On capacity of wireless ad hoc networks with MIMO MMSE receivers
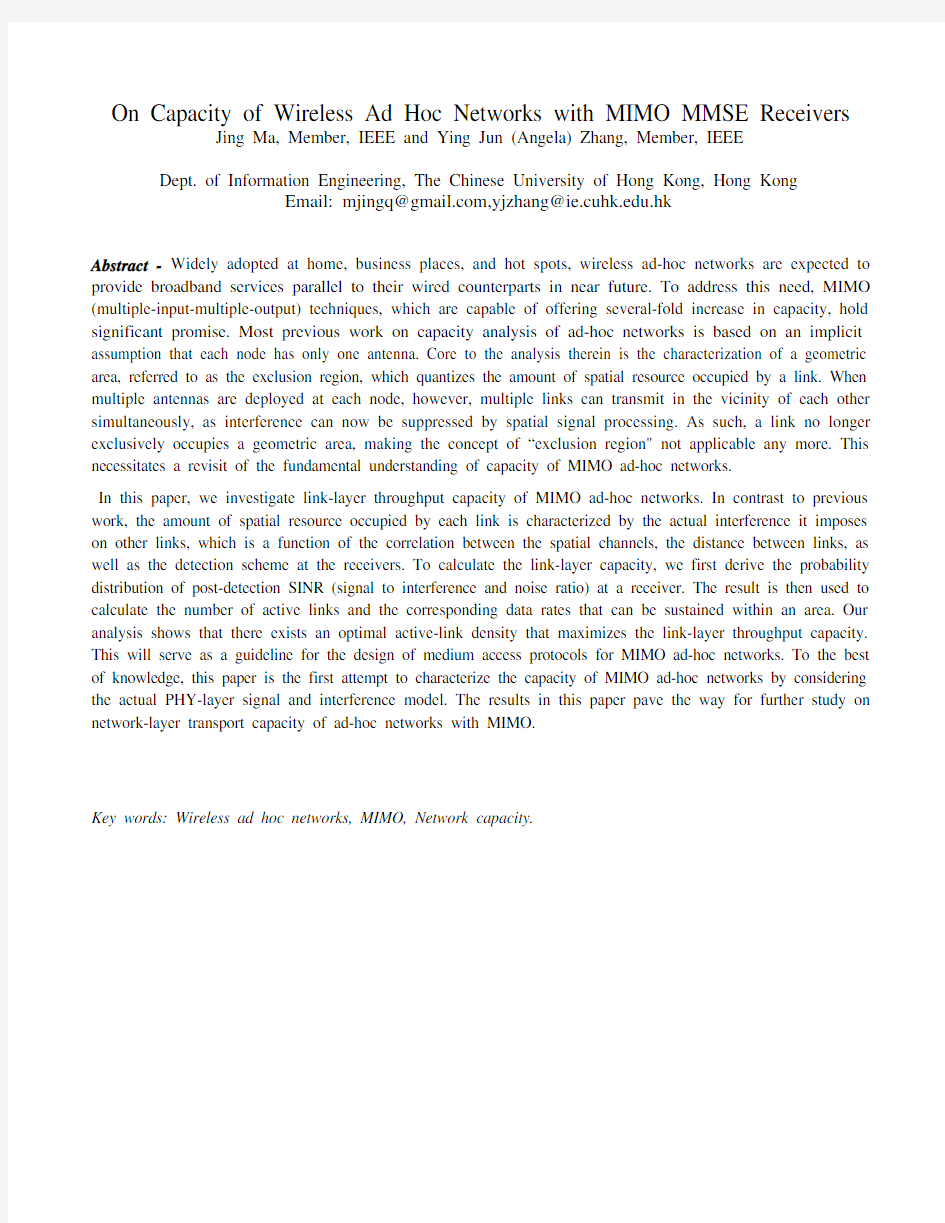

On Capacity of Wireless Ad Hoc Networks with MIMO MMSE Receivers Jing Ma, Member, IEEE and Ying Jun (Angela) Zhang, Member, IEEE
Dept. of Information Engineering, The Chinese University of Hong Kong, Hong Kong
Email: mjingq@https://www.360docs.net/doc/774182458.html,,yjzhang@https://www.360docs.net/doc/774182458.html,.hk
Abstract - Widely adopted at home, business places, and hot spots, wireless ad-hoc networks are expected to provide broadband services parallel to their wired counterparts in near future. To address this need, MIMO (multiple-input-multiple-output) techniques, which are capable of offering several-fold increase in capacity, hold significant promise. Most previous work on capacity analysis of ad-hoc networks is based on an implicit assumption that each node has only one antenna. Core to the analysis therein is the characterization of a geometric area, referred to as the exclusion region, which quantizes the amount of spatial resource occupied by a link. When multiple antennas are deployed at each node, however, multiple links can transmit in the vicinity of each other simultaneously, as interference can now be suppressed by spatial signal processing. As such, a link no longer exclusively occupies a geometric area, making the concept of “exclusion region" not applicable any more. This necessitates a revisit of the fundamental understanding of capacity of MIMO ad-hoc networks.
In this paper, we investigate link-layer throughput capacity of MIMO ad-hoc networks. In contrast to previous work, the amount of spatial resource occupied by each link is characterized by the actual interference it imposes on other links, which is a function of the correlation between the spatial channels, the distance between links, as well as the detection scheme at the receivers. To calculate the link-layer capacity, we first derive the probability distribution of post-detection SINR (signal to interference and noise ratio) at a receiver. The result is then used to calculate the number of active links and the corresponding data rates that can be sustained within an area. Our analysis shows that there exists an optimal active-link density that maximizes the link-layer throughput capacity. This will serve as a guideline for the design of medium access protocols for MIMO ad-hoc networks. To the best of knowledge, this paper is the first attempt to characterize the capacity of MIMO ad-hoc networks by considering the actual PHY-layer signal and interference model. The results in this paper pave the way for further study on network-layer transport capacity of ad-hoc networks with MIMO.
Key words: Wireless ad hoc networks, MIMO, Network capacity.
I. I NTRODUCTION
MIMO (Multi-Input Multi-Output) systems where multiple antennas are deployed at both transmitter and receiver, open up a new dimension, i.e., space, to significantly improve the spectral efficiency of wireless communication systems. Foschini and Telatar [1]-[2] show that MIMO provides a linear growth of capacity with the number of antennas. Moreover, the extra degree of freedom, i.e., space, offered by multiple antennas enables interference cancelation at receiving stations, which allows spectrum to be reused more aggressively [3]-[7]. On the other hand, MANET (mobile ad hoc network) is likely to play a major role in next-generation home networks and hot spots, thanks to its simplicity, cost effectiveness, and simple reconfiguration [8]. One of the major challenges faced by MANET is the increasing demand for data-rate-intensive applications similar to those in its wired counterpart. Deploying multiple antennas at each node is a promising solution to improve network capacity and link reliability required by these applications. To fully exploit the benefits of MIMO in ad hoc networks, it is essential to have a thorough understanding of the fundamental impact of the use of MIMO on overall network performance.
Most previous work on capacity analysis of ad hoc networks is based on an implicit assumption that only one antenna is used at each node [9]. Under this assumption, an active link exclusively occupies a geometric area, referred to as the exclusion region, to avoid collisions with other links. The larger the exclusion region, the more spatial resource a link occupies, and the capacity of a network is essentially determined by the amount of spatial resource occupied by underlying links. When multiple antennas are deployed at each node, however, an active link no longer exclusively occupies a spatial area, making the original definition of “exclusion region" not applicable any more. In fact, multiple adjacent links can transmit at the same time, as long as the mutual interference can be suppressed by spatial signal processing. As such, previous work on capacity analysis is not directly applicable to ad hoc networks with MIMO links.
The impact of multiple antennas on network capacity was previously studied under different contexts. In [23]-[24], Zhang and Liew derived the upper and lower bounds on network capacity when directional antennas with realizable generic patterns are used. It is well known that directional antennas do not work well in indoor and urban environments where a large number of local scatterers cause severe multipath effects and large angular spread. Unfortunately, most application scenarios of ad hoc networks perceive rich-scattering channels. As a result, the previous analysis based on directional antennas does not apply to general MIMO ad hoc networks. In [15], Chen et al studied the ergodic single-hop capacity of ad-hoc networks when single-user detection is employed at each receiver. Though simple, single-user detectors
do not exploit the interference cancelation capability of MIMO. As a result, the capacity calculated in [15] is far below the actual achievable capacity of MIMO ad hoc networks.
In this paper, we investigate link-layer throughput capacity, defined as the total data rate that can be successfully delivered through all single-hop links within a unit area, of MIMO ad-hoc networks. In particular, MMSE (minimum mean square error), the optimal linear multiuser detection, is assumed to be deployed at receiving nodes, as it has the highest interference suppression capability among all linear detection schemes including ZF (zero forcing) and single user detection [25]. In contrast to previous work, we characterize the amount of spatial resource occupied by each link by the actual interference active links impose on each other, taking into consideration the actual behavior of multipath fading and MIMO systems.
One of the key challenges in this work is the characterization of the distribution of post-detection SINR (signal to interference and noise ratio) when transmitters are randomly located. SINR distribution of MMSE detectors was previously studied in [26]-[28], [19]. In [27] and [28], the authors proved asymptotic Normality of post-detection SINR for equal and non-equal interference power, respectively. In [19], Li et al improved the accuracy by modeling SINR using a Gamma or a generalized Gamma distribution. All these papers assumed that the signal strength of interfering data streams as detected at the receiver is deterministic and known. In ad-hoc networks, however, active nodes are randomly located, and hence the received interference power is also random. Moreover, previous work often assumed that the number of interferers is smaller than or comparable to the number of receive antennas. While the assumption is reasonable for traditional cellular networks, it is not true in ad hoc networks where the number of simultaneously transmitting stations could be much larger than the number of antennas at a receiving node. The main contributions of this paper can be summarized as follows.
? We derive a closed-form expression for the distribution of received SINR of an active link with
maximum ratio transmission and a multiuser MMSE receiver. In contrast to [26]-[28], [19], signal power, interference power, and the number of interferers are all random variables due to the randomness in the location and activity of transmitting nodes. The analytical results are validated by numerical simulations.
