The Subdwarf Luminosity Function
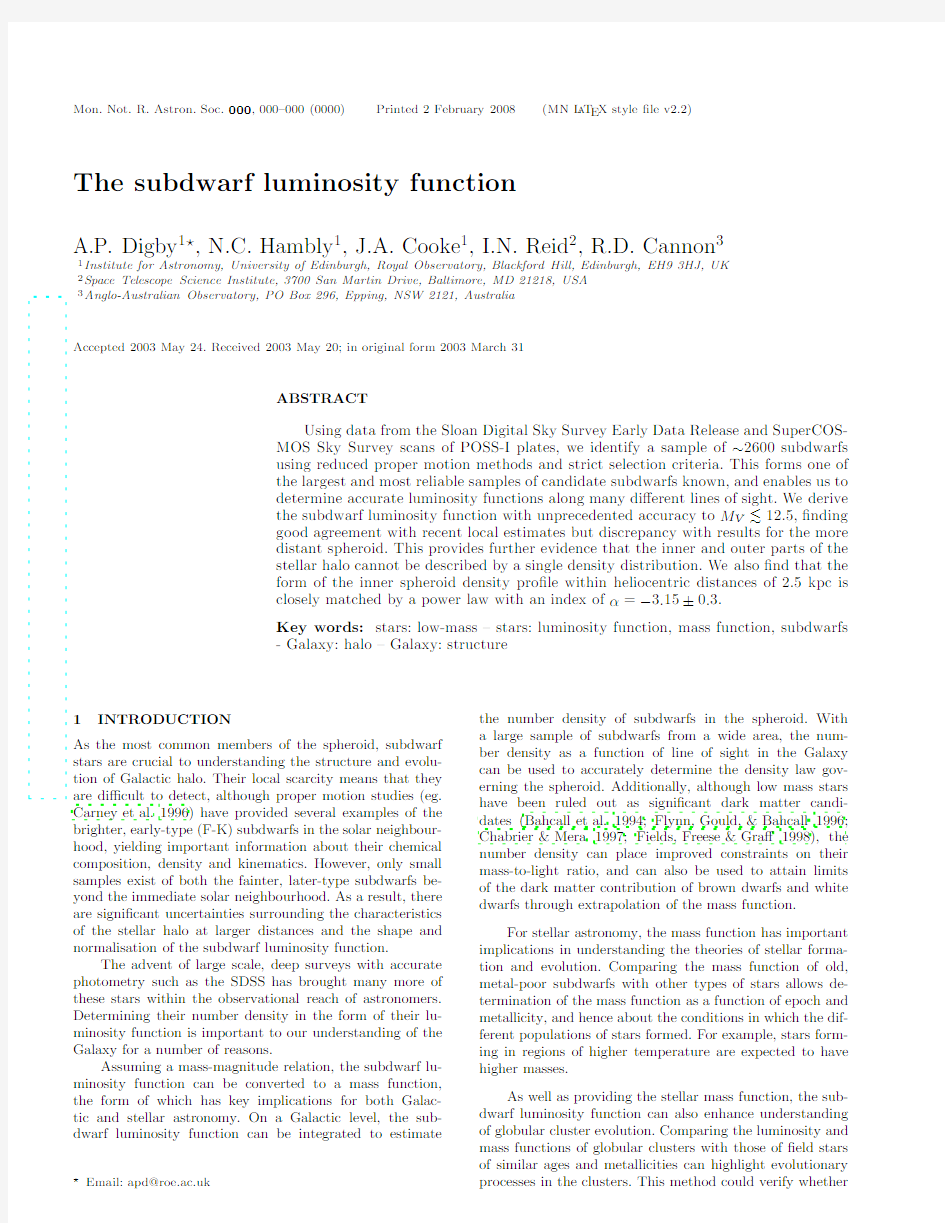
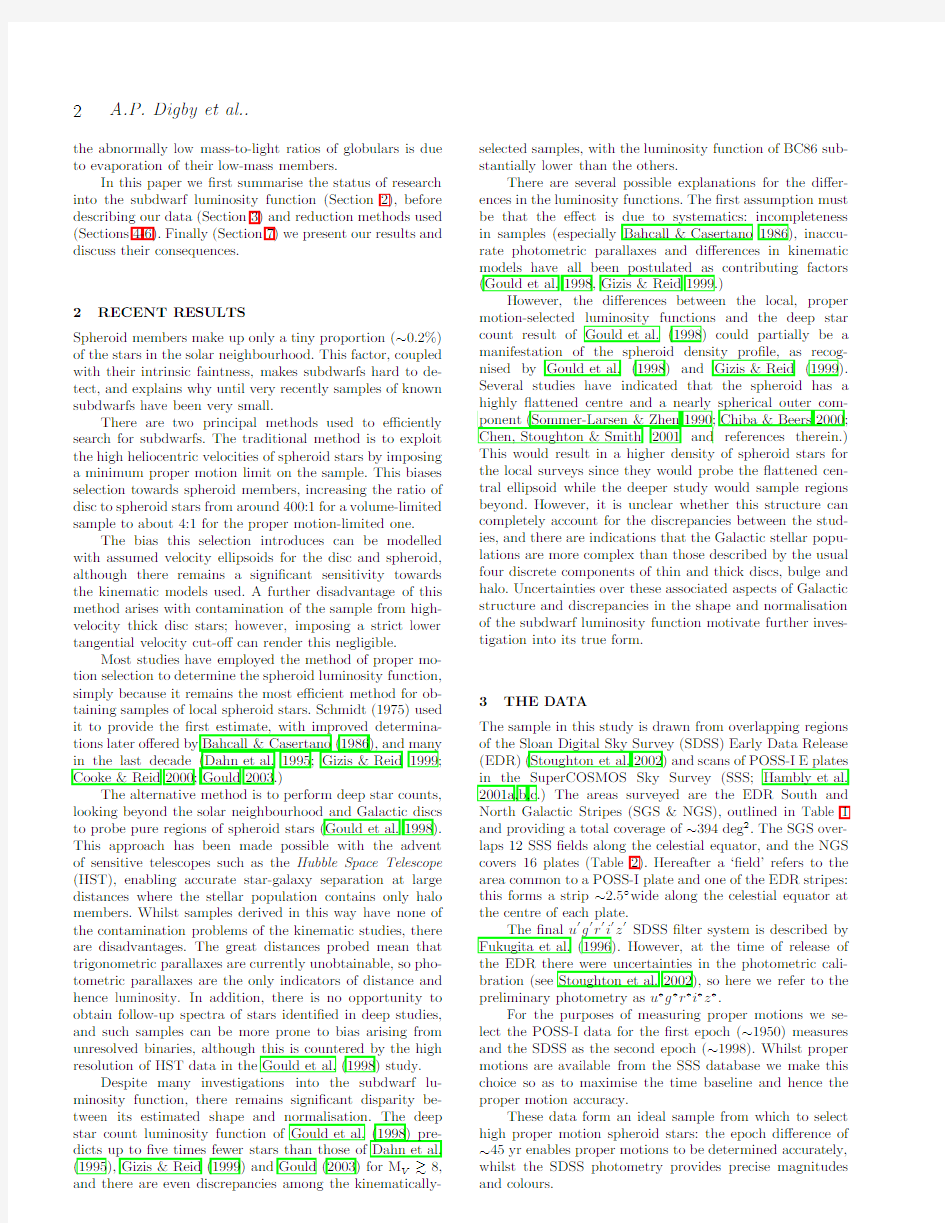
a r X i v :a s t r o -p h /0304056v 2 29 O c t 2003
Mon.Not.R.Astron.Soc.000,000–000(0000)Printed 2February 2008
(MN L A T E X style ?le v2.2)
The subdwarf luminosity function
A.P.Digby 1?,N.C.Hambly 1,J.A.Cooke 1,I.N.Reid 2,R.D.Cannon 3
1
Institute for Astronomy,University of Edinburgh,Royal Observatory,Blackford Hill,Edinburgh,EH93HJ,UK
2Space
Telescope Science Institute,3700San Martin Drive,Baltimore,MD 21218,USA
3Anglo-Australian Observatory,PO Box 296,Epping,NSW 2121,Australia
Accepted 2003May 24.Received 2003May 20;in original form 2003March 31
ABSTRACT
Using data from the Sloan Digital Sky Survey Early Data Release and SuperCOS-MOS Sky Survey scans of POSS-I plates,we identify a sample of ~2600subdwarfs using reduced proper motion methods and strict selection criteria.This forms one of the largest and most reliable samples of candidate subdwarfs known,and enables us to determine accurate luminosity functions along many di?erent lines of sight.We derive the subdwarf luminosity function with unprecedented accuracy to M V 12.5,?nding good agreement with recent local estimates but discrepancy with results for the more distant spheroid.This provides further evidence that the inner and outer parts of the stellar halo cannot be described by a single density distribution.We also ?nd that the form of the inner spheroid density pro?le within heliocentric distances of 2.5kpc is closely matched by a power law with an index of α=?3.15±0.3.
Key words:stars:low-mass –stars:luminosity function,mass function,subdwarfs -Galaxy:halo –Galaxy:structure
1INTRODUCTION
As the most common members of the spheroid,subdwarf stars are crucial to understanding the structure and evolu-tion of Galactic halo.Their local scarcity means that they are di?cult to detect,although proper motion studies (eg.Carney et al.1996)have provided several examples of the brighter,early-type (F-K)subdwarfs in the solar neighbour-hood,yielding important information about their chemical composition,density and kinematics.However,only small samples exist of both the fainter,later-type subdwarfs be-yond the immediate solar neighbourhood.As a result,there are signi?cant uncertainties surrounding the characteristics of the stellar halo at larger distances and the shape and normalisation of the subdwarf luminosity function.
The advent of large scale,deep surveys with accurate photometry such as the SDSS has brought many more of these stars within the observational reach of astronomers.Determining their number density in the form of their lu-minosity function is important to our understanding of the Galaxy for a number of reasons.
Assuming a mass-magnitude relation,the subdwarf lu-minosity function can be converted to a mass function,the form of which has key implications for both Galac-tic and stellar astronomy.On a Galactic level,the sub-dwarf luminosity function can be integrated to estimate
?
Email:apd@https://www.360docs.net/doc/1c4710903.html,
the number density of subdwarfs in the spheroid.With a large sample of subdwarfs from a wide area,the num-ber density as a function of line of sight in the Galaxy can be used to accurately determine the density law gov-erning the spheroid.Additionally,although low mass stars have been ruled out as signi?cant dark matter candi-dates (Bahcall et al.1994;Flynn,Gould,&Bahcall 1996;Chabrier &Mera 1997;Fields,Freese &Gra?1998),the number density can place improved constraints on their mass-to-light ratio,and can also be used to attain limits of the dark matter contribution of brown dwarfs and white dwarfs through extrapolation of the mass function.For stellar astronomy,the mass function has important implications in understanding the theories of stellar forma-tion and https://www.360docs.net/doc/1c4710903.html,paring the mass function of old,metal-poor subdwarfs with other types of stars allows de-termination of the mass function as a function of epoch and metallicity,and hence about the conditions in which the dif-ferent populations of stars formed.For example,stars form-ing in regions of higher temperature are expected to have higher masses.
As well as providing the stellar mass function,the sub-dwarf luminosity function can also enhance understanding of globular cluster https://www.360docs.net/doc/1c4710903.html,paring the luminosity and mass functions of globular clusters with those of ?eld stars of similar ages and metallicities can highlight evolutionary processes in the clusters.This method could verify whether
2 A.P.Digby et al..
the abnormally low mass-to-light ratios of globulars is due to evaporation of their low-mass members.
In this paper we?rst summarise the status of research into the subdwarf luminosity function(Section2),before describing our data(Section3)and reduction methods used (Sections4-6).Finally(Section7)we present our results and discuss their consequences.
2RECENT RESULTS
Spheroid members make up only a tiny proportion(~0.2%) of the stars in the solar neighbourhood.This factor,coupled with their intrinsic faintness,makes subdwarfs hard to de-tect,and explains why until very recently samples of known subdwarfs have been very small.
There are two principal methods used to e?ciently search for subdwarfs.The traditional method is to exploit the high heliocentric velocities of spheroid stars by imposing a minimum proper motion limit on the sample.This biases selection towards spheroid members,increasing the ratio of disc to spheroid stars from around400:1for a volume-limited sample to about4:1for the proper motion-limited one.
The bias this selection introduces can be modelled with assumed velocity ellipsoids for the disc and spheroid, although there remains a signi?cant sensitivity towards the kinematic models used.A further disadvantage of this method arises with contamination of the sample from high-velocity thick disc stars;however,imposing a strict lower tangential velocity cut-o?can render this negligible.
Most studies have employed the method of proper mo-tion selection to determine the spheroid luminosity function, simply because it remains the most e?cient method for ob-taining samples of local spheroid stars.Schmidt(1975)used it to provide the?rst estimate,with improved determina-tions later o?ered by Bahcall&Casertano(1986),and many in the last decade(Dahn et al.1995;Gizis&Reid1999; Cooke&Reid2000;Gould2003.)
The alternative method is to perform deep star counts, looking beyond the solar neighbourhood and Galactic discs to probe pure regions of spheroid stars(Gould et al.1998). This approach has been made possible with the advent of sensitive telescopes such as the Hubble Space Telescope (HST),enabling accurate star-galaxy separation at large distances where the stellar population contains only halo members.Whilst samples derived in this way have none of the contamination problems of the kinematic studies,there are disadvantages.The great distances probed mean that trigonometric parallaxes are currently unobtainable,so pho-tometric parallaxes are the only indicators of distance and hence luminosity.In addition,there is no opportunity to obtain follow-up spectra of stars identi?ed in deep studies, and such samples can be more prone to bias arising from unresolved binaries,although this is countered by the high resolution of HST data in the Gould et al.(1998)study.
Despite many investigations into the subdwarf lu-minosity function,there remains signi?cant disparity be-tween its estimated shape and normalisation.The deep star count luminosity function of Gould et al.(1998)pre-dicts up to?ve times fewer stars than those of Dahn et al. (1995),Gizis&Reid(1999)and Gould(2003)for M V 8, and there are even discrepancies among the kinematically-selected samples,with the luminosity function of BC86sub-stantially lower than the others.
