Prediction of mineral scale formation in geothermal and oilfield operations using the UNIQUAC model
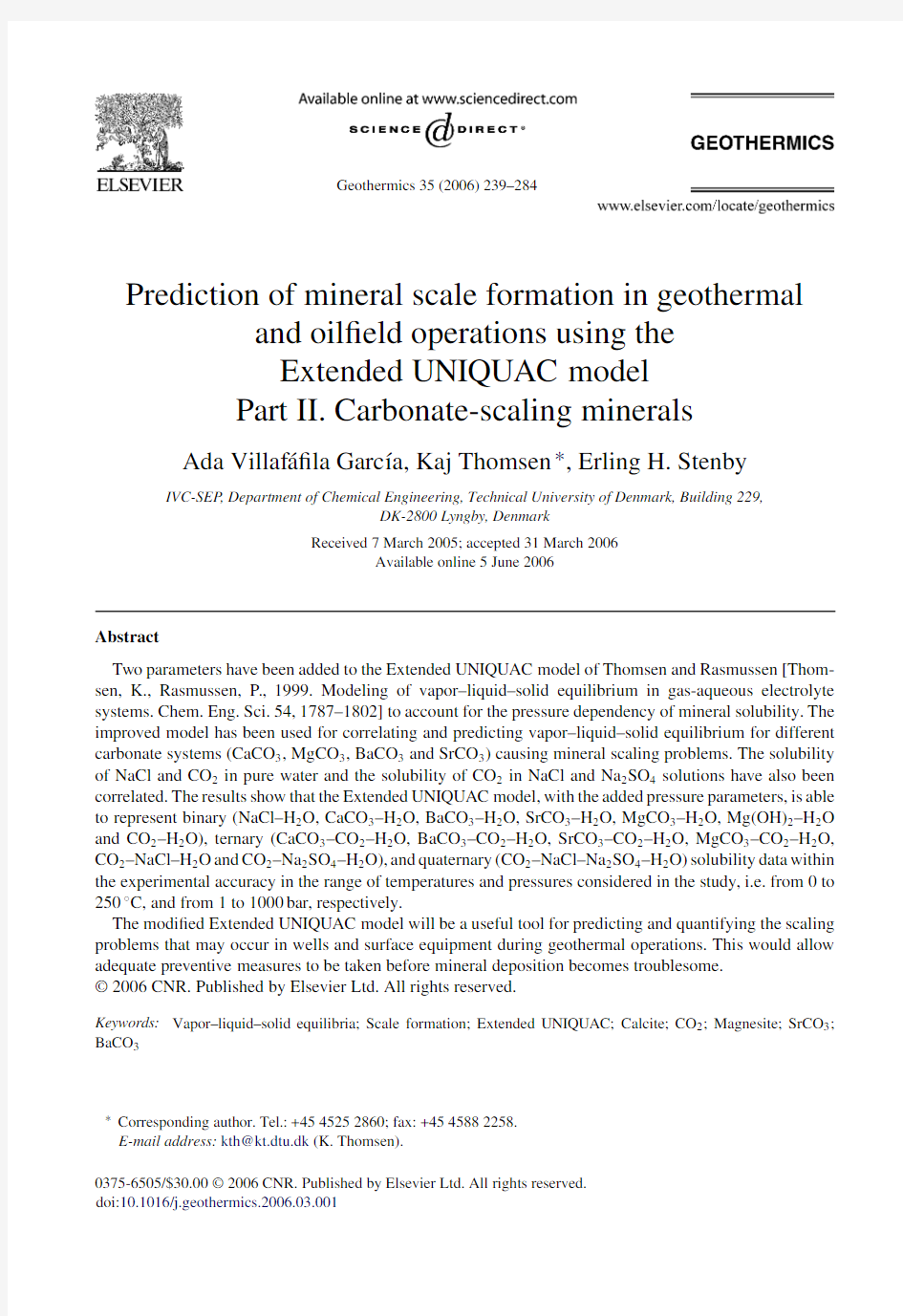
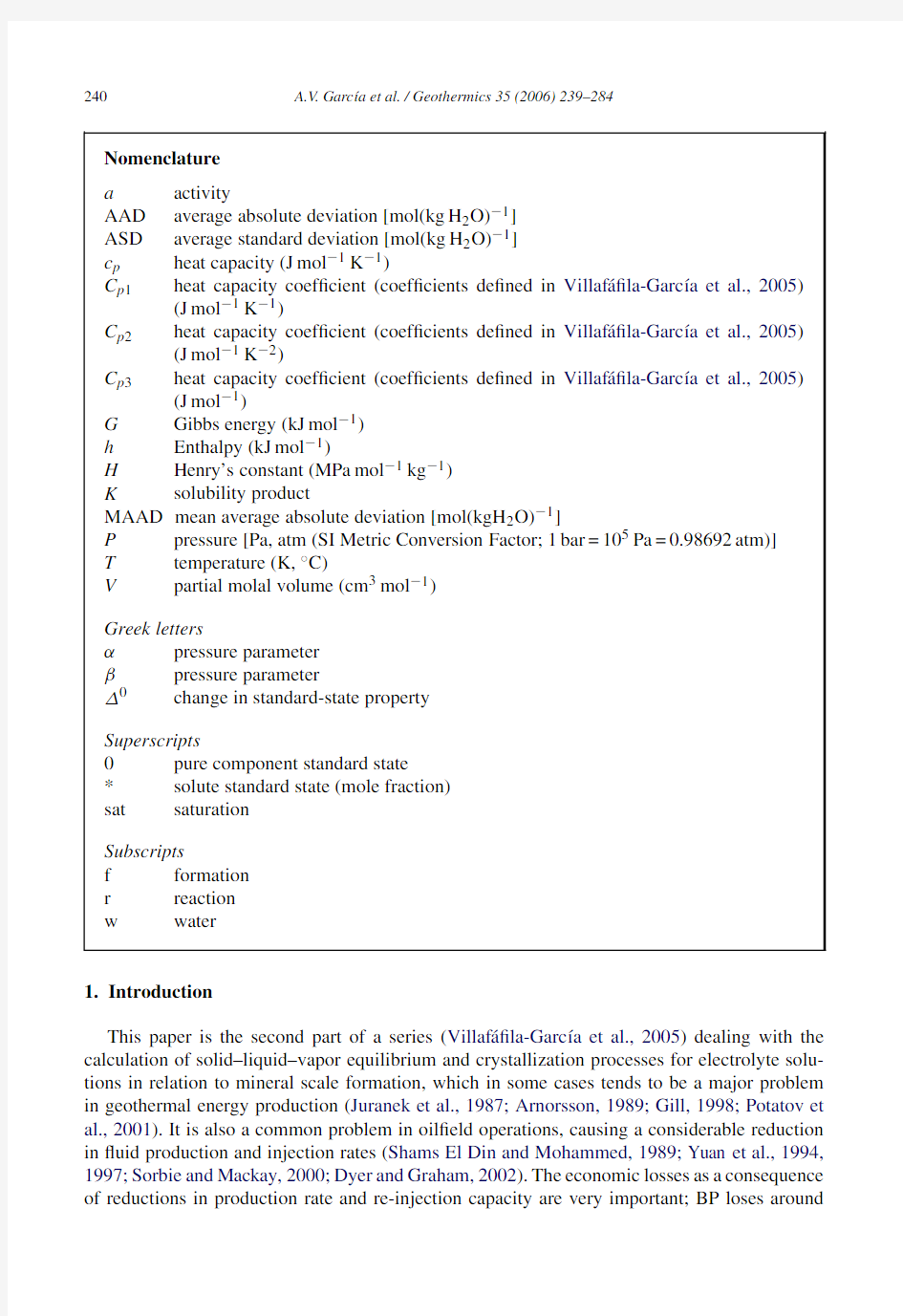
Geothermics35(2006)239–284
Prediction of mineral scale formation in geothermal
and oil?eld operations using the
Extended UNIQUAC model
Part II.Carbonate-scaling minerals
Ada Villaf′a?la Garc′?a,Kaj Thomsen?,Erling H.Stenby IVC-SEP,Department of Chemical Engineering,Technical University of Denmark,Building229,
DK-2800Lyngby,Denmark
Received7March2005;accepted31March2006
Available online5June2006
Abstract
Two parameters have been added to the Extended UNIQUAC model of Thomsen and Rasmussen[Thom-sen,K.,Rasmussen,P.,1999.Modeling of vapor–liquid–solid equilibrium in gas-aqueous electrolyte systems.Chem.Eng.Sci.54,1787–1802]to account for the pressure dependency of mineral solubility.The improved model has been used for correlating and predicting vapor–liquid–solid equilibrium for different carbonate systems(CaCO3,MgCO3,BaCO3and SrCO3)causing mineral scaling problems.The solubility of NaCl and CO2in pure water and the solubility of CO2in NaCl and Na2SO4solutions have also been correlated.The results show that the Extended UNIQUAC model,with the added pressure parameters,is able to represent binary(NaCl–H2O,CaCO3–H2O,BaCO3–H2O,SrCO3–H2O,MgCO3–H2O,Mg(OH)2–H2O and CO2–H2O),ternary(CaCO3–CO2–H2O,BaCO3–CO2–H2O,SrCO3–CO2–H2O,MgCO3–CO2–H2O, CO2–NaCl–H2O and CO2–Na2SO4–H2O),and quaternary(CO2–NaCl–Na2SO4–H2O)solubility data within the experimental accuracy in the range of temperatures and pressures considered in the study,i.e.from0to 250?C,and from1to1000bar,respectively.
The modi?ed Extended UNIQUAC model will be a useful tool for predicting and quantifying the scaling problems that may occur in wells and surface equipment during geothermal operations.This would allow adequate preventive measures to be taken before mineral deposition becomes troublesome.
?2006CNR.Published by Elsevier Ltd.All rights reserved.
Keywords:Vapor–liquid–solid equilibria;Scale formation;Extended UNIQUAC;Calcite;CO2;Magnesite;SrCO3; BaCO3
?Corresponding author.Tel.:+4545252860;fax:+4545882258.
E-mail address:kth@kt.dtu.dk(K.Thomsen).
0375-6505/$30.00?2006CNR.Published by Elsevier Ltd.All rights reserved.
doi:10.1016/j.geothermics.2006.03.001
240 A.V.Garc′?a et al./Geothermics35(2006)239–284
Nomenclature
a activity
AAD average absolute deviation[mol(kg H2O)?1]
ASD average standard deviation[mol(kg H2O)?1]
c p heat capacity(J mol?1K?1)
C p1heat capacity coef?cient(coef?cients de?ned in Villaf′a?la-Garc′?a et al.,2005)
(J mol?1K?1)
C p2heat capacity coef?cient(coef?cients de?ned in Villaf′a?la-Garc′?a et al.,2005)
