临床应用 (tDCS) Challenges and future directions
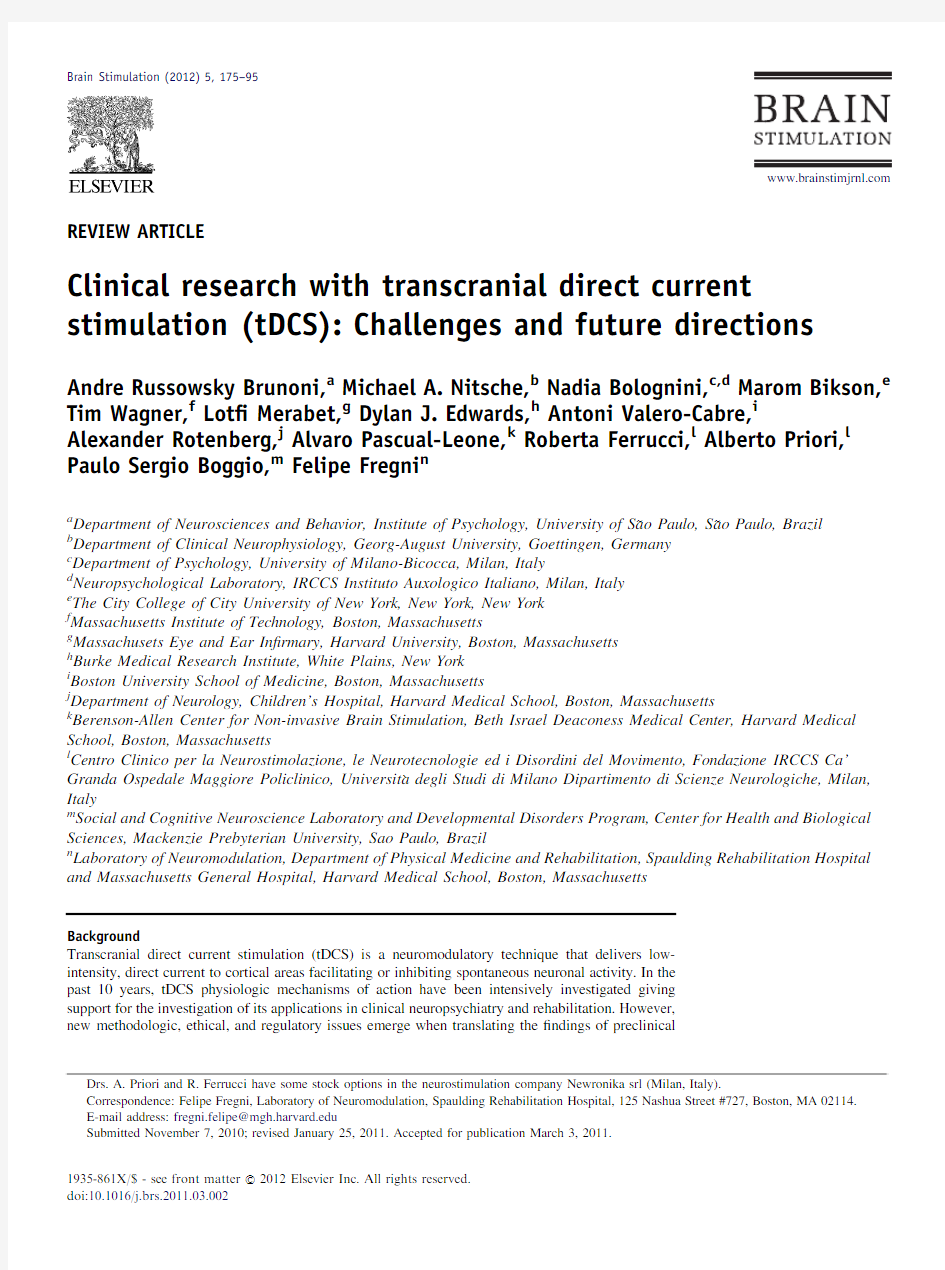
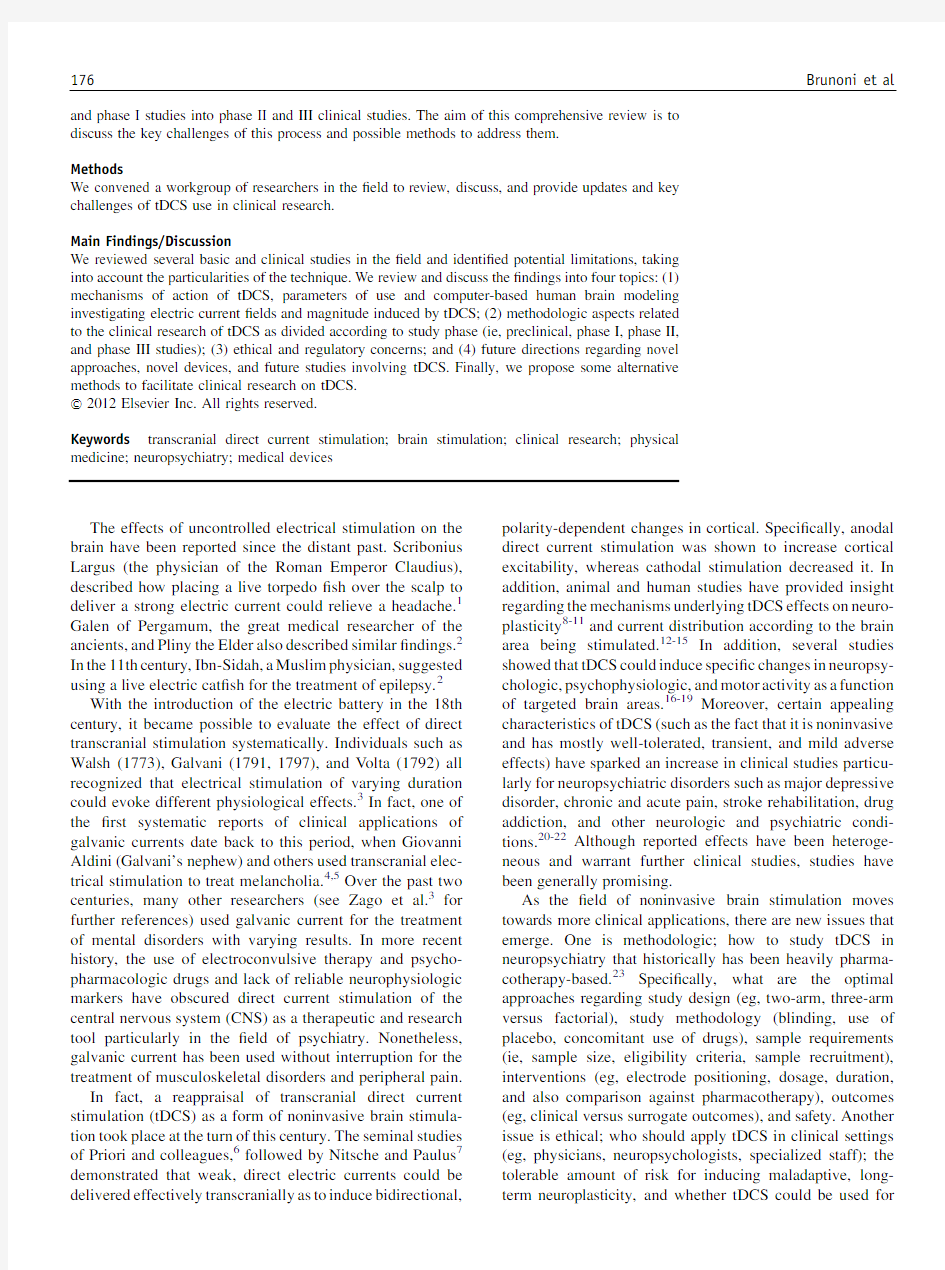
REVIEW ARTICLE
Clinical research with transcranial direct current stimulation (tDCS):Challenges and future directions
Andre Russowsky Brunoni,a Michael A.Nitsche,b Nadia Bolognini,c,d Marom Bikson,e Tim Wagner,f Lot?Merabet,g Dylan J.Edwards,h Antoni Valero-Cabre,i
Alexander Rotenberg,j Alvaro Pascual-Leone,k Roberta Ferrucci,l Alberto Priori,l Paulo Sergio Boggio,m Felipe Fregni n
a
Department of Neurosciences and Behavior,Institute of Psychology,University of S ~a o Paulo,S ~a o Paulo,Brazil b Department of Clinical Neurophysiology,Georg-August University,Goettingen,Germany c
Department of Psychology,University of Milano-Bicocca,Milan,Italy d
Neuropsychological Laboratory,IRCCS Instituto Auxologico Italiano,Milan,Italy e
The City College of City University of New York,New York,New York f
Massachusetts Institute of Technology,Boston,Massachusetts g
Massachusets Eye and Ear In?rmary,Harvard University,Boston,Massachusetts h
Burke Medical Research Institute,White Plains,New York i
Boston University School of Medicine,Boston,Massachusetts j
Department of Neurology,Children’s Hospital,Harvard Medical School,Boston,Massachusetts k
Berenson-Allen Center for Non-invasive Brain Stimulation,Beth Israel Deaconess Medical Center,Harvard Medical School,Boston,Massachusetts l
Centro Clinico per la Neurostimolazione,le Neurotecnologie ed i Disordini del Movimento,Fondazione IRCCS Ca’Granda Ospedale Maggiore Policlinico,Universit a degli Studi di Milano Dipartimento di Scienze Neurologiche,Milan,Italy m
Social and Cognitive Neuroscience Laboratory and Developmental Disorders Program,Center for Health and Biological Sciences,Mackenzie Prebyterian University,Sao Paulo,Brazil n
Laboratory of Neuromodulation,Department of Physical Medicine and Rehabilitation,Spaulding Rehabilitation Hospital and Massachusetts General Hospital,Harvard Medical School,Boston,Massachusetts
Background
Transcranial direct current stimulation (tDCS)is a neuromodulatory technique that delivers low-intensity,direct current to cortical areas facilitating or inhibiting spontaneous neuronal activity.In the past 10years,tDCS physiologic mechanisms of action have been intensively investigated giving support for the investigation of its applications in clinical neuropsychiatry and rehabilitation.However,new methodologic,ethical,and regulatory issues emerge when translating the ?ndings of preclinical
Drs.A.Priori and R.Ferrucci have some stock options in the neurostimulation company Newronika srl (Milan,Italy).
Correspondence:Felipe Fregni,Laboratory of Neuromodulation,Spaulding Rehabilitation Hospital,125Nashua Street #727,Boston,MA 02114.E-mail address:fregni.felipe@https://www.360docs.net/doc/571179832.html,
Submitted November 7,2010;revised January 25,2011.Accepted for publication March 3,2011.1935-861X/$-see front matter ó2012Elsevier Inc.All rights reserved.doi:10.1016/j.brs.2011.03.002
Brain Stimulation (2012)5,175–95
https://www.360docs.net/doc/571179832.html,
and phase I studies into phase II and III clinical studies.The aim of this comprehensive review is to discuss the key challenges of this process and possible methods to address them.
Methods
We convened a workgroup of researchers in the?eld to review,discuss,and provide updates and key challenges of tDCS use in clinical research.
Main Findings/Discussion
We reviewed several basic and clinical studies in the?eld and identi?ed potential limitations,taking into account the particularities of the technique.We review and discuss the?ndings into four topics:(1) mechanisms of action of tDCS,parameters of use and computer-based human brain modeling investigating electric current?elds and magnitude induced by tDCS;(2)methodologic aspects related to the clinical research of tDCS as divided according to study phase(ie,preclinical,phase I,phase II, and phase III studies);(3)ethical and regulatory concerns;and(4)future directions regarding novel approaches,novel devices,and future studies involving tDCS.Finally,we propose some alternative methods to facilitate clinical research on tDCS.
ó2012Elsevier Inc.All rights reserved.
Keywords transcranial direct current stimulation;brain stimulation;clinical research;physical medicine;neuropsychiatry;medical devices
The effects of uncontrolled electrical stimulation on the brain have been reported since the distant past.Scribonius Largus(the physician of the Roman Emperor Claudius), described how placing a live torpedo?sh over the scalp to deliver a strong electric current could relieve a headache.1 Galen of Pergamum,the great medical researcher of the ancients,and Pliny the Elder also described similar?ndings.2 In the11th century,Ibn-Sidah,a Muslim physician,suggested using a live electric cat?sh for the treatment of epilepsy.2 With the introduction of the electric battery in the18th century,it became possible to evaluate the effect of direct transcranial stimulation systematically.Individuals such as Walsh(1773),Galvani(1791,1797),and V olta(1792)all recognized that electrical stimulation of varying duration could evoke different physiological effects.3In fact,one of the?rst systematic reports of clinical applications of galvanic currents date back to this period,when Giovanni Aldini(Galvani’s nephew)and others used transcranial elec-trical stimulation to treat melancholia.4,5Over the past two centuries,many other researchers(see Zago et al.3for further references)used galvanic current for the treatment of mental disorders with varying results.In more recent history,the use of electroconvulsive therapy and psycho-pharmacologic drugs and lack of reliable neurophysiologic markers have obscured direct current stimulation of the central nervous system(CNS)as a therapeutic and research tool particularly in the?eld of psychiatry.Nonetheless, galvanic current has been used without interruption for the treatment of musculoskeletal disorders and peripheral pain.
In fact,a reappraisal of transcranial direct current stimulation(tDCS)as a form of noninvasive brain stimula-tion took place at the turn of this century.The seminal studies of Priori and colleagues,6followed by Nitsche and Paulus7 demonstrated that weak,direct electric currents could be delivered effectively transcranially as to induce bidirectional,polarity-dependent changes in cortical.Speci?cally,anodal direct current stimulation was shown to increase cortical excitability,whereas cathodal stimulation decreased it.In addition,animal and human studies have provided insight regarding the mechanisms underlying tDCS effects on neuro-plasticity8-11and current distribution according to the brain area being stimulated.12-15In addition,several studies showed that tDCS could induce speci?c changes in neuropsy-chologic,psychophysiologic,and motor activity as a function of targeted brain areas.16-19Moreover,certain appealing characteristics of tDCS(such as the fact that it is noninvasive and has mostly well-tolerated,transient,and mild adverse effects)have sparked an increase in clinical studies particu-larly for neuropsychiatric disorders such as major depressive disorder,chronic and acute pain,stroke rehabilitation,drug addiction,and other neurologic and psychiatric condi-tions.20-22Although reported effects have been heteroge-neous and warrant further clinical studies,studies have been generally promising.
As the?eld of noninvasive brain stimulation moves towards more clinical applications,there are new issues that emerge.One is methodologic;how to study tDCS in neuropsychiatry that historically has been heavily pharma-cotherapy-based.23Speci?cally,what are the optimal approaches regarding study design(eg,two-arm,three-arm versus factorial),study methodology(blinding,use of placebo,concomitant use of drugs),sample requirements (ie,sample size,eligibility criteria,sample recruitment), interventions(eg,electrode positioning,dosage,duration, and also comparison against pharmacotherapy),outcomes (eg,clinical versus surrogate outcomes),and safety.Another issue is ethical;who should apply tDCS in clinical settings (eg,physicians,neuropsychologists,specialized staff);the tolerable amount of risk for inducing maladaptive,long-term neuroplasticity,and whether tDCS could be used for
176Brunoni et al
enhancing neuropsychologic performance in healthy subjects;?nally,regulatory issues also need to be discussed. In contrast to transcranial magnetic stimulation(TMS),which is delivered through a sophisticated device,tDCS can be administered with devices already manufactured and used in pain and cosmetic medicine.These devices deliver direct current to the joints and/or the skin.Also,contrary to TMS, these devices are affordable and readily accessible and can be purchased by nontrained individuals,including patients.
The last question is why conducting clinical research on tDCS.Among others,we can identify three main reasons:
(1)there is a theoretical clinical basis for tDCS as
a substitutive treatment for pharmacotherapy,such as patients with poor drug tolerability or those with adverse pharmacologic interactions(eg,elderly people who use several drugs).For instance,one group that would poten-tially bene?t from further investigation of tDCS safety is pregnant women with unipolar depression,as there is a lack of acceptable pharmacologic alternatives for this condition24;(2)using tDCS as an augmentative treat-ment d for example,tDCS and restraint therapy for stroke25; or tDCS and pharmacotherapy for chronic pain or major depression.Again,side effects and noninvasiveness make tDCS an appealing strategy to boost the effects of other treatments in addition to its neurophysiologic effects on membrane resting threshold that likely underlie its syner-gistic effects.And,(3)tDCS is inexpensive;being therefore attractive to areas lacking in resources.If proven effective, tDCS will be an interesting option for developing countries.
The purpose of this review is to assess the current stage of tDCS development and identify its potential limitations in current clinical studies as to provide a comprehensive framework for designing future clinical trials.This review is divided in four parts.The?rst part reviews the mechanisms of action of tDCS,parameters of use and computer-based human brain modeling investigating electric current?elds and magnitude induced by tDCS.Given the conciseness of this section,the reader is invited to consult more recent reviews focusing exclusively on the mechanisms of action and technical development.26,27The second section covers methodologic aspects related to the clinical research applica-tion of tDCS.This section is divided according to study phase (ie,preclinical,phase I,phase II,and phase III studies).The third section focuses on ethical and regulatory concerns.The last section concludes with a presentation of what are ex-pected in the near future regarding novel approaches,novel devices,and future studies involving tDCS.
