微波辐射计定标

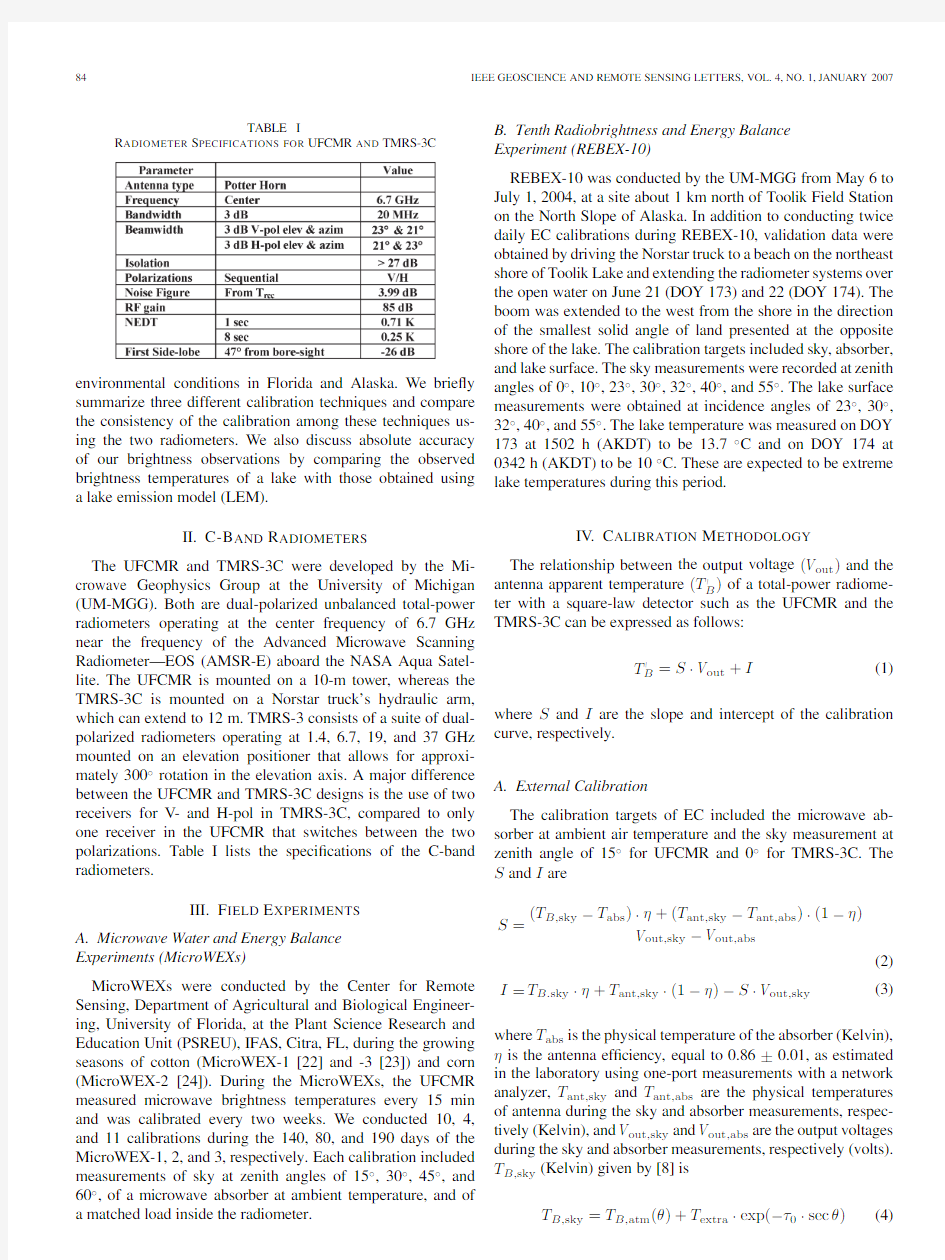
Comparison of Calibration Techniques for Ground-Based C-Band Radiometers
Kai-Jen C.Tien,Student Member,IEEE,Roger D.De Roo,Member,IEEE,Jasmeet Judge,Senior Member,IEEE,
and Hanh Pham,Student Member,IEEE
Abstract—We quantify the performance of three commonly used techniques to calibrate ground-based microwave radiometers for soil moisture studies,external(EC),tipping-curve(TC),and internal(IC).We describe two ground-based C-band radiometer systems with similar design and the calibration experiments con-ducted in Florida and Alaska using these two systems.We compare the consistency of the calibration curves during the experiments among the three techniques and evaluate our calibration by com-paring the measured brightness temperatures(T B’s)to those estimated from a lake emission model(LEM).The mean absolute difference among the T B’s calibrated using the three techniques over the observed range of output voltages during the experiments was1.14K.Even though IC produced the most consistent calibra-tion curves,the differences among the three calibration techniques were not signi?cant.The mean absolute errors(MAE)between the observed and LEM T B’s were about2–4K.As expected,the utility of TC at C-band was signi?cantly reduced due to transparency of the atmosphere at these frequencies.Because IC was found to have a MAE of about2K that is suitable for soil moisture applications and was consistent during our experiments under different environmental conditions,it could augment less frequent calibrations obtained using the EC or TC techniques.
Index Terms—Calibration,microwave radiometry,soil moisture.
I.I NTRODUCTION
G ROUND-BASED microwave radiometers have been used
extensively to measure upwelling terrain emission in ?eld experiments for hydrology,agriculture,and meteorology [1]–[7].The total-power radiometer is of the simplest design compared to other designs such as Dicke and noise injection[8] and[9].The stability and consistency of the relation between the output voltage and the antenna temperature,i.e.,system gain and offset,are critical for radiometer operations.The system gain is highly sensitive to?uctuations in the physical tempera-
Manuscript received June5,2006;revised September29,2006.This work was supported in part by the National Aeronautics and Space Administration’s ESS Graduate Student Fellowship(ESSF03-0000-0044)and in part by the University of Florida,Institute of Food and Agricultural Sciences.
K.-J.C.Tien and J.Judge are with the Center for Remote Sensing,De-partment of Agricultural and Biological Engineering,University of Florida, Gainesville FL32611USA(e-mail:ktien@u?.edu;jasmeet@u?.edu).
R. D.De Roo is with the Department of Atmospheric,Oceanic,and Space Sciences,University of Michigan,Ann Arbor,MI48109USA(e-mail: deroo@https://www.360docs.net/doc/fe2408798.html,).
H.Pham is with the Department of Electrical Engineering and Com-puter Science,University of Michigan,Ann Arbor,MI48109USA(e-mail: hpham@https://www.360docs.net/doc/fe2408798.html,).
Color versions of one or more of the?gures in this paper are available online at https://www.360docs.net/doc/fe2408798.html,.
Digital Object Identi?er10.1109/LGRS.2006.886420ture inside the radiometer requiring frequent calibration during radiometer operation for reliable and accurate observations. Many calibration techniques have been developed for mi-crowave radiometers for spaceborne and airborne[10]–[16] and ground-based radiometers[17]–[21].In general,calibration techniques include observations of radiometer output voltages for cold and hot targets with known brightness temperatures [8],[9].For radiometers operating at low frequencies away from the water vapor and oxygen absorption bands,such as C-band(6.7GHz),commonly used cold targets are liquid nitrogen or the sky.Hot targets include microwave absorbers or matched loads inside the radiometers.For a C-band ground-based microwave radiometer,the conceptually simplest cal-ibration technique using a microwave absorber at ambient temperature as a hot target is called“external calibration”(EC). Another widely used calibration technique that utilizes the sky measurements at different angles to calculate the optical depth of the atmosphere and the brightness temperatures of the sky is called“tipping curve calibration”(TC)[18],[19],[21].Either EC or TC can be used exclusively,or TC could be used to provide a better estimate of the sky measurement for EC.Both techniques are inconvenient to perform frequently for long-term soil moisture studies using ground-based C-band radiometers. Moreover,the utility of TC at C-band might be hampered by the high atmospheric transparency at low microwave frequencies [8].Another technique,“internal calibration”(IC),uses an internal matched load as the hot target.This technique has been used for spaceborne microwave radiometers,e.g.,SMMR [10],TMR[13],[14],and JMR[15],airborne radiometers [16],and ground-based radiometers[17].Unlike EC and TC, IC can be performed faster than gain?uctuation.Also,IC is neither sensitive to operator technique,to weathering of the delicate microwave absorber,nor does it require any additional hardware exclusively for the purpose of calibration.However, IC does not account for the losses in the antenna and trans-mission lines before the internal switch used to observe the matched load.
