CHU-MEL Effluents Discharged in the Cotonou Lagoon (Benin).”International Journal of Environmental Protection and Policy 7 (4): 109-116.[18]Tolosana, S., & Ehrlich, R. 2000. “Composition of LiquidEffluent Discharged by Medical Institutions in Cape Town.” South African Journal of Science 96 (8): 417-420.。
The remediation of heavy metals contaminated sediment

Journal of Hazardous Materials 161(2009)633–640Contents lists available at ScienceDirectJournal of HazardousMaterialsj o u r n a l h o m e p a g e :w w w.e l s e v i e r.c o m /l o c a t e /j h a z m atReviewThe remediation of heavy metals contaminated sedimentJian-feng Peng,Yong-hui Song ∗,Peng Yuan,Xiao-yu Cui,Guang-lei QiuChinese Research Academy of Environmental Sciences,100012Beijing,Chinaa r t i c l e i n f o Article history:Received 23June 2007Received in revised form 19March 2008Accepted 21April 2008Available online 24April 2008Keywords:Heavy metal Sediment DistributionInfluence factorsIn situ and ex situ remediationa b s t r a c tHeavy metal contamination has become a worldwide problem through disturbing the normal functions of rivers and lakes.Sediment,as the largest storage and resources of heavy metal,plays a rather important role in metal transformations.This paper provides a review on the geochemical forms,affecting factors and remediation technologies of heavy metal in sediment.The in situ remediation of sediment aims at increasing the stabilization of some metals such as the mobile and the exchangeable fractions;whereas,the ex situ remediation mainly aims at removing those potentially mobile metals,such as the Mn-oxides and the organic matter (OM)fraction.The pH and OM can directly change metals distribution in sediment;however oxidation–reduction potential (ORP),mainly through changing the pH values,indirectly alters metals distribution.Mainly ascribed to their simple operation mode,low costs and fast remediation effects,in situ remediation technologies,especially being fit for slight pollution sediment,are applied widely.However,for avoiding metal secondary pollution from sediment release,ex situ remediation should be the hot point in future research.©2008Elsevier B.V.All rights reserved.Contents 1.Introduction.........................................................................................................................................6342.Metals species in sediment .........................................................................................................................6343.Influence factors for heavy metal distributions ....................................................................................................6343.1.The influence of pH values...................................................................................................................6343.2.The influence of OM species.................................................................................................................6353.3.The influence of ORP ........................................................................................................................6353.4.The influence of some other factors.........................................................................................................6364.Remediation technology............................................................................................................................6364.1.In situ remediation technology..............................................................................................................6364.1.1.Amendments.......................................................................................................................6364.1.2.Sand cap............................................................................................................................6364.1.3.Phytoremediation..................................................................................................................6364.2.Ex situ remediation technology.............................................................................................................6374.2.1.Washing............................................................................................................................6374.2.2.Electrochemical remediation......................................................................................................6374.2.3.Flotation ...........................................................................................................................6384.2.4.Ultrasonic-assisted extraction.....................................................................................................6384.2.5.Immobilization ....................................................................................................................6385.Conclusions .........................................................................................................................................638Acknowledgement..................................................................................................................................639References ..........................................................................................................................................639∗Corresponding author.Tel.:+861084928381.E-mail address:pjf1995@ (J.-f.Peng).0304-3894/$–see front matter ©2008Elsevier B.V.All rights reserved.doi:10.1016/j.jhazmat.2008.04.061634J.-f.Peng et al./Journal of Hazardous Materials161(2009)633–6401.IntroductionNowadays,heavy metals originating from anthropogenic activities are frequently detected in sediments and water columns of river/lake,which cause a considerable number of the world’s rivers/lakes severely contaminated[1–4].Heavy metals pollution had gradually become a major concern world-wide.In aquatic environment,heavy metal is usually distributed as follows:water-soluble species,colloids,suspended forms and sedimentary phases.However,unlike organic pollutants,natural processes of decomposition do not remove heavy metals.On the contrary,they usually are enriched in sediment by organisms or some other compounds.In some conditions,more than99%of heavy metal entering into river can be stored in river sediments in various forms[5].However,heavy metals cannotfix in sediment forever.With the variation of the physical–chemical characteris-tics of water conditions,part of thesefixed metals will re-enter the overlying water and become available to living organisms.Thus, sediment often acts as both carriers and potential sources for metals in aquatic environment[1].Heavy metals usually possess signifi-cant toxicity to aquatic organisms,and then affect human health through food chain.Therefore,investigating the transformation and distributions mechanisms of heavy metal in sediment becomes necessary.The main purpose of this paper is to provide a review on the geochemical forms,affecting factors and remediation technologies of heavy metal in sediment,depending on literatures and practi-cal working experiences.Only based on these,the optimization of remediation technologiesfitting for polluted sediment can be achieved.2.Metals species in sedimentIn sediments,metals can be bound to various compartments in different ways:occluded in amorphous materials;adsorbed on clay surfaces or iron/manganese oxyhydroxides;presenting in lattice of secondary minerals like carbonates,sulfates or oxides;complexed with organic matter(OM)or lattice of primary minerals such as silicates[6,7].Since each form has different remobilization poten-tial,and then affects its respective bioavailability and toxicity,the measurement of total metal may not be able to provide exact infor-mation about the characteristics of pollution[8].To clearly investigate the toxicity of heavy metal to aquatic biota, in the past decades,different sequential extraction procedures for partitioning the metals bound to various mineral components had been developed[9].Based on these,the community Bureau of Reference of the Commission of the European Union initiated a major effort to harmonize these extraction procedures.Their works produced the definition of an extraction protocol(the BCR protocol)and a purely operational definition of sequential frac-tionation.For enlarging the research scopes,depending on the different partition conditions among various metals,some assis-tant sequential extraction procedure were also established and popularized.Some primary characteristics of them are shown in Table1.In all of these sequential extraction methods,the four steps method mainly established by Tessier et al.won the most popu-lar application[6].This method partitions the heavy metals into five fractions:extractable and exchangeable,carbonate bound,iron and manganese oxides bound,organic matter bound and residual metal.Exchangeable fraction,usually extracted with magnesium chlo-ride solution or sodium acetate solution(1M)at pH8.2for1h,refers to the metals directly adsorbing on sediments[16].Through some typical sorption–adsorptions processes,these metals can be exchangeable and are in equilibrium with the ionic content in water. Generally,this fraction is usually used to represent the environmen-tally available components.Carbonates fraction,continuously extracted with NaOAc or HOAc solution(1M)at pH5.0for5h,mainly refers to the met-als that are precipitated or co-precipitated with carbonate.This fraction is sensitive to pH variations.Fe–Mn oxides fraction includes the soluble metal oxides/hydroxides under slightly acidic pH as well as the metal associated with reducible amorphous Fe–Mn oxyhydroxides, which was extracted with25%(v/v)acetic acid containing some NH2OH·HCl at96◦C for6h.This fraction can be dissolved with oxidation–reduction potential(ORP)varying.Organic fraction may be associated with various forms of organic material such as living organisms,detritus or coatings on mineral particles through complexation or bioaccumulation process.It is extracted mainly with0.02M nitric acid and30%hydrogen peroxide at pH2.0and85◦C.This kind of metals can exist in sediment for longer periods,and can also be released with OM decomposition.Residual fraction,namely the metals still remained in sediment after the above extraction procedures,usually presents as consoli-dated