The electrophysiology of tDCS
Mechanisms of action
TDCS differs from other noninvasive brain stimulation techniques such as transcranial electrical stimulation(TES)and TMS.TDCS does not induce neuronal?ring by supra-threshold neuronal membrane depolarization but rather modulates spontaneous neuronal network activity.27,28At the neuronal level,the primary mechanism of action is a tDCS polarity-dependent shift(polarization)of resting membrane potential.Although anodal DCS generally enhances cortical activity and excitability,cathodal DCS has opposite effects.7,29,30Animal studies have shown that changes in excitability are re?ected in both spontaneous ?ring rates31,32;and responsiveness to afferent synaptic inputs.33,34It is this primary polarization mechanism that underlies the acute effects of low-intensity DC currents on cortical excitability in humans.6
However,tDCS elicits after-effects lasting for up to1 hour.9,35Therefore,its mechanisms of action cannot be solely attributed to changes of the electrical neuronal membrane potential.In fact,further research showed that tDCS also modi?es the synaptic microenvironment,for instance,by modifying synaptic strength NMDA receptor-dependently or altering GABAergic activity.36-38TDCS also interferes with brain excitability through modulation of intracortical and corticospinal neurons.10,39The effects of tDCS might be similar to those observed in long-term potentiation(LTP),as shown by one recent animal study that applied anodal motor cortex stimulation and showed a lasting increase in postsynaptic excitatory potentials.8 Experiments with peripheral nerve39and spinal cord40 stimulation showed that DC effects are also nonsynaptic, possibly involving transient changes in the density of protein channels localized below the stimulating electrode.
Given that a constant electric?eld displaces all polar molecules and most of the neurotransmitters and receptors in the brain have electrical properties,tDCS might also in?uence neuronal function by inducing prolonged neuro-chemical changes.38,40For instance,magnetic resonance spectroscopy showed that after anodal tDCS brain myoino-sitol signi?cantly increased,whereas n-acetyl-aspartate failed to change.41
In addition to the‘‘direct’’tDCS effects described previ-ously,‘‘indirect’’effects are also observed.This is seen in connectivity-driven alterations of distant cortical and subcor-tical areas.42,43Interestingly,tDCS modulates not only single neuron activity and evoked neuronal activity,but also spon-taneous neuronal oscillations.Ardolino et al.39found that below the cathodal electrode,the slow EEG activity in the theta and delta band increases.Animal and modeling studies suggest that a network of tightly coupled active neurons(eg, oscillations)may be more sensitivity to applied weak current than neurons in isolation.44-46
Although most early tDCS studies have been performed in the motor cortex,it should be noticed that tDCS does not only induce long-lasting alterations of motor-evoked poten-tials,but also affects somatosensory and visual-evoked potentials.This activity is dependent on the area stimu-lated.47-49Ferrucci et al.50and Galea et al.51provided evidence that tDCS can in?uence the human cerebellum.
Clinical research with tDCS177
Cogiamanian et al.40and Winkler et al.52demonstrated that transcutaneous DC stimulation modulates conduction along the spinal cord and the segmental re?ex pathways.
An important aspect when discussing the mechanisms of tDCS is the magnitude and location of the current induced in cortical tissues.Several modeling studies have been conducted to address this issue and will be discussed in a later section.
Finally,constant electrical?elds in?uence several different tissues(vessels,connective tissue)and pathophysiologic mechanisms(in?ammation,cell migration,vascular motility); in addition,their effects are observed on multiple cellular structures(cytoskeleton,mithocondria,membrane).With that said,tDCS may also in?uence nonneuronal components of the CNS.Support for this theory is observed below anodal tDCS electrode as it can induce prolonged brain vasodilatation.53 In conclusion,the mechanisms of action of DCS remain to be completely elucidated,an issue that can have important repercussions for future clinical applications. These mechanisms likely involve different synaptic and nonsynaptic effects on neurons and effects on nonneuronal cells and tissues within the CNS.
Pharmacologic investigation of tDCS
In tDCS research,pharmacologic studies use diverse drugs to block and/or enhance the activity of neurotransmitters and its receptors to observe how and whether tDCS-induced cortical excitability is modi?ed.Therefore,such studies aim to enhance our knowledge about the mechanisms of action of tDCS with regard to neuromodulation and neuroplasticity.
Evidence suggests that blocking voltage-gated sodium and calcium channels decreases the excitability enhancing effect of anodal tDCS.In contrast,cathodal tDCS-generated excitability reductions are not affected.36,37These?ndings are in line with the assumption that tDCS induces shifts in membrane resting threshold of cortical neurons.
Regarding neurotransmitters,it has been shown that NMDA-glutamatergic receptors are involved in inhibitory and facilitatory plasticity induced by tDCS.Blocking NMDA receptors abolishes the after-effects of stimulation, whereas enhancement of NMDA receptor ef?cacy by d-cycloserine enhances selectively facilitatory plasticity.9,54 In contrast,GABAergic modulation with lorazepam results in a delayed then enhanced and prolonged anodal tDCS-induced excitability elevation55(Table1).
Regarding the monoaminergic neurotransmitters, amphetamines(that increase monoaminergic activity) seem to enhance tDCS-induced facilitatory plasticity.56 For the dopaminergic system,tDCS-generated plasticity is modulated in a complex dosage-and subreceptor-dependent manner.Application of the dopamine precursor l-dopa converts facilitatory plasticity into inhibition,and prolongs inhibitory plasticity,57whereas blocking D2 receptors seems to abolish tDCS-induced plasticity,58D2 agonists,applied at high or low dosages,decrease plasticity. Furthermore,plasticity is restituted by medium dosage D2 agonists.59Interestingly,the acetylcholine reuptake-inhibitor rivastigmine affects tDCS-induced plasticity in a similar fashion as l-dopa.11For the serotoninergic system, the5-HT reuptake-inhibitor citalopram enhances facilita-tory plasticity and also converts inhibitory plasticity into facilitation.60
From a clinical point of view,these results show that pharmacotherapy and tDCS interact,which might be an issue when studying clinical samples receiving both
Table1Pharmacologic agents that interact with tDCS effects on cortical excitability
Drug Class Effect
Amine metabolism
Citalopram SERT blocker Enhancement of the duration of facilitatory anodal effects;
Facilitation of cathodal tDCS effects59
Amphetamine NET/DAT competitive inhibitor Enhancment of the duration of facilitatory anodal effects.55
L-Dopa Dopamine precursor For anodal:excitability turns into inhibition;For cathodal:effects are
enhanced12
Sulpiride D2-receptor blocker Abolishment of tDCS-induced plasticity57
Pergolide Dopamine agonist agent Enhancement of the duration of cathodal tDCS effects57,58
Amino acid metabolism
Lorazepam GABA allosteric modulator Anodal effects are delayed,but then enhanced and prolonged.100 Rivastigmine Cholinesterase inhibitor Abolishment of anodal tDCS effects;stabilization of cathodal tDCS
effects11
Dextromethorpan NMDA antagonist agent Abolishment of the after-effects of anodal and cathodal tDCS.36,37 D-cycloserine NMDA agonist agent Enhancement of the duration of anodal effects;no effects during
cathodal stimulation.54
Voltage-sensitive channel blockers
Carbamazepine Voltage-sensitive sodium channel blocker Abolishment of the depolarizing effects of anodal tDCS.36,37 Flunarizine Voltage-sensitive calcium channel blocker Similar effects of Carbamazepine.
tDCS5transcranial direct current stimulation;NET5norepinephrine transporter;DAT5dopamine transporter;GABA5gamma-aminobutyric acid; NMDA5n-methyl-d-aspartic acid;SERT5serotonin transporter.
178Brunoni et al
interventions.In fact,the complex nonlinear interaction makes it dif?cult to foresee the speci?c effects of pathophysiologic alterations or drug application on the amount and direction of tDCS-induced plasticity;thus demanding further empirical research on this topic.
Parameters of stimulation
TDCS parameters can vary widely and several factors need to be de?ned.These factors include electrode size and positioning,intensity,duration of stimulation,number of sessions per day,and interval between sessions.By varying these parameters,different amounts of electric current can be delivered,thus inducing diverse physiologic and adverse effects.Another potential concern is that tDCS devices are not worldwide standardized.These devices can be easily constructed using standard equipment and technology in engineering laboratories of colleges and universities.In fact,more than a dozen different tDCS devices can be found throughout neuromodulation laboratories worldwide. Electrode positioning
Although tDCS electrical?elds are relatively nonfocal, electrode positioning is critical.For instance,a previous study showed that changing the electrode reference from DLPFC to M1eliminated tDCS effects on working memory.16Other studies have shown that phosphene-thresholds are modulated only during occipital(visual cortex)DCS and not other areas.49,61Likewise,a tDCS trial for major depression showed that only DLPFC stimulation (and not occipital stimulation)ameliorated symptoms.62 Although current evidence suggests site-dependent effects, other issues have yet to be explored d for instance,one open question is whether and how brain stimulation in one area in?uences adjacent and more distant areas.
TDCS studies usually use one anode and one cathode electrode placed over the scalp to modulate a particular area of the CNS.Electrode positioning is usually deter-mined according to the International EEG10-20System. Given the focality of tDCS,this appears appropriate.For instance,studies exploring the motor cortex place elec-trodes over C3or C4;for the visual system,electrodes are typically placed over O1or O2(for a review of tDCS studies exploring different brain areas see Utz et al.63).
In this study,some terms used to describe tDCS montages should be discussed:when one electrode is placed bellow the neck,the entire montage is usually described as ‘‘unipolar.’’In contrast,montages with two electrodes on the head are termed usually‘‘bipolar.’’However,this nomen-clature might be inaccurate as technically the DC stimula-tion is always generated via two poles(electrodes) generating an electric dipole between the electrodes. Therefore,an alternative nomenclature of‘‘mono-cephalic’’and‘‘bi-cephalic’’is proposed to differentiate between ‘‘unipolar’’and‘‘bipolar’’setups,respectively.Researchers in the?eld also use the terms‘‘reference’’and‘‘stimulating’’electrode to refer to the‘‘neutral’’and‘‘active’’electrode, respectively.However,the term‘‘reference’’electrode may also be problematic,especially for bicephalic montages because the‘‘reference’’electrode is not physiologically inert and can contribute to activity modulation as well.This could be a potential confounder depending on the main study question.Nonetheless,researchers use these terms to highlight that(in their study)they are under the assumption that in their particular montage one electrode is being explored as the‘‘stimulating,’’whereas the other is the ‘‘reference.’’
In contrast,having the possibility to increase and decrease activity in different brain areas simultaneously may be advantageous.For instance,this could be useful in conditions involving an imbalanced interhemispheric activity(ie,in stroke).64In scenarios whether the reference electrode poses a confounding effect,an extracephalic reference electrode could theoretically aid in avoiding this issue.However,this might increase the risk of shunting the electric current through the skin(which would then not reach brain tissue)or displacing the current.Ultimately,the choice of montage will be application speci?c;for example, a recent study comparing different tDCS setups showed that,although bicephalic setups were effective,the monoce-phalic setup was no different than sham stimulation.65 Finally,in a monocephalic setup,using very high currents there is the potential risk of in?uencing brain stem activity, including respiratory control(note that this risk is theoret-ical and was only observed in one historical report).66 Nevertheless,in choosing the extracephalic position,the researcher must be con?dent that a signi?cant electric?eld will be induced on the target brain area.
Moreover,because current?ow direction/electrical?eld orientation relative to neuronal orientation might determine the effects of tDCS,7it might be that the effects of an ex-tracephalic electrode differs relevantly from that of a bipolar electrode arrangement.Alternatively,enhancing the size of one electrode,thus reducing current density,might enable functional monocephalic stimulation also with a bicephalic electrode montage.58
Direct current stimulation can also be delivered over noncortical brain areas.Ferrucci et al.50stimulated the cere-bellum showing changes in performance in a cognitive task for working memory.Galea et al.51explored the inhibitory effects of the cerebellum on motor-evoked potentials (MEPs)triggered by TMS over the motor cortex.This revealed that tDCS could modify MEPs in a polarity-speci?c manner. In addition,Cogiamanian et al.40observed that cathodal transcutaneous DC over the thoracic spinal cord suppressed tibial somatosensory-evoked potentials.Furthermore,Winkler et al.52observed that transcutaneous DCS over the spinal cord modulates the postactivation depression of the H-re?ex. Preliminary data indicates spinal DCS also in?uences noci-ception67suggesting that the spinal cord as a target for trans-cutaneous DCS.Challenges for stimulation in this area must be considered such as location of induced electrical?elds.
Clinical research with tDCS179
Modeling tDCS
During tDCS,current is generated across the brain;different montages result in distinct current?ow through the brain and thus the ability to adjust montage allows customization and optimization of tDCS for speci?c applications(see above). Though tDCS montage design often follow basic assump-tions(eg,‘‘increased/decreased excitability under the anode/ cathode’’),computational models of brain current?ow during tDCS(so called‘‘forward’’models)provide more accurate insight into detailed current?ow patterns,and in some cases show that the basic assumptions are not valid. When interpreting the results of such simulations,it is important to recognize that the intensity of current?ow in any speci?c brain region does not translate in any simple linear manner to the degree of brain modulation.However,it seems reasonable to predict that regions with more current ?ow are more likely to be affected by stimulation,whereas regions with little or no current?ow will be spared the direct effects of stimulation.
Computational models of tDCS range in complexity from concentric sphere models to individualized high-resolution models based an individual’s structural magnetic resonance imaging(MRI).The appropriate level of detail depends on the available computational resources and the clinical question being asked(see technical note below). Regardless of complexity,all models share the primary outcome of correctly predicting brain current?ow during transcranial stimulation to guide clinical practice in a mean-ingful manner.
Most clinical studies use tDCS devices that apply direct electric currents via a constant current source,but even within this space there are in?nite variations of dosage and montage that can be leveraged,with the help of models,to optimize outcomes.The current is sent through patch electrodes(surface areas typical range from25to35cm2 but can vary by an order of magnitude)attached to the scalp surface.Total current injected ranges in magnitude are typically from0.5to2mA.Steps taken to improve tDCS speci?city(including the use of larger‘‘return’’sponges and extracephalic electrodes)have been proposed but more analysis is required to determine the role of electrode-montage in neuromodulation and targeting.Modeling approaches are instrumental toward this goal.For example, modeling studies have recently predicted a profound role of the‘‘return’’electrode position in modulating overall current?ow including under the‘‘active’’(or‘‘stimulating’’) electrode.68Speci?cally,for a?xed active electrode position on the head,changing the position of the return electrode (including cephalic and extracephalic positions)in?uences current?ow through the presumed target region directly under the active electrode.Therefore,in addition to consid-ering the role of scalp shunting and action on deep brain structures(see above)when determining electrode distance, the complete design of electrode montage may subtly modu-late cortical current?ow.69Again,computer modeling can provide valuable insight into this process.
Recent modeling studies suggest that individual anatom-ical differences may affect current?ow through the cortex. In comparison to TMS,which uses MEPs to index its potency,there is no similar rationale for titrating tDCS dosage.A related issue is the modi?cation of tDCS dose montages for individuals with skull defects or stroke-related lesions.Such individuals may be candidates for tDCS therapy but defects/lesions are expected to distort current?ow.For example,any defect/injury?lled with cerebrospinal?uid(CSF),including those related to stroke of traumatic brain injury,is expected to preferentially ‘‘shunt’’current?ow.15Ideally,tDCS would be adjusted in a patient-speci?c(defect/lesion speci?c)manner to take advantage of such distortions in guiding current?ow to targeted regions,while simultaneously avoiding any safety concerns(such as current hot spots).
Evidence from modeling studies suggests that for typical tDCS signi?cant amounts of current can reach broad cortical areas especially between and under the electrode surface.12,13Modeling studies also show that electrode montage is critical to the amount of current shunted through the skin.
Electrode montage is critically associated to the amount of current being shunted through the skin,how much is delivered to the brain,and to what targets.The overall theme emerging from modeling efforts is that despite clinical success in applying simplifying rules in dose design,all the details and aspects of electrode montage design combine to in?uence current?ow such that these simplifying rules are applicable but only within a limited parameter range.For example,average current density (total current/electrode area)at the‘‘active’’electrode may be a useful metric to normalize speci?c neurophysiologic outcomes(eg,TMS evoked MEPs),there is no universal relationship between current density and brain modulation when one considers the full spectrum of possible electrode montages.13,70
Recent modeling data taking into consideration gyri and sulci geometry have shown that electric current can concentrate on the edge of gyri.71Therefore,the effects might not be homogeneous throughout the stimulated area.Increased appreciation of the complexity of current ?ow through the head(re?ecting the complexity of neuro-anatomy),reinforces the use of applying computational models to assist in tDCS dose design72rather than simply relying on some heuristic rules(eg,‘‘increased excitability under the anode’’).
In addition to predicting brain current?ow,modeling studies also provide insight into electrode design by predicting current?ow patterns through the skin.Modeling studies has reinforced that current is not passed uniformly through the skin but rather tends to concentrate near electrode edges or skin inhomogeneities.13Electrode design can be simple saline-soaked cotton or sponge pads or speci?cally designed patches with unique shapes and materials to maximize stimulation magnitude and focality.
