哈尔滨工业大学 计算传热学 Introduction to LBM
热能英语第三版哈工大部分翻译
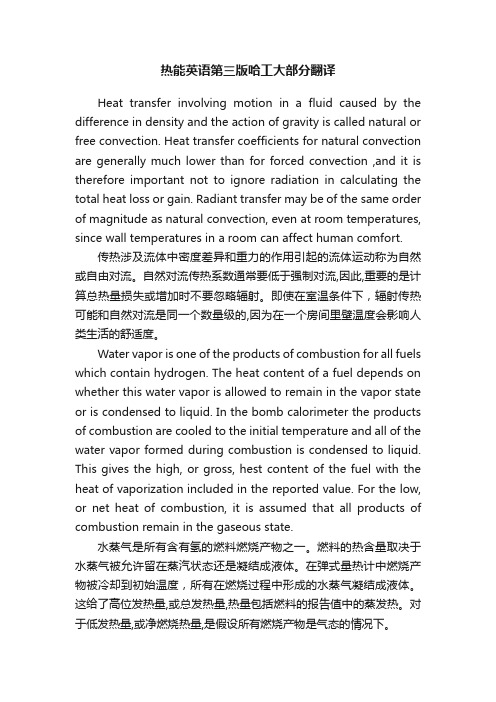
热能英语第三版哈工大部分翻译Heat transfer involving motion in a fluid caused by the difference in density and the action of gravity is called natural or free convection. Heat transfer coefficients for natural convection are generally much lower than for forced convection ,and it is therefore important not to ignore radiation in calculating the total heat loss or gain. Radiant transfer may be of the same order of magnitude as natural convection, even at room temperatures, since wall temperatures in a room can affect human comfort.传热涉及流体中密度差异和重力的作用引起的流体运动称为自然或自由对流。
自然对流传热系数通常要低于强制对流,因此,重要的是计算总热量损失或增加时不要忽略辐射。
即使在室温条件下,辐射传热可能和自然对流是同一个数量级的,因为在一个房间里壁温度会影响人类生活的舒适度。
Water vapor is one of the products of combustion for all fuels which contain hydrogen. The heat content of a fuel depends on whether this water vapor is allowed to remain in the vapor state or is condensed to liquid. In the bomb calorimeter the products of combustion are cooled to the initial temperature and all of the water vapor formed during combustion is condensed to liquid. This gives the high, or gross, hest content of the fuel with the heat of vaporization included in the reported value. For the low, or net heat of combustion, it is assumed that all products of combustion remain in the gaseous state.水蒸气是所有含有氢的燃料燃烧产物之一。
哈尔滨工业大学2008-2009学年春季学期《传热学》期末考试试题与答案
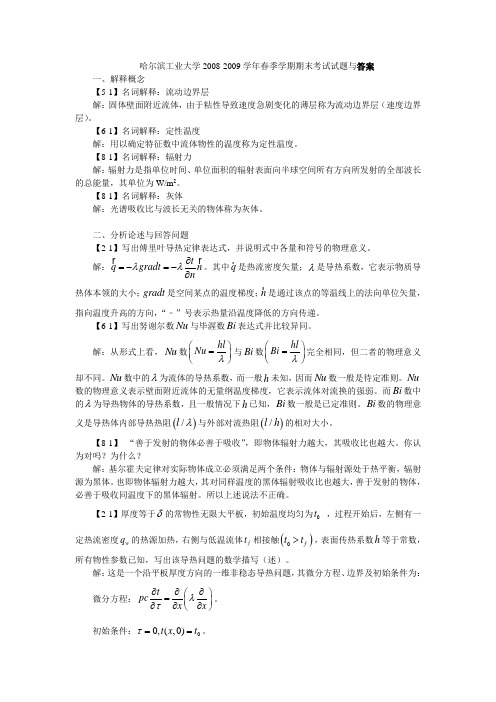
哈尔滨工业大学2008-2009学年春季学期期末考试试题与答案一、解释概念【5-1】名词解释:流动边界层解:固体壁面附近流体,由于粘性导致速度急剧变化的薄层称为流动边界层(速度边界层)。
【6-1】名词解释:定性温度解:用以确定特征数中流体物性的温度称为定性温度。
【8-1】名词解释:辐射力 解:辐射力是指单位时间、单位面积的辐射表面向半球空间所有方向所发射的全部波长的总能量,其单位为W/m 2。
【8-1】名词解释:灰体解:光谱吸收比与波长无关的物体称为灰体。
二、分析论述与回答问题【2-1】写出傅里叶导热定律表达式,并说明式中各量和符号的物理意义。
解:t q gradt n nλλ∂=-=-∂。
其中q是热流密度矢量;λ是导热系数,它表示物质导热体本领的大小;gradt 是空间某点的温度梯度;n是通过该点的等温线上的法向单位矢量,指向温度升高的方向,“﹣”号表示热量沿温度降低的方向传递。
【6-1】写出努谢尔数Nu 与毕渥数Bi 表达式并比较异同。
解:从形式上看,Nu 数hl Nu λ⎛⎫=⎪⎝⎭与Bi 数hl Bi λ⎛⎫= ⎪⎝⎭完全相同,但二者的物理意义却不同。
Nu 数中的λ为流体的导热系数,而一般h 未知,因而Nu 数一般是待定准则。
Nu数的物理意义表示壁面附近流体的无量纲温度梯度,它表示流体对流换的强弱。
而Bi 数中的λ为导热物体的导热系数,且一般情况下h 已知,Bi 数一般是已定准则。
Bi 数的物理意义是导热体内部导热热阻()/l λ与外部对流热阻()/l h 的相对大小。
【8-1】 “善于发射的物体必善于吸收”,即物体辐射力越大,其吸收比也越大。
你认为对吗?为什么?解:基尔霍夫定律对实际物体成立必须满足两个条件:物体与辐射源处于热平衡,辐射源为黑体。
也即物体辐射力越大,其对同样温度的黑体辐射吸收比也越大,善于发射的物体,必善于吸收同温度下的黑体辐射。
所以上述说法不正确。
【2-1】厚度等于δ的常物性无限大平板,初始温度均匀为0t ,过程开始后,左侧有一定热流密度w q 的热源加热,右侧与低温流体f t 相接触()0f t t >,表面传热系数h 等于常数,所有物性参数已知,写出该导热问题的数学描写(述)。
哈工大供热工程课程设计室内采暖设计说明书
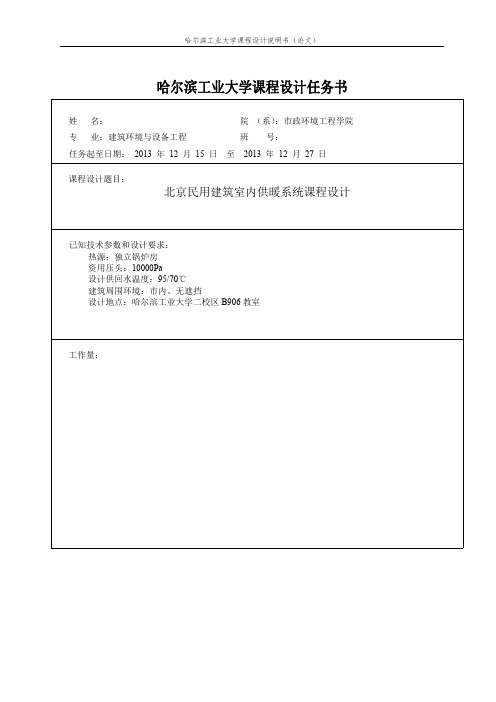
