Physiological regulation of lipoprotein lipase
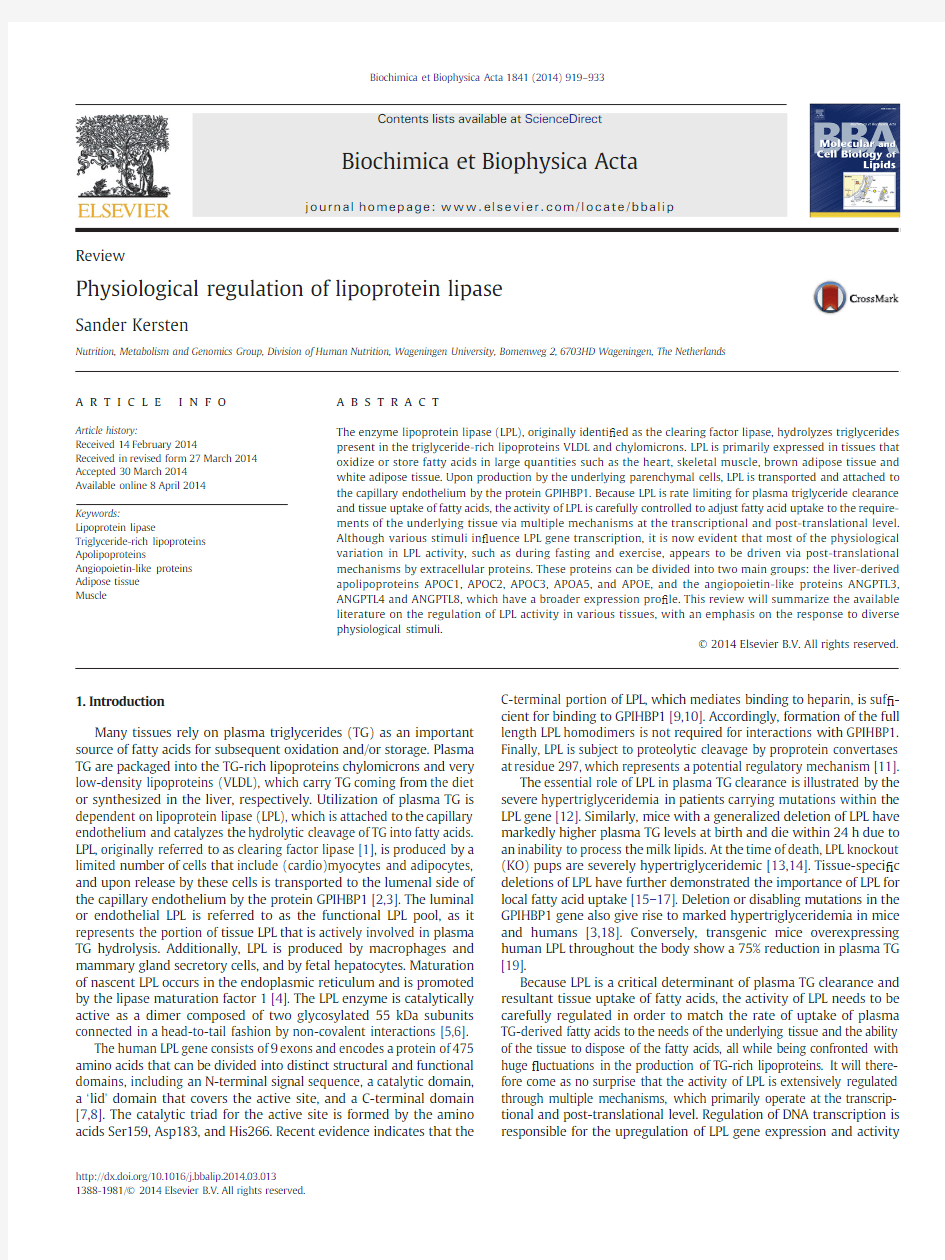
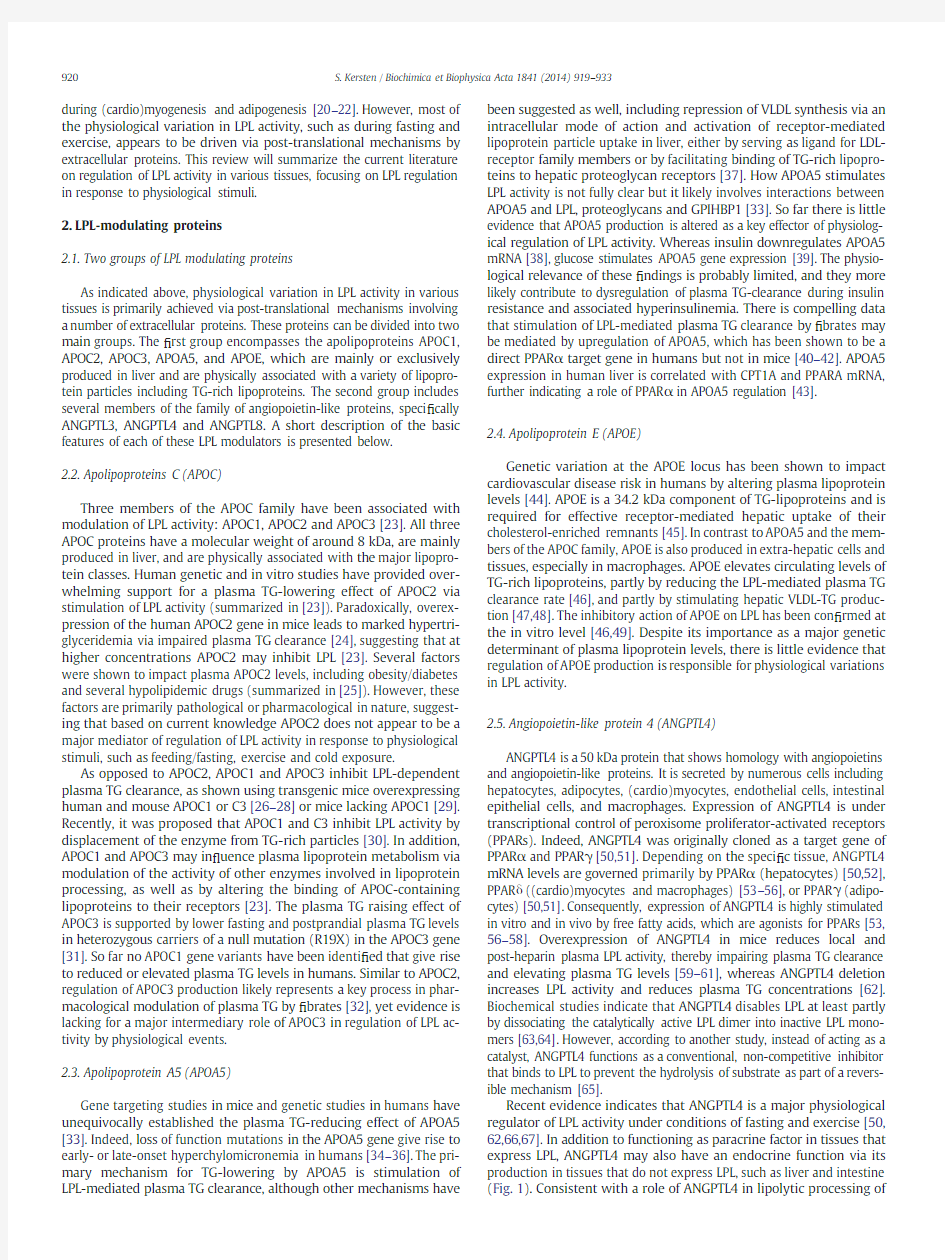
Review
Physiological regulation of lipoprotein lipase
Sander Kersten
Nutrition,Metabolism and Genomics Group,Division of Human Nutrition,Wageningen University,Bomenweg 2,6703HD Wageningen,The Netherlands
a b s t r a c t
a r t i c l e i n f o Article history:
Received 14February 2014
Received in revised form 27March 2014Accepted 30March 2014Available online 8April 2014Keywords:
Lipoprotein lipase
Triglyceride-rich lipoproteins Apolipoproteins
Angiopoietin-like proteins Adipose tissue Muscle
The enzyme lipoprotein lipase (LPL),originally identi ?ed as the clearing factor lipase,hydrolyzes triglycerides present in the triglyceride-rich lipoproteins VLDL and chylomicrons.LPL is primarily expressed in tissues that oxidize or store fatty acids in large quantities such as the heart,skeletal muscle,brown adipose tissue and white adipose tissue.Upon production by the underlying parenchymal cells,LPL is transported and attached to the capillary endothelium by the protein GPIHBP1.Because LPL is rate limiting for plasma triglyceride clearance and tissue uptake of fatty acids,the activity of LPL is carefully controlled to adjust fatty acid uptake to the require-ments of the underlying tissue via multiple mechanisms at the transcriptional and post-translational level.Although various stimuli in ?uence LPL gene transcription,it is now evident that most of the physiological variation in LPL activity,such as during fasting and exercise,appears to be driven via post-translational mechanisms by extracellular proteins.These proteins can be divided into two main groups:the liver-derived apolipoproteins APOC1,APOC2,APOC3,APOA5,and APOE,and the angiopoietin-like proteins ANGPTL3,ANGPTL4and ANGPTL8,which have a broader expression pro ?le.This review will summarize the available literature on the regulation of LPL activity in various tissues,with an emphasis on the response to diverse physiological stimuli.
?2014Elsevier B.V.All rights reserved.
1.Introduction
Many tissues rely on plasma triglycerides (TG)as an important source of fatty acids for subsequent oxidation and/or storage.Plasma TG are packaged into the TG-rich lipoproteins chylomicrons and very low-density lipoproteins (VLDL),which carry TG coming from the diet or synthesized in the liver,respectively.Utilization of plasma TG is dependent on lipoprotein lipase (LPL),which is attached to the capillary endothelium and catalyzes the hydrolytic cleavage of TG into fatty acids.LPL,originally referred to as clearing factor lipase [1],is produced by a limited number of cells that include (cardio)myocytes and adipocytes,and upon release by these cells is transported to the lumenal side of the capillary endothelium by the protein GPIHBP1[2,3].The luminal or endothelial LPL is referred to as the functional LPL pool,as it represents the portion of tissue LPL that is actively involved in plasma TG hydrolysis.Additionally,LPL is produced by macrophages and mammary gland secretory cells,and by fetal hepatocytes.Maturation of nascent LPL occurs in the endoplasmic reticulum and is promoted by the lipase maturation factor 1[4].The LPL enzyme is catalytically active as a dimer composed of two glycosylated 55kDa subunits connected in a head-to-tail fashion by non-covalent interactions [5,6].
The human LPL gene consists of 9exons and encodes a protein of 475amino acids that can be divided into distinct structural and functional domains,including an N-terminal signal sequence,a catalytic domain,a ‘lid ’domain that covers the active site,and a C-terminal domain [7,8].The catalytic triad for the active site is formed by the amino acids Ser159,Asp183,and His266.Recent evidence indicates that the C-terminal portion of LPL,which mediates binding to heparin,is suf ?-cient for binding to GPIHBP1[9,10].Accordingly,formation of the full length LPL homodimers is not required for interactions with GPIHBP1.Finally,LPL is subject to proteolytic cleavage by proprotein convertases at residue 297,which represents a potential regulatory mechanism [11].
The essential role of LPL in plasma TG clearance is illustrated by the severe hypertriglyceridemia in patients carrying mutations within the LPL gene [12].Similarly,mice with a generalized deletion of LPL have markedly higher plasma TG levels at birth and die within 24h due to an inability to process the milk lipids.At the time of death,LPL knockout (KO)pups are severely hypertriglyceridemic [13,14].Tissue-speci ?c deletions of LPL have further demonstrated the importance of LPL for local fatty acid uptake [15–17].Deletion or disabling mutations in the GPIHBP1gene also give rise to marked hypertriglyceridemia in mice and humans [3,18].Conversely,transgenic mice overexpressing human LPL throughout the body show a 75%reduction in plasma TG [19].
Because LPL is a critical determinant of plasma TG clearance and resultant tissue uptake of fatty acids,the activity of LPL needs to be carefully regulated in order to match the rate of uptake of plasma TG-derived fatty acids to the needs of the underlying tissue and the ability of the tissue to dispose of the fatty acids,all while being confronted with huge ?uctuations in the production of TG-rich lipoproteins.It will there-fore come as no surprise that the activity of LPL is extensively regulated through multiple mechanisms,which primarily operate at the transcrip-tional and post-translational level.Regulation of DNA transcription is responsible for the upregulation of LPL gene expression and activity
Biochimica et Biophysica Acta 1841(2014)919–
933
https://www.360docs.net/doc/415523865.html,/10.1016/j.bbalip.2014.03.013
1388-1981/?2014Elsevier B.V.All rights
reserved.
Contents lists available at ScienceDirect
Biochimica et Biophysica Acta
j o u r n a l h o m e p a g e :w w w.e l s e v i e r.c o m/l o c a t e /b b a l i p
during(cardio)myogenesis and adipogenesis[20–22].However,most of the physiological variation in LPL activity,such as during fasting and exercise,appears to be driven via post-translational mechanisms by extracellular proteins.This review will summarize the current literature on regulation of LPL activity in various tissues,focusing on LPL regulation in response to physiological stimuli.
2.LPL-modulating proteins
2.1.Two groups of LPL modulating proteins
As indicated above,physiological variation in LPL activity in various tissues is primarily achieved via post-translational mechanisms involving a number of extracellular proteins.These proteins can be divided into two main groups.The?rst group encompasses the apolipoproteins APOC1, APOC2,APOC3,APOA5,and APOE,which are mainly or exclusively produced in liver and are physically associated with a variety of lipopro-tein particles including TG-rich lipoproteins.The second group includes several members of the family of angiopoietin-like proteins,speci?cally ANGPTL3,ANGPTL4and ANGPTL8.A short description of the basic features of each of these LPL modulators is presented below.
2.2.Apolipoproteins C(APOC)
Three members of the APOC family have been associated with modulation of LPL activity:APOC1,APOC2and APOC3[23].All three APOC proteins have a molecular weight of around8kDa,are mainly produced in liver,and are physically associated with the major lipopro-tein classes.Human genetic and in vitro studies have provided over-whelming support for a plasma TG-lowering effect of APOC2via stimulation of LPL activity(summarized in[23]).Paradoxically,overex-pression of the human APOC2gene in mice leads to marked hypertri-glyceridemia via impaired plasma TG clearance[24],suggesting that at higher concentrations APOC2may inhibit LPL[23].Several factors were shown to impact plasma APOC2levels,including obesity/diabetes and several hypolipidemic drugs(summarized in[25]).However,these factors are primarily pathological or pharmacological in nature,suggest-ing that based on current knowledge APOC2does not appear to be a major mediator of regulation of LPL activity in response to physiological stimuli,such as feeding/fasting,exercise and cold exposure.
As opposed to APOC2,APOC1and APOC3inhibit LPL-dependent plasma TG clearance,as shown using transgenic mice overexpressing human and mouse APOC1or C3[26–28]or mice lacking APOC1[29]. Recently,it was proposed that APOC1and C3inhibit LPL activity by displacement of the enzyme from TG-rich particles[30].In addition, APOC1and APOC3may in?uence plasma lipoprotein metabolism via modulation of the activity of other enzymes involved in lipoprotein processing,as well as by altering the binding of APOC-containing lipoproteins to their receptors[23].The plasma TG raising effect of APOC3is supported by lower fasting and postprandial plasma TG levels in heterozygous carriers of a null mutation(R19X)in the APOC3gene [31].So far no APOC1gene variants have been identi?ed that give rise to reduced or elevated plasma TG levels in humans.Similar to APOC2, regulation of APOC3production likely represents a key process in phar-macological modulation of plasma TG by?brates[32],yet evidence is lacking for a major intermediary role of APOC3in regulation of LPL ac-tivity by physiological events.
2.3.Apolipoprotein A5(APOA5)
Gene targeting studies in mice and genetic studies in humans have unequivocally established the plasma TG-reducing effect of APOA5 [33].Indeed,loss of function mutations in the APOA5gene give rise to early-or late-onset hyperchylomicronemia in humans[34–36].The pri-mary mechanism for TG-lowering by APOA5is stimulation of LPL-mediated plasma TG clearance,although other mechanisms have been suggested as well,including repression of VLDL synthesis via an intracellular mode of action and activation of receptor-mediated lipoprotein particle uptake in liver,either by serving as ligand for LDL-receptor family members or by facilitating binding of TG-rich lipopro-teins to hepatic proteoglycan receptors[37].How APOA5stimulates LPL activity is not fully clear but it likely involves interactions between APOA5and LPL,proteoglycans and GPIHBP1[33].So far there is little evidence that APOA5production is altered as a key effector of physiolog-ical regulation of LPL activity.Whereas insulin downregulates APOA5 mRNA[38],glucose stimulates APOA5gene expression[39].The physio-logical relevance of these?ndings is probably limited,and they more likely contribute to dysregulation of plasma TG-clearance during insulin resistance and associated hyperinsulinemia.There is compelling data that stimulation of LPL-mediated plasma TG clearance by?brates may be mediated by upregulation of APOA5,which has been shown to be a direct PPARαtarget gene in humans but not in mice[40–42].APOA5 expression in human liver is correlated with CPT1A and PPARA mRNA, further indicating a role of PPARαin APOA5regulation[43].
2.4.Apolipoprotein E(APOE)
Genetic variation at the APOE locus has been shown to impact cardiovascular disease risk in humans by altering plasma lipoprotein levels[44].APOE is a34.2kDa component of TG-lipoproteins and is required for effective receptor-mediated hepatic uptake of their cholesterol-enriched remnants[45].In contrast to APOA5and the mem-bers of the APOC family,APOE is also produced in extra-hepatic cells and tissues,especially in macrophages.APOE elevates circulating levels of TG-rich lipoproteins,partly by reducing the LPL-mediated plasma TG clearance rate[46],and partly by stimulating hepatic VLDL-TG produc-tion[47,48].The inhibitory action of APOE on LPL has been con?rmed at the in vitro level[46,49].Despite its importance as a major genetic determinant of plasma lipoprotein levels,there is little evidence that regulation of APOE production is responsible for physiological variations in LPL activity.
2.5.Angiopoietin-like protein4(ANGPTL4)
ANGPTL4is a50kDa protein that shows homology with angiopoietins and angiopoietin-like proteins.It is secreted by numerous cells including hepatocytes,adipocytes,(cardio)myocytes,endothelial cells,intestinal epithelial cells,and macrophages.Expression of ANGPTL4is under transcriptional control of peroxisome proliferator-activated receptors (PPARs).Indeed,ANGPTL4was originally cloned as a target gene of PPARαand PPARγ[50,51].Depending on the speci?c tissue,ANGPTL4 mRNA levels are governed primarily by PPARα(hepatocytes)[50,52], PPARδ((cardio)myocytes and macrophages)[53–56],or PPARγ(adipo-cytes)[50,51].Consequently,expression of ANGPTL4is highly stimulated in vitro and in vivo by free fatty acids,which are agonists for PPARs[53, 56–58].Overexpression of ANGPTL4in mice reduces local and post-heparin plasma LPL activity,thereby impairing plasma TG clearance and elevating plasma TG levels[59–61],whereas ANGPTL4deletion increases LPL activity and reduces plasma TG concentrations[62]. Biochemical studies indicate that ANGPTL4disables LPL at least partly by dissociating the catalytically active LPL dimer into inactive LPL mono-mers[63,64].However,according to another study,instead of acting as a catalyst,ANGPTL4functions as a conventional,non-competitive inhibitor that binds to LPL to prevent the hydrolysis of substrate as part of a revers-ible mechanism[65].
Recent evidence indicates that ANGPTL4is a major physiological regulator of LPL activity under conditions of fasting and exercise[50, 62,66,67].In addition to functioning as paracrine factor in tissues that express LPL,ANGPTL4may also have an endocrine function via its production in tissues that do not express LPL,such as liver and intestine (Fig.1).Consistent with a role of ANGPTL4in lipolytic processing of
920S.Kersten/Biochimica et Biophysica Acta1841(2014)919–933
TG-rich lipoproteins in humans,a loss of function gene variant of ANGPTL4is associated with reduced plasma TG levels [68].
Apart from its role as LPL inhibitor,which is mediated by the N-terminal portion and proteolytic fragment,ANGPTL4is able to bind speci ?c integrins via its C-terminal portion [69].This mechanism of action of ANGPTL4likely accounts for the modulating effect of ANGPTL4on angiogenesis,endothelial migration &permeability,tumorigenesis,and renal glomerular function [70].
2.6.Angiopoietin-like protein 3(ANGPTL3)
ANGPTL3was discovered as plasma lipid modulating factor via positional cloning in KK/San mice,a mutant strain of KK obese mice with markedly lower plasma free fatty acid (FFA)and TG levels [71].In humans,mutations within the ANGPTL3gene give rise to fa-milial hypolipidemia,characterized by low plasma levels of total cholesterol,LDL-C,HDL-C,and triglycerides,or combinations
thereof
Fig.1.Role of ANGPTLs in regulating plasma TG clearance.A)ANGPTL3and ANGPTL8serve as endocrine regulators of plasma TG clearance via their near exclusive production in liver (at least in human)and their LPL inhibitory action in peripheral tissues.B)Despite being produced in liver as well,ANGPTL4functions primarily as a paracrine regulator of plasma TG clearance in peripheral tissues via local inhibition of
LPL.
Fig.2.Summary of the main physiological stimuli regulating LPL activity in various tissues.The principal tissues characterized by a high level of LPL activity are shown.
921
S.Kersten /Biochimica et Biophysica Acta 1841(2014)919–933
[72].Furthermore,genome-wide association studies revealed that a com-mon sequence variant at a locus near the ANGPTL3gene is associated with plasma TG[73,74].Studies using various animal models of ANGPTL3 overexpression or deletion have corroborated the stimulatory effect of ANGPTL3on plasma TG,which is achieved by suppressing plasma TG clearance via inhibition of LPL activity[62,75,76].The biochemical mechanism for LPL inhibition by ANGPTL3is not fully clear but seems to be stoichiometrically distinct from LPL inhibition by ANGPTL4 [77,78].Similar to the C-class of apolipoproteins,ANGPTL3is produced exclusively in liver,where its expression is downregulated by leptin and insulin[79,80],and upregulated by the nuclear receptor LXR[81].Because ANGPTL3is produced by the liver only,which does not express LPL,it functions as an endocrine rather than paracrine factor.How regulation of ANGPTL3synthesis in liver may contribute to tissue-speci?c regulation of LPL activity is unclear(Fig.2).Recent data suggest that ANGPTL3may cooperate with the related protein ANGPTL8[82],which has a somewhat broader expression pro?le.
2.7.Angiopoietin-like protein8(ANGPTL8)
ANGPTL8(also referred to as RIFL,lipasin and betatrophin)is the most recently cloned member of the angiopoietin-like protein family. It has substantial homology with ANGPTL3and ANGPTL4but lacks the C-terminal?brinogen-like domain that is characteristic for this family [83,84].In mice ANGPTL8is expressed mainly in liver and white and brown adipose tissue,with lower expression in kidney and intestine, while in humans expression appears to be speci?c for liver.Deletion of ANGPTL8lowers plasma TG[85],whereas overexpression of ANGPTL8raises plasma TG,likely by inhibiting LPL activity[82–84]. ANGPTL8may inhibit LPL activity directly,or indirectly by promoting cleavage and activation of ANGPTL3[82,84].A variant of the human ANGPTL8gene was associated with lower plasma LDL-C and HDL-C levels but not with plasma TG.Expression of ANGPTL8in white adipose tissue and liver is markedly induced by refeeding,which is likely mediated by insulin[82–84],whereas expression of ANGPTL8in brown adipose tissue is induced by cold[86].Intriguingly,refeeding/in-sulin induces LPL activity in white adipose tissue,and cold exposure induces LPL activity in brown adipose tissue,raising questions about the physiological relevance and rationale of the observed ANGPTL8 regulation.
