A supercritical or transcritical Rankine cycle with ejector using low-grade heat
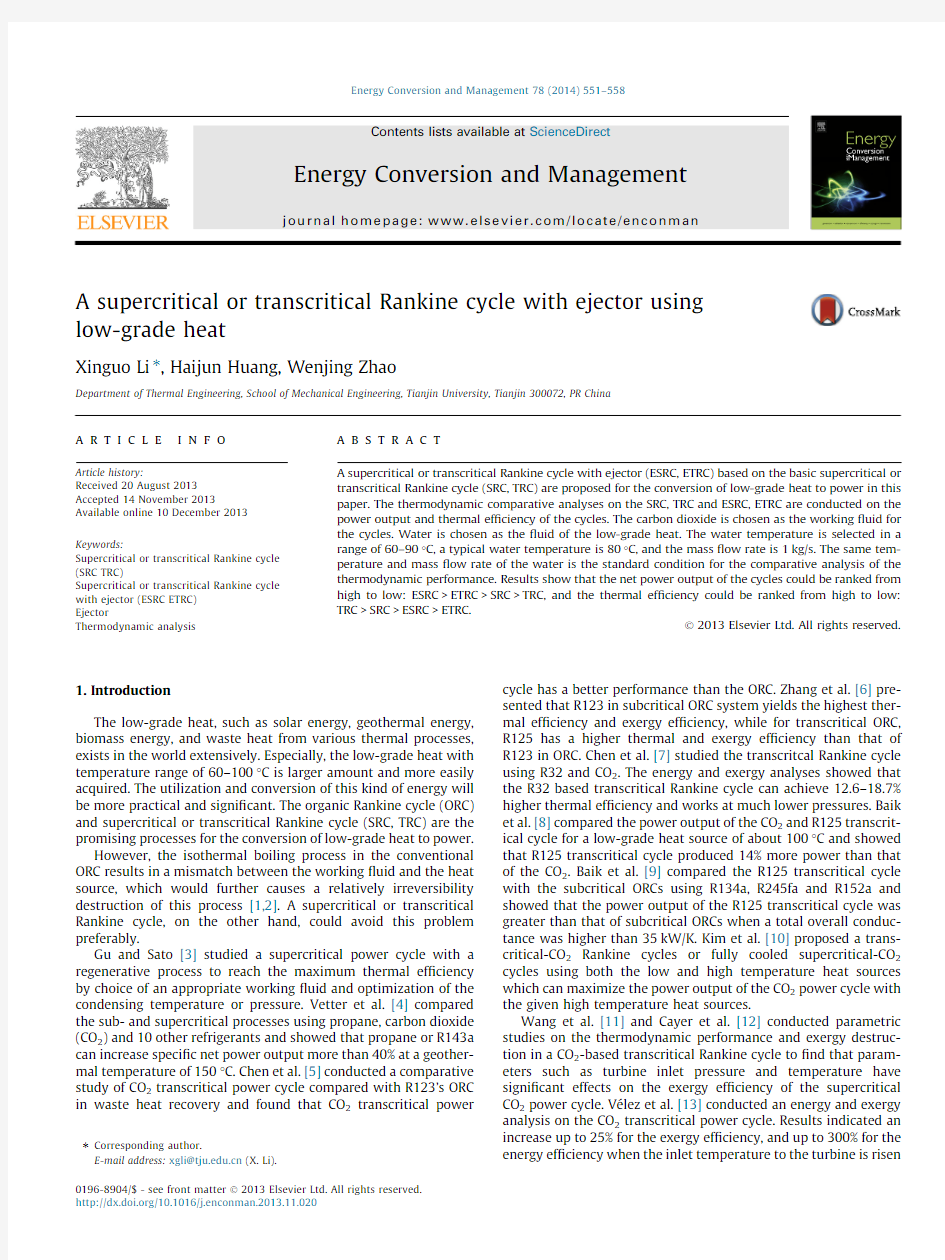

A supercritical or transcritical Rankine cycle with ejector using low-grade
heat
Xinguo Li ?,Haijun Huang,Wenjing Zhao
Department of Thermal Engineering,School of Mechanical Engineering,Tianjin University,Tianjin 300072,PR China
a r t i c l e i n f o Article history:
Received 20August 2013Accepted 14November 2013
Available online 10December 2013Keywords:
Supercritical or transcritical Rankine cycle (SRC TRC)
Supercritical or transcritical Rankine cycle with ejector (ESRC ETRC)Ejector
Thermodynamic analysis
a b s t r a c t
A supercritical or transcritical Rankine cycle with ejector (ESRC,ETRC)based on the basic supercritical or transcritical Rankine cycle (SRC,TRC)are proposed for the conversion of low-grade heat to power in this paper.The thermodynamic comparative analyses on the SRC,TRC and ESRC,ETRC are conducted on the power output and thermal ef?ciency of the cycles.The carbon dioxide is chosen as the working ?uid for the cycles.Water is chosen as the ?uid of the low-grade heat.The water temperature is selected in a range of 60–90°C,a typical water temperature is 80°C,and the mass ?ow rate is 1kg/s.The same tem-perature and mass ?ow rate of the water is the standard condition for the comparative analysis of the thermodynamic performance.Results show that the net power output of the cycles could be ranked from high to low:ESRC >ETRC >SRC >TRC,and the thermal ef?ciency could be ranked from high to low:TRC >SRC >ESRC >ETRC.
ó2013Elsevier Ltd.All rights reserved.
1.Introduction
The low-grade heat,such as solar energy,geothermal energy,biomass energy,and waste heat from various thermal processes,exists in the world extensively.Especially,the low-grade heat with temperature range of 60–100°C is larger amount and more easily acquired.The utilization and conversion of this kind of energy will be more practical and signi?cant.The organic Rankine cycle (ORC)and supercritical or transcritical Rankine cycle (SRC,TRC)are the promising processes for the conversion of low-grade heat to power.However,the isothermal boiling process in the conventional ORC results in a mismatch between the working ?uid and the heat source,which would further causes a relatively irreversibility destruction of this process [1,2].A supercritical or transcritical Rankine cycle,on the other hand,could avoid this problem preferably.
Gu and Sato [3]studied a supercritical power cycle with a regenerative process to reach the maximum thermal ef?ciency by choice of an appropriate working ?uid and optimization of the condensing temperature or pressure.Vetter et al.[4]compared the sub-and supercritical processes using propane,carbon dioxide (CO 2)and 10other refrigerants and showed that propane or R143a can increase speci?c net power output more than 40%at a geother-mal temperature of 150°C.Chen et al.[5]conducted a comparative study of CO 2transcritical power cycle compared with R123’s ORC in waste heat recovery and found that CO 2transcritical power cycle has a better performance than the ORC.Zhang et al.[6]pre-sented that R123in subcritical ORC system yields the highest ther-mal ef?ciency and exergy ef?ciency,while for transcritical ORC,R125has a higher thermal and exergy ef?ciency than that of R123in ORC.Chen et al.[7]studied the transcritcal Rankine cycle using R32and CO 2.The energy and exergy analyses showed that the R32based transcritical Rankine cycle can achieve 12.6–18.7%higher thermal ef?ciency and works at much lower pressures.Baik et al.[8]compared the power output of the CO 2and R125transcrit-ical cycle for a low-grade heat source of about 100°C and showed that R125transcritical cycle produced 14%more power than that of the CO 2.Baik et al.[9]compared the R125transcritical cycle with the subcritical ORCs using R134a,R245fa and R152a and showed that the power output of the R125transcritical cycle was greater than that of subcritical ORCs when a total overall conduc-tance was higher than 35kW/K.Kim et al.[10]proposed a trans-critical-CO 2Rankine cycles or fully cooled supercritical-CO 2cycles using both the low and high temperature heat sources which can maximize the power output of the CO 2power cycle with the given high temperature heat sources.
Wang et al.[11]and Cayer et al.[12]conducted parametric studies on the thermodynamic performance and exergy destruc-tion in a CO 2-based transcritical Rankine cycle to ?nd that param-eters such as turbine inlet pressure and temperature have signi?cant effects on the exergy ef?ciency of the supercritical CO 2power cycle.Vélez et al.[13]conducted an energy and exergy analysis on the CO 2transcritical power cycle.Results indicated an increase up to 25%for the exergy ef?ciency,and up to 300%for the energy ef?ciency when the inlet temperature to the turbine is risen
0196-8904/$-see front matter ó2013Elsevier Ltd.All rights reserved.https://www.360docs.net/doc/4e6661917.html,/10.1016/j.enconman.2013.11.020
Corresponding author.
E-mail address:xgli@https://www.360docs.net/doc/4e6661917.html, (X.Li).
from60to150°C.B?y?kog?lu and Yal??nkaya[14]investigated a geothermal power cycle using CO2at supercritical condition,and a parametric study was performed to test the proposed system un-der different reservoir conditions.Schuster et al.[15]conducted an exergy analysis and comparison and indicated that the system ef?-ciency could improve approximately8%for the supercritical parameters because of the better exergetical ef?ciency.Sarkar and Bhattacharyya[16]analyzed the effects of different operating conditions and cycle performance on the optimized supercritical CO2recompression cycle.
In nuclear reactors,solar energy collectors and coal-?red plant adapted CO2capture process et al.,the supercritical CO2power cy-cle attracted more and more interests recently[17–20].Zhang et al. [21,22]investigated the performance of a solar supercritical Rankine system using CO2and showed that heat collection ef?ciency for the CO2-based solar collector is measured at8.78–9.45%which presents a potential future for the solar powered CO2Rankine system.Song et al.[23]analyzed a transcritical CO2 power cycle driven by solar energy and showed that there is an optimum turbine inlet pressure where the net power output and the system ef?ciency both reach maximum values.In addition, the condensation temperature has a signi?cant impact on the sys-tem performance.Halimi and Suh[24]developed a optimized supercritical cycle analysis code to analyze the design of a super-critical CO2driven Brayton cycle for a fusion reactor.
Carbon dioxide(CO2)with the advantages of abundant in nature,non-toxic,non-?ammable and inexpensive has been exten-sively studied as the working?uid in the supercritical or transcrit-ical Rankine cycles.However,the low critical temperature of 31.18°C might be a disadvantage for the condensation process.
Both the ORC and supercritical or transcritical Rankine cycle (SRC and TRC)have their own advantages.Although the SRC and TRC can obtain a better thermal match than the ORC,the SRC and TRC normally needs high pressure that may lead to dif?culties in operation and a safety concern.