oxides,co-precipitates,and strongly held complexes,which keeps relatively stable and does not show significant transforma-tion in various conditions.Normally,the summation of the mobile and the exchangeable fractions can be used to assess the environmentally available com-ponents.The fractions bound to Mn oxides and organic material are supposed to represent the potentially mobile component under changing conditions,which are reviewed as the most important components in sediments for metals binding.While the residual fraction represents the more stable metal forms associated with anthropogenic or geogenic components,the influence of which to ecological system is much less than the others in major condi-tions.3.Influence factors for heavy metal distributionsIt has been validated that each environmental factor presents unique influence on metals distribution in sediment.The influences of some factors,such as pH,ORP and OM are more crucial than the others.Only a slight variation of them,the distributions of metals would be producing some significant variations.Correspondingly, some other factors(e.g.salinity,temperature)can only alter met-als distribution to a less extent.In this paper,only those relatively important factors are introduced.3.1.The influence of pH valuesThe pH is a key parameter controlling heavy metal transfer behavior in sediment.Normally,with pH decreasing in sediment, the competition between H+and the dissolved metals for ligands (e.g.OH−,CO32−,SO42−,Cl−,S2−and phosphates)becomes more and more significant.It subsequently decreases the adsorption abilities and bioavailability of the metals,and then increases the mobility of heavy metal.Sometimes,only with a few lower of pH units,thefixation percentage of heavy metals on sediment parti-cles may range from almost a100%to none[17].In sediment,due to the OM degradation and the acid volatile sulfide oxidation,the pH of sediment usually decreases from the neutral in the initial to acid,sometimes even decreasing to pH1.2,which often results in some metals being released into water again even under stable water conditions[15,18].J.-f.Peng et al./Journal of Hazardous Materials161(2009)633–640635Table1Characteristic of different sequential extraction proceduresSequence number Total extraction steps Extracted metal species Reference1Three steps Acid soluble fraction;reducible fraction;oxidizablefraction;residual fraction[10,11]Exchangeable fraction;OM fraction;carbonate fraction;residual fraction[12]2Four steps Extractable/exchangeable fraction;carbonate fraction;ironand manganese oxides fraction;OM fraction;residualfraction[6]3Five steps Exchangeable Cations fraction;carbonates fraction;easilyreducible fraction(e.g.manganese oxides);moderatelyreducible fraction(e.g.amorphous iron oxyhydroxides);oxidizable fraction(organic phase+sulfides);residualfraction[1,8]Exchangeable metals fraction;iron and manganese oxidesfraction;weakly bound to organic matter fraction;stronglybound to organic matter fraction;sulfide phase fraction;residual fraction.[13]4Six steps Mobile fraction;exchangeable fraction;Mn-oxide fraction;organic fraction;amorphous Fe oxides fraction;crystallineFe oxides fraction;residual fraction.[14]5Seven steps Mobile fraction;exchangeable fraction;Mn-oxidesfraction;OM fraction;Fe oxides(anthropogenic)fraction;Fe oxides(geogenic)fraction;sulfide fraction;residualfraction.[15]In sediment,there exists a limit pH controlling heavy metal mobility.And the trace metal would be released only as they reaching such pH value.For different metals,this limit pH is dif-ferent correspondingly,which can be found in Table2.Therefore, under similar pH value,the potential mobility of heavy metals in sediment is different significantly.For example,when pH was con-trolled at4.0,the potential mobility of metal decrease as follows: Zn>Cd>Ni>As>Cu>Pb[19].3.2.The influence of OM speciesOrganic compounds in sediment,frequently existing in consid-erable amounts in particle form,play an important role in heavy metal transformation.For example,in the sediment of some rivers or lakes,the heavy metal bound to OM generally takes up the largest fraction.Additionally,in sediment,the solubility of organic matters usually directly determines the mobility of heavy metals.Normally, the complexation of metal ions with insoluble organic compounds can strongly lower their mobility,whereas the formation of sol-uble metal complexes with dissolved organic compounds would enhance their mobility[20].In natural rivers or lakes,OM is mainly composed of humic and fulvic substances.The complexation reaction between heavy metals and organic complexants is usually recognized as the most important reaction pathway,due to this reaction determining,to a large extent,the speciation and bioavailability of metal,and then influencing the mobility of trace metal in natural water environ-ment.However,in severely polluted river,due to the complexity of organic matter,the reaction types between organic complexes andTable2The limit pH values of different metals in sedimentMetal species pH limitZn 6.0–6.5 Cd 6.0Ni 5.0–6.0 As 5.5–6.0 Cu 4.5Pb 4.0Al 2.5Fe 2.5metals are difficult to predict.In most conditions,precipitation,co-precipitation orflocculation usually plays the most important role in heavy metalfixation.3.3.The influence of ORPIt is generally accepted that sediment ORP is also a most impor-tant factors controlling heavy metal mobility[5].In anaerobic sediment,Acid volatile sulfide(AVS),a key component controlling the activities of some divalent cationic metals,usually present nat-urally[21].Initially,the majority of AVS contained in the anaerobic sediment is bound to iron as solid iron monosulfide(FeS),crys-talline mackinawite(FeS),pyrrhotite(FeS),greigite(Fe3S4),or exits as free sulfides.However,if divalent metals,such as cadmium,cop-per,chromium,lead or zinc are present,the iron in iron-sulfide are displaced and one of these heavy metals rapidly bind to AVS with stronger affinity[22].Finally,in those sediment contaminated,the metal bound with sulfide usually takes up a rather high propor-tion.When ORP in sediment increases,the oxidization rate of metal sulfides and the degradation rate of organic compounds will increase correspondingly.Both can accelerate the release of the adsorbed/complexing heavy metal[23].The reaction can be expressed as:MS2+(15/4)O2+(7/2)H2O M(OH)3+2SO42−+4H+. The release of H+ions into porewater would decrease the pH of sediment and then cause a secondary release of heavy metals.Part of this released material will be re-adsorbed,especially onto the more labile binding fractions.For example,with ORP in sediment increase,the Cd bound to organic sulfide,a stable metal forms, would decrease from65%to30%and form a more mobile form [1,24].Accordingly,with the annual variations of ORP in sediment,the heavy metal presents seasonal release andfixation.These phe-nomena are very significant in some seasonallyflooding rivers. For example,in Mulde reservoir,approximately18t of Zn can be released from sediment into water only due to the sediment being disturbed and oxidized inflooding,which led to a significant increase of Zn concentration in water[1].Therefore,in the dredging process of river or lake,for decreasing the release of metal from sediment,oxidation of sediment should be avoided.636J.-f.Peng et al./Journal of Hazardous Materials161(2009)633–6403.4.The influence of some other factorsExcept pH,OM and ORP,some other factors,such as temper-ature,salinity,metal species and retention time,can also affect the distributions of heavy metal in sediment.For example,due to the differences of cation exchange capacity among different metal, their mobility capacity varies correspondingly,and usually ranges as follows:Cs>Zn>Cd>Fe>Ag>Co>Mn[25];with temperature increasing,the adsorption content of heavy metal on sediment often decreases gradually;with salinity in porewater increasing,the total adsorption content of heavy metal would decrease ascribed to the competition among heavy metal and some other cations [26].Additionally,long time kinetic adsorption–desorption exper-iments also show that the metal freshly associated with particles presents less stable and higher potential bioavailability than those associated for a long time.4.Remediation technologyJust like soil remediation,a two-tiered remediation strategy has also been adopted for remedying the sediment contaminated by heavy metal.Thefirst tier aimed at in situ increasing the stabiliza-tion of metals on sediment particles(e.g.immobilization)and the second tier aimed at ex situ extracting or separating metals from sediment(e.g.washing,flotation)[4,27].Thefirst strategy focus on improving metal stabilization,in which mainly by enhancing metal sorption,precipitation and complexation capacity on sediment,the potential mobility or bioavailability of the toxic metals to environment are lowered.Due to these stabilization techniques usually being carried out“in situ”, their remediation cost is relatively low[28,29].Noteworthily,these techniques just improve the immobility of heavy metal on sedi-ment and their total content in sediment does not lower.Therefore, in some special conditions,part of these immobilized metals will be released into the water again.The other strategy is extraction,in which sediment polluted is dredged from the river bed and heavy metal is extracted from the sediment through a series of chemical,physical,biological methods in a specially designed reactor.This kind of remediation techniques is usually carried out“ex situ”,and can remove almost all mobile metal.However,it also implies the sediment structure deterioration and high costs,which limit their popularization on vast contami-nated areas[30,31].Compared with ex situ remediation techniques,the key advantage of in situ stabilization approach is that only need a simple mixture of amendments with sediment.And the major disadvantage of this approach is that thefinal product of reme-diation,although existing in inactive form,still remains in sediment.4.1.In situ remediation technologyDue to low cost and non-disruptive to natural hydrological con-ditions than conventional ex situ extraction technologies,in situ metal immobilization technologies developed rapidly in the past several decades.Several typical