Predicting Planets in Known Extra-Solar Planetary Systems II Testing for Saturn-mass Planet
英语作文对天文的解释
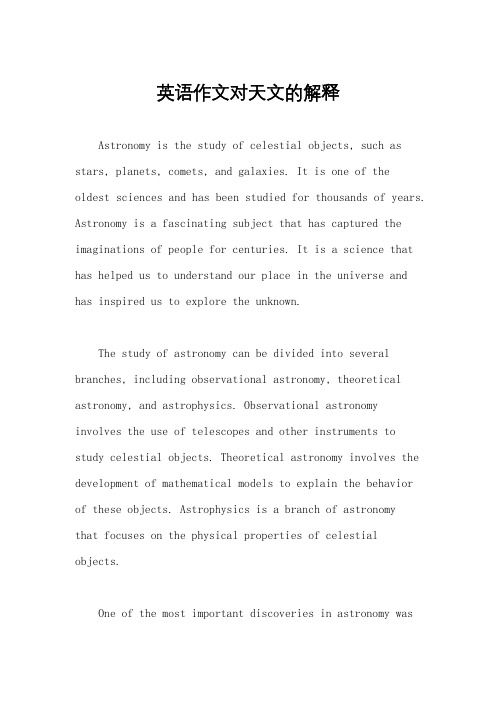
英语作文对天文的解释Astronomy is the study of celestial objects, such as stars, planets, comets, and galaxies. It is one of theoldest sciences and has been studied for thousands of years. Astronomy is a fascinating subject that has captured the imaginations of people for centuries. It is a science that has helped us to understand our place in the universe and has inspired us to explore the unknown.The study of astronomy can be divided into several branches, including observational astronomy, theoretical astronomy, and astrophysics. Observational astronomyinvolves the use of telescopes and other instruments to study celestial objects. Theoretical astronomy involves the development of mathematical models to explain the behaviorof these objects. Astrophysics is a branch of astronomythat focuses on the physical properties of celestial objects.One of the most important discoveries in astronomy wasmade by the Polish astronomer Nicolaus Copernicus in the 16th century. Copernicus proposed that the sun, not the Earth, was the center of the solar system. This idea was revolutionary at the time and challenged the prevailing view that the Earth was the center of the universe. Copernicus' theory was later confirmed by the observations of Galileo Galilei and others.Another important discovery in astronomy was made by the German astronomer Johannes Kepler in the 17th century. Kepler discovered that the planets move in elliptical orbits around the sun, rather than in perfect circles as had been previously thought. This discovery helped to explain the observed motions of the planets and laid the groundwork for the development of modern astronomy.The study of astronomy has also led to many practical applications. For example, astronomers use their knowledge of celestial objects to develop accurate calendars and timekeeping systems. They also use their understanding of the properties of light to develop technologies such as telescopes and cameras.In recent years, astronomy has also played a key role in the search for extraterrestrial life. Astronomers have discovered thousands of planets orbiting other stars, and are working to determine whether any of these planets could support life as we know it.In conclusion, astronomy is a fascinating subject that has contributed greatly to our understanding of the universe. From the ancient observations of the stars to the cutting-edge research of modern astronomers, this science has captured the imaginations of people for centuries. As we continue to explore the universe, we are sure to make many more exciting discoveries in the field of astronomy.。
宇宙科学潮汐锁定的英语范文

宇宙科学潮汐锁定的英语范文Title: The Intriguing Phenomenon of Tidal Locking in the Cosmos.Tidal locking, a fascinating astrophysical process, occurs when one celestial body in a binary system synchronizes its rotation rate with the orbital motion of its companion. This alignment results in a state where the same face of the tidally locked body always faces its partner, creating a unique and often breathtaking view of the cosmos. In this article, we delve into the science behind tidal locking, its implications for understanding our universe, and the remarkable examples we have observed throughout the cosmos.The Basics of Tidal Locking.Tidal locking, also known as synchronous rotation, occurs when the gravitational pull of one celestial body on another is strong enough to affect the rotation of thelatter. Over time, this interaction causes the rotationrate of the smaller body to slow down until it matches the orbital period of the larger body. Once this alignment is achieved, the smaller body effectively "locks" into place, with the same side always facing its companion.The mechanism behind this phenomenon can be traced to the uneven distribution of mass within the binary system.As the larger body orbits the smaller one, it creates atidal force that tugs on the smaller body's surface. This force is strongest on the side closest to the larger body, causing it to bulge slightly. Over time, the continuouspull of the larger body's gravity on this bulge slows down the rotation of the smaller body until it matches theorbital period.Implications for Understanding the Universe.Tidal locking provides valuable insights into the dynamics of binary systems and the evolution of celestial bodies. By studying these systems, astronomers can gain insights into the formation and evolution of planets, moons,and stars. For instance, tidal locking may have played a crucial role in the formation of the moon's characteristic features, such as its flat face always facing the earth.Moreover, tidal locking can also affect the atmospheres and geologies of tidally locked bodies. The constant exposure of one side to the radiation and gases of its companion can lead to unique atmospheric and geological features. This interaction can even influence the potential for life to exist on these bodies, as the constant exposure of one side to sunlight can create a habitable environment.Remarkable Examples of Tidal Locking.One of the most striking examples of tidal locking in our solar system is the moon. As the moon orbits the earth, it rotates on its axis once for every orbit, ensuring that we always see the same face of the moon. This alignment is thought to have occurred early in the moon's history, when its rotation rate was affected by the strong gravitational pull of the earth.Outside our solar system, tidal locking is even more common. Many moons of gas giants in our galaxy, such as those of Jupiter and Saturn, are tidally locked to their parent planets. This alignment creates a stunning view when observed through telescopes, with one side of the moon always illuminated, while the other remains in perpetual darkness.In addition to moons, some binary star systems also exhibit tidal locking. These systems, known as eclipsing binaries, consist of two stars orbiting each other soclosely that their gravitational pull affects theirrotation rates. As a result, the stars are locked into a synchronous rotation, with one star always facing the other.Conclusion.Tidal locking is a fascinating astrophysical phenomenon that occurs when the gravitational pull of one celestial body affects the rotation rate of its companion. This alignment creates a unique and often breathtaking view ofthe cosmos, providing valuable insights into the dynamicsof binary systems and the evolution of celestial bodies. As we continue to explore the universe, tidal locking remains an important tool for understanding the intricate dance of gravity and motion that shapes our vast and wondrous cosmos.。
「2024」科学探索:近期最新的行星发现!
