Design of SnO2 ZnO hierarchical nanostructures for enhanced ethanol
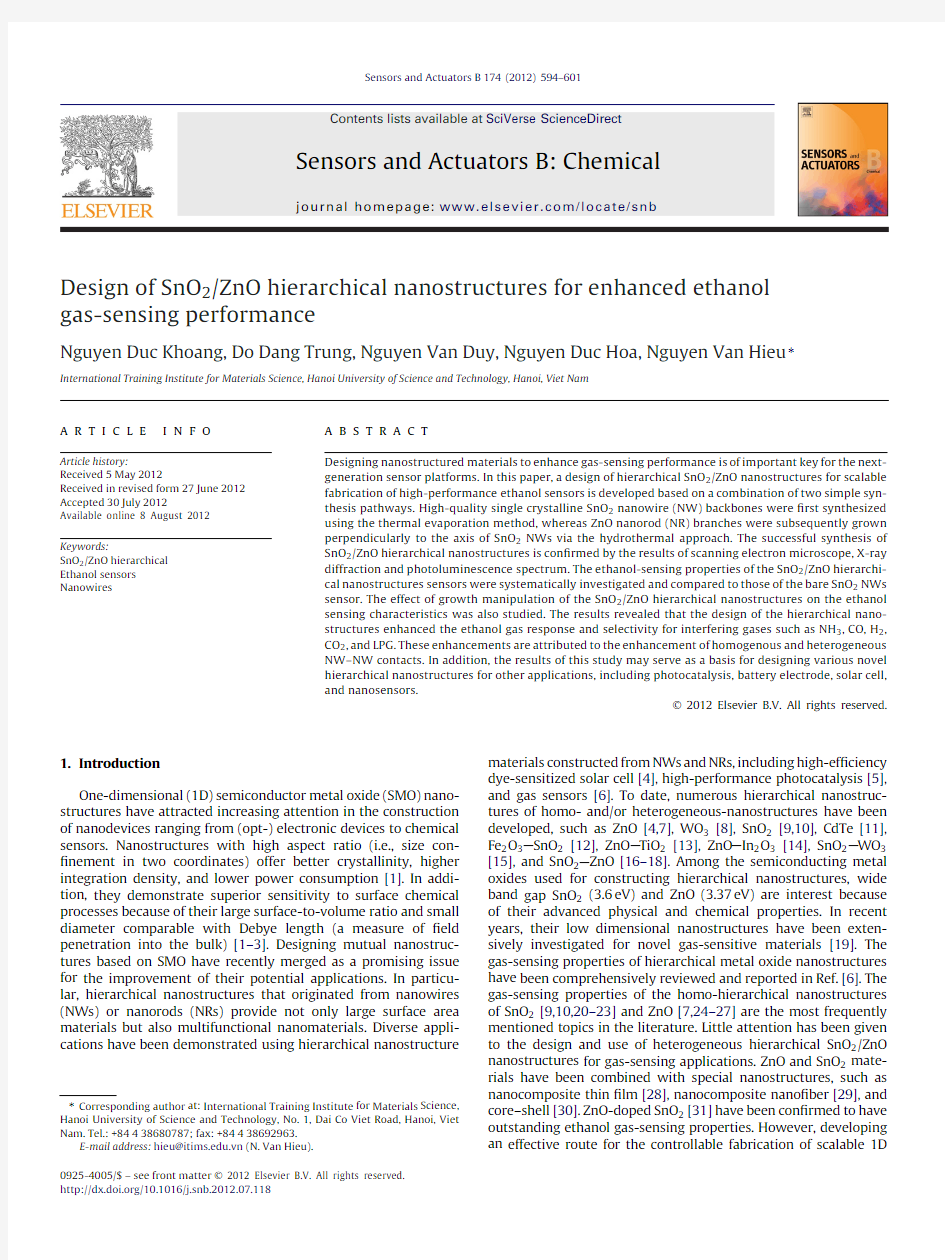
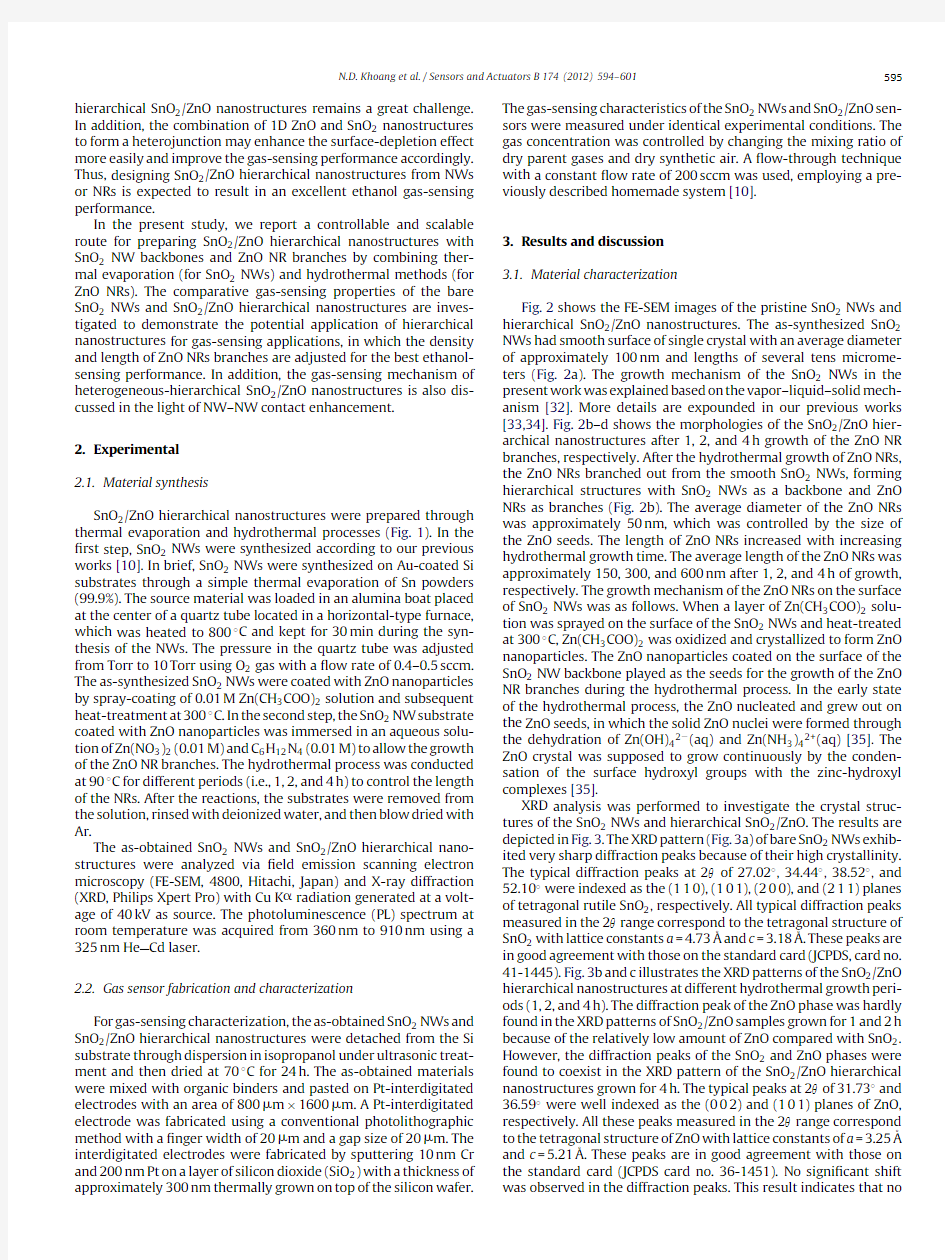
Sensors and Actuators B 174 (2012) 594–601
Contents lists available at SciVerse ScienceDirect
Sensors and Actuators B:
Chemical
j o u r n a l h o m e p a g e :w w w.e l s e v i e r.c o m /l o c a t e /s n
b
Design of SnO 2/ZnO hierarchical nanostructures for enhanced ethanol gas-sensing performance
Nguyen Duc Khoang,Do Dang Trung,Nguyen Van Duy,Nguyen Duc Hoa,Nguyen Van Hieu ?
International Training Institute for Materials Science,Hanoi University of Science and Technology,Hanoi,Viet Nam
a r t i c l e
i n f o
Article history:
Received 5May 2012
Received in revised form 27June 2012Accepted 30July 2012
Available online 8 August 2012
Keywords:
SnO 2/ZnO hierarchical Ethanol sensors Nanowires
a b s t r a c t
Designing nanostructured materials to enhance gas-sensing performance is of important key for the next-generation sensor platforms.In this paper,a design of hierarchical SnO 2/ZnO nanostructures for scalable fabrication of high-performance ethanol sensors is developed based on a combination of two simple syn-thesis pathways.High-quality single crystalline SnO 2nanowire (NW)backbones were ?rst synthesized using the thermal evaporation method,whereas ZnO nanorod (NR)branches were subsequently grown perpendicularly to the axis of SnO 2NWs via the hydrothermal approach.The successful synthesis of SnO 2/ZnO hierarchical nanostructures is con?rmed by the results of scanning electron microscope,X-ray diffraction and photoluminescence spectrum.The ethanol-sensing properties of the SnO 2/ZnO hierarchi-cal nanostructures sensors were systematically investigated and compared to those of the bare SnO 2NWs sensor.The effect of growth manipulation of the SnO 2/ZnO hierarchical nanostructures on the ethanol sensing characteristics was also studied.The results revealed that the design of the hierarchical nano-structures enhanced the ethanol gas response and selectivity for interfering gases such as NH 3,CO,H 2,CO 2,and LPG.These enhancements are attributed to the enhancement of homogenous and heterogeneous NW–NW contacts.In addition,the results of this study may serve as a basis for designing various novel hierarchical nanostructures for other applications,including photocatalysis,battery electrode,solar cell,and nanosensors.
? 2012 Elsevier B.V. All rights reserved.
1.Introduction
One-dimensional (1D)semiconductor metal oxide (SMO)nano-structures have attracted increasing attention in the construction of nanodevices ranging from (opt-)electronic devices to chemical sensors.Nanostructures with high aspect ratio (i.e.,size con-?nement in two coordinates)offer better crystallinity,higher integration density,and lower power consumption [1].In addi-tion,they demonstrate superior sensitivity to surface chemical processes because of their large surface-to-volume ratio and small diameter comparable with Debye length (a measure of ?eld penetration into the bulk)[1–3].Designing mutual nanostruc-tures based on SMO have recently merged as a promising issue for the improvement of their potential applications.In particu-lar,hierarchical nanostructures that originated from nanowires (NWs)or nanorods (NRs)provide not only large surface area materials but also multifunctional nanomaterials.Diverse appli-cations have been demonstrated using hierarchical nanostructure
?Corresponding author at:International Training Institute for Materials Science,Hanoi University of Science and Technology,No.1,Dai Co Viet Road,Hanoi,Viet Nam.Tel.:+84438680787;fax:+84438692963.