There are several possible explanations for the di?er-ences in the luminosity functions.The?rst assumption must be that the e?ect is due to systematics:incompleteness in samples(especially Bahcall&Casertano1986),inaccu-rate photometric parallaxes and di?erences in kinematic models have all been postulated as contributing factors (Gould et al.1998,Gizis&Reid1999.)
However,the di?erences between the local,proper motion-selected luminosity functions and the deep star count result of Gould et al.(1998)could partially be a manifestation of the spheroid density pro?le,as recog-nised by Gould et al.(1998)and Gizis&Reid(1999). Several studies have indicated that the spheroid has a highly?attened centre and a nearly spherical outer com-ponent(Sommer-Larsen&Zhen1990;Chiba&Beers2000; Chen,Stoughton&Smith2001and references therein.) This would result in a higher density of spheroid stars for the local surveys since they would probe the?attened cen-tral ellipsoid while the deeper study would sample regions beyond.However,it is unclear whether this structure can completely account for the discrepancies between the stud-ies,and there are indications that the Galactic stellar popu-lations are more complex than those described by the usual four discrete components of thin and thick discs,bulge and halo.Uncertainties over these associated aspects of Galactic structure and discrepancies in the shape and normalisation of the subdwarf luminosity function motivate further inves-tigation into its true form.
3THE DATA
The sample in this study is drawn from overlapping regions of the Sloan Digital Sky Survey(SDSS)Early Data Release (EDR)(Stoughton et al.2002)and scans of POSS-I E plates in the SuperCOSMOS Sky Survey(SSS;Hambly et al. 2001a,b,c.)The areas surveyed are the EDR South and North Galactic Stripes(SGS&NGS),outlined in Table1 and providing a total coverage of~394deg2.The SGS over-laps12SSS?elds along the celestial equator,and the NGS covers16plates(Table2).Hereafter a‘?eld’refers to the area common to a POSS-I plate and one of the EDR stripes: this forms a strip~2.5?wide along the celestial equator at the centre of each plate.
The?nal u′g′r′i′z′SDSS?lter system is described by Fukugita et al.(1996).However,at the time of release of the EDR there were uncertainties in the photometric cali-bration(see Stoughton et al.2002),so here we refer to the preliminary photometry as u?g?r?i?z?.
For the purposes of measuring proper motions we se-lect the POSS-I data for the?rst epoch(~1950)measures and the SDSS as the second epoch(~1998).Whilst proper motions are available from the SSS database we make this choice so as to maximise the time baseline and hence the proper motion accuracy.
These data form an ideal sample from which to select high proper motion spheroid stars:the epoch di?erence of ~45yr enables proper motions to be determined accurately, whilst the SDSS photometry provides precise magnitudes and colours.
The Subdwarf Luminosity Function3 Table1.SDSS EDR regions
North Galactic Stripe145?-236?0?228
Table2.The survey?elds in the EDR SGS&NGS regions
South Galactic Stripe
09320343+0028186-401954.0 2.66
03630319+0031181-451951.7 4.60
14530255+0035175-491955.8 4.61
12830231+0038168-531954.9 4.59
08520207+0041159-561953.8 4.61
03620143+0044149-591951.7 4.64
12590119+0046138-611954.8 4.61
11960055+0047125-621954.7 4.58
05910031+0048112-621952.7 4.59
03190007+0048100-601951.6 4.60
04312343+004890-581951.9 4.64
08342319+004880-541953.8 1.81
4 A.P.Digby et al..
(2)Small scale errors are then removed by a one-dimensional error mapper,which calculates x and y errors as a function of both x and y by evaluating the mean shift for stars in each 2arcminute strip in both directions over the ?eld.Median and then linear ?lters are applied to smooth and reduce the noise in the functions,which are then used to again shift the star and galaxy SDSS positions with respect to the SuperCOSMOS measures.This process is iterated un-til the mean shifts in each of the x and y strips is less than a tolerance level (0.008times the rms x or y shift.)
(3)The two-dimensional error mapper is then applied again to remove any large scale shifts introduced by the one-dimensional algorithm.
(4)At this point the stars have zero mean proper mo-tion,whilst the mean galaxy displacement is non-zero,since stars dominate the number counts at these magnitudes.The galaxies are used to shift the proper motion zero point by de-termining a least-squares ?t of the galaxy positions to ?nd a six-coe?cient linear plate model accounting for zero-point,scale and orientation.A global translation is then applied using this model to reset the galaxies to zero mean proper motion,with the SDSS measures transformed by x SDSS =a +bx SSS +cy SSS y SDSS
=
d +ex SSS +fy SSS
where a,b,c,d,e and f are the plate coe?cients determined from the least-squares ?t to the galaxy positions.
The results of applying this error mapper can be seen in Figure 1,which shows a typical survey ?eld covering a strip along the centre of the Schmidt plate that is 6.?25wide and 2.?
5high.The ?rst panel shows the ?eld after the SSS mean large-scale distortion algorithm has been used,but before the error mapping algorithm described above has been ap-plied,with the large scale ‘swirl pattern’of the photographic data still evident.After the error mapper there are only very small residual systematic errors in position,and the remain-ing random errors have an rms of only ~0.3arcsec.4.4
Photometric-dependent astrometric errors
Systematic biases dependent on the magnitude of targets are particularly prevalent in photographic astronomy,and could a?ect the selection or derived astrometric parameters of stars.These systematic magnitude errors cannot be cor-rected for in the data,since this could remove real e?ects present,such as brighter stars tending to have higher proper motions due to their mean closer proximity to the Sun.How-ever,as shown in Figure 2,the in?uence of these errors is likely to be small for the high proper motion sample:their size is only 8mas yr ?1(with a ~45-yr epoch di?erence)for a sample selected with a proper motion cut ?ve times larger (see Section 4.6.2),and they have a systematic varia-tion with magnitude only at the ~0.1arcsec level (~2mas yr ?1).Additionally,this systematic error applies to the error di?erence with magnitude across the whole 15 r ? 19.5range,whereas the subdwarf sample is dominated by stars with r ? 17(Chen et al.2001;Gould 2003).The more pronounced errors at r ? 16seen in Figure 2are therefore unlikely to have a large e?ect on the derived proper motions in this study,and any magnitude-dependent errors should not lead to signi?cant astrometric or selection e?ects.
Figure 1.Before and after the error mapper is applied in ?eld 0363in the SGS,which covers a 2.?5-high strip along the centre of the Schmidt plate.The ‘lollipops’show the relative size of the binned,smoothed and ?ltered positional errors at each point in the ?eld.Note that the binning and smoothing means that these plots show systematic,not random,errors and that the scale of the errors in the bottom ?gure is ?ve times smaller than that in the top ?gure.
Figure 2.The rms of the random positional errors in x and y as a function of magnitude for a typical ?eld in the NGS,once the error mapping algorithm has been applied.In the magnitude range of interest (dashed lines)there is only variation with mag-nitude at the ~0.1arcsec level,and the errors are smallest where the majority of the subdwarf sample is found (r ?≈18).These considerations indicate that systematic magnitude e?ects will not signi?cantly a?ect the derived astrometric parameters.
The Subdwarf Luminosity Function5 Similarly,systematic colour errors are expected to have
little e?ect on the astrometry of the sample.Biases aris-
ing from variations in atmospheric e?ects are unlikely since
all observations were taken on or near the meridian,and the
telescopes used in the survey are at similar latitudes:Apache
Point Observatory where the SDSS observations were made
is at32?N46’,and the Palomar Observatory is at33?N21’.
Discrepancies in positions between the SDSS and SSS data
due to di?erential colour refraction will also contribute only
a small e?ect,since the SDSS r?and POSS-I E passbands
are very similar(centred atλ~6400?A),and only relative re-
fraction di?erences are important when determining proper
motions.
4.5Proper motion accuracy
4.5.1Quasar motions
The proper motions of all the stars in each?eld are mea-
sured after error mapping,and an external check on the
accuracy of these is obtained from analysis of the quasars
in each?eld.The Veron and Veron10th QSO catalogue
(Veron-Cetty&Veron2001)and the SDSS QSO catalogue
(Schneider et al.2002)provide a mean of135and172
quasars per?eld in our data for the SGS and NGS respec-
tively,which are used to estimate the proper motion accu-
racy.
The mean spatial discrepancies between the quasars’
SDSS and SuperCOSMOS coordinates are divided by the
epoch di?erence to estimate the rms proper motion error
for each?eld,under the assumption that the quasars should
have zero motion.Due to variations in the quality of the
plate data this error deviates signi?cantly from?eld to?eld,
but is~5.0-9.0mas yr?1for the SGS and~5.0-10.0mas
yr?1for the NGS.However,it should be noted that the true
proper motion errors of the proper motion sample are liable
to be less than these values,since the quasars are fainter and
have poorer centroiding than the majority of the subdwarfs
in this study.The zero point of the quasar proper motions
is consistent with zero,as shown in Figure3.
4.5.2Comparison with SSS proper motions
The accuracy of the derived proper motions can also be as-
certained by comparison with those published in the Super-
COSMOS Sky Survey(Hambly et al.2001c).These are mea-
sured from the B J and R plates of the survey,with system-
atic positional errors removed as in this study(Section4.3)
and di?erential colour refraction e?ects also accounted for.
The SSS proper motions are currently derived from data at
two epochs,with a median epoch di?erence between the B J
and R plates of15yr,although future releases will soon
utilise measures from four plates where available.
To compare the proper motions derived here with the
SSS results we have analysed SSS UKJ/UKR?eld866,
which overlaps the POSS-I?elds1440and1424in the NGS.
The B J and R plates in?eld866have an epoch di?erence
of17yr,so with good plate quality this comparison uses
SSS proper motions that are likely to be of above average
accuracy.
The stars in our sample are paired with the SSS data,
with image quality criteria(such as restrictions on blended
Figure3.Proper motion zero points as measured from quasars
for the NGS?elds(points)and the combined NGS dataset(open
circle).
objects,see Section4.6.1)applied as in our analysis.Our
proper motions derived from the SDSS/SSS data are then
compared with the SSS proper motions for each star.Fig-
ure4shows this comparison for stars with15.0 r? 19.5,
along with1σand2σdeviations from a perfect correlation,
assuming standard deviations of10mas yr?1and8mas
yr?1for the SSS and SDSS/SSS proper motions respectively.
There is good correlation between the measures,with a lin-
ear correlation coe?cient of~0.85and~99%of the stars
falling with the2σerror bars,indicating negligible system-
atic e?ects.Note that there are virtually no stars that have
discrepantly high SDSS/SSS proper motions compared to
the SSS measures,although there are some for which the
SSS proper motion estimate appears to be signi?cantly too
high.This is likely to be due to the facts that the SSS re-
sults are derived from a smaller time baseline and from pho-
tographic material at both epochs,increasing the likelihood
of objects with spurious proper motions entering the SSS
sample.The excellent consistency of the SDSS/SSS proper
motions compared to the SSS measures for stars with high
SDSS/SSS proper motions demonstrates the good reliability
of our strict high proper motion sample selection and sug-
gests negligible contamination from objects with false mo-
tions.
4.6The high proper motion sample
4.6.1Image quality criteria
Prior to the proper motion cut being applied,the data are
subject to criteria to ensure that only objects with stellar
6 A.P.Digby et
al..
Figure https://www.360docs.net/doc/1c4710903.html,parison of the proper motions derived in this study (μSDSS /SSS )with those from the SuperCOSMOS Sky Sur-vey (μSSS )in NGS ?elds 1424and 1440.Some ~99%of stars fall within the 2σboundaries of a perfect correlation,and the linear correlation coe?cient is 0.85.The lack of stars along the μSDSS /SSS axis shows close agreement between the SDSS/SSS measures and the corresponding SSS estimates for stars with high SDSS/SSS proper motions,demonstrating the good relia-bility and negligible contamination of our high proper motion sample.
and high quality images are included in the ?nal sample.From the SSS data the following characteristics of each im-age are restricted (see Hambly et al.2001a for more details):?Blend:Objects appearing as blended in the SSS database are rejected.
?Pro?le Classi?cation Statistic,η:This quanti?es the ‘stellarness’of an object by comparing the residuals of the areal pro?le of an image with that of an average stellar tem-plate.Objects with η 4σare rejected.
?Quality:During processing the SSS data are assigned a quality ?ag,which is a?ected by circumstances such as an image being very large,bright,fragmented or close to a bright star or plate boundary.Images with a quality value of greater than 127are rejected.
?Ellipticity:The ellipticity of an image is calculated from the weighted semi-minor and semi-major axes given by the SSS processing.Only objects with e 0.25are included in the sample.4.6.2
Proper motion selection
A lower proper motion limit is applied to the sample of paired stars for two principal reasons:to amplify the pro-portion of spheroid stars selected and to create a ‘cleaner’proper motion sample.The proper motion selection magni-?es the contribution from the higher-velocity spheroid popu-lation,since they are e?ectively sampled over larger volumes than the lower-velocity disc stars (Reid 1997;Cooke &Reid 2000).The number of stars of each population in a proper
motion selected sample is proportional to the mean popula-tion tangential velocity:N (μ>μmin )∝ρ0 V T 3,
(1)
with ρ0the local space density of the population (Hanson 1983;Reid 1984).This therefore ampli?es the contribution of the higher velocity population above the ratio of the local space densities by the amount:
A μ=
V 1T
The Subdwarf Luminosity Function
7
Figure 5.The numbers of high proper motion stars in the SGS (solid line)and NGS (dashed line),expressed as a fraction of the total number of paired stars and plotted as a function of magni-tude.For the magnitude range of the sample (between the dotted lines)the proportion in each stripe agree to within a few per-cent,indicating no systematic errors in the derivation of proper motions or high proper motion selection.
5METHODS:THE SUBDW ARF SAMPLE 5.1
Reduced proper motion
Although applying a lower proper motion limit produces a cleaner sample of fast-moving stars,this will consist not only of subdwarfs but also white dwarfs and high velocity members of the disc.In order to identify the candidate sub-dwarfs,the reduced proper motion of each star is used as a discriminator.De?ned as
H =m +5log μ+5=M +5log V T ?3.379,
(4)
this parameter separates e?ectively high-velocity (V T ),low-luminosity (M)subdwarfs from the white dwarf and thin disc populations.This is seen in the reduced proper motion diagram (RPMD)of H r ?plotted against (r ??i ?):Figures 6and 7show the RPMDs for all stars with μ>μmin in the SGS and NGS respectively.The fainter and higher tangential velocity subdwarfs form a distinct sequence below the thin disc main sequence,whilst the white dwarfs populate the bluest part of the diagram.