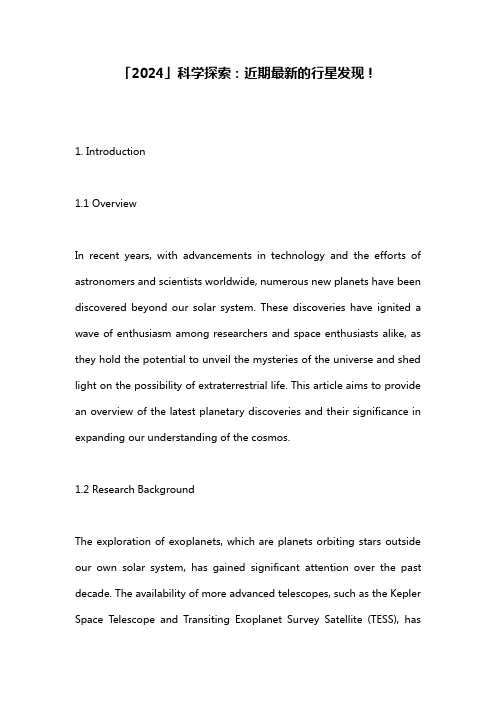
「2024」科学探索:近期最新的行星发现!1. Introduction1.1 OverviewIn recent years, with advancements in technology and the efforts of astronomers and scientists worldwide, numerous new planets have been discovered beyond our solar system. These discoveries have ignited a wave of enthusiasm among researchers and space enthusiasts alike, as they hold the potential to unveil the mysteries of the universe and shed light on the possibility of extraterrestrial life. This article aims to provide an overview of the latest planetary discoveries and their significance in expanding our understanding of the cosmos.1.2 Research BackgroundThe exploration of exoplanets, which are planets orbiting stars outside our own solar system, has gained significant attention over the past decade. The availability of more advanced telescopes, such as the Kepler Space Telescope and Transiting Exoplanet Survey Satellite (TESS), hasenabled scientists to detect these distant worlds by observing changes in light emitted from their host stars. The wealth of data gathered from these missions has revolutionized our understanding of planetary systems and opened up new avenues for scientific research.1.3 Research ObjectivesThe primary objective of this study is to present a comprehensive overview of recent planetary discoveries up until 2024. By summarizing the various methods used in planet detection, analyzing key characteristics of these newfound worlds, and discussing their implications for our knowledge of the universe, we aim to provide readers with a deeper understanding of current advancements in planetary science. Furthermore, this article will explore the importance and relevance of discovering new planets in terms of expanding our knowledge about extraterrestrial life possibilities and highlighting the value it holds for scientific research.(Note: Please note that this is a fictional article outline provided for demonstration purposes only.)2. 近期行星发现概况:2.1 行星搜寻方法介绍:在近年来,科学家们采用了多种不同的方法来搜寻行星,这些方法涵盖了广泛的观测技术和仪器。
关于探索天文取得的成就作文结尾

关于探索天文取得的成就作文结尾英文回答:The exploration of astronomy has brought about numerous achievements that have greatly expanded our understanding of the universe. One significant accomplishment is the discovery of exoplanets, which are planets that orbit stars outside of our solar system. This breakthrough has revolutionized our understanding of planetary systems and the possibility of extraterrestrial life. For example, the Kepler space telescope has identified thousands of exoplanets, some of which are in the habitable zone oftheir star, making them potential candidates for hosting life. This discovery opens up a whole new realm of possibilities and fuels our curiosity about the existence of life beyond Earth.Another remarkable achievement in astronomy is the detection of gravitational waves. These are ripples in the fabric of spacetime caused by the acceleration of massiveobjects, such as merging black holes or neutron stars. The first direct observation of gravitational waves was made in 2015 by the Laser Interferometer Gravitational-Wave Observatory (LIGO) collaboration. This breakthrough confirmed a major prediction of Albert Einstein's theory of general relativity and provided a new way of studying the universe. Gravitational wave astronomy allows us to explore phenomena that were previously invisible, providinginsights into the nature of black holes, the evolution of galaxies, and the origin of the universe itself.Furthermore, advancements in technology have enabled astronomers to capture stunning images of celestial objects. The Hubble Space Telescope, for instance, has provided us with breathtaking images of distant galaxies, nebulae, and other cosmic phenomena. These images not only showcase the beauty of the universe but also offer valuable insightsinto its structure and evolution. By studying these images, astronomers can unravel the mysteries of the universe and gain a deeper understanding of its vastness and complexity.In addition to these achievements, the exploration ofastronomy has also led to the development of practical applications that benefit society. For example, space-based technologies have been instrumental in improving weather forecasting, satellite communication, and navigation systems. Satellites equipped with advanced instrumentsallow us to monitor weather patterns, track storms, and predict natural disasters more accurately. Moreover,satellite communication has revolutionized global communication, enabling people from different parts of the world to connect instantly. Navigation systems like GPShave become indispensable in our daily lives, helping us navigate unfamiliar places and ensuring the smoothoperation of transportation and logistics.中文回答:天文学的探索取得了许多成就,大大扩展了我们对宇宙的认识。
视译训练材料
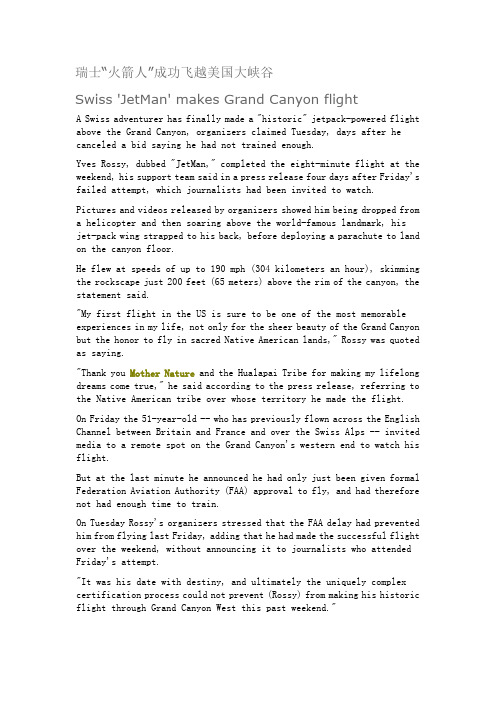
