大洋中脊岩浆活动研究-南海海洋所-2020
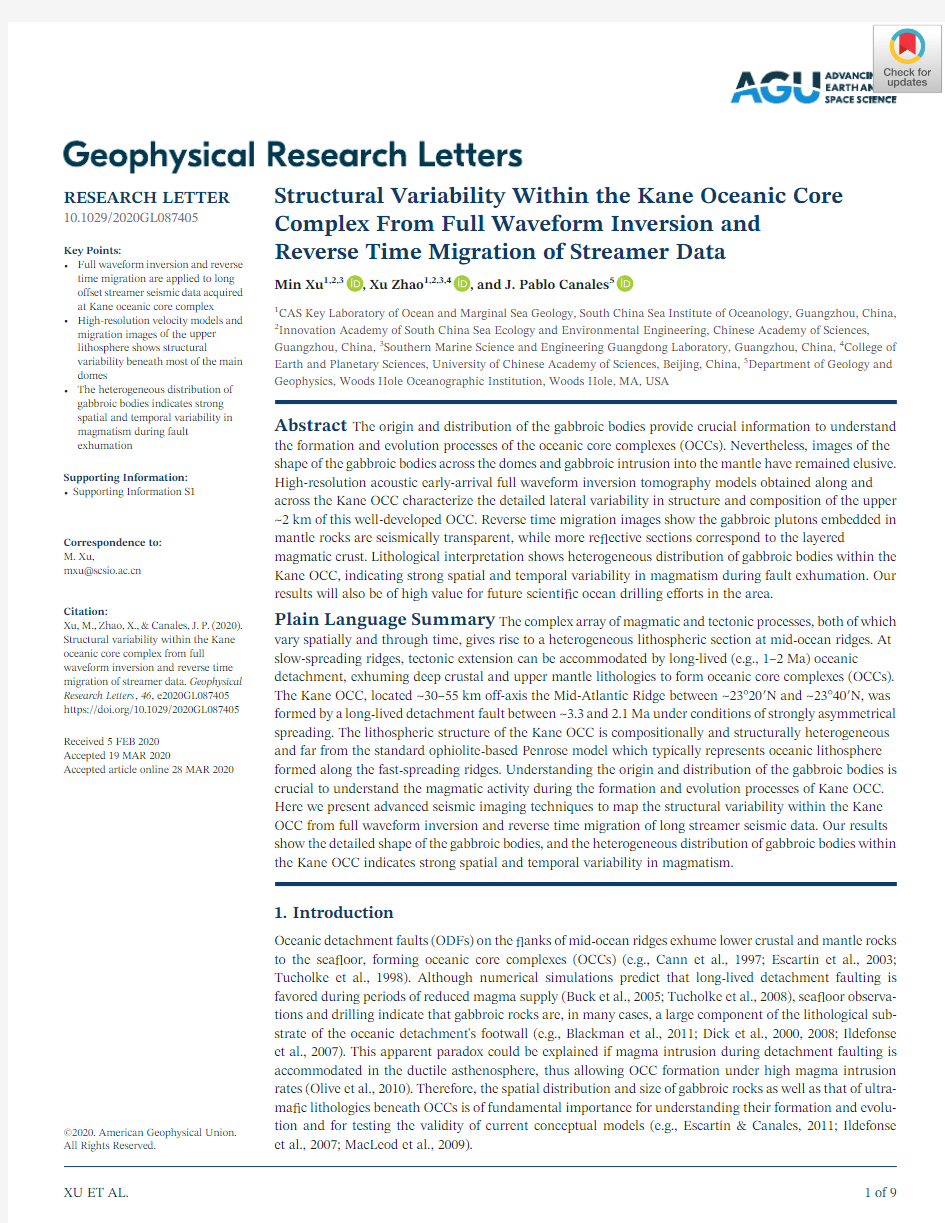
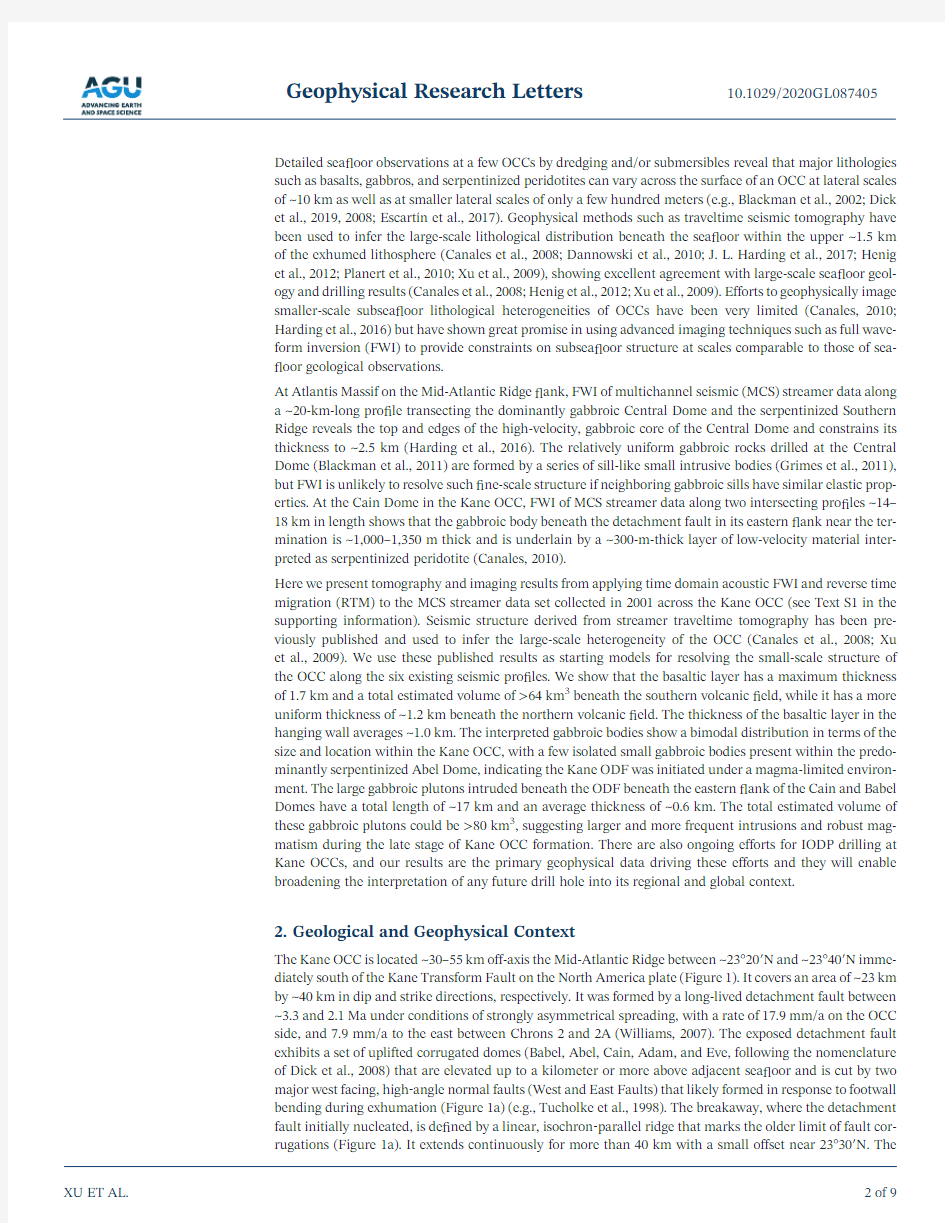
Structural Variability Within the Kane Oceanic Core Complex From Full Waveform Inversion and Reverse Time Migration of Streamer Data Min Xu 1,2,3,Xu Zhao 1,2,3,4,and J.Pablo Canales
51CAS Key Laboratory of Ocean and Marginal Sea Geology,South China Sea Institute of Oceanology,Guangzhou,China,2Innovation Academy of South China Sea Ecology and Environmental Engineering,Chinese Academy of Sciences,Guangzhou,China,3Southern Marine Science and Engineering Guangdong Laboratory,Guangzhou,China,4College of Earth and Planetary Sciences,University of Chinese Academy of Sciences,Beijing,China,5Department of Geology and Geophysics,Woods Hole Oceanographic Institution,Woods Hole,MA,USA Abstract The origin and distribution of the gabbroic bodies provide crucial information to understand the formation and evolution processes of the oceanic core complexes (OCCs).Nevertheless,images of the shape of the gabbroic bodies across the domes and gabbroic intrusion into the mantle have remained elusive.High ‐resolution acoustic early ‐arrival full waveform inversion tomography models obtained along and
across the Kane OCC characterize the detailed lateral variability in structure and composition of the upper
~2km of this well ‐developed OCC.Reverse time migration images show the gabbroic plutons embedded in
mantle rocks are seismically transparent,while more re ?ective sections correspond to the layered
magmatic crust.Lithological interpretation shows heterogeneous distribution of gabbroic bodies within the
Kane OCC,indicating strong spatial and temporal variability in magmatism during fault exhumation.Our
results will also be of high value for future scienti ?c ocean drilling efforts in the area.Plain Language Summary The complex array of magmatic and tectonic processes,both of which vary spatially and through time,gives rise to a heterogeneous lithospheric section at mid ‐ocean ridges.At slow ‐spreading ridges,tectonic extension can be accommodated by long ‐lived (e.g.,1–2Ma)oceanic detachment,exhuming deep crustal and upper mantle lithologies to form oceanic core complexes (OCCs).The Kane OCC,located ~30–55km off ‐axis the Mid ‐Atlantic Ridge between ~23°20′N and ~23°40′N,was formed by a long ‐lived detachment fault between ~3.3and 2.1Ma under conditions of strongly asymmetrical spreading.The lithospheric structure of the Kane OCC is compositionally and structurally heterogeneous and far from the standard ophiolite ‐based Penrose model which typically represents oceanic lithosphere formed along the fast ‐spreading ridges.Understanding the origin and distribution of the gabbroic bodies is
crucial to understand the magmatic activity during the formation and evolution processes of Kane OCC.
Here we present advanced seismic imaging techniques to map the structural variability within the Kane
OCC from full waveform inversion and reverse time migration of long streamer seismic data.Our results
show the detailed shape of the gabbroic bodies,and the heterogeneous distribution of gabbroic bodies within
the Kane OCC indicates strong spatial and temporal variability in magmatism.
