HIV-1缺损慢病毒载体介导的高效基因转移与抑制HIV-1复制
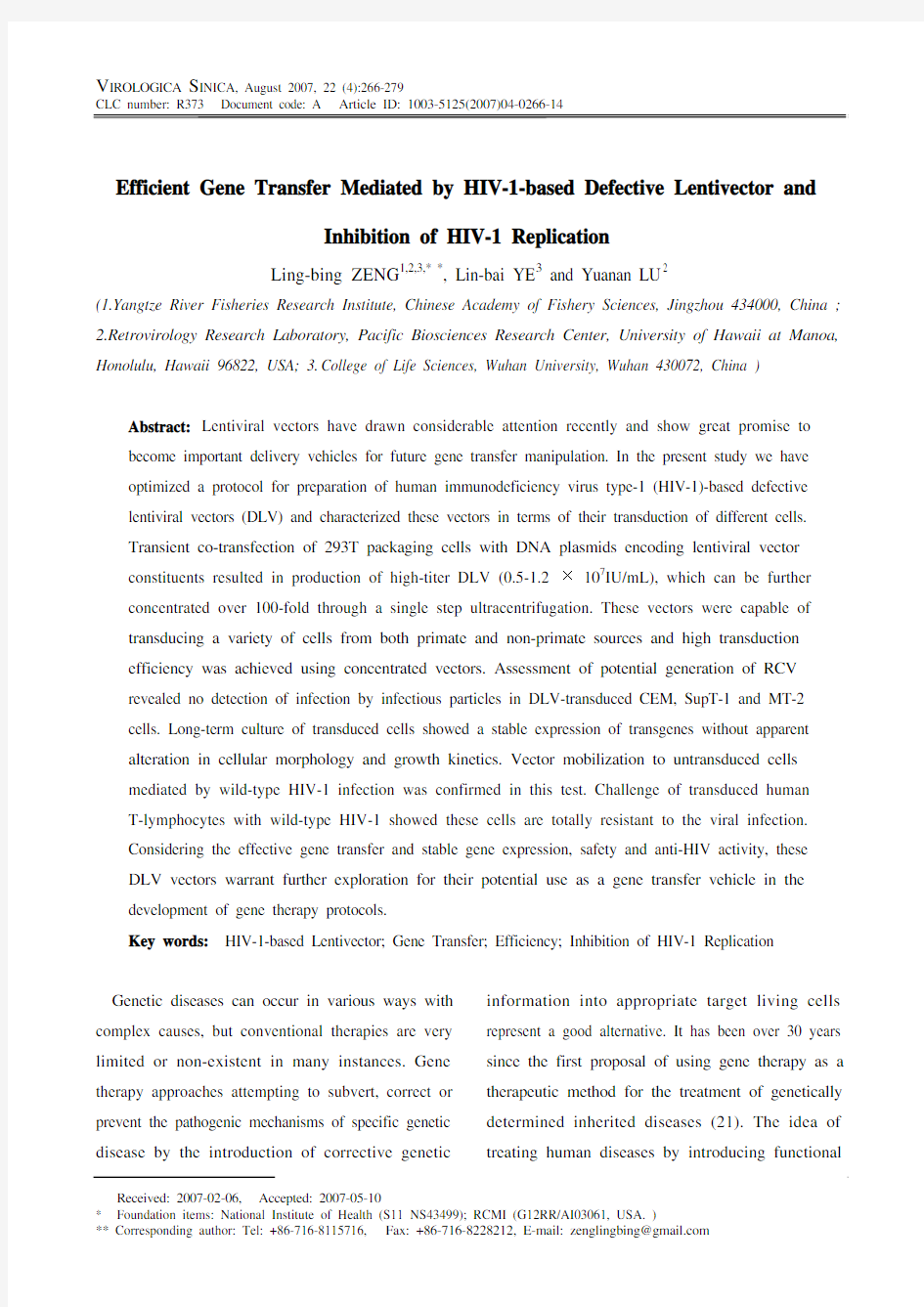

V IROLOGICA S INICA, August 2007, 22 (4):266-279
Received: 2007-02-06, Accepted: 2007-05-10
* Foundation items: National Institute of Health (S11 NS43499); RCMI (G12RR/AI03061, USA. )
** Corresponding author: Tel: +86-716-8115716, Fax: +86-716-8228212, E-mail: zenglingbing@https://www.360docs.net/doc/7418489838.html,
ZENG et al. Efficient Gene Transfer and Inhibition of HIV-1 Replication 267
genes into living cells to achieve therapeutic purpose has now become a clinical reality (3). The first successful attempt of using molecular-based ther- apeutic techniques for the treatment of human recessive hereditary deficiency was documented in 2000 when Cavazzana-Calvo and his co-workers demonstrated they were able to overcome severe combined immunodeficiency (SCID-X1), an X-linked inherited disorder in children, through retrovirus- mediated transfer of complementary DNA containing a gamma c and ex vivo infection of CD34+ cells (8). Successful completion of this clinical gene therapy showed great promise for the use of gene therapy to provide full correction of genetic disease phenotype and associated clinical benefits. Today, human gene therapy represents a promising new form of medicine which is under active development. The potential application of gene transfer technology has now been extended to the treatment of a variety of diseases including infectious diseases, AIDS, neurological disorders and cancer (7, 20, 27, 35).
Successful gene therapy approaches are largely dependent on the development of safe and efficient gene-delivery systems to transport therapeutic genes into the target destination. The vectors, or gene delivery systems, play a crucial role as effective tools for genetic modification of the majority of somatic cells in vitro and in vivo in the development of human gene therapy protocols (12, 25, 42). Vectors derived from a variety of viruses including retroviruses, adenoviruses, adeno-associated viruses, baculoviruses and herpesviruses, are currently being developed and evaluated for their potential use as gene transfer vehicles (27). Among these viral vectors, gene- transfer vectors based on lentiviruses, particularly HIV-1-derived vectors, have been widely used for gene therapy applications since their initial constr- uction in 1991 (36) and the interest in using such systems in applied settings will continue to grow (18, 31, 46). Present studies have shown that HIV-1-based vectors are attractive gene delivery tools due to their relatively large coding capacity, efficient gene transfer, ability to establish long-lasting transgene expression, ability to integrate into genomes of nondividing cells and to inhibit wild type HIV-1 replication in the absence of any anti-HIV-1 insert (2, 4, 5, 11, 42). Because of the potential for possible clinical gene transfer applications in the future, lentiviral vectors mediated gene transfer have been tested for their ability to infect various types of cells in vitro, in vivo and ex vivo, including hepatocytes (19, 41), hematopoietic cells (1, 14), stem cells (30, 40), monocyte-derived dendritic cells (22), lymphocytes (13, 47), monocytes/ macrophages (29, 34, 38), and neurons (33, 44). All these cells are important targets in human gene therapy (4, 25, 35).
To further explore the use of a lentiviral vector as a potential gene transfer tool in human gene therapy, it would be necessary and important to develop an in vitro transfection protocol for consistent production of high yields of vector virus. The objectives of this study are directed towards optimization of in vitro protocols for DLV vector production and vector mediated gene transduction. We have established a method for preparation of high-titer vector and vector concentration through a simple one-step ultracentrifu- gation. Constructed HIV-1-based vectors pseudotyped with a VSV-G envelope protein are highly infectious to human T-cell lines and cells derived from a variety of mammalian species. DLV-mediated gene delivery
268V IROLOGICA S INICA V ol.22, No 4
into human T-cells facilitate a stable and long-term transgene expression and transductant cells become refractory to the infection by wild type HIV-1, suggesting that the transduced cells are protected from HIV-1 infection. We also demonstrated that these vectors are likely to be safe to use since we detected no generation of replication-competent virus (RCV) through vector recombination in transduced cells.
MATERIALS AND METHODS
Plasmids
All three HIV-1-based defective vectors, DHIV (DHIV-Rev+), DHIV-Rev- and DHIV-CTE, encoding selected cis-acting elements,express a reporter gene, green fluorescent protein (GFP), under the control of HIV-1 LTR. The vector, DHIV-Rev+, has deletions that affect structural (gag/pol and env) and accessory genes (vif, vpr, and nef), but contains all cis-acting elements required for vector packaging and tran- sduction (43). The vector, DHIV-Rev-, has a deletion in the second coding exon of Rev in DHIV-Rev+. The rev-independent vector, DHIV-CTE, was produced by replacing the RRE in DHIV-Rev- with the constitutive transport element (CTE) from Moson-Pfizer monkey virus (26, 29). All plasmids were prepared using Qiagen plasmid-Prep kits (Qiagen). DNA yield was determined by measuring the concentration of DNA in the elute by recording the absorbance at 260 nm in a Beckman DU-640 spectrophotometer (Beckman).
Cell cultures
Human T-cell line, CEM (human T4-lympho- blastoid cell line, obtained through the AIDS Research and Reference Reagent Program, Division of AIDS, NIAID, NIH), COS-7 (Africa green monkey kidney cell) and Sup-T1 (Non-Hodgkin’s T-cell lymphoma cells) were propagated in RPMI-1640 medium (Sigma), while 293T (Human embryonic kidney cell), NIH3T3 (Mouse embryo cell), MT-2 (Human T-cell leukemia cell) and PA 317 (Mouse embryo hybridoma cell) cells were maintained in Debecco’s Modified Eagle’s Medium (Sigma) and Vero (Monkey kidney cell), HeLa (Human cervix epithelial adenocarcinoma cell), WI38 (Human lung fibroblast cell) and HTB-14 (Human glioblastoma cell)cells were maintained in Minimum Essential Medium Eagle (Sigma). All these cell cultures were incubated at 37°C with 5% CO2 in proper media supplemented with 10% heat-inactivated fetal bovine serum (FBS) (HyClone), 100U/mL penicillin, 100ug/mL streptomycin, and 0.292mg/mL L-glutamine (Sigma). Cells were observed and documented using a phase-contrast inverted micros- cope (Olympus IX70).
