硫氮掺杂碳纳米管
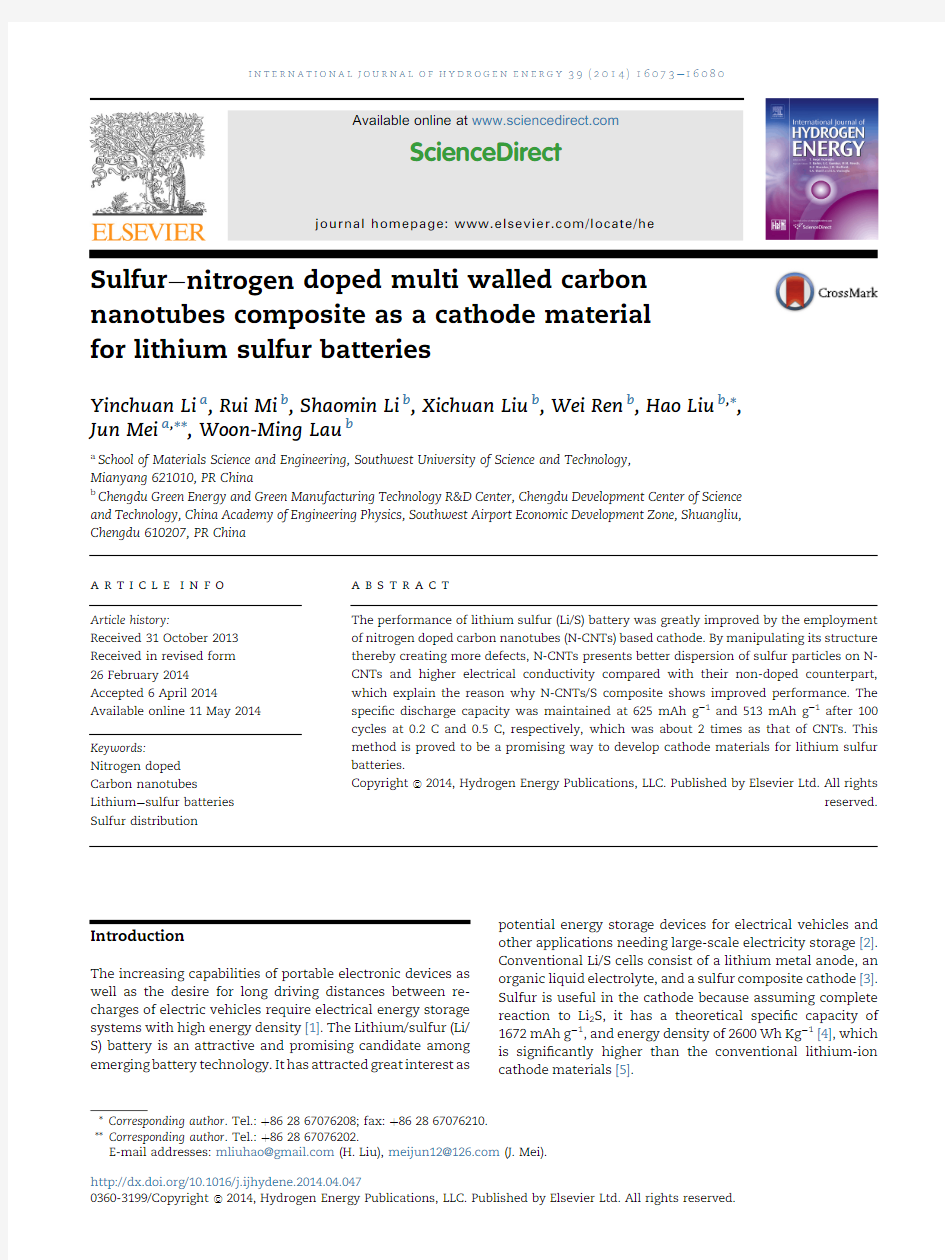
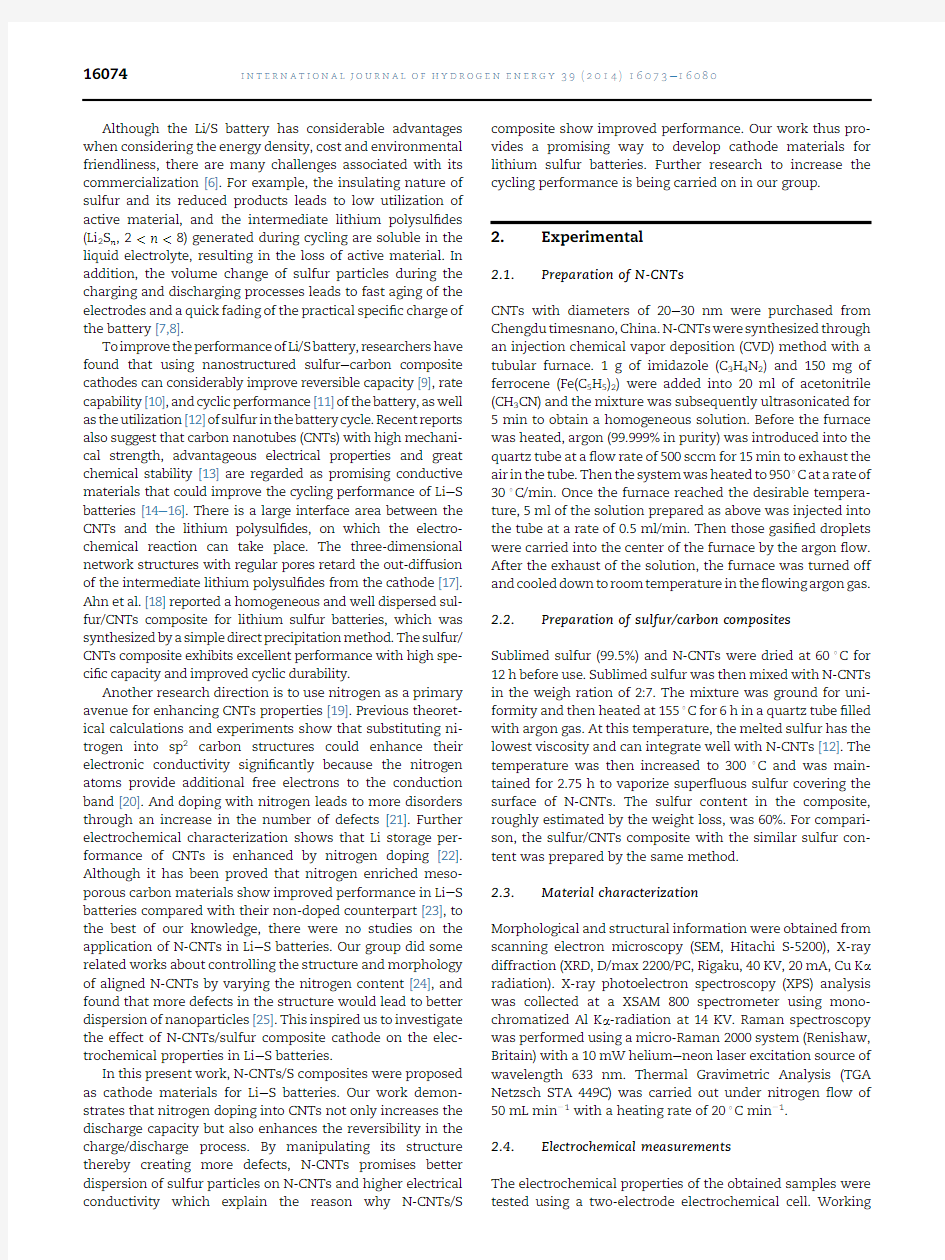
Sulfur e nitrogen doped multi walled carbon nanotubes composite as a cathode material for lithium sulfur batteries
Yinchuan Li a ,Rui Mi b ,Shaomin Li b ,Xichuan Liu b ,Wei Ren b ,Hao Liu b ,*,Jun Mei a ,**,Woon-Ming Lau b
a
School of Materials Science and Engineering,Southwest University of Science and Technology,Mianyang 621010,PR China b
Chengdu Green Energy and Green Manufacturing Technology R&D Center,Chengdu Development Center of Science and Technology,China Academy of Engineering Physics,Southwest Airport Economic Development Zone,Shuangliu,Chengdu 610207,PR China
a r t i c l e i n f o
Article history:
Received 31October 2013Received in revised form 26February 2014Accepted 6April 2014Available online 11May 2014Keywords:Nitrogen doped Carbon nanotubes Lithium e sulfur batteries Sulfur distribution
a b s t r a c t
The performance of lithium sulfur (Li/S)battery was greatly improved by the employment of nitrogen doped carbon nanotubes (N-CNTs)based cathode.By manipulating its structure thereby creating more defects,N-CNTs presents better dispersion of sulfur particles on N-CNTs and higher electrical conductivity compared with their non-doped counterpart,which explain the reason why N-CNTs/S composite shows improved performance.The speci?c discharge capacity was maintained at 625mAh g à1and 513mAh g à1after 100cycles at 0.2C and 0.5C,respectively,which was about 2times as that of CNTs.This method is proved to be a promising way to develop cathode materials for lithium sulfur batteries.