1.Introduction
Oceanic detachment faults (ODFs)on the ?anks of mid ‐ocean ridges exhume lower crustal and mantle rocks
to the sea ?oor,forming oceanic core complexes (OCCs)(e.g.,Cann et al.,1997;Escartín et al.,2003;
Tucholke et al.,1998).Although numerical simulations predict that long ‐lived detachment faulting is
favored during periods of reduced magma supply (Buck et al.,2005;Tucholke et al.,2008),sea ?oor observa-
tions and drilling indicate that gabbroic rocks are,in many cases,a large component of the lithological sub-
strate of the oceanic detachment's footwall (e.g.,Blackman et al.,2011;Dick et al.,2000,2008;Ildefonse
et al.,2007).This apparent paradox could be explained if magma intrusion during detachment faulting is
accommodated in the ductile asthenosphere,thus allowing OCC formation under high magma intrusion
rates (Olive et al.,2010).Therefore,the spatial distribution and size of gabbroic rocks as well as that of ultra-
ma ?c lithologies beneath OCCs is of fundamental importance for understanding their formation and evolu-
tion and for testing the validity of current conceptual models (e.g.,Escartín &Canales,2011;Ildefonse
et al.,2007;MacLeod et al.,2009).
?2020.American Geophysical Union.All Rights Reserved.RESEARCH LETTER
10.1029/2020GL087405
Key Points:
?Full waveform inversion and reverse
time migration are applied to long
offset streamer seismic data acquired
at Kane oceanic core complex
?High ‐resolution velocity models and
migration images of the upper
lithosphere shows structural
variability beneath most of the main
domes
?The heterogeneous distribution of
gabbroic bodies indicates strong
spatial and temporal variability in
magmatism during fault
exhumation
Supporting Information:
?Supporting Information S1Correspondence to:M.Xu,mxu@https://www.360docs.net/doc/522606862.html,
Citation:
Xu,M.,Zhao,X.,&Canales,J.P.(2020).
Structural variability within the Kane
oceanic core complex from full
waveform inversion and reverse time
migration of streamer data.Geophysical
Research Letters ,46,e2020GL087405
https://https://www.360docs.net/doc/522606862.html,/10.1029/2020GL087405
Received 5FEB 2020
Accepted 19MAR 2020
Accepted article online 28MAR 2020
Detailed sea?oor observations at a few OCCs by dredging and/or submersibles reveal that major lithologies such as basalts,gabbros,and serpentinized peridotites can vary across the surface of an OCC at lateral scales of~10km as well as at smaller lateral scales of only a few hundred meters(e.g.,Blackman et al.,2002;Dick et al.,2019,2008;Escartín et al.,2017).Geophysical methods such as traveltime seismic tomography have been used to infer the large‐scale lithological distribution beneath the sea?oor within the upper~1.5km of the exhumed lithosphere(Canales et al.,2008;Dannowski et al.,2010;J.L.Harding et al.,2017;Henig et al.,2012;Planert et al.,2010;Xu et al.,2009),showing excellent agreement with large‐scale sea?oor geol-ogy and drilling results(Canales et al.,2008;Henig et al.,2012;Xu et al.,2009).Efforts to geophysically image smaller‐scale subsea?oor lithological heterogeneities of OCCs have been very limited(Canales,2010; Harding et al.,2016)but have shown great promise in using advanced imaging techniques such as full wave-form inversion(FWI)to provide constraints on subsea?oor structure at scales comparable to those of sea-?oor geological observations.
At Atlantis Massif on the Mid‐Atlantic Ridge?ank,FWI of multichannel seismic(MCS)streamer data along a~20‐km‐long pro?le transecting the dominantly gabbroic Central Dome and the serpentinized Southern Ridge reveals the top and edges of the high‐velocity,gabbroic core of the Central Dome and constrains its thickness to~2.5km(Harding et al.,2016).The relatively uniform gabbroic rocks drilled at the Central Dome(Blackman et al.,2011)are formed by a series of sill‐like small intrusive bodies(Grimes et al.,2011), but FWI is unlikely to resolve such?ne‐scale structure if neighboring gabbroic sills have similar elastic prop-erties.At the Cain Dome in the Kane OCC,FWI of MCS streamer data along two intersecting pro?les~14–18km in length shows that the gabbroic body beneath the detachment fault in its eastern?ank near the ter-mination is~1,000–1,350m thick and is underlain by a~300‐m‐thick layer of low‐velocity material inter-preted as serpentinized peridotite(Canales,2010).
Here we present tomography and imaging results from applying time domain acoustic FWI and reverse time migration(RTM)to the MCS streamer data set collected in2001across the Kane OCC(see Text S1in the supporting information).Seismic structure derived from streamer traveltime tomography has been pre-viously published and used to infer the large‐scale heterogeneity of the OCC(Canales et al.,2008;Xu et al.,2009).We use these published results as starting models for resolving the small‐scale structure of the OCC along the six existing seismic pro?les.We show that the basaltic layer has a maximum thickness of1.7km and a total estimated volume of>64km3beneath the southern volcanic?eld,while it has a more uniform thickness of~1.2km beneath the northern volcanic?eld.The thickness of the basaltic layer in the hanging wall averages~1.0km.The interpreted gabbroic bodies show a bimodal distribution in terms of the size and location within the Kane OCC,with a few isolated small gabbroic bodies present within the predo-minantly serpentinized Abel Dome,indicating the Kane ODF was initiated under a magma‐limited environ-ment.The large gabbroic plutons intruded beneath the ODF beneath the eastern?ank of the Cain and Babel Domes have a total length of~17km and an average thickness of~0.6km.The total estimated volume of these gabbroic plutons could be>80km3,suggesting larger and more frequent intrusions and robust mag-matism during the late stage of Kane OCC formation.There are also ongoing efforts for IODP drilling at Kane OCCs,and our results are the primary geophysical data driving these efforts and they will enable broadening the interpretation of any future drill hole into its regional and global context.
2.Geological and Geophysical Context
The Kane OCC is located~30–55km off‐axis the Mid‐Atlantic Ridge between~23°20′N and~23°40′N imme-diately south of the Kane Transform Fault on the North America plate(Figure1).It covers an area of~23km by~40km in dip and strike directions,respectively.It was formed by a long‐lived detachment fault between ~3.3and2.1Ma under conditions of strongly asymmetrical spreading,with a rate of17.9mm/a on the OCC side,and7.9mm/a to the east between Chrons2and2A(Williams,2007).The exposed detachment fault exhibits a set of uplifted corrugated domes(Babel,Abel,Cain,Adam,and Eve,following the nomenclature of Dick et al.,2008)that are elevated up to a kilometer or more above adjacent sea?oor and is cut by two major west facing,high‐angle normal faults(West and East Faults)that likely formed in response to footwall bending during exhumation(Figure1a)(e.g.,Tucholke et al.,1998).The breakaway,where the detachment fault initially nucleated,is de?ned by a linear,isochron‐parallel ridge that marks the older limit of fault cor-rugations(Figure1a).It extends continuously for more than40km with a small offset near23°30′N.The
termination is de ?ned by a valley between the smooth detachment fault surface and the rougher adjacent
terrain of the fossil hanging wall that marks the young limit of fault corrugations (Figure 1a).It sinuously
follows corrugated domes in the detachment surface.
Extensive geological sampling on the Kane OCC has been done by dredge and ROV on R/V Knorr Cruise
180‐2(Dick et al.,2008)and complemented by sampling done along the southern wall of the Kane
Transform Fault (northern wall of the OCC)during submersible dives of the KANAUT Expedition
(Auzende et al.,1994)(Figure 1b).Details of the lithological structure of the Kane OCC are given by Dick
et al.(2008).The geological sampling indicates that mantle peridotites,gabbros,and diabase dikes are
exposed in the detachment footwall and in outward facing high ‐angle normal fault scarps and slide scars
along the East Fault that cut through the detachment.The central and western parts of the Kane OCC are
predominantly ultrama ?c,while both peridotites and gabbros are exposed along the northern edge of the
OCC (e.g.,Dick et al.,2008).Slide scars along the East Fault expose massive outcrops of serpentinized peri-
dotite at the center of the OCC,providing direct evidence of the footwall composition there (Dick et al.,2008).
There are two volcanic ?elds erupted off axis (Figure 1a),which might be related to stress distribution in the
detachment fault as it was exhumed,with lower part in compression and upper part in extension as the foot-
wall rolled over (Parnell ‐Turner et al.,2017).This differential stress could force any melt at depth in the foot-
wall to erupt on the detachment surface and result in sur ?cial volcanism along the active East Fault
(Tucholke et al.,2001).
The subsurface velocity structure shows an excellent agreement with large ‐scale sea ?oor geology and
drilling/diving results (Canales et al.,2008;Xu et al.,2009)(Figure 1b).Areas with low uppermost velocities
near the sea ?oor (<3.4km/s)and low vertical velocity gradients (<~1s ?1)correlate with extrusive volcanics
and hummocky morphology,primarily in the fossil hanging wall and volcanic ?elds.Intermediate shallow
velocities (3.4–4.2km/s)and intermediate ‐velocity gradients (1–3s ?1)correlate with massive outcrops of
serpentinized peridotite,dominating the central (Abel Dome and western half of Cain Dome)and southeast-
ern parts of the OCC.High velocities near the sea ?oor (>4.2km/s)and large ‐velocity gradients (>3s ?1)
are
Figure 1.(a)Shaded ‐relief map of the Kane OCC with simpli ?ed tectonic interpretation (adapted from Dick et al.,2008).Black lines locate seismic pro ?les with shooting direction indicated by arrows.Red stars with shot numbers show positions of two example shot gathers displayed in Figure S1.The black star in the inset shows the study location on the MAR.(b)Interpretation of upper basement composition along the Kane MCS pro ?les based on TT velocity characteristics (Xu et al.,2009).Along the pro ?les,red presents pillow basalts (±sheeted dikes),yellow is dominantly gabbros,and green indicates serpentinized peridotites.Pie diagrams show lithological proportions by weight of samples obtained from ROV/submersible dives and dredges on two cruises:KANAUT Expedition (Auzende et al.,1994)and R/V Knorr Cruise 180‐2(Dick et al.,2008);inset at bottom shows the key to lithology.Dredge,Jason II,and Nautile tracks are shown in red.
interpreted as large gabbro plutons and are mostly present beneath the Babel Dome and eastern half of the Cain Dome(Xu et al.,2009)(Figure1b).