E-mail address:hieu@https://www.360docs.net/doc/c512341737.html,.vn (N.Van Hieu).
materials constructed from NWs and NRs,including high-ef?ciency dye-sensitized solar cell [4],high-performance photocatalysis [5],and gas sensors [6].To date,numerous hierarchical nanostruc-tures of homo-and/or heterogeneous-nanostructures have been developed,such as ZnO [4,7],WO 3[8],SnO 2[9,10],CdTe [11],Fe 2O 3SnO 2[12],ZnO TiO 2[13],ZnO In 2O 3[14],SnO 2WO 3[15],and SnO 2ZnO [16–18].Among the semiconducting metal oxides used for constructing hierarchical nanostructures,wide band gap SnO 2(3.6eV)and ZnO (3.37eV)are interest because of their advanced physical and chemical properties.In recent years,their low dimensional nanostructures have been exten-sively investigated for novel gas-sensitive materials [19].The gas-sensing properties of hierarchical metal oxide nanostructures have been comprehensively reviewed and reported in Ref.[6].The gas-sensing properties of the homo-hierarchical nanostructures of SnO 2[9,10,20–23]and ZnO [7,24–27]are the most frequently mentioned topics in the literature.Little attention has been given to the design and use of heterogeneous hierarchical SnO 2/ZnO nanostructures for gas-sensing applications.ZnO and SnO 2mate-rials have been combined with special nanostructures,such as nanocomposite thin ?lm [28],nanocomposite nano?ber [29],and core–shell [30].ZnO-doped SnO 2[31]have been con?rmed to have outstanding ethanol gas-sensing properties.However,developing an effective route for the controllable fabrication of scalable 1D
0925-4005/$–see front matter ? 2012 Elsevier B.V. All rights reserved.https://www.360docs.net/doc/c512341737.html,/10.1016/j.snb.2012.07.118
N.D.Khoang et al./Sensors and Actuators B174 (2012) 594–601595
hierarchical SnO2/ZnO nanostructures remains a great challenge. In addition,the combination of1D ZnO and SnO2nanostructures to form a heterojunction may enhance the surface-depletion effect more easily and improve the gas-sensing performance accordingly. Thus,designing SnO2/ZnO hierarchical nanostructures from NWs or NRs is expected to result in an excellent ethanol gas-sensing performance.
In the present study,we report a controllable and scalable route for preparing SnO2/ZnO hierarchical nanostructures with SnO2NW backbones and ZnO NR branches by combining ther-mal evaporation(for SnO2NWs)and hydrothermal methods(for ZnO NRs).The comparative gas-sensing properties of the bare SnO2NWs and SnO2/ZnO hierarchical nanostructures are inves-tigated to demonstrate the potential application of hierarchical nanostructures for gas-sensing applications,in which the density and length of ZnO NRs branches are adjusted for the best ethanol-sensing performance.In addition,the gas-sensing mechanism of heterogeneous-hierarchical SnO2/ZnO nanostructures is also dis-cussed in the light of NW–NW contact enhancement.
2.Experimental
2.1.Material synthesis
SnO2/ZnO hierarchical nanostructures were prepared through thermal evaporation and hydrothermal processes(Fig.1).In the ?rst step,SnO2NWs were synthesized according to our previous works[10].In brief,SnO2NWs were synthesized on Au-coated Si substrates through a simple thermal evaporation of Sn powders (99.9%).The source material was loaded in an alumina boat placed at the center of a quartz tube located in a horizontal-type furnace, which was heated to800?C and kept for30min during the syn-thesis of the NWs.The pressure in the quartz tube was adjusted from Torr to10Torr using O2gas with a?ow rate of0.4–0.5sccm. The as-synthesized SnO2NWs were coated with ZnO nanoparticles by spray-coating of0.01M Zn(CH3COO)2solution and subsequent heat-treatment at300?C.In the second step,the SnO2NW substrate coated with ZnO nanoparticles was immersed in an aqueous solu-tion of Zn(NO3)2(0.01M)and C6H12N4(0.01M)to allow the growth of the ZnO NR branches.The hydrothermal process was conducted at90?C for different periods(i.e.,1,2,and4h)to control the length of the NRs.After the reactions,the substrates were removed from the solution,rinsed with deionized water,and then blow dried with Ar.
The as-obtained SnO2NWs and SnO2/ZnO hierarchical nano-structures were analyzed via?eld emission scanning electron microscopy(FE-SEM,4800,Hitachi,Japan)and X-ray diffraction (XRD,Philips Xpert Pro)with Cu K?radiation generated at a volt-age of40kV as source.The photoluminescence(PL)spectrum at room temperature was acquired from360nm to910nm using a 325nm He Cd laser.
2.2.Gas sensor fabrication and characterization
For gas-sensing characterization,the as-obtained SnO2NWs and SnO2/ZnO hierarchical nanostructures were detached from the Si substrate through dispersion in isopropanol under ultrasonic treat-ment and then dried at70?C for24h.The as-obtained materials were mixed with organic binders and pasted on Pt-interdigitated electrodes with an area of800?m×1600?m.A Pt-interdigitated electrode was fabricated using a conventional photolithographic method with a?nger width of20?m and a gap size of20?m.The interdigitated electrodes were fabricated by sputtering10nm Cr and200nm Pt on a layer of silicon dioxide(SiO2)with a thickness of approximately300nm thermally grown on top of the silicon wafer.The gas-sensing characteristics of the SnO2NWs and SnO2/ZnO sen-sors were measured under identical experimental conditions.The gas concentration was controlled by changing the mixing ratio of dry parent gases and dry synthetic air.A?ow-through technique with a constant?ow rate of200sccm was used,employing a pre-viously described homemade system[10].
3.Results and discussion
3.1.Material characterization
Fig.2shows the FE-SEM images of the pristine SnO2NWs and hierarchical SnO2/ZnO nanostructures.The as-synthesized SnO2 NWs had smooth surface of single crystal with an average diameter of approximately100nm and lengths of several tens microme-ters(Fig.2a).The growth mechanism of the SnO2NWs in the present work was explained based on the vapor–liquid–solid mech-anism[32].More details are expounded in our previous works [33,34].Fig.2b–d shows the morphologies of the SnO2/ZnO hier-archical nanostructures after1,2,and4h growth of the ZnO NR branches,respectively.After the hydrothermal growth of ZnO NRs, the ZnO NRs branched out from the smooth SnO2NWs,forming hierarchical structures with SnO2NWs as a backbone and ZnO NRs as branches(Fig.2b).The average diameter of the ZnO NRs was approximately50nm,which was controlled by the size of the ZnO seeds.The length of ZnO NRs increased with increasing hydrothermal growth time.The average length of the ZnO NRs was approximately150,300,and600nm after1,2,and4h of growth, respectively.The growth mechanism of the ZnO NRs on the surface of SnO2NWs was as follows.When a layer of Zn(CH3COO)2solu-tion was sprayed on the surface of the SnO2NWs and heat-treated at300?C,Zn(CH3COO)2was oxidized and crystallized to form ZnO nanoparticles.The ZnO nanoparticles coated on the surface of the SnO2NW backbone played as the seeds for the growth of the ZnO NR branches during the hydrothermal process.In the early state of the hydrothermal process,the ZnO nucleated and grew out on the ZnO seeds,in which the solid ZnO nuclei were formed through the dehydration of Zn(OH)42?(aq)and Zn(NH3)42+(aq)[35].The ZnO crystal was supposed to grow continuously by the conden-sation of the surface hydroxyl groups with the zinc-hydroxyl complexes[35].
XRD analysis was performed to investigate the crystal struc-tures of the SnO2NWs and hierarchical SnO2/ZnO.The results are depicted in Fig.3.The XRD pattern(Fig.3a)of bare SnO2NWs exhib-ited very sharp diffraction peaks because of their high crystallinity. The typical diffraction peaks at2?of27.02?,34.44?,38.52?,and 52.10?were indexed as the(110),(101),(200),and(211)planes of tetragonal rutile SnO2,respectively.All typical diffraction peaks measured in the2?range correspond to the tetragonal structure of SnO2with lattice constants a=4.73?A and c=3.18?A.These peaks are in good agreement with those on the standard card(JCPDS,card no. 41-1445).Fig.3b and c illustrates the XRD patterns of the SnO2/ZnO hierarchical nanostructures at different hydrothermal growth peri-ods(1,2,and4h).The diffraction peak of the ZnO phase was hardly found in the XRD patterns of SnO2/ZnO samples grown for1and2h because of the relatively low amount of ZnO compared with SnO2. However,the diffraction peaks of the SnO2and ZnO phases were found to coexist in the XRD pattern of the SnO2/ZnO hierarchical nanostructures grown for4h.The typical peaks at2?of31.73?and 36.59?were well indexed as the(002)and(101)planes of ZnO, respectively.All these peaks measured in the2?range correspond to the tetragonal structure of ZnO with lattice constants of a=3.25?A and c=5.21?A.These peaks are in good agreement with those on the standard card(JCPDS card no.36-1451).No signi?cant shift was observed in the diffraction peaks.This result indicates that no
596N.D.Khoang et al./Sensors and Actuators B 174 (2012) 594–
601
Fig.1.Experimental steps to prepare the SnO 2/ZnO hierarchical nanostructures:(a)the deposition of Au catalytic layer;(b)the growth of the bare SnO 2nanowires;(c)the decoration of ZnO nanoparticles on the SnO 2nanowires surface;(d)the hydrothermal growth of ZnO nanorods;the actual SEM images of the SnO 2nanowires (e),the nanoparticles-decorated SnO 2nanowires (f),and the SnO 2/ZnO hierarchical nanostructures (h).
interface reaction exists between ZnO and SnO 2for the formation of the Zn 2SnO 4phase.
The optical characteristics of the SnO 2NWs and SnO 2/ZnO hier-archical nanostructures were also studied through PL at room temperature (Fig.4).The PL spectrum of the bare SnO 2NWs (curve 1)exhibited a broad emission peak at a visible region of 620nm
(2.0eV),which was smaller than the band gap width of the SnO 2NWs (3.6eV).Hence,the visible emission peaks cannot be ascribed to the direct recombination of a conduction electron in the Sn4d band and a hole in the O2p valence band.The semiconducting behavior of SnO 2is attributed to the oxygen vacancies in the crys-tal structure,which is also crucial to their optical properties [36]
.
Fig.2.FE-SEM images of the bare SnO 2nanowires (a),the SnO 2/ZnO hierarchical nanostructures grown at 1h (b),2h (c),and 4h (d).
N.D.Khoang et al./Sensors and Actuators B 174 (2012) 594–601
597
SnO 2 JCPDS :46-1088I n t e n i s i t y (a .u .
)
70
656055504540353025202θ(Degree)
Fig.3.The XRD patterns of the bare SnO 2nanowires (a),SnO 2/ZnO hierarchical nanostructures grown at 1h (b),2h (c),and 4h (d).
I n t e n s i t y (a .u .)
I n t e n s i t y (a .u .)
Wavelength (nm)
Fig.4.The PL spectrum of the bare SnO 2nanowires,hierarchical nanostructures
grown at 1
h,2h (c),and 4h (a)and its magni?cation at emission peak at 385nm (b).
a i r /R g a s )
R e s p o n s e (R 253
2
2
Fig.5.The gas response of the bare SnO 2nanowires sensor and the SnO 2/ZnO (grown for 2h)sensors to C 2H 5OH,NH 3,CO,H 2,CO 2,and LPG gases.
Therefore,the emission peak at approximately 620nm is believed
to originate from the luminescence centers formed by tin inter-stitials or dangling bonds in the SnO 2NWs.The oxygen vacancies with high density interact with interfacial tin and from a consid-erable amount of trapped states within the band gap,giving rise to a high PL intensity at room temperature [6].The PL spectra of the SnO 2/ZnO hierarchical nanostructures are also presented in Fig.4,in which curves (2),(3),and (4)correspond to the spec-tra of the ZnO NRs after 1,2,and 4h of growth,respectively.Some differences were found in the PL spectrum of the bare SnO 2NWs.Aside from the emission peak at 620nm,the PL spectra of the SnO 2/ZnO hierarchical nanostructures showed a weak emis-sion peak at 385nm (approximately 3.2eV).This peak could be attributed to the attached ZnO NRs because the PL spectrum of pure ZnO NRs shows emission peaks at approximately 380and 520nm.These peaks correspond to the near band-edge emission and deep-level/trap-state emission,respectively [37].In addition,the intensity of these emission peaks at 385and 620nm increased with increasing length of the ZnO NR branches.