The colour index (r ??i ?)was chosen because of its ef-fectiveness at separating subdwarfs from higher-metallicity stars on colour-magnitude diagrams,due to their lower opac-ities and higher temperatures at a given mass and luminosity resulting in a larger proportion of their ?ux being emitted at optical wavelengths (Gizis 1997;Gizis &Reid 1999).The (r ??i ?)index is a good temperature/spectral type indica-
tor for the M dwarfs which dominate this survey (see Sec-tion 5.5.1;Lenz et al.1998;Hawley et al.2002),so on a re-duced proper motion or colour-magnitude diagram it enables clear distinction between the solar-metallicity stars and the hotter (bluer)subdwarfs at each absolute magnitude.The (r ??i ?)colour is similar to (R ?I C ),proven to be e?ective at separating subdwarfs in this manner,and covers the TiO and CaH spectral features important for subdwarf classi?ca-tion (Gizis 1997;Gizis &Reid 1999).We investigated other appropriate SDSS indices for use in the RPMD,but (r ??i ?)proved to be the most e?ective for subdwarf identi?cation.5.2
Reddening and extinction
With all the ?elds at high Galactic latitudes (|b | 37?),interstellar extinction and reddening are unlikely to have a signi?cant impact on this study.To con?rm this we use the high-resolution COBE/DIRBE dust maps of Schlegel et al.(1998)to estimate the reddening and extinction at each ?eld centre,using the given wavelength coe?cients to convert E B ?V and A V to E r ??i ?and A r ?.We ?nd that the majority (23out of 28)of the SGS and NGS ?elds have E r ??i ?<0.04,and that the overall mean is E r ??i ?=0.03.The maximum reddening is E r ??i ?=0.08(for ?eld 0932),and only three ?elds have E r ??i ? 0.05,two of which are narrow ?elds at the end of a stripe and hence contain only a small fraction of the total sample.In applying these estimates we assume that there is no di?erential reddening across the ?elds,and that all obscuration is in the foreground.This assumption is valid for our study,since the reddening layer scale height is ~100pc (Chen et al.1999),and our survey is sensitive to very few stars within this distance (see Section 5.5.2.)
With photometric accuracy of ~3%in the r ?and i ?bands of the EDR (Stoughton et al.2002),the size of the reddening e?ect is comparable to that of the σr ??i ?≈0.04colour errors.Inspection of the reduced proper motion dia-grams (Figures 6and 7)shows that adjustments of this order would have little e?ect on the “tightness”of the population locii and subsequent subdwarf selection.The mean extinc-tion over all ?elds is A r =0.126,which if not corrected for would result in derived photometric parallaxes being under-estimated by up to ~5%,less than the expected distance errors.These considerations suggest that the e?ects of red-dening and extinction on the study are negligible,and so corrections are not applied to the data.5.3
Tangential velocity cut-o?
Although the RPMD separates the subdwarfs from the thin disc stars and white dwarfs,there is some overlap on the dia-gram with the locus of thick disc stars.These have relatively high velocities,and hence are responsible for the objects ly-ing between the old disc and subdwarf sequences.Thick disc stars have a much higher local number density than subd-warfs,and the inclusion of even a tiny proportion of this population in the sample can result in a signi?cant overesti-mation of the spheroid density (Bahcall &Casertano 1986).
However,the contamination of the sample by these stars can be avoided by the introduction of a cut-o?in tangen-tial velocity.Figure 8shows calculated tangential velocity distributions of the disc and spheroid populations in the di-rection of one of the SGS ?elds,assuming a velocity ellipsoid
8 A.P.Digby et
al..
Figure 6.The RPMD for stars with μ μmin in the SGS.The three separate sequences of white dwarfs,subdwarfs and thin disc stars are
clear.
Figure 7.The RPMD for stars with μ μmin in the NGS.
described by a solar motion and Gaussian dispersions (Table 3)and following the method given in Murray (1983,p285).
For our assumed velocity ellipsoids we adopt the disc
kinematics derived from two analyses of local M dwarfs (Reid,Hawley &Gizis 1995;Hawley,Gizis &Reid 1996),and the spheroid ellipsoid derived from the study of a large
Figure 8.Simulated tangential velocity distributions for the thin and thick disc and spheroid (in order of increasing velocity)in the direction of ?eld 0363in the SGS
Table 3.Adopted velocity ellipsoids.The assumed disc (Reid et al.1995;Hawley et al.1996)and spheroid (Chiba &Beers 2000)stellar kinematics used in the tangential velocity calculations.All speeds are in kms ?1,and U,V,W denote the usual Galactic coordinate triad in the respective directions of the Galactic center,direction of rotation and NGP.
Spheroid -26-199-1214110694Thin Disc 10-5-7412721Thick Disc 10-23-7524532
The Subdwarf Luminosity Function
9
and closely follow
the majority of ?eld star data,espe-cially in the infrared (Bara?e et al.1997,1998;Chabrier 2003).However,the metal-rich versions ([m/H] ?1.0)of these models su?er from discrepancies with the lower end of the observed main sequence (V ?I C 2.0)in op-tical colours (Bara?e et al.1998;Chabrier 2003).Whilst lower-metallicity models are expected to be less a?ected in this way,when matched to ?eld stars they still yield poor reproductions of the observed colours at redder wave-lengths (Bara?e et al.1997;Chabrier 2003),and exhibit possible systematic errors in metallicity when used to es-timate abundances from colour-magnitude diagrams (Gizis 1997;Gould et al.1998).These factors could signi?cantly a?ect the ability of the models to provide the reliable opti-cal colour-magnitude relations required here,calculating an expected absolute magnitude for each subdwarf given an ob-served colour and metallicity estimate.Therefore,whilst the latest theoretical model atmospheres provide good matches to most of the stellar physics and observed parameters,the possibility of systematic colour o?sets,perhaps wavelength-dependent,leads us to choose to employ an empirical colour-magnitude relation for this study.
An obstacle in applying a colour-magnitude relation to halo stars is the large range of metallicities present in the spheroid,and hence the spread of absolute magnitude for a given colour.Without spectra to obtain metallicities we deal with this degeneracy in two ways:by using one approx-imate colour magnitude relation for selecting subdwarfs on the RPMD,and a more accurate estimate which predicts metallicity from observed colours for deriving photometric parallaxes.5.4.2
The colour-magnitude relation for selecting subdwarfs
The colour-magnitude relation used to select subdwarfs from the RPMD is obtained in a similar way to that of Gizis &Reid (1999).We derive separate relations for low and high metallicity subdwarfs,following the classi?cations de?ned by Gizis (1997)of stars with [Fe/H]≈?1.2±0.3as subwarfs (sd),and with [Fe/H]≈?2.0±0.5as extreme subwarfs (esd).
To derive a colour-magnitude relation we use calibrat-ing subdwarfs from the compilations of Gizis (1997)and Reid et al.(2001).Both of these studies use the spectral classi?cation scheme described in Gizis (1997)to identify subdwarfs,and give accurate trigonometric parallaxes for their samples.These are derived principally from the USNO (Monet et al.1992)and Yale (4th ed;van Altena et al.1995)catalogues in the case of Gizis,and from the Hipparcos catalogue (Perryman et al.1997)in the case of Reid et al.(2001).
We consider only subdwarfs with σπ/π<0.2so as to en-sure accuracy of the absolute magnitudes,producing a total of 35subdwarfs and extreme subdwarfs from Gizis,and 38from Reid et al.We plot these stars on a M I ,(V ?I C )Hertzsprung-Russell diagram (Johnson V and Cousins I C ),and ?t a colour-magnitude relation to the sdM and esdM separately.Stellar colour-magnitude relations are poorly matched by a linear relation due to an in?ection in the sub-dwarf main sequence at V-I C ~1.5(Bara?e et al.1997),so we match a cubic spline to the parallax data (Figure 9.)
Figure 9.Colour-magnitude relations derived from subdwarfs with accurate trigonometric parallaxes.Squares denote subdwarfs and triangles extreme subdwarfs;?lled symbols are subdwarfs from Gizis (1997)and open symbols are those from Reid et al.(2001)
There is currently no published SDSS photometry for stars with accurate trigonometric parallaxes,so it is nec-essary to ?rst derive the colour-magnitude relation in the standard Johnson/Cousins photometric systems,and then convert the colours and magnitudes to SDSS r ?and r ??i ?.This is done by means of the relation M r ?=r ??V +(V ?I C )+M I ,
(5)
combined with two-colour relations to convert from SDSS (r ??i ?)to (r ??V )and (V ?I C ).
These two-colour relations should ideally be obtained from subdwarfs with accurate photometry and similar metallicities to those in our sample.However,of the sub-dwarfs in the SDSS Standard Star Catalogue (Smith et al.2002),very few have published V I C photometry and span only a small range in colour.We therefore ?t linear two-colour relations between the Johnson/Cousins and SDSS systems for higher-metallicity disc stars instead.Despite this,applying these ?ts to the few subdwarf stars with SDSS and V I C magnitudes suggests that the disc metallicity ?ts apply well to the spheroid metallicity stars.The relations derived are
r ??V =?0.889(r ??i ?)?0.040,(6)V ?I C =2.097(r ??i ?)+0.429,(7)
and are shown in Figure 10.5.4.3
Selecting candidate subdwarfs
With an approximate colour-magnitude relation with which to estimate M r ?given (r ??i ?)for an assumed subdwarf,we can make use of Equation (4)to plot lines of constant V T on the RPMD.
Figure 11shows the RPMD for the high proper motion stars in one of our ?elds (POSS-I ?eld 0465in the NGS),with the lines corresponding to 200and 500km s ?1that we use to identify our candidate subdwarfs.These limits clearly provide a good match to the observed subdwarf se-quence,and are applied on a ?eld-by-?eld basis to account
10 A.P.Digby et
al..
Figure10.Two-colour relations from the SDSS to John-son/Cousins photometric systems for disc stars in the SDSS stan-dard star catalogue.There is little to suggest that these relations are strongly dependent on metallicity.
for the varying kinematics between?elds.The lower veloc-ity bound is applied to avoid contamination from thick disc stars,whereas the upper limit is applied to prevent selec-tion of white dwarfs.With knowledge of the expected tan-gential velocity
distributions for the disc and spheroid stel-lar populations(Section 5.3)we can estimate the fraction of spheroid and thick disc stars that are expected to fall within this velocity range to compensate for selection e?ects (Section6.4).The numbers of high proper motion stars and subdwarfs passing all selection criteria(including metallicity constraints for subdwarfs)are shown in Table4.
5.5Metallicities and photometric parallaxes
5.5.1Estimating metallicities
The division of the subdwarf colour-magnitude relation into two metallicity ranges is clearly a poor approximation,and one that is unsuitable for use in obtaining photometric paral-laxes of the candidate stars.The spread of nearly two magni-tudes in luminosity between metal-rich and metal-poor sub-dwarfs at(V?I C)≈2could lead to signi?cant errors in the distance estimates,so a more robust method which ac-counts for the absolute magnitude-metallicity degeneracy is required.
The highly accurate?ve band photometry of the SDSS is ideally suited for estimating metallicities from colours. The photometric precision is su?cient to clearly separate stars of di?ering metallicities when plotted in metallicity-sensitive colours such as(u??g?)and(g??r?)(Figure13). We use all?ve photometric bands matched to model atmo-Table4.The numbers of high proper motion stars(N hpm)and subdwarfs(N sd)passing all magnitude,proper motion,quality and metallicity selection criteria for each?eld.
SGS NGS
093229615
036375768
145370381
128374785
085270980
036281491
125980489
1196790107
059187596
031986484
043184790
083432032
015125644
1402703143
1613700125
1440814145
1424866145
0465910164
1595853130
1578802128
1405947125
14011005125
0471947111
140081389
139770469
046776276
047060056
131828221
Figure11.The RPMD for high proper motion stars in NGS ?eld0465,with the subdwarf(solid lines)and extreme subdwarf (dashed lines)selection lines corresponding to V T=200and500 kms?1overlaid.
sphere grids in order to derive a metallicity estimate for each subdwarf candidate.
The model atmospheres of Lenz et al.(1998)use Ku-rucz ATLAS9models(Kurucz1993)to give grids of four synthetic SDSS colours for stars with e?ective temperatures (T ef f)from3500to40000K and varying metallicity and log g.Adopting a value of log g=4.5and given the observed colours,we use a chi-square statistic and interpolate in the grids to estimate the best-?t temperature and metallicity
The Subdwarf Luminosity Function11
for each subdwarf.Stars with poor metallicity-temperature ?ts are eliminated by rejecting all candidates with a resid-ual greater than twenty times the rms value of the residuals of the grid?t.This leads to some3%of candidates being rejected,although their highly unusual colours means that most of these objects will not be subdwarfs.
It is clear from inspection of the metallicities derived that there is a zero point error in the estimates,with the median metallicity for each stripe of
[m/H]~?2.4consid-erably lower than the[Fe/H]~?1.5expected for spheroid stars(Laird et al.1988;Nissen&Schuster1991).There are a number of likely contributing causes of this:one is that the [m/H]=?5.0model only extends blueward of(g??r?)≈0.7,and so the metallicities of subdwarfs redder than this have to be extrapolated from the[m/H] ?2.0models.Even subdwarfs with(g??r?) 0.7rely on interpolation between the[m/H]=?5.0and?2.0models–a very large metallicity spread which will inevitably introduce errors.Additionally, inaccuracies in the model atmospheres will lead to uncertain-ties in the derived metallicities,particularly for redder stars. However,the observed o?set is largely irrelevant to this ap-plication as we are interested in only the relative metallicities of the sample.Aside from the zero point error,the derived
metallicities and temperatures show good consistency,both with the expected correlations on a temperature-colour di-
agram(Figure12)and in colour-colour plots(Figure13).
Additionally,the metallicity distributions of both the SGS and NGS are in close agreement(Figure14),indicating no
signi?cant systematic di?erence(whether intrinsic or not)
between the samples.