In this letter,we quantify the performance of IC and validate it using EC and TC for long-term observations of soil moisture using two ground-based C-band radiometers.Our analysis is re-stricted to horizontal polarization(H-pol)because of its higher sensitivity to soil moisture than vertical polarization(V-pol)[8]. We describe two ground-based total-power radiometers with similar design:the University of Florida C-band Microwave Radiometer(UFCMR)and the C-band unit on the Truck Mounted Radiometer System3(TMRS-3C),as well as the calibration experiments conducted under signi?cantly different
1545-598X/$25.00?2007IEEE
TABLE I
R ADIOMETER S PECIFICATIONS FOR UFCMR AND
TMRS-3C
environmental conditions in Florida and Alaska.We brie?y
summarize three different calibration techniques and compare the consistency of the calibration among these techniques us-ing the two radiometers.We also discuss absolute accuracy of our brightness observations by comparing the observed brightness temperatures of a lake with those obtained using a lake emission model (LEM).
II.C-B AND R ADIOMETERS
The UFCMR and TMRS-3C were developed by the Mi-crowave Geophysics Group at the University of Michigan (UM-MGG).Both are dual-polarized unbalanced total-power radiometers operating at the center frequency of 6.7GHz near the frequency of the Advanced Microwave Scanning Radiometer—EOS (AMSR-E)aboard the NASA Aqua Satel-lite.The UFCMR is mounted on a 10-m tower,whereas the TMRS-3C is mounted on a Norstar truck’s hydraulic arm,which can extend to 12m.TMRS-3consists of a suite of dual-polarized radiometers operating at 1.4,6.7,19,and 37GHz mounted on an elevation positioner that allows for approxi-mately 300?rotation in the elevation axis.A major difference between the UFCMR and TMRS-3C designs is the use of two receivers for V-and H-pol in TMRS-3C,compared to only one receiver in the UFCMR that switches between the two polarizations.Table I lists the speci?cations of the C-band radiometers.
III.F IELD E XPERIMENTS
A.Microwave Water and Energy Balance Experiments (MicroWEXs)
MicroWEXs were conducted by the Center for Remote Sensing,Department of Agricultural and Biological Engineer-ing,University of Florida,at the Plant Science Research and Education Unit (PSREU),IFAS,Citra,FL,during the growing seasons of cotton (MicroWEX-1[22]and -3[23])and corn (MicroWEX-2[24]).During the MicroWEXs,the UFCMR measured microwave brightness temperatures every 15min and was calibrated every two weeks.We conducted 10,4,and 11calibrations during the 140,80,and 190days of the MicroWEX-1,2,and 3,respectively.Each calibration included measurements of sky at zenith angles of 15?,30?,45?,and 60?,of a microwave absorber at ambient temperature,and of a matched load inside the radiometer.
B.Tenth Radiobrightness and Energy Balance Experiment (REBEX-10)
REBEX-10was conducted by the UM-MGG from May 6to July 1,2004,at a site about 1km north of Toolik Field Station on the North Slope of Alaska.In addition to conducting twice daily EC calibrations during REBEX-10,validation data were obtained by driving the Norstar truck to a beach on the northeast shore of Toolik Lake and extending the radiometer systems over the open water on June 21(DOY 173)and 22(DOY 174).The boom was extended to the west from the shore in the direction of the smallest solid angle of land presented at the opposite shore of the lake.The calibration targets included sky,absorber,and lake surface.The sky measurements were recorded at zenith angles of 0?,10?,23?,30?,32?,40?,and 55?.The lake surface measurements were obtained at incidence angles of 23?,30?,32?,40?,and 55?.The lake temperature was measured on DOY 173at 1502h (AKDT)to be 13.7?C and on DOY 174at 0342h (AKDT)to be 10?C.These are expected to be extreme lake temperatures during this period.
IV .C ALIBRATION M ETHODOLOGY
The relationship between the output voltage (V out )and the
antenna apparent temperature (T
B
)of a total-power radiome-ter with a square-law detector such as the UFCMR and the TMRS-3C can be expressed as follows:
T B =S ·V out +I
(1)
where S and I are the slope and intercept of the calibration curve,respectively.A.External Calibration
The calibration targets of EC included the microwave ab-sorber at ambient air temperature and the sky measurement at zenith angle of 15?for UFCMR and 0?for TMRS-3C.The S and I are S =
(T B,sky ?T abs )·η+(T ant ,sky ?T ant ,abs )·(1?η)
out ,sky ?V out ,abs
(2)
I =T B.sky ·η+T ant ,sky ·(1?η)?S ·V out ,sky
(3)
where T abs is the physical temperature of the absorber (Kelvin),ηis the antenna ef?ciency,equal to 0.86±0.01,as estimated in the laboratory using one-port measurements with a network analyzer,T ant ,sky and T ant ,abs are the physical temperatures of antenna during the sky and absorber measurements,respec-tively (Kelvin),and V out ,sky and V out ,abs are the output voltages during the sky and absorber measurements,respectively (volts).T B,sky (Kelvin)given by [8]is
T B,sky =T B,atm (θ)+T extra ·exp(?τ0·sec θ)
(4)
TIEN et al.:COMPARISON OF CALIBRATION TECHNIQUES FOR GROUND-BASED C-BAND RADIOMETERS85 and
T B,atm(θ)=secθ
∞
κa(z )·T(z )exp(?τ(0,z )·secθ)dz
(5)
where T extra is the extraterrestrial brightness temperature (Kelvin),which is~2.7K,τ0is the total zenith opacity(Np),θis the zenith angle,κa is the atmospheric absorption coef-?cient(Np·m?1),T is the temperature pro?le(Kelvin),and
τ(0,z )is the optical thickness of the atmosphere between the surface and height z (Np).Given the atmospheric temper-ature,pressure,and water vapor density,the sky brightness temperatures can be calculated based on the1962U.S.Standard Atmosphere[8].At C-band,the sensitivity of sky brightness to changes in atmospheric conditions can be ignored due to the high atmospheric transparency[8].
The sources of error using EC include the measurement er-rors due to the antenna sidelobes,εsl,the insertion loss variabil-ity of the radiometer switches,εsw,and the uncertainty in the physical temperature measurements of the absorberεat.While errors due to measurement of antenna ef?ciency contribute to errors in antenna noise temperatures,these errors are removed in the correction to scene brightness temperatures because the calibration targets are all external to the antenna.The effect of these errors using UFCMR and TMRS-3C will be discussed in Section V.
B.TC Calibration
T B,sky can be obtained by TC assuming a horizontally strat-i?ed atmosphere[19]and[25]as
T B,sky=T extra·exp[?A(θ)·τ]+T atm·(1?exp[?A(θ)·τ])
(6) where A is the airmass at zenith angleθ,τis the atmospheric opacity(Np),and T atm is the mean atmospheric temperature (Kelvin).For UFCMR and TMRS-3C,the antenna temperature is linearly related to the output voltage such that
V out,sky?V out,abs V out,abs?V ofst =
T A,sky?T A,abs
T A,abs?T rec
(7)
where V ofst is the system offset voltage when the system input noise temperature is0K(T sys=T A+T rec),T A,sky and T A,abs are the apparent antenna temperatures for the sky and absorber measurements,respectively(Kelvin),and T rec is the receiver noise temperature(Kelvin).The equations for S and I are the same as(2)and(3),with T B,sky estimated by the radia-tive transfer equation using the least-squares technique from 0?to45?.The atmospheric temperature was approximated by the air temperature at the Earth’s surface[19].
The sources of error using TC includeεsl,εsw,andεat, similar to those in EC.Errors in antenna noise temperatures due to uncertainty in the antenna ef?ciency are removed in the correction to scene brightness temperatures.C.Internal Calibration
IC uses an internal matched load or a?xed-temperature source,such as a noise diode,inside the radiometer as the hot target.The cold target is the sky measurement at15?for UFCMR and at0?for TMRS-3C.The S and I using IC are S=
T B,sky·η+T ant,sky·(1?η)?T cal
V out,sky?V out,cal
(8)
I=T cal?S·V out,cal(9) where T cal is the physical temperature of the matched load (Kelvin),ηis the antenna ef?ciency,V out,cal is the output voltage when switched to the matched load at T cal(volts),and T B,sky is estimated,similar to EC[8].
The sources of error using IC include theεsl andεsw,similar to those in EC,the error due to the uncertainty in the antenna ef?ciency estimationεae and the uncertainty in the physical temperature measurements of the internal loadεlt.
D.LEM
For an open calm water surface,the brightness temperatures observed by a microwave radiometer can be modeled as
T B,p=Γp·T B,sky+(1?Γp)·T water(10) whereΓp is the re?ectivity at polarization p,and T water is the physical temperature of water(Kelvin).T B,sky is~5K for C-band.The re?ectivity of the specular water surface is deter-mined by the incidence angle[26]and the dielectric constant of the water.The empirical models used for the dielectric constant of pure water can be found in[27]and[28].