3.2.Gas-sensing properties
The effects of heterogeneous hierarchical structure on the gas-sensing performance of the materials were determined by measuring the bare SnO 2NWs and SnO 2ZnO (2h)sensors with different test gases (C 2H 5OH,NH 3,CO,H 2,CO 2,and LPG)at a ?xed concentration of 100ppm and an operating temperature of 400?C.As shown in Fig.5,the responses (i.e.,R a /R g ,where R a is the resis-tance in air,and R g is the resistance in ethanol gas)of the bare SnO 2NW sensors to C 2H 5OH,NH 3,CO,H 2,CO 2,and LPG were not much different.They were proximately in the range of 1.2–2.2.Meanwhile,the responses of the SnO 2ZnO hierarchical sensors to those gases were larger (i.e.,1.5–6.2).The highest enhancement in response was observed for ethanol gas.This result indicates the potential application of the sensors for screening inebriated drivers.
The gas-sensing performances of the SnO 2/ZnO hierarchical structures are dependent on the length of the ZnO NR branches.Therefore,to obtain the best ethanol-sensing performance of SnO 2/ZnO hierarchical sensors,we measured the response of the bare SnO 2NWs and SnO 2/ZnO sensors.The branched ZnO NRs were grown at different times (i.e.,1,2,and 4h)with ethanol gas (25–500ppm)at an operating temperature of 400?C.The ethanol-sensing transient results are shown in Fig.6,in which the graphs were plotted with the same scale for quickly comparing the sensor response.Apparently,the SnO 2/ZnO hierarchical sensors exhibited better ethanol response than bare SnO 2NWs.All the SnO 2/ZnO hierarchical sensors showed very stable sensing and recovery char-acteristics.The responses to 25–500ppm ethanol gas of SnO 2/ZnO
598N.D.Khoang et al./Sensors and Actuators B 174 (2012) 594–
601
Fig.6.Dynamic sensing response to ethanol gas (25–500ppm)of the bare SnO 2nanowires (a)the SnO 2/ZnO hierarchical nanostructures grown at 1h (b),2h (c),and 4h (d).
hierarchical sensor prepared from the branched ZnO NRs grown for 1,2,and 4h were in the range of 2.3–13.1,3.0–16.2,and 1.7–8.1,respectively.This result suggests that 2h of ZnO branch growth is optimal in designing SnO 2/ZnO hierarchical nanostructures for the best gas-sensing applications.The highest response to 25ppm ethanol of the SnO 2/ZnO hierarchical sensor was approximately 3.This sensor has the capacity to detect ethanol even at lower concen-trations down to sub-ppm level.In practical applications of ethanol sensors to screen intoxicated drivers,the sensor should be able to detect an ethanol concentration of approximately 200ppm,which corresponds to approximately 0.5g of C 2H 5OH per liter of blood [38].Therefore,these results suggest that SnO 2/ZnO hierarchical nanostructure sensors are effective for the enhanced detection of low ethanol gas limits.
The sensor response plotted as a function of ethanol gas con-centration is shown in Fig.7.As shown in the ?gure,the response increased with increasing ethanol gas concentration.In addition,the SnO 2/ZnO hierarchical nanostructures with ZnO NRs grown for 2h clearly exhibited the best response to ethanol gas.Its response to 25–500ppm ethanol was approximately threefold and ?vefold higher than that of bare SnO 2NWs sensors,respectively.This result suggests that the enhancement of the response becomes evident
100
10
a /R g )
1R e s p o n s e (R C 2H 5OH conc. (pp m)
Fig.7.Ethanol response of the bare SnO 2nanowires and the SnO 2/ZnO hierarchical
nanostructures sensors as a function of ethanol gas concentration.
for low and high ethanol gas concentrations.The response of oxide semiconductor gas sensors is usually depicted as [39]:
R g =R a (1+K [C ])?ˇ
(1)
where R g and R a are the sensor’s resistance in ethanol and air,respectively,C is the gas concentration in ppm,and ˇand K are constants.The data of sensor response (S =R a /R g )versus ethanol gas concentration (C )can be expressed as follows:
S =(1+K [C ])ˇ
(2)
The ?tted parameters ˇand K are particularly useful because
they provide meaningful information for comparing sensor per-formances.The sensor’s response switches from zero order to ?rst order when [C ]=K ?1.Therefore,the inverse of K is called the sen-sitivity threshold [39].The power-law exponent ˇis related to the slope of the log–log plot of the sensor response versus ethanol con-centration.Thus,it can be understood as the ability of the sensor to distinguish similar concentrations [39].Table 1shows the ?tting parameters for the observed data presented in Fig.7.Evidently,the SnO 2/ZnO sensor can detect ethanol gas down to a concentration of <10ppm.The exponent ˇof the SnO 2/ZnO sensors was relatively larger than that of the SnO 2NW sensors.This result indicates that the SnO 2/ZnO sensors have better resolution in detecting ethanol gas.
In future studies,the sensitivity and selectivity of hierarchical sensors for sensing particular target gases can be further improved through functionalization with catalytic nanoparticles,such as Pd,Pt,Ag,Au,RuO 2,Co 3O 4,and NiO.
3.3.Gas-sensing mechanism
We proposed a sensing mechanism to explain the enhance-ment of the gas-sensing performance of SnO 2/ZnO hierarchical
Table 1
The ?tted parameters (ˇand K )in expression (2)obtained from experimental data presented in Fig.7.
Sensor
ˇ
1/K
SnO 2NWs
0.51100SnO 2/ZnO (1h)0.605SnO 2/ZnO (2h)0.606SnO 2/ZnO (4h)
0.62
19
N.D.Khoang et al./Sensors and Actuators B174 (2012) 594–601
599
Fig.8.The schematic illustration of the gas sensing mechanism of the bare SnO2nanowires(a)and the SnO2/ZnO hierarchical nanostructures(b)gas sensors.
nanostructures compared with SnO2NWs(Fig.8).The electri-cal current transport from our NWs and hierarchical sensors is performed by percolating the network of the NWs,where the con-ductance between two adjacent electrodes is determined by the availability of the conduction paths through the overlapping NWs [40].The gas-sensing performance of NWs and hierarchical NWs can be detected using two possible mechanisms[40,41].The?rst mechanism is based on the coaxial cable model,whereas the sec-ond mechanism is based on the percolating network theory.In the ?rst mechanism,the electron transport inside the NWs(or NRs) with diameters approximately100nm can be modulated by the electron depletion layer,which surrounds the NW surface and is formed by adsorbing the oxygen molecules on the NWs surface, extracting electrons from the conduction band of the NWs to form oxygen ions(O2?,O?,or O2?).The electron depletion layer is sup-posed to narrow the conducting channel of the NWs.When the sensors are exposed to ethanol gas,the ethanol molecules react to these oxygen ions,release electrons back into the conduction band, and reduce the depletion layer,thereby enhancing the conducting channel.The diameter of NWs plays an important role in gas-sensing performance.NWs with diameters in the range of Debye length(10–20nm for SnO2NWs)exhibit high gas response.For the second mechanism,the percolation network of electrons should pass through potential barriers at junctions between NWs.The potential barriers at the contact points between NWs effectively modulate the electron transport between two adjacent electrodes by adsorbing or desorbing gas molecules[40,41].Therefore,the second mechanism elicits a more effective in?uence on the gas-sensing performance of NW sensors[40].When the sensors are exposed to ethanol gas,the ethanol molecules react with oxygen ion at the junctions,thereby lowering the potential barrier height and enhancing the electron transport through the network NWs[1].Based on these two gas-sensing mechanisms,the ethanol response enhancement of SnO2/ZnO hierarchical nanostructures compared with SnO2NWs can be qualitatively attributed to the addition of SnO2/ZnO hetero-junctions and ZnO/ZnO junctions(Fig.8).These junctions can be considered as additional active sites,resulting in the enhancement of sensor response.
The chemical mechanism should also be taken into account in elucidating the ethanol response enhancement of SnO2/ZnO hierarchical nanostructures.The chemical-sensing mechanism of ethanol gas is related to its decomposition and/or oxidation of ethanol molecules.The decomposition of ethanol molecules at ele-vated temperatures depends on the acid-base properties of sensing materials[42].Given that ZnO is a basic oxide[7,28],SnO2/ZnO hierarchical nanostructures may exhibit more basic properties than SnO2NWs.Therefore,dehydrogenation is favored,as depicted in Eq.(3):
C2H5OH(g)→CH3CHO(g)+H2(g)(basicoxide)(3) The CH3CHO intermediate is subsequently oxidized to form CO2 and H2O,as depicted in Eq.(4):
CH3CHO(ad)+5O
ˉ→2CO
2+2H2O+5e
ˉ(4)
These equations indicate that the electron-donating effect of ethanol gas is stronger than that of the other gases.This phe-nomenon explains why the response to ethanol gas is higher than that to the other gases at equivalent concentration(Fig.5).Besides, the3D hierarchical assembly of ZnO NRs on the core SnO2NWs makes the SnO2/ZnO hierarchical nanostructures more porous, which may also contribute to gas response enhancement.
600N.D.Khoang et al./Sensors and Actuators B174 (2012) 594–601
4.Conclusion
We reported a controllable route for the preparation of SnO2/ZnO hierarchical nanostructures by combining two conven-tional methods.The backbone SnO2NWs were?rst prepared using the thermal evaporation method.The ZnO NR branches were then grown on the SnO2NWs to form a3D hierarchical assem-bly.The gas-sensing characterizations showed that the ethanol gas-sensing performance of SnO2/ZnO hierarchical nanostructures was improved compared with that of the bare SnO2NWs.This improvement in ethanol gas response could be attributed to an additional formation of SnO2/ZnO hetero-junction.The present study may serve as a basis for designing other novel hierarchical nanostructures from oxide NWs,such as In2O3,WO3/ZnO,(p-type) Co3O4/ZnO,and(p-type)CuO/ZnO hierarchical nanostructures for gas-sensing applications.
Acknowledgement
This research is funded by Vietnam National Foundation for Science and Technology Development(NAFOSTED)under grant number103.02-2011.40.
References
[1]Z.L.Wang,Functional oxide nanotubes:materials,properties and potential
application in nanosystems and biotechnology,Annual Review of Physical Chemistry55(2004)159–196.
[2]J.G.Lu,P.Chang,Z.Fan,Quasi-one-dimensional metal oxide materials-
synthesis,properties and applications,Materials Science and Engineering:R 52(2006)49–91.
[3]A.Kolmakov,M.Moskovits,Chemical sensing and catalysis by one-dimensional
metal-oxide nanostructures,Annual Review of Materials Research34(2004) 150–180.
[4]S.H.Ko,D.Lee,H.W.Kang,K.H.Nam,J.Y.Yeo,S.J.Hong,Nanoforest of hydrother-
mally grown hierarchical ZnO nanowires for a high ef?ciency dye-sensitized solar cell,Nano Letters11(2011)666–671.
[5]B.Li,Y.Wang,Facile synthesis and enhanced photocatalytic performance of
?ower-like ZnO hierarchical microstructures,Journal of Physical Chemistry C 114(2010)890–896.
[6]J-.H.Lee,Gas sensors using hierarchical and hollow oxide nanostructures:
overview,Sensors and Actuators B140(2009)319–336.