From brief review of the literatures above,the researches on the supercritical or transcritical Rankine cycles are mainly focused on the temperature of low-grade heat in medium to high(>100°C) conditions.But,most of the temperature is lower than100°C which is larger amount and more easily acquired,as solar energy, low temperature geothermal and waste heat from various thermal processes et al.To use this kind of energy,especially to increase the power output from this low temperature energy by power cycles will be more practical and signi?cant to the research and application.
In view of this consideration,a supercritical or transcritical Rankine cycle with ejector is proposed in this paper.With the ejector,the power output of the cycle could be increased compared to the basic supercritical or transcritical Rankine cycle.
2.Principle of the supercritical or transcritical Rankine cycle with ejector
Fig.1shows the process of the supercritical or transcritical Rankine cycle(SRC,TRC)in the T–S diagram.The major difference between the supercritical and transcritical Rankine cycle is whether the cooling process takes place at a pressure below the critical pressure.As shown in Fig.1a,the working?uid in the supercritical Rankine cycle(SRC)processes is found entirely above its critical pressure.While,in transcritical Rankine cycle(TRC),the cooling process goes through the two-phase region below the crit-ical pressure,as shown in Fig.1b.
Based on the basic SRC and TRC,an ejector and a second-stage heater are introduced to the SRC and TRC to form the supercritical or transcritical Rankine cycle with ejector(ESRC,ETRC),as shown in Figs.2and3.In the ETRC and ESRC,the supercritical?uid from the second-stage heater works as the primary?uid in the ejector to entrain the exhaust from the expander so as to decrease the expander’s backpressure and increase the pressure difference in
Nomenclature
h speci?c enthalpy(J kgà1)
m mass?ow rate(kg sà1)
Q heat transfer(W)
s speci?c entropy(J kgà1Kà1)
P pressure(bar)
T temperature(K)
u entrainment ratio of ejector or mass?ow ratio in?rst-stage heater
W power(W)
Greek symbols
g ef?ciency(%)
Subscripts
dry dry?uid(expansion)
cool(c)cooling
cr critical exp expander
heat(h)heating
in inlet
max maximum
oc environment
opt optimum
out outlet
net net output
pp pinch point
r reduced parameter sum sum
th thermal
w water
wet wet?uid(expansion) wf working?uid
1–7state points
552X.Li et al./Energy Conversion and Management78(2014)551–558
the expander that results in an increase of the power output of the cycles.
2.1.Process and principle of the ESRC and ETRC
(1)The?rst cycle5–6–1–3–4–5:One part of subcooled(or sat-
urated)liquid from the cooler(or condenser)is pumped to the?rst-stage heater in process5–6,and then heated to the supercritical state in process6–1;the supercritical?uid expands in the expander,generating power in process1–3;
the exhaust?uid from the expander(state3)will work as the secondary?uid of the ejector to the ejection process.
(2)The second cycle5–7–2–4–5:The other part of subcooled
(or saturated)liquid from the cooler(or condenser)is pumped to the second-stage heater in process5–7,and heated to the supercritical state(or superheated vapor in the ETRC)in process7–2;the supercritical?uid(or super-heated vapor)(state2)will work as the primary?uid of the ejector to the ejection process.
(3)The primary?uid(state2)and the secondary?uid(state3)
are mixed and diffused in the ejector to form the mixed?uid (state4).The mixed?uid(state4)from the ejector enters to the cooler(or condenser)to be cooled to the subcooled(or saturated)?uid(state5);then pumped to the two heaters by the?uid pumps in process5–6and process5–7respec-tively,completed the cycle.
2.2.Process and principle of the ejector
Fig.4shows the con?guration and process of the ejector.A typical ejector consists of a motive nozzle,a suction nozzle or receiving chamber,a mixing section and a diffuser.A high pressure (P h2)primary?uid(state2)from second-stage heater in the ESRC or ETRC expands in the motive nozzle where its internal energy converts to kinetic energy.This high speed primary?uid entrains a low pressure(P3)secondary?uid(state3)from the expander in the suction nozzle.Both?uids enter the mixing section where they exchange momentum,kinetic and internal energies and become a mixed?uid(state4)outlet from the ejector and inlet to the cooler or condenser with a middle pressure(P c).
The result of the ejection process is that the outlet pressure from the expander(P3)as the secondary?uid is lower than the cooling or condensation pressure(P c)of the mixed?uid.It means that the expander’s backpressure of P3in the ESRC or ETRC is decreased compared to the basic cycle of SRC or TRC in which the backpressure is Pc that results in an increase of the power output in the ETRC and ESRC compared to the TRC and SRC.
3.Thermodynamic analysis of the cycle
3.1.Speci?cations and conditions for calculations
1.The carbon dioxide CO2is chosen as the working?uid for the
supercritical or transcritical Rankine cycles(SRC,TRC,ESRC and ETRC).
2.Water is chosen as the?uid of the low-grade heat.The water
temperature is selected in a range of60–90°C,a typical water temperature is80°C,and the mass?ow rate is1kg/s.The same temperature and mass?ow rate of the water is the standard condition for the comparative analysis of the thermodynamic performance.
3.A pinch point temperature difference(D T_pp)between the heat
source water and the working?uid is set to8°C.The maximum outlet and inlet temperature of the CO2in the heater of the cycles is set to lower than the inlet and outlet temperature of the water by D T_pp respectively in the calculation.
4.In the SRC and ESRC,the outlet temperature of the CO2from the
cooler is set to30°C.In the TRC and ETRC,the outlet of CO2 from the condenser is set as saturated liquid,and the bubble temperature of CO2is set the same as30°C.
5.Carbon dioxide CO2is a wet?uid.A wet expansion can be trans-
ferred to dry expansion with the outlet of the expander shifted to the saturated(or super heat)vapor,and the inlet to the expander will be shifted to the super heat state.
6.In the ETRC and ESRC,the outlet pressure from the expander
(P3),i.e.,the pressure of the second?uid in the ejector,is set to0.95or0.9times the cooling pressure(P c),and a pressure drop ratio(c)is de?ned as c=P3/P c=0.95or0.9.
7.In consideration of the comparability,a reduced parameter of
the pressure(P r=P/P cr)is used to illustrate the cycle’s thermo-dynamic performance of the working?uids.
8.The environment state is set to:P oc=101.325kPa,
T oc=298.15K.
3.2.The simulation of the ejector
In consideration of the calculation load and to simplify the calculation process,an idealized thermodynamic process of the ejector in the ESRC and ETRC is assumed and considered as the enthalpy balance and the entropy balance in the ejection process of the ejector,as follows:
h4?eh3?uth2T=e1tuTe1TS4?es3?uts2T=e1tuTe2TThe entrainment ratio(u)of the ejector,i.e.,the mass?ow ratio in the?rst-stage heater,is:
X.Li et al./Energy Conversion and Management78(2014)551–558553
u?m wf1=m wf2e3TThe total mass?ow ratio(m wf)in the cycle is:
m wf?m wf1tm wf2e4TIn the ejection process of the ejector,there should exist the pressure losses and irreversible processes to cause the increase of entropy in the ejection process.For the unsound theory and model of the ejection process for the supercritical?uid,this problem will be necessary for a further study in the future.
3.3.Thermodynamic performance of the cycle
The thermodynamic analyses and comparisons between the ESRC,ETRC and the basic SRC,TRC will be conducted.And the emphasis will be on the thermodynamic performance at the max-imum net power output(W net_max)of the cycles.The calculated thermodynamic performance includes:heating load(Q heat),power output from the expander(W out),pumping power(W pump),net power output(W net),thermal ef?ciency(g th),and the operating condition parameters.The simulation procedures for the cycle’s thermodynamic performance are built based on the refrigerant’s physical properties by REFPROP from NIST[25]on Matlab.Fig.5 shows the?ow chart of the simulation procedure for the ESRC.
An idealized thermodynamic process of the cycles is assumed and considered as no pressure loss,no heat loss,and isentropic processes are assumed for the expander and the pump.
The heating load(Q heat)from heat source water in the heater is: Q heat?m wf?eh heat outàh heat inTe5TThe cooling load(Q cool)in the cooler(or condenser)is:
Q cool?m wf?eh cool inàh cool outTe6TThe power output(W out)from the expander is:
W out?m wf?eh exp inàh exp outTe7TThe pumping power(W pump)by the pump is:
W pump?m wf?eh pump outàh pump inTe8TThe net power output(W net)of the cycle is:
W net?W outàW pumpe9TThe thermal ef?ciency(g th)of the cycle is:
g th ?W net=Q heate10T
4.Results and discussion
For different working?uids have different pressure in the heat-
ing or cooling processes in the cycle,a reduced parameter of the
pressure(P r=P/P cr)will be used to illustrate the thermodynamic
performance of the cycles.This reduced parameter of the pressure
will be more intuitive and comparable to the evaluation of thermo-
dynamic performance of different working?uids.
Figs.6–12present the thermodynamic performance of the basic
supercritical or transcritical Rankine cycle(SRC,TRC)and the
supercritical or transcritical Rankine cycle with ejector(ESRC,
ETRC)with working?uid of CO2.
Fig.5.Flow chart of the simulation procedure for the ESRC. 554X.Li et al./Energy Conversion and Management78(2014)551–558
It is found that the variation of the net power output (W net )in the dry expansion and wet expansion is the same in most range of the operation conditions.In the calculation of the dry expansion,the outlet of the expansion will be shifted manually to the satu-rated vapor if it falls into the two-phase region to avoid the wet expansion.So the outlet temperature of the CO 2from the heater will be increased until it reaches the maximum which is lower than the inlet water temperature by D T _pp of 8°C,and the calculation will be terminated.
X.Li et al./Energy Conversion and Management 78(2014)551–558
555
In the optimum calculation,the outlet temperature of the CO2 from the heater in those four cycles always reaches the maximum of72°C which is lower than the water temperature of80°C by
D T_pp of8°C.
https://www.360docs.net/doc/4e6661917.html, power output(W net)
As shown in Fig.6,the net power output(W net)of the TRC,SRC, ETRC and ESRC with CO2at water temperature of80°C increases and then decreases with the?rst-stage heating pressure(P h1), and exists the maximum net power output(W net_max).The W net_max is9.25kW,9.45kW,10.19kW,10.32kW for TRC,SRC,ETRC,ESRC respectively at the pressure drop ratio(c=P3/P c)of0.95in ETRC and ESRC,as shown in Table1.And the increases of the W net_max in the SRC,ETRC,ESRC is2.14%,10.18%and11.60%compared to the TRC respectively.The corresponding optimum?rst-stage heat-ing pressure(P h1_opt)is122.91bar,128.58bar,124.74bar and 131.34bar for TRC,SRC,ETRC and ESRC respectively,or the reduced parameter of pressure(P r1)is1.67,1.74,1.69and1.78. The W net of the cycles can be ranked from high to low: ESRC>ETRC>SRC>TRC.