3.Regulation of LPL in adipose tissue
3.1.Lipid sources for adipocytes
Adipocytes can acquire fatty acids for storage from three principal sources.The?rst source is de novo lipogenesis in adipocytes from glucose and acetate.The importance of this pathway is highly dependent on the animal species,and appears to be relatively insigni?cant in humans[87,88].The second source is circulating FFA.Since circulating FFA mostly originate from adipose tissue,uptake of plasma FFA by adipose tissue essentially represents recycling of stored fatty acids and therefore does not contribute to net fat storage.The third and by far the most important source are circulating TG-rich lipoproteins in the form of VLDL and chylomicrons,which explains the high expression of LPL in adipose tissue.It should be noted that part of the fatty acids carried by VLDL may have arrived in the liver as FFA and their uptake by adipocytes can also be considered recycling of stored fatty acids.
3.2.Mechanism of nutritional regulation of plasma TG clearance in adipose tissue
The adipose tissue continuously switches from being a net storage organ in the fed or post-prandial state to a net release organ in the fasted or post-absorptive state.Although LPL-mediated extracellular lipolysis and intracellular lipolysis mediated by the lipase trilogy ATGL–HSL–MGL are never completely turned“off”,the?ux through these pathways and the activities of the participating enzymes vary widely with nutri-tional status[89].Clearance of TG-rich lipoproteins in adipose tissue is markedly elevated in the fed state compared to the fasted state[90], which is likely at least partly mediated by the presence and relative absence of insulin,respectively.Regulation of plasma TG-derived fatty acid uptake in adipose tissue occurs via three interrelated pathways that are described below.
3.2.1.Fatty acid spillover
The?rst pathway in?uencing plasma TG-derived fatty acid uptake is fatty acid spillover,which describes the loss of plasma TG-derived fatty acids into the venous blood without being directly taken up by the underlying tissue.Although LPL is usually considered as a fat storage enzyme,it should be realized that a major proportion of the fatty acids released by LPL-catalyzed lipolysis are“lost”as spillover[91].Estimates of the magnitude of fatty acid spillover vary but it is well accepted that spillover is higher in the fasted state[92],reaching estimates of100%, compared to the post-prandial state.There is evidence that fatty acid spillover decreases with each successive meal and more profoundly affects chylomicrons compared with VLDL[93].Many molecular details of the process of fatty acid spillover remain murky,mostly due to our lack of insight about the transport of fatty acids from the endothelial surface to the underlying adipocytes.For example,it could be envisioned that all fatty acids generated by plasma TG hydrolysis are taken up by adipocytes but that a portion leaves the cell again and ends up back in the circulation.If that is true,spillover may be controlled at the level of intracellular fatty acid esteri?cation.Alternatively,it is possible that a portion of the released fatty acids never leaves the blood-stream or the endothelial cells in the?rst place,which implies possible regulation at the level of trans-endothelial transport of fatty acids. Although numerous lines of evidence corroborate the existence of fatty acid spillover,its purported magnitude is seemingly dif?cult to reconcile with the detailed and tissue-speci?c regulation of LPL activi-ty[90].Teusink and colleagues have proposed a model in which fatty acids released from LPL exchange locally with plasma FFA[90].In this model,the purpose of LPL is to increase the local concentration of fatty acids close to the endothelium,which provides the driving force for the uptake of fatty acids into the underlying tissues.According to this scenario,a fatty acid spillover of close to100%does not contradict with an important role of LPL-catalyzed TG clearance in local fatty acid uptake.
3.2.2.Regulation of LPL synthesis
Early studies have indicated that fasting is associated with a pronounced decrease in LPL activity in adipose tissue(Fig.2)[94,95], which may be mediated at the level of LPL production or via other mechanisms.LPL mRNA and protein production vary with nutritional status,with LPL expression being lowest after fasting[96,97].Fluctuations in LPL mRNA during the feeding–fasting cycle may be related to changes in circulating glucocorticoids and insulin.Currently,the effects of gluco-corticoids on adipose LPL mass and activity are rather ambiguous.Kern and colleagues found that the synthetic glucocorticoid dexamethasone decreased adipocyte LPL mRNA in vitro and in rats,resulting in corre-sponding changes in the LPL synthetic rate and LPL activity[98].In line with these data,dexamethasone raised serum TG levels in mice[99].By contrast,dexamethasone and cortisol increased LPL mRNA and activity in human adipose organ cultures,as did insulin[100,101].Similarly,treat-ment of arthritic patients with prednisolone resulted in enhanced adipose LPL mass and activity[102].Whether these differences in LPL response re?ect species-speci?c differences is unclear.
Insulin has been shown to play a central role in the postprandial response of adipose LPL activity[103].Even though increases in LPL gene expression by insulin have been observed in adipocytes in vitro [104,105],changes in LPL mRNA probably only account for a small portion of the stimulation of LPL activity by insulin(see below).
922S.Kersten/Biochimica et Biophysica Acta1841(2014)919–933
Another feeding-related mechanism that may result in changes in LPL mRNA is mediated by glucose-dependent insulinotropic polypeptide (GIP).It was shown that GIP increases LPL activity and mRNA in isolated human adipocytes via a pathway involving PI3-K activation,PKB,AMPK, TORC2,and CREB,and that is activated by resistin[106,107].Whether GIP also plays a role in in vivo regulation of adipose LPL activity is unclear.
Transcription of the LPL gene is stimulated during adipogenesis by the adipogenic transcription factor PPARγ[108],and is stimulated by fatty acids and other PPARγagonists in differentiated adipocytes [109–111].Fasting reduces expression of PPARγin adipose tissue [112],providing a potential explanation for the reduction in LPL expression during fasting.Regulation of LPL expression by PPARγin humans is supported by induction of LPL mRNA by rosiglitazone in human adipose tissue[113].Another transcription factor that activates LPL gene transcription and that is reduced in adipose tissue upon fasting is SREBP-1[114,115].Whether SREBP-1plays a role in in vivo regulation of LPL expression remains unclear.
Taken together,it is clear that LPL expression in adipose tissue is governed via multiple transcription factors,yet it is unclear which of these pathways dominate regulation of LPL mRNA during feeding and fasting.
3.2.3.Post-translational regulation of LPL
Fluctuations in adipose LPL mRNA and protein mass with feeding and fasting are relatively small compared to the amplitude of changes in LPL activity[66,116,117].In fact,in some species LPL mRNA and activity move in opposite directions during fasting,with LPL activity decreasing and LPL mRNA or mass increasing[118,119],pointing to important regulation at the post-transcriptional and post-translational level.In humans,adipose LPL activity is markedly higher in the fasted state compared with the fed state[120].Based on the pioneering work in the1960s on the in?uence of transcriptional inhibitors on regulation of lipase activity in adipose tissue[121–123],Olivecrona was able to show that adipose LPL activity is primarily regulated during fasting at the post-translational level via a shift of extracellular LPL toward an inactive form[124],which is dependent on switching on a gene other than LPL[125,126].The identity of this fasting-induced gene has been unequivocally determined to be ANGPTL4[63,64],originally referred to as the fasting induced adipose factor FIAF[50].The importance of ANGPTL4in mediating nutritional regulation of LPL activity in adipose tissue is demonstrated by the observation that ANGPTL4KO mice have elevated basal LPL activity and do not show any decrease in adipose LPL activity during fasting[66].As a result,ANGPTL4KO mice have markedly reduced plasma TG,to the point of being almost undetectable in fasted ANGPTL4KO mice.In the same vein,injection of monoclonal antibodies against ANGPTL4reduced plasma TG,elevat-ed adipose LPL activity,and increased clearance of circulating TG into adipose tissue[127],whereas overexpression of ANGPTL4reduces uptake of plasma TG-derived fatty acids into adipose tissue and raises plasma TG levels[63].
The importance of ANGPTL4in governing LPL activity during feeding and fasting can easily be reconciled with the key role of insulin in regulating adipose LPL activity in vitro and in vivo[103,108,128]. Indeed,it was found that insulin potently suppresses ANGPTL4mRNA in cultured adipocytes[129],and in rat adipose tissue[66],likely via the transcription factor FoxO1[130],while raising adipose LPL activity. In humans,insulin reduces circulating ANGPTL4levels,as revealed during hyperinsulinemic clamps[131].Other proposed effects of insulin on LPL include stimulation of release of active enzyme from the cell and increased uptake of inactive extracellular LPL[132].The suppressive effect of insulin on LPL activity was found to be mediated by phos-phatidylinositol3-kinase and mTOR-dependent signaling pathways [133].
Induction of adipose ANGPTL4expression during fasting may be related to the loss of the inhibitory effect of insulin,and/or may be mediated by increased plasma FFA[58].Plasma FFA not only are highly potent activators of ANGPTL4gene expression in adipocytes[134],but also cause marked inhibition of LPL activity[109,135],suggesting a link between elevated FFA and LPL activity via ANGPTL4.Other potential explanations for the increase in adipose ANGPTL4mRNA during fasting are elevated circulating levels of glucocorticoids.It has been observed that dexamethasone directly stimulates ANGPTL4transcription in adipose tissue and also raises serum TG levels in mice[99].Whether induction of ANGPTL4by glucocorticoids leads to modulation of adipose LPL activity remains to be demonstrated.
Overall,these studies indicate that ANGPTL4very likely represents the long sought-after factor that governs regulation of LPL activity during fasting and feeding in fat tissue and therefore can be considered
a key determinant of fat storage.
3.3.Regulation of adipose LPL activity by hypoxia
Since the1960s it is known that high altitude simulation raises circu-lating TG levels[136]and that hypoxia may impact LPL activity[137].In support of these data,plasma TG went up twofold in subjects during a 40day simulated ascent of Mt.Everest[138].While little information is available about the mechanisms underlying hypertriglyceridemia during chronic continuous hypoxia,it is plausible that the mechanisms are similar to those operating under conditions of chronic intermittent hypoxia.It was shown that chronic intermittent hypoxia inhibits plasma lipoprotein clearance and adipose LPL activity in association with upregulation of ANGPTL4[139],which is a direct target of hypoxia inducible factor HIF1a in adipose tissue[140].However,mice treated with monoclonal antibodies against ANGPTL4still exhibited decreased uptake of TG-derived fatty acids into adipose tissue during chronic intermittent hypoxia,despite restoration of LPL activity,suggesting that inhibition of LPL is not the principal mechanism accounting for impaired TG tissue uptake in hypoxic mice[127].
3.4.Regulation of adipose LPL activity by stress and catecholamines
Both short-term and long-term stress elicited by immobilization lead to a pronounced decrease in LPL activity in adipose tissue,whereas immobilization causes an increase in plasma LPL activity[141].The effect of immobilization stress,which is supposedly mediated by catecholamines,is very fast,leading to reciprocal changes in plasma and adipose LPL activity within minutes.The speed of the events suggest that stress leads to release of LPL into the bloodstream from its attach-ment to GPIHBP1and/or heparan sulfate proteoglycans at the endothelial surface[142],but the mechanism underlying this effect remains unclear.
Catecholamines are important mediators of the stress response and are known to directly impact LPL activity in adipose tissue.In vitro studies indicate that catecholamines reduce adipocyte LPL activity via downregulation of LPL gene transcription[105],reducing LPL translation by stimulating the interaction of a PKA-containing RNA binding complex to the LPL3′UTR[143,144],inducing LPL protein degradation[132],and induction of ANGPTL4mRNA(our unpublished observation).In brief, stress and catecholamines reduce adipose LPL activity via a number of complementary mechanisms.
3.5.Regulation of adipose LPL activity by LPS and TNFα
Sepsis is a common surgical problem associated with profound chang-es in production of cytokines and hormones leading to marked metabolic perturbations including hypertriglyceridemia[145].Development of hypertriglyceridemia may serve as an adaptive response to increase the capacity for binding and neutralization of endotoxins[146],and thereby protect against sepsis-associated complications[147].Studies in rats have shown that endotoxin exposure causes hypertriglyceridemia by reducing plasma TG clearance,pointing to a potential role of LPL[148]. Compelling evidence indicates that endotoxins decrease LPL activity in adipose tissue[148–151],and in skeletal muscle and heart[149,151,
923
S.Kersten/Biochimica et Biophysica Acta1841(2014)919–933
152].The effect of endotoxin is conveyed via TNFα[153],which has been consistently found to reduce LPL activity in vivo and in vitro[154–158]. TNFαrepresses LPL activity by downregulating LPL transcription and thereby LPL mRNA,and furthermore a more recent study indicates that TNFαupregulates a gene whose product inactivates extracellular LPL [159].So far the identity of the TNFα-induced LPL inhibitor remains elusive,but it probably does not represent ANGPTL4,as ANGPTL4expres-sion was not altered by TNFαin3T3-L1adipocytes[160].
4.Regulation of LPL in skeletal muscle
4.1.Lipid sources for myocytes
Myocytes acquire fatty acids from plasma FFA and circulating TG-rich lipoproteins[90].The absolute and relative importance of each of these two sources is in?uenced by a variety of factors,with nutritional status and physical exercise being the dominant ones.In addition,fuel use is determined by?ber type[161].Type1oxidative slow-twitch?bers are heavily reliant on lipid fuels,whereas Type IIB glycolytic fast twitch?bers prefer glucose and glycogen.When fatty acid uptake exceeds the rate of fatty acid oxidation,fatty acids can be stored as TG in the muscle within lipid droplets,which is referred to as intra-myocellular lipid.Intra-myocellular lipid is believed to serve as a readily available fuel during endurance exercise[162].
4.2.Nutritional regulation of skeletal muscle LPL activity
Substantial fatty acid spillover also occurs in skeletal muscle, although it has been suggested that the extent of fatty acid spillover is less in muscle than in adipose tissue[163].The same considerations that have been expressed for spillover in adipose tissue also apply to spillover in muscle and will therefore not be further elaborated on here.
In contrast to adipose tissue,where LPL is mostly extracellular,in skeletal muscle a much higher proportion of LPL is intracellular.Accord-ingly,changes in total LPL activity in skeletal muscle more closely re?ect total LPL mass rather than functional LPL directly involved in endothelial hydrolysis of TG-rich lipoproteins,which explains why in general a relatively close correspondence is found between changes in muscle LPL mRNA and total LPL activity.A limited number of studies have employed heparin perfusion or prepared heparin eluates from fresh muscle tissue to determine the activity of the functional LPL pool[164, 165].Unfortunately,the technique is not straightforward and its use has not been widespread.
In contrast to LPL in adipose tissue,most studies indicate that fasting and starvation raise total LPL activity in skeletal muscle[97,117,166], the extent of which is somewhat dependent on the type of muscle studied[167].Results are not all consistent as Eckel and colleagues reported a signi?cantly higher muscle heparin-releasable LPL activity in human subjects in the fed state compared with the fasting state [120].At the mRNA level,LPL was found to be increased by fasting in skeletal muscle in mice[168]and humans[169](our unpublished observations).Little is known about the molecular mechanisms involved in governing muscle LPL during fasting,including regulation of the endothelial LPL pool.In contrast to its effect in adipose tissue, infusion of insulin decreased skeletal muscle LPL activity in humans [128].It has been suggested that the increase in skeletal muscle LPL activity and mRNA during fasting is mediated by an increase in LPL transcription[170],and is driven by the transcription factor Foxo1 [171].Similar to the situation in adipose tissue,expression of the LPL inhibitor ANGPTL4is markedly increased during fasting in mouse and human skeletal muscle[63,67,160],likely via elevated plasma FFA[56, 58],which may offset any increase in LPL mRNA and/or mass and may thereby lead to decreased functional LPL activity.In vitro in cultured myocytes,fatty acids and other agonists of PPARδand RXR cause reciprocal induction of ANGPTL4mRNA and reduction in heparin-releasable LPL activity,respectively[67,172,173],suggesting that fatty acids may regulate LPL activity in muscle during fasting via PPARδ/ RXR and ANGPTL4.
In summary,whereas LPL mRNA and total activity in skeletal muscle appear to be increased upon fasting,there is no evidence that functional LPL activity is elevated as well.The marked induction in expression of ANGPTL4during fasting may be predicted to lead to a decrease in functional LPL activity.In terms of nutritional physiology,it can be argued that LPL activity in muscle should increase during fasting to allow greater reliance on lipid fuels at the expense of carbohydrate. Alternatively,it stands to reason that the increased?ux of plasma FFA into muscle during fasting should be compensated by decreased uptake of plasma TG-derived fatty acids in order to limit fasting-induced lipid overload,which would argue for a decrease in LPL activity.
4.3.Regulation of skeletal LPL activity by acute exercise
One of the unique features of skeletal muscle is that it exhibits extreme metabolic plasticity,as shown by its ability to adjust fuel use depending on the metabolic circumstances,a property referred to as metabolic?exibility.In addition,skeletal muscle is able to massively increase the rate of ATP synthesis during physical exercise.Initiation of exercise commands immediate adjustments in fuel provision to the muscle to permit suf?cient ATP generation,including changes in the supply and uptake of lipid fuels.Numerous studies in humans and rodents have demonstrated that a single bout of physical exercise leads to a marked increase in LPL activity,protein,and mRNA in the exercising muscle[97,164,174–179].The physiological rationale behind the rapid exercise-induced stimulation of LPL activity is to increase the supply of fatty acids to the cell for subsequent oxidation to CO2and H2O and generate ATP.In addition,it may promote the post-exercise restoration of intramyocellular TG that have been used during exercise.
Currently,there is a scarcity of information on the physiological and molecular mechanisms involved in the induction of muscle LPL expres-sion by acute exercise.One possibility is via elevated catecholamines, which were found to stimulate skeletal muscle LPL activity in humans [180].In agreement with this notion,chronicβ-adrenergic activation stimulated muscle LPL activity in rats[181].A role of contraction-induced signaling molecules such as AMPK can be hypothesized but concrete evidence is lacking.Recently,we found that ANGPTL4is selec-tively induced during exercise in non-exercising muscle compared with exercising muscle,which is explained by the opposing effect of plasma FFA(stimulatory,via PPARδ)and AMPK(inhibitory)on ANGPTL4gene expression[67].It was suggested that selective induction of ANGPTL4 in non-exercising muscle reduces local fatty acid uptake presumably to prevent fat overload,while directing fatty acids to the active skeletal muscle as fuel.
4.4.Regulation of skeletal LPL activity by exercise training
It is well established that regular exercise lowers circulation TG levels[182],suggesting that exercise training,representing a series of incremental training bouts,may increase muscle LPL activity.Consistent with this notion,numerous studies in rodents have shown that exercise training signi?cantly increases muscle heparin releasable LPL activity, LPL mass,and LPL mRNA(Fig.2)[164,165,175,183].Cross-sectional and intervention studies have con?rmed that endurance exercise train-ing stimulates LPL activity in humans[184–186].Conversely,detraining leads to a decrease in muscle LPL activity,with variable changes in LPL immunoreactive mass and mRNA levels,depending on the duration of inactivity[187,188].
The increase in muscle LPL abundance following exercise training can be considered an adaptive change that promotes fuel supply to the muscle and thereby may promote oxidative capacity.Conversely, the decrease in LPL activity in inactive muscle may serve as an adaptive mechanism to minimize unnecessary lipid accumulation and potential lipotoxicity[187].How exercise training and/or chronic inactivity lead
924S.Kersten/Biochimica et Biophysica Acta1841(2014)919–933
to alterations in LPL activity and mRNA is unclear.Hamilton and colleagues found that the decrease in rat muscle heparin-releasable LPL activity upon physical inactivity was independent of a change in LPL mRNA concentration,and concluded that inactivity results in upregulation of a gene encoding an LPL inhibitor,the identity of which remains obscure[187].
5.Regulation of LPL in heart
5.1.Lipid sources for heart
Cardiac contractility depends on the adequate delivery of oxygen and energy substrates to the heart followed by oxidation of energy substrates to yield ATP.The energy requirements of the contracting heart are mainly met by fatty acid oxidation,with the remainder of energy coming from oxidation of glucose and lactate[189,190]. Although fatty acids are thus essential for cardiac contractility,excessive uptake of fatty acids causes lipid overload or lipotoxicity and may disturb cardiac function,possibly leading to cardiomyopathy[191]. Most of the fatty acids taken up and oxidized by the heart are derived from LPL-dependent hydrolysis of circulating TG-rich lipoproteins [90].In contrast to skeletal muscle,circulating FFA are of relatively minor importance as fuel for the heart.Accordingly,LPL activity in the heart needs to be carefully regulated to match plasma TG hydrolysis and consequent lipid uptake into cardiomyocytes with cardiac contrac-tility and the cellular requirement for ATP.Loss of LPL speci?cally in the heart is associated with elevated plasma levels and impaired clearance of TG-rich lipoproteins and a compensatory increase in glucose uptake and glucose oxidation,which cannot prevent age-related cardiac dysfunction as revealed by decreased fractional shortening and interstitial and perivascular?brosis[192].
5.2.Nutritional regulation of cardiac LPL activity
As in skeletal muscle,a very high proportion of LPL in heart is intracellular and cannot be released by heparin,which should be taken into account when interpreting total LPL activity data.Early investigations have demonstrated that fasting causes an increase in heparin releasable LPL activity in the heart(Fig.2)[193,194],which has been corroborated in several more recent studies[195–197],with some exceptions[116].In contrast,insulin decreases cardiac heparin-releasable LPL activity[198].Similar to what is observed in adipose tissue,fasting provokes a more pronounced change in cardiac heparin-releasable LPL activity compared with total cardiac LPL activity[193, 199,200].Heparin-agarose chromatography of heparin-released LPL indicated that the proportion of active dimeric LPL is higher in the fasted state than in the fed state.Transcription inhibition by actinomycin D increased heparin-releasable LPL activity in hearts of fed rats and increased the relative abundance of active LPL[201].Accordingly,it has been hypothesized that feeding is associated with the production of an LPL inhibitor that converts active LPL into inactive LPL[201].
An attractive candidate is ANGPTL4,which shows increased expres-sion in the heart after an oral fat load[53].Overexpression of ANGPTL4 in the heart reduces cardiac heparin-releasable LPL activity.In addition, cardiac ANGPTL4overexpression raises circulating TG levels,lowers cardiac TG levels and causes left-ventricular dysfunction[60].Expres-sion of ANGPTL4in the heart is induced by dietary fatty acids via the transcription factor PPARδand is likely part of a feedback mechanism aimed at limiting fatty acid uptake and protecting against cardiac overload and lipotoxicity[53].Intriguingly,expression of ANGPTL4 was also found to be increased in the fasted state relative to the ad libitum fed state[63].Accordingly,it is somewhat doubtful whether ANGPTL4is responsible for the relative inactivation of cardiac LPL in the fed state.
Intracellular factors that impact LPL activity in cardiomyocytes include PPARαand AMPK.Activation of PPARαin cultured cardiomyocytes using synthetic or endogenous agonists gives rise to decreased total cellular LPL activity,which is mediated by post-transcriptional and post-translational mechanisms,presumably involving ANGPTL4as cardiac PPARαtarget [202,53].Conversely,activation of AMPK leads to increased heparin-releasable LPL activity in heart,whereas AMPK inhibition causes a marked decrease in LPL activity[199,203].Finally,cold exposure,which increases energetic demands on the heart,was found to increase total and heparin-releasable LPL activity in the rat heart[204,205].The molecular mecha-nisms involved in modulation of cardiac LPL activity by AMPK and cold remain to be elucidated.
