Using thermally coupled reactive distillation columns in biodiesel production
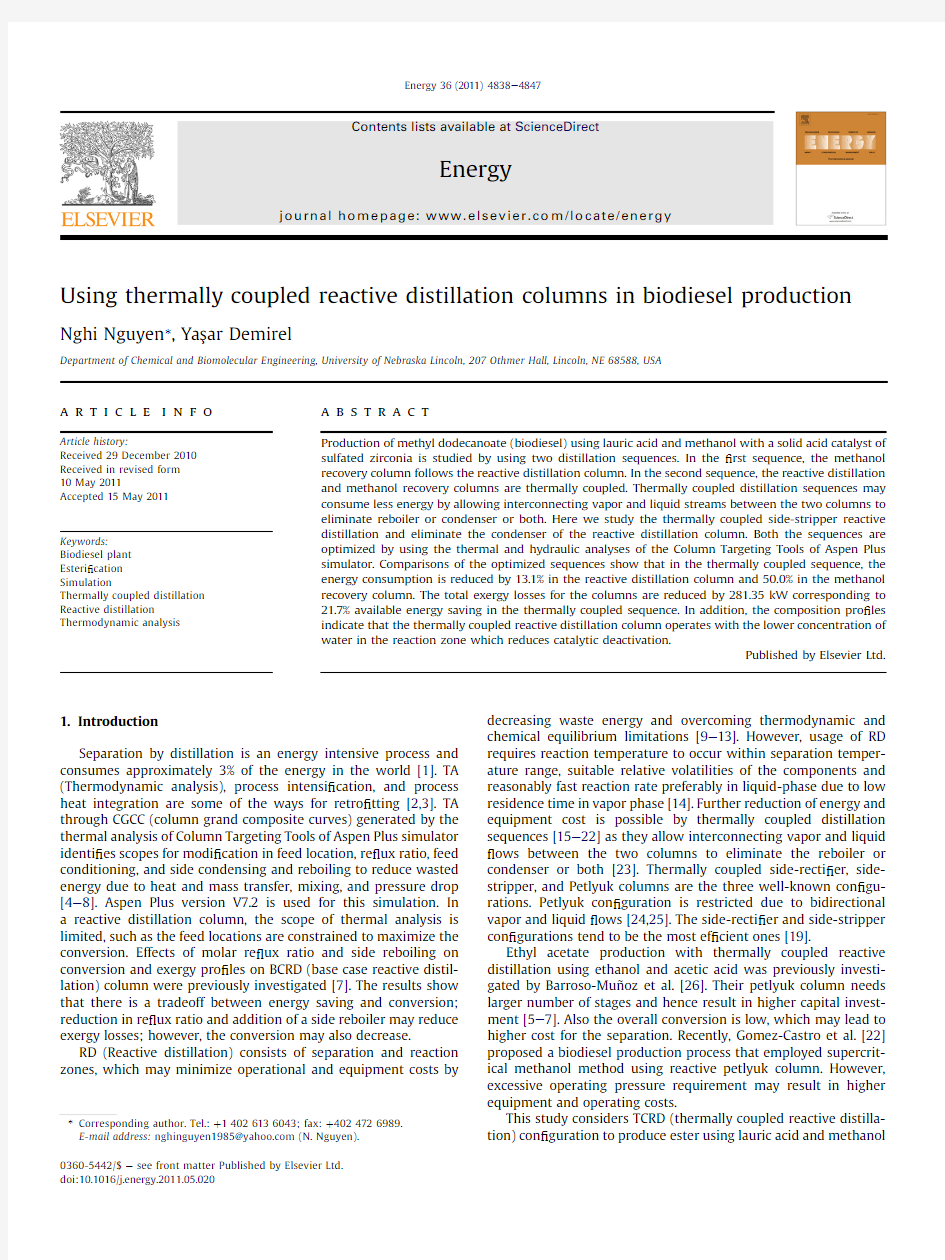

Using thermally coupled reactive distillation columns in biodiesel production
Nghi Nguyen *,Yas ?ar Demirel
Department of Chemical and Biomolecular Engineering,University of Nebraska Lincoln,207Othmer Hall,Lincoln,NE 68588,USA
a r t i c l e i n f o
Article history:
Received 29December 2010Received in revised form 10May 2011
Accepted 15May 2011
Keywords:
Biodiesel plant Esteri ?cation Simulation
Thermally coupled distillation Reactive distillation
Thermodynamic analysis
a b s t r a c t
Production of methyl dodecanoate (biodiesel)using lauric acid and methanol with a solid acid catalyst of sulfated zirconia is studied by using two distillation sequences.In the ?rst sequence,the methanol recovery column follows the reactive distillation column.In the second sequence,the reactive distillation and methanol recovery columns are thermally coupled.Thermally coupled distillation sequences may consume less energy by allowing interconnecting vapor and liquid streams between the two columns to eliminate reboiler or condenser or both.Here we study the thermally coupled side-stripper reactive distillation and eliminate the condenser of the reactive distillation column.Both the sequences are optimized by using the thermal and hydraulic analyses of the Column Targeting Tools of Aspen Plus https://www.360docs.net/doc/745093111.html,parisons of the optimized sequences show that in the thermally coupled sequence,the energy consumption is reduced by 13.1%in the reactive distillation column and 50.0%in the methanol recovery column.The total exergy losses for the columns are reduced by 281.35kW corresponding to 21.7%available energy saving in the thermally coupled sequence.In addition,the composition pro ?les indicate that the thermally coupled reactive distillation column operates with the lower concentration of water in the reaction zone which reduces catalytic deactivation.