(J mol?1K?2)
C p3heat capacity coef?cient(coef?cients de?ned in Villaf′a?la-Garc′?a et al.,2005)
(J mol?1)
G Gibbs energy(kJ mol?1)
h Enthalpy(kJ mol?1)
H Henry’s constant(MPa mol?1kg?1)
K solubility product
MAAD mean average absolute deviation[mol(kgH2O)?1]
P pressure[Pa,atm(SI Metric Conversion Factor;1bar=105Pa=0.98692atm)]
T temperature(K,?C)
V partial molal volume(cm3mol?1)
Greek letters
αpressure parameter
βpressure parameter
?0change in standard-state property
Superscripts
0pure component standard state
*solute standard state(mole fraction)
sat saturation
Subscripts
f formation
r reaction
w water
1.Introduction
This paper is the second part of a series(Villaf′a?la-Garc′?a et al.,2005)dealing with the calculation of solid–liquid–vapor equilibrium and crystallization processes for electrolyte solu-tions in relation to mineral scale formation,which in some cases tends to be a major problem in geothermal energy production(Juranek et al.,1987;Arnorsson,1989;Gill,1998;Potatov et al.,2001).It is also a common problem in oil?eld operations,causing a considerable reduction in?uid production and injection rates(Shams El Din and Mohammed,1989;Yuan et al.,1994, 1997;Sorbie and Mackay,2000;Dyer and Graham,2002).The economic losses as a consequence of reductions in production rate and re-injection capacity are very important;BP loses around
A.V.Garc′?a et al./Geothermics35(2006)239–284241 4million bbls per year in North Sea oil production(Graham and Mackay,2003).Apart from this aspect,large investments are required to repair damaged equipment and to inhibit mineral scaling.Safety problems may also arise,as a consequence of plugging of?ow lines and/or valve failures.
The formation of carbonate scale is mainly associated with changes in?uid pressure and pH,while sulfate scale occurs mainly as a result of mixing incompatible brines.The effect of temperature on carbonate and sulfate scaling depends on the system analyzed,and varies from one system to another.For example,calcite solubility decreases with increasing temperature,while BaSO4solubility increases with temperature up to around125?C,and behaves in the opposite way for higher temperatures.Carbon dioxide is generally present in signi?cant amounts in geothermal and oil?eld?uids at reservoir temperature and pressure.The decrease of pressure along?ow paths greatly reduces the solubility of carbonate minerals,leading in some cases to mineral deposition (CaCO3,MgCO3,BaCO3,SrCO3)on the inner walls of pipes and other equipment.Of all the carbonate minerals,calcite(CaCO3)seems the most likely to precipitate when pressure decreases and CO2is released(Wat et al.,1992).