6.Regulation of LPL in brown adipose tissue
6.1.Lipid sources for brown adipocytes
The physiological function of brown adipose tissue is to generate heat and maintain body temperature as part of cold-adaptive thermogenesis [206].In many animal species,cold provokes chronic expansion of BAT mass as well as changes in BAT morphology and speci?c enzymatic activity,together leading to a marked increase in total thermogenic capacity.Recent studies in human subjects indicate cold-induced hyper-metabolic activity in multifocal regions along the neck,supraclavicular regions,mediastinum,and paraspinal regions,suggesting adult human possess BAT or a BAT-like tissue[207–210].BAT is a highly oxidative tissue and therefore requires the provision of large amounts of glucose and lipid fuels[206].A major source of lipid for brown adipocytes are fatty acids generated via intracellular lipolysis of locally stored TG.The second lipid source are plasma FFA originating from lipolysis in white adipose tissue.The third lipid source and most relevant to the present article are circulating TG-rich lipoproteins in the form of VLDL and chylomicrons,which explains the high expression of LPL in brown adipo-cytes.The importance of LPL in uptake of TG-derived fatty acids in BAT was recently demonstrated using adipocyte-speci?c LPL KO animals [211,212].
6.2.Regulation of LPL activity in bat by cold
Concomitant with the gradual expansion of the brown adipose tissue mass in rats and mice,cold exposure leads to a marked increase in total LPL activity in BAT(Fig.2)(expressed per depot)[213,214],as well as an increase in post-heparin plasma LPL activity[204].As a consequence,cold increases extraction of plasma TG-derived fatty acids into BAT and reduces plasma TG levels[214,215].In addition, cold exposure increases speci?c LPL activity in BAT[216–218].Induction of speci?c LPL activity by cold is most prominent during the early hours and then declines to reach a level that still signi?cantly exceeds baseline LPL activity[219].The increase in LPL activity is mediated by a transcrip-tion and translation-dependent mechanism,is paralleled by a similar increase in LPL mRNA,and can be mimicked byβ-adrenergic agonists, suggesting thatβ-adrenergic stimulation is the key mechanism for LPL induction during cold[181,215,217,219–221].Subsequent experiment in cultured brown adipocytes from rat showed thatβ3-adrenergic agonists but notβ1-orβ2-adrenergic agonists directly stimulate LPL mRNA[222].Surprisingly,β-adrenergic stimulation tended to decrease LPL mRNA in cultured brown adipocytes from mouse[221].
In contrast to mice and especially rats,hamsters show a decrease in size of the BAT depot following acute and chronic cold exposure, concurrent with a marked increase in LPL activity[223].The increase in LPL activity upon cold exposure clearly exceeds the increase in LPL protein and/or mRNA,suggesting a disproportion increase in the active fraction of LPL[220].Cold-induced activation of LPL activity in BAT in hamsters is not dependent on T3[224],and similar to rats and mice can be reproduced byβ-adrenergic activation[225].
In summary,cold markedly stimulates LPL activity in BAT,causing a reduction in plasma TG and potentially leading to alterations in other lipoproteins species,feeding speculations that BAT may be a fruitful
925
S.Kersten/Biochimica et Biophysica Acta1841(2014)919–933
target for the treatment of hypertriglyceridemia and other disorders in plasma lipoproteins[215].
6.3.Regulation of LPL activity in bat by other factors
Other factors that have been shown to in?uence LPL in BAT include PPARγagonists.PPARγactivation increases speci?c LPL activity in BAT of rats,concomitant with a huge increase in BAT mass[226],leading to a marked increase in uptake of TG-derived fatty acids in BAT[227, 228].Studies in fetal rat primary brown adipocytes indicate that PPARγactivation increases LPL mRNA and activity[229],suggesting that PPARγstimulates LPL activity in vivo via direct transcriptional regulation of LPL[111].
The impact of fasting on LPL activity in BAT remains unclear. Whereas short term fasting increased speci?c LPL activity in BAT in rats[216],3days of fasting decreased total LPL activity,which returned to control values by day3of refeeding[230].Similar to in white adipose tissue,insulin induced LPL activity in BAT[231].
7.Regulation of LPL in macrophages
7.1.Role of lipoprotein lipase in macrophages
It has long been recognized that macrophages have the ability to hydrolyze TG within chylomicrons via lipase activity and oxidize or re-esterify the released fatty acids[232–235].The TG-depleted and cholesterol-enriched remnant is subsequently taken up by macro-phages via an APOE-mediated process[236].Most of the published studies on LPL in macrophages have addressed the potential impact of LPL on foam cell formation and atherogenesis,showing expression of LPL in macrophages and foam cells in atherosclerotic plagues[237, 238],and a stimulatory role of LPL in foam cell formation from TG-rich lipoproteins or oxidized LDL[239,240].Consistent with these data, various types of gene targeting studies indicate stimulation of in vivo atherosclerosis by macrophage LPL.Indeed,macrophage-speci?c over-expression of LPL promotes the formation of atherosclerotic lesions and accumulation of macrophage-derived foam cells[241–244],which occurs in the absence of any changes in circulating lipoproteins, whereas the opposite is observed in macrophage speci?c LPL KO mice [245,246].Given the link between LPL and atherogenesis,data on regulation of LPL in macrophages have been mainly viewed in relation to its potential impact on atherosclerosis.
Remarkably,the basic question why macrophages abundantly express LPL and what may be the role of LPL in fuel provision to the macrophage or other macrophage functions has remained unanswered. It has been proposed that fatty acids released upon macrophage LPL-catalyzed hydrolysis of TG are an important fuel for macrophages when glucose availability is limited and during periods of intense metabolic activity,such as phagocytosis[247].In agreement with this notion,proliferating macrophages secrete large amounts of LPL activity,whereas in nonproliferating,quiescent cells,LPL activity is much lower[248].A role of LPL in fuel provision in macrophages auto-matically raises the question why none of the other leukocytes including dendritic cells express LPL.A complicating factor is that the TG-rich lipo-proteins that serve as substrate for LPL-mediated hydrolysis cannot easily cross the endothelium and thus hardly get into direct contact with tissue macrophages.An exception may be the mesenteric lymph nodes,where extremely high concentrations of chylomicrons directly pass macro-phages in the subscapular sinus of the mesenteric lymph node[55]. One could postulate that macrophages in mesenteric lymph nodes survey newly synthesized chylomicrons for immunogenic lipids via LPL-mediated lipolysis and present them to adjacent natural killer T cells, thereby conferring a major immunological role onto LPL.Overall,the limited understanding of the role of macrophage LPL in immunity hampers interpretation of the functional implications of macrophage LPL regulation by a variety of stimuli.7.2.Regulation of macrophage LPL activity by in?ammatory parameters
A major portion of the LPL secreted by macrophages remains associated with the cell membrane and can be released by heparin. This heparin-releasable secreted LPL activity has been the primary output parameter in most studies on LPL regulation.The level of secreted LPL activity is highly dependent on the activation state of macrophages.Fully activated macrophages obtained from Corynebacte-rium parvum-injected mice secrete very low levels of LPL activity when compared with unstimulated macrophages,while thioglycollate-elicited in?ammatory macrophages secrete much higher levels of LPL activity[249,250].
Lipopolysaccharide(LPS)inhibits secreted LPL activity,which is like-ly mediated at the level of LPL gene transcription(Fig.2)[250–253].The inhibitory effect of LPS may be partially conveyed by numerous cytokines released in response to LPS,including TNFα,interferonγand IL-1β[254].Inhibition of secreted LPL activity by TNFαhas been observed in mouse peritoneal macrophages but not in J774.1,BMDM and human monocyte-derived macrophages[252,253,255].
Interferonγdecreases macrophage LPL at least in part via a reduc-tion in LPL synthesis and gene transcription,possibly via CK2and PI3K signaling and regulation of Sp1/Sp3binding[256,257].Another cytokine that has been shown to inhibit LPL activity is transforming growth factorβ[258],whereas macrophage colony-stimulating factor, C-reactive protein,platelet-derived growth factor and elevated concen-trations of hydrogen peroxide(H2O2)were found to stimulate secreted LPL activity and/or LPL mRNA[259–262].
7.3.Regulation of macrophage LPL activity by nuclear hormone receptors
Several studies have found a marked suppression of secreted LPL activity by the glucocorticoid receptor agonist dexamethasone in mouse macrophages[263–265].Interestingly,dexamethasone stimulated LPL transcription in human THP-1and monocyte-derived macrophages [266],suggesting a possible species difference in regulation of LPL by glucocorticoids.
Agonists for PPARαand PPARγincrease LPL mRNA expression in human macrophages[267,268].Interestingly,Staels and colleagues re-ported that PPAR activators decrease secreted LPL mass and enzyme ac-tivity[267],whereas Renier observed an increase in the same parameters[268].Activation of PPARδdid not affect LPL mRNA in mac-rophages but markedly reduced secreted LPL activity,which is likely caused by concomitant induction of ANGPTL4[269,270].Similarly,upreg-ulation of ANGPTL4likely explains the reduction in secreted LPL activity upon incubation of macrophages with oleic acid and TG-rich lipoproteins[271].
Another nuclear receptor that has been suggested to be involved in LPL regulation is LXR,which is activated by oxysterols.Prolonged treat-ment with the synthetic LXR agonist T0301719stimulated LPL expression in peritoneal macrophages by about2-fold[272].In contrast,the natural LXR agonist25-hydroxycholesterol reduced LPL mRNA and secreted LPL activity in human monocyte-derived macrophages[273].The same response was observed for7β-hydroxycholesterol,which is not a LXR agonist,arguing against an involvement of LXR.Incubation of human monocytes-macrophages with highly oxidized LDL,which is enriched with oxysterols,also decreased LPL mRNA and secretion[274].Based on this limited information,the role of LXR in regulation of macrophage LPL by oxLDL and oxysterols remains unclear.
Other factors that have been shown to in?uence LPL in macrophages include cAMP activators,which potently repress LPL activity[263,275, 276],and glucose,which causes marked upregulation of LPL mRNA expression and immunoreactive mass[277].Insulin has no effect on LPL secretion,even though peritoneal macrophages isolated from insulin-de?cient mice secreted70%less LPL activity than control mice [265,278].
926S.Kersten/Biochimica et Biophysica Acta1841(2014)919–933
8.Regulation of LPL in other tissues
8.1.LPL activity in the lung
In addition to its well established expression and function in adipose tissue,muscle,and macrophages,LPL enzyme activity has been detected in other tissues and cells,including lung,lactating mammary gland, brain,and kidney.Expression of LPL in lung is related to the abundance of LPL-expressing macrophages[279].It has been proposed that LPL activ-ity in lung cancer tissue predicts shorter survival in patients with non-small cell lung cancer[280].Based on its macrophage origin,it can be speculated that LPL activity in lung is primarily under control of in?am-matory mediators,although no actual data are available.
8.2.LPL activity in the kidney
In contrast to adipose tissue and heart,LPL activity in kidney exhibits very pronounced inter-species variation,with highest activity in mink, followed by Chinese hamster and mouse,and low activity in rat and guinea pig[281].Food deprivation decreases LPL activity in kidney without corresponding changes in LPL mRNA or mass via a mechanism reliant on changes in gene transcription.LPL in kidney has been located to the mesanglial cells[282],where it promotes VLDL-induced lipid accumulation[283].In addition,LPL has been located to tubular epithelial cells and the renal vascular endothelium[197,281,284].
8.3.LPL activity in mammary gland
LPL activity is known to be highly elevated in the lactating mammary gland to extract circulating TG for incorporation into milk fat.Both secretory mammary epithelial cells and interstitial cells located in the connective tissue adjacent to mammary alveoli,which may represent regressed lipid-depleted mammary adipocytes,have been suggested to serve as source of LPL in the lactating mammary gland[284,285].In line with(residual)adipocytes being the primary carrier of LPL activity, adipocyte and mammary gland LPL activity respond in the same manner to metabolic stimuli.Indeed,experimental hyperinsulinemia induced LPL activity and mRNA in mammary gland of rats[286].Conversely, fasting decreased milk and heparin-releasable LPL activity in mouse/ rat mammary gland,whereas cell-associated LPL was less affected and LPL mRNA remained unaltered,pointing to a post-transcriptional mechanism[287,288].
Prolactin,which coordinates milk production during lactation,has been suggested to be a major stimulator of LPL activity in the mammary gland[289].Indeed,prolactin stimulated heparin-releasable LPL activity and LPL mRNA in cultured mammary tissues derived from mid-pregnant mice[290].However,Da Costa and Williamson did not?nd a major effect of prolactin and instead point to insulin as key hormone governing mammary gland LPL activity and plasma TG clearance in rats[291].No data are available about the potential mechanisms underlying insulin and prolactin action on LPL.
8.4.LPL activity in the brain
Due to the blood brain barrier,circulating lipoproteins are unable to reach the brain,except perhaps for small HDL particles.Most of the lipoproteins inside the central nervous system are likely produced by astrocytes.Currently,many aspects of lipoprotein metabolism in the brain remain enigmatic,including the role of neurons in regulation of lipoprotein metabolism and the major functions of lipoproteins in the brain[292].
Interestingly,LPL activity has also been found in the brain,in particu-lar in the hypothalamus,cortex,cerebellum,and midbrain of adult rats [293].In situ hybridization revealed strong LPL mRNA signals in pyrami-dal neurons of the rat hippocampus,the brain cortex and in the interme-diate lobe of the pituitary gland[294],while another study also found high levels of LPL activity and mRNA in the caudal spinal cord and in Purkinje cells of the cerebellum[295].Uptake and incorporation of fatty acids from labeled TG demonstrated the functionality of LPL in cultured brain cells,as well as in vivo in the CNS in general[293,295].LPL in brain has been associated with Alzheimer disease at the cellular and genetic level,but results obtained are too inconsistent to reach?rm conclusions.Recent evidence indicates that LPL in brain plays a role in regulation of food intake and in provision of essential fatty acids[296]. Speci?cally,neuron-speci?c LPL-de?cient mice were hyperphagic and obese.In contrast,genetic or chemical inactivation of LPL speci?cally in the hippocampus in rodents did not affect food intake but promoted body weight gain via decreased locomotor activity and energy expendi-ture,concomitant with increased parasympathetic tone[297].
In all brain regions tested except the hippocampus,LPL activity was either decreased by fasting,or showed no change[293,298,299].In rat hippocampus LPL activity was increased by fasting[298].Since lack of LPL leads to increased food intake,it can be hypothesized that fasting may exert an orexigenic effect partly via reduced LPL activity.No additional information is available about regulation of LPL activity in various brain regions by other(physiological)stimuli.
9.Conclusion
The cellular uptake of fatty acids needs to be adjusted to local requirements and thus highly?uctuates between different tissues and between different physiological and nutritional states.By catalyzing the hydrolysis of circulating TG,LPL serves as one of the central gatekeepers that controls local fatty acid uptake.Consistent with this important function,the activity of LPL is subject to multiple regulatory mechanisms via a number of regulatory proteins.Some of these mechanisms are locally driven and,for instance,may serve to limit uptake of fatty acids from TG-rich lipoproteins when other fuels such as plasma FFA are plentiful.Others are effectors of hormonal signals that coordinate fuel metabolism and fatty acid uptake between various tissues,depending on the physiological and nutritional state.The last decade has witnessed the emergence of a novel class of proteins named ANGPTLs whose primary function is to inhibit LPL activity. These ANGPTLs likely turn out to represent the elusive mediators in the local and hormonal control of LPL activity and resultant fatty acid uptake that were predicted from more ancient studies.Indeed, regulation of ANGPTL4was shown to be responsible for the reduction in LPL activity in white adipose tissue during fasting.Nevertheless,the current picture is still very far from complete.There is a clear need for appropriate experiments to try to demonstrate the role of each of these LPL modulators in the regulation of LPL activity in response to various local,hormonal and also pharmacological cues.Overall,the multitude of regulatory in?uences converging onto LPL illustrates the essential role of LPL-mediated plasma TG hydrolysis in cellular lipid homeostasis.
References
[1] E.D.Korn,Clearing factor,a heparin-activated lipoprotein lipase.I.Isolation and
characterization of the enzyme from normal rat heart,J.Biol.Chem.215(1955) 1–14.
[2] B.S.Davies,A.P.Beigneux,R.H.Barnes II,Y.Tu,P.Gin,M.M.Weinstein,C.Nobumori,
R.Nyren,I.Goldberg,G.Olivecrona,A.Bensadoun,S.G.Young,L.G.Fong,GPIHBP1 is responsible for the entry of lipoprotein lipase into capillaries,Cell Metab.12 (2010)42–52.
[3] A.P.Beigneux,B.S.Davies,P.Gin,M.M.Weinstein,E.Farber,X.Qiao,F.Peale,S.
Bunting,R.L.Walzem,J.S.Wong,W.S.Blaner,Z.M.Ding,K.Melford,N.
Wongsiriroj,X.Shu,F.de Sauvage,R.O.Ryan,L.G.Fong,A.Bensadoun,S.G.
Young,Glycosylphosphatidylinositol-anchored high-density lipoprotein-binding protein1plays a critical role in the lipolytic processing of chylomicrons,Cell Metab.5(2007)279–291.
[4]M.Peterfy,O.Ben-Zeev,H.Z.Mao,D.Weissglas-Volkov,B.E.Aouizerat,C.R.
Pullinger,P.H.Frost,J.P.Kane,M.J.Malloy,K.Reue,P.Pajukanta,M.H.Doolittle,Mu-tations in LMF1cause combined lipase de?ciency and severe hypertriglyceridemia, Nat.Genet.39(2007)1483–1487.
927
S.Kersten/Biochimica et Biophysica Acta1841(2014)919–933
[5]P.H.Iverius,A.M.Ostlund-Lindqvist,Lipoprotein lipase from bovine milk.Isolation
procedure,chemical characterization,and molecular weight analysis,J.Biol.Chem.
251(1976)7791–7795.
[6]Y.Kobayashi,T.Nakajima,I.Inoue,Molecular modeling of the dimeric structure of
human lipoprotein lipase and functional studies of the carboxyl-terminal domain, Eur.J.Biochem.269(2002)4701–4710.
[7]R.S.Holmes,J.L.Vandeberg,L.A.Cox,Comparative studies of vertebrate lipoprotein
lipase:a key enzyme of very low density lipoprotein metabolism,Comp.Biochem.
Physiol.Part D Genomics Proteomics6(2011)224–234.
[8]H.van Tilbeurgh,A.Roussel,https://www.360docs.net/doc/415523865.html,louel,C.Cambillau,Lipoprotein lipase.Molecu-
lar model based on the pancreatic lipase X-ray structure:consequences for heparin binding and catalysis,J.Biol.Chem.269(1994)4626–4633.
[9]P.Gin,C.N.Goulbourne,O.Adeyo,A.P.Beigneux,B.S.Davies,S.Tat,C.V.Voss,A.
Bensadoun,L.G.Fong,S.G.Young,Chylomicronemia mutations yield new insights into interactions between lipoprotein lipase and GPIHBP1,Hum.Mol.Genet.21 (2012)2961–2972.
[10]R.A.Sendak,A.Bensadoun,Identi?cation of a heparin-binding domain in the distal
carboxyl-terminal region of lipoprotein lipase by site-directed mutagenesis,J.Lipid Res.39(1998)1310–1315.
[11]J.Liu,H.Afroza,D.J.Rader,W.Jin,Angiopoietin-like protein3inhibits lipoprotein
lipase activity through enhancing its cleavage by proprotein convertases,J.Biol.
Chem.285(2010)27561–27570.
[12]S.Santamarina-Fojo,H.B.Brewer Jr.,The familial hyperchylomicronemia syndrome.
New insights into underlying genetic defects,JAMA265(1991)904–908.
[13]T.Coleman,R.L.Seip,J.M.Gimble,D.Lee,N.Maeda,C.F.Semenkovich,COOH-
terminal disruption of lipoprotein lipase in mice is lethal in homozygotes,but heterozygotes have elevated triglycerides and impaired enzyme activity,J.Biol.
Chem.270(1995)12518–12525.
[14]P.H.Weinstock,C.L.Bisgaier,K.Aalto-Setala,H.Radner,R.Ramakrishnan,S.
Levak-Frank,A.D.Essenburg,R.Zechner,J.L.Breslow,Severe hypertriglyc-eridemia,reduced high density lipoprotein,and neonatal death in lipoprotein lipase knockout https://www.360docs.net/doc/415523865.html,d hypertriglyceridemia with impaired very low density lipoprotein clearance in heterozygotes,J.Clin.Invest.96(1995) 2555–2568.
[15] A.Augustus,H.Yagyu,G.Haemmerle,A.Bensadoun,R.K.Vikramadithyan,S.Y.
Park,J.K.Kim,R.Zechner,I.J.Goldberg,Cardiac-speci?c knock-out of lipoprotein lipase alters plasma lipoprotein triglyceride metabolism and cardiac gene expression, J.Biol.Chem.279(2004)25050–25057.
[16]H.Wang,L.A.Knaub,D.R.Jensen,D.Young Jung,E.G.Hong,H.J.Ko,A.M.Coates,I.J.
Goldberg,B.A.de la Houssaye,R.C.Janssen,C.E.McCurdy,S.M.Rahman,C.Soo Choi,
G.I.Shulman,J.K.Kim,J.E.Friedman,R.H.Eckel,Skeletal muscle-speci?c deletion of
lipoprotein lipase enhances insulin signaling in skeletal muscle but causes insulin resistance in liver and other tissues,Diabetes58(2009)116–124.
[17]P.H.Weinstock,S.Levak-Frank,L.C.Hudgins,H.Radner,J.M.Friedman,R.Zechner,
J.L.Breslow,Lipoprotein lipase controls fatty acid entry into adipose tissue,but fat mass is preserved by endogenous synthesis in mice de?cient in adipose tissue lipoprotein lipase,Proc.Natl.Acad.Sci.U.S.A.94(1997)10261–10266.
[18] A.P.Beigneux,R.Franssen,A.Bensadoun,P.Gin,K.Melford,J.Peter,R.L.Walzem,M.M.
Weinstein,B.S.Davies,J.A.Kuivenhoven,J.J.Kastelein,L.G.Fong,G.M.Dallinga-Thie,S.
G.Young,Chylomicronemia with a mutant GPIHBP1(Q115P)that cannot bind
lipoprotein lipase,Arterioscler.Thromb.Vasc.Biol.29(2009)956–962.
[19]M.Shimada,H.Shimano,T.Gotoda,K.Yamamoto,M.Kawamura,T.Inaba,Y.
Yazaki,N.Yamada,Overexpression of human lipoprotein lipase in transgenic mice.Resistance to diet-induced hypertriglyceridemia and hypercholesterolemia, J.Biol.Chem.268(1993)17924–17929.
[20]P.Cornelius,S.Enerback,G.Bjursell,T.Olivecrona,P.H.Pekala,Regulation of
lipoprotein lipase mRNA content in3T3-L1cells by tumour necrosis factor, Biochem.J.249(1988)765–769.
[21]R.H.Eckel,W.Y.Fujimoto,J.D.Brunzell,Development of lipoprotein lipase in
cultured3T3-L1cells,https://www.360docs.net/doc/415523865.html,mun.78(1977)288–293. [22] A.Singh-Bist,M.C.Komaromy,F.B.Kraemer,Transcriptional regulation of lipopro-
tein lipase in the heart during development in the rat,Biochem.Biophys.Res.
Commun.202(1994)838–843.
[23]M.C.Jong,M.H.Hofker,L.M.Havekes,Role of ApoCs in lipoprotein metabolism:
functional differences between ApoC1,ApoC2,and ApoC3,Arterioscler.Thromb.
Vasc.Biol.19(1999)472–484.
[24]N.S.Shachter,T.Hayek,T.Leff,J.D.Smith, D.W.Rosenberg, A.Walsh,R.
Ramakrishnan,I.J.Goldberg,H.N.Ginsberg,J.L.Breslow,Overexpression of apolipo-protein CII causes hypertriglyceridemia in transgenic mice,J.Clin.Invest.93(1994) 1683–1690.