180Brunoni et al
Modeling con?rms that decreasing the salinity of the pads reduces peak current concentration at the edges(even as the total current applied and average current density is ?xed).73
In summary,modeling studies are expected to play a critical role in the development of next-generation tDCS technologies and approaches.Notably,tDCS devices have not drastically changed since the time when the battery was ?rst discovered.Thus,conventional technology has certain limitations.These include focality(area stimulated),depth of penetration,and targeting-location control.To overcome these and other limitations,technologies using arrays of electrodes74such as‘‘High De?nition’’tDCS(HD-tDCS)71 and others(eg,simultaneous EEG monitoring during tDCS as to adjust dosage and parameters)have been recently proposed.Ultimately,as we begin integrating modern tech-nology with transcranial stimulation techniques,clinical control and ef?cacy will undoubtedly improve.
On a?nal technical note:Though there has been a recent emphasize to develop increasingly accurate and complex models,71,72,75certain universal technical issues should be considered for high-precision models,beginning with:(1) high-resolution(eg,1mm)anatomic scans so that the entire model work?ow should preserve precision.Any?nite-element human head model is limited by the precision and accuracy of tissue dimensions(masks)and conductivity values incorporated(inhomogeneity and anisotropy).One hallmark of precision is the cortical surface used in the ?nal?nite-element mask solver should represent realistic sulci and gyri;(2)Simultaneously,a priori knowledge of tissue anatomy and factors known to shape current?ow are applied to further re?ne segmentation.Particularly crit-ical are discontinuities not present in nature that result from limited scan resolution;notably both unnatural perforations in planar tissues(eg,holes in cerebrospinal?uid where brain contacts skull)and microstructures(eg,incomplete or voxelized vessels)can produce signi?cant aberrations in predicted current?ow.Addition of complexity without proper parameterization can evidently decrease prediction accuracy.An improper balance between these factors can lead to distortions in brain current?ow of an order of magnitude or more d uncontrolled additional complexity can in fact induce distortion.We thus emphasize that the most appropriate methodology(ranging from concentric spheres to individualized models)ultimately depends on the clinical question being addressed.
The clinical research of tDCS
Studies in nonhumans(Preclinical)
Previous animal studies have assessed safety limits of tDCS current intensity.In one study,58rats received tDCS with varying current densities for up to270minutes and histologic evaluation was conducted to assess neuronal
lesion.Results suggest that brain lesions occurred when
current density was at least two orders of magnitude higher
than typically used in humans88and may re?ect increase in
brain temperature never observed using conventional tDCS
protocols.14Another interesting insight from this study is
that duration of tDCS only becomes a safety issue when
the intensity of stimulation is near the threshold associated
with neuronal lesion.Other animals studies conducted with
different goals have also shown that tDCS used with
charges similar to human studies do not induce histological
lesions.89
Finally,animal studies are useful for test dosing and
exploring physiologic aspects of tDCS mechanisms.In
contrast,such studies are rare,and positioning of the
electrodes as well as different cortical architecture,might
be critical.Still,animal models might be important for
answering speci?c questions not possible to be done in
humans.
Studies on healthy volunteers(Phase I)
In drug-based trials,phase I studies are nonrandomized,
noncontrolled clinical(human)trials designed to address
safety and optimal dosage of drugs.This is performed by
assessing the adverse effects/safety and dosage or the drugs.
In this section,previous tDCS studies that address these
questions and present issues that remain unsolved(dose
parameters was above discussed)are reviewed(Table2). Safety/toxicity
Although tDCS differs in many aspects from other
noninvasive neuromodulatory therapies in that it does not
induce neuronal action potential and uses weak electric
currents,there are safety concerns that must be addressed.
If the electrochemical products generated by these currents
contact the skin,skin irritation may occur;in addition,
tissue heating associated with nonintact skin(therefore this
is especially important in people with skin diseases and/or
in protocols using daily tDCS applications and/or high
electric currents)may induce skin burning92d although mild redness is more likely related to local,vasodilatation
skin changes rather than skin damage.93In fact,considering
there is no direct contact between the brain and the elec-
trode and also the distance,electrochemical or heating
lesions to the neuronal tissue is less likely.Moreover,
experimental and modeling studies suggest no signi?cant
temperature increases for typical tDCS protocols.7,71,73
TDCS has been tested in thousands of subjects world-
wide with no evidence of toxic effects to date.In addition to
the hundreds of studies exploring tDCS effects in diverse
contexts,some studies have focused speci?cally on safety.
For instance,in a large retrospective study,Poreisz et al.94
reviewed adverse effects in77healthy subjects and25
patients who underwent a total of5671mA stimulation
sessions.Results show the most common effects were
Clinical research with tDCS181
mild tingling sensations(75%),light itching sensation (30%),moderate fatigue(35%),and headache(11.8%); and most of these effects did not differ from those of placebo stimulation.In another study,164sessions of stim-ulation were analyzed.Authors found only mild adverse effects with a low prevalence(0.11%in active and0.08% in sham stimulation group).95Other initial studies90,91,96-99 also reported only mild,benign,and transient side effects. In fact,the most severe adverse event reported is skin lesions on the site of electrode placement.92
Historically,the most severe adverse effect was observed in the?rst study of tDCS.During the1960s Lippold and Redfearn66related a brief respiratory and motor paralysis in a bifrontal electrode montage with the current reference placed on the leg.No loss of consciousness was reported and respiration returned to normal when the current was stopped.This was attributed to the fact that the subject received10times the intended intensity,probably3mA.27 General exclusion criteria for noninvasive brain stimu-lation also apply for tDCS.Subjects must be free of unstable medical conditions,or conditions that may increase the risk of stimulation such as uncontrolled epilepsy;although epileptic seizures have not been observed in a pilot study with patients with active epilepsy.100Also,subjects must have no metallic implants near the electrodes.
Finally,it should be underscored that most of these observations were extracted from single stimulation studies in healthy subjects without medications.Less is known about the adverse effects of daily(or even twice daily)tDCS in patients with neuropsychiatric disorders who use phar-macotherapy.In such conditions,the adverse effects can be magni?ed and therefore they should be actively inquired during trials.For instance,some single-patient studies report that tDCS can induce mania/hypomania in patients with major depression.101-103Therefore,we suggest that a medical monitor should supervise tDCS treatment in such contexts of increased risk of signi?cant adverse effects. Dosage
TDCS dosage is de?ned by the following parameters:(1) current dosage(measured in amperes);(2)duration of stimulation;and(3)electrode montage(size and position of all electrodes).Current density(current dose divided by electrode size)is also an important parameter in considering dosage;especially for de?ning safety58;see the following review for additional information.104The most common elec-trode sizes are of25-35cm2with currents of1-2mA(gener-ating densities ranging from0.28-0.80A/m2)for up to20-40 minutes.However,the current that effectively reaches neuronal tissue depends on other less controllable factors. These include skin resistance,skull resistance,resistance of intracranial structures(eg,blood vessels,cerebrospinal?uid, and meninges)and the resistance of brain tissue,which varies according to cell type and structure(eg,glial cells,pyramidal neurons,white matter,and so on).Moreover,patients with skull defects,brain lesions and other conditions will in?uence current amount and delivery.In addition,the baseline cortical excitability is different in people using pharmacotherapy(eg, benzodiazepines,55anticonvulsants,105antidepressants,69 and others106)and/or presenting neuropsychiatric disorders (eg,major depression,107schizophrenia,108?bromyalgia,109 migraine,110and others);an issue that is likely to interfere with the chosen dosage.Finally,other variables in?uence baseline cortical excitability such as gender,111age,112and smoking.113Hence,the same amount of current is likely to have nonuniform effects in subjects with different conditions. For instance,one study showed that low(25mg)and high (200mg)doses of l-dopa abolished tDCS-induced effects on cortical excitability,whereas an intermediate(100mg) dosage increased inhibitory effects.114Notwithstanding, these studies should be regarded as exploratory and thus repli-cated in other contexts and samples,especially in clinical populations.
Therefore,in the context of clinical research,such individual factors are a source of variability and,if important enough,may result in negative?ndings.To avoid this,one alternative is to standardize the source of error in the sample. For instance,using saline-soaked sponges to minimize skin resistance(which can also be measured by an ohmmeter adapted in the tDCS device d some devices do give the resistance),excluding patients under pharmacotherapy,or controlling when it is not feasible(eg,benzodiazepines), avoiding sample heterogeneity using speci?c diagnostic criteria,particularly when working with a small,neuropsy-chiatric subject pool.Future studies addressing the interac-tion of tDCS and drugs in psychopharmacology will continue to explore and identify which drugs do not interfere with tDCS effects and which ones could block or enhance tDCS-excitability effects.10,27
Initial studies measuring brain excitability demonstrate that currents as low as0.28A/m2present depolarizing and hyperpolarizing effects.6,7In addition,phase I/II studies ad-dressed the effects of varying dose and/or time of stimula-tion on cortical excitability and/or neuropsychologic tasks. Ohn et al.115tested the effects on working memory during 30minutes of stimulation,showing that performance increased in a time-dependent fashion.Other studies showed the cognitive effects induced by tDCS are depen-dent on the current intensity;demonstrating effects such as enhanced verbal?uency improvement at2mA(versus lower improvement at1mA)18;and working memory improvement at2mA(versus no improvement at1mA) (See Box1for other tDCS studies on cognition).116Never-theless,it remains unclear whether there is a linear(dose versus effect)curve associated with direct current stimula-tion and the in?uence of each parameter(dose,current density,stimulation duration)on these effects.It is known that increasing current densities will increase the depth of the electrical?eld,thus affecting different populations of neurons.However,at greater intensity tDCS might be pain-ful to the subjects.For these reasons,a more effective approach designed to prolong tDCS effects is to increase
Clinical research with tDCS183
the stimulation duration as opposed to the current density.7,27,35,37
Short applications(ie,seconds to a few minutes)of anodal/cathodal tDCS result in excitability shifts during stimulation but no after-effects.However,no long-term effects are seen.In contrast,10minutes or more of stimulation can elicit prolonged after-effects,which can be sustained for over an hour.7,27,39The exact duration of effects depends on the targeted cortical area and on the type of variable assessed.
For clinical purposes,longer-lasting effects are crucial. Single-dose tDCS interventions have relatively short-lived after-effects.Multiple stimulation sessions are required to induce a signi?cant manipulation in synaptic ef?cacy.117,118 In fact,repeated sessions of tDCS may have cumulative effects associated with greater magnitude and duration of behavioral effects.For example,cathodal tDCS applied over5consecutive days is associated with cumulative motor function improvement lasting up to2weeks after the end of stimulation.This is an effect which is not observed when sessions are applied weekly(as opposed to daily).98Whether this approach is appropriate to maxi-mize and stabilize the electrophysiologic effects of tDCS remains under investigation.The optimal repetition rate and duration to promote tDCS-induced plasticity also remains to be determined.In animal experiments,repetition of tDCS during the after-effects of a?rst stimulation session has been shown to enhance ef?cacy.32However, repeated plasticity induction may result in homeostatically driven antagonistic effects.119Recently,Monte-Silva and coworkers118directly compared the effects induced by single sessions of cathodal tDCS over the motor cortex to the effects of repetitive stimulation during or after the after-effects of the?rst stimulation.The results showed that increasing cathodal tDCS duration(1mA,with no interstimulation interval)resulted in longer-lasting after-effects,typically over1hour(tDCS duration from9to 18min prolonged the after-effects from60to90minutes). Interestingly,when the second stimulation was performed during the after-effects of the?rst,a prolongation and enhancement of tDCS-induced effects for up to120 minutes after stimulation was observed.In contrast,when the second session was performed3or24hours after the ?rst,tDCS effects on cortical excitability were mixed. This was shown with a primary reduction or abolishment of the initial effects of cathodal tDCS,followed by a later reoccurrence of tDCS-induced cortical inhibition.Such neurophysiologic evidence is indicative of a stimulation timing-dependent plasticity regulation in the human motor cortex.Understanding the interaction of the consecutive stimulation protocols appears crucial to effectively target spontaneous changes of cortical activity and excitability (See Box2for a discussion on‘‘of?ine’’vs.‘‘online’’stim-ulation).Hence,implementing more effective procedures of plasticity induction procedures in clinical settings is crucial d in fact these results need to be replicated in clinical populations.
Studies on patients with neuropsychiatric conditions(Phase II/III)
Phases II and III studies relate to using an intervention in clinical samples.Phase II studies are typically small and use targeted samples to obtain additional information regarding optimal parameters of stimulation.Phase III are
pivotal 184Brunoni et al
studies,involving larger samples.In the United States,two positive phase III trials are required for approving a drug or device by the Food and Drug Agency(FDA)(See Box3for phase II/III studies in major depression).
As mentioned previously,several studies have explored the therapeutic application of tDCS in several neuropsy-chiatric disorders.The results of these studies reveal long-lasting tDCS effects and have promoted its use in clinical settings.Because clinical development of tDCS is being conducted mainly in academia,studies are not widely standardized regarding variables and population samples, therefore limiting conclusions.These?ndings are also limited by small sample sizes and experimental design.In fact,a similar scenario has been observed for TMS5to10 years ago.120This section aims to comprehensively review the main issues of the later phases of clinical trial develop-ment for tDCS.
Issues related to recruitment and eligibility Recruiting subjects for tDCS clinical trials presents a chal-lenge.Proposing an intervention alternative to the main-stream pharmacotherapy might be seen by prospective patients and referring physicians in nonacademic settings as suspicious.This issue can be particularly important when a large sample size is required and/or if the eligibility criteria exclude refractory patients who are more prone to enroll in research protocols.Likewise,referral physicians,due to time constraints in the ambulatory setting,usually prefer to treat drug-na€?ve patients themselves.Indeed,daily visits for1-to-2weeks to research centers might sound unappealing and/or unaffordable even to refractory patients.In such contexts,it is advisable to have multiple referral sources and to use broad recruitment strategies.Building trust with potential volunteers is imperative.One cost-effectiveness approach could be using explanatory videos in lay language.26
Another issue is sample heterogeneity.In pivotal clinical trials comparing tDCS against pharmacotherapy,large samples are typically required and patient heterogeneity might be larger than for drug trials for the same condition. This is due to the fact that the severity of the condition ranges from drug-na€?ve to refractory subjects.Targeting only the former would create dif?cult enrollment(for the reasons mentioned previously),although targeting only the latter decreases overall generalizability.Possible solutions include strati?cation during randomization(refractory versus nonrefractory),post hoc analysis controlling for refractoriness,or increasing sample size to address some of the issues associated with heterogeneity.
Blinding issues
It appears easier to conduct sham-controlled trials using tDCS compared with TMS.TMS induces itching and pain sensations over the stimulation site,whereas tDCS induces a mild tingling sensation that usually rapidly fades. Therefore,sham protocols begin with active tDCS,which is switched off within a minute.In addition,the tingling sensation relates to the velocity in which the current is either increased or decreased.In fact,an increase of current delivery from0.1to0.2mA/s generates no discomfort for most subjects.121Interestingly,some subjects in the sham group continue feeling some tingling even after the current is discontinued.
These sensations are related to the total amount of charge delivered.Although this has yet to be systematically evaluated,this relationship can be a potential issue when delivering relatively high charges(.1.5-2mA/s)and/or higher current densities.There is evidence that electrolyte solutions with lower NaCl concentrations(15mM)are perceived as more comfortable during tDCS than those solutions with higher NaCl concentrations(220
mM). Clinical research with tDCS185
Because the ionic strength of deionized water is much less than that of all NaCl solutions,there is a signi?cantly larger voltage required to carry current through the skin compared with NaCl solutions.Thus,it is recommended the use of solutions with relatively low NaCl concentration,in the range15mM to140mM,as tDCS at these concentrations is more likely to be perceived as comfortable,requires low voltage and still allows good conduction of current.122It has also been proposed to apply topical anesthetics to alle-viate this issue.27
An additional blinding issue is the local vasodilatation after tDCS.This causes the skin to turn red that might not be acknowledged by the subject but might be seen by the staff and other patients.In clinical protocols,such redness can be evident after several days of stimulation.This can become a logistical issue,demanding stimulated patients to leave the setting immediately,avoiding contact with other people(patients and researchers)as to avoid blinding breaking.Another approach would be to interview patients before(and not after)being stimulated.If a rater notices evidence of redness on the scalp of a patient,another blinded rater should substitute him/her,although this matter is more important in sham-controlled studies as in studies using active groups differing only regarding polarity(and not scalp site of stimulation)cathodal and anodal stimula-tion cannot be distinguished between each other.Also,30 seconds of active stimulation in the sham protocols might also lead to local redness.
Clinical protocols should assess post hoc the effective-ness of blinding;though investigators need to be aware that potential differences might occur because active tDCS is more effective than sham tDCS.It is not easy to detangle unblinding versus response because effectiveness.Other alternatives are(1)to avoid crossover trials,especially when the crossover happens in the same section,as to avoid subjects noticing the differences;(2)to apply active protocols but switching polarity so that adverse effects do not threaten blinding even if noticed,although the issue would be whether changing polarity would be an appro-priate control condition,when the reference electrode is not physiologically inert.