Published by Elsevier Ltd.
1.Introduction
Separation by distillation is an energy intensive process and consumes approximately 3%of the energy in the world [1].TA (Thermodynamic analysis),process intensi ?cation,and process heat integration are some of the ways for retro ?tting [2,3].TA through CGCC (column grand composite curves)generated by the thermal analysis of Column Targeting Tools of Aspen Plus simulator identi ?es scopes for modi ?cation in feed location,re ?ux ratio,feed conditioning,and side condensing and reboiling to reduce wasted energy due to heat and mass transfer,mixing,and pressure drop [4e 8].Aspen Plus version V7.2is used for this simulation.In a reactive distillation column,the scope of thermal analysis is limited,such as the feed locations are constrained to maximize the conversion.Effects of molar re ?ux ratio and side reboiling on conversion and exergy pro ?les on BCRD (base case reactive distil-lation)column were previously investigated [7].The results show that there is a tradeoff between energy saving and conversion;reduction in re ?ux ratio and addition of a side reboiler may reduce exergy losses;however,the conversion may also decrease.
RD (Reactive distillation)consists of separation and reaction zones,which may minimize operational and equipment costs by
decreasing waste energy and overcoming thermodynamic and chemical equilibrium limitations [9e 13].However,usage of RD requires reaction temperature to occur within separation temper-ature range,suitable relative volatilities of the components and reasonably fast reaction rate preferably in liquid-phase due to low residence time in vapor phase [14].Further reduction of energy and equipment cost is possible by thermally coupled distillation sequences [15e 22]as they allow interconnecting vapor and liquid ?ows between the two columns to eliminate the reboiler or condenser or both [23].Thermally coupled side-recti ?er,side-stripper,and Petlyuk columns are the three well-known con ?gu-rations.Petlyuk con ?guration is restricted due to bidirectional vapor and liquid ?ows [24,25].The side-recti ?er and side-stripper con ?gurations tend to be the most ef ?cient ones [19].
Ethyl acetate production with thermally coupled reactive distillation using ethanol and acetic acid was previously investi-gated by Barroso-Mu?oz et al.[26].Their petlyuk column needs larger number of stages and hence result in higher capital invest-ment [5e 7].Also the overall conversion is low,which may lead to higher cost for the separation.Recently,Gomez-Castro et al.[22]proposed a biodiesel production process that employed supercrit-ical methanol method using reactive petlyuk column.However,excessive operating pressure requirement may result in higher equipment and operating costs.
This study considers TCRD (thermally coupled reactive distilla-tion)con ?guration to produce ester using lauric acid and methanol
*Corresponding author.Tel.:t14026136043;fax:t4024726989.E-mail address:nghinguyen1985@https://www.360docs.net/doc/745093111.html, (N.
Nguyen).Contents lists available at ScienceDirect
Energy
journal ho me page:www.elsevier.co m/lo
cate/energy
0360-5442/$e see front matter Published by Elsevier Ltd.doi:10.1016/j.energy.2011.05.020
Energy 36(2011)4838e 4847
with a solid acid catalyst of sulfated zirconia(SO4à2/ZrO2)[27e29]. In this proposed biodiesel plant,TCSRD(thermally coupled side-stripper reactive distillation)con?guration has been considered, since using the reactive distillation column as a side-recti?er may cause lower reaction rate because the reaction zone temperature must be within the separation temperature of methanol and water.Also,using the methanol recovery column as a recti?er may disrupt the reaction zone with the side feed and withdraw [10e12,15e19].2.TA(thermodynamic analysis)
TA determines the net enthalpy de?cits and exergy losses due to irreversibility at each stage of a column to identify the scope and extent of retro?ts for improvements by reducing the irreversibility and/or distributing them evenly[30e33].The Column Targeting Tools of Aspen Plus performs thermal analysis and hydraulic anal-yses.Thermal analysis produces the CGCC(column grand composite curves)and the exergy loss pro?les for rigorous column calculations
Fig.1.Process?ow diagrams for biodiesel plant:(a)base case design;(b)thermally coupled design.
N.Nguyen,Y.Demirel/Energy36(2011)4838e48474839
based on the PNMTC (Practical Near-Minimum Thermodynamic Condition).These pro ?les represent the cumulative heating and cooling requirements for the column to operate at PNMTC.This approximation takes into account the inef ?ciencies introduced through pressure drops,mixing,and heat and mass transfer.
2.1.CGCC (column grand composite curve)
The CGCC displays the net enthalpies for the actual and ideal operations at each stage,and theoretical minimum cooling and heating requirements in the temperature range of separation.The CGCCs are available as stage-enthalpy (Stage-H)and T e H (tem-perature e enthalpy)pro ?les and help in identifying the following scopes:(i)feed location (appropriate placement),(ii)re ?ux ratio (re ?ux ratio vs.number of stages),(iii)feed conditioning (heating or cooling),and (iv)side condensing and reboiling.The area between the actual and the ideal operations in a CGCC should be small.A sharp enthalpy change occurring on the condenser side indicates that a feed has been introduced too high up in the column and should be moved down.Similarly,a feed introduced too low in the column will cause a sharp enthalpy change on the reboiler side and should be moved up.Appropriate feed placement removes the distortions on the CGCC and may reduce the condenser or reboiler duty.Re ?ux ratio reduction lowers the condenser and reboiler duties and decreases operating costs.However,it will increase the number of stages and increase capital costs to preserve the https://www.360docs.net/doc/745093111.html,er must carefully analyze to determine whether savings in operating costs compensate higher capital costs.Feed preheating or cooling can reduce thermal loss on the feed https://www.360docs.net/doc/745093111.html,ing heat sources available in the plant are desirable and side condensing and side reboiling provides the column with a cheaper cold or hot utility [34,35].