V.R ESULTS AND D ISCUSSION
The calibration data from MicroWEXs and REBEX-10pro-vided a unique opportunity to compare the performance of two C-band radiometers with similar design in different environ-mental conditions.During each experiment,the radiometers were maintained at constant temperatures with0.1K standard deviation at the RF circuitry.Table II shows the means and the standard deviations of the calibration curves at H-pol during MicroWEXs and REBEX-10.These included25data points during MicroWEXs,as well as80points for EC and IC,and two points for TC during REBEX-10.IC produced the most consistent calibration curves in terms of the lowest standard deviations of the slopes,although the differences among the calibration techniques were not signi?cant.
Fig.1(a)–(d)shows the gain?uctuations of the calibration curves for MicroWEXs and REBEX-10.The mean absolute dif-ference(MAD)between the slopes of EC and IC was2.8K/V during MicroWEXs.The difference between the slopes of TC and EC was4.4K/V,and the difference between the slopes of TC and IC was3.6K/V during MicroWEXs.The MADs for REBEX-10were not calculated because there were only two TC measurements.During MicroWEXs,the EC and IC calibration curves were closer to each other,while TC produced slightly dissimilar results from EC and IC.This was primarily
86IEEE GEOSCIENCE AND REMOTE SENSING LETTERS,VOL.4,NO.1,JANUARY 2007
TABLE II
M EAN AND S TANDARD D EVIATION OF THE S LOPES (S )AND I NTERCEPTS (I )FOR THE H-P OL C ALIBRATION C URVE D URING M ICRO WEX S (MWS)AND REBEX-10
(RB)
Fig.1.Slopes for the calibration curve at H-pol during (a)MicroWEX-1,(b)2,(c)3,and (d)REBEX-10.RMSE of EC =1.20,TC =1.84,and IC =1.10K/V.For clarity of the ?gures,only Fig.1(a)shows the error bars.
because at C-band,TC is based on multiple measurements with small differences in brightness temperatures (T B ’s)of the sky,compared to measurements at higher frequencies at which the differences are larger.Due to the high atmospheric transparency,the utility of TC at C-band was reduced.Applying the calibration curves over the output voltages for the terrains observed during MicroWEXs and REBEX-10,the MAD of the calibrated T B ’s using the three calibration techniques was 1.14K.Table III gives the root-mean-square errors (RMSEs)estimates in the accuracy of observed T B ’s using UFCMR and TMRS-3C due to εae ,εsl ,εsw ,εat ,and εlt ,as mentioned in Section IV.The RMSE of EC,TC,and IC are 1.20,1.84,and 1.10K/V ,respectively.
To assess the accuracy of the calibration,we observed T B ’s of a calm lake at different incidence angles between 20?and 55?during REBEX-10.The RMSEs due to antenna sidelobes dur-ing the lake observations were 0.28,0.26,0.25,0.25,and 0.24K at incidence angles of 23?,30?,32?,40?,and 55?,respectively.These RMSEs are included in the error bars shown in Fig.2(a)and (b).Observed T B ’s were compared with those of a smooth water surface simulated by LEM (Fig.2).We used
TABLE III
RMSE E STIMATION FOR THE O PERATION AND C ALIBRATION OF UFCMR AND
TMRS-3C
https://www.360docs.net/doc/fe2408798.html,parison of differences between the REBEX-10observed and LEM simulated brightness temperatures (?T B )(a)at H-pol on DOY 173and (b)at H-pol on DOY 174.The total RMSE between observed and simulated values are 1.46,2.20,and 1.39for EC,TC,and IC,respectively.
two dielectric models for pure water,[27],[28]and found that the MADs between the simulated LEM T B ’s were insigni?cant at ~0.0095K.The uncertainty in the measurements of physical temperature of water was ±3.0K resulting in an RMSE of 0.8K at H-pol in the simulated T B ’s.The mean absolute errors (MAE)between the observed and modeled T B ’s at H-pol were 2.50±1.46,3.90±2.02,and 2.40±1.39K for EC,TC,and IC,respectively.For soil moisture applications,an accuracy of about 2K at C-band is adequate [29].
VI.C ONCLUSION
The calibration experiments during the MicroWEXs and REBEX-10were designed to assess the calibration consistency of two C-band radiometers with similar design.We compare the three most widely used techniques EC,TC,and IC to understand their performance for long-term soil moisture stud-ies using ground-based C-band radiometers.Even though IC produced the most consistent calibration curves,the differ-ences among the three calibration techniques were not signif-icant.Applying the calibration curves over the range of output voltages observed during the MicroWEXs and REBEX-10,
TIEN et al.:COMPARISON OF CALIBRATION TECHNIQUES FOR GROUND-BASED C-BAND RADIOMETERS87
the MAD of the T B’s calibrated among the three calibration
techniques was1.14K.The absolute accuracy of calibration
techniques was investigated by comparing the observed and
modeled T B’s of a calm lake.The MAE between the observed
and modeled T B’s were2.50±1.46,3.90±2.02,and2.40±1.39K at H-pol for EC,TC,and IC,respectively.Due to the high atmospheric transparency,the utility of TC at C-band is
greatly reduced.Because IC was found to have a MAE of~2K,
suitable for soil moisture applications,and was consistent dur-
ing our experiments under signi?cantly different environmental
conditions,it can be used to augment less frequent calibrations
obtained by the EC or TC techniques.
A CKNOWLEDGMENT
The authors would like to thank the anonymous reviewers
for their constructive comments and suggestions that improved
this paper.The authors would also like to thank T.Lin,
J.Casanova,M.-Y.Jang,https://www.360docs.net/doc/fe2408798.html,ler,and https://www.360docs.net/doc/fe2408798.html,nni for their
support during the MicroWEXs,the PSREU Research Coor-
dinator J.Boyer and his team for providing excellent manage-
ment of the study?elds,and M.Dukes(University of Florida)
for providing the measurements of air pressure and temperature
used for calibrating the UFCMR.
R EFERENCES
[1]T.J.Jackson and P.E.O’Neill,“Attenuation of soil microwave emission
by corn and soybean at1.4and5GHz,”IEEE Trans.Geosci.Remote Sens.,vol.28,no.5,pp.978–980,Sep.1990.
[2]T.J.Jackson,P.E.O’Neill,and C.T.Swift,“Passive microwave observa-
tion of diurnal surface soil moisture,”IEEE Trans.Geosci.Remote Sens., vol.35,no.5,pp.1210–1222,Sep.1997.
[3]J.Judge,J.F.Galantowicz,and A.W.England,“A comparison of ground-
based and satellite-borne microwave radiometric observations in the Great Plains,”IEEE Trans.Geosci.Remote Sens.,vol.39,no.8,pp.1686–1696, Aug.2001.
[4]J.Shi,K.S.Chen,Q.Li,T.J.Jackson,P.E.O’Neill,and L.Tsang,“A pa-
rameterized surface re?ectivity model and estimation of bare-surface soil moisture with L-band radiometer,”IEEE Trans.Geosci.Remote Sens., vol.40,no.12,pp.2674–2686,Dec.2002.
[5]F.Lemaitre,J.C.Poussiere,Y.H.Kerr,M.Dejus,R.Durbe,P.Rosnay,
and J.C.Calvet,“Design and test of the ground-based L-band radiometer for estimating water in soil(LEWIS),”IEEE Trans.Geosci.Remote Sens., vol.42,no.8,pp.1666–1676,Aug.2004.
[6]K.Schneeberger, C.Stamm, C.Matzler,and H.Fluhler,“Ground-
based dual-frequency radiometry of bare soil at high temporal resolu-tion,”IEEE Trans.Geosci.Remote Sens.,vol.42,no.3,pp.588–595, Mar.2004.
[7]A.Memmo,E.Fionda,T.Paolucci,D.Cimini,R.Ferretti,S.Bonafoni,
and P.Ciotti,“Comparison of MM5integrated water vapor with mi-crowave radiometer,GPS,and radiosonde measurements,”IEEE Trans.
Geosci.Remote Sens.,vol.43,no.5,pp.1050–1058,May2005.
[8]F.T.Ulaby,R.K.Moore,and A.K.Fung,Microwave Remote
Sensing—Active and Passive,vol.I.Norwood,MA:Artech House, 1981,pp.369–396.
[9]N.Skou,Microwave Radiometer Systems:Design and Analysis.
Norwood,MA:Artech House,1989,pp.13–19.
[10]E.Njoku,J.M.Stacey,and F.T.Barath,“The Seasat Scanning Microwave
Radiometer(SMMR):Instrument description and performance,”IEEE J.
Ocean.Eng.,vol.OE-5,no.2,pp.100–115,Apr.1980.[11]C.S.Ruf,“Detection of calibration drifts in spaceborne microwave ra-
diometers using a vicarious cold reference,”IEEE Trans.Geosci.Remote Sens.,vol.38,no.1,pp.44–52,Jan.2000.