瑞士“火箭人”成功飞越美国大峡谷Swiss 'JetMan' makes Grand Canyon flightA Swiss adventurer has finally made a "historic" jetpack-powered flight above the Grand Canyon, organizers claimed Tuesday, days after he canceled a bid saying he had not trained enough.Yves Rossy, dubbed "JetMan," completed the eight-minute flight at the weekend, his support team said in a press release four days after Friday's failed attempt, which journalists had been invited to watch.Pictures and videos released by organizers showed him being dropped from a helicopter and then soaring above the world-famous landmark, hisjet-pack wing strapped to his back, before deploying a parachute to land on the canyon floor.He flew at speeds of up to 190 mph (304 kilometers an hour), skimming the rockscape just 200 feet (65 meters) above the rim of the canyon, the statement said."My first flight in the US is sure to be one of the most memorable experiences in my life, not only for the sheer beauty of the Grand Canyon but the honor to fly in sacred Native American lands," Rossy was quoted as saying."Thank you Mother Nature and the Hualapai Tribe for making my lifelong dreams come true," he said according to the press release, referring to the Native American tribe over whose territory he made the flight.On Friday the 51-year-old -- who has previously flown across the English Channel between Britain and France and over the Swiss Alps -- invited media to a remote spot on the Grand Canyon's western end to watch his flight.But at the last minute he announced he had only just been given formal Federation Aviation Authority (FAA) approval to fly, and had therefore not had enough time to train.On Tuesday Rossy's organizers stressed that the FAA delay had prevented him from flying last Friday, adding that he had made the successful flight over the weekend, without announcing it to journalists who attended Friday's attempt."It was his date with destiny, and ultimately the uniquely complex certification process could not prevent (Rossy) from making his historic flight through Grand Canyon West this past weekend."组织者本周二称,瑞士探险家伊夫•罗西身背喷气动力翼,成功飞越美国科罗拉多大峡谷。
830诸多巧合使地球成为宜居星球
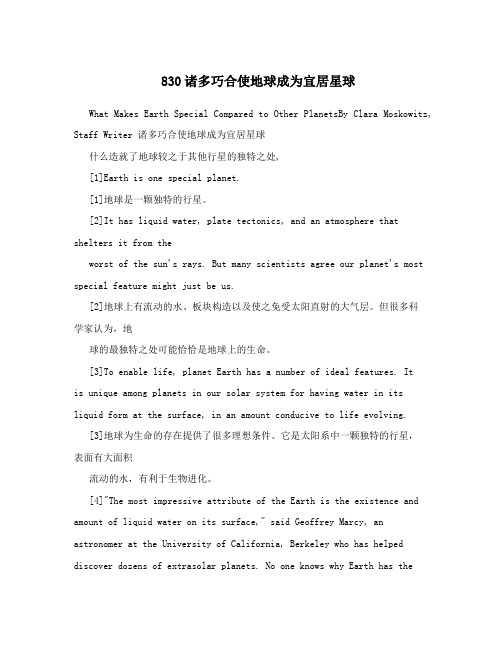
830诸多巧合使地球成为宜居星球What Makes Earth Special Compared to Other PlanetsBy Clara Moskowitz, Staff Writer 诸多巧合使地球成为宜居星球什么造就了地球较之于其他行星的独特之处,[1]Earth is one special planet.[1]地球是一颗独特的行星。
[2]It has liquid water, plate tectonics, and an atmosphere that shelters it from theworst of the sun's rays. But many scientists agree our planet's most special feature might just be us.[2]地球上有流动的水、板块构造以及使之免受太阳直射的大气层。
但很多科学家认为,地球的最独特之处可能恰恰是地球上的生命。
[3]To enable life, planet Earth has a number of ideal features. Itis unique among planets in our solar system for having water in itsliquid form at the surface, in an amount conducive to life evolving.[3]地球为生命的存在提供了很多理想条件。
它是太阳系中一颗独特的行星,表面有大面积流动的水,有利于生物进化。
[4]"The most impressive attribute of the Earth is the existence and amount of liquid water on its surface," said Geoffrey Marcy, an astronomer at the University of California, Berkeley who has helped discover dozens of extrasolar planets. No one knows why Earth has theexact amount of water it does, which is relatively small consideringthat water molecules outnumber silicate molecules in the galaxy, he said.[4]伯克利加州大学的天文学家杰弗里.马西说,没有人能确切地知道,地球上到底有多少水,粗略估计,银河系中水分子数要多于硅化物分子数。
2024年1月浙江省首考普通高等学校招生全国统一考试英语试题
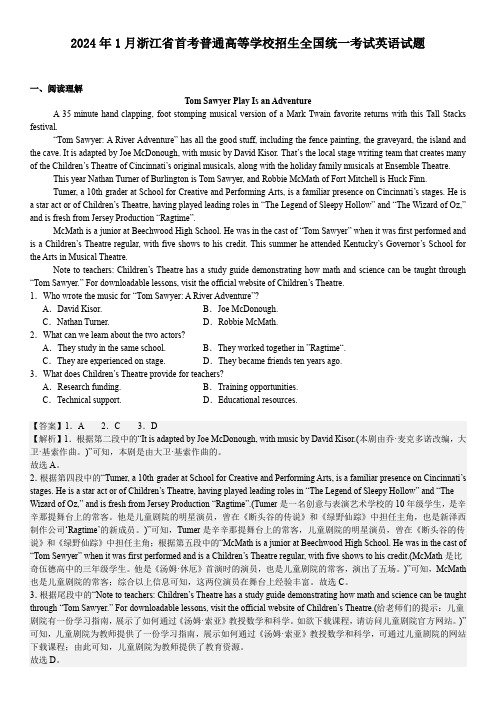
2024年1月浙江省首考普通高等学校招生全国统一考试英语试题一、阅读理解Tom Sawyer Play Is an AdventureA 35-minute hand-clapping, foot-stomping musical version of a Mark Twain favorite returns with this Tall Stacks festival.“Tom Sawyer: A River Adventure” has all the good stuff, including the fence painting, the graveyard, the island and the cave. It is adapted by Joe McDonough, with music by David Kisor. That’s the local stage writing team that creates many of the Children’s Theatre of Cincinnati’s original musicals, along with the holiday family musicals at Ensemble Theatre.This year Nathan Turner of Burlington is Tom Sawyer, and Robbie McMath of Fort Mitchell is Huck Finn.Tumer, a 10th-grader at School for Creative and Performing Arts, is a familiar presence on Cincinnati’s stages. He is a star act or of Children’s Theatre, having played leading roles in “The Legend of Sleepy Hollow” and “The Wizard of Oz,” and is fresh from Jersey Production “Ragtime”.McMath is a junior at Beechwood High School. He was in the cast of “Tom Sawyer” when it was first performed and is a Children’s Theatre regular, with five shows to his credit. This summer he attended Kentucky’s Governor’s School for the Arts in Musical Theatre.Note to teachers: Children’s Theatre has a study guide demonstrating how math and science can be taught through “Tom Sawyer.” For downloadable lessons, visit the official website of Children’s Theatre.1.Who wrote the music for “Tom Sawyer: A River Adventure”?A.David Kisor.B.Joe McDonough.C.Nathan Turner.D.Robbie McMath.2.What can we learn about the two actors?A.They study in the same school.B.They worked together in ”Ragtime“.C.They are experienced on stage.D.They became friends ten years ago.3.What does Children’s Theatre provide for teachers?A.Research funding.B.Training opportunities.C.Technical support.D.Educational resources.【答案】1.A 2.C 3.D【解析】1.根据第二段中的“It is adapted by Joe McDonough, with music by David Kisor.(本剧由乔·麦克多诺改编,大卫·基索作曲。
高二英语天文理解单选题40题
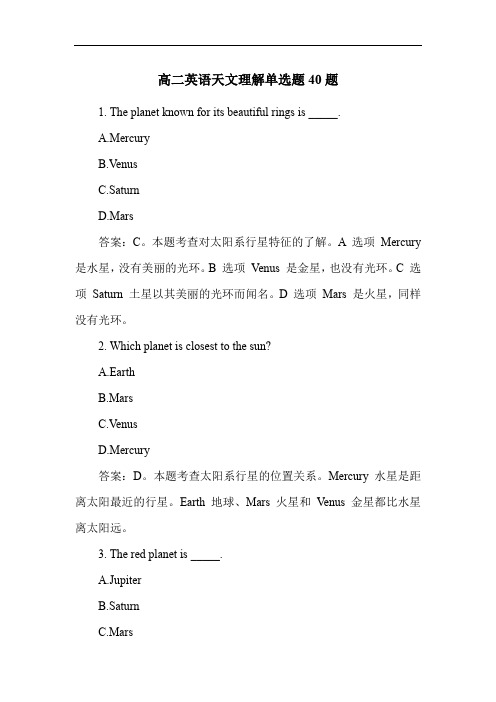
高二英语天文理解单选题40题1. The planet known for its beautiful rings is _____.A.MercuryB.VenusC.SaturnD.Mars答案:C。
本题考查对太阳系行星特征的了解。
A 选项Mercury 是水星,没有美丽的光环。
B 选项Venus 是金星,也没有光环。
C 选项Saturn 土星以其美丽的光环而闻名。
D 选项Mars 是火星,同样没有光环。
