taurine zebrafish brain
【高中生物】斑马鱼如何长出新的神经元

【高中生物】斑马鱼如何长出新的神经元
研究人员已经发现了使得斑马鱼的大脑能够在其受到创伤性损害之后再生的机制。
与哺乳动物不同,这些在淡水中生长的小鲦鱼因为脑部损伤所致的炎症会伴有新神经元的产生。
如今,Nikos Kyritsis及其同事展示,在损伤反应中,斑马鱼脑部的炎症会激活特定的信号传导分子及神经胶质细胞,后者可促进替代神经元的生长。
研究人员在不造成损伤的情况下,用药物引发了斑马鱼脑中的炎症,实验结果显示,被称作放射状胶质细胞的特定细胞仍然可受到诱导而产生新的细胞。
然而,他们说,炎症信号受到抑制的斑马鱼则无法产生新的神经元或鳍细胞。
Kyritsis和其他的研究人员发现,一种叫做半胱氨酰白三烯受体1??或Cystlr1的蛋白质的表达对斑马鱼的再生过程是至关重要的。
当他们在斑马鱼脑内注射一种叫做LTC4的该蛋白的配体时,研究人员观察到,在没有任何炎症时新的神经元也会产生。
因此,他们鉴于这些结果提出,在放射状胶质细胞内,炎症一定是通过与LTC4信号传导级联反应进行耦合才能使斑马鱼的受损神经元重新生长。
他们表示,他们的发现可能最终会在治疗创伤性脑损伤及神经退行性疾病中找到治疗性的应用。
感谢您的阅读,祝您生活愉快。
獭尾肝——精选推荐

獭尾肝獭尾肝左肝叶向左后⽅的突起称为獭尾肝(beaverlliver),别名包围肝、象⿐肝是临床并不少见的肝脏正常变异。
发⽣机制出⽣前,肝脏营养靠脐静脉输送。
脐静脉在左叶间裂处向右⾃然弯曲与门静脉连接,同时营养左右肝叶。
出⽣后,肝脏营养靠肝动脉、门静脉输送,脐静脉闭合,肝内⾎管合并、退化,数⽬减少,门静脉主⼲与其左⽀的夹⾓成锐⾓,致使左肝供⾎、⽣长发育均不及右叶,左外叶后部肝组织逐渐退化,遗留的残迹称为肝纤维附件或肝纤维垂,其内可有少许肝组织和迷⾛肝管,若其内仍有完整肝组织,形成的肝叶就是獭尾肝,见于5%成⼈。
超声表现:①肝左外缘向左后⽅延长、弯曲,尖端超过腋中线,包绕部分脾脏;②延长部分肝组织与正常左外叶⾎管相连,与其余肝组织密度相同③左叶间裂位于椎体右缘右侧(提⽰獭尾肝只与左外叶有关,⽽不是整个左肝叶增⼤);④延长部分与左外叶连接处可相对狭窄。
上述4项诊断标准中,①,②,③项为必备条件,④为辅助条件。
鉴别诊断:①獭尾肝为正常变异,其肝组织含有正常的肝、胆管组织,同样可以发⽣肝硬化和各种肝占位。
肝硬化的左叶增⼤⼀般为⼀致性,没有相对狭窄段和向后弯曲,其肝缘圆钝,常有肝右叶相对萎缩、第⼀肝门向右后⽅移位、肝硬化结节等肝硬化其他表现,与獭尾肝不难鉴别。
②陈旧性脾包膜下⾎肿鉴别。
③与脾梗死鉴别。
獭尾肝图⽚男性,24岁,此⼈⽐较瘦低烧1周就诊脾脏包膜下探及新⽉形低回声区,低回声区与脾脏分界⽐较清晰,⽆明显彩⾊⾎流信号。
个⼈感觉这个⾸先考虑獭尾肝不排除其它当时没有仔细区分这个低回声与肝左外叶的关系咳纹肝 (粗⼤膈肌束压迫肝脏)定义咳纹肝粗⼤膈肌束对肝造成的压痕,属膈肌正常解剖变异,粗⼤的膈肌束由于紧贴并陷⼊肝脏表⾯,陷⼊肝表⾯的膈肌束在超声图像上可⾛⾏于肝实质内, 易被误诊为肝内病变.肝右叶的膈⾯因长期咳嗽或束腰等导致膈肌勒压的痕迹,称肝副裂(咳纹肝)。
应与主裂标志、肝内病理性结节相鉴别。
正常解剖解剖肝脏表⾯被覆的薄层纤维结缔组织成为肝被膜(也称为格⾥森被膜)。
研究表明:鱼类身上的某种基因可能拯救失聪者
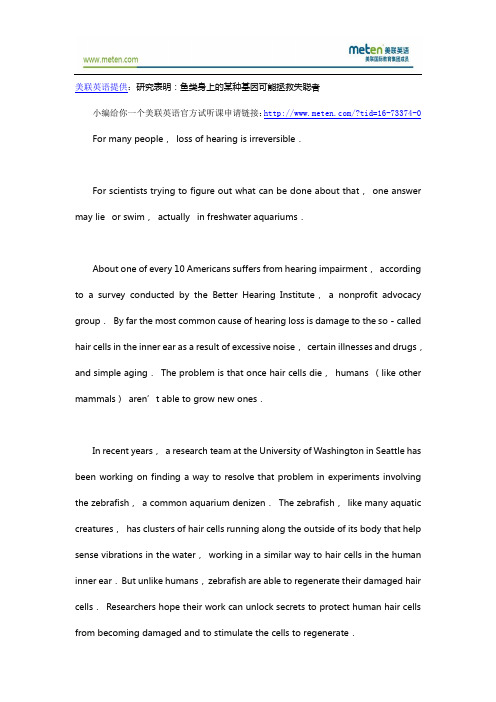
美联英语提供:研究表明:鱼类身上的某种基因可能拯救失聪者小编给你一个美联英语官方试听课申请链接:/?tid=16-73374-0 For many people,loss of hearing is irreversible.For scientists trying to figure out what can be done about that,one answer may lie or swim,actually in freshwater aquariums.About one of every 10 Americans suffers from hearing impairment,according to a survey conducted by the Better Hearing Institute,a nonprofit advocacy group.By far the most common cause of hearing loss is damage to the so-called hair cells in the inner ear as a result of excessive noise,certain illnesses and drugs,and simple aging.The problem is that once hair cells die,humans (like other mammals)aren’t able to grow new ones.In recent years,a research team at the University of Washington in Seattle has been working on finding a way to resolve that problem in experiments involving the zebrafish,a common aquarium denizen.The zebrafish,like many aquatic creatures,has clusters of hair cells running along the outside of its body that help sense vibrations in the water,working in a similar way to hair cells in the human inner ear.But unlike humans,zebrafish are able to regenerate their damaged hair cells.Researchers hope their work can unlock secrets to protect human hair cells from becoming damaged and to stimulate the cells to regenerate.Hair cells,which took their name because under the microscope they look like cells with little hairs growing out of them,are an essential link in hearing.The filament hairs,or cilia,bend with vibrations caused by sound waves entering the ear.That induces the hair cell to create an electrical signal that is passed on to the auditory nerve and sent to the brain.Devices such as hearing aids,which amplify sounds,and cochlear implants,which stimulate the auditory nerve directly,help people hear,but neither restores hearing to normal.Until the mid-1980s,researchers thought warm-blooded vertebrates,including humans,weren’t able to regenerate hair cells.Then,researchers around the country began observing that hair cells grew back in birds whose hearing was damaged either by noise or drugs.They also determined that hair-cell regeneration can result in improved hearing;in experiments,song birds that had grown new hair cells were able to resume singing