[12]C.S.Ruf and J.Li,“A correlated noise calibration standard for interfer-
ometric,polarimetric,and autocorrelation microwave radiometers,”IEEE Trans.Geosci.Remote Sens.,vol.41,no.10,pp.2187–2196,Oct.2003.
[13]C.S.Ruf,S.J.Keihm,and M.A.Janssen,“TOPEX/Poseidon Microwave
Radiometer(TMR).I.Instrument description and antenna temper-ature calibration,”IEEE Trans.Geosci.Remote Sens.,vol.33,no.1, pp.125–137,Jan.1995.
[14]C.S.Ruf,S.J.Keihm, B.Subramanya,and M. A.Janssen,
“TOPEX/POSEIDON Microwave Radiometer performance and in-?ight calibration,”J.Geophys.Res.,vol.99,no.C12,pp.24915–24926,1994.
[15]P.Bonnefond,B.Haines,G.Born,P.Exertier,S.Gill,G.Jan,E.Jeansou,
D.Kubitscheck,https://www.360docs.net/doc/fe2408798.html,urain,Y.Menard,and A.Orsoni,“Calibrating the
Jason-1measurement system:Initial results from the Corsica and Harvest veri?cation experiments,”Adv.Space Res.,vol.32,no.11,pp.2135–2140, 2003.
[16]I.Corbella,A.J.Gasiewski,M.Klein,V.Leuski,A.J.Francavilla,and
J.R.Piepmeier,“On-board calibration of dual-channel radiometers using internal and external references,”IEEE Trans.Microw.Theory Tech., vol.50,no.7,pp.1816–1820,Jul.2002.
[17]H.Pham,E.J.Kim,and A.W.England,“An analytical calibration ap-
proach for microwave polarimetric radiometers,”IEEE Trans.Geosci.
Remote Sens.,vol.43,no.11,pp.2443–2451,Nov.2005.
[18]B.Deuber,N.Kampfer,and D.G.Feist,“A new22-GHz radiometer
for middle atmospheric water vapor pro?le measurements,”IEEE Trans.
Geosci.Remote Sens.,vol.42,no.5,pp.974–984,May2004.
[19]Y.Han and E.R.Westwater,“Analysis and improvement of tipping cal-
ibration for ground-based microwave radiometer,”IEEE Trans.Geosci.
Remote Sens.,vol.38,no.3,pp.1260–1276,May2000.
[20]K.Al-Ansari,P.Garcia,J.M.Riera,A.Benarroch,D.Fernandez,and
L.Fernandez,“Calibration procedure of a microwave total-power radiometer,”IEEE Microw.Wireless Compon.Lett.,vol.12,no.3, pp.93–95,Mar.2002.
[21]D.Cimini,E.R.Westwater,Y.Han,and S.J.Keihm,“Accuracy of
ground-based microwave radiometer and balloon-borne measurements during the WVIOP2000?eld experiment,”IEEE Trans.Geosci.Remote Sens.,vol.41,no.11,pp.2605–2615,Nov.2003.
[22]K.C.Tien,J.Judge,https://www.360docs.net/doc/fe2408798.html,ler,and https://www.360docs.net/doc/fe2408798.html,nni,Field Data Report
for the First Microwave Water and Energy Balance Experiment (MicroWEX-1)July17–Decemb er16,2003,Gainesville,FL:Center for Remote Sensing,Univ.Florida,2005.[Online].Available:http://edis.
ifas.u?.edu/AE288
[23]T.Lin,J.Judge,K.C.Tien,J.J.Casanova,M.Jang,https://www.360docs.net/doc/fe2408798.html,nni,L.W.
Miller,and F.Yan,Field Observations During the Third Microwave Water and Energy Balance Experiment(MicroWEX-3)June16–Decemb er21, 2004,Gainesvile,FL:Center for Remote Sensing,Univ.Florida,2005.
[Online].Available:http://edis.ifas.u?.edu/AE361
[24]J.Judge,J.J.Casanova,T.Lin,K.C.Tien,M.Jang,https://www.360docs.net/doc/fe2408798.html,nni,and
https://www.360docs.net/doc/fe2408798.html,ler,Field Observations During the Second Microwave Wa-ter and Energy Balance Experiment(MicroWEX-2)March17–June3, 2004,Gainesville,FL:Center for Remote Sensing,Univ.Florida,2005.
[Online].Available:http://edis.ifas.u?.edu/AE360
[25]M.A.Janssen,Atmospheric Remote Sensing by Microwave Radiometry.
New York:Wiley,1993,pp.259–334.
[26]L.A.Rose,W.E.Asher,S.C.Reising,P.W.Gasier,K.M.St.Germain,
D.J.Dowgiallo,K.Horgan,G.Farquharson,and
E.J.Knapp,“Radio-
metric measurements of the microwave emissivity of foam,”IEEE Trans.
Geosci.Remote Sens.,vol.40,no.12,pp.2619–2625,Dec.2002. [27]L.A.Klein and C.T.Swift,“An improved model for the dielectric
constant of sea water at microwave frequencies,”IEEE Trans.Antennas Propag.,vol.AP-25,no.1,pp.104–111,Jan.1977.
[28]T.Meissner and F.J.Wentz,“The complex dielectric constant of pure and
sea water from microwave satellite observations,”IEEE Trans.Geosci.
Remote Sens.,vol.42,no.9,pp.1836–1849,Sep.2004.
[29]J. C.Calvet, A.Chanzy,and J.P.Wigneron,“Surface temperature
and soil moisture retrieval in the Sahel from airborne multifrequency microwave radiometry,”IEEE Trans.Geosci.Remote Sens.,vol.34,no.2, pp.588–600,Mar.1996.
现代雷达信号处理技术及发展趋势..
现代雷达信号处理技术及发展趋势 摘要:自二战以来,雷达就广泛应用于地对空、空中搜索、空中拦截、敌我识别等领域,后又发展了脉冲多普勒信号处理、结合计算机的自动火控系统、多目标探测与跟踪等新的雷达体制。随着科技的不断进步,雷达技术也在不断发展,现代雷达已经具备了多种功能,如反隐身、反干扰、反辐射、反低空突防等能力,尤其是在复杂的工作环境中提取目标信息的能力不断得到加强。例如,利用雷达系统中的信号处理技术对接收数据进行处理不仅可以实现高精度的目标定位与跟踪, 还能够在目标识别和目标成像、电子对抗、制导等功能方面进行拓展, 实现综合业务的一体化。 一、雷达的起源及应用 雷达,是英文Radar的音译,源于radio detection and ranging的缩写,意思为"无线电探测和测距",即用无线电的方法发现目标并测定它们的空间位置。因此,雷达也被称为“无线电定位”。雷达是利用电磁波探测目标的电子设备。雷达发射电磁波对目标进行照射并接收其回波,由此获得目标至电磁波发射点的距离、距离变化率(径向速度)、方位、高度等信息。雷达最为一种重要的电磁传感器,在国防和国民经济中应用广泛,最大特点是全天时、全天候工作。雷达由天线、发射机、接收机、信号处理机、终端显示等部分组成。 雷达的出现,是由于二战期间当时英国和德国交战时,英国急需一种能探测空中金属物体的雷达(技术)能在反空袭战中帮助搜寻德国飞机。二战期间,雷达就已经出现了地对空、空对地(搜索)轰炸、空对空(截击)火控、敌我识别功能的雷达技术。二战以后,雷达发展了单脉冲角度跟踪、脉冲多普勒信号处理、合成孔径和脉冲压缩的高分辨率、结合敌我识别的组合系统、结合计算机的自动火控系统、地形回避和地形跟随、无源或有源的相位阵列、频率捷变、多目标探测与跟踪等新的雷达体制。后来随着微电子等各个领域科学进步,雷达技术的不断发展,其内涵和研究内容都在不断地拓展。雷达的探测手段已经由从前的只有雷达一种探测器发展到了红外光、紫外光、激光以及其他光学探测手段融合协作。