? Based on the SINR distribution, we analyze link-layer throughput capacity, calculated as the sum data rate that can be delivered by all links within a unit area. In contrast to [15] where the data rate of each link is represented by ergodic capacity, per-link data rate is defined as in this paper, where is the transmission rate of each link and is the
=(1)out Th P q ?q out P
probability of transmission failure given . This definition more accurately reflects the characteristics of today's ad-hoc networks, where multipath fading varies slowly compared with the transmission time of a packet. Unlike the mean value analysis in [19], the PDF (probability density function) of SINR is needed in this paper to calculate , which makes the job here much more challenging. Our work on link-layer capacity paves the way for further study on network-layer transport capacity of ad-hoc networks with MIMO links.
q out P ? The analysis suggests that there exists an optimal density of simultaneously transmitting links that maximizes the link-layer capacity. Through numerical study, we calculate the optimal density under various scenarios. In real implementation, the optimal active-link density can be mapped to an optimal transmission probability in the MAC (medium access control) layer. This result serves as a guideline for the design of MAC protocols of next-generation ad-hoc networks with MIMO links.
? Our analysis is based on the assumption that CSI (channel state information) from all transmitting nodes to the receiver of a tagged link is available at the receiver. In practice, it is likely that a receiver only knows CSI from a few neighboring links. In this paper, we also study the link-layer capacity and the corresponding optimal link density when only local CSI is available.
The remainder of the paper is organized as follows. The system and signal models are presented in Section II. In Section III, we derive the distribution of post-detection SINR in ad hoc networks when MMSE receivers are deployed. The link-layer throughput capacity is then given in Section IV. Numerical examples and discussions are presented in Section V, where we also study the impact of partial CSI on the capacity and optimal link density. Finally, the paper is concluded in Section VI.
II. S YSTEM M ODEL
We first describe the notation used in this paper for readers' convenience. Throughout the paper, scalars are given by normal letters, vectors by boldface lower case letters, and matrices by boldface upper case letters. Besides, the following notations are used. T X : Transpose operation *X : Hermitian transpose []ij X : th element of (,)i j X Tr()X : trace of matrix
X
E()i : Expectation Var()i : Variance
A. System Description
Consider an ad hoc network as demonstrated in Fig. 1, where mobile nodes are uniformly distributed within an area. Each node is equipped with antennas. For simplicity, we ignore the edge effects and assume that each link has the same statistical characteristics. Without loss of generality, let Link 0 be the tagged link. At a given time, there are other links sending data at the same time as Link 0, resulting in co-channel interference. As a result, the data received by the tagged link, given as follows, is a superposition of desired signal, interference, and noise.
m K
000=K
k k k 0
++y x x n
(1)
In the above, is an m m channel matrix, representing the channel fading from the transmitter of the th link to the receiver of the tagged link. Assuming a rich scattering environment and quasi-static Rayleigh flat fading channels, we can model the elements of as i.i.d. complex Gaussian random variables. Likewise, denotes the transmit signal vector of Link ; k H ×k k H 0x k k p the transmit power of Link
; k k α the path loss from the transmitter of Link to the receiver of the tagged link; and the
AWGN (additive white Gaussian noise) with zero mean and unit variance.
k 0n Note that the number of interferers, , is a random variable depending on the transmission probability of links. Since the neighborhood observed by each link is statistically identical, we assume that the interferers are randomly located within a disc of radius K K R centered at the tagged receiver, where R is the largest distance at which an interferer can cause non-negligible interference to the receiver. Furthermore, let ε denote the minimum separation between interferers and the tagged receiver. Assume that ε is small enough so that it does not affect the uniform distribution of nodes. Thus, the probability density function of the distance between a node and the tagged receiver is given by
222()=
d x
f x R ε?
(2)
Let be the distance between the th transmitter to the tagged receiver and k c k θ be the path loss exponent. In particular, is the length of the tagged link. We can calculate the received power from the th interferer as
0c k
000
=()k k k
c p p c θαα
(3)
whose PDF is
2/2
00000000022(2)/2()()=()(.()p k k p c c c f x p R x R θθθ
αθθαααθεε+?≤≤?x p
(4)
When =4θ,
44
000000()=()()p k k c c f x p R αααε?≤≤x p j v r
(5)
B. Maximum Ratio Transmission and MMSE Reception
It was proved in [29] that SVD (singular value decomposition) based space-time vector coding allows the collection of signal power in space and it is a theoretical means to achieve high capacity for MIMO systems. By SVD, can be decomposed into
k H
(6)
*,,,=1
=,
r k
k k j k j k j λ∑H u where ,1,2,k k k k
λλ≥≥≥ λ are the eigenvalues, and are the left singular vector and right singular vector, respectively, and is the rank of . Note that the left and right singular vectors have the same distribution as normalized complex Gaussian random vectors [20]-[21]. Likewise, the distribution of the square of the largest singular value ,k j u H ,k j v 2,1k k r k λ is given by [17] as a finite linear combination of elementary Gamma densities:
2
1222,,1
=1
=0
()=>0,!l l nx
m mn n n l
k n l n x e f
x g x l λ+???∑
∑
(7)
where are computed and listed in [17] for most antenna configurations of interest. The ,n l g τth moment of 2,1k λ is
()2
22,2,1=1
=0
()E []=.!
m mn n n l k n l g l n l ττ
!τλ?+∑
∑
(8)
In an interference-limited environment such as ad hoc networks, an active link should transmit only
one data stream at a time to optimize the system performance [11]-[13]. In this case, the single data stream should be transmitted on the largest singular mode of the channel for SNR (signal to noise ratio) maximization. Such scheme, known as MRT (maximum ratio transmission), configures the transmit
antenna weight using the right singular vector corresponding to the dominant singular value.1 For example, the transmit antenna weight of Link 0 is . Similarly, the transmit beamforming vector of Link , denoted by , is the dominant singular vector of the channel matrix between its own transmitter-receiver pair. Therefore, the received signal in (1) becomes
0,1v k k t
000,10=K
k k k k b b 0++y v t n
0,10,100
=K
k k k b b ++u n (9) where 0y is
a vector with the th element being the received signal on the th receive antenna. Denote by , whose elements are still i.i.d. complex Gaussian random variables with zero mean and unit variance [2], since has unit norm and is independent of . 1m ×?k
h i i k k H t k t k H Define equivalent channel matrix as G
(10)
0,10,11??=[,,,],K
λG u h h and the transmit power matrix as
0011=.K K p p p ααα??????????
??P
(11)
We can then rewrite (9) into a matrix form as
1/200=,+y GP b n
(12)
where is .
b 01[,,,]T K b b b Upon receiving the signal, the tagged receiver attempts to obtain an estimate of from the received signal b 0y . Being the optimal linear detector, MMSE detector minimizes the mean square error between
and its estimate. Specifically, the decision statistics b is obtained by linearly combining the received signal vector as follows: b
1
To implement MRT, transmitter-side CSI is needed. Transmitter-side CSI is easily achievable in wireless networks with
two-way communications. In case it is not available, random antenna selection or space time coding can be deployed instead of MRT. Our analysis can be easily extended to these cases with slight modification. Specifically, it is the distribution of 0,1λ that needs to be modified in the analysis.
*0=b V y
(13)
where and ()1
*1=K ?++V I G PG GP 1K +I is a (1)(1)K K +×+ Identity matrix. With MMSE, the post-detection SINR of the tagged link can be calculated as [25]
()01*11,1
1
SINR =
1K ?+???+????I G PG
(14)
where denotes the element of a matrix. The distribution of SINR was previously studied in [26]-[28], [19]. However, their work assumes that interference power (i.e., 1,1[]?(1,1)th k k p α for ) is deterministic and known. This assumption, however, is not applicable to ad hoc networks where interfering links are randomly located. In this paper, we focus on MMSE receivers, for it achieves the optimal performance in terms of BER (bit error rate) or SINR among all linear detectors. Our conclusions, however, can easily be extended to other suboptimal detectors such as ZF (zero forcing) and single-user detection.
>0k Note that eqns. (13) and (14) have assumed that the tagged receiver has the knowledge of for all . Although not realistic, this assumption allows us to investigate the fundamental limit of wireless
MIMO network capacity without taking into account implementation details. This assumption will later be removed in Section V in Fig. 8 and Fig. 9, where the receiver only knows the CSI from its neighboring interfering nodes. ?k h k
III. SINR D ISTRIBUTION OF MMSE
In this section, we derive the distribution of SINR in ad hoc networks when MMSE detection is deployed. To this end, we first compute the mean and variance of SINR in subsections III.A to III.E. The PDF of SINR is then presented in III.F.
A. Simplified Form of SINR We define 1/2=G
P G
0,10,11=,K ??u ….
(15)
and then have
2
**
000,1
0,10,11
**10,10,111
=p αλλ????,
????????
u G G
G G u G G (16)
where is G with first column removed. 1
?G Before going further, we first describe the following lemma. Lemma 1: Write a matrix into
A
*
1,1
1,1
1,1
1,1=,a
a a ??????????
A A
(17)
where is the element of , 1,1a (1,1)th A 1,1a ? is the first column of with the first element removed and is with the first column and row removed. Then, A 1,??A 1A
()1
1*11,11,11,11,11,1[]=()a a a .
????????A A
(18)
By using Lemma 1, (14) can be simplified as
()
01
*11,1
1
SINR =
1K ?+???
+???
?I G G
()
1
22***000,1000,10,1111
10,1
=.K p p αλαλ??????+u G I G G G u
(19)
Denoting the SVD of as 1
?G
(20)
1
=,?G WDZ where the i th diagonal element of is , we then can derive the SINR as D i d ()1
22*
****0000,1000,10,10,1SINR =K p p αλαλ??+u WDZ I Z D DZ Z D W u *
()
(
)
1
2*
*11*000,10,10,1=m m p αλ????+u W I I D D W u
2**1*000,10,10,1=()m p αλ?+u W I DD W u
(21)
2*000,10,10,1=p αλu Bu ,where is defined as . B *1*()m ?+W I DD W B. Conditional Mean of SINR
It is easy to see that is deterministic function of and . Given a channel realization, the conditional mean of given is
B 0NR G P SI B
(22)
2
*0000,10,10,1E(SINR |)=E()E().
p αλB u Bu j Likewise,
*
*()()*()()
0,1
0,10,10,10,10,1=1E()=E E m i i i j ii ij i i j u u B u u B ≠????+????????
∑∑u Bu ()()()2*()()
0,10,10,1=1
=E ||E m
i i ii ij i i j
B u u B ≠+∑∑u
()*()()
0,1
0,11=
Tr()E i j ij i j
u u B m ≠+∑B (23) where ij B is the th element of , and is the th element of . Since is a normalized complex Gaussian random vector as mentioned in Section II, it is not difficult to prove that (,)i j B ()
0,1
i u i 0,1u 0,1u
()*()()
0,10,1E =0i j u u i j .