Copyright a2014,Hydrogen Energy Publications,LLC.Published by Elsevier Ltd.All rights
reserved.
Introduction
The increasing capabilities of portable electronic devices as well as the desire for long driving distances between re-charges of electric vehicles require electrical energy storage systems with high energy density [1].The Lithium/sulfur (Li/S)battery is an attractive and promising candidate among emerging battery technology.It has attracted great interest as
potential energy storage devices for electrical vehicles and other applications needing large-scale electricity storage [2].Conventional Li/S cells consist of a lithium metal anode,an organic liquid electrolyte,and a sulfur composite cathode [3].Sulfur is useful in the cathode because assuming complete reaction to Li 2S,it has a theoretical speci?c capacity of 1672mAh g à1,and energy density of 2600Wh Kg à1[4],which is signi?cantly higher than the conventional lithium-ion cathode materials [5].
*Corresponding author .Tel.:t862867076208;fax:t862867076210.**Corresponding author .Tel.:t862867076202.
E-mail addresses:mliuhao@https://www.360docs.net/doc/4213931753.html, (H.Liu),meijun12@https://www.360docs.net/doc/4213931753.html, (J.
Mei).
Available online at https://www.360docs.net/doc/4213931753.html,
ScienceDirect
journal homepage:
https://www.360docs.net/doc/4213931753.html,/locate/he
i n t e r n a t i o n a l j o u r n a l o f h y d r o g e n e n e r g y 39(2014)16073e 16080
https://www.360docs.net/doc/4213931753.html,/10.1016/j.ijhydene.2014.04.047
0360-3199/Copyright a2014,Hydrogen Energy Publications,LLC.Published by Elsevier Ltd.All rights reserved.