their original songs with perfect pitch again.But there is no indication that mammals can regenerate hair cells.And why some animals,even within the same species,are more vulnerable to hair-cell death,while others are more resistant to it,is a mystery.’I literally walked around for years wondering about this variability,’says Ed Rubel,a professor of hearing sciences who leads part of the University of Washington research effort.There are two main approaches to efforts aimed at inducing hair cells to regenerate.Some research groups are attempting to get stem cells --undifferentiated cells that can develop into various specialized cells --to turn into hair cells,either by transplanting them from other parts of the body,or by stimulating stem cells naturally occurring in the inner ear to transform themselves.Albert Edge,an associate professor at Harvard Medical School and a researcher at the Massachusetts Eye and Ear Infirmary,says his team has been able to turn mouse stem cells into hair cells in a laboratory dish,though it isn’t clear whether those cells are functional or not.Other researchers,like those at the University of Washington,are focused on understanding the molecules and genetics involved with hair-cell regeneration,and how to mimic this process in animals that don’t spontaneously regenerate hair cells.Scientists say aspects of such research,likely will be the first to have applications in humans.One encouraging angle:Dr.Rubel,in collaboration with another University of Washington scientist,David Raible,has identified chemicals that seem to protect hair cells from damage.In this experiment,zebrafish are exposed to a dye that highlights living hair cells.Then,one or two of the zebrafish --the young ones used in the lab measure just 1/8 of an inch long --are placed in each of 96 shallow holes contained on a plate.Different chemicals are administered to each fish group that might confer protection to thehair cells.Finally,another chemical known to kill the fish hair cells is added.Under a microscope,researchers then examine the fish to look for cases where the dye is still evident,signaling that the cells are still alive and suggesting that the protective chemical appears to have done its job.Those chemicals found to confer protection on fish hair cells are currently also being tested on mice and rats.The idea is that,once a drug is discovered that effectively protects hair cells from dying and is safe for humans,the medicine could be used to help protect the hearing of patients receiving drugs known for killing hair cells,like chemotherapeutic agents.Dr.Rubel’s and Dr.Raible’s teams also are studying the genetics of zebrafish to identify markers that confer hair-cell protection.Last year,their labs jointly identified several genetic mutations and drug-like compounds that seemed to protect hair cells from death,publishing their findings in the journal PLoS Genetics.In a separate study,published in 2007 in Hearing Research,they identified several drugs that also appear to be protective and were already approved for other purposes by the Food and Drug Administration.No tests have been performed on humans,however.The teams also are working on a separate group of studies to understand the genes and other molecules that allow the regeneration of hair cells in zebrafish,birds and mice.Surrounding cells known as support cells can both turn into hair cells or generate new hair cells.Dr.Rubel’s lab is investigating both processes.’If we understand the template of genes that are expressed by the cells we would want to divide,then we could tap into that template’to mimic regeneration efforts in mammals,he says.One finding identified a developmental protein that appears to be