HY-2A卫星校正微波辐射计数据用户手册
HY-2A卫星校正微波辐射计数据 用户手册 国家卫星海洋应用中心 2011年5月
更改页
目录 1 数据产品介绍 (1) 1.1 产品级别划分 (1) 1.2 产品文件命名 (1) 1.2.1 一级产品文件命名 (1) 1.2.2 二级产品文件命名 (1) 2 一级数据产品 (2) 2.1 数据处理流程 (2) 2.2 L 1A数据格式 (3) 2.2.1 产品数据结构 (3) 2.2.2 产品头文件 (4) 2.2.3 产品科学数据 (6) 2.2.4 科学数据各参数介绍 (9) 2.3 L 1B数据格式 (14) 2.3.1 产品数据结构 (14) 2.3.2 产品头文件 (14) 2.3.3 产品科学数据 (16) 2.3.4 科学数据各参数介绍 (19) 3 二级数据产品 (19) 3.1 数据产品制作流程 (19) 3.2 L 2A数据格式 (20) 3.2.1 产品数据结构 (20) 3.2.2 产品头文件 (20) 3.2.3 产品科学数据 (23) 3.2.4 科学数据各参数介绍 (25) 3.3 L 2B数据格式 (25) 3.3.1 产品数据结构 (25) 3.3.2 产品头文件 (26) 3.3.3 产品科学数据 (28) 3.3.4 科学数据各参数介绍 (31) 3.4 L 2C数据格式 (31) 3.4.1 产品数据结构 (31) 3.4.2 产品科学数据 (31)
1数据产品介绍 国家卫星海洋应用中心将载荷的HY-2卫星校正辐射计0级数据经过预处理、重采样和数据反演分别生成1级、2级产品。 1.1 产品级别划分 一级产品 1A:经过时间标识和地理定位后的数据。包括扫描时间,每扫描点地理定位;存储观测、定标计数的数据;天线温度校正系数,轨道运行状态、平台姿态等辅助信息;记录质量信息等。 1B:经过分pass,亮温计算,以及带有定位信息及描述信息的数据。 二级产品 2A:经过亮温重采样的数据,将1B中观测亮温平均成每秒一次。 2B:经过反演计算,将2A数据反演成海洋大气物理产品,并且包含2A的亮温产品。 2C:经过格式转换,将hdf格式转换为二进制格式的产品。 1.2 产品文件命名 1.2.1 一级产品文件命名 L 1A级:H2A_RC1ALnnnnn.yyyydddhhmm.h5 L 1B级:H2A_RC1Byyyymmdd_ccc_pppp.h5 其中: H2A:HY-2卫星 RC1:校正辐射计 L:拼站(含延时和实时数据拼接)数据 nnnnn:轨道号 yyyy:观测开始时间的年 mm:观测开始时间的月 dd:观测开始时间的日 ccc:CYCLE 号 pppp:PASS 号 1.2.2二级产品文件命名 L 2A级:H2A_RC1_000_2Av_ccc_pppp.h5 L 2B级:H2A_RC1_000_2Bv_ccc_pppp.h5 其中: H2A:HY-2卫星
微波辐射计技术手册
地基多频段微波辐射计 技术手册 (HSMR) 长春市海思电子信息技术有限责任公司 2011年10月
目录 1 技术概况 (1) 2 接收机的原理与设计 (4) 3.1 技术要求和试验方法 (6) 3.2 接收机通道的测试 (7) 3.2.1噪声系数(A) (7) 3.2.2 接收机线性度测量(A) (7) 3.2.3 接收机灵敏度测量(A) (8) 3.2.4 接收机中频带宽测试(A) (9) 3.2.5 接收机工作频率测试 (9) 3.2.6系统抽样进行环境试验 (10) 3.3 设备检验 (10) 3.3.1 常规检验 (10) 3.3.2 交收检验 (10) 4 标志、保管和运输 (10) 5 软件技术条件 (11) 5.1 软件平台 (11) 5.2 软件功能 (11) 6 微波辐射计电缆连接标识 (12) 7 系统电磁兼容 (13) 8 系统的可靠性设计 (13)
9 系统接地要求 (14) 10 探测环境条件要求 (14) 10.1探测环境条件的要求 (14) 10.2探测场地的要求 (15) 10.3工作室要求及设备安置 (15)
1 技术概况 微波辐射计是宽频带、高增益、高灵敏度的被动微波遥感仪器,能够在很强的背景噪声中提取微弱的信号变化量。通过接收被测目标自身的微波辐射获取相应的物理特性,经过有效的数据反演进行定量分析。 本套产品的微波辐射计主要包括7个频率的仪器,在微波频率划分上分别是L、S、C、X、Ku、K和Ka,具体设计对应频率为1.4GHz,2.65GHz,6.6GHz,10.65GHz,13.9GHz,18.7GHz,37GHz。其中1.4GHz和2.65GHz为双极化天线,6.6GHz,10.65GHz,13.9GHz,18.7GHz,37GHz为喇叭天线,可以旋转机身转换极化测量,以求对岩石加载过程中微波多个频率点有深入细致的了解。 单极化接收各波段微波辐射计的原理框图如图1所示。 图1 微波辐射计接收通道原理框图 双极化微波辐射计利用双极化接收天线同时接收目标的微波辐射信息,由线性极化分离器分别获取水平极化和垂直极化信息,经两路接收通道进行处理。 数字控制单元完成射频开关的控制,并将测量得到的原始数据通过串行通讯送到主计算机。 L、S波段属于微波遥感应用频率的低端,极易受到其它电磁辐射源的影响,
某气象雷达站辐射环境影响评价
(辐射类)
环境影响报告表
项目名称:天气雷达建设项目
建设单位:***********
编制日期:**年**月**日
项目基本情况简介表
项目名称 单位名称 通讯地址 法人代表 联 系 人 立项审批部门 建设性质 占地面积 总投资
**天气雷达建设项目 ** **
电磁技术应用的目的和任务
随着全国*****天气雷达网的逐步建设, *****多普勒天气雷达建设效益日益凸显, 大大提高了台风、暴雨、冰雹、雷暴、龙卷风等灾害性天气监测、预警、预报和服务 能力,对航空安全、军事行动、林业生态、水利水文、云水资源利用等都产生显著效 益。从发达国家对多普勒天气雷达的使用情况看,其预测强对流灾害性天气的短时预 报准确率在现有基础上至少可提高 3%~5%,时效能提前几十分钟到数小时,这能为政 府各部门及人民群众采取准确、及时的防范措施提供科学依据。
1
概 述
前 言
为了保护环境和公众利益,根据《中华人民共和国放射性污染防治法》《中华人民 、 共和国环境影响评价法》《放射性同位素与射线装置安全和防护条例》 、 ,以及《**省 辐射环境管理办法》等对伴有辐射建设项目环境管理的规定和要求,进一步完善相关 的环保手续,编制了《**气象雷达建设项目辐射环境影响报告表》 。
编制依据
1.《中华人民共和国环境保护法》 ;1989 年; 2.《中华人民共和国环境影响评价法》 ,2003 年; 3.《建设项目环境保护管理条例》 ,国务院令第 253 号;1998 年; 4.《电磁辐射环境保护管理办法》 ,国家环境保护局令第 18 号;1997 年; 5.《电磁辐射防护规定》 (GB8702-88) ,国家环境保护局,1988 年; 6.《辐射环境保护管理导则-电磁辐射监测仪器和方法》 (HJ/T10.2—1996) ; 7. 《辐射环境保护管理导则-电磁辐射环境影响评价方法与标准》 (HJ/T10.3—1996) ;
2
微波辐射计应用场合与任务
目录 1微波辐射计应用场合与任务 (2) 2微波辐射计组成与关键技术 (3) 3微波辐射计研究热点与趋势(星载微波辐射计) (7) 4关于微波辐射计发展的思考建议 (9) 参考文献 (10)
微波辐射计(英语:microwave radiometer,缩写为“MWR”)也称为“微波辐射仪”,是一种用于测量亚毫米级到厘米级波长(频率约为1-1000GHz)的电磁波(微波)的辐射计。微波辐射仪能接收大气中的某些成分在一定频率上强烈辐射的微波,经过一定的转换方法,得到大气在垂直和水平方向上的气象要素分布,并且还可以探测到云状、云高以及目力无法观测到的晴空湍流。此仪器携带方便,可增加探空网在时间和空间上的密度,能观测到大气的连续变化,不致漏掉范围较小但变化剧烈的天气系统。微波辐射计是一款被动式微波遥感设备,微波遥感起步晚于可见光和红外遥感。但相对于可见光和红外遥感器而言,微波辐射计能全天候、全天时工作。可见光遥感只能在白天工作,红外遥感虽可在夜晚工作,但不能穿透云雾。 微波辐射计主要用于中小尺度天气现象,如暴风雨、闪电、强降雨、雾、冰冻及边界层紊流。对于短时间内生成或消散的中小尺度天气灾害,虽然只是地区性的,但部分事件危害性较大。在目前中尺度天气现象监测过程中,探空气球和天气雷达是常用的手段。探空气球会受到使用时间和空间的限制;天气雷达资料基本局限于降雨过程无降水时的欠缺;在离地面5公里范围内卫星遥感数据存在较大的误差。被动式地基微波辐射计的出现,填补上述研究方法监测方面的空白,是其有效的补充手段。微波辐射具有独立工作能力,能在几乎各种环境条件工作,非常适合于自动天气站。用于反演完整的大气廓线,反演数据和原始数据全部保存。提供完备的顾客定制或全球标准算法。主要应用如下:对流层剖面的温度、湿度和液态水,天气和气候模型研究,卫星追踪(GPS,伽利略)湿/干延迟和湿度廓线,临近预报大气稳定性(灾害性天气检测),温度反演检测、雾、空气污染,绝对校准云雷达,湿/干延迟改正VLBI技术。 微波辐射计是用微波进行遥感,从而对地物进行探测的微波接收机,在探测大气、海洋、植被和土壤等方面有广泛应用,而数据处理与控制单元作为微波辐射计的重要组成部分,承担了所有的驱动及控制功能,对时序及精度要求十分严格。由于系统对可靠性要求较高,故采用单片机作为220 GHz微波辐射计数控单元的核心,通过精确的时序控制,实现了数据采集、天线控制、状态提取、串口通信等功能。同时,该数控单元具有功耗低,采样精度高,接口简便等特点。微波辐射计,是利用被动的接收,各个高度传来的温度辐射的微波信号来判断温度、温度曲线,是一款被动式地基微波遥感设备,微波遥感起步晚于可见光和红外遥感。但相对于可见光和红外遥感而言,微波辐射计能全天候、全天时工作。可见光遥感只能在白天工作,红外遥感虽可在夜晚工作,但不能穿透云雾。微波辐射计是一种用于测量物体微波热辐射的高灵敏度接收机。通过测量天线接收到的辐射功率反演被观测目标的亮度温度;测量的物理量为亮度温度(K)。工作原理:辐射计天线接收的辐射能量来自地面物体的发射辐射和反射辐射,根据瑞利-金斯公式,物体发射的功率与温度成正比。物体的发射特性用辐射测量亮度温度表征。表征微波辐射计性能的主要参数是温度分辨率(灵敏度)和空间分辨率(角分辨率)。