Although the Li/S battery has considerable advantages when considering the energy density,cost and environmental friendliness,there are many challenges associated with its commercialization[6].For example,the insulating nature of sulfur and its reduced products leads to low utilization of active material,and the intermediate lithium polysul?des (Li2S n,2 To improve the performance of Li/S battery,researchers have found that using nanostructured sulfur e carbon composite cathodes can considerably improve reversible capacity[9],rate capability[10],and cyclic performance[11]of the battery,as well as the utilization[12]of sulfur in the battery cycle.Recent reports also suggest that carbon nanotubes(CNTs)with high mechani-cal strength,advantageous electrical properties and great chemical stability[13]are regarded as promising conductive materials that could improve the cycling performance of Li e S batteries[14e16].There is a large interface area between the CNTs and the lithium polysul?des,on which the electro-chemical reaction can take place.The three-dimensional network structures with regular pores retard the out-diffusion of the intermediate lithium polysul?des from the cathode[17]. Ahn et al.[18]reported a homogeneous and well dispersed sul-fur/CNTs composite for lithium sulfur batteries,which was synthesized by a simple direct precipitation method.The sulfur/ CNTs composite exhibits excellent performance with high spe-ci?c capacity and improved cyclic durability. Another research direction is to use nitrogen as a primary avenue for enhancing CNTs properties[19].Previous theoret-ical calculations and experiments show that substituting ni-trogen into sp2carbon structures could enhance their electronic conductivity signi?cantly because the nitrogen atoms provide additional free electrons to the conduction band[20].And doping with nitrogen leads to more disorders through an increase in the number of defects[21].Further electrochemical characterization shows that Li storage per-formance of CNTs is enhanced by nitrogen doping[22]. Although it has been proved that nitrogen enriched meso-porous carbon materials show improved performance in Li e S batteries compared with their non-doped counterpart[23],to the best of our knowledge,there were no studies on the application of N-CNTs in Li e S batteries.Our group did some related works about controlling the structure and morphology of aligned N-CNTs by varying the nitrogen content[24],and found that more defects in the structure would lead to better dispersion of nanoparticles[25].This inspired us to investigate the effect of N-CNTs/sulfur composite cathode on the elec-trochemical properties in Li e S batteries. In this present work,N-CNTs/S composites were proposed as cathode materials for Li e S batteries.Our work demon-strates that nitrogen doping into CNTs not only increases the discharge capacity but also enhances the reversibility in the charge/discharge process.By manipulating its structure thereby creating more defects,N-CNTs promises better dispersion of sulfur particles on N-CNTs and higher electrical conductivity which explain the reason why N-CNTs/S composite show improved performance.Our work thus pro-vides a promising way to develop cathode materials for lithium sulfur batteries.Further research to increase the cycling performance is being carried on in our group. 2.Experimental 2.1.Preparation of N-CNTs CNTs with diameters of20e30nm were purchased from Chengdu timesnano,China.N-CNTs were synthesized through an injection chemical vapor deposition(CVD)method with a tubular furnace.1g of imidazole(C3H4N2)and150mg of ferrocene(Fe(C5H5)2)were added into20ml of acetonitrile (CH3CN)and the mixture was subsequently ultrasonicated for 5min to obtain a homogeneous solution.Before the furnace was heated,argon(99.999%in purity)was introduced into the quartz tube at a?ow rate of500sccm for15min to exhaust the air in the tube.Then the system was heated to950 C at a rate of 30 C/min.Once the furnace reached the desirable tempera-ture,5ml of the solution prepared as above was injected into the tube at a rate of0.5ml/min.Then those gasi?ed droplets were carried into the center of the furnace by the argon?ow. After the exhaust of the solution,the furnace was turned off and cooled down to room temperature in the?owing argon gas. 2.2.Preparation of sulfur/carbon composites Sublimed sulfur(99.5%)and N-CNTs were dried at60 C for 12h before use.Sublimed sulfur was then mixed with N-CNTs in the weigh ration of2:7.The mixture was ground for uni-formity and then heated at155 C for6h in a quartz tube?lled with argon gas.At this temperature,the melted sulfur has the lowest viscosity and can integrate well with N-CNTs[12].The temperature was then increased to300 C and was main-tained for2.75h to vaporize super?uous sulfur covering the surface of N-CNTs.The sulfur content in the composite, roughly estimated by the weight loss,was60%.For compari-son,the sulfur/CNTs composite with the similar sulfur con-tent was prepared by the same method. 