turned on in animals able to regenerate hair cells.In one study,a team member found a type of protein increased in a chick (which can regenerate hair cells)after its cells were damaged.But in running the same experiment in a mouse (which can’t regenerate hair cells),the protein didn’t increase,suggesting the protein could be involved in regeneration.Scientists involved in the experiments say there could be therapeutic trials to prevent hearing loss using drugs within a decade.However,finding a cure for hearing loss using hair-cell regeneration is likely to be at least 20 years away,they say.’Hearing aids are Band-aids on a problem that already exists,’says Nancy Freeman,director of the regenerative and development program in hearing loss at the National Institute on Deafness and Other Communication Disorders.’The hope with this type of [regeneration] approach is that at the end of the day you’d end up with something that natively restores function.’对许多人来讲,听力的丧失是一件无法挽回的事。
动物界的“最强大脑”
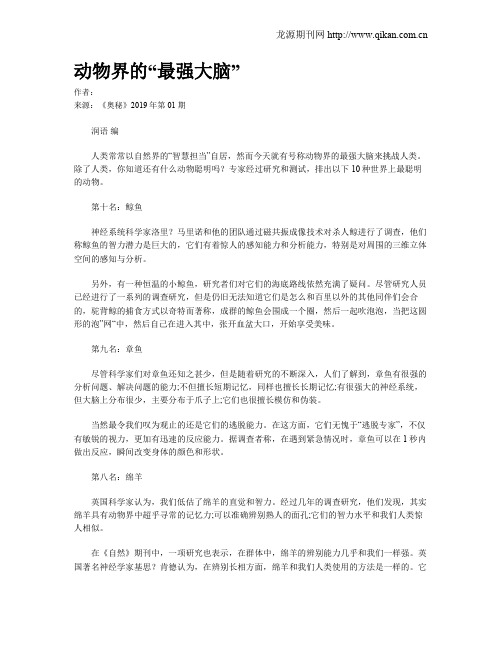
动物界的“最强大脑”作者:来源:《奥秘》2019年第01期润语编人类常常以自然界的“智慧担当”自居,然而今天就有号称动物界的最强大脑来挑战人类。
除了人类,你知道还有什么动物聪明吗?专家经过研究和测试,排出以下10种世界上最聪明的动物。
第十名:鲸鱼神经系统科学家洛里?马里诺和他的团队通过磁共振成像技术对杀人鲸进行了调查,他们称鲸鱼的智力潜力是巨大的,它们有着惊人的感知能力和分析能力,特别是对周围的三维立体空间的感知与分析。
另外,有一种恒温的小鲸鱼,研究者们对它们的海底路线依然充满了疑问。
尽管研究人员已经进行了一系列的调查研究,但是仍旧无法知道它们是怎么和百里以外的其他同伴们会合的,驼背鲸的捕食方式以奇特而著称,成群的鲸鱼会围成一个圈,然后一起吹泡泡,当把这圆形的泡”网“中,然后自己在进入其中,张开血盆大口,开始享受美味。
第九名:章鱼尽管科学家们对章鱼还知之甚少,但是随着研究的不断深入,人们了解到,章鱼有很强的分析问题、解决问题的能力;不但擅长短期记忆,同样也擅长长期记忆;有很强大的神经系统,但大脑上分布很少,主要分布于爪子上;它们也很擅长模仿和伪装。
当然最令我们叹为观止的还是它们的逃脱能力。
在这方面,它们无愧于“逃脱专家”,不仅有敏锐的视力,更加有迅速的反应能力。
据调查者称,在遇到紧急情况时,章鱼可以在1秒内做出反应,瞬间改变身体的颜色和形状。
第八名:绵羊英国科学家认为,我们低估了绵羊的直觉和智力。
经过几年的调查研究,他们发现,其实绵羊具有动物界中超乎寻常的记忆力;可以准确辨别熟人的面孔;它们的智力水平和我们人类惊人相似。
在《自然》期刊中,一项研究也表示,在群体中,绵羊的辨别能力几乎和我们一样强。
英国著名神经学家基思?肯德认为,在辨别长相方面,绵羊和我们人类使用的方法是一样的。
它们的世界也是一个充满阶段的社会,它们甚至也会通过其它羊的长相来确定伴侣和朋友(友谊大概会持续几周时间)。
原来,有审美感的不仅仅是我们人类。
世界上大脑最大的动物
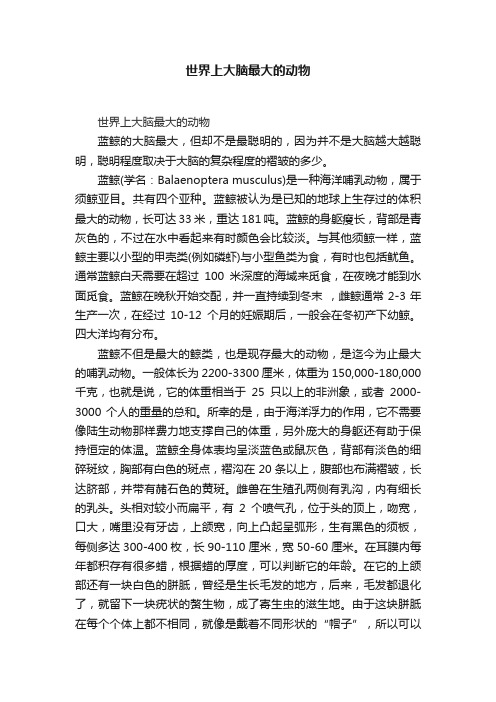
世界上大脑最大的动物世界上大脑最大的动物蓝鲸的大脑最大,但却不是最聪明的,因为并不是大脑越大越聪明,聪明程度取决于大脑的复杂程度的褶皱的多少。
蓝鲸(学名:Balaenoptera musculus)是一种海洋哺乳动物,属于须鲸亚目。
共有四个亚种。
蓝鲸被认为是已知的地球上生存过的体积最大的动物,长可达33米,重达181吨。
蓝鲸的身躯瘦长,背部是青灰色的,不过在水中看起来有时颜色会比较淡。
与其他须鲸一样,蓝鲸主要以小型的甲壳类(例如磷虾)与小型鱼类为食,有时也包括鱿鱼。
通常蓝鲸白天需要在超过100米深度的海域来觅食,在夜晚才能到水面觅食。
蓝鲸在晚秋开始交配,并一直持续到冬末,雌鲸通常2-3年生产一次,在经过10-12个月的妊娠期后,一般会在冬初产下幼鲸。
四大洋均有分布。
蓝鲸不但是最大的鲸类,也是现存最大的动物,是迄今为止最大的哺乳动物。
一般体长为2200-3300厘米,体重为150,000-180,000千克,也就是说,它的体重相当于25只以上的非洲象,或者2000-3000个人的重量的总和。
所幸的是,由于海洋浮力的作用,它不需要像陆生动物那样费力地支撑自己的体重,另外庞大的身躯还有助于保持恒定的体温。
蓝鲸全身体表均呈淡蓝色或鼠灰色,背部有淡色的细碎斑纹,胸部有白色的斑点,褶沟在20条以上,腹部也布满褶皱,长达脐部,并带有赭石色的黄斑。
雌兽在生殖孔两侧有乳沟,内有细长的乳头。
头相对较小而扁平,有2个喷气孔,位于头的顶上,吻宽,口大,嘴里没有牙齿,上颌宽,向上凸起呈弧形,生有黑色的须板,每侧多达300-400枚,长90-110厘米,宽50-60厘米。
在耳膜内每年都积存有很多蜡,根据蜡的厚度,可以判断它的年龄。
在它的上颌部还有一块白色的胼胝,曾经是生长毛发的地方,后来,毛发都退化了,就留下一块疣状的赘生物,成了寄生虫的滋生地。
由于这块胼胝在每个个体上都不相同,就像是戴着不同形状的“帽子”,所以可以据此区分不同的个体。
211103631_海水养殖鱼类病毒性神经坏死病流行特点及综合防治措施
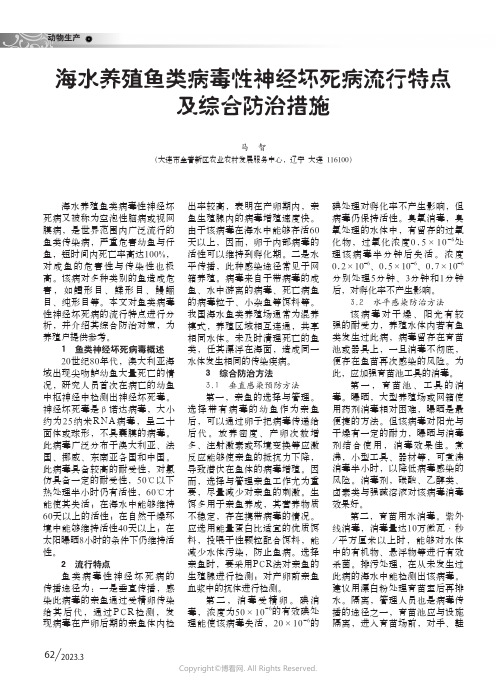
动物生产 622023.3海水养殖鱼类病毒性神经坏死病流行特点及综合防治措施马 智(大连市金普新区农业农村发展服务中心,辽宁 大连 116100)海水养殖鱼类病毒性神经坏死病又被称为空泡性脑病或视网膜病,是世界范围内广泛流行的鱼类传染病,严重危害幼鱼与仔鱼,短时间内死亡率高达100%,对成鱼的危害性与传染性也极高。
该病对多种类别的鱼造成危害,如鳕形目、鲽形目、鳗鲡目、纯形目等。
本文对鱼类病毒性神经坏死病的流行特点进行分析,并介绍其综合防治对策,为养殖户提供参考。
1 鱼类神经坏死病毒概述20世纪80年代,澳大利亚海域出现尖吻鲈幼鱼大量死亡的情况,研究人员首次在病亡的幼鱼中枢神经中检测出神经坏死毒。
神经坏死毒是β诺达病毒,大小约为25纳米RNA病毒,呈二十面体或球形,不具囊膜的病毒。
此病毒广泛分布于澳大利亚、法国、挪威、东南亚各国和中国。
此病毒具备较高的耐受性,对氯仿具备一定的耐受性,50℃以下热处理半小时仍有活性,60℃才能使其失活;在海水中能够维持60天以上的活性;在自然干燥环境中能够维持活性40天以上;在太阳曝晒8小时的条件下仍维持活性。
2 流行特点鱼类病毒性神经坏死病的传播途径为:一是垂直传播,感染此病毒的亲鱼通过受精卵传染给其后代,通过P CR 检测,发现病毒在产卵后期的亲鱼体内检出率较高,表明在产卵期内,亲鱼生殖腺内的病毒增殖速度快。
由于该病毒在海水中能够存活60天以上,因而,卵子内部病毒的活性可以维持到孵化期。
二是水平传播,此种感染途径常见于网箱养殖。
病毒来自于带病毒的成鱼、水中游离的病毒、死亡病鱼的病毒粒子、小杂鱼等饵料等。
我国海水鱼类养殖场通常为混养模式,养殖区域相互连通,共享相同水体。
未及时清理死亡的鱼类,任其漂浮在海面,造成同一水体发生相同的传染疾病。
3 综合防治方法3.1 垂直感染预防方法第一,亲鱼的选择与管理。
选择带有病毒的幼鱼作为亲鱼后,可以通过卵子把病毒传递给后代。
放养密度、产卵次数增多、注射激素或环境变换等应激反应能够使亲鱼的抵抗力下降,导致潜伏在鱼体的病毒增殖。
十三种令人惊异的动物大脑水蛭有32个大脑!!