Using the equilibrium compositions of light (L)and heavy (H)key components minimum vapor and liquid ?ow rates leaving the same stage with the same temperatures can be estimated from the following mass balances [4,5]
_V min ?1y *L
_D L t_L min x *L
(1)
_L min
?1x *H
_V L y *
H à_D H (2)
The enthalpies for the minimum vapor and liquid ?ows are obtained from the molar ?ow ratios
H V min ?
H *V
_V min
_V
* (3)
H L min ?H *
L
_L min _L
* (4)
where _V *and _L *
are the molar ?ows of equilibrium,and H *V and H *L
are enthalpies of equilibrium vapor and liquid streams leaving the same stage,respectively.From the enthalpy balances at each stage,the net enthalpy de ?cits are calculated by
H def ?H L min àH V min tH D ebefore the feed stage T(5)
H def ?H L min àH V min tH D àH feed eafterthefeedstage T(6)
After adding the individual stage-enthalpy de ?cits to the condenser duty,the enthalpy values are cascaded,and plotted in
T a b l e 1S t r e a m s p r o p e r t i e s o f t h e b a s e c a s e d e s i g n .
L A U R I C
M E T H
S 3B
S 2A
S 1
S 2B
E S T E R A
E S T E R B
E S T E R C
E S T E R D W A T M E T
S 3A
W A T A
W A T B
M a s s F l o w (k g /h r )20032.14
3364.433029.986394.4120032.146394.4121560.9721560.9721560.9721560.974865.573029.981835.591835.59T e m p e r a t u r e ( C )
25.0025.0065.0744.54100.00110.00311.29288.12234.6825.00124.1264.3697.5925.00P r e s s u r e (b a r )5.56.06.06.05.56.06.06.06.01.05.51.01.01.0V a p o r F r a c t i o n 00000000000000E n t h a l p y (k c a l /m o l )à176.42à56.98à56.07à56.55à168.25à54.69à124.13à127.72à135.57à160.12à60.57à56.09à66.83à68.13E n t r o p y (c a l /m o l -K )à324.48
à57.53
à54.14
à55.85
à300.06
à50.88à241.91à248.01
à262.48
à323.59
à40.80à54.18
à35.14
à38.99M a s s F l o w (k g /h r )W a t e r 0.00E t000.00E t001.80E t011.80E t010.00E t001.80E t011.33E t001.33E t001.33E t001.33E t001.82E t031.80E t011.80E t031.80E t03M e t h a n o l 0.00E t003.36E t033.01E t036.38E t030.00E t006.38E t031.26E t021.26E t021.26E t021.26E t023.05E t033.01E t033.47E t013.47E t01L a u r i c A c i d 2.00E t040.00E t004.07E -364.07E -362.00E t044.07E -362.64E t002.64E t002.64E t002.64E t008.98E -024.07E -368.98E -028.98E -02M e t e s t e r 0.00E t00
0.00E t001.48E -171.48E -170.00E t001.48E -172.14E t042.14E t042.14E t042.14E t048.48E -011.48E -178.48E -018.48E -01
M a s s F r a c t i o n W a t e r 0.00000.00000.00590.00280.0000
0.0028
0.00010.00010.00010.00010.37360.00590.98060.9806M e t h a n o l 0.00001.00000.99410.99720.00000.99720.00580.00580.00580.00580.62620.99410.01890.0189L a u r i c A c i d 1.00000.00000.00000.00001.00000.00000.00010.00010.00010.00010.00000.00000.00000.0000M e t e s t e r
0.00000.0000
0.0000
0.0000
0.0000
0.0000
0.99400.99400.99400.99400.00020.0000
0.0005
0.0005
N.Nguyen,Y.Demirel /Energy 36(2011)4838e 4847
4840
the CGCC.This is called the top-down calculation procedure,which will be the same with the bottom-up calculations for a stage without any feed.At the feed stage,mass and energy balances differ from a stage without feed.For the two procedures to yield similar results,the enthalpy de ?cit at the feed stage becomes [4,5]
_H def ;F ?_Q C t_D
H D tH L
x D ày *F
y *F àx *F
àH V
x D àx *F
y *F àx *F
!
(7)
The values of y *F and x *F may be obtained from an adiabatic ?ash
for a single phase feed,or from the constant relative volatility estimated with the converged compositions at the feed stage and feed quality.This procedure can be reformulated for multiple feeds and side products as well as for different choices of the key components.For nonideal multicomponent systems pinch may exist in rectifying and stripping sections.
A horizontal distance between the CGCC pinch point and the vertical axis represents the excessive use of heat,and therefore the scope for reduction in re ?ux ratio [36].For smaller re ?ux ratios,the CGCC will move toward the vertical axis,and hence reduce the reboiler and condenser duties,which may be estimated by
_Q R à_Q R ;min ?_Q C à_Q C ;min ?_D l
R à
x D ày *F
y *F *F
!
