Determination of the Strong Phase in D0 - K+pi- Using Quantum-Correlated Measurements
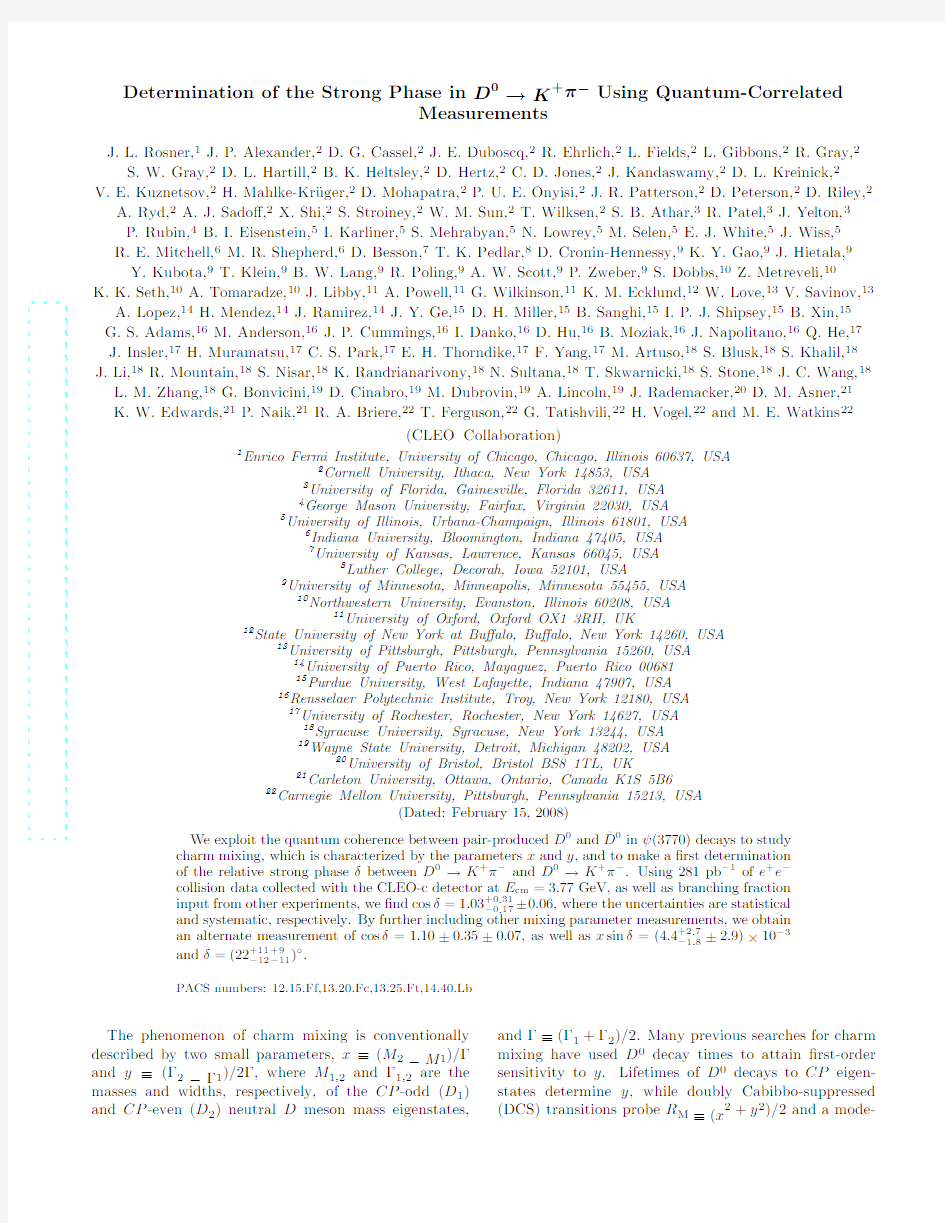

a r X i v :0802.2264v 1 [h e p -e x ] 15 F e
b 2008
Determination of the Strong Phase in D 0→K +π?Using Quantum-Correlated
Measurements
J.L.Rosner,1J.P.Alexander,2D.G.Cassel,2J.E.Duboscq,2R.Ehrlich,2L.Fields,2L.Gibbons,2R.Gray,2
S.W.Gray,2D.L.Hartill,2B.K.Heltsley,2D.Hertz,2C.D.Jones,2J.Kandaswamy,2D.L.Kreinick,2
V.E.Kuznetsov,2H.Mahlke-Kr¨u ger,2D.Mohapatra,2P.U.E.Onyisi,2J.R.Patterson,2D.Peterson,2D.Riley,2
A.Ryd,2A.J.Sado?,2X.Shi,2S.Stroiney,2W.M.Sun,2T.Wilksen,2S.