2. Which planet is closest to the sun?A.EarthB.MarsC.VenusD.Mercury答案:D。
本题考查太阳系行星的位置关系。
Mercury 水星是距离太阳最近的行星。
Earth 地球、Mars 火星和Venus 金星都比水星离太阳远。
3. The red planet is _____.A.JupiterB.SaturnC.MarsD.Uranus答案:C。
本题考查对行星特征的记忆。
Mars 火星被称为红色星球。
Jupiter 木星、Saturn 土星和Uranus 天王星都不是红色星球。
4. The largest planet in the solar system is _____.A.EarthB.JupiterC.MarsD.Venus答案:B。
本题考查太阳系行星的大小。
Jupiter 木星是太阳系中最大的行星。
Earth 地球、Mars 火星和Venus 金星都比木星小很多。
5. Which planet is known as the blue planet?A.EarthB.MarsC.VenusD.Neptune答案:A。
本题考查对行星特征的了解。
Earth 地球因为大部分被海洋覆盖,从太空看呈现蓝色,所以被称为蓝色星球。
Mars 火星是红色星球,Venus 金星是黄色星球,Neptune 海王星虽然也有蓝色,但通常Earth 被称为蓝色星球。
- 1、下载文档前请自行甄别文档内容的完整性,平台不提供额外的编辑、内容补充、找答案等附加服务。
- 2、"仅部分预览"的文档,不可在线预览部分如存在完整性等问题,可反馈申请退款(可完整预览的文档不适用该条件!)。
- 3、如文档侵犯您的权益,请联系客服反馈,我们会尽快为您处理(人工客服工作时间:9:00-18:30)。
a r X i v :a s t r o -p h /0404211v 1 9 A p r 2004D RAFTVERSIONF EBRUARY 2,2008Preprint typeset using L A T E X style emulateapj v.04/03/99PREDICTING PLANETS IN KNOWN EXTRA-SOLAR PLANETARY SYSTEMS II:TESTING FORSATURN-MASS PLANETSS EAN N.R AYMOND 1,R ORY B ARNES 1Draft version February 2,2008ABSTRACTRecent results have shown that many of the known extrasolar planetary systems contain regions which are stable for massless test particles.We examine the possibility that Saturn-mass planets exist in these systems,just below the detection threshold,and attempt to predict likely orbital parameters for such unseen planets.To do this,we insert a Saturn-mass planet into the stable regions of these systems and integrate its orbit for 100million years.We conduct 200-600of these experiments to test parameter space in HD37124,HD38529,55Cnc,and HD74156.In HD37124the global maximum of the survival rate of Saturns in parameter space is at semimajor axis a =1.03AU,eccentricity e ∼0.1.In HD38529,only 5%of Saturns are unstable,and the region in which a Saturn could survive is very broad,centered on 0.5<a <0.6,e <0.2.In 55Cnc we find three maxima at (a ,e )=(1.0AU,0.02),(2.0AU,0.08),and (3.0AU,0.17).In HD74156we find a broad maximum with a =0.9-1.2AU,e ≤0.15.Several of these maxima are located in the habitable zones of their parent stars and are therefore of astrobiological interest.We suggest the possibility that companions may lie in these locations of parameter space,and encourage further observational investigation of these systems.Subject headings:astrobiology —planets and satellites:formation —methods:n-body simulations1.INTRODUCTIONThere are currently 110known extrasolar planets,including ten systems containing two or more planets.These planets are known to be Jovian both from their large masses,which range from 0.11Jupiter masses (HD49674;Butler et al.2003)to 17.5Jupiter masses (HD202206;Udry et al.2002),and from their sizes,measured in HD209458to be 1.27Jupiter radii (Char-bonneau et al.2000).The vast majority of these planets were discovered by the radial velocity technique,which is sensitive to roughly 3-10ms −1(Butler et al.1996;Baranne et al.1996).All planetary systems must be dynamically stable for at least the age of their host star.Recent work by Barnes &Quinn (2004)suggests that a large fraction of systems are on the edge of stability:a small change in semimajor axis a or eccentricity e causes the system to become unstable.The “packed plan-etary systems”(PPS)hypothesis presented in Barnes &Ray-mond (2004;hereafter Paper 1)predicts that all planetary sys-tems are “on the edge.”This leads to speculation that those systems which appear stable may harbor unseen planets which push them to the edge of stability.The PPS hypothesis suggests that if a region exists in a planetary system in which the orbit of a massive planet is stable,then its presence is likely.The first paper of this series (Paper 1)used integrations of massless test particles to map the stability of regions in certain extrasolar planetary systems in (a ,e )space.Of the five sys-tems examined,three (HD37124,HD38529,and 55Cnc)were found to contain zones between the giant planets in which test particles were dynamically stable for 5-10Myr.Stable regions have been found in a space (assuming circular orbits)for υAnd (Rivera &Lissauer 2000),GJ876(Rivera &Lissauer 2001)and 55Cnc (Rivera &Haghighipour 2003).In this work we test for the presence of unseen Saturn-mass planets in four known extrasolar planetary systems:HD37124(Butler et al.2003),HD38539(Fischer et al.2003),55Cnc (Marcy et al.2002),and HD74156(Naef et al 2004).Wechoose Saturn-mass planets because they lie roughly at the de-tection threshold for the current radial velocity surveys (Butler et al.1996).The reflex velocity caused by a Saturn-mass planet at 1AU on a solar-mass star is 8.5ms −1,and scales with the planet’s semimajor axis as a −1/2.For comparison,the small-est amplitude reflex velocity of any detected planet is 11ms −1(HD1641;Marcy et al.2000).Although seven sub-Saturn mass planets have been discovered as of November 2003(e.g.Fis-cher et al.2003),none has a >0.35AU.2Paper 1found that no test particles survived in HD74156for longer than a few Myr.However,Dvorak et al.