4.2.Thermal ef?ciency(g th)
As shown in Fig.7,the thermal ef?ciency(g th)of the TRC and SRC increases with the P h1throughout.But the g th of the ETRC and ESRC increases and then decreases with the P h1,and exists the maximum of g th(g th_max).The g th_max is6.41%for ETRC and 6.37%for ESRC,and the corresponding P h1is117.51bar, 124.17bar respectively at the pressure drop ratio(c=P3/P c)of
0.95in ETRC and ESRC.But this condition of the maximum of g th
(g th_max)is different to the condition of the maximum net power output(W net_max).
In the condition of the W net_max,the g th of the TRC,SRC,ETRC and ESRC is8.19%,7.88%,6.23%,and6.30%.Different to the increase of the W net_max,the g th of the SRC,ETRC and ESRC isà3.89%,
Table1
Performance comparison among the cycles at different expander’s outlet pressure in the condition of the maximum net power output(W net_max)and water temperature of80°C and?ow rate of1kg/s.
Cycle TRC SRC ETRC ESRC
1.Pressure drop ratio c(P3/P c)in expander in ETRC and ESRC///0.950.9_wet0.9_dry0.950.9_wet0.9_dry
2.Water temperature outlet from heater
First-stage heater°C53.0551.3653.4855.3654.5551.8954.2952.15 Second-stage heater°C//40.9444.1444.9140.8843.4444.26
3.Cycle parameters of working?uid CO2
First-stage heater Pressure(P h1)bar122.91128.58124.74133.25129.54131.34144.17132.66
Pressure ratio of
P r1(P h1/P cr)
/ 1.67 1.74 1.69 1.81 1.76 1.78 1.95 1.80
Inlet temperature(T6)°C45.0543.3645.4747.3646.5543.8946.2944.15
Outlet temperature(T1)°C7272727272727272
Mass?ow rate(m wf1)kg/s 1.02 1.07 1.03 1.10 1.07 1.10 1.21 1.11
Heating load(Q h1)kW112.88119.97111.10103.21106.60117.72107.70116.65 Second-stage heater Pressure(P h2)bar//79.7289.3491.8583.3092.8696.09
Pressure ratio of
P r2(P h2/P cr)
/// 1.08 1.21 1.25 1.13 1.26 1.30
Inlet temperature(T7)°C//32.9436.1436.9132.8835.4436.26
Outlet temperature(T2)°C//45.4847.3646.5543.8946.2944.15
Mass?ow rate(m wf2)kg/s//0.440.480.480.410.510.57
Heating load(Q h2)kW//52.4346.9240.3046.0345.3533.00 Cooler or condenser Pressure(P c)bar72.1473.7872.1472.1472.1473.7873.7873.78
Pressure ratio of P rc
(P c/P cr)
/0.9778 1.00010.97780.97780.9778 1.0001 1.0001 1.0001
Inlet temperature(T4)°C34.0834.0134.6531.9031.8833.8631.7931.79
Outlet temperature(T5)°C3030303030303030
Mass?ow rate(m wf)kg/s 1.02 1.07 1.48 1.58 1.54 1.50 1.71 1.67
Cooling load(Q cool)kW103.63110.52153.33139.46136.27153.42142.43139.14
4.Parameters in ejection
Primary?uid(state2)Pressure(P h2)bar//79.7289.3491.8583.3092.8696.09
Temperature(T2)°C//45.4847.3646.5543.8946.2944.15 Second?uid(state3)Pressure(P3)bar//68.5364.9264.9270.0966.4066.40
Pressure ratio of
P r3(P3/P cr)
///0.928910.880020.880020.9500950.900090.90009
Temperature(T3)°C//30.0125.3925.3930.0526.3726.37
Quality kg/kg//sup-heat0.96sat.vap sup-heat0.86sat.vap Mixed?uid(state4)Pressure(P c)bar//72.1472.1472.1473.7873.7873.78
Temperature(T4)°C//34.6531.9031.8833.8631.7931.79 Entrainment ratio(u)/// 2.34 2.28 2.25 2.68 2.38 1.95 Pressure ratio(inlet/outlet)in expander/// 1.82 2.05 2.00 1.87 2.17 2.00
5.Performance of the cycles
Total heating load(Q h_sum=Q h1+Q h2)kW112.88119.97163.52150.13146.91163.75153.04149.65 Power output from expander(W out)kW17.4118.1119.2822.4421.7320.1924.4721.99 Pumping power(W pump)kW8.168.679.0911.7711.099.8613.8611.48 Maximum net power output(W net_max)kW9.259.4510.1910.6710.6410.3210.6210.51 Thermal ef?ciency(g th)%8.197.88 6.237.117.24 6.30 6.947.02
6.Increase of the performance compared to TRC
Increase of the W net_max%/ 2.1410.1815.3415.0311.6014.7813.64 Increase of the g th%/à3.89à23.94à13.27à11.62à23.07à15.34à14.28 556X.Li et al./Energy Conversion and Management78(2014)551–558
à23.94%andà23.07%lower than that of the TRC respectively,as shown in Table1.
4.3.Heating load(Q h)
Fig.8presents the variation of the heating load(Q h)with the ?rst-stage heating pressure(P h1).At the same P h1,the heating load in the?rst-stage(Q h1)of the ETRC and ESRC is the same as the heating load in the TRC and SRC respectively,and the Q h1decreases with the P h1.But the heating load in the second-stage(Q h2)of the ETRC and ESRC increases with the P h1,and the total heating load (Q h_sum=Q h1+Q h2)in the ETRC and ESRC increases with P h1.
There exists the maximum of the thermal ef?ciency(g th_max)in the ETRC and ESRC which is different from the TRC and SRC,as shown in Fig.7.The reason is that the Q h in TRC and SRC is decreas-ing throughout but the Q h_sum in ETRC and ESRC is increasing due to the Q h2.With the P h1higher than the optimum P h1_opt which is 122.91bar,128.58bar,124.74bar,131.34bar for TRC,SRC,ETRC and ESRC,as present in Section3.1,the W net decreases.But the negative slope or the decreased tendency of the Q h is higher than that of the W net in the TRC and SRC that the g th of the TRC and SRC does not decrease,but increase.On the contrary,due to the in-crease of the Q h_sum throughout in the ETRC and ESRC,the g th will decrease after the increase that the maximum of the thermal ef?ciency(g th_max)comes out,as shown in Fig.7.
https://www.360docs.net/doc/4e6661917.html,parison of the maximum net power output(W net_max)
The performance of the ETRC and ESRC in the condition of the maximum net power output(W net_max)is calculated with different outlet pressure(P3)of the expander(the pressure drop ratio c at 0.95and0.9),as shown in Table1.The lower the P3(or the c)is, the higher the W net_max and g th of the ETRC and ESRC is.For exam-ple,the W net_max at c of0.9is4.68%in wet expansion and4.40%in dry expansion higher than the W net_max at c of0.95in the ETRC.But the lower the P3(or the c)is,the more possibility the wet expan-sion will occur for the wet working?uid of the CO2.
In summary,an ejector is introduced to the basic cycles of the TRC and SRC to form the ETRC and ESRC.The outlet pressure of the expander in the ETRC and ESRC is decreased or the pressure dif-ference in the expander is increased by the ejector that results in an increase of the net power output of the cycle.The net power output is higher in the ETRC and ESRC compared to the TRC and SRC.
4.5.Effect of the cooling pressure
It is well known that the lower the condensation condition(i.e., the condensation pressure or condensation temperature)is,the higher the thermal performance of the power cycle will be.The optimum calculation reveals that the cooling pressure(P c)in the SRC and ESRC is as lower as possible to close to the critical pressure of the CO2.The P c had to be set to a de?nite value as the reduced parameter of the cooling pressure(P rc)is set to1.0001,as shown in Table1.The lower the cooling pressure(P rc)is,the higher the thermal performance of the W net and g th is,as shown in Fig.9. But the heating pressure(P h1)will increase with the P rc in the SRC and ESRC,as shown in Fig.10.
4.6.Performance(W net_max and g th)with water temperature
Figs.11and12present the maximum net power output (W net_max)and the corresponding thermal ef?ciency(g th)of the TRC,SRC,ETRC and ESRC with CO2at pressure drop ratio(c)of
0.95in water temperature range of60–90°C.The W net_max and
g th of the cycles increase with the water temperature almost line-arly.The thermal performance and the analyses of the cycles at dif-ferent water temperature are similar to the outcomes at water temperature of80°C.The net power output of the cycles could be ranked from high to low:ESRC>ETRC>SRC>TRC;and the thermal ef?ciency could be ranked from high to low: TRC>SRC>ESRC>ETRC in the water temperature range of60–90°C.
5.Conclusions
An ejector is introduced to the basic supercritical or transcritical Rankine cycle(SRC,TRC)to form the supercritical or transcritical Rankine cycle with ejector(ESRC,ETRC).The outlet pressure of the expander in the ETRC and ESRC is decreased or the pressure dif-ference in the expander is increased by the ejector that results in an increase of the net power output of the cycle.
1.The net power output(W net)of the cycles could be ranked from
high to low:ESRC>ETRC>SRC>TRC,and the thermal ef?-ciency(g th)could be ranked from high to low:TRC>SRC>ESR-
C>ETRC with working?uid of CO2at the pressure drop ratio
(c=outlet pressure of expander(P3)/cooling pressure(P c))of
0.95in ETRC and ESRC in water temperature range of60–90°C.
2.The increases of the maximum net power output(W net_max)in
the SRC,ETRC,ESRC is2.14%,10.18%and11.60%compared to the TRC,but the corresponding g th of the SRC,ETRC and ESRC isà3.89%,à23.94%andà23.07%lower than that of the TRC respectively at c of0.95in ETRC and ESRC.
3.The lower the outlet pressure(P3)of the expander is,the higher
the W net_max and g th of the ETRC and ESRC is.In the ETRC,the W net_max at c of0.9is4.68%in wet expansion and4.40%in dry expansion higher than the W net_max at c of0.95.
4.The cooling pressure in the SRC and ESRC by the optimum
calculation demonstrates to be as lower as possible to near the critical pressure of the CO2.
Acknowledgement
The authors gratefully acknowledge the?nancial support from the National Natural Science Foundation of China(Grant No. 51276122).
References
[1]Chen HJ,Goswami DY,Stefanakos EK.A review of thermodynamic cycles and
working?uids for the conversion of low-grade heat.Renew Sust Energy Rev 2010;14(9):3059–67.