大脑是机体最为复杂、神秘的器官。
正是因为其神秘,才显得有趣,才引得那么多的科学家,去探索。
下面就是科学家们发现的13中令人惊异的动物大脑。
海鞘吃自己的大脑海鞘是两性动物,产生蝌蚪状的幼虫,分散到各处,寻找栖息之所。
海鞘在幼体阶段,与鱼类、鸟类、爬行动物和哺乳动物有相同的解剖特点,但随着它们的成长,它们失去了自己的思维(从字面上)。
它们消化自己控制运动的脑神经节,因为它们不再需要它了。
巨型乌贼通过大脑进食巨型乌贼进食时不得不将食物咬成小块,因为它们每吞咽一次,食物必须通过其环形的大脑,然后进入食管。
对于这样一个大型的动物,巨型乌贼的大脑是出奇的小。
新西兰奥克兰科技大学乌贼专家史蒂夫·奥谢(Steve O)查理王小猎犬的头骨变得过小,无法承受大脑的重量它可能患有名叫“骨髓空洞症(syringomyelia)”的遗传疾病,即这种动物的头骨变得过小,无法承受大脑的重量。
用神经学家Claire Rusbridge的话说,这就好像“10英尺的脚要塞进6英尺的鞋中一样”。
三分之一的查理王小猎犬都受着这种遗传病的煎熬。
海豚的大脑比人类的大你知道吗?海豚的大脑实际上比我们人类的还大。
瓶鼻海豚(bottlenose dolphins)等鲸类动物有能力识别、记忆和解决问题,使它们正式成为在动物王国中智力最接近人类的动物。
海豚的大脑皮层比人类的更为复杂。
美国埃默里大学的海豚专家洛里·马里诺(Lori Marino)说,“按神经组织分析,海豚与人类都拥有心理状况。
研究显示海豚,尤其瓶鼻海豚的智商甚高,它们拥有独特的个性,拥有自我的观念,甚至为将来做打算!”蚂蚁的大脑被寄生真菌操控在这个例子里,我们不是要讲蚂蚁的大脑有多么特别,而是讲它对一些种类的寄生真菌如何做出反应。
冬虫夏草(Cordyceps)真菌感染蚂蚁,并把它们变成行尸走肉,操纵它们的行为,以驱散它们的孢子和繁殖能力。
有点像一场噩梦,冬虫夏草真菌以蚂蚁的非重要器官为食,其细丝成长为蚂蚁的大脑,促使蚂蚁爬上附近植物的顶部,然后,真菌杀死蚂蚁,以蘑菇的形式从它的头部发芽。
实验室新明星——斑马鱼
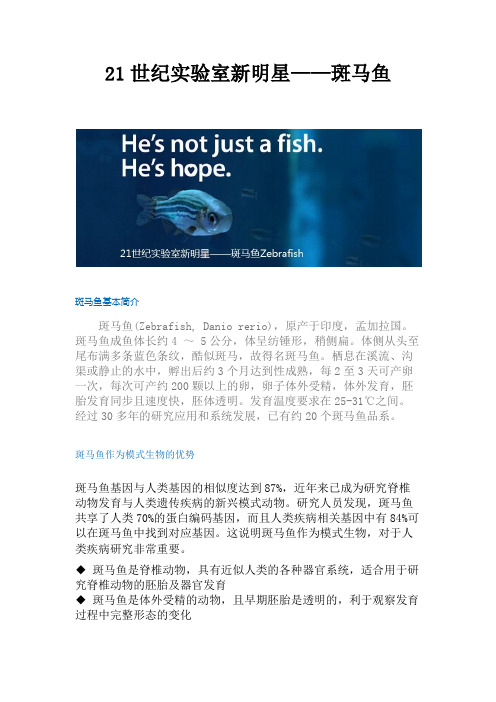
21世纪实验室新明星——斑马鱼斑马鱼基本简介斑马鱼(Zebrafish, Danio rerio),原产于印度,孟加拉国。
斑马鱼成鱼体长约4 ~ 5公分,体呈纺锤形,稍侧扁。
体侧从头至尾布满多条蓝色条纹,酷似斑马,故得名斑马鱼。
栖息在溪流、沟渠或静止的水中,孵出后约3个月达到性成熟,每2至3天可产卵一次,每次可产约200颗以上的卵,卵子体外受精,体外发育,胚胎发育同步且速度快,胚体透明。
发育温度要求在25-31℃之间。
经过30多年的研究应用和系统发展,已有约20个斑马鱼品系。
斑马鱼作为模式生物的优势斑马鱼基因与人类基因的相似度达到87%,近年来已成为研究脊椎动物发育与人类遗传疾病的新兴模式动物。
研究人员发现,斑马鱼共享了人类70%的蛋白编码基因,而且人类疾病相关基因中有84%可以在斑马鱼中找到对应基因。
这说明斑马鱼作为模式生物,对于人类疾病研究非常重要。
◆斑马鱼是脊椎动物,具有近似人类的各种器官系统,适合用于研究脊椎动物的胚胎及器官发育◆斑马鱼是体外受精的动物,且早期胚胎是透明的,利于观察发育过程中完整形态的变化◆斑马鱼养殖设备比老鼠简单,且花费也低◆斑马鱼成熟快,且繁殖力强,利于遗传学之研究◆斑马鱼可以很容易进行诱发突变及基因转植,利于研究基因功能斑马鱼在基因研究中的应用今年早前,英国桑格研究所完成了斑马鱼的参考基因组,并比较了斑马鱼与人类基因组的异同,进行了系统性的全基因组分析。
研究人员发现,斑马鱼共享了人类70%的蛋白编码基因,且人类疾病相关基因中有84%可以在斑马鱼中找到对应基因。
斑马鱼作为脊椎动物可以用来进行大规模的饱和突变,提供丰富的遗传资源,为人类疾病研究和药物研发开辟新的道路。
近年应用于斑马鱼研究的热门基因组编辑技术(genome editing):■锌指核酸酶锌指核酸酶(ZFNs)不是自然存在的,而是一种人工改造的核酸内切酶,由一个 DNA 识别域和一个非特异性核酸内切酶构成,其中DNA识别域赋予特异性,在DNA特定位点结合,而非特异性核酸内切酶赋剪切功能,两者结合就可在DNA特定位点进行定点断裂。
- 1、下载文档前请自行甄别文档内容的完整性,平台不提供额外的编辑、内容补充、找答案等附加服务。
- 2、"仅部分预览"的文档,不可在线预览部分如存在完整性等问题,可反馈申请退款(可完整预览的文档不适用该条件!)。
- 3、如文档侵犯您的权益,请联系客服反馈,我们会尽快为您处理(人工客服工作时间:9:00-18:30)。
TAURINE PREVENTS ENHANCEMENT OF ACETYLCHOLINESTERASE ACTIVITY INDUCED BY ACUTE ETHANOL EXPOSURE AND DECREASES THE LEVEL OF MARKERS OF OXIDATIVE STRESS IN ZEBRAFISH BRAIND.B.ROSEMBERG,a,b*R.F.DA ROCHA,a,cE.P.RICO,a,b A.ZANOTTO-FILHO,a,c R.D.DIAS,dM.R.BOGO,e,f C.D.BONAN,d,f J.C.F.MOREIRA,a,cF.KLAMT a,c,f AND D.O.SOUZA a,ba Programa de Pós-graduação em Bioquímica,Departamento de Bio-química,Instituto de Ciências Básicas da Saúde,Universidade Fed-eral do Rio Grande do Sul.Rua Ramiro Barcelos2600-Anexo,90035-003,Porto Alegre,RS,Brazilb Instituto Nacional de Ciência e Tecnologia em Excitotoxicidade e Neuroproteção(INCT-EN)90035-003,Porto Alegre,RS,Brazilc Centro de Estudos em Estresse Oxidativo(Lab.32),Departamento de Bioquímica,ICBS,Universidade Federal do Rio Grande do Sul,Av. Ramiro Barcelos,2600-Anexo,CEP90035-003,Porto Alegre,RS, Brazild Laboratório de Neuroquímica e Psicofarmacologia,Departamento de Biologia Celular e Molecular,Faculdade de Biociências,Pontifícia Universidade Católica do Rio Grande do Sul.Avenida Ipiranga,6681, 90619-900,Porto Alegre,RS,Brazile Laboratório de Biologia Genômica e Molecular,Departamento de Biologia Celular e Molecular,Faculdade de Biociências,Pontifícia Universidade Católica do Rio Grande do Sul.Avenida Ipiranga,6681, 90619-900,Porto Alegre,RS,Brazilf Instituto Nacional Translacional em Medicina(INCT-TM),90035-003, Porto Alegre,RS,BrazilAbstract—Ethanol(EtOH)is a drug widely consumed throughout the world that promotes several neurochemical disorders.Its deleterious effects are generally associated with modifications in oxidative stress parameters,signaling transduction pathways,and neurotransmitter systems,lead-ing to distinct behavioral changes.Taurine(2-aminoethane-sulfonic acid)is a-amino acid not incorporated into pro-teins found in mM range in the central nervous system(CNS). The actions of taurine as an inhibitory neurotransmitter,neu-romodulator,and antioxidant make it attractive for studying a potential protective role against EtOH-mediated neurotoxic-ity.In this study,we investigated whether acute taurine cotreatment or pretreatment(1h)prevent EtOH-induced changes in acetylcholinesterase(AChE)activity and in oxi-dative stress parameters in zebrafish brain.The results showed that EtOH exposure(1%in volume)during1h in-creased AChE activity,whereas the cotreatment with400mg·L؊1taurine prevented this enhancement.A similar pro-tective effect of150and400mg·L؊1taurine was also ob-served when the animals were pretreated with this amino acid.Taurine treatments also prevented the alterations pro-moted in superoxide dismutase and catalase activities by EtOH,suggesting a modulatory role in enzymatic antioxidant defenses.The pretreatment with150and400mg·L؊1taurine significantly increased the sulfydryl levels as compared to control and EtOH groups.Moreover,150and400mg·L؊1 taurine significantly decreased thiobarbituric acid reactive species(TBARS)levels,but the cotreatment with EtOH plus 400mg·L؊1taurine did not prevent the EtOH-induced lipoper-oxidation.In contrast,the pretreatment with150and400 mg·L؊1taurine prevented the TBARS increase besides de-creased the basal levels of lipid peroxides.Altogether,our data showed for thefirst time that EtOH induced oxidative stress in adult zebrafish brain and reinforce the idea that this vertebrate is an attractive alternative model to evaluate the beneficial effect of taurine against acute EtOH exposure.©2010IBRO.Published by Elsevier Ltd.All rights reserved. Key words:taurine,ethanol,acetylcholinesterase,oxidative stress parameters,zebrafish,brain.The zebrafish(Danio rerio)is emerging as a promising model organism for experimental studies in different bio-medical areas.As a relatively simple vertebrate species, zebrafish is an ideal animal model for laboratory research because they are inexpensive,low-maintenance,and abundantly produce offspring(Gerlai et al.,2006;Egan et al.,2009).Because zebrafish genes are highly conserved sharing a70–80%homology to those of humans(Barba-zuk et al.,2000),it is a tempting vertebrate model for modeling behavioral and functional parameters related to human pathogenesis and for clinical treatments screening, including alcohol abuse and therapeutic strategies.Ethanol(EtOH)is a drug widely consumed throughout the world.Alcoholic consumption is linked to the occur-rence of several pathological conditions such as various forms of cancer,liver failure,brain damage,and fetal inju-ries(Quertemont et al.,2005;Dalitz et al.,2008).The cerebral effects of acute EtOH exposure in central nervous system(CNS)lead to an impairment of motor coordination, sensory perception and cognition,which can be correlated