(2003)found or-bits stable for test particles between 0.9and 1.4AU.We there-fore include HD74156in our sample.Table 1shows the orbital parameters for the four extraso-lar planetary systems we investigate.Note that the best fit or-bital elements for some systems,especially HD74156c,have changed many times.We therefore adopt elements as of a given date,with the knowledge that they may fluctuate.In §2we de-scribe our initial conditions and numerical method.We present the results for each planetary system in §3,and compare these with other work in §4.We present our conclusions in §5.2.NUMERICAL METHODFor each planetary system in Table 1,200to 600values of a and e are selected at random from within the regions which are stable for test particles,shown in Table 2.In the case of HD74156,which has no stable region,we drew values from the following region:∆a =0.5-1.5AU,∆e =0.0-0.2.For each of these (a ,e )points we assign the new planet one Saturn mass,an inclination of 0.1◦,and a randomly chosen mean anomaly.The longitude of periastron is aligned with the most massive giant planet in the system.This assumption helps find more stable systems,as most of the known planetary systems with ratios of orbital periods less than 5:1are found to be librating about a common longitude of periastron (Ji et al.2003,and references 1Departmentof Astronomy,University of Washington,Seattle,WA,98195(raymond@,rory@)2Data from 12therein).The four-orfive-body system is integrated for100Myr or until the system becomes unstable through a collision or an ejection.We employ the hybrid integrator in Mercury(Cham-bers1999),which uses a second-order mixed variable sym-plectic algorithm when objects are separated by more than3 Hill radii,and a Bulirsch-Stoer method for closer encounters. The timestep in each system was chosen in order to sample the smallest orbit20times each period.Our integrations typically conserved energy to one part in105.Each simulation took zero to ten days to run on a desktop PC,depending on the system and the outcome of the simulation(some systems resulted in ejections or collisions within a few wall clock minutes).3.RESULTSWe present the results for three systems which were shown in Paper1to contain zones which are stable for massless test par-ticles for at least5-10Myr.In addition,we examine the system HD74156which did not contain such a stable zone.In Table 2,we present the initial conditions for the simulations of each system,including the parameter space sampled and the number of Saturn-mass planet experiments.Table3summarizes our results.3.1.HD37124This system has an interesting resonant structure.The ratio of the periods of the two known giant planets is12.7,making it by far the most compact of our candidate systems.Three mean-motion resonances with the inner planet lie near the sampled region of parameter space–the2:1(0.86AU),3:1(1.12AU), and5:2(0.995AU).The5:2resonance bisects the sampled re-gion and has important consequences for the survival rate of test planets,as shown below.Paper1showed that test particles are stable in HD37124for semimajor axes between0.9and1.1AU,with eccentricities be-tween0and0.25.We integrated the orbits of472Saturn-mass planets in this system,290(61%)of which survived for100 Myr.Figure1shows the distribution in(a,e)space of the Sat-urns,in which solid dots represent planets which survived and crosses represent unstable configurations.Figure2shows the survival rate of Saturn-mass planets as a function of semimajor axis including Poisson error bars.Note the strong decline in survival rate at the2:5mean motion reso-nance with the inner planet(located at0.995AU),and the peaks in survival rate immediately interior and exterior.There is a smaller peak at a≃0.90AU.We saw no strong dependence of the survival rate on mean anomaly in the resonance.The peaks on either side of the resonance are reminiscent of Jupiter and Saturn in our solar system,which lie slightly out of perfect5:2 resonance,and whose orbits are stable.Figure3shows the data averaged into bins in both a and e such that each bin contains roughly25points.The shade of each square represents the fraction of planets in that bin which survived,and has a Poisson error of20%.Over-plotted are con-tours of constant survival rate,also spaced by20%,to show the underlying distribution and the location of maxima.The outer edge of the system’s habitable zone is marked by the black dashed line.Three local maxima are evident in the Figure:1) a∼0.92AU,e∼0.12,2)a∼1.02AU,e∼0.1,and3)the absolute maximum at a∼0.98AU,e∼0.07.Each of these maxima is located in the habitable zone of the system,although maximum2is at the outer edge.In the absence of a test planet,the longitudes of periastron of the inner and outer giant planet librate about each other with an amplitude of roughly31◦and a precession period of171kyr. With the insertion of a Saturn-mass test planet,wefind evidence for secular resonances in various configurations of test planets. Fig.4shows the time evolution of the longitudes of periastron of each planet in two cases.The initial orbital elements for the test planet in these systems were(a,e)=(0.90AU,0.11)(top panel)and(1.01AU,0.08)(bottom panel).The top panel shows a system in which the Saturn-mass test planet is in a strong sec-ular resonance with the inner giant planet,as the orientation of the two planets’orbits are tracking each other with time.The bottom panel