3.Early‐Arrival FWI
After preprocessing(Text S1),we applied acoustic early‐arrival FWI to the MCS data(Text S2).FWI models are derived by fully exploiting kinematic and dynamic information from early arrival seismic refraction data (Figures S1and S2of the supporting information).Our FWI runs were performed from low to high fre-quency in two main steps:6‐8‐10‐15and6‐8‐15‐20Hz.The starting velocity models were the traveltime tomography models of Xu et al.(2009).The in?uence of unrealistically high velocities of the starting models below~2‐km depth was assessed through an FWI test with a modi?ed starting model in which all velocity values larger than7.0km/s are set to7.0km/s for Pro?le K1,and the test gives us con?dence that our results in the upper~2km are robust and not signi?cantly in?uenced by the unconstrained velocities>2km below sea?oor in the starting models(Figure S7).Ricker source wavelets with central frequency of5and8Hz (Figure S3a)were used for the two‐step runs,respectively.The model updates occur not only in the shallow part but also deeper than the?rst‐arrival ray coverage predicted by the traveltime tomography models (Figure2).The validity of the?nal velocity models can be qualitatively assessed by comparing the observed traces with synthetic traces predicted by the traveltime tomography and the?nal FWI velocity models. Although could not?t all the later wiggles,the waveform inversion has been able to?t a signi?cant part of the data,showing a clear improvement in the match between observed and predicted early‐arrival refrac-tions(Figure S6).Checkerboard resolution tests indicate that FWI models can resolve features as small as 0.5km by0.5km(Figure S8h)for most of the models'domain,except at the ends of pro?les where data cov-erage and resolution are poor.In some shallow regions best sampled by the data,features with thicknesses of ~0.25km are resolvable(Figures S8i and S8j).In addition,despite the small drop in mis?t function obtained during the inversions(Figure S9)and the nonuniqueness of the inversions,the large‐scale similarity and clear improvement in the small‐scale structures(Figure S10)and deeper illumination of the FWI models (Figure2)with respect to traveltime tomography models indicate that the?nal models are realistic,and pro-vide con?dence when interpreting them.
The large lateral velocity variations(Figure3)in both strike and dip directions can be seen clearly by display-ing the FWI models relative to a1‐D reference model(Figure S3b).The most striking feature of the FWI models are the high‐velocity bodies beneath the detachment surface at the Cain Dome(the central sections of Dip Pro?les K1,K4,and K8,and the northern two thirds of Strike Pro?le K7),and at the southern end of K5(Figures2and3).These high‐velocity anomalies are embedded within a more intermediate background velocity,which is prevalent near the terminations and western?ank of Cain Dome and beneath the Abel and the Eve Domes.Low‐velocity zones are present primarily in the hanging wall at the eastern ends of the Dip Pro?les K4,K1,and K8and coincident with the sea?oor volcanic?elds,for example,in the northern and southern parts of K6,and the western end of K8.There is also a small patch of low velocities located at the western end of K1.
4.RTM
The RTM images were derived from migrating preprocessed MCS shot gathers(Figure S11)based on high‐?delity FWI models(Figure2)to investigate the structural variability within the Kane OCC(Text S3).To aid interpretation,RTM images(Figure S12)are shown together with P wave velocity variations (relative to a1‐D structure with sea?oor velocity of3.7km/s and vertical velocity gradient of1.75s?1, Figure S3b)to highlight the correlation between velocity variations and the RTM imaged features.The RTM images reveal a geometrically complex re?ection image underlying the OCC domes and extending to across the eastern hanging wall and volcanic?elds,showing distinct re?ectivity patterns in shallow litho-sphere compared to normal crust(Figure3).
Consistent with velocity variations,RTM images show evidence for the heterogeneous structure of the upper lithosphere at Kane OCC,with more re?ective and chaotic image sections correlated to the low‐velocity zones.The low re?ectivity and/or seismically transparent sections are present beneath easternmost Cain Dome along the Dip Pro?les K4and K1,the Pro?les K8,K5,and K6,and at the northern two thirds of K7.The higher and more chaotic re?ectivity,and in some cases with layered re?ectors,subparallel to the
sea ?oor are mostly present in the hanging wall and underneath the interpreted volcanic ?elds.The
transition from transparent domes to chaotic hanging wall is abrupt,as seen near the termination along
Pro ?les K1and K4.In some cases the chaotic re ?ectivity overlaps a more seismically transparent section,
as found along K6and the western ?ank of the Cain Dome along Pro ?les K1and K4.
5.Discussion
The lithospheric structure exhumed by ODFs is far from the standard ophiolite ‐based Penrose model.The
geological interpretation of the traveltime tomography models is basically based on in situ geological sam-
ples and sea ?oor morphology at Kane and Atlantis Massif OCCs,and dominant gabbro recovered from
the 1.4‐km ‐deep IODP Hole U1309D drilled on the ODF of the Atlantis Massif (Blackman et al.,2011).
Our new FWI models (Figure 2)and RTM images (Figure 3)illuminate in great detail the lateral structural
heterogeneity up to ~2km beneath the Kane OCC,providing an opportunity to discuss the thickness of the
volcanic units,size,and thickness of the gabbroic bodies across the domes and to use this information to
quantify magmatism during OCC evolution.Based on the velocity structures,velocity variations,and re ?ec-
tion images,we identify four lithological domain features (Figure 4)in our RTM images:basalt,gabbro,ser-
pentinized mantle,and less altered mantle and/or mantle with variable amounts of gabbro.
5.1.Thickness of the Volcanic Units
FWI models show that low ‐velocity zones are systemically correlated with pillow basalts on top of volcanic
?elds that formed along the East Fault,and volcanic terrain with hummocky morphology in the hanging
wall (Figure 2).RTM images of these low ‐velocity zones show a more re ?ective and chaotic section typical
of slow ‐spreading upper magmatic crust (e.g.,Mutter &Karson,1992)(Figure 3).We thus interpret these
low ‐velocity zones as dominantly extrusive volcanics,with a mixture of an uncertain proportion
of
Figure 2.Two ‐dimensional full waveform inversion models of P wave velocity beneath Kane OCC.Contour interval is 0.5km/s.Pro ?le locations are shown in the inset.Morphological features are labeled at the top of the pro ?les.Thick dashed lines indicate locations of breakaway (B)and termination (T).Thin dashed lines show crossings between dip and strike pro ?les.The limit of the ray coverage for the traveltime tomography inversion is indicated by a solid white line.Models are shown with no vertical exaggeration.
underlying sheeted dikes (Figure 4).The basaltic sections are present along the western part of Pro ?le K8,
both ends of Pro ?le K6,the western part of Pro ?le K1,northern part of Pro ?le K5,and the eastern end of
dip pro ?les in the hanging wall.
Beneath the southern volcanic ?eld,the basaltic layer has a maximum thickness of 1.7km,protruding
downward at the center (at ?5‐km model distance of Pro ?les K8and K6),with a total estimated volume
of at least 64km 3.Beneath the northern Mt.Ararat volcanic ?eld,the basaltic layer has a more uniform
thickness of ~1.2km toward the northern transform wall,and extends shallowly beneath the northwestern
section of Cain Dome (K6)and northern section of Abel Dome (K5).The basaltic layer at the eastern ends of
the dip pro ?les in the hanging wall has an average thickness of ~1.0km and extends further west toward the
elevated domes from north to south (Figure 4).An ~1‐km ‐thick basaltic layer next to the breakaway is
located in the west of Abel Dome along Pro ?le K1(Figure 3),probably corresponding to the volcanic crust
in which the detachment initiated.
5.2.The Shape of the Gabbroic Bodies Across the Domes
New FWI models and RTM images illuminate in great detail the shape of the larger gabbroic bodies beneath
Cain and Babel Domes and show evidence for smaller gabbroic units beneath the other domes (Figure 4).
The interpreted gabbroic bodies show a bimodal distribution in terms of size and location within the
Kane https://www.360docs.net/doc/522606862.html,rge gabbroic bodies are observed beneath the Cain Dome along Pro ?les K4,K1,and K7and
also shown beneath the Babel Dome along Pro ?le K7.Lithological variations from the breakaway to termi-
nation along Dip Pro ?les K4and K1across the Cain Dome are very similar (Figure 4).A large elongated gab-
broic body with an average thickness of ~600m is located beneath the central and eastern sections of Cain
Dome along Pro ?les K4and K1.The size in the dip direction of this large gabbroic body as measured along
pro ?les K4and K1is ~5.5and 10km,respectively.The large gabbroic body beneath the Cain Dome extends
continuously to the north beneath the Babel Dome with a maximum thickness of ~1.0km as imaged
along
Figure 3.RTM images overlain on velocity variations relative to a 1‐D model (Figure S3b).The shaded area indicates data gap of MCS acquisition of Pro ?le K4.Models and images are shown with no vertical exaggeration.Pro ?le locations are indicated in the left inset.The stippled regions were excluded from interpretation.
Pro ?le K7.It is shown as several discrete gabbroic bodies next to each other and has a total along ‐strike length of ~17km.To the south,this gabbroic body does not extend into the Eve Dome.It seems to ?nger southward from the southern ?ank of Cain Dome,as evidenced by the alternating high ‐and intermediate ‐velocity bodies imaged along Pro ?le K8between the termination and ?3‐km model distance.Our new FWI models and RTM images also reveal the presence of several elongated gabbroic bodies of smal-ler size with length of 0.5–3.0km and thickness of 200–500m.Many of these are embedded in a predomi-nately serpentinized peridotite beneath the Abel Dome (K5),western ?ank of Cain Dome (K6),and eastern ?ank of the Cain Dome (K4and K8).Other similarly sized gabbroic bodies are located beneath the volcanic ?elds and hanging wall (K6and K8).
Lithological distribution along the strike pro ?les shows greater variability (Figure 4).A prominent gabbroic body with length of ~2km and thickness of ~0.5km exists at the southern section of Pro ?le K5approaching Adam Dome.Several small isolated gabbroic bodies are decentralized in their distribution with respect to the Abel Dome.Pro ?le K6crosses the two volcanic ?elds to the south and north.Gabbroic bodies are found dis-persed beneath the interpreted basalt,and two smaller ones are located beneath the southern section of Cain Dome.
The southern section of Pro ?le K7shows a slighter smaller velocity and not so transparent seismic image compared to the northern section (Figure 3);thus,we interpret that area as predominantly serpentinized mantle although a mixture of gabbro and serpentinized mantle might be the
case.
Figure 4.Lithological structure interpretations along the seismic pro ?les.Images are shown with no vertical exaggeration.
5.3.Magmatism During OCC Evolution Understanding the origin and distribution of the gabbroic bodies is crucial to determining the formation and evolution processes of OCCs (Ildefonse et al.,2007).The heterogeneous distribution of gabbroic bodies within the Kane OCC (Figure 4)indicates strong spatial and temporal variability in magmatism.