2.3.Material characterization Morphological and structural information were obtained from scanning electron microscopy(SEM,Hitachi S-5200),X-ray diffraction(XRD,D/max2200/PC,Rigaku,40KV,20mA,Cu K a radiation).X-ray photoelectron spectroscopy(XPS)analysis was collected at a XSAM800spectrometer using mono-chromatized Al K a-radiation at14KV.Raman spectroscopy was performed using a micro-Raman2000system(Renishaw, Britain)with a10mW helium e neon laser excitation source of wavelength633nm.Thermal Gravimetric Analysis(TGA Netzsch STA449C)was carried out under nitrogen?ow of 50mL minà1with a heating rate of20 C minà1. 2.4.Electrochemical measurements The electrochemical properties of the obtained samples were tested using a two-electrode electrochemical cell.Working i n t e r n a t i o n a l j o u r n a l o f h y d r o g e n e n e r g y39(2014)16073e16080 16074 electrodes were prepared by casting the slurry homoge-neously on an Al foil as a current collector.The slurry was produced by uniformly mixing the active material(CNTs or N-CNTs,80wt.%on dry solids basis),carbonaceous additive (acetylene black,10wt.%)and a poly vinylidene di?uoride (PVDF,10wt.%on dry solids basis)in N-methylpyrrolidone (NMP)solvent.The loading of all sample are around 0.45e0.55mg cmà2.The electrodes were dried in a vacuum oven at60 C for20h.Lithium foil was used as the counter electrode.The electrolyte was composed of1M lithium bis(-tri?uoromethane sulfone)imide(LiTFSI)dissolved in1,3-dioxolane(DOL)/dimethoxy ethane(DME)in a1:1(volume) mixture and Celgard2400microporous polypropylene?lm was used as a separator.The CR-2032-type coin cells were assembled in an argon-?lled glove box(moisture and oxygen concentration<0.1ppm).The cells were charged and dis-charged over a voltage range of1.5e2.6V(vs Lit/Li)at different rates using an Arbin BT-2000Battery Test System.Electro-chemical impedance spectroscopy(EIS)measurements were performed using CHI760D electrochemical workstation.The impedance spectra were obtained by applying an AC voltage of5.0mV over the frequency range from0.1to100KHz at room temperature.Cyclic voltammetry measurements were carried out on the CHI760D electrochemical workstation over the potential range 1.5e2.6vs.Lit/Li at a scan rate of 0.1mV sà1 3.Results and discussion 3.1.Morphological and nitrogen doping characterization Typical SEM images of CNTs and N-CNTs are shown in Fig.1. Both samples have uniform distributions in diameters.The diameter of CNTs and N-CNTs are in the same range.The bamboo-like structure in N-CNTs indicates that nitrogen atoms were introduced into the carbon network[26]. Fig.2shows XRD patterns of CNTs and N-CNTs as well as N-CNTs/S composite.Both CNTs and N-CNTs samples exhibit a broad(002)diffraction peak at2q around26 and a weak(1 00)diffraction peak around43 in the hexagonal graphitic carbon structure(Fig.2(a)).Both peaks of N-CNTs slightly shift to lower2q values compared with those of CNTs.The(100) line shift is assigned to the relaxation and distortions caused by the introduction of C e N bond(shorter than C e C bond) within the sp2carbon layer[27].The(002)peak shift was associated with the expansion of the interlayer distance be-tween the two graphitic layers to relax the distortion caused by nitrogen doping in the sp2carbon layer[28].The XRD pat-terns given in Fig.2(b)con?rm the nanostructure of the N-CNTs/S.The re?ections of the sulfur are consistent with Fddd orthorhombic https://www.360docs.net/doc/4213931753.html,pared with the pattern of the raw elemental sulfur,the XRD spectrum of the N-CNTs/S did not exhibit many changes except for the appearance N-CNTs peaks(Fig.2(a)),indicating that no phase transformation occurred during heat treatment and the crystal structure of sulfur still remains a Fddd orthorhombic structure.The weight loss of CNTs and N-CNTs after sulfur incorporation was recorded by TGA(Fig.3).The sulfur contents of CNTs/S and N-CNTs/S were around60%. The XPS spectra shown in Fig.4further con?rm the incorporation of nitrogen in N-CNTs.A full scan spectrum of N-CNTs is illustrated in Fig.4(a).Three strong peaks at290, 401,and530eV are attributed to C1s,N1s and O1s,respec-tively.The atomic concentration of N can be estimated by the area ratio of N peak to the sum of C and N peaks.In this work, the nitrogen content in N-CNTs is2.34at.