[25] A.A.Kei,T.D.Filippatos,V.Tsimihodimos,M.S.Elisaf,A review of the role of
apolipoprotein C-II in lipoprotein metabolism and cardiovascular disease,Metabolism 61(2012)906–921.
[26]K.Aalto-Setala,P.H.Weinstock,C.L.Bisgaier,L.Wu,J.D.Smith,J.L.Breslow,Further
characterization of the metabolic properties of triglyceride-rich lipoproteins from human and mouse apoC-III transgenic mice,J.Lipid Res.37(1996)1802–1811. [27]J.F.Berbee,C.C.van der Hoogt,D.Sundararaman,L.M.Havekes,P.C.Rensen,Severe
hypertriglyceridemia in human APOC1transgenic mice is caused by apoC-I-induced inhibition of LPL,J.Lipid Res.46(2005)297–306.
[28]M.C.Jong,P.C.Rensen,V.E.Dahlmans,H.van der Boom,T.J.van Berkel,L.M.
Havekes,Apolipoprotein C-III de?ciency accelerates triglyceride hydrolysis by lipoprotein lipase in wild-type and apoE knockout mice,J.Lipid Res.42(2001) 1578–1585.
[29]M.Westerterp,W.de Haan,J.F.Berbee,L.M.Havekes,P.C.Rensen,Endogenous
apoC-I increases hyperlipidemia in apoE-knockout mice by stimulating VLDL production and inhibiting LPL,J.Lipid Res.47(2006)1203–1211.[30]https://www.360docs.net/doc/415523865.html,rsson,E.Vorrsjo,P.Talmud,A.Lookene,G.Olivecrona,Apolipoproteins C-I
and C-III inhibit lipoprotein lipase activity by displacement of the enzyme from lipid droplets,J.Biol.Chem.288(2013)33997–34008.
[31]T.I.Pollin,C.M.Damcott,H.Shen,S.H.Ott,J.Shelton,R.B.Horenstein,W.Post,J.C.
McLenithan,L.F.Bielak,P.A.Peyser,B.D.Mitchell,https://www.360docs.net/doc/415523865.html,ler,J.R.O'Connell,A.R.
Shuldiner,A null mutation in human APOC3confers a favorable plasma lipid pro?le and apparent cardioprotection,Science322(2008)1702–1705.
[32]S.Kersten,Peroxisome proliferator activated receptors and lipoprotein metabo-
lism,PPAR Res.2008(2008)132960.
[33]S.K.Nilsson,J.Heeren,G.Olivecrona,M.Merkel,Apolipoprotein A-V;a potent
triglyceride reducer,Atherosclerosis219(2011)15–21.
[34]K.Albers,C.Schlein,K.Wenner,P.Lohse,A.Bartelt,J.Heeren,R.Santer,M.Merkel,
Homozygosity for a partial deletion of apoprotein A-V signal peptide results in intracellular missorting of the protein and chylomicronemia in a breast-fed infant, Atherosclerosis233(2014)97–103.
[35] C.Marcais,B.Verges,S.Charriere,V.Pruneta,M.Merlin,S.Billon,L.Perrot,J.Drai,A.
Sassolas,L.A.Pennacchio,J.Fruchart-Najib,J.C.Fruchart,V.Durlach,P.Moulin, Apoa5Q139X truncation predisposes to late-onset hyperchylomicronemia due to lipoprotein lipase impairment,J.Clin.Invest.115(2005)2862–2869.
[36] C.Priore Oliva,L.Pisciotta,G.Li Volti,M.P.Sambataro,A.Cantafora,A.Bellocchio,A.
Catapano,P.Tarugi,S.Bertolini,S.Calandra,Inherited apolipoprotein A-V de?cien-cy in severe hypertriglyceridemia,Arterioscler.Thromb.Vasc.Biol.25(2005) 411–417.
[37]J.C.Gonzales,P.L.Gordts,E.M.Foley,J.D.Esko,Apolipoproteins E and AV mediate
lipoprotein clearance by hepatic proteoglycans,J.Clin.Invest.123(2013) 2742–2751.
[38]M.Nowak,A.Helleboid-Chapman,H.Jakel,G.Martin,D.Duran-Sandoval,B.Staels,
E.M.Rubin,L.A.Pennacchio,M.R.Taskinen,J.Fruchart-Najib,J.C.Fruchart,Insulin-
mediated down-regulation of apolipoprotein A5gene expression through the phosphatidylinositol3-kinase pathway:role of upstream stimulatory factor,Mol.
Cell.Biol.25(2005)1537–1548.
[39]M.Nowak,A.Helleboid-Chapman,H.Jakel,E.Moitrot,C.Rommens,L.A.Pennacchio,J.
Fruchart-Najib,J.C.Fruchart,Glucose regulates the expression of the apolipoprotein A5gene,J.Mol.Biol.380(2008)789–798.
[40]X.Prieur,H.Coste,J.C.Rodriguez,The human apolipoprotein AV gene is regulated
by peroxisome proliferator-activated receptor-alpha and contains a novel farnesoid X-activated receptor response element,J.Biol.Chem.278(2003) 25468–25480.
[41]N.Vu-Dac,P.Gervois,H.Jakel,M.Nowak,E.Bauge,H.Dehondt,B.Staels,L.A.
Pennacchio,E.M.Rubin,J.Fruchart-Najib,J.C.Fruchart,Apolipoprotein A5,a crucial determinant of plasma triglyceride levels,is highly responsive to peroxisome proliferator-activated receptor alpha activators,J.Biol.Chem.278(2003) 17982–17985.
[42]X.Prieur,P.Lesnik,M.Moreau,J.C.Rodriguez,C.Doucet,M.J.Chapman,T.Huby,
Differential regulation of the human versus the mouse apolipoprotein AV gene by PPARalpha.Implications for the study of pharmaceutical modi?ers of hypertri-glyceridemia in mice,Biochim.Biophys.Acta1791(2009)764–771.
[43]P.Hahne,F.Krempler,F.G.Schaap,S.M.Soyal,H.Hof?nger,https://www.360docs.net/doc/415523865.html,ler,H.Oberko?er,
W.Strobl,W.Patsch,Determinants of plasma apolipoprotein A-V and APOA5gene transcripts in humans,J.Intern.Med.264(2008)452–462.
[44]J.M.Ordovas,V.Mooser,The APOE locus and the pharmacogenetics of lipid
response,Curr.Opin.Lipidol.13(2002)113–117.
[45] A.D.Cooper,Hepatic uptake of chylomicron remnants,J.Lipid Res.38(1997)
2173–2192.
[46]P.C.Rensen,T.J.van Berkel,Apolipoprotein E effectively inhibits lipoprotein lipase-
mediated lipolysis of chylomicron-like triglyceride-rich lipid emulsions in vitro and in vivo,J.Biol.Chem.271(1996)14791–14799.
[47]G.Gerritsen,P.C.Rensen,K.E.Kypreos,V.I.Zannis,L.M.Havekes,K.Willems van
Dijk,ApoC-III de?ciency prevents hyperlipidemia induced by apoE overexpression, J.Lipid Res.46(2005)1466–1473.
[48] A.R.Mensenkamp,M.C.Jong,H.van Goor,M.J.van Luyn,V.Bloks,R.Havinga,P.J.
Voshol,M.H.Hofker,K.W.van Dijk,L.M.Havekes,F.Kuipers,Apolipoprotein E participates in the regulation of very low density lipoprotein-triglyceride secretion by the liver,J.Biol.Chem.274(1999)35711–35718.
[49]W.J.McConathy,C.S.Wang,Inhibition of lipoprotein lipase by the receptor-binding
domain of apolipoprotein E,FEBS Lett.251(1989)250–252.
[50]S.Kersten,S.Mandard,N.S.Tan,P.Escher,D.Metzger,P.Chambon,F.J.Gonzalez,B.
Desvergne,W.Wahli,Characterization of the fasting-induced adipose factor FIAF,a novel peroxisome proliferator-activated receptor target gene,J.Biol.Chem.275 (2000)28488–28493.
[51]J.C.Yoon,T.W.Chickering,E.D.Rosen,B.Dussault,Y.Qin,A.Soukas,J.M.Friedman,
W.E.Holmes,B.M.Spiegelman,Peroxisome proliferator-activated receptor gamma target gene encoding a novel angiopoietin-related protein associated with adipose differentiation,Mol.Cell.Biol.20(2000)5343–5349.
[52]M.Bunger,H.M.van den Bosch,J.van der Meijde,S.Kersten,G.J.Hooiveld,M.
Muller,Genome-wide analysis of PPARalpha activation in murine small intestine, Physiol.Genomics30(2007)192–204.
[53] A.Georgiadi,L.Lichtenstein,T.Degenhardt,M.V.Boekschoten,M.van Bilsen,B.
Desvergne,M.Muller,S.Kersten,Induction of cardiac Angptl4by dietary fatty acids is mediated by peroxisome proliferator-activated receptor beta/delta and protects against fatty acid-induced oxidative stress,Circ.Res.106(2010) 1712–1721.
[54]Y.Jia,J.Y.Kim,H.J.Jun,S.J.Kim,J.H.Lee,M.H.Hoang,K.Y.Hwang,S.J.Um,H.I.Chang,
S.J.Lee,The natural carotenoid astaxanthin,a PPAR-alpha agonist and PPAR-gamma antagonist,reduces hepatic lipid accumulation by rewiring the transcriptome in lipid-loaded hepatocytes,Mol.Nutr.Food Res.56(2012)878–888.
928S.Kersten/Biochimica et Biophysica Acta1841(2014)919–933
[55]L.Lichtenstein,F.Mattijssen,N.J.de Wit,A.Georgiadi,G.J.Hooiveld,R.van der Meer,Y.
He,L.Qi,A.Koster,J.T.Tamsma,N.S.Tan,M.Muller,S.Kersten,Angptl4protects against severe proin?ammatory effects of saturated fat by inhibiting fatty acid uptake into mesenteric lymph node macrophages,Cell Metab.12(2010)580–592.
[56]H.Staiger,C.Haas,J.Machann,R.Werner,M.Weisser,F.Schick,F.Machicao,N.
Stefan,A.Fritsche,H.U.Haring,Muscle-derived angiopoietin-like protein4is induced by fatty acids via peroxisome proliferator-activated receptor(PPAR)-delta and is of metabolic relevance in humans,Diabetes58(2009)579–589. [57]I.J.A,N.S.Tan,L.Gelman,S.Kersten,J.Seydoux,J.Xu,D.Metzger,L.Canaple,P.
Chambon,W.Wahli,B.Desvergne,In vivo activation of PPAR target genes by RXR homodimers,EMBO J.23(2004)2083–2091.
[58]S.Kersten,L.Lichtenstein,E.Steenbergen,K.Mudde,H.F.Hendriks,M.K.Hesselink,
P.Schrauwen,M.Muller,Caloric restriction and exercise increase plasma ANGPTL4 levels in humans via elevated free fatty acids,Arterioscler.Thromb.Vasc.Biol.29 (2009)969–974.
[59]S.Mandard,F.Zandbergen,E.van Straten,W.Wahli,F.Kuipers,M.Muller,S.
Kersten,The fasting-induced adipose factor/angiopoietin-like protein4is physical-ly associated with lipoproteins and governs plasma lipid levels and adiposity,J.
Biol.Chem.281(2006)934–944.
[60] A.Xu,https://www.360docs.net/doc/415523865.html,m,K.W.Chan,Y.Wang,J.Zhang,R.L.Hoo,J.Y.Xu,B.Chen,W.S.Chow,
A.W.Tso,https://www.360docs.net/doc/415523865.html,m,Angiopoietin-like protein4decreases blood glucose and
improves glucose tolerance but induces hyperlipidemia and hepatic steatosis in mice,Proc.Natl.Acad.Sci.U.S.A.102(2005)6086–6091.
[61]X.Yu,S.C.Burgess,H.Ge,K.K.Wong,R.H.Nassem,D.J.Garry,A.D.Sherry,C.R.Malloy,J.
P.Berger,C.Li,Inhibition of cardiac lipoprotein utilization by transgenic overexpres-sion of Angptl4in the heart,Proc.Natl.Acad.Sci.U.S.A.102(2005)1767–1772.
[62] A.Koster,Y.B.Chao,M.Mosior,A.Ford,P.A.Gonzalez-DeWhitt,J.E.Hale,D.Li,Y.Qiu,C.
C.Fraser,
D.D.Yang,J.G.Heuer,S.R.Jaskunas,P.Eacho,Transgenic angiopoietin-like
(angptl)4overexpression and targeted disruption of angptl4and angptl3:regulation of triglyceride metabolism,Endocrinology146(2005)4943–4950.
[63]L.Lichtenstein,J.F.Berbee,S.J.van Dijk,K.W.van Dijk,A.Bensadoun,I.P.Kema,P.J.
Voshol,M.Muller,P.C.Rensen,S.Kersten,Angptl4upregulates cholesterol synthe-sis in liver via inhibition of LPL-and HL-dependent hepatic cholesterol uptake, Arterioscler.Thromb.Vasc.Biol.27(2007)2420–2427.
[64]V.Sukonina,A.Lookene,T.Olivecrona,G.Olivecrona,Angiopoietin-like protein4
converts lipoprotein lipase to inactive monomers and modulates lipase activity in adipose tissue,Proc.Natl.Acad.Sci.U.S.A.103(2006)17450–17455.
[65]https://www.360docs.net/doc/415523865.html,fferty,K.C.Bradford,D.A.Erie,S.B.Neher,Angiopoietin-like protein4inhibi-
tion of lipoprotein lipase:evidence for reversible complex formation,J.Biol.Chem.
288(2013)28524–28534.
[66]O.Kroupa,E.Vorrsjo,R.Stienstra,F.Mattijssen,S.K.Nilsson,V.Sukonina,S.Kersten,G.
Olivecrona,T.Olivecrona,Linking nutritional regulation of Angptl4,Gpihbp1,and Lmf1to lipoprotein lipase activity in rodent adipose tissue,BMC Physiol.12(2012)13.
[67]M.Catoire,S.Alex,N.Paraskevopulos,F.Mattijssen,G.Schaart,A.Kneppers,I.
Evers-van Gogh,M.Mensink,P.J.Voshol,G.Olivecrona,N.S.Tan,M.K.C.
Hesselink,J.F.Berbée,P.C.N.Rensen,E.Kalkhoven,P.Schrauwen,S.Kersten,Fatty acid-inducible ANGPTL4governs lipid metabolic response to exercise,Proc.Natl.
Acad.Sci.U.S.A.111(2014)E1043-E152.
[68]S.Romeo,L.A.Pennacchio,Y.Fu,E.Boerwinkle,A.Tybjaerg-Hansen,H.H.Hobbs,J.
C.Cohen,Population-based resequencing of ANGPTL4uncovers variations that
reduce triglycerides and increase HDL,Nat.Genet.39(2007)513–516.
[69]Y.Y.Goh,M.Pal,H.C.Chong,P.Zhu,M.J.Tan,L.Punugu,https://www.360docs.net/doc/415523865.html,m,Y.H.Yau,C.K.Tan,
R.L.Huang,S.M.Tan,M.B.Tang,J.L.Ding,S.Kersten,N.S.Tan,Angiopoietin-like4 interacts with integrins beta1and beta5to modulate keratinocyte migration,Am.
J.Pathol.177(2010)2791–2803.
[70]P.Zhu,Y.Y.Goh,H.F.Chin,S.Kersten,N.S.Tan,Angiopoietin-like4:a decade of
research,Biosci.Rep.32(2012)211–219.
[71]R.Koishi,Y.Ando,M.Ono,M.Shimamura,H.Yasumo,T.Fujiwara,H.Horikoshi,H.
Furukawa,Angptl3regulates lipid metabolism in mice,Nat.Genet.30(2002) 151–157.
[72]I.Minicocci,A.Montali,M.R.Robciuc,F.Quagliarini,V.Censi,https://www.360docs.net/doc/415523865.html,bbadia,C.Gabiati,
G.Pigna,M.L.Sepe,F.Pannozzo,D.Lutjohann,S.Fazio,M.Jauhiainen,C.Ehnholm,
M.Arca,Mutations in the ANGPTL3gene and familial combined hypolipidemia:a clinical and biochemical characterization,J.Clin.Endocrinol.Metab.97(2012) E1266–E1275.
[73]S.Kathiresan,O.Melander,C.Guiducci,A.Surti,N.P.Burtt,M.J.Rieder,G.M.Cooper,
C.Roos,B.F.Voight,A.S.Havulinna,B.Wahlstrand,T.Hedner,
D.Corella,
E.S.Tai,J.
M.Ordovas,G.Berglund,E.Vartiainen,P.Jousilahti,B.Hedblad,M.R.Taskinen,C.
Newton-Cheh,V.Salomaa,L.Peltonen,L.Groop,D.M.Altshuler,M.Orho-Melander,Six new loci associated with blood low-density lipoprotein cholesterol, high-density lipoprotein cholesterol or triglycerides in humans,Nat.Genet.40 (2008)189–197.
[74] C.J.Willer,S.Sanna,A.U.Jackson,A.Scuteri,L.L.Bonnycastle,R.Clarke,S.C.Heath,
N.J.Timpson,S.S.Najjar,H.M.Stringham,J.Strait,W.L.Duren,A.Maschio,F.
Busonero,A.Mulas,G.Albai,A.J.Swift,M.A.Morken,N.Narisu,D.Bennett,S.
Parish,H.Shen,P.Galan,P.Meneton,S.Hercberg,D.Zelenika,W.M.Chen,Y.Li, L.J.Scott,P.A.Scheet,J.Sundvall,R.M.Watanabe,R.Nagaraja,S.Ebrahim,D.A.
Lawlor,Y.Ben-Shlomo,G.Davey-Smith,A.R.Shuldiner,R.Collins,R.N.Bergman, M.Uda,J.Tuomilehto,A.Cao,F.S.Collins,https://www.360docs.net/doc/415523865.html,katta,https://www.360docs.net/doc/415523865.html,throp,M.Boehnke,D.
Schlessinger,K.L.Mohlke,G.R.Abecasis,Newly identi?ed loci that in?uence lipid concentrations and risk of coronary artery disease,Nat.Genet.40(2008)161–169.
[75]K.Fujimoto,R.Koishi,T.Shimizugawa,Y.Ando,Angptl3-null mice show low plas-
ma lipid concentrations by enhanced lipoprotein lipase activity,Exp.Anim.55 (2006)27–34.
[76]T.Shimizugawa,M.Ono,M.Shimamura,K.Yoshida,Y.Ando,R.Koishi,K.Ueda,T.
Inaba,H.Minekura,T.Kohama,H.Furukawa,ANGPTL3decreases very low density
lipoprotein triglyceride clearance by inhibition of lipoprotein lipase,J.Biol.Chem.
277(2002)33742–33748(JID—2985121R).
[77]L.Shan,X.C.Yu,Z.Liu,Y.Hu,L.T.Sturgis,M.L.Miranda,Q.Liu,The angiopoietin-like
proteins ANGPTL3and ANGPTL4inhibit lipoprotein lipase activity through distinct mechanisms,J.Biol.Chem.284(2009)1419–1424.
[78]W.K.Sonnenburg,D.Yu,E.C.Lee,W.Xiong,G.Gololobov,B.Key,J.Gay,N.
Wilganowski,Y.Hu,S.Zhao,M.Schneider,Z.M.Ding,B.P.Zambrowicz,G.
Landes,D.R.Powell,U.Desai,GPIHBP1stabilizes lipoprotein lipase and prevents its inhibition by angiopoietin-like3and angiopoietin-like4,J.Lipid Res.50 (2009)2421–2429.
[79]K.Inukai,Y.Nakashima,M.Watanabe,S.Kurihara,T.Awata,H.Katagiri,Y.Oka,S.
Katayama,ANGPTL3is increased in both insulin-de?cient and-resistant diabetic states,https://www.360docs.net/doc/415523865.html,mun.317(2004)1075–1079.
[80]M.Shimamura,M.Matsuda,Y.Ando,R.Koishi,H.Yasumo,H.Furukawa,I.
Shimomura,Leptin and insulin down-regulate angiopoietin-like protein3,a plas-ma triglyceride-increasing factor,https://www.360docs.net/doc/415523865.html,mun.322(2004) 1080–1085.
[81]R.Kaplan,T.Zhang,M.Hernandez,F.X.Gan,S.D.Wright,M.G.Waters,T.Q.Cai,
Regulation of the angiopoietin-like protein3gene by LXR,J.Lipid Res.44(2003) 136–143.
[82] F.Quagliarini,Y.Wang,J.Kozlitina,N.V.Grishin,R.Hyde,E.Boerwinkle,D.M.
Valenzuela,A.J.Murphy,J.C.Cohen,H.H.Hobbs,Atypical angiopoietin-like protein that regulates ANGPTL3,Proc.Natl.Acad.Sci.U.S.A.109(2012)19751–19756.
[83]G.Ren,J.Y.Kim,C.M.Smas,Identi?cation of RIFL,a novel adipocyte-enriched
insulin target gene with a role in lipid metabolism,Am.J.Physiol.Endocrinol.
Metab.303(2012)E334–E351.
[84]R.Zhang,Lipasin,a novel nutritionally-regulated liver-enriched factor that regulates
serum triglyceride levels,https://www.360docs.net/doc/415523865.html,mun.424(2012)786–792. [85]Y.Wang,F.Quagliarini,V.Gusarova,J.Gromada,D.M.Valenzuela,J.C.Cohen,H.H.
Hobbs,Mice lacking ANGPTL8(Betatrophin)manifest disrupted triglyceride metabolism without impaired glucose homeostasis,Proc.Natl.Acad.Sci.U.S.A.
110(2013)16109–16114.
[86]Z.Fu,F.Yao,A.B.Abou-Samra,R.Zhang,Lipasin,thermoregulated in brown fat,is a
novel but atypical member of the angiopoietin-like protein family,Biochem.
https://www.360docs.net/doc/415523865.html,mun.430(2013)1126–1131.
[87]M.K.Hellerstein,De novo lipogenesis in humans:metabolic and regulatory aspects,
Eur.J.Clin.Nutr.53(Suppl.1)(1999)S53–S65.
[88]https://www.360docs.net/doc/415523865.html,liotis,https://www.360docs.net/doc/415523865.html,elis,E.Rogdakis,Comparative approach of the de novo fatty acid
synthesis(lipogenesis)between ruminant and non ruminant mammalian species: from biochemical level to the main regulatory lipogenic genes,Curr.Genomics11 (2010)168–183.
[89]S.G.Young,R.Zechner,Biochemistry and pathophysiology of intravascular and
intracellular lipolysis,Genes Dev.27(2013)459–484.
[90] B.Teusink,P.J.Voshol,V.E.Dahlmans,P.C.Rensen,H.Pijl,J.A.Romijn,L.M.Havekes,
Contribution of fatty acids released from lipolysis of plasma triglycerides to total plasma fatty acid?ux and tissue-speci?c fatty acid uptake,Diabetes52(2003) 614–620.
[91]M.Hultin,R.Savonen,T.Olivecrona,Chylomicron metabolism in rats:lipolysis,
recirculation of triglyceride-derived fatty acids in plasma FFA,and fate of core lipids as analyzed by compartmental modelling,J.Lipid Res.37(1996)1022–1036.
[92]K.N.Frayn,S.W.Coppack,B.A.Fielding,S.M.Humphreys,Coordinated regulation of
hormone-sensitive lipase and lipoprotein lipase in human adipose tissue in vivo: implications for the control of fat storage and fat mobilization,Adv.Enzym.
Regul.35(1995)163–178.
[93]T.Ruge,L.Hodson,J.Cheeseman,A.L.Dennis,B.A.Fielding,S.M.Humphreys,K.N.