?≠
(24)
Hence, we have the conditional expectation of given as shown in (25). 0SINR B
()()
12
*0000,1
1E(SINR |)=
E()Tr m p m
αλ?+B W I D *D W ()
()1
2*000,1
1=E()Tr m
p m
αλ?+I
DD
2000,1
2=1
11=E()1m i i p m d αλ??
?+??∑?m j (25) C. Conditional Second Moment of SINR
We now derive the conditional second moment of SINR given .
B Denoting as , and ()40,1E(||)(1)i u i ≤≤1a ()2()2
0,10,1E(||||)()i j u u i ≠ as , respectively, we first
derive (26) from (23). 2a
()*
2
()4*()2()2*()2()2*0,1
0,10,1
0,10,10,10,1=1
E ()
=E(||)E(||||)E(||||)m
i i j i j ii ii ij ij ii jj i i j
i j
u
B B u u B B u u B B ≠≠++∑∑∑u Bu
()()*()*()
**1
2120,10,10,10,111
22
,,,11221212
E()i j j i i j i j i j i j i i j j u u u u B B ≠≠≠≠+
∑
()4*()2()2*()2()2*
0,10,10,10,10,1=1
=E(||)E(||||)E(||||)m
i i j i j ii ii ij ij ii jj i i j
i j u B B u u B B u u B B ≠≠++∑∑∑
*
*
*
1222=1
=1
=Tr()Tr ()Tr()2m
m
ii
ii ii ii i i a B B a a a B B ++?∑∑B B B B *
(26)
()*
*
*
21=1
=Tr()Tr ()Tr()(2)m
ii jj i a a ++?∑B B B B 2a B B ,where the second equality is due to the fact that
(
)()()*()*()
1212
0,10,10,10,11
1
2
21212
E =0,,i j j i u u u u i j i
j i i j j ?≠≠≠≠
(27)
Since is a normalized complex Gaussian random vector, the PDF of is 0,1u ()2
0,1
|i u |
22
()0,1
()=(1)(1),0 1.
m i u f
x m x x ???≤≤
(28)
Therefore, we have ()2
0,11
E(||)=
,i u m
(29)
and
()4
0,12
E(||)=
.(1)
i u m m + (30)
We can then derive the pdf of on the condition of as
()20,1|i u |y ()2
0,1||=j u
3
22()()0,10,1
21(|)=01.
11m i j u u m x y f x y x y y y ???
???≤≤???????
(31)
Then, we have as
follows. ()2()2
0,10,1E(||||)i j u u
11()2()2
0,10,1()2
22
00
||()()0,10,10,1
E(||||)=(|)()y
i j j u i j u u u u xyf
x y f
y dxdy ?∫
∫
3
1120
21=(1)(1)11m y
m m x y y m y x d y y ????????????????
∫∫
xdy
1
=
(1m m )
+ (32)
From eqns. (26) and (32), we have
()()*2
**0,10,11
E ()=
Tr()Tr ()Tr()(1)
m m ++u Bu B B B B
2
2
2=1=11
11=(1)11m m i i i i m m d d ??
?????+?????+++??????
∑∑2?? (33) We are now ready to derive the second moment of as
0SINR
()2224*0000,10,10,1E(SINR |)=E()E ()p αλB u 2
Bu
22
224
000,12=1=1111=E()(1)11m m i i i i p m m d d αλ????????+??????+++??????
∑∑2. (34) D. Mean of SINR
In order to compute the mean of , we first introduce the method of asymptotic analysis of random matrix [18]. 0SINR Definition of η transform:
Given a nonnegative random variable χ, the η transform is defined as
1()=E 1χηγγχ?
?
?
?+??
(35)
Theorem 1 [18]: Let be an m K matrix whose entries are i.i.d. complex Gaussian variables with
variance
H ×1
m
. Let be a Hermitian nonnegative random matrix, independent of , whose empirical eigenvalue distribution converges almost surely to a nonrandom limit. The empirical eigenvalue distribution of converges almost surely, as with T K K *HTH ×H ,K m →∞K
m
β→, to a distribution whose η-transform satisfies
1=
,
1()η
βηγη??T
(36)
where for simplicity we have abbreviated *
()=ηγηHTH . *
()η
?HTH and ()η?T stand for the η
transform of the eigenvalues of and T , respectively.
*HTH Theorem 1 can be applied to find the empirical eigenvalue distribution of , i.e., the empirical
distribution of . Rewrite the matrix as
*11??G G 2i d
**11111
=.m ?????G G P
(37)
where is with first column removed. Then, from Theorem 1, the empirical eigenvalue
distribution of converges almost surely to a distribution whose 1?P P G G *1?? 1η transform
satisfies
*11*1
11
1()
=,1((m K
m ηγ))
ηγηγ???????G G P G G
(38)
where 1
()m ηγ?P is
the η transform of the eigenvalue of 1m ?P . We now begin to derive 1
()m ηγ?P . Since 1?P is a diagonal matrix, the empirical eigenvalue
distribution of is the distribution of its diagonal elements of 1?P k k p α. Given the distribution of k k p α in (3), we derive the η transform of 1m ?P as
11()=E 1m k k
m p ηγγα????
?+??
P
=1tan tan ????
?
(39)
Substituting (39) to (38), we have
1
*11
1()=
tan ηγ????G G
1tan ?? (40)
We are now ready to derive the mean of as
0SINR ()00E(SINR )=E E(SINR |)B B
2000,1
2=111=E()E 1m i i p m d αλ??
?+??
∑.?
(41) Note that
*211=111E =1m i i m d η??????+??∑G G (1),
(42)
which can be computed by (40) as
1
*11
1(1)=
tan η????G G
1tan ?? (43)
At last, we have the mean of as
0SINR 20000,1
2=111E(SINR )=E()E 1m i i p m d αλ??
??
+??
∑
(44)
2
000,1*11
=E()(p αλη??G G
1)0E. Variance of SINR
We now begin to derive the various of .
0SINR 00Var(SINR )=E (Var(SINR |))Var(E(SINR |))+B B B
(45)
0E(Var(SINR |)),≈B where the approximation is due to the fact that converges to zero as the rank of becomes very large. 0Var(E(SINR |))B B
00Var(SINR )E (Var(SINR |))≈B B
()2
200=E E(SINR |)E (SINR |)?B B B
22
224
000,122=1=1111=E E()(1)11m m i i i i p m m d d αλ??????????+?????+++??????????∑∑B
2
220,12=111E ()1m
i i m d λ????
????+?
???
∑
2422()
0,10,122
002=1E()E ()1E 1m i i i p m
d λλα???????≈????+????
∑
222422
000,1
0,121=(E()E ())E 1i p d αλλ??
?????????+???? 22
242
20
0,1
0,1
221=(E()E ())E 1(1)i i i d p d d αλλ??
????++??
2
()()22422000,10,1=E()E ()(1)(1p αλληη′?+), (46)
where approximation (i ) is due to the following inequality:
22
22=1=111.11m m
i i i i m d d ???≤???++???∑∑?
??
(47)
where the equality holds if all are equal. i d s ′F. Probability Density Function of
0SINR The close-form PDF of SINR is known to be difficult to derive. Fortunately, it can be seen from (21) that the SINR is a summation of many positive terms. Therefore, a Gamma distribution can e used to approximate the SINR according to central limit theorem for causal functions [22] as follows.
/1
SINR 0
()=,>0,()x b
a a e f x x
x b a ???Γ
(48)
where , , and 200=E (SINR )/Var(SINR )a 00=Var(SINR )/E(SINR )b ()a Γ is the gamma function. Then, the CDF (cumulative distribution function) of is
0SINR
/1SINR SINR 0
1()=()=
.
()x
x a t
F x f x dx t e dt a ??Γ∫∫b
(49)
In Fig. 2 and Fig. 3, we respectively plot the CDF of SINR with 2 and 20 interfering nodes when there are 4 antennas at each station. Assume that the length of the tagged link, , is normalized to 1 and the average received SNR 0c 000p N α is equal to 20 dB. The interfering links are uniformly distributed within a disc with . The minimum separation between the tagged receiver and interferers is
=3R =0.1ε. From the figures, we can see that although the analytical results come from asymptotic analysis, they match the simulation results very well even with a small number of antennas.
IV. L INK -L AYER T HROUGHPUT C APACITY
In this section, we investigate the link-layer throughput capacity of wireless ad hoc networks, which is defined as the total data rate that can be successfully delivered through all single-hop links per unit area . Assume that an active link transmits at data rate . The transmission is successful only when SINR at the receiver side is higher than a threshold, , which is a function of . To be more specific, the relationship between q and is defined as q th SINR q SINR th
2=(1SINR log th q ).+
(50)
Denote by the probability of transmission failure of a link, which is calculated from the CDF of
out P
0SINR derived in the last section.