3 R 3m 0.649 0.285 0.185 mm
-6-
哈尔滨工业大学课程设计说明书(论文)
取保温层厚度 3 19 cm ,验算 D 值为 3.57,仍属 III 型,满足计算要求。 因此顶棚保温层厚度为 19cm,则顶棚传热系数为
K 1 1 1.1 W / (㎡·℃) Rn Ri Rw 0.115 0.017 0.011 0.043
查表得tp. min -15.9 ℃,tw.e 0.3t w 0.7tp. min -11 .76 ℃。查表得供暖室内计算温度tn 与 围护结构内表面温度n 的外墙允许温差 ty 6 ℃,因此有
R 0. min
(tn tw.e) 1 (18 11 .76) Rn 0.115 0.57 6 ㎡·℃/ W t y 6
哈尔滨工业大学课程设计说明书(论文)
哈尔滨工业大学课程设计任务书
姓 专 名: 业:建筑环境与设备工程 至 院 (系) :市政环境工程学院 班 号:
任务起至日期: 2013 年 12 月 15 日 课程设计题目:
2013 年 12 月 27 日
北京民用建筑室内供暖系统课程设计
已知技术参数和设计要求: 热源:独立锅炉房 资用压头:10000Pa 设计供回水温度:95/70℃ 建筑周围环境:市内、无遮挡 设计地点:哈尔滨工业大学二校区 B906 教室
R 0. min
(tn tw.e) 1 (18 9) Rn 0.115 0.776 t y 4
㎡·℃/ W
求除保温层以外的各材料层总的热阻及内、外表面换热阻之和 R0 : 钢筋混凝土板 1 30 mm , 1 1.74 , R1
LBM相变传热与流体流动数值分析PPT课件

11.1 计算流体动力学与计算传热学
微观方法:目前适用于纳米尺度和纳秒量级的模拟
• 微观方法假设条件最小,原理上应用范围不受限制。但是 分子动力学方法需要跟踪大量分子的运动,描述每一个分 子的动力学行为,因此所需的计算量非常之大,对计算机 的存储量和计算速度有着非常高的要求。
• 计算条件有限,目前还仅仅局限于纳米尺度的系统和纳秒 时间内的演化过程。
内容介绍
LBM起源与发展 LBM基础理论 LBM基本模型 LBM边界处理方法 LBM应用实例
第1页/共55页
11.1 计算流体动力学与计算传热学
• 流体在物理空间上是由大量分子所构成的离散系统,分 子之间尺度远远大于分子本身尺度,分子通过相互之间 的热运动频繁碰撞从而交换动量和能量。因此,流体的 微观结构在时间和空间上非常复杂,具有非均匀性、离 散性和随机性。
第14页/共55页
11.2 格子Boltzmann方法起源与发展
概述:
Macroscopic
Macroscopic Physics:
• A result of collective behavior of many microscopic particles.
• Not sensitive to underlying microscopic dynamics.
程不能反映正确的非线性和耗散效应。其宏观动力学方程也不 满足 Navier-Stokes 方程。
第19页/共55页
11.2 格子Boltzmann方法起源与发 展
➢ LBM 起源
(2)格子气自动机—— FHP 模型和 FCHC 模型
• 缺 乏 对 称 性 的 问 题 在 1986 年 得 以 解 决 , Frisch 、 Hasslacher 和 Pomeau他们提出了一个具有足够对称性 的二维正六边形的LGA模型,即FHP模型。
哈工程传热学
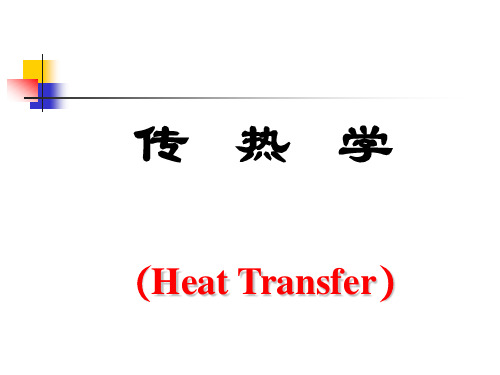
q Φ
ΦΦqΦqtqt❖ww1t1tAwtwrRtttw1w1wwA1111ttwwAttwtw2tt2tww2w2w2222RRrrRr导 单Rttrtttttt热 位热 导阻 热热阻
AA
tw1
Q
tw2
A
导热热阻图示
❖
R
A
导热热阻
2.对流换热(Convection)
(1)热对流:流体(气体或液体)中温度不同的各部分 之间,由于发生相对的宏观运动而把热量 由一处传递到另一处的现象。
火
卫星上装有的太阳能吸收
箭
装置能提供卫星工作所需
升
的部分能量。
空
5. 传热学的应用
建筑环境
建筑上,利用空气导热系数
空
小的特点,制成的空心砖具
心
有良好的保温效果。
砖
实 心 砖 室外环境仿真
5. 传热学的应用
医药卫生 传热学广泛应用于激光手 术、肿瘤高温治疗、低温 外科、移植器官冷冻储存、 疾病热诊断等技术中
定h
2.对流换热(Convection)
(6)表面传热系数的大致范围 表面传热系数的数值范围
过程
自然对流
空气 水
气体
强制对流 高压水蒸气
水
水的相变 沸腾
换热 蒸汽凝结
[W/(m2.K)] 1~10
200~1000 20~100
500~3500 1000~15000 2500~35000 5000~25000
274.7(W / m)
讨论: 计算结果表明, 对于表面温度为几到几十摄氏 度的一类表面的散热问题, 自然对流散热量与辐射具 有相同的数量级,必须同时予以考虑。
1.2 传热过程和传热系数
哈工大传热学大作业--传热学的新领域
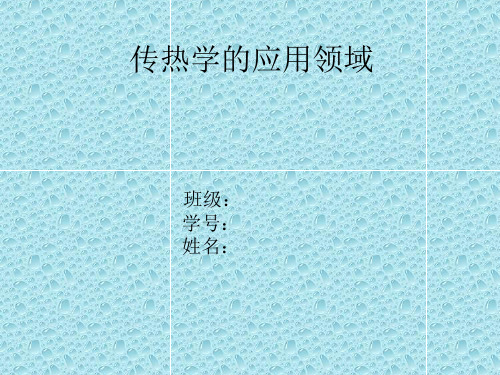
3.机械加工以及金属加工的传热学应用
①金属切削刀具的散热问题与刀具的强度决定了刀的使用寿命和被加工表面的质 量与加工精度。金属切削加工时,材料弹性和塑形变形做的功以及前后刀面 与工件表面的摩擦做功产生的热量都需要通过切屑、工件、刀具和周围介质 散失到环境中,而切削刃的磨损情况与散热的快慢最为密切。当工件材料或 者刀具材料的导热系数大时,切削区散热良好,刀具的磨损减轻,使用寿命 较长,反之,刀具因温度过高发生组织性能转变,磨损加剧,因而需要使用 不同的切削液来加快散热,延长刀具寿命。 ②刀具的散热影响了切削用量的选择,进而影响加工表面的质量,通过对刀具切 削区温度场建立传热模型进行分析,可以更合理的设计刀具结构和选择切削 量,从而提高零件的加工精度,这方面在超精密加工中显得尤为重要。