B.Athar,3R.Patel,3J.Yelton,3P.Rubin,4B.I.Eisenstein,5I.Karliner,5S.Mehrabyan,5N.Lowrey,5M.Selen,5E.J.White,5J.Wiss,5R.E.Mitchell,6M.R.Shepherd,6D.Besson,7T.K.Pedlar,8D.Cronin-Hennessy,9K.Y.Gao,9J.Hietala,9Y.Kubota,9T.Klein,https://www.360docs.net/doc/807865786.html,ng,9R.Poling,9A.W.Scott,9P.Zweber,9S.Dobbs,10Z.Metreveli,10
K.K.Seth,10A.Tomaradze,10J.Libby,11A.Powell,11G.Wilkinson,11K.M.Ecklund,12W.Love,13V.Savinov,13
A.Lopez,14H.Mendez,14J.Ramirez,14J.Y.Ge,https://www.360docs.net/doc/807865786.html,ler,15
B.Sanghi,15I.P.J.Shipsey,15B.Xin,15G.S.Adams,16M.Anderson,16J.P.Cummings,16I.Danko,16D.Hu,16B.Moziak,16J.Napolitano,16Q.He,17J.Insler,17H.Muramatsu,17
C.S.Park,17E.H.Thorndike,17F.Yang,17M.Artuso,18S.Blusk,18S.Khalil,18J.Li,18R.Mountain,18S.Nisar,18K.Randrianarivony,18N.Sultana,18T.Skwarnicki,18S.Stone,18J.C.Wang,18
L.M.Zhang,18G.Bonvicini,19D.Cinabro,19M.Dubrovin,19A.Lincoln,19J.Rademacker,20D.M.Asner,21K.W.Edwards,21P.Naik,21R.A.Briere,22T.Ferguson,22G.Tatishvili,22H.Vogel,22and M.E.Watkins 22
(CLEO Collaboration)
1
Enrico Fermi Institute,University of Chicago,Chicago,Illinois 60637,USA
2
Cornell University,Ithaca,New York 14853,USA 3
University of Florida,Gainesville,Florida 32611,USA 4
George Mason University,Fairfax,Virginia 22030,USA 5
University of Illinois,Urbana-Champaign,Illinois 61801,USA 6
Indiana University,Bloomington,Indiana 47405,USA 7
University of Kansas,Lawrence,Kansas 66045,USA
8
Luther College,Decorah,Iowa 52101,USA
9
University of Minnesota,Minneapolis,Minnesota 55455,USA 10
Northwestern University,Evanston,Illinois 60208,USA
11
University of Oxford,Oxford OX13RH,UK
12
State University of New York at Bu?alo,Bu?alo,New York 14260,USA
13
University of Pittsburgh,Pittsburgh,Pennsylvania 15260,USA 14
University of Puerto Rico,Mayaguez,Puerto Rico 0068115
Purdue University,West Lafayette,Indiana 47907,USA 16
Rensselaer Polytechnic Institute,Troy,New York 12180,USA 17
University of Rochester,Rochester,New York 14627,USA 18
Syracuse University,Syracuse,New York 13244,USA 19
Wayne State University,Detroit,Michigan 48202,USA
20
University of Bristol,Bristol BS81TL,UK
21
Carleton University,Ottawa,Ontario,Canada K1S 5B6
22
Carnegie Mellon University,Pittsburgh,Pennsylvania 15213,USA
(Dated:February 15,2008)
We exploit the quantum coherence between pair-produced D 0and ˉD
0in ψ(3770)decays to study charm mixing,which is characterized by the parameters x and y ,and to make a ?rst determination
of the relative strong phase δbetween D 0→K +π?and ˉD
0→K +π?.Using 281pb ?1of e +e ?collision data collected with the CLEO-c detector at E cm =3.77GeV,as well as branching fraction
input from other experiments,we ?nd cos δ=1.03+0.31
?0.17±0.06,where the uncertainties are statistical and systematic,respectively.By further including other mixing parameter measurements,we obtain
an alternate measurement of cos δ=1.10±0.35±0.07,as well as x sin δ=(4.4+2.7?1.8±2.9)×10
?3
and δ=(22+11?12+9?11)?