[2]Chen HJ,Goswami DY,Stefanakos EK,Rahman MM.A supercritical Rankine
cycle using zeotropic mixture working?uids for the conversion of low-grade heat into power.Energy2011;36(1):549–55.
[3]Gu ZL,Sato H.Optimization of cyclic parameters of a supercritical cycle for
geothermal power generation.Energy Convers Manage2001;42(12):1409–16.
[4]Vetter C,Wiemer HJ,Kuhn https://www.360docs.net/doc/4e6661917.html,parison of sub-and supercritical Organic
Rankine Cycle for power generation from low-temperature/low-enthalpy geothermal wells,considering speci?c net power output and ef?ciency.Appl Therm Eng2013;51(1–2):871–9.
[5]Chen Y,Lundqvist P,Johansson A,Platell P.A comparative study of the carbon
dioxide transcritical power cycle compared with an organic rankine cycle with R123as working?uid in waste heat recovery.Appl Therm Eng2006;26(17–
18):2142–7.
[6]Zhang SJ,Wang HX,Guo T.Performance comparison and parametric
optimization of subcritical organic Rankine cycle(ORC)and transcritical power cycle system for low-temperature geothermal power generation.Appl Energy2011;88(8):2740–54.
[7]Chen HJ,Goswami DY,Rahman MM,Stefanakos EK.Energetic and exergetic
analysis of CO2-and R32-based transcritical Rankine cycles for low-grade heat conversion.Appl Energy2011;88(8):2802–8.
[8]Baik YJ,Kim MS,Chang KC,Kim SJ.Power-based performance comparison
between carbon dioxide and R125transcritical cycles for a low-grade heat source.Appl Energy2011;88(3):892–8.
X.Li et al./Energy Conversion and Management78(2014)551–558557
[9]Baik YJ,Kim MS,Chang KC,Lee YS,Yoon HK.A comparative study of power
optimization in low-temperature geothermal heat source driven R125 transcritical cycle and HFC organic Rankine cycles.Renew Energy 2012;54:78–84.
[10]Kim YM,Kim CG,Favrat D.Transcritical or supercritical CO2cycles using both
low-and high-temperature heat sources.Energy2012;43(1):402–15.
[11]Wang JF,Sun ZX,Dai YP,Ma SL.Parametric optimization design for
supercritical CO2power cycle using genetic algorithm and arti?cial neural network.Appl Energy2010;87(4):1317–24.
[12]Cayer E,Galanis N,Nesreddine H.Parametric study and optimization of a
transcritical power cycle using a low temperature source.Appl Energy 2012;87(4):1349–57.
[13]Vélez F,Chejne F,Antolin G,Quijano A.Theoretical analysis of a transcritical
power cycle for power generation from waste energy at low temperature heat source.Energy Convers Manage2012;60:188–95.
[14]B?y?kog?lu A,Yal??nkaya R.Effects of different reservoir conditions on carbon
dioxide power cycle.Energy Convers Manage2013;72:125–33.
[15]Schuster A,Karellas S,Aumann R.Ef?ciency optimization potential in
supercritical oranic Rankine cycle.Energy2010;35(2):1033–9.
[16]Sarkar J,Bhattacharyya S.Optimization of recompression supercritical-CO2
power cycle with reheating.Energy Convers Manage2009;50(8):1939–45. [17]Le Moullec Yann.Conceptual study of a high ef?ciency coal-?red power plant
with CO2capture using a supercritical CO2Brayton cycle.Energy 2013;49(1):32–46.[18]Sarkar J.Second law analysis of supercritical CO2recompression Brayton cycle.
Energy2009;34(9):1172–8.
[19]Singh R,Miller SA,Rowlands AS,Jacobs PA.Dynamic characteristics of a direct-
heated supercritical carbon-dioxide Brayton cycle in a solar thermal power plant.Energy2013;50(1):194–204.
[20]Singh R,Rowlands AS,Miller SA.Effects of relative volume-ratios on dynamic
performance of a direct-heated supercritical carbon-dioxide closed Brayton cycle in a solar-thermal power plant.Energy2013;55(15):1025–32.
[21]Zhang XR,Yamaguchi H,Uneno D,Fujima K,Enomoto M,Sawada N.Analysis of
a novel solar energy-powered Rankine cycle for combined power and heat
generation using supercritical carbon dioxide.Renew Energy 2006;31(12):1839–54.
[22]Zhang XR,Yamaguchi H,Fujima K,Enomoto M,Sawada N.Theoretical analysis
of a thermodynamic cycle for power and heat production using supercritical carbon dioxide.Energy2007;32(4):591–9.
[23]Song YH,Wang JF,Dai YP,Zhou EM.Thermodynamic analysis of a transcritical
CO2power cycle driven by solar energy with lique?ed natural gas as its heat sink.Appl Energy2012;92:194–203.
[24]Halimi B,Suh https://www.360docs.net/doc/4e6661917.html,putational analysis of supercritical CO2Brayton cycle
power conversion system for fusion reactor.Energy Convers Manage 2012;63:38–43.
[25]Lemmon EW,Huber ML,McLinden MO.NIST reference?uid thermodynamic
and transport properties REFPROP8.In:NIST Standard Reference Database.
vol.23;2007.
558X.Li et al./Energy Conversion and Management78(2014)551–558
Rotork电动执行机构调试步骤
Rotork电动执行机构调试步骤 1设定开关阀门方式 首先将执行机构上的红色旋钮转到停止位置(或就地位置),然后用遥控器进入设定和调整执行器的功能菜单,找到C2(关阀方式) 这个菜单,然后在这个菜单下找到CT(Close on Torque用力矩关闭阀门;Close on Limit 用限位关闭阀门) 这个选项,然后确认,这样关阀的的方式就变为了力矩关。开阀的方式同上,它的菜单为C3,找到OL,然后确认就OK了。 2设定开关阀门的位置 首先将执行机构上的红色旋钮转到就地位置,在设定和调整执行器的功能菜单下找到LC(Set Close Torque Move to Close ) 设定关位菜单,然后顺时针旋动执行机构上的黑色旋钮,阀门会向下运动直到停止,这时用遥控确认一下,这样阀门的关闭位置就定好了。 此时将阀门的行程指示牌和阀门的关闭位置对齐。 再找到LO(Set Open Limit Move to Open)设定开位菜单,然后逆时针旋动执行机构上的黑色旋钮,阀门会向上运动,当阀门移动到行程指示盘的全开位置时旋动执行机构上的黑色旋钮到停止位 置。此时用遥控器确认一下,开位就确定好了。 3锁紧行程螺母 旋动执行机构上的黑色旋钮让阀门运行到全关位置,将行程螺母旋到最下面,然后再往上退三分之一圈,然后锁死就行了。最后将手轮中心密封盖用聚四氟乙烯带密封并完全上紧,确保执行机
构中心套筒内不进水。最后将执行机构上的红色旋钮旋转到远方位置,并用挂锁锁定就行了。 注意事项:电动切手动的时候,把红色旋钮打到停止(stop)位置,然后将手/电动切换手柄切到手动位置,然后操作手轮
表面粗糙度符号
表面粗糙度符号、代号 1. 图样上所标注的表面粗糙度符号、代号是该表面完工后的要求。 2. 表面粗糙度的画法。 3. 有关表面粗糙度的各项规定应按功能要求给定。若仅需要加工(采用去除材 料的方法或不去除材料的方法)但对表面粗糙度的其他规定没有要求时,允许只注表面粗糙度符号。 4. 表面粗糙度参数和各项规定注写的位置。 a1、a2 —— 粗糙度高度参数的允许值(μm ); b —— 加工方法、镀涂或其他表面处理; c —— 取样长度(mm ); d —— 加工纹理方向符号; e —— 加工余量(mm ); f —— 粗糙度间距参数值(mm )或轮廓支撑长度率 5. 图样上表示零件表面粗糙度的符号。 b