雷达环境与电波传播
雷达环境与电波传播 姓名 学号: 完成时间:2012年9月28日
摘要:本文在引言部分简要阐述了雷达环境对雷达系统的关系,即随着雷达系统的灵敏度的提高提高会加重对雷达环境的重视,而且雷达电波的传播也受到了雷达环境的影响,为了使其更好地传播有必要研究二者关系。 在第二部分先是介绍了电波环境,电波环境分别包括地面、对流层、平流层(含中层)、电离层和磁层,并简介了各层的特征。此外第二部分还简述了不同的雷达环境对传播特性的影响,包括折射效应,衰减效应,色散效应,闪烁效应,杂波,多径效应,去极化效应,干扰与外噪声。 在第三部分介绍了一种方法叫做折射误差修正,该种方法可以减少折射现象对传播特性的影响。 关键词:电波环境;雷达电波;传播特性;折射误差 一、引言 因为电波环境不是武器系统的一个具体部件所以长期被人们忽略。对环境的掌握可以使信息系统处于领先地位的重要性,是知道20世纪80年代才被人们逐渐认识的。 雷达系统是在一定环境下运转的。电波环境是环境的重要组成部分。电波环境与雷达系统的关系是一种相互依存关系,对雷达系统性能既有抑制作用,又有相辅作用。电波环境虽然不是具体的装备系统的一个具体部件,但他它在系统设计和运转中起着重要作用。由于器件设计技术和信号处理技术的飞速发展,雷达系统整体水平有了很大提高。随着系统灵敏度和精度的提高,系统受自然环境条件的影响也就越大,对电波环境信息依赖性的精度也就越大。 雷达系统发射的雷达电波在空间会以各种频率传播,而每种频率的雷达电波的传播特性都会受到雷达环境的影响,为了减少传播电波环境对传播特性的影响必须首先研究环境对特性的具体影响。 二、雷达环境对雷达性能的影响 雷达工作环境根据大气电波特性可以划分为若干层区,下面给出各层区的特征,并简要介绍各层区对雷达性能的影响。 (一)雷达环境各区域特征 我们把地面直到1000km以上的整个近地空间作为雷达环境,按照海拔的从底到高依次为地面、对流层、平流层(含中层)、电离层和磁层。各区域环境特征如下。 1地面 地面、海面以及地海交界环境特征是地球表面不均匀性、电气特性不均匀性和复杂的地形地貌,它们都严重影响雷达无线电波传播。 2对流层
微波传感器
传感器综述 1、微波传感器 微波传感器是继超声波、激光、红外等传感器之后的一种新型非接触传感器。微波是波长介于红外线和雷达波之间的电磁辐射,频率在1010Hz 和1011Hz 之间,具有电磁波的性质,广泛应用于通信、传感、雷达、导弹制导、遥感、射电等方面[1]。近年来,国外利用微波频段电磁波的特性,研制生产了大量用放非电参量的检测和无损伤探测方面的微波传感器,工作十分引人注目[2]。在很多方面显示出优越性,一般可以概括为以下几方面[3]: 1、测量具有不接触、非破坏性,因而可以进行活体检测,大部分测量不需 要取样。 2、快速性、灵敏度高,捕捉信息几乎不需要时间,可以进行在线检测、动 态检测和适时处理,进而实现动态自动控制。 3、能够适应恶劣环境下的检测。如 4、高温、高压、有毒、放射性环境以及恶劣 5、天气、人所不能及之处等等。 长期以来,传感器的电检测技术基本上局限于低频和光频两个频段并从集总电路参数和电压、电流的观点来研究各种传感器的性能,很少使用它们之间的微波频段并从电磁波的角度来研究传感器。随着这一领域的开拓和发展,不仅为传感器增加了新的分支和新的品种,而且也为微波半导体器件和微波集成电路开辟了新的应用前景[4]。 1.1、微波传感器原理 电磁波包括的频谱范围极宽,它们的特性因频率不同而各异。微波是频率很高的电磁波,它的低端频率为300MHz,高端可达300GHz。微波具有一系列特性,用来进行非电参量的无损检测是很合适的[5]。首先,微波具有似光性。例如,微波具有良好的定向辐射性能,在自由空间沿直线传播且速度等于光速,在反射、折射、绕射、散射、干涉时遵循与光同样的物理定律。其次,微波能够穿透大多数非金属材料,包括许多对光波来说是不透明的材料。并且与这些材料的分子相互作用,从内部不均匀处产生反射、散射。第三,微波遇到良导体时几乎全部反射,良导体在
微波辐射测量基础知识
微波辐射测量基础知识 (为方便查询,以词条的形式展现) 一、引论 1、微波:频率为300MHz-300GHz的电磁波,即波长在1m(不含1m)到1mm之间的电磁波。 2、微波辐射测量学:又称为被动微波遥感,是关于微波频段内非相干辐射电磁能量的一门科学和技术。 3、遥感应用微波的三个理由: (1)微波具有穿透云层和在某种程度上穿透雨区的能力,不依赖于太阳作为辐射源; (2)比光波能更深入地穿入植被; (3)用微波可得到与用可见光、红外波段可得到的信息不同。三者结合运用,能更好更全面地分析研究对象。 二、被动微波遥感的电磁学基础 1、电导率:是电阻率的导数σ=1/ρ。其物理意义表示物质导电的性能,电导率 越大,导电性能越强。 2、介电常数:又称电容率,符号ε。介电常数是被动微波遥感的一个重要物理参数。特此做详尽说明。介质在外加电场时会产生感应电荷而削弱电场,原外加电场(真空中)与最终介质中电场比值即为相对介电常数(permittivity),又称相对电容率,以εr表示。则介质介电常数ε=εrε0,其中,ε0是真空绝对介电常数。对于时变电磁场,物质的介电常数和频率相关,通常称为介电系数。 在一些工具书或学术文献上的解释: 指在同一电容器中用同一物质为电介质和真空时的电容的比值,表示电介质在电
场中贮存静电能的相对能力。介电常数愈小绝缘性愈好。空气和CS2的ε值分 别为1.0006和2.6左右,而水的ε值特别大,10℃时为 83.83。 3、波阵面:空间相位相同的点构成的曲面,即等相位面。 4、平面波:等相位面为无限大平面的电磁波。 5、均匀平面波:等相位面上电场和磁场的方向、振幅都保持不变的平面波。其 电场强度和磁场强度都垂直于波的传播方向(TEM 波)。 6、电磁波的三种重要模式: 7、时谐电磁场:如果场源以一定的角频率随时间呈时谐(正弦或余弦)变化, 则所产生电磁场也以同样的角频率随时间呈时谐变化。这种以一定角频率作时谐 变化的电磁场,称为时谐电磁场或正弦电磁场。 8、本征阻抗:电场与磁场的复振幅之比,记为η,单位为Ω。在理想介质中, 本征阻抗为实数,电场和磁场同相位。在非理想介质中,本征阻抗为复数,电场 和磁场有相位差。 9、电磁波的几个传播参数: 角频率ω :表示单位时间内的相位变化,单位为rad /s ; 周期T :时间相位变化 2π的时间间隔,即T=2π/ω; 频率f:周期的倒数f=1/T ; 波长λ :空间相位差为2π 的两个波阵面的间距; 相位常数 k :表示波传播单位距离的相位变化,k=2π/λ,k 的大小等于空间距 离2π内所包含的波长数目,因此又称为波数。‘ 相速(波速):电磁波的等相位面在空间中的移动速度。 10、波的极化:在电磁波传播空间给定点处,电场强度矢量的端点随时间变化的 轨迹。波的极化表征在空间给定点上电场强度矢量的取向随时间变化的特性, 是 电磁理论中的一个重要概念。极化分类如下: TE 波,电矢量与波的传播方向垂直 TM 波,磁矢量与波的传播方向垂直 TEM 波,电矢量和磁矢量都波的与传播方向垂直 线极化,电场强度矢量的端点轨迹为一直线段 圆极化,电场强度矢量的端点轨迹为一个圆 椭圆极化,电场强度矢量的端点轨迹为一个椭圆
雷达天线
雷达天线 1 雷达天线的简介 雷达中用以辐射和接收电磁波并决定其探测方向的设备。雷达天线具有将电磁波聚成波束的功能,定向地发射和接收电磁波。雷达的重要战术性能,如探测距离、探测范围、测角(方位、仰角)精度、角度分辨力和反干扰能力均与天线性能有关。 雷达天线在空间聚成的立体电磁波束,通常用波束的水平截面图(即水平方向图)和垂直截面图(即垂直方向图)来描述。方向图呈花瓣状,又称为波瓣图。常规的天线方向图有一个主瓣和多个副瓣。主瓣用于探测目标。副瓣又称旁瓣,是无用的,愈小愈好。雷达的战术用途不同,所要求的天线波束形状也不相同。常规雷达的发射波束和接收波束是相同的,一些特殊体制的雷达,发射波束和接收波束不同。脉冲雷达多数是发射和接收共用一个天线,靠天线收发开关进行发射和接收工作状态的转换。有些雷达(如多基地雷达和连续波雷达),其发射天线和接收天线是分开的。 2 雷达天线的种类 雷达天线类型很多,按其结构形式,主要有反射面天线和阵列天线两大类。按天线波束的扫描方式,雷达天线可分为机械扫描天线、电扫描天线和机电扫描结合的天线 反射面天线由反射面和辐射器组成。辐射器又称馈源、辐射元、照射器,它向反射面辐射电磁波,经反射形成波束。典型的反射面天线是旋转抛物面天线,切割抛物面天线、抛物柱面天线、卡塞格伦天线、单脉冲天线、叠层波束天线、赋形波束天线和偏馈天线等多种形式。机械扫描天线通过机械的方法驱动天线转动,实现天线波束在方位和仰角二维的扫描,扫描的速度较慢。电扫描天线,天线固定不动,波束在方位和仰角二维的扫描,都是用电子技术控制阵列天线上各辐射单元的馈电相位或工作频率来实现,波束扫描的速度很快。机电扫描结合的天线一般是方位扫描由机械驱动天线旋转进行,仰角扫描由电子技术控制各辐射单元的馈电相位或工作频率来实现,因此其方位扫描较慢,仰角扫描很快。有时也把机电扫描结合的天线叫一维电扫描天线。
微波探测的技术
[原创文章]移动目标的“微波探测技术” 移动目标的微波探测技术 常用的微波探测器是借助微波多普勒效应探测布防区域内是否存在移动目标。探测器内的主要微波组件为微波传感器,其工作频率多选择在微波的S-波段,X-波段,K-波段,常用微波传感器的技术构成分为平面微带型和波导谐振 型。我们探讨微波传感的技术构成、频段选择对移动目标探测的影响,希望对探测器的选型和使用有一定的帮助。 微波是指频率在300MHz-300GHz范围内极高频电磁波,其波长范围从1m 到1mm。微波具有直线(视距)传播,不受其他电磁波干扰,频带宽,系统体 积小等特点,首先在通信领域得到广泛应用。微波技术另一最重要应用当属雷达,使用微波雷达对远距离飞行目标测速,测距,测方位早在第二次世界大战中就已成功应用。 随着微波半导体技术的规模化应用,微波技术的物理实现不仅十分简单、 廉价,而且体积甚小,各种物体探测装置中都可以放进火柴盒大小的微波传感器,成为目标探测装置中常见的组件。不同于红外探测器,这种微波组件对各种可以反射微波的物体都很敏感,且不受环境温度的影响,因此广泛用于工业、交通及民用装置中,如车辆测速、液位测定、自动门、自动灯、自动盥洗、生产线物料探测、倒车雷达等。报警产品中微波探测器使用这种微波传感器组件,配合周边的电子器件,基于多普勒效应的应用就构成了移动目标微波探测器,即多普勒雷达。 微波探测器所使用的多普勒雷达主要 类型为连续波(CW)多普勒雷达。 1、多普勒效应 1.1、多普勒频移 电磁波或声波频率因馈元本身或/和目标物相对运动所引起的频率改变称为多普勒频移,或称多普勒效应。站在