5.5.2Photometric parallaxes
With a metallicity estimate for each candidate subdwarf,a
metallicity-dependent colour-magnitude relation is used to
provide a more reliable indication of each star’s intrinsic lu-minosity.A median colour-magnitude relation M I is derived by?tting a single cubic spline to all the parallax subdwarfs
in Section5.4.2.The absolute magnitude M?I of each star in the sample is then estimated by o?setting from this median magnitude by an amount proportional to the star’s devia-tion from the sample median metallicity at that particular colour.The estimated absolute magnitude is given by:
M?I= M I+d[m/H]w dM I
σ[m/H] 2,(10)Figure12.The correlation between e?ective temperature and r??i?,with model predictions for[m/H]=?1.0,?2.0,?5.0(in order of increasing temperature at r??i?~0.2)from Lenz et al. (1998).For the majority of stars(those with r??i? 0.4)there is close agreement with the models and good internal consistency of the metallicity and temperature estimates.The poor?t of the models for r??i? 0.4is likely to be mostly due to less accurate temperature interpolations over this range,arising from the larger colour spread and the fact that the[m/H]=?5.0model does not extend to these redder wavelengths.However,since the majority of the sample lies blueward of r??i?≈0.5and the metallicities are not used in an absolute sense,the e?ect of these discrepancies will be limited.
whereσtrue
[m/H]
is the assumed intrinsic metallicity spread, given by the rms metallicity deviation from the median for 0.1 (r??i?) 0.25,andσ[m/H]is the rms metallicity spread at a given colour.This weighting therefore assumes that the metallicity range for the sample is independent of colour.
The derivative of absolute magnitude with respect to metallicity at each colour is derived from the theoretical colour-magnitude relations for stars of di?erent metallicities provided by Bara?e et al.(1997).The metallicity-absolute magnitude relation varies considerably with colour(Fig-ure15),but is approximately linear.The slope of this rela-tion is therefore a constant and is estimated from the mod-els for each star’s colour to provide the last term in Equa-tion(8).The models of Bara?e et al.(1997)and the paral-lax subdwarfs are given in the(M I,V?I C)colour plane; conversion to SDSS colours is achieved using Equation(5).
It is stressed that this modi?cation of the colour mag-nitude relation based upon estimated metallicity is only an approximation.It is only intended to adjust the predicted magnitude of each star according to its likely metallicity in the right direction and by roughly the right amount.In light of this the adjustment of absolute magnitude from the me-dian is restricted to a maximum of±1.5mag,to prevent
12 A.P.Digby et
al..
Figure 13.Colour-colour plots for subdwarfs in the SGS (top)and NGS (bottom),with model atmosphere predictions for stars of [m/H]=+1.0,0.0,?1.0,?2.0and ?5.0(from bottom to top)overlaid.There is no evidence for any systematic metallicity dif-ference between the
samples.
Figure 14.The estimated metallicity distributions for the SGS (solid line)and NGS (dashed line).Although the metallicities are clearly not accurate in an absolute sense from comparison with the [Fe/H]~?1.5expected for spheroid stars,there is good agreement between the samples and no systematic metallicity dif-ference between the two.The median metallicities of ?2.5for the SGS and ?2.3for the NGS agree well within the expected
errors.
Figure 15.The correlation of absolute magnitude with metal-licity from the models of Bara?e et al.(1997),for (V ?I C )from 1.0(top)to 2.8(bottom)in steps of 0.2.
any stars being assigned inappropriate values.However,it can be seen from the sample estimated colour-magnitude diagram (Figure 16)that this method provides a far more realistic and accurate distribution of absolute magnitudes than a simple division of the colour-magnitude relation into two metallicity ranges.
With an estimated absolute magnitude for each star a distance can then be derived,assuming no signi?cant red-dening e?ects due to the high latitude of the ?elds (|b | 37?).The distances sampled are in the range ~260pc to ~2.8kpc,and the distributions for the SGS and NGS are shown in Figure 17.That proportionally fewer stars with distances over 2kpc are found in the SGS compared to the NGS is an e?ect expected in a non-uniform spheroid den-sity distribution.Whereas the NGS is directed principally towards the inner quadrants of the Galaxy,the SGS lies to-wards the outer quadrants where the space density of stars will be lower with a non-uniform distribution.
5.6Tests for sample systematic errors
The luminosity functions derived from the two independent SGS and NGS samples not only provide an excellent probe of the spheroid density distribution,but also can be compared to ensure that our results are free from signi?cant systematic errors –a source of concern with earlier studies (Section 2).
Prior to comparing the results from each stripe,how-ever,we must ensure that the two samples are self-consistent and free from systematic e?ects.Inconsistency between the samples could arise from either a systematic disparity in the selection of each sample,or from an intrinsic di?erence in the constituent stars of each.
The Subdwarf Luminosity Function
13 Figure16.Estimated absolute magnitudes for all subdwarfs in
the SGS and NGS,derived from a median colour-magnitude rela-
tion?tted to calibrating subdwarfs(solid line)and o?set accord-
ing to the metallicity estimate of each star.
5.6.1Sampling systematic errors
Systematic errors arising from the sample selection are the
most likely,with a whole range of possible causes such as a
di?erence in astrometric or photometric accuracy between
the stripes.However,we can test for any signi?cant di?er-
ence of this type by investigating the completeness of each
subdwarf sample.We do this in a somewhat crude manner
by comparing cumulative proper motion number counts:as-
suming a uniform stellar density and that proper motion
and distance are inversely proportional(μ∝d?1),a plot
of log cumulative number count(ΣN)against logμshould
have a gradient of?3:
ΣN∝V∝d3∝μ?3.(11)
Figure
18shows the histogram of cumulative proper
motion counts for all paired stars in the NGS and SGS after
the error mapper has been applied.We?t a straight line to
the points between the global proper motion limits for each
stripe:μmin=40.5mas yr?1andμmax=160mas yr?1.
Both give a close?t to the expected gradient:?2.92±0.06
for the SGS and?2.99±0.06for the NGS.Similar plots
for just the candidate subdwarfs in each stripe are shown
in Figure19,where gradients of?3.10are found for each
stripe.
There is some evidence for incompleteness in the non-
linearity of the histograms,especially for the subdwarf sam-
ples where proper motion errors lead to incompleteness to-
wards the proper motion limits.This is particularly relevant
to the higher proper motions where the errors will be more
dominant,and this explains the rapid tailing o?of the distri-
butions for logμ 2.1caused by the imposition of the upper
proper motion limit.However,the number counts for the
population from which the subdwarfs are selected(Figure
Figure17.The distributions of estimated distances for the SGS
(top)and NGS(bottom).
18)demonstrate that it is complete for40.5mas yr?1 μ
160mas yr?1,and the good match of all of the histograms to
the expected gradient of?3indicate that there are no signif-
icant signs of incompleteness a?ecting the samples.It must
be also stressed that these are only approximate tests for
completeness,and will have some unreliability introduced
by the assumption of uniform stellar density,which is par-
ticularly invalid for stars at large distances which tend to
have lower proper motions.
5.6.2Radial metallicity gradient
An intrinsic di?erence between the samples that could have
a signi?cant e?ect on the results is a di?erence in median
metallicity between the SGS and NGS.Although the radial
14 A.P.Digby et
al..
Figure 18.Cumulative proper motion number counts for all stars in the SGS (top)and NGS (bottom).The straight line ?ts between the proper motion limits in each stripe are shown (dashed line),along with the expected line of gradient of ?3.0(dotted
line).
Figure 19.Cumulative proper motion number counts for can-didate subdwarfs in the SGS (lower line)and NGS (upper line).The straight line ?ts between 1.6 log μ 2.1(dashed lines)both have gradients of ?3.1
metallicity gradient of the inner spheroid is thought to be small (?[F e/H ]/?r ≈?0.012dex kpc ?1,Bekki &Chiba 2001),with both samples in opposite radial Galactic direc-tions this could nevertheless introduce a source of error.The assumption of a single colour-magnitude relation for both of the samples would mean that stars in the sample with the higher metallicity would have their absolute magnitudes overestimated,and hence their distances and contribution to
the luminosity function would be underestimated.The de-rived luminosity function of each sample would therefore be expected to di?er.This e?ect would be less pronounced with the photometric parallax relation de?ned in Section 5.5.2that partially accounts for metallicity variations,but is an issue that should still be addressed.
However,our data display little evidence of metallicity gradient.The two-colour diagrams for the subdwarf sample in each stripe in Figure 13show no systematic di?erence,and the estimated metallicity distribution and median metallic-ity of each (Figure 14)are very similar.It is therefore highly unlikely that there is any strong systematic metallicity dif-ference between the samples.
These checks of sample consistency indicate that there are no large systematic di?erences between the samples aris-ing from sampling errors or intrinsic variations.A further and ?nal test of the sample completenesses can be ascer-tained following the luminosity function calculations from the value of V /V max (Section 7).
6METHODS:THE LUMINOSITY FUNCTION 6.1
The generalised V max method
With a ?nal sample size of 918candidate subdwarfs in the SGS and 1696in the NGS,the luminosity function can be accurately derived.We achieve this using a modi?cation of the V max method of Schmidt (1968):each star contributes 1/V max to the luminosity function,where V max is the maxi-mum volume that the star could have been detected in,given the proper motion and magnitude limits of the survey.This technique therefore implicitly corrects for any bias arising from the proper motion selection.
Schmidt’s original 1/V max method assumes that the sample is selected from a uniformly-distributed popula-tion.This certainly is not the case for our subdwarf sam-ple;indeed,we intend to use it to determine the vari-ation in spheroid density.We therefore adopt the ‘gen-eralised V max ’method (Stobie,Ishida,&Peacock 1989;Tinney,Reid,&Mould 1993),which extends Schmidt’s method to non-uniformly distributed samples.Given that each star in the survey can be detected to minimum and maximum distances d min ,d max in a ?eld of solid angle ?,the luminosity function is de?ned as:Φsample =
1
n ⊙
ds,(13)
where s is the heliocentric distance and n (s )is the adopted spheroid density law (Section 6.3).6.2
Proper motion and magnitude limits
The distance limits depend on the minimum and maximum proper motion and magnitudes in each ?eld.The upper and lower proper motion limits employed are μmin =40.5mas yr ?1and μmax =160mas yr ?1(Section 4.6.2).
Ascertaining the magnitude limits for each ?eld is less straightforward as the data come from two quite di?erent sources,and there is signi?cant variation in the depth of
The Subdwarf Luminosity Function
15
Figure 20.The log number -magnitude histogram for all un-paired (top)and paired (bottom)stars in the NGS,with the approximate upper and lower r ?magnitude limits for the NGS ?elds shown.The actual magnitude limits adopted for each ?eld are ascertained from where the log(N (r ?))curve in the ?eld’s histogram departs from a steady increase.
the plate material.However,the fact that the datasets are paired and that the SDSS data probe much fainter (r ?~23)than the POSS-I plates means that only the r ?magnitudes need be considered.
The POSS-I plates reach incomplete levels at R ~20,at which magnitude the SDSS data are certainly complete.Stars need to appear in both datasets to pass the pairing criteria,so the faint magnitude limit can be de?ned solely in terms of the much more accurate SDSS r ?magnitude.A (log number,r ?magnitude)histogram is plotted for all of the paired stars in each ?eld,and an upper limit is conser-vatively de?ned from the magnitude at which log (N (r ?))departs from a steady increase (Figure 20;Table 5.)The brighter limit is less crucial due to the smaller likelihood of subdwarfs having such magnitudes,but a limit of r ?~15is applied to encompass the range that is likely to be complete as indicated by the histogram.
With proper motion limits μmin ,μmax and magnitude
limits r ?min
,r ?
max for each ?eld,the limiting distances for a subdwarf of distance d and magnitude r ?are therefore de?ned as d min =d max
μ
μmin
,10
0.2(r ?max ?r ?
)
.(15)
6.3The spheroid density law
Recent studies of the structure of the Galactic spheroid have
indicated that its density pro?le is ?attened and follows a power law:an axial ratio of (c/a)≈0.6and ρ(r )~r ?3(Gould et al.1998;Sluis &Arnold 1998;Yanny et al.2000;Ivezi′c et al.2000;Chen et al.2001;Siegel et al.2002;Gould 2003).We therefore adopt a spheroid density law of the form n
r 2c +R 2+z 2/q 2
α
,(16)
Table 5.Magnitude limits and spheroid and thick disc discovery fractions for SGS (top)and NGS (bottom)?elds.The minimum magnitude limit for all ?elds is r ?=15.0and the proper motion limits are μmin =40.5mas yr ?1and μmax =160mas yr ?1.
093218.761.150.01036319.562.560.01145319.063.570.01128319.264.160.02085219.164.390.02036219.264.270.02125919.163.850.03119619.163.130.03059119.362.130.04031919.260.850.04043119.059.310.04083418.957.500.04
where n ⊙is the local spheroid density,αis the power law index and q =(c/a )is the axial ratio,and we assume R 0=8.0kpc and r c =1.0kpc.Converting the Galactocentric cylindrical coordinates R and z in terms of heliocentric co-ordinates (s,l,b ),where s is the distance from the Sun and l and b are Galactic latitude and longitude:n
r 2c +R 20+s 2?2sR 0cos l cos b +s 2sin 2b 1?q
2
16 A.P.Digby et al..
tangential velocity calculations as described in Section https://www.360docs.net/doc/1c4710903.html,ing our adopted velocity ellipsoids,χh varies between 0.58and 0.64
in the SGS,and 0.49and 0.64in the NGS (Table 5),and scales the spheroid luminosity function as Φtrue h
=1
(χtd /χh )(n td /n h )+1
,(20)
where χtd and χh are the fractions of thick disc and
spheroid stars with 200km s ?1 V T 500km s ?1and n td and n h are the local number densities of thick disc and spheroid stars.The discovery fractions χtd and χh are known from the calculations described in Sec-tion 5.3,whilst the relative normalisation of thick disc to spheroid stars is taken from independent studies.Assum-ing a thick to thin disc density ratio of 1:10(Reid et al.1995;Chen,Stoughton &Smith 2001;Siegel et al.2002)and a combined disc to spheroid normalisation of 400:1(Chabrier &Mera 1997;Holmberg &Flynn 2000),we adopt a thick disc to spheroid ratio of n td :n h =40:1.With this consideration of thick disc contamination,the true spheroid luminosity function is therefore derived from Φtrue h
=λh
V 2max
.(22)
Allowing for scaling:
σ2Φtrue
=
λh
log Φ=
log Φ
σ2
log Φ
log Φ
=
1
dM V
.(26)
The derivative is evaluated from
M V
=M r ??(r ??V )
(27)=
M r ??[(?0.889(r ?
?i ?