0=Pr(SINR (51) SINR 0 =(SINR th F )Therefore, the throughput of a communicating link is given by =(1).out Th P q ? (52) If the radius of the network R is very large so that the edge effect is negligible, we can assume that each link experiences homogeneous channel and interference conditions. As a result, the throughput is the same for all links in the network. When there are 1K + active links (one tagged link and interfering links) simultaneously transmitting in the network, we can evaluate the capacity of the network as the summation of the throughput of all the links. K (53) (1)=(1)(1)out C K K P q ++? .In wireless ad hoc networks, the number of active links varies from time to time due to the random-access nature of links. Assume that there are in total links per unit area and each link transmits with a probability L t p . Then, the average number of active links is equal to 200=K R ρπ (54) where 0=t Lp ρ is the average number of active links per unit area. When is large, the number of active links follows Poisson distribution. The probability of having L 1K + active links in the network is given by 10 0Pr(1)=. (1)!K K K e K K ?+++ (55) Finally, we have the link-layer throughput capacity of the network as 2 =0 1= (1)Pr(K C C K K R π∞ 1).++∑ (56) V. S IMULATION AND N UMERICAL R ESULTS As shown in the last section, link-layer capacity of wireless networks heavily depends on the number of simultaneously active links within a unit area. This section investigates the impact of the density of active links on the capacity through numerical results. Moreover, the effect of incomplete channel state information is studied. Similar to Fig. 2 and Fig. 3, we normalize the length of the tagged link to 1 and assume that the average SNR at the tagged receiver is 20dB. The SNR threshold for the communication pair is 10dB. For simplicity, assume that all transmitters have the same transmission power. Around the tagged receiver, interfering links are uniformly distributed in the space. The minimum separation between the tagged receiver and interferers is SINR th =0.1ε. Given an average density of active links 0ρ, the number of active links is randomly generated according to Poisson distribution in (56). We first validate the analytical results derived in the previous sections by comparing them with simulation results. In Fig. 4 and Fig. 5, the mean and second moment of SINR are plotted against average link density when there are 2, 4, and 6 antennas at each node, respectively. It is not surprising that both mean and second moment decrease as the link density increases. The figures show that our analytical results match the simulations well. In Fig. 6, link-layer throughput capacity defined in (56) is plotted against the density of active links, 0ρ. From the figure, we can see that when the active-link density is low, capacity increases with the number of active links, as the interference can be well handled by multiple antennas. However, when 0ρ exceeds a certain level, co-channel interference becomes so severe that link-layer capacity starts to decrease. As expected, the optimal density of active links that maximizes link-layer capacity increases with the number of antennas, for more co-channel interference can be tolerated when there are a larger number of antennas at each station. Moreover, link-layer capacity increases as the number of antennas increases. For example, the maximal capacity for networks with 2, 4, and 6 antennas is about 0.25, 0.78, and 1.53 bps/Hz/, respectively. A close observation of the figure reveals an interesting fact: The maximal capacity increases faster than the number of antennas. In particular, the normalized maximal capacity (normalized by the number of antennas) is equal to 0.125, 0.195, and 0.255 bps/Hz/, respectively. This provides a strong incentive in deploying multiple antennas in future wireless networks. 2m 2m In Fig. 7, the optimal active-link density, denoted by *ρ, is plotted as a function of the number of antennas at each station. To validate the analysis, simulation results are also plotted in the figure. The figure shows that our analysis can accurately predict the optimal density of active links in wireless networks with MIMO links. In wireless networks, active-link density is directly related to the transmission probability of existing links, as shown in the last section. In traditional wireless networks, transmission probability is usually selected according to the network contention level. In this paper, we argue that the optimal transmission probability should be determined by the characteristics of PHY-layer co-channel interference as well as the interference cancelation capability at each receiver. As Fig. 7 shows, the optimal transmission probability can be accurately calculated through our analysis. The observations in Fig. 6 and Fig. 7 serves as a guideline in designing the transmission probability in wireless networks with MIMO links. So far, we have assumed CSI at each receiving node. That is, the receiver knows the channel matrices (see eqn. (10)) from all interfering nodes. In practice, however, it is difficult for a receiver to monitor the CSI on all links. Hence, it would be interesting to investigate network capacity in a more practical scenario where only the CSI from neighboring interferers is available. In Fig. 8, we assume that a receiving node only estimates the channel from interferers that are located within distance 2 from the receiver. Interference from other interferers is treated as noise. By restricting the channel-monitoring range, the computational complexity due to channel estimation and MMSE detection can be significantly reduced. The figure shows that the maximum throughput is slightly reduced from to when the channel-monitoring range is restricted to 2. Intuitively, the larger the channel-estimation range, the higher the capacity. In real implementation, one can trade off between computational complexity and achievable capacity. ?k h 0.82/bps m 20.71/bps m In this