6.当允许在表面粗糙度参数的所有实测值中超过规定值的个数少于总数的 16%时,应在图样上标注表面粗糙度参数的上限值或下限值。 7.当要求在表面粗糙度参数的所有实测值中不得超过规定值时,应在图样上 标注表面粗糙度参数的最大值或最小值。 8.表面粗糙度高度参数轮廓算术平均偏差R a值的标注见下表,R a在代号中用 数值表示(单位为微米μm),参数值前可不标注参数代号。
9.表面粗糙度高度参数轮廓微观不平度十点高度R z、轮廓最大高度R y值(单位 为微米μm)的标注见下表,前需标注出相应的参数代号。 10.取样长度应标注在符号长边的横线下面,见图1。 图1 若按GB 10610—1989第6.1条中表1、表2的有关规定选用对应的取样长度时,在图样上可省略标注。 11.若需要标注表面粗糙度间距参数轮廓的单峰平均间距S值、轮廓微观不平 度的平均间距S m值或轮廓支承长度率tp时,应注在符号长边的横线下面,
表面粗糙度符号及意义 (1)
表面粗糙度符号及意义表面粗糙度高度参数的标注 符号意义及说明 R a R z、R y 代号意义 代号 意义 基本符号,表示表面可用任何方法获得。当不加注粗糙度参数值或有关说明(例如:表面处理、局部热处理状况等)时,仅适用于简化代号标注 用任何方法 获得的表面粗 糙度,R a的上限 值为3.2μm 用任何方法获 得的表面粗糙 度,R y的上限值 为3.2μm 用去除材料 方法获得的表 面粗糙度,R a 的上限值为3.2 μm 用不去除材料 方法获得的表面 粗糙度,R z的上 限值为200μm 基本符号加一 短划,表示表面是用去除材料的方法获得。例如:车、铣、钻、磨、剪、切、抛光、腐蚀、电火花加工、气剖等 用不去除材 料方法获得的 表面粗糙度,R a 的上限值为3.2 μm 用去除材料方 法获得的表面粗 糙度,R z的上限 值为3.2μm,下 限值为1.6μm 基本符号加一 小圆,表示表面是用不去除材料的方法获得。例如:铸、锻、冲压变形、热轧、冷轧、粉末冶金等。或者是用于保持原供应状况的表面(包括保持上道工序的状况) 用去除材料 方法获得的表 面粗糖度,R a 的上限值为3.2 μm,R a的下限 值为1.6μm 用去除材料方 法获得的表面粗 糙度,R a的上限 值为3.2μm,R y 的上限值为12.5 μm 用任何方法 获得的表面粗 糙度,R a的最大 值为3.2μm 用任何方法获 得的表面粗糙 度,R y的最大值 为3.2μm 在上述三个符 号的长边上均可加一横线,用于标注有关参数和说明 用去除材料 方法获得的表 面粗糙度,R a 的最大值为3.2 μm 用不去除材料 方法获得的表面 粗糙度,R y的最 大值为200μm
ROTORK电动执行机构参数设置及操作方法
ROTORK电动执行机构参数设置及操作方法 作者:杨潇波 2013年10月22日来源:浏览量:1181 字号:T | T 1结构ROTORKIQ系列电动执行器是对阀门进行就地及远程电动控制的非侵入式自控设备。它由一个电机、减速齿轮、现场控制反转启动器、带电逻辑控制力矩、限位和监视装置组成。 ROTOKIQ系列使用非侵入式手持红外线IQ设定器 1 结构 ROTORK IQ系列电动执行器是对阀门进行就地及远程电动控制的非侵入式自控设备。它由一个电机、减速齿轮、现场控制反转启动器、带电逻辑控制力矩、限位和监视装置组成。ROTOKIQ系列使用非侵入式手持红外线IQ设定器完成对力矩、限位以及一级、二级设定的。IQ的结构如图1所示。 图 1 IQ的结构 2 IQ执行器的操作方法 2.1 手动操作
压下手动/自动手柄,使其处于手动位置。旋转手轮以挂上离合器,此时松开手柄,手柄将自动弹回初始位置,手轮将保持啮合状态,直到执行器被电动操作,手轮将自动脱离,回到电机驱动状态。如果需要,可用一个带6.5mm铁钩的挂锁将离合器锁定在任何状态。 2.2 电动操作 检查电源电压,应与执行器铭牌上的标识相符,然后即可开启电源。无需检查相位。如果没有进行初步检查,则不要进行电气操作,至少要用红外线设定器来完成初级设定。 选择现场/停止/远程操作:红色选择器可选择现场或远程两种操作,当选择器锁定在就地或远程位置时,停止功能仍然有效。选择器也可锁定在停止状态,以防止现场或远程的电动操作。逆时针旋转红色选择器旋钮至现场位置,相邻的黑色旋钮可分别转至开和关的位置;顺时针旋转红色旋钮则停止运行。如果逆时针旋转红色选择器旋钮至远程位置,远程控制只能用于开和关,此时顺时针旋转红色旋钮仍可使执行器停止运行。 3 IQ执行器的功能检查参数设置 3.1 基本参数设定方法 ROTORK IQ系列执行器是全世界首家推出无需打开电气端盖即可进行调试和查询的阀门执行器。当执行器与阀门连接可靠后,接通主电源,并将执行器选择在就地或停止位,使用所提供的红外线设定器进入执行器的设定程序,即可对行程末端的限位方式、力矩值和限位以及其它所有控制和指示功能进行设定。(IQ的设定和调整在执行器主电源接通和断开时均可完成。)设定器的按键名称及作用(图2):
表面粗糙度符号及数值说明[1]
表面粗糙度符号及其标注说明 粗糙度是衡量零件表面粗糙程度的参数,它反映的是零件表面微观的几何形状误差,必须借助放大镜等进行测量。它是由于零件加工过程中刀具与加工表面之间的摩擦、挤压以及加工时的高频振动等方面的原因造成的。表面粗糙度对零件的工作精度、耐磨性、密封性、耐蚀性以及零件之间的配合都有着直接的影响。 粗糙度的评定常用轮廓算术平均偏差Ra、轮廓最大高度Ry、微观不平度十点高度Rz三个参数表示。数值越小,零件的表面越光滑,数值越大零件的表面越粗糙。 1、轮廓算术平均偏差Ra 取样长度:取样长度是指具有粗糙度几何特征的一段长度,在取样长度内应该具有几个波峰和波谷。测量时可选5倍的取样长度作为测量长度进行测量。 Ra是指在取样长度内,轮廓偏距绝对值的算术平均值,可以表示为:
关于表面粗糙度的数值和表面特征、获得方法、应用举例请参见下表。 从上图中也可以看出,粗糙度参数的数值.基本上成倍数的关系。标注时应当选用这些数值,不能选用其他的数值。 2、轮廓最大高度Ry 3、轮廓不平度十点高度Rz
标注 2.1代号及意义 粗糙度代号可以分为:符号,粗糙度项目及数值。 常用标注参数是Ra, 标注Ra时Ra可以省略,标注Rz和Ry时,在粗糙度数值前加对应的符号Rz和Ry。 2.2 标注原则 1)、在同一图样上每一表面只注一次粗糙度代号,且应注在可见轮廓线、尺寸界线、引出线或它们的延长线上,并尽可能靠近有关尺寸线。 2)、当零件的大部分表面具有相同的粗糙度要求时,对其中使用最多的一种,代(符)号,可统一注在图纸的右上角。并加注“其余”二字。 3)、在不同方向的表面上标注时,代号中的数字及符号的方向必须下图的规
L直行程电子式电动执行器说明书
封面
目录 1、产品概述 2、主要技术参数 3、产品型号规格 4、结构与原理 5、安装 6、调整 7、外形尺寸 8、配套支架 9、故障和解决办法 10、订货须知 11、安全使用注意事项 (1)(1)(2)(3)(7)(8)(11)(12)(13)(13)(14)
使用本执行器时,请先认真阅读和理解本说明书,通过正确的使用和维护,充分发挥其效能。 1 产品概述 3810L系列直行程电子式电动执行器有以 220V交流单相电源和 380V三相交流电源两种驱动电源的机型,接受来自调节器控制信号(D C4 ~ 20m A或 D C1 ~ 5V),实现预定直线往复运动的新型执行器。本系列执行器被用作调节阀的执行机构时,几乎具备了调节阀本身所要求的各种动作变换功能以及阀开度信号功能和手动功能。因此被广泛应于发电、冶金、石化、轻工及环保等工业部门。 本系列执行器有以下主要特点: 1.1 执行器设计有伺服系统(无需另配伺服放大器),只需接入D C4 ~ 20m A(或D C1 ~5V)信号和对应的电源即可工作。内设接线端子,接线极为简单方便。 1.2 执行器的关键部件 — 控制器,采用最先进的混合集成电路,用树脂密封浇铸,外形为匣状,体积小,可靠性高。 1.3 驱动量的反馈检测采用高性能导电塑料电位器,分辨率< 0.4%。 1.4 具备自诊断功能,当发生故障时,控制器上的指示灯会立即发出指示信号。 1.5 用状态选择开关可以设定断信号时,(阀芯处于全开,全闭或自锁状态。) 1.6 用状态选择开关可以设定(正、反动作。) 1.7 用状态选择开关可以设定输入信号为(D C4~20m A或D C1~5V。) 1.8 调整工作零点(始点)和行程(终点)简单易行。 1.9 当突然断电时,能确保阀芯自锁。 1.10 采用同步皮带转动,有效降低了噪音。 1.11 延时保护功能,额定负载时,能实行状态自锁,故障发生时,能立即起 动保护,并可反向运行取消延时保护。 2 主要技术参数 2.1 电源 单相A C220V±10%,50H z,三相A C380V±10%,50H z(需订货时注明) 2.2 耗电功率(额定负载时) 规格 A型执行器 50V A; 规格 B 型执行器 150V A; 规格 C 型执行器 220V A。 2.3 输入信号 D c4 ~ 20m A或 D C1 ~ 5V。 1
粗糙度 符 及其表示方法
表面粗糙度符号、代号及其注法 本标准等效采用国际标准ISO1302—1992《技术制图——标注表面特征的方法》。 1主题内容与适用范围 本标准规定了零件表面粗糙度符号、代号及其在图样上的注法。 本标准适用于机电产品图样及有关技术文件。其他图样和技术文件也可参照采用。 2引用标准 GB1031表面粗糙度参数及其数值 GB/T13911金属镀覆和化学处理表示方法 GB3505表面粗糙度术语表面及其参数 GB4054涂料涂覆标记 GB10610触针式仪器测量表面粗糙度的规则和方法 GB12472木制件表面粗糙度参数及其数值 3表面粗糙度符号、代号 3.1图样上所标注的表面粗糙度符号、代号是该表面完工后的要求。 3.2有关表面粗糙度的各项规定应按功能要求给定。若仅需要加工(采用去除材料的方法 或不去除材料的方法)但对表面粗糙度的其他规定没有要求时,允许只注表面粗糙度符号。 3.3图样上表示零件表面粗糙度的符号见表1。 表1 符号意义及说明 基本符号,表示表面可用任何方法获得。当不加注粗糙度参数值或有关 说明(例如:表面处理、局部热处理状况等)时,仅适用于简化代号标注 基本符号加一短划,表示表面是用去除材料的方法获得。例如:车、铣、 钻、磨、剪切、抛光、腐蚀、电火花加工、气割等 基本符号加一小圆,表示表面是用不去除材料的方法获得。例如:铸、 锻、冲压变形、热轧、冷轧、粉末冶金等。 或者是用于保持原供应状况的表面(包括保持上道工序的状况) 在上述三个符号的长边上均可加一横线,用于标注有关参数和说明 在上述三个符号上均可加一小圆,表示所有表面具有相同的表面粗糙度 要求 3.4当允许在表面粗糙度参数的所有实测值中超过规定值的个数少于总数的16%时,应 在图样上标注表面粗糙度参数的上限值或下限值。 当要求在表面粗糙度参数的所有实测值中不得超过规定值时,应在图样上标注表面 粗糙度参数的最大值或最小值。 3.5表面粗糙度高度参数轮廓算术平均偏差R a值的标注见表2,R a在代号中用数值表示(单位为微米),参数值前可不标注参数代号。 表2
表面粗糙度符号及意义
1、表面粗糙度符号及意义 符号 意义 表面粗糙度参数和各项规定注写的位置 基本符号,单独使用这符 号是没有意义的 α1、α2——粗糙度高度参数的允许值(μm ); b ——加工方法、镀涂或其他表面处理; c ——取样长度(mm ); d ——加工纹理方向符号; e ——加工余量(mm ); f ——粗糙度间距参数值(mm )或轮廊支承长度率 基本符号上加一短划,表示表面粗糙度是用去除材料方法获得。例如:车、铣、钻、磨、剪切、抛光、腐蚀、电火花加工等 基本符号加一小圆,表示表面粗糙度是用不去除材 料的方法获得。例如:铸、锻、冲压变形、热轧、冷 轧、粉末冶金等 或者是用于保持原供应状况的表面(包括保持上道工序的状况) 以上三个符号的长边可加一横线,用于标注参数;在长边与横线间可加一小 圆,表示所有表面具有相同的表面粗糙度要求。 2、表面粗糙度高度参数的标注 R a 值 R z ,R y 值 代号 意义 代号 意义 用任何方法获得的表面,R a 的最大允许值为3.2μm 用任何方法获得的表面,R Y 的最大允许值为3.2μm 用去除材料获得的表面, R a 的最大允许值为3.2μm 用不去除材料方法获得的表面,R z 的最大允许值为 200μm 用不去除材料获得的表面,R a 的最大允许值为 3.2μm 用去除材料方法获得的表面,R z 的最大允许值(R zmax )为3.2μm ,最小允许值(R zmin )为1.6μm 用去除材料方法获得的表面,R a 的最大允许值(R amax )为3.2μm ,最小的允许值 (R amin )为1.6μm 用去除材料方法获得的表面,R a 的最大允许值为3.2μm ,R Y 的最大允许值为 12.5μm 3、表面粗糙度符号的画法
罗托克中文说明书
电动执行机构IQ系列 安装和维护手册 出版物编号 E170C2 出版日期 .