in situ remediation technologies are introduced as follows.4.1.1.AmendmentsAmendment,usually possessing high cation exchange capacity, can lower metal mobility and bioavailability in sediment by precip-itation or sorption,thereby decreasing their solubility.The in situ immobilization of metals,usually using inexpensive amendments such as minerals(e.g.apatite,zeolites,steel shot,or beringite),is considered as a promising alternative to the currently available remediation pared to the amendments used in soil, that used in sediment usually has higher sorption capacity,lower water solubility(K sp=10−40),higher stability under reducing and oxidizing conditions,and lower cost[28].Apatite is usually selected as an ideal amendment for sediment remediation.Generally,it is formulated in the form of Ca10–a−b Na a Mg b(PO4)6−x(CO3)x F2+0.4x with isomorphic substitution of carbon-ate for phosphate,F for hydroxy,and minor substitution of Ca2+ by Na+and Mg2+atoms.In sediment remediation process,metal firstly incorporates into the apatite lattice through ion exchange with Ca2+.This reaction can stimulate apatite dissolution and phos-phate is released correspondingly.Due to the lower solubility between metal ions and phosphate,a new metal-phosphate solid phase(such as Ca10−x Pb x(PO4)6(OH)2)would form[32].Through thesefixing process,apatite minerals can effectively immobilize almost all Pb,Mn,Co,Cu,Cd,Zn,Mg,Ba,U,and Th in sediment [28,33].4.1.2.Sand capNormally,decreasing the direct contact area between water and the contaminated sediment is a good choice for lowering the release content of heavy metal.Therefore,capping the contaminated sedi-ment with sandy materials,such as clean sediment,sand,or gravel becomes an effective remediation technique.Through physical iso-lation,chemical isolation or sediment stabilization,the mobile and the exchangeable metals are transformed from the contaminated sediment into the clean cap and combined with particles in more stable forms.When properly designed,the placement of a rela-tively coarse-grained cap does not disturb or mix with underlying very softfine-grained sediments.Some researchers also showed that a good cap thickness was approximately50cm;and through capping the sediment by sands materials,the heavy metal concen-tration in water could reduce to80%[1].Additionally,compared with other in situ remediation methods,this approach has a lower cost.However,this remediation technique can only reduce the trans-fer rate of metal in sediment,while their immobilization effect for heavy metal is small.Therefore,for enhancing their immobiliza-tion capacity,some amendments(such as apatite,rock phosphate, lime or zeolite)can also be added into the sand cap.These reac-tive materials would demobilize heavy metals from solution and enhance the cap quality.For example,Jacobs and F¨orstner found that through adding the natural zeolite in sand cap,theirfixa-tion capacity for heavy metals and organic contaminants increases sharply[34].4.1.3.PhytoremediationPhytoremediation is the use of plants to extract,sequester,or detoxify pollutants.This technology is widely viewed as an eco-logically responsible alternative to the environmentally destructive chemical remediation methods currently practiced[35].This tech-nology is popularly applied in soil remediation,and also shows some excellent remediation effects in some shallow rivers,lakes and wetlands.At present,this technology had presents good immo-bilization effects for Zn,Fe,Mn and Cd in sediment.Phytoremediation is comprised of two tiers,one by plants themselves and the other by the root colonizing microbes,which degrades the toxic compounds to further non-toxic metabolites. Normally,hydrophytes have the ability to uptake and accumu-late various heavy metals by the action of phytochelatins and metal lothioneins[36].However,mass balance experiments show that metal uptake by hydrophytes were not high enough for phy-toextraction.This indicates that in hydroremediation,the direct uptake of hydrophytes is small,and the indirect reactions,such as stimulation of microbial activity,redox reactions/formation andJ.-f.Peng et al./Journal of Hazardous Materials161(2009)633–640637precipitation of insoluble metal compounds in the rhizosphere, may play a relative important role[37].Therefore,the direct uptake capacity of phytoremediation for heavy metal can be achieved by most plant species able to grow in the contaminated sediment.Cor-respondingly,the choice of hydrophytes would not depend on their apparent uptake capacity,but on their practical immobility capacity for metal.4.2.Ex situ remediation technologyIn situ remediation techniques are usually applied for the sedi-ment slightly polluted by heavy metal.However,for the sediments heavily polluted,their remediation effects can be ignored to a large extent.Under such conditions,ex situ sediment remediation becomes thefirst choice[38].Most ex situ remediation technolo-gies for soil or mineral ores can be used for dredged sediment. However,due to higher workload and different environmental char-acteristics in sediment,some technologies have higher costs and more complex when used in sediment remediation.Only those promising alternative technologies are introduced as follows.4.2.1.WashingSediment washing is a relatively simple and useful ex situ reme-diation technology,which involves through adding washing water, heavy metal can be transferred from the dredged sediment to wash solution.To enhance the performance of sediment washing,various additives can be employed,such as acid washing(e.g.H2SO4and HNO3),chelating agents(e.g.EDTA,DTPA and EDDS)or surfactants (e.g.rhamnolipid).These additives can assist in the solubilization, dispersal and desorption of metal from dredged sediments.This technology is most appropriate for the weaker bound metals in the form of exchangeable,hydroxides,carbonates and reducible oxides fraction.Residual fractions,the most difficult ones to remove,are not affected during the washing process[38,39].Additionally,fine grain sediments are difficult to decontaminate through washing solutions,therefore washing is most applicable to sands and grav-els.Acid leaching,as a typical washing method,refers to the reme-diation of sediment through extracting the metals with sulfuric acid.Considering the sources of leaching agent,acid leaching can be divided into abiotic leaching and microbial leaching[40,41].In abiotic solid-bed leaching,the H2SO4is supplied to the sediment by circulating water with a rate highly dependent on the solid-bed height and percolationflow.However,in microbial solid-bed leaching,elemental sulfur added to the sediment is oxidized to sul-furic acid within the package which,in turn,solubilize the heavy metals.Here,the percolationflow and the solid-bed height do not affect the rate of metal solubilization[42,43].Both methods present perfect removal efficiencies(>90%)for total extractable Ni,Zn,Cu and Cr.And the pH ranges are controlled depending on the heavy metal species.For example,Al is markedly solubilized at pH<4,and Fe at pH<2.4.However,due to a lesser requirement for acid and lime,which subsequently lower the operational difficulties of the microbial solid-bed leaching,the microbial process is80%cheaper than an abiotic solid-bed leaching[29].Additionally,when solid-bed leaching is performed on a larger scale,microbial leaching is much faster than abiotic leaching[40].Therefore,microbial solid-bed leaching presents great attraction in sediment remediation.Chelating agents used in sediment remediation usually possess higher chelating affinity for metal.They can combine with aqueous metal to form chelate complex,which subsequently lower the metal concentration in water.Through the adsorption/release balance between sediment and water,the toxic and bioavailable metals adsorbed on sediment will be transferred to chelate complex grad-ually,andfinally removed with solution.This method is especially suitable for treating the dredged sediment contaminated concur-rently by organic pollutants and heavy metal.EDTA,as a chelating agent popularly applied,can effectively remove Cd,Cu,Pb and Zn with the removal efficiencies ranging between65and86%[44]. However,due to possible adverse health and environmental effects, EDTA is currently under scrutiny.Therefore,the optimization of chelating agents should be the research direction in future.Surfactants,especially biosurfactants,usually possess excellent surface active properties,anionic nature and low toxicity.Through adsorbing onto sediment surface,complexing with metal,detach-ing the metal from the sediment into the porewater and hence associating with surfactant micelles,surfactant can effectively remove the metals adsorbed on sediment particles.For example, when rhamnolipid,a glycolipid biosurfactant,without additives was applied,the removal of heavy metals from sediments was up to37%of Cu,13%of Zn,and27%of Ni[45].4.2.2.Electrochemical remediationElectrochemical remediation involves applying a low DC current or a low potential gradient to electrodes that are inserted into the sediment and encompass the contaminated zone[46].When DC electricfields are applied to the contaminated sediment,migration of charged ions occurs.Positive ions are attracted to the negatively charged cathode,and negative ions move to the positively charged anode.For example,under an induced electric potential,the anionic Cr(VI)migrated towards the anode,while the cationic Cr(III),Ni(II) and Cd(II)migrated towards the cathode.Once the remediation process is over,the contaminants that are accumulated at the elec-trodes are eventually extracted by methods such as electroplating, precipitation/co-precipitation,pumping water near the electrodes, or complexing with ion-exchange resins[16].Because the electric conductivity is the highest in thefine particles of the sediment on which also most metals are adsorbed,and the electricfield is the strongest where the metals are mainly found.This method is well suited forfine-grained dredged sediment.In electrochemical remediation,there are four mechanisms, namely electromigration,electro-osmosis,electrophoresis and dif-fusion,affecting the migration of metals in an imposed electricfield. Electromigration is considered as the dominant transfer mecha-nism