to oxidative stress and modifications of neurotransmitter systems and intricate signaling pathways(Hanchar et al., 2005;Belmeguenai et al.,2008).Acetylcholine(ACh)is a neurotransmitter that elicits its effects through nicotinic and muscarinic receptors.In the extracellular space,acetylcholinesterase(EC 3.1.1.7;*Correspondence to:D.B.Rosemberg,Departamento de Bioquímica,Instituto de Ciências Básicas da Saúde,Universidade Federal do RioGrande do Sul.Rua Ramiro Barcelos2600-Anexo,90035-003,PortoAlegre,RS,Brazil.Tel:ϩ55-51-3308-5557;fax:ϩ55-51-3308-5540.E-mail address:dbrosemberg@(D.B.Rosemberg).Abbreviations:ACh,acetylcholine;AChE,acetylcholinesterase;CAT,catalase;CNS,central nervous system;DTNB,5,5=-dithionitrobis2-ni-trobenzoic acid;EtOH,ethanol;GSH,reduced glutathione;ROS,re-active oxygen species;SOD,superoxide dismutase;TAR,total anti-oxidant reactivity;TBARS,thiobarbituric acid reactive species;TRAP,total antioxidant potential.Neuroscience171(2010)683–6920306-4522/10$-see front matter©2010IBRO.Published by Elsevier Ltd.All rights reserved. doi:10.1016/j.neuroscience.2010.09.030683AChE)is the enzyme responsible for terminating the cho-linergic transmission by degradation of ACh into choline and acetate.Since the cholinergic system can also be involved in the modulation of behavioral and cognitive functions(Sarter and Bruno,2004;Furey et al.,2008) the levels of ACh in extracellular milieu must be tightly regulated and the screening for molecules able to mod-ulate directly and/or indirectly AChE activity could be a pharmacological strategy to lead the maintenance of brain homeostasis.Taurine(2-aminoethanesulfonic acid)is a simple sul-fur-containing-amino acid,which is not incorporated into proteins and is found free in virtually all animal cells.In particular,high concentrations of taurine are detected in electrically excitable tissues such as brain,retina,heart, and skeletal muscles(Huxtable,1992;Saransaari and Oja, 2000;Oja and Saransaari,2007).In the CNS,taurine plays a critical role for brain function,being implicated in cell volume regulation and also in neuromodulation or inhibi-tory neurotransmission(Banerjee et al.,2008).In certain tissues such as brain,intracellular taurine concentrations can range up to50mM,whereas extracellular concentra-tions are in the micromolar range(Huxtable,1992).The intracellular taurine accumulation results primarily from up-take by an efficient transport system(TauT protein)in the plasma membrane which utilizes transmembrane gradi-ents of Naϩand ClϪas the driving force,as well from intracellular biosynthesis of taurine(Kozlowski et al.,2008; Kang,2009).Several roles of taurine have been reported, including its trophic actions during the CNS development; antioxidant functions,the ability for modifying protein phos-phorylation,maintenance of calcium homeostasis,and membrane integrity(Wu et al.,2005;Oliveira et al.,2010; Junyent et al.,2010).Although the mechanisms involved in taurine actions still remains poorly understood,it is con-ceivable that its extracellular effects are mediated by open-ing the chloride channels through the interaction with GABAAreceptors,glycine receptors,or putative taurine receptors(Albrecht and Schousboe,2005).Due to its bio-chemical properties,taurine interacts with other transmitter systems and acts as a neuroprotector against various types of injury,including alcohol abuse(Oja and Saran-saari,2007;Chen et al.,2009).The teratogenic properties of EtOH have been previ-ously established in zebrafish(Dlugos and Rabin,2003; Reimers et al.,2006).It has been shown that EtOH mod-ulates distinct behavioral parameters in this species such as swimming activity,aggression,group preference,and pigment response,possibly through alterations in neuro-transmitter systems and in cell signaling cascades(Gerlai et al.,2000;Rico et al.,2007;Peng et al.,2009).Concern-ing the cholinergic signaling,zebrafish presents a unique situation among vertebrates because its genome does not encode a functional butyrylcholinesterase,being AChE the only ACh-hydrolyzing enzyme in this organism(Behra et al.,2004).It was reported that brain AChE activity in ze-brafish is altered after acute EtOH exposure,suggesting an involvement of cholinergic parameters on EtOH-medi-ated responses(Rico et al.,2007).Moreover,previous study characterized the expression profile of TauT in ze-brafish during embryogenesis,showing a high degree of homology to mammalian taurine transporter(Kozlowski et al.,2008).Although it has been previously reported that the EtOH-mediated toxicity in zebrafish embryos can be partially attenuated by antioxidants(Reimers et al.,2006), little is known about the effects of EtOH and taurine on the brain function of adult zebrafish.In this context,the influ-ence of acute EtOH exposure on oxidative stress param-eters and the beneficial actions of taurine in this species still remain obscure.Therefore,in the present study we focused for thefirst time our attention on the potential neu-roprotective effects of taurine in zebrafish brain.First,we evaluated whether acute cotreatment or pretreatment with taurine prevent EtOH-induced enhancement of AChE activ-ity.In addition,the effect of acute EtOH exposure on oxidative stress parameters and the influence of both taurine treat-ments on neurochemical redox profile were also studied.EXPERIMENTAL PROCEDURESAnimalsAdult males and females of the“wild type”(shortfin—SF)ze-brafish(Danio rerio)strain(3–6months-old,weighing0.43Ϯ0.07 g)were obtained from a commercial supplier(Delphis,RS,Brazil) and acclimated for at least2weeks in50-L thermostated aquar-ium before the experiments.All tanks werefilled with unchlori-nated water previously treated with132L.LϪ1AquaSafe®(Tetra,USA)and kept under mechanical and chemicalfiltration at a targeted temperature of26Ϯ2°C and water pH and conductivity at7.0–8.0and1,500–1,600S.cmϪ1,respectively.Illumination was provided by ceiling-mountedfluorescent light tubes on a12-h light-dark photoperiod(on7:00h;off19:00h).Fish were fed twice a day to satiety with commercial alcon BASIC®(Alcon,Brazil)as flakefish food.For the experiments,fish were euthanized by decapitation and the brains were quickly dissected.Each inde-pendent experiment was performed using biological preparations from a pool of three animals for AChE experiments and10animals for oxidative stress evaluation.All animals used were naive being healthy and free of any signs of disease and maintained in accor-dance to the National Institute of Health Guide for Care and Use of Laboratory Animals.The protocols were approved by the Eth-ical Committee of the Federal University of Rio Grande