11
那么:基于对流方式的节能途径是: • 加大换热温差 • 可明显提高换热效率,但实际操作有一定难度。 主要要考虑有哪些场合涉及流体加热或冷却。 • 提高流体流速,增加紊流程度 • 注意控制流体与受热(冷却)面的相对运动方 向 • 设计合理的有利于流体运动的截面形状 • 例如炉膛形状,不仅影响散热面积,而且影响 换热效率, • 设法增大换热面积 肋片、翅片、排管… 炉膛内工件的合理堆放…
6.节能的传热学途径 (基于导热、对流、辐射)
• 总体概述:传热学是研究热量传递规律的一门科学, 它在解决许多工程问题中得到了非常广泛的应用。在研 究节能问题时,通过传热学寻找合适的途径是最根本的 措施 • 在研究节能中的传热学问题时,一般可以分成两种类 型: • 一类是强化传热过程的问题。比如,如何使工件快速 而均匀地达到加热要求,即尽可能地提高热效率,减少 能源的浪费。 • 另一类就是力求削弱传热,比如:各种加热炉的热量 尽可能少地向外界传递或散失,其他各类保温措施也都 属于此类。从节能观点来看,就是减少能量的无谓支出。
工程热力学 01(哈工大)
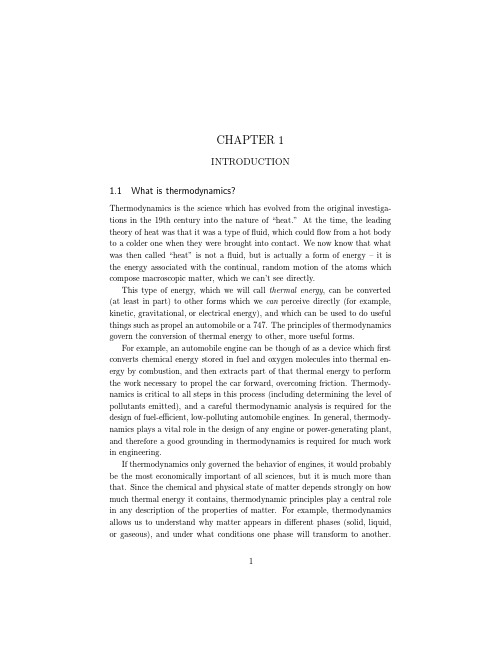
CHAPTER1INTRODUCTION1.1What is thermodynamics?Thermodynamics is the science which has evolved from the original investiga-tions in the19th century into the nature of“heat.”At the time,the leading theory of heat was that it was a type offluid,which couldflow from a hot body to a colder one when they were brought into contact.We now know that what was then called“heat”is not afluid,but is actually a form of energy–it is the energy associated with the continual,random motion of the atoms which compose macroscopic matter,which we can’t see directly.This type of energy,which we will call thermal energy,can be converted (at least in part)to other forms which we can perceive directly(for example, kinetic,gravitational,or electrical energy),and which can be used to do useful things such as propel an automobile or a747.The principles of thermodynamics govern the conversion of thermal energy to other,more useful forms.For example,an automobile engine can be though of as a device whichfirst converts chemical energy stored in fuel and oxygen molecules into thermal en-ergy by combustion,and then extracts part of that thermal energy to perform the work necessary to propel the car forward,overcoming friction.Thermody-namics is critical to all steps in this process(including determining the level of pollutants emitted),and a careful thermodynamic analysis is required for the design of fuel-efficient,low-polluting automobile engines.In general,thermody-namics plays a vital role in the design of any engine or power-generating plant, and therefore a good grounding in thermodynamics is required for much work in engineering.If thermodynamics only governed the behavior of engines,it would probably be the most economically important of all sciences,but it is much more than that.Since the chemical and physical state of matter depends