for metal due to the transfer rate from electromigration is one to two orders greater than the others[46].Using inert elec-trodes,the electrode reactions will produce H+at the anode and OH−at the cathode,which means that if pH is not controlled,the H+in the anode migrate through the sediment towards the cath-ode,whereas the OH−migrate towards the anode.Depending on the extent of migration of H+and OH−,pH will vary across the sed-iment.An increase in the OH−concentration causes an increase in the pH near the cathode.It has been proved that when heavy metals enter into basic conditions,they are likely to be adsorbed onto soil particles or form precipitate as hydroxides,oxyhydroxides,etc.,and in acidic conditions,those ions desorb,solubilize and migrate.The high pH region in the proximity to the cathode is the main obstacle to heavy metal removal.In order to solubilize the metal hydroxides and carbonates formed,or other species adsorbed onto sediment particles,as well as protonate organic functional groups,acidification may be a very effective method.Generally,in electrochemical remediation pro-cess,the development of an acidic front is often couple with a successful remediation[47].However,this acid addition also has some evident drawbacks.Achieving these acidic conditions might be difficult due to higher sediment buffering capacity;in addition, acidification of dredged sediment may be not an environmen-tally acceptable method.Surfactants can increase the solubility and mobility of heavy metals during electrochemical remediation, depending on its function on decreasing the potential of sediment。
Materials Characterization
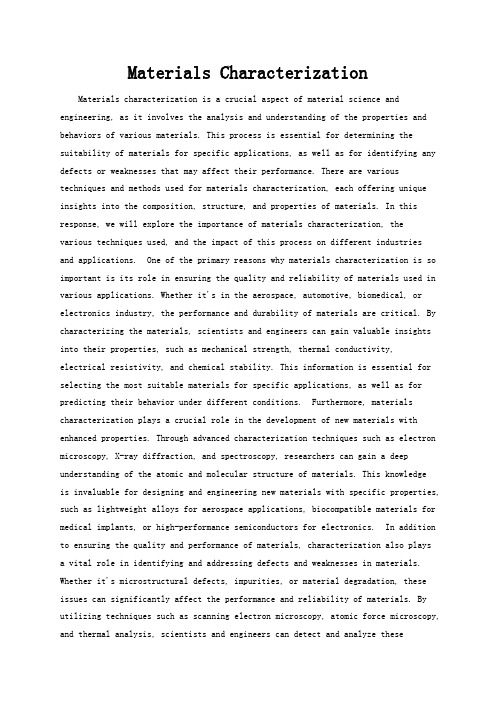
Materials Characterization Materials characterization is a crucial aspect of material science and engineering, as it involves the analysis and understanding of the properties and behaviors of various materials. This process is essential for determining the suitability of materials for specific applications, as well as for identifying any defects or weaknesses that may affect their performance. There are various techniques and methods used for materials characterization, each offering unique insights into the composition, structure, and properties of materials. In this response, we will explore the importance of materials characterization, thevarious techniques used, and the impact of this process on different industriesand applications. One of the primary reasons why materials characterization is so important is its role in ensuring the quality and reliability of materials used in various applications. Whether it's in the aerospace, automotive, biomedical, or electronics industry, the performance and durability of materials are critical. By characterizing the materials, scientists and engineers can gain valuable insights into their properties, such as mechanical strength, thermal conductivity,electrical resistivity, and chemical stability. This information is essential for selecting the most suitable materials for specific applications, as well as for predicting their behavior under different conditions. Furthermore, materials characterization plays a crucial role in the development of new materials with enhanced properties. Through advanced characterization techniques such as electron microscopy, X-ray diffraction, and spectroscopy, researchers can gain a deep understanding of the atomic and molecular structure of materials. This knowledgeis invaluable for designing and engineering new materials with specific properties, such as lightweight alloys for aerospace applications, biocompatible materials for medical implants, or high-performance semiconductors for electronics. In addition to ensuring the quality and performance of materials, characterization also playsa vital role in identifying and addressing defects and weaknesses in materials. Whether it's microstructural defects, impurities, or material degradation, these issues can significantly affect the performance and reliability of materials. By utilizing techniques such as scanning electron microscopy, atomic force microscopy, and thermal analysis, scientists and engineers can detect and analyze thesedefects, allowing them to take corrective actions to improve the material'squality and reliability. The impact of materials characterization extends beyond the realm of research and development, as it also plays a crucial role in quality control and failure analysis in various industries. For example, in the automotive industry, materials characterization is used to ensure the quality and safety of vehicle components, such as engine parts, chassis, and safety systems. In the medical field, materials characterization is essential for evaluating the biocompatibility and performance of medical devices and implants. Moreover, in the electronics industry, characterization techniques are used to assess thereliability and performance of electronic components and semiconductor materials. In conclusion, materials characterization is a fundamental process in material science and engineering, with far-reaching implications for various industries and applications. By gaining a deep understanding of the composition, structure, and properties of materials, scientists and engineers can ensure the quality, reliability, and performance of materials, as well as develop new materials with enhanced properties. Moreover, materials characterization plays a crucial role in identifying and addressing defects and weaknesses in materials, as well as in quality control and failure analysis in different industries. As technology continues to advance, the development of new and improved characterization techniques will further enhance our ability to understand and manipulate materials, driving innovation and progress in various fields.。
Heavy metal2011
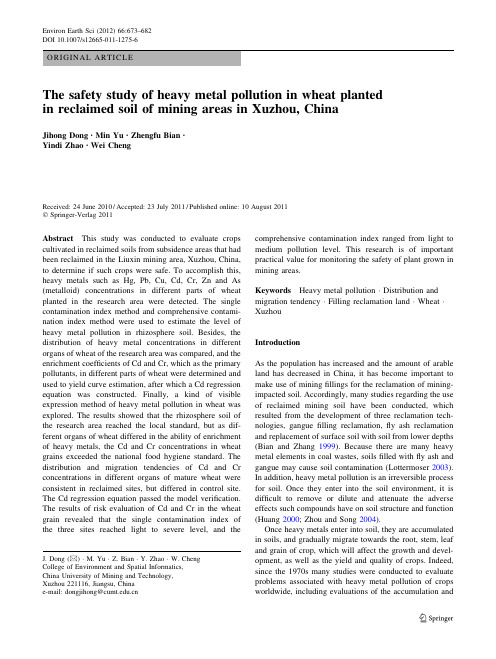
ORIGINAL ARTICLEThe safety study of heavy metal pollution in wheat planted in reclaimed soil of mining areas in Xuzhou,ChinaJihong Dong •Min Yu •Zhengfu Bian •Yindi Zhao •Wei ChengReceived:24June 2010/Accepted:23July 2011/Published online:10August 2011ÓSpringer-Verlag 2011Abstract This study was conducted to evaluate crops cultivated in reclaimed soils from subsidence areas that had been reclaimed in the Liuxin mining area,Xuzhou,China,to determine if such crops were safe.To accomplish this,heavy metals such as Hg,Pb,Cu,Cd,Cr,Zn and As (metalloid)concentrations in different parts of wheat planted in the research area were detected.The single contamination index method and comprehensive contami-nation index method were used to estimate the level of heavy metal pollution in rhizosphere soil.Besides,the distribution of heavy metal concentrations in different organs of wheat of the research area was compared,and the enrichment coefficients of Cd and Cr,which as the primary pollutants,in different parts of wheat were determined and used to yield curve estimation,after which a Cd regression equation was constructed.Finally,a kind of visible expression method of heavy metal pollution in wheat was explored.The results showed that the rhizosphere soil of the research area reached the local standard,but as dif-ferent organs of wheat differed in the ability of enrichment of heavy metals,the Cd and Cr concentrations in wheat grains exceeded the national food hygiene standard.The distribution and migration tendencies of Cd and Cr concentrations in different organs of mature wheat were consistent in reclaimed sites,but differed in control site.The Cd regression equation passed the model verification.The results of risk evaluation of Cd and Cr in the wheat grain revealed that the single contamination index of the three sites reached light to severe level,and thecomprehensive contamination index ranged from light to medium pollution level.This research is of important practical value for monitoring the safety of plant grown in mining areas.Keywords Heavy metal pollution ÁDistribution and migration tendency ÁFilling reclamation land ÁWheat ÁXuzhouIntroductionAs the population has increased and the amount of arable land has decreased in China,it has become important to make use of mining fillings for the reclamation of mining-impacted soil.Accordingly,many studies regarding the use of reclaimed mining soil have been conducted,which resulted from the development of three reclamation tech-nologies,gangue filling reclamation,fly ash reclamation and replacement of surface soil with soil from lower depths (Bian and Zhang 1999).Because there are many heavy metal elements in coal wastes,soils filled with fly ash and gangue may cause soil contamination (Lottermoser 2003).In addition,heavy metal pollution is an irreversible process for soil.Once they enter into the soil environment,it is difficult to remove or dilute and attenuate the adverse effects such compounds have on soil structure and function (Huang 2000;Zhou and Song 2004).Once heavy metals enter into soil,they are accumulated in soils,and gradually migrate towards the root,stem,leaf and grain of crop,which will affect the growth and devel-opment,as well as the yield and quality of crops.Indeed,since the 1970s many studies were conducted to evaluate problems associated with heavy metal pollution of crops worldwide,including evaluations of the accumulation andJ.Dong (&)ÁM.Yu ÁZ.Bian ÁY.Zhao ÁW.Cheng College of Environment and Spatial Informatics,China University of Mining and Technology,Xuzhou 221116,Jiangsu,China e-mail:dongjihong@Environ Earth Sci (2012)66:673–682DOI 10.1007/s12665-011-1275-6migration of heavy metals from soils to crops(Liao et al. 1999;Luksˇien_e and Racˇait_e2008;Jia et al.2010),enrich-ment and distribution of heavy metals in crops(Wu et al. 1998;Barman et al.2000;Mo et al.2002),the factors affecting heavy metal absorption by crops(Liu et al.2004), the correlation of heavy metal content between crops and soils(Feng et al.2002;Voegelin et al.2003)and the mechanisms by which heavy metals migrate and accumu-late(Sterckeman et al.2000;Ettler et al.2005;Pradeep et al.2005;Shen2007).Although the heavy metal pollution has attracted widespread attention(Hu et al.2003;Long et al.2004),very few studies have been conducted to evaluate the food security of reclaimed soil and crop heavy metal pollution to date(Lu2004).This