Geological interpretation of Dip Pro ?les K4and K1indicates that mantle was exhumed near the breakaway underneath Abel Dome,suggesting the Kane ODF was formed under a very low magma environment and initially cut through a thin crust.Strike Pro ?le K5,however,shows a strong gabbroic intrusion to the south near the Adam Dome where sea ?oor gabbroic samples were recovered (Dick et al.,2008).Thus,the ODF reached gabbros at shallow depths,indicating robust magmatism beneath Adam Dome.The small isolated gabbroic bodies imbedded in serpentinized peridotite beneath the Abel Dome shown along Pro ?le K5can be attributed to a decreasing melt ?ux to the north,producing smaller,more rapidly crystallized intrusions.Dominantly serpentinized mantle are inferred to be present beneath the western section of the Cain Dome along Pro ?les K4,K1,and K6,suggesting magma ‐limited conditions during the exhumation of the central part of Kane OCC,whereas magmatic sections during this period mostly developed at centers beneath the volcanic ?elds to the south and to the north.
Gabbroic bodies overlaid by volcanic basalts are shown in the western side of Pro ?le K8,and both ends of Pro ?le K6,where irregular,hummocky morphology indicates volcanic ?elds are present (Dick et al.,2008).The spatial association of the volcanic ?elds with East Fault suggests that the magma was extruded along the fault during exhumation of the ODF (Dick et al.,2008),caused by the bending ‐related differential stress (Tucholke et al.,2001).
A signi ?cant change in the distribution of gabbroic bodies happens beneath the Babel Dome and central and eastern sections of Cain Dome.The large gabbroic plutons were intruded beneath the ODF,suggesting robust magmatism during the late stage of Kane OCC formation.This gabbroic unit probably represents lar-ger and more frequent intrusions,with a total estimated volume of >80km 3beneath the Cain and Babel Domes.This late stage northern magmatism is also evidenced by the more elevated sea ?oor in the hanging wall adjacent to Babel Dome and Cain Dome than the Eve Dome.References Auzende,J.‐M.,Cannat,M.,Gente,P.,Henriet,J.P.,Juteau,T.,Karson,J.,et al.(1994).Observation of sections of oceanic crust and mantle cropping out on the southern wall of Kane FZ (N.Atlantic).Terra Nova ,6(2),143–148.https://https://www.360docs.net/doc/522606862.html,/10.1111/j.1365‐3121.1994.tb00647.x Blackman,D.K.,Ildefonse,B.,John,B.E.,Ohara,Y.,Miller,D.J.,Abe,N.,et al.(2011).Drilling constraints on lithospheric accretion and evolution at Atlantis Massif,Mid ‐Atlantic Ridge 30°N.Journal of Geophysical Research ,116,B07103.https://https://www.360docs.net/doc/522606862.html,/10.1029/2010JB007931Blackman,D.K.,Karson,J.A.,Kelley,D.S.,Cann,J.R.,Früh ‐Green,G.L.,Gee,J.S.,et al.(2002).Geology of the Atlantis Massif (Mid ‐Atlantic Ridge,30°N):Implications for the evolution of an ultrama ?c oceanic core complex.Marine Geophysical Researches ,23(5/6),443–469.https://https://www.360docs.net/doc/522606862.html,/10.1023/B:MARI.0000018232.14085.75Buck,W.R.,Lavier,L.L.,&Poliakov,A.N.B.(2005).Modes of faulting at mid ‐ocean ridges.Nature ,434(7034),719–723.https://https://www.360docs.net/doc/522606862.html,/10.1038/nature03358Canales,J.P.(2010).Small ‐scale structure of the Kane Oceanic Core Complex,Mid ‐Atlantic Ridge 23°30′N,from waveform tomography of multichannel seismic data.Geophysical Research Letters ,37,L21305.https://https://www.360docs.net/doc/522606862.html,/10.1029/2010GL044412Canales,J.P.,Tucholke,B.E.,Xu,M.,Collins,J.A.,&Dubois,D.L.(2008).Seismic evidence for large ‐scale compositional heterogeneity of oceanic core complexes.Geochemistry,Geophysics,Geosystems ,9,Q08002.https://https://www.360docs.net/doc/522606862.html,/10.1029/2008GC002009Cann,J.R.,Blackman,D.K.,Smith,D.K.,McAllister,E.,Janssen,B.,Mello,S.,et al.(1997).Corrugated slip surfaces formed at ridge ‐transform intersections on the Mid ‐Atlantic Ridge.Nature ,385(6614),329–332.https://https://www.360docs.net/doc/522606862.html,/10.1038/385329a0Dannowski,A.,Grevemeyer,I.,Ranero,C.R.,Ceuleneer,G.,Maia,M.,Phipps Morgan,J.,&Gente,P.(2010).Seismic structure of an oceanic core complex at the Mid ‐Atlantic Ridge,22°19′N.Journal of Geophysical Research ,115,B07106.https://https://www.360docs.net/doc/522606862.html,/10.1029/2009JB006943Dick,H.J.B.,Kvassnes,A.J.S.,Robinson,P.T.,MacLeod,C.J.,&Kinoshita,H.(2019).The Atlantis Bank Gabbro Massif,Southwest Indian Ridge.Progress in Earth and Planetary Science ,6(1),1–70.https://https://www.360docs.net/doc/522606862.html,/10.1186/s40645‐019‐0307‐9Dick,H.J.B.,Natland,J.H.,Alt,J.C.,Bach,W.,Bideau,D.,Gee,J.S.,et al.(2000).A long in situ section of the lower ocean crust:Results of ODP Leg 176drilling at the Southwest Indian ridge.Earth and Planetary Science Letters ,179(1),31–51.https://https://www.360docs.net/doc/522606862.html,/10.1016/s0012‐821x(00)00102‐3Dick,H.J.B.,Tivey,M.A.,&Tucholke,B.E.(2008).Plutonic foundation of a slow ‐spread ridge segment:Oceanic core complex at Kane Megamullion,23°30′N,45°20′W.Geochemistry,Geophysics,Geosystems ,9,Q05014.https://https://www.360docs.net/doc/522606862.html,/10.1029/2007GC001645Escartín,J.,&Canales,J.P.(2011).Detachments in oceanic lithosphere:Deformation,magmatism,?uid ?ow and ecosystems.AGU Chapman Conference on Oceanic Detachments.Agros,Cyprus,8–15May 2010.Eos,Transactions,American Geophysical Union ,92(4),31.https://https://www.360docs.net/doc/522606862.html,/10.1029/2011EO040003Escartín,J.,Mével,C.,MacLeod,C.J.,&McCaig,A.M.(2003).Constraints on deformation conditions and the origin of oceanic detach-ments:The Mid ‐Atlantic Ridge core complex at 15°45′N.Geochemistry,Geophysics,Geosystems ,4(8),1067.https://https://www.360docs.net/doc/522606862.html,/10.1029/2002GC000472
Acknowledgments Seismic data acquisition was funded by
NSF Grant OCE99‐87004.Data ?les can
be obtained from Interdisciplinary
Earth Data Alliance (IEDA)(https://
https://www.360docs.net/doc/522606862.html,/10.1594/IEDA/314508)
(Tucholke &Collins,20142014).The
velocity models and migrated seismic
sections shown in the paper are freely
available for download from 4TU.
Centre for Research Data (doi:10.4121/
uuid:3ef55160-4a5a-4d1a-b734-
fe2b8d2871ae).Full waveform
inversion was performed with the
software TomoPlus (GeoTomo LLC)
licensed to SCSIO.This research was
supported by the National Natural
Science Foundation of China (41676044
and 91858207)and Special Foundation
for National Science and Technology
Basic Research Program of China
(2018FY100505).M.X.acknowledges
supports from Guangdong NSF
research team project
(2017A030312002),K.C.Wong
Education Foundation (GJTD ‐2018‐13),
Key Special Project for Introduced
Talents Team of Southern Marine
Science and Engineering Guangdong
Laboratory (GML2019ZD0205),and the
Strategic Priority Research Program of
the Chinese Academy of Sciences
(XDA13010105).J.P.C.acknowledges
support from the Independent Research
and Development Program at WHOI.
J.P.Wang and X.R.Mu from China
University of Petroleum are thanked for
helping with the RTM setup.
Escartín,J.,Mével,C.,Petersen,S.,Bonnemains,D.,Cannat,M.,Andreani,M.,et al.(2017).Tectonic structure,evolution,and the nature of oceanic core complexes and their detachment fault zones(13°20′N and13°30′N,Mid Atlantic Ridge).Geochemistry,Geophysics, Geosystems,18,1451–1482.https://https://www.360docs.net/doc/522606862.html,/10.1002/2016GC006775
Grimes,C.B.,Cheadle,M.J.,John,B.E.,Reiners,P.W.,&Wooden,J.L.(2011).Cooling rates and the depth of detachment faulting at oceanic core complexes:Evidence from zircon Pb/U and(U‐Th)/He ages.Geochemistry,Geophysics,Geosystems,12,Q0AG01.
https://https://www.360docs.net/doc/522606862.html,/10.1029/2010GC003391
Harding,A.J.,Arnulf,A.F.,&Blackman,D.K.(2016).Velocity structure near IODP Hole U1309D,Atlantis Massif,from waveform inversion of streamer data and borehole measurements.Geochemistry,Geophysics,Geosystems,17,1990–2014.https://https://www.360docs.net/doc/522606862.html,/10.1002/ 2016GC006312
Harding,J.L.,van Avendonk,H.J.A.,Hayman,N.W.,Grevemeyer,I.,Peirce,C.,&Dannowski,A.(2017).Magmatic‐tectonic conditions for hydrothermal venting on an ultraslow‐spread oceanic core complex.Geology,45(9),839–842.https://https://www.360docs.net/doc/522606862.html,/10.1130/G39045.1 Henig,A.S.,Blackman,D.K.,Harding,A.J.,Canales,J.P.,&Kent,G.M.(2012).Downward continued multi‐channel seismic refraction analysis of Atlantis Massif oceanic core complex,30°N Mid‐Atlantic Ridge.Geochemistry,Geophysics,Geosystems,13, Q0AG07.https://https://www.360docs.net/doc/522606862.html,/10.1029/2012GC004059
Ildefonse,B.,Blackman,D.K.,John,B.E.,Ohara,Y.,Miller,D.J.,MacLeod,C.J.,&Party,I.O.E.S.(2007).Oceanic core complexes and crustal accretion at slow‐spreading ridges.Geology,35(7),623–626.https://https://www.360docs.net/doc/522606862.html,/10.1130/G23531A.1
MacLeod,C.J.,Searle,R.C.,Murton,B.J.,Casey,J.F.,Mallows,C.,Unsworth,S.C.,et al.(2009).Life cycle of oceanic core complexes.