.
PACS numbers:12.15.Ff,13.20.Fc,13.25.Ft,14.40.Lb
The phenomenon of charm mixing is conventionally
described by two small parameters,x ≡(M 2?M 1)/Γand y ≡(Γ2?Γ1)/2Γ,where M 1,2and Γ1,2are the masses and widths,respectively,of the CP -odd (D 1)and CP -even (D 2)neutral D meson mass eigenstates,
and Γ≡(Γ1+Γ2)/2.Many previous searches for charm mixing have used D 0decay times to attain ?rst-order sensitivity to y .Lifetimes of D 0decays to CP eigen-states determine y ,while doubly Cabibbo-suppressed (DCS)transitions probe R M ≡(x 2+y 2)/2and a mode-
2 dependent quantity,y′.For the most widely used DCS
mode,D0→K+π?,y′≡y cosδ?x sinδ,where?δ
is the phase of K+π?|D0 / K+π?|ˉD0 ≡re?iδ.We
adopt a convention in whichδcorresponds to a strong
phase,which vanishes in the SU(3)limit[1].To date,
δhas not been measured,so measurements of y and y′
have not been directly comparable.The magnitude r of
the amplitude ratio is approximately0.06.
In this Letter,we implement the method described in
Ref.[2]for measuring y and cosδusing quantum correla-
tions at theψ(3770)resonance[1,3],where D0ˉD0pairs
produced in e+e?collisions are in a C-odd eigenstate.
We extract these parameters from decay rates to single
tags(ST),which are individually reconstructed D0or
ˉD0candidates,and double tags(DT),which are events
where both D0andˉD0are reconstructed.CP violation
in D and K decays are negligible second order e?ects.
To?rst order in x and y,the rateΓD0ˉD0(i,j)for C-
odd D0ˉD0decay to?nal state{i,j}follows from the
anti-symmetric amplitude M ij:
ΓD0ˉD0(i,j)∝M2ij= A iˉA j?ˉA i A j 2
=| i|D2 j|D1 ? i|D1 j|D2 |2,(1)
where A i≡ i|D0 ,ˉA i≡ i|ˉD0 ,and we have used
=[|D0 ±|ˉD0 ]/√
|D1
2
K?π+1+R WS1+R WS
S±22
3
TABLE II:D?nal states reconstructed in this analysis.