strongly on how much thermal energy it contains,thermodynamic principles play a central role in any description of the properties of matter.For example,thermodynamics allows us to understand why matter appears in different phases(solid,liquid, or gaseous),and under what conditions one phase will transform to another.1CHAPTER1.INTRODUCTION2The composition of a chemically-reacting mixture which is given enough time to come to“equilibrium”is also fully determined by thermodynamic principles (even though thermodynamics alone can’t tell us how fast it will get there).For these reasons,thermodynamics lies at the heart of materials science,chemistry, and biology.Thermodynamics in its original form(now known as classical thermodynam-ics)is a theory which is based on a set of postulates about how macroscopic matter behaves.This theory was developed in the19th century,before the atomic nature of matter was accepted,and it makes no reference to atoms.The postulates(the most important of which are energy conservation and the impos-sibility of complete conversion of heat to useful work)can’t be derived within the context of classical,macroscopic physics,but if one accepts them,a very powerful theory results,with predictions fully in agreement with experiment.When at the end of the19th century itfinally became clear that matter was composed of atoms,the physicist Ludwig Boltzmann showed that the postu-lates of classical thermodynamics emerged naturally from consideration of the microscopic atomic motion.The key was to give up trying to track the atoms in-dividually and instead take a statistical,probabilistic approach,averaging over the behavior of a large number of atoms.Thus,the very successful postulates of classical thermodynamics were given afirm physical foundation.The science of statistical mechanics begun by Boltzmann encompasses everything in classical thermodynamics,but can do more also.When combined with quantum me-chanics in the20th century,it became possible to explain essentially all observed properties of macroscopic matter in terms of atomic-level physics,including es-oteric states of matter found in neutron stars,superfluids,superconductors,etc. Statistical physics is also currently making important contributions in biology, for example helping to unravel some of the complexities of how proteins fold.Even though statistical mechanics(or statistical thermodynamics)is in a sense“more fundamental”than classical thermodynamics,to