Rotork设定器可按现场要求对执行器的控制、指示、保护功能进行设定。所有的执行器在投入使用前,有必要检查其与过程控制系统要求的兼容性。请阅读本手册。 当Rotork工作人员或指定代理商按照合同规定进行现场调试或/和验收时,执行器组态的相关文件应让客户留档备查。
本手册提供如下介绍: * 手动和电动(就地和远程)操作。 * 执行器的准备和安装。 * 根据有关阀门正确操作的要求,对执行器进行初级设定。 * 根据现场具体控制和指示的要求,对执行器进行二级设定。 * 维护 - 故障排除。 * 销售和服务。RotorkIQ系列执行器- 全世界首家推出无需打开电气端盖即可进行调试和查询的阀门执行器。 使用所提供的红外线设定器进入执行器的设定程序,即使在危险区域,也可安全、快捷地对力矩值、限位以及其它所有控制和指示功能进行设定。IQ的设定和调整在执行器主电源接通和断开时均可完成。 标准诊断功能可对控制系统、阀门和执行器的状态进行诊断,并通过执行器的显示屏上的图标和帮助屏幕来显示。 按一下设定器的按键即可在显示屏上对相应阀位的瞬时力矩进行监视。 内置的数据记录器可获取操作和阀门力矩数据,可提醒用户根据需要对阀门进行维护。运行于PC机的IQ Insight软件和/或Rotork本安型通讯器可访问数据记录器,可对执行器的所有功能进行组态和记录。 执行器是否带有设定器,可根据接线端子箱盖上的黄色标签来识别。 有关Rotork IQ和其它系列执行器的资料,可浏览我们的网站。
目录 页码 1 健康与安全 2 2 保存 3 3 IQ系列执行器的操作 3 手动操作 3 电动操作 3 执行器的显示- 4 阀位指示 执行器的显示- 5 报警指示 4 准备驱动轴套7 4.1 IQ7至IQ3 5 7 A和Z型推力底座 4.2 IQ7至IQ35 7 B型非推力底座 4.3 IQ40至IQ95 8 A和Z型推力底座 4.4 IQ40至IQ95 9 B型非推力底座5 执行器的安装 10 提升杆式阀门- 11 顶部安装 带齿轮箱的阀门- 11 侧面安装 非提升杆式阀门- 11 顶部安装 手轮密封 11 IQM调节型执行器 12 IQML线性推力装置 12 IQML线性行程的调整 12 6 接线 14 地线的连接 14 端子箱盖的拆卸 14 电缆入口 14 端子的接线 15 端子箱盖的复位 15 7 设定 16 设定程序 16 设定器 17 进入设定程序 18 设定方式-口令 18 新口令 18 检查方式 18 程序路径分支- 19 分支点 (Cr) 7.8 执行器的显示 19 设定 / 检查方式 返回阀位指示 19 8 设定- 初级设定功能 20 初级设定功能目录 21 9 设定 - 二级设定功能 30 二级设定功能目录 30 10 维护和故障排除 61 帮助显示 63 IrDA诊断和组态 68 11 重量和尺寸 70 二进制、十六进制、十进制 71 换算表
电子式执行机构
一、产品[电子式执行机构]的详细资料: 产品型号:PS 产品名称:电子式执行机构 产品特点:PS系列电子式执行机构,电子式执行机构 PSL、PSQ执行机构是德国PS Automation GmbH执行机构生产商推出的产品。PS系列电子式执行机构可用于对管道的阀门和风门的调节及开与关的控制,其主要特点如下: 二、特点: (1)一体化结构设计,位置变送器和伺服放大器做为两个独立部件均可直接装入执行机构内部,直接接受4-20mA控制信号,输出 4-20mA或1-5VDC阀位反馈信号,具有自诊断功能,使用和调校十分方便。 (2)功能模块式结构设计,通过不同可选功能的组合,实现从简单到复杂的控制,满足不同应用要求。 (3)结构简单,体积小巧,重量轻,便于安装和维护,机械零件全部采用CNC加工制作,工艺精湛。 (4)传动全部采用小齿隙密封齿轮,具有效率高、噪声低、寿命长、稳定可靠、无需再加油等特点。 (5)具有多种运行速度,可满足各种控制系统的要求,以保证系统的快速响应及稳定性。 (6)PSL系列同阀门的连接采用柔性盘簧连接,可避免阀杆与输出轴不同轴给阀门带来的影响,可预置阀门关断力保证阀门的可靠 关断,防止泄漏。 (7)PSQ系列有转矩开关保护,可防止因阀门产生过大转矩而损坏阀杆。 (8)驱动电机采用高性能稀土磁性材料制作的高速同步电机,运行平稳。具有体积小、力矩大、抗堵转、控制精度高等特点。 (9)可设置分段调节,即由一台调节器输出的双时间比例信号控制两台执行机构(4-12mA对应PSL1的全开全闭,12-20mA对应 PSL2的全开全闭)。 (10)阀位反馈元件采用全密封高精度多圈电位器,具有体积小、精度高、死区小、使用寿命长等特点。
表面粗糙度及表面粗糙度的标注方法
一.表面粗糙度的符号 注意:极限值表示参数的实测值中允许少于总数的16%的实测值超过规定值,高度参数常用Ra,在图中标注时常省略。无max min则表示是上极限或下极限,如果有则表示最大值和最小值,单位为微米 基本符号,表示可使用任何方法获得 基本符号加一短划,表示表面用去除材料的方法获得 表示用不去除材料方法获得(铸锻冲压等) 表示所有表面具有相同的表面粗糙度要求 二.表面粗糙度的代号 1. d' =h/10;H=1.4h;h为字体高度 a1、a2--粗糙度高度参数的允许值(mm); b加工方法、镀涂或其他表面处理; c取样长度(mm); d加工纹理方向符号; e加工余量(mm); f粗糙度间距参数值(mm)或轮廊支承长度率。 2.零件的加工表面的粗糙度要求由指定的加工方法获得,用文字标注在符号上边的横线,加工方法也可在图样的技术要求中说明 3.加工纹理方向: = 纹理平行于标注符号的视图的投影面 ⊥纹理垂直于标注符号的视图的投影面 x 纹理呈两相交的方向 M 纹理呈多方向 c 纹理呈近似同心圆 R 纹理呈近似的放射状 p 纹理无方向或凸起的细粒状 4.加工余量:注在符号的左侧,标注时数值要加上括号,单位为毫米 5.参数S Sm Tp l的标注,应标注在符号长边的横线下面,并且必须在参数值前注写参数的符号 三。表面粗糙度符号、代号在图样上的标注 一般标注在可见轮廓线、尺寸界线、引出线或它们的延长线上,符号的尖端必须从材料外指向表面,代号中数字及符号的注写方向必须与尺寸数字方向一致
标准规定在同一图样上,每一表面一般只标注一次。当零件的大部分表面具有相同的表面粗糙度要求时,对其中使用最多的一种代号可以统一注在图样的右上角,并加注“其余”两字当零件所有表面具有相同的表面粗糙度要求时,其代号可在图样的右上角统一标注序号标注规定及说明图例 1当零件的大部分表面具有相同的表由粗糙度要求时,对其中使用最多的一种代(符)号可统一注在图样的右上角,并加注‘其余”两字,且应是图样上其它代(符)号高度的1.4倍 2 代号中数字注写方向应与尺寸数字方向一致;倾斜表面的代号及数字标控方向应符合图右规定 3 带有横线的表面粗糙度应按右图方式标注
机械制图表面粗糙度符号1
机械制图表面粗糙度符号、代号及其注法 浏览22742发布时间10/09/11表面粗糙度符号、代号及其注法 Mechanical drawings— Surface roughness symbols and methods of indicating 1993-11-09 批准1994-07-01 实施 国家质量技术监督局发布 本标准等效采用国际标准ISO 1302—1992《技术制图——标注表面特征的方法》。 1 主题内容与适用范围 本标准规定了零件表面粗糙度符号、代号及其在图样上的注法。 本标准适用于机电产品图样及有关技术文件。其他图样和技术文件也可参照采用。 2 引用标准 GB 1031 表面粗糙度参数及其数值 GB/T 13911 金属镀覆和化学处理表示方法 GB 3505 表面粗糙度术语表面及其参数 GB 4054 涂料涂覆标记 GB 10610 触针式仪器测量表面粗糙度的规则和方法 GB 12472 木制件表面粗糙度参数及其数值 3 表面粗糙度符号、代号 3.1图样上所标注的表面粗糙度符号、代号是该表面完工后的要求。 3.2有关表面粗糙度的各项规定应按功能要求给定。若仅需要加工(采用去除材料的方法或不去除材料的方法)但对表面粗糙度的其他规定没有要求时,允许只注表面粗糙度符号。 3.3图样上表示零件表面粗糙度的符号见表1。 表1 符号意义及说明 基本符号,表示表面可用任何方法获得。当不加注粗糙度参 数值或有关说明(例如:表面处理、局部热处理状况等)时,仅
适用于简化代号标注 基本符号加一短划,表示表面是用去除材料的方法获得。例如:车、铣、钻、磨、剪切、抛光、腐蚀、电火花加工、气割等 基本符号加一小圆,表示表面是用不去除材料的方法获得。例如:铸、锻、冲压变形、热轧、冷轧、粉末冶金等。 或者是用于保持原供应状况的表面(包括保持上道工序的状况) 在上述三个符号的长边上均可加一横线,用于标注有关参数和说明 在上述三个符号上均可加一小圆,表示所有表面具有相同的表面粗糙度要求
罗托克电动阀培训讲义