Frayn,F.Karpe,Fasted to fed traf?cking of fatty acids in human adipose tissue reveals a novel regulatory step for enhanced fat storage,J.Clin.Endocrinol.
Metab.94(2009)1781–1788.
[94] C.H.Hollenberg,Effect of nutrition on activity and release of lipase from rat adipose
tissue,Am.J.Physiol.197(1959)667–670.
[95] A.Cherkes,R.S.Gordon Jr.,J.Lipid Res.1(1959)97–101.
[96]S.Enerback,H.Semb,J.Tavernier,G.Bjursell,T.Olivecrona,Tissue-speci?c regula-
tion of guinea pig lipoprotein lipase;effects of nutritional state and of tumor necro-sis factor on mRNA levels in adipose tissue,heart and liver,Gene64(1988) 97–106.
[97]https://www.360docs.net/doc/415523865.html,du,H.Kapsas,W.K.Palmer,Regulation of lipoprotein lipase in adipose and
muscle tissues during fasting,Am.J.Physiol.260(1991)R953–R959.
[98]J.M.Ong,R.B.Simsolo,B.Saffari,P.A.Kern,The regulation of lipoprotein lipase gene
expression by dexamethasone in isolated rat adipocytes,Endocrinology130 (1992)2310–2316.
[99]S.K.Koliwad,T.Kuo,L.E.Shipp,N.E.Gray,F.Backhed,A.Y.So,R.V.Farese Jr.,J.C.
Wang,Angiopoietin-like4(ANGPTL4,fasting-induced adipose factor)is a direct glucocorticoid receptor target and participates in glucocorticoid-regulated triglyc-eride metabolism,J.Biol.Chem.284(2009)25593–25601.
[100]S.K.Fried,C.D.Russell,N.L.Grauso,R.E.Brolin,Lipoprotein lipase regulation by insulin and glucocorticoid in subcutaneous and omental adipose tissues of obese women and men,J.Clin.Invest.92(1993)2191–2198.
[101]M.Ottosson,P.Marin,K.Karason,A.Elander,P.Bjorntorp,Blockade of the gluco-corticoid receptor with RU486:effects in vitro and in vivo on human adipose tissue lipoprotein lipase activity,Obes.Res.3(1995)233–240.
[102]T.Kidani,K.Sakayama,H.Masuno,N.Takubo,Y.Matsuda,H.Okuda,H.Yamamoto, Active-dimeric form of lipoprotein lipase increases in the adipose tissue of patients with rheumatoid arthritis treated with prednisolone,Biochim.Biophys.Acta1584 (2002)31–36.
[103] F.Picard,N.Naimi,D.Richard,Y.Deshaies,Response of adipose tissue lipoprotein lipase to the cephalic phase of insulin secretion,Diabetes48(1999)452–459.
929
S.Kersten/Biochimica et Biophysica Acta1841(2014)919–933
[104]J.M.Ong,T.G.Kirchgessner,M.C.Schotz,P.A.Kern,Insulin increases the synthetic rate and messenger RNA level of lipoprotein lipase in isolated rat adipocytes,J.
Biol.Chem.263(1988)12933–12938.
[105]M.V.Raynolds,P.D.Awald,D.F.Gordon,A.Gutierrez-Hartmann,D.C.Rule,W.M.
Wood,R.H.Eckel,Lipoprotein lipase gene expression in rat adipocytes is regulated by isoproterenol and insulin through different mechanisms,Mol.Endocrinol.4 (1990)1416–1422.
[106]S.J.Kim,C.Nian,C.H.McIntosh,GIP increases human adipocyte LPL expression through CREB and TORC2-mediated trans-activation of the LPL gene,J.Lipid Res.
51(2010)3145–3157.
[107]S.J.Kim,C.Nian,C.H.McIntosh,Resistin knockout mice exhibit impaired adipocyte glucose-dependent insulinotropic polypeptide receptor(GIPR)expression, Diabetes62(2013)471–477.
[108] C.F.Semenkovich,M.Wims,L.Noe,J.Etienne,L.Chan,Insulin regulation of lipopro-tein lipase activity in3T3-L1adipocytes is mediated at posttranscriptional and posttranslational levels,J.Biol.Chem.264(1989)9030–9038.
[109] E.Z.Amri,L.Teboul,C.Vannier,P.A.Grimaldi,G.Ailhaud,Fatty acids regulate the expression of lipoprotein lipase gene and activity in preadipose and adipose cells,Biochem.J.314(Pt2)(1996)541–546.
[110]J.Auwerx,K.Schoonjans,J.C.Fruchart,B.Staels,Regulation of triglyceride metabo-lism by PPARs:?brates and thiazolidinediones have distinct effects,J.Atheroscler.
Thromb.3(1996)81–89.
[111]K.Schoonjans,J.Peinado-Onsurbe,A.M.Lefebvre,R.A.Heyman,M.Briggs,S.Deeb,
B.Staels,J.Auwerx,PPARalpha and PPARgamma activators direct a distinct tissue-
speci?c transcriptional response via a PPRE in the lipoprotein lipase gene,EMBO J.
15(1996)5336–5348.
[112] A.Vidal-Puig,M.Jimenez-Linan,B.B.Lowell,A.Hamann,E.Hu,B.Spiegelman,J.S.
Flier,D.E.Moller,Regulation of PPAR gamma gene expression by nutrition and obesity in rodents,J.Clin.Invest.97(1996)2553–2561.
[113]G.D.Tan,G.Olivecrona,H.Vidal,K.N.Frayn,F.Karpe,Insulin sensitisation affects lipoprotein lipase transport in type2diabetes:role of adipose tissue and skeletal muscle in response to rosiglitazone,Diabetologia49(2006)2412–2418.
[114]J.B.Kim,P.Sarraf,M.Wright,K.M.Yao,E.Mueller,G.Solanes,B.B.Lowell,B.M.
Spiegelman,Nutritional and insulin regulation of fatty acid synthetase and leptin gene expression through ADD1/SREBP1,J.Clin.Invest.101(1998)1–9.
[115]K.Schoonjans,L.Gelman,C.Haby,M.Briggs,J.Auwerx,Induction of LPL gene expression by sterols is mediated by a sterol regulatory element and is indepen-dent of the presence of multiple E boxes,J.Mol.Biol.304(2000)323–334. [116] D.H.Bessesen,A.D.Robertson,R.H.Eckel,Weight reduction increases adipose but decreases cardiac LPL in reduced-obese Zucker rats,Am.J.Physiol.261(1991) E246–E251.
[117]M.C.Sugden,M.J.Holness,R.M.Howard,Changes in lipoprotein lipase activities in adipose tissue,heart and skeletal muscle during continuous or interrupted feeding, Biochem.J.292(Pt1)(1993)113–119.
[118]M.Bergo,G.Olivecrona,T.Olivecrona,Regulation of adipose tissue lipoprotein lipase in young and old rats,Int.J.Obes.Relat.Metab.Disord.21(1997)980–986. [119]M.H.Doolittle,O.Ben-Zeev,J.Elovson,D.Martin,T.G.Kirchgessner,The response of lipoprotein lipase to feeding and fasting.Evidence for posttranslational regulation, J.Biol.Chem.265(1990)4570–4577.
[120]T.J.Yost,D.R.Jensen,B.R.Haugen,R.H.Eckel,Effect of dietary macronutrient com-position on tissue-speci?c lipoprotein lipase activity and insulin action in normal-weight subjects,Am.J.Clin.Nutr.68(1998)296–302.
[121]G.R.Eagle,D.S.Robinson,The ability of actinomycin D to increase the clearing-factor lipase activity of rat adipose tissue,Biochem.J.93(1964)10C–11C. [122] D.R.Wing,D.S.Robinson,Clearing-factor lipase in adipose tissue.Studies with puromycin and actinomycin,Biochem.J.106(1968)667–676.
[123] D.R.Wing,M.R.Salaman,D.S.Robinson,Clearing-factor lipase in adipose tissue.
Factors in?uencing the increase in enzyme activity produced on incubation of tissue from starved rats in vitro,Biochem.J.99(1966)648–656.
[124]M.Bergo,G.Olivecrona,T.Olivecrona,Forms of lipoprotein lipase in rat tissues:in adipose tissue the proportion of inactive lipase increases on fasting,Biochem.J.313 (Pt3)(1996)893–898.
[125]M.Bergo,G.Wu,T.Ruge,T.Olivecrona,Down-regulation of adipose tissue lipopro-tein lipase during fasting requires that a gene,separate from the lipase gene,is switched on,J.Biol.Chem.277(2002)11927–11932.
[126]G.Wu,G.Olivecrona,T.Olivecrona,The distribution of lipoprotein lipase in rat adipose tissue.Changes with nutritional state engage the extracellular enzyme,J.
Biol.Chem.278(2003)11925–11930.
[127]Q.Yao,M.K.Shin,J.C.Jun,K.L.Hernandez,N.R.Aggarwal,J.R.Mock,J.Gay,L.F.
Drager,V.Y.Polotsky,Effect of chronic intermittent hypoxia on triglyceride uptake in different tissues,J.Lipid Res.54(2013)1058–1065.
[128]R.V.Farese Jr.,T.J.Yost,R.H.Eckel,Tissue-speci?c regulation of lipoprotein lipase activ-ity by insulin/glucose in normal-weight humans,Metabolism40(1991)214–216. [129]T.Yamada,N.Ozaki,Y.Kato,Y.Miura,Y.Oiso,Insulin downregulates angiopoietin-like protein4mRNA in3T3-L1adipocytes,https://www.360docs.net/doc/415523865.html,mun.347 (2006)1138–1144.
[130]T.Kuo,T.C.Chen,S.Yan,F.Foo,C.Ching,A.McQueen,J.C.Wang,Repression of glucocorticoid-stimulated angiopoietin-like4(Angptl4)gene transcription by insulin,J.Lipid Res.(2014).
[131] D.H.van Raalte,M.Brands,M.J.Serlie,K.Mudde,R.Stienstra,H.P.Sauerwein,S.
Kersten,M.Diamant,Angiopoietin-like protein4is differentially regulated by glucocorticoids and insulin in vitro and in vivo in healthy humans,Exp.Clin.
Endocrinol.Diabetes120(2012)598–603.
[132]G.E.Chiappe de Cingalani,J.W.Goers,M.Giannotti,C.I.Caldiz,Comparative effects of insulin and isoproterenol on lipoprotein lipase in rat adipose cells,Am.J.Physiol.
270(1996)C1461–C1467.[133] F.B.Kraemer,D.Takeda,V.Natu,C.Sztalryd,Insulin regulates lipoprotein lipase activity in rat adipose cells via wortmannin-and rapamycin-sensitive pathways, Metabolism47(1998)555–559.
[134]P.Gonzalez-Muniesa,C.de Oliveira,F.Perez de Heredia,M.P.Thompson,P.
Trayhurn,Fatty acids and hypoxia stimulate the expression and secretion of the adipokine ANGPTL4(angiopoietin-like protein4/fasting-induced adipose factor) by human adipocytes,J.Nutrigenet.Nutrigenomics4(2011)146–153.
[135]J.L.Kirkland,C.H.Hollenberg,S.Kindler,D.A.Roncari,Long-chain fatty acids decrease lipoprotein lipase activity of cultured rat adipocyte precursors,Metabo-lism43(1994)144–151.
[136] A.Louhija,Hypertriglyceridemia in rats at simulated high altitudes,Experientia25 (1969)248–249.
[137]https://www.360docs.net/doc/415523865.html,penskii,The effect of experimental hypoxic hypoxia on the blood clearing factor,Patol.Fiziol.Eksp.Ter.9(1965)88–89.
[138]P.M.Young,M.S.Rose,J.R.Sutton,H.J.Green,A.Cymerman,C.S.Houston,Operation Everest II:plasma lipid and hormonal responses during a simulated ascent of Mt.
Everest,J.Appl.Physiol.66(1989)1430–1435.
[139]L.F.Drager,J.Li,M.K.Shin,C.Reinke,N.R.Aggarwal,J.C.Jun,S.Bevans-Fonti,C.
Sztalryd,S.M.O'Byrne,O.Kroupa,G.Olivecrona,W.S.Blaner,V.Y.Polotsky,Inter-mittent hypoxia inhibits clearance of triglyceride-rich lipoproteins and inactivates adipose lipoprotein lipase in a mouse model of sleep apnoea,Eur.Heart J.33 (2012)783–790.
[140] B.Wang,I.S.Wood,P.Trayhurn,Dysregulation of the expression and secretion of in?ammation-related adipokines by hypoxia in human adipocytes,P?ugers Arch.
—Eur.J.Physiol.455(2007)479–492.
[141] D.Ricart-Jane,P.Cejudo-Martin,J.Peinado-Onsurbe,M.D.Lopez-Tejero,M.Llobera, Changes in lipoprotein lipase modulate tissue energy supply during stress,J.Appl.
Physiol.99(2005)1343–1351.
[142] A.Casanovas,N.Parramon,F.de la Cruz,O.Andres,J.Terencio,M.D.Lopez-Tejero, M.Llobera,Retroperitoneal white adipose tissue lipoprotein lipase activity is rapid-ly down-regulated in response to acute stress,J.Lipid Res.48(2007)863–868. [143]G.Ranganathan,D.Phan,I.D.Pokrovskaya,J.E.McEwen,C.Li,P.A.Kern,The trans-lational regulation of lipoprotein lipase by epinephrine involves an RNA binding complex including the catalytic subunit of protein kinase A,J.Biol.Chem.277 (2002)43281–43287.
[144] A.Yukht,R.C.Davis,J.M.Ong,G.Ranganathan,P.A.Kern,Regulation of lipoprotein lipase translation by epinephrine in3T3-L1cells.Importance of the3′untranslated region,J.Clin.Invest.96(1995)2438–2444.
[145]J.S.Samra,L.K.Summers,K.N.Frayn,Sepsis and fat metabolism,Br.J.Surg.83 (1996)1186–1196.
[146] A.M.Barcia,H.W.Harris,Triglyceride-rich lipoproteins as agents of innate immuni-ty,Clin.Infect.Dis.41(Suppl.7)(2005)S498–S503.
[147]T.E.Read,C.Grunfeld,Z.L.Kumwenda,M.C.Calhoun,J.P.Kane,K.R.Feingold,J.H.
Rapp,Triglyceride-rich lipoproteins prevent septic death in rats,J.Exp.Med.182 (1995)267–272.
[148]G.J.Bagby,C.B.Corll,R.R.Martinez,Triacylglycerol kinetics in endotoxic rats with suppressed lipoprotein lipase activity,Am.J.Physiol.253(1987)E59–E64. [149]G.J.Bagby,J.A.Spitzer,Lipoprotein lipase activity in rat heart and adipose tissue during endotoxic shock,Am.J.Physiol.238(1980)H325–H330.
[150]M.Kawakami,A.Cerami,Studies of endotoxin-induced decrease in lipoprotein lipase activity,J.Exp.Med.154(1981)631–639.
[151] F.Picard,D.Arsenijevic,D.Richard,Y.Deshaies,Responses of adipose and muscle lipoprotein lipase to chronic infection and subsequent acute lipopolysaccharide challenge,https://www.360docs.net/doc/415523865.html,b.Immunol.9(2002)771–776.
[152]I.Gouni,K.Oka,J.Etienne,L.Chan,Endotoxin-induced hypertriglyceridemia is mediated by suppression of lipoprotein lipase at a post-transcriptional level,J.
Lipid Res.34(1993)139–146.
[153] B.Beutler,J.Mahoney,N.Le Trang,P.Pekala,A.Cerami,Puri?cation of cachectin,a lipoprotein lipase-suppressing hormone secreted by endotoxin-induced RAW264.
7cells,J.Exp.Med.161(1985)984–995.
[154]S.K.Fried,R.Zechner,Cachectin/tumor necrosis factor decreases human adipose tissue lipoprotein lipase mRNA levels,synthesis,and activity,J.Lipid Res.30 (1989)1917–1923.
[155] C.Grunfeld,R.Gulli,A.H.Moser,L.A.Gavin,K.R.Feingold,Effect of tumor necrosis factor administration in vivo on lipoprotein lipase activity in various tissues of the rat,J.Lipid Res.30(1989)579–585.
[156] A.G.Mackay,J.D.Oliver,M.P.Rogers,Regulation of lipoprotein lipase activity and mRNA content in rat epididymal adipose tissue in vitro by recombinant tumour necrosis factor,Biochem.J.269(1990)123–126.
[157]H.Semb,J.Peterson,J.Tavernier,T.Olivecrona,Multiple effects of tumor necrosis factor on lipoprotein lipase in vivo,J.Biol.Chem.262(1987)8390–8394. [158]R.Zechner,T.C.Newman,B.Sherry,A.Cerami,J.L.Breslow,Recombinant human cachectin/tumor necrosis factor but not interleukin-1alpha downregulates lipoprotein lipase gene expression at the transcriptional level in mouse3T3-L1 adipocytes,Mol.Cell.Biol.8(1988)2394–2401.
[159]G.Wu,P.Brouckaert,T.Olivecrona,Rapid downregulation of adipose tissue lipoprotein lipase activity on food deprivation:evidence that TNF-alpha is involved,Am.J.Physiol.Endocrinol.Metab.286(2004)E711–E717.
[160]S.Dutton,P.Trayhurn,Regulation of angiopoietin-like protein4/fasting-induced adipose factor(Angptl4/FIAF)expression in mouse white adipose tissue and 3T3-L1adipocytes,Br.J.Nutr.100(2008)18–26.
[161] E.F.Coyle,Physical activity as a metabolic stressor,Am.J.Clin.Nutr.72(2000) 512S–520S.
[162]V.B.Schrauwen-Hinderling,M.K.Hesselink,P.Schrauwen,M.E.Kooi, Intramyocellular lipid content in human skeletal muscle,Obesity(Silver Spring) 14(2006)357–367.
930S.Kersten/Biochimica et Biophysica Acta1841(2014)919–933
[163]https://www.360docs.net/doc/415523865.html,es,Y.S.Park,D.Walewicz,C.Russell-Lopez,S.Windsor,W.L.Isley,S.W.
Coppack,W.S.Harris,Systemic and forearm triglyceride metabolism:fate of lipo-protein lipase-generated glycerol and free fatty acids,Diabetes53(2004)521–527. [164]M.T.Hamilton,J.Etienne,W.C.McClure,B.S.Pavey,A.K.Holloway,Role of local contractile activity and muscle?ber type on LPL regulation during exercise,Am.
J.Physiol.275(1998)E1016–E1022.
[165]L.B.Oscai,R.W.Tsika,D.A.Essig,Exercise training has a heparin-like effect on lipo-protein lipase activity in muscle,Can.J.Physiol.Pharmacol.70(1992)905–909. [166] D.W.Quig,https://www.360docs.net/doc/415523865.html,yman,P.J.Bechtel,L.R.Hackler,The in?uence of starvation and refeeding on the lipoprotein lipase activity of skeletal muscle and adipose tissue of lean and obese Zucker rats,J.Nutr.113(1983)1150–1156.
[167]S.Samec,J.Seydoux,A.P.Russell,J.P.Montani,A.G.Dulloo,Skeletal muscle hetero-geneity in fasting-induced upregulation of genes encoding UCP2,UCP3, PPARgamma and key enzymes of lipid oxidation,P?ugers Arch.—Eur.J.Physiol.
445(2002)80–86.
[168]T.B.Hakvoort,P.D.Moerland,R.Frijters, A.Sokolovic,https://www.360docs.net/doc/415523865.html,bruyere,J.L.
Vermeulen,E.Ver Loren van Themaat,T.M.Breit,F.R.Wittink,A.H.van Kampen,
A.J.Verhoeven,https://www.360docs.net/doc/415523865.html,mers,M.Sokolovic,Interorgan coordination of the murine
adaptive response to fasting,J.Biol.Chem.286(2011)16332–16343.
[169]H.Pilegaard,B.Saltin,P.D.Neufer,Effect of short-term fasting and refeeding on transcriptional regulation of metabolic genes in human skeletal muscle,Diabetes 52(2003)657–662.
[170] A.L.Hildebrandt,P.D.Neufer,Exercise attenuates the fasting-induced transcrip-tional activation of metabolic genes in skeletal muscle,Am.J.Physiol.Endocrinol.
Metab.278(2000)E1078–E1086.
[171]Y.Kamei,J.Mizukami,S.Miura,M.Suzuki,N.Takahashi,T.Kawada,T.Taniguchi,O.
Ezaki,A forkhead transcription factor FKHR up-regulates lipoprotein lipase expres-sion in skeletal muscle,FEBS Lett.536(2003)232–236.
[172]P.J.Davies,S.A.Berry,G.L.Shipley,R.H.Eckel,N.Hennuyer,D.L.Crombie,K.M.
Ogilvie,J.Peinado-Onsurbe,C.Fievet,M.D.Leibowitz,R.A.Heyman,J.Auwerx, Metabolic effects of rexinoids:tissue-speci?c regulation of lipoprotein lipase activity,Mol.Pharmacol.59(2001)170–176.
[173]M.R.Robciuc,P.Skrobuk,A.Anisimov,V.M.Olkkonen,K.Alitalo,R.H.Eckel,H.A.
Koistinen,M.Jauhiainen,C.Ehnholm,Angiopoietin-like4mediates PPAR delta effect on lipoprotein lipase-dependent fatty acid uptake but not on beta-oxidation in myotubes,PLoS One7(2012)e46212.
[174]J.S.Greiwe,J.O.Holloszy,C.F.Semenkovich,Exercise induces lipoprotein lipase and GLUT-4protein in muscle independent of adrenergic-receptor signaling,J.Appl.
Physiol.89(2000)176–181.
[175]L.B.Oscai,R.A.Caruso,A.C.Wergeles,Lipoprotein lipase hydrolyzes endogenous triacylglycerols in muscle of exercised rats,J.Appl.Physiol.52(1982)1059–1063. [176]H.Pilegaard,C.Keller,A.Steensberg,J.W.Helge,B.K.Pedersen,B.Saltin,P.D.
Neufer,In?uence of pre-exercise muscle glycogen content on exercise-induced transcriptional regulation of metabolic genes,J.Physiol.541(2002)261–271. [177]V.B.Schrauwen-Hinderling,M.K.Hesselink,E.Moonen-Kornips,G.Schaart,M.E.
Kooi,W.H.Saris,P.Schrauwen,Short-term training is accompanied by a down reg-ulation of ACC2mRNA in skeletal muscle,Int.J.Sports Med.27(2006)786–791. [178]R.L.Seip,K.Mair,T.G.Cole,C.F.Semenkovich,Induction of human skeletal muscle lipoprotein lipase gene expression by short-term exercise is transient,Am.J.
Physiol.272(1997)E255–E261.
[179]K.Vissing,J.L.Andersen,P.Schjerling,Are exercise-induced genes induced by exercise?FASEB J.19(2005)94–96.
[180]S.B.Pedersen,J.F.Bak,P.Holck,O.Schmitz,B.Richelsen,Epinephrine stimulates human muscle lipoprotein lipase activity in vivo,Metabolism48(1999)461–464. [181]Y.Deshaies,A.Geloen,A.Paulin,A.Marette,L.J.Bukowiecki,Tissue-speci?c alter-ations in lipoprotein lipase activity in the rat after chronic infusion of isoproterenol, Horm.Metab.Res.(Hormon-und Stoffwechselforschung=Hormones et metabolisme),25(1993)13–16.
[182] E.P.Plaisance,P.W.Grandjean,A.J.Mahurin,Independent and combined effects of aerobic exercise and pharmacological strategies on serum triglyceride concentra-tions:a qualitative review,Phys.Sportsmed.37(2009)11–19.