analyze practical problems we usually take the macroscopic approach.For example,to carry out a thermodynamic analysis of an aircraft engine,its more convenient to think of the gas passing through the engine as a continuumfluid with some specified properties rather than to consider it to be a collection of molecules.But we do use statistical thermodynamics even here to calculate what the appropriate property values(such as the heat capacity)of the gas should be.CHAPTER1.INTRODUCTION3 1.2Energy and EntropyThe two central concepts of thermodynamics are energy and entropy.Most other concepts we use in thermodynamics,for example temperature and pres-sure,may actually be defined in terms of energy and entropy.Both energy and entropy are properties of physical systems,but they have very different characteristics.Energy is conserved:it can neither be produced nor destroyed, although it is possible to change its form or move it around.Entropy has a different character:it can’t be destroyed,but it’s easy to produce more entropy (and almost everything that happens actually does).Like energy,entropy too can appear in different forms and be moved around.A clear understanding of these two properties and the transformations they undergo in physical processes is the key to mastering thermodynamics and learn-ing to use it confidently to solve practical problems.Much of this book is focused on developing a clear picture of energy and entropy,explaining their origins in the microscopic behavior of matter,and developing effective methods to analyze complicated practical processes1by carefully tracking what happens to energy and entropy.1.3Some TerminologyMostfields have their own specialized terminology,and thermodynamics is cer-tainly no exception.A few important terms are introduced here,so we can begin using them in the next chapter.1.3.1System and EnvironmentIn thermodynamics,like in most other areas of physics,we focus attention on only a small part of the world at a time.We call whatever object(s)or region(s) of space we are studying the system.Everything else surrounding the system (in principle including the entire universe)is the environment.The boundary between the system and the environment is,logically,the system boundary. The starting point of any thermodynamic analysis is a careful definition of the system.EnvironmentSystemBoundarySystem1Rocket motors,chemical plants,heat pumps,power plants,fuel cells,aircraft