Vector production
HIV-1-based vectors pseudotyped with VSV-G were produced by transiently transfecting human embryonic kidney 293T cells with a packaging construct, a VSV-G envelope construct and a transfer construct containing GFP gene (43). Twenty four hours before transfection, 293T packaging cells at exponential growth phase were subcultured in T-75 flasks (Corning) at a density of 6×106 cells/flask and incubated at 37°C with 5% CO2. The growth medium was replaced with fresh medium 2-3 h prior to the transfection. DNA mixture containing 6.25 μg packaging plasmid, 6.25 μg transfer plasmid and 2.50 μg VSV-G plasmid was prepared per TC-75 cm2 flask and added to the cell monolayer drop-by-drop. Chloroquine solution was added immediately after to yield a final concentration of 25 μM/mL. Eight hours following the transfection, the growth medium was
ZENG et al. Efficient Gene Transfer and Inhibition of HIV-1 Replication 269
replaced with 8 mL/flask of fresh DMEM supplem- ented with 2% FBS. Vector production at post transfection days 1, 2, 3 was titered in CEM cells using 10-fold serial dilution method. Vector titer was determined by visually counting the GFP positive cells of the endpoint dilution using fluorescence micros- copy (23, 28).
Vector concentration
Preparation of large-scale production of HIV-1 based vectors was conducted in TC-150 cm2 flasks (Corning) using the same protocol. 293T packaging cells were seeded at 1.2 - 1.5×107 cells/flask in 25 mL DMEM medium 24 h prior to transfection and 6-10 flasks were used each time. Transfection was conducted using double concentrated DNA mixture and vector produced from different batches were stored at –80°C. To concentrate DLV, vectors stored at –80°C were thawed and pooled. Following a low speed centrifugation (1 800×g) for 30 min at 4°C, recovered supernatant was filtered through a 0.45 μm filter unit to remove cellular debris (Nalgene). Filtrate containing the vector was ultra-centrifuged at 113 000×g for 2.5 h at 4°C using a Beckman SW28 rotor. Vector pellet in each tube was resuspended in 0.2mL RPMI-1640 medium containing no FBS or antibiotics. V ector aliquots (0.1-0.2 mL/tube) were stored at –80°C for future titration and transduction.
Transduction efficiency of DLV in CEM cells
The transduction efficiency of HIV-1-based defective vectors for human T-cells (CEM) was tested using different vector multiplicities of infection (MOI) of 1, 5, 20 and 50. CEM cells at their exponential growth phase were harvested and counted. For DLV trans- duction, 1.0×105 cells were pelleted in a 1.5-mL sterile tube and resuspended with 0.5 mL of diluted DLV stocks. Following 1 hr vector adsorption at the presence of 8 μg/mL polybrene at 37 °C and gently tapping the tube every 15 min, the cells were washed once, and then resuspended with 1.0 mL medium supplemented with 5% heat inactivated FBS and seeded into 2 wells of a 24-well plate (0.5 mL/well). Following seventy-two hours incubation, GFP positive cells were visually counted under a fluorescence microscopy and the transduction efficiency were determined by recording the percentage of the GFP+ cells within a transduced cell population. Transduction with DLV in different cell lines
11 cell lines commonly used for gene transduction including CEM, MT2, Sup-T1, COS-7, PA317, 293T, NIH3T3, HeLa, Vero, WI38 and HTB-14 were tested and compared for their sensitivity to DLV infection in vitro. All the cells were harvested at their exponential growth phase and the concentration was adjusted to 2.0×105 cells/mL. A DLV stock was 10-fold diluted (10-1to10-6) with medium containing 8μg/mL Polybrene. DLV transduction was conducted by resuspending different cell lines with 1.0 mL of the same DLV dilution. Following 1 h adsorption, infected cell suspension was inoculated into a 96-well plate, with 0.1 mL/well cells (2.0×104 cells/well), and 4 wells/dilution/cell line. The 96-well plates were covered with sensitive films and incubated at 37°C with 5% CO2. At post infection day 3, GFP+ cells were visually counted under a fluorescence micros- copy and the sensitivity of these cells to DLV infection was determined by examining the calculated vector titer (averaged of 4 well at endpoint dilution). GFP+ cell Cloning and long-term expression of GFP CEM cells transduced with three HIV-1-based defective vectors were cloned using the limited
270V IROLOGICA S INICA V ol.22, No 4
dilution method. In brief, transduced cells were diluted with the conditioned RPMI growth medium to the concentration of 10 cells/mL, and then inoculated at 0.1 mL/well into a 96-well plate. Following a period of 4-day cultivation at 37°C, GFP positive clones derived from a single cell were identified, transferred into a 12-well plate after 7-10 days and then transferred into TC-25 cm2 flasks (Corning). The long-term expression of GFP gene was determined by microscopical examination of transduced cells at different passage times (1 week to 1.5 year) and/or by PCR and RT-PCR detection.
Assays for replication-competent virus (RCV)
To evaluate if replication-competent virus is generated in transduced cells through vector recombi- nation, two independent methods were used: 1) p24 assay to measure virus production in transduced cells. In brief, medium specimens were collected from DLV-transduced CEM cells at different passages (10~40 passages) and stored at –80°C. RCV gene- ration in the medium was determined by measuring viral p24 antigen production (Coulter, Beckman). 2) Infectivity assay to measure infectious RCV. In this assay, collected medium specimens were filtrated through 0.22 μM filters to remove cellular debris and dead cells. Recovered filtrates were diluted with equal volume of fresh growth medium RPMI-1640 (for CEM and Sup-T1 cells) or DMEM (for MT-2 cells) and then used to infect these indicator cells for a period of at least 1 month. The emergence of GFP positive cells was checked every other day under a fluorescence microscopy.
Inhibition of wild type HIV-1 replication
Three cell populations (including a DLV-transduced GFP+ clone, normal CEM, a mixed cell population containing 1/2 GFP+ clone and 1/2 normal CEM cells) were infected with a same HIV-1 stock (MOI = 0.1) in the presence of 8 μg/mL polybrene. Following 1 h adsorption at 37°C, cells were washed twice with DPBS and seeded into TC-25 cm2 flasks. Syncytial formation induced by HIV-1 infection was examined daily and viral replication was assayed by measuring p24 viral antigen production (ELISA kit from Coulter). Cell viability was determined by trypan blue staining and GFP+ cells were visually counted using fluor- escence microscopy.
Vector mobilization
Measurement of DLV mobilization was conducted in two ways. 1) Direct measurement of the increase of GFP+ cells in a transduced CEM population. DLV- transduced CEM cells and a GFP+ clone were mixed with normal CEM cells to generate two sets of CEM populations containing 10%, 30% and 50% GFP+ cells. These cells together with normal CEM and GFP+ clone were super-infected with wild-type HIV-1 -IIIB at a MOI of 0.1. The vector mobilization was determined by the increase of the number of counted GFP+ cells within the cell population. 2) Infection of normal CEM cells with conditioned medium. Culture supernatant from the above super-infected groups was collected and centrifuged at 1 800 g, 4°C for 30 min, then filtered through a 0.22 μm filter (Costar). Conditioned media were prepared by mixing with these cell-free culture supernatants with fresh medium at the ratio of 1:1. Normal CEM cells were cultured with this conditioned media and the emergence of GFP positive cells were checked by fluorescent microscopy.
RESULTS
ZENG et al. Efficient Gene Transfer and Inhibition of HIV-1 Replication 271
Vector production and concentration
The DLV pseudotyped with the VSV-G envelope protein used in this study was generated in 293T cells according to the well-established calcium phosphate co-precipitation method. Transfection was evidenced by the presence of large percentage of GFP+cells (>70%) at day 1 post-transfection which extended to the whole cell population by day 3. This was accompanied by rapid increase of vector yield from 5.6×106 IU/mL at day 1 to 10.7×106 IU/mL at day 3 (Fig. 1A). To define the vector production by time, vector generated from each post-transfection day was separately quantified. Although many vectors were assembled shortly after transfection (5-7.5×106 IU/mL at day 1), the majority of vectors were produced at day 2 (>10×106 IU/mL) as shown in Fig. 1B. Vector production dropped to less than 6×106 IU/mL at day 3. Because of the cellular fusion and detachment of large percent of transfected cells, vector production was dramatically reduced at transfection day 4 and subsequent days (data not shown).
To define the localization of DLV , we com- paratively analyzed daily production of DLV released
into cell culture medium (cell-free vector) and vectors associated with the packaging cells (cell-associated vector). The majority of assembled DLV (>97.5%) was released in the cell-free form in the cell culture medium while only a small portion of vector (<2.5%) was associated with the packaging cells (Fig. 1B). This led to the exclusion of the cellular pellet to be used for vector isolation and concentration.
To generate high-titer vector stocks, we have established a one-step ultracentrifugation protocol to concentrate vector produced in large-scale or from different batches of viral transfection. As shown in Table 1, the vector virus was effectively concentrated using this method and concentrated vector yielded a final titer up to 9.83±2.25×108 IU/mL. This vector concentration method is relative simple, rapid, easily reproducible, and it allowed a high recovery rate of infectious vector (90%) (Table 1). DLV-mediated transduction efficiency
All three DLV vectors were capable of infecting CEM cells and they shared the same pattern of transduction efficiency, which is largely related to the
concentration of DLV stocks.
Fig. 1. DLV (DHIV-Rev -) production in 293T packaging cell line DLV vector was produced under the same transfection protocol and titration of vector by limited dilution infectivity assay using CEM cells. A: Accumulative DLV production measured at selected post transfection time. B: Comparative analysis of vector produced in cell-free medium supernatant and associated within the 293T packaging cells at different post transfection times. The result of each test represented an average of three experiments.