paper, we have assumed that the optimal linear detector, MMSE, is deployed at each receiver. In real systems, suboptimal detectors such as zero-forcing (ZF) detector are also widely used due to the easy implementation. In the case of ZF, V in eqn. (13) satisfies *=,+V G (57) where denotes the psudo inverse of matrix . For comparison purpose, we investigate the link-layer capacity when ZF detector is deployed in Fig. 9. Note that the number of interferences a ZF detector can handle is no more than +G G 1m ?, where is the number of antennas. In the figure, we assume that the strongest interferences are canceled by the ZF detector. Similar to the case of MMSE detector, the figure shows that there exists an optimal active-link density when ZF detector is deployed. However, the maximum capacity is reduced by more than compared with the MMSE detector. Due to the lower interference cancelation capability of ZF compared with MMSE, the optimal link density is also reduced. m 1m ?30% VI. C ONCLUSION In this paper, we have investigated the link-layer throughput capacity of wireless ad hoc networks when multiple antennas are deployed at each node. In contrast to previous work where network capacity is calculated as if each link exclusively occupies a geometric area, we have argued that it is indeed the characteristics of PHY-layer interference and the interference cancelation capability of receivers that determines the network capacity. This is especially true in networks with MIMO links, where links can transmit simultaneously in the vicinity of each other, with co-channel interference being reduced via space-domain signal processing. One key contribution of this work is the characterization of distribution of post-detection SINR of MMSE receivers when the number and locations of interferers are random. The PHY-layer SINR is then translated into MAC-layer throughput capacity in wireless ad hoc networks. We have shown that there exists an optimal transmission probability that maximizes network throughput capacity. In particular, the optimal transmission probability is determined by the number of antennas as well as the multiuser detection scheme deployed at each node. This observation serves as a guideline for the design of MAC protocols in future wireless ad-hoc networks with MIMO links. References: [1] G. J. Foschini and M. J. Gans, ``On limits of wireless communications in a fding environment when using multiple antennas," Wireless Personal Commun.: Kluwer Academic Press, no. 6, pp. 311-335, 1998. [2] E. Telatar, ``Capacity of multi-antenna Gaussian channels," Eur. Trans. Telecom ETT, vol. 10,no. 6, pp. 585-596, Nov. 1998. [3] Q. H. Spencer, C. B. Peel, A. L. Swindlehurst, and M. Haardt, ``An introduction to the multi-user MIMO downlink," IEEE Commun. Mag., pp. 60-67, Oct. 2004. [4] G. Caire and S. Shamai, ``On the achievable throughput of a multiantenna Gaussian broadcast channel,'' IEEE Trans. Inf. Theory, vol.49, pp. 1691-1706, July 2003. [5] P. Viswanath and D. Tse, ``Sum capacity of the vector Gaussian broadcast channel and uplink-downlink duality ," IEEE Trans. Inf. theory, vol. 49, pp. 1912-1921, Aug. 2003. [6] W. Rhee and J. M. Cioffi, ``On the capacity of multiuser wireless channels with multiple antennas," IEEE Trans. Inf. Theory, vol. 49, pp. 2580-2595, Oct. 2003. [7] W. Yu, W. Rhee, S. Boyd, and J. M. Cioffi, ``Iterative water filling for Gaussian vector multiple-access channels," IEEE Trans. Inf. Theory, vol. 50, no. 1, pp. 145-152, Jan. 2004. [8] G. Anastasi, M. Conti, and E. Gregori, IEEE 802.11 Ad Hoc Networks: Protocols, Performance and Open Issues, New York: IEEE Press?CWiley, 2004. [9] P. Gupta and P. R. Kumar, ``The capacity of wireless networks," IEEE Trans. Inf. Theory, vol. 46, pp. 388-404, Mar. 2000. [10] S. Toumpis and A. J. Goldsmith, ``Capacity regions for wireless ad hoc networks," IEEE Trans. Wireless Commun., vol. 2, pp. 736-748, Jul. 2003. [11] R. S. Blum, ``MIMO capacity with interfrence," IEEE J. Selected Area Commun., vol. 21, no. 5, pp. 793-801, June 2003. [12] R. S. Blum, ``On the capacity of cellular systems with MIMO," IEEE Comm. Lett., vol. 6, no. 6, pp. 242-244, June, 2002. [13] W. Choi and J.G. Andrews, ``On spatial multiplexing in cellular MIMO-CDMA systems with linear receivers," in Proc. IEEE Int. Conf. Commun., vol. 4, pp. 2277-2281, May, 2005. [14] Y. Tokgoz and B. D. Rao, ``Performance analysis of maximum ratio transmission based multi-celluar MIMO systems," IEEE Trans. On Wireless Comm., vol. 5, no. 1, pp. 83-89, Jan. 2006. [15] B. Chen and M. J. Gans, ``MIMO communications in Ad Hoc networks," IEEE Trans. on Sig. Processing, vol. 54, no. 7, pp. 2773-2783, June 2006. [16] M. Zorzi, J. Zeidler, A. Anderson, B. Rao, J. Proakis, A. L. Swindlehurst and M. Jensen, ``Cross-layer issues in MAC protocol design for MIMO ad hoc networks," IEEE Wireless Comm., vol. 13, no. 4, pp.62-76, Aug. 2006. [17] P. A. Dighe, R. K. Mallik, and S. S. Jamuar, ``Analysis of transmit receive diversity in Rayleigh fading," IEEE Trans. Commun., vol. 51, no. 4, pp. 694-703, Apr. 2003. [18] A. M. Tulino and S. Verdu, Random Matrix Theory and Wireless Communications, Delft : Now, 2004. [19] P. Li, D. Paul, R. Narasimhan, and J. Cioffi, ``On the distribution of SINR for the MMSE MIMO receiver and performance analysis," IEEE Trans. on Inf. Theory, Vol. 52, No. 1, pp. 271-286, Jan. 2006. [20] R. J. Muirhead, Aspects of Multivariate Statistical Theory. Wiley, 1982. [21] N. R. Goodman, ``Statistical analysis based on a certain multivariate complex gaussian distribution (an introduction)," Annals of Mathematical Statistics, vol. 34, pp. 152-177, 1963. [22] A. Papoulis, The Fourier Integral and its Applications. New York: McGraw-Hill, 1962. [23] J. Zhang and S. C. Liew, ``Capacity improvement of wireless ad hoc networks with directional antennae," ACM MobiCom'05, Aug. 2005. [24] J. Zhang and S. C. Liew, ``Capacity improvement of wireless ad hoc networks with directional fast ap设置教程 ap可以为原来的有线网络提供无线接入,满足家庭和小型企业的多种上网需求。 