Type Final States
K?π+25374±16864.70±0.04
K+π?25842±16965.62±0.04
K+K?4740±7157.25±0.09
π+π?2098±6072.92±0.13
K0Sπ0π02435±7412.50±0.06
K0Sπ07523±9329.73±0.05
K0Sη1051±4310.34±0.06
K0Sω3239±6312.48±0.04
E20/c4?p2D/c2,where p D is the D0candidate mo-mentum and E0is the beam energy,and?E≡E D?E0, where E D is the sum of the D0candidate daughter en-ergies.We extract ST and DT yields from M distribu-tions using unbinned maximum likelihood?ts(ST)or by counting candidates in signal and sideband regions(DT). Because most K0L mesons and neutrinos produced at CLEO-c are not detected,we only reconstruct modes with these particles in DTs,where the other D in the event is fully reconstructed.Ref.[8]describes the miss-ing mass technique used to identify K0Lπ0candidates. For semileptonic decays,we use inclusive,partial recon-struction to maximize e?ciency,demanding only that the electron be identi?ed.Electron identi?cation utilizes a multivariate discriminant[9]that combines measure-ments from the tracking chambers,the electromagnetic calorimeter,and the ring imagingˇCerenkov counter. Table III gives yields and e?ciencies for8ST modes and58DT modes,where the DT modes have been grouped into categories.Fifteen of the DT modes are for-bidden by CP conservation and are not included in the nominal?ts.In general,crossfeed among signal modes and backgrounds from other D decays are smaller than 1%.Modes with K0Sπ0π0have approximately3%back-ground,and yields for{K?π±,K?π±}and{S±,S±}are consistent with being entirely from background. External inputs to the standard?t include measure-ments of R M,R WS,B K?π+,and B S
±
,as well as an in-dependent B K0
L
π0
from CLEO-c,as shown in Table IV. R WS is required to constrain r2,and thus,to convert r cosδand rx sinδto cosδand x sinδ.We also perform an extended?t that uses the external mixing parameter measurements shown in Table V.These?ts incorporate the full covariance matrix for these inputs,accounting for statistical overlap with the yields in this analysis.Covari-ance matrices for the?ts in Ref.[16]have been provided by the CLEO,Belle,and BABAR collaborations.
TABLE IV:Averages of external measurements used in the standard and extended?ts.Charge-averaged D0branching fractions are denoted by?nal state.
Parameter Average
y0.00662±0.00211[13,14,15]
x0.00811±0.00334[15]
r20.00339±0.00012[16]
y′0.0034±0.0030[16]
x′20.00006±0.00018[16]
Systematic uncertainties include those associated with e?ciencies for reconstructing tracks,K0S decays,π0de-cays,and for hadron identi?cation(see Refs.[5,7]). Other sources of e?ciency uncertainty include:?E re-quirements(0.5–5.5%),ηreconstruction(4.0%),electron identi?cation(1.0%),modeling of particle multiplicity and detector noise(0.1–1.3%),simulation of initial and?-nal state radiation(0.5–1.2%),and modeling of resonant substructure in K0Sπ0π0(0.7%).We also include addi-tive uncertainties of0.0–0.9%to account for variations of yields with?t function.
These systematic uncertainties are included in the co-variance matrix given to the?tter,which propagates them to the?t parameters.The other?t inputs deter-mined in this analysis are ST and DT yields and e?cien-cies,crossfeed probabilities,background branching frac-tions and e?ciencies,and statistical uncertainties on all
4
of these measurements.Quantum correlations between signal and background modes are accounted for using as-sumed values of amplitude ratios and strong phases that are systematically varied and found to have negligible ef-fect.We validated our analysis technique in a simulated C-odd
D0ˉD0sample15times the size of our data sample. Table VI shows the results of the data?ts,excluding the15same-CP DT modes.Our standard?t includes the measurements in Table IV but not Table V.In this ?t,x sinδis not determined reliably,so we?x it to zero, and the associated systematic uncertainty is±0.03for
cosδand negligible for all other parameters.We obtain a?rst measurement of cosδ,consistent with being at the boundary of the physical region.Our branching fraction results do not supersede other CLEO-c measurements. TABLE VI:Results from the standard?t(with Table IV in-puts)and the extended?t(with Table IV/V inputs).Uncer-tainties are statistical and systematic,respectively.Charge-averaged D0branching fractions are denoted by?nal state. Parameter Standard Fit Extended Fit
χ2?t/ndof30.1/4655.3/57
The likelihood curve for cosδ,shown in Fig.1a,is com-puted as L=e?(χ2?χ2min)/2at various?xed values of cosδ.It is highly non-Gaussian,so we assign asymmet-ric uncertainties(which still do not fully capture the non-linearity)by?nding the values of cosδwhere?χ2=1 to obtain cosδ=1.03+0.31
?0.17±0.06.This non-linearity stems from the use of r cosδto determine cosδ,which causes the uncertainty on cosδto scale roughly like1/r. Because r2is obtained from R WS,an upward shift in y lowers the derived value of r2(for positive r cosδ),and the resultant uncertainty on cosδincreases,as illustrated by Fig.1b.For values of|cosδ|<1,we also compute L as a function of|δ|,and we integrate these curves within the physical region to obtain95%con?dence level(CL) limits of cosδ>0.07and|δ|<75?.