paper aimed to evaluate the status of heavy metal pollution in wheat cul-tivated in reclaimed soil.This study is of practical value to the monitoring of food cultivated in mining areas. Materials and methodsStudy areaThe testingfield was located in the Town of Liuxin in Tongshan County,which is20km away from Xuzhou, northwest of Jiangsu province in China(33°430–4°580N, 116°220–118°400E)(Fig.1).The area has a warm temper-ate semi-humid monsoonal climate,with annual average rainfall of800–930mm,and annual average temperature of13.8°C.The treatment soils included two areas:area A (34°25024N,117°08026E)wasfilled with gangue and area B(34°23043N,117°07029E)withfly ash.Area A was reclaimed in1998by placing gangue into an area that had subsided due to coal mining and then covering the gangue with40–45cm clay as topsoil.Area B was reclaimed in 2001by placingfly ash into an area that had subsided due to coal mining subsidence through pipeline transportation, then covering thefly ash with40–50cm sandy soil as topsoil.The control soil(area C34°23043N,117°06050E) consisted of untreated land near the treatment sites.The control soil had a depth of greater than1m and the soil texture was clay.The cropping systems,the crops and the influence of groundwater were the same for the control soil and the reclaimed soils.The cropping system of the testing field was wheat–rice rotation.Figure2is the photo taken at the same time.Soil samplingBased on the area and shape of the research site,five seedling samples were collected from each research area following a snake-shape pattern.Additionally,three other samples were selected from the sites as accessory samples. For each sampling site,soil samples at different depths–topsoil,20,40cm,interface between covering soil and filling layer and60cm–were collected.All soil and plant samples were kept in packages and tagged.All the plant sample collection was carried out in four periods.In period one(2006.3)15wheat seeding samples were collected;in period two(2006.6)15mature wheat samples were col-lected;in period three(2007.3–2007.5)15wheat seeding samples were collected in March,and15mature wheat samples were collected in May;in period four(2007.9)15 mature wheat samples were collected.Eighty-seven(87) plant samples were collected in all(including12accessory samples).Analytical methodsSoil samples were air-dried and crumbled to sieve through a ten mesh(\2mm).Soil samples(approx.0.2g)were dissolved with a mixture of HNO3(approx.4ml)and HClO4(approx.2ml)then heated.The plant samples were cut into different parts(root,stem,grain,shell),then washed with tap water and then rinsed with deionized water.After being air-dried,the plant samples were ground and digested with a mixture of HNO3(approx.4ml)and HCLO4(approx.2ml).The concentrations of As,Hg,Pb, Cu,Cd,Cr and Zn in different parts of the wheat plants were detected by ICP-AES,with Hg and As being evalu-ated by atomicfluorescence spectrometry(AF-160, AF-2500)and Cr,Zn,Cd,Pb and Cu being evaluated by flame atomic absorption spectrometry.Visual C??and Photoshop software were used for simulating heavy metal concentrations in different parts of the wheat in colors.All the data analysis was carried out using EXCEL and SPSS13.0.The single contamination index method and compre-hensive contamination index method were used to evaluate the heavy metal pollution level of grain.1.Single contamination index method:The formula is I i=C i/S i,where I i is the single con-tamination index of contamination i;C i is the actual con-centration of contamination i;S i is the standard of estimate.If I i\1means unpolluted;1\I i B2means light polluted;2\I i B3means medium polluted;I i[3means severe polluted.prehensive contamination index method:The formula isI¼ffiffiffiffiffiffiffiffiffiffiffiffiffiffiffiffiffiffiffiffiffiffiffiffiffiffiffiffiI i maxðÞ2þI iÀÁ22s:where I is the comprehensive contamination index of one surveyed point;I i max is the biggest comprehensive con-tamination index of contamination;"I i is average value ofcontamination index of each contamination,which isdetermined by "I i ¼1P n i ¼1I i :If I \1means unpolluted,1\I B 2means light pol-luted,2\I B 3means medium polluted and I [3meansseverely polluted;the bigger the value,the more severe the pollution.Results and discussion Selection criteriaHeavy metals are common pollutants in soil that have an impact on the security of crops.The uptake of heavy metals by crops such as grains and vegetables cultivated in con-taminated soils,as well as livestock that feed on such crops,may lead to cancer and other chronic diseases in human through the food chain.Therefore,there are clear definitions of the concentrations of heavy metal pollutants allowed in crops in China and other countries,some of which are presented in Table 1.Fig.1Location map of the studyareaFig.2Sampling location and crop growing situationAs shown in Table1,the allowable concentrations of Pb,Hg,Cd and As in crops are clearly defined in many major countries and regions,and these limits are basically consistent,but there are more stringent standards for heavy metal pollutants in China than in other countries.Based on the limits provided in Table1,Cd,Cr,Cu, Pb,Zn,Hg and As were selected as evaluation elements for this paper.The food hygiene standard of the People’s Republic of China(GB2715-2005)was taken as the standard.However,it only contains regulations for Pb, Cd,Hg and As;therefore,the agricultural professional standard of the People’s Republic of China(NY861-2004) was used as a reference for the evaluation of Cr,Cu and Zn.Analysis of heavy metal concentrations in rhizosphere soilHeavy metal concentrations,which were absorbed by plants,have some relationship with the total amount of heavy metals in soil.As the research sites were used for agriculture,this paper adopted secondary assessment standard according to the soil environment quality standard of China(GB15618-1995)as control standard in rhizo-sphere soil.The results are shown in Table2.The heavy metal concentrations of the three sites have reached the secondary assessment standard of the soil environment quality standard and found suitable for agri-cultural purposes.Table1Permitted levels of heavy metals in crops(mg/kg)Species of grain WheatPollution element Cd Cr Cu Pb Zn Hg As Agricultural professional standard of China a0.11100.4500.020.7 Hygiene standard for grains in China b0.10.20.020.1 Pollution limits in crops in China c0.110.20.020.1 CAC criterion d0.20.2American criterion e1EU criterion f0.1–0.20.2Hong Kong criterion g0.1–2160.5 1.4–10 Australian criterion h0.10.2a The Agricultural Professional Standard of the People’s Republic of China(NY861-2004)b The Hygiene Standard for Grains of the People’s Republic of China(GB2715-2005)c Maximum Content of Contaminants in Food of the Peoples Republic of China(GB2762-2005)d CAC criterion:Maximum Levels for Lead(Codex Stan230–2001,Rev.1-2003),Maximum Levels for Cadmium(CODEX STAN248–2005), General Standard of Pollution and Toxins in food(Codex Stan193)e American Criterion:Maximum Content of Contaminants External Bulletin Opinion Feedback of the American Government(2004)f Maximum Content of Contaminants in Food(79/700/EEC instruction,98/53/EC committee instruction,2001/22/EC instruction,2004/16/EC instruction,2002/63/EC committee instruction)g Maximum Allowable Concentration of Metals of Natural Occurrence(30/06/1997),Maximum Allowable Concentration of Metals in Crops (30/06/1997),Insurant Regulation in Crops L.N.230of2003h Food Standard Code Part1.4Contaminants and Residues Standard1.4.1,Contaminants and Natural ToxicantsTable2Comparison between heavy metal concentrations in rhizosphere soil of different sites and soil environment quality standard(GB15618-1995)(mg/kg)Cd Cr Cu Pb Zn Hg AsGangue siteAverage value0.4044.4931.8612.9291.350.03424.29 Standard deviation0.15 3.68 1.97 1.17 2.270.003 3.70 Fly ash siteAverage value0.2231.5217.69 4.4948.220.01514.27 Standard deviation0.10 6.218.16 3.18 6.240.003 5.62 Control siteAverage value0.4240.7031.3313.23108.470.05120.13 Standard deviation0.14 1.700.82 1.2510.450.012 1.06 Secondary assessment standard 1.0250100350300 1.025Analysis of heavy metal concentrations in different organs of wheatAnalysis of heavy metal concentrations in wheat grain Once heavy metals enter into human bodies through the food chain,they are accumulated and not degraded.Wheat grain is an edible part of wheat;heavy metals in wheat grain will enter human bodies through it.Therefore,the heavy metal concentrations of wheat grain in different reclamation soils were evaluatedfirst and compared with the national food hygiene standard.Results are shown in Table3.As shown in Table3,with the exception of Cr and Cd, none of the wheat contained heavy metals in levels that exceeded the food hygiene standard.The average value of Cd concentration infly ash site and control site exceed the standard and is close to the standard in gangue site. However,the average values of Cr concentration in all the sites exceed the standard.Based on this,the heavy metal concentrations in different wheat organs in different growth period are analyzed.Analysis of heavy metal concentrations in stemsTable4shows heavy metal concentrations in immature seedlings and mature stems.The results of the present study revealed the following:(1)The Cd and Hg concentrations are lower in immature seedlings than mature stems;(2)The Cr,Cu and Zn concentrations are greater in immature seedlings than mature stems;(3)The regulations of Pb and As are not too obvious.Pb concen-tration is lower in immature seedlings than in mature stems of wheat grown at the gangue andfly ash site,while the reverse is true at the other sites.Additionally,As concen-tration is lower in immature seedlings than in the stems of wheat grown at the gangue site,while the opposite is true for wheat grown at the other two sites;(4)there are greater differences in the heavy metal concentration of the same metal in immature wheat seedlings and mature stems dur-ing various stages of growth.Analysis of heavy metal concentrations in rootsTable5shows that all the heavy metal concentrations accumulated in immature roots were lower than in mature roots of wheat grown in gangue andfly ash sites.In addition,Cr and Zn