Earth and Planetary Science Letters,287(3–4),333–344.https://https://www.360docs.net/doc/522606862.html,/10.1016/j.epsl.2009.08.016
Mutter,J.C.,&Karson,J.A.(1992).Structural processes at slow‐spreading ridges.Science,257(5070),627–634.https://https://www.360docs.net/doc/522606862.html,/10.1126/ science.257.5070.627
Olive,J.‐A.,Behn,M.D.,&Tucholke,B.E.(2010).The structure of oceanic core complexes controlled by the depth distribution of magma emplacement.Nature Geoscience,3(7),491–495.https://https://www.360docs.net/doc/522606862.html,/10.1038/ngeo888
Parnell‐Turner,R.,Sohn,R.A.,Peirce,C.,Reston,T.J.,MacLeod,C.J.,Searle,R.C.,&Sima,N.M.(2017).Oceanic detachment faults generate compression in extension.Geology,45(10),923–926.https://https://www.360docs.net/doc/522606862.html,/10.1130/G39232.1
Planert,L.,Flueh,E.R.,Tilmann,F.,Grevemeyer,I.,&Reston,T.(2010).Crustal structure of a rifted oceanic core complex and its con-jugate side at the MAR at5°S:Implications for melt extraction during detachment faulting and core complex formation.Geophysical Journal International,181(1),113–126.https://https://www.360docs.net/doc/522606862.html,/10.1111/j.1365‐246X.2010.04504.x
Tucholke,B.E.,Behn,M.D.,Buck,R.,&Lin,J.(2008).The role of melt supply in oceanic detachment faulting and formation of mega-mullions.Geology,36(6),455–458.https://https://www.360docs.net/doc/522606862.html,/10.1130/G24639A.1
Tucholke,B.E.,&Collins,J.A.(2014).Raw multi‐channel seismic shot data from the northern Mid‐Atlantic Ridge acquired during R/V Maurice Ewing expedition EW0102(2001).Interdisciplinary earth data Alliance(IEDA),doi:https://https://www.360docs.net/doc/522606862.html,/10.1594/IEDA/314508. Tucholke,B.E.,Fujioka,K.,Ishitara,T.,Hirth,G.,&Kinoshita,M.(2001).Submersible study of an oceanic megamullion in the central North Atlantic.Journal of Geophysical Research,106(B8),16,145–16,161.https://https://www.360docs.net/doc/522606862.html,/10.1029/2001JB000373
Tucholke,B.E.,Lin,J.,&Kleinrock,M.C.(1998).Megamullions and mullion structure de?ning oceanic metamorphic core complexes on the Mid‐Atlantic ridge.Journal of Geophysical Research,103(B5),9857–9866.https://https://www.360docs.net/doc/522606862.html,/10.1029/98jb00167
Williams,C.M.(2007).Oceanic lithosphere magnetization:Marine magnetic investigations of crustal accretion and tectonic processes in mid‐ocean ridge environments,(Doctoral dissertation).Retreved from Mass.Inst.of Technol.,Woods Hole Oceanogr.Inst.,Woods Hole, Mass.https://https://www.360docs.net/doc/522606862.html,/10.1575/1912/2031.
Xu,M.,Canales,J.P.,Tucholke,B.E.,&Dubois,D.L.(2009).Heterogeneous seismic velocity structure of the upper lithosphere at Kane oceanic core complex,Mid‐Atlantic Ridge.Geochemistry,Geophysics,Geosystems,10,Q10001.https://https://www.360docs.net/doc/522606862.html,/10.1029/2009GC002586
2012-2020上海轨道交通详细规划
上海市轨交最新规划图 轨道交通2012-2020年详细规划图,部分站点名尚未确定。 规划背景及概况 (1) 上海城市轨道交通网络运营现状 目前,上海城市轨道交通已呈现网络化特征,网络效应初步显现。2007年随着―三线两段‖(6号线、8号线一期、9号线一期开通试运营,1号线向北延伸3个车站,4号线实现环线运营)开通后,上海城市轨道交通网络运营线路总数达到8条,运营线路总长度达到235km,覆盖全市13个行政区域,形成了―一条环线、七条射线、九个换乘站、九站共线‖的网络运营格局。建成线路运营情况总体呈现出客流总量逐年增加、客流效益显著提高、运营服务水平逐步提升的特点。近年轨道交通在城市公共交通体系中发挥出了重要作用。 表1 上海轨道交通现状运营线路一览表 序号线路名称线路范围运营线路长度(km) 车站数(座) 1 轨道交通1号线莘庄—富锦路36.9 28 2 轨道交通2号线淞虹路—张江高科24.2 17 3 轨道交通3号线上海南站—江杨北路40.2 28 4 轨道交通4号线环线33.8 17 5 轨道交通5号线莘庄—闵行开发区16. 6 11 6 轨道交通6号线港城路—灵岩南路31.1 27 7 轨道交通8号线市光路—耀华路21.9 21
8 轨道交通9号线松江新城—宜山路30.5 12 合计235 161 (2) 2005年编制的近期建设规划 2005年4月,上海申通地铁集团有限公司组织编制了《上海市轨道交通近期规划》,至2012年,包括已运营的线路长度,上海将形成轨道交通网络规模约567km,见表2。在上述规划基础上,我院编制完成了《上海市城市快速轨道交通近期建设规划环境影响报告书》,并通过了国家环境保护部的审批。 表2 2012年形成的基本网络 线路起讫长度(km) 车站 (座) 1号线莘庄~富锦路37.8 28 2号线徐泾~浦东机场64.0 31 3号线上海南站~江杨北路40.7 29 4号线虹桥路~宝山路22.3 17 5号线莘庄~闵行开发区17.2 11 6号线港城路~济阳路33.1 28 7号线罗店~浦东博览中心45.0 33 8号线市光路~航天公园41.9 32 9号线松江新城~民生路46.3 23 10号线虹桥枢纽~新江湾城36.0 31 11号线北段嘉定~安亭~罗山路66.9 34 11号线南段龙阳路~临港新城59.5 12 12号线七莘路~上川路39.5 31 13号线一期华江路~南京西路16.3 14 合计567 354 (3) 新一轮近期规划的建设项目规模和构成 今年,在原有轨道交通网络规划的基础上,结合―支持城市重点地区开发建设、服务郊区及保障性住房建设、提升对外交通枢纽配套能力、继续支持浦东新区开发开放、完善和加密中心城轨道交通网络‖等原则。上海申通地铁集团有限公司又一次组织编制了近期建设规划,新一轮建设项目在2010-2020年期间共 13项,包括5条延伸线和8条新建线,线路总长合计约310km,车站189座,见表3。至此,2020年上海城市轨道交通网络总规模将达到约 877km,见图1。 表3新一轮近期建设规划建设项目(2010-2020年)
上海城市轨道交通规划
上海城市轨道交通规划 自1863年在英国伦敦出现第一条地下铁道以来,城市轨道成为世界各国解决城市交通问题的首选方案,并在世界40多个国家的130多个城市快速发展。城市交通成为一个国家现代化进程的标尺。 回索历史的胶片,中国的地铁始建于1965年,比世界发达国家晚了整整一个世纪!到二十世纪末,在北京、天津、上海和广州四个已运营的地铁系统中,总长仅80公里,而法国巴黎的地铁即超过300公里。 1958年8月,北京中南海。周恩来总理在一次会上提出:“西方卡不住我们的油脖子,中国也要修地下铁道”。9月,中铁四局集团的前身铁道部北京地下铁道工程局在北京市正式成立,很快就开始了北京地铁一号线的筹建,在西方实施经济技术封锁的情况下,克服重重困难,进行了线路比选、地质钻探、勘测设计、方案研究、施工组织等大量工作,后因三年自然灾害而暂缓施工。1965年3月,中铁四局集团抽调所属第一工程处、地下铁道工程技术研究组、钢筋混凝土预制构件工厂、机械厂筹建组、机械经租站、修配厂及机关部分人员重新组建铁道部北京地下铁道工程局,开始了新中国第一条地铁——北京地铁一号线的艰难困苦的掘进。 步入新世纪,城市轨道交通作为疏通堵塞的唯一选择,成为中国经济增长的新亮点。据悉,中国“十五”期间城市交通投资达8000亿元,其中2000亿元用于地铁建设。城市规划建设地铁和轻轨线路30多条,总长650公里。北京、上海、天津、广州在加速地铁里程的拓展,深圳、南京、青岛、重庆、沈阳、长春、成都和哈尔滨在动工兴建地铁,杭州、大连、兰州、昆明、西安、鞍山、合肥、佛山和乌鲁木齐在积极筹建地铁。首都北京现有地