)?0.040],
(28)
from Equation (6).By varying (r ??i ?),M r ?can be plot-ted against M V ,a spline ?tted and the derivative evaluated
(Figure 21).Hence dM r ?/dM V can be calculated for a given M r ?,and repeating this for each M r ?bin in the luminosity function completes the transformation.6.8
Constraining the spheroid density pro?le
With a wide range of lines of sight,the SGS and NGS sam-ples are ideally suited to constraining the density distribu-tion of the spheroid.Indeed,a non-uniform density law has
to be assumed in order to compare the luminosity functions of the di?erent samples.Figure 22shows the number densi-ties (luminosity function integrated over 5 M r ? 10)for each ?eld plotted against Galactic longitude when derived using a conventional V max method under the assumption of uniform space density.It is clear from comparison with the models that at the distances sampled the varying density of the spheroid has a strong e?ect and so has to be taken into account when deriving the luminosity function.
In order to constrain the density distribution a power law of the form given in Equation (17)is assumed,and sub-dwarf number densities are calculated for each ?eld in the SGS and NGS for a range of power law indices and axial ratios.The power law index αis allowed to vary from ?2.0to ?4.4in steps of 0.05,and the axial ratio from 0.2to 1.0in intervals of 0.05.
The generalised V max method (Section 6.1)should en-sure that the derived number densities are constant and in-dependent of line of sight,so how well a (α,q )power law model ?ts the data is ascertained by measuring how close
The Subdwarf Luminosity Function
17
Figure 21.The relations between M r ?and M V (solid line)and
dM r ?
σ2i +σ2j
,(29)
where n i and σi are the number density and its standard
deviation for each of nf ?elds.The best-?t (α,q )density model is therefore the model with the minimum value of χ2,and the likelihood of each model can be evaluated by comparing its statistic value with the minimum.
7RESULTS AND DISCUSSION 7.1
Spheroid density pro?le
Figure 23shows the results of ?tting spheroid density mod-els (α,q )to the combined SGS and NGS data,with con-tours of equal χ2plotted.Whilst the axial ratio q can-not be constrained with these data,the power law in-dex αcan.Although there is a slight degeneracy with q ,the best-?t power law index is α=?3.15±0.30for the range 0.55 q 0.85,which is the value of the axial ratio derived from recent studies (Sluis &Arnold 1998;Gould,Flynn &Bahcall 1998;Robin,Reyl′e ,&Cr′e z′e 2000;Chen,Stoughton &Smith 2001;Siegel et al.2002).This power law index is largely in agreement with the re-cent results of Gould et al.(1998)(α=?3.13±0.23);Sluis &Arnold (1998)(α=?3.2±0.3);Yanny et al.(2000)(α=?3.2±0.3);Ivezi′c et al.(2000)(α=?3);Chen,Stoughton &Smith (2001)(α=?2.55±0.3);Siegel et al.(2002)(α=?2.75)and Gould (2003)(α=?3.1±1.0).This r ?3distribution di?ers signi?cantly from
Figure 22.The subdwarf number densities (points)in each ?eld derived from a conventional V max method assuming uniform pop-ulation density.The lines show the expected relative number den-sities at a distance of 1kpc from a spheroid density power law with (α,q )=(?3.0,0.55).Is is clear that the uniform density assump-tion is invalid for this sample and so a non-uniform distribution must be assumed in the calculation of the luminosity functions.Accordingly,these data can be used to constrain the form of the density pro?le.
the r ?2power law of the dark matter halo,providing fur-ther evidence for the theory that faint halo stars consti-tute at most a negligible fraction of Galactic dark mat-ter (Bahcall et al.1994;Flynn et al.1996;Chabrier &Mera 1997;Fields et al.1998).
These results are only able to constrain q https://www.360docs.net/doc/1c4710903.html,-parison of the limits of q ≈0.55±0.06set by Chen et al.(2001)with the SDSS EDR data and inspection of Equa-tion (17)shows that this is due to the much smaller distances probed by this study.Small values of s in Equation (17)
cause the q term to be negligible compared to the r 2c +R 2
0terms,and so variations of l and b are insensitive to q .The low distances are a result of the relatively bright lower mag-nitude limit of r ?~19set by the POSS-I R plates;using a wider range of Galactic coordinates from future SDSS re-leases will help to overcome this degeneracy.
A similar problem a?icts independent analysis of the density distributions of the SGS and NGS.The smaller ranges of Galactic longitude and distances (Figure 17)sam-pled in the SGS and the decreased sensitivity to the density pro?le parameters in this direction means that neither αor q can be adequately constrained for the SGS taken in iso-lation.The NGS alone does allow limits to be placed on α
18 A.P.Digby et
al..
Figure23.Contours of equalχ2constraining the spheroid den-sity models(α,q).Although q cannot be adequately constrained with these data,we?ndα=?3.15±0.30.The dashed lines show the one standard error interval from the best-?tα.The standard error inαis calculated from bootstrap resampling.
and q,and these agree well with the values derived for the joint SGS/NGS sample,albeit with larger errors.
The standard error of theαderived for the SGS and NGS is obtained from bootstrap resampling.The best-?t αis calculated for each of1000bootstrap samples,all of the same size as the original sample and chosen from it by randomly selecting stars with replacement.The standard deviation ofαfor these bootstrap samples is an estimate of the standard error in the power law index.
7.2The subdwarf luminosity function
The combined luminosity functions of the SGS and NGS ?elds for the best-?t power lawα=?3.15are shown in Figure24(the luminosity functions for our samples are in-sensitive to the value of q.)The results for each stripe are in excellent accordance with each other,with all but one mag-nitude bin agreeing within the1σerror bars.This suggests that there are no systematic spatial e?ects in the analysis of the two samples.
There is also good agreement with the kinematic stud-ies of Gizis&Reid(1999),Dahn et al.(1995)(scaled by 0.75to account for Casertano,Ratnatunga,&Bahcall1990 kinematics as in Gould et al.1998)and Gould(2003).This indicates that the correction of our sample to the solar neighbourhood data by an r?3.15power law is a good ap-proximation,and that this distribution therefore well de-scribes the inner spheroid out to2.5kpc.The disagreement with the result of Gould et al.(1998)is pronounced,how-ever,and this may be due to systematic errors a?ecting their sample:for example,Gizis&Reid(1999)postulated that the choice of local calibrating subdwarfs in Gould et al. (1998)could cause their luminosity function to be underesti-mated.Alternatively,there could be a real e?ect behind the discrepancy.The inability of the Gould et al.(1998)data to match the local luminosity functions when?tted with a(α,q)=(?3.13,0.8)density power law indicates that this model does not accurately describe the spheroid.As we?nd that an r?3.15power law accurately?ts our data out to2.5kpc,this provides more evidence that a di?er-ence in the axial ratios of the inner and outer spheroid (Sommer-Larsen&Zhen1990)may be responsible for the discrepancy of the Gould et al.(1998)result.However,there is growing evidence that the usual four-component model for the Galaxy(with discrete thin and thick discs,halo and bulge)is inadequate,and that a model with more continu-ous distributions in stellar age,kinematics and metallicity is more appropriate(Chiba&Beers2000;Siegel et al.2002; Yanny et al.2003).It is possible that the de?ciencies of this over-simplistic picture of Galactic structure is behind the discrepancies seen here.
A further feature evident from Figure24is the preci-sion of our results.That our error bars are so small re?ects the size of our sample,with only the Gould(2003)lumi-nosity function exhibiting comparable errors.However,our subdwarf selection is more rigorous that of Gould(2003), who identi?ed~4500subdwarfs simply by taking cuts by eye in the reduced proper motion plane and whose sample is perhaps more susceptible to thick disc contamination.
Figure25plots the luminosity functions for stars of dif-ferent metallicities,with the samples divided into subdwarfs with metallicities either greater or less than the median of [m/H]≈?2.4.As in the study of Gizis&Reid(1999),we ?nd that the very metal-poor subdwarfs extend to fainter absolute magnitudes and tend to have a higher space den-sity than the more metal-rich ones.
7.3The V/V max test
The overall completeness of the subdwarf samples can be estimated by using the V/V max test.For each star the ratio of the volume V(corresponding to its distance d)to V max is calculated(Equation30),and the mean of this quantity should be0.5for a complete survey evenly sampling the survey volume.The error in this mean is1/(12N)1
V max =
d
The Subdwarf Luminosity Function
19
Figure 24.The combined luminosity function for the SGS (solid line,open circles)and NGS (solid line,open squares)assuming the best-?t spheroid density power law α=?3.15.The SGS and NGS results are in excellent agreement with each other and agree well with the results of Dahn et al.(1995)/Casertano et al.(1990)(dot-dash,asterisks),Gizis &Reid (1999)(dashed,?lled squares)and Gould (2003)(dotted,?lled triangles),but not with the outer spheroid sample of Gould et al.(1998)(solid line,?lled circles).This lends weight to the suggestion that a single power law cannot describe the density distributions of both the inner and outer spheroid.[Note that the Dahn et al.1995luminosity function has been scaled by 0.75to re?ect use of the Casertano et al.1990kinematics,as in Gould et al.1998.]The small error bars of this study’s luminosity function re?ect the much greater size of the subdwarf sample compared to the other kinematic studies;Gould (2003)has a larger sample size,although his is more likely to su?er from thick disc contamination.
8SUMMARY AND FUTURE WORK
We have demonstrated the e?ectiveness of the method of reduced proper motion at selecting spheroid stars,and have used it to obtain one of the largest samples of known spheroid subdwarfs.From this we derive the subdwarf lu-minosity functions to unprecedented accuracy in two dia-metrically opposite lines of sight in the Galaxy.
The large samples along di?erent lines of sight in this study have enabled us to constrain the form of the spheroid density distribution,out to heliocentric distances of 2.5kpc,to a power law with an index of α=?3.15±0.3.This is in accordance with other recent results (Gould et al.1998;Sluis &Arnold 1998;Yanny et al.2000;Ivezi′c et al.2000;Chen et al.2001;Siegel et al.2002).We are unfortunately unable to adequately constrain the spheroid ?attening pa-rameter q with this study (we can only rule out a very ?at spheroid),due to insu?cient survey depth.
Our luminosity functions agree well with other recent
local derivations,so that with this result the solar neigh-bourhood subdwarf luminosity function is now well de?ned to M V ≈12.5.Our data corrected by an r ?3.15spheroid density distribution closely match the local luminosity func-tions,suggesting that this power law well describes the in-ner spheroid density pro?le.On the other hand,our result further con?rms the discrepancy between the local luminos-ity functions and the outer spheroid luminosity function of Gould et al.(1998)corrected by an r ?3.1power law.This provides additional evidence that either the Gould et al.(1998)result is a?ected by systematic errors,or that the inner and outer spheroid follow quite di?erent density dis-tributions.
A progression of this study to larger volumes will enable limits to be placed on q and will provide stronger constraints on α.Adding the SIRTF First Look ?eld from the EDR will immediately increase the sample size by ~18%,and will provide additional lines of sight,important for determining
20 A.P.Digby et
al..
Figure 25.The luminosity functions for stars with metallici-ties either greater (dashed line)or less than (solid line)the me-dian metallicity of [m/H ]≈?2.4in the SGS (circles)and NGS (squares).As in Gizis &Reid (1999),we ?nd that more metal-poor subdwarfs have a higher space density.
Figure 26. V/V max for ?elds in the SGS and NGS .There is no evidence for incompleteness in the samples.
α.Progressive SDSS data releases will signi?cantly increase the scienti?c return of this work.With the ?rst formal SDSS data release (DR1)some 20%of the photometry is now avail-able,increasing to ~50%in January 2004.This represents a huge increase on the 5%from the EDR,and will mean that tens of thousands of subdwarfs will be found with the methods described in this paper.These will yield even more
accurate estimates of the subdwarf luminosity function and will provide much tighter constraints on the spheroid density law through the increase in sample size and lines of sight.
A further development of this study that is already un-der way is to obtain spectra of the candidate subdwarfs.Con?rmation of their spectral type is important for deter-mining the accuracy of the RPM selection methods,and for ascertaining the true level of thick disc contamination in the samples.In addition,radial velocities will enable the full 3D angular momentum properties of these spheroid stars to be investigated over large scales,with accuracy su?cient for the detection of spheroid kinematic substructure (see eg.Helmi et al.1999;Helmi &de Zeeuw 2000).Spectra of sev-eral hundred candidates have already been obtained using the 6dF multi-?bre spectrograph on the UKST at AAO.
A subsequent step to be made in the near future is to convert the luminosity functions into an accurate subdwarf mass function by use of a mass-magnitude relation.As de-scribed in Section 1,this has a number of important appli-cations,and is particularly important to the theories of star formation and evolution.Future analyses will also take into consideration the expected binarity fraction of the samples in the derivation of the luminosity functions.
This work clearly demonstrates the great potential of combining the old-style photographic surveys with the newer CCD programmes.This combination makes optimum use of both datasets,utilising the accurate astrometry and long time baselines available from surveys such as the SSS,whilst taking advantage of the accurate CCD photometry from such studies as the SDSS.
Acknowledgements
We are very grateful to the referee,Ra?aele Grat-ton,for prompt and helpful suggestions,and to Andy Gould and Gilles Chabrier for useful comments. A.P.D is supported by a UK PPARC studentship,and this work makes use of the SuperCOSMOS Sky Survey (https://www.360docs.net/doc/1c4710903.html,/sss/index.html)and the Sloan Digital Sky Survey Archive.
Funding for the creation and distribution of the SDSS Archive has been provided by the Alfred P.Sloan Founda-tion,the Participating Institutions,the National Aeronau-tics and Space Administration,the National Science Foun-dation,the U.S.Department of Energy,the Japanese Mon-bukagakusho,and the Max Planck Society.The SDSS Web site is https://www.360docs.net/doc/1c4710903.html,/.
The SDSS is managed by the Astrophysical Research Consortium (ARC)for the Participating Institutions.The Participating Institutions are The University of Chicago,Fermilab,the Institute for Advanced Study,the Japan Participation Group,The Johns Hopkins University,Los Alamos National Laboratory,the Max-Planck-Institute for Astronomy (MPIA),the Max-Planck-Institute for Astro-physics (MPA),New Mexico State University,University of Pittsburgh,Princeton University,the United States Naval Observatory,and the University of Washington.
REFERENCES
van Altena W.F.,Lee J.T.,Ho?eit E.D.,1995,New Haven,CT:Yale University Observatory,4th ed.