罗托克电动阀培训稿 目前我公司的罗托克电动阀主要使用的是IQ系列的产品其执行器型号有IQ10,IQ12,IQ18,IQ20,IQ25,IQ35, IQ40, IQ70, IQ90, IQ91,IQ95等。 (1)罗托克的操作: 1.手动操作:压下手动/自动手柄,使其处于手动位置。旋转手轮以挂上离合器,此时松开手柄,手柄将自动弹回初始位置,手轮将保持啮合状态,直到执行器被电动操作,手轮将自动脱离,回到电机驱动状态。 2.电动操作:检察电源电压,应与执行器铭牌上的标识相符,然后即可开启电源。无需检查相位。如果没有进行初步检查,则不要进行电气操作,至少要用红外线设定器来完成初级设定。选择现场/停止/远程操作:红色选择器可选择现场或远程两种操作。当选择器锁定在就地或远程位置时,停止功能仍然有效。选择器也可锁定在停止状态,以防止现场或远程的电动操作。顺时针旋转红色选择器旋钮至现场位置,相邻的黑色旋钮可分别转至开和关的位置;逆时针旋转红色旋钮则停止运行。逆时针旋转红色选择器旋钮至远程位置,远程控制只能用于开和关,此时顺时针旋转红色旋钮仍可使执行器停止运行。 (2)罗托克指示灯: 1.红色指示灯表示阀门打开, 2.黄色指示灯黄灯表示在中间(就是在运行过程中), 3.绿色指示灯表示阀门关闭,液晶显示屏有两种阀位指示方式:1.阀位一动力电源接通,动力电源接通后,执行器液晶显示屏的淡黄色背景灯和一个指示阀位的指示灯也将点亮,显示屏上可看到阀门打开的百分数或表示行程末端的符号。2.阀位一动力电源断开。由黄色指示灯和百分比开度值表示主电源关闭后,液晶显示器由电池供电,继续显示执行器的阀位。无论何种情况,电池都不向背景灯和阀位指示灯供电。
直行程电子式电动执行器
直行程电子式电动执行器 381(361)系列直行程(381L)及角行程(381R),是以AC220单相交流电为电源,接受来自DCS、PLC系统和其他操作器的DC4~20mA,标准信号来控制执行器进行开关阀门或自动调节。电子控制模块采用最先进的混合集成电路,包含完整的保护功能,可现场控制或远程控制,被设计成匣子形式,并用树脂浇铸密封。驱动反馈量采用高性能导电塑料电位器、精度高、寿命长等特点,可与各种角行程调节机构组合成高性能、高可靠性的电动调节阀。因此被广泛用于冶金、发电、石化、造纸、轻工及环保等工业部门。 执行器主要特点: 1、执行器设计有伺服系统(无需另配伺服放大器),只需接入DC4~20mA(或DC1~5V)信号和AC220V单相电源即可工作。内设接线端子,接线极为简单方便。 2、执行器的关键部件─控制器,采用最先进的混合集成电路,用树脂密封浇铸,外形为匣状,体积小,可靠性高。 3、驱动量的反馈检测采用高性能导电塑料电位器,分辨率<0.4%。 4、具备自诊断功能,当发生故障时,控制器上的指示灯会立即发出指示信号。 5、用状态选择开关可以设定断信号时,(阀芯处于全开,全闭或自锁状态)。 6、用状态选择开关可以设定(正、反动作)。 7、用状态选择开关可以设定输入信号为(DC4~20mA或DC1~5V)。 8、调整工作零点(始点)和行程(终点)简单易行。 9、当突然断电时,能确保阀芯自锁。 10、采用同步皮带传动,有效降低了噪音。
11、延时保护功能,额定负载时,能实行状态自锁,故障发生时,能立即起动保护,并可反向运行取消延时保护。 技术参数: 电源:AC220±50%,50HZ。 耗电功率(额定负载时) 规格A型执行器50VA; 规格B型执行器150VA; 规格C型执行器220VA。 输入信号:DC4~20mA或DC1~5V。 输出信号:DC4~20mA(负载电阻500Ω以下)。 控制精度:基本误差:±1% 回差≤1% 死区≤1% 工作行程调整范围:“零点”±25%“行程”20%~100% 外部配线:输入输出信号应采用屏蔽电缆。电源线不得与信号线共用一根电缆。
PS系列电子式执行机构
PS系列电子式执行机构 一、特点 PSL、PSQ执行机构是德国PS Automation GmbH执行机构生产商推出的产品。PS系列电子式执行机构可用于对管道的阀门和风门的调节及开与关的控制,其主要特点如下: (1)一体化结构设计,位置变送器和伺服放大器做为两个独立部件均可直接装入执行机构内部,直接接受4-20mA控制信号,输出4-20mA或1-5VDC阀位反馈信号,具有自诊断功能,使用和调校十分方便。 (2)功能模块式结构设计,通过不同可选功能的组合,实现从简单到复杂的控制,满足不同应用要求。 (3)结构简单,体积小巧,重量轻,便于安装和维护,机械零件全部采用CNC加工制作,工艺精湛。 (4)传动全部采用小齿隙密封齿轮,具有效率高、噪声低、寿命长、稳定可靠、无需再加油等特点。 (5)具有多种运行速度,可满足各种控制系统的要求,以保证系统的快速响应及稳定性。 (6)PSL系列同阀门的连接采用柔性盘簧连接,可避免阀杆与输出轴不同轴给阀门带来的影响,可预置阀门关断力保证阀门的可靠关断,防止泄漏。 (7)PSQ系列有转矩开关保护,可防止因阀门产生过大转矩而损坏阀杆。 (8)驱动电机采用高性能稀土磁性材料制作的高速同步电机,运行平稳。具有体积小、力矩大、抗堵转、控制精度高等特点。 (9)可设置分段调节,即由一台调节器输出的双时间比例信号控制两台执行机构(4-12mA对应PSL1的全开全闭,12-20mA对应PSL2的全开全闭)。 (10)阀位反馈元件采用全密封高精度多圈电位器,具有体积小、精度高、死区小、使用寿命长等特点。 (11)行程可调,便于与阀门连接。 (12)全部电器元件均采用世界名牌产品,质量可靠,使用寿命长。 (13)电器部件布线严谨并与传动部件完全隔离,提高了执行机构运行性的可靠。 二、产品结构 PS系列执行机构电器部件布线严谨并与传动部件完全隔离,提高了执行机构运行的可靠性,传动部件采用CNC加工的小齿隙密封齿轮传动,具有效率高、噪音低、寿命长、稳定可靠,不会因工艺环境,如介质入口力的波动而造成执行机构共振等特点。 1.PSL直行程执行机构 PSL直行程执行机构主要是由相互隔离的电器控制部分和齿轮传动部分组成。电机作为连接两隔离部分的中间部件,电机按控制要求输出转矩,通过多极正齿轮传递到梯形丝杆上,丝杆通过杆螺纹变换转矩为推力。此杆螺纹能自锁,并且将直线行程通过与阀门的适配器传递到阀杆。执行机构出轴带有一个防止转动的止转销,出轴的径向锁定装置可以作为位置指示器。锁定装置连有一个连杆,连杆随输出轴同步运行,通过与连杆连接的线路板将输出轴位移转换成电信号,提供给伺服放大器做为比较信号和阀位反馈输出,同时执行机构的行程也可由开关板上的两个主限位开关来限制,并由两个机械限位档块来调整限定位置。 2.PSQ角行程执行机构 PSQ角行程执行机构主要是由相互隔离的电器控制部分和齿轮传动部分组成,电机做为连接两隔离部分的中间部件。电机转矩通过主齿轮传送到行星齿轮,主齿轮驱动行星齿轮带动中空的齿轮。中空齿轮比二级齿轮少三个齿,因此可以由行星齿轮驱动,行星齿轮转一周中空齿轮转动三个齿,行星齿轮的外部始终啮合一个蜗杆齿轮,运行时也不分开,这种设计使电机在故障或阀门转矩意外增加时可直接通过手轮进行手动控制。 执行机构有两个螺纹止档机构限位,也有两限位开关来实现电限位,每个转向的行程范围可以通过限位开关的位置来控制,阀门开度由一个凸起的显示器指示,独特的转矩开关可调整从50%-100%的最大转矩,实现转矩保护,以防止阀门被卡死时造成的阀杆损坏。
机械制图_表面粗糙度符号分析
表面粗糙度符号、代号及其注法 Mechanical drawings— Surface roughness symbols and methods of indicating 1993-11-09 批准 1994-07-01 实施 国家质量技术监督局发布 本标准等效采用国际标准ISO 1302—1992《技术制图——标注表面特征的方法》。 1 主题内容与适用范围 本标准规定了零件表面粗糙度符号、代号及其在图样上的注法。 本标准适用于机电产品图样及有关技术文件。其他图样和技术文件也可参照采用。 2 引用标准 GB 1031 表面粗糙度参数及其数值 GB/T 13911 金属镀覆和化学处理表示方法 GB 3505 表面粗糙度术语表面及其参数 GB 4054 涂料涂覆标记 GB 10610 触针式仪器测量表面粗糙度的规则和方法 GB 12472 木制件表面粗糙度参数及其数值 3 表面粗糙度符号、代号 3.1图样上所标注的表面粗糙度符号、代号是该表面完工后的要求。 3.2有关表面粗糙度的各项规定应按功能要求给定。若仅需要加工(采用去除材料的方法或不去除材料的方法)但对表面粗糙度的其他规定没有要求时,允许只注表面粗糙度符号。 3.3图样上表示零件表面粗糙度的符号见表1。 表1
3.4当允许在表面粗糙度参数的所有实测值中超过规定值的个数少于总数的16%时,应在图样上标注表面粗糙度参数的上限值或下限值。 当要求在表面粗糙度参数的所有实测值中不得超过规定值时,应在图样上标注表面粗糙度参数的最大值或最小值。 3.5表面粗糙度高度参数轮廓算术平均偏差R a值的标注见表2,R a在代号中用数值表示(单位为微米),参数值前可不标注参数代号。 表2 3.6表面粗糙度高度参数轮廓微观不平度十点高度R z、轮廓最大高度R y值(单位为微米)的标注见表3,参数值前需标注出相应的参数代号。 表3 3.7取样长度应标注在符号长边的横线下面,见图1。
电动执行机构