铁一号线、环线和复八线,总长54公里,已全部贯通运营。全长27.7公里的地铁五号线已动工。北京规划地铁网络12条新线,总长达408公里。 上海地铁发展简史 早在1956年,上海市就开始地铁建设的前期准备,1956年8月,上海市政建设交通办公室向市人委提交《上海市地下铁道初步规划(草案)》,上海地下铁道建设开始提到市领导的议事日程。 1958年8月,上海市地下铁道筹建处成立,以“平战结合”的功能要求,对上海地下铁道开始规划设计、方案论证和试验研究。当时苏联专家断言上海是软土地层,含水量多,因此不宜建设隧道工程。1959年8月,上海警备区领导机关提出:上海地下铁道应以“平战结合、以战为主”的指导思想规划建设,地铁尽可能深埋入基岩层。市地铁筹建处组织科研、大专院校和设计单位,对上海地下铁道的埋设深度作浅、中、深3种方案的研究。对深埋方案探索后认为:如将地铁置于地下300~350米的基岩层,对功能要求、工程技术和建设经济均不合理。 1960年2月,上海市隧道工程局在浦东塘桥开始作盾构掘进试验。 1963年3月,上海市城市建设局隧道处继续在浦东塘桥用直径4.2米盾构,分别在覆土4米和12米处,建成25.2米和37.8米的装配式钢筋混凝土管片衬砌试验隧道,用于验证粉沙性土质和淤混质粘土质中建设隧道的可行性。 1964年11月,上海市委决定结合战备在地铁规划线上的衡山路段实施地铁扩大试验工程。至1967年7月,完成一井一站和600米区间的两条隧道后,因“文化大革命”中止。11年后,地铁试验工程才得以继续,1978年,漕溪路段试验工程批准开工,在漕溪公园的地底下,又尝试了第二条试验隧道的掘进,投资达四千多万人民币,上下行总长1290米。至1983年底,完成一井一站和圆形隧道913米、矩形隧道274米。试验成果:盾构掘进的轴线误差和地表沉陷都可控制在允许的范围之内;隧道用单层装配式钢筋混凝土管片衬砌可满足地铁隧道结构要求,防水达到同期国际标准;初步掌握槽壁地下连续墙的设计与施工技术。细心的乘客可以发觉这段线路采用结构法修筑地下连续水泥墙(方形隧道),与此后采用的盾构掘进(圆形隧道)有明显不同。这段线路现在作为上海轨道交通一号线的正式路线使用。 十一届三中全会后,随着改革开放形势的发展,市区“乘车难”的矛盾日渐突出。1983年初,市基本建设委员会、市科学技术委员会组织有关专家探讨上海的多平面、大容量快速有轨交通工程。4月,市计委向市政府上报《关于建设本市南北快速有轨交通项目建议书》,建议建设南起金山卫、北抵宝山、纵贯南北的快速有轨交通干线,穿越市区的中段为地下铁道。8月,市政府批准项目建议书,并成立上海市南北快速有轨交通线项目筹备组,组织有关单位和国内外专家开展项目的可行性研究。 1985年3月,上海市地铁公司成立,接替上海市南北快速有轨交通线项目筹备组的地铁工程项目可行性研究。1986年7月,市政府向国务院上报建设新龙华至新客站地下铁道的请示报告。8月,国务院批准立项。1988年2月,国务院批准工程可行性研究报告,同时成立上海市地铁工程建设指挥部,组织实施工程建设,由上海市市政工程管理局副局长石礼安兼任指挥。
上海2020年22条轨交规划图
2020年22条轨交规划图 请登录后查看与下载附件... 轨道交通2010-2020年详细规划图,站点名尚未确定。
【新民网讯】上海市环境科学研究院近日通过“上海环境热线”网站对《上海市城市快速轨道交通近期建设规划(2010-2020年)》进行了环境影响评价公示,首次完整披露了上海在2010年—2020年间将新建8条地铁新线、延伸5条既有线路的规划。 该院通过公告表示:本次信息公示后,公众可向指定地址发送电子邮件、电话、信函、面谈等方式发表关于该规划及环评工作的意见看法。在该环评报告书编制过程中和报告基本编制完成,报送审批前,还将采取调查问卷、公众告示等方式进一步征求公众意见。 同时新民网网友也可在本新闻跟帖中提出意见和建议,新民网将整理意见并转交上海市 环境科学研究院。 公示全文如下: 规划背景及概况 (1) 上海城市轨道交通网络运营现状 目前,上海城市轨道交通已呈现网络化特征,网络效应初步显现。2007年随着“三线两段”(6号线、8号线一期、9号线一期开通试运营,1号线向北延伸3个车站,4号线实现环线运营)开通后,上海城市轨道交通网络运营线路总数达到8条,运营线路总长度达到235km,覆盖全市13个行政区域,形成了“一条环线、七条射线、九个换乘站、九站共线”的网络运营格局。建成线路运营情况总体呈现出客流总量逐年增加、客流效益显著提高、运营服务水平逐步提升的特点。近年轨道交通在城市公共交通体系中发挥出了重要作用。
(2) 2005年编制的近期建设规划 2005年4月,上海申通地铁集团有限公司组织编制了《上海市轨道交通近期规划》,至2012年,包括已运营的线路长度,上海将形成轨道交通网络规模约567km,见表2。在上述规划基础上,我院编制完成了《上海市城市快速轨道交通近期建设规划环境影响报告书》, 并通过了国家环境保护部的审批。 (3) 新一轮近期规划的建设项目规模和构成 今年,在原有轨道交通网络规划的基础上,结合“支持城市重点地区开发建设、服务郊区及保障性住房建设、提升对外交通枢纽配套能力、继续支持浦东新区开发开放、完善和加密中心城轨道交通网络”等原则。上海申通地铁集团有限公司又一次组织编制了近期建设规划,新一轮建设项目在2010-2020年期间共13项,包括5条延伸线和8条新建线,线路总
到2020年,上海规划建造地铁线18条
到2020年,上海规划建造地铁线18条。 在2010年世博会之前将建成规模约400公里的运营网络。2012年之前,12号线、13号线也将建成通车,全市轨道交通运营总里程将达到510公里左右。据统计,地铁网络中除浦东新区外,拥有线路数、车站数最多的区域将是徐汇区。 2010年,建成的线路是:1(北延伸到泰和路),2(虹桥临空园区-浦东机场),3(北延伸到铁力路),4,5,6(港城路到主题公园),7(祁连山路外环路到新国际博览中心),8(新江湾城到三林镇和上南路外环线),9(松江新城到杨高路内环线),10(西郊公园到军工路新江湾城)11(嘉定-石龙路)12(漕河泾到高桥)13(金沙江路外环线-汉中路) 其中,1,2,5,9,10,11,13号线还有2010年以后的延伸规划。 上海地铁1号线:莘庄至富锦路。 远期规划向南延伸至金山新城,北延伸至崇明。 途经站点:莘庄(南起讫站)(换乘5号线)-外环路-莲花路-锦江乐园-上海南站(换乘3号线)-漕宝路-上海体育馆(换乘4号线)-徐家汇-衡山路-常熟路-陕西南路-黄陂南路-人民广场(换乘2号线)-新闸路-汉中路-上海火车站(转乘3、4号线)-中山北路-延长路-上海马戏城-汶水路-彭浦新村-共康路-通河新村-呼兰路-共富新村-宝安公路-友谊西路-富锦路(北起讫站) 上海地铁2号线:淞虹路至张江高科。 2号线将西延伸至虹桥机场,东至浦东国际机场。2010年前不延伸到虹桥机场,只到临空园区。 途经站点:淞虹路(西起讫站)-北新泾-威宁路-娄山关路-中山公园(换乘3、4号线)-江苏路-静安寺-南京西路-人民广场(换乘1号线)-南京东路-
上海2020前地铁建设规划图
[编辑本段]开工总表 2010 二号线东延伸段(浦东机场——龙阳路)及西延伸段(淞虹路——诸光路)及七号线北延伸段(锦秋路——罗店)、十号线(新江湾城——虹桥综合交通枢纽东站/航华新村)、十三号线世博段(淮海中路——长清路)通车;十九号线、二十号线、二十一号线开工。 2011 十四号线、十五号线、十六号线、十七号线、十八号线、二十二号线开工。 2012 五号线二期(东川路——海湾大学城)、十一号线二期(江苏路——济阳路)通车。 2013 十二号线(凌空路——友东路)、十三号线(华江路——孙桥)、二十一号线(龙阳路——临港新城)通车。 2015 八号线三期(中国航天科普园——航头)、九号线南段(松江新城——枫泾)及二期东段(民生路——凌空路)、十号线二期(五号沟——新江湾城)、十一号线三期(济阳路——迪斯尼乐园)、十三号线西延伸段(华江路——华新)、十四号线(安亭北站——合庆)、十五号线(锦秋路——南桥新城)、十七号线(共青森林公园——虹桥综合交通枢纽)、十九号线(凌空路——新民/城桥新城)、二十号线(虹桥综合交通枢纽——朱家角)、二十二号线(闵行开发区——虹桥综合交通枢纽)、机场快速轨道交通二期(虹桥综合交通枢纽——龙阳路)通车;沿江线开工。 2016 一号线北延伸段三期(城桥新城——富锦路)及南延伸段二期(莘庄——松江新站)、十八号线(长江西路——航头)通车。 2017 十七号线北延伸段(共青森林公园——吴淞第二邮轮港)、十二号线西延伸段(青浦工业园区——友东路)、六号线北延伸段(高桥滨江森林公园——港城路)通车。 2018年二号线南延伸段(浦东机场——浦东客站)、十六号线(带状环线)通车。 2020年十三号线西延伸段二期(华新——白鹤)、沿江线(新江湾城——关港/友谊河)、机场快速轨道交通西延伸段(青浦站——虹桥综合交通枢纽)及南延伸段(浦东机场——浦东客站)、二十二号线二期(闵行开发区——卫清路) 通车。[1] 近期规划 只列出新建线延伸线不列出 上海轨道交通20号线 上海轨道交通20号线,为上海市2010~2013年(待有关招投标审批程序完成后,初定于2010年上半年开工建设,工期约3年)规划建设的一条重要轨道交通路线。东起虹桥火车站站,沿崧泽大道南侧平行西行跨越A5公路后接转沿盈港东路、盈港路西进青浦城区,进入朱家角地区后走向沿318国道南侧平行至东风路舟,全程33.2公里,含终点站共设11站。目前环评已经完成,红线保留工作基本完成,近期开工可能性极大。在新一轮上海新开工轨道交通建设序列中,该线排序第一。 站数:11站 虹桥火车站(地下换乘车站,与10号、2号线同站不同层面实现零换乘) 徐泾新区站(地下车站,崧泽大道路嘉金高架东侧) 徐泾镇站(高架车站,崧泽大道明珠路口) 方家窑站(高架车站,预留未来其他轨道交通换乘站,盈港东路方黄路口) 赵巷站(高架车站,盈港东路赵重路口) 汇金路站(半下沉广场式车站,华科东路汇金路口) 华青路站(地下车站,盈港路北、华青路以西,夏阳派出所南侧广场) 青浦客运中心站(地下车站,盈港路以北,胜利路以西,上达河以南空地) 雕塑公园站(半下沉广场式车站,朱家角三汾荡)
上海地铁2030官方规划
上海地铁2030官方规划 《上海市城市总体规划(2017-2035年)》正式公布了,其中受人关注的是对未来城市交通建设的规划。未来,上海将形成城际线、市区线、局域线“三个1000公里”的轨道交通网络。 根据规划,到2020年底,上海市轨交运营规模将超过830公里。 ▲最新规划图▼
上海市轨道交通,近期建设规划(2017—2025)项目实施后,车站总数将达到600余座!上海地铁线路将增加到24条线路!届时上海地铁运营规模将超1000公里!之前的环评公示上看,上海地铁还将新增,19号、20号、21号、23号、崇明岛线、嘉闵线、机场联络线等!