do Sul under the number2007950.ChemicalsEthanol(C2H6O;CAS number64-17-5)was purchased from Merck(Darmstadt,Germany).All other reagents used were pur-chased from Sigma(St.Louis,MO,USA).Experimental designFor acute EtOH exposure,fish were placed in3-L aquarium and kept in a solution of1%EtOH in volume during1h.The same time of exposure and EtOH concentration have been successfully tested in adult zebrafish(Gerlai et al.,2000;Dlugos and Rabin, 2003;Rico et al.,2007)leading to alterations in behavioral and cholinergic signaling parameters of this species.In addition,the blood alcohol levels achieved with it are expected to be in the range seen in the human clinic after mild to moderate acute alcohol consumption(Gerlai et al.,2008).Acute taurine treat-ments were performed using distinct concentrations(42,150,and 400mg·LϪ1)during1h,the same time used for EtOH exposure. Taurine chosen concentrations correspond to a range previouslyD.B.Rosemberg et al./Neuroscience171(2010)683–692 684used in other studies related to taurine effects on several bio-chemical parameters,which vary from0.33to3.2mM(Wu et al., 2005;Kong et al.,2006;Rosemberg et al.,2010b).Two treatment protocols with taurine were performed:(i)concomitant treatment with EtOH;and(ii)a pretreatment during1h before EtOH expo-sure.Importantly,the acute treatments procedure(e.g.,the origin and quality of the system water and the timing of taurine delivery, etc.)was identical for allfish.Different experimental groups were analyzed:(1)control(1h into water);(2)EtOH-exposed during 1h;(3)taurine-treated during1h;(4)concomitant taurine treat-ment with EtOH during1h(cotreatment);(5)1h into water before transference to another aquariaϩ1h into water(pretreatment—control group unexposed to taurine);(6)1h into water before transference to another aquariaϩEtOH exposure during1h(pre-treatment—EtOH group unexposed to taurine);(7)taurine-treated during1h before transference to another aquariaϩEtOH expo-sure during1h(pretreatment—experimental group);and(8)tau-rine-treated during1h before transference to another aquariaϩ1 h into water(pretreatment—control group treated with taurine). Determination of AChE activityThe brains were homogenized on ice in60volumes(v/w)of Tris–citrate buffer(50mM Tris,2mM EDTA,2mM EGTA,pH7.4, with citric acid)using a Potter–Elvehjen-type glass homogenizer. The rate of hydrolysis of acetylthiocholine iodide(0.88mM)was determined in afinal volume of300L,with33L of100mM phosphate buffer,pH7.5mixed to 2.0mM5,5=-dithionitrobis 2-nitrobenzoic acid(DTNB).Samples containing5g protein and the reaction medium specified above were preincubated for10 min at25°C.The hydrolysis of acetylthiocholine iodide was mon-itored in a microplate reader by the formation of thiolate dianion of DTNB at412nm for2–3min(intervals of30s)(Ellman et al., 1961).Controls without the homogenate preparation were per-formed in order to determine the non-enzymatic hydrolysis of the substrate.AChE activity was expressed asmol thiocholine (SCh).hϪ1.mg proteinϪ1.All experiments were performed in quadruplicate.Oxidative stress analysesZebrafish brains were dissected out in ice immediately after the fish were euthanized and homogenized in 1.0mL phosphate buffer saline(PBS)pH7.4,containing in mM:137NaCl,10.1Na2HPO4,and1.76KH2PO4.The homogenates were centrifuged(700ϫg,5min)to remove cellular debris.Supernatants were collected and used to all biochemical assays described herein. Antioxidant enzyme activities determination Superoxide dismutase(EC1.15.1.1,SOD)activity was assessed by quantifying the inhibition of superoxide-dependent adrenaline auto-oxidation at480nm(Misra and Fridovich,1972),and the results were expressed as Units SOD.mg proteinϪ1.Catalase (EC1.11.1.6;CAT)activity was assessed by measuring the rate ofdecrease in H2O2absorbance at240nm(Aebi,1984),and theresults were expressed as Units CAT.mg proteinϪ1.For SOD assay,protein amounts ranged from15–60g,while CAT activity was determined using30–50g protein.To better understand the effect promoted by the treatments upon these two oxidant-detox-ifying enzymes that work in sequence converting superoxide an-ion to water,a ratio SOD/CAT activities was calculated.An imbal-ance between their activities is thought to facilitate oxidative-dependent alterations in the cellular environment,which could culminate in oxidative stress.Non-enzymatic antioxidant defensesThe non-enzymatic antioxidant potential of zebrafish brains was estimated by the total antioxidant potential(TRAP)and total an-tioxidant reactivity(TAR)(Lissi et al.,1995).The reaction wasinitiated by adding luminol(5-Amino-2,3-dihydro-1,4-phthala-zinedione,4mM)—an external probe to monitoring radical pro-duction—and AAPH(2,2=-azobis[2-methylpropionamidine]dihy-drochloride,10mM)—a free radical source that produces peroxylradical at a constant rate—in glycine buffer(0.1M)pH8.6at roomtemperature,resulting in a steady luminescence emission(systemcounts).Chemiluminescence was read in a liquid scintillationcounter(Wallace,1409)as counts per minutes.Sample additiondecreases the luminescence proportionately to its antioxidant po-tential.The luminescence emission was followed for40min afterthe addition of the sample(10g protein)in a TRAP protocol,andthe area under the curve was quantified.In the TAR protocol,results were calculated as percentage of radical production(sys-tem counts considered as100%of radical production).Total reduced thiol contentOxidative alterations in proteins can be evaluated by the level ofprotein thiol content in samples.Briefly,samples(40g protein) were mixed to35L of0.2mM EDTA,100mM boric acid buffer, pH8.5.DTNB(0.01M dissolved in ethanol)was added and theintense yellow color developed was measured at412nm after1h(Ellman,1959).Total reduced sulfydryl content was estimated in afinal volume of210L and the results were expressed asmol SH.mg proteinϪ1.Thiobarbituric acid reactive species(TBARS)The formation of TBARS during an acid-heating reaction,which iswidely performed for measurement of lipid redox state(Draperand Hadley,1990),was used as an index of lipid peroxidation.Briefly,300L of samples(80–100g protein)were mixed with 600L of15%trichloroacetic acid(TCA)and centrifuged (10.000ϫg,10min).Supernatants(100L)were mixed with100L of0.67%thiobarbituric acid(TBA,4,6-Dihydroxypyrimidine-2-thiol)and heated at100°C for30min.TBARS levels weredetermined by the absorbance at532nm using1,1,3,3-tetrame-thoxypropane(TMP)as standard.Results were expressed asnmol TBARS.mg proteinϪ1.Protein quantificationFor AChE activity assays,the protein was measured by the Coo-massie Blue method(Bradford,1976).For oxidative stress