ABB机器人的手动操作
ABB[a]/-J-3ABB机器人的手动操作 3、1任务目标 ?掌握手动操作机器人运动的三种模式。 ?使用“增量”模式来控制机器人的运动。 ?熟练使用手动操纵的快捷方式。 ?掌握ABB机器人转数计数器更新操作。 3、2任务描述 手动操纵机器人运动一共有三种模式:单轴运动、线性运动与重定位运动。如何使用这三种模式手动操作机器人运动就是项目的主要内容。 建立一个工作站,ABB型号为IRB120,Y轴上建模长方体,长200mm,宽200mm,高400mm,在长方体的内角上进行重定位运动,之后恢复到机械远点。(手动操作练习需要教师指导,同时需要上机练习) 3、3知识储备 3、3、1手动操作三种模式 1、单轴运动 一般地,ABB机器人就是由六个伺服电动机分别驱动机器人的六个关节轴,那么每次手动操纵一个关节轴的运动,就称之为单轴运动。 图2 IRB 120机器人的关节轴 2、线性运动 机器人的线性运动就是指安装在机器人第六轴法兰盘上工具的TCP在空间中作线性运动。
3、重定位运动 机器人的重定位运动就是指机器人第六轴法兰盘上的工具TCP点在空间中绕着坐标轴旋转的运动,也可 以理解为机器人绕着工具TCP点作姿态调整的运动。 3、3、2RobotStudio中的建模功能 当使用RobotStudio进行机器人的仿真验证时,如节拍、到达能力等,如果对周边模型要求不就是非常细 致的表述时,可以用简单的等同实际大小的基本模型进行代替,从而节约仿真验证的时间。 如果需要精细的3D模型,可以通过第三方的建模软件进行建模,并通过*、sat格式导入到RobotStudio中 来完成建模布局的工作。 1、使用RobotStudio建模功能进行3D模型的创建 1.单击“新建”菜单命令组,创建一 个新的空工作站。 2.在“建模”功能选项卡中,单击 “创建”组中的“固体”菜单,选择 “矩形体”。
通信系统建模与仿真课程设计
通信系统建模与仿真课程设计2011 级通信工程专业1113071 班级 题目基于SIMULINK的基带传输系统的仿真姓名学号 指导教师胡娟 2014年6月27日
1任务书 试建立一个基带传输模型,采用曼彻斯特码作为基带信号,发送滤波器为平方根升余弦滤波器,滚降系数为0.5,信道为加性高斯信道,接收滤波器与发送滤波器相匹配。发送数据率为1000bps,要求观察接收信号眼图,并设计接收机采样判决部分,对比发送数据与恢复数据波形,并统计误码率。另外,对发送信号和接收信号的功率谱进行估计。假设接收定时恢复是理想的。 2基带系统的理论分析 1.基带系统传输模型和工作原理 数字基带传输系统的基本组成框图如图1 所示,它通常由脉冲形成器、发送滤波器、信道、接收滤波器、抽样判决器与码元再生器组成。系统工作过程及各部分作用如下。 g T(t) n 定时信号 图 1 :数字基带传输系统方框图 发送滤波器进一步将输入的矩形脉冲序列变换成适合信道传输的波形g T(t)。这是因为矩形波含有丰富的高频成分,若直接送入信道传输,容易产生失真。 基带传输系统的信道通常采用电缆、架空明线等。信道既传送信号,同时又因存在噪声n(t)和频率特性不理想而对数字信号造成损害,使得接收端得到的波形g R(t)与发送的波形g T(t)具有较大差异。 接收滤波器是收端为了减小信道特性不理想和噪声对信号传输的影响而设置的。其主要作用是滤除带外噪声并对已接收的波形均衡,以便抽样判决器正确判决。 抽样判决器首先对接收滤波器输出的信号y(t)在规定的时刻(由定时脉冲cp控制)进行抽样,获得抽样信号{r n},然后对抽样值进行判决,以确定各码元是“1”码还是“0”码。 2.基带系统设计中的码间干扰和噪声干扰以及解决方案
ABB机器人操作培训 S C IRB 说明书 完整版
S4C IRB 基本操作 培训教材 目录 1、培训教材介绍 2、机器人系统安全及环境保护 3、机器人综述 4、机器人启动 5、用窗口进行工作 6、手动操作机器人 7、机器人自动生产 8、编程与测试 9、输入与输出 10、系统备份与冷启动 11、机器人保养检查表 附录1、机器人安全控制链 附录2、定义工具中心点 附录3、文件管理 1、培训教材介绍 本教材解释ABB机器人的基本操作、运行。 你为了理解其内容不需要任何先前的机器人经验。 本教材被分为十一章,各章分别描述一个特别的工作任务和实现的方法。各章互相间有一定联系。因此应该按他们在书中的顺序阅读。 借助此教材学习操作操作机器人是我们的目的,但是仅仅阅读此教材也应该能帮助你理解机器人的基本的操作。 此教材依照标准的安装而写,具体根据系统的配置会有差异。
机器人的控制柜有两种型号。一种小,一种大。本教材选用小型号的控制柜表示。大的控制柜的柜橱有和大的一个同样的操作面板,但是位于另一个位置。 请注意这教材仅仅描述实现通常的工作作业的某一种方法,如果你是经验丰富的用户,可以有其他的方法。 其他的方法和更详细的信息看下列手册。 《使用指南》提供全部自动操纵功能的描述并详细描述程序设计语言。此手册是操作员和程序编制员的参照手册。 《产品手册》提供安装、机器人故障定位等方面的信息。 如果你仅希望能运行程序,手动操作机器人、由软盘调入程序等,不必要读8-11章。 2、机器人系统安全及环境保护 机器人系统复杂而且危险性大,在训练期间里,或者任何别的操作过程都必须注意安全。无论任何时间进入机器人周围的保护的空间都可能导致严重的伤害。只有经过培训认证的人员才可以进入该区域。请严格注意。 以下的安全守则必须遵守。 ?万一发生火灾,请使用二氧化碳灭火器。 ?急停开关(E-Stop)不允许被短接。 ?机器人处于自动模式时,不允许进入其运动所及的区域。 ?在任何情况下,不要使用原始盘,用复制盘。 ?搬运时,机器停止,机器人不应置物,应空机。 ?意外或不正常情况下,均可使用E-Stop键,停止运行。在编程,测试及维修时必须注意既使在低速时,机器人仍然是非常有力的,其动量很大,必须将机器人置于手动模式。 ?气路系统中的压力可达0.6MP,任何相关检修都要断开气源。 ?在不用移动机器人及运行程序时,须及时释放使能器(Enable Device)。?调试人员进入机器人工作区时,须随身携带示教器,以防他人无意误操作。?在得到停电通知时,要预先关断机器人的主电源及气源。 ?突然停电后,要赶在来电之前预先关闭机器人的主电源开关,并及时取下夹具上的工件。 ?维修人员必须保管好机器人钥匙,严禁非授权人员在手动模式下进入机器人软件系统,随意翻阅或修改程序及参数。 安全事项在《用户指南》安全一章中有详细说明。 如何处理现场作业产生的废弃物 现场服务产生的危险固体废弃物有:废工业电池、废电路板、废润滑油和废油脂、粘油回丝或抹布、废油桶。
系统建模与仿真
一、基本概念 1、数字正弦载波调制 在通信中不少信道不能直接传送基带信号,必须用基带信号对载波波形的某些参量进行控制,使得载波的这些参量随基带信号的变化而变化,即所谓数字正弦载波调制。 2、数字正弦载波调制的分类。 在二进制时, 数字正弦载波调制可以分为振幅键控(ASK)、移频键控(FSK)和移相键控(PSK)三种基本信号形式。如黑板所示。 2、高斯白噪声信道 二、实验原理 1、实验系统组成 2、实验系统结构框图
图 1 2FSK信号在高斯白噪声信道中传输模拟框图 各个模块介绍p12 3、仿真程序 x=0:15;% x表示信噪比 y=x;% y表示信号的误比特率,它的长度与x相同FrequencySeparation=24000;% BFSK调制的频率间隔等于24KHz BitRate=10000;% 信源产生信号的bit率等于10kbit/s SimulationTime=10;% 仿真时间设置为10秒SamplesPerSymbol=2;% BFSK调制信号每个符号的抽样数等于2 for i=1:length(x)% 循环执行仿真程序 SNR=x(i);% 信道的信噪比依次取中的元素 sim('project_1');% 运行仿真程序得到的误比特率保存在工作区变量BitErrorRate中 y(i)=mean(BitErrorRate); end hold off% 准备一个空白的图 semilogy(x,y);%绘制的关系曲线图,纵坐标采用对数坐标 三、实验结论
图 4 2FSK信号误比特率与信噪比的关系曲线图 系统建模与仿真(二) ——BFSK在多径瑞利衰落信道中的传输性能 一、基本概念 多径瑞利衰落信道 二、实验原理 1、实验系统组成
系统建模与仿真项目驱动设计报告
系统建模与仿真项目驱动设计报告 学院:电气工程与自动化学院 专业班级:自动化143班 学号:2420142928 学生姓名:李荣 指导老师:杨国亮 时间:2016年6月10号
仿真技术是一门利用物理模型或数学模型模拟实际环境进行科学实验的技术,具有经济、可靠、实用、安全、灵活和可多次重复使用的优点。 本文中将使用Matlab软件实现一个简单的控制系统仿真演示,可实现对一些连续系统的数字仿真、连续系统按环节离散化的数字仿真、采样控制系统的数字仿真以及系统的根轨迹、伯德图、尼克尔斯图和奈氏图绘制。 本设计完成基本功能的实现,基于Matlab的虚拟实验仿真的建立和应用,培养了我们的兴趣,提高了我们的实践能力。 关键字:Matlab;系统数字仿真;根轨迹;伯德图。
第一章概述 (4) 1.1 设计目的 (4) 1.2 设计要求 (4) 1.3设计内容 (4) 第二章 Matlab简介 (6) 2.1 Matlab的功能特点 (6) 2.2 Matlab的基本操作 (6) 第三章控制系统仿真设计 (8) 3.1 控制系统的界面设计 (8) 3.2 控制系统的输入模型设计 (9) 3.3 欧拉法的Matlab实现 (12) 3.4 梯形法的Matlab实现 (14) 3.5 龙格-库塔法的Matlab实现 (15) 3.6 双线性变换法的Matlab实现 (16) 3.7 零阶保持器法的Matlab实现 (17) 3.8 一阶保持器法的Matlab实现 (18) 3.9 系统PID控制的Matlab实现 (19) 3.10 系统根轨迹的绘制 (21) 3.11系统伯德图的绘制 (22) 3.12系统尼克尔斯图的绘制 (23)
电力系统建模及仿真课程设计
某某大学 《电力系统建模及仿真课程设计》总结报告 题目:基于MATLAB的电力系统短路故障仿真于分析 姓名 学号 院系 班级 指导教师
摘要:本次课程设计是结合《电力系统分析》的理论教学进行的一个实践课程。 电力系统短路故障,故障电流中必定有零序分量存在,零序分量可以用来判断故障的类型,故障的地点等,零序分量作为电力系统继电保护的一个重要分析量。运用Matlab电力系统仿真程序SimPowerSystems工具箱构建设计要求所给的电力系统模型,并在此基础上对电力系统多中故障进行仿真,仿真波形与理论分析结果相符,说明用Matlab对电力系统故障分析的有效性。实际中无法对故障进行实验,所以进行仿真实验可达到效果。 关键词:电力系统;仿真;短路故障;Matlab;SimPowerSystems Abstract: The course design is a combination of power system analysis of the theoretical teaching, practical courses. Power system short-circuit fault, the fault current must be zero sequence component exists, and zero-sequence component can be used to determine the fault type, fault location, the zero-sequence component as a critical analysis of power system protection. SimPowerSystems Toolbox building design requirements to the power system model using Matlab power system simulation program, and on this basis, the power system fault simulation, the simulation waveforms with the theoretical analysis results match, indicating that the power system fault analysis using Matlab effectiveness. Practice can not fault the experiment, the simulation can achieve the desired effect. Keywords: power system; simulation; failure; Matlab; SimPowerSystems - 1 - 目录 一、引言 ............................................ - 3 -
《生产物流系统建模和仿真》课程设计报告
《生产物流系统建模与仿真》课程设计 2012-2013学年度第一学期 姓名孙会芳 学号 099094090 班级工093 指导老师暴伟霍颖
目录 一、课程任务书 (3) 1.题 目............................................................... (3) 2.课程设计内容 (3) 3.课程设计要求 (4) 4.进度安排 (4) 5.参考文献 (4) 二、课程设计正文 (5) 1、题目 (5) 2、仿真模型建立 (5) (1)实体元素定义 (5) (2)元素可视化的设置 (6) (3)元素细节设计 (8) (4 ) 模型运行和数据.................................................................. . (10) (5)模型代码 (12) (6)模型改进 (16) 3.实验感想 (17)