272V IROLOGICA S INICA V ol.22, No 4
Table 1. DLV production in 293T cells and concentration by ultracentrifugation
Before concentration After concentration
DHIV-Rev- Titers
(IU/mL) V olume
(mL)
Titers
(IU/mL)
V olume
(mL)
Increase in
titer
(×times)
Decrease in
volume
(×times)
Recovery rate
(%)
Test 1 5.0 × 106 500 7.5
× 108 3.0 150.0 166.7 90.0 Test 2 7.5 × 106 415 1.0
× 109 2.7 133.3 153.7 86.7 Test 3 1.0 × 107 430 1.2
× 109 3.0 120.0 143.3 83.7
As shown in Fig. 2, approximately 30% cells were successfully transduced when a low-titer of DLV stock was used (MOI = 1.0). This transduction efficiency increased to about 60% at a MOI of 5 and reached over 80% at a MOI of 20, indicating that the transduction efficiency is directly correlated with DLV titer. More than 90% of CEM cells became GFP+ when a more concentrated DLV preparation (MOI = 50) was used for the transduction. In addition, human glioblastoma cells (HTB-14) available in this labo- ratory were also tested for their susceptibility for DLV transduction. We demonstrated that DLV were highly infectious to HTB-14 cells with a transduction efficiency of 50% at MOI of 1.0 and over 90% at MOI 20 (data not shown). This finding may suggest DLV have the ability to transduce human microglial cells,
Fig. 2. Transduction efficiency with DLV in human T- lymphocyte cell line CEM. Each transduction was conducted with the use of 1.0×105 cells and transduction efficiency was determined at post infection day 3. The result of each test represented an average of two experiments. which are very important target cells of HIV-1 infection in the human central nervous system. Transduction of vectors in different cell lines Infectivity of these DLV to other cell lines were determined and compared by the titration of the same DLV preparation in ten different cells derived from human and other mammalian species. All these cells appeared to be susceptible to DLV infection. However, efficiency of DLV-mediated gene transduction of these cells differed remarkably (>10 000 times). As shown in Fig. 3, human lymphoid cells (CEM, MT-2, SupT1 and HTB-14) were the most sensitive to DLV infection while HeLa, 293T and WI38 cells were comparatively less sensitive, and murine-derived NIH3T3 and PA317 cells were the least sensitive. The ability to infect a wide spectrum of cell types suggests the potential use of these DLV pseudotyped with VSV-G in gene transfer for human and other mammalian species.
Cloning of transduced cells and long-term expression of the GFP gene
Cloning culture of DLV-transduced CEM cells were conducted in 96-well plates using the limited dilution method. The GFP positive cell clones derived from individual transduced cells were cultured with conditioned medium prepared from normal CEM cultures. By days 10-14, clonal cultures formed sizable cell colonies, then were transferred to a 12-well plate for 7-10 days before propagated in TC-25 cm2 flasks. Clonal cells were subcultured more than 60 times in
ZENG et al. Efficient Gene Transfer and Inhibition of HIV-1 Replication 273
vitro in a period of 1.5 years and showed no change in GFP expression in transduced cells (Fig.4A, fluorescence light; 4B, normal light). This long-term
stable transgene expression was also demonstrated by
analyzing nucleic acid extracted from transduced
CEM cells by RT-PCR (Fig. 4C).
Fig. 3. Transduction of different cell lines with DLV. Cells were harvested at their exponential growth phase and adjusted to a concentration of 2.0×105 cells/mL. 1 mL of this cell preparation was pelleted down an Eppendorf tube and for each vector 6 tubes of this cell pellet were prepared. Master preparation of DLV was 10-fold serially diluted (10-1 to 10-6) with serum-free medium containing 8ug/mL Polybrene. Infection of cells with DLV was conducted by resuspending the prepared cell pellet with 1.0 mL DLV preparation from 10-1 to 10-6, separately. Following 1 h adsorption, infected cells was inoculated into a 96-well plate at a concentration of 0.1 mL/well for cells (2.0×104 cells/well) and 4 wells/dilution of vector for each cell line. The 96-well plate was covered with sensitive film and incubated at 37°C with 5% CO 2. At day 3 post infection, GFP+ cells were visually counted and documented under either normal or fluorescent light (Olympus IX 70) by using mounted Olympus digital camera and software MagnaFire. The infectivity of DLV to 11 cells to DLV was determined by comparing vector titer (average of GFP positive cells from
4 wells at the endpoint dilution).
Fig. 4. Stable long-term expression of GFP gene in DLV-transduced CEM cells. A: Photomicrograph of GFP+ transductant CEM cells at passage 60 under fluorescence light; B: Photomicrograph of GFP+ transductant CEM cells at passage 60 under normal light; C: RT-PCR detection of transgene (GFP) expression in transductant CEM cells. Lane 1: 1kb plus DNA ladder (Invitrogen); 2: Total RNA from the 9th passage DLV-transduced CEM; 3: Total RNA fron the 48th DLV-transduced CEM; 4: Untransduced CEM; 5: Negative Control (water); 6: Positive control (plasmid DHIV-Rev-). Gel running: 2% agarose, 50V 90min, EB staining, Bio-Rad FX molecular imager scanning. Target fragment size: 373bp.
274V IROLOGICA S INICA V ol.22, No 4
Generation of replication-competent virus (RCV) The potential for the generation of RCV through vector recombination during vector production, tran- sduction and long-term culture of transduced cells was investigated using both p24 ELISA and viral infectivity assays. We have detected no RCV in all the samples we tested (data not shown). Initially, we examined the conditioned media collected from both early (8-10 passages) and later (>40 passages) cultures of different clones of DLV-transduced CEM cells and p24 antigen was not detected in these clones over a period of 1 year. The medium samples were then tested for infectious virus by inoculating the media to several human T-lymphocytes including CEM, Sup- T1 and MT-2 cells. Following in vitro cultivation for at least one month, these cultures showed neither any GFP+cells nor p24 production. Over a dozen of the transduced cultures were analyzed and generation of RCV was not detected (Data not shown). In addition, the negative detection of RCV was not due to the use of lower DLV preparations since present study included the use of 5 different DLV stocks with an average vector titer of more than 109 IU/mL. Inhibition of wild-type HIV-1 replication and vector mobilization
The lentiviral vector-mediated inhibitory effect on viral replication was demonstrated by challenging transduced CEM with a wild type HIV-1 strain (Fig.