When combined with previous measurements of y and y′,our measurement of cosδalso gives x sinδ.Table VI shows the results of such an extended?t that includes external inputs from both Table IV and Table V.The FIG.1:Standard?t likelihood including both statistical and systematic uncertainties for cosδ(a),and simultaneous like-lihood for cosδand y(b)shown as contours in increments of 1σ,whereσ=
?χ2.The hatched regions contain95%of the area in the physical regions.For δ,the?t fails to converge beyond the limits of the plot. resultant value of y includes the CLEO-c measurement from the standard?t,but the precision is dominated by the external y measurements.The overall uncer-tainty on cosδincreases to±0.36because of the non-linearity discussed above.However,unlike the standard ?t,the likelihood for cosδis nearly Gaussian,as shown in Fig.2a.The correlation coe?cient between cosδand x sinδis0.56,and we assign asymmetric uncertainties of x sinδ=(4.4+2.7
?1.8±2.9)×10?3.By repeating the?t at
5
various simultaneously ?xed values of cos δand sin δ,we
also determine δ=(22+11?12+9?11)?
.The corresponding 95%CL intervals within the physical region are cos δ>0.39,x sin δ∈[0.002,0.014],and δ∈[?7?,+61?].Perform-ing this extended ?t with y ,x 2,and x sin δ?xed to zero results in a change in χ2of 25.1,or a signi?cance of 5.0σ.
By observing the change in 1/σ2
y as each ?t input is removed,we identify the major contributors of informa-tion on y to be the {S ±,e }yields (90%)and {Kπ,e }yields (10%).For cos δ,the {Kπ,S ±}DT yields and the ST yields simultaneously account for 100%.We also ?nd that no single input or group of inputs exerts a pull larger than 3σon cos δor y .Moreover,removing all external inputs gives branching fractions consistent with those in Table IV.Finally,if we determine y only from K +K ?and π+π?input,as in previous direct measurements,the result is consistent with the value in Table VI.
We also allow for a C -even D 0ˉD
0admixture in the initial state,which is expected to be O (10?8)[18],by including the 15{S ±,S ±}DT yields in the ?t.These modes limit the C -even component,which can modify the other yields as described in Ref.[2].In both the standard and extended ?ts,we ?nd a C -even fraction consistent with zero with an uncertainty of 2.4%,and neither the ?tted parameters nor their uncertainties are shifted noticeably from the values in Table VI.
In summary,using 281pb ?1of e +e ?collisions pro-duced at the ψ(3770),we make a ?rst determination of
the strong phase δ,with cos δ=1.03+0.31
?0.17±0.06.By further including external mixing parameter measure-ments in our analysis,we obtain an alternate measure-ment of cos δ=1.10±0.35±0.07,as well as x sin δ=
(4.4+2.7?1.8±2.9)×10?3
and δ=(22+11?12+9?11)?.
We thank Alexey Petrov,William Lockman,Alan Schwartz,Bostjan Golob,and Brian Petersen for help-ful discussions.We gratefully acknowledge the e?ort of the CESR sta?in providing us with excellent luminosity and running conditions.This work was supported by the A.P.Sloan Foundation,the National Science Foundation,the U.S.Department of Energy,and the Natural Sciences and Engineering Research Council of Canada.