concentrations in immature roots were also lower than that in mature roots in control site,while the other heavy metal concentrations in immature roots were higher than that in mature roots.Based on the data presented,there is great Cr and Cd pollution in wheat.Therefore,it is necessary to evaluate the capability of these heavy metals to be taken up by wheat,as well as their distribution after uptake.Table3Heavy metal concentrations of wheat grains in different sites(mg/kg)Cd Cr Cu Pb Zn Hg AsGangue siteAverage value0.09 3.21 6.170.1344.38––Standard deviation0.04 1.65 1.16–8.03––Fly ash siteAverage value0.18 1.37 4.06–33.030.001–Standard deviation0.040.610.64– 6.26––Control siteAverage value0.14 2.34 5.29–39.060.003–Standard deviation0.040.940.81–8.460.001–Standard0.1 1.0100.2500.020.1Table4Heavy metal concentrations of wheat seedlings and mature stems (mg/kg)Comparative object Sites Cd Cr Cu Pb Zn Hg AsImmature seedlings Gangue site0.0214.13 6.910.3042.690.000.13 Fly ash site0.0316.33 3.930.6027.680.000.18Control site0.0428.088.440.3563.160.000.26 Stems Gangue site0.1513.49 4.150.6127.880.020.15 Fly ash site0.2015.77 2.240.8522.850.020.16Control site0.2420.97 3.840.2837.540.020.13Distribution and migration of Cr and CdDistribution of Cd and Cr in different organs in mature wheatTo reveal the percentage of Cd and Cr concentrations in different organs of wheat,Cd and Cr concentrations in different organs of wheat were divided by the total con-centration in wheat.The distributions of Cd and Cr con-centrations in wheat are shown in Fig.3.According to the results,it was determined that Cd concentrations of different organs in mature wheat occur-red in the following order:gangue sites:root [shell [stem [grain;fly ash sites:root [shell [stem [grain;control sites:stem [root [grain [shell;Cr concentra-tions in different organs in mature wheat were as follows:gangue sites:root [shell [stem [grain;fly ash sites:root [shell [stem [grain;control sites:shell [stem [root [grain.The distribution and migration of Cd and Cr in different organs were consistent in mature wheat from the reclaimed soils,while they differed in wheat from the control soil.Simulation color mapsAccording to the raw data,wheat can be divided into an edible part,rejected part,stems,roots and rhizosphere soil (Fig.4).Based on Cd and Cr concentrations in different organs of wheat analyzed before,different colors corresponding to different heavy metal concentrations were assigned formore intuitive observation.Mapping was conducted using the Visual C ??software,with raw data generated using a color map table and Photoshop used to generate the final map.Heavy metal concentrations were detected in different organs of wheat and then assigned a color as described below.The results are shown in Fig.5.As shown in Fig.5,Cd concentrations tend to be 0in immature seedlings and between 0.3and 0.4in mature roots in the three sites.It means very little Cd is absorbed by immature wheat,and there is no certain relationship between Cd concentration in immature wheat with Cd concentration in rhizosphere soil.However,there is great change of Cd distribution in mature wheat.Specifically,Cd concentrations in grain are similar in fly ash and control site and higher than in gangue site.There is significant increaseTable 5Heavy metal concentrations of wheat immature and mature roots (mg/kg)Comparative object Sites Cd Cr Cu Pb Zn Hg As Immature rootsGangue site 0.2211.189.33 1.68109.510.00 1.61Fly ash site 0.3110.399.540.8029.560.00 2.83Control site0.26 6.4910.140.7674.820.09 1.42Mature rootsGangue site 0.4874.9811.68 4.60180.560.01 1.92Fly ash site 0.4865.3714.780.9167.380.01 5.48Control site0.1815.539.560.2899.380.021.01Fig.3Percentage of Cd and Cr in different organs in mature wheatRootStemwheatin the roots of reclaimed sites,higher than the control site;the concentrations in inedible parts also are higher.Cr concentrations are higher in immature seedlings at the control site than at the reclaimed sites and generally the same in immature roots of all the sites.The concentrations in grain,stem and shell are similar in mature wheat.Finally,the Cr concentration in roots is much higher in reclaimed sites than in control site.Simulation color maps expressing the regulation of heavy metal content in different parts of the wheat are then constructed for each site,and the results show that this method has a good effect.Enrichment coefficient of Cd and CrPollutants are primarily absorbed by plants through roots in the soil.The enrichment coefficient of plant (b i)reflects the heavy metal enrichment capacity of wheat and the accu-mulating strength from soil to wheat.The enrichment coefficients of different parts of the mature wheat for Cd and Cr are shown in Fig.6,respectively.The enrichment capacity of Cr is found to be stronger than that of Cd in different parts of wheat cultivated at different sites.Additionally,the coefficients of Cd and Cr in the roots and stem are relatively higher in wheat grown at the gangue and fly ash site,while the distribution of Cd is more uniform in different organs of wheat cultivated atthe control site.In addition,Cr distributes uniformly in the roots,stems and shells,but presents in lower levels in grain.Clearly,the enrichment capabilities of Cd and Cr varied among different parts of wheat.As plants absorb heavy metals in soils through the roots,and then migrate to other parts of the plant,the absorption and enrichment capacity of heavy metals in plants depends on many soil factors,such as the heavy metal concentra-tions,soil physical and chemical properties,texture,organic matter content and the variety of plant.To confirm the influence of the factors on the wheat enrichment capacity,correlation analysis between the enrichment coefficient and Cd and Cr concentrations in soil was con-ducted (Table 6).From Table 6,it can be seen that the relevance between Cr,Cd and enrichment coefficient of different parts in wheat was not strong,except for Cd and enrichment coefficient of stem and grain appeared comparatively strong.Since the factor that influences the enrichment ability of plant could not be determined in this experiment,more experiments should be conducted in the next research procedure.Regression equation of Cd and CrSPSS software was used to conduct curve estimation for the Cd enrichment coefficient and root enrichment coeffi-cient rate in the grain,stem and shell of mature wheatfromFig.5colored mapping graph of Cd andCrFig.6Cd and Cr enrichment coefficient in different parts of mature wheatdifferent sites.There’re many models include linearfitting, compositefitting,growthfitting,logarithmfitting,expo-nentialfitting,inversefitting and so on,adopt which model depending on whether R2approach largely to1,so the powerfitting was adopted in this research.An F test was used to test the regression equation.The results are shown in Table7.Based on the data presented in Table7,a suitable model was found to describe the relationship among wheat grain, shell and stem enrichment with Cd.The accumulated rate of grain and enrichment coefficient of the root was found to have a linear relationship that was described by the fol-lowing regression equations:Gangue sites:b grain=-0.32?0.4679b root Fly ash sites:b grain=-0.38?1.0439b root Control sites: b grain=-0.309?0.6199b root where b grain is the enrichment coefficient of grain and b root is the enrichment coefficient of rootHowever,the relationships among wheat grain,shell and stem enrichment with Cr were complicated,so no suitable model was found to describe those relationships. Evaluation of ecological risk of wheat grainTo further consider the influence of Cd and Cr on wheat grain,the single contamination index method and comprehensive contamination index method were used to evaluate the heavy metal pollution level of grain.The results are shown in Table8.Table8indicates that the average value of single contamination index of the three sites ranged as gangue site:Cr[Cd[Zn[Pb[Cu[Hg=As,fly ash site: Cd[Cr[Zn[Cu[Pb=Hg=As,control site:Cr[ Cd[Zn[Cu[Pb=Hg=As.The contamination index of Cr in gangue site is the largest,reaching3.21,the severe pollution level;two sample points of Cd reached light pollution level;the other elements did not exceed the standard.Cd and Cr infly ash site reached light pollution level with contamination indices of1.78and1.37;the other elements did not exceed the standard.In the control site, the contamination index of Cd is1.66and achieves the light pollution level,while Cr achieves medium even sever pollution level with the contamination index of2.9.As the comprehensive contamination index,the wheat grain in gangue site has reached medium pollution level with the average value of2.36,due to the Cr pollution. While infly ash site the comprehensive contamination index of wheat grain ranged from1.03to1.77,the average value was1.37which caused the site to be lightly polluted by Cd and Cr.The comprehensive contamination index of wheat grain in control site ranged from1.50to3.26,and the average value was 2.13which means the site has reached medium pollution.Totally speaking,the wheatTable6Interrelated coefficient between Cr and Cd concentrations and enrichment coefficient of different parts in wheat Element Sites Roots Stems Shells GrainsCd Gangue site0.5990.3940.604-0.705 Fly ash site-0.109-0.4560.609-0.791Control site-0.1500.8900.263-0.348 Cr Gangue site-0.4500.2990.6350.146 Fly ash site-0.0810.189-0.460-0.340Control site-0.4010.0200.294-0.445Table7Relationship of the Cd enrichment coefficient in different parts of mature wheat (mg/kg)The data made bold and italic indicate the equation do not satisfy the F test of the mode,it should be rejected Sites Variable(Y)Independentvariable(t)Model Equation Testing of modelR2F F0.05(1.3) Gangue site Grains Roots Linearity Y=-0.320?0.467t0.96993.57310.13 Shells Roots Linearity Y=-0.023?0.377t0.94349.26410.13Stems Roots Linearity Y=0.067?0.261t0.79211.43410.13 Fly ash site Grains Roots Linearity Y=-0.038?1.043t0.87721.32010.13 Shells Roots Linearity Y=0.340?0.250t0.7619.55110.13Stems Roots Inverse Y=0.672-1.181/t0.7077.23410.13 Control site Grains Roots Linearity Y=-0.038?1.043t0.87721.32010.13 Shells Roots S Y=exp(-1.563-0.327/t)0.0570.18310.13Stems Roots Linearity Y=0.309?0.619t0.95869.26810.13grown in the study area has been polluted,causing certain harm to people.ConclusionsThis study showed the situation of heavy metal pollution in wheat grown in different periods of the research area,and the main conclusions are as follows:1.Distribution of heavy metal concentrations in differentorgans of wheat:All heavy metals taken up by wheat present in lower concentrations in immature roots than in mature roots in plants grown in the reclamation soils.In addition,the Cr and Zn concentrations are lower in immature roots than in mature roots in plants grown in the control soils,while the other heavy metals present in higher concentrations in the imma-ture roots than in the mature roots.Cd and Hg concentrations are lower in immature seed-lings than in mature stems,while the Cr,Cu and Zn con-centrations are higher in immature seedlings than in mature stems at the three sites.Pb concentration is lower in immature seedlings than in mature stems of plants from the gangue andfly ash sites,while the reverse is true in plants at the control site.As concentration is lower in immature seedlings than in the stems for plants from the gangue site, while the reverse is true in plants from the other two sites.Because the enrichment capability and sensitivity of heavy metals is different in different parts of wheat,the levels of Cd and Cr concentrations in wheat grain exceeded that prescribed by the food hygiene standards of China. 