%.The position of the main C1s peak at290eV con?rms the graphite structure of carbon which corresponds to sp2C e C bond[29,30].Deconvo-lution of the N1s peak was carried out to understand the bonding environment of nitrogen atoms incorporated in N-CNTs.As shown in Fig.4(b),the peak at398.8eV was attrib-uted to the pyridine-like nitrogen which bonds with two sp2 carbons,while the peak at400.9eV could correspond to the graphite-like nitrogen which bonds with three sp2carbons mostly located inside the graphitic carbon plane[31].The peak located at405.1eV could be ascribed to the chemisorbed ni-trogen oxide on the graphite layers[32]. 3.2.Electrochemical performance The cyclic voltammograms(CV)of the CNTs/S and N-CNTs/S composite electrodes are shown in Fig.5(a)and(b).Two reduction peaks are observed from both electrodes.The?rst peak around2.3V is attributable to the reduction of sulfur to Fig.1e SEM micrographs of(a)pristine CNTs and(b)N-CNTs. i n t e r n a t i o n a l j o u r n a l o f h y d r o g e n e n e r g y39(2014)16073e1608016075 lithium polysul?des and the second peak around 2.05V to the reduction of longer-chain polysul?des to lithium sul?des [33].Compared with CNTs/S electrode,the cathodic and anodic peaks of N-CNTs/S electrode show a complete overlapping through cycles,suggesting an effective retention of capacity and prevention of the shuttle mechanism [34].Fig.5(c)and (d)show the discharge/charge pro?les of CNTs/S and N-CNTs/S electrodes at different current densities.The N-CNTs/S composite-based cells deliver a higher capacity in the initial cycle at 0.1C (168mA g à1),0.2C (336mA g à1),0.5C (840mA g à1),respectively. Cycling performance of N-CNTs/S cathode at 0.2C and 0.5C is presented in Fig.6,together with that of the CNTs/S without nitrogen doping.A reversible capacity of around 625mAh/g was observed after 100cycles of charge and discharge.The discharge capacity at 0.5C also shows good cycling stability,and the reversible capacity was around 513mAh/g after 100cycles.N-CNTs/S electrode also exhibits higher coulombic ef?ciencies during cycling processes at both rates.Obviously,these results show improved performance in speci?c capacity as compared to CNTs/S composite. To understand the improvement in Li/S batteries’perfor-mance coming from nitrogen doping,further characterization to CNTs/S and N-CNTs/S composites were carried out.The energy dispersive spectroscopy (EDS)mapping in Fig.7pre-sents homogenous distribution of sulfur and carbon in the NCNTs/S composite,indicating that sulfur forms highly-dispersed nano particle. Fig.3e TGA of CNTs/S and N-CNTs/S composites. Fig.4e XPS full scan spectra of CNTs and N-CNTs (a),XPS N1s spectra of N-CNTs (b). Fig.2e XRD patterns of (a)CNTs and N-CNTs including a magni?ed view in the range of 23e 31 inserted,(b)N-CNTs/S composite. i n t e r n a t i o n a l j o u r n a l o f h y d r o g e n e n e r g y 39(2014)16073e 16080 16076 In addition,Raman spectroscopy was carried out to study the reason why nitrogen doping improved dispersion of sulfur on N-CNTs.Fig.8shows the Raman spectra of CNTs and N-CNTs.Both samples exhibit two obvious peaks at w 1345and w 1570cm à1,corresponding to the D and G bands,respec-tively.The D band denotes the disordered graphite structure,whereas the G band indicates the presence of crystalline graphitic carbon [35].The intensity ratio of D to G bands (I D /I G )is used to evaluate the disorder in carbon materials [36].The I D /I G ratios of CNTs and N-CNTs are 0.99and 1.16,respectively.The higher I D /I G ratio implies more defects [37]which facilitate the distribution of sulfur on N-CNTs. The impedance spectra of CNTs/S and N-CNTs/S electrodes were analyzed and ?tted to the equivalent circuit as shown in Fig.9.In the equivalent circuit,Rs is the total resistance of electrolyte,electrode and separator.Rct and CPE1are the resistance and capacitance of the ?lm formed on the electrode surface,which is related to the formation of SEI.Zw is known as the Warburg resistance and is related to the frequency dependence of ion diffusion/transportation in the electrolyte to the electrode surface [38].The ?tting values from the equivalent circuit are presented in Table 1.It is evident that the N-CNTs based electrode has a lower resistance (Rs ?4.35,Rct ?13.03)than those of the CNTs based electrode (Rs ?5.03,Rct ?14.13).It indicates that the N-CNTs/S electrode pos-sesses faster charge-transfer kinetics [23].Hence,the improved dispersion of S on N-CNTs and the reduced impedance of N-CNTs give rise to higher reversible speci?c capacity with cycling. 