SURPASS 5 PROFITRON系列变频智能型电动执行机构 一.SURPASS 5 PROFITRON电动执行机构的分类 1、根据机械接口的不同,SURPAss 5 PROFITRoN被分为三大类: ●多回转电动执行机构 执行机构输出轴的转动必须大于360。,才能完成被控对象的全行程。 ●直行程电动执行机构 执行机构输出轴的运动为直线运动(而不是转动)。 ●角行程电动执行机构 执行机构输出轴的转动小于360。 (通常为90。),就可完成被控对象的全行程;根据所配减速箱的不同,角行程执行机构又可分为两种:a.直连式:通过法兰和输出轴与阀门实现机械连接。B.底座曲柄式:安装在基座上,通过曲柄与被控对象实现机械连接。 2、根据控制模式的不同,一般电动执行机构被分为三大类: ●开关型电动执行机构 要么处于全开位置,要么处于全关位置的执行机构。且在两次启动之间有足够的停止时间,以使电机冷却至正常温度。启动次数通常不超过60次/小时。 ●介于开关型和调节型之间的电动执行机构 可以处于全开位置、全关位置或二者之间的任意位置的执行机构。启动次数可达600次/小时。 ●调节型电动执行机构 处于频繁动作状态的执行机构。启动次数可达。1200次/小时。 每一台SURPAss 5 PROFITRON新一代智能型电动执行机构的三种控制模式全部具备(开关型为DC 12V低电平或无源干接点控制)! 二.SURPASS 5 PROFITRON系列智能型电动执行机构的主要技术和性能特点 1.汉显菜单人机对话 suRPAss 5 PROFITRON新一代智能型电动执行机构采用了液晶显示、全中文操作界面,极具人性化!"设置向导"将以人机对话方式引导您轻松完成整个参数设置过程。 2.功能强大而结构简单 设计巧妙的控制系统与独特的应用软件相结合,保证了电动执行机构具有极其优越的性能:功能强大而结构简单,把以往只有通过机械零部件才能实现的部分功能被整合到电子控制系统中,机械结构得以简化,控制精度和工作可靠性却得以提高。符合"简洁至上"的设计理念。 3.宽范围力矩设定 同一台智能型电动执行机构,其关断力矩可在最大输出力矩的30%至100%范围内由用户自行设定(分8档拨码设置)。因此,在设计选型时,多台执行器只需选一种型号。一方面,简化选型和调试;另一方面,可以减少备品和备件的数量,从而减少库存。 4.电子式限位精确可靠 suRPASS 5 PROFITRoN新一代智能型电动执行机构淘汰了传统的机械式限位开关和力矩开关,具备电子式限位保护和电子式过力矩保护功能。通过信号单元和精密的塑导电位器,可精密地检测阀门所处的位置。一旦到达极限位置,cPu控制无刷电机驱动器立即切断电机电源,从而实现限位保护。并向控制系统输出开、关位提示信号(无源干接点信号)。通过检测驱动器供给电机的电流和电压,可测量执制系统输出报警信号(无源干接点信号)。一方面,不用象传统执行机构那样需对限位开关和力矩开关进行复杂的调整,使现场调试变得行机构的实际工作力矩,一旦达到事先设定好的关断力矩,CPU控制无刷电机驱动器立即切断电机电源,从而实现过力矩保护。并向控极为简单;另一方面,因无机械联动机构,故无磨损、无偏移,控制精确而无需维护. 5.柔性启动和柔性到达极限位置 SURPASS 5 PROFITRoN在远程调节控制状态下,在每次启动瞬间,执行机构都会自动以最低转速启动,既具备"柔性启动"功能。启动电流永远都不会超过电机的额定电流,此功能对于启动频繁的调节阀来说具有极其重要的意义:减少了温升,延长电机使用寿命,提高了可靠性。假如执行机构在开、关阀门时,以全速驱动阀门直到碰到阀座,虽然可以实现一个被称作 "关紧"的功能,但代价是对阀门的动态负荷很大。在力矩检测和实际关断电机之问的时问延迟段内,电机仍然会全力以赴,即使当电机在电气上被断开以后,电机中储存的动态能量仍将继续增加过载力矩,这无疑将对阀门造成机械损伤。而SURPASS 5 PROFITRON新一代智能型电动执行机构在运行到接近"全开"和"全关"两个极限位置时,会自动以最低的速度运行,可避免因惯性造成的冲击力矩对阀门的冲撞。因此,阀门、阀座、密封及其它机械部件都受到了精心呵护,延长了使用寿命。 6.轻松设定关方向 可轻松设定执行机构的关方向,无需开盖调整接线,适应任何现场需求。 7."曲线式"运行速度 在远程调节控制状态下,当给定信号与阀位信号差值较大(连续运行区段较长,大于全行程的5%时),执行机构将以低速一高速一低速的"曲线式"运行。较好的解决了运行速度与定位精度的矛盾,从而实现控制过程最优化 8.自动检测和处理信号故障 在自动调节控制模式下,执行器会自动检测4~20mA模拟控制信号回路是否正常,如出现断路、短路等故障,执行器会自动按事先设定好(运行至全开、保持原位、运行至全关)的模式采取保护措施。 9.采用直流无刷电机作驱动 执行机构的动力源选用了性能极其优越的直流无刷电机,不仅具有体积小、重量轻、惯量小(有无源磁能制动特性);而且具有最优越的调速性能(优于变频调速),主要表现在调速范围宽、低速性能好(启动转矩大、启动电流小)、运行平衡、噪音低、效率高等方面。目前无刷直流电机已广泛应用于数控机床的进给驱动、机器人的伺服驱动、电脑硬盘驱动、以及新一代家用电 器的变速驱动中。 10.内部射频干扰过滤器 执行机构内部集成了射频干扰过滤器,将驱动器可能造成对供电电源的干扰以及可能会出现的供电电源对内部 整个控制系统的干扰进行双向隔离,以确保安全使用。 11.整体结构采用模块化 SURPASS 5 PROFITRON智能化控制单元由两块标准的电路板组成:带驱动器的电源板和带微处理器的主控板。如有故障,在更换备件时快速而又简便。 12."非侵入式"无贯通设计 采用"非侵入式"键控(面板操作按钮与电子舱体无贯通)设计,各连接口均采取密封处理措施,确保执行机构 具有较高的防水、防尘特性,SLIRPASS 5 PROFITRON外壳的防护等级为IP67。 13.可分体安装 如现场安装空间不够、现场温度过高、现场振动过大或安装现场不便于调试,则可将智能控制单元(电子舱)和机械部分分开安装。既可解决以上问题,又丝毫不影响执行器的性能。 14.优质铝合金外壳 本执行机构的外部箱体材质为特制铝合金,具有重量轻、强度高、防腐性能好等优点。外部所采用的螺钉均为不锈钢材质。无需喷漆即可在工厂一般的环境中使用。即使防护漆表面出现划痕,也不会导致严重的裂隙腐蚀现象。 三.SURPASS 5 PROFITRON电动执行机构功能概述 ◎模拟量控制信号 DC 0~10mA、4~20mA 输入电阻200Ω、100Ω; DC 1~5V 输入电阻30KΩ; DC 0~10V 输入电阻60KΩ ◎低电平开关量控制信号(共负极、带隔离)
电动执行器故障维修-电子式电动执行器
电动执行器故障维修 一.指示灯故障 1. 故障现象:给电动执行机通电后发现电源指示灯不亮,伺放板无反馈,给信号不动作。 故障判断和检修过程:因电源指示灯不亮,首先检查保险管是否开路,经检查保险管完好,综合故障现象,可以推断故障有可能发生在伺放板的电源部分,接着检查电源指示灯,用万用表检测发现指示灯开路,更换指示灯故障排除。 结论:电源指示灯开路会造成整个伺放板不工作。 2.故障现象:(调试中发现)电动执行器的执行机构通电后,给信号开可以,关不动作。 故障判断和检修过程:先仔细检查反馈线路,确认反馈信号无故障,给开信号时开指示灯亮,说明开正常,给关信号时关指示灯不亮,说明关可控硅部分有问题,首先检查关指示灯,用万用表检测发现关指示灯开路,将其更换后故障排除。 结论:关和开指示灯不亮(开路)时可控硅不动作。 二.电阻电容
1.故障现象:PSL210 执行机构通电后,给定一个信号(例75%),执行机构会全开到底,然后回到指定位置(75%)。 故障判断和检修过程:根据以上故障现象,首先要判断是伺放板和执行机构那一个有问题。将伺放板从执行机构上拆下,直接将电源线接到X5/1和X5/4端子上,执行机构关方向动作,将电源线接到X5/1和X5/2端子上,执行机构开方向动作,如果执行机构动作不正常,说明故障在执行器上。用万用表测电机绕组正常,再测电容两边的电阻发现有一个开路,将其更换后故障排除。 结论:遇到以上故障现象时,首先要判断故障发生在那一个部分上,最后确定根源。 2. 故障现象:执行机构通电后给关信号(4mA)执行机构先全开后再全关。 故障判断和检修过程:先拆除伺放板,直接给执行机构通电发现仍然存在原故障,检查电阻,电阻阻值正常,说明电阻没问题,检查电机绕组,发现阻值正常,电机没问题。由此故障推断有可能电容坏,重新更换电容,故障排除。 结论:出现该问题时首先怀疑电阻和电容。
粗糙度符号及意义
1、表面粗糙度的概念 零件加工时,由于刀具在零件表面上留下的刀痕及切削时金属表面的塑性变形等影响,使零件存在着间距较小的轮廓峰谷。这种表面上具有较小间距的峰谷所组成的微观几何形状特性,称为表面粗糙度。 表面粗糙度是衡量零件质量的标志之一,它对零件的配合、耐磨性、抗腐蚀性、密封性和外观都有影响。因此,零件表面的粗糙度的要求与使用要求、加工方法、加工成本紧密相关,因此,在满足使用要求的前提下,合理选用表面粗糙度参数。 2、表面粗糙度的参数 目前,在生产中评定零件表面质量的主要参数是使用轮廓算术平均偏差Ra。它是在取样长度l(用于判别具有表面粗糙度特征的一段基准线长度)内,轮廓偏距y(表面轮廓上的点至基准线的距离)绝对值的算术平均值。 Ra的取值及取样长度见表1。 表1 Ra及l, ln选用值 有时,还使用Rz(微观不平度十点高度)和Ry(轮廓最大高度)。 3、表面粗糙度的标注方法 (1)表面粗糙度代号 图样上表示零件表面的粗糙度符号的画法如图1所示。 图1 表面粗糙度符号的比例 图样上表示零件表面粗糙度的符号如表2。
表2 表面粗糙度符号 (2)表面粗糙度代号的标注方法 代号和参数的注写方向如图2所示。当零件大部分表面具有相同的表面粗糙度时,对其中使用最多的一种符号、代号可统一标注在图样的右上角,并加注“其余”两字,统一标注的代号及文字高度,应是图形上其它表面所注代号和文字的1.4倍。 图2 表面粗糙度代号的基本标注方法 不同位置表面代号的注法,符号的尖端必须从材料外指向表面,代号中数字的方向与尺寸数字方向一致,如图3所示。 图3 不同位置表面上表面粗糙度代号的标注