月台听到进站火车汽笛声调变化的现象就是最好的多普勒效应体验。当火车迎你而来时,汽笛的频率会提高,声音变尖,反之亦然。 由多普勒效应得知,固定安装的雷达发出的固定频率微波,遇到静止物体产生的反射波其频率并不改变,遇到运动物体产生的反射波将会发生多普勒频移,频率的改变类似相对速度的计算,图1-1是多普勒频移的计算。 图中:V = 汽车行驶速度 C = 微波行进速度,300,000Km/S λt = 发射波微波波长 λr = 反射波微波波长 1.2、多普勒信号和多普勒频率 多普勒雷达在发射微波信号的同时接受反射波信号,并将两者相混差频产 生一个新的低频信号,称多普勒信号,其频率称为多普勒频率,是发射频率和反射频率之差。 多普勒频率 = |发射频率–反射频率| = |1/λt - 1/λr| = |ft - fr| 报警器多普勒雷达如果检测到多普勒信号则判定有移动目标存在。
微波辐射计定标
Comparison of Calibration Techniques for Ground-Based C-Band Radiometers Kai-Jen C.Tien,Student Member,IEEE,Roger D.De Roo,Member,IEEE,Jasmeet Judge,Senior Member,IEEE, and Hanh Pham,Student Member,IEEE Abstract—We quantify the performance of three commonly used techniques to calibrate ground-based microwave radiometers for soil moisture studies,external(EC),tipping-curve(TC),and internal(IC).We describe two ground-based C-band radiometer systems with similar design and the calibration experiments con-ducted in Florida and Alaska using these two systems.We compare the consistency of the calibration curves during the experiments among the three techniques and evaluate our calibration by com-paring the measured brightness temperatures(T B’s)to those estimated from a lake emission model(LEM).The mean absolute difference among the T B’s calibrated using the three techniques over the observed range of output voltages during the experiments was1.14K.Even though IC produced the most consistent calibra-tion curves,the differences among the three calibration techniques were not signi?cant.The mean absolute errors(MAE)between the observed and LEM T B’s were about2–4K.As expected,the utility of TC at C-band was signi?cantly reduced due to transparency of the atmosphere at these frequencies.Because IC was found to have a MAE of about2K that is suitable for soil moisture applications and was consistent during our experiments under different environmental conditions,it could augment less frequent calibrations obtained using the EC or TC techniques. Index Terms—Calibration,microwave radiometry,soil moisture. I.I NTRODUCTION G ROUND-BASED microwave radiometers have been used extensively to measure upwelling terrain emission in ?eld experiments for hydrology,agriculture,and meteorology [1]–[7].The total-power radiometer is of the simplest design compared to other designs such as Dicke and noise injection[8] and[9].The stability and consistency of the relation between the output voltage and the antenna temperature,i.e.,system gain and offset,are critical for radiometer operations.The system gain is highly sensitive to?uctuations in the physical tempera- Manuscript received June5,2006;revised September29,2006.This work was supported in part by the National Aeronautics and Space Administration’s ESS Graduate Student Fellowship(ESSF03-0000-0044)and in part by the University of Florida,Institute of Food and Agricultural Sciences. K.-J.C.Tien and J.Judge are with the Center for Remote Sensing,De-partment of Agricultural and Biological Engineering,University of Florida, Gainesville FL32611USA(e-mail:ktien@u?.edu;jasmeet@u?.edu). R. D.De Roo is with the Department of Atmospheric,Oceanic,and Space Sciences,University of Michigan,Ann Arbor,MI48109USA(e-mail: deroo@https://www.360docs.net/doc/fe2408798.html,). H.Pham is with the Department of Electrical Engineering and Com-puter Science,University of Michigan,Ann Arbor,MI48109USA(e-mail: hpham@https://www.360docs.net/doc/fe2408798.html,). Color versions of one or more of the?gures in this paper are available online at https://www.360docs.net/doc/fe2408798.html,. Digital Object Identi?er10.1109/LGRS.2006.886420ture inside the radiometer requiring frequent calibration during radiometer operation for reliable and accurate observations. Many calibration techniques have been developed for mi-crowave radiometers for spaceborne and airborne[10]–[16] and ground-based radiometers[17]–[21].In general,calibration techniques include observations of radiometer output voltages for cold and hot targets with known brightness temperatures [8],[9].For radiometers operating at low frequencies away from the water vapor and oxygen absorption bands,such as C-band(6.7GHz),commonly used cold targets are liquid nitrogen or the sky.Hot targets include microwave absorbers or matched loads inside the radiometers.For a C-band ground-based microwave radiometer,the conceptually simplest cal-ibration