Fast(迅捷)FW150RM无线路由器完成AP模式的设置,一共需要5个配置步骤:1、设置电脑IP;2、设备连接;3、设置FW150RM;4、再次设置电脑IP;5、修改FW150RM管理IP地址. 步骤一:设置电脑IP 迅捷FW150RM无线路由器在AP模式下并未启用DHCP 服务器,所以无法给电脑自动分配IP地址,因此需要用户自己给电脑的无线网卡配置固定IP地址。将电脑无线网络连接IP地址设置为192.168.1.X(1 X 252),子网掩码设置为:255.255.255.0,如下图所示:设置方法可以参考:电脑固定IP地址设置方法 步骤二:设备连接 1、FW150RW连接到网络:用一根网线一段连接在有线网络中的路由器或者交换机上,另一端与Fast FW150RW进行连接。 2、电脑与FW150RW连接:这里电脑只能用无线的方式与FW150RW路由器进行连接,用电脑上的无线网卡搜索附近的无线网络,找到FW150RW的SSID后,点击连接即可。注意:FW150RM默认SSID是FAST_XXXXXX( XXXXXX 是FW150RM无线MAC地址后六位),且并未设置无线安全,所以这里可以直接进行连接。 步骤三:设置FW150RW路由器 1、登录管理界面:在浏览器中输入192.168.1.253 并按下回车键,输入登陆用户名及密码均为admin,打开FW150RM的管理界面。 2、运行设置向导:首次登录后会自动运行设置向导,如果 位运行可以点击左侧的设置向导下一步。 3、选择工作模式:选择AP 模式下一步。 4、配置无线网络:如果需要可以修改SSID 选择WPA-PSK/WPA2-PSK 设置PSK密码(本例为abcdefgh) 下一步。 5、重启FW150RW:点击重启。 重启完成后,无线AP模式的配置就生效了,笔记本、手机等无线终端就可以连接迅捷FW150RW的无线网络上网了。 步骤四:再次设置电脑IP 这里需要把刚才电脑的无线网卡的IP地址重新配置为自动获得,这样电脑才能通过FW150RW的无线网络正常上网,其它需要上网的无线终端也建议把IP地址配置为自动获得,配置方法可以参考:电脑自动获取IP地址的设置方法。 步骤五:更改FW150RW管理IP地址 并不是一定要更改迅捷(Fast)FW150RW的管理IP地址,只有当原来的局域网中192.168.1.253这个IP地址已经被使用了,为了避免IP地址冲突,才需要修改的;如果192.168.1.253这个IP 地址未被使用,则无需更改FW150RW的管理地址了。 修改方法:登录管理界面网络参数LAN口设置,修改IP之后点击保存,路由器自动重启 FW300RM AP模式设置 1、运行设置向导:进入路由器的管理界面后,点击设置向导点击下一步。 FW300RM的设置向导 2、选择工作模式:这里需选择AP:接入点模式点击下一步。 实验一 常用仪器的使用 一、实验目的 (1) 了解双踪示波器、函数信号发生器、数字万用表的原理框图和主要技术指标。 (2) 掌握用双踪示波器测量信号的幅度、频率和相位。 (3) 掌握万用表的正确使用方法。 二、实验仪器 (1) 双踪示波器; (2) 低频信号发生器; (3) 数字式(或指针式)万用表。 三、实验原理 在电子技术实验里,测试和定量分析电路的静态和动态的工作状况时,最常用的电子仪器有:示波器、低频信号发生器、直流稳压电源、晶体管毫伏表、数字式(或指针式)万用表等。它们之间的连接方式如下图所示。 输出信号 图1-1电子技术实验中测量仪器、仪表连接框图 示波器:用来观察电路中各点的波形,以监视电路是否正常工作,同时还用于测量波形的周期、幅度、相位差及观察电路的特性曲线等。 函数信号发生器:为电路提供各种频率和幅度的输入信号。 直流稳压电源:为电路提供电源。 数字式(或指针式)万用表:用于测量电路的静态工作点和直流信号的值等。 四、实验内容及步骤: 熟悉仪器(仪器使用简单步骤见附录) 1.学会正确使用函数信号发生器 2.学会正确使用数字示波器 3.熟悉并学会使用数字式万用表 4.熟悉模拟电路实验箱 五、实验步骤 1、使用函数信号发生器输出频率的调节方法 (1)使用Sine按键,波形图标变为正弦信号,并在状态区左侧出现“Sine”字样。按Sine → 频率/周期→ 频率,设置频率参数值。配合面上的“频率调节”旋钮可使信号发生器输出频率在1HZ~10MHZ的范围改变。 屏幕中显示的频率为上电时的默认值,或者是预先选定的频率。在更改参数时,如果当前频率值对于新波形是有效的,则继续使用当前值。若要设置波形周期,则再次按频率/ 周期软键,以切换到周期软键(当前选项为反色显示)。 使用数字键盘,输入所需的频率值。直接输入所选参数值,然后选择频率所需单位,按下对应于所需单位的软键。也可以使用左右键选择需要修改的参数值的数位,使用旋钮改变该数位值的大小。 (2)根据手册通过设置频率/周期、幅值/高电平、偏移/低电平、相位,可以得到不同参数值的正弦波。 2、双踪示波器的使用 (1)使用前的检查与校准 (2)交流信号电压幅值的测量 使低频信号发生器信号频率为1kHz、信号幅度为5V,适当选择示波器灵敏度选择开关“V/div”的位置,使示波器屏上能观察到完整、稳定的正弦波,则此时上纵向坐标表示每格的电压伏特数,根据被测波形在纵向高度所占格数便可读出电压的数值,置于表1-1 中要求的位置并测出其结果记入表中。 注意:若使用10:1 探头电缆时,应将探头本身的衰减量考虑进去。 (3) 交流信号频率值的测量 将示波器扫描速率中的“微调”置于校准位置,在预先校正好的条件下,此时扫描速率开关“t/div”的刻度值表示屏幕横向坐标每格所表示的时间值。根据被测信号波形在横向所占的格数直接读出信号的周期,若要测量频率只需将被测的周期求倒数即为频率值。按表1-5 所示频率,由信号发生器输出信号,用示波器测出其周期,再计算频率,并将所测结果与已知频率比较。 检测机构一览表 序号机构名称备注 1. C-001 信息产业部第四研究所(电子工业安全与电磁兼容检测中心) 2. C-002 信息产业部第三研究所(国家广播电视产品检测中心) 3. C-003 上海市电子仪表标准计量测试所 4. C-004 中国赛宝实验室 5. C-005 广州日用电器检测所 6. C-006 中国家用电器研究院 7. C-007 机械工业电线电缆质量检测中心(北京) 8. C-008 上海电缆研究所 9. C-009 上海电器设备检测所 10. C-010 成都电气检验所 11. C-011 上海电气器具检验测试所 12. C-012 国家电光源质量监督检验中心(北京) 13. C-013 国家灯具质量监督检验中心(上海) 14. C-014 中国泰尔实验室 15. C-015 信息产业部通信计量中心 16. C-016 上海市劳动保护科学研究所特种电器检测站 17. C-017 上海出入境检验检疫局电器检测室 18. C-018 浙江检验检疫技术中心 19. C-019 江苏检验检疫机电产品检测中心 20. C-020 深圳出入境检验检疫局工业品检测技术中心 21. C-021 沈阳出入境检验检疫局电子电器产品检测中心 22. C-022 深圳电子产品质量检测中心 23. C-023 广州出入境检验检疫局电气安全实验室 24. C-024 (C-205) (Q-012) 国家质量技术监督局广州电气安全检验所 25. C-026 浙江方圆检测股份有限公司 26. C-027 (SL-036) 福建省中心检验所 27. C-028 上海市产品质量监督检验所 28. C-029 四川省产品质量监督检验所 29. C-030 成都市产品质量监督检验所 30. C-031 深圳市计量质量检测研究院 31. C-032 (SL-007) 大连市产品质量监督检验所 32. C-033 山东省计量科学研究所 一、常见仪器的分类 一般根据仪器的主要用途的不同,可将常见化学实验仪器分为下列8类: (一)计量类 用于量度质量、体积、温度、密度等的仪器。这类仪器中多为玻璃量器。主要有滴定管、移液管、量筒、量杯等。 (二)反应类 用于发生化学反应的仪器,也包括一部分可加热的仪器。这类仪器中多为玻璃或瓷质烧器。主要有试管、烧瓶、蒸发皿、坩埚等。(三)容器类 用于盛装或贮存固体、液体、气体等各种化学试剂的试剂瓶等。(四)分离类 用于进行过滤、分液、萃取、蒸发、灼烧、结晶、分馏等分离提纯操作的仪器。主要有漏斗、分液漏斗、蒸发皿、烧瓶、冷凝器、坩埚、烧杯等。 (五)固体夹持类 用于固定、夹持各种仪器的用品或仪器。主要有铁夹、铁圈、铁架台、漏斗架等。 (六)加热类 用于加热的用品或仪器。主要有试管、烧杯、烧瓶、蒸发皿、坩埚等。 (七)配套类 用于组装、连接仪器时所用的玻璃管、玻璃阀、橡胶管、橡胶塞等用品或仪器。 (八)其它类 不便归属上述各类的其它仪器或用品。 二、中学化学常见仪器的名称和使用 (一)计量仪器 1.量杯 量杯属量出式(符号Ex)量器,它用于量度从量器中排出液体的体积。排出液体的体积为该液体在量器内时从刻度值读取的体积数。 量杯有2种型式。面对分度表时,量杯倾液嘴向右,便于左手操作,称为左执式量杯。倾液嘴向左,则称为右执式量杯。250 mL以内的量杯均为左执式,500 mL以上者,则属于右执式。 2.温度计 温度计是用于测量温度的仪器。其种类很多,有数码式温度计,热敏温度计痔。而实验室中常用为玻璃液体温度。 温度计可根据用途和测量精度分为标准温度计和实用温度计2类。标准温度汁的精度高,它主要用于校正其它温度计。实用温度计是指所供实际测温用的温度计,主要有实验用温度计、工业温度计、气象温度计、医用温度计等。中学常用棒式工业温度汁。其中酒精温度计的量程为100℃,水银温度计用200℃和360℃2种量程规格。 使用注意事项 匿名通信的研究 1 匿名通信概述 (应用背景介绍) 在网络出现的最初几十年里,它主要用于在各大学的研究人员之间传送电子邮件,以及共同合作的职员间。在这种条件下,信息的安全性未能引起足够的注意。但是现在,Internet已经被越来越多地用于个人和商业通信中,如众多市民使用网络来处理银行事务、购物和纳税等重要的商业交易,信息安全逐渐成为一个潜在的巨大问题。对信息本身的保密已有相对成熟的保护信息的数据加密算法。但报文头部信息很难通过加密算法来隐藏,例如源地址和目的地址、报文长度等。 攻击者则可以利用协议所存在的这些漏洞发动窃听与流量分析攻击来获取通信双方的地址,进而推断出一些有价值的信息。侦听是指攻击者对通信链路进行非法窃听,获取重要信息。而流量分析是指攻击者可以对链路中信息的时间、速率、源及目的地等信息进行分析,从而窃取通信双方的地址以及相关重要信息。例如根据传输信包的控制信息部分以及信息特征等可见部分,推断出信息的来源、去向、数据量和一些隐含的意义。随着一些特殊部门如军事、国防、政府部门对网络安全性要求的提高,以及民用上对网络个人隐私要求的提高,迫切需要更安全的网络。匿名通信是指通过一定的方法将业务流中的通信关系加以隐藏,使窃听者无从直接获知或推知双方的通信关系或通信的一方。匿名通信的一个重要目的就是隐藏通信双方的身份或通信关系,从而实现网络用户的个人通信隐私及对涉密通信的更好的保护。目前,有关网络匿名通信技术的研究已逐渐引起网络安全研究人员的重视,成为网络安全研究领域的重要分支之一。 2 基本匿名通信机制 2.1 MIX种类和实现机制 1、Chaum的MIX系统模型 MIX的设想最早由David Chaum在1981年提出[c01],在他的无法跟踪电子邮件系统中提出了理想MIX应能完成的功能以及MIX系统的基本结构与实现。 MIX是指网络上的一些特殊的机器,它完成存储转发功能,其基本作用是改变信息的编码、顺序和长度,使得用户无法推知输出与输入之间的关系。即偷听者即使在链路上获得了MIX的输入数据包和输出数据包,也无法推知哪个是哪个的输出,从而无法推知数据流的来源,使通信关系得到隐藏。MIX的基本结构如图 -1所示。 商用无线AP FAT模式设置方法商用无线ap fat模式的设置第一步、操作准备 1、切换模式开关 在AP的壳体侧面上找到FAT/FIT模式开关,将模式开关切换到FAT档。 2、供电并连接 部分AP需采用802.3af/at标准PoE网线供电,请可以使用标准PoE供电交换机(PoE供电器)给AP供电,设置时建议勿接入网络,接线图如下。 注意。TL-AP451C、TL-AP301C使用DC(直流电源)或Passive PoE供电。将电脑连接到AP的网线口,使用电源适配器供电,如下图。 商用无线ap fat模式的设置第二步、登陆界面 1、设置操作电脑 请将操作电脑的本地连接设置静态IP,IP地址。192.168.1.10,子网掩码为255.255.255.0,无需设置网关、DNS。 2、输入管理地址 打开浏览器,清空地址栏并输入192.168.1.254,回车后页面会弹出登录框。 注意。如果管理地址有修改过,请使用修改后的地址登录。 3、输入登录信息 在登录框中,输入无线AP默认的用户名和密码,均为admin,点击登录,进入管理界面。 注意。如果有修改过登录的用户名和密码,请使用修改后 的用户名和密码登录。 商用无线ap fat模式的设置第三步、无线设置 1、进入无线设置 登录AP界面后,点击无线进行无线设置。 2、修改已有无线设置 如果无线服务中已经有无线信号,您可以点击设置图标进行修改无线设置(建议删除无用信号,加密有用信号)。如下图。 具体设置过程如下图。。 注意。类型设置为访客网络时,访客网络的主机不能与其他无线网络主机通信。启用无线网络内部隔离后,连接到同一个无线网络的主机之间不能相互通信。以上功能如果需要垮AP生效(多个AP之间),需要结合VLAN设置,详细可以参考相关文档。 3、增加新的无线信号 在无线服务中点击新增,可以添加新的无线信号。如下图。 4、保存设置 设置完成后,界面会提示您有未保存的配置,是否现在保存? 的提示,请务必点击右侧保存配置的按钮保存配置。 商用无线ap fat模式的设置第四步、地址修改 AP的默认IP地址为192.168.1.254 ,如果局域网有多个AP,为了便于管理,需要将管理地址设置为相同网段不冲突的地址。 如第一个AP的IP地址为192.168.1.254,第二个AP的IP 地址为192.168.1.253,第三个AP的IP地址为192.168.1.252...,依次类推。