The?rst practical step towards scale prevention is its prediction.In that context,we need a reliable thermodynamic model able to calculate mineral solubility in natural waters,under con-ditions of varying brine composition,temperature and pressure.The experimental data available on the systems of interest in the context of the present study are of poor quality and in limited amounts,especially at high temperatures and pressures.The use of a simple but accurate model thus offers clear advantages.Here,the activity coef?cients of the species present in the aqueous phase will be determined by the Extended UNIQUAC thermodynamic model of Thomsen and Rasmussen(1999).The fugacities of the species in the vapor phase will be determined by the Soave–Redlich–Kwong(SRK)cubic equation of state(Soave,1972),using classical mixing rules.
The Extended UNIQUAC model only requires two parameters per species plus two binary parameters per species pair,and accurately represents multicomponent systems with common ions.In order to account for pressure dependency,two pressure parameters have been added to the model.The results obtained through this modi?cation are very satisfactory over the pressure range investigated(1–1000bar).
The?rst part of this series(Villaf′a?la-Garc′?a et al.,2005)was focused on sulfate-scaling minerals,whereas this study is directed at the carbonate-scaling minerals.The most common carbonate scales found in the oil and geothermal industry are the salts of calcium,barium and strontium(Li et al.,1995).Dyer and Graham(2002)also added magnesium carbonates to this list;all these minerals will be studied here.The solubility of NaCl at high tempera-ture and pressure will also be analyzed.Both Na+and Cl?are the main ions in seawater and thus of interest in the present work.Although halite is highly soluble in water,it could precip-itate when the salinity of the formation brine is very high,because of lower NaCl solubility at decreasing temperature or as a consequence of boiling as pressure drops(Dyer and Graham, 2002).
2.Thermodynamics of solubility
The thermodynamic basis used in this paper for the calculation of equilibria was discussed in detail in Villaf′a?la-Garc′?a et al.(2005)and consists of the classical thermodynamic equa-tions.The temperature dependency and the modi?cations made to the Extended UNIQUAC thermodynamic model to account for the pressure dependency of solubility can also be found there.
242 A.V.Garc′?a et al./Geothermics35(2006)239–284
The fugacity coef?cients for both H2O(g)and CO2(g)are calculated by the SRK cubic equation-
of-state using classical mixing rules.The Henry’s constant for carbon dioxide(H CO
2)is calculated
according to Rumpf and Maurer(1993):
ln H CO
2=192.876?9624.4
T
+1.441×10?2T?28.749ln T(1)
where T is the temperature in Kelvin and H CO
2is given in MPa mol?1kg?1.The empirical
correlation(Eq.(1))was based on selected literature data for the solubility of carbon dioxide in pure water.The temperature range of applicability of Eq.(1)is0–200?C.Nevertheless,we applied it up to250?C,obtaining very satisfactory results.The standard-state partial molar volume used for calculating the Poynting correction follows a temperature dependency as given by Rumpf and Maurer(1993):
ˉV?
CO2
=77.83350021?327.8959231×10?3T+594.8558514×10?6T2(2)
In Eq.(2),T is the temperature in Kelvin andˉV?CO
2
is given in cm3mol?1.
3.Results
The procedure followed for estimating parameters and analysing the available experimental data is presented in Villaf′a?la-Garc′?a et al.(2005).Not all data found in the published scienti?c literature were used in our parameter estimations,mainly because experimental conditions and/or methods were not mentioned or were ambiguous.Only the information and sources used are given in this paper.The complete list of data considered is given by Villaf′a?la-Garc′?a(2006).All data were checked before being incorporated in our study.The?rst check was performed within each source of data,analyzing the trend of the solubility as the temperature,pressure and/or salt concentration increase.For the salts analyzed in the present study at high-pressure values, solubility increases with pressure.Regarding temperature and salt concentration,the behavior varies from one system to another(e.g.CaCO3solubility decreases with rising temperature, whereas NaCl solubility increases).The trend followed by each system studied is well known and should be easy to recognize in the experimental data.The experimental procedure and device used to perform the measurements were also studied in order to identify methodological errors.Finally, data from different sources at the same conditions were compared and outliers were rejected.It should be noted that,for certain systems,there were few data available at high temperature and pressure,making such a comparison dif?cult or even impossible.The problem is exacerbated for experiments performed with solutions containing a second salt,instead of being pure water.In this case,the number of points that can be compared is reduced drastically.
The data sources,as well as the ranges of temperature and pressure,the number of experimental points and the average absolute deviation with respect to the Extended UNIQUAC calculations, are given in the following section.The average standard deviation(ASD),the average absolute deviation(AAD)and the mean average absolute deviation(MAAD)are calculated as described in Villaf′a?la-Garc′?a et al.(2005).
Table1shows the values for the ASD calculated from the experimental data reported for the different systems studied here.The surface area and volume parameters for the extended model obtained from the present work,together with the values required for other species obtained by Thomsen and Rasmussen(1999),are given in Table2.The binary energy interaction parameters for our model are shown in Tables3and4.Finally,the two new pressure parameters added to the
A.V.Garc′?a et al./Geothermics35(2006)239–284243 Table1
Calculated average standard deviation(ASD)values for different systems
System ASD(m)
NaCl–H2O7.60×10?2
CO2–H2O 4.10×10?2
CO2–NaCl–H2O 2.20×10?2
CO2–Na2SO4–H2O 2.70×10?2
CaCO3–CO2–H2O 1.30×10?2
BaCO3–CO2–H2O 2.32×10?2
SrCO3–CO2–H2O
MgCO3–CO2–H2O 1.80×10?2
m:molal.
extended model to account for pressure dependency(see Eq.22in Villaf′a?la-Garc′?a et al.,2005), are given in Table5.The parameters obtained based on the present work are marked in bold.
After publishing the?rst paper(Villaf′a?la-Garc′?a et al.,2005),we were able to further optimize the model parameters for the sulfate-scaling minerals.These optimized parameters are included in Tables2–5.
The objective minimizing function for all the systems discussed in this paper(except for NaCl–H2O)is also mentioned in Villaf′a?la-Garc′?a et al.(2005).For the system NaCl–H2O,a residual deviation is preferred as objective function,as sodium chloride is very soluble in water.
It will be shown that the Extended UNIQUAC model is able to give a very good representation of the solid–liquid–vapor phase equilibria in the binary,ternary and quaternary systems over the entire investigated temperature,pressure and concentration range.In most cases,the absolute deviation between Extended UNIQUAC calculations and the experimental data is within experimental accuracy.
The values for the standard-state Gibbs free energy,enthalpy and heat capacity for the different crystalline phases analyzed are taken(when available)from the NIST Chemical Thermodynamics Database(1990).These values are also reported in Table6.