[183]J.M.Ong,R.B.Simsolo,M.Saghizadeh,J.W.Goers,P.A.Kern,Effects of exercise training and feeding on lipoprotein lipase gene expression in adipose tissue, heart,and skeletal muscle of the rat,Metabolism44(1995)1596–1605. [184]H.Lithell,J.Orlander,R.Schele,B.Sjodin,J.Karlsson,Changes in lipoprotein-lipase activity and lipid stores in human skeletal muscle with prolonged heavy exercise, Acta Physiol.Scand.107(1979)257–261.
[185] E.A.Nikkila,M.R.Taskinen,S.Rehunen,M.Harkonen,Lipoprotein lipase activity in adipose tissue and skeletal muscle of runners:relation to serum lipoproteins, Metabolism27(1978)1661–1667.
[186]M.R.Taskinen,E.A.Nikkila,Effect of acute vigorous exercise on lipoprotein lipase activity of adipose tissue and skeletal muscle in physically active men,Artery6 (1980)471–483.
[187]L.Bey,M.T.Hamilton,Suppression of skeletal muscle lipoprotein lipase activity during physical inactivity:a molecular reason to maintain daily low-intensity activity,J.Physiol.551(2003)673–682.
[188]R.B.Simsolo,J.M.Ong,P.A.Kern,The regulation of adipose tissue and muscle lipoprotein lipase in runners by detraining,J.Clin.Invest.92(1993)2124–2130. [189]K.Abozguia,K.Clarke,L.Lee,M.Frenneaux,Modi?cation of myocardial substrate use as a therapy for heart failure,Nat.Clin.Pract.Cardiovasc.Med.3(2006)490–498. [190]W.C.Stanley,F.A.Recchia,G.D.Lopaschuk,Myocardial substrate metabolism in the normal and failing heart,Physiol.Rev.85(2005)1093–1129.
[191]T.S.Park,H.Yamashita,W.S.Blaner,I.J.Goldberg,Lipids in the heart:a source of fuel and a source of toxins,Curr.Opin.Lipidol.18(2007)277–282.
[192] A.S.Augustus,J.Buchanan,T.S.Park,K.Hirata,H.L.Noh,J.Sun,S.Homma,J.
D'Armiento,E.D.Abel,I.J.Goldberg,Loss of lipoprotein lipase-derived fatty acids
leads to increased cardiac glucose metabolism and heart dysfunction,J.Biol.
Chem.281(2006)8716–8723.
[193]J.Borensztajn,D.S.Robinson,The effect of fasting on the utilization of chylomicron triglyceride fatty acids in relation to clearing factor lipase(lipoprotein lipase) releasable by heparin in the perfused rat heart,J.Lipid Res.11(1970)111–117. [194] C.H.Hollenberg,The effect of fasting on the lipoprotein lipase activity of rat heart and diaphragm,J.Clin.Invest.39(1960)1282–1287.
[195]M.Kuwajima,D.W.Foster,J.D.McGarry,Regulation of lipoprotein lipase in differ-ent rat tissues,Metabolism37(1988)597–601.
[196]T.Ruge,M.Bergo,M.Hultin,G.Olivecrona,T.Olivecrona,Nutritional regulation of binding sites for lipoprotein lipase in rat heart,Am.J.Physiol.Endocrinol.Metab.
278(2000)E211–E218.
[197]T.Ruge,G.Wu,T.Olivecrona,G.Olivecrona,Nutritional regulation of lipoprotein lipase in mice,Int.J.Biochem.Cell Biol.36(2004)320–329.
[198]N.Sambandam,M.A.Abrahani,E.St Pierre,O.Al-Atar,M.C.Cam,B.Rodrigues, Localization of lipoprotein lipase in the diabetic heart:regulation by acute changes in insulin,Arterioscler.Thromb.Vasc.Biol.19(1999)1526–1534.
[199] D.An,T.Pulinilkunnil,D.Qi,S.Ghosh,A.Abrahani,B.Rodrigues,The metabolic “switch”AMPK regulates cardiac heparin-releasable lipoprotein lipase,Am.J.
Physiol.Endocrinol.Metab.288(2005)E246–E253.
[200]G.Liu,T.Olivecrona,Synthesis and transport of lipoprotein lipase in perfused guinea pig hearts,Am.J.Physiol.263(1992)H438–H446.
[201]G.Wu,L.Zhang,J.Gupta,G.Olivecrona,T.Olivecrona,A transcription-dependent mechanism,akin to that in adipose tissue,modulates lipoprotein lipase activity in rat heart,Am.J.Physiol.Endocrinol.Metab.293(2007)E908–E915.
[202]R.Carroll,D.L.Severson,Peroxisome proliferator-activated receptor-alpha ligands inhibit cardiac lipoprotein lipase activity,Am.J.Physiol.Heart Circ.Physiol.281 (2001)H888–H894.
[203] D.Hauton,Does long-term metformin treatment increase cardiac lipoprotein lipase?Metabolism60(2011)32–42.
[204]M.P.Rogers,D.S.Robinson,Effects of cold exposure on heart clearing factor lipase and triglyceride utilization in the rat,J.Lipid Res.15(1974)263–272.
[205]Y.Cheng,D.Hauton,Cold acclimation induces physiological cardiac hypertrophy and increases assimilation of triacylglycerol metabolism through lipoprotein lipase,Biochim.Biophys.Acta1781(2008)618–626.
[206] B.Cannon,J.Nedergaard,Brown adipose tissue:function and physiological signif-icance,Physiol.Rev.84(2004)277–359.
[207] A.M.Cypess,S.Lehman,G.Williams,I.Tal,D.Rodman,A.B.Gold?ne,F.C.Kuo,E.L.
Palmer,Y.H.Tseng,A.Doria,G.M.Kolodny,C.R.Kahn,Identi?cation and importance of brown adipose tissue in adult humans,N.Engl.J.Med.360(2009)1509–1517. [208]M.Saito,Y.Okamatsu-Ogura,M.Matsushita,K.Watanabe,T.Yoneshiro,J.Nio-Kobayashi,T.Iwanaga,M.Miyagawa,T.Kameya,K.Nakada,Y.Kawai,M.Tsujisaki, High incidence of metabolically active brown adipose tissue in healthy adult humans: effects of cold exposure and adiposity,Diabetes58(2009)1526–1531.
[209]W.D.van Marken Lichtenbelt,J.W.Vanhommerig,N.M.Smulders,J.M.Drossaerts,
G.J.Kemerink,N.D.Bouvy,P.Schrauwen,G.J.Teule,Cold-activated brown adipose
tissue in healthy men,N.Engl.J.Med.360(2009)1500–1508.
[210]K.A.Virtanen,M.E.Lidell,J.Orava,M.Heglind,R.Westergren,T.Niemi,M.
Taittonen,https://www.360docs.net/doc/415523865.html,ine,N.J.Savisto,S.Enerback,P.Nuutila,Functional brown adipose tissue in healthy adults,N.Engl.J.Med.360(2009)1518–1525.
[211] A.Bartelt,C.Weigelt,M.L.Cherradi,A.Niemeier,K.Todter,J.Heeren,L.Scheja, Effects of adipocyte lipoprotein lipase on de novo lipogenesis and white adipose tissue browning,Biochim.Biophys.Acta1831(2013)934–942.
[212]I.Garcia-Arcos,Y.Hiyama,K.Drosatos,K.G.Bharadwaj,Y.Hu,N.Huiping Son,S.M.
O'Byrne,C.L.Chang,R.J.Deckelbaum,M.Takahashi,M.Westerterp,J.C.Obunike,H.
Jiang,H.Yagyu,W.S.Blaner,I.J.Goldberg,Adipose speci?c lipoprotein lipase de?-ciency more profoundly affects brown than white fat biology,J.Biol.Chem.288 (2013)14046–14058.
[213]M.Goubern,https://www.360docs.net/doc/415523865.html,ury,L.Zizine,R.Portet,Effect of temperature on lipoprotein lipase and lipogenic enzyme activities in brown adipose tissue of hypophysectomized rats, Horm.Metab.Res.(Hormon-und Stoffwechselforschung=Hormones et metabolisme), 17(1985)176–180.
[214]L.Mantha,Y.Deshaies,Beta-adrenergic modulation of triglyceridemia under increased energy expenditure,Am.J.Physiol.274(1998)R1769–R1776. [215] A.Bartelt,O.T.Bruns,R.Reimer,H.Hohenberg,H.Ittrich,K.Peldschus,M.G.Kaul,U.
I.Tromsdorf,H.Weller,C.Waurisch,A.Eychmuller,P.L.Gordts,F.Rinninger,K.
Bruegelmann,B.Freund,P.Nielsen,M.Merkel,J.Heeren,Brown adipose tissue activity controls triglyceride clearance,Nat.Med.17(2011)200–205.
[216]R.Bertin,M.Triconnet,R.Portet,Effects of cold acclimation on the activity of lipo-protein lipase in adipose tissues of genetically obese Zucker rats,Comp.Biochem.
Physiol.B81(1985)797–801.
[217] C.Carneheim,J.Nedergaard,B.Cannon,Beta-adrenergic stimulation of lipoprotein lipase in rat brown adipose tissue during acclimation to cold,Am.J.Physiol.246 (1984)E327–E333.
[218]M.W.Radomski,T.Orme,Response of lipoprotein lipase in various tissues to cold exposure,Am.J.Physiol.220(1971)1852–1856.
[219] C.Carneheim,J.Nedergaard,B.Cannon,Cold-induced beta-adrenergic recruitment of lipoprotein lipase in brown fat is due to increased transcription,Am.J.Physiol.
254(1988)E155–E161.
[220]M.Klingenspor,C.Ebbinghaus,G.Hulshorst,S.Stohr,F.Spiegelhalter,K.Haas,G.
Heldmaier,Multiple regulatory steps are involved in the control of lipoprotein lipase activity in brown adipose tissue,J.Lipid Res.37(1996)1685–1695. [221]P.Kuusela,A.Jacobsson,M.Klingenspor,S.Rehnmark,G.Heldmaier,B.Cannon,J.
Nedergaard,Contrasting adrenergic effects on lipoprotein lipase gene expression in the brown adipose tissue of intact mice and in cultured brown adipocytes from mice,Biochim.Biophys.Acta1345(1997)327–337.
931
S.Kersten/Biochimica et Biophysica Acta1841(2014)919–933
[222]P.Kuusela,S.Rehnmark,A.Jacobsson,B.Cannon,J.Nedergaard,Adrenergic stimu-lation of lipoprotein lipase gene expression in rat brown adipocytes differentiated in culture:mediation via beta3-and alpha1-adrenergic receptors,Biochem.J.321 (Pt3)(1997)759–767.
[223]M.Klingenspor,S.Klaus,H.Wiesinger,G.Heldmaier,Short photoperiod and cold activate brown fat lipoprotein lipase in the Djungarian hamster,Am.J.Physiol.
257(1989)R1123–R1127.
[224]R.J.Reiter,S.Klaus,C.Ebbinghaus,G.Heldmaier,U.Redlin,D.Ricquier,M.K.
Vaughan,S.Steinlechner,Inhibition of5′-deiodination of thyroxine suppresses the cold-induced increase in brown adipose tissue messenger ribonucleic acid for mitochondrial uncoupling protein without in?uencing lipoprotein lipase activity,Endocrinology126(1990)2550–2554.
[225]J.M.Hamilton,T.J.Bartness,G.N.Wade,Effects of norepinephrine and denervation on brown adipose tissue in Syrian hamsters,Am.J.Physiol.257(1989)R396–R404. [226]https://www.360docs.net/doc/415523865.html,plante,H.Sell,K.L.MacNaul,D.Richard,J.P.Berger,Y.Deshaies,PPAR-gamma activation mediates adipose depot-speci?c effects on gene expression and lipopro-tein lipase activity:mechanisms for modulation of postprandial lipemia and differential adipose accretion,Diabetes52(2003)291–299.
[227]https://www.360docs.net/doc/415523865.html,plante,W.T.Festuccia,G.Soucy,P.G.Blanchard,A.Renaud,J.P.Berger,G.
Olivecrona,Y.Deshaies,Tissue-speci?c postprandial clearance is the major determinant of PPARgamma-induced triglyceride lowering in the rat,Am.J.
https://www.360docs.net/doc/415523865.html,p.Physiol.296(2009)R57–R66.
[228]https://www.360docs.net/doc/415523865.html,plante,W.T.Festuccia,G.Soucy,Y.Gelinas,https://www.360docs.net/doc/415523865.html,londe,Y.Deshaies,Involve-ment of adipose tissues in the early hypolipidemic action of PPARgamma agonism in the rat,https://www.360docs.net/doc/415523865.html,p.Physiol.292(2007)R1408–R1417. [229]T.Teruel,R.Hernandez,E.Rial,A.Martin-Hidalgo,M.Lorenzo,Rosiglitazone up-regulates lipoprotein lipase,hormone-sensitive lipase and uncoupling protein-1,and down-regulates insulin-induced fatty acid synthase gene expres-sion in brown adipocytes of Wistar rats,Diabetologia48(2005)1180–1188. [230]S.K.Fried,J.O.Hill,M.Nickel,M.DiGirolamo,Novel regulation of lipoprotein lipase activity in rat brown adipose tissue:effects of fasting and caloric restriction during refeeding,J.Nutr.113(1983)1870–1874.
[231]M.Goubern,R.Portet,Circadian rhythm and hormonal sensitivity of lipoprotein lipase activity in cold acclimated rats,Horm.Metab.Res.(Hormon-und Stoffwechselforschung=Hormones et metabolisme),13(1981)73–77. [232] A.Chait,P.H.Iverius,J.D.Brunzell,Lipoprotein lipase secretion by human monocyte-derived macrophages,J.Clin.Invest.69(1982)490–493.
[233] A.J.Day,Oxidation of14C-labelled chylomicron fat and14C-labelled unesteri?ed fatty acids by macrophages in vitro and the effect of clearing factor,Q.J.Exp.
Physiol.Cogn.Med.Sci.45(1960)220–228.
[234] A.J.Day,P.M.Harris,Esterolytic and lipolytic-enzymes of macrophages,Q.J.Exp.
Physiol.Cogn.Med.Sci.45(1960)213–219.
[235] E.M.Mahoney,J.C.Khoo,D.Steinberg,Lipoprotein lipase secretion by human monocytes and rabbit alveolar macrophages in culture,Proc.Natl.Acad.Sci.U.S.
A.79(1982)1639–1642.
[236] A.J.Evans,C.G.Sawyez,B.M.Wolfe,P.W.Connelly,G.F.Maguire,M.W.Huff,Evi-dence that cholesteryl ester and triglyceride accumulation in J774macrophages induced by very low density lipoprotein subfractions occurs by different mecha-nisms,J.Lipid Res.34(1993)703–717.
[237]S.Yla-Herttuala,B.A.Lipton,M.E.Rosenfeld,I.J.Goldberg,D.Steinberg,J.L.Witztum, Macrophages and smooth muscle cells express lipoprotein lipase in human and rabbit atherosclerotic lesions,Proc.Natl.Acad.Sci.U.S.A.88(1991)10143–10147. [238]K.D.O'Brien,D.Gordon,S.Deeb,M.Ferguson,A.Chait,Lipoprotein lipase is synthe-sized by macrophage-derived foam cells in human coronary atherosclerotic plaques,J.Clin.Invest.89(1992)1544–1550.
[239]W.L.Hendriks,H.van der Boom,L.C.van Vark,L.M.Havekes,Lipoprotein lipase stimulates the binding and uptake of moderately oxidized low-density lipoprotein by J774macrophages,Biochem.J.314(Pt2)(1996)563–568.
[240]S.I.Skarlatos,H.L.Dichek,S.S.Fojo,H.B.Brewer,H.S.Kruth,Absence of triglyceride accumulation in lipoprotein lipase-de?cient human monocyte-macrophages incu-bated with human very low density lipoprotein,J.Clin.Endocrinol.Metab.76 (1993)793–796.
[241]V.R.Babaev,S.Fazio,L.A.Gleaves,K.J.Carter,C.F.Semenkovich,M.F.Linton,Macro-phage lipoprotein lipase promotes foam cell formation and atherosclerosis in vivo, J.Clin.Invest.103(1999)1697–1705.
[242]V.R.Babaev,M.B.Patel,C.F.Semenkovich,S.Fazio,M.F.Linton,Macrophage lipo-protein lipase promotes foam cell formation and atherosclerosis in low density lipoprotein receptor-de?cient mice,J.Biol.Chem.275(2000)26293–26299. [243]T.Ichikawa,J.Liang,S.Kitajima,T.Koike,X.Wang,H.Sun,M.Morimoto,H.Shikama,T.
Watanabe,N.Yamada,J.Fan,Macrophage-derived lipoprotein lipase increases aortic atherosclerosis in cholesterol-fed Tg rabbits,Atherosclerosis179(2005)87–95. [244]K.Wilson,G.L.Fry,D.A.Chappell,C.D.Sigmund,J.D.Medh,Macrophage-speci?c expression of human lipoprotein lipase accelerates atherosclerosis in transgenic apolipoprotein e knockout mice but not in C57BL/6mice,Arterioscler.Thromb.
Vasc.Biol.21(2001)1809–1815.
[245]M.Takahashi,H.Yagyu,F.Tazoe,S.Nagashima,T.Ohshiro,K.Okada,J.Osuga,I.J.
Goldberg,S.Ishibashi,Macrophage lipoprotein lipase modulates the development of atherosclerosis but not adiposity,J.Lipid Res.54(2013)1124–1134.
[246]M.Van Eck,R.Zimmermann,P.H.Groot,R.Zechner,T.J.Van Berkel,Role of macrophage-derived lipoprotein lipase in lipoprotein metabolism and atheroscle-rosis,Arterioscler.Thromb.Vasc.Biol.20(2000)E53–E62.
[247] B.Yin,J.D.Loike,Y.Kako,P.H.Weinstock,J.L.Breslow,S.C.Silverstein,I.J.Goldberg, Lipoprotein lipase regulates Fc receptor-mediated phagocytosis by macrophages maintained in glucose-de?cient medium,J.Clin.Invest.100(1997)649–657. [248]R.Goldman,O.Sopher,Control of lipoprotein lipase secretion in mouse macro-phages,Biochim.Biophys.Acta1001(1989)120–126.[249]S.R.Behr,F.B.Kraemer,Effects of activation on lipoprotein lipase secretion by macrophages.Evidence for autoregulation,J.Exp.Med.164(1986)1362–1367. [250] D.I.Goldberg,J.C.Khoo,Regulation of lipoprotein lipase secretion by mouse perito-neal macrophages,https://www.360docs.net/doc/415523865.html,mun.142(1987)1–6.
[251]M.R.Hill,K.Kelly,X.Wu,F.Wanker,H.Bass,C.Morgan,C.Wang,J.M.Gimble, Lipopolysaccharide regulation of lipoprotein lipase expression in murine macro-phages,Infect.Immun.63(1995)858–864.
[252]O.Sopher,R.Goldman,Bacterial lipopolysaccharide suppresses the expression of lipoprotein lipase in murine macrophages:a process independent of tumor necrosis factor or interleukin1,Immunol.Lett.15(1987)261–265.
[253]J.R.White,A.Chait,S.J.Klebanoff,S.Deeb,J.D.Brunzell,Bacterial lipopolysaccharide reduces macrophage lipoprotein lipase levels:an effect that is independent of tumor necrosis factor,J.Lipid Res.29(1988)1379–1385.
[254]T.S.Tengku-Muhammad,T.R.Hughes,A.Cryer,D.P.Ramji,Differential regulation of lipoprotein lipase in the macrophage J774.2cell line by cytokines,Cytokine8 (1996)525–533.
[255]G.Friedman,T.Chajek-Shaul,R.Gallily,O.Stein,E.Shiloni,J.Etienne,Y.Stein,Mod-ulation of lipoprotein lipase activity in mouse peritoneal macrophages by recombi-nant human tumor necrosis factor,Biochim.Biophys.Acta963(1988)201–207. [256]S.M.Harris,E.J.Harvey,T.R.Hughes,D.P.Ramji,The interferon-gamma-mediated inhibition of lipoprotein lipase gene transcription in macrophages involves casein kinase2-and phosphoinositide-3-kinase-mediated regulation of transcription factors Sp1and Sp3,Cell.Signal.20(2008)2296–2301.
[257]L.Jonasson,G.K.Hansson,G.Bondjers,L.Noe,J.Etienne,Interferon-gamma inhibits lipoprotein lipase in human monocyte-derived macrophages,Biochim.Biophys.
Acta1053(1990)43–48.
[258]S.A.Irvine,P.Foka,S.A.Rogers,J.R.Mead,D.P.Ramji,A critical role for the Sp1-binding sites in the transforming growth factor-beta-mediated inhibition of lipoprotein lipase gene expression in macrophages,Nucleic Acids Res.33(2005) 1423–1434.
[259]T.Inaba,M.Kawamura,T.Gotoda,K.Harada,M.Shimada,J.Ohsuga,H.Shimano,Y.
Akanuma,Y.Yazaki,N.Yamada,Effects of platelet-derived growth factor on the synthesis of lipoprotein lipase in human monocyte-derived macrophages, Arterioscler.Thromb.Vasc.Biol.15(1995)522–528.
[260] F.Maingrette,L.Li,G.Renier,C-reactive protein enhances macrophage lipoprotein lipase expression,J.Lipid Res.49(2008)1926–1935.
[261]N.Mori,T.Gotoda,S.Ishibashi,H.Shimano,K.Harada,T.Inaba,F.Takaku,Y.Yazaki, N.Yamada,Effects of human recombinant macrophage colony-stimulating factor on the secretion of lipoprotein lipase from macrophages,Arterioscler.Thromb.
11(1991)1315–1321.
[262]G.Renier,A.C.Desfaits,https://www.360docs.net/doc/415523865.html,mbert,R.Mikhail,Role of oxidant injury on macro-phage lipoprotein lipase(LPL)production and sensitivity to LPL,J.Lipid Res.37 (1996)799–809.
[263]S.R.Behr,F.B.Kraemer,Regulation of the secretion of lipoprotein lipase by mouse macrophages,Biochim.Biophys.Acta889(1986)346–354.
[264]R.Goldman,Control of lipoprotein lipase secretion by macrophages:effect of macrophage differentiation agents,J.Leukoc.Biol.47(1990)79–86.
[265]M.Kawakami,T.Murase,S.Ishibashi,N.Mori,F.Takaku,Lipoprotein lipase in mouse peritoneal macrophages:the effects of insulin and dexamethasone,J.
Biochem.100(1986)1373–1378.
[266]W.S.Domin,A.Chait,S.S.Deeb,Transcriptional activation of the lipoprotein lipase gene in macrophages by dexamethasone,Biochemistry30(1991)2570–2574. [267] F.G.Gbaguidi,G.Chinetti,https://www.360docs.net/doc/415523865.html,osavljevic,E.Teissier,J.Chapman,G.Olivecrona,J.C.
Fruchart,S.Griglio,J.Fruchart-Najib,B.Staels,Peroxisome proliferator-activated receptor(PPAR)agonists decrease lipoprotein lipase secretion and glycated LDL uptake by human macrophages,FEBS Lett.512(2002)85–90.
[268]L.Li,M.C.Beauchamp,G.Renier,Peroxisome proliferator-activated receptor alpha and gamma agonists upregulate human macrophage lipoprotein lipase expression, Atherosclerosis165(2002)101–110.
[269]L.A.Bojic,C.G.Sawyez,D.E.Telford,J.Y.Edwards,R.A.Hegele,M.W.Huff,Activation of peroxisome proliferator-activated receptor delta inhibits human macrophage foam cell formation and the in?ammatory response induced by very low-density lipoprotein,Arterioscler.Thromb.Vasc.Biol.32(2012)2919–2928.
[270] E.Makoveichuk,V.Sukonina,O.Kroupa,P.Thulin,E.Ehrenborg,T.Olivecrona,G.