anal-yses,the protein concentration was determined according toPeterson(1977).Bovine serum albumin was used as a proteinstandard.StatisticsData were expressed as meanϮstandard error of the mean(SEM)and P-values were considered significant for PՅ0.05.Differenceswithin the experimental groups were determined by one or two-wayanalysis of variance(ANOVA).Comparison among means was car-ried out using Bonferroni’s multiple comparison test as post hoc.Allexperiments were performed at least in triplicate.RESULTSTaurine prevents alterations in AChE activity promoted by acute EtOH exposureAs previously reported by our group(Rico et al.,2007),we demonstrate that acute EtOH exposure(1%in volume) increased AChE activity in zebrafish brain(37%,nϭ6) when compared to control group(Fig.1).When the ani-mals were treated concomitantly with taurine(42and150D.B.Rosemberg et al./Neuroscience171(2010)683–692685mg·L Ϫ1)and EtOH,the AChE activity did not change in comparison to EtOH group.However,taurine cotreatment at 400mg·L Ϫ1prevented zebrafish brain from EtOH-in-duced enhancement of AChE activity (n ϭ6)(Fig.1A).Next,we evaluated the effect of taurine pretreatment on EtOH-induced AChE stimulation (Fig.1B).A two-way ANOVA revealed a significant effect of taurine pretreat-ment ϫEtOH exposure interaction (F (3,32)ϭ3.63,P Ͻ0.05).Post hoc analyses indicated that pretreatment with 150and 400mg·L Ϫ1taurine prevented the effects promoted by EtOH in AChE activity.To verify whether taurine alters AChE activity,the an-imals were treated with the same concentrations tested (42,150,and 400mg·L Ϫ1).The results demonstrated that AChE activity remained similar to control group (n ϭ4).Taurine prevents changes in antioxidant enzyme activities induced by EtOHSOD and CAT play a key role in the control of reactive oxygen species (ROS)levels and in the prevention of oxidative damage.Acute exposure to EtOH significantly decreased SOD activity (49%,n ϭ3)(Fig.2)and concom-itantly increased CAT activity (89%,n ϭ4)(Fig.3).The cotreatment with 400mg·L Ϫ1taurine prevented the EtOH-induced changes in SOD (Fig.2A)and CAT activities (Fig.3A)(n ϭ4).The influence of taurine pretreatment on the effects of EtOH in SOD (Fig.2B)and CAT activities (Fig.3B)was also evaluated.A two-way ANOVA revealed a significant effect of taurine pretreatment (F (2,28)ϭ6.15,P Ͻ0.05)and EtOH exposure (F (1,28)ϭ4.5,P Ͻ0.05).Post hoc analyses indicated that pretreatment with 150and 400mg·L Ϫ1tau-rine prevented the EtOH-induced decrease in SOD activ-ity.In relation to CAT activity,a two-way ANOVA revealed a significant effect of taurine pretreatment ϫEtOH expo-sure interaction (F (2,19)ϭ10.37,P Ͻ0.05)and EtOH expo-sure (F (1,19)ϭ8.79,P Ͻ0.05).Post hoc analyses indicated that pretreatment with 150and 400mg·L Ϫ1taurine also prevented the EtOH-induced increase in CAT activity.Both taurine concentrations per se did not significantly alter SOD and CAT activities in zebrafish brain.As depicted in Fig.4,EtOH induced an imbalance in the SOD/CAT ratio (n ϭ4;P Ͻ0.05).Both cotreatment (400mg·L Ϫ1)(Fig.4A)and pretreatment (150and 400mg·L Ϫ1)(Fig.4B)with taurine reversed SOD/CAT ratio to the basal level (n ϭ5).Non-enzymatic antioxidant defenses are altered neither by EtOH exposure nor taurine treatments The effects promoted by EtOH,taurine and both cotreat-ment and pretreatment on non-enzymaticantioxidantFig.2.Effect of EtOH and taurine treatments in SOD activity.(A)Cotreatment with taurine and EtOH.The groups were represented as control (CRL),ethanol (EtOH),taurine-treated (TAU)and taurine-co-treated animals (COT).Data represent mean ϮSEM of at least three independent experiments.*Significant difference compared to control (one-way ANOVA followed by Bonferroni’s test as post hoc,P Յ0.05).(B)Pretreatment with taurine followed by water (control group)or EtOH exposure.The treatments were represented as taurine-un-treated (UNT)and taurine-treated (TAU).Data represent mean ϮSEM of at least three independent experiments.*Significant difference compared to UNT from control group.#Significant difference com-pared to UNT from EtOH group (two-way ANOVA followed by Bonfer-roni’s test as post hoc,P Յ0.05).Fig.1.Effect of taurine on the EtOH-induced enhancement of AChE activity after acute exposure.(A)Cotreatment with taurine and EtOH.The groups were represented as control (CRL),ethanol (EtOH),tau-rine-cotreated animals.Data represent mean ϮSEM of at least three independent experiments.*Significant difference compared to control (one-way ANOVA followed by Bonferroni’s test as post hoc,P Յ0.05).(B)Pretreatment with taurine followed by water (control group)or EtOH exposure.The treatments were represented as taurine-un-treated (UNT)and taurine-treated (TAU).Data represent mean ϮSEM of at least three independent experiments.*Significant difference compared to UNT from control group.#Significant difference com-pared to UNT from EtOH group (two-way ANOVA followed by Bonfer-roni’s test as post hoc,P Յ0.05).D.B.Rosemberg et al./Neuroscience 171(2010)683–692686defenses were also evaluated.Neither EtOH exposure nor taurine treatments significantly changed TRAP (ac-cording to decreased radical production during TRAP experiment)and TAR in zebrafish brain (n ϭ3)(data not shown).Effect of taurine treatments on total reduced thiol contentEtOH,at the concentration and time of exposure tested,did not induce any significant changes in the total reduced thiol content of zebrafish brain (Fig.5).Similarly,150and 400mg·L Ϫ1taurine did not alter total thiol content.Fur-thermore,the cotreatment with 400mg·L Ϫ1taurine did not significantly change the basal total reduced thiol content as compared to control and EtOH-treated groups (n ϭ4)(Fig.5A).However,a two-way ANOVA revealed a significant effect of EtOH exposure (F (1,20)ϭ12.47,P Ͻ0.05)and taurine pretreatment (F (2,20)ϭ5.27,P Ͻ0.05).Post hoc analyses indicated that pretreatment with 150and 400mg·L Ϫ1taurine followed by EtOH exposure induced a sig-nificant increase in total reduced sulfydryl content (52%and 64%,respectively,n ϭ4)as compared to control and EtOH-treated groups (n ϭ5)(Fig.5B).Protective effect of taurine against EtOH-induced lipid peroxidationTo evaluate whether EtOH exposure alters lipid peroxida-tion in zebrafish brain,we assessed the formation of TBARS during an acid-heating reaction.Acute EtOH ex-posure significantly increased by 40%(n ϭ3)the lipid per-oxidation (Fig.6).When the animals were treated with 150and 400mg·L Ϫ1taurine,there was a significant decrease of TBARS levels (30%and 50%,respectively)as com-pared to the control (n ϭ3).Interestingly,the cotreatment with taurine (400mg·L Ϫ1)did not prevent the increase of TBARS levels induced by EtOH (n ϭ5)(Fig.6A).Regarding the taurine pretreatment experiment (Fig.6B),a two-way ANOVA revealed significant effects of taurine pretreatment (F (2,23)ϭ74.45,P Ͻ0.05),taurine pret-reatment ϫEtOH exposure interaction (F (2,23)ϭ6.27,P Ͻ0.05)and EtOH exposure (F (1,23)ϭ4.75,P Ͻ0.05).Post hoc analyses indicated that pretreatment with 150and 400mg·L Ϫ1taurine prevented EtOH-induced enhancement of TBARS formation and the lipid peroxidation levels were statistically lower than that determined in control group (34%and 41%,respectively,n ϭ3).Fig.4.Protective effect of taurine in SOD/CAT ratio.