Table2
Extended UNIQUAC model volume(r)and surface area(q)parameters(Villaf′a?la-Garc′?a et al.,2005),?tted to experi-mental data
r q
H2O0.92 1.40
CO2(aq)0.752.45
Na+ 1.40 1.20
H+0.140.1×10?15
Ba2+15.6714.48
Ca2+ 3.87 1.48
Sr2+7.1412.89
Mg2+ 5.41 2.54
OH?9.408.88
Cl?10.3910.20
SO42?12.7912.44
CO32?10.8310.77
HCO3?8.088.68
The parameters we obtained are shown in bold.
244
A.V .Garc′?a et al./Geothermics 35(2006)239–284
Table 3
u 0ij =u 0ji parameters for calculating Extended UNIQUAC interaction energy parameters (Villaf′a ?la-Garc′?a et al.,2005)[u ij =u 0ij +u T ij (T ?298.15)]
H 2O
CO 2(aq)H +
Na +
Ca 2+
Ba 2+
Sr 2+
Mg 2+
OH ?
Cl ?
SO 42?
CO 32?
HCO 3?
H 2O
CO 2(aq)8.83825302.25H +1×10410100
Na +733.2863172.3910100Ca 2+496.352325001010?1000Ba 2+?0.378625*********.125000Sr 2+543.11?100.71010?103.9?402.7825000Mg 2+?2.04282?581.21010?70.956155.2324628.529?400.580OH ?600.4952250010101398.1164.637825002500736.421562.88Cl ?1523.393161310101443.21805.591403.171895.8820491895.522214.81SO 42?752.87921942.41010845.141258.103250025001407.211225.672036.061265.83CO 32?361.387725001010547.952500250025001001588.032724.941216.761458.344HCO 3?
577.0502
526.31
1010
1101.9
2500
2500
2500
100
2500
1736.62
990.48
800.0081
771.038
The parameters we obtained are shown in bold.
A.V .Garc′?a et al./Geothermics 35(2006)239–284
245
Table 4
u T ij =u T ji parameters for calculating Extended UNIQUAC interaction energy parameters (Villaf′a ?la-Garc′?a et al.,2005)[u ij =u 0ij +u T ij (T ?298.15)]
H 2O
CO 2(aq)H +
Na +
Ca 2+
Ba 2+
Sr 2+
Mg 2+
OH ?
Cl ?
SO 42?
CO 32?
HCO 3?
H 2O
CO 2(aq)0.862930.3587H +0
00
Na +0.48719?0.43600Ca 2+?8.065400?4.6560Ba 2+0.58244002.3380
0Sr 2+ 1.274200?0.62?4.253300
Mg 2+?3.5542?2.8550 1.3394 5.19210?1.4370OH ?8.54550020.278 3.6084000 5.6169
Cl ?14.63115.015015.63511.1414.8915.68912.13213.62814.436SO 42?9.49054.7896011.68150.446010 2.27918.590212.4078.3194CO 32? 3.351600 3.7820001 2.7496 5.72677.0067?1.3448HCO 3?
?0.38795
?3.734
1.829
01
14.035
6.9646
1.7241
?0.0198
The parameters we obtained are shown in bold.
246 A.V.Garc′?a et al./Geothermics35(2006)239–284
Table5
Pressure dependency parametersαandβfor the Extended UNIQUAC model to calculate solubility products(see text)
αβ
BaSO4 1.636629×10?3 3.333826×10?7
SrSO4 1.035686×10?37.153236×10?7
CaSO4 2.328718×10?3?8.421355×10?7
CaSO4·2H2O 1.078224×10?3 3.078141×10?7
NaCl9.927134×10?5?1.358719×10?8
CaCO3?3.916945×10?3?2.092925×10?5
3.1.NaCl–H2O system
Some results for the system NaCl–H2O at high pressures were presented in Villaf′a?la-Garc′?a et al.(2005).A more detailed study of sodium chloride solubility at high pressure,including additional experimental data,follows.The volume and surface area parameters for Na+and Cl?, together with all the binary interaction parameters required for the binary system NaCl–H2O,are taken from Thomsen and Rasmussen(1999).The pressure parameters are estimated on the basis of solid–liquid equilibrium data at high pressures.
The few studies found on the solubility of sodium chloride in pure water at pressures higher than atmospheric are reported in Table7.The experimental conditions and the number of measurements performed,whose results were used in the present work,are also given.
Cohen et al.(1911)withdrew?uid samples from their pressure bomb for analysis after reducing the experimental pressure,a procedure that can lead to solid deposition and can cause erroneous measurements.Sill(1916)was aware of this problem and developed a new pressure bomb to avoid it.The agreement between both sources is very good,which seems to prove that the results from Cohen et al.(1911)were not affected by solid deposition prior to analysis.
Schroeder et al.(1935)reported both experimental temperature and NaCl solubility,but they did not mention the experimental pressure(which was the saturation pressure of the solution).In
Table6
Thermodynamic properties and related parameters
r G?
f (kJ mol?1) r H?
f
(kJ mol?1)
C p1(J mol?1K?1)C p2(J mol?1K?2)C p3(J mol?1)
CO2(aq)?385.98?413.824300 CaCO3?1129.057?1208.72681.8800
Mg(OH)2?833.51?921.140777.0300
Na2SO4?1270.16?1387.08128.200 NaCl?384.1258?411.958445.1307400 MgCO3?1012.1?1095.875.5200 MgCO3·3H2O?1723.487?1953.29301.496800 MgCO3·5H2O?2197.745?2524.95367.36700
3MgCO3·Mg(OH)2·3H2O?4602.818?5069.43478.698600 BaCO3?1136.054?1222.90785.500 SrCO3?1139.745?1227.6331259.146863?2.827237?21149.44 CaCO3·MgCO3?2140.89?2302.72157.400
Bold numbers are taken from the NIST Chemical Thermodynamics Database(1990).The rest of the values were?tted to experimental data.The heat capacity coef?cients C p1,C p2,and C p3were de?ned in Villaf′a?la-Garc′?a et al.(2005).
A.V.Garc′?a et al./Geothermics35(2006)239–284247 Table7
Experimental NaCl–H2O solid–liquid equilibrium datasets used for parameter estimation
T(?C)P(bar)Number of data AAD(m)Reference
0/301/4014(14) 2.24×10?2Moeller(1862)
241/150010(8) 6.63×10?3Cohen et al.(1911)
251/735.57(7) 1.33×10?2Sill(1916)
301/191114(6)7.24×10?3Adams and Hall(1931) 75/3001/57.59(9) 5.06×10?1Liu and Lindsay(1972) 101.9/168.31/5.32(2) 1.11×10?1Eddy and Menzies(1940) 150/350 3.5/137.78(7) 4.36×10?1Schroeder et al.(1935) MAAD(m) 1.57×10?1
The number of data used for parameter estimation is given in parentheses.AAD:average absolute deviation;m:molal; MAAD:mean average absolute deviation.
order to use these data,the total pressure was calculated as
p~=a w P sat w(3)
where P sat w is the saturation pressure for pure water at a given temperature T.The water activity, a w,was obtained using the NaCl model of Archer(1992).The saturation pressure of water was calculated based on the NIST/ASME steam properties formulation for general and scienti?c use (Harvey et al.,1996).