(8)
where l is the heat of vaporization and R is the re ?ux ratio [4,5].The horizontal distance of the CGCC from the temperature axis,however,determines the targets for installing a side reboiler or side condenser at suitable temperatures [30].For example,a sharp change on the reboiler side may be due to subcooled feed,and a feed preheater with a heat duty depending on the change can be installed [37].On the other hand,a sharp change in the enthalpy represents inappropriate feed conditioning,such as feed quality or temperature.Feed conditioning is usually preferred to side condensing or reboiling,since the side heat exchangers are effective at suitable temperature levels or stages only.2.2.Exergy loss pro ?les
Exergy (E x )is the accessible work potential and de ?nes the maximum amount of work that may be performed theoretically by bringing a resource into equilibrium with its surrounding through a reversible process.Speci ?c exergy is de ?ned by
e x ?D h àT o D s ?eh àh o TàT o es às o T
(9)
where h is the molar enthalpy,s is the molar entropy,and T o is the reference state temperature.The reference temperature and pressure states are 298.15K and 1atm,respectively.Aspen Plus uses ideal gas heat of formation and ideal gas Gibbs free energy of formation to compute enthalpies,entropies,and Gibbs free energies;these formation energies may be set equal to zero if there is no reaction occurred [38].Exergy is a function of both the physical properties of a system and its environment.Exergy Loss pro ?les are available as stage-exergy loss and temperature-exergy loss pro ?les,and measure the irreversibility in the column due to momentum loss (pressure driving force),thermal loss (tempera-ture driving force/mixing),and chemical potential loss (mass transfer driving force/mixing).These pro ?les can be used to examine the degradation of accessible work for the column.Higher value of exergy loss means more thermodynamic imper-fection,consequently,higher energy consumption to achieve a desirable separation.For a steady state system,the exergy balance is not conserved [39]
T a b l e 2S t r e a m s p r o p e r t i e s o f t h e t h e r m a l l y c o u p l e d d e s i g n .
L A U R I C
M E T H
S 3B
S 2A
S 1
S 2B
E S T E R A
E S T E R B
E S T E R C
E S T E R D
W A T M E T
S 4B
S 4A
S 3A
W A T A
W A T B
M a s s F l o w (k g /h r )20032.143364.433029.986394.4120032.146394.4121526.7121526.7121526.7121526.715036.00136.19136.193029.981869.831869.83T e m p e r a t u r e ( C )
25.0025.0065.5244.76100.00110.00274.96250.36194.2125.00155.5097.7391.4664.3696.3425.00P r e s s u r e (b a r )9.09.59.59.59.09.59.59.59.51.09.09.01.01.01.01.0V a p o r F r a c t i o n 0000000000100000E n t h a l p y (k c a l /m o l )à176.42à56.98à56.06à56.55à168.25à54.69à129.49à133.06à140.92à159.90à51.97à66.47à66.63à56.09à67.01à68.30E n t r o p y (c a l /m o l -K )à324.48
à57.53à54.10
à55.84
à300.06à50.88à251.07
à257.62
à273.34
à323.10
à19.83
à36.55
à36.95à54.18
à35.67
à39.51
M a s s F l o w (k g /h r )W a t e r 0.00E t000.00E t001.80E t011.80E t010.00E t001.80E t011.79E -011.79E -011.79E -01
1.79E -011.93E t031.18E t021.18E t021.80E t01
1.80E t031.80E t03M e t h a n o l 0.00E t003.36E t033.01E t036.38E t030.00E t006.38E t031.34E t021.34E t021.34E t021.34E t023.06E t031.40E t011.40E t013.01E t033.41E t013.41E t01L a u r i c A c i d
2.00E t040.00E t007.56E -327.56E -322.00E t047.56E -32
3.79E t013.79E t013.79E t013.79E t019.99E t003.49E -013.49E -017.56E -329.64E t009.64E t00M e t e s t e r 0.00E t00
0.00E t006.53E -156.53E -15
0.00E t006.53E -15
2.14E t042.14E t042.14E t042.14E t04
3.30E t01
3.97E t00
3.97E t006.53E -15
2.90E t01
2.90E t01
M a s s F r a c t i o n W a t e r 0.00000.00000.00590.00280.00000.00280.00000.00000.00000.00000.38380.86550.86550.00590.96110.9611M e t h a n o l 0.00001.00000.99410.99720.00000.99720.00620.00620.00620.00620.60760.10280.10280.99410.01820.0182L a u r i c A c i d 1.00000.00000.00000.00001.00000.00000.00180.00180.00180.00180.00200.00260.00260.00000.00520.0052M e t e s t e r
0.00000.0000
0.0000
0.00000.0000
0.0000
0.99200.99200.9920
0.9920
0.0066
0.0292
0.0292
0.0000
0.0155
0.0155
N.Nguyen,Y.Demirel /Energy 36(2011)4838e 48474841
_E x ;loss
?
X in
_ne x t_Q 1àT o
s t_W s à
X out
_ne x t_Q 1àT o
T s t_W s (10)
where _W s and _E x ;loss are the shaft work and exergy losses,
respectively.
For distillation columns,the minimum exergy ?ow rate required
for separation is directly proportional to the differences between the exergy of the product and feed streams
_E x ;min ?
X
out
_ne
x àX
in
_ne
x (11)
Table 3
Comparison of operating conditions and con ?gurations of reactive distillation column RD101and distillation column T101of base case and thermally coupled designs.