Study design
Four approaches in tDCS clinical trials for neuropsychiatric disorders are possible:(1)to compare active versus tDCS sham in a superiority trial;(2)to compare tDCS versus another therapy(eg,acupuncture,pharmacotherapy)as a superiority or noninferiority trial;(3)to combine tDCS with another therapy(eg,physical therapy,pharmaco-therapy)versus sham tDCS and another therapy as a superiority trial;and(4)combination of these approaches.
Two-arm designs are suitable when comparing active versus tDCS sham,an approach commonly used in pilot,‘‘proof-of-concept’’studies.This approach is effective in studies exploring the mechanisms of action of tDCS,for example,with neuroimaging or serum
measurements. 186Brunoni et al
Three-arm and‘‘double-dummy’’(ie,placebo pill1 active tDCS versus pharmacotherapy1sham tDCS)designs are adequate for comparing tDCS against another therapy. The placebo arm is interesting for increasing assay sensi-tivity,although ethical concerns might impede using placebo groups when there are reasons to believe that treatment ef?cacy among study arms is imbalanced(principle of clinical equipoise).123
Another option is a factorial(232)design,which could be useful to test tDCS with and/or against another therapy of interest.For instance,in a trial testing tDCS for chronic pain, patients could be randomized to four groups:only tDCS,only pharmacotherapy,tDCS,and pharmacotherapy and sham plus placebo.In fact,such a design is the most robust as it tests two interventions simultaneously and also one inter-vention against another,making them optimal for pivotal studies.Although comprehensive,this approach is more demanding regarding resources,sample size,and logistics.
The n-of-one(n51)trial is a possible approach when the researcher is con?dent that tDCS effects are short-lasting(which is not usually the case for studies using multiple sessions of tDCS).In this design,one subject is randomized to receive repeated randomized allocations of the tDCS treatment.This is helpful especially to address different parameters of stimulation for single session protocols.
Attrition
Attrition(or‘‘dropout’’)is the premature discontinuation of participation in a trial occurring either immediately after the baseline visit or at any time before endpoint.The speci?c reasons for attrition in tDCS trials should still be investigated.Although some might be the same for pharmacotherapy,one reason more speci?c for tDCS trials is the dif?culty to comply with required daily visits to the research center(that usually occur during the?rst2weeks of the study).In intention-to-treat trials,this issue can be particularly perturbing as such subjects will maintain the same baseline scores at endpoint and thus diminish the effect size between groups.To avoid attrition in tDCS trials, some measures can be taken such as:(1)concede one or two nonconsecutive missing visits,which are replaced at the end of the daily stimulation phase and(2)using a‘‘run-in’’period,that is,a phase before trial onset in which subjects receive either active or placebo/sham treatment (usually for1week)as a method to preemptively screen and discard nonadherent subjects.Although the usefulness of run-in phases is controversial in pharmacotherapy given the potential for selection bias,the rationale for using tDCS is to select subjects that can commit to the stimulation protocol requirements.
Finally,although uncommon,another issue is skin burn. This would prevent further stimulations,breaking blinding, and also forcing the investigators to withdraw treatment, leading to a study dropout.Skin burning can be avoided by diminishing electric density(ie,increasing electrode size and/or diminish electric current)and electric resistance(by using rubber electrodes involved with saline-soaked sponges)over the stimulation site.
Statistical issues
Being that most tDCS trials are exploratory and using small samples,they are particularly vulnerable to type I and type II errors.
Type I(false-positive)errors occur in exploratory studies performing several statistical tests,being the case of many phase II tDCS trials.In this scenario,investigators need to decide whether to claim?ndings as exploratory or to determine a priori the statistical method for the primary outcome,differentiating other statistical analyses as secondary.
Type II(false-negative)errors occur in small studies and are related to underpowered trials.Again,most phase II tDCS trials recruit small samples and are prone to this error. To avoid this,researchers must perform sample size calculations when designing the trial.Another approach is to use adaptive designs,which allow sample increasing during the study,although this method may be challenging for researchers and readers to interpret the data.In this context,given that most of tDCS trials are conducted with limited resources,the best choice of primary study outcome is a continuous outcome and two time points so as to increase statistical power(and consider other analyses as secondary). Although baseline differences are usually not signi?cant in tDCS trials probably because trials have a relatively homo-geneous population,one option is to calculate normalized differences from baseline.In this case a simple approach to calculate sample size is to use independent two-sample t test provided in most statistical software packages.
Pilot versus pivotal studies for tDCS
Most phase II studies are also referred as‘‘proof-of-concept’’or‘‘pilot’’studies.These studies typically use small,high-targeted samples that represent the more severe spectrum of a disease to address the ef?cacy of a given treatment in optimal conditions.They also use several surrogate endpoints and perform many exploratory anal-yses.Exploratory phase II studies are necessary as they provide data to be used in subsequent trials.Furthermore, data of small studies can be pooled together in metaanal-yses.However,the validity of these analyses can be contested when approving clinical interventions.128,129 An additional challenge for pilot studies is the explor-atory nature and thus an important degree of risk regarding outcomes that is normally not seen in animal models in neuromodulation research.This hinders the ability to test the clinical ef?cacy of tDCS for a particular condition for the?rst time.In such context,a negative?nding might be due to tDCS parameters or a poor neurobiologic model(eg, a negative?nding in a pain trial with anodal stimulation over the DLPFC area might represent,besides being a true-negative,either the use of incorrect tDCS parameters,
Clinical research with tDCS187
a misconception in the neurologic model;thus the DLPFC area being unrelated to pain pathophysiology).This issue poses an additional challenge in tDCS research.
Therefore,pivotal(phase III)studies are necessary to validate tDCS as an effective treatment when proof-of-concept trials showed encouraging results.Future phase III studies should include:(1)sample size estimation based on prior,pilot trials or metaanalyses;(2)robust blinding method(eg:using tDCS devices that can be automatically turned off as to keep both patients and appliers unaware of the intervention delivered)and(3)assessment of sample heterogeneity,either targeting particular samples(eg, medication-free patients)or identifying potential sources of heterogeneity(eg,degree of refractoriness,number of depressive episodes,depression severity,and others)and controlling for them during study design(strati?ed random-ization approaches)or statistical analysis.
Surrogate outcomes
Although several de?nitions for surrogate(or substitutive) outcomes exist,they are typically understood as laboratory measurements that substitute clinically meaningful outcomes for being in a prior step in the pathophysiologic pathway of the disease.130In neuromodulation research, this also includes neuropsychologic tests and neuroimaging scans.The advantage of using surrogate outcomes is avoid-ing long-term,expensive research.This is achieved by substituting‘‘hard’’outcomes(death or serious events)for ‘‘soft’’measurements that take place earlier.Furthermore, surrogate outcomes must have high accuracy and low vari-ability;otherwise their utility is limited(Table3).
One surrogate outcome that is often used is TMS-indexed cortical excitability,a neurophysiologic measure-ment.According to the protocol used,it indexes and detects changes in brain activity.131For instance,measurement of motor threshold d the lowest intensity to elicit motor-evoked potentials of more than50uV in at least50%of trials d is used for studying whether different tDCS proto-cols change motor cortical excitability.Also,measurement of the silent period d the period of electromyographic suppression(or voluntary muscle activity)after one single suprathreshold TMS pulse d can be also used for addressing whether and how tDCS affects the inhibitory cortical inter-neurons that are recruited during this task.Moreover, paired-pulse TMS is also used for studying inhibitory or excitatory cortical mechanisms elicited after one supra-threshold pulse and is another method that can be coupled with tDCS for indexing cortical excitability.Nonetheless, all these methods are limited to the motor cortex and thus might not necessarily re?ect net brain cortical excitability and/or cortical excitability of speci?c brain areas.
Neuropsychologic tests are able to measure brain activity in some areas,especially those that cannot be indexed through TMS.Moreover,cognitive de?cits are a common consequence of brain injury,stroke,epilepsy, neurodegenerative,and other neurologic disorders.Hence,the rehabilitation of cognitive function,such as language, spatial perception,attention,memory,calculation,and praxis represents an expanding area of neurologic rehabilitation and has recently attracted growing attention within the scienti?c community.For instance,changes in the activity of the prefrontal cortex can be measured using tests of working memory and attention,whereas temporoparietal stimulation can be evaluated using working memory tests.A drawback of several neuro-psychologic tests is the need of a control group to adjust for learning effects biases.Performance is also in?uenced by educational level and,therefore,the results of one study might not be valid for similar samples in different countries.
Neurophysiologic measurements are another possible approach to surrogate outcomes.Besides TMS,brain activity can be measured using electrodes,which can be interpreted using several methods.These include the qual-itative EEG,which measures spontaneous neuronal?ring; the event-related potentials(ERPs),which modi?es accord-ing to the brain area provoked;the quantitative EEG (qEEG),which maps brain activity;and,?nally,new approaches that provide a three dimensional brain imaging based on electromagnetic reconstruction of the brain(which in fact are not widely accepted due to the‘‘inverse problem solution.’’For a review on this topic,see Pascual-Marqui et al.132).Such measurements lack speci?city d simple psychological,cognitive,or motor task recruits several brain networks and thus the measured ERP can be an epiphenom-enon of another brain region rather than a relevant?nding (ie,a‘‘noise’’and not a‘‘signal’’).Another issue is that the devices measuring brain activity must be adapted to decrease the electrical noise generated by the tDCS device; or,alternatively,the measurement must be collected either before or after(but not throughout)tDCS delivery.
Neuroimaging methods are divided into two branches: the?rst uses radiotracers and is represented by the positron-emission tomography(PET)and the single-photon emis-sion computed tomography(SPECT),which assess brain metabolism through the emission of gamma rays.The advantage of PET/SPECT in tDCS research is that the radiotracer can be injected during brain stimulation,thus providing‘‘real-time’’brain imaging.However,the spatial resolution of such methods is poor.Because they obligatory require using radiotracers,the radiation dose needs to be carefully controlled and monitored.The second branch of neuroimaging is the MRI.This technique presents high spatial resolution.There are several methodologic approaches for MRI,which allows evaluation of different aspects of brain activity.For example,functional MRI (fMRI)explores the paramagnetic properties of hemoglobin to infer brain metabolism(based on blood oxygen satura-tion),whereas magnetic resonance spectroscopy(MRS) analyzes the magnetic?elds of relevant molecules(eg, glutamate,GABA)and provides a noninvasive‘‘chemical biopsy’’of the brain.Some of these techniques such as
188Brunoni et al
fMRI lack temporal resolution as it does not measure electrical activity changes directly (it does indirectly via changes in cerebral ?ow).Diffusion tensor imaging (DTI)focuses on the white matter ?bers,revealing the neural connectivity between brain areas.Finally,voxel-based morphometry (VBM)is a computational analysis of morphologic images that makes inferences about brain activity based on the differences of brain tissue concentra-tion among areas.For tDCS,these methods present the advantage of high spatial resolution;allowing to assess subtle changes in the stimulated area.For instance,one study used VBM to assess neuroplastic changes after 5days of TMS over the superior temporal cortex;showing macroscopic gray matter changes in the region.133Even though,the reliability of some methods of MRI are currently under dispute.134Moreover,tDCS is not used concomitantly with MRI yet due to serious risks of over-heating and thus an ‘‘online’’visualization of the stimulated area is not possible although this technical dif?culty might be resolved in the near future.
Finally,there is a wide range of blood measurements used in neuropsychiatry research for surrogate outcomes.135One biomarker under intensive investigation is the brain-derived neurotrophic factor (BDNF).This marker plays an important role in synaptogenesis and neuroplasticity and
is thus believed to be linked with some neuropsychiatric disor-ders,for instance,BDNF serum levels are low in depressed patients and increase after antidepressant treatment.136A recent study showed BDNF expression also increases after tDCS.8Additional biomarkers used in neuropsychiatry include in?ammatory proteins such as interleucin-1,interleu-cin-6,and TNF-alpha 137;hypothalamic-pituitary-adrenal activity,which is measured by serum and salivary cortisol 138;and oxidative stress proteins such as nitric oxide and other neuroin?ammatory protein markers.139,140These biomarkers present two important drawbacks:?rst,because of the blood-brain barrier,serum levels might not re?ect ‘‘real-time’’brain activity (or even brain activity at all);second,serum levels can only express the net brain activity,and do not represent a speci?c area.Therefore,perhaps the most effective use in tDCS research is to index disease improvement in phase II/III studies (Also see Box 4for a discussion on surrogate outcomes).
TDCS in children
As the brain is under intensive development during child-hood and adolescence d particularly the prefrontal cortex,141intensive research is currently being made to explore how cognition,emotion,behavior,and
other
190
Brunoni et al
functions evolve.Having neuromodulatory properties, tDCS would be an interesting tool to explore which brain areas are particularly important in each stage of develop-ment both in healthy and pathologic conditions,such as epilepsy,cerebral palsy,autism,and mental de?ciency. However,because of its potential to induce neuroplastic changes,tDCS should be used carefully especially during important phases of brain development associated with intensive plasticity and also other processes such as synaptic pruning.
A further step would be using tDCS for treating neuropsychiatric disorders in children,but this has not been tested yet.In a review of TMS studies in children,no adverse effects were reported,but its use is still limited for some reasons,including lack of established safety guide-lines.142Notwithstanding,tDCS is a promising tool for children neurology and psychiatry.
The ethics of tDCS
TDCS is a putative candidate for adjuvant therapy for a range of neuropsychiatric conditions.tDCS is a valuable tool in neuroscience research,as its focality can be used to explore several brain aspects.Studies regarding tDCS ethics reveals its ability to induce changes in behavior such as in moral judgment,143deception,144,145and decision-making.146For instance,one recent study showed tDCS affected utilitarian behavior.Similarly to other studies in tDCS,the polarity-dependent effects resulted in a sel?sh versus sel?ess behavior in women.143Although the effects were short-lasting(volunteers were not exposed to daily stimulation),the targeted area is similar than used in studies exploring the long-lasting tDCS effects.Therefore,the ethical concern is whether tDCS could induce maladaptive behavior changes,and if so,to what intensity and extent of time.
Diverse tDCS studies on healthy subjects have shown positive changes in attention and memory.84,85,147From the scope of neuroethics,the issue is whether tDCS enhances cognition in healthy subjects.Can tDCS be used to boost performance in speci?c situations(eg,before school tests)? Another issue is that the cognitive effects described (increased attention and memory)from tDCS are in some aspects similar to amphetamines.Despite therapeutic appli-cations,amphetamines are sold illegally as a recreational and performance enhancer drug(with the suggestive name of‘‘speed’’).As a tDCS device is easily built and inexpensive(contrary to TMS),it could also be used for nonresearch and nontherapeutic objectives by lay people. In fact,there are online videos in popular web sites such as Youtube explaining how to build and use a tDCS device.148Although it should be underscored that all the enhancement effects were present for a short period,it is possible that prolonged daily stimulation could increase the time span of such effects,thus inducing maladaptive changes.In contrast,other legal substances such as caffeine are also frequently used as cognitive boosters.
In fact,because applications in these?elds are currently in the research stage,?xed protocols and safety guidelines are yet to be de?ned.Research and development of any new devices provides an opportunity for brain science and clinical care to advance,and also challenges the medical and wider communities to address potential dangers and complications,ethical and moral quandaries,and issues of healthcare economics and distributive justice.For innova-tive neurotechnologies,these are major potential pitfalls to look out for.Intervening in the brain is always fraught with the potential for serious consequences.Despite these concerns,only by conducting carefully planned clinical and experimental studies can we provide the impetus to advance care for people with brain,emotional or psycho-logic,or neuropsychiatric disorders.
Conclusion
The current paper addresses the main aspects of the clinical research of tDCS.This technique has a wide range of potential applications and can be used to explore the basic aspects of neurosciences as well as for the treatment of neuropsychiatric disorders.TDCS has unique characteris-tics such as ability to induce antagonistic effects in cortical excitability according to the parameters of stimulation; concomitant(‘‘online’’)use with neuropsychologic and psychophysiologic tests;noninvasiveness and thus absence of pharmacokinetics interactions,being a putative substi-tutive/augmentative agent in neuropsychiatry;and low-cost and portability,making it suitable for increasing access to novel therapies.However,such characteristics also bring challenges regarding clinical design,neuroethics and legal issues.In this paper,we aimed to provide an overview of tDCS in clinical research;thereby providing knowledge for conducting proper clinical trials using this promising approach.
Acknowledgments
We are thankful to Erin Connors for copyediting this manuscript.We are also grateful to Scala Institute and Mackenzie University(Sao Paulo,Brazil)for the additional support to organize this working group meeting in the II International Symposium in Neuromodulation.This working group meeting was the2nd International tDCS club workshop,which took place in Sao Paulo,Brazil,in March2010,at the Social and Cognitive Neuroscience Laboratory of Mackenzie Presbiterian University.The?rst meeting was held in Milan,Italy in2008. D.E.was supported by NIH grant R21HD060999and F.F.was supported by NIH grant(5R21DK081773-03).
Clinical research with tDCS191
References
https://www.360docs.net/doc/571179832.html,rgus S.De compositionibus medicamentorum.Paris1529.
2.Kellaway P.The part played by the electric?sh in the early history of
bioelectricity and electrotherapy.Bull Hist Med1946;20:112-137.
3.Zago S,Ferrucci R,Fregni F,Priori A.Bartholow,Sciamanna,
Alberti:pioneers in the electrical stimulation of the exposed human cerebral cortex.Neuroscientist2008;14(5):521-528.