[7]J.Zhang,S.Wang,M.Xu,Y.Wang,B.Zhu,S.Zhang,W.Huang,S.Wu,Hierar-
chically porous ZnO architectures for gas sensor application,Crystal Growth& Design9(2009)3532–3537.
[8]J.Zhou,Y.Ding,S.Z.Deng,L.Gong,N.S.Xu,Z.L.Wang,Three-dimensional tung-
sten oxide nanowire networks,Advanced Materials17(2005)2107–2110. [9]Q.Wan,J.Huang,Z.Xie,T.Wang,E.N.Dattoli,W.Lu,Branched SnO2nanowires
on metallic backbones for ethanol sensors application,Applied Physics Letters 92(2008)102101–102103.
[10]L.V.Thong,L.T.N.Loan,N.V.Hieu,Comparative study of gas sensor performance
of SnO2nanowires and their hierarchical nanostructures,Sensors and Actuators B112(2010)112–119.
[11]L.Manna,https://www.360docs.net/doc/c512341737.html,liron,A.Meisel,E.C.Scher,A.P.Alivisatos,Controlled growth of
tetrapod-branched inorganic nanocrystals,Nature Materials2(2003)382–385.
[12]D.F.Zhang,L.D.Sun,C.J.Jia,Z.G.Yan,L.P.You,C.H.Yan,Hierarchical assembly
of SnO2nanorod arrays on Fe2O3nanotubes:a case of interfacial lattice com-patibility,Journal of the American Chemical Society127(2005)13492–13493.
[13]N.X.Wang,C.H.Sun,Y.Zhao,S.Y.Zhou,P.Chen,L.Jiang,Fabrication of three-
dimensional ZnO/TiO2,heteroarchitectures via a solution process,Journal of Materials Chemistry18(2008)3909–3911.
[14]https://www.360docs.net/doc/c512341737.html,o,J.G.Wen,Z.F.Ren,Hierarchical ZnO nanostructures,Nano Letters2
(2002)1287–1291.
[15]S.Sen,P.Kanitkar,A.Sharma,K.P.Muthe,A.Rath,S.K.Deshpandee,M.Kaur,R.C.
Aiyer,S.K.Guptaa,J.V.Yakhmi,Growth of SnO2/W18O49nanowire hierarchical heterostructure and their application as chemical sensor,Sensors and Actuators B147(2010)453–460.
[16]C.Cheng,B.Liu,H.Yang,W.Zhou,L.Sun,R.Chen,S.F.Yu,J.Zhang,H.Gong,H.
Sun,H.J.Fan,Hierarchical assembly of ZnO nanostructures on SnO2backbone nanowires:low-temperature hydrothermal preparation and optical properties, ACS Nano3(2009)3069–3076.
[17]W.W.Wang,Y.J.Zhu,L.X.Yang,ZnO–SnO2hollow spheres and hierarchical
nanosheets:hydrothermal preparation,formation mechanism,and photocat-alytic properties,Advanced Functional Materials17(2007)59–64.
[18]X.Jia,H.Fan,Preparation and ethanol sensing properties of the superstructure
SnO2/ZnO,Materials Research Bulletin45(2010)1496–1500.
[19]https://www.360docs.net/doc/c512341737.html,nini,Metal oxide nano-crystals for gas sensing,Analytica Chimica Acta
568(2007)28–40.[20]L.Qin,J.Xu,X.Dong,Q.Pan,Z.Cheng,Q.Xiang,F.Li,The template-free synthe-
sis of square-shaped SnO2nanowires:the temperature effect and acetone gas sensors,Nanotechnology19(2008)185705.
[21]H.-R.Kim,K.-I.Choi,J.-H.Lee,S.A.Akbar,Highly sensitive and ultra-fast
responding gas sensors using self-assembled hierarchical SnO2spheres,Sen-sors and Actuators B136(2009)138–143.
[22]D.Chen,T.Li,L.Yin,R.Zhang,X.Li,Fabrication of hierarchical SnO2nanocrystals
and their sensing to volatile organic compound vapors,Advances in Materials Research266(2011)1–4.
[23]L.-Y.Jiang,X.-L.Wu,Y.-G.Guo,L.-J.Wan,SnO2-based hierarchical nanomi-
crostructures:facile synthesis and their applications in gas sensors and lithium-ion batteries,Journal of Physical Chemistry C113(2009)14213–14219.
[24]N.Zhang,K.Yu,Q.Li,Z.Q.Zhu,Q.Wan,Room-temperature high-sensitivity
H2S gas sensor based on dendritic ZnO nanostructures with macroscale in appearance,Journal of Applied Physics103(2008)104305.
[25]J.Liu,Z.Guo,F.Meng,Y.Jia,T.Luo,M.Li,J.Liu,Novel single-crystalline
hierarchical structured ZnO nanorods fabricated via a wet-chemical route: combined high gas sensing performance with enhanced optical properties, Crystal Growth&Design9(2009)1716–1722.
[26]Y.Zhang,J.Xu,Q.Xiang,H.Li,Q.Pan,P.Xu,Brush-like hierarchical ZnO nano-
structures:synthesis,photoluminescence and gas sensor properties,Journal of Physical Chemistry C113(2009)3430–3435.
[27]S.Ma,R.Li,W.Xu,X.Gou,Facile synthesis of ZnOnanorod arrays and hierar-
chical nanostructures for photocatalysis and gas sensor application,Journal of Hazardous Materials192(2011)730–740.
[28]K.-W.Kim,P.-S.Cho,S.-J.Kim,J.-H.Lee,C.-Y.Kang,J.-S.Kim,S.-J.Yoon,The
selective detection of C2H5OH using SnO2–ZnO thin?lm gas sensors prepared by combinatorial solution deposition,Sensors and Actuators B123(2007) 318–324.
[29]Z.Song,Z.Wang,Y.Liu, C.Wang,L.Li,A highly sensitive ethanol sen-
sor based on mesoporous ZnO–SnO2nano?bers,Nanotechnology20(2009) 075501–075505.
[30]I.-S.Hwang,S.-J.Kim,J.-K.Choi,J.Choi,H.Ji,G.-T.Kim,G.Cao,J.-H.Lee,Synthe-
sis and gas sensing characteristics of highly crystalline ZnO–SnO2core–shell nanowires,Sensors and Actuators B148(2010)595–600.
[31]J.H.Yu,G.M.Choi,Selective CO gas detection of CuO-and ZnO-doped SnO2gas
sensor,Sensors and Actuators B75(2001)56–61.
[32]R.S.Wagner,W.C.Ellis,Vapor–liquid–solid mechanism of single crystal growth,
Applied Physics Letters4(1964)89–90.
[33]N.V.Hieu,L.T.N.Loan,T.Trung,N.D.Chien,A facile thermal evaporation route
for large-area synthesis of tin oxide nanowires:characterizations and their use for liquid petroleum gas sensor,Current Applied Physics10(2010)636–641.
[34]L.V.Thong,N.D.Hoa,D.T.T.Le,D.T.Viet,P.D.Tam,A.T.Le,N.V.Hieu,On-chip
fabrication of SnO2-nanowire gas sensor:the effect of growth time on sensor performance,Sensors and Actuators B146(2010)361–367.
[35]B.Weintrau,Y.Deng,Z.L.Wang,Position-controlled seedless growth of ZnO
nanorod arrays on a polymer substrate via wet chemical synthesis,Journal of Physical Chemistry C111(2007)10162–10165.
[36]W.Wang,C.XU,G.Wang,Y.Liu,C.Zheng,Synthesis and Raman scattering study
of rutile SnO2nanowires,Journal of Applied Physics92(2002)2740–2742. [37]P.Yang,H.Yan,S.Mao,R.Russo,J.Johnson,R.Saykally,N.Morris,J.Pham,R.
He,H.-J.Choi,Controlled growth of ZnO nanowires and their optical properties, Advanced Functional Materials12(2002)323–331.
[38]J.-J.Ho,Y.K.Fang,K.H.Wu,W.T.Hsieh,C.H.Chen,G.S.Chen,M.S.Ju,J.-J.Lin,S.B.
Hwang,High sensitivity and ethanol gas sensor integrated with a solid-state heater and thermal isolation improvement structure of legal drink–drive limit detecting,Sensors and Actuators B50(1998)227–233.
[39]P.K.Clifford,D.T.Tuma,Characteristics of semiconductor gas response.I.Steady
state gas response,Sensors and Actuators B3(83)(1982)233–254.
[40]V.V.Sysoev,J.Goschnick,T.Schneider,E.Strelcov,A.Kolmakov,A gradient
microarray electronic nose based on percolating SnO2nanowires sensing ele-ments,Nano Letters7(2007)3182–3188.
[41]Y.-J.Choi,I.-S.Hwang,J.-G.Park,K.J.Choi,J.-H.Park,J.-H.Lee,Novel fabrica-
tion of an SnO2nanowire gas sensor with high sensitivity,Nanotechnology19 (2008)095508(4pp.).
[42]T.Jinkawa,G.Sakai,J.Tamaki,N.Miura,N.Yamazoe,Relationship between
ethanol gas sensitivity and surface catalytic property of tin oxide sensors mod-i?ed with acidic or basic oxides,Journal of Molecular Catalysis A:Chemical155 (2000)193–200.
Biographies
Nguyen Duc Khoang received his MSc degree in Materials Science at International Training Institute for Material Science(ITIMS),Hanoi University of Science and Tech-nology(HUST),in Vietnam in2007.He is currently pursuing his PhD degree at the ITIMS,where he is working on the synthesis and applications of hybrid materials of metal oxides and carbon nanotubes.
Do Dang Trung received Engineering degree in Chemistry at the Faculty of Chemical Engineering,Hanoi University of Science Technology(HUST),Vietnam,in2004.He received MSc degree in Materials Science at the International Training Institute for Materials Science(ITIMS),HUST,in Vietnam,in October,2010.He is currently pur-suing his PhD degree at ITIMS,where he is working on development of?ammable and explosive gas detectors based on nanomaterials.
N.D.Khoang et al./Sensors and Actuators B174 (2012) 594–601601
Nguyen Van Duy is currently working as a research lecturer at International Training Institute for Material Science(ITIMS),Hanoi University of Science and Technology (HUST).He received PhD degree from the Department of Electrical and Electron-ics Engineering at Sungkyunkwan University,South Korea,in2011.His current research interests include nanomaterials,nanofabrications,characterizations,and applications to electronic devices,gas sensors,and biosensors.
Nguyen Duc Hoa obtained his PhD degree in Materials Science and Engineering in 2009at Chungnam National University in Korea.He awarded JSPS fellowship and conducted the research at National Institute for Materials Science(NIMS,Japan) from2009to2011.His research activity has covered a wide range of nanostructured materials from synthesis,fundamental,and applications.He is the author of several dozens of scienti?c articles published in reputed journals.Currently,he is a lecturer and scientist at Hanoi University of Science and Technology,Vietnam.
Nguyen Van Hieu joined the International Training Institute for Material Science (ITIMS)at Hanoi University of Science and Technology(HUST)in2004,where he is currently associate professor.He received his PhD degree from the Fac-ulty of Electrical Engineering at University of Twente in The Netherlands in2004. He worked as a post-doctoral fellow at the Korea University from2006to2007. His current research interests include functional nanostructures,gas sensors,and biosensors.