Base Case Design
Thermally Coupled Design Condition &Con ?gurations RD101T101Total
RD101T101Total
Number of stages 30123012S1feed stage 3__3__S2B feed stage 29__29__S4B feed stage
____1__WATMET feed stage __9__10S1temperature, C 100__100__S2B temperature, C 110__110__Molar re ?ux ratio
0.1 1.55__ 2.02S4B ?ow rate,kmol/hr ____7__Reaction stages
3e 29__3e 29__Distillate rate,kmol/hr 1969520395Column diameter,m 1.04 1.100.86 1.25Liquid hold up,cm 30.043__
0.043__
Condenser duty,kW
à2168.38à2375.23à4543.610.00à2809.62à2809.62
Condenser temperature, C 124.1264.36155.5064.36Condenser pressure,bar 5.5 1.09.0 1.0Side condenser stage ______6
Side condenser duty,kW __
__
__
à600.00à600.00Reboiler duty,kW 5634.952136.237771.18
4898.971067.385966.35
Boilup rate,kmol/hr 177.80186.19217.5389.63Bottoms rate,kmol/hr 104101104101Reboiler temperature, C 311.2997.59274.9696.34Reboiler pressure,bar 6.0 1.09.5 1.0Total Conversion,mol%99.99__
99.76__
Exergy loss,kW
1189.04
108.36
1297.40907.31
108.743217
1016.05
https://www.360docs.net/doc/745093111.html,parison of operating conditions for reactive distillation column RD101:(a)temperature pro ?les;(b)composition pro ?les;(c)reaction pro ?les;(d)exergy loss pro ?les.
N.Nguyen,Y.Demirel /Energy 36(2011)4838e 4847
4842
The sign of_E x;min depends on the energy stored in the inlet and outlet streams.
Thermodynamic ef?ciency(h)is the ratio between the total useful work output and the total exergy input.When_E x;min is greater than zero,the h is calculated by Refs.[4,5]
h?
_E
x;min
_E
x;min
t_E
x;loss
(12)
If the value of_E x;min is negative,the h is given by
h?
_E
x;min
_E
x;min
à_E
x;loss
(13)
Exergy loss is always greater than zero in every irreversible
processes,hence,h must lie between0and 1.A conventional
distillation column receives heat in the reboiler,performs the
separation work,and releases the rest of the amount in the
condenser.Thus,it resembles a thermal engine and the ef?ciency is
typically much less than1.
3.Reactive distillation
Reactive distillation column RD101shown in Fig.1a and b has30
stages,rectifying section located at the top,reaction zone located at
the middle,and stripping section located at the bottom of the
column.The column operates with a total condenser and a
kettle
Fig.3.Hydraulic analysis and enthalpy de?cit pro?les for column RD101:(a)stage-liquid?ow rate pro?les of base case design;(b)stage-liquid?ow rate pro?les of thermally coupled design;(c)stage-vapor?ow rate pro?les of base case design;(d)stage-vapor?ow rate pro?les of the thermally coupled design;(e)stage-enthalpy de?cit curves of base case design;(f)stage-enthalpy de?cit curves of the thermally coupled design.
N.Nguyen,Y.Demirel/Energy36(2011)4838e48474843
reboiler.The activity coef ?cient model of UNIQUAC containing size and shape parameters is used to estimate the phase equilibrium because of the presence of the components with wide range of molecular weights within the column.The esteri ?cation reaction takes place in the reaction zone from stages 3e 29with solid acid catalyst of sulfated zirconia (SO 4à2/ZrO 2)[27e 29].Operation temperature of the catalyst ranging from 130to 200 C and high activity even at high methanol/lauric acid ratio make it a suitable candidate for the RD application.The reverse hydrolysis reaction is negligible and the kinetic rate constants is given by k (T )?1.2?105exp(e 55,000/RT )[12].The units for reaction rate,concentration,and activation energy expressed as kmol/(m 3h),kmol/m 3,and kJ/kmol,respectively.For each reaction stage,an estimated volumetric hold up of 0.043m 3is used [12].The bottom separation zones,stage 30,separates the desired product,methyl dodecanoate,while the top separation zone,stages 1and 2,removes the water and unreacted methanol.4.Biodiesel plant
A biodiesel production plant using reactive distillation sequence requires at least two columns for biodiesel separation and meth-anol recovery as seen in Fig.1a [13].Fig.1b proposes a thermally coupled reactive distillation column sequence utilizing methanol and lauric acid (oil)to produce product methyl dodecanoate (bio-diesel).Tables 1and 2summarize the stream properties for both the sequences.Stream LAURIC is preheated by stream ESTER
B in heat exchanger HX101to 100
C and enters to the top of the reaction zone at stage 3of RD101.Stream METH combined with stream S3B in mixer M101is preheated to 110 C in heat exchanger HX102before it is fed to the bottom of the reaction zone at stage 29of RD101.The feeds enter at the both ends of the reaction zone to maximize conversion [7,10e 14].Although the conversion nearly reaches completion in 23reactive stages although,27stages are set for ensuring ?exibility in the operation.Both feed streams are preheated to minimize the loss of exergy caused by the tempera-ture gradient.The plant produces around 99.2wt%of 21,527kg/h of methyl dodecanoate and dilute concentration of methanol in water as summarized in Tables 1and 2.The inlet streams conditions are identical while there is a slight difference of the product concen-trations in the outlet streams between the two sequences of distillation columns.