engines,...CHAPTER1.INTRODUCTION4Figure1.1:Control masses and control volumes.1.3.2Open,closed,and isolated systemsAny system can be classified as one of three types:open,closed,or isolated. They are defined as follows:open system:Both energy and matter can be exchanged with the environ-ment.Example:an open cup of coffee.closed system:energy,but not matter,can be exchanged with the environ-ment.Examples:a tightly capped cup of coffee.isolated system:Neither energy nor matter can be exchanged with the envi-ronment–in fact,no interactions with the environment are possible at all.Example(approximate):coffee in a closed,well-insulated thermos bottle.Note that no system can truly be isolated from the environment,since no thermal insulation is perfect and there are always physical phenomena which can’t be perfectly excluded(gravitationalfields,cosmic rays,neutrinos,etc.). But good approximations of isolated systems can be constructed.In any case, isolated systems are a useful conceptual device,since the energy and mass con-tained inside them stay constant.1.3.3Control masses and control volumesAnother way to classify systems is as either a control mass or a control volume. This terminology is particularly common in engineering thermodynamics.A control mass is a system which is defined to consist of a specified piece or pieces of matter.By definition,no matter can enter or leave a control mass. If the matter of the control mass is moving,then the system boundary moves with it to keep it inside(and matter in the environment outside).A control volume is a system which is defined to be a particular region of space.Matter and energy may freely enter or leave a control volume,and thus it is an open system.CHAPTER1.INTRODUCTION5 1.4A Note on UnitsIn this book,the SI system of units will be used exclusively.If you grew up anywhere but the United States,you are undoubtedly very familiar with this system.Even if you grew up in the US,you have undoubtedly used the SI system in your courses in physics and chemistry,and probably in many of your courses in engineering.One reason the SI system is convenient is its simplicity.Energy,no matter what its form,is measured in Joules(1J=1kg-m2/s2).In some other systems, different units are used for thermal and mechanical energy:in the English sys-tem a BTU(“British Thermal Unit”)is the unit of thermal energy and a ft-lbf is the unit of mechanical energy.In the cgs system,thermal energy is measured in calories,all