三、参考文献 (18) 《生产物流系统建模与仿真》课程设计任务书 1. 题目 离散型流水作业线系统仿真 2. 课程设计内容 系统描述与系统参数: (1)一个流水加工生产线,不考虑其流程间的空间运输。 (2)两种工件A,B分别以正态分布和均匀分布的时间间隔进入系统,A进入队列Q1, B进入队列Q2,等待检验。(学号最后位数对应的仿真参数设置按照下表进行) (3)操作工人labor1对A进行检验,每件检验用时2分钟,操作工人labor2对B进行检验,每件检验用时2分钟。 (4)不合格的工件废弃,离开系统;合格的工件送往后续加工工序,A的合格率为65%,B的合格率为95%。 (5)工件A送往机器M1加工,如需等待,则在Q3队列中等待;B送往机器M2加工,如需等待,则在Q4队列中等待。 (6)A在机器M1上的加工时间为正态分布(5,1)分钟;B在机器M2上的加工时间为正态分布(8,1)分钟。
系统建模与仿真设计报告一
设计一产生十种不同分布的独立的随机数 一、设计内容及要求 任务:产生十种不同分布的独立的随机数,并进行检验。 要求:对随机数进行的统计性检验包括频率检验、参数检验、独立性检验。 二、设计环境及工具 Windows7、MatlabR2010b 三、设计思想及方法 (1) 在对雷达系统进行仿真时,首当其冲的问题就是对电磁环境 的仿、真。其中无用的电磁信号包括三大类,即杂波、噪声和干扰,在模拟仿真时相比于有用的电磁信号也是不可或缺的。其所谓的仿真就是在已知随机变量的统计特性及其参数的情况下,研究如何在计算机上产生服从给定统计特性和参数的随机变量。 (2) 在雷达、导航、声呐、通信和电子对抗等系统中,应用最多 的概率统计模型还是正态分布或高斯分布、指数分布、瑞利分布、莱斯分布或广义瑞利分布、韦尔分布、对数-正态分布、m分布、拉普拉斯分布、复合k分布等。 (3) 在这些随机总体中畸形随机抽样,实际上都是以[0,1]区间上 的均匀分布随机总体为基础的。原则上讲,只要已知[0,1]区间上的均匀分布随机数序列,总可以通过某种方法(数学方法)来获得某已知分布的简单子样。只要给定的均匀分布随机数列满足均匀
且相互独立打的要求,经过严格的数学变换或者严格的数学方法,所产生的任何分布的简单子样都会满足具有相同总体分布和相互独立的要求。 四、设计过程及结果 本次设计的十种随机数包括均匀分布、高斯分布、指数分布、广 义指数分布、瑞利分布、广义瑞利分布、韦尔分布、拉普拉斯分布、柯西分布和2χ分布,使用Matlab 完成设计并给出具体的参数,代码附在最后。 1.均匀分布 已知随机变量ε在[0,1]区间上服从均匀分布,则有概率密度函数 1,01 ()0,x f x ≤≤?=?? 其他 其分布函数为 0,0F(),01 x x x x x ? =≤≤??≥? 1,1 在计算机上利用数学方法产生随机数的方法有平方取中法、移位 寄存器法和各种同余法等,这里我们采用剩同余法产生均匀随机序列,其递推公式为 1(mod )n n x x M λ+= 通常取2k M =,k 为计算机的尾数字长 021b x =+,b 为正整数,x 初始值须为奇数 323a λ=±,a 为正整数
生产系统建模与仿真课程设计
1. 设计分析 1.1问题描述 系统由四台加工中心、五个托盘和装夹工具、一套搬运轨道和小车、一个 工件装夹区组成,其布局如图1所示。系统所包含的主要时间类别及大致时间 如下: (1) 工件安装时间。是指待加工工件装夹并固定在托盘上的时间,由于模具工 件均为长方体,因此,该时间比较稳定,大约 2mi ns 左右。 (2) 小车等待时间。工件安装完成后,如机床都在工作状态,则小车需等待有 机床完成 工作后,开始运出待加工工件。该等待时间不是固定的值,需要计算 得出。 (3) 机床等待时间。当有多个机床处于空置状态时,由于运输容量的限制,有 的机床就 处于空置等待状态,该状态所经历的时间,就是该机床的等待时间。 (4) 工件运出时间。将已安装好工件的托盘,从安装区运出至数控设备。大约 2mi ns 。 (5) 更换托盘时间。将设备上装载已加工好的零件的托盘与小车上装载待加工 工件的托 盘进行更换。大约需要1min 。 (6) 工件运回时间。更换托盘后,将载有已加工好的工件的托盘运回安装区, 并卸载。 大约需要3mins 。 图1系统布局图 1.2设计内容 1任务队列如表1所示,计算该队列条件下的任务总完成时间、四台设备各自 的设备等待 搬运 轨道 小车
时间,绘制四台设备的工序图。 2对任务队列进行排序优化,阐述优化的思路和方法,计算优化后的任务总完成时间、四台设备各自的设备等待时间,绘制四台设备的工序图。 表1设计案例参数表(单位:分钟) 1.3设计中的主要因素及系统分析 在本次的设计条件中,系统中共有20个任务,每个任务的加工时间是不相等的,而且只有一套运输设备,各个设备的功能完全一致。所以制约的加工的最大因素便是运输的制约。按照原始的顺序,进行加工,画出原始工序图。 再对原始任务工序图进行分析,并数据计算。计算出20个任务的总加工时间, 各个设备的等待时间,小车的等待时间。分析我们所得的数据结果,找出制约整个工序的主要问题所在,并进行改善。 在这个系统中共有20个加工时间各不相同的任务,按照顺序移动的方式来进行加工。在分析之前我们需先进行以下假设: (1)加工开始前,五个托盘分别位于四台加工中心及装夹区; (2)小车运出至每台加工中心的时间相等,运回至每台加工中心的时间也 相等。 (3)系统运行中不会出现故障等影响加工时间的意外 (4)小车一次只能进行一次托盘更换,最多只能运回一个工件,也最多只能运出
ABB机器人的手动操作
ABB[a]/-J-3 ABB 机器人的手动操作 3.1 任务目标 掌握手动操作机器人运动的三种模式。 使用“增量”模式来控制机器人的运动。 熟练使用手动操纵的快捷方式。 掌握ABB 机器人转数计数器更新操作。 3.2 任务描述 手动操纵机器人运动一共有三种模式:单轴运动、线性运动和重定位运动。如何使用这三种模式手动操作机器人运动是项目的主要内容。 建立一个工作站,ABB 型号为IRB120,Y 轴上建模长方体,长200mm,宽200mm,高400mm,在长方体的内角上进行重定位运动,之后恢复到机械远点。(手动操作练习需要教师指导,同时需要上机练习)3.3 知识储备 3.3.1 手动操作三种模式 1.单轴运动 一般地,ABB 机器人是由六个伺服电动机分别驱动机器人的六个关节轴,那么每次手动操纵一个关节轴的运动,就称之为单轴运动。 2.线性运动 图2 IRB 120 机器人的关节轴
机器人的线性运动是指安装在机器人第六轴法兰盘上工具的TCP 在空间中作线性运动。 3.重定位运动 机器人的重定位运动是指机器人第六轴法兰盘上的工具TCP 点在空间中绕着坐标轴旋转的运动,也可以理解为机器人绕着工具TCP 点作姿态调整的运动。 3.3.2RobotStudio 中的建模功能 当使用RobotStudio 进行机器人的仿真验证时,如节拍、到达能力等,如果对周边模型要求不是非常细致的表述时,可以用简单的等同实际大小的基本模型进行代替,从而节约仿真验证的时间。 如果需要精细的3D 模型,可以通过第三方的建模软件进行建模,并通过*.sat 格式导入到RobotStudio 中来完成建模布局的工作。 1.使用RobotStudio 建模功能进行3D 模型的创建
系统建模与仿真
系统建模仿真技术的历史现状和发展趋势分析 工程133 胡浩3130212026 【摘要】:经过半个多世纪的发展,仿真技术已经成为对人类社会发展进步具有重要影响的一门综合性技术学科。本文对建模与仿真技术发展趋势作了较全面分析。仿真建模方法更加丰富,更加需要仿真模型具有互操作性和可重用性,仿真建模VVA与可信度评估成为仿真建模发展的重要支柱;仿真体系结构逐渐形成标准,仿真系统层次化、网络化已成为现实,仿真网格将是下一个重要发展方向;仿真应用领域 更加丰富,向复杂系统科学领域发展,并将更加贴近人们的生活。 工程系统的仿真,起源于自动控制技术领域。从最初的简单电子、机械系统,逐步发展到今天涵盖机、电、液、热、气、电、磁等各个专业领域,并且在控制器和执行机构两个方向上飞速发展。 控制器的仿真软件,在研究控制策略、控制算法、控制系统的品质方面提供了强大的支持。随着执行机构技术的发展,机、电、液、热、气、磁等驱动技术的进步,以高可靠性、高精度、高反应速度和稳定性为代表的先进特征,将工程系统的执行品质提升到了前所未有的水平。相对控制器本身的发展,凭借新的加工制造技术的支持,执行机构技术的发展更加富于创新和挑战,而对于设计、制造和维护高性能执行机构,以及构建一个包括控制器和执行机构的完整的自动化系统也提出了更高的要求。 AMESIM软件正是能够提供平台级仿真技术的工具。从根据用户需求,提供液压、机械、气动等设计分析到复杂系统的全系统分析,
到引领协同仿真技术的发展方向,AMESIM的发展轨迹和方向代表了工程系统仿真技术的发展历程和趋势。 一、系统仿真技术发展的现状 工程系统仿真作为虚拟设计技术的一部分,与控制仿真、视景仿真、结构和流体计算仿真、多物理场以及虚拟布置和装配维修等技术一起,在贯穿产品的设计、制造和运行维护改进乃至退役的全寿命周期技术活动中,发挥着重要的作用,同时也在满足越来越高和越来越复杂的要求。因此,工程系统仿真技术也就迅速地发展到了协同仿真阶段。其主要特征表现为: 1、控制器和被控对象的联合仿真:MATLAB+AMESIM,可以覆盖整个自动控制系统的全部要求。 2、被控对象的多学科、跨专业的联合仿真:AMESIM+机构动力学+CFD+THERMAL+电磁分析 3、实时仿真技术 实时仿真技术是由仿真软件与仿真机等半实物仿真系统联合实现的,通过物理系统的实时模型来测试成型或者硬件控制器。 4、集成进设计平台 现代研发制造单位,尤其是设计研发和制造一体化的大型单位,引进PDM/PLM系统已经成为信息化建设的潮流。在复杂的数据管理流程中,系统仿真作为CAE工作的一部分,被要求嵌入流程,与上下游工具配合。
生产系统建模与仿真课程设计
1.1问题描述 系统由四台加工中心、五个托盘和装夹工具、一套搬运轨道和小车、一个工件装夹区组成,其布局如图1所示。系统所包含的主要时间类别及大致时间如下: (1) 工件安装时间。是指待加工工件装夹并固定在托盘上的时间,由于模具工件均为长方体,因此,该时间比较稳定,大约2mins 左右。 (2) 小车等待时间。工件安装完成后,如机床都在工作状态,则小车需等待有机床完成工作后,开始运出待加工工件。该等待时间不是固定的值,需要计算得出。 (3) 机床等待时间。当有多个机床处于空置状态时,由于运输容量的限制,有的机床就处于空置等待状态,该状态所经历的时间,就是该机床的等待时间。 (4) 工件运出时间。将已安装好工件的托盘,从安装区运出至数控设备。大约2mins 。 (5) 更换托盘时间。将设备上装载已加工好的零件的托盘与小车上装载待加工工件的托盘进行更换。大约需要1min 。 (6) 工件运回时间。更换托盘后,将载有已加工好的工件的托盘运回安装区,并卸载。大约需要3mins 。 图1 系统布局图 CNC CNC CNC CNC 搬运轨道 小车 工件装夹区
1 任务队列如表1所示,计算该队列条件下的任务总完成时间、四台设备各自的设备等待时间,绘制四台设备的工序图。 2 对任务队列进行排序优化,阐述优化的思路和方法,计算优化后的任务总完成时间、四台设备各自的设备等待时间,绘制四台设备的工序图。 表1 设计案例参数表(单位:分钟) 安装运出运回更换 2 2 3 1 任务1 任务2 任务3 任务4 7 15 21 5 任务5 任务6 任务7 任务8 12 33 17 38 任务9 任务10 任务11 任务12 8 19 22 25 任务13 任务14 任务15 任务16 16 27 31 6 任务17 任务18 任务19 任务20 245118 11 1.3设计中的主要因素及系统分析 在本次的设计条件中,系统中共有20个任务,每个任务的加工时间是不相等的,而且只有一套运输设备,各个设备的功能完全一致。所以制约的加工的最大因素便是运输的制约。按照原始的顺序,进行加工,画出原始工序图。再对原始任务工序图进行分析,并数据计算。计算出20个任务的总加工时间,各个设备的等待时间,小车的等待时间。分析我们所得的数据结果,找出制约整个工序的主要问题所在,并进行改善。 在这个系统中共有20个加工时间各不相同的任务,按照顺序移动的方式来进行加工。在分析之前我们需先进行以下假设: (1)加工开始前,五个托盘分别位于四台加工中心及装夹区; (2)小车运出至每台加工中心的时间相等,运回至每台加工中心的时间也相等。
ABB机器人中文手册
1 介绍 本手册解释ABB机器人的基本操作、运行。 你为了理解其内容不需要任何先前的机器人经验。 手册被分为章,各章分别描述一个特别的工作任务和实现的方法。 各章互相间有一定联系。因此应该按他们在书中的顺序阅读。 借助此手册学习操作操作机器人是我们的目的,但是仅仅阅读此手册也应该能帮助你理解机器人的基本的操作。 此手册依照标准的安装而写,具体根据系统的配置会有差异。 控制柜有两种型号。一种小,一种大。本手册选用小型号的控制柜表示。大的控制柜的柜橱有和大的一个同样的操作面板,但是位于另一个位置。 请注意这手册仅仅描述实现通常的工作作业的某一种方法,如果你是经验丰富的用户,可以有其他的方法。 其他的方法和更详细的信息看下列手册。 《使用指南》提供全部自动操纵功能的描述并详细描述程序设计语言。此手册是操作员和程序编制员的参照手册。 《产品手册》提供安装、机器人故障定位等方面的信息。 如果你仅希望能运行程序,手动操作机器人、由软盘调入程序等,不必要读8-11章。 2 系统安全及环境保护 机器人系统复杂而且危险性大,在训练期间里,或者任何别的操作过程都必须注意安全。无论任何时间进入机器人周围的保护的空间都可能导致严重的伤害。只有经过培训认证的人员才可以进入该区域。请严格注意。 以下的安全守则必须遵守。 ?万一发生火灾,请使用二氧化炭灭火器。 ?急停开关(E-Stop)不允许被短接。 ?机器人处于自动模式时,不允许进入其运动所及的区域。 ?在任何情况下,不要使用原始盘,用复制盘。 ?搬运时,机器停止,机器人不应置物,应空机。