The modeling results obtained for the system NaCl–H2O at high pressure can be divided into two different groups,according to their accuracy:up to150?C,and from150to300?C.For the?rst group,the results are very satisfactory,with a MAAD between that of the Extended UNIQUAC calculations and that of the experimental data(1.5×10?3m;“m”stands for molal) that is,lower than the ASD(7.6×10?3m).We can therefore conclude that the calculations for the temperature range0–150?C are within experimental accuracy.Fig.1,which represents the solubility diagram for the binary NaCl–H2O system at30?C,shows this good accuracy.Although
Fig.1.Experimental and calculated solid–liquid phase diagram for the NaCl–H2O system at30?C.
248 A.V.Garc′?a et al./Geothermics35(2006)239–284
only experimental data up to1000bar were used for parameter estimation(higher pressures are unusual in geothermal and oil wells),Fig.1presents data up to2000bar.The?gure shows that the prediction made by the Extended UNIQUAC model for such large pressures is very good.It can therefore be concluded that,although the parameters are estimated from data up to1000bar,the range of applicability of the extended model is not limited to that pressure.The predictions based on the extended model over the1000to around2000bar pressure range are within experimental accuracy.The MAAD between our model calculations and the experimental data[eight points reported by Adams and Hall(1931)and two points given by Cohen et al.(1911)]is2.2×10?3m NaCl,well below the ASD of7.6×10?3m.
Fig.1also shows that the in?uence of pressure on sodium chloride solubility is not strong,as it only increases from6.17m NaCl at1bar to6.45m NaCl at2000bar.About16g of halite will therefore precipitate from each kilogram of water saturated with NaCl at2000bar if the pressure is reduced to one atmosphere.
The good agreement between the model and the experimental data observed for the?rst dataset is not found for the second dataset,which covers temperatures between150and300?C.The MAAD between the Extended UNIQUAC calculations and the experimental data at high temper-atures is5.3×10?1m,which is larger than the ASD.There are only a few data sources covering that range of temperatures.Schroeder et al.(1935)and Liu and Lindsay(1972)report quite close solubility values at similar T and P conditions.Liu and Lindsay did not measure the solubility directly.Instead,they used an indirect method to obtain,from vapor pressure data,values for both the osmotic coef?cient and the concentration of saturated solutions.Liu and Lindsay(1972)claim that their method gives considerable precision.The lack of additional information on the system at high temperatures makes it dif?cult to determine the reliability of both datasets.
The AAD for each of the sources used for the NaCl–H2O system is shown in Table7.The ASD for the experimental data at pressures higher than1atm is7.6×10?2m NaCl,while that for data obtained at atmospheric pressure is5.3×10?2m.According to the International Critical Tables (1928),the solubility of sodium chloride in water is estimated experimentally with an accuracy of 0.2%(3.4×10?2m)below55?C and1atm,and of0.5%(8.6×10?2m)in the60–110?C range. Above these temperatures the accuracy is unknown(Eddy and Menzies,1940).It is likely that the standard deviation at pressures higher than1atm is larger than these values,as the experiments are more dif?cult to perform.For all the sources in Table7the AAD is lower than the ASD,except for the results of Schroeder et al.(1935),Eddy and Menzies(1940)and Liu and Lindsay(1972), which happen to be the sources reporting experimental data at the highest temperatures.
The results obtained by the Extended UNIQUAC model at atmospheric pressure and tem-peratures up to110?C,and the sources at these conditions,are not mentioned here,but can be found in our data bank at IVC-SEP;this database is available on-line(http://www.ivc-sep.kt.dtu.dk/databank/).All the calculations we performed at atmospheric pressure are within experimental accuracy.The MAAD between Extended UNIQUAC calculations and the experi-mental data from all data sources corresponding to atmospheric pressure is4.6×10?2m NaCl, while the ASD for these experimental data is higher(5.3×10?2m).
3.2.CO2solubility in pure water
Most papers dealing with calcite solubility in CO2–saturated water do not report the CO2 concentration in the liquid,but only the CaCO3solubility and the partial pressure of CO2.In order to be able to use these data,we need to know the amount of CO2present in the solution at the experimental conditions.We therefore developed a program for calculating CO2solubility
A.V.Garc′?a et al./Geothermics35(2006)239–284249 Table8
Calculated vs.experimental CO2solubility in CaCO3–H2O solutions
T(?C)P(bar)CO2solubility in pure water(m)RD(%)
Malinin(1963)Our model
15010.90.0530.056 5.66 15020.20.1330.138 3.76 15021.70.1460.151 3.42 15035.10.2620.2630.38 15054.20.4090.4110.56 15054.30.4100.4140.98 15057.50.4430.437?1.35 15059.20.4570.450?1.53 150145.10.9550.973 1.88 150200.1 1.192 1.228 3.02 22557.90.3180.337 5.97 22580.40.5230.553 5.74 225131.40.955 1.002 4.92 225135.30.978 1.034 5.73 225199.1 1.458 1.518 4.12 225200.1 1.468 1.525 3.88 225398.2 2.660 2.767 4.02 225402.1 2.680 2.788 4.03 RD:relative deviation;m:molal.
in water as a function of temperature and pressure.The Extended UNIQUAC model was used to compute activity coef?cients in the liquid phase,while gas phase fugacities were calculated according to the SRK cubic equation-of-state,using classical mixing rules.
It was assumed that the CO2solubility in CaCO3solutions is very close to that in pure water at the same conditions,based on the fact that calcite solubility in water is so low that it will probably not in?uence the gas solubility signi?cantly.In Table8,CO2solubility values in CaCO3–H2O solutions reported by Malinin(1963)are compared with those obtained using the extended model. The highest relative deviation is found to be5.66%and the average relative deviation,3.07%. These low values indicate that the in?uence of calcite on CO2solubility in the liquid phase can essentially be neglected.
Table9shows all the publications used for the system CO2–H2O,the ranges of temperature and pressure covered in them,the number of data points reported in these papers,the number of data points used in the present work,and the AAD between our calculations and the experimental data.The calculated CO2solubilities in pure water compare well with the experimental data,with a MAAD of3.7×10?2m CO2,while the ASD for the experimental data is4.1×10?2m CO2.
The calculated and experimental CO2solubility in pure water at150?C is shown in Fig.2;there is excellent agreement between the results of the Extended UNIQUAC model and the experimental data,even at very large pressures.Only data up to1000bar were used for parameter estimation, but Fig.2shows that predictions performed by our model at larger pressures(up to1200bar)are very accurate.