4.Aldini G.Essai theorique et experimental sur le galvanisme.
Paris1804.
5.Arndt R.Die electricitat in der psychiatrie.Arch Psychiat Nervenk-
rankh1869;2:259-261.
6.Priori A,Berardelli A,Rona S,Accornero N,Manfredi M.Polariza-
tion of the human motor cortex through the scalp.Neuroreport1998;
9(10):2257-2260.
7.Nitsche MA,Paulus W.Excitability changes induced in the human
motor cortex by weak transcranial direct current stimulation.
J Physiol2000;527(Pt3):633-639.
8.Fritsch B,Reis J,Martinowich K,et al.Direct current stimulation
promotes BDNF-dependent synaptic plasticity:potential implications for motor learning.Neuron2010;66(2):198-204.
9.Nitsche MA,Liebetanz D,Antal A,Lang N,Tergau F,Paulus W.
Modulation of cortical excitability by weak direct current stimulation–technical,safety and functional aspects.Suppl Clin Neurophysiol 2003;56:255-276.
10.Nitsche MA,Seeber A,Frommann K,et al.Modulating parameters
of excitability during and after transcranial direct current stimulation of the human motor cortex.J Physiol2005;568(Pt1):291-303. 11.Kuo MF,Paulus W,Nitsche MA.Boosting focally-induced brain
plasticity by dopamine.Cereb Cortex2008;18(3):648-651.
12.Wagner T,Fregni F,Fecteau S,Grodzinsky A,Zahn M,Pascual-
Leone A.Transcranial direct current stimulation:a computer-based human model study.Neuroimage2007;35(3):1113-1124.
13.Miranda PC,Lomarev M,Hallett M.Modeling the current distribu-
tion during transcranial direct current stimulation.Clin Neurophysiol 2006;117(7):1623-1629.
14.Bikson M,Datta A,Elwassif M.Establishing safety limits for transcranial
direct current stimulation.Clin Neurophysiol2009;120(6):1033-1034.
15.Datta A,Bikson M,Fregni F.Transcranial direct current stimulation
in patients with skull defects and skull plates:high-resolution compu-tational FEM study of factors altering cortical current?ow.Neuro-image2010;52(4):1268-1278.
16.Fregni F,Boggio PS,Nitsche M,et al.Anodal transcranial direct
current stimulation of prefrontal cortex enhances working memory.
Exp Brain Res2005;166(1):23-30.
17.Boggio PS,Alonso-Alonso M,Mansur CG,et al.Hand function
improvement with low-frequency repetitive transcranial magnetic stimulation of the unaffected hemisphere in a severe case of stroke.
Am J Phys Med Rehabil2006;85(11):927-930.
18.Iyer MB,Mattu U,Grafman J,Lomarev M,Sato S,Wassermann EM.
Safety and cognitive effect of frontal DC brain polarization in healthy individuals.Neurology2005;64(5):872-875.
19.Fecteau S,Pascual-Leone A,Zald DH,et al.Activation of prefrontal
cortex by transcranial direct current stimulation reduces appetite for risk during ambiguous decision making.J Neurosci2007;27(23): 6212-6218.
20.George MS,Aston-Jones G.Noninvasive techniques for probing neu-
rocircuitry and treating illness:vagus nerve stimulation(VNS),trans-cranial magnetic stimulation(TMS)and transcranial direct current stimulation(tDCS).Neuropsychopharmacology2010;35(1):301-316.
21.Fregni F,Pascual-Leone A.Technology insight:noninvasive brain
stimulation in neurology-perspectives on the therapeutic potential of rTMS and tDCS.Nat Clin Pract Neurol2007Jul;3(7):383-393.
22.Nitsche MA,Boggio PS,Fregni F,Pascual-Leone A.Treatment of
depression with transcranial direct current stimulation(tDCS):
a review.Exp Neurol2009;219(1):14-19.23.Brunoni AR,Tadini L,Fregni F.Changes in clinical trials method-
ology over time:a systematic review of six decades of research in psychopharmacology.PLoS One2010;5(3):e9479.
24.Zhang X,Liu K,Sun J,Zheng Z.Safety and feasibility of repetitive
transcranial magnetic stimulation(rTMS)as a treatment for major depression during pregnancy.Arch Womens Ment Health2010;
13(4):369-370.
25.Bolognini N,Pascual-Leone A,Fregni https://www.360docs.net/doc/571179832.html,ing non-invasive brain
stimulation to augment motor training-induced plasticity.
J Neuroeng Rehabil2009;6:8.
26.Zaghi S,Acar M,Hultgren B,Boggio PS,Fregni F.Noninvasive
brain stimulation with low-intensity electrical currents:putative mechanisms of action for direct and alternating current stimulation.
Neuroscientist2010;16(3):285-307.
27.Nitsche MA,Cohen LG,Wassermann EM,et al.Transcranial direct
current stimulation:state of the art2008.Brain Stimul2008;1(3): 206-223.
28.Priori A,Hallett M,Rothwell JC.Repetitive transcranial magnetic
stimulation or transcranial direct current stimulation?Brain Stimul 2009;2(4):241-245.
29.Bindman LJ,Lippold OC,Redfearn JW.Relation between the size
and form of potentials evoked by sensory stimulation and the back-ground electrical activity in the cerebral cortex of the rat.J Physiol 1964;171:1-25.
30.Purpura DP,McMurtry JG.Intracellular activities and evoked poten-
tial changes during polarization of motor cortex.J Neurophysiol 1965;28:166-185.
31.Creutzfeldt OD,Fromm GH,Kapp H.In?uence of transcortical d-c
currents on cortical neuronal activity.Exp Neurol1962;5:436-452.
32.Bindman LJ,Lippold OC,Redfearn JW.The action of brief polar-
izing currents on the cerebral cortex of the rat(1)during current ?ow and(2)in the production of long-lasting after-effects.
J Physiol1964;172:369-382.
33.Jefferys JG.In?uence of electric?elds on the excitability of
granule cells in guinea-pig hippocampal slices.J Physiol1981;
319:143-152.
34.Bikson M,Inoue M,Akiyama H,et al.Effects of uniform extracel-
lular DC electric?elds on excitability in rat hippocampal slices in vitro.J Physiol2004;557(Pt1):175-190.
35.Nitsche MA,Paulus W.Sustained excitability elevations induced by
transcranial DC motor cortex stimulation in humans.Neurology 2001;57(10):1899-1901.
36.Liebetanz D,Nitsche MA,Tergau F,Paulus W.Pharmacological
approach to the mechanisms of transcranial DC-stimulation-induced after-effects of human motor cortex excitability.Brain 2002;125(Pt10):2238-2247.
37.Nitsche MA,Fricke K,Henschke U,et al.Pharmacological modula-
tion of cortical excitability shifts induced by transcranial direct current stimulation in humans.J Physiol2003;553(Pt1):293-301.
38.Stagg CJ,Best JG,Stephenson MC,et al.Polarity-sensitive modula-
tion of cortical neurotransmitters by transcranial stimulation.
J Neurosci2009;29(16):5202-5206.
39.Ardolino G,Bossi B,Barbieri S,Priori A.Non-synaptic mecha-
nisms underlie the after-effects of cathodal transcutaneous direct current stimulation of the human brain.J Physiol2005;
568(Pt2):653-663.
40.Cogiamanian F,Vergari M,Pulecchi F,Marceglia S,Priori A.Effect
of spinal transcutaneous direct current stimulation on somatosensory evoked potentials in humans.Clin Neurophysiol2008;119(11): 2636-2640.
41.Rango M,Cogiamanian F,Marceglia S,et al.Myoinositol content in
the human brain is modi?ed by transcranial direct current stimulation in a matter of minutes:a1H-MRS study.Magn Reson Med2008;
60(4):782-789.
42.Boros K,Poreisz C,Munchau A,Paulus W,Nitsche MA.Premotor
transcranial direct current stimulation(tDCS)affects primary motor excitability in humans.Eur J Neurosci2008;27(5):1292-1300.
192Brunoni et al
https://www.360docs.net/doc/571179832.html,ng N,Siebner HR,Ward NS,et al.How does transcranial DC stim-
ulation of the primary motor cortex alter regional neuronal activity in the human brain?Eur J Neurosci2005;22(2):495-504.
44.Parra LC,Bikson M.Model of the effect of extracellular?elds on
spike time coherence.Conf Proc IEEE Eng Med Biol Soc2004;6: 4584-4587.
45.Deans JK,Powell AD,Jefferys JG.Sensitivity of coherent oscilla-
tions in rat hippocampus to AC electric?elds.J Physiol2007;
583(Pt2):555-565.
46.Frohlich F,McCormick DA.Endogenous electric?elds may guide
neocortical network activity.Neuron2010;67(1):129-143.
47.Accornero N,Li V oti P,La Riccia M,Gregori B.Visual evoked
potentials modulation during direct current cortical polarization.
Exp Brain Res2007;178(2):261-266.
48.Matsunaga K,Nitsche MA,Tsuji S,Rothwell JC.Effect of transcra-
nial DC sensorimotor cortex stimulation on somatosensory evoked potentials in humans.Clin Neurophysiol2004;115(2):456-460. 49.Antal A,Kincses TZ,Nitsche MA,Bartfai O,Paulus W.Excitability
changes induced in the human primary visual cortex by transcranial direct current stimulation:direct electrophysiological evidence.
Invest Ophthalmol Vis Sci2004;45(2):702-707.
50.Ferrucci R,Marceglia S,Vergari M,et al.Cerebellar transcranial
direct current stimulation impairs the practice-dependent pro?ciency increase in working memory.J Cogn Neurosci2008;20(9): 1687-1697.
51.Galea JM,Jayaram G,Ajagbe L,Celnik P.Modulation of cerebellar
excitability by polarity-speci?c noninvasive direct current stimula-tion.J Neurosci2009;29(28):9115-9122.
52.Winkler T,Hering P,Straube A.Spinal DC stimulation in humans
modulates post-activation depression of the H-re?ex depending on current polarity.Clin Neurophysiol2010;121(6):957-961.
53.Merzagora AC,Foffani G,Panyavin I,et al.Prefrontal hemodynamic
changes produced by anodal direct current stimulation.Neuroimage 2010;49(3):2304-2310.
54.Nitsche MA,Jaussi W,Liebetanz D,Lang N,Tergau F,Paulus W.
Consolidation of human motor cortical neuroplasticity by D-cycloserine.Neuropsychopharmacology2004;29(8):1573-1578.
55.Nitsche MA,Liebetanz D,Schlitterlau A,et al.GABAergic modula-
tion of DC stimulation-induced motor cortex excitability shifts in hu-mans.Eur J Neurosci2004;19(10):2720-2726.
56.Nitsche MA,Grundey J,Liebetanz D,Lang N,Tergau F,Paulus W.
Catecholaminergic consolidation of motor cortical neuroplasticity in humans.Cereb Cortex2004;14(11):1240-1245.
57.Kuo MF,Unger M,Liebetanz D,et al.Limited impact of homeostatic
plasticity on motor learning in humans.Neuropsychologia2008;
46(8):2122-2128.
58.Nitsche MA,Doemkes S,Karakose T,et al.Shaping the effects of
transcranial direct current stimulation of the human motor cortex.
J Neurophysiol2007;97(4):3109-3117.
59.Monte-Silva K,Kuo MF,Thirugnanasambandam N,Liebetanz D,
Paulus W,Nitsche MA.Dose-dependent inverted U-shaped effect of dopamine(D2-like)receptor activation on focal and nonfocal plas-ticity in humans.J Neurosci2009;29(19):6124-6131.
60.Nitsche MA,Kuo MF,Karrasch R,Wachter B,Liebetanz D,
Paulus W.Serotonin affects transcranial direct current-induced neu-roplasticity in humans.Biol Psychiatry2009;66(5):503-508.
61.Antal A,Kincses TZ,Nitsche MA,Paulus W.Manipulation of phos-
phene thresholds by transcranial direct current stimulation in man.
Exp Brain Res2003;150(3):375-378.
62.Boggio PS,Rigonatti SP,Ribeiro RB,et al.A randomized,double-
blind clinical trial on the ef?cacy of cortical direct current stimulation for the treatment of major depression.Int J Neuropsychopharmacol 2008;11(2):249-254.
63.Utz KS,Dimova V,Oppenlander K,Kerkhoff G.Electri?ed minds:
transcranial direct current stimulation(tDCS)and galvanic vestibular stimulation(GVS)as methods of non-invasive brain stimulation in
neuropsychology:a review of current data and future implications.
Neuropsychologia2010;48(10):2789-2810.
64.Williams JA,Pascual-Leone A,Fregni F.Interhemispheric modula-
tion induced by cortical stimulation and motor training.Phys Ther 2010;90(3):398-410.
65.Mahmoudi H,Haghighi AB,Petramfar P,Jahanshahi S,Salehi Z,
Fregni F.Transcranial direct current stimulation:electrode montage in stroke.Disabil Rehabil2010November26(Epub ahead of print).
66.Redfearn JW,Lippold OC,Costain R.A preliminary account of the
clinical effects of polarizing the brain in certain psychiatric disorders.
Br J Psychiatry1964;110:773-785.
67.Cogiamanian F,Vergari M,Ardolino G,et al.Effects of transcuta-
neous spinal cord direct current stimulation(tsDCS)on the lower limb nociceptive?exion re?ex in humans.Proceedings of the XLI Congress of the Italian Neurological Society;2010:Neurological Sciences.
68.Datta A,Rahman A,Scaturro J,Bikson M.Electrode montages for
tDCS and weak transcranial electrical stimulation role of"return"
electrode’s position and size.Clin Neurophysiol2010;121: 1976-1978.
69.Moliadze V,Antal A,Paulus W.Electrode-distance dependent after-
effects of transcranial direct and random noise stimulation with ex-tracephalic reference electrodes.Clin Neurophysiol2010;121(12): 2165-2171.
70.Datta A,Elwassif M,Battaglia F,Bikson M.Transcranial current
stimulation focality using disc and ring electrode con?gurations: FEM analysis.J Neural Eng2008;5(2):163-174.
71.Datta A,Bansal V,Diaz J,Patel J,Reato D,Bikson M.Gyri-precise
head model of transcranial direct current stimulation:Improved spatial focality using a ring electrode versus conventional rectangular pad.Brain Stimul2009;2(4):201-207.
72.Sadleir RJ,Vannorsdall TD,Schretlen DJ,Gordon B.Transcranial
direct current stimulation(tDCS)in a realistic head model.Neuro-image2010;51(4):1310-1318.
73.Minhas P,Bansal V,Patel J,et al.Electrodes for high-de?nition trans-
cutaneous DC stimulation for applications in drug delivery and elec-trotherapy,including tDCS.J Neurosci Methods2010;190(2): 188-197.
74.Faria P,Leal A,Miranda https://www.360docs.net/doc/571179832.html,paring different electrode con?g-
urations using the10-10international system in tDCS:a?nite element model analysis.Conf Proc IEEE Eng Med Biol Soc2009;
1596-1599.
75.Suh HS,Kim SH,Lee WH,Kim TS.Realistic simulation of transcra-
nial direct current stimulation via3-d high-resolution?nite element analysis:effect of tissue anisotropy.Conf Proc IEEE Eng Med Biol Soc2009;638-641.
76.Elmer S,Burkard M,Renz B,Meyer M,Jancke L.Direct current
induced short-term modulation of the left dorsolateral prefrontal cortex while learning auditory presented nouns.Behav Brain Funct 2009;5(1):29.
77.Marshall L,Molle M,Siebner HR,Born J.Bifrontal transcranial
direct current stimulation slows reaction time in a working memory task.BMC Neurosci2005;6(1):23.
78.Boggio PS,Fregni F,Valasek C,et al.Temporal lobe cortical electri-
cal stimulation during the encoding and retrieval phase reduces false memories.PLoS One2009;4(3):e4959.
79.Marshall L,Molle M,Hallschmid M,Born J.Transcranial direct
current stimulation during sleep improves declarative memory.
J Neurosci2004;24(44):9985-9992.
80.Fecteau S,Knoch D,Fregni F,Sultani N,Boggio P,Pascual-Leone A.
Diminishing risk-taking behavior by modulating activity in the prefrontal cortex:a direct current stimulation study.J Neurosci 2007;27(46):12500-12505.
81.Hecht D,Walsh V,Lavidor M.Transcranial direct current stimulation
facilitates decision making in a probabilistic guessing task.J Neurosci 2010;30(12):4241-4245.
Clinical research with tDCS193
82.Kincses TZ,Antal A,Nitsche MA,Bartfai O,Paulus W.Facilitation
of probabilistic classi?cation learning by transcranial direct current stimulation of the prefrontal cortex in the human.Neuropsychologia 2004;42(1):113-117.
83.Stone DB,Tesche CD.Transcranial direct current stimulation modu-
lates shifts in global/local attention.Neuroreport2009;20(12): 1115-1119.
84.Sparing R,Thimm M,Hesse MD,Kust J,Karbe H,Fink GR.Bidi-
rectional alterations of interhemispheric parietal balance by non-invasive cortical stimulation.Brain2009;132(Pt11):3011-3020. 85.Bolognini N,Fregni F,Casati C,Olgiati E,Vallar G.Brain polariza-
tion of parietal cortex augments training-induced improvement of visual exploratory and attentional skills.Brain Res2010;1349:76-89.