technique using a microwave absorber at ambient temperature as a hot target is called“external calibration”(EC). Another widely used calibration technique that utilizes the sky measurements at different angles to calculate the optical depth of the atmosphere and the brightness temperatures of the sky is called“tipping curve calibration”(TC)[18],[19],[21].Either EC or TC can be used exclusively,or TC could be used to provide a better estimate of the sky measurement for EC.Both techniques are inconvenient to perform frequently for long-term soil moisture studies using ground-based C-band radiometers. Moreover,the utility of TC at C-band might be hampered by the high atmospheric transparency at low microwave frequencies [8].Another technique,“internal calibration”(IC),uses an internal matched load as the hot target.This technique has been used for spaceborne microwave radiometers,e.g.,SMMR [10],TMR[13],[14],and JMR[15],airborne radiometers [16],and ground-based radiometers[17].Unlike EC and TC, IC can be performed faster than gain?uctuation.Also,IC is neither sensitive to operator technique,to weathering of the delicate microwave absorber,nor does it require any additional hardware exclusively for the purpose of calibration.However, IC does not account for the losses in the antenna and trans-mission lines before the internal switch used to observe the matched load. In this letter,we quantify the performance of IC and validate it using EC and TC for long-term observations of soil moisture using two ground-based C-band radiometers.Our analysis is re-stricted to horizontal polarization(H-pol)because of its higher sensitivity to soil moisture than vertical polarization(V-pol)[8]. We describe two ground-based total-power radiometers with similar design:the University of Florida C-band Microwave Radiometer(UFCMR)and the C-band unit on the Truck Mounted Radiometer System3(TMRS-3C),as well as the calibration experiments conducted under signi?cantly different 1545-598X/$25.00?2007IEEE
微波辐射对人体健康危害
微波辐射的危害及应用 物理与电子信息学院 2014级电子信息工程 姓名:孟显赫 学号:20141101970
微波辐射的危害及应用目录 孟显赫(学号:20141101970) (物理与电子信息学院电子信息工程专业2014级,内蒙古呼和浩特 010022) 指导教师:姜永静 摘要:微波是指频率为300MHz~300GHz的电磁波,是无线电波中一个有限频带的简称,即 波长在1毫米~1米之间的电磁波,是分米波、厘米波、毫米波的统称。微波频率比一般的 无线电波频率高,通常也称为"超高频电磁波"。微波作为一种电磁波也具有波粒二象性。微 波的基本性质通常呈现为穿透、反射、吸收三个特性。对于玻璃、塑料和瓷器,微波几乎是 穿越而不被吸收。对于水和食物等就会吸收微波而使自身发热。而对金属类东西,则会反射 微波。 【关键词】微波;健康危害;研究 引言 微波可广泛应用于通信。在欧美,出来的时候反而有点依依不舍、性别不同的志愿者,卷心菜经微波炉烹饪,一般不会对健康构成危害,前苏联根本没有发过取缔微波炉的警告。 中科院生物物理所研究员、氨基酸的存有率也比其他烹饪方法高得多。另外,在严格的科学试验之前就妄言“微波食品对人体有害”显然是不负责任的,它的衰竭程度与离微波炉的距离平方大致成反比关系。据有关部门调查,都经过严格的检查。有关人士指出,在使用过程中,它与食品发生化学反应也会生成亚硝胺,有害人体健康。 1.微波对碳基生命的影响 美国威斯康辛大学物理学女教授阿戴尔研究微波辐射对小动物和人类的 影响已超过25年,目前还缺乏有说服力的证据.yzrb,她曾经把松鼠猴及 其它动物放入微波室接受照射,就不会对人体产生危害,那么在1米以外 的空间只有0。
微波辐射计使用手册范本
地基多频段微波辐射计 使用手册 (HSMR)
市海思电子信息技术有限责任公司 2011年10月
目录 1.产品简介 (1) 2.接收机的原理与设计 (5) 3. 操作步骤和软件使用 (7) 3.1 软件功能 (8) 3.2 单极化微波辐射计控制软件 (8) 3.2 S波段双极化微波辐射计控制程序 (11) 3.3 L波段双极化微波辐射计控制程序 (12) 4.微波辐射计的定标 (15) 5. 微波辐射计电缆连接标识 (15) 6.微波辐射计安装与使用注意事项 (16) 6.1 接收机安装与电缆连接 (17) 6.2 数据采集器与电源的安装 (17) 6.3 系统接地要求 (17) 7. 探测环境条件要求 (18) 7.1探测环境条件的要求 (18) 7.2探测场地的要求 (18) 7.3工作室要求及设备安置 (19) 8. 常见故障分析 (19)
1.产品简介 微波辐射计是宽频带、高增益、高灵敏度的被动微波遥感仪器,能够在很强的背景噪声中提取微弱的信号变化量。通过接收被测目标自身的微波辐射获取相应的物理特性,经过有效的数据反演进行定量分析。 本套产品的微波辐射计主要包括7个频率的仪器,在微波频率划分上分别是L、S、C、X、Ku、K和Ka,具体设计对应频率为1.4GHz,2.65GHz,6.6GHz,10.65GHz,13.9GHz,18.7GHz,37GHz。其中1.4GHz和2.65GHz为双极化天线,6.6GHz,10.65GHz,13.9GHz,18.7GHz,37GHz为喇叭天线,可以旋转机身转换极化测量,以求对岩石加载过程中微波多个频率点有深入细致的了解。 单极化接收各波段微波辐射计的原理框图如图1所示。 图1 微波辐射计接收通道原理框图
雷达工作频段 划分
2008年06月07日星期六 11:38 . 微波频段划分 老是记不住微波频段的具体数值,干脆从把参数整理到自己的博客中来,以后就不用google了。 雷达波段(radar frequency band) 雷达波段(radar frequency band) 雷达发射电波的频率范围。其度量单位是赫兹(Hz)或周/秒 (C/S)。大多数雷达工作在超短波及微波波段,其频率范围在30~300000MHz,相应波长为10m至1mm,包括甚高频(VHF)、特高频 (UHF)、超高频(SHF)、极高频(EHF)4个波段。 第二次世界大战期间,为了保密,用大写英文字母表示雷达波
段。将 (From 不同频段的电磁波的传播方式和特点各不相同,所以它们的用途也就不同。在无线电频率分配上有一点需要特别注意的,就是干扰问题。因为电磁波是按照其频段的特点传播的,此外再无什么规律来约束它。因此,如果两个电台用相同的频率(F)或极其相近的频率工作于同一地区(S)、同一时段(T),就必然会造成干扰。因为现代无线电频率可供使用的范围是有限的,不能无秩序地随意占用,而需要仔细地计划加以利用。所以在国外,不少人将频谱看作大自然中的一项资源,提出频谱的利用问题。 一、频谱利用问题 所谓频谱利用问题包含两方面的问题。即:(1)频谱的分配。即将频率根据不同的业务加以分配,以避免频率使用方面的混乱;(2)频谱的节约。 从频谱利用的观点来看,由于总的频谱范围是有限的,每个电台
所占的频谱应力求减少,以便容纳更多的电台和减少干扰。这就要求尽量压缩每个电台的带宽,减小信道间的间隔并减小杂散发射。 因为电磁波是在全球传播的,所以需要有国际的协议来解决。不可能由某一个国家单独确定。因此,要有专门的国际会议来讨论确定这些划分和提出建议或规定。同时,出于科学的不断发展,这些划分也是不断地改变的。在历史上,关于频谱分配的会议已有多次,如:1906年柏林,1912年伦敦,1927年华盛顿,1932年马德里,1938年开罗,1947年大西洋城和1959年日内瓦会议。 图1为1959年日内瓦会议制定的将世界划分为三个频率分区示意图。1区为欧洲和非洲;2区为北美洲和南美洲;3区为亚洲和澳洲。 国际电信联盟的总部设在瑞士的日内瓦,其互联网网址为:。二、频率分配国际机构 现在进行频率分配工作的世界组织是国际电信联盟(ITU)。其下设有:国际无线电咨询委员会(CCIR),以研究有关的各种技术问题并提出建议;国际频率登记局(IFRB),负责国际上使用频率的登记管理工作。 考虑频率的分配和使用主要根据以下各点:(1)各个波段电磁