规划中的线路, 地铁19号线▼ 轨道交通19号线起自闵行梅陇,终至宝山杨行。主要沿济明路、浦东南路、江杨南路走行。全长约40km,设站30余座。 地铁20号线▼ 轨道交通20号线一期起自上海西站,终至共青森林公园。主要沿交通路、场中路、嫩江路走行。全长约20km,设站10余座。 地铁21号线▼
轨道交通21号线一期起自国际旅游度假区,终至浦东新区高行。上海装修网了解到,该线路主要沿哥白尼路、广兰路、杨高北路、东靖路走行。全长约28km,设站10余座。 地铁22号线▼ 纵贯浦西中外环间的22号线路,可以直接对接20、18号线,串起机场拓展区、静安大宁、普陀桃浦、中环、长宁临空、闵行金虹桥等区域。 地铁23号线▼
轨道交通23号线一期起自闵行开发区,终至徐家汇。线路主要沿东川路、龙吴路走行。全长约29km,设站20余座。 地铁24号线▼ 为贯穿中心城的南北走向线路,主要沿何杨铁路支线、杨树浦路、北洋泾路、锦绣路、陈行公路走行,经杨浦区、浦东新区。 25号线是本次规划中新增的。
2020上海地铁规划线路图
2020上海地铁规划线路图(含地铁线详解) 除了《上海地铁规划2010年版图细说((1-7号线)》和《上海地铁规划2010年版图细说(8-13号)》 里所说的13条地铁线,将在2020之前建设完毕并且投入使用。上海地铁的14-18号线为2020 年的地铁远景规划,预计在2010-2020年间全部建设完成。 上海地铁14号线:即原来的M6线,起讫点为嘉定江桥至陆行。 走向:嘉定江桥由A20东侧起经铜川路、曹杨路、武宁南路、华山路、常熟路、长乐路、金陵东路过江到世纪大道、浦东大道、金桥路、川桥路到陆行(申江路和金穗路之间)结束 设站:陆行、金港路、金沪路、碧云路(和9号线换乘)、云山路(和6号线换乘)、居家桥路、罗山路、源深路(和18号线换乘)、浦东大道(和4号线换乘)、浦东南路、陆家嘴(和2号
线换乘)、豫园(和10号线换乘)、大世界(和8号线换乘)、淮海中路(和13号线换乘)、长乐路(和12号线换乘)、静安寺(和2、7号线换乘)、武定路、武宁路(和13号线换乘)、曹杨路(和3、4,11号线换乘)、真如西村路、真光新村,丰庄,嘉定江桥 上海地铁15号线:即原来的轻轨L1线,起讫点为锦秋路-闵行紫竹圆区。 走向:锦秋路,南大路,西站,沿中环(真北路、北虹路、虹许路、虹梅路)、钦州南路、桂林东街、上海南站、老沪闵路,银都路,到闵行紫竹圆区结束。 设站:闵行紫竹圆区、银都路,莘朱东路、上中路、华东理工大学、上海南站(和1、3号线换乘)、上海师大、钦州南路虹梅路、虹梅路漕宝路(和12号线换乘)、宜山路虹梅路(和9号线换乘)、虹许路(和10号线换乘)、虹古路、北新泾(和2号线换乘)、金沙江路(和13号线换乘)、武宁路、铜川路(和14号线换乘)、上海西站(和11、16号线换乘),南大路,锦秋路 上海地铁16号线:即原来的轻轨L2线,起讫点为吴泾-虹口公园。 走向:吴泾—银都路—莲花路—顾戴路—合川路—龙溪路—淞虹路—万镇路-->上海西站站(11,15)-->交通路站-->新村路站(7)-->志丹路站-->大宁公园站-->上海马戏城站(1)-->广粤路站-->虹口足球场站(3,8) 设站:虹口足球场(和3、8号线换乘)、广粤路、上海马戏城(和1号线换乘)、万荣路、沪太路、新村路(和7号线换乘)、上海西站(和11、15号线换乘)、万镇路,淞虹路,龙溪路,合川路,顾戴路,莲花路,银都路,吴泾 上海地铁17号线:即原来的轻轨L3线,起讫点为虹桥交通枢纽-军工路。 走向:由虹桥交通枢纽出发,经嘉定江桥,南翔,南大路,场中路、万安路、三门路、嫩江路到军工路共青森林公园西南结束。 设站:军工路、嫩江路(和8号线换乘)、民庆路、三门路(和10号线换乘)、同江路(和18号线换乘)、江湾镇(和3号线换乘)、江杨南路、彭浦新村(和1号线换乘)、刘场路、大场东街、场中路大场(和7号线换乘)、南大路,南翔,嘉定江桥,虹桥交通枢纽。 上海地铁18号线(L5):即原来的轻轨L5线,起讫点为共康新村-南汇航头。
上海市城市快速轨道交通近期建设规划(2010-2020年)
上海市城市快速轨道交通近期建设规划(2010-2020年) 环境影响评价公示 发布单位:上海市环境科学研究院 发布日期:2009年4月2日 一、规划背景及概况 (1) 上海城市轨道交通网络运营现状 目前,上海城市轨道交通已呈现网络化特征,网络效应初步显现。2007年随着“三线两段”(6号线、8号线一期、9号线一期开通试运营,1号线向北延伸3个车站,4号线实现环线运营)开通后,上海城市轨道交通网络运营线路总数达到8条,运营线路总长度达到235km,覆盖全市13个行政区域,形成了“一条环线、七条射线、九个换乘站、九站共线”的网络运营格局。 建成线路运营情况总体呈现出客流总量逐年增加、客流效益显著提高、运营服务水平逐步提升的特点。近年轨道交通在城市公共交通体系中发挥出了重要作用。 表1 上海轨道交通现状运营线路一览表 (2) 2005年编制的近期建设规划 2005年4月,上海申通地铁集团有限公司组织编制了《上海市轨道交通近期规划》,至2012年,包括已运营的线路长度,上海将形成轨道交通网络规模约567km,见表2。 在上述规划基础上,我院编制完成了《上海市城市快速轨道交通近期建设规划环境影响报告书》,并通过了国家环境保护部的审批。 表2 2012年形成的基本网络
(3) 新一轮近期规划的建设项目规模和构成 今年,在原有轨道交通网络规划的基础上,结合“支持城市重点地区开发建设、服务郊区及保障性住房建设、提升对外交通枢纽配套能力、继续支持浦东新区开发开放、完善和加密中心城轨道交通网络”等原则。上海申通地铁集团有限公司又一次组织编制了近期建设规划,新一轮建设项目在2010-2020年期间共13项,包括5条延伸线和8条新建线,线路总长合计约310km,车站189座,见表3。至此,2020年上海城市轨道交通网络总规模将达到约877km,见图1。 表3 新一轮近期建设规划建设项目(2010-2020年)
2020年后上海将有21条地铁线路,总里程超过800公里
从原装进口的地铁列车模拟驾驶室、新概念触摸屏列车组装互动游戏,到模拟真实列车调度的全自动列车运行沙盘,都让参观者与上海地铁亲密接触。澎湃新闻记者杨一图国内首座地铁博物馆——上海地铁博物馆10月28日开始试运营。 新落成的上海地铁博物馆位于吴中路1779号,紧邻上海地铁10号线紫藤路站。博物馆总建筑面积5000平方米,其中一期面积为2800平方米。步入序厅,震撼的180度宽屏环幕影片,吸引着参观者进入轨道交通的“精彩世界”。地铁全网规划图显示,在不久的将来,上海地铁将增至21条线路,崇明三岛将和浦东通过轨交连接。2020年左右,上海地铁运营总里程将超过800公里。 上世纪50年代末,轨道交通建设的先驱们就在黄浦江畔的芦苇棚里憧憬着上海的地铁梦。时至今日,上海已形成15条线路(含磁悬浮),567公里、333座地铁站的庞大地铁网络。 未来上海轨道交通网络将持续扩容,馆内的上海地铁全网规划图中除了展示现有地铁线路和已完成规划选线的线路,还首次出现了新线路的编号——19、20、21以及崇明集运等线路。 目前,正在建设的有12号、13号线及9号线3期等,14、15、17、18号等4条新线路正进行着前期工作,这4条线路的建设将持续至2020年前后。 规划显示,2015年上海轨交设计里程将超过600公里,365座车站,轨道交通客运量占公共交通客运量的比重达到50%;2017年设计里程达668公里,车站411座;2020年前后将超过800公里,共509座车站。同时,优化地面公交线网和枢纽布局,实现地面公交和轨道交通的便捷换乘。 在全网规划图上,19号线从浦东榕桥路至崇明三岛,20号线从莘庄至共青森林公园,途径上海西部和北部地区,莘庄站将成为1、5、20号线三线换乘站。21号从龙吴路开始,在上海西站与11、15号线换乘后,呈东西走向至虹口足球场。上海迪士尼除了现在的11号线,还有一条地铁线路通达,就是从2号线的广兰路至迪士尼,全线设7个车站,并在张江路站与13号线换乘。此外还有崇明集运线。 上海最早地铁规划追溯至1956年 地铁博物馆内,一份份泛黄的珍贵地铁史料文件讲述了上海地铁从无到有的历程。馆内最早的档案要属《上海市地下铁道初步规划(草案)》了。1956年8月,上海市人民委员会市政建设交通办公室根据战备要求,向市政府提交《上海市地下铁道初步规划(草案)》,上海地下铁道建设由此开始了重要的征程。这份泛黄的文件至今仍可清晰看到,显示最初上海地铁规划呈环形放射状。主线南北向为漕河泾到吴淞。东西向是西郊公园到黎平路,交叉中心即人民广场。而径线则规划了周家渡——真如,塘桥至大场等。 此外,从原装进口的地铁列车模拟驾驶室、新概念触摸屏列车组装互动游戏,到模拟真实列车调度的全自动列车运行沙盘,都让参观者与上海地铁亲密接触。上海地铁博物馆还首次引进小型5D
上海地铁10号线线路图
上海地铁10号线线路图 上海轨道交通10号线,编号M1,是国内首条无人驾驶轨道交通线,一期由新江湾城站至虹桥火车站,支线在龙溪路站连接支线,抵达航中路站。线路全长36千米,其中龙溪路站以东及支线部分于2010年4月10日先期开通试运营,而主线龙溪路站以西于2010年11月28日开通。第二期将由新江湾城站延伸至基隆路,长10.08公里,共设6站,为上海2010~2020年规划建设线路。由于沿途经过新天地、豫园老城厢、南京路、淮海路、四川路、五角场城市副中心等上海中心区域,因此被称为“白金线路”。 未来发展规划: 浦东东北部的外高桥区域将新增一条通往虹桥枢纽的轨道交通线——日前,市规土局《关于轨道交通10号线(新江湾城-基隆路)选线专项规划》公示,透露了轨道交通10号线将进一步延伸,穿过黄浦江后到达基隆路。 轨道交通10号线是本市轨道交通网络中一条重要的市区级线路,一期工程已经运营通车,全长36.2公里,纵穿杨浦、虹口、黄浦、闸北、徐汇、长宁、闵行等区,并串联起虹桥火车站、虹桥机场等多个客运交通枢纽和大型客流集散点,共设31个车站。 10号线二期工程将由新江湾城出发向东延伸,设国帆路站、双江路站、高桥西站、高桥站、港城路站(换乘6号线)、基隆路站。 10号线未来将新增如下换乘站:港城路站(换乘6号线)