The distillate,stream WATMET,of column RD101is fed to stage 9and 10of column T101of the BCRD and TCSRD sequences,respectively.Aspen Plus NQ curves (plots of heat load Q versus total number of stages N)are used to determine the number of stages and optimum feed locations based on an objective function [5,38].Column T101recovers methanol from water and recycles.The column operates with 12stages,with a kettle reboiler and a total condenser.The activity coef ?cient model of NRTL is used for pre-dicting the equilibrium and liquid properties in column T101.The top product,stream S3A,containing mostly methanol is pressur-ized before it is recycled.The bottom product,stream WATA,is treated as a waste.Stream ESTERA and S3A are tear streams for both sequences,while stream S4A and WATMET are tear streams only for the TCSRD sequence.
a
b 0.99195
0.992050.992150.992250.992355
8
11
14
17
20
E s t e r M a s s
F r a c t i o n
Stream S4A flow (kmol/hr) 4.85
4.904.95
5.005.055.10
5
8
11
14
17
20
R e b o i l e r D u t y (M W )
Stream S4A flow (kmol/hr)
Fig.4.Sensitivity analysis of stream S4A ?ow rate on:(a)ester mass fraction in the bottom product stream;(b)column RD101reboiler duty.
a
b
60
7080901000
3
6912
T e m p e r a t u r e (C )
Stage
Base case
Thermally coupled
-5
515253545036912
E x e r g y L o s s (k W )
Stage
Base case
Thermally coupled
https://www.360docs.net/doc/745093111.html,parison of operating conditions for distillation column T101:(a)temperature pro ?les;(b)exergy loss pro ?les.
N.Nguyen,Y.Demirel /Energy 36(2011)4838e 4847
4844
Table 4
Some of the streams properties for the base case and thermally coupled designs given in Fig.1.
Base Case Design
Thermally Coupled Design Stream _n (kmol/h)h (kJ/mol)s (J/mol-K)E x (kW)_n (kmol/h)h (kJ/mol)s (J/mol-K)E x (kW)S1100à703.98à1255.47à9157.24100à703.98à1255.47à9157.24S2B 200à228.82à212.87à9186.08200à228.82à212.87à9186.08ESTERA 104à519.37à1012.14à6286.31104à541.79à1050.46à6603.83S4B
____
__
__
25à278.11à152.94à1614.67WATMET 196à253.45à170.72à11027.47221à217.43à82.96à11828.90S4A ____
__
__
25à278.77à154.61à1615.77S3A 95à234.69à226.71à4409.4295à234.69à226.71à4409.42WATA
101
à279.60
à147.01
à6614.73
101
à280.37
à149.25
à
6617.34
Fig.6.Hydraulic analysis and enthalpy de ?cit pro ?les for column T101:(a)stage-liquid ?ow rate pro ?les of base case design;(b)stage-liquid ?ow rate pro ?les of thermally coupled design;(c)stage-vapor ?ow rate pro ?les of base case design;(d)stage-vapor ?ow rate pro ?les of thermally coupled design;(e)stage-enthalpy de ?cit curves of base case design;(f)stage-enthalpy de ?cit curves of the thermally coupled design.
N.Nguyen,Y.Demirel /Energy 36(2011)4838e 48474845
5.Results and discussions 5.1.Column RD101
Table 3compares the operating conditions and con ?gurations for reactive distillation column RD101in base case and thermally coupled sequences.The base case sequence operates with a total condenser,duty of à2168.38kW,and a molar re ?ux ratio of 0.1,while the thermally coupled design has no condenser,and has the reboiler duty of 735.98kW less than the base case sequence.However,higher pressure is required to achieve a comparable conversion due to the interconnecting vapor ?ow from the top of the column in thermally coupled sequence.Interconnecting side stream S4B,with molar ?ow rate of 7kmol/h,cools down top of the column RD101as seen in Fig.1b.
The temperature,composition,conversion,and exergy loss pro ?les in RD101of both the sequences are shown in Fig.2.In the thermally coupled column,the reaction zone temperature pro ?le remains almost constant.The composition pro ?les (Fig.2b)indicate that the base case sequence contains higher water concentration in the reaction zone,which causes liquid e liquid separation leading to solid catalyst deactivation.Both sequences have comparable conversions as shown in Fig.2c.The exergy loss pro ?les,shown in Fig.2d,indicate that the exergy losses in the stages 4e 28for both reactive distillation columns overlap [38].Exergy loss mainly occurs at the feed stages and in the reboilers.For column RD101,the total exergy loss of the thermally coupled design is 23.7%less than the base case sequence.The hydraulic analyses shown in Fig.3a e d indicate that actual vapor and liquid ?ows overlap with the ther-modynamic ideal minimum vapor and liquid ?ows,respectively,from stages 3e 28.The hydraulic maximum ?ow (HMF)is higher for base case sequence for both actual liquid and vapor ?ows.No ?ooding or dried up stages have occurred.Fig.3e and f shows the stage-enthalpy CGCCs for the base case and thermally coupled sequences produced by the thermal analysis;the ideal and actual pro ?les in the separation zones are close to each other.