如何解析红外光谱图解读
如何解析红外光谱图 一、预备知识 (1)根据分子式计算不饱和度公式: 不饱和度Ω=n4+1+(n3-n1)/2其中: :化合价为4价的原子个数(主要是C原子), n 4 :化合价为3价的原子个数(主要是N原子), n 3 n :化合价为1价的原子个数(主要是H,X原子) 1 (2)分析3300~2800cm-1区域C-H伸缩振动吸收;以3000 cm-1为界:高于3000cm-1为不饱和碳C-H伸缩振动吸收,有可能为烯,炔,芳香化合物;而低于3000cm-1一般为饱和C-H伸缩振动吸收; (3)若在稍高于3000cm-1有吸收,则应在 2250~1450cm-1频区,分析不饱和碳碳键的伸缩振动吸收特征峰,其中炔 2200~2100 cm-1,烯 1680~1640 cm-1 芳环 1600,1580,1500,1450 cm-1若已确定为烯或芳香化合物,则应进一步解析指纹区,即1000~650cm-1的频区,以确定取代基个数和位置(顺、反,邻、间、对); (4)碳骨架类型确定后,再依据官能团特征吸收,判定化合物的官能团; (5)解析时应注意把描述各官能团的相关峰联系起来,以准确判定官能团的存在,如2820,2720和1750~1700cm-1的三个峰,说明醛基的存在。 二、熟记健值 1.烷烃:C-H伸缩振动(3000-2850cm-1)C-H弯曲振动(1465-1340cm-1) 一般饱和烃C-H伸缩均在3000cm-1以下,接近3000cm-1的频率吸收。 2.烯烃:烯烃C-H伸缩(3100~3010cm-1),C=C伸缩(1675~1640 cm-1),烯烃C-H 面外弯曲振动(1000~675cm-1)。 3.炔烃:炔烃C-H伸缩振动(3300cm-1附近),三键伸缩振动(2250~2100cm-1)。 4.芳烃:芳环上C-H伸缩振动3100~3000cm-1, C=C 骨架振动1600~1450cm-1, C-H 面外弯曲振动880~680cm-1。 芳烃重要特征:在1600,1580,1500和1450cm-1可能出现强度不等的4个峰。C-H面外弯曲振动吸收880~680cm-1,依苯环上取代基个数和位置不同而发生变化,在芳香化合物红外谱图分析中,常用判别异构体。
红外谱图解析基本知识
红外谱图解析基本知识 基团频率区 中红外光谱区可分成4000 cm-1 ~1300(1800)cm-1和1800 (1300 )cm-1 ~ 600 cm-1两个区域。最有分析价值的基团频率在4000 cm-1 ~ 1300 cm-1 之间,这一区域称为基团频率区、官能团区或特征区。区内的峰是由伸缩振动产生的吸收带,比较稀疏,容易辨认,常用于鉴定官能团。 在1800 cm-1 (1300 cm-1 )~600 cm-1 区域内,除单键的伸缩振动外,还有因变形振动产生的谱带。这种振动基团频率和特征吸收峰与整个分子的结构有关。当分子结构稍有不同时,该区的吸收就有细微的差异,并显示出分子特征。这种情况就像人的指纹一样,因此称为指纹区。指纹区对于指认结构类似的化合物很有帮助,而且可以作为化合物存在某种基团的旁证。 基团频率区可分为三个区域 (1) 4000 ~2500 cm-1 X-H伸缩振动区,X可以是O、N、C或S等原子。 O-H基的伸缩振动出现在3650 ~3200 cm-1 范围内,它可以作为判断有无醇类、酚类和有机酸类的重要依据。 当醇和酚溶于非极性溶剂(如CCl4),浓度于0.01mol. dm-3时,在3650 ~3580 cm-1 处出现游离O-H基的伸缩振动吸收,峰形尖锐,且没有其它吸收峰干扰,易于识别。当试样浓度增加时,羟基化合物产生缔合现象,O-H基的伸缩振动吸收峰向低波数方向位移,在3400 ~3200 cm-1 出现一个宽而强的吸收峰。 胺和酰胺的N-H伸缩振动也出现在3500~3100 cm-1 ,因此,可能会对O-H伸缩振动有干扰。 C-H的伸缩振动可分为饱和和不饱和的两种: 饱和的C-H伸缩振动出现在3000 cm-1以下,约3000~2800 cm-1 ,取代基对它们影响很小。如-CH3 基的伸缩吸收出现在2960 cm-1和2876 cm-1附近;R2CH2基的吸收在2930 cm-1 和2850 cm-1附近;R3CH基的吸收基出现在2890 cm-1 附近,但强度很弱。 不饱和的C-H伸缩振动出现在3000 cm-1以上,以此来判别化合物中是否含有不饱和的C-H键。 苯环的C-H键伸缩振动出现在3030 cm-1附近,它的特征是强度比饱和的C-H浆键稍弱,但谱带比较尖锐。 不饱和的双键=C-H的吸收出现在3010~3040 cm-1范围内,末端= CH2的吸收出现在3085 cm-1附近。 叁键oCH上的C-H伸缩振动出现在更高的区域(3300 cm-1 )附近。 (2) 2500~1900 cm-1为叁键和累积双键区,主要包括-CoC、-CoN等叁键的伸缩振动,以及-C =C=C、-C=C=O等累积双键的不对称性伸缩振动。 对于炔烃类化合物,可以分成R-CoCH和R¢-C oC-R两种类型: R-CoCH的伸缩振动出现在2100~2140 cm-1附近; R¢-C oC-R出现在2190~2260 cm-1附近; R-C oC-R分子是对称,则为非红外活性。 -C oN 基的伸缩振动在非共轭的情况下出现2240~2260 cm-1附近。当与不饱和键或芳香核共轭时,该峰位移到2220~2230 cm-1附近。若分子中含有C、H、N原子,-C oN基吸收比较强而尖锐。若分子中含有O原子,且O原子离-C oN基越近,-C oN基的吸收越弱,甚至观察不到。
纳米氧化锌的奇妙颜色
纳米氧化锌的奇妙颜色 --作者冯铸(高级工程师,工程硕士宝鸡天鑫工业添加剂有限公司销售经理) 纳米级活性氧化锌有多种生产方式,而每种生产方式及各个生产方式的工艺差别的不同,使得最终产品的颜色不同,即呈现微黄色的程度不同。 一、物质颜色的由来 物质的颜色都是其反光的结果。白光是混合光,由各种色光按一定的比例混合而成。如果某物质在白光的环境中呈现黄色(比如纳米氧化锌),那是因为此物体吸收了部分或者全部的蓝色光。物质的颜色是由于其对不同波长的光具有选择性吸收作用而产生的。 不同颜色的光线具有不同的波长,而不同的物质会吸收不同波长的色光。物质也只能选择性的吸收那些能量相当于该物质分子振动能变化、转动能变化及电子运动能量变化的总和的辐射光。换句话说,即使是同一物质,若其内能处在不同的能级,其颜色也会不同。比如氧化锌,不论是普通形式的,还是纳米形式的,高温时颜色均很黄,温度降低时颜色变浅。原因在于在不同温度时,氧化锌的分子能及电子能的跃迁能量不同,因此,对各种色光的吸收不同。 二、粗颗粒的氧化锌与纳米氧化锌的结构区别,及由此导致的分子内能差异 粗颗粒的直接法或间接法氧化锌是离子晶体。通常来说,锌原子与氧原子以离子键形式存在。由于其颗粒较粗,每个颗粒中氧原子与锌原子的数量相当多,而且两种原子的数量是一样的(按分子式ZnO看,是1:1)。但对于纳米氧化锌,其颗粒相当细,使得颗粒表面的未成键的原子数目大增。也就是说,纳米氧化锌不能再看成具有无限多理想晶面的理想晶体,在其表面,会有无序的晶间结构及晶体缺陷存在。表面这些与中心部分不同的原子的存在,使得其具有很强的与其他物质反应的能力,也就是我们通常所说的活性。 研究表明:在纳米氧化锌中,至少存在三种状态的氧,他们是晶格氧(位于颗粒内部)、表面吸附氧及羟基氧(--OH),而且,颗粒中锌的数量大于氧的数量,不是1:1的状况。这一点与普通氧化锌完全不同。纳米氧化锌的表面存在氧空缺,有许多悬空键,易于与其他原子结合而发生反应,这也是纳米氧化锌在橡胶中、催化剂中作为活性剂应用的基本原理。 由于纳米氧化锌与普通氧化锌的上述不同。使得其颗粒中分子能及电子能的跃迁变化能级不同,因此,其颜色也不同。普通氧化锌是白色,而纳米氧化锌是微黄色。 三、纳米氧化锌随时间及环境湿度变化,其颜色的变化 对于纳米氧化锌,由于其颗粒表面存在吸附氧及羟基氧,而这两种氧的数量会随着时间的变化而发生变化,比如水分的吸附及空气中氧气的再吸附与剥离等。这两种氧的数量的变化,必然会引起颗粒中分子及电子能级的变化,对光的吸收也不相同,因此,纳米氧化锌的颜色变浅。 四、纳米氧化锌的颜色与纯度的关系 纯的纳米氧化锌,其颜色是纯微黄的,显得色泽很亮。 当纳米氧化锌含杂质,如铁、锰、铜、镉等到了一定程度,会使氧化锌的颜色在微黄色中带有土色的感觉,那是因为铁、锰、铜、镉等的氧化物均为有色物质,相互混合后,几种色光交混,显出土白色。而纳米氧化锌(或者活性氧化锌,轻质氧化锌)随着时间变化而发生的颜色变化,会被土色所掩盖,而使颜色显得变化极小;当纳米氧化锌中含杂质再高时,其颜色会变得很深,更无法观测到其颜色随时间变化的情况。 如前所述,物质的颜色是其对外界光线选择性的吸收引起的。因此,在我们比较氧化锌的颜色时,最好在户外光亮的地方观察比较确切。选择不同的环境做比较,会得到不同的比较结果,这也体现了光反射的趣味性。 五、关于纳米氧化锌颜色的另外一种解释 纳米氧化锌是经碱式碳酸锌煅烧而得。在此过程中,如果碱式碳酸锌未能完全分解,纳米氧化锌的颜色就会显得白一些,因为碱式碳酸锌为纯白色。此外,在南方与北方生产,或在潮湿的雨天与干燥的天气下生产,也会影响颜色。因为纳米氧化锌可与湿空气及二氧化碳反应生成碱式碳酸锌,发生了煅烧过程的逆反应。这种变化对产品质量的影响有多大,现在尚难断定,因为碱式碳酸锌本身也是具有催化作用的,适于在脱硫剂及橡胶行业使用;而在饲料行业,碱式碳酸锌具有与氧化锌同样的功能,它也是一种饲料添加剂,同时,在饲料行业,我们关心的问题主要是重金属的含量是否达到标准要求。
常见高分子红外光谱谱图解析
常见高分子红外光谱谱图解析1. 红外光谱的基本原理 1)红外光谱的产生 能量变化 ν νhc h= = E - E = ?E 1 2 ν ν h ?E = 对于线性谐振子 μ κ π ν c 2 1 = 2)偶极矩的变化 3)分子的振动模式 多原子分子振动 伸缩振动对称伸缩 不对称伸缩 变形振动AX2:剪式面外摇摆、面外扭摆、面内摇摆 AX3:对称变形、反对称变形 . 不同类型分子的振动 线型XY2: 对称伸缩不对称伸缩 弯曲
弯曲型XY2: 不对称伸缩对称伸缩面内弯曲(剪式) 面内摇摆面外摇摆卷曲 平面型XY3: 对称伸缩不对称伸缩面内弯曲 面外弯曲 角锥型XY3: 对称弯曲不对称弯曲
面内摇摆 4)聚合物红外光谱的特点 1、组成吸收带 2、构象吸收带 3、立构规整性吸收带 4、构象规整性吸收带 5、结晶吸收带 2 聚合物的红外谱图 1)聚乙烯 各种类型的聚乙烯红外光谱非常相似。在结晶聚乙烯中,720 cm-1的吸收峰常分裂为双峰。要用红外光谱区别不同类型的聚乙烯,需要用较厚的薄膜测绘红外光谱。这些光谱之间的差别反映了聚乙烯结构与线性—CH2—链之间的差别,主要表现在1000-870㎝-1之间的不饱和基团吸收不同,甲基浓度不同以及在800-700㎝-1之间支化吸收带不同。