86.Fertonani A,Rosini S,Cotelli M,Rossini PM,Miniussi C.Naming
facilitation induced by transcranial direct current stimulation.Behav Brain Res2010;208(2):311-318.
87.Sparing R,Dafotakis M,Meister IG,Thirugnanasambandam N,
Fink GR.Enhancing language performance with non-invasive brain stimulation d a transcranial direct current stimulation study in healthy humans.Neuropsychologia2008;46(1):261-268.
88.Liebetanz D,Koch R,Mayenfels S,Konig F,Paulus W,Nitsche MA.
Safety limits of cathodal transcranial direct current stimulation in rats.Clin Neurophysiol2009;120(6):1161-1167.
89.Fregni F,Liebetanz D,Monte-Silva KK,et al.Effects of transcranial
direct current stimulation coupled with repetitive electrical stimula-tion on cortical spreading depression.Exp Neurol2007;204(1): 462-466.
90.Rigonatti SP,Boggio PS,Myczkowski ML,et al.Transcranial direct
stimulation and?uoxetine for the treatment of depression.Eur Psychiatry2008;23(1):74-76.
91.Ferrucci R,Bortolomasi M,Vergari M,et al.Transcranial direct
current stimulation in severe,drug-resistant major depression.
J Affect Disord2009;118(1-3):215-219.
92.Palm U,Keeser D,Schiller C,et al.Skin lesions after treatment
with transcranial direct current stimulation(tDCS).Brain Stimul 2008;1(4):386-387.
93.Durand S,Fromy B,Bouye P,Saumet JL,Abraham P.Vasodilatation
in response to repeated anodal current application in the human skin relies on aspirin-sensitive mechanisms.J Physiol2002;540(Pt1): 261-269.
94.Poreisz C,Boros K,Antal A,Paulus W.Safety aspects of transcranial
direct current stimulation concerning healthy subjects and patients.
Brain Res Bull2007;72(4-6):208-214.
95.Tadini L,El-Nazer R,Brunoni AR,et al.Cognitive,mood and EEG
effects of noninvasive cortical stimulation with weak electrical currents.J ECT2010October5.(Epub ahead of print).
96.Nitsche MA,Liebetanz D,Lang N,Antal A,Tergau F,Paulus W.
Safety criteria for transcranial direct current stimulation(tDCS)in humans.Clin Neurophysiol2003;114(11):2220-2222.author reply 2-3.
97.Fregni F,Boggio PS,Nitsche MA,Rigonatti SP,Pascual-Leone A.
Cognitive effects of repeated sessions of transcranial direct current stimulation in patients with depression.Depress Anxiety2006;
23(8):482-484.
98.Boggio PS,Nunes A,Rigonatti SP,Nitsche MA,Pascual-Leone A,
Fregni F.Repeated sessions of noninvasive brain DC stimulation is associated with motor function improvement in stroke patients.
Restor Neurol Neurosci2007;25(2):123-129.
99.Loo C,Martin D,Pigot M,Arul-Anandam P,Mitchell P,Sachdev P.
Transcranial direct current stimulation priming of therapeutic repet-itive transcranial magnetic stimulation:a pilot study.J ECT2009;
25(4):256-260.
100.Fregni F,Thome-Souza S,Nitsche MA,Freedman SD,Valente KD, Pascual-Leone A.A controlled clinical trial of cathodal DC polariza-tion in patients with refractory epilepsy.Epilepsia2006;47(2): 335-342.101.Arul-Anandam AP,Loo C,Mitchell P.Induction of hypomanic episode with transcranial direct current stimulation.J ECT2010;
26(1):68-69.
102.Baccaro A,Brunoni AR,Bensenor IM,Fregni F.Hypomanic episode in unipolar depression during transcranial direct current stimulation.
Acta Neuropsychiatrica2010;22(6):316-318.
103.Brunoni AR,Valiengo L,Zanao T,Oliveira J,Bensenor IM,Fregni F.
Manic psychosis following transcranial direct current stimulation and sertraline.J Neuropsychiatry Clin Neurosci(in press).
104.Miranda PC,Faria P,Hallett M.What does the ratio of injected current to electrode area tell us about current density in the brain during tDCS?Clin Neurophysiol2009;120(6):1183-1187.
105.Fregni F,Boggio PS,Valle AC,et al.Homeostatic effects of plasma valproate levels on corticospinal excitability changes induced by1Hz rTMS in patients with juvenile myoclonic epilepsy.Clin Neurophy-siol2006;117(6):1217-1227.
106.Ziemann U.Pharmacology of TMS.Suppl Clin Neurophysiol2003;
56:226-231.
107.Bajwa S,Bermpohl F,Rigonatti SP,Pascual-Leone A,Boggio PS, Fregni F.Impaired interhemispheric interactions in patients with major depression.J Nerv Ment Dis2008;196(9):671-677.
108.Soubasi E,Chroni E,Gourzis P,Zisis A,Beratis S, Papathanasopoulos P.Cortical motor neurophysiology of patients with schizophrenia:a study using transcranial magnetic stimulation.
Psychiatry Res2010;176(2-3):132-136.
109.Mhalla A,de Andrade DC,Baudic S,Perrot S,Bouhassira D.Alter-ation of cortical excitability in patients with?bromyalgia.Pain2010;
149(3):495-500.
110.Brighina F,Palermo A,Fierro B.Cortical inhibition and habituation to evoked potentials:relevance for pathophysiology of migraine.
J Headache Pain2009;10(2):77-84.
111.Chaieb L,Antal A,Paulus W.Gender-speci?c modulation of short-term neuroplasticity in the visual cortex induced by transcranial direct current stimulation.Vis Neurosci2008;25(1):77-81.
112.Ferri R,Del Gracco S,Elia M,Musumeci SA.Age-related changes of cortical excitability in subjects with sleep-enhanced centrotempo-ral spikes:a somatosensory evoked potential study.Clin Neurophy-siol2000;111(4):591-599.
https://www.360docs.net/doc/571179832.html,ng N,Hasan A,Sueske E,Paulus W,Nitsche MA.Cortical hypo-excitability in chronic smokers?A transcranial magnetic stimulation study.Neuropsychopharmacol2008;33(10):2517-2523.
114.Monte-Silva K,Liebetanz D,Grundey J,Paulus W,Nitsche MA.
Dosage-dependent non-linear effect of L-dopa on human motor cortex plasticity.J Physiol2010;588(Pt18):3415-3424.
115.Ohn SH,Park CI,Yoo WK,et al.Time-dependent effect of transcra-nial direct current stimulation on the enhancement of working memory.Neuroreport2008;19(1):43-47.
116.Boggio PS,Ferrucci R,Rigonatti SP,et al.Effects of transcranial direct current stimulation on working memory in patients with Parkinson’s disease.J Neurol Sci2006;249(1):31-38.
117.Froc DJ,Chapman CA,Trepel C,Racine RJ.Long-term depression and depotentiation in the sensorimotor cortex of the freely moving rat.J Neurosci2000;20(1):438-445.
118.Monte-Silva KK,Kuo MF,Liebetanz D,Paulus W,Nitsche MA.
Shaping the optimal repetition interval for cathodal transcranial direct current stimulation(tDCS).J Neurophysiol2010;103(4): 1735-1740.
119.Siebner HR,Lang N,Rizzo V,et al.Preconditioning of low-frequency repetitive transcranial magnetic stimulation with transcra-nial direct current stimulation:evidence for homeostatic plasticity in the human motor cortex.J Neurosci2004;24(13):3379-3385. 120.Brunoni AR,Fregni F.Clinical trial design in noninvasive brain stim-ulation psychiatric research.Int J Methods Psychiatry Res(in press). 121.Gandiga PC,Hummel FC,Cohen LG.Transcranial DC stimulation (tDCS):a tool for double-blind sham-controlled clinical studies in brain stimulation.Clin Neurophysiol2006;117(4):845-850.
194Brunoni et al
软件操作说明书
智能蓝精灵 门禁考勤管理软件 使 用 说 明 书
软件使用基本步骤
一.系统介绍―――――――――――――――――――――――――――――2二.软件的安装――――――――――――――――――――――――――――2三.基本信息设置―――――――――――――――――――――――――――2 1)部门班组设置 -------------------------- 3 2)人员资料管理 -------------------------- 3 3)数据库维护 ---------------------------- 3 4)用户管理 ------------------------------ 3 四.门禁管理―――――――――――――――――――――――――――――4 1)通迅端口设置―――――――――――――――――――――――――4 2)控制器管理――――――――――――――――――――――――――4 3)控制器设置 ---------------------------- 6 4)卡片资料管理―――――――――――――――――――――――――11 5)卡片领用注册 ------------------------- 12 6)实时监控 ----------------------------- 13 五.数据采集与事件查询――――――――――――――――――――――――13六.考勤管理―――――――――――――――――――――――――――――14 1 )班次信息设置――――――――――――――――――――――――――14 2 )考勤参数设置--------------------------------------------------------- 15 3 )考勤排班------------------------------------------------------------- 15 4 )节假日登记―――――――――――――――――――――――――――16 5 )调休日期登记――――――――――――――――――――――――――16 6 )请假/待料登记―――――――――――――――――――――――――17 7 )原始数据修改――――――――――――――――――――――――――17 8 )考勤数据处理分析――――――――――――――――――――――――17 9 )考勤数据汇总―――――――—――――――――――――――――――18 10 )考勤明细表—―――――――――――――――――――――――――18 11 )考勤汇总表――――――――――――――――――――――――――18 12 )日打卡查询――――――――――――――――――――――――――18 13 )补卡记录查询—――――――――――――――――――――――――19
TDCS系统
铁路线上有三道安全保障:CTCS系统、LKJ、人工调度。 每列动车上都装有CTCS系统,即中国列车运行控制系统。它能把一条线上所有运行列车所在位置、列车之间的关系及她们的运行速度确定下来,列车按照规定走,就能保证在一条线上,所有运行的列车就是有序的,安全的。如果前车停驶,系统应给后车发出信息,在停驶车后面有一个安全区间,其她列车必须在到达安全区间前停下来。 第二重,LKJ系统,即列车运行监控装置。列车监控装置这套系统原先不就是为了动车运行的,它就是原 来系统里的电力机车与内燃机车上采用的装置,就是用来保障列车运行安全的。列车在正线运行时,用CTCS 系统,下了正线后,就没有CTCS系统了,这时用LKJ系统。 除以上两套系统,还有人工调度。当列车运行发生故障,CTCS系统会把情况反映到调度室,调度室应该马上就知道列车停驶了。调度室通过车站或机务部门去联系,首先可以问最近的车站,车站可以跟司机联系,了解列车出了什么问题。 TDCS(Train Operation Dispatching Command System)就是覆盖全路的调度指挥管理系统,能及时、准确地为全路各级调度指挥管理人员提供现代化的调度指挥管理手段与平台。TDCS系统就是个全路联网的调度指挥系统,它由部中心TDCS系统,铁路局TDCS系统,车站系统三层机构有机地组成的,它采用数字化、网络化、信息化技术,就是对传统调度指挥模式的革命性突破,它极大地减轻了调度员的劳动强度,提高了运输生产的效率。在TDCS系统基础上建设调度集中,就是铁路跨越式发展的必经之路,所以TDCS系统为铁路调度实现现代化打下坚实基础。 TDCS系统的重点在直接指挥车站的路局TDCS系统一层,路局TDCS实现对全路局的行车进行实时、集中、透明指挥,用自动化的手段调整运输方案,通过计算机网络下达行车计划与调度命令,实现自动报点与车次号自动跟踪,改变过去车站值班员用电话向调度员人工报点、调度员用电话向车站下达计划与命令,车站手抄再复诵的落后方式。列车实际运行图自动绘制,自动过表,车站行车日志自动生成。这些都大大减轻了行车调度员与车站值班员工作强度。TDCS工程建成后,优化了运输调度指挥管理手段、提高了调度管理水平 调度办公-无纸化 行调台延续多年的一张图,一只笔,一把尺,一块像皮的工作模式将被现代化的TDCS 行调子系统所替代,调度员通过简单的点击鼠标即可实现运行线的自动铺画,调整,下达阶段计划与调度命令等操作。列车运行的到发点由系统自动采集,实际运行线自动生成。每班的运行图可打印输出。以计算机替代重复、简单的作业环节,减少作业员的工作环节、劳动强度。 流程管理-程序化 通过详细描述列调工作中的设备、规则、方式、流程等条件,由程序智能控制作业流程,规范作业过程管理。
超纯水系统操作说明书
水处理设备(超纯水系统) 操 作 说 明 书
目录 一、超纯水设备工艺流程图: (2) 二、工艺流程说明: (2) 1.原水箱 (2) 2.原水泵 (2) 3.多介质过滤器 (3) 4.活性碳过滤器 (3) 5.阻垢剂加药系统 (3) 6.软化器 (4) 7.精密保安过滤器 (4) 8.高压泵 (4) 9.两级反渗透RO机 (5) 10、二级纯水箱 (12) 11、EDI输送泵 (12) 12、前置紫外杀菌器 (13) 13、0.22μ微滤系统 (13) 14、EDI装置 (13) 15、EDI超纯水箱 (17) 16、输送泵 (17) 17、核级树脂 (17) 18、后置紫外线杀菌器 (18) 19、终端0.22μ微滤系统 (19) 三、设备操作指南: (19)
四、设备维护与保养:(以原水水质与纯水水质而定) (19) 附表1:水处理设备运行记录表 (21) 附表2:水处理设备维修保养记录表 (22) 附录3:售后服务承诺 (23) 一、超纯水设备工艺流程图: 二、工艺流程说明: 1.原水箱 原水箱作为储水装置,调节系统进水量与原水泵抽送量之间的不平衡,避免原水泵启停过于频繁,箱内设置液位,原水进水阀根据液位高低进行自动补水,原水泵根据水池液位情况自动启停。 操作:原水箱顶部设置手动及自动电动进水阀,可进行手动及自动补水; 手动补水时不受液位控制,只能手动控制。自动补水阀补水时受液位控制,
当水箱液位降到设定中液位时,自动阀开启自动补水;当水箱液位达到设定高液位时,自动阀关闭停止补水,从而达到自动的性能。 2.原水泵 作用:原水泵将原水增压后输送到下道工序,保证多介质过滤器、活性炭过滤的操作压力及运行流量。 操作:原水泵可分手动和自动操作,自动运行时,原水泵将与原水箱液位联动,原水箱液位低时原水泵停止运行,中水位时重新启动;手动操作时除原水箱液位液位不与原水泵连锁外,其他和自动一样;其他有关说明及注意事项详见水泵说明书。 3.多介质过滤器 作用:在水质预处理系统中,多介质过滤器压力容器内不同粒径的石英砂按一定级配装填,经絮凝的原水在一定压力下自上而下通过滤料层,从而使水中的悬浮物得以截留去除,多介质过滤器能够有效去除原水中悬浮物、细小颗粒、全价铁及胶体、菌藻类和有机物。其出水SDI15(污染指数)小于等于5,完全能够满足反渗透装置的进水要求。 操作:多介质过滤器的反洗操作采用自动控制器,过滤器应定期清洗。冲洗周期一般为5~7个工作日,具体将根据进水浊度而定。 4.活性碳过滤器 功能:在水质预处理系统中,活性炭过滤器能够吸附前级过滤中无法去除的余氯以防止后级反渗透膜受其氧化降解,同时还吸附从前级泄漏过来的小分
ERP系统操作说明书(完整版)
在使用本软件时,需要对IE作如下设置: 1)需设置工具->Internet属性->浏览历史记录->设置->设置检查所存网页的较新 2)把“格安信息协同工作”网站加入可信任站点:工具->Internet属性->安全->可信站点->站点->加入该站点IP,如图所示: 系统使用流程 1.立项:市场部人员点击导航栏->项目管理->填写立项选项,申请一个新的项目立项,下 面的附件管理可以添加该项目立项所需附件,在确认立项前可以修改相关内容,如图所示:
注意:在填写新的立项时一定要设置状态为“立项”,否则该项目无法进行确认。 2.确认立项:填写完立项后,执行部门的部门经理就可以对项目进行确认了。如果没有问 题,点击导航栏->项目管理->确认立项选项,然后点击提交审批,在审批过程中,可以 3.审批:总经办人员对项目进行审批,点击导航栏->项目管理->立项审批或从首页提示中 直接点击进入,如图所示,同意立项则点击审批按钮。
4.财务审核:财务人员点击导航栏->项目管理->立项财务审核或从首页提示中直接点击进 入,财务人员可以根据项目情况选择下面的修改项目信息对该项目进行修改,该项目无问题后,点击下方“财务审批”按钮进行审核。 5.部门经理制作预算:首先点击导航栏->项目管理->收入预算,对该项目预计收入进行添 加, 注意:此处预算与员工报销时的费用密切相关,必须仔细且与财务名目一致,如果细类不准确,如办公费预算不足了,即使总预算未超,员工也无法进行该项费用报销 然后点击导航栏->项目管理->估算经费,对该项目预计花费进行添加,
最后点击导航栏->项目管理->提交预算审批,对该项目预算进行提交,等待审批。 6.预算审批:预算审批人员对预算进行审批。 7.预算财务审核:财务人员对预算进行审核。 8.指定项目经理:该项目承接部门负责人指定项目经理, 点击导航栏->项目管理->指定项 目经理,选中被批准过的项目,点击选中该项目,在弹出的界面选择下面的添加,指定项目经理及其任职时间。
TDCS系统介绍
列车调度指挥系统 TDCS 2006年4 月 卡斯柯信号有限公司 CASCO SIGNAL LTD.