?意外或不正常情况下,均可使用E-Stop键,停止运行。 在编程,测试及维修时必须注意既使在低速时,机器人仍然是非常有力的,其动量很大,必须将机器人置于手动模式。 ?气路系统中的压力可达0.6MP,任何相关检修都要断气源。 ?在不用移动机器人及运行程序时,须及时释放使能器(Enable Device)。 ?调试人员进入机器人工作区时,须随身携带示教器,以防他人无意误操作。 ?在得到停电通知时,要预先关断机器人的主电源及气源。 ?突然停电后,要赶在来电之前预先关闭机器人的主电源开关,并及时取下夹具上的工件。 ?维修人员必须保管好机器人钥匙,严禁非授权人员在手动模式下进入机器人软件系统,随意翻阅或修改程序及参数。 安全事项在《用户指南》安全一章中有详细说明。 如何处理现场作业产生的废弃物 现场服务产生的危险固体废弃物有:废工业电池、废电路板、废润滑油和废油脂、粘油回丝或抹布、废油桶。 普通固体废弃物有:损坏零件和包装材料。 ?现场服务产生的损坏零件由我公司现场服务人员或客户修复后再使用;废包装材料,我方现场服务人员建议客户交回收公司回收再利用。 ?现场服务产生的废工业电池和废电路板,由我公司现场服务人员带回后交还供应商;或由客户保管,在购买新电池时作为交换物。废润滑油及废油脂、废油桶、粘油废棉丝和抹布,由我方现场服务人员建议客户分类收集后交给专业公司处理。 3 综述 3.1 S4C系统介绍: 常规型号: IRB 1400,IRB 2400,IRB 4400,IRB 6400 IRB 指 ABB 机 器 人, 第一位数(1,2,4,6)指机器人大小 第二位数( 4 )指机器人属于S4或S4C系统。 无论何型号,机器人控制部分基本相同。
物流系统建模与仿真软件简介
一、物流系统建模与仿真软件简介 由于物流系统变得越来越复杂并且内部关联性越来越强。仿真是公司检验其物流系统及决策是否真的高效的唯一可用技术了。在设计一个新的工厂或系统,对已由系统添加新设备或重新优化,仿真都是非常必要的。同时仿真还用来提供直觉的和经验的决策支持。在当今市面上,仿真可用使用专用软件来实现。由于存在着如此多的仿真软件,如何正确的选择软件至关重要。下面列举出典型的系统仿真软件[3]。 软件名称简介 (1)20-sim 20-sim是由Twente大学控制实验室开发的运行于Windows系统下的建模与仿真软件。作为著名软件包TUTSIM的后续产品,它完全支持图形建模,让用户在直观和友善的方式对动态系统进行设计和分析,同MATLAB和Simulink可以方便的进行建模与仿真的交互。使用20-sim,我们可以仿真动态系统(例如电力、机械、水动力系统或它们的组合系统)的各种行为。 (2)arena该软件可以用来模拟服务、制造、运输、物流、供应链和其它系统。(3)Automod该软件提供了真实的三维虚拟现实动画,使得仿真模型非常用以理 解;提供了高级的特征让用户可以仿真复杂的活动,如机器人、设 备工具、生产线等的运动和转动。该软件还为用户提供了一套基于 专家系统的物料搬运系统,它是根据工业自动化的真实运行经验开 发的。这些包括输送链、自动存储和检索系统,桥式起重机等。(4)Awesim Awesim提供生产系统动态模型的仿真机。动画使用图形界面构建, 用户可以对交互式仿真进行特定的控制。 (5)Easy5由波音公司开发的用来模拟和仿真包含水力、风力、机械、热、电 子和数字等子系统的动态系统软件包。包括了一整套控制系统建 模、分析和设计功能。 (6)Idef该软件是一种流程图析软件,可以非常容易的适用流程图来绘制和 表述流程。它能够提供比传统流程图更多的信息。流程中包含的流 程、流程约束、人和其他资源能够被整合到一起。 (7)Intrax该软件能够提供许多被建模和仿真实际流程的管理决策。它能够被 用来执行战略(同战略视图,同步价值链视图相符合的现实),流 程改善(工序改善、生产力改善、节约循环时间),同步价值链(动 态视觉,同步约束)和日常运作(可对比的运作替代方案,短期变 化影响力的检验)等的模拟和仿真。 (8)Manufacturing Engineering 该软件提供离散仿真功能来解决制造问题和设计制造方案。它在广阔的应用领域中预测产出率,人工和其他的绩效。 (9)Matlab该软件是组合的数字计算、高级图形技术和可视化、高级编程语言 的集成计算机算环境。Simulink式用来对动态系统进行建模、仿真 和分析的交互式工具。它可以构建图形化的结构图,模拟动态系统, 评估系统绩效和精炼设计。 (10)Modsim该软件可以用来仿真像港口,铁路网和航空管制等的管理模型。还 可以用来仿真制造系统。 (11)Promodel该软件可以对制造系统、仓储系统和物流系统的评估、规划或重新 设计进行仿真。典型应用包括精益制造的实施,周期事件的降低, 设备投资决策,产出率和能力分析,识别和排除瓶颈,资源分配等。
制造系统建模与仿真在工业工程中的应用 0713020
制造系统建模与仿真在工业工程中的应用0713020 工业工程刘鹏 [摘要]介绍了企业发展和建模的必要性和必然性,分析了制造系统的建模与仿真在优化企业资源中的作用及意义,详细地论述了制造系统的建模与仿真在优化企业资源中的具体应用。 关键词:制造系统;建模与仿真;企业优化;仿真应用 系统建模与仿真技术的含义 系统建模与仿真技术是以相似原理、模型理论、系统技术、信息技术以及建模与仿真应用领域的有关专业技术为基础,以计算机系统、与应用相关的物理效应设备及仿真器为工具,利用模型参与已有或设想的系统进行研究、分析、设计、加工生产、试验、运行、评估、维护、和报废(全生命周期)活动的一门多学科的综合性技术。 仿真科学和技术的通用性和战略性 仿真的通用性表现在一切基础学科(如物理、化学、天文?)都可以通过仿真来研究;并可以极大地提高研究的安全性。仿真的战略性表现在一切复杂巨系统的研究都离不开仿真技术,可以说研究复杂巨系统采用仿真技术是唯一的途径。正如宋健院士所说:“系统仿真是科学实验的利器。 国内仿真技术发展 在我国仿真技术经过半个多世纪的发展,已经从军工走向国民经济。已经从工程走向非工程;已经从确定的小系统走向不确定的复杂巨系统。最初的仿真技术只是用计算机来求解方程,为了实时性,大
都采用电子模拟计算机。现在的仿真技术已经融合了信息技术、网络技术、系统技术、控制技术和高性能的计算技术,以完全崭新的面貌出现在我们的面前。 现在,摆在我们仿真工作者面前的任务是:在虚拟世界与真实世界之间架起一座桥梁;通过仿真技术构筑起一个平台,来勾画出创新型国家的轮廓,例如,国家正投入几个亿,来建设国家级研究经济模型的仿真实验室。 仿真技术,一方面反映了我国仿真技术和仿真技术应用发展的现状,另一方面,又对我国仿真技术今后的发展方向产生了指导作用。近年来,我国仿真技术及其应用的发展是十分迅猛的。仿真技术的发展,使人感到震惊。研究天文、地理、宇宙进化论等等,要依靠仿真,几乎没有哪个领域能离开仿真技术。凡是能写成方程的都要进行仿真。故应鼓励仿真界的科技人员发挥聪明才智,搞好仿真技术。 仿真技术的广度、深度、高度的提高,正反映了我国仿真技术和应用的发展。例如,“面向复杂性地理问题的虚拟研讨厅体系研究”,“复杂系统建模中的几个问题”等都是有代表性的好文章,反映了我国仿真技术已经在军事和国民经济的一些复杂巨系统研究建设中发挥越来越重要的作用。 1、制造系统的建模与仿真在优化企业资源中的作用及意义 计算机仿真技术作为一门高新技术,其方法学建立在计算机能力的基础之上。随着计算机技术的发展,仿真技术也得到迅速的发展,其应用领域及其作用也越来越大。尤其在航空、航天、国防及其他大
物流系统建模与仿真课程设计概要
课程设计物流系统建模与仿真 专业年级2011级物流工程指导教师张莹莹 小组成员 重庆大学自动化学院 物流工程系 2014年9 月12 日
课程设计指导教师评定成绩表 项目分 值 优秀 (100>x≥90) 良好 (90>x≥80) 中等 (80>x≥ 70) 及格 (70>x≥60) 不及格 (x<60) 评 分参考标准参考标准参考标准参考标准参考标准 学习态度15 学习态度认 真,科学作风 严谨,严格保 证设计时间并 按任务书中规 定的进度开展 各项工作 学习态度比较 认真,科学作 风良好,能按 期圆满完成任 务书规定的任 务 学习态度 尚好,遵守 组织纪律, 基本保证 设计时间, 按期完成 各项工作 学习态度尚 可,能遵守组 织纪律,能按 期完成任务 学习马虎, 纪律涣散, 工作作风 不严谨,不 能保证设 计时间和 进度 技术水平 与实际能力25 设计合理、理 论分析与计算 正确,实验数 据准确,有很 强的实际动手 能力、经济分 析能力和计算 机应用能力, 文献查阅能力 强、引用合理、 调查调研非常 合理、可信 设计合理、理 论分析与计算 正确,实验数 据比较准确, 有较强的实际 动手能力、经 济分析能力和 计算机应用能 力,文献引用、 调查调研比较 合理、可信 设计合理, 理论分析 与计算基 本正确,实 验数据比 较准确,有 一定的实 际动手能 力,主要文 献引用、调 查调研比 较可信 设计基本合 理,理论分析 与计算无大 错,实验数据 无大错 设计不合 理,理论分 析与计算 有原则错 误,实验数 据不可靠, 实际动手 能力差,文 献引用、调 查调研有 较大的问 题 创新10 有重大改进或 独特见解,有 一定实用价值 有较大改进或 新颖的见解, 实用性尚可 有一定改 进或新的 见解 有一定见解观念陈旧 论文(计算 书、图纸)撰写质量50 结构严谨,逻 辑性强,层次 清晰,语言准 确,文字流畅, 完全符合规范 化要求,书写 工整或用计算 机打印成文; 图纸非常工 整、清晰 结构合理,符 合逻辑,文章 层次分明,语 言准确,文字 流畅,符合规 范化要求,书 写工整或用计 算机打印成 文;图纸工整、 清晰 结构合理, 层次较为 分明,文理 通顺,基本 达到规范 化要求,书 写比较工 整;图纸比 较工整、清 晰 结构基本合 理,逻辑基本 清楚,文字尚 通顺,勉强达 到规范化要 求;图纸比较 工整 内容空泛, 结构混乱, 文字表达 不清,错别 字较多,达 不到规范 化要求;图 纸不工整 或不清晰 指导教师评定成绩:
系统建模与仿真习题答案(forstudents)分解
第一章习题 1-1什么是仿真?它所遵循的基本原则是什么? 答:仿真是建立在控制理论,相似理论,信息处理技术和计算技术等理论基础之上的,以计算机和其他专用物理效应设备为工具,利用系统模型对真实或假想的系统进行试验,并借助专家经验知识,统计数据和信息资料对试验结果进行分析和研究,进而做出决策的一门综合性的试验性科学。 它所遵循的基本原则是相似原理。 1-2在系统分析与设计中仿真法与解析法有何区别?各有什么特点? 答:解析法就是运用已掌握的理论知识对控制系统进行理论上的分析,计算。它是一种纯物理意义上的实验分析方法,在对系统的认识过程中具有普遍意义。由于受到理论的不完善性以及对事物认识的不全面性等因素的影响,其应用往往有很大局限性。 仿真法基于相似原理,是在模型上所进行的系统性能分析与研究的实验方法。 1-3数字仿真包括那几个要素?其关系如何? 答: 通常情况下,数字仿真实验包括三个基本要素,即实际系统,数学模型与计算机。由图可见,将实际系统抽象为数学模型,称之为一次模型化,它还涉及到系统辨识技术问题,统称为建模问题;将数学模型转化为可在计算机上运行的仿真模型,称之为二次模型化,这涉及到仿真技术问题,统称为仿真实验。 1-4为什么说模拟仿真较数字仿真精度低?其优点如何?。 答:由于受到电路元件精度的制约和容易受到外界的干扰,模拟仿真较数字仿真精度低 但模拟仿真具有如下优点: (1)描述连续的物理系统的动态过程比较自然和逼真。 (2)仿真速度极快,失真小,结果可信度高。 (3)能快速求解微分方程。模拟计算机运行时各运算器是并行工作的,模拟机的解题速度与原系统的复杂程度无关。 (4)可以灵活设置仿真试验的时间标尺,既可以进行实时仿真,也可以进
系统建模与仿真课程设计
生产系统建模与仿真课程设计任务书 问题描述: 系统由四台加工中心、两个托盘及装夹工具A 和B 、一套搬运轨道和小车、一个工件装夹区组成,其布局如图1所示。系统所包含的主要时间类别及大致时间如下: (1) 工件安装时间。是指待加工工件装夹并固定在托盘上的时间,由于模具工件均为长方体,因此,该时间稳定在3mins 左右。 (2) 小车等待时间。工件安装完成后,如机床都在工作状态,则小车需等待有机床完成工作后,开始运出待加工工件。该等待时间不是固定的值,需要计算得出。 (3) 机床等待时间。当有多个机床处于空置状态时,由于运输容量的限制,有的机床就处于空置等待状态,该状态所经历的时间,就是该机床的等待时间。 (4) 工件运出时间。将已安装好工件的托盘,从安装区运出至数控设备。大约2mins 。 (5) 更换托盘时间。将设备上装载已加工好的零件的托盘与小车上装载待加工工件的托盘进行更换。大约需要1min 。 (6) 工件运回时间。更换托盘后,将载有已加工好的工件的托盘运回安装区,并卸载。大约需要3mins 。 图1 系统布局图 设计内容:
1 任务队列如表1所示,计算该队列条件下的任务总完成时间、四台设备各自的设备等待时间,绘制四台设备的工序图。 2 对任务队列进行排序优化,阐述优化的思路和方法,计算优化后的任务总完成时间、四台设备各自的设备等待时间,绘制四台设备的工序图。 A: 2*10+QQ号第2位。 B: 3*12+QQ号第1位。
目录 一、课程设计背景 (1) 二、问题分析 (2) 2.1初步分析 (2) 2.2原始条件分析 (2) 2.3小车运动分析 (2) 2.4加工中心加工分析 (3) 2.5运动分析原则 (3) 2.6时间分析 (3) 三、原始加工顺序分析 (3) 3.1原始加工顺序工序图 (4) 3.2原始数据分析 (4) 四、目标优化 (5) 4.1原始加工零件时间分析 (5) 4.2优化分析思路 (5) 4.3优化过程 (5) 4.4选择原则 (7) 4.5加工顺序表 (7) 4.6优化后的零件工序图 (7) 4.7优化后零件的时间指标 (7) 五、优化方案的结果分析 (8) 5.1优化结果比较 (8)