[1]M.Gronau,Y.Grossman and J.L.Rosner,Phys.Lett.
B 508,37(2001).
[2]D.M.Asner and W.M.Sun,Phys.Rev.D 73,034024
(2006)[Erratum-ibid.77,019901(E)(2008)].
[3]R.L.Kingsley,S.B.Treiman,F.Wilczek and A.Zee,
Phys.Rev.D 11,1919(1975);L.B.Okun,B.M.Pon-tecorvo and V.I.Zakharov,Lett.Nuovo Cim.13,218(1975);R.L.Kingsley,Phys.Lett.B 63,329(1976);M.Goldhaber and J.L.Rosner,Phys.Rev.D 15,1254(1977);I.I.Bigi and A.I.Sanda,Phys.Lett.B 171,320(1986);I.I.Bigi,SLAC-PUB-4000;I.I.Bigi,UND-HEP-89-BIG01,also SLAC-R-343,pp.169–195;Z.Z.Xing,Phys.Rev.D 55,196(1997); D.Atwood and A.A.Petrov,Phys.Rev.D 71,054032(2005).[4]W.M.Sun,Nucl.Instrum.Meth.A 556,325(2006).[5]S.Dobbs et al.[CLEO Collaboration],Phys.Rev.D 76,
112001(2007)[arXiv:0709.3783[hep-ex]].
[6]Y.Kubota et al.[CLEO Collaboration],Nucl.In-strum.Methods Phys.Res.,Sec.A 320,66(1992);D.Pe-terson et al.,Nucl.Instrum.Methods Phys.Res.,Sec.A 478,142(2002);M.Artuso et al.,Nucl.Instrum.Meth-ods Phys.Res.,Sec.A 502,91(2003);R.A.Briere et al.[CLEO-c/CESR-c Taskforces &CLEO-c Collaboration],Cornell LEPP preprint CLNS 01/1742(2001).[7]Companion paper,to be submitted to PRD.
[8]Q.He et al.[CLEO Collaboration],arXiv:0711.1463[hep-ex].
[9]T.E.Coan et al.[CLEO Collaboration],Phys.Rev.Lett.
95,181802(2005).
[10]E.M.Aitala et al.[E791Collaboration],Phys.Rev.D
57,13(1998);J.M.Link et al.[FOCUS Collaboration],Phys.Lett.B 618,23(2005);A.Abulencia et al.[CDF Collaboration],Phys.Rev.D 74,031109(2006).
[11]E.M.Aitala et al.[E791Collaboration],Phys.Rev.Lett.
77,2384(1996); C.Cawl?eld et al.[CLEO Collabora-tion],Phys.Rev.D 71,077101(2005);K.Abe et al.[Belle Collaboration],Phys.Rev.D 72,071101(2005);B.Aubert et al.[BABAR Collaboration],Phys.Rev.D 76,014018(2007).
[12]S.Eidelman et al.,Phys.Lett.B 592,1(2004).
[13]W.-M.Yao et al.,Journal of Physics G 33,1(2006).[14]M.Staric et al.[Belle Collaboration],Phys.Rev.Lett.
98,211803(2007).
[15]D.M.Asner et al.[CLEO Collaboration],Phys.Rev.D
72,012001(2005);L.M.Zhang et al.[BELLE Collabo-ration],Phys.Rev.Lett.99,131803(2007).
[16]R.Godang et al.[CLEO Collaboration],Phys.Rev.Lett.
84,5038(2000);L.M.Zhang et al.[BELLE Collabora-tion],Phys.Rev.Lett.96,151801(2006);B.Aubert et al.[BABAR Collaboration],Phys.Rev.Lett.98,211802(2007).
[17]A.Abulencia et al.[CDF Collaboration],Phys.Rev.D
74,031109(2006).
[18]A.Petrov,private communication.