Olivecrona,Inactivation of lipoprotein lipase occurs on the surface of THP-1macro-phages where oligomers of angiopoietin-like protein4are formed,Biochem.
https://www.360docs.net/doc/415523865.html,mun.425(2012)138–143.
[271]O.Sofer,M.Fainaru,Z.Schafer,R.Goldman,Regulation of lipoprotein lipase secre-tion in murine macrophages during foam cell formation in vitro.Effect of triglyceride-rich lipoproteins,Arterioscler.Thromb.12(1992)1458–1466. [272]Y.Zhang,J.J.Repa,K.Gauthier,D.J.Mangelsdorf,Regulation of lipoprotein lipase by the oxysterol receptors,LXRalpha and LXRbeta,J.Biol.Chem.276(2001) 43018–43024.
[273]L.M.Hulten,H.Lindmark,U.Diczfalusy,I.Bjorkhem,M.Ottosson,Y.Liu,G.
Bondjers,O.Wiklund,Oxysterols present in atherosclerotic tissue decrease the expression of lipoprotein lipase messenger RNA in human monocyte-derived macrophages,J.Clin.Invest.97(1996)461–468.
[274] D.Stengel,M.Antonucci,W.Gaoua,C.Dachet,P.Lesnik,D.Hourton,E.Ninio,M.J.
Chapman,S.Griglio,Inhibition of LPL expression in human monocyte-derived macro-phages is dependent on LDL oxidation state:a key role for lysophosphatidylcholine, Arterioscler.Thromb.Vasc.Biol.18(1998)1172–1180.
[275]J.Gardette,D.Margelin,J.C.Maziere,J.Bertrand,J.Picard,Effect of dibutyryl cyclic AMP and theophylline on lipoprotein lipase secretion by human monocyte-derived macrophages,FEBS Lett.225(1987)178–182.
[276]R.N.Melmed,G.Friedman,T.Chajek-Shaul,O.Stein,Y.Stein,Lipoprotein lipase activity in cultured macrophage cell line J774(2)and its increase in variants
932S.Kersten/Biochimica et Biophysica Acta1841(2014)919–933
de?cient in adenylate cyclase and cyclic AMP-dependent protein kinase,Biochim.
Biophys.Acta762(1983)58–66.
[277]M.R.Sartippour,https://www.360docs.net/doc/415523865.html,mbert,https://www.360docs.net/doc/415523865.html,framboise,P.St-Jacques,G.Renier,Stimulatory effect of glucose on macrophage lipoprotein lipase expression and production, Diabetes47(1998)431–438.
[278]S.R.Behr,F.B.Kraemer,Insulin de?ciency decreases lipoprotein lipase secretion by murine macrophages,Diabetes37(1988)1076–1081.
[279]H.Podgornik,M.Sok,I.Kern,J.Marc,D.Cerne,Lipoprotein lipase in non-small cell lung cancer tissue is highly expressed in a subpopulation of tumor-associated macrophages,Pathol.Res.Pract.209(2013)516–520.
[280]Z.Trost,M.Sok,J.Marc,D.Cerne,Increased lipoprotein lipase activity in non-small cell lung cancer tissue predicts shorter patient survival,Arch.Med.Res.40(2009) 364–368.
[281]T.Ruge,L.Neuger,V.Sukonina,G.Wu,S.Barath,J.Gupta,B.Frankel,B.Christophersen, K.Nordstoga,T.Olivecrona,G.Olivecrona,Lipoprotein lipase in the kidney:activity varies widely among animal species,Am.J.Physiol.Renal Physiol.287(2004) F1131–F1139.
[282]S.A.Irvine,J.Martin,T.R.Hughes,D.P.Ramji,Lipoprotein lipase is expressed by glomerular mesangial cells,Int.J.Biochem.Cell Biol.38(2006)12–16.
[283]J.Li,H.Li,Y.B.Wen,X.W.Li,Very-low-density lipoprotein-induced triglyceride accumulation in human mesangial cells is mainly mediated by lipoprotein lipase, Nephron.Physiology110(2008)1–10.
[284]L.Camps,M.Reina,M.Llobera,S.Vilaro,T.Olivecrona,Lipoprotein lipase:cellular origin and functional distribution,Am.J.Physiol.258(1990)C673–C681. [285] D.R.Jensen,D.H.Bessesen,J.Etienne,R.H.Eckel,M.C.Neville,Distribution and source of lipoprotein lipase in mouse mammary gland,J.Lipid Res.32(1991) 733–742.
[286]P.Ramos,A.Martin-Hidalgo,E.Herrera,Insulin-induced up-regulation of lipopro-tein lipase messenger ribonucleic acid and activity in mammary gland,Endocrinol-ogy140(1999)1089–1093.
[287] D.R.Jensen,S.Gavigan,V.Sawicki,D.L.Witsell,R.H.Eckel,M.C.Neville,Regulation of lipoprotein lipase activity and mRNA in the mammary gland of the lactating mouse,Biochem.J.298(Pt2)(1994)321–327.[288]P.Lopez-Luna,J.Olea,E.Herrera,Effect of starvation on lipoprotein lipase activity in different tissues during gestation in the rat,Biochim.Biophys.Acta1215 (1994)275–279.
[289]K.M.Ostrom,A review of the hormone prolactin during lactation,Prog.Food Nutr.
Sci.14(1990)1–43.
[290]J.Hang,J.A.Rillema,Prolactin's effects on lipoprotein lipase(LPL)activity and on LPL mRNA levels in cultured mouse mammary gland explants,Proc.Soc.Exp.
Biol.Med.214(1997)161–166.
[291]T.H.Da Costa,D.H.Williamson,Regulation of rat mammary-gland uptake of orally administered[1-14C]triolein by insulin and prolactin:evidence for bihormonal control of lipoprotein lipase activity,Biochem.J.300(Pt1)(1994)257–262. [292]H.Wang,R.H.Eckel,What are lipoproteins doing in the brain?Trends Endocrinol.
Metab.25(2014)8–14.
[293]R.H.Eckel,R.J.Robbins,Lipoprotein lipase is produced,regulated,and functional in rat brain,Proc.Natl.Acad.Sci.U.S.A.81(1984)7604–7607.
[294]I.J.Goldberg,D.R.Soprano,M.L.Wyatt,T.M.Vanni,T.G.Kirchgessner,M.C.Schotz, Localization of lipoprotein lipase mRNA in selected rat tissues,J.Lipid Res.30 (1989)1569–1577.
[295] D.H.Bessesen,C.L.Richards,J.Etienne,J.W.Goers,R.H.Eckel,Spinal cord of the rat contains more lipoprotein lipase than other brain regions,J.Lipid Res.34(1993) 229–238.
[296]H.Wang,G.Astarita,M.D.Taussig,K.G.Bharadwaj,N.V.DiPatrizio,K.A.Nave,D.
Piomelli,I.J.Goldberg,R.H.Eckel,De?ciency of lipoprotein lipase in neurons modi?es the regulation of energy balance and leads to obesity,Cell Metab.13 (2011)105–113.
[297]M.Abdelrahman,A.Sivarajah,C.Thiemermann,Bene?cial effects of PPAR-gamma ligands in ischemia–reperfusion injury,in?ammation and shock,Cardiovasc.Res.
65(2005)772–781.
[298]O.Ben-Zeev,M.H.Doolittle,N.Singh,C.H.Chang,M.C.Schotz,Synthesis and regu-lation of lipoprotein lipase in the hippocampus,J.Lipid Res.31(1990)1307–1313. [299]L.A.Gavin,R.R.Cavalieri,M.Moeller,F.A.McMahon,J.N.Castle,R.Gulli,Brain lipoprotein lipase is responsive to nutritional and hormonal modulation,Metabolism 36(1987)919–924.
933
S.Kersten/Biochimica et Biophysica Acta1841(2014)919–933
国家名英语缩写
AC => 上升岛, AD => 安道尔, AE => 阿拉伯联合酋长国, AF => 阿富汗, AG => 安提瓜和巴布达, AI => 安圭拉, AL => 阿尔巴尼亚, AM => 亚美尼亚, AN => 荷兰安的列斯群岛, AO => 安哥拉, AQ => 南极洲, AR => 阿根廷, AS => 美属萨摩亚群岛, AT => 奥地利, AU => 澳洲, AW => 阿卢巴, AZ => 阿塞拜疆, BA => 波士尼亚共和国和Herzegowina , BB => 巴巴多斯, BD => 孟加拉, BE => 比利时, BF => BurkinaFaso , BG => 保加利亚, BH => 巴林, BI => 布隆迪, BJ => 贝宁湾, BM => 百慕达群岛, BN => 汶莱, BO => 玻利维亚, BR => 巴西, BS => 巴哈马, BT => 不丹, BV => 布威岛, BW => 博茨瓦纳, BY => 白俄罗斯, BZ => 伯利兹, CA => 加拿大, CC => 椰子树(装以龙骨)岛, CD => 扎伊尔, CF => 中非共和国, CG => 刚果, CH => 瑞士, CI => 棚d Ivoire , CK => 科克群岛, CL => 智利, CM => 喀麦隆, CN => 中国, CO => 哥伦比亚, CR => 哥斯达黎加, CU => 古巴, CV => 维德岛, CX => 圣诞岛, CY => 赛普勒斯, CZ => 捷克, DE => 德国, DJ => 吉布地, DK => 丹麦, DM => 多米尼加共和国, DO => 多米尼加共和国, DZ => 阿尔及利亚, EC => 厄瓜多尔, EE => 爱沙尼亚, EG => 埃及, EH => 西撒哈拉, ER => 厄立特里安, ES => 西班牙, ET => 埃塞俄比亚, EU => 欧盟,
世界各国国旗图片+英文名称及缩写(珍藏版)
亚洲 6不丹王国全称:KingdOm of BhUtan 缩写:BHU 1阿富汗 全称:AfghaniStan 缩写:AFG 7文莱 5孟加拉共和国 全称:BrUnei DarUSSaIam 缩写:BRU 2亚美尼亚 全称:The RePUbliC Of Armenia 缩写:ARM 8缅甸 全称:The Union of Myanmar 缩写:MYA 3阿塞拜疆 全称:The RePUbIiC of AZerbaijan 缩写:AZE 9柬埔寨 全称:The KingdOm of CambOdia 缩写:CAM 4巴林 全称:The KingdOm of Bahrain 缩写:BRN 10 中国英文名称The People's RePUbIiC of China 缩写:CHN 全称:The People's RePUbIiC of BangIadeSh 缩写:BAN
11塞浦路斯 全称:The RePUbliC Of CyPrUS 缩写:CYP 16伊拉克 全称:The RePUbIiC of Iraq 缩写:IRQ 全称:DemOCratiC RePUbIiC of TimOr-LeSte 缩写:TLS 13印度 全称:The RePUbIiC Oflndia 缩写:IND 14印度尼西亚 全称:The RePUbIiC of IndOneSia 缩写:INA 19约旦 全称:The HaShemite KingdOm of JOrdan 缩写: JoR 15伊朗 全称:The ISIamiC RePUbIiC of Iran 缩写:IRI 20哈萨克斯坦 全称:The RePUbIiC of KaZakhStan 缩写: KAZ 12东帝汶 17 以色列The State of ISraeI 缩写:ISR 18日本 全称:JaPan缩写:JPN
各个国家的公司名称翻译及缩写
各个国家的公司名称翻译及缩写 SA系法语Societe Anonym、意大利语Societa Anonima和西班牙语Sociedad Anonima的简称,均译为“股份公司”。 交易国别是我国国际收支统计记录中一个重要的申报要素。在对交易对方和交易国别的核查过程中,我们发现很多国家和地区的收付款人(公司)名称中存在各式各样的表示企业类型的缩略语,比如:Co.Ltd、Corp.、S.A.、GmbH和BHD SDN等。经过调查,我们发现上述现象存在的主要原因主要是:(1)各国语言文字不同,同一词汇在不同的国家具有不同的称谓,比如,“公司”在英语里一般简称Co.、Inc.或Corp.,在瑞典语里的简称则是AB;(2)各国法律制度和使用习惯存在差异,导致一些国家和地区的企业名称比较独特,比如,新加坡法律规定私人企业名称中必须出现Pte.字样,而其他国家则绝少有此现象。由于有的缩略语为某个国家独有,有的则是同一语种国家和地区所共有。因此,我们能通过交易对方中的缩略语来排除掉或推定出某一笔国际收支交易的国别。 有鉴于此,我们以近两年全国部分地区的近20万条国际收支统计交易记录为索引,对我国主要贸易伙伴国的《公司法》、《投资法》进行了查阅和研究,梳理出许多在国际收支统计信息中出现频率较高、可用于国际收支交易国别判断的交易对方缩略语,现列举和剖译如下: 一、SDN BHD与马来西亚的关系 SDN系马来西亚语Sendirian的缩写,意即“私人”。BHD系Berhad的缩写,意为“公司”。 SDN BHD是指“私人有限公司”,单BHD一般指“公众有限公司”。 在马来西亚,企业一般注册为个人企业、合伙人企业或私人有限公司,其中以SDN BHD私人有限公司最为常见。如: CSP CORPORATION M ALAYSIABHD UNITED MS ELECTRICAL MFG(M) SDN BHD 除新加坡、文莱(马来语是两国正式语言)企业名称偶尔出现SDN BHD外,其他国家企业名称基本上没有SDN BHD字样,因此,如果交易对方中出现SDN BHD,而交易国别不是马来西亚,则基本上可认定交易国别有误。 二、GmbH是德语区国家除德国外还有奥地利、列支敦士登、瑞士、比利时和卢森堡 GmbH系德文Gesellschaft Mit Beschrankter Haftung的缩写,等于英文中的Limited liability company,即“有限责任公司”。 有限责任公司为介于大型股份公司与小型合伙企业之间的企业形态,为目前德国采用最为广泛的企业形式。如: B.TEAM EDV.EDITION B.BREIDENSTEIN GMBH(德国) MESSE FRANKFURT MEDJEN UND SERVICE GMBH(德国) 此类公司形式主要是德语区存在,除德国外,将德语作为母语之一的还有奥地利、列支敦士登、瑞士、比利时和卢森堡,上述国家企业名称中都有可能出现GmbH。如:Hutchison 3G Austria GmbH即为地址在维也纳的奥地利企业。 因此,绝不可将交易对方中出现GMBH的交易记录一概认为是德国的。 三、AG(德国和瑞士)、S.A.(南欧、南美)
全球世界国家名称及缩写代码
国家名称及缩写 Af -------Afghanistan 阿富汗 AL -------Albania 阿尔巴尼亚 DZ -------Algeria 阿尔及利亚 AS -------American Samoa 东萨摩亚 AD -------Andorra 安道尔 AO -------Angola 安哥拉 Av -------Anguilla 安圭拉岛 AQ -------Antarctica 南极洲 AG -------Antigua and Barbuda 安提瓜和巴布达AR -------Argentina 阿根廷 AM -------Armenia 亚美尼亚 AA -------Aruba 阿鲁巴 AU -------Australia 澳大利亚 AT -------Austria 奥地利 AZ -------Azerbaijan 阿塞拜疆 BH -------Bahrain 巴林 BB -------Barbados 巴巴多斯 BD -------Bangladesh 孟加拉 BY -------Belarus 白俄罗斯 BE -------Belgium 比利时
BZ -------Belize 伯里兹 BJ -------Benin 贝宁 BM -------Bermuda 百慕大 BS -------Bahamas 巴哈马 BF -------Bahamas 巴哈马 BT -------Bhutan 不丹 BW -------Botswana 博茨瓦纳 BO -------Bolivia 玻利维亚 BA -------Bosnia and Herzegovina 波黑 BV -------Bouvet Island 布韦岛 BR -------Brazil 巴西 IO -------British Indian Ocean Territory 英属印度洋领地BN -------Brunei Darussalam 文莱布鲁萨兰 BG -------Bulgaria 保加利亚 BF -------Burkina Faso 布基纳法索 BI -------Burundi 布隆迪 KH -------Cambodia (Internet) 柬埔寨 CB -------Cambodia (CIA World Fact Book) 柬埔寨CM -------Cameroon 喀麦隆 CA -------Canada 加拿大 CV -------Cape Verde 佛得角 KY -------Cayman Islands 开曼群岛
国家英文名称及缩写
亚洲: 1阿富汗英文全称:Afghanistan 英文缩写:AFG 2亚美尼亚英文全称:The Republic of Armenia英文缩写:ARM 3阿塞拜疆英文全称:The Republic of Azerbaijan英文缩写:AZE 4巴林英文全称:The Kingdom of Bahrain英文缩写:BRN 5孟加拉共和国The People's Republic of Bangladesh英文缩写:BAN 6不丹王国英文全称:Kingdom of Bhutan英文缩写:BHU 7文莱英文全称:Brunei Darussalam英文缩写:BRU 8缅甸英文全称:The Union of Myanmar英文缩写:MY A
9柬埔寨英文全称:The Kingdom of Cambodia英文缩写:CAM 10中国英文名称The People's Republic of China英文缩写CHN 11塞浦路斯The Republic of Cyprus英文缩写:CYP 12东帝汶英文全称:Democratic Republic of Timor-Leste英文缩写:TLS 13印度英文全称:The Republic of India英文缩写:IND 14印度尼西亚英文全称:The Republic of Indonesia英文缩写:INA 15伊朗英文全称:The Islamic Republic of Iran英文缩写:IRI
16伊拉克英文全称:The Republic of Iraq英文缩写:IRQ 17以色列The State of Israel英文缩写:ISR 18日本英文全称:Japan英文缩写:JPN 19约旦英文全称:The Hashemite Kingdom of Jordan英文缩写:JOR 20哈萨克斯坦英文全称:The Republic of Kazakhstan英文缩写:KAZ 21朝鲜英文全称:Democratic People's Republic of Korea英文缩写:PRK 22韩国英文全称:Republic of Korea英文缩写:KOR
世界各国国名中英文及其简写
序号缩写中文英文 1 AE 阿联酋United Arab Emirates 2 AF 阿富汗Afghanistan 3 AL 阿尔巴尼亚Albania 4 AO 安哥拉Angola 5 AR 阿根廷Argentina 6 AT 奥地利Austria 7 AU 澳大利亚Australia 8 AZ 阿塞拜疆Azerbaijan 9 BD 孟加拉Bangladesh 10 BE 比利时Belgium 11 BG 保加利亚Bulgaria 12 BH 巴林Bahrain 13 BI 布隆迪Burundi 14 BJ 贝宁Benin 15 BM 百慕大Bermuda 16 BN 文莱Brunei 17 BO 玻利维亚Bolivia 18 BR 巴西Brazil 19 BS 巴哈马Bahamas 20 BT 不丹Bhutan 21 BW 博茨瓦纳Botswana 22 CA 加拿大Canada 23 CF 中非共和国Central Africa 24 CG 刚果Congo 25 CH 瑞士Switzerland 26 CK 库克群岛Cook Is. 27 CL 智利Chile 28 CM 喀麦隆Cameroon 29 CN 中国China 30 CO 哥伦比亚Colombia 31 CR 哥斯达黎加Costa Rica 32 CU 古巴Cuba 33 CV 佛得角群岛Cape Verde Is. 34 CY 塞浦路斯Cyprus 35 CZ 捷克共和国Czech 36 DE 德国Germany 37 DK 丹麦Denmark 38 DZ 阿尔及利亚Algeria 39 EC 厄瓜多尔Ecuador 40 EE 爱沙尼亚Estonia 41 EG 埃及Egypt 42 ES 西班牙Spain 43 ET 埃塞俄比亚Ethiopia
酒店中常用的英文缩写及各国家名称的英文
酒店中常用的英文缩写 部门 DEPARTMENT DEPT 职位 POSITION POS 行政办 EXECUTIVE OFFICE EO 财务部 FINANCE DEPARTMENT FIC 会计部 ACCOUNTING ACCT 采购部 PURCHASING DEPARTMENT PD 酒水仓 BEVERAGE STORE 食品仓 FOOD STORE 日用品仓 GENERAL STORE 市场及销售部 SALES & MARKETING DEPARTMENT S & M 前厅部 FRONT OFFICE DEPARTMENT F.O (F/O) 管家部 HOUSEKEEPING HSKP 洗衣房 LAUNDRY ROOM LR 布草房 LINEN ROOM 游泳池 SWIMMING POOL 餐饮部 FOOD & BEVERAGE DEPARTMENT F & B 中餐厅 CHINESE RESTAURANT CHN REST 西餐厅 WEST RESTAURANT WEST REST 日本餐厅 JAPANESE RESTAURANT 大堂吧 LOBBY BAR 送餐部 ROOM SERVICE RM SVC 宴会中心 BANQUET CENTRE 会议厅 CONFERENCE HALL 管事部 STEWARD 娱乐部 ENTERTAINMENT DEPARTMENT ENT 夜总会 NIGHT CLUB 桑拿 SAUNA 健身中心 HEALTH CENTRE 美容美发中心 BEAUTY & BARBER CENTRE 保龄球室 BOWLING BALL 工程部 ENGINEERING DEPARTMENT ENG 保安部 SECURITY DEPARTMENT SEC 人力资源部 HUMAN & RESOURCES DEPARTMENT HR 培训部 TRAINING DEPARTMENT 员工饭堂 STAFF CANTEEN 员工宿舍 STAFF DORMITORY 医务室 CLINIC ROOM 图书馆 LIBRARY 前厅各分部: 接待处 RECEPTION RECP 行政楼层 EXECUTIVE FLOOR E/F