2.The distribution and migration of Cd and Cr areconsistent in different organs of mature wheat plants cultivated in reclaimed soils,and the sequence of concentration from high to low is root[shell[ stem[grain.Conversely,the distribution of the metals in plants grown in the control soil differed, with the distribution of Cd from high to low being stem[root[grain[shell and the distribution of Cr being shell[stem[root[grain.3.The distribution and migration of Cd and Cr indifferent parts of mature wheat are evaluated using the root enrichment coefficient rate,performing curve estimation and constructing a Cd regression equation.These methods are able to describe the accumulation of these heavy metals in crops bined with the assessment results of soil quality,although all the sites reached the secondary assessment standard according to the soil environment,the heavy metals may be influenced by enrichment capability and sensitivity and migrate to different parts of the wheat, thus polluting wheat and endangering humans.4.The risk assessment of wheat grain showed that assingle contamination index,there is light to severe Cd,Table8Heavy metal risk assessment results of wheat seedsSites Sample points Single contamination index ComprehensivecontaminationindexLevelI Cd I Cr I Cu I Pb I Zn I Hg I As I comGangue site10.60 4.510.77– 1.12–– 3.27Severe20.60 5.400.63 3.150.97–– 3.97Severe30.70 2.210.66–0.86–– 1.63Light4 1.20 1.560.46–0.69–– 1.17Light5 1.40 2.360.58–0.81–– 1.75LightAverage value0.90 3.210.62–0.89–– 2.36Medium Fly ash site1 1.20 1.360.41–0.73–– 1.03Light2 2.00 1.420.46–0.74–– 1.49Light3 2.20 2.360.47–0.78–– 1.77Light4 1.800.810.37–0.59–– 1.32Light5 1.700.910.32–0.48–– 1.25LightAverage value 1.78 1.370.41–0.66–– 1.37Light Control site1 1.10 2.960.52–0.77–– 2.16Medium2 1.80 4.470.59– 1.02–– 3.26Severe3 1.80 2.410.42–0.73–– 1.79Light4 2.10 2.620.46–0.72–– 1.95Light5 1.50 2.020.38–0.55–– 1.50LightAverage value 1.66 2.900.47–0.75–– 2.13Medium。
heavy metals
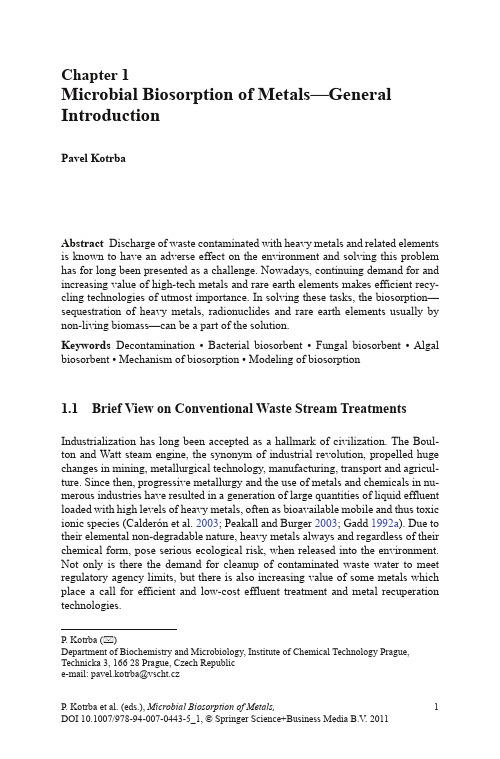
1Abstract Discharge of waste contaminated with heavy metals and related elements is known to have an adverse effect on the environment and solving this problem has for long been presented as a challenge. Nowadays, continuing demand for and increasing value of high-tech metals and rare earth elements makes efficient recy-cling technologies of utmost importance. In solving these tasks, the biosorption—sequestration of heavy metals, radionuclides and rare earth elements usually by non-living biomass—can be a part of the solution.Keywords Decontamination • Bacterial biosorbent • Fungal biosorbent • Algal biosorbent • Mechanism of biosorption • Modeling of biosorption1.1 B rief View on Conventional Waste Stream TreatmentsIndustrialization has long been accepted as a hallmark of civilization. The Boul-ton and Watt steam engine, the synonym of industrial revolution, propelled huge changes in mining, metallurgical technology, manufacturing, transport and agricul-ture. Since then, progressive metallurgy and the use of metals and chemicals in nu-merous industries have resulted in a generation of large quantities of liquid effluent loaded with high levels of heavy metals, often as bioavailable mobile and thus toxic ionic species (Calderón et al. 2003; Peakall and Burger 2003; Gadd 1992a ). Due to their elemental non-degradable nature, heavy metals always and regardless of their chemical form, pose serious ecological risk, when released into the environment. Not only is there the demand for cleanup of contaminated waste water to meet regulatory agency limits, but there is also increasing value of some metals which place a call for efficient and low-cost effluent treatment and metal recuperation technologies.P. Kotrba et al. (eds.), Microbial Biosorption of Metals, DOI 10.1007/978-94-007-0443-5_1, © Springer Science+Business Media B.V . 2011Chapter 1Microbial Biosorption of Metals—General Introduction Pavel KotrbaP. Kotrba ( )Department of Biochemistry and Microbiology, Institute of Chemical Technology Prague, Technicka 3, 166 28 Prague, Czech Republic e-mail: pavel.kotrba@vscht.cz2P. Kotrba Conventional procedures for heavy metal removal from aqueous industrial ef-fluents involve precipitation, ion exchange, electrochemical methods and reverse osmosis. Another promising approach is solvent extraction. Conversion of metal ions to insoluble forms by chemical precipitation is the most common method, reducing the metal content of solution to the levels of mg l−1. The cheapest pre-cipitation technique relies on alkalization of the metal solution (usually with lime) to achieve formation of insoluble metal species, namely of hydroxides. Chemical precipitation could also be achieved by the addition of other coagulants, such as of potash alum, sodium bisuphite, sulphide or iron salts. Though it is cost effec-tive; such precipitation lacks the specificity, produces large volumes of high water content sludge and has low performance at low metal concentrations. Although adsorption using activated carbon is generally expensive (and not suitable for many metal species), it is an efficient method for the removal of metallic mercury following chemical reduction (e.g., with hydrazine) of mercuric ions in heavily contaminated process waters.Ion exchange employing manmade synthetic organic resins is the most common method. It becomes the method of choice especially for its capacity to reduce the metal contents to μg l−1 levels in relatively large volumes of effluent, some possi-bility to formulate metal-selective resin and well established procedures for metal recovery from and reuse of the ion exchanger. This method is, however, relatively expensive, which therefore makes the processing of concentrated metal solutions cost intensive. Precautions should also be taken to prevent the poisoning of ion exchanger by organics and solids in solution.Electro-winning, employing electro-deposition of metals on anodes is popu-larly used for the recuperation of metals in mining and metallurgical operations as well as in electrical industries and electronics. Electrodialysis involves the use of ion selective semi-permeable membranes fitted between the charged electrodes attracting respective ions (in the case of metal cations, the anode compartment is smaller to concentrate the metal in). The main disadvantage of electrodialysis operation is clogging of the membrane by metal hydroxides formed during the process. Like electrodialysis, reverse osmosis and ultrafiltration employ semi-permeable membranes which allow water to pass, while solutes, including heavy metals, remain contained in retentate. The advantages of membrane-based pro-cesses involve some selectivity of metal separation and tolerance to changes in pH. One disadvantage of membrane-based approaches is that they are cost intensive.Reactive two-phase extraction complexing extractants specifically (or preferen-tially) dissolved in organic solvents has been suggested as another technological alternative (Schwuger et al. 2001). This approach may provide a viable method for the selective recuperation of metals, e.g., of platinum group metals from spent cata-lysts (Marinho et al. 2010). Suitable extractants for platinum involve organophos-phorus compounds, aliphatic amines and ammonium quaternary salts. The main disadvantages of this process are the difficult recovery of metals from organic phase and the toxicity of extractants.3 1 Microbial Biosorption of Metals—General Introduction1.2 B io-based Methods for Waste Water Treatment and Environment RestorationThe natural capacity of microrganisms, fungi, algae and plants to take up heavy metal ions and radionuclides and, in some cases, to promote their conversion to less toxic forms has sparked the interest of (micro)biologists, biotechnologists and environmental engineers for several decades. Consequently, various concepts for “bio-removal” of metals from waste streams and bioremediations of contami-nated environment are being proposed, some of which were brought to pilot or industrial scale (Bargar et al. 2008; Macek et al. 2008; Muyzer and Stams 2008; Singh et al. 2008; Chaney et al. 2007; Sheoran and Sheoran 2006; Volesky 2004; Lloyd et al. 2003; Ruml and Kotrba 2003; Baker et al. 2000; Gadd 1992b; see also Chap. 2). The “bio” prefix refers to the involvement of biological entity, which is living organisms, dead cells and tissues, cellular components or products. The ultimate goal of these efforts is to provide an economical and eco-friendly tech-nology, efficiently working also at metal levels below 10 mg l−1. These are the features that living as well as dead biomass could be challenged for. There are generally three routes to follow considering “bio-removal” of metallic species from solutions. The first two rely on properties of living cells and