目录 1 概述 (4) 1.1TDCS系统背景描述 (4) 1.2相关术语 (4) 1.3TDCS系统特点 (5) 1.4系统的技术条件 (5) 1.4.1设备正常运行适应的环境技术指标 (6) 1.4.2设备运输及储存要求 (6) 1.4.3产品绝缘电阻、耐压指标 (6) 1.4.4系统接地要求 (7) 1.4.5数据传输系统指标 (7) 1.4.6硬件接口、软件接口所遵循的协议标准 (7) 1.4.7系统防尘要求 (8) 1.4.8系统可靠性 (8) 2 TDCS系统构成 (9) 2.1全路TDCS系统四层体系结构 (9) 2.2铁路局TDCS系统结构 (10) 2.2.1调度所设备 (12) 2.2.1.1电源设备: (12) 2.2.1.2微机设备: (12) 2.2.1.3网络设备: (14) 2.2.1.4调度台设备 (14) 2.2.1.4.1行调度台设备 (15) 2.2.2车站设备 (16) 2.2.2.1车站计算机及网络设备由以下设备构成: (17) 2.2.2.1.1设备构成 (17) 2.2.2.1.2车站子系统的功能如下: (17) 2.2.2.2分机采集及控制设备由以下设备构成: (17) 2.2.2.2.1设备构成 (17) 2.2.2.2.2采集设备功能 (18) 2.3与其它设备的接口 (18) 2.3.1与TMIS系统接口 (18) 2.3.2无线车次号及无线调度命令 (19) 3 铁路局TDCS系统功能 (21) 3.1铁路局TDCS系统总体功能要求 (21) 3.2调度监督功能要求 (21) 3.3运行图显示功能 (22) 3.4调度命令及统计功能 (23) 3.5铁路局大屏功能 (23) 3.5.1分界口运输状况宏观显示 (23) 主要干线运输状况宏观显示及展开内容 (25)
软件系统需求说明书
专 组号:小组成员: 完成时间:
目录 1.系统概述 (3) 1.1. 系统功能简介 (3) 1.2 系统用户角色 (3) 2.理由 (3) 3.项目范围 (3) 4.系统假设 (3) 5.系统定义 (4) 6.用户场景 (5) 7.用户用例 (5) 7.1 用户用例步骤 (5) 7.2系统需求 (9) 7.2.1 功能需求 (9) 7.2.2 非功能需求 (12) 8.文档历史 (14)
1.系统概述 1.1. 系统功能简介 教务处工作人员根据设置的用户名和密码,登录到学生信息管理系统,并对学生提交的信息修改进行审核,,系统优先级高; 档案管理员添加、查看、删除、修改学生的基本信息, 系统优先级高; 老师查看自己所管班级的学生的信息, 系统优先级高; 学生修改、查看自己的某些信息, 系统优先级高; 1.2 系统用户角色 2.理由 由于现在的学校规模在逐渐的扩大,设置的专业类别、分支机构及老师、学生人数越来越多,对于过去的学生信息管理系统,不能满足当前学生信息管理的服务性能要求。本报告对于开发新的<<学生信息管理系统>>面临的问题及解决方案进行初步的设计与合理的安排,对用户需求进行了全面细致的分析,更清晰的理解学生信息管理系统业务需求,深入描述软件的功能和性能与界面,确定该软件设计的限制和定义软件的其他有效性需求,对开发计划进行了总体的规划确定开发的需求与面临困难的可行性分析。 3.项目范围 学生信息管理系统是典型的信息管理系统,其开发主要包括后台数据库的建立、维护以及前端应用程序的开发两个方面。对于前者要求建立起数据一致性和完整性强、数据安全性好的数据库。而对于后者则要求应用程序具有功能完备,易使用等特点。学生信息管理系统对全校学生实行统一的管理,可以方便的进行增添、查询、修改、删除学生信息的工作。为了使本系统成功达到用户的要求,需要在2012.12.28之前完成本系统的开发测试,并写提交相关的技术文档。通过与用户的沟通,及时获得用户的最新需求以便于本系统的完善。 4.系统假设 本项目的开发时间为2012.9.9—2012.12.28 开发人员人数:3人 技术文档写作人员人数3人
反渗透系统控制操作说明书
反渗透系统控制操作说明书 一、 概述 如图所示是反渗透系统的工艺流程图,系统主要有以下几个部分组成:原水箱、原水泵、加药装置、砂滤器、炭滤器、精滤器、高压泵、RO 装置、除盐水箱、除盐水泵。 从外部过来的原水从原水泵输出后经砂滤器、炭滤器、精滤器过滤后RO 装置处理后进入除盐水箱,除盐水供给用户使用。RO 装置在正常运行时第隔一段时间再生一次,以保证装置的除盐水能力。 二、 技术参数 电源:三相四线(660V/50HZ );控制电源:(220V/50HZ ) 装机容量:40KW 环境温度:—20℃---—60℃ 相对湿度:不大于80%(25℃) 工作环境:矿井 防护等级:矿用隔爆型 电机防护等级:MA 原水泵:660V/50HZ ,2.2KW 数量:2台 高压泵:660V/50HZ ,7.5KW 数量:1台 除盐水泵:660V/50HZ ,4KW 数量:2台 原水箱 原水泵 W1 Y1 H1 H2 加药装置高压泵 F1 B1B2 B3 Y2 砂滤器炭滤器 Y3 加药装置Y4 精滤器 F2 除盐水箱 除盐水泵 W2 B4B5 F3 F4 RO装置
加药泵:660V/50HZ ,0.55KW 数量:4台 阀门:DC24V 50W 数量:5台 机箱外形尺寸:1200×1000×800 电机绝缘等级:F 级 控制方式:手动/自动 三、 操作方式 如图所示是控制箱的面板布置图: 参数显示 手动/自动ON/OFF 原水泵A/B 除盐水A/B 除盐水远程/本机 原水进水阀 开/关反渗透进水阀 开/关反渗透浓水阀 开/关 反渗透淡水阀 开/关备用 原水泵A启动原水泵A停止原水泵B启动原水泵B停止高压泵启动高压泵停止除盐水泵A启动除盐水泵A停止除盐水泵B启动除盐水泵B停止加药泵1启动加药泵1停止加药泵2启动加药泵2停止加药泵3启动加药泵3停止加药泵4启动加药泵4停止 反渗透启动 反渗透停止 系统在就地PLC 控制状态下,包含自动/手动控制: 3.1手动操作 手动时,可按工艺要求启动每台用电设备;操作时将“手动/自动”开关拔到“手动”位置: 泵的操作:按启动键启动泵,按停止键停上泵的运行。 阀的操作:将旋钮开转到开位置时阀打打,转到关位置时阀门关闭。 3.2自动操作
软件的系统操作手册
3DMS监控平台软件使用说明书 版本:4.23 深圳市亚美达通讯设备有限公司
目录 1、系统登录 (3) 2、主界面 (4) 2.1标题栏 (4) 2.2菜单栏 (4) 2.3工具栏 (4) 2.4状态栏 (4) 2.5树形区 (4) 2.6地图区 (5) 2.7简明信息区 (6) 2.8报警区 (6) 3、监控站点界面 (7) 3.1组态图形 (7) 3.2数据列表 (8) 3.3单灯 (8) 3.4监控点资料 (9) 4、配电箱端的远程操作 (10) 4.1遥测数据 (11) 4.2设置自动开关灯时间 (11) 4.3手动开关灯 (12) 4.4校准时钟 (13) 4.5设置采集器参数 (13) 5、单灯监控 (14) 5.1报警信息 (14) 5.2监测数据 (14) 5.3单灯手动开关灯控制 (15) 5.4单灯配置管理 (15) 6、报表 (17) 6.1监控数据 (17) 6.2故障记录 (17) 6.3监控点数据 (18) 6.4操作记录 (18) 7、数据配置 (19) 7.1监控点管理 (19) 7.2设备管理 (19) 7.3监控项管理 (20) 7.4人员管理 (20) 7.5字典管理 (21) 7.6时间表管理 (21) 8、常见问题 (22)
1、系统登录 启动客户端软件(3DMS.exe),出现登录界面,输入正确的用户名和登录密码,点击登录按钮即可进入监控软件。
2、主界面 主界面采用Windows标准风格,分为: 2.1标题栏:上方第一行,包括软件名称、Windows标准缩放按钮。 2.2菜单栏:上方第二行,为软件功能的菜单。 2.3工具栏:上方第三行,软件常用功能的快捷方式图标。 2.4状态栏:最下方一行,显示服务器连接状态和登录用户信息。 2.5树形区:左侧,按层次显示所有监控站点,可在监控站点名称上单击右键弹出菜单,执行常用功能,亦可在监控站点名称上双击左
TDCS培训资料
同时有无线车次号自动输入和校核系统 下图为本系统结构示意图 铁道部调度指挥中心 TMIS 铁道局调度指挥中心 现有其它系统 分界口基层调度指挥网车站基层调度指挥网 基层网 系统可靠、准确的TDCS系统车站设备作为整个系统中采集基本信息的单元,对TDCS 统设备包括:TDCS工作起着至关重要的作用。车站电源设备 信息采集及显示设备 网络通信设备 设备配置 TDCS系统最基础的部分,是系统动态信息的来源。它包括TDCS基层网车站设备是 电源屏等。TDCS/CTC、车务终端、路由器、集线器、分机(双机) 系统车务终端设备连接示意图如下:TDCS
2 柜机I-SDI )1图( 3 ( 统系端终务车 )2图
器换转议协 )3图( 4 信息采集系统简介第一节 由它所完成的基本信息采集及处理系统的一项重要功能,TDCS车站信息采集系统是 结果,已成为运输部门科学决策的重要参考,也是计算机辅助调度指挥信息系统可靠运 行的重要基础之一。 车站系统主要功能是通过串口(微机联锁站)或信息采集设备采集本站的设备信息 通道将本站采集的信息传送到调度所及相邻车站,并通过2M和无线车次信息,并且经过网络传送到车务运转室车务终端设备。 主板介绍AIO 一、 .概述1 486DX4为CPU主板的ALL-IN-ONE显示接口、双以太接口、VGA。板上集成了100- 软盘接口、FDC硬盘接口、IDE接口、I/O路双向96路差分模拟量输入、32总线接口、CAN DOC2000板上还有一个鼠标接口等电路此
外,键盘接口、串行接口、RS-232个2并行接口、 配置信息。主板使用CMOS保存EEPROM 电子盘插座,可安装操作系统和应用程序;板上 系统进行工作。WINDOWS NT)上安装的嵌入式DOC英文缩写DISKONCHIP,电子盘( .表示灯定义2 该机复位按钮,需用细尖物插按;:RESET 卡正常运行状态;CAN绿灯闪烁时为卡未初始化,CAN绿灯稳亮时为绿灯: CAN 红灯亮时为该机得电,灭时为该机断电;电源红灯: Eth22网段物理性连接正常,灭灯时表示该机第2红灯:红灯亮时表示该机第 网段物理性连接断开; 2网段内部已初始化,闪烁时表示该机第2绿灯:绿灯稳亮时表示该机第Eth2 ;网段正与外界通信(有数据交换) 1网段物理性连接正常,灭灯时表示该机第1红灯:红灯亮时表示该机第Eth1
MES系统操作说明书
MES系统操作说明文档(针织) 一,管理物料(路径:针织厂->MM物料->管理物料(成衣))。如图 操作说明: 1,使用菜单栏中的新建按钮新增款号,维护款式信息。 2,填写货号,货号描述等相关信息,在没有图像数据处右键调用可上传款式图像,完成后保存。二,尺码管理(路径:针织厂->PB公用->尺码管理)。如图
1,使用新建按钮 2,填写红色尺码分组名称, 3,使用尺码分组下面的新增按钮 4,填写尺码编码和尺码名称, 5,红色字体部份为必填项。 三,生产订单(路径:针织厂->SD订单->生产订单(服装))。如图 操作说明 1,图上1为菜单栏图2为已制订单信息,可以点击行查询相关订单明细数据,图3为订单详细。 2,使用系统菜单右边上的’<’号可隐藏菜单栏从而扩大订单明细界面。 3,通过速查下方的向下键可设置查询条件查询已制作过的订单信息。 4,使用菜单栏中的新建按钮新增一新的订单。 5,填写3处的表头部份,如客户名称,订单类型,合同号,加工类型,制作单日期等相关容,下单总量为系统自动生成不需求录入。 6,如果在下拉列表中未能找到相应的客户,使用按钮。
1),使用菜单栏新建按钮后填写客户信息,红色字体为必填项,维护完成后保存即可。 7, 1),选择款式批次子页面,新增款式批次信息。 2),填写相应生产工厂,货号,货名,成份,纱支,针型为系统自动带出,批号,工厂,交期,交地为必填项。 8, 1),选择颜色尺码子页面,新增纱线色号。
2),使用新建按钮,增加色号和色名以及相应的RGB值,红色字体为必填项,保存即可。 注:如无色名,可直接填写色号。 9,选择尺码。
车站概念
深圳北站——“立体化车站”设计 引申:“立体快巴”概念 与“引爆新能源汽车” 发展“立体快巴”无需占用新的道路资源。“路上最多的车是私家车,但最矮的车也是私家车,城市立交桥底的高度大约是4.5至5.5米,…立体快巴?就是利用了2米以上、4.5米以下的空间。”宋有洲说,一般巴士和快速公交系统(BRT)占用路面资源多,而地铁投资成本高、建设时间长,“立体快巴”刚好能避免以上缺点,并能令塞车情况减少20%至30%。 这种零排放的“立体快巴”是我国完全自主创新、全世界独有的产品。每公里造价约500 0万元人民币,较地铁每公里造价5亿至8亿低了九成,一年可建40公里,而建同样里程的地铁则需3年。 “最为重要的是,快巴比地铁和轻轨灵活。”宋有洲介绍,快巴的车厢是可以卸载的,在非高峰期,可以只开一节或者两节车厢,发车间隔时间也可以自由调整。 为了让充电方便,沿途还设置了充电桩,车辆行驶时只要接触就能充上电。此外,夜间不使用时,“立体快巴”可停泊在车站,不需另占停车场,也不影响下方车辆继续通过。 在张文波看来,“立体快巴”最大优点是,在不改变平面交通形态的条件下,使交通立体化,使载客量可达到地铁的载客量,降低城市公共交通投入,并提高运行效率。 他表示,门头沟之所以选择“立体快巴”,是因为其环保、高效、节能的运营模式,符合该区中芬生态城的发展定位,“先期试验线将选在门头沟区中芬生态谷与市区连接线上,距离约为9公里。” 根据目前规划,未来将在门头沟建设189公里的“立体快巴”轨道,除先期的9公里试验线外,门头沟至首都机场将建约120公里的轨道,中芬生态谷将建约60公里的环线。这些轨道之间将彼此贯通,以实现车辆顺畅通行。 广州地铁5号线“小北站”:
污水处理实用工艺及操作说明书
污水处理工艺及操作说明 、工程名称 富德(白山)麦矿泉有限公司年产100万吨天然麦饭石泉水建设项目 、工程概况 1、污水水量 污水处理部分50T/d。 2、进水水质(详见水质检测报告) 般生活污水如下表: 3、出水水质 处理设备的出水水质达到国家回用标准 4、处理工艺 污水拟采用MBF工艺处理。设备材质:碳钢防腐。控制系统具有手动和自动控制功能,可根据实际需要进行合理选择运行方式。 MBR 污水处理,是现代污水处理的一种常用方式,其采用膜生物反应器(MembraneBioreactor,简称MBR技术是生物处理技术与膜分离技术相结合的 一种新技术,取代了传统工艺中的二沉池,它可以高效地进行固液分离,得到直接使用的稳定中水。又可在生物池内维持高浓度的微生物量,工艺剩余污泥少,极有效地去除氨氮,出水悬浮物和浊度接近于零,出水中细菌和病毒被大幅度去除,能耗低,占地面积小。
三、工艺流程图 图1工艺流程图 四、参考资料 1、 《城市区域环境噪声标准》(GB3096-93 ; 2、 《室外排水设计规范》(GBJ14-87; 3、 《给水排水工程结构设计规范》(GBJ69-84; 4、 《污水综合排放标准》(GB8978-2002 。 5、 《给排水设计手册》 & 《给水排水工程结构设计规范》(GB50069-2002 7、 《MBR S 计手册》 8、 《膜生物反应器 -- 在污水处理中的研究和应用》 wikf r*珈丰 I 口林 atm 图例 空吒If 蜒 tH
9、《简明管道工手册》 五、整体工艺 污水流经人工格栅,捞除大颗粒的悬浮物及杂质后流入污水调节池内,在调节池内进行水质、水量调节,由污水提升泵将污水提升至缺氧池,经缺氧池水解酸化后,流入MBF生化处理系统。整个生化处理系统由前端处理及反应池组成,根据进水水质和出水要求,决定需要及设计前端处理池。反应池内装填浸没式膜,构成MBR好氧的运行模式。 MBRz应池出水可直接进入回用水池,投加消毒剂进行消毒,消毒池出水自流进入清水池,各项水质指标达标后,排放或打入中水管网进行回用。 系统产生的栅渣送至指定垃圾存放地点,与其它垃圾一并处理。 装置有七大主要单元组成:(1)调节池(2)缺氧池(3)MBR A(4)消毒池(5)事故池(6)污泥池(7)清水池。 设备调试结束后,MBR S统进入正式运行阶段。正式运行的监控应由经过培训的专业人员实施。 当MBR系统正式运行时,应先按照设计值设定自控装置,设定结束后,整个系统即可按照设定实现阀门、设备的启动和关闭以及仪表的监控,实现自动运行。 MBR正式运行期间,间歇产水、反洗由自控装置控制完成,操作人员应注意监控,保证稳定运行。同时,操作人员应定期检查污泥性状、曝气效果、产水浊度、跨膜压差等,防止不良状况的累积,减小可能的突发事件造成的影响。如果条件允许,有关以下项目也需要检查和管理:各槽罐的浮渣发生情况、液位、各装置的流量、各机器的电流、活性污泥的MLSS DO水温、pH等。原水、产水的水质如遇特殊情况,可以联系我公司寻求解决方案。 膜组件在运行期间,由于长期进行活性污泥的过滤,膜丝表面会有无机盐和蛋白质等有机物吸附之上,膜压会缓慢上升,为防止膜的过度污堵和抽吸压力过高,需要对膜进行间歇产水、定时反洗、定期加药反洗或离线清洗。 间歇产水和定时反洗由自控系统自动完成。 加药反洗或离线清洗需要操作人员根据运行情况按照标准程序操作完成。详细的操作程序如下: 跨膜压差的读取按照本公司推荐的标准流量运转时,由泵抽吸经膜组件出水引起的初始跨膜压差为1米水柱左右。但是,配管的架设方法和仪表类的位置不同,压差计(真空