全球各国国名的英文缩写大全
有几个国家的缩写比较特殊,在开头列出(补充中) 荷兰 - NL (Netherlands) 西班牙 - ES (Spain) 南斯拉夫 - YU (Yugoslavia) 下面的排列顺序为按照国家英文全名以字典顺序排列检索时找“ - ” 后面的英文全名,字母顺序 AF - Afghanistan 阿富汗 AL - Albania 阿尔巴尼亚 DZ - Algeria 阿尔及利亚 AS - American Samoa 东萨摩亚 AD - Andorra 安道尔 AO - Angola 安哥拉 Av - Anguilla 安圭拉岛 AQ - Antarctica 南极洲 AG - Antigua and Barbuda 安提瓜和巴布达 AR - Argentina 阿根廷 AM - Armenia 亚美尼亚 AA - Aruba 阿鲁巴 AU - Australia 澳大利亚 AT - Austria 奥地利 AZ - Azerbaijan 阿塞拜疆 BF - Bahamas 巴哈马 BH - Bahrain 巴林 BB - Barbados 巴巴多斯 BD - Bangladesh 孟加拉 BY - Belarus 白俄罗斯 BE - Belgium 比利时 BZ - Belize 伯里兹 BJ - Benin 贝宁 BM - Bermuda 百慕大 BS - Bahamas 巴哈马 BT - Bhutan 不丹 BW - Botswana 博茨瓦纳 BO - Bolivia 玻利维亚 BA - Bosnia and Herzegovina 波黑 BV - Bouvet Island 布韦岛 BR - Brazil 巴西 IO - British Indian Ocean Territory 英属印度洋领地
各个国家英文名称缩写
A字头 AE-阿联酋(UNITED EMIRATES) AF-阿富汗(AFGHANISTAN) AL-阿尔巴尼亚(ALBANIA) AM-亚美尼亚(ARMENIA) AO-安哥拉(ANGOLA) AR-阿根廷(ARGENTINA) AT-奥地利(AUSTRIA) AU-澳大利亚(AUSTRALIA) AZ-阿塞拜疆(AZERBAIJAN(REPUBLIC)) B字头 BD-孟加拉(BANGLADESH) BE-比利时(BELGIUM) BF-布基纳法索(BURKINA FASO) BG-保加利亚(BULGARIA) BH-巴林(BAHREIN) BI-布隆迪(BURUNDI) BJ-贝宁(BENIN) BL-巴勒斯坦() BN-文莱(BRUNEI DARUSSALAM) BO-玻利维亚(BOLIVIA) BR-巴西(BRAZIL) BW-博茨瓦纳(BOTSWANA) BY-白俄罗斯(BYELORUSSIA) C字头 CA-加拿大(CANADA) CF-中非(CENTRAL AFRICA) CG-刚果(CONGO) CH-瑞士(SWITZERLAND) CL-智利(CHILE) CM-喀麦隆(CAMEROON) CN-中国(CHINA) CO-哥伦比亚(COLOMBIA) CR-哥斯达黎加(COSTA RICA) CS-捷克(CZECH REPUBIC) CU-古巴(CUBA) CY-塞浦路斯(CYPRUS) D字头 DE-德国(GERMANY / Deutschland) DK-丹麦(DENMARK) DO-多米尼加共和国(DOMINICAN REPUBLIC)
DZ-阿尔及利亚(ALGERIA) E字头 EC-厄瓜多尔(ECUADOR) EE-爱沙尼亚(ESTONIA) EG-埃及(EGYPT) ES-西班牙(SPAIN) ET-埃塞俄比亚(ETHIOPIA) F字头 FI-芬兰(FINLAND) FJ-斐济(FIJI) FR-法国(FRANCE) G字头 GA-加蓬(GABON) GB-英国(UNITED KINGDOM) GD-格林纳达(GRENADA) GE-格鲁吉亚(GEORGIA) GH-加纳(GHANA) GN-几内亚(GUINEA) GR-希腊(GREECE) GT-危地马拉(GUATEMALA) H字头 HK-香港特别行政区(HONG KONG) HN-洪都拉斯(HONDURAS) HU-匈牙利(HUNGARY) I字头 ID-印度尼西亚(INDONESIA) IE-爱尔兰(IRELAND) IL-以色列(ISRAEL) IN-印度(INDIA) IQ-伊拉克(IRAQ) IR-伊朗(IRAN) IS-冰岛(ICELAND) IT-意大利(ITALY) J字头 JM-牙买加(JAMAICA) JO-约旦(JORDAN) JP-日本(JAPAN)
世界各国国名及其简写
世界各国国名及其简写: --------------------------------------------------- A字母 --------------------------------------------------- AD Andorra 安道尔共和国 AE United Arab Emirates 阿拉伯联合酋长国AF Afghanistan 阿富汗 AG Antigua and Barbuda 安地卡及巴布达 AI Anguilla 安圭拉岛 AL Albania 阿尔巴尼亚 AM Armenia 亚美尼亚 AN Netherland Antilles 荷兰大小安第列斯群岛AO Angola 安哥拉 AQ Antarctica 南极洲 AR Argentina 阿根廷 AS American Samoa 美洲萨摩亚 AT Austria 奥地利 AU Australia 澳大利亚 AW Aruba 阿卢巴岛 AZ Azerbaijan 阿卢巴岛 --------------------------------------------------- B字母 --------------------------------------------------- BA Bosnia-Herzegovina 波斯尼亚 BB Barbados 巴贝多 BD Bangladesh 孟加拉共和国 BE Belgium 比利时 BF Burkina Faso 布基那法索 BG Bulgaria 保加利亚 BH Bahrain 巴林 BI Burundi 蒲隆地 BJ Bermuda 百慕达 BM Bermuda 百慕达 BN Brunei Darussalam 汶莱达累斯萨拉姆 BO Bolivia 玻利维亚 BR Brazil 巴西 BS Bahamas 巴哈马 BT Buthan BV Bouvet Island BW Botswana 波扎那 BY Bielorussia BZ Belize 贝里斯--------------------------------------------------- C字母 --------------------------------------------------- CA Canada 加拿大 CC Cocos (Keeling) Isl. 椰子岛 CF Central Africa Rep. 中非洲 CG Congo 刚果 CH Switzerland 瑞士 CI Ivory Coast 象牙海岸 CK Cook Islands CL Chile 智利 CM Cameroon 喀麦隆 CN China 中国 CO Colombia 安哥伦比亚 CR Costa Rica 哥斯达黎加 CS Czechoslovakia 捷克斯拉夫 CU Cuba 古巴 CV Cape Verde 维德角 CX Christmas Island 圣诞岛 CY Cyprus 塞普勒斯 CZ zech Republic --------------------------------------------------- D字母 --------------------------------------------------- DE Germany 德国 DJ Djibouti 吉布地 DK Denmark 丹麦 DM Dominica 多米尼克 DO Dominican Republic 多明尼加共和国DZ Algeria 阿尔及利亚 --------------------------------------------------- E字母 --------------------------------------------------- EC Ecuador 厄瓜多尔 EE Estonia 爱沙尼亚 EG Egypt 埃及 EH Western Sahara 西撒哈拉 ER Eritrea 厄立特里亚 ES Spain 西班牙 ET Ethiopia 衣索比亚 --------------------------------------------------- F字母 ---------------------------------------------------
世界各国国名及缩写大全
世界各国国名及缩写大全 AF - Afghanistan 阿富汗 AL - Albania 阿尔巴尼亚 DZ - Algeria 阿尔及利亚 AS - American Samoa 东萨摩亚 AD - Andorra 安道尔 AO - Angola 安哥拉 AV- Anguilla 安圭拉岛 AQ - Antarctica 南极洲 AG - Antigua and Barbuda 安提瓜和巴布达 AR - Argentina 阿根廷 AM - Armenia 亚美尼亚 AA - Aruba 阿鲁巴 AU - Australia 澳大利亚 AT - Austria 奥地利 AZ - Azerbaijan 阿塞拜疆 BF - Bahamas 巴哈马 BH - Bahrain 巴林 BB - Barbados 巴巴多斯 BD - Bangladesh 孟加拉 BY - Belarus 白俄罗斯 BE - Belgium 比利时 BZ - Belize 伯里兹 BJ - Benin 贝宁 BM - Bermuda 百慕大 BS - Bahamas 巴哈马 BT - Bhutan 不丹 BW - Botswana 博茨瓦纳 BO - Bolivia 玻利维亚 BA - Bosnia and Herzegovina 波黑 BV - Bouvet Island 布韦岛 BR - Brazil 巴西 IO - British Indian Ocean Territory 英属印度洋领地BN - Brunei Darussalam 文莱布鲁萨兰 BG - Bulgaria 保加利亚 BF - Burkina Faso 布基纳法索 BI - Burundi 布隆迪 KH - Cambodia (Internet) 柬埔寨 CB - Cambodia (CIA World Fact Book) 柬埔寨CM - Cameroon 喀麦隆 CA - Canada 加拿大 CV - Cape Verde 佛得角 KY - Cayman Islands 开曼群岛
国家 地区 名称与英文缩写
国家(地区)名称与英文缩写对照表 国家(地区)名 称英文 缩写 国家(地区)名称 英文 缩写 国家(地区)名称 英文 缩写 阿富汗AFG 英国GBR 尼日利亚NGR 荷属安的列斯AHO 几内亚比绍GBS 尼日尔NIG 阿尔巴尼亚ALB 格鲁吉亚GEO 挪威NOR 阿尔及利亚ALG 赤道几内亚GEQ 瑙鲁NRU 安道尔AND 德国GER 新西兰NZL 安哥拉ANG 加纳GHA 阿曼OMA 安提瓜和巴布 达 ANT 希腊GRE 巴基斯坦PAK 阿根廷ARG 格林纳达GRN 巴拿马PAN 亚美尼亚ARM 危地马拉GUA 巴拉圭PAR 阿鲁巴ARU 几内亚GUI 秘鲁PER 美属萨摩亚ASA 关岛GUM 菲律宾PHI 澳大利亚AUS 圭亚那GUY 巴勒斯坦PLE 奥地利AUT 海地HAI 帕劳PLW 阿塞拜疆AZE 中国香港HKG 巴布亚新几内亚PNG 巴哈马BAH 洪都拉斯HON 波兰POL 孟加拉国BAN 匈牙利HUN 葡萄牙POR 巴巴多斯BAR 牙买加IAM 朝鲜PRK 布隆迪BDI 印度尼西亚INA 波多黎各PUR 比利时BEL 印度IND 卡塔尔QAT 贝宁BEN 伊朗IRI 罗马尼亚ROU 百慕大BER 爱尔兰IRL 南非RSA 不丹BHU 伊拉克IRQ 俄罗斯RUS 波黑BIH 冰岛ISL 卢旺达RWA 伯利兹BIZ 以色列ISR 萨摩亚SAM 白俄罗斯BLR 美属维尔京群岛ISV 塞内加尔SEN 玻利维亚BOL 意大利ITA 塞舌尔SEY 博茨瓦纳BOT 英属维尔京群岛IVB 新加坡SIN 巴西BRA 约旦JOR 圣基茨和尼维斯SKN
巴林BRN 日本JPN 塞拉利昂SLE 文莱BRU 哈萨克斯坦KAZ 斯洛文尼亚SLO 保加利亚BUL 肯尼亚KEN 所罗门群岛SOL 布基纳法索BUR 吉尔吉斯斯坦KGZ 索马里SOM 中非CAF 基里巴斯KIR 塞尔维亚SRB 柬埔寨CAM 韩国KOR 斯里兰卡SRI 加拿大CAN 沙特阿拉伯KSA 圣多美和普林西比STP 开曼群岛CAY 科威特KUW 苏丹SUD 刚果(布)CGO 老挝LAO 瑞士SUI 乍得CHA 拉脱维亚LAT 苏里南SUR 智利CHI 利比亚LBA 斯洛伐克SVK 中国CHN 利比里亚LBR 瑞典SWE 科特迪瓦CIV 圣卢西亚LCA 斯威士兰SWZ 喀麦隆CMR 莱索托LES 叙利亚SYR 刚果(金)COD 黎巴嫩LIB 坦桑尼亚TAN 库克群岛COK 列支敦士登LIE 汤加TGA 哥伦比亚COL 立陶宛LTU 泰国THA 科摩罗COM 卢森堡LUX 塔吉克斯坦TJK 佛得角CPV 马达加斯加MAD 土库曼斯坦TKM 哥斯达黎加CRC 摩洛哥MAR 东帝汶TLS 克罗地亚CRO 马来西亚MAS 多哥TOG 古巴CUB 马拉维MAW 中华台北TPE 塞浦路斯CYP 摩尔多瓦MDA 特立尼达和多巴哥TRI 捷克CZE 马尔代夫MDV 突尼斯TUN 丹麦DEN 墨西哥MEX 土耳其TUR 吉布提DJI 蒙古MGL 图瓦卢TUV 多米尼克DMA 马绍尔群岛MHL 阿联酋UAE 多米尼加DOM 马其顿MKD 乌干达UGA 厄瓜多尔ECU 马里MLI 乌克兰UKR 埃及EGY 马耳他MLT 乌拉圭URU 厄立特里亚ERI 黑山MNE 美国USA 萨尔瓦多ESA 摩纳哥MON 乌兹别克斯坦UZB 西班牙ESP 莫桑比克MOZ 瓦努阿图VAN
国家名缩写
AT at 奥地利 AD ad 安道尔 AE ae 阿联酋 AF af 阿富汗 AI ai 安奎拉 AL al 阿尔巴尼亚 AM am 亚美尼亚 AO ao 安哥拉 AQ aq 南极洲 AR ar 阿根廷 AS as 美属萨摩亚群岛 AU au 澳大利亚 AZ az 阿塞拜疆 BA ba 波斯尼亚和黑塞哥维那BB bb 巴巴多斯 BD bd 孟加拉 BE be 比利时 BF bf 布基那法索 BG bg 保加利亚 BH bh 巴林 BI bi 布隆迪 BJ bj 贝宁 BM bm 百慕大 BN bn 文莱 BO bo 玻利维亚 BR br 巴西 BS bs 巴哈马 BT bt 不丹 BW bw 博茨瓦纳 BZ bz 伯里兹 CA ca 加拿大 CF cf 中非共和国 CG cg 刚果 CH ch 瑞士 CI ci 象牙海岸 CK ck 库克群岛 CL cl 智利 CM cm 喀麦隆 CN cn 中国 CO co 哥伦比亚 CR cr 哥斯达黎加 CS cs 捷克斯洛伐克(前)CU cu 古巴 CV cv 佛得角群岛
CY cy 塞浦路斯 CZ cz 捷克共和国 DE de 德国 DJ dj 吉布提 DK dk 丹麦 DM dm 多米尼加 DO do 多米尼加共和国DZ dz 阿尔及利亚 EC ec 厄瓜多尔 EE ee 爱沙尼亚 EG eg 埃及 EH eh 西撒哈拉 ER er 厄立特利亚 ES es 西班牙 ET et 埃塞俄比亚 FI fi 芬兰 FJ fj 斐济 FK fk 马尔维那斯群岛FM fm 密克罗尼西亚FR fr 法国 GA ga 加蓬 GB gb 英国 GD gd 格林纳达 GE ge 乔治亚 GF gf 法属圭亚那 GH gh 加纳 GI gi 直布罗陀 GL gl 格陵兰(岛)GM gm 赞比亚 GN gn 几内亚 GP gp 瓜德罗普 GQ gq 赤道几内亚 GR gr 希腊 GT gt 危地马拉 GU gu 关岛 GW gw 几内亚比绍GY gy 圭亚那 HK hk 香港 HN hn 洪都拉斯 HR hr 克罗地亚 HT ht 海地 HU hu 匈牙利 ID id 印度尼西亚 IE ie 爱尔兰
世界各国的中英文名称对照及英文简写
UA:乌克兰Ukraine DE:德国Germany U.K.:英国 GB:大不列颠Great Britain RUS:俄罗斯Russia PL: 波兰Poland English Name 国家(地区) 首都(首府) 所属大洲域名后缀Afghanistan 阿富汗喀布尔西南亚AF Albania 阿尔巴尼亚地拉那欧洲AL Algeria 阿尔及利亚阿尔及尔北非洲DZ American Samoa 美属萨摩亚群岛帕果帕果大洋洲AS Andorra 安道尔安道尔欧洲AD Angola 安哥拉罗安达非洲AO Anguilla 安圭拉瓦利美洲AI Antarctica 南极洲\\ \\ AQ Antigua and Barbuda 安提瓜和巴布达圣约翰美洲Argentina 阿根廷布宜诺斯艾利斯南美洲AR Armenia 亚美尼亚埃里温亚洲AM Australia 澳大利亚堪培拉大洋洲AU Austria 奥地利维也纳欧洲AT Azerbaijan 阿塞拜疆巴库亚洲AZ Bahamas 巴哈马拿骚北美洲BS Bahrain 巴林麦纳麦亚洲BH Bangladesh 孟加拉达卡亚洲BD Barbados 巴巴多斯布里奇敦北美洲BB Belarus 白俄罗斯明斯克欧洲 Belize 伯利兹贝尔莫潘北美洲BZ Belgium 比利时布鲁塞尔欧洲BE
Benin 贝宁波多诺伏非洲BJ Bermuda 百慕大汉密尔顿北美洲BM Bhutan 不丹廷布亚洲BT Bolivia 玻利维亚苏克雷南美洲BO Bosnia & Herzegovina 波斯尼亚和黑塞哥维那萨拉热窝欧洲BA Botswana 博茨瓦纳哈博罗内非洲BW Brazil 巴西巴西利亚南美洲BR Brunei 文莱斯里巴加湾市亚洲BN Bulgaria 保加利亚索非亚欧洲BG Burkina Faso 布基纳法索瓦加杜古非洲BF Burundi 布隆迪布琼布拉非洲BI Cambodia 柬埔寨金边亚洲KH Cameroon 喀麦隆雅温得非洲CM Canada 加拿大渥太华北美洲CA Cape verde islands 佛得角群岛普拉亚非洲CV Cayman Islands 开曼群岛乔治敦美洲KY Central Africa 中非共和国班吉非洲CF Chad 乍得恩贾梅纳非洲TD Chile 智利圣地亚哥南美洲CL China 中国北京亚洲CN Cook Islands 库克群岛阿瓦鲁阿大洋洲CK Colombia 哥伦比亚波哥大南美洲CO Comoros 科摩罗群岛莫罗尼非洲KM The Republic of Congo 刚果共和国/刚果(布) 布拉柴维尔非洲CG The Democratic Republic Of Congo 刚果民主共和国/刚果(金) 金沙萨非洲ZR Costarica 哥斯达黎加圣何塞北美洲CR Cote d'Ivoire/Ivory Coast 科特迪瓦/象牙海岸亚穆苏克罗非洲CI Croatia 克罗地亚萨格勒布欧洲HR Cuba 古巴哈瓦那北美洲CU
各个国家缩写
阿尔巴尼亚AL Albania 阿尔及利亚DZ Algeria 阿富汗AF Afghanistan 阿根廷AR Argentina 阿拉伯联合酋长国AZ Azerbaijan 阿拉伯联合酋长国AE United Arab Emirates 阿拉伯也门共和国 阿拉斯加 阿鲁巴AW Aruba 阿鲁巴岛 阿曼 阿曼OM Oman 阿塞拜疆共和国 阿森松 埃及EG Egypt 埃塞俄比亚ET Ethiopia 爱尔兰IE Ireland 爱沙尼亚EE Estonia 安道尔AD Andorra 安哥拉AO Angola 安圭拉AI Angola 安提瓜和巴布达AG Ntigua and Barbuda 奥地利AT Austria 澳大利亚AU Australia 巴巴多斯BB Barbados 巴布亚新几内亚PG Papua,Territory of 巴哈马BS Bahamas 巴基斯坦PK Pakistan 国家或地区名称(中文)地区代码国家或地区名称(英文)巴拉圭PY Paraguay 巴勒斯坦 巴林BH Bahrain 巴拿马PA Panama 巴西BR Brazil 白俄罗斯共和BY White Russia 白俄罗斯共和BY Byelorussian SSR 百慕大BM Bermuda 保加利亚BG Bulgaria 北马里亚纳群岛 贝宁BJ Benin 比利时BE Belgium 冰岛IS Iceland 波多黎各(美)PR Puerto Rico 波兰PL Poland
波斯尼亚和黑塞哥维那BA Bosnia Hercegovina 玻利维亚BO Bolivia 伯利兹BZ Belize 博茨瓦纳BW Botswana 不丹BT Bhutan 不列颠岛(美) VI Vigin Islands(U.S.) 不列颠岛(英) VG Virgin Islands(British) 布基纳法索BF Burkina Faso 布隆迪BI Burundi 布维岛BV Bouvet Island 朝鲜KP North Korea 赤道几内亚GQ Equatorial Guinea 大西洋东区(海事卫星) 大西洋区(海事卫星) 丹麦DK Denmark 德国DE Grmany 东帝TP East Timor 东非共同体 东萨摩亚 多哥TG Togo 多米尼加共和国DO Dominica 多明哥DM Gominica 俄罗斯联邦RU Russia 厄瓜多尔EC Ecuador 厄立特里亚 法国FR France 法属玻里尼西亚PF French Polynesia 法属圭亚那GF French Guiana 国家或地区名称(中文)地区代码国家或地区名称(英文)法属南方领土TF French Southern Territoties 梵蒂冈(罗马教庭)V A V atican 菲律宾PH Philippines 斐济FJ Fiji 芬兰FI Finland 佛得角CV Cape Verde,Republic of 福克兰群岛FK Falkland Islands 冈比亚GM Gambia 刚果(布)CG Congo 刚果(金) 哥伦比亚CO Colombia 哥斯达黎加CR Costa rica 格林纳达GD Grenada 格陵兰GL Greenland 格鲁吉亚GE Georgia
世界国家的英文名称及缩写
世界国家的英文名称及缩写: AFG 阿富汗Afghanistan ALB 阿尔巴尼亚Albania ALG 阿尔及利亚Algeria AND 安道尔Andorra ANG 安哥拉Angola ANT 安提瓜和巴布达Antigua and Barbuda ARG 阿根廷Argentina ARM 亚美尼亚Armenia AUS 澳大利亚Australia AUT 奥地利Austria AZE 阿塞拜疆Azerbaijan BAH 巴哈马Bahamas BAN 孟加拉国Bangladesh BAR 巴巴多斯Barbados BEL 比利时Belgium BEN 贝宁Benin BDI 布隆迪Burundi BHU 不丹Bhutan BIH 波黑Bosnia and Herzegovina BIZ 伯利兹Belize BLR 白俄罗斯Belarus BOL 玻利维亚Bolivia BOT 博茨瓦纳Botswana BRA 巴西Brazil BRN 巴林Bahrain BRU 文莱Brunei BUL 保加利亚Bulgaria BUR 布基纳国Burkina Faso CAF 中非Central African Republic CAM 柬埔寨Cambodia CAN 加拿大Canada CHA 乍得Chad CGO 刚果Congo CHI 智利Chile CHN 中国China CIV 科特迪瓦Cote d'Ivoire CMR 喀麦隆Cameroon COD 刚果(金) Congo (Congo-Kinshasa) COL 哥伦比亚Colombia COM 科摩罗Comoros CPV 佛得角Cape Verde CRC 哥斯达黎加Costa Rica CRO 克罗地亚Croatia CUB 古巴Cuba CYP 塞浦路斯Cyprus CZE 捷克Czech DEN 丹麦Denmark DJI 吉布提Djibouti DMA 多米尼克Dominica DOM 多米尼加Dominican Republic ECU 厄瓜多尔Ecuador EGY 埃及Egypt ERI 厄立特里亚Eritrea ESA 萨尔瓦多El Salvador ESP 西班牙Spain EST 爱沙尼亚Estonia ETH 埃塞俄比亚Ethiopia FIJ 斐济Fiji FIN 芬兰Finland FSM 密克罗尼西亚Micronesia FRA 法国France GAB 加蓬Gabon GAM 冈比亚Gambia GBR 英国United Kingdom GEO 格鲁吉亚Georgia GEQ 赤几Equatorial Guinea GER 德国Germany GHA 加纳Ghana GNB 几比Guinea-Bissau GRE 希腊Greece GRN 格林纳达Grenada GUA 危地马拉Guatemala GUI 几内亚Guinea GUY 圭亚那Guyana HAI 海地Haiti
世界各国国旗 英文名称及缩写
世界各国(地区)国旗英文名称及缩写亚洲: 1阿富汗英文全称:Afghanistan 英文缩写:AFG 2亚美尼亚英文全称:The Republic of Armenia英文缩写:ARM 3阿塞拜疆英文全称:The Republic of Azerbaijan英文缩写:AZE 4巴林英文全称:The Kingdom of Bahrain英文缩写:BRN 5孟加拉共和国The People's Republic of Bangladesh英文缩写:BAN 6不丹王国英文全称:Kingdom of Bhutan英文缩写:BHU 7文莱英文全称:Brunei Darussalam英文缩写:BRU
8缅甸英文全称:The Union of Myanmar英文缩写:MY A 9柬埔寨英文全称:The Kingdom of Cambodia英文缩写:CAM 10中国英文名称The People's Republic of China英文缩写CHN 11塞浦路斯The Republic of Cyprus英文缩写:CYP 12东帝汶英文全称:Democratic Republic of Timor-Leste英文缩写:TLS 13印度英文全称:The Republic of India英文缩写:IND 14印度尼西亚英文全称:The Republic of Indonesia英文缩写:INA
15伊朗英文全称:The Islamic Republic of Iran英文缩写:IRI 16伊拉克英文全称:The Republic of Iraq英文缩写:IRQ 17以色列The State of Israel英文缩写:ISR 18日本英文全称:Japan英文缩写:JPN 19约旦英文全称:The Hashemite Kingdom of Jordan英文缩写:JOR 20哈萨克斯坦英文全称:The Republic of Kazakhstan英文缩写:KAZ 21朝鲜英文全称:Democratic People's Republic of Korea英文缩写:PRK