involve active metal uptake—bioaccumulation (i.e., plasma membrane mediated transport of metal ion into cellular compartment) and eventual chemical conversion of mobile metal to insoluble forms. The later may occur in the cytoplasm, at the cell surface or in the solution by precipitation of metal ion with metabolites, via redox reac-tions or by their combination. The effectiveness of the process will depend on the (bio)chemistry of particular metal and on metabolic activity of eligible organism, which is in turn affected by the presence of metal ions. To this point, the use of metallotolerant species or physical separations of the production of metal-pre-cipitating metabolite from metal precipitation in contaminated solution produce viable methods. For their importance in the treatment of industrial liquid streams as well as of the environmental pollution are some of these approaches discussed in Chap. 9. Several of them are to various extents dependent on or involve the metabolism-independent metal uptake event at the cell wall by polysaccharides, associated molecules, and functional groups. This metal sequestration capacity is commonly known as biosorption, which itself represents the third potent way of “bio-removal” of metals from solution.Biosorption is a general property of living and dead biomass to rapidly bind and abiotically concentrate inorganic or organic compounds from even very di-luted aqueous solutions. As a specific term, biosorption is used to depict a method that utilizes materials of biological origin—biosorbents formulated from non-living biomass—for the removal of target substances from aqueous solutions. Biosorp-tion “traditionally” covers sequestration of heavy metals as well as rare earth ele-ments and radionuclides or metalloids, but the research and applications extended to the removal of organics, namely dyes (Kaushik and Malik 2009; see also some examples with magnetic biocomposite biosorbents in Chap. 13), and biosorption is4P. Kotrba being proposed for the recovery of high-value proteins, steroids, pharmaceuticals and drugs (V olesky 2007).Decades of biosorption research provided a solid understanding of the mecha-nism underlying microbial biosorption of heavy metals and related elements. It in-volves such physico-chemical processes as adsorption, ion-exchange, chelatation, complexation and microprecipitation. These depend on the type and ionic form of metal, the type of metal binding site available from microbial biomass, as well as on various external environmental factors (see Chap. 3). Accumulated knowledge resulted in the development of suitable modelling approaches comprehensively described in Chap. 4. When properly used, these models explain the equilibrium biosorption data, the kinetics in batch reactors and the dynamics in biosorption col-umns both for single and multimetal systems and provide a powerful tool for the de-sign and development of the actual biosorption process. It should be noted here that it was due to a poor understanding of mechanisms and kinetics of AlgaSORB TM and AMT-Bioclaim TM processes commercialized in the early 1990s that hindered the adequate assessment of process performance and limitations, and thus the expected widespread industrial application of biosorption.Biosorbents are derived from raw biomass selected for its superior metal-sequestering capacity. Investigated biomass types are of such diverse origins as bacterial, cyanobacterial, fungal (including filamentous fungi and unicellular yeasts), algal, plant or even animal (chitosan). This book covers development in major areas exploiting bacterial biomass (Chap. 5), fungal biomass (Chap. 6) and algal biomass (including macroalgae; Chap. 7) for the biosorption of heavy metal and radionuclides as well as for the sequestration of precious and rare earth elements (Chap. 8). It is noteworthy to add that the potential of plant-based biosorbents formulated from agricultural waste is attaining growing attention (Demirbas 2008; Sud et al. 2008; a few examples with magnetic biocomposite biosorbents are given in Chap. 13). The cheapest microbial biomass could be procured from selected fermentation industries as waste by-product or could be harvested from its natural habitat when it is produced in sufficient quantity there (e.g., marine macroalgae). Independent propagation of biomass under specific conditions optimizing its metallosorption properties is another option. Some ef-forts have been also devoted to modifications of yeast (Chap. 10) and bacterial (Chap. 11) cell walls through their genetic engineering, resulting in a surface display of particular amino acid sequences providing additional (even selective) metal-binding sites.When derived from dead raw biomass featuring high metal uptake, the biosor-bent for its practical application should exhibit some additional characteristics im-proving its stability and favoring hydrodynamic process conditions. To this end, biosorbent particle size, density and porosity, hardness, resistance to a broad range of physical and chemical conditions could be tailored by an appropriate immo-bilization method. Conventional strategies of biosorbent formulation from differ-ent types of microbial biomass are described in respective chapters as well as in Chap. 12. Chapter 13 further sets the biosorbent design forward to “smart materi-als”, the magnetically responsive biocomposites improving biosorbents applicabil-1 Microbial Biosorption of Metals—General Introduction5 ity by enabling their selective magnetic separation even from solutions containing suspended solids.1.3 F uture Thrusts in BiosorptionCompared with conventional or some biological methods for removing metal ions from industrial effluents, the biosorption process offers the advantages of low op-erating cost, minimization of the use of chemicals, no requirements for nutrients or disposal of biological or inorganic sludge, high efficiency at low metal concen-trations, and no metal toxicity issues. The operation of biosorption shares many common features with ion-exchange technology and, despite shorter life cycle and less selectivity options, biosorbents could be considered direct competitors of ionex resins. The high cost of the ion-exchange process limits its application. Not all industries producing metal bearing effluents have financial resources for such sophisticated treatment and most opt only for basic decontamination techniques to meet regulation limits. The accumulated knowledge already provides a solid basis for the commercial exploitation of biosorption processes. Huge markets already exist (V olesky 2007) and they may even grow with progressively stricter legisla-tion worldwide and increase demand on metal resources. Future efforts to improve selectivity and shelf life of biosorbents, further information on biosorption mecha-nisms and reliability and performance of biosorption models as well as more pilot scale demonstrations should bring convincing marketing arguments for large-scale applications. Biosorption also has the potential to find an industrial application in the future separation technologies with renewable biosorbents complementing con-ventional methods in hybrid or integrated installations.ReferencesBaker AJM, McGrath SP, Reeves RD, Smith JAC (2000) Metal hyperaccumulator plants: a review of the ecology and physiology of a biological source for phytoremediation of metal-polluted soil. In: Terry N, Bañuelos GS (eds) Phytoremediation of contaminated soil and water. CRC Press, Boca Raton, pp 85–107Bargar JR, Bernier-Latmani R, Giammar DE, Tebo BM (2008) Biogenic uraninite nanoparticles and their importance for uranium remediation. Elements 4:407–412Calderón J, Ortiz-Pérez D, Yáñez L, Díaz-Barriga F (2003) Human exposure to metals. Pathways of exposure, biomarkers of effect, and host factors. Ecotoxicol Environ Saf 56:193–103 Chaney RL, Angle JS, Broadhurst CL, Peters CA, Tappero RV, Sparks DL (2007) Improved under-standing of hyperaccumulation yields commercial phytoextraction and phytomining technolo-gies. J Environ Qual 36:1429–1443Demirbas A (2008) Heavy metal adsorption onto agro-based waste materials: a review. J Hazard Mater 157:220–229Gadd GM (1992a) Metals and microorganisms: a problem of definition. FEMS Microbiol Lett 79:197–203P. Kotrba 6Gadd GM (1992b) Microbial control of heavy metal pollution. In: Fry JC, Gadd GM, Herbert RA, Jones CW, Watson-Craik IA (eds) Microbial control of pollution. Cambridge University Press, Cambridge, pp 59–87Kaushik P, Malik A (2009) Fungal dye decolourization: recent advances and future potential. En-viron Int 35:127–141Lloyd JR, Lovley DR, Macaskie LE (2003) Biotechnological application of metal-reducing micro-organisms. Adv Appl Microbiol 53:85–128Macek T, Kotrba P, Svatoš A, Demnerová K, Nováková M, Macková M (2008) Novel roles for GM plants in environmental protection. Trends Biotechnol 26:146–152Marinho RS, Afonso JC, da Cunha JW (2010) Recovery of platinum from spent catalysts by liq-uid–liquid extraction in chloride medium. J Hazard Mater 179:488–494Muyzer G, Stams AJ (2008) The ecology and biotechnology of sulphate-reducing bacteria. Nat Rev Microbiol 6:441–454Peakall D, Burger J (2003) Methodologies for assessing exposure to metals: speciation, bioavail-ability of metals, and ecological host factors. Ecotoxicol Environ Saf 56:110–121Ruml T, Kotrba P (2003) Microbial control of metal pollution: an overview. In: Fingerman M, Nagabhushanam R (eds) Recent advances in marine biotechnology, vol 8. Science Publishers Inc., Enfield, pp 81–153Schwuger MJ, Subklew G, Woller N (2001) New alternatives for waste water remediation with complexing surfactants. Colloid Surface A 186:229–242Sheoran AS, Sheoran V (2006) Heavy metal removal mechanism of acid mine drainage in wet-lands: a critical review. Mineral Eng 19:105–116Singh S, Kang SH, Mulchandani A, Chen W (2008) Bioremediation: environmental clean-up through pathway engineering. Curr Opin Biotechnol 19:437–444Sud D, Mahajan G, Kaur MP (2008) Agricultural waste material as potential adsorbent for se-questering heavy metal ions from aqueous solutions—a review. Biores Technol 99:6017–6027 V olesky B (2004) Sorption and biosorption. BV-Sorbex Inc., St. LambertV olesky B (2007) Biosorption and me. Water Res 4017–4029。