shows a case in which the test planet’s longitude of periastron is librating about that of the outer giant planet.At the same time,the test planet’s orbit tracks that of the inner gi-ant planet for over half of its precession cycle of∼7.5kyr(e.g. 231to237kyr).An additional,1.5kyr oscillation is superim-posed on the evolution of the test planet.The secular dynamics of the test planet in this case are affected by both giant planets in a complex way,yet the system is stable.We expect simple systems in secular resonance to be stable because of the avoid-ance of close approaches between planets.The presence of the Saturn-mass test planets makes an analytical treatment of the secular resonance structure of the system beyond the scope of this paper.Note in Fig.4that the precession rates of both the inner and outer giant planets are different in the top and bottom panels,due to the different locations of the test planet.3.2.HD38529The resonant structure of HD38529is quite different from that of HD37124,as the separation between the two known planets is much larger.The stable region for test particles lies between0.27and0.82AU,with eccentricities up to0.3.The inner edge is cut off by the1:3resonance with the inner planet. We see no evidence of secular resonances playing a significant role in the dynamics.We integrated the orbits of200Saturns in this system,of which191(95.5%)survived for100Myr.Figure5shows the data binned and over-plotted with contours as in Fig.3.The only unstable regions in this system lie at small semimajor axes and high eccentricities.The vast majority of the zone which is stable for massless test particles is also stable for Saturn-mass planets.It is therefore difficult to dynamically constrain the lo-cation of such a planet beyond the results of Paper1,although its orbit would likely be stable in the given region.3.3.55CancriThis system is interesting dynamically,as it is composed of an interior pair of planets in3:1mean motion resonance with a distant,separated companion.Paper1showed that there exists a large region between the inner pair and the outer planet which is stable for test particles,at0.7AU<a<3.4AU,with ec-centricities up to0.2.This stable region is bounded at its inner edge by the1:5resonance with the inner planet at0.72AU,and at its outer edge by the5:2resonance with the outer planet at 3.2AU.Several mean motion resonances with the outer planet are located in the stable region,notably the3:1resonance at 2.84AU,the4:1resonance at2.34AU,and the5:1resonance at2.02AU.We integrated the orbits of Saturns in512locations within this zone,and384(75%)of these survived for100Myr.Fig-ure6shows the distribution in(a,e)space of our experiments, in the same format as Figs.3and5,with Poisson errors of∼320%per bin.We see three local maxima:1)a relatively nar-row maximum at a∼1.0AU,e∼0.03,2)a broad maximum centered roughly at a∼2.0AU,e∼0.08but which extends to higher values of a,and3)a∼3AU,e∼0.17.Region1is of great astrobiological interest,as it lies in the habitable zone of its parent star,which is bounded by the black dashed lines.Re-gion3is bordered by the3:1(2.84AU)and5:2(3.2AU)mean motion resonances with the outer planet.We see no clear trend of survival rate with mean anomaly near these resonances.3.4.HD74156Paper1found that no test particles survived in this system for longer than1Myr.The region in which they survived the longest was for a between0.5and1.5AU at relatively low eccentricities.The1:5mean motion resonance(with the in-ner planet)is at0.82AU and the5:1resonance(with the outer planet)at1.3AU are located at the outskirts of the region we investigate.Therefore,only very high order mean motion reso-nances are found in the center.Wefind no evidence of secular resonances in the region.We performed600integrations of Saturn-mass planets in this system with a in the above mentioned region,and e between0 and0.2.Of these600Saturns,296(49%)survived for100Myr. Figure7shows the distribution of the surviving planets in these simulations.We see three small islands of stability at(a,e)≃: 1)(1.0AU,0.02),2)(1.0AU,0.1),and3)(1.2AU,0.13).These three islands lie at a slightly higher survival rate than the sur-rounding,larger region of stability between0.9-1.2AU with e≤0.15,in which the survival rate is75%.We see a strong trend in the survival rate of planets as a func-tion of semimajor axis,as shown in Fig.8.The fraction of sys-tems which are stable for100Myr increases sharply between 0.8and1.0AU,thenflattens off and decreases slightly past1.2 AU.The stable zones found in Fig.7lie at the peak of the curve.4.DISCUSSIONMenou&Tabachnik(2003;hereafter MT)investigated the possibility of Earth-sized planets residing in the habitable zones (HZs)of known extrasolar planetary systems.The location of the HZ is a function of the luminosity(and therefore mass)of the host star,as well as the atmospheric composition of the planet(Kasting et al.1993).For each system MT integrated the orbits of100massless test particles in the HZ for106years. They considered all four of our systems.The HZs for each sys-tem are as follows–HD37124:0.6-1.2AU,HD38529:1.4-3 AU,HD74156:0.6-1.2AU,and55Cnc:0.7-1.3AU.MT found no surviving planets in the HZ of HD37124.Their stability