Carbon dioxide solubility increases considerably with pressure,changing from0.028m at 7.7bar to2.8m at1500bar.Fig.3shows the solubility of CO2in water at a constant pressure of100bar.Carbon dioxide solubility in water decreases very steeply with temperature in the 25–100?C range,whereas the temperature effects become almost negligible between100and
250 A.V.Garc′?a et al./Geothermics35(2006)239–284
Table9
Experimental CO2–H2O vapor–liquid equilibrium datasets used for parameter estimation
T(?C)P(bar)Number of data AAD(m)Reference
0/250.1/119(19) 5.25×10?4Morgan and Maass(1931)
0/2513(3) 1.72×10?3Kiss et al.(1937)
0/50 1.00E+0018(18) 6.00×10?4Harned and Davis(1943)
0/601/965(65)8.68×10?3Wasmund and Bultmann(1980)
0/1001/9695(95) 2.84×10?2Zelvenskii(1937)
0.2/40 1.00E+003(3)7.24×10?4Markham and Kobe(1941a)
1/59/219(9)9.61×10?3Malegaonkar et al.(1997)
1/151/2253(53)8.19×10?3Anderson(2002)
1/35 1.00E+008(8) 1.15×10?3Murray and Riley(1971)
100/2003/8149(49) 3.65×10?2M¨u ller et al.(1988)
5/650.5/0.810(10) 2.96×10?4Zheng et al.(1997)
10/301/2015(15) 1.63×10?2Bartholome and Friz(1956)
11.5/400.1/0.924(24) 1.20×10?3Novak et al.(1961)
12/4050/50642(42) 1.01×10?1Wiebe and Gaddy(1940)
13.3/74.7119(19) 1.87×10?3Morrison and Billett(1952)
15/25 1.00E+002(2) 1.22×10?3Geffcken(1904)
15/5515(5) 1.37×10?3Postigo et al.(1978)
15.6/93.3 6.9/202.716(16) 2.77×10?2Gillespie and Wilson(1982)cited in
Diamond and Akin?ev(2003)
20/3418(8) 6.88×10?4Kunerth(1992)
21.2/21.814(4) 2.07×10?3Curry and Hazelton(1938)
22.8/25.516(6) 3.93×10?4Van Slyke(1939)
2511(1) 3.79×10?4Markham and Kobe(1941b) 2521.7/53.37(7) 3.98×10?2Yang et al.(2000)
25/45 1.00E+004(4) 1.01×10?3Yeh and Peterson(1964)
25/754820(20)8.94×10?3Malinin and Savel’eva(1972)
25/1504810(10) 1.53×10?2Malinin and Kurovskaya(1975)
30/809.3/39.313(13)8.79×10?3Matous et al.(1969)
33/2138/5.87(6) 1.98×10?2Cramer(1982)
5.00E+0110.6/587(7) 2.23×10?3Rumpf et al.(1994)
50/8040/14129(29) 1.09×10?2Bamberger et al.(2000)
50/10025/70929(29) 6.65×10?2Wiebe and Gaddy(1939)
50/200 1.5/5433(33)9.17×10?3Zawisza and Malesinska(1981)
50/350200/3500108(14) 1.41×10?1T¨o dheide and Franck(1963)
80/19820.4/10233(33) 2.16×10?2Nighswander et al.(1989)
101/12023.3/70326(26)9.91×10?2Prutton and Savage(1945)
110/350100/1500121(39) 2.19×10?1Takenouchi and Kennedy(1964) 114/3485/16236(23) 5.97×10?3Ellis(1959b)
177/33425/19915(6) 3.70×10?2Ellis and Golding(1963)
MAAD(m) 3.65×10?2
The number of data used for parameter estimation is given in parentheses.AAD:average absolute deviation;m:molal; MAAD:mean average absolute deviation.
200?C.For higher temperatures,the carbon dioxide solubility decreases again with increasing temperature.The shape of the experimental curve is very well reproduced by the Extended UNI-QUAC model.Table9shows the value of the MAAD between the model calculations and the experimental data used in this work.This value,3.6×10?2m CO2,is lower than the experi-mental ASD(4.1×10?2m CO2);it can therefore be concluded that the model calculations and predictions for the CO2–H2O system are within experimental accuracy.
A.V.Garc′?a et al./Geothermics35(2006)239–284251
Fig.2.Experimental and calculated vapor-liquid phase diagram for the CO2–H2O system at150?C.
3.3.CO2solubility in aqueous solutions of NaCl and Na2SO4
In order to apply our model to the calculation of vapor–liquid–solid equilibria in natural waters, the main constituents of these waters have to be accounted for.We therefore had to study and model the carbon dioxide solubility in different electrolyte solutions,such as those containing NaCl and Na2SO4.The parameters for the interactions between CO2,HCO3?,CO32?and Na+, Cl?and SO42?were determined by Thomsen and Rasmussen(1999)on the basis of vapor–liquid equilibrium(VLE)and solid–liquid equilibrium(SLE)data.Their experiments were performed at temperatures and pressures ranging from0to110?C and from1to100bar,respectively. These conditions are too restricted for the purposes of the present work.Extrapolation to higher
Fig.3.Experimental and calculated vapor–liquid phase diagram for the CO2–H2O system at100bar.
252 A.V.Garc′?a et al./Geothermics35(2006)239–284
Table10
Experimental CO2–NaCl–H2O vapor–liquid equilibrium datasets used for parameter estimation
T(?C)P(atm)Number of data AAD(m)Reference
0/50190(90)9.72×10?4Harned and Davis(1943)
0.2/40115(15) 1.32×10?3Markham and Kobe(1941a)
15/35127(27) 1.28×10?3Yasunishi and Yoshida(1979) 20/35116(16) 1.73×10?3Vazquez et al.(1994)
2518(8) 1.51×10?3Onda et al.(1970)
25/15048.927(27) 2.54×10?2Malinin and Kurovskaya(1975) 25/754813(13) 4.34×10?2Malinin and Savel’eva(1972) 40/160.8 4.6/96.463(63) 1.51×10?2Rumpf et al.(1994)
2517(7) 1.92×10?3Yasunishi et al.(1979)
250/450100/140086(30) 1.29×10?1Takenouchi and Kennedy(1965) 100/500200/2500178(32) 1.21×10?1Naumov et al.(1974)
MAAD(m) 3.09×10?2
The number of data used for parameter estimation is given in parentheses.AAD:average absolute deviation;m:molal; MAAD:mean average absolute deviation.
temperatures and pressures did not give accurate results,so parameter estimations were carried out on the basis of VLE data over wider ranges of temperature and pressure.
The VLE behavior of aqueous solutions may be quite different from that of pure water.The thermodynamic properties of the solution may vary signi?cantly,as a consequence of the inter-actions between molecules and ionic species.Depending on the type of salt,gas solubility may increase(salting-in effect)or decrease(salting-out effect).For carbon dioxide solubility in NaCl and Na2SO4solutions,the salting-out effect is large,but the general form of the solubility curves is more or less the same as for the system CO2–H2O(Takenouchi and Kennedy,1965).