点击系统设置管理中可以设置管理IP地址,修改 H3C WLAN产品配置方法 本篇主要介绍在开通H3C两款WLAN(WA1208E,WA2220X)的时候需要配置的3个重要的参数,即: 1:信道的选择 2:SSID的配置 3:远程管理IP的填写方法 一:首先,先介绍针对WA1208E这款产品的配置方法。需要做的准备是在电脑上配置与WLAN相同网段的IP地址,以便通过电脑登陆WLAN产品进行配置,方法如下: 1:双击打开网上邻居,然后选择查看网络连接,可以看见本地连接,右键本地连接属性后, 如图所示: 2:在上图中选择Internet协议(TCP/IP),选择后如图所示: TCP/IP属性输入IP地址192.168.0.x (x 介于1和254之间,注意50不要用),子网掩码255.255.255.0 ,WA1208E默认管理地址为192.168.0.50 ,PC机ping通192.168.0.50即可. 3.打开PC机的开始栏运行菜单,输入telnet 192.168.0.50,输入正确的用户名和密码(WA1208E预设用户名admin,密码wa1208),进入了设备的配置界面。 4.(1)进入系统视图 (2)根据电信的要求,现场统一修改SSID成ChinaNet(注意大小写): [H3C]ssid ChinaNet [H3C-ssid-ChinaNet]bind domain system [H3C-ssid-ChinaNet]quit [H3C]interface Wireless-access 2/1 [H3C-Wireless-access2/1]undo bind ssid wa1208e //去除系统默认的SSID [H3C-Wireless-access2/1]bind ssid ChinaNet [H3C-Wireless-access2/1]quit (3)配置信道,注意:相邻的AP之间需要用不同的信道隔开。 例如:以11g为例,将工作信道改变到2号信道上 泰尔终端实验室(CTTL-terminals)成立于2014年元月1日,行政隶属于工业和信息化部电信研究院(CATR)。泰尔终端实验室是在电信研究院相关检验机构不断发展和融合的基础上诞生的,现在的实验室是由工业和信息化部电信研究院所属的电信传输研究所(RITT)、通信计量中心(TMC)通过业务重组和资源优化整合而重新成立的。泰尔终端实验室是集信息通信技术发展研究,信息通信产品标准、测试方法、通信计量标准、计量方法研究,以及国内外产品的测试、验证、技术评估、测试仪表计量以及通信软件的评估、验证为一体的高科技组织。实验室现有员工400余人,博士后1人,博士20人,具有硕士以上学位者超过百人。实验室面积超过5万平方米,仪器仪表设备资产在7亿元人民币。实验室定位以电子信息通信产业为主要领域,以市场需求为导向,向政府组织、行业组织、运营企业、产品设计制造业以及社会公众提供全方位技术支撑和服务,实验室的主要任务是电子信息通信科学新技术发展研究、相关标准研究与制定、技术可行性和标准符合性技术实验和测试。实验室建立了以ISO/IEC 17025 为标准的质量体系,并通过了中国合格评定国家认可委员会(CNAS)以及德国国家认可机构有限公司(DakkS)等国内外认证组织的认证。实验室现在已经获得PTCRB、GCF、CCF、CTIA、WIFI alliance、SIG、USB IF、NFC forum等行业组织的授权,也获得了Orange、Vodafone、中国移动、中国电信及中国联通等运营商的授权,同时也是CCC、FCC、CE等授权实验室。实验室专注从事终端产品及相关领域的检测与认证工作,其检测能力覆盖了各类电子信息通信终端、多媒体通信设备、电子产品及芯片、汽车电子,测试能力包括:一致性、电磁兼容、天线、生物电磁学、电气安全、信息安全、软件验证、短距离通信的相关接口、节能环保、有害物质检测等。实验室已成为中国面向国内外的综合性、规模化电子信息通信设备检验和试验的基地。 China telecommunication Technology Labs-Terminals(CTTL-terminals) was founded on Jan. 1st, 2014, under the supervision of China Academy of Telecommunication Research of MIIT (CATR). Based on the development and merging of related testing bodies of CATR, the present CTTL-terminals was reestablished by an amalgamation of the Research Institute of Telecommunication Transmission (RITT) and the Telecommunication and Metrology Center(TMC), by means of business reengineering as well as optimization and integration of resources. CTTL-terminals is a high-tech organization being engaged in research on the following fields: development of information and communication technology(ICT), standards and testing methods for information and communication products, telecommunication metrolog y standards and methods; as well as in missions of testing, verification, technology 化学实验教学中心功能 根据学校教务处和设备处等相关部门的指导性意见,配合学院发展的需要,原材料科学与化学工程学院的中心实验室更名为化学实验教学中心,并设立三个分实验室:基础化学实验室、专业化学实验室、理化检测中心,共同承担了教学、科研、地方服务的任务。各分实验室的主要功能分述如下: 基础化学实验室: 本分室主要承担校级公共平台(生命、环境、医学等专业)化学实验类课程、院内化学专业和应用化学专业的基础化学实验类课程的教学工作,包含大学化学、普通化学、无机及分析化学、无机化学、分析化学、有机化学、物理化学、仪器分析等实验课程。 其中,大学化学实验课程面向07年学校大类平台招生改革而设置,给自然科学大类的一年级学生开设。普通化学实验课程为工科类一年级学生开设。无机及分析化学实验、有机化学实验为生命类和医学类一年级学生开设。这四门实验课程所开设的实验项目以无机化学和分析化学相关的基础项目为主,包含少量的简易综合性和趣味性实验,以培养学生宽基础的知识体系和实验能力,并辅以激发学生的学习兴趣。 无机化学、分析化学、有机化学、物理化学的实验课程为学院内本科生的基础课程。同时承担基础实验教学、开放实验和学生毕业论文设计实验,其开出的实验项目分为基础性、综合性和设计性三个层次,部分包含选做实验,根据不同的教学情况进行选择。分述如下:无机化学实验是化学专业学生的第一门必修的、独立的基础实验课。它是以实验为手段来研究无机化学中的重要理论、典型元素及其化合物的变化规律,以及相应的仪器、装置、基本操作的一门课程。着力于培养学生具有宽广的基础知识和熟练的基本技能、能够适应未来社会发展需要的专业人才。教学内容着眼于为学生今后的学习发展奠定基础。学生在学习无机化学专业理论知识的同时,通过实验研究活动,学习和掌握无机化学专业的基本实验技术,研究元素的单质及其化合物的重要性质,熟悉重要无机化合物的制备方法;加深理解和掌握无机化学基本理论和基础知识;比较牢固地掌握化学实验的基本知识和操作技能;培养学生严谨的科学态度;培养学生准确观察化学反应现象,处理实验数据的能力,达到训练学生基本理论知识的综合应用能力;培养学生分离、分析与鉴别物质,合成、制备物质及将所学知识与生产实际结合起来的能力。 通过分析化学实验的学习,学生可以掌握定量化学分析及可见吸光光度分析和部分仪器分析实验的基本知识、基本操作和典型的分析方法;通过实验加深对有关理论的理解,并能灵活运用所学的理论知识指导实验设计与操作;确立“量”的概念、“相对误差”的概念和“有效数字”的概念;培养严谨的科学作风和良好的实验素养,激发实验兴趣和探索精神,提高分析问题和解决实际问题的能力。 有机化学实验是化学专业本科的一门基础实验课程。其主要目的是:通过有机化学实验验证、巩固和深入理解所学的有机化学理论知识;通过实验,使学生正确地掌握基础化学实验的基本操作方法和技能技巧,培养学生独立工作和独立思考的能力,养成严谨的科学态度和良好的科学思维方法。为后续课程的学习、为培养合格的化学教学工作者和化学化工技术人才打下扎实的基础。 通过物理化学实验课程的学习,使学生巩固物理化学理论课中所学习的基本概念、基本理论;掌握通用仪器的基本操作,掌握物理化学中常用的基本实验方法和实验技能,为学生今后做专业基础实验,专业实验和毕业论文打下坚实的基础。 无线AP配置教程 今天开始了工作以来的第一次上中夜班,在工作间抽了一点点时间来做此教程,希望能够增强自己的文字表述能力。 下面对Strix Access无线AP的配置过程做下详细讲解 一、前期准备工作 (1)每个AP在调试之前,应先检查其AP模块组合方式是否正确。(注:针对有外置天线接口的模块,应在不使用外置天线时将其天线控制档位打至INT.档,反之则将其打至EXT.档。)(2)在进行调试前应对每个无线AP进行通电检查,看其电源工作情况是否正常;并且统一进行一次初始化设置。(注:初始化设置方法:a.先用回形针插入电源接口附近的RESET孔中不放,再接通电源;b.当看到模块中的指示灯均同时出现快闪10后即可松开回形针,等待指示灯稳定不闪时AP初始化完成。) 二、Strix Access无线AP的配置方法与过程 (1)无线Strix Access配置过程分两种方式: 第一种为:DHCP服务动态分配IP地址配置法; 第二种为:手动设置IP地址配置法。 (2)下面先讲解DHCP服务器方式: A.具体操作流程拓朴图如下: B.在设置DHCP服务器时就先将其系统自带防火墙关闭,并设置其本地连接IP地址为:192.168.1.254;子网掩码为: 255.255.255.0;默认网关为:192.168.1.254;首选DNS地址为:192.168.1.254;备用DNS地址为:192.168.1.253;(注:本文中所使用的DHCP服务软件为:DHCPSRV 1.5版本;程序只包含三个文件:一个为DHCPSRV.INI程序配置文件,一个为README.TXT程序介绍文件,一个为DHCPSRV.EXE运行程序文件;)如下图所示: a.具体IP配置方法为: ①右击“桌面”上的“网上邻居”图标,选择“属性”快捷菜单项,打开“网络连接”窗口,如下和图二所示: 图一: 民勤县第三中学化学实验室简介 民勤三中现有化学实验室1个,其中仪器室1个,准备室一个,学生实验室2个。各室的水电到位,布局合理,有防火、防盗、换气设备,双人双锁管理,仪器设备按省级标准配置齐全。实验室完全达到两名学生一组进行分组实验的条件。 实验室设有演示台,供电到位,实验室的演示台和学生实验桌采用防酸碱阻燃面板,长、宽、高及材质符合要求。教师演示台有电源总控制设备,集中控制学生实验电源,配有触电保护器。化学实验室演示台和学生实验桌旁设置水槽,实验室内悬挂有名言警句。仪器室与实验室毗邻设置,配备满足仪器存放的仪器橱。对危险品和毒品有完整的管理和领用制度,配有准备台、工具箱(常用类)等。所有仪器按配备标准顺序上架、入柜。教学演示实验及学生分组实验能全部开出。此外,学校还经常及时增补教学实验材料及仪器设备,满足教师演示及学生分组实验的需求。所有实验室采光良好,灯管垂直黑板安装;保持自然通风。 实验室实物流水账、管理明细账记录规范;仪器存放、分类、编号、贴签入柜,摆放科学有序,存取方便;药品、仪器分室存放。 实验教学坚持做到期初有计划、期末有总结,各项制度、实验员工作职责都张贴在墙上,以便经常对照学习。实验的周计划,每学期初就公布上墙,做到合理安排,一周工作早知道。学生进入实验室必须遵守学生实验规则,实验结束要填好实验记录单。仪器损坏按有关制度赔偿。各室登记台帐,既有电子台账,又有纸质台账,做到帐、 物、卡相符,并及时做好新增仪器的登记和仪器的报损工作。实验室工作以教学为中心,以提高教学质量为目的,加强实验教学环节。保证紧密配合教学工作。服务教学一线,结合新课程要求,开足开齐演示实验、学生实验和探究性实验,演示实验开出率达100%,分组实验的开出率达85%以上。 华为AC+AP无线配置方法 组网示意图: 前提条件: 1)所有设备工作正常 2)依据上述组网建立测试环境 1)正确配置AC使AP发放SSID:“SSID-Temp1”、“SSID-Temp2”和“SSID-Temp3”,且对应业务vlan为:vlan-1,vlan-2,vlan-3; 配置Switch [Switch]vlan batch 100 101 102 103 [Switch]interface GigabitEthernet0/0/1 [Switch-GigabitEthernet0/0/1]description Link-to-AP [Switch-GigabitEthernet0/0/1]port link-type trunk [Switch-GigabitEthernet0/0/1]port trunk pvid vlan 100 [Switch-GigabitEthernet0/0/1]port trunk allow-pass vlan 100 101 102 103 [Switch-GigabitEthernet0/0/1]quit [Switch]interface GigabitEthernet0/0/2 [Switch-GigabitEthernet0/0/2]description Link-to-AC [Switch-GigabitEthernet0/0/2]port link-type trunk [Switch-GigabitEthernet0/0/2]port trunk allow-pass vlan 100 101 102 103 [Switch-GigabitEthernet0/0/2]quit 配置AC 1.