低压聚乙烯(热压薄膜) 中压聚乙烯(热压薄膜) 高压聚乙烯(热压薄膜)
2.聚丙烯 无规聚丙烯
等规聚丙烯的红外光谱中,在1250-830 cm-1区域出现一系列尖锐的中等强度吸收带(1165、998、895、840 cm-1)。这些吸收与聚合物的化学结构和晶型无关,只与其分子链的螺旋状排列有关。 3.聚异丁烯 CH3 H2 C C n CH3
氧化锌纳米材料简介
目录 摘要 (1) 1.ZnO材料简介 (1) 2.ZnO材料的制备 (1) 2.1 ZnO晶体材料的制备 (1) 2.2 ZnO纳米材料的制备 (2) 3. ZnO材料的应用 (3) 3.1 ZnO晶体材料的应用 (3) 3.2 ZnO纳米材料的应用 (5) 4.结论 (7) 参考文献 (9)
氧化锌材料的研究进展 摘要介绍了氧化锌(ZnO)材料的性质,简单综述一下近几年ZnO周期性晶体材料和ZnO纳米材料的新进展。 关键词:ZnO;晶体材料;纳米材料 1.ZnO材料简介 氧化锌材料是一种优秀的半导体材料。难溶于水,可溶于酸和强碱。作为一种常用的化学添加剂,ZnO广泛地应用于塑料、硅酸盐制品、合成橡胶、润滑油、油漆涂料、药膏、粘合剂、食品、电池、阻燃剂等产品的制作中。ZnO的能带隙和激子束缚能较大,透明度高,有优异的常温发光性能,在半导体领域的液晶显示器、薄膜晶体管、发光二极管等产品中均有应用。此外,微颗粒的氧化锌作为一种纳米材料也开始在相关领域发挥作用。纳米ZnO粒径介于1-100nm之间,是一种面向21世纪的新型高功能精细无机产品,表现出许多特殊的性质,如非迁移性、荧光性、压电性、吸收和散射紫外线能力等,利用其在光、电、磁、敏感等方面的奇妙性能,可制造气体传感器、荧光体、变阻器、紫外线遮蔽材料、图像记录材料、压电材料、压敏电阻、高效催化剂、磁性材料和塑料薄膜等[1–5]。下面我们简单综述一下,近几年ZnO周期性晶体材料和ZnO纳米材料的新进展。 2.ZnO材料的制备 2.1 ZnO晶体材料的制备 生长大面积、高质量的ZnO晶体材料对于材料科学和器件应用都具有重要意义。尽管蓝宝石一向被用作ZnO薄膜生长的衬底,但它们之间存在较大的晶格失配,从而导致ZnO外延层的位错密度较高,这会导致器件性能退化。由于同质外延潜在的优势,高质量大尺寸的ZnO晶体材料会有利于紫外及蓝光发射器件的制作。由于具有完整的晶格匹配,ZnO同质外延在许多方面具有很大的潜力:能够实现无应变、没有高缺陷的衬底-层界面、低的缺陷密度、容易控制材料的极性等。除了用于同质外延,ZnO晶体
Ag ZnO纳米复合材料的制备
运城学院应用化学系 文献综述 Ag/ZnO纳米复合材料的制备 学生姓名王新光 学号2010080412 专业班级应用化学1004班 批阅教师 成绩 2013年06月
Ag/ZnO纳米复合材料的制备 1. 研究背景 纳米技术是一门交叉性很强的综合学科,研究的内容涉及现代科技的广阔领域。纳米科技现在已经包括纳米生物学、纳米电子学、纳米材料学、纳米机械学、纳米化学等学科。从包括微电子等在内的微米科技到纳米科技,人类正越来越向微观世界深入,人们认识、改造微观世界的水平提高到前所未有的高度。我国著名科学家钱学森也曾指出,纳米左右和纳米以下的结构是下一阶段科技发展的一个重点,会是一次技术革命,从而将引起21世纪又一次产业革命。 2.制备方法 2.1采用沉淀法制备 周广、邓建成、王升文[1]采用配位均匀共沉淀法制备了平均粒径约为20 nm的Ag/ZnO纳米复合材料。利用XRD、TEM及UV-Vis等技术对样品进行了表征,并将其与用浸渍光分解法和光还原沉积法制备的样品在形貌结构及催化降解甲基橙溶液和工业废水性能方面进行了比较。结果表明,采用配位均匀共沉淀法制备的样品,表现出更加优异的催化降解性能。 庹度[2]采用沉淀法制备了纳米氧化锌,并以它为前驱物,采用高温分解法对纳米氧化锌进行了载银改性处理,制备了载银氧化锌复合纳米粒子,考察了载银前后纳米粒子的粒径与结构。研究发现,采用沉淀法制备的纳米氧化锌尺寸较为均匀,粒径约为170nm,分散性也较好;载银后的复合纳米粒子粒径略有增加,这来源于银在纳米氧化锌粒子外的成功包覆。 斯琴高娃、照日格图、姚红霞、嘎日迪[3]以ZnCl2.2H2O和无水(NH4)2CO3为原料,采用直接沉淀法制备了纳米氧化锌.TG-DTG-DTA、IR分析结果表明,前驱体为碱式碳酸锌[Zn5(OH)6(CO3)2].前驱体经300℃煅烧1 h、2 h、3 h后分别得到粒径不
植物生长素的作用机理
植物生长素的作用机理 陶喜斌 2014310218 种子科学与工程
摘要;经过多位科学家的研究,发现了与植物生长有关的重要激素——生长素。生长素在植物芽的生长,根的生长,果实的生长,种子休眠等方面有重要作用。那么,生长素是如何发挥这这些作用? 1;什么是生长素 生长素(auxin)是一类含有一个不饱和芳香族环和一个乙酸侧链的内源激素,英文简称IAA,国际通用,是吲哚乙酸(IAA;。4-氯-IAA、5-羟-IAA、萘乙酸(NAA)、吲哚丁酸等为类生长素。1872年波兰园艺学家谢连斯基对根尖控制根伸长区生长作了研究~后来达尔文父子对草的胚芽鞘向光性进行了研究。1928年温特证实了胚芽的尖端确实产生了某种物质,能够控制胚芽生长。1934年, 凯格等人从一些植物中分离出了这种物质并命名它为吲哚乙酸,因而习惯上常把吲哚乙酸作为生长素的同义词。 2;植物生长素的生理作用 生长素有多方面的生理效应,这与其浓度有关。低浓度时可以促进生长,高浓度时则会抑制生长,甚至使植物死亡,这种抑制作用与其能否诱导乙烯的形成有关。生长素的生理效应表现在两个层次上。 在细胞水平上,生长素可刺激形成层细胞分裂~刺激枝的细胞伸长、抑制根细胞生长~促进木质部、韧皮部细胞分化,促进插条发根、调节愈伤组织的形态建成。 在器官和整株水平上,生长素从幼苗到果实成熟都起作用。生长素控制幼苗中胚轴伸长的可逆性红光抑制~当吲哚乙酸转移至枝条下侧即产生枝条的向地性~当吲哚乙酸转移至枝条的背光侧即产生枝条的向光性~吲哚乙酸造成顶端 优势~延缓叶片衰老~施于叶片的生长素抑制脱落,而施于离层近轴端的生长素促进脱落~生长素促进开花,诱导单性果实的发育,延迟果实成熟。 生长素对生长的促进作用主要是促进细胞的生长,特别是细胞的伸长。植物感受光刺激的部位是在茎的尖端,但弯曲的部位是在尖端的下面一段,这是因为尖端的下面一段细胞正在生长伸长,是对生长素最敏感的时期,所以生长素对其生长的影响最大。趋于衰老的组织生长素是不起作用的。生长素能够促进果实的发育和扦插的枝条生根的原因是;生长素能够改变植物体内的营养物质分配,在生长素分布较丰富的部分,得到的营养物质就多,形成分配中心。生长素能够诱 导无籽番茄的形成就是因为用生长素处理没有受粉的番茄花蕾后,番茄花蕾的子房就成了营养物质的分配中心,叶片进行光合作用制造的养料就源源不断地运到子房中,子房就发育了。 生长素在植物体作用很多,具体有;1.顶端优势 2.细胞核分裂、细胞纵向伸长、细胞横向伸长3.叶片扩大4.插枝发根5.愈伤组织6.抑制块根7.气孔开放8.延长休眠9.抗寒 3;生长素的作用机理 3.1生长素作用机理的解释 激素作用的机理有各种解释,可以归纳为二; 一、是认为激素作用于核酸代谢,可能是在DNA转录水平上。它使某些基因活化,形成一些新的mRNA、新的蛋白质(主要是酶;,进而影响细胞内的新陈代谢,引起生长发育的变化。 二、则认为激素作用于细胞膜,即质膜首先受激素的影响,发生一系列膜结构与功能的变化,使许多依附在一定的细胞器或质膜上的酶或酶原发生相应
纳米氧化锌的研究进展
学号:201140600113 纳米氧化锌的制备方法综述 姓名:范丽娜 学号: 201140600113 年级: 2011级 院系:应用化学系 专业:化学类
纳米氧化锌的制备方法综述 姓名:范丽娜学号: 201140600113 内容摘要:介绍了纳米氧化锌的应用前景及国内外的研究现状,对制 备纳米氧化锌的化学沉淀法、溶胶凝胶法、微乳液法、水热合成法、 化学气相法的基本原理、影响因素、产物粒径大小,操作过程等进行 了详细的分析讨论;提出了每种创造工艺的优缺点,指出其未来的研 究方向是生产具有新性能、粒径更小、大小均一、形貌均可调控、生 产成本低廉的纳米氧化锌。同时也有纳米氧化锌应用前景的研究。 Describes the application of zinc oxide prospects and research status, on the preparation of ZnO chemical precipitation, sol-gel method, microemulsion, hydrothermal synthesis method, chemical vapor of the basic principles, factors, product particle size, operating procedure, carried out a detailed analysis and discussion; presents the advantages and disadvantages of each creation process, pointing out its future research direction is the production of new properties, particle size is smaller, uniform size, morphology can be regulated, production cost of zinc oxide. There is also promising research ZnO. 关键字:纳米氧化锌制备方法影响研究展望 正文:纳米氧化锌是一种多功能性的新型无机材料,其颗粒大小约在1~100纳米。由于晶粒的细微化,其表面电子结构和晶体结构发生 变化,产生了宏观物体所不具有的表面效应、体积效应、量子尺寸效 应和宏观隧道效应以及高透明度、高分散性等特点。近年来发现它在
红外图谱解析
红外图谱解析 首先应该对各官能团的特征吸收熟记于心,因为官能团特征吸收是解析谱图的基础。 对一张已经拿到手的红外谱图: (1)首先依据谱图推出化合物碳架类型:根据分子式计算不饱和度,公式: 不饱和度=F+1+(T-O)/2 其中: F:化合价为4价的原子个数(主要是C原子), T:化合价为3价的原子个数(主要是N原子), O:化合价为1价的原子个数(主要是H原子), F、T、O分别是英文4,3,1的首字母。 举个例子:比如苯:C6H6,不饱和度=6+1+(0-6)/2=4,3个双键加一个环,正好为4个不饱和度; (2)分析3300~2800cm-1区域C-H伸缩振动吸收;以3000 cm-1为界:高于3000cm-1为不饱和碳C-H伸缩振动吸收,有可能为烯, 炔, 芳香化合物,而低于3000cm-1一般为饱和C-H 伸缩振动吸收; (3)若在稍高于3000cm-1有吸收,则应在2250~1450cm-1频区,分析不饱和碳碳键的伸缩振动吸收特征峰,其中: 炔2200~2100 cm-1 烯1680~1640 cm-1 芳环1600,1580,1500,1450 cm-1 若已确定为烯或芳香化合物,则应进一步解析指纹区,即1000~650cm-1的频区,以确定取代基个数和位置(顺反,邻、间、对); (4)碳骨架类型确定后,再依据其他官能团,如C=O, O-H, C-N 等特征吸收来判定化合物的官能团; (5)解析时应注意把描述各官能团的相关峰联系起来,以准确判定官能团的存在,如2820,2720和1750~1700cm-1的三个峰,说明醛基的存在。 解析的过程基本就是这样吧,至于制样以及红外谱图软件的使用,一般的有机实验书上都有比较详细的介绍的,这里就不唠叨了。 这是一个令人头疼的问题,有事没事就记一两个吧: 1.烷烃:C-H伸缩振动(3000-2850cm-1) C-H弯曲振动(1465-1340cm-1)