3.3.1.CO2solubility in NaCl solutions
Table10shows the sources found in the literature reporting carbon dioxide solubility in sodium chloride solutions at different temperatures and pressures.The number of experimental determi-nations for each source and the number of data used in the present study are also given.
The effect of pressure on CO2solubility in NaCl solutions reported by Naumov et al.(1974) does not agree with the data of Malinin and Kurovskaya(1975).The?rst source gives solubility values at100?C and100bar,very close to those reported by Malinin and Kurovskaya(1975)at 100?C and48bar,covering a NaCl concentration range from0.5to6m.The latter study is in good agreement with Malinin and Savel’eva(1972),whose results concur with those of many other authors listed in Table10.On the other hand,Naumov et al.(1974)agree quite well with Takenouchi and Kennedy(1965),especially at high temperatures(250and300?C).At lower temperatures(150and200?C),the in?uence of NaCl concentration on CO2solubility reported by both sources is somewhat different.The values given by Naumov et al.(1974)at0.5and5m are very close to those of Takenouchi and Kennedy(1965)at1and4.3m,respectively.After analyzing all these datasets it seems clear that a general disagreement exists among the different sources.The regions of overlap of the different studies are rather narrow,and a clear conclusion about the reliability of the various sources cannot be reached.We therefore decided to use them all in the present work.
At30and35?C and1atm total pressure,there is some scatter among the data reported by Harned and Davis(1943),Yasunishi and Yoshida(1979),and Vazquez et al.(1994).The dif-
A.V.Garc′?a et al./Geothermics35(2006)239–284253
Fig.4.Experimental and calculated vapor–liquid phase diagram for the CO2–NaCl–H2O system at160?C. ferences are,however,not very large and can be regarded as reasonable experimental standard deviations.
The experimental and calculated phase diagram for the system CO2–NaCl–H2O at a constant temperature of160?C and NaCl concentrations of4and6m is given in Fig.4.According to the experimental data plotted in this?gure,carbon dioxide solubility increases with increasing pres-sure,and decreases for increasing salt concentration.Fig.4shows that the Extended UNIQUAC model reproduces this trend.
Fig.5presents experimental results in the60–70bar pressure range,together with the model calculations,at both4and6m NaCl.Data at different pressures were used instead of isobaric data because of the lack of the latter.The?gure includes calculations performed by the extended
Fig.5.Experimental and calculated vapor–liquid phase diagram for the CO2–NaCl–H2O system at60–70bar.Lines represent calculations at a constant pressure of60bar;solid dots and triangles represent model results at the experimental pressure(in the60–70bar range),which are compared to the experimental data(open dots and triangles).
254 A.V.Garc′?a et al./Geothermics35(2006)239–284
model at the experimental conditions(black dots and triangles),together with the calculations at 60bar constant pressure(lines).The salting-out effect observed in Fig.4can also be observed here.According to the experimental data plotted in Fig.5,CO2solubility decreases considerably with increasing temperature,passing from0.53m at40?C to0.22m at160?C,for4m NaCl solutions.Fig.5shows that this trend is also accurately replicated by the Extended UNIQUAC model.
The extended model reproduces the solubility of carbon dioxide in NaCl solutions very well, even for very concentrated solutions(close to the saturation of NaCl).The MAAD between the model calculations and the experimental data is3.1×10?2m,while the ASD for the experi-mental results is2.2×10?2m.The latter value is mainly based on data obtained at atmospheric conditions,because of the lack of experimental measurements at the same NaCl concentration, temperature and pressure.If only data at pressures higher than1atm are considered in the calcu-lation of ASD,its value increases to0.15m.
Table10shows the AAD for each of the CO2–NaCl–H2O sources used in the present work. The value of AAD for all the sources at atmospheric pressure is smaller than2.2×10?2m.For some sources dealing with higher pressures(Malinin and Kurovskaya,1975;Rumpf et al.,1994) the value is lower than,or very close to,2.2×10?2m.Only for the investigations performed at high pressures,the AAD between the Extended UNIQUAC calculations and the experimental data is larger than2.2×10?2m(but always lower than0.15m).It can therefore be concluded that the results of the Extended UNIQUAC calculations are within experimental accuracy and that the model represents correctly the salting-out effect,as well as the temperature and pressure dependence of CO2solubility in NaCl solutions(from very dilute to almost saturated solutions).
3.3.2.CO2solubility in Na2SO4solutions
Sodium sulfate also has a relatively large salting-out effect on aqueous CO2solutions,reducing the solubility of the gas.Very few experimental data on this solution system were found in the literature.Furthermore,most of the data reported had been measured at pressures around1atm and at relatively low temperatures.The sources found in the literature reporting CO2solubility in Na2SO4solutions are given in Table11;the range of temperatures and pressures covered and the number of measurements performed and used in this work for parameter estimation are also shown in Table11.
The experimental and calculated phase diagrams for the system CO2–Na2SO4–H2O at a con-stant temperature of140?C,and at pressures in the80–90bar range,are shown in Figs.6and7, respectively.Carbon dioxide in Na2SO4solutions behaves in a very similar way as in NaCl Table11
Experimental CO2–Na2SO4–H2O vapor–liquid equilibrium datasets used for parameter estimation
T(?C)P(atm)Number of data AAD(m)Reference
15/35126(26) 1.30×10?3Yasunishi and Yoshida(1979) 2513(3) 3.74×10?4Onda et al.(1970)
2515(5)7.86×10?4Yasunishi et al.(1979)
25/4018(8)8.76×10?4Markham and Kobe(1941a) 40/160 4.2/93.8102(102)7.05×10?3Rumpf and Maurer(1993) MAAD(m) 5.32×10?3
The number of data used for parameter estimation is given in parentheses.AAD:average absolute deviation;m:molal; MAAD:mean average absolute deviation.
A.V.Garc′?a et al./Geothermics35(2006)239–284255
Fig.6.Experimental and calculated vapor–liquid phase diagram for the CO2–Na2SO4–H2O system at140?C. solutions;a salting-out effect is observed in these two?gures.With regard to temperature,the solubility of CO2decreases quite rapidly with temperature over the0–100?C range,while the slope of the solubility curve is much smaller at higher temperatures.The solubility of CO2in1m Na2SO4solution decreases from0.6m at50?C to0.4m at160?C.
The calculated solubility given by the extended UNIQUAC model compares very well with the experimental data shown in Table11.The value of the MAAD(5.3×10?3m)is well below the ASD(2.7×10?2m).
3.3.3.CO2solubility in NaCl–Na2SO4solutions
Only the data of Yasunishi et al.(1979),who studied the CO2–NaCl–Na2SO4–H2O system, were used for parameter estimation.These authors performed15measurements of CO2solubility in aqueous solutions of NaCl and Na2SO4at25?C and1atm.The modeling results for this quater-
Fig.7.Experimental and calculated vapor–liquid phase diagram for the CO2–Na2SO4–H2O system at80–90bar.Lines represent calculations at a constant pressure of80bar;solid dots and triangles represent model results at the experimental pressure(in the80–90bar range),which are compared to the experimental data(open dots and triangles).