配置接口和VLAN信息,AC为DHCP Server,为AP和client分配地址 [AC]vlan batch 100 101 102 103 [AC]interface GigabitEthernet0/0/1 [AC-GigabitEthernet0/0/1]description Link-to-SW [AC-GigabitEthernet0/0/1]port link-type trunk [AC-GigabitEthernet0/0/1]port trunk allow-pass vlan 100 101 102 103 [AC-GigabitEthernet0/0/1]quit TYE—2000KN压力试验机操作规程 一.一般规定: (1)本机由本室指定的试验人员进行操作,非本室人员未经许可,不得随意摆弄,以防事故发生。 (2)试验操作人员在试验前必须对本机进行检查,看贮油是否足够,油管接头是否松动,如油不足应加油,松动的油管接头应拧紧。(3)每次试验完毕,应及时关闭油泵,切断电源后应清理试台上的渣物,并盖好防尘罩。 二.操作方法: (1)首次对试样的最大荷载,应有所估计,然后根据所估计的最大荷载,合理选择并悬挂应力弹簧,校正指针回零。 (2)将试件放在下压板中心,顺时针旋动上压板手轮直至上压板接触试件。 (3)接通电源开动油泵,关闭加油阀,打开送油阀,按要求速度加荷,至试件破坏后,立即徐徐松开回油阀,关闭送油阀,读取从动指针所指荷载值并记录之(大弹簧读取外圈值,小簧读取内圈值)。(4)反时针转动上压板手轮,取出已破坏的试件。 ZBSX-92型振筛机操作规程 一. 一般规定: 使用人员在使用前,仔细阅读使用说明书,了解本机的有关事项,方可使用。 二. 操作步骤: 2.1接通电源。 2.2先将装有试样的套筛固定在振筛机上; 2.3将旋扭转至要求定时位,即可开始工作,若中途停机,应切断电源开关,让定时片中自行停止位置,不可强行扭回。 2.4将筛好的试样分别称重。 三. 注意事项: 1.1开机前,加足润滑油至螺孔面。 3.2转动旋扭时,不应过快,过猛,以免损坏零件。 3.3每隔6个月更换机油一次,使用时注意避免盖板上脦根导杆变形。 101型电热恒温干燥箱 一. 一般规定 使用人员在使用前,仔细阅读使用说明书,了解本机的有关事项,方可使用。 二. 操作方法 1. 打开电源开关。 2. 将温控仪设定所需温度,温控仪绿灯灭,此时箱内开始升温,同时打开鼓风机开关,使鼓风机工作,并注意鼓风机运转状况是否正常。 3. 恒温时,可关闭辅助加热开关,只留一组工作,以免功率过大,影响恒温波动度。 三. 注意事项 1. 本箱装置时必须用导线连接通地。 2. 本箱非防爆型,故带腐蚀性及易燃性物品禁止放入箱内干燥, cisco无线AP配置方法 无线接入点(AP)在无线网络中充当用户的中心通信点,可以连接有线和无线网络。使用Web浏览器和命令行接口(CLI)都可以完成配置。一般来说,AP 可以配置两个接口以太网接口和无线端口。 a:配置方式 1:控制台端口(需要翻转线) 2:Telnet(远程登录) 3:W 无线接入点(AP)在无线网络中充当用户的中心通信点,可以连接有线和无线网络。使用Web浏览器和命令行接口(CLI)都可以完成配置。 一般来说,AP可以配置两个接口以太网接口和无线端口。 a:配置方式 1:控制台端口(需要翻转线) 2:Telnet(远程登录) 3:Web浏览器(这是最简单的配置方法,只需要在图形界面(GUI)上就可以操作) b:配置过程 1:图形界面(GUI)根据IOS的不同,一般有两种基本的GUI界面。GUI配置简单这里就不说明了。 2:通过Telnet在MS-Dos中输入 Telnet ip-address 3:使用CLI(命令行)配置是本文的重点。下面我将详细给大家说明。 首先连接物理线缆,打开超级终端(在系统“开始”菜单中的“通讯”中)。 超级终端的配置参数如下: * 每秒比特数:9600; *数据位:8; *奇偶效验:无; *停止位:1; *流控:Xon/Xoff或者无; 进入命令行的界面如下: User Access Verification Username: Cisco Password: Ap>show version //可以查看当前版本信息 一:设置系统名 Ap>enable Ap#config t Ap(config)#hostname myap //设置系统名,默认是ap 一、常见仪器得分类 一般根据仪器得主要用途得不同,可将常见化学实验仪器分为下列8类: (一)计量类 用于量度质量、体积、温度、密度等得仪器。这类仪器中多为玻璃量器。主要有滴定管、移液管、量筒、量杯等. (二)反应类 用于发生化学反应得仪器,也包括一部分可加热得仪器。这类仪器中多为玻璃或瓷质烧器.主要有试管、烧瓶、蒸发皿、坩埚等。(三)容器类 用于盛装或贮存固体、液体、气体等各种化学试剂得试剂瓶等。 (四)分离类 用于进行过滤、分液、萃取、蒸发、灼烧、结晶、分馏等分离提纯操作得仪器.主要有漏斗、分液漏斗、蒸发皿、烧瓶、冷凝器、坩埚、烧杯等。 (五)固体夹持类 用于固定、夹持各种仪器得用品或仪器。主要有铁夹、铁圈、铁架台、漏斗架等. (六)加热类 用于加热得用品或仪器。主要有试管、烧杯、烧瓶、蒸发皿、坩埚等。 (七)配套类 用于组装、连接仪器时所用得玻璃管、玻璃阀、橡胶管、橡胶塞等用品或仪器。 (八)其它类 不便归属上述各类得其它仪器或用品。 二、中学化学常见仪器得名称与使用 (一)计量仪器 1。量杯 量杯属量出式(符号Ex)量器,它用于量度从量器中排出液体得体积.排出液体得体积为该液体在量器内时从刻度值读取得体积数。量杯有2种型式。面对分度表时,量杯倾液嘴向右,便于左手操作,称为左执式量杯。倾液嘴向左,则称为右执式量杯。250 mL以内得量杯均为左执式,500mL以上者,则属于右执式。 2。温度计 温度计就是用于测量温度得仪器。其种类很多,有数码式温度计,热敏温度计痔。而实验室中常用为玻璃液体温度。 温度计可根据用途与测量精度分为标准温度计与实用温度计2类.标准温度汁得精度高,它主要用于校正其它温度计。实用温度计就是指所供实际测温用得温度计,主要有实验用温度计、工业温度计、气象温度计、医用温度计等。中学常用棒式工业温度汁。其中酒精温度计得量程为100℃,水银温度计用200℃与360℃2种量程规格。 使用注意事项 一.无线局域网的特点及应用环境 (1) 二.无线网络标准 (2) 四.无线局域网的架构 (3) 五.解决问题 (4) 六. 安全建议 (5) 七.施工细节 (6) 一.无线局域网的特点及应用环境 1. 无线局域网的特点 无线局域网的出现使有线网络所遇到的问题迎刃而解,它可以使用户任意对有线网络进行扩展和延伸。只是在有线网络的基础上通过无线接入器、无线网桥、无线网卡等无线设备使无线通信得以实现。在不进行传统的布线的同时,提供有线局域网的所有功能,无线局域网内可以使用无线AC来管理大量的AP,当AP的增加时,就可接入无线AC而形成一个大型的集中管理系。 2.无线局域网具有传统有线网络无法比拟的特点: 灵活性,不受线缆的限制,可以随意增加和配置工作站; 低成本,无线局域网不再需要大量的工程布线,同时节省了线路维护的费用; 移动性,不受时间、空间的限制,用户可在网络中漫游; 易安装,对于有线网络来说,无线局域网的组建、配置和维护更为容易。而且,通信范围不受环境条件的限制,网络传输覆盖范围大大的拓展,室外可以传输几十公里、室内可以传输数十、几百米。在网络数据传输方面也有与有线网络等效的安全加密措施。 3.所有这些无线局域网的特点使其可以广泛使用在以下的领域: 移动办公的环境:大型企业、医院等移动工作的人员应用的环境; 难以布线的环境:历史建筑、校园、工厂车间、城市建筑群、大型的仓库等不能布线或者难于布线的环境; 频繁变化的环境:活动的办公室、零售商店、售票点、医院、以及野外勘测、试验、军事、公安和银行金融等,以及流动办公、网络结构经常变化或者临时组建的局域网; 特殊项目的局域网:航空公司、机场、货运公司、码头、展览和交易会等; 小型网络用户:办公室、家庭办公室(SOHO)用户; 二.无线网络标准 1.IEEE 80 2.11标准 IEEE 802.11标准是无线局域网的标准之一,是无线领域目前最为成熟的网络标准。该标准定义了OSI七层模型中的物理层(PHY)和媒体访问控制层(MAC)的协议规范,其中MAC层是重点。它的制定使得各厂商之间的无线产品在物理层上实现互操作,而逻辑链路层(LLC)是一致的,即MAC层以下对网络应用是透明的。在MAC层以下,802.11规定了三种发送及接收技术:扩频(Spread Spectrum)技术;红外(Infared)技术;窄带(NarrowBand)技术。扩频技术又分为直接序列(Direct Sequence,DS)扩频技术和跳频(Frequency Hopping,FH)扩频技术。 2.IEEE 802.11b 标准 3.IEEE802.11a标准 4.IEEE802.11g标准 5.IEEE802.11n标准 三.无线局域网设计原则 1根据校园的实际情况,考虑用户需求、覆盖范围、用户密度、建筑结构、业务构成等各方面的需求,校园无线局域网建设的主要是作为校园有线网络的补充, 无线充电技术 无线充电技术(Wireless charging technology;Wireless charge technology )。无线充电技术,源于无线电力输送技术。无线充电,又称作感应充电、非接触式感应充电,是利用近场感应,也就是电感耦合,由供电设备(充电器)将能量传送至用电的装置,该装置使用接收到的能量对电池充电,并同时供其本身运作之用。由于充电器与用电装置之间以电感耦合传送能量,两者之间不用电线连接,因此充电器及用电的装置都可以做到无导电接点外露。[1] 概述 麻省理工学院的研究团队在2007年6月7日美国《科学》杂志的网站上发表了他们的研究成果。研究小组把共振运用到电磁波的传输上而成功“抓住”了电磁波。他们利用铜制线圈作为电磁共振器,一团线圈附在传送电力方,另一团在接受电力方。当传送方送出某特定频率的电磁波后,经过电磁场扩散到接受方,电力就实现了无线传导。这项被他们称为“无线电力”的技术经过多次试验,已经能成功为一个两米外的60瓦灯泡供电。这项技术的最远输电距离还只能达到2.7米, 但研究者相信,电源已经可以在这范围内为电池充电。而且只需要安装一个电源,就可以为整个屋里的电器供电。 共振原理 麻省理工学院的科研组不是第一个提出无线能量转换的组织。科学家早在19世纪就发现了电磁转换现象,从理论上说,电力可转化为通过无形的介质传播的电磁波,实现电力的无线输送。但是电磁波向四面八方辐射,能量大量散失,因此“无线输电”的研究始终进展不大,19世纪的物理学家和工程师尼古拉·特斯拉进行了远程无线能量转换系统实验,但是当他的财力用尽后,这项最有野心的尝试(29米高的瓦登克莱弗塔)宣告失败。其他尝试包括激光等定向能量转换机制。然而,它们与麻省理工学院的工作不同,这些都需要连续的可视线路,这对住宅周围的电力设施不好。 无线充电技术给两个手机无线充电[2] 研究组成员,助理教授马林·索亚克教授和他的科研组正在改进这个设备。“这是一项还未得到发展的系统,它证明能量转换行得通。但 常见化学实验仪器的名称及功能 实验仪器是进行化学实验的重要工具。“工欲善其事,必先利其器”,实验工具的齐备与否,直接影响到实验的成功与失败。根据不同的实验目的,应选择相应的实验方法,用不同的实验仪器才能进行实验。而实验仪器的构造和性能又决定了它特有的操作方法和不同的适用范围。所以必须对化学仪器的有关知识及功能有一个完整的了解,才能掌握它、正确地使用它,迸而在熟练基础上达到得心应手;完成好各种实验。 这里向大家介绍常见化学仪器的分类及各种仪器的名称、性能、规格和使用注意事项。 一、常见仪器的分类 一般根据仪器的主要用途的不同,可将常见化学实验仪器分为下列8类: (一)计量类 用于量度质量、体积、温度、密度等的仪器。这类仪器中多为玻璃量器。主要有滴定管、移液管、量筒、量杯等。 (二)反应类 用于发生化学反应的仪器,也包括一部分可加热的仪器。这类仪器中多为玻璃或瓷质烧器。主要有试管、烧瓶、蒸发皿、坩埚等。 (三)容器类 用于盛装或贮存固体、液体、气体等各种化学试剂的试剂瓶等。 (四)分离类 用于进行过滤、分液、萃取、蒸发、灼烧、结晶、分馏等分离提纯操作的仪器。主要有漏斗、分液漏斗、蒸发皿、烧瓶、冷凝器、坩埚、烧杯等。 (五)固体夹持类 用于固定、夹持各种仪器的用品或仪器。主要有铁夹、铁圈、铁架台、漏斗架等。 (六)加热类 用于加热的用品或仪器。主要有试管、烧杯、烧瓶、蒸发皿、坩埚等。 (七)配套类 用于组装、连接仪器时所用的玻璃管、玻璃阀、橡胶管、橡胶塞等用品或仪器。 (八)其它类 不便归属上述各类的其它仪器或用品。 二、中学化学常见仪器的名称和使用 (一)计量仪器 1.量杯 量杯属量出式(符号Ex)量器,它用于量度从量器中排出液体的体积。排出液体的体积为该液体在量器内时从刻度值读取的体积数。 量杯有2种型式。面对分度表时,量杯倾液嘴向右,便于左手操作,称为左执式量杯。倾液嘴向左,则称为右执式量杯。250 mL以内的量杯均为左执式,500 mL以上者,则属于右执式。 2.温度计fast ap设置教程.doc
实验一 常用仪器的使用
检测机构一览表
化学实验室常见仪器的名称及功能
匿名通信的研究
商用无线APFAT模式设置方法
华三_AP配置方法
中国泰尔实验室简介
化学实验室功能简介
无线AP的配置教程
化学实验室实验室简介
华为AC+AP无线的配置方法
实验仪器操作流程
CISCO 无线AP配置方法
化学实验室常见仪器的名称及功能
无线AC+AP方案
无线充电技术简介
常见化学实验室仪器的名称及功能1