5). When normal CEM was infected with HIV-1, viral replication was detected shortly ( Fig. 5. Inhibitory effect of DLV on HIV-1IIIB replication. Viral infection of normal CEM (CEM-N) was monitored and compared with the same infection conducted in both a DLV-transduced CEM clone (GFP+ clone) and a cell population composing of equal number of normal CEM and GFP+ cells. Data show HIV-1 replication was significantly suppressed in the mixed culture and completely inhibited in the GFP+ clone. ZENG et al. Efficient Gene Transfer and Inhibition of HIV-1 Replication 275 when these clones were challenged with a more concentrated HIV-1 dose (MOI = 1.0). Further analysis of these infected cells revealed that GFP+ clones showed a consistent high percentage of cell viability (>92%) during the course of infection. In contrast, HIV-1 infection resulted in significant drop of cell viability to less than 15% for control CEM at postinfection day 21 as compared to 60% for the mixed population (Fig. 6A). The percentage of GFP+ cells gradually increased from the initial 50% to 80% following HIV-1 infection while no change was observed for the uninfected population (Fig. 6B). These data clearly suggest the resistance of GFP+ cells to HIV-1 infection and possibly vector mobi- lization. To define vector mobilization to untransduced cells, partially transduced CEM cells containing 10%, 30% and 50% GFP+ cells were infected with a low dose of HIV-1 (MOI = 0.01). We observed that the initial percentage of GFP+ cells increased very slowly for the 10% GFP+ population but rapidly increased for the 50% GFP+ group, and reached more than 75% by day 21. Examination of the normal CEM cells cultured with the conditioned media prepared from 50% GFP+ cells infected with HIV-1 revealed the appearance of GFP+ cells (Data not shown). This phenomenon was also observed when Sup-T1 and MT-2 cells were cultured with the cell-free condi- tioned medium. These data indicated the generation of replication-competent DLV in the HIV-1 infected transductant cultures, which confirmed the mobil- zation of DLV . DISCUSSION The application of viral vectors as a gene transfer systems play a crucial role in the development of human gene therapy protocols. Lentiviral vectors based on the HIV-1-genome are emerging as the most attractive and promising vehicles for delivering therapeutic genes into human target cells. These vectors have the ability to efficiently integrate into a wide variety of cells and provide a highly efficient, stable environment for long-term transgene expression. One of major factors affecting DLV-mediated gene transduction of target cells is the production of high titer vector virus. 293T packaging cell line derived from human kidney has been the most widely used vector-producing system for HIV-1-based vector (5,10,17,23,26,29,34,37). To enhance the production of high-titer of DLV in 293T cells, it is very important Fig. 6. Comparative analysis of cell viability and percentage of a transduced CEM cell population (50% GFP+) infected (I) or Uninfected (UI) with HIV-1IIIB . A: Kinetics of cell viability of a 50% GFP+ CEM cells compared to normal CEM cells. B: Percentage of GFP+ cells gradually increased with HIV-1 infection compared to no change for the uninfected cell population. CEM/GFP-I = HIV-1 infected transducatant CEM (50% GFP+) cells, CEM-GFP-UI = Transductant CEM (50% GFP+) cells without HIV-1 infection, CEM-I = normal CEM cells infected with HIV-1. 276V IROLOGICA S INICA V ol.22, No 4 to seed cells at the exponential growth phase at the appropriate density. Our data show that seeding 6.5×106 cells per TC-75 cm2 flask one day prior to the transfection led to the formation of an approximately 70% cell monolayer in the next day. This cell density appeared to be superior to the other two cell seeding densities (50% and 95%) for the production of lentiviral vector. Vector systems based on lentiviruses has been an important tool for human gene therapy. However, use of these vectors for gene transfer was limited for a number of years initially because of several vector- related problems including low vector titers and narrow host ranges. It was reported that the innate envelopes of lentiviruses are much more fragile and attempts to concentrate these vectors through ultracen- trifugation would cause the loss of vector infectivity (1,6). Over time, advances in the understanding of lentiviral vector design and gene transfer have occurred and progress has been made in overcoming the identified limitations. Present studies have shown that vectors based on murine retroviruses and HIV-1 can be pseudotyped with an amphotropic envelope glycoprotein. In particular, the G-protein of vesicular stomatitis virus (VSV-G), a single-stranded RNA virus belonging to the rhabdoviridae family, has been widely used for vector production. Because of much wider spectrum of receptors for VSV-G including ubiquitous anionic phospholipids, the pseudotyped vectors exhibit a much broader host cell range than vectors using the conventional amphotropic env (6, 16, 39). More importantly, because of the physical stability of VSV-G-containing envelopes, it is possible to concentrate pseudotyped vectors by a hundred and thousand fold through ultracentrifugation (1, 6, 10, 29, 45). We have established a protocol for effective concentration of DLV which includes the use of 0.45 μm filters to remove small cellular debris and fragments and the addition of small volume of 50% sucrose which acts as a cushion in the centrifuge tubes to allow the formation of a vector pellet which could be readily resuspended in small volume of buffer. Under these laboratory conditions, we can easily concentrate DLV over 100-fold with the recovery rate as high as 90%. This vector concentration protocol has been routinely performed in this laboratory and the concentrated vector titer is in the range of 0.50-1.25×109 IU/mL. Production of high yield vector is crucially important and essential for human gene therapy because high titer of vector stocks promotes high efficiency of gene transfer. This means that a high percentage of transduced target cells can be readily obtained without going through a long-time drug- selection step. Furthermore, high efficiency of gene transfer is of great importance in human gene therapy since it allows a shortened in vitro cell manipulation time, which would largely reduce the risk of the biological characteristics and function of target cells. We have shown that the efficiency in transducing human lymphoid cells is directly correlated with the titer of vector virus. As we demonstrated in this study, DLV-mediated transduction efficiency reached over 90% at a MOI of 50, compared to only 30% when target cells were transduced with a lower titer of vector stock (MOI = 1). There are several major concerns regarding the use of HIV-1 based vector as gene delivery system, including the possible adverse generation of RCV. These concerns have led to the new design and ZENG et al. Efficient Gene Transfer and Inhibition of HIV-1 Replication 277 production of self-inactivating lentiviral vectors and vectors containing different viral elements with improved safety (32, 43, 48). We have examined the generation of the RCV from five batches of vector production with an average titer of approximately 109 IU/mL; the results showed no RCV were generated. To date, generation of infectious virus has not been reported from second or third generation lentiviral vectors (24), and it is unlikely that we will observe the presence of RCV in the DLV-transduced CEM cells in the 1.5 years duration of this study. Together with the demonstration of unchanged morphology and growth kinetics of transductant cells, our preliminary data suggest the constructed DLV represents a safe gene transfer system (9,17). However, further analysis of these vectors would be necessary using other cell culture systems and animal models prior to clinical testing. Several investigators have recently reported that