256 A.V.Garc′?a et al./Geothermics35(2006)239–284
Fig.8.Experimental and calculated CO2solubility in NaCl–Na2SO4–H2O solutions of ionic strength(I),at25?C and 1bar.
nary system are also very satisfactory,with a MAAD of9.3×10?4m.Fig.8shows experimental and calculated CO2solubilities in NaCl–Na2SO4solutions as a function of the ionic strength of the solution,at25?C and1atm.
3.4.CaCO3–H2O and CaCO3–CO2–H2O systems
The solubility of calcite is strongly affected by the amount of carbonic acid present in the solution(i.e.CaCO3solubility increases rapidly with CO2concentration).In that sense,calcite solubility studies(and,in general,any carbonate mineral study)can be divided into different groups according to the amount of carbon dioxide used in the experiments.In most cases,pure CO2is bubbled into a calcite–water solution so that the amount of CO2in the liquid phase equals the gas solubility in the solution,at the experimental temperature and pressure.A second line of study deals with calcite solubility in pure water,free of CO2,which is achieved by vigorous boiling of the solution.Finally,some authors perform experiments by adding a constant amount of CO2while varying the temperature and/or pressure.
Table12shows the sources reporting calcite solubility in pure water and in CO2–H2O solutions, together with the range of temperatures and pressures investigated,and the number of experimental points.Some,e.g.Engel(1889),Treadwell and Reuter(1898),Mitchell(1923),Miller(1952), Yanat’eva(1955a),Ellis(1959a),Yanat’eva(1960),Segnit et al.(1962),Ellis(1963)and Malinin (1963),used a gas phase over the solution formed by pure carbon dioxide(plus the water vapor corresponding to the experimental conditions).Others,e.g.Schloesing(1872),Leather and Sen (1910),Kendall(1912),Johnston(1915),Wells(1915a),Frear and Johnston(1929),Weyl(1959), Sippel and Glover(1964),Sharp and Kennedy(1965),Plummer and Busenberg(1982),and Wolf et al.(1989),measured calcite solubility in CO2–H2O solutions,where the amount of dissolved CO2was below its saturation values.Most of these studies used a gas phase over the solution formed by air,or mixtures of carbon dioxide and N2or air(in different proportions).
The data given by Segnit et al.(1962)show very large scatter,especially at100?C,where CaCO3solubility varies from8.5×10?3to11.2×10?3m.They state that their results agree satisfactorily with those of Ellis(1959a),but disagree somewhat with those obtained by Miller
A.V.Garc′?a et al./Geothermics35(2006)239–284257 Table12
Experimental CaCO3–H2O SLE and CaCO3–CO2–H2O solid–liquid–vapor equilibrium(SLVE)data sets used for param-eter estimation
T(?C)P(bar)Number of data AAD(m)Reference
0/5512(2)8.4×10?4Yanat’eva(1955a)
0/1021/10198(98) 1.6×10?3Miller(1952)
0.1/89.71141(133) 3.8×10?4Plummer and Busenberg(1982) 10/6018(4)8.3×10?5Wolf et al.(1989)
10/70130(30) 1.1×10?4Weyl(1959)
15/40149(49) 4.9×10?4Leather and Sen(1910)
1617(7) 5.3×10?4Johnston(1915)
16112(12) 4.4×10?11Schloesing(1872)
161/64(4) 2.2×10?4Engel(1889)
2519(9)9.3×10?4Frear and Johnston(1929)
25/10016(2) 3.7×10?3Kendall(1912)
30/80 3.4/17951(51) 1.6×10?3Sippel and Glover(1964) 7011(1) 4.0×10?4Yanat’eva(1960)
75/200 5.5/68.2260(254) 4.9×10?4Segnit et al.(1962)
98/302 1.9/142.359(59) 6.1×10?4Ellis(1959a)
100/3002/15034(34) 4.9×10?4Ellis(1963)
150/22510.9/40218(18) 3.3×10?4Malinin(1963)
MAAD(m)7.4×10?4
The number of data used for parameter estimation is given in brackets.AAD:Average absolute deviation;m:molal; MAAD:mean average absolute deviation.
(1952)at75and100?C,at pressures above10atm.Nevertheless,the disagreement among all these sources was not extreme and their results were used to estimate the required parameters [with the exception of four points given by Segnit et al.(1962)that were in greatest disagreement with the main trend].
The experimental and calculated phase diagrams for the CaCO3–CO2–H2O system at a constant temperature of100?C and at a constant pressure of40bar are shown in Figs.9and10,respectively.
Fig.9.Experimental and calculated solid–liquid phase diagram for the CaCO3–CO2–H2O system at100?C.Gas phase formed by CO2and H2O.
258 A.V.Garc′?a et al./Geothermics35(2006)239–284
Fig.10.Experimental and calculated solid-liquid phase diagram for the CaCO3–CO2–H2O system at40bar.Gas phase formed by CO2and H2O.
The gas phase over the solution used for all the measurements reported in these?gures was pure CO2saturated with water vapor.Therefore,the increase of calcite solubility with pressure also reveals the higher solubility of that mineral at higher CO2concentrations.A very sharp decrease of calcite solubility is observed for increasing temperature.
A large scatter in the experimental results can be observed in Fig.9.The experimental standard deviation was calculated using data from different sources.When an author gives more than one point for the same conditions,the average of these values is compared against the other sources reporting data at the same temperature and pressure.The scatter shown in Fig.9for the measure-ments of Segnit et al.(1962)is therefore not included in the calculation of the standard deviation.
Although in most cases the CO2content in the system was calculated,the results obtained for the calcite solubility are very close to experimental data.The MAAD between the Extended UNIQUAC model results and the experimental measurements(i.e.7.4×10?4m)is lower than the experimental standard deviation(i.e.1.3×10?3m).This con?rmed that the extended model is also applicable to the system CaCO3–CO2–H2O.
Ellis(1959a)and Malinin(1963)reported CO2concentrations in the liquid phase,as well as calcite solubilities.In both cases,the experimental values were used in our parameter estimation study instead of the model calculations.We should stress that the differences between the reported values and those calculated using the extended model were very small.
3.5.BaCO3–H2O and BaCO3–CO2–H2O systems
Very few studies on the solubility of witherite(BaCO3)in pure water and in water under various CO2partial pressures have been found in literature.Of these,only two report solubility values at temperatures above25?C or pressures higher than atmospheric.The list of experimental data used for parameter estimation is presented in Table13,which shows the total number of data found in each source,the number of values we chose for parameter estimation,and the range of temperatures and pressures considered.