上海市轨道交通10号线二期工程线路起自一期工程终点站新江湾城站北端,沿淞沪路过黄浦江后,再沿港城路至外高桥保税区的基隆路站。线路主要途径杨浦区、浦东新区2个行政区。线路全长约10.080km,其中地下线(盾构)长度3.155km,明挖段长度0.228km,过渡段长度0.337km,高桥段长度6.36km;设站6座,其中地下站1座,高架站5座;设港城路停车场1座;在港城路停车场内设1座主变电所。预计2016年建成。10号线二期工程是10号线的组成部分,是上海轨道交通网络中北部越江通道,连接市中心区和浦东新区,服务于浦东新区北部及杨浦区北部地区,与10号线一期工程贯通运营 更多详情请访问媒力·中国官网:https://www.360docs.net/doc/522606862.html,
2020-2030上海地铁规划图
上海地铁规划图2020、2030年高清及12-21号线线路图 目前中在建的还有12、13、14、21号线,将在2013年建成;世博会结束后,年内还将开工15、17、20号线。远期,中将达到33条线,1700公里规模,并与贯通全市各要点的高速城际铁路统一票务、有效换乘。 2020年规划图(高清) 2030年规划图(高清) 目前上海地铁在建的还有12、13、14、21号线,将在2013年建成;世博会结束后,年内还将开工15、17、20号线。远期,上海地铁规划将达到33条线,1700公里规模,并与贯通全市各要点的高速城际铁路统一票务、有效换乘。 上海地铁14号线:即原来的M6线,起讫点为嘉定( )至陆行。 走向:嘉定江桥由A20东侧起经铜川路、曹杨路、( )南路、华山路、常熟路、长乐路、金陵东路过江到世纪大道、浦东大道、( )路、川桥路到陆行(申江路和金穗路之间)结束 设站:陆行、金港路、金沪路、( )路(和9号线换乘)、云山路(和6号线换乘)、居家桥路、罗山路、源深路(和18号线换乘)、浦东大道(和4号线换乘)、浦东南路、( )(和2号线换乘)、豫园(和10号线换乘)、大世界(和8号线换乘)、淮海中路(和13号线换乘)、长乐路(和12号线换乘)、( )寺(和2、7号线换乘)、武定路、武宁路(和13号线换乘)、曹杨路(和3、4,11号线换乘)、( )路、真光新村,丰庄,嘉定江桥 上海地铁15号线:即原来的轻轨L1线,起讫点为锦秋路-闵行紫竹圆区。 走向:锦秋路,南大路,西站,沿中环(真北路、北虹路、虹许路、虹梅路)、钦州南路、桂林东街、上海南站、老沪闵路,银都路,到闵行紫竹圆区结束。 设站:闵行紫竹圆区、银都路,莘朱东路、上中路、华东理工大学、上海南站(和1、3号线换乘)、上海师大、钦州南路虹梅路、虹梅路漕宝路(和12号线换乘)、宜山路虹梅路(和9号线换乘)、虹许路(和10号线换乘)、虹古路、北新泾(和2号线换乘)、金沙江路(和13号线换乘)、武宁路、铜川路(和14号线换乘)、上海西站(和11、16号线换乘),南大路,锦秋路 上海地铁16号线:即原来的轻轨L2线,起讫点为吴泾-虹口公园。
2010-2020上海轨道交通+最新规划图
2009年4月最新规划图 轨道交通2010-2020年详细规划图,站点名尚未确定。来源:新民网 【新民网讯】上海市环境科学研究院近日通过―上海环境热线‖网站对《上海市城市快速轨道交通近期建设规划(2010-2020年)》进行了环境影响评价公示,首次完整披露了上海在2010年—2020年间将新建8条地铁新线、延伸5条既有线路的规划。 该院通过公告表示:本次信息公示后,公众可向指定地址发送电子邮件、电话、信函、面谈等方式发表关于该规划及环评工作的意见看法。在该环评报告书编制过程中和报告基本编制完成,报送审批前,还将采取调查问卷、公众告示等方式进一步征求公众意见。 同时新民网网友也可在本新闻跟帖中提出意见和建议,新民网将整理意见并转交上海市环境科学研究院。 公示全文如下: 规划背景及概况 (1) 上海城市轨道交通网络运营现状 目前,上海城市轨道交通已呈现网络化特征,网络效应初步显现。2007年随着―三线两段‖(6号线、8号线一期、9号线一期开通试运营,1号线向北延伸3个车站,4号线实现环线运营)开通后,上海城市轨道交通网络运营线路总数达到8条,运营线路总长度达到235km,覆盖全市13个行政区域,形成了―一条环线、七条射线、九个换乘站、九站共线‖的网络运营格局。建成线路运营情况总体呈现出客流总量逐年增加、客流效益显著提高、运营服务水平逐步提升的特点。近年轨道交通在城市公共交通体系中发挥出了重要作用。 表1 上海轨道交通现状运营线路一览表 序号线路名称线路范围运营线路长度(km) 车站数(座) 1 轨道交通1号线莘庄—富锦路36.9 28 2 轨道交通2号线淞虹路—张江高科24.2 17 3 轨道交通3号线上海南站—江杨北路40.2 28 4 轨道交通4号线环线33.8 17
上海轨道交通2017-2025规划重点利好郊区板块分析
上海轨道交通2017-2025规划重点利好郊区板块分析 近十年来,上海的轨道交通建设极大地带动了郊区板块房地产市场的发展和周边配套的成熟。我们通过选取临港新城、嘉定新城、松江新城、罗店以及浦江五个郊区板块开通地铁前后两年的成交数据进行对比发现,地铁在开通前一年之内或者后一年之内对郊区板块的住宅成交量提升显著。根据《上海市轨道交通近期建设规划(2017-2025)》,到2025年上海将拥有轨交线路29条,总长约1050公里,成为中国所有城市中轨道交通里程数最长的城市之一。我们由此统计了预计2017-2025年上海轨道交通建成通车的线路(见下表),并分析每条线路重点利好的郊区板块(首次接通地铁的板块)。由下表可知,2017年底-2025年,轨道交通重点利好的板块有:青浦区沿线板块、曹路板块、南桥新城、华漕北部、吴淞片区、紫竹高新区、上海东站以及崇明等。 上海市轨道交通2017-2025规划重点利好郊区板块一览表 紫竹高新区和上海东站因有多条轨道交通接入,再加上板块内有市级甚至国家级大型规划陆续实施与落地,所以从短中期发展前景来看,这两个板块房地产市场发展潜力较大,本文将重点分析。01紫竹高新区未来规划接入该区域的地铁线路 目前仅地铁5号线东川路靠近紫竹板块,距离紫竹高新区直
线距离约3公里,距离较远,对板块带动作用不明显,另有地铁15号线在建和23号线规划中。 15号线:正在修建中,将设永德路站、紫竹高新区站,预计2020年通车。 23号线:正在规划中,预计将设华泾站、吴泾站、紫竹高新区站,预计2025年通车。 紫竹高新区 紫竹高新园区位于闵行滨江东段,享有先天的7公里滨江岸线资源。在上海市“十三五”规划中,紫竹科学园区被定位成南上海的科创走廊。园区在总体规划上借鉴世界先进发展创新创业园区的运行模式,合理分区、积极开放、广纳高素质人才,努力成为世界一流的科学园区。 至2016年末,紫竹高新区入驻外资企业181家,累计吸引投资总额60.87亿美元,合同引进外资31.08亿美元。园区内初步形成了企业孵化器、加速器、产业承载空间等创业生态体系链,已集聚了一批以“零号湾”为首的具有影响力的市级资质孵化加速机构。目前在孵项目总数达225个,在孵企业近200家,整体项目已融资可公开总额超过14600万元,产业类型包括智能硬件、医疗健康、电子信息、环保及新材料、大数据等四新经济产业。 房地产市场分析 吴泾板块紫竹科学园区坐落于吴泾板块内,另外板块内还有
上海轨道交通10号线线路图
上海轨道交通10号线线路介绍 上海轨道交通10号线,编号M1,是国内首条无人驾驶轨道交通线,一期由新江湾城站至虹桥火车站,支线在龙溪路站连接支线,抵达航中路站。线路全长36千米,其中龙溪路站以东及支线部分于2010年4月10日先期开通试运营,而主线龙溪路站以西于2010年11月28日开通。第二期将由新江湾城站延伸至基隆路,长10.08公里,共设6站,为上海2010~2020年规划建设线路。由于沿途经过新天地、豫园老城厢、南京路、淮海路、四川路、五角场城市副中心等上海中心区域,因此被称为“白金线路”。 未来发展规划: 浦东东北部的外高桥区域将新增一条通往虹桥枢纽的轨道交通线——日前,市规土局《关于轨道交通10号线(新江湾城-基隆路)选线专项规划》公示,透露了轨道交通10号线将进一步延伸,穿过黄浦江后到达基隆路。 轨道交通10号线是本市轨道交通网络中一条重要的市区级线路,一期工程已经运营通车,全长36.2公里,纵穿杨浦、虹口、黄浦、闸北、徐汇、长宁、闵行等区,并串联起虹桥火车站、虹桥机场等多个客运交通枢纽和大型客流集散点,共设31个车站。 10号线二期工程将由新江湾城出发向东延伸,设国帆路站、双江路站、高桥西站、高桥站、港城路站(换乘6号线)、基隆路站。 10号线未来将新增如下换乘站:港城路站(换乘6号线)
上海市轨道交通10号线二期工程线路起自一期工程终点站新江湾城站北端,沿淞沪路过黄浦江后,再沿港城路至外高桥保税区的基隆路站。线路主要途径杨浦区、浦东新区2个行政区。线路全长约10.080km,其中地下线(盾构)长度3.155km,明挖段长度0.228km,过渡段长度0.337km,高桥段长度6.36km;设站6座,其中地下站1座,高架站5座;设港城路停车场1座;在港城路停车场内设1座主变电所。预计2016年建成。10号线二期工程是10号线的组成部分,是上海轨道交通网络中北部越江通道,连接市中心区和浦东新区,服务于浦东新区北部及杨浦区北部地区,与10号线一期工程贯通运营 更多详情请访问媒力·中国官网:https://www.360docs.net/doc/522606862.html,