4. Conclusions The performance of Li/S battery was greatly improved by the employment of N-CNTs based cathode,which was synthesized using an injection CVD method and the Fig.5e Cyclic voltammograms of CNTs/S (a)and N-CNTs/S (b)electrodes at a scan rate of 0.1mV s L 1in a voltage range of 1.5e 2.6V.Initial discharge/charge pro?les of CNTs/S (c)and N-CNTs/S (d)electrodes at different rates. Fig.6e Cycle performance at 0.2C (336mA g L 1 )and 0.5C (840mA g L 1)of CNTs/S and N-CNTs/S electrodes. i n t e r n a t i o n a l j o u r n a l o f h y d r o g e n e n e r g y 39(2014)16073e 16080 16077 addition of sulfur by heat treatment.The speci?c discharge capacity was maintained at 625mAh g à1and 513mAh g à1after 100cycles at 0.2C and 0.5C,respectively,which was about 2times as that of CNTs.This improved performance is attributed to the enhanced electronic conductivity of electrode and more uniform sulfur dispersion on the nanotubes resulting from nitrogen doping,which were proved by reduced resistance examined from EIS and highly dispersed sulfur particles observed from EDS,separately.Although the achieved capacity is not the highest,consid-ering the straightforward composition method of N-CNTs and sulfur,this method is proved to be a promising way to enhance the performance of carbon nanotubes based cathode materials of Li e S batteries. Fig.7e EDS mapping of CNTs/S (a)and N-CNTs/S (b). Fig.8e Raman spectra of CNTs and N-CNTs. Fig.9e EIS of CNTs/S and N-CNTs/S electrodes after 30cycles of CV measurements at a scan rate of 0.5mV s L 1;insert:Randles equivalent circuit used for analysis of impedance spectra. i n t e r n a t i o n a l j o u r n a l o f h y d r o g e n e n e r g y 39(2014)16073e 16080 16078 Acknowledgments The authors appreciate the support of the Science and Technology Foundation of China Academy of Engineering Physics(No.2012B0302041).We are indebted to Margaret Yau,Jiahui Lin,Kun Luo,Cong Wang,Changzhen Man,Jie Chen and Yongzheng Zhang for their kind help and fruitful discussions. r e f e r e n c e s [1]Manthiram A,Fu Y,Su Y-S.In charge of the world: electrochemical energy storage.J Phys Chem Lett 2013;4:1295e7. [2]Bruce PG,Freunberger SA,Hardwick LJ,Taracon JM.Li-O2and Li-S batteries with high energy storage.Nat Mater 2012;11:19e29. [3]Manthiram A,Fu Y,Su Y-S.Challenges and prospects of lithium-sulfur batteries.Acc Chem Res2013;46:1125e34. [4]Zhang Y,Wu X,Feng H,Wang L,Zhang A,Xia T,et al.Effect of nanosized Mg0.8Cu0.2O on electrochemical properties of Li/ S rechargeable batteries.Int J Hydrogen Energy 2009;34:1556e9. [5]Barchasz C,Lepretre JC,Alloin F,Patoux.New insights into limiting parameters of the Li/S rechargeable cell.J Power Sources2012;199:322e30. [6]Yang Y,Zheng G,Cui Y.Nanostructured sulfur cathodes. Chem Soc Rev2013;42:3018e32. [7]Jayaprakash N,Shen J,Moganty SS,Corona A,Archer LA. Porous hollow carbon@sulfur composites for high-power lithium-sulfur batteries.Angew Chem Int Ed2011;50:5904e8. [8]Seh Z,Li W,Cha J,Zheng G,Yang Y,McDowell MT,et al. Sulphur-TiO2yolk-shell nanoarchitecture with internal void space for long-cycle lithium-sulphur batteries.Nat Commun 2013;4:1331e6. [9]Zheng G,Yang Y,Cha JJ,Hong SS,Cui Y.Hollow carbon nano?ber-encapsulated sulfur cathodes for high speci?c capacity rechargeable lithium batteries.Nano Lett 2011;11:4462e7. [10]Ji L,Rao M,Aloni S,Wang L,Cairns EJ,Zhang Y.Porous carbon nano?ber-sulfur composite electrodes for lithium/ sulfur cells.Energy Environ Sci2011;4:5053e9. [11]Lu S,Cheng Y,Wu X,Liu J.Signi?cantly improved long-cycle stability in high-rate Li-S batteries enabled by coaxial graphene wrapping over sulfur coated carbon nano?bers. Nano Lett2013;13:2485e9. [12]Ji X,Lee KT,Nazar LF.A highly ordered nanostructured carbon-sulphur cathode for lithium-sulphur batteries.Nat Mater2009;8:500e6. [13]Li X,Yang J,Hu Y,Wang J,Li Y,Cai M,et al.Novel approach toward a binder-free and current collector-free anode con?guration:highly?exible nanoporous carbon nanotube electrodes with strong mechanical strength harvesting improved lithium stage.J Mater Chem2012;22:18847e53. [14]Guo J,Xu Y,Wang C.Sulfur-impregnated disordered carbon nanotubes cathode for lithium-sulfur batteries.Nano Lett 2011;11:4288e94.