5.2.Column T101
The base case and thermally coupled distillation columns T101operate with molar re ?ux ratios of 1.55and 2.02,respectively,as shown in Table 2.Design speci ?cation is used to set the ?ow rate of water to 1kmol/h in the distillate by varying the molar re ?ux ratio.The thermally coupled column has a side withdrawal with a ?ow rate of 7kmol/h at stage 10,which is connected to the top stage of the reactive distillation column RD101.Both the reboiler duty and ester mass fraction in the bottom decrease as the ?ow rate of stream S4A is reduced as indicated in Fig.4.The ester mass fraction is 99.2wt%when stream S4A ?ow rate is 7kmol/h.Stream S4A is pressurized by pump P102to match the pressure in column RD101.The reboiler and condenser duties required for the base case sequence are 2136.23kW and à2375.23kW,respectively,while they are 1067.38kW and à3409.62kW,respectively,for the ther-mally coupled sequence.
Fig.5compares the temperature and exergy loss pro ?les for column T101.Hydraulic analysis (Fig.6a e d)shows that the actual liquid and vapor ?ow rates are between the TIMF (thermodynamic ideal minimum ?ow)and HMF.Stream WATMET is introduced at stages 9and 10of the base case and thermally coupled sequences,respectively,to minimize the distortions due to inappropriate feed placement as shown in Fig.6e and f.A signi ?cant area beneath the CGCC pinch point between the ideal and actual enthalpy pro ?les of the thermally coupled column (Fig.6f)suggests the scope for side condensing.A side condenser with a heat duty of à600kW is installed on stage 6to reduce the area gap.
The stream properties and exergy values estimated by Eq.(9)are shown in Table 4.Table 5summarizes the total exergy lossess and thermodynamic ef ?ciencies of the both sequences.The ef ?ciency of column RD101increases from 46%to 63%,while the total exergy loss reduces by 281.35kW in thermally coupled sequence.
6.Conclusions
Thermally coupled side-stripper reactive distillation sequence requires less energy and use one less condenser and hence results in lower capital investment and operating cost compared with the base case reactive distillation sequence.The thermally coupled sequence reduces the energy consumption by 13.1%in the reactive distillation column and 50.0%in the methanol recovery column.In the thermally coupled reactive distillation column,the content of water in the reaction zone is lower.This decreases liquid e liquid separation and protects solid catalyst from deactivation.The total exergy losses for the columns are reduced from 1297.40kW to 1016.05kW indicating a signi ?cant amount of available energy saving and operation with less thermodynamic imperfections in thermally coupled sequence.
Nomenclature
AF actual ?ow
CGCC column grand composite curve BCRD base case reactive distillation _D distillate ?ow rate,kmol/h e x molar exergy,kW/kmol E x total Exergy,kW h enthalpy,kJ/kmol H total enthalpy,kJ
HMF hydraulic maximum ?ow _L liquid ?ow rate,kmol/h _m mass ?ow rate,kg/h _n
molar ?ow rate,kmol/h
PNMTC practical near-minimum thermodynamic condition _Q heat ?ow (heat transfer rate),kW _Q C condenser duty,kW _Q R reboiler duty,kW
R re ?ux ratio,universal gas constant RD reactive distillation
s molar entropy,kJ/kmol K T temperature,K
TA thermodynamic analysis
TCRD thermally coupled reactive distillation TIMF thermodynamic ideal minimum ?ow x liquid mole fraction y vapor mole fraction V vapor ?ow rate,kmol/h _W work,kW
Table 5
Minimum exergy of separation and thermodynamic ef ?ciency estimations based on the converged simulation.
Base Case Design
Thermally Coupled Design
Column _E x ;min (kW)_E x ;loss (kW)h
_E x ;min (kW)_E x ;loss (kW)h
Saved _E
x (kW)RD1011029.54
1189.040.461525.26907.310.63281.73T101 3.33
107.360.03
à813.62
108.740.88
à0.38Total
1297.40
1016.05
281.35
N.Nguyen,Y.Demirel /Energy 36(2011)4838e 4847
4846
Greek symbols
h ef?ciency
l heat of vaporization,J/mol
Subscripts
C condenser
D distillate
def de?cit
F feed
V vapor
H heavy
L light
min minimum
R reboiler
s stream,shaft
*equilibrium condition
o reference state
References
[1]Khalifa M,Emtir M.Rigorous optimization of heat-integrated and Petlyuk
column distillation con?gurations based on feed conditions.Clean Technol Environ Policy2009;11:107e13.
[2]Demirel Y.Retro?t of distillation columns by thermodynamic analysis.Sep Sci
Technol2006;41:791e817.
[3]Nakaiwa M,Huang K,Owa M,Akiya T,Sato M,Takamatsu T.Energy savings in
heat-integrated distillation columns.Energy1997;22:621e5.
[4]Demirel Y.Thermodynamic analysis of separation systems.Sep Sci Technol
2004;39:3897e942.
[5]Nguyen N,Demirel Y.Retro?t of distillation columns in biodiesel production
plants.Energy2010;35:1625e32.
[6]Demirel Y.Assessment of thermodynamic performances for distillation
columns.Int J Exergy2006;3:345e61.
[7]Nguyen N,Demirel Y.Reactive distillation column esteri?cation of lauric with
methanol:equilibrium vs.nonequilibrium approaches;2010.AIChE Annual Meeting;2010Nov7e12,Salt Lake City,Utah.
[8]Araújo AB,Brito RP,Vasconcelos LS.Exergetic analysis of distillation processes
e a case study.Energy2007;32:1185e93.
[9]Kiss AA,Pragt JJ,van Strien CJG.Reactive dividing-wall columns e defying
equilibrium restrictions.Chem Prod Process Model2009;4.Article2.