HIV-1-based defective vectors can efficiently inhibit wild-type HIV-1 replication (2, 4, 5, 11, 42). In particular, this occurs when the vectors do not encode any specifically designed antiviral insert. We have demonstrated stable transduction of various cell types with HIV-1-based vectors. Analysis of transductant CEM cells showed that they are refractory to HIV-1 infection; this was observed by monitoring p24 production, syncytial formation and cell viability counting. Potential mechanisms of this DLV-mediated anti-HIV-1 effect include TAR and RRE decoy effects of the vectors, the competition of vectors for the substrate necessary for reverse transcription and RNA encapsidation (2, 5, 15). Another possible reason for the inhibition of HIV-1 replication involves the formation of defective HIV/vector recombinants caused by the dimerization of HIV-1 and vector RNAs at the dimmer linkage structure (DLS). The defective heterodimers could be packaged into virions and responsible for HIV-1 inhibition by either competing for the packaging or, later, interfering with the strand transfer (34). All these suggest the occurrence of some fatal changes in virus infectivity after the wild-type HIV-1 enters the transduced cells. In addition, demonstration of vector mobilization indicated the successful package of defective vector genome in the newly assembled virus particles transduced cells, either in heterologous HIV/vector recombinant RNA or in homologous vector/vector RNA. Because of the relatively smaller size, it is possible that defective vector genome is somewhat more preferably packaged in encapsulation. Thus the transduced cells could serve as a filter to remove the toxicity of the wild-type HIV-1(5, 26). Another interesting property of the vectors is their ability to be trafficked by wild-type HIV-1 to untransduced cells (26). These HIV-1-based vectors are engineered to contain cis-acting elements neces- sary for replication and packaging (i.e., the long terminal repeats and the primer binding site). Therefore, they have the potential to be packaged into the HIV-1 capsid and envelope to form new virus particles with the vector genome. We have employed two methods to determine the vector mobilization and demonstrated the trafficking of DLV into the untransduced cells following a low-dose HIV-1 infection. This property may prove to be extremely useful for the purpose of HIV-1 gene therapy which allows the protection of a large number of target cells by initially transducing a small number of cells. In conclusion, considering the high transduction 278V IROLOGICA S INICA V ol.22, No 4 efficiency in various cell types, stable transgene expression, no generation of RCV, and anti-HIV activity, these newly constructed HIV-1-based vectors are represent a valuable gene transfer system for human gene therapy. We are currently characterizing these vectors interaction with wild-type virus to understand the molecular mechanisms of their anti- HIV-1 effect. Acknowledgment We thank Dr. Vivek Nerurkar, the director of Retrovirology Research Laboratory, PBRC, UH and all other staffs and faculties at RRL for their kind help with our researches.This work was supported by a grant from National Health Institute, USA (S11 NS43499) and RCMI, USA (G12RR/AI03061) and conducted at Retrovirology Research Laboratory, Pacific Biosciences Research Center, University of Hawaii at Manoa, USA. References 1.Akkina R K, Walton R M, Chen M L, et al. 1996. High-efficiency gene transfer into CD34+ cells with a human immunodeficiency virus type 1-based retroviral vector pseudotyped with vesicular stomatitis virus envelope glycoprotein G. J Virol, 70: 2581-2585. 2.An D S, Morizono K, Li Q, et al. 1999. An inducible human immunodeficiency virus type 1 (HIV-1) vector which effectively suppresses HIV-1 replication. J Virol, 73: 7671-7677. 3.Anderson W F. 1998. Human gene therapy. Nature, 392: 25-30. 4.Buchschacher G L, Wong-stall F. 2000. Development of lentiviral vectors for gene therapy for human diseases. Blood, 95: 2499-2504. 5.Bukovsky A A, Song J P, Naldini L. 1999. Interaction of human immunodeficiency virus-derived vectors with wild-type virus in transduced cells. J Virol, 73: 7087-7092. 6.Burns J C, Friedmann T, Driever W, et al. 1993. Vesicular stomatitis virus G glycoprotein pseudotyped retroviral vectors: concentration to very high titer and efficient gene transfer into mammalian and nonmammalian cells. Proc Natl Acad Sci USA, 90: 8033-8037. 7.Cai K, Sham M K, Tam P, et al. 2003. Lung cancer gene therapy. Gene Ther Mol Biol, 7: 255-272. 8.Cavazzana-Calvo M, Hacein-Bey S, de Saint Basile G, et al. 2000. Gene therapy of human severe combined immunodeficiency (SCID)-X1 disease. Science, 288: 669- 672. 9.Chang L, Urlacher V, Iwakuma T, et al. 1999. Efficacy and safety analyses of a recombinant human immunode- ficiency virus 1 derived vector system. Gene Ther, 6: 715- 728. 10.Coleman J E, Huentelman M J, Kasparov S, et al. 2003. Efficient large-scale production and concentration of HIV-1 -based lentiviral vectors for use in vivo. Physiol Genomics, 12: 221-228. 11.Corbeau P, Wong-staal F. 1996. Anti-HIV effects of HIV vectors. Virology, 243: 268-274. 12.Costantini L C, Bakowska J C, Breakefield X O, et al. 2000. Gene therapy in the CNS. Gene Ther, 7: 93-109. 13.Costello E, Munoz M, Buetti E, et al. 2002. Gene transfer into stimulated and unstimulated T lymphocytes by HIV-1 -derived lentiviral vectors. Gene Ther, 7: 596-604. 14.Deola S, Scaramuzza S, Birolo R S, et al. 2004. Mobilized blood CD34+ cells transduced and selected with a clinically applicable protocol reconstitute lymphopoiesis in SCID-hu mice. Hum Gene Ther, 15: 305-311. 15.Dropulic B, Hermankova M, Pitha P A. 1996. A conditionally replicating HIV-1 vector interferes with wild-type HIV-1 replication and spread. Proc Natl Acad Sci USA, 93: 11103-11108. 16.Emi N, Friedmann T, Yee J K. 1991. Pseudotype formation of murine leukemia virus with the G protein of vesicular stomatitis virus. J Virol, 65: 1202-1207. 17.Escarpe P, Zayek N, Chin P, et al. 2003. Development of a sensitive assay for detection of replication-competent recombinant lentivirus in large-scale HIV-based vector preparation. Mol Ther, 8: 332-341. 18.Federico M. 1999. Lentiviruses as gene delivery vectors. Curr Opin Biotechnol, 10: 448-453. 19.Follenzi A, Battaglia M, Lombardo A, et al. 2004. Targeting lentiviral vector expression to hepatocytes limits transgene-specific immune response and establishes long- term expression of human antihemophilic factor IX in mice. Blood, 103: 3700-3709. ZENG et al. Efficient Gene Transfer and Inhibition of HIV-1 Replication 279 20.Follenzi A, Gupta S. 2004. The promise of lentiviral gene therapy for liver cancer. J Hepatol, 40: 337-240. 21.Friedmann T, Roblin R. 1972. Gene therapy for human genetic disease. Science, 175: 949-955. 22.Gruber A, Kan-Mitchell J, Kuhen K L, et al. 2000. Dendritic cells transduced by multiply deleted HIV-1 vectors exhibit normal phenotypes and functions and elicit an HIV-specific cytotoxic T-lymphocyte response in vitro. Blood, 96: 1327-1333. 23.Ikeda Y, Taceuchi Y, Martin F, et al. 2003. Continuous high-titer HIV-1 vector production. Nat Biotechnol, 21: 569-572. 24.Kappes J C, Wu X. 2003. Safety consideration in vector development. Somat Cell Mol Genet, 26:147-158. 