criterion requires a particle to remain in the HZ at all times, limiting its eccentricity such that the particle’s aphelion and perihelion remain in the HZ.Paper1used over500test par-ticles to systematically map out the region in HD37124which is stable for test particles,finding it to be centered at1AU.The eccentricities in this stable region are small enough to keep test particles in the HZ of the system throughout their orbits.In ad-dition,wefind three local maxima of the survival rate Saturn-mass planets in this system,all of whose orbits remain in the HZ.For HD38529our results are consistent with MT,as the sta-ble region from Paper1lies well outside the HZ,and the region we investigated with Saturns does not overlap with the HZ.In the case of55Cnc our results are again consistent with MT,who find that a significant fraction of low-inclination test particles survive at1.0AU,with eccentricities centered on0.09.The sta-ble region for55Cnc from Paper1encompasses the HZ entirely for eccentricities below0.25.In addition,Table3shows a max-imum in the survival rate of Saturns at(a,e)=(1.0AU,0.03), very close to the value from MT.MT’s results for HD74156are consistent with Paper1,but we have found two regions in the HZ which are stable for Saturn-mass planets in83%of cases. However,this may be due to the fact that the orbital elements used by MT are different than those we have used here.In par-ticular,the semimajor axis of the outer planet used here is0.35 AU larger(3.82AU vs3.47AU),increasing the separation of the two giant planets and therefore possibly causing the region in between to become more stable for an additional companion. Note that the current value for HD74156c is3.40AU(Naef et al.2004).Dvorak et al.(2003)investigated the possibility of an unseen planet in HD74156,using both test particles and massive ones. Theyfind a broad,relatively stable region for test particles be-tween0.9and1.4AU,with the most stable location being at a =1.25AU and e<0.2.This is a region in which Paper1found no stable test particle orbits.Fig.8shows a plateau in surviv-ability between1.0and1.25AU.Dvorak et al.(2003)found no trend in the results of their simulations of massive planets,and concluded that the presence of an unseen companion in the sys-tem was unlikely.Further observations will shed light on this issue,although the75%survival rate of Saturns for the entire region with0.9AU<a<1.2AU,e≤0.15suggests that this is a real possibility.Note again that the best-fit orbit of the outer planet in this system has recently been revised to a=3.40AU, e=0.58(Naef et al.2004).The closer proximity and higher ec-centricity of this planet strongly affects the dynamics between the two known plants.Both Dvorak et al.(2003)and Paper I assume the orbital elements from Table1in their calculations.5.CONCLUSIONSWe have found specific locations in four known extrasolar planetary systems in which Saturn-mass planets could exist on stable orbits.Such a planet would lie just below the detec-tion threshold of current radial velocity surveys,and may be detected in the near future.Table3summarizes our results, detailing the location in(a,e)space of each maximum in the survival rate for each of our four candidate systems.If an ad-ditional planet is discovered in the stable region of one of these systems,it would mark thefirst successful prediction of a planet since John Couch Adams predicted the existence of Neptune in 1845based on perturbations to Uranus’orbit.Does the presence of a stable region imply the presence of a planet?Must all systems contain as many planets as they can? Laskar(1996)speculated that“a planetary system will always be in this state of marginal stability,as a result of its gravita-tional interactions.”The“packed planetary systems”(PPS)hy-pothesis,presented in Paper1(see also Barnes&Quinn,2004), extends this idea by suggesting that all systems contain as many planets as they can dynamically support without self-disrupting. All systems may be on the edge of stability,but observational constraints prevent the detection of smaller or more distant bod-ies which push apparently stable systems to this edge.The formation scenario of a planet of any size in between two gas giant planets is of great interest.In the Solar System no stable regions exist between the orbits of the gas giants.The detailed formation scenario of a smaller giant planet between two others is unclear,be it through gravitational instability(e.g. Mayer et al.2002)or core-accretion(Pollack et al.1996).Gas giant planets at small orbital radii may have formed farther out4in the protoplanetary disk and migrated inward,which further complicates this formation scenario.Certain stable regions in HD37124,55Cnc and HD74156are located in the habitable zones of their parent stars(see Table 3).Clearly,the discovery of a planet of any size in these re-gions is of great astrobiological importance,as any giant planet would likely have one or more large moons.Understanding the formation of terrestrial planets in these systems is vital.In the upcoming third paper of the“predicting planets”series(Ray-mond&Barnes2004)we present results of simulations of ter-restrial planet formation in between the known giant planets in the same four systems examined here.6.ACKNOWLEDGMENTSWe thank Tom Quinn and Andrew West for many helpful dis-cussions,and Chance Reschke for his assistance in the comple-tion of the simulations presented in this paper.This work was funded by grants from the NASA Astrobiology Institute,the NSF,and a NASA GSRP.These simulations were performed on computers donated by the University of Washington Stu-dent Technology Fund.These simulations were performed un-der CONDOR.3REFERENCESBaranne,A.et al.1996,A&AS,119,373Barnes,R.