[15]Liang X,Wen Z,Liu Y,Zhang H,Jin J,Wu M,et al.A composite of sulfur and polpyrrole-multi walled carbon combinatorial nanotubes as cathode for Li/S battery.J Power Sources2012;206:409e13. [16]Wei W,Wang J,Zhou L,Yang J,Schumann B,Nuli https://www.360docs.net/doc/4213931753.html,T enhanced sulfur composite cathode material for high rate lithium battery.Electrochem Commun2011;13:399e402. [17]Zheng W,Liu Y,Hu X,Zhang C.Novel nanosized adsorbing sulfur composite cathode materials for the advanced secondary lithium batteries.Electrochim Acta 2006;51:1330e5. [18]Ahn W,Kim K-B,Jung K-N,Shin K-H,Jin C-S.Synthesis and electrochemical properties of a sulfur-multi walled carbon nanotubes composite as a cathode material for lithium sulfur batteries.J Power Sources2012;202:394e9. [19]Pattinson SW,Diaz RE,Stelmashenko NA,Windle AH, Ducati C,Stach EA,et al.In situ observation of the effect of nitrogen on carbon nanotube synthesis.Chem Mater 2013;25:2921e3. [20]Wiggins-Camacho JD,Stevenson KJ.Effect of nitrogen concentration on capacitance,density of states,electronic conductivity,and morphology of N-doped carbon nanotube electrodes.J Phys Chem C2009;113:19082e90. [21]Nxumalo EN,Nyamori VO,Coville NJ.CVD synthesis of nitrogen doped carbon nanotubes using ferrocene/aniline mixtures.J Organomet Chem2008;693:2942e8. [22]Ren W,Li D,Liu H,Mi R,Zhang Y,Dong L.Lithium storage performance of carbon nanotubes with different nitrogen contents as anodes in lithium ions batteries.Electrochim Acta2013;105:75e82. [23]Sun X-G,Wang X,Mayes RT,Dai S.Lithium-Sulfur batteries based on nitrogen-doped carbon and an lonic-liquid electrolyte.ChemSusChem2012;5:2079e85. [24]Liu H,Zhang Y,Li R,Sun X,Desilets S,Abou-Rachid A,et al. Structural and morphological control of aligned nitrogen- doped carbon nanotubes.Carbon2010;48:1498e507. [25]Chen Y,Wang J,Liu H,Li R,Sun X,Ye S,et al.Enhanced stability of Pt electrocatalysts by nitrogen doping in CNTs for PEM fuel cells.Electrochem Commun2009;11:2071e6. [26]Li X,Liu J,Zhang Y,Li Y,Liu H,Meng X,et al.High concentration nitrogen doped carbon nanotube anodes with superior Litstorage performance for lithium rechargeable battery application.J Power Sources2012;197:238e45. [27]Ghosh K,Kumar M,Maruyama T,And Y.Tailoring the?eld emission property of nitrogen-doped carbon nanotubes by controlling the graphitic/pyridinic substitution.Carbon 2010;48:191e200. [28]Kim SY,Lee J,Na CW,Park J,Seo K,Kim B.N-doped double- walled carbon nanotubes synthesized by chemical vapor deposition.Chem Phys Lett2005;413:300e5. [29]Pels JR,Kapteijn F,Moulijn JA,Zhu Q,Thomas KM.Evolution of nitrogen functionalities in carbonaceous materials during pyrolysis.Carbon1995;33:1641e53. [30]Maldonado S,Morin S,Stevenson KJ.Structure,composition, and chemical reactivity of carbon nanotubes by selective nitrogen doping.Carbon2006;44:1429e37. [31]Morant C,Andrey J,Prieto P,Mendiola D,Sanz JM,Elizalde E. XPS characterization of nitrogen-doped carbon nanotubes. Phys Status Solidi A2006;203:1069e75. [32]Zhao L,Baccile N,Gross S,Zhang Y,Wei W,Sun Y,et al. Sustainable nitrogen-doped carbonaceous materials from biomass derivatives.Carbon2010;48:3778e87. [33]Ji L,Rao M,Zheng H,Zhang L,Li Y,Duan W,et al.Graphene oxide as a sulfur immobilizer in high performance lithium/ sulfur cells.J Am Chem Soc2011;133:18522e5. [34]Zu C,Manthiram A.Hydroxylated graphene-sulfur nanocomposites for high-rate lithium-sulfur batteries.Adv Funct Mater2013;3:1008e12. i n t e r n a t i o n a l j o u r n a l o f h y d r o g e n e n e r g y39(2014)16073e1608016079 [35]Li X,Geng D,Zhang Y,Meng X,Li R,Sun X.Superior cycle stability of nitrogen-doped graphene nanosheets as anodes for lithium ion batteries.Electrochem Commun 2011;13:822e5. [36]Chuang C,Huang J,Chen W,Lee C,Chang Y.Role of amorphous carbon nanowires in reducing the turn-on?eld of carbon?lms prepared by microwave-heated CVD.Diam Relat Mater2004;13:1012e6.[37]Li Y,Wang J,Li X,Liu J,Geng D,Yang J,et al.Nitrogen-doped carbon nanotubes as cathode for lithium-air batteries. Electrochem Commun2011;13:668e72. [38]Zhang Y,Liu H,Zhu Z,Woog Ka-wai,Mi R,Mei J,et al.A green hydrothermal approach for the preparation of graphene/a-MnO23D network as anode for lithium ion battery.Electrochim Acta2013;108:465e71. i n t e r n a t i o n a l j o u r n a l o f h y d r o g e n e n e r g y39(2014)16073e16080 16080 Sulfur e nitrogen doped multi walled carbon nanotubes composite as a cathode material for lithium sulfur batteries Yinchuan Li a ,Rui Mi b ,Shaomin Li b ,Xichuan Liu b ,Wei Ren b ,Hao Liu b ,*,Jun Mei a ,**,Woon-Ming Lau b a School of Materials Science and Engineering,Southwest University of Science and Technology,Mianyang 621010,PR China b Chengdu Green Energy and Green Manufacturing Technology R&D Center,Chengdu Development Center of Science and Technology,China Academy of Engineering Physics,Southwest Airport Economic