25.Kay M A, Glorioso J C, Naldini L. 2001. Viral vectors for gene therapy: the art of tuning infectious agents into vehicles of therapeutics. Nat Med, 7: 33-40. 26.Klimatcheva E, Planelles V, Day S L, et al. 2001. Defective lentiviral vectors are efficiently trafficked by HIV-1 and inhibit its replication. Mol Ther, 3: 928-939. 27.Kootstra N A, Verma I M. 2003. Gene therapy with viral vectors. Annu Rev Pharmacol Toxicol, 43: 413-439. 28.Kwon Y J, Hung G, Anderson W F, et al. 2003. Determination of infectious retrovirus concentration from colony-forming assay with quantitative analysis. J Virol, 77: 5712-5720. 29.Lu Y, Liu C, Zeng L, et al. 2003. Effficient gene transfer into human monocytes-derived macrophages using defective lentiviral vectors. Cell Mol Biol, 49: 1151-1156. 30.Ma Y, Ramizane A, Lewis R, et al. 2003. High-level sustained transgene expression in human embryonic stem cells using lentiviral vectors. Stem Cells, 21: 111-117. 31.Mautino M R. 2002. Lentiviral vectors for gene therapy of HIV-1 infection. Curr Gene Ther, 2:3-43. 32.Miyoshi H, Blomer U, Takahashi M, et al. 1998. Development of a self-inactivating lentiviral vector. J Virol, 72: 8150-5157. 33.Naldini L, Blomer U, Gallay P, et al. 1996. In vivo gene delivery and stable transduction of nondividing cells by a lentiviral vector. Science, 272: 263-267. 34.Neil S, Martin F, Ikeda Y, et al. 2001. Postentry restriction to human immunodeficiency virus-based vector trans- duction in human monocytes. J Virol, 75: 5448-5456. 35.Nobel G J. 2004. Genetic, cellular and immune approaches to disease therapy: past and future. Nat Med, 10: 135-141. 36.Poznansky M, Lever A, Bergeron L, et al. 1991. Gene transfer into human lymphocytes by a defective human immunodeficiency virus type 1 vetcor. J Virol, 65: 532- 536. 37.Reiser J. 2000. Production and concentration of pseudotyped HIV-1-based gene transfer vectors. Gene Ther, 7: 910-913. 38.Schroers R, Sinha I, Segall H, et al. 2000. Transduction of human PBMC-derived dendritic cells and macrophages by an HIV-1-based lentiviral vector system. Mol Ther, 1: 171- 179. 39.Sharma S, Cantwell M, Kipps T J, et al. 1996. Effect infectious of a human T-cell line and of human primary peripheral blood leukocytes with a pseudotyped retrovirus vector. Proc Natl Acad Sci USA, 93: 11842-11847. 40.Sutton R E, Wu H T M, Rigg R, et al. 1998. Human immunodeficiency virus type 1 vectors efficiently trans- duced human hematopoietic stem cells. J Virol, 72: 5781-5788. 41.Tendeloo V F I V, Broeckhoven C V, Berneman Z N. 2001. Gene therapy: principles and applications to hematopoietic cells. Leukemia. 15: 523-544. 42.Verma I M, Somia N. 1997. Gene therapy-promises, problems and prospects. Nature, 389: 239-242. 43.White S M, Renda M, Nam N Y, et al. 1999. Lentivirus vectors using human and simian immunodeficiency virus elements. J Virol, 73: 2832-2840. 44.Wong L F, Azzouz M, Walmsley L E, et al. 2004. Transduction patterns of pseudotyped lentiviral vectors in the nervous system. Mol Ther, 9: 101-111. 45.Yee J K, Friedmann T, Burns J C. 1994. Generation of high-titer pseudotyped retroviral vector with very broad host range. Methods Cell Biol, 43: 99-112. 46.Zaia J A. 2003. Problems and solutions to successful gene-transfer based therapies for HIV. Clin Appl Immu Rev, 10: 1-13. 47.Zhang Y, Lu H, Liwang P, et al. 2003. Optimization of gene expression in nonactivated circulating lymphocytes. Mol Ther, 8: 629-636. 48.Zufferey R, Dull T, Mandel R J, et al. 1998. Self-inactivating lentivirus vector for safe and efficient in vivo gene delivery. J Virol, 72: 9873-9880. 浅析非病毒载体基因转移技术的现状和展望 摘要:目前基因治疗已经成为科学家治疗多种难治性疾病的一种新手段,基因导入技术是基因治疗的核心也是最基本的技术。目前研究较多的基因导入技术共分为两大类:一,病毒载体基因导入法;二,非病毒载体基因导入法。前者转染效率高,但存在安全性和免疫原性等问题。因此,近年来人们对非病毒类载体系统给予了更多的关注。 关键词:非病毒载体基因转移技术现状和展望 非病毒载体基因转移方法又分为物理方法和化学方法。物理方法如:注射法、基因枪法、电穿孔法、超声波法等都是借助物理力量穿透细胞膜达到基因转移的目的;化学方法则是借助天然的或者人工合成的化合物辅助完成基因转移。尽管近年来在非病毒基因转移领域中取得了显著成效,但总体而言,非病毒载体相对于病毒载体来说转移基因的效率要低,在体内的基因转移尤其如此。现在把目前较常用的非病毒载体基因转移方法的优势和局限综述如下。 1 物理方法 就是基于物理力量造成细胞膜的瞬间缺损,从而使质粒DNA进入细胞内的方法。如基因枪法、电穿孔法、超声波法等,还有近年来发现的激光相关辅助方法。 注射法 直接将质粒DNA注射入组织细胞中达到基因转移的目的。有学者成功地将裸露的质粒DNA注射入肌肉、肝脏、皮肤等组织,但基因表达水平较低。注射法中,细胞表面的某些受体起了一定的作用,它们能够特异或者非特异性地结合DNA并且介导DNA的内吞,但这些受体的详细作用机制不甚清楚。由于注射法有其独特优点如:方法简单,不需特殊试剂且毒性低而受到欢迎。此外,借助显微操作系统进行的显微注射法是目前国际上公认的制备转基因和基因剔除动物模 型的首选。 基因枪法 基因枪法是一种全新的基因导入技术,它以压缩气体(氦或氮)转换成的气体冲击波为动力,把附着于高速微弹上的DNA直接射入细胞、组织和细胞器,基因枪导入的基因被证明可在广泛类型的细胞中得到瞬时的、高效率的表达。基因枪法是皮肤、黏膜以及手术局部暴露组织较理想的基因转移方法,因而基因枪被认为是将来DNA疫苗的良好免疫工具。但是基因枪法用于基因治疗还需要进一步改进,如通过对微弹颗粒表面结构的改良使其可以结合更多的DNA或者使结合 LentiCRISPRv2 and lentiGuide-Puro: lentiviral CRISPR/Cas9 and single guide RNA CRISPR (C lustered R egularly I nterspaced S hort P alindromic R epeats) is a microbial nuclease system involved in defense against invading phages and plasmids. CRISPR loci in microbial hosts contain a combination of CRISPR-associated (Cas) genes as well as non-coding RNA elements capable of programming the specificity of the CRISPR-mediated nucleic acid cleavage. Lentiviral CRISPR/Cas can infect a broad variety of mammalian cells by co-expressing a mammalian codon-optimized Cas9 nuclease along with a single guide RNA (sgRNA) to facilitate genome editing (Shalem*, Sanjana*, et al., Science 2014). Protocols for cloning into the lentiviral transfer plasmid and general considerations for producing lentivirus are described below. Separate protocols are available for amplifying the genome-scale CRISPR knock-out (GeCKO) libraries. This protocol is for creating individual lentiviral CRISPR plasmids targeting a single genomic locus. lentiCRISPRv2 (one vector system): This plasmid contains two expression cassettes, hSpCas9 and the chimeric guide RNA. The vector can be digested using BsmB I, and a pair of annealed oligos can be cloned into the single guide RNA scaffold. The oligos are designed based on the target site sequence (20bp) and needs to be flanked on the 3' end by a 3bp NGG PAM sequence, as shown on the next page. lentiGuide-Puro (two vector system): This plasmid expressed only the chimeric guide RNA. It does not contain Cas9. Please use lentiCas9-Blast (a separate lentiviral construct that delivers hSpCas9 and blasticidin resistance) to first integrate Cas9 into your cell line. The lentiGuide-Puro vector can be digested using BsmB I, and a pair of annealed oligos can be cloned into the single guide RNA scaffold. The oligos are designed based on the target site sequence (20bp) and needs to be flanked on the 3' end by a 3bp NGG PAM sequence, as shown on the next page. Which vector to use: lentiCRISPRv2 is identical to the original lentiCRISPRv1 but produces nearly 10X higher titer virus. lentiGuide-Puro produces >100X higher titer virus over lentiCRISPRv1 and should be used in cell lines where Cas9 has already been integrated in (e.g. using the separate lentiCas9-Blast lentivirus). For applications where Cas9 cannot first be introduced (e.g. primary cells), lentiCRISPRv2 is recommended. After transduction, use puromycin to select for cells with lentiCRISPRv2 or lentiGuide-Puro. Lentiviral production: Before starting any lentiviral work, please ensure compliance with your Environmental Health and Safety office and government/organization/university. Briefly, to make lentivirus, a transfer plasmid (e.g. lentiCRISPRv2 or lentiGuide-Puro) must be co-transfected into HEK293(F)T cells with the packaging