生长素的作用机理
生长素的作用机理 生长素是发现最早的一类植物激素也是植物五大类激素中的一种.它参与着植物体内很多的生理作用如细胞的伸长生长、形成层的细胞分裂、维管组织的分化、叶片和花的脱落、顶端优势、向性、生根和同化物的运输等。所以研究生长素的作用机理对认识植物生长发育的许多生理过程有着不可估量的意义。 目前对激素作用的机理有各种解释,可以归纳为二:一是认为激素作用于核酸代谢,可能是在DNA转录水平上。它使某些基因活化,形成一些新的mRNA、新的蛋白质(主要是酶),进而影响细胞内的新陈代谢,引起生长发育的变化。另一则认为激素作用于细胞膜,即质膜首先受激素的影响,发生一系列膜结构与功能的变化,使许多依附在一定的细胞器或质膜上的酶或酶原发生相应的变化,或者失活或者活化。酶系统的变化使新陈代谢和整个细胞的生长发育也随之发生变化。此外,还有人认为激素对核和质膜都有影响;或认为激素的效应先从质膜再经过细胞质,最后传到核中。 虽然对激素作用机理有不同的解释,但是,无论哪一种解释都认为,激素必须首先与细胞内某种物质特异地结合,才能产生有效的调节作用。这种物质就是激素的受体。生长素作用于细胞时,首先与受体结合。经过一系列过程,引起细胞壁介质酸化和影响蛋白质合成,最终导致细胞的变化。 1.生长素受体结合蛋白(ABP1) ABP包括位于内质网膜上的ABP-I、可能位于液泡膜上的ABP-∏、位于质膜上ABP -III 以及生长素运输抑制剂 N1-naphthylp- hthalamic acid(NPA)和2,3,5一三碘苯甲酸(TIBA)的结合蛋白4类。 内质网上的ABP1合成后运输到细胞质膜上发挥生长素受体作用。生长素与细胞质膜上的ABP1结合后,钝化的坞蛋白转变为活性状态,并进一步激活质子泵将膜内H+泵到膜外,引起质膜的超极化,胞壁松弛,于是引起细胞的生长反应。内质网上的ABP1可能只是起贮藏库的作用。由于发育或其他信号引起的质膜上ABP1量的改变是通过内质网上的ABP1输出增加或减少调节的。由此可见,ABP1的分布和数量可以调节IAA功能的行使。 研究还发现,各种植物的ABP基因结构相似,编码的前体蛋白都具有主要的功能性结构序列。在氨基末端有一疏水信号序列,利于ABP在内质网膜间的穿透和转移,起信号转导作用;在羧基末端的KDEL四肽结构则使得ABP定位于内质网中的特定区域。研究认为,ABP1是一个同型二聚体糖蛋白,其亚基由163个氨基酸残基组成。如玉米的ABP1由3个组氨酸残基和1个谷氨酸残基组成1个结合部位,内含1个金属阳离子,这个部位极其疏水。在第2和第5位的半胱氨酸残基间还有1个二硫键,当生长素结合到这个部位时,羧酸酯与金属离子结合,而芳香环则与第151位的色氨酸残基等疏水性氨基酸残基结合。对ABP1羧基末端高度保守的氨基酸残基作定点突变时,发现第177位的半胱氨酸残基、第175位的天冬氨酸残基和第176位的谷氨酸残基是ABP1折叠和在质膜上起作用的重要残基,ABP1构象变化引发质膜信号传递。 2.信号转导 生长素信号传导分为两条主要途径:(1)质膜上的生长素结合蛋白(ASP)可能起接收细胞外生长素信号的作用,并将细胞外信号向细胞内传导.从而诱导细胞伸长。2)细胞中存在的细胞液/细胞核可溶性结合蛋白(SABP)与生长素结合,在转录和翻译水平上影响基因表达。生长素要引发细胞内的生化反应和特定基因表
纳米氧化锌的表面改性
文章编号:1005-7854(2004)02-0050-03 纳米氧化锌的表面改性 马正先 1,2 ,韩跃新2,印万忠2,王泽红2,袁致涛2,于富家2,马云东 3 (11济南大学,济南250022;21东北大学,沈阳110004;31辽宁工程技术大学,阜新123000) 摘 要:在新开发的纳米氧化锌应用中,大多是将氧化锌直接混入有机物中,而把氧化锌直接添加到 有机物中有相当大的困难,因此必须对纳米氧化锌进行表面改性。以自制纳米氧化锌为原料,采用钛酸酯偶联剂为改性剂对其进行了表面改性处理。试验发现,改性剂用量是影响改性效果的最重要影响因素,且其用量远远超出普通粉体用量,最后找出了最佳改性条件。借助于T EM 、IR 等测试手段,对纳米氧化锌粉体改性前后的变化进行了表征与分析。试验结果表明,最佳改性条件为:改性剂用量为40%,改性时间约为30min 。 关键词:纳米氧化锌;表面改性;红外光谱;钛酸酯偶联剂中图分类号:TB383 文献标识码:A SU RFACE M ODIFICA T ION OF N ANOM ET ER -SIZED ZINC OXIDE MA Zheng -x ian 1,2,HAN Yue -x in 2,YIN Wan -z hong 2,WANG Ze -hong 2, Y UAN Zhi -tao 2,Y U Fu -j ia 2,MA Yun -dong 3 (11Jinan University ,Jinan 250022,China;2.Northeaster n Univer sity ,Shengy ang 110004,China; 31L iaoning Technical University ,Fux in 123000,China) ABSTRAC T:In application of new ly prepared nano -sized zinc ox ide,it is directly added into organic compound mostly,w hich is difficult comparatively.So,it is indispensable that surface modification of nano -sized zinc ox ide is done.The tests on surface modification of sel-f made nano -sized zinc oxide w ere carried out w ith titanate as cou -pling agent.Results indicate that the use level of coupling agent is the most important factor to influence the modification and its dosage is w ell over that needed for common pow der.By m eans of IR and TEM ,unmodified and modified nano -sized zinc oxides are investigated and the optimal modifying conditions are the agent dosage of 40%and modifying time of about 30min. KEY WORDS:Nano -sized zinc ox ide;Surface modification;IR -spectrum ;T itanate coupling agent 收稿日期:2003-09-05 基金项目:国家自然科学基金项目(50374021) 作者简介:马正先,机械学院副教授、博士,主要从事粉体制备 与处理及其设备的研究。 1 引 言 氧化锌的用途十分广泛,主要用于橡胶、油漆、涂料、印染、玻璃、医药、化工和陶瓷等工业112。纳米氧化锌因其全新的纳米特性体现出许多新的物理化学性能,使它在众多领域表现出巨大的应用前景。纳米氧化锌除了作为微米级或亚微米级氧化锌的替 代产品外,在抗菌添加剂、防晒剂、催化剂与光催化剂、气体传感器、图像记录材料、吸波材料、导电材料、压电材料、橡胶添加剂等新的应用场合也正在或 即将投入应用12-62。在这些应用过程中,大多是与有机物相混的,而氧化锌作为无机物直接添加到有机物中有相当大的困难:1颗粒表面能高,处于热力学非稳定状态,极易聚集成团,从而影响了纳米颗粒的实际应用效果;o氧化锌表面亲水疏油,呈强极性,在有机介质中难于均匀分散,与基料之间没有结合力,易造成界面缺陷,导致材料性能下降。所以,必须对纳米氧化锌进行表面改性,以消除表面高能 第13卷 第2期2004年6月 矿 冶M INING &M ET ALLURGY Vol.13,No.2 June 2004
红外谱图的解析
红外谱图的解析经验 (1)首先依据谱图推出化合物碳架类型:根据分子式计算不饱和度,公式:不饱和度=F+1+(T-O)/2 (2) 分析3300-2800区域C-H伸缩振动吸收;以3000 为界:高于3000为不饱和碳C-H伸缩振动吸收,有可能为烯,炔,芳香化合物,而低于3000一般为饱和C-H伸缩振动吸收; (3)若在稍高于3000有吸收,则应在 2250-1450频区,分析不饱和碳碳键的伸缩振动吸收特征峰,其中:炔 2200-2100,烯 1680-1640,芳环 1600,1580,1500,1450,若已确定为烯或芳香化合物,则应进一步解析指纹区,即1000-650的频区 ,以确定取代基个数和位置(顺反,邻、间、对); (4)碳骨架类型确定后,再依据其他官能团,如 C=O,O-H,C-N 等特征吸收来判定化合物的官能团; (5)解析时应注意把描述各官能团的相关峰联系起来,以准确判定官能团的存在,如2820,2720和1750-1700的三个峰,说明醛基的存在。 1、烷烃:C-H伸缩振动(3000-2850) C-H弯曲振动(1465-1340),一般饱和烃C-H伸缩均在3000以下,接近3000的频率吸收。 2、烯烃:烯烃C-H伸缩(3100-3010) C=C伸缩(1675-1640) 烯烃C-H面外弯曲振动(1000-675)。 3、炔烃:伸缩振动(2250-2100) 炔烃C-H伸缩振动(3300附近)。 4、芳烃:3100-3000, 芳环上C-H伸缩振动 1600-1450, C=C 骨架振动 880-680C-H。 芳香化合物重要特征:一般在1600,1580,1500和1450,可能出现强度不等的4个峰。 880-680,C-H面外弯曲振动吸收,依苯环上取代基个数和位置不同而发生变化 ,在芳香化合物红外谱图分析中,常常用此频区的吸收判别异构体。 5、醇和酚:主要特征吸收是O-H和C-O的伸缩振动吸收, O-H 自由羟基O-H的伸缩振动:3650-3600,为尖锐的吸收峰, 分子间