[10]Omota F,Dimian AC,Bliek A.Fatty acid esteri?cation by reactive distillation.
Part1:equilibrium-based design.Chem Eng Sci2003;98:3159e74.
[11]Omota F,Dimian AC,Bliek A.Fatty acid esteri?cation by reactive distillation:
part2e kinetics-based design for sulphated zirconia catalysts.Chem Eng Sci 2003;58:3175e85.
[12]Dimian AC,Bildea CS,Omota F,Kiss AA.Innovative process for fatty acid esters
by dual reactive https://www.360docs.net/doc/745093111.html,put Chem Eng2009;33:743e50.
[13]Kiss AA,Dimian AC,Rothenberg G.Biodiesel by catalytic reactive distillation
powered by metal oxides.Energy Fuels2008;22:598e604.
[14]William L,Cheng-Ching Y.Reactive distillation design and control.New York:
Wiley;2008.[15]Malinen I,Tanskanen J.Thermally coupled side-column con?gurations
enabling distillation boundary crossing.1.An overview and solving proce-dure.Ind Eng Chem Res2009;48:6387e404.
[16]Malinen I,Tanskanen J.Thermally coupled side-column con?gurations
enabling distillation boundary crossing. 2.Effects of intermediate heat exchangers.Ind Eng Chem Res2009;48:6372e86.
[17]Rong B-G,Kraslawski A,Turunen I.Synthesis of heat-integrated thermally
coupled distillation systems for multicomponent separations.Ind Eng Chem Res2003;42:4329e39.
[18]Rong B-G,Turunen I.Synthesis of new distillation systems by simultaneous
thermal coupling and heat integration.Ind Eng Chem Res2006;45:3830e42.
[19]Agrawal R,Fidkowski Z.Are thermally coupled distillation columns always
thermodynamically more ef?cient for ternary distillations?Ind Eng Chem Res 1998;37:3444e54.
[20]Asprion N,Kaibel G.Dividing wall columns:fundamentals and recent
advances.Chem Eng Process Process Intensif2010;49:139e46.
[21]Wang S-J,Lee H-Y,Ho J-H.Plantwide design of ideal reactive distillation
processes with thermal coupling.Ind Eng Chem Res2010;49:3262e74. [22]Gomez-Castro FI,Rico-Ramirez V,Segovia-Hernandez JG,Hernandez S.
Feasibility study of a thermally coupled reactive distillation process for bio-diesel production.Chem Eng Process Process Intensif2010;49:262e9. [23]Errico M,Rong B-G,Tola G.Process intensi?cation for the retro?t of a multi-
component distillation plant e an industrial case study.Ind Eng Chem Res 2008;47:1975e80.
[24]Petlyuk FB.Distillation theory and its application to optimal design of sepa-
ration units.Cambridge:United Kingdom;2004.
[25]Hernandez S,Segovia-Hernandez JG,Rico-Ramirez V.Themodynamically
equivalent distillation schemes to the Petlyuk column for ternary mixtures.
Energy2006;31:2167e83.
[26]Barroso-Mu?oz FO,Hernández S,Segovia-Hernández JG,Hernández-Escoto H,
Aguilera-Alvarado AF.Thermally coupled distillation systems:study of an energy-ef?cient reactive case.Chem Bio Eng2007;2:115e20.
[27]Kansedo JB.Synthesis of biodiesel from palm oil and sea mango oil using
sulfated zirconia catalyst(thesis).Malaysia:Universiti Sains Malaysia;2009.
[28]Garcia CM,Teixeira S,Marciniuk LL,Schuchardt U.Transesteri?cation of soybean
oil catalyzed by sulfated zirconia.Bioresour Technol2008;99:6608e13.
[29]Kiss AA,Dimian AC,Rothenberg.Solid acid catalysts for biodiesel production
e towards sustainable energy.Adv Synth Catal2006;348:75e81.
[30]Agrawal R,Herron DM.Ef?cient use of an intermediate reboiler and
condenser in a binary distillation.AIChE J1998;44:1303e15.
[31]Rivero R.Exergy simulation and optimization of adiabatic and diabatic binary
distillation.Energy2001;26:561e93.
[32]De Koeijer GM,Rivero R.Entropy production and exergy loss in experimental
distillation columns.Chem Eng Sci2003;58:1587e97.
[33]Suphanit B,Bischert A,Narataruksa P.Exergy loss analysis of heat transfer across
the wall of the dividing-wall distillation column.Energy2007;32:2121e34. [34]Bandyopadhyay S,Malik RK,Shenoy UV.Temperature-enthalpy curve for
energy targeting of distillation https://www.360docs.net/doc/745093111.html,put Chem Eng1998;22: 1733e44.
[35]Dhole VR,Linnhoff B.Distillation column https://www.360docs.net/doc/745093111.html,put Chem Eng1993;17:
549e60.
[36]Chang H,Li Jr W.A new exergy method for process analysis and optimization.
Chem Eng Sci2005;60:2771e84.
[37]Bandyopadhyay S.Effect of feed on optimal thermodynamic performance of
a distillation column.Chem Eng J2002;88:175e86.
[38]Aspen Engineering Suite,https://www.360docs.net/doc/745093111.html,;2004.
[39]Demirel Y.Nonequilibrium thermodynamics transport and rate processes in
physical,chemical and biological processes.2nd ed.Amsterdam:Elsevier;
2007.
N.Nguyen,Y.Demirel/Energy36(2011)4838e48474847