other energy in ergs.The reason for this is that these units were chosen before it was understood that thermal energy was like mechanical energy, only on a much smaller scale.2Another advantage of SI is that the unit of force is indentical to the unit of(mass x acceleration).This is only an obvious choice if one knows about Newton’s second law,and allows it to be written asF=m a.(1.1)In the SI system,force is measured in kg-m/s2,a unit derived from the3primary SI quantities for mass,length,and time(kg,m,s),but given the shorthand name of a“Newton.”The name itself reveals the basis for this choice of force units.The units of the English system werefixed long before Newton appeared on the scene(and indeed were the units Newton himself would have used).The unit of force is the“pound force”(lbf),the unit of mass is the“pound mass”(lbm)and of course acceleration is measured in ft/s2.So Newton’s second law must include a dimensional constant which converts from Ma units(lbm ft/s2) to force units(lbf).It is usually writtenF=1g cm a,(1.2)whereg c=32.1739ft-lbm/lbf-s2.(1.3) Of course,in SI g c=1.2Mixed unit systems are sometimes used too.American power plant engineers speak of the “heat rate”of a power plant,which is defined as the thermal energy which must be absorbed from the furnace to produce a unit of electrical energy.The heat rate is usually expressed in BTU/kw-hr.CHAPTER1.INTRODUCTION6In practice,the units in the English system are now defined in terms of their SI equivalents(e.g.one foot is defined as a certain fraction of a meter,and one lbf is defined in terms of a Newton.)If given data in Engineering units,it is often easiest to simply convert to SI,solve the problem,and then if necessary convert the answer back at the end.For this reason,we will implicitly assume SI units in this book,and will not include the g c factor in Newton’s2nd law.。
哈尔滨工业大学计算传热学第四章扩散方程的数值解法及其应用资料重点
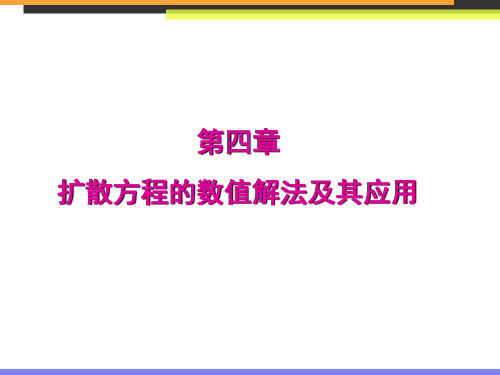
1
y
xw
TP
aETE
aNTN
aSTS
1
y
xw
Tf
Scxy
kB
kB
所以对第三类边界条件不仅有附加常数源项,而且还有 附加源项的斜率项
aPTP aETE aNTN aSTS (Sc•ad Sc )xy
aP aE 0 aN aS (Sp•ad Sp )xy
Sp•ad
y xy
a)算术平均线性分布
ke
kp
xe+ xe
kE
xe xe
e
••
•
W
Pxe xe E
xe
b)调和平均
qe
TE TP
xe
ke
TE Te
xe
kE
Te Tp
xe
kp
TE
xe
kE
TP
xe
kP
ke
kP kExe xek p xekE
当 xe xe
kP kE
算术平均
ke
kP
kE 2
kP 2
第四章 扩散方程的数值解法及其应用
§4.1 一维稳态导热
1 • d [kF(x) dT ] S 0
F (x) dx
dx
F(x):与坐标系和截面形状有关的计算因子
S:内热源。
w
e
△x
e d
dT
[kF (x) ]dx
w dx
dx
e
F (x) • Sdx 0
w
•
W
xw
•
P
xe
•
E
keFe
W PE
ap
Fe k e
xe