高中生物生长素的生理作用 教材分析 新课标 人教版 必修3精编版
生长素的生理作用教材分析 要点提炼 一、生长素的生理作用 1.两重性 生长素在植物体内起作用的方式和动物体内激素相似,它不直接参与细胞代谢,而是给 细胞传递一种调节代谢的信息,即促进细胞的伸长。研究发现,对于同一器官而言,生长素 对其作用具有两重性,即低浓度的生长素促进生长,高浓度的生长素抑制生长。浓度的高、 低是以生长素最适浓度为界划分的,低于最适浓度为“低浓度”,高于最适浓度为“高浓度”。 在低浓度范围内,浓度越高促进生长的效果越明显;高浓度范围内浓度越高,促进效果越差, 甚至抑制生长。由于不同的器官其生长素的最适浓度不同,所以同一浓度的生长素作用于不 同器官,其生长素的最适浓度不同。如10-6mol/L时,对茎有促进作用,对芽和根则起抑制 作用。所以生长素发挥作用因浓度、植物细胞的成熟情况和器官种类不同而有较大差异。一 般情况下,生长素浓度低时促进生长,浓度过高时则会抑制生长,甚至杀死植物。幼嫩的细 胞对生长素敏感,老细胞则比较迟钝。生长素的作用表现出两重 性:既能促进生长,又能抑制生长;既能促进发芽,又能抑制发芽;既能防止落花落果,又 能疏花疏果。 2.顶端优势 (1)观察猜想——顶端优势的原因 很早以前,人们注意到很多植物的顶芽生长很快,越接近顶芽的侧芽发育越慢,远离顶 芽的侧芽发育成侧枝,甚至使整个植物体呈现“塔”形,科学家就把这种顶芽优先生长,侧芽 生长受抑制的现象,称为顶端优势。是什么原因导致顶端优势呢?一位科学家做过一个小实 验:取各种情况基本相同的蚕豆苗,随机分成三组,第一组不作处理,第二组去除顶芽,在 切口处放一富含生长素的琼脂块,第三组去除顶芽,切口处放一块不含生长素的琼脂块。请 你猜想一下,各组的实验结果是什么?该实验能说明顶端优势的原因是什么?顶端优势实验结果:第一组蚕豆保持顶端优势;第二组蚕豆保持顶端优势;第三组侧芽发育,顶 端优势解除。 分析:比较第二、三组可以看出侧芽是否发育,顶端优势是否解除,关键取决于是否不 断地向侧芽运输生长素。综合分析以上三组实验可以总结出顶端优势的原因:顶芽产生的生 长素逐渐向下运输,枝条上部的侧芽附近生长素浓度较高,由于侧芽对生长素比较敏感,因 此它的发育受到抑制,植株表现出顶端优势。去掉顶芽后,侧芽附近的生长素来源受阻,浓 度降低,于是抑制就被解除,侧芽萌动加快生长。 (2)顶端优势的应用 在生产实践中,顶端优势有很多应用,如对于棉花,农民会适时摘心(去除顶芽),促进侧枝 发育,使之多开花多结果,提高产量;对于果树,可以适时剪枝,或利用顶端优势,或解除 顶端优势,可以塑造一定的树形,使之更好地进行光合作用,提高水果的产量和品质;对于 想获取木材的树木,在种植时注意保护顶芽,以获得高、直的树干。思维拓展 从分析实验中可以培养多项能力,注意比较各组实验的不同之处,再联系实验结果的不 同,可分析得出结论——顶端优势是由于顶芽合成的生长素不断在侧芽积累的结果。多做类 似训练,有助于提高分析能力、综合能力。 知识拓展: 植物的向重力性 如果我们把任何一种植物的幼苗横放,几小时以后,就可以看到它的茎向上弯曲,而根 向下弯曲生长的现象,这就是向重力性的表现,向重力性是指植物在重力的影响下,保持一
纳米氧化锌材料
纳米氧化锌材料研究现状 [摘要]总之,纳米ZnO作为一种新型无机功能材料,从它的许多独特的用途可发现其在日常生活和科研领域具有广阔的市场和诱人的应用前景。随着研究的不断深入与问题的解决,将有更多的优异性能将会被发现。同时更为廉价的工业化生产方法也将会成为现实,纳米ZnO材料将凭借其独特的性能进入我们的日常生活。随着科技的发展,相信纳米ZnO材料的性能及应用将会得到更大的提高和普及,并在新能源、环保、信息科学技术、生物医学、安全、国防等领域发挥重要的作用。 [关键词]纳米ZnO; 表面效应; 溶胶-凝胶法;纳米复合材料 一、纳米氧化锌体的制备 目前,制备纳米氧化锌的方法很多,归纳起来有属于液相法的沉淀法、溶胶-凝胶法、水热法、溶剂热法等,也有属于气相法的化学气相反应法等,而固相法在纳米氧化锌的制备领域则较少见。 a、沉淀法 沉淀法是指使用某些沉淀剂如OH-、CO 32-、C 2 O 4 2-等,或在一定的温度下使 溶液发生水解反应,从而析出产物,洗涤后得到产品[2]。沉淀法一般有分为均匀沉淀法、络合沉淀法、共沉淀法等。 均匀沉淀法工艺成本低、工艺简单,为研究纳米氧化锌结构与性能及应用之间的关系提供了方便。曾宪华[3]等人以常见且廉价的六水硝酸锌和氢氧化钠为以甲醇溶液作为溶剂在常温常压条件下,用均匀沉淀法直接制备了平均粒径为11 nm的纳米氧化锌粉体。以下是他们的用共沉淀法制备的纳米ZnO的扫描电子显微镜(SEM)照片。
络合沉淀法,制备的纳米Zn0不团聚,分散性好,粒径均匀。李冬梅[4]等人采用络合沉淀法制备了粉体平均粒径52 nm,分散性好的纳米氧化锌粉体,并对产品结构性能进行了表征。所得ZnO粉体平均粒径48 nm.分散性好,收率高。 共沉淀法是将含两种或两种以上的阳离子加入到沉淀剂中,使所有的离子同时完全沉淀。在共沉淀中,如何使组成材料的多种离子同时沉淀和如何避免烧结过程中的硬团聚问题是共沉淀法的关键。韩丽[5]的研究表明采用二水醋酸锌、硝酸锌和草酸为基础反应原料,利用共沉淀的方法可合成纳米氧化锌微细晶粒的过程中,利用超声波技术对反应的中间体进行分散和洗涤,由于超声空化作用所产生的局部高温、高压或冲击波和微射流等,使得到的ZnO微晶可以在能量的冲击下得到很好的分散,因此可以阻止团聚现象的产生。 b、溶胶-凝胶法 溶胶-凝胶法是指金属有机或无机化合物加入到溶胶中,制成干胶后进行热处理得到氧化物或其它固体化合物的方法。溶胶凝胶法制备化学微粒的主要优点是反应温度低、化学均匀性好、颗粒细。杨淼[6]等人采用溶胶-
ZnO纳米材料的制备与应用
ZnO纳米材料制备及其应用研究进展 前言:ZnO晶体材料具有六方纤维矿结构,属于直接跃迁宽带半导体材料,其室温下带隙约为3.35eV。具有大的激子结合能,约为60meV,比GaN激子结合能(~25meV)还要大,而且与InGaN材料的晶格较为匹配,因此有利于这两种材料的集成,这些性质在光电子器件制备领域都是十分优越的材料特性。众所周知,由于纳米材料具有大的比表面积、以及量子尺寸效应等特殊性质,近几年来,人们对于ZnO纳米材料的研究工作以极快的速度进展。人们采用了多种方法,诸如分子束外延、热蒸发、化学气相沉积、射频溅射、电化学沉积、溶胶凝胶法,以及脉冲激光沉积等,制备出了多种纳米结构的ZnO材料,诸如纳米棒、纳米线、纳米管、纳米带、纳米颗粒,以及纳米花状结构等。这就使得ZnO纳米材料既具有ZnO 晶体所具有的优异本征特性,又可具有多种变化的纳米结构。可以预期,它在光、声、电纳米器件等诸多应用领域将有着广阔的应用前景。本文将就ZnO纳米材料的制备方法、纳米结构、及其应用研究进展作一简要介绍。 1 ZnO纳米材料制备方法 1.1 等离子体辅助分子束外延(Plasma—assistedMBE 1日本的Yefan Chen等人利用等离子体辅助分子束外延方法在蓝宝石衬底上制备出了纳米ZnO薄膜。锌束流是由蒸发锌元素材料而提供的。而活性氧是由微波等离子体源所产生的。氧等离子体的发光谱研究表明,在氧束流中包含有O ,O ,O“等多种粒子,但是在加大流速的情况下,将以原子氧成分为主。蓝宝石衬底在进行化学侵蚀后,放到850~C高温下净化处理,最后再放入氧等离子体中处理。衬底处理过后,放人反应室进行薄膜生长。调控束流、和微波等离子体功率维持以原子氧为主的反应气氛,衬底温度控制在450—750oC,在蓝宝石C一面(0001)上便可生长出ZnO薄膜。 1.2 热蒸发(Thermal evaporation) Kazuki Bando等人采用热蒸发的方法制备出了ZnO纳米带。将ZnO粉末放入陶瓷舟内,将陶瓷舟及一段陶瓷管同时放入炉膛内加热。在1450~(2温度下加热3h,并以50sccm的流量通入氩气。便可在陶瓷管壁上形成白色ZnO物质。在扫描电子显微镜(SEM)下观察,则是由宽度各不相同的纳米带状结构组成。 1.3 溶胶一凝胶法(SOl—gel technique) D.Basak等人利用溶胶一凝胶法在蓝宝石(0001)晶面上制备了ZnO薄膜J。以乙酸锌[zn(CH CO0) ·2H 0]为先驱物,加入包含有2.86ml二甲基胺(dimethylamine)稳定剂的脱水异丙基醇(dehydrated isopropyl aleoho1)中,制成0.6M 的溶胶。而后用磁力搅拌器进行搅拌,一直到
红外谱图解析基本知识
红外谱图解析基本知识-CAL-FENGHAI-(2020YEAR-YICAI)_JINGBIAN
红外谱图解析基本知识 基团频率区 中红外光谱区可分成4000 cm-1 ~1300(1800) cm-1和1800 (1300 ) cm-1 ~ 600 cm-1两个区域。最有分析价值的基团频率在4000 cm-1 ~ 1300 cm-1之间,这一区域称为基团频率区、官能团区或特征区。区内的峰是由伸缩振动产生的吸收带,比较稀疏,容易辨认,常用于鉴定官能团。 在1800 cm-1(1300 cm-1)~600 cm-1区域内,除单键的伸缩振动外,还有因变形振动产生的谱带。这种振动基团频率和特征吸收峰与整个分子的结构有关。当分子结构稍有不同时,该区的吸收就有细微的差异,并显示出分子特征。这种情况就像人的指纹一样,因此称为指纹区。指纹区对于指认结构类似的化合物很有帮助,而且可以作为化合物存在某种基团的旁证。 基团频率区可分为三个区域 (1) 4000 ~2500 cm-1 X-H伸缩振动区,X可以是O、N、C或S等原子。 O-H基的伸缩振动出现在3650 ~3200 cm-1范围内,它可以作为判断有无醇类、酚类和有机酸类的重要依据。 当醇和酚溶于非极性溶剂(如CCl4),浓度于. dm-3时,在3650 ~3580 cm-1处出现游离O-H基的伸缩振动吸收,峰形尖锐,且没有其它吸收峰干扰,易于识别。当试样浓度增加时,羟基化合物产生缔合现象,O-H基的伸缩振动吸收峰向低波数方向位移,在3400 ~3200 cm-1出现一个宽而强的吸收峰。 胺和酰胺的N-H伸缩振动也出现在3500~3100 cm-1,因此,可能会对O-H伸缩振动有干扰。 C-H的伸缩振动可分为饱和和不饱和的两种: 饱和的C-H伸缩振动出现在3000 cm-1以下,约3000~2800 cm-1,取代基对它们影响很小。如-CH3基的伸缩吸收出现在2960 cm-1和2876 cm-1附近;R2CH2基的吸收在2930 cm-1和2850 cm-1附近;R3CH基的吸收基出现在2890 cm-1附近,但强度很弱。