(A)Cotreatment with taurine and EtOH.The groups were represented as control (CRL),ethanol (EtOH),taurine-treated (TAU)and taurine-cotreated animals (COT).Data represent mean ϮSEM of at least three independent experiments.*Significant difference compared to control (one-way ANOVA followed by Bonferroni’s test as post hoc,P Յ0.05).(B)Pre-treatment with taurine followed by water (control group)or EtOH exposure.The treatments were represented as taurine-untreated (UNT)and taurine-treated (TAU).Data represent mean ϮSEM of at least three independent experiments.*Significant difference com-pared to UNT from control group.#Significant difference compared to UNT from EtOH group (two-way ANOVA followed by Bonferroni’s test as post hoc,P Յ0.05).Fig.3.Effect of EtOH and taurine treatments in CAT activity.(A)Cotreatment with taurine and EtOH.The groups were represented as control (CRL),ethanol (EtOH),taurine-treated (TAU)and taurine-co-treated animals (COT).Data represent mean ϮSEM of at least three independent experiments.*Significant difference compared to control (one-way ANOVA followed by Bonferroni’s test as post hoc,P Յ0.05).(B)Pretreatment with taurine followed by water (control group)or EtOH exposure.The treatments were represented as taurine-un-treated (UNT)and taurine-treated (TAU).Data represent mean ϮSEM of at least three independent experiments.*Significant difference compared to UNT from control group.#Significant difference com-pared to UNT from EtOH group (two-way ANOVA followed by Bonfer-roni’s test as post hoc,P Յ0.05).D.B.Rosemberg et al./Neuroscience 171(2010)683–692687DISCUSSIONStudies have demonstrated that the behavioral changes induced by acute EtOH exposure in mammalian models are generally associated to its influence on several neuro-transmitter systems (Hanchar et al.,2005;Belmeguenai et al.,2008).Due to the susceptibility of zebrafish to environ-mental and pharmacological manipulations and the char-acterization of distinct neurotransmitter parameters in this species,it is rapidly becoming a popular vertebrate model in behavioral and neuroscience research (Egan et al.,2009;Rico et al.,2010;Rosemberg et al.,2010a ).It has been shown that acute EtOH exposure during 1h in the tank water induced significant alterations in behavioral tasks of zebrafish and also increased the levels of dopa-mine,serotonin,and its metabolite 5-HIAA in whole brain extracts (Chatterjee and Gerlai,2009).Zebrafish may be especially suitable for modeling the effects of EtOH be-cause of the simplicity of alcohol delivery (Gerlai et al.,2000).The EtOH mixed in their environment is absorbed by the blood vessels of the gill and the skin of the fish so that blood alcohol levels reach equilibrium with the external alcohol concentration quickly (Gerlai et al.,2000;Dlugos and Rabin,2003;Chatterjee and Gerlai,2009).Despite to the relative differences in comparison to mammalian me-tabolism of EtOH ingestion,the alcohol mixed to the fish tank is rapidly diffused through systemic circulation andreaches distinct tissues.There is evidence suggesting that hepatic alcohol dehydrogenase and aldehyde dehydroge-nase play a role in alcohol metabolism in fish,since 32h of continuous 2%EtOH exposure caused characteristics signs of acute alcoholic liver disease in zebrafish larvae,including hepatomegaly,steatosis and changes in hepatic gene expression (Passeri et al.,2009).Furthermore,it has been shown that significant brain alcohol levels can be detected 15min after exposure to 0.5%(v/v)EtOH in adult zebrafish brain,reaching a steady-state level that is main-tained for at least 24h without significant difference among distinct strains (Dlugos and Rabin,2003).These data are in accordance with studies involving other fish species (Ryback et al.,1969;Galizio et al.,1985)in that,following a few hours of exposure,an equilibrium between the level of alcohol in the tank and EtOH content in brain was reached.Previous report demonstrated that the brain al-cohol level of the zebrafish was achieved when brain al-cohol levels were approximately 90%of the tank alcohol level (Dlugos and Rabin,2003;Gerlai et al.,2006).Thus,it is comprehensive that the physiological responses promoted by acute EtOH exposure might involve alter-ations in neurotransmitter systems and also in oxidative stress parameters of zebrafish,suggesting that studies which comprise both approaches are tempting fortheFig.6.Effect of EtOH and taurine on lipid peroxidation measured as TBARS formation in zebrafish brain.(A)Cotreatment with taurine and EtOH.The groups were represented as control (CRL),ethanol (EtOH),taurine-treated (TAU)and taurine-cotreated animals (COT).Data rep-resent mean ϮSEM of at least three independent experiments.*Sig-nificant difference compared to control (one-way ANOVA followed by Bonferroni’s test as post hoc,P Յ0.05).(B)Pretreatment with taurine followed by water (control group)or EtOH exposure.The treatments were represented as taurine-untreated (UNT)and taurine-treated (TAU).Data represent mean ϮSEM of at least three independent experiments.*Significant difference compared to UNT from control group.#Significant difference compared to UNT from EtOH group (two-way ANOVA followed by Bonferroni’s test as post hoc,P Յ0.05).Fig.5.Effect of EtOH and taurine on total thiol content in zebrafish brain.(A)Cotreatment with taurine and EtOH.The groups were rep-resented as control (CRL),ethanol (EtOH),taurine-treated (TAU)and taurine-cotreated animals (COT).Data represent mean ϮSEM of at least three independent experiments.(B)Pretreatment with taurine followed by water (control group)or EtOH exposure.The treatments were represented as taurine-untreated (UNT)and taurine-treated (TAU).Data represent mean ϮSEM of at least three independent experiments.*Significant difference compared to UNT from control group.#Significant difference compared to UNT from EtOH group (two-way ANOVA followed by Bonferroni’s test as post hoc,P Յ0.05).D.B.Rosemberg et al./Neuroscience 171(2010)683–692688。