Development Zone,Shuangliu,Chengdu 610207,PR China a r t i c l e i n f o Article history: Received 31October 2013Received in revised form 26February 2014Accepted 6April 2014Available online 11May 2014Keywords:Nitrogen doped Carbon nanotubes Lithium e sulfur batteries Sulfur distribution a b s t r a c t The performance of lithium sulfur (Li/S)battery was greatly improved by the employment of nitrogen doped carbon nanotubes (N-CNTs)based cathode.By manipulating its structure thereby creating more defects,N-CNTs presents better dispersion of sulfur particles on N-CNTs and higher electrical conductivity compared with their non-doped counterpart,which explain the reason why N-CNTs/S composite shows improved performance.The speci?c discharge capacity was maintained at 625mAh g à1and 513mAh g à1after 100cycles at 0.2C and 0.5C,respectively,which was about 2times as that of CNTs.This method is proved to be a promising way to develop cathode materials for lithium sulfur batteries. Copyright a2014,Hydrogen Energy Publications,LLC.Published by Elsevier Ltd.All rights reserved. Introduction The increasing capabilities of portable electronic devices as well as the desire for long driving distances between re-charges of electric vehicles require electrical energy storage systems with high energy density [1].The Lithium/sulfur (Li/S)battery is an attractive and promising candidate among emerging battery technology.It has attracted great interest as potential energy storage devices for electrical vehicles and other applications needing large-scale electricity storage [2].Conventional Li/S cells consist of a lithium metal anode,an organic liquid electrolyte,and a sulfur composite cathode [3].Sulfur is useful in the cathode because assuming complete reaction to Li 2S,it has a theoretical speci?c capacity of 1672mAh g à1,and energy density of 2600Wh Kg à1[4],which is signi?cantly higher than the conventional lithium-ion cathode materials [5]. *Corresponding author .Tel.:t862867076208;fax:t862867076210.**Corresponding author .Tel.:t862867076202. E-mail addresses:mliuhao@https://www.360docs.net/doc/4213931753.html, (H.Liu),meijun12@https://www.360docs.net/doc/4213931753.html, (J. Mei). Available online at https://www.360docs.net/doc/4213931753.html, ScienceDirect journal homepage: https://www.360docs.net/doc/4213931753.html,/locate/he i n t e r n a t i o n a l j o u r n a l o f h y d r o g e n e n e r g y 39(2014)16073e 16080 https://www.360docs.net/doc/4213931753.html,/10.1016/j.ijhydene.2014.04.047 0360-3199/Copyright a2014,Hydrogen Energy Publications,LLC.Published by Elsevier Ltd.All rights reserved. 选择性氮掺杂的碳纳米管的结构、组成和化学 摘要 掺杂有一系列氮含量为(0-10%)的碳纳米管(CNT)通过使用二茂铁,NH3和二甲苯或吡啶在一个浮动催化剂CVD上进行合成的方法。XPS和Raman显微镜用来定量评估掺氮碳纳米管的组成和结构特性(N-CNTs)。XPS分析表明C1s 光谱轨迹随着氮掺杂N1sXPS光谱发生的移位和扩大显示出三种主要类型的氮协调(吡啶,镍铬合金和季),伴随着吡啶型选择率从0增加到4.5%。一阶拉曼光谱出现的五峰由于氮含量不同在峰强度和宽度上有所不同。D和G带集合强度的比例随着氮含量线形变化。用碘滴定的方法来测量所制备的N-CNTs还原位点的数量。这是通过掺杂氮的方法对碳纳米管化学活性有决定性影响的第一份报告。针对规律性增长和CNTs的选择性掺杂氮已经报道的方法,提出了一种新的方法来系统地研究纳米碳组成和结构对化学和电化学活性在应用上的影响。 1 简介 石墨烯晶格中杂原子(硼、硫、磷和氮)掺杂兑SP2碳材料的物化特性有着不同的影响。其中氮的取代掺杂尤其受到重视,因为其对硬度、导电性和化学活性显著改变进行了理论预测和实验观察。掺氮碳材料合成的几种方法已经在应用中,包括溅射沉积、含氮聚合物石墨化和预先形成的碳爆漏在升温过程中已形成反应气体(HCN和NH3)。虽然前两条线路通常制得的材料可以分别用作惰性涂料和吸附剂。后一条线路特别有希望合成可以增强化学反应中电子转移过程的活性碳,可以应用在电池和燃料电池中。虽然许多研究已经评估了掺氮碳的结构组成特性之间的关系,但是掺氮对物理化学特性的影响没有得到充分界定。举个例子,碳表面积、表面功能和石墨化程度由于采用碳材料和前处理及加工过程的不同而有相当大的差异。进一步,掺杂氮的过程是一个采用活化条件的复杂过程(比如反应气体浓度、时间、温度),因此,关于掺氮碳会得到许多不同的甚至相互矛盾的结论。 一个引人注目的替代方案可以使其直接生长和纳米碳进行氮的取代掺杂,这个方法使用到气相前体而不是像传统方法那样使用液相或者固相前体。通过化学气相沉积技术合成的气相纳米碳对于物化性质有着很好的控制能力,比如杂原子掺杂、结晶度和边缘暴露程度。我们实验室之前的报告已经描述在碳纳米管电极上掺氮对于氧化还原和、过氧化氢分解和邻苯二酚氧化反应的影响。在此,我们提出对于采用吡啶和NH3,通过改进的流化催化剂合成碳纳米管进行控制增长 氮掺杂提升碳纳米管钌催化剂活性 2016-06-05 14:02来源:内江洛伯尔材料科技有限公司作者:研发部 氮掺杂提升碳纳米管钌 催化剂活性 最近研究发现,杂原子如N等的引入可进一步调变CNTs的结构,尤其是电子结构.Czerw等通过理论计算其局域态密度发现,本为半导体的锯齿碳纳米管(17,0) 经过掺杂吡啶型N以后呈现金属特性;Amadou等通过测量pH值计算等电点的办法发现,N的掺杂使得CNTs等电点变大,表明其具有弱碱性.研究表明,N的掺杂使CNTs 负载的催化剂上肉桂醛加氢、氨分解和甲醇氧化等反应性能有所提高.这些作者认为, 由于氮掺杂的碳纳米管中增强的π键和N原子的供电子作用以及丰富的结构缺陷, 促进了催化剂粒子分布均匀. 合成氨是最重要的工业反应之一,碳负载的Ru系催化剂被认为是继铁之后的第二代氨合成催化剂. 由于Ru催化合成氨反应涉及N2分子解离吸附, 具有较高的电子密度和导电能力的催化剂有利于N2的解离吸附, 从而提高反应活 性.Liang等报道CNTs担载的Ru基催化剂活性明显高于传统活性炭负载的催化剂, 他们将其归因于CNTs具有良好的石墨化程度和高导电性. 碱金属如K等的添加因其供电子作用进一步提高了Ru催化合成氨反应性能。 Guo等发现, 位于CNTs管外壁的Ru粒子因受其修饰作用, Ru表面的电子密度相对高于负载在管腔内的, 因而其催化合成氨反应的活性更高. 由于N掺杂CNTs具有良好的导电性能和N原子的供电子作用, 可能会促进合成氨反应。 中国科学院大连化学物理研究所催化基础国家重点实验室包信和小组 以乙腈为碳源和氮源, 采用化学气相沉积法制备了氮掺杂的碳纳米管. 电子显微镜观察表明, 样品形貌为中空的多壁纳米管, 管腔大小10~15 nm, 壁厚10~20 nm. X 射线光电子能谱结果表明, 氮已掺杂到碳纳米管结构中, 主要以吡啶型氮和取代型氮存在. 结合X射线衍射和拉曼光谱结果发现, 随着制备温度的升高, 氮掺杂量减少, 但纳米管的石墨化程度提高. 与未掺杂碳纳米管相比, 氮掺杂碳纳米管负载的Ru催化剂上催化合成氨反应活性增加, 于650℃制得的掺氮碳纳米管负载的Ru催化剂活性相对最高, 这可能是由于载体中氮掺杂和管壁石墨化的综合作用所致。硫氮掺杂碳纳米管
选择性氮掺杂的碳纳米管的结构、组成和化学
氮掺杂提升碳纳米管钌催化剂活性