&Quinn,T.,2004,ApJ,in press,astro-ph/0401171Barnes,R.&Raymond,S.N.,2004(Paper1),ApJ submitted,astro-ph/0402542Butler,R.P.,Marcy,G.W.,Williams,E.,McCarthy,C.,Dosanjh,P.,&V ogt, S.S.1996,PASP,108,500Butler,R.P.,Marcy,G.W.,V ogt,S.S.,Fischer,D.A.,Henry,G.W.,Laughlin, G.,&Wright,J.T.2003,ApJ,582,455Chambers,J.E.1999,MNRAS,304,793Charbonneau,D.,Brown,T.M.,Latham,D.W.,&Mayor,M.2000,ApJ,529, L45Dvorak,R.,Pilat-Lohinger,E.,Funk,B.,&Freistetter,F.2003,A&A,410,L13 Fischer,D.A.et al.2003,ApJ,586,1394Fischer,D.A.,Butler,R.P.,Marcy,G.W.,V ogt,S.S.,&Henry,G.W.2003, ApJ,590,1081Ji,J.,Liu,L.,Kinoshita,H.,Zhou,J.,Nakai,H.,&Li,G.2003,ApJ,591,L57 Kasting,J.F.,Whitmire,D.P.,&Reynolds,R.T.1993,Icarus,101,108Laskar,J.1996,Celestial Mechanics and Dynamical Astronomy,64,115 Laughlin,G.,Chambers,J.,&Fischer,D.A.2002,ApJ,579,455Marcy,G.W.,Butler,R.P.,&V ogt,S.S.2000,ApJ,536,L43Marcy,G.W.et al.2001,ApJ,555,418Mayer,L.,Wadsley,J.,Quinn,T.,&Stadel,J.,2002,Science,298,1756 Menou,K.&Tabachnik,S.2003,ApJ,583,473Naef,D.,Mayor,M.,Beuzit,J.L.,Perrier,C.,Queloz,D.,Sivan,J.P.,&Udry, S.2004,A&A414,351Pollack et al.1996,Icarus,124,62Raymond,S.N.&Barnes,R.2004,ApJ,submitted,astro-ph/Rivera&Lissauer2000,ApJ,530,454Rivera&Lissuaer2001,ApJ,558,392Rivera&Haghighipour2003,S cientific Frontiers in Research on Extrasolar Planets,ASP Conference Series,294,205Udry,S.,Mayor,M.,Naef,D.,Pepe,F.,Queloz,D.,Santos,N.C.,&Burnet, M.2002,A&A,390,2673CONDOR is publicly available at/condorF IG. 1.—The distribution in(a,e)space of472Saturn-mass planets in HD37124.Solid dots represent systems which were stable for100Myr,and crosses represent unstable configurations.5F IG.2.—The survival rate of Saturn-mass planets in HD37124as a function of semimajor axis,with Poisson error bars.Note the strong instability at the2:5 mean motion resonance,and the stable regions immediately interior and exterior.F IG.3.—The data for HD37124from Fig.1,binned on the a and e axes.The shade of each bin represents the fraction of planets in that bin which survived for 100Myr,with Poisson error of roughly20%.Contours of constant survival rate are over-plotted to bring out structure,spaced by20%.The black dashed line is the outer edge of the system’s habitable zone.Note the three local maxima,including one on either side of the2:5resonance at0.995AU.6F IG.4.—Evolution of the orientation of orbits(measured by the longitude of periastron)for two test systems of HD37124.The orbital elements of the Saturn-mass test planets are(a,e)=(0.90AU,0.11)(top)and(1.01AU,0.08)(bottom).Both systems were stable for100Myr.T ABLE1O RBITAL P ARAMETERS OF S ELECTED P LANETARY S YSTEMSSystem Planet M(M J)a(AU)eϖT(JD)b0.5497.0c 2.95265.0b0.12987.7c 3.6814.7b0.11599.0c0.24161.0d 5.9201.0b0.28185.0c 3.82272.01Bestfit values as of August22,2002.The current bestfit for planet c is a=3.40AU,e=0.58(Naef et al.2003).7F IG.5.—Binned data from200simulations of Saturns in HD38529with contours of constant survival rate over-plotted,as in Fig.3.Contours of constant survival rate are spaced by25%.The only unstable systems lie at low a and high e.F IG.6.—Binned data from512simulations of Saturns in55Cnc,with contours of constant survival rate spaced by20%.The black dashed lines indicate the boundaries of the system’s habitable zone.Note the maxima at(a,e)≃(1.03AU,0.03),(2.0AU,0.08),and(3.0AU,0.17).8F IG.7.—Binned data from600simulations of Saturns in HD74156,formatted as in Fig.3,with contours of constant survival rate spaced by20%.The dashed lines indicate the boundaries of the system’s habitable zone.The absolute maximum is located at(a,e)≃(1.0AU,0.02)and two local maximum are at(1.0AU, 0.10)and(1.2AU,0.13).F IG.8.—Survival rate of Saturns in HD74156as a function of semimajor axis,with statistical error bars.Note the strong increase toward1AU and the plateau between1.0and1,3AU.9 T ABLE2I NITIAL C ONDITIONS FOR S IMULATIONSSystem∆a(AU)∆e N(Saturns)0.9–1.1472HD385290.0–0.30.7–3.2512HD7415610.0–0.21Paper1found that no test particles in HD74156sur-vived for longer than1Myr.In our simulations,however,we sample the given region of parameter space.T ABLE3S IMULATION R ESULTSSystem Stable Region(a,e)1Survival Rate2(0.92AU,0.12)∗(0.98AU,0.07)∗(1.02AU,0.1)∗(0.3-0.8AU,0.0-0.15)(1.0AU,0.03)∗(2.0AU,0.08)(3.0AU,0.17)(1.0AU,0.02)∗(1.0AU,0.10)∗(1.2AU,0.13)1Local maxima of the survival rate,i.e.the center ofeach bin from Figs3,5,6,and7in which the survival rateis a maximum.The exact location of the stable region isuncertain on the order of the bin size.2Survival Rate for all simulations in the binned region inwhich the stable region is located.See Figs3,5,6,and7.∗Stable regions which lie in the habitable zone of theirparent stars,as defined by Kasting et al.(1993).。