plasmids pVSVg (AddGene 8454) and psPAX2 (AddGene 12260). As a positive control for viral production, we often use a CMV-EGFP lentiviral transfer plasmid (eg. AddGene 19319). Target design notes and online resources: For application of Cas9 for site-specific genome editing in eukaryotic cells and organisms, we have computationally identified suitable target sites for the S. pyogenes Cas9 and calculated most likely off-targets within the genome. Please visit https://www.360docs.net/doc/7418489838.html, to access these Cas9 target design tools. Complete plasmid sequences, protocols, a discussion forum and additional information can be found at the Zhang Lab GeCKO website: https://www.360docs.net/doc/7418489838.html,/gecko/ . Citation: Please reference the following publications for the use of this material. Improved lentiviral vectors and genome-wide libraries for CRISPR screening. Sanjana NE*, Shalem O*, Zhang F. Nature Methods (2014). Genome-scale CRISPR-Cas9 knockout screening in human cells. Shalem O*, Sanjana NE*, Hartenian E, Shi X, Scott DA, Mikkelsen T, Heckl D, Ebert BL, Root DE, Doench JG, Zhang F (2014). Science, 343, 83-7. DOI: 10.1126/science.1247005 过表达慢病毒载体构建和包装手册 Version1.0 吉凯基因 二零一一年五月 目录 简介 (3) 第一部分过表达慢病毒载体的制备 实验流程 (4) 实验材料 (5) 过表达克隆制备 (6) 第二部分慢病毒包装与滴度检测 实验流程 (17) 实验材料 (18) L e n t i v i r u s病毒包装 (21) 病毒的收获及浓缩 (22) L e n t i v i r u s滴度测定 (24) 参考文献 (33) 简介 慢病毒(Lentivirus)载体是以人类免疫缺陷型病毒(HIV)为基础发展起来的基因治疗载体,它对分裂细胞和非分裂细胞均具有感染能力,并可以在体内较长期的表达且安全性高。吉凯基因提供的慢病毒为“自杀”性病毒,即病毒感染目的细胞后不会再感染其他细胞,也不会利用宿主细胞产生新的病毒颗粒。慢病毒中的毒性基因已经被剔除并被外源性目的基因所取代,属于假型病毒。但该病毒仍然具有可能的潜在的生物学危险,吉凯基因建议不要使用编码已知或可能会致癌的基因的假型病毒,除非已经完全公认某个基因肯定没有致癌性,否则均不建议采用假型病毒进行生物学实验。 吉凯基因慢病毒载体系统由GV慢病毒载体系列、pHelper 1.0载体和pHelper 2.0载体三质粒组成。GV慢载体中含有HIV的基本元件5’LTR和3’LTR以及其他辅助元件,例如WRE (woodchuck hepatitis virus posttranscriptional regulatory element)。通常根据不同的实验目的针对GV载体改造以进行基因功能研究。pHelper 1.0载体中含有HIV病毒的gag基因,编码病毒主要的结构蛋白;pol基因,编码病毒特异性的酶;rev基因,编码调节gag和pol基因表达的调节因子。pHelper 2.0载体中含有单纯疱疹病毒来源的VSV-G基因,提供病毒包装所需要的包膜蛋白。 吉凯基因过表达慢病毒产品可通过对GV慢病毒载体的改造和病毒包装,获得带有特定基因序列的慢病毒颗粒,以满足不同的实验需求。 本手册为吉凯基因RNAi慢病毒载体的构建和病毒包装的通用操作流程,目的是为了方便大家交流使用,部分细节内容未能做到一一详述,敬请谅解。同时希望大家能够针对手册中的错误和问题,提出宝贵的意见。 基因转移技术 什么是基因转移技术? 基因转移技术是将特定的外源基因信息转入到受体细胞或生物并使其表达的一种基因工程技术。基因转移技术已广泛用于基因的结构和功能分析、基因表达与调控、基因治疗与转基因动物模型建立等研究方向。 基因转移方法有哪几类? 一、化学转染 1.磷酸钙法 该技术通过将磷酸盐溶液和含有DNA的氯化钙溶液进行缓慢混合,形成DNA-磷酸钙共沉淀复合物。复合物能粘附于细胞膜上,通过细胞内吞作用进入细胞浆中。 优点:实验室中转染哺乳动物细胞最广泛使用的方法。试剂易获得,成本低,可用于瞬时转染和稳定转染。 缺点:重复性差,转染效率低。对基因和细胞的选择要求较高。 2.DEAE-葡聚糖法 DEAE-葡聚糖是最早开发的转染试剂之一。它是一种可溶的聚阳离子碳水化合物,通过与带负电的DNA结合形成聚集物。携带正电荷的复合物与带负电荷的细胞膜结合,通过细胞内吞作用进入细胞中。与磷酸钙转染过程中形成的复合物颗粒相比,其粒径更小。 优点:该试剂价格便宜,并且过程简便、效率较高。一般常用于瞬时转染,DNA使用量较少。 缺点:不适用于稳定转染。 3.脂质体法 脂质体分为单层脂质体和多层脂质体。常用的阳离子脂质体与带负电的DNA结合,形成DNA-阳离子脂质体复合物,从而吸附到带负电的细胞膜表面,通过细胞内吞作用进入细胞。脂质体介导的基因转移的效率可以通过整合病毒蛋白来提高,从而促进病毒包膜和细胞膜之间的主动融合。这种融合粒子被称为病毒体。 优点:能够在活体内应用,毒性低、重复性好。适用性广,在很多细胞中能得到有效的瞬时转染和稳定转染效果。 缺点:试剂难以自制,商品较为昂贵,转染效果在不同细胞类型中差异较大。 慢病毒介导的RNAi技术 一、概述 慢病毒(Lentivirus)载体是以HIV-1(人类免疫缺陷I型病毒)为基础发展起来的基因治疗载体。和一般的逆转录病毒载体不同,它对分裂细胞和非分裂细胞均具有感染能力。该载体可以将外源基因有效地整合到宿主染色体上,从而达到持久性表达。并且它可以有效地感染神经元细胞、肝细胞、心肌细胞、肿瘤细胞、内皮细胞、干细胞等多种类型的细胞,因此具有广阔的应用前景。 目前慢病毒被广泛地应用于RNAi的研究中。由于体外合成siRNA对基因表达的抑制作用通常是短暂的,因而使其应用受到较大的限制。采用事先在体外构建能够表达siRNA的载体, 然后转入细胞内转录siRNA的策略,不但对基因表达抑制效果不逊色于体外合成siRNA,而且稳定整合表达载体的细胞中,可以发挥长期阻断基因表达的作用。但上述两种方法,一是受到转染试剂转染效率的影响,在一些细胞内无法有效敲低(Knockdown)一些基因;二是传统方法得到稳定细胞株通常需要挑取单克隆,进行克隆化,这样费时费力。利用慢病毒介导的方法可以解决上述问题,这是因为慢病毒介导的RNAi具有下述优点: ● 慢病毒几乎可以感染所有种类的细胞,不存在某些细胞难以转染的问题。 ● 慢病毒可以在细胞基因组上稳定整合表达siRNA的元件,siRNA表达效率比较均一,因此,无需挑取单克隆。 ● 慢病毒载体具有嘌罗霉素(Puromycin)抗性基因,Puromycin可以在2-3天内杀死细胞,只有整合了病毒载体的细胞能够存活,这样缩短了得到稳定细胞株的时间。 慢病毒表达载体中,是由RNA聚合酶Ⅲ启动子来指导RNA合成的,这是因为RNA聚合酶Ⅲ有明确的起始和终止序列,而且合成的RNA不会带poly A尾。当RNA聚合酶Ⅲ遇到连续4个或5个T时,它指导的转录就会停止,在转录产物3’端形成1~4个U。U6和H1 RNA启动子是两种RNA聚合酶Ⅲ依赖的启动子,其特点是启动子自身元素均位于转录区的上游,适合于表达~21nt RNA和~50nt RNA茎环结构(stem loop)。在siRNA表达载体中,构成siRNA的正义与反义链,可由各自的启动子分别转录,然后两条链互补结合形成siRNA;也可由载体直接表达小发卡状RNA(small hairpin RNA, shRNA),载体内的位于RNA 聚合酶Ⅲ启动子和4~5T转录终止位点之间的茎环结构序列,转录后可折叠成具有1~4 个U 3 ’ 突出端的茎环结构,在细胞内进一步加工成siRNA。慢病毒介导的RNAi和其它方法的比较见图1。 解剖科学进展 Progress of Anatomical Sciences 2010 Mar,16(2):131~134 慢病毒载体介导的RNA干扰抑制PC12细胞MyD88基因的表达 * 崔万鹏,刘晓湘,方秀斌(中国医科大学 基础医学院神经生物学教研室, 辽宁 沈阳 110001) 【摘要】 目的 研究慢病毒介导的RNA干扰对PC12细胞的转染效率和对MyD88基因的抑制效率。方法 构建针对大鼠MyD88基因3个靶点的ShRNA慢病毒载体,PC12细胞按照不同的转染条件分为 正常组(DMEM完全培养液)、DMEM加入polybrene组、EN.iS(Enhance infection solution)组、EN.iS加入polybrene组。荧光显微镜下观察不同组的转染效率,采用Real-time PCR和Western blot检测不同的靶点对MyD88的不同沉默效率。结果 病8毒滴度为1 × 10 TU/ml,MOI为100时,PC12细胞在EN.iS组的转染效率最高,转染试剂polybrene对转染效率无明显影响,沉默效率最高的靶点是5'-CATAC GCAACCAGCAGAAA-3'。结论 慢病毒介导的RNA干扰是一种高效的基因沉默手段,可以对PC12细胞中MyD88的功能进行长期的研究。 【关键词】 PC12细胞;慢病毒;RNA干扰;髓样分化因子初次应答基因88 【中图分类号】 Q189 【文献标识码】 A 【文章编号】 1006-2947(2010)02-0131-04 Lentivirus mediated RNA interference knockdowns the expression of MyD88 in PC12 cells 【Abstract】 Objective To investigate the transfection efficiency of lentivirus and silencing efficiency of MyD88 by lentivirus mediated RNA interference in PC12 cell line. Methods Recombinant lentivirus vectors with shRNA targeting rat myeloid differentiation primary response gene 88(MyD88) were constructed and transfected into pheochromocytoma cells (PC12) in vitro, the tranfection efficiency was observed under fluorescence microscope and the expression of MyD88 was 8determined by Real-time PCR and Western Blot respectively. Results The viral titer of lentivirus was 1×10 TU/ml and the MOI(multiplicity of infection) was 100, PC12 cells exhibited the highest transfection efficiency in enhanced infection solution(EN.iS), with no remarkable effect on the transfection efficiency in the presence of the transfection reagent polybrene. According to the result of Real-time PCR and Western Blot, the most efficient targeting sequence was 5'-CATAC GCAACCAGCAGAAA-3'. Conclusion Lentivirus mediated RNA interference is an effective means to silence gene expression in PC12 cells,which can generate stable transfected PC12 cell line with MyD88 expression knocking down. 【Key words 】 Pc12 cell line; lentivirus; RNA interference; myeloid differentiation primary response gene88 * CUI Wan-peng,LIU Xiao-xiang,FANG Xiu-bin (Department of Neurobiology, China Medical University, Liaoning Shenyang 110001 China) [1]Lentivirus 是逆转录病毒的一种,可将自身携带作用。近来研究发现,MyD88 基因敲除的小鼠在肾的基因整合如宿主基因组。利用Lentivirus 构建的脏和心肌缺血再灌注中,能够大大减少炎症反应导[1]shRNA表达载体,与化学合成和基于瞬时表达载体致的组织损伤和功能紊乱。我们通过RNA干扰抑制构建的shRNA相比,一方面可以替代瞬时表达载体PC12 细胞中Myd88的表达,建立稳定表达shRNA细使用,另一方面,可用于转染依靠传统转染试剂难胞株,研究氧糖剥夺(oxygen-glucose deprivation)于转染的原代细胞、悬浮细胞等,并且可以整合到导致的TLR4介导的MyD88依赖的信号通路的激活导被感染的细胞的基因组,进行长时间的稳定表达。致的细胞损伤的体外模型。MyD88作为 Toll-like receptor4(TLR4)的主要胞内接头蛋白,在TLR4激活引起的炎症反应中起到关键 1 材料和方法 1.1 材料和试剂 DMEM高糖培养基购自GIBCO,胎牛血清和马血 【收稿日期】2009-11-17 【基金项目】辽宁省高等学校科研项目(No.2008813) *通讯作者(To whom correspondence should be addressed) 脂质体介导I L22、TNF2α联合基因转导体内抗肝细胞癌的实验研究① 李东复 申吉子② 闫 峻② 邵国光 (吉林大学第二医院消化内科,长春130041) 周晓琦 杨春荣 (吉林大学第二医院电诊科,长春130041) 中国图书分类号 R73517 R73013 文献标识码 A 文章编号 10002484X(2003)0720483204 [摘 要] 目的:探讨脂质体介导I L22cDNA、T NF2αcDNA联合基因转导体内抗肿瘤效果。方法:建立裸鼠肝癌动物模型,在荷瘤部位将脂质体包裹的I L22cDNA和T NF2αcDNA直接注入瘤体中,观察肿瘤大小变化,并检测此2种基因在肿瘤组织中的表达情况。结果:I L22基因和T NF2α基因联合治疗组肿瘤生长明显受到抑制,抗肿瘤效果显著优于单纯治疗组和对照组。结论:I L22和T NF2α基因联合治疗组对肿瘤生长抑制效果明显优于单纯治疗组与对照组,荷瘤鼠生存期明显延长(P<0105)。 [关键词] 肝细胞癌;脂质体;细胞因子基因;基因转导 Combined I L22cDNA with TNF2αcDNA genetherapy for hepatocellular carcinoma LI Dong2Fu,SHEN Ji2Zi,Y AN Jun et al.Second Hospital,Jilin Univer sity,Changchun130041,China [Abstract] Objective:T o explore antitum or effects of combined interleukin22(I L22)gene and tum or necrosis factor(T NF2α)gene therapy in nude mice m odel with hepatocellular carcinoma.Methods:Nude mice m odel with hepatocellualr carcinoma was established with BelH7402 cell line.Plasmid vector was used to trans fect BelH7402and nude mice m odel with the gene for human I L22or T NF2αalone or in combination by lipofectamine reagent and tum or size in nude mice m odel with BelH7402carcinoma was measured before and after treatment to evaluate the response of the different treatment and control groups.R esults:Tum or and BelH7402trans fected with I L22or T NF2αgene by lipos ome mediated were success ful.G rowth rates of BelH7402trans fected with I L22or T NF2αgene were not significantly changed,growth rate of tum or in nude mice m odel was significantly inhibited in combined I L22and T NF2αgenetherapy group as compared with the other groups(P<0105).Conclu2 sion:C ombination of the tw o genes in genetherapy may be additive of synergistic in antitum or effect. [K ey w ords] Hepatocellular carcinoma;Cellular factor gene;G ene trans fection;G enetherapy 肝癌基因治疗的方法已发展有免疫基因疗法、抑癌基因疗法、反义基因疗法和自杀基因疗法。近年来又出现前药转换基因疗法、多药耐药基因疗法和反基因策略等。但是这一新的治疗手段在技术上还存在许多问题,如缺乏高效和定向的载体系统;病毒载体应用中可能产生有传染性的野生型病毒,有可能随机整合于宿主基因,从而使癌基因活化或抑癌基因失活;基因进入人体后的可控性尚难定论,某些基因的过度表达可能会产生毒副作用;所以至今为止体内型转基因治疗尚不能在临床上应用。因此,当前重要的是发展经济有效、安全易行的基因运载体系,使目的基因能达到肿瘤部位,并且表达的产物能受机体控制,使基因治疗能够按照要求用于临床,成为肝脏肿瘤的一种有效治疗方法。本文脂质体介导I L22cDNA、T NF2αcDNA联合基因转导体内抗肝细胞癌的实验研究,旨在探讨联合基因 ①吉林省科技厅基金资助项目 ②研究生 作者简介:李东复(1956年-),男,主任医师,教授,主要从事肝病免疫学研究。 转导持续基因产物表达的抗肿瘤效应,为肝癌基因治疗的临床应用提供理论与实验依据。 1 材料与方法 111 主要材料与试剂 BelH7402人肝癌细胞,本校免疫学教研究室冻存;脂质体Lipofectamine由联星生物工程试剂公司提供;I L22、T NF2α检测试剂盒由深圳晶美公司提供;质粒型真核表达载体BMG Neo、pcDNA3由上海第二军医大学免疫学教研室、本院中心实验室提供;人I L22cDNA、人T NF2αcDNA由外周血制备;裸小鼠,6~8周龄,由北京大学医学部实验动物中心提供。 112 体外基因转导与表达细胞培养 亲本肝癌细胞BelH7402及I L22、T NF2α基因转导细胞株均培养于含10%小牛血清I M DM培养基中待用。 113 I L22cDNA、T NF2αcDNA真核表达载体构建 质粒提取、酶切,连接按《分子克隆》有关章节进行。人I L22cDNA插入到BMG Neo的XhoI位点,人T NF2αcDNA插入到pcDNA3HindⅢ与BamHI位点,提取BMG Neo2hI L22,pcDNA3hT NF酶切后,018%琼脂糖凝胶电泳鉴定。 慢病毒包装系统简介及应用 一、慢病毒包装简介及其用途 慢病毒(Lentivirus)载体是以HIV-1(人类免疫缺陷I 型病毒)为基础发展起来的基因治疗载体。区别一般的逆转录病毒载体,它对分裂细胞和非分裂细胞均具有感染能力。慢病毒载体的研究发展得很快,研究的也非常深入。该载体可以将外源基因有效地整合到宿主染色体上,从而达到持久性表达。在感染能力方面可有效地感染神经元细胞、肝细胞、心肌细胞、肿瘤细胞、内皮细胞、干细胞等多种类型的细胞,从而达到良好的基因治疗效果,在美国已经开展了临床研究,效果非常理想,因此具有广阔的应用前景。 目前慢病毒也被广泛地应用于表达RNAi的研究中。由于有些类型细胞脂质体转染效果差,转移到细胞内的siRNA半衰期短,体外合成siRNA对基因表达的抑制作用通常是短暂的,因而使其应用受到较大的限制。采用事先在体外构建能够表达siRNA的载体,然后转移到细胞内转录siRNA的策略,不但使脂质体有效转染的细胞种类增加,而且对基因表达抑制效果也不逊色于体外合成siRNA,在长期稳定表达载体的细胞中,甚至可以发挥长期阻断基因表达的作用。在所构建的siRNA表达载体中,是由RNA聚合酶Ⅲ启动子来指导RNA合成的,这是因为RNA聚合酶Ⅲ有明确的起始和终止序列,而且合成的RNA不会带poly A尾。当RNA聚合酶Ⅲ遇到连续4个或5个T时,它指导的转录就会停止,在转录产物3' 端形成1~4个U。U6和H1 RNA启动子是两种RNA聚合酶Ⅲ依赖的启动子,其特点是启动子自身元素均位于转录区的上游,适合于表达~21ntRNA和~50ntRNA茎环结构(stem loop)。在siRNA表达载体中,构成siRNA 的正义与反义链,可由各自的启动子分别转录,然后两条链互补结合形成siRNA;也可由载体直接表达小发卡状RNA(small hairpin RNA, shRNA),载体包含位于RNA聚合酶Ⅲ启动子和4~5T转录终止位点之间的茎环结构序列,转录后即可折叠成具有1~4个U 3 '突出端的茎环结构,在细胞内进一步加工成siRNA。构建载体前通常要通过合成siRNA的方法,寻找高效的siRNA,然后从中挑选符合载体要求的序列,将其引入siRNA表达载体。 慢病毒载体(Lentiviral vector)较逆转录病毒载体有更广的宿主范围,慢病毒能够有效感染非周期性和有丝分裂后的细胞。慢病毒载体能够产生表达shRNA的高滴度的慢病毒,在周期性和非周期性细胞、干细胞、受精卵以及分化的后代细胞中表达shRNA,实现在多种类型的细胞和转基因小鼠中特异而稳定的基因表达的功能性沉默,为在原代的人和动物细胞组织中快速而高效地研究基因功能,以及产生特定基因表达降低的动物提供了可能性。 慢病毒表达载体包含了包装、转染、稳定整合所需要的遗传信息。慢病毒包装质粒可提供所有的转录并包装RNA到重组的假病毒载体所需要的所有辅助蛋白。为产生高滴度的病毒颗粒,需要利用表达载体和包装质粒同时共转染细胞,在细胞中进行病毒的包装,包装好的假病毒颗粒分泌到细胞外的培养基中,离心取得上清液后,可以直接用于宿主细胞的感染,目的基因进入到宿主细胞之后,经过反转录,整合到基因组,从而高水平的表达效应分子。 二、这一系统的目的,主要是为了解决以下问题: 1. 对于一些较难转染的细胞,如原代细胞、干细胞、不分化的细胞等,能大大提高目的基因转导效率,而且目的基因整合到宿主细胞基因组的几率大大增加,这就为RNAi,cDNA克隆以及报告基因的研究提供了一个有利的途径。 2. 进行稳转细胞株的筛选; 外源基因进入细胞主要有四种方法:电击法、磷酸钙法和脂质体介导法和病毒介导法。电击法是在细胞上短时间暂时性的穿孔让外源质粒进入;磷酸钙法和脂质体法是利用不同的载体物质携带质粒通过直接穿膜或者膜融合的方法使得外源基因进入细胞;病毒法是利用包装了外源基因的病毒感染细胞的方法使得其进入细胞。但是由于电击法和磷酸钙法的实验条件控制较严、难度较大;病毒法的前期准备较复杂、而且可能对于细胞有较大影响;所以现在对于很多普通细胞系,一般的瞬时转染方法多采用脂质体法。 学习和掌握外源基因导入真核细胞的主要方法—脂质体介导的转染。了解外源基因进入的一般性方法,观测外源蛋白的表达(绿色荧光蛋白),为染色准备实验材料。 上图所示是脂质体介导转染的示意图,它显示了外源质粒进入细胞的一般过程。 外源基因进入细胞主要有四种方法:电击法、磷酸钙法和脂质体介导法和病毒介导法。电击法是在细胞上短时间暂时性的穿孔让外源质粒进入;磷酸钙法和脂质体法是利用不同的载体物质携带质粒通过直接穿膜或者膜融合的方法使得外源基因进入细胞;病毒法是利用包装了外源基因的病毒感染细胞的方法使得其进入细胞。但是由于电击法和磷酸钙法的实验条件控制较严、难度较大;病毒法的前期准备较复杂、而且可能对于细胞有较大影响;所以现在对于很多普通细胞系,一般的瞬时转染方法多采用脂质体法。 本次实验采用的脂质体是promega公司的TransFast脂质体试剂,它是一种阳离子脂质体和中性脂质体的混合物,是对于本次实验中采用的293T细胞优化的转染试剂。 293T细胞 MyoD表达质粒和EGFP表达质粒 DMEM培养基 链霉素/青霉素(双抗) PBS(磷酸盐缓冲溶液) 酒精灯 废液缸 血球计数板 涡旋振荡器 35mm培养皿 转染管 细胞传代 (2) 弃掉培养皿中的培养基,用1ml的PBS溶液洗涤两次。 (5) 用Tip头多次吹吸,使细胞完全分散开。 细胞转染 1)转染试剂的准备 2)选择合适的混合比例(1:1-1:2/脂质体体积:DNA质量)来转染细胞。在一个转染管中加入合适体积的无血清培养基。加入合适质量的MyoD或者EGFP的DNA,震荡后在加入合适体积的转染试剂, 再次震荡。 7)加入混合液,将细胞放回培养箱中培养一个小时。 第二次细胞传代 在转染后24小时,观察实验结果并记录绿色荧光蛋白表达情况。 再次进行细胞传代,按照免疫染色合适的密度 北京市朝阳区2020届高三一模 1.下图为细胞膜的结构模式图,说法正确的是( ) A. 磷脂双分子层是细胞膜的基本支架,③为疏水端,④为亲水端 B. 细胞癌变时,细胞表面发生变化,①减少使细胞的黏着性降低 C. 同种生物不同细胞的细胞膜上①、②、⑤的种类和数量完全相同 D. 性激素、甘油等小分子物质从A 侧运输到B 侧需要有②或⑤参与 『答案』B 『解析』 『分析』 据图分析可知,图示为细胞膜结构图,①表示糖蛋白,②⑤表示蛋白质,③④分别表示磷脂亲水头部和疏水的尾部,A 侧面为细胞膜的外侧,B 为内侧。 『详解』A 、磷脂由亲水的③头部和疏水的④尾部构成,A 错误; B 、癌细胞的表现发生了变化,由于细胞膜上的①糖蛋白等物质减少,使得癌细胞彼此之间的黏着性低,容易在体内分散和转移,B 正确; C 、膜的功能主要由蛋白质承担,故不同功能的细胞,其细胞膜上蛋白质的种类和数量不完全相同,C 错误; D 、性激素、甘油从A 侧运输到B 侧(进入细胞)的方式为自由扩散,不需要②或⑤的参与,D 错误。 故选B 『点睛』本题主要考查细胞膜的结构和功能,意在考查考生识图和理解能力,明确流动镶嵌模型是解答本题的关键。 2.下列有关生物多样性的叙述,正确的是( ) A. 群落演替过程中生物多样性一般会逐渐降低 B. 建立动植物园是保护生物多样性最有效的措施 。 C. 湿地能调节气候,体现了生物多样性的直接价值 D. 生物多样性包括基因多样性、物种多样性、生态系统多样性 『答案』D 『解析』 『分析』 1.生物多样性是指生物圈内所有的植物、动物和微生物,它们所拥有的全部基因以及各种各样的生态系统共同构成的。 2.生物多样性的价值有:直接使用价值、间接使用价值和潜在使用价值。 『详解』一般情况下,群落演替过程中生物种类增多,生物多样性增加,A错误。建立自然保护区是保护生物多样性最有效的措施,B错误。湿地能调节气候,是属于生物多样性在生态上的功能,属于生物多样性的间接使用价值,C错误。生物多样性包括基因多样性、物种多样性、生态系统多样性,D正确。 3.有一种变异发生在两条非同源染色体之间,它们发生断裂后片段相互交换,仅有位置的改变,没有片段的增减。关于这种变异的说法错误的是() A. 这种变异使染色体结构改变,在光学显微镜下可见 B. 该变异一定导致基因突变,为生物进化提供原材料 C. 该变异可导致染色体上基因的排列顺序发生变化 D. 该变异是可遗传变异的来源,但不一定遗传给后代 『答案』B 『解析』 『分析』 根据题干分析,“该类型的变异是两条非同源染色体发生断裂后相互交换,仅有位置的改变,没有染色体片段的增减”,属于染色体结构变异中的易位,据此分析作答。 『详解』A、由以上分析可知,该变异属于染色体结构变异,在显微镜下可以观察,A正确;BC、该变异属于染色体结构变异中的易位,可导致染色体上基因的排列顺序发生变化,但易位通常不会造成基因突变,B错误,C正确; D、可遗传变异包括突变(基因突变和染色体变异)和基因重组,故该变异属于可遗传变异,但不一定能遗传给后代,D正确。 故选B。 『点睛』本题考查染色体结构变异的相关知识,意在考查考生的识记能力、审题能力和理解 慢病毒载体包装构建过程 原理:慢病毒载体可以将外源基因或外源的shRNA有效地整合到宿主染色体上,从而达到持久性表达目的序列的效果。在感染能力方面可有效地感染神经元细胞、肝细胞、心肌细胞、肿瘤细胞、内皮细胞、干细胞等多种类型的细胞,从而达到良好的的基因治疗效果。对于一些较难转染的细胞,如原代细胞、干细胞、不分化的细胞等,使用慢病毒载体,能大大提高目的基因或目的shRNA的转导效率,且目的基因或目的shRNA整合到宿主细胞基因组的几率大大增加,能够比较方便快捷地实现目的基因或目的shRNA的长期、稳定表达。 概念:慢病毒载体是指以人类免疫缺陷病毒-1 (H IV-1) 来源的一种病毒载体,慢病毒载体包含了包装、转染、稳定整合所需要的遗传信息,是慢病毒载体系统的主要组成部分。携带有外源基因的慢病毒载体在慢病毒包装质粒、细胞系的辅助下,经过病毒包装成为有感染力的病毒颗粒,通过感染细胞或活体组织,实现外源基因在细胞或活体组织中表达。 辅助成分:慢病毒载体辅助成分包括:慢病毒包装质粒和可产生病毒颗粒的细胞系。 慢病毒载体包含了包装、转染、稳定整合所需要的遗传信息。慢病毒包装质粒可提供所有的转录并包装RNA 到重组的假病毒载体所需要的所有辅助蛋白。为产生高滴度的病毒颗粒,需要利用表达载体和包装质粒同时共转染细胞,在细胞中进行病毒的包装,包装好的假病毒颗粒分泌到细胞外的培养基中,离心取得上清液后,可以直接用于宿主细胞的感染,目的基因进入到宿主细胞之后,经过反转录,整合到基因组,从而高水平的表达效应分子。 基本原理:慢病毒载体系统由两部分组成,即包装成分和载体成分。 包装成分:由HIV-1基因组去除了包装、逆转录和整合所需的顺式作用序列而构建,能够反式提供产生病毒颗粒所必需的蛋白。包装成分通常被分开构建到两个质粒上,一个质粒表达Gag和Pol蛋白,另一个质粒表达Env蛋白,其目的也是降低恢复成野生型病毒的可能。将包装成分与载体成分的3个质粒共转染细胞(如人肾293T细胞),即可在细胞上清中收获只有一次性感染能力而无复制能力的、携带目的基因的HIV-1载体颗粒。 载体成分:与包装成分互补,即含有包装、逆转录和整合所需的HIV顺式作用序列,同时具有异源启动子控制下的多克隆位点及在此位点插入的目的基因。 为降低两种成分同源重组恢复成野生型病毒的可能,需尽量减少二者的同源性,如将包装成分上5′LTR换成巨细胞病毒(CMV)立即早期启动子、3′LTR换成SV40 polyA等。 一、实验流程(1和2为并列步骤) 1.慢病毒过表达质粒载体的构建 设计上下游特异性扩增引物,同时引入酶切位点,PCR(采用高保真KOD酶,3K内突变率为0%)从模板中(CDNA质粒或者文库)调取目的基因CDS区(coding sequence)连入T载体。将CDS区从T载体上切下,装入慢病毒过表达质粒载体。 2.慢病毒干扰质粒载体的构建 合成siRNA对应的DNA颈环结构,退火后连入慢病毒干扰质粒载体 3. 慢病毒载体的包装与浓缩纯化 制备慢病毒穿梭质粒及其辅助包装原件载体质粒,三种质粒载体分别进行高纯度无内毒素抽提,共转染293T细胞,转染后6 h 更换为完全培养基,培养24和48h后,分别收集富含浅析非病毒载体基因转移技术的现状和展望
慢病毒载体使用手册
过表达慢病毒载体构建和包装手册 version1
基因转移技术
慢病毒介导的RNAi技术
慢病毒载体介导的RNA干扰抑制PC12细胞MyD88基因的表达
脂质体介导IL22
慢病毒系统简介及应用
脂质体转染原理步骤
北京市朝阳区2020届高三一模生物试题(解析版)
慢病毒载体包装构建过程