11、车站功能说明
1.车站内定时背景音乐播放 公共广播系统通过软件编程、下载主机自动运行,可以预先编辑自动运行程序,平时在火车车站的不同区域为售票、检票、进站、候车、乘降、出站、换乘等播报不同的服务用语和有关注意事项,为提供各项服务,维持车站秩序,有效疏导乘客,乘车先下后上,缩短列车站停时间,确保列车正点,创造了条件,同时可为车站工作人员提供其它作业广播; 2、车站语音广播 公共广播系统配置有远程寻呼站和广播寻呼话筒,通过话筒或寻呼站,实现对一个或多个分区回路的进行广播讲话或广播通知等功能,当发生重大活动、集会、赛事、节日等引起火车客流突变时,作为实施应急客运组织的重要手段,为确保大客流运营组织和乘客安全提供了保障,其中远程寻呼站可可安置距离机房1000米内的任意地方,实现远程广播讲话,实现工作人员不需要到达机房就可进行广播讲话。 3、多套节目播放 公共广播系统配置有MP3播放器、CD播放器、调谐器,满足车站多套节目播放需求,不同分区播放不同音乐,临时插播、广播而不影响其他区域音乐的正常播放。 4、分区广播 公共广播系统将车站或分多个区域,进行分区域广播,通过主机功率分区和控制,可进行不同区域节目独立控制,或多个区域节目控制; 5、多套分控 公共广播系统在已有的网络基础上(或自建局域网)覆盖区域,可用个人电脑接入局域网,安装分控软件,即可享多项分控控制功能,多分控建设在日常使用,简化系统使用;6、定时节目播放 公共广播系统采用软件编程,下载主机自动运行,通过预先编程,可以实现7天24小时自动无人值守运行; 7、定时开关机 公共广播系统采用定时电源管理,通过预先编程,可以实现软件自动控制设备电源自动关闭和打开; 8、公共广播 公共广播系统通过配置有远程寻呼话筒,通过话筒,实现对一个或多个分区回路的进行广播讲话或广播通知等功能,远程寻呼站安装在服务台,工作人员通过远程寻呼站可以进行远程广播,实现远程广播讲话,实现工作人员不需要到达机房就可进行广播讲话。
软件使用说明书模板.最新版本
变电所联网监控系统使用说明书 **发展有限公司 2013.04.01
目录 第一部分设计说明______________________________________ 2一系统概况____________________________________________ 2二系统网络结构________________________________________ 2 三、监控内容及方式_____________________________________ 3 3.1 联网变电所_________________________________________ 3 3.2 监控内容___________________________________________ 4 四、使用环境___________________________________________ 4 五、设计依据___________________________________________ 5第二部分系统功能介绍__________________________________ 6 2.1 主菜单_____________________________________________ 8 2.2 历史曲线__________________________________________ 11 2.3 报警查询__________________________________________ 12 2.4 操作查询__________________________________________ 13 2.5 报表查询__________________________________________ 14 2.6 系统说明__________________________________________ 15 2.7 报警确认__________________________________________ 15第三部分系统故障及维护_______________________________ 16 3.1 系统故障处理______________________________________ 16 3.2 使用注意__________________________________________ 16
TDCS
TDCS TDCS(Train Operation Dispatching Command System)是覆盖全路的调度指挥管理系统,能及时、准确地为全路各级调度指挥管理人员提供现代化的调度指挥管理手段和平台。TDCS 以现代计算机技术、计算机网络技术、通信技术、多媒体技术、数据库技术为基本技术手段,实现对列车在车站和区间运行的实时监视,动态调整、自动生成列车运行三小时阶段计划,实现列车调度命令的自动下达和实迹运行图的自动描绘;实现分界口交接列车数、列车运行正点率、行车密度、早晚点原因、重点列车跟踪等实时宏观统计分析并形成相关统计报表;为各级调度人员提供列车的动态运行情况,便于机车合理调配,提高运输能力和安全程度;显示铁路路网、沿线线路、车站、重要列车和救援列车分布等主要信息,为铁路事故救援、灾害抢险、防洪等提供决策参考。 TDCS系统采用各种新技术与铁路信号技术的特点相互融合,把传统的以车站为单位的分散信号系统逐步改造成为一个全国统一的网络信号系统,由提高安全提高效率向提高运输效能转变,由单一功能向综合功能转变,由模拟传输向数字传输转变,由手工绘制向辅助及自动绘制转变;通过建立一个融先进通信、信号、计算机网络、数据传输、多媒体技术为一体的现代化信息系统,为各级调度人员提供先进的调度指挥和处理手段,提高应变和处理能力,减少调度人员通话和手工制表数量,改善调度指挥人员的工作条件,从而提高铁路运输组织的科学性、劳动生产效率和铁路服务质量,为铁道部生产布局的调整打下了坚实的基础。 系统结构 中心局域网采用高性能的交换机组成双100M 高速以太网,所有设备通过双网卡连接到双局域网上,确保各节点数据传输的可靠性。 车站局域网采用高性能的交换机组成双100M 高速以太网,所有车站设备通过双网卡连接到双局域网上,确保各节点数据传输的可靠性。 调度中心子系统中各子系统之间为通过双冗余局域网实现的以太网网络接口,接口为RJ45 接口规范、网络介质为 5 类双绞线,速率为100M。 调度中心子系统的局域网底层网络协议均符合IEEE802.3 标准。网络节点之间的通信高层协议采用国际通用的互联网TCP/IP 协议。 调度中心与车站之间的网络子系统为双环路广域网连接方式,中心到车站
CIPSIP工艺流程操作说明书
CIPSIP工艺流程操 作说明书
无锡华裕药制有限公司 灭菌系统工艺自控部分 HMI操作说明书 江苏迈登普洁净系统工程有限公司 电气工程部 -4一、配置说明
1.硬件配置 触摸屏:昆仑通态 TPC1061Ti 分辨率为1024 X 600, 10.2" TFT液晶屏, ARM CPU,主频600MHz, 128M DDR2,128M NAND Flash 可编程控制器:CPU S7-200 2.软件配置 1)触摸屏 2)可编程控制器
二、操作要求 1).洁净无油空压0.5MPa以上压力 2).操作空压0.6~0.8MPa 3).纯蒸汽0.15MPa以上压力、130℃以上温度4).电源50HZ,AC220V 三、触摸屏画面及功能简介
1.主流程画面 电源启动,触按屏显示初始画面。 1)“主流程画面”的组成 *在画面的上方是带有公司名称及工艺名称的页眉,右上角日期和时间显示。 *画面中间是由38个气动阀门及3个手动隔膜阀组成的流程示意图。另外有两个温度(TT)显示和两个压力(PT)显示。其中温度的单位为℃,压力的单位为MPa。 *显示 A.电机状态:关闭为灰色;运行为绿色 B.阀门状态:关闭为白色;打开为绿色 C.仪表参数:实时显示 2)画面下方按钮 *系统自动按钮:自动操作模式下,系统自动切换阀门不需手动操
作。 *报警浏览按钮:点击此按钮进入报警浏览画面(后面详述) *SIP操作按钮:点击此按钮进入SIP操作画面(后面详述) *CIP操作按钮:点击此按钮进入实时警报画面(后面详述) *阀门手动控制按钮:点击此按钮进入阀门控制画面(后面详述)*阀门开启条件:详见EXCEL表 整个系统为自动化过程,除非操作人员强行停止,此过程中断。 2.“报警浏览”画面
CIP、SIP实用工艺流程操作说明书
华裕药制 灭菌系统工艺自控部分 HMI操作说明书 迈登普洁净系统工程 电气工程部 2016-4
一、配置说明 1.硬件配置 触摸屏:昆仑通态 TPC1061Ti 分辨率为1024 X 600, 10.2" TFT液晶屏, ARM CPU,主频600MHz, 128M DDR2,128M NAND Flash 可编程控制器:CPU S7-200 2.软件配置 1)触摸屏 2)可编程控制器
二、操作要求 1).洁净无油空压0.5MPa以上压力 2).操作空压0.6~0.8MPa 3).纯蒸汽0.15MPa以上压力、130℃以上温度4).电源50HZ,AC220V 三、触摸屏画面及功能简介
1.主流程画面 电源启动,触按屏显示初始画面。 1)“主流程画面”的组成 *在画面的上方是带有公司名称及工艺名称的页眉,右上角日期和时间显示。 *画面中间是由38个气动阀门及3个手动隔膜阀组成的流程示意图。另外有两个温度(TT)显示和两个压力(PT)显示。其中温度的单位为℃,压力的单位为MPa。 *显示 A.电机状态:关闭为灰色;运行为绿色 B.阀门状态:关闭为白色;打开为绿色 C.仪表参数:实时显示 2)画面下方按钮 *系统自动按钮:自动操作模式下,系统自动切换阀门不需
手动操作。 *报警浏览按钮:点击此按钮进入报警浏览画面(后面详述)*SIP操作按钮:点击此按钮进入SIP操作画面(后面详述)*CIP操作按钮:点击此按钮进入实时警报画面(后面详述)*阀门手动控制按钮:点击此按钮进入阀门控制画面(后面详述) *阀门开启条件:详见EXCEL表 整个系统为自动化过程,除非操作人员强行停止,此过程中断。 2.“报警浏览”画面
4软件系统操作详细说明书
软件系统操作详细说明书: 1)单击桌面上图标HotelLock system3.3出现屏幕的欢迎界面。5秒钟过后,在操作员登陆 窗口上选择操作员并输入密码;单击登陆进入系统主界面;系统默认的超级管理级的操作员SYSTEM的密码是0。点击“登陆”按钮进入主界面。 软件系统菜单操作详情指南 1.系统管理 系统设置菜单包括:系统设置、软件注册、数据库链接、读数据卡、数据备份、数据恢复和退出系统。 1)系统设置(系统管理——系统设置) 初次使用该软件时,请先对软件系统的一些系统参数进行设置。其中包括酒店名称(本酒店名称)、选择门锁类型、通迅端口、设置您们想设定的默认宾客的入住天数和默认退房时间以及退房时间间隔(时间间隔的意思就是让宾客卡在您设定的到期的时间范围后再多给客人几分钟的开门有效期限),系统参数设置完,需要点击“保存”(发卡器要连接在选择的端口上),退出系统。 2)软件注册:(系统管理——软件注册), 软件会随机生成“用户码”,请把“用户码”用电话告之我公司、我公司通过该“用户码”反馈“授权码”输入后点击“注册”, 3)读数据卡(系统管理——读数据卡) 读数据卡:该软件处于感应状态门锁使用时才能发数据卡,客户使用感应门锁时(RF),要提取门锁数据,先发数据卡,对着门锁读一次,门锁即处于信息发送(红外发射),使用手执机接收红外信息(手执机接收红外信息,对准红外发射管)。当手执机在接收红外发射住处时,绿灯闪烁,信息读取完毕时,门锁会发出“嘀”一声,绿灯停止闪烁,说明信息发送成功。与前台发卡器USB连接,点击菜单系统管理、读数据卡、读卡,这里我们门锁上的开
门信息共可以存储198条。 4)数据备份(系统管理——数据备份) 选择保存路径,默认保存路径是C:\Programfiles\locksoft\db,然后输入保存文件名,默认文件名是为当前的日期时间,最后点击保存,系统提示“文件数据备份完毕”。 5)数据恢复(系统管理——数据恢复) 数据恢复时系统会提示“恢复数据会清除现有记录,是否继续”,点击“确定”后,在出现的对话框中选择数据备份文件后,点击打开,系统会提示“文件数据恢复完毕”。 6)退出系统(系统管理——退出系统) 2.客房管理 客房管理菜单包括:客房类型设置、客房资料设置、客人查询和客房查询。 1)客房类型设置(客房管理——客房资料) 点击添加,输入客房类型,人数(即房间入住的人数)房价后点保存。如修改客房类型点击编辑,修改完毕后点保存。如要删除,请选中要删除的记录,点删除即可。 2)客房资料设置(客房管理——客房资料设置) 进入客房资料设置对话框中,点添加,输入房号、楼号、楼层、区域编号、客房类型、客房状态。点保存。如客房房号按顺序排列请点击自动增加房号。如要删除,请选中要删除的客房资料,点删除即可。 3)客人查询(客房管理——客人查询)查询在住客人、所有客人、退房客人一些及信息。4)客房查询(客房管理——客房查询)查询客房状态的一些信息。 3.员工设置 员工设置菜单包括:员工资料、系统登陆和更改密码、语言选项。 1)员工资料:(员工设置——员工资料) 设置系统登陆时的用户,密码和权限及其它相关信息。
车站的作用与分类
火车车站的作用与分类 我国的铁路车站数以千计,而且每年都在变化之中。当新线开通时,会增加若干车站;当旧线改造后,也可能减少若干车站。 车站是办理客货运输的基地,旅客上下车和货物的装卸车以及相关的作业都是在车站进行的。它是铁路与旅客、货主间的纽带,铁路运输与国民经济的发展、市场的需求的关系如何,车站是最好的观察窗口。 另外,车站也是铁路运输的基层生产单位。在车站里,除了办理客货运输各项作业还要办理与列车运行有关的各项作业。例如列车的接发、会让、越行;车列的解体、编组;机车 的换挂、整备;车辆的检查、修理等。 车站有多种分类法,如果按照车站所担负的任务量,以及它在国家政治、经济方面的地位来划分,车站可分为特等站、一等站、二等站、三等站、四等站、五等站共六个等级。显而易见,像北京站、上海站、郑州站等一定是特等站。 如果按车站技术作业的不同来划分,可分为编组站、区段站和中间站。编组站和区段站又统称为技术站。 如果按业务性质来划分,可分为客运站、货运站和客货运站。 中间站 中间站是为沿线城乡人民及工农业生产服务,提高铁路区段通过能力,保证行车安全而设的车站。一般设在技术站之间区段内(或在支线上),它主要办理列车的到发、会让和越行,以及客货运业务。有些中间站还进行机车给水。 中间站设备规模虽然较小,但是数量很多,它遍布全国铁路沿线中、小城镇和农村,在发展地方工农业生产,沟通城乡物资交流中起着很重要的作用。中间站的设置位置,既要符合线路通过能力的要求,又要适当满足地方工农业生产发展的需要,并应考虑地形、地质等自然条件。
中间站的作业和设备 中间站的主要作业有: ——列车的到发、通过、会让和越行; ——旅客的乘降和行李、包裹的承运、保管与交付; ——货物的承运、装卸、保管与交付; ——沿零摘挂列车的车辆摘挂和到货场或专用线取送车辆的调车作业。 有的中间站如有工业企业线接轨或加力牵引起终点以及机车折返时,尚需办理工业企业的取送车、补机的摘挂和机车整备等作业。 为了完成上述作业,中间站应根据作业的性质和工作量大小而设置以下设备: 客运设备——包括旅客站舍(售票房、候车室、行包房)、旅客站台、雨棚和跨越设备(天桥、地道、平过道)等; 货运设备——包括货物仓库、货物站台和货运室、装卸机械等; 站内线路——包括到发线、牵出线和货物线等,它们分别用于接发列车、进行调车和货物装卸作业; 信号及通信设备。 此外,某些中间站还设有机车整备设备和列车检查设备等。 会让站和越行站 在我国铁路上,还有数量不多的主要用来提高线路通过能力而设置的车站,称为会让站和越行站。根据《铁路技术管理规程》规定,会让站和越行站均包括在中间站之内。 会让站设在单线铁路上,主要办理列车的到发和会让,也办理少量的客货运业务。因此,会让站应铺设到发线、旅客乘降设备,并设置信号及通信设备、技术办公用房,但没有专门