Impulse Radio Systems with Multiple Types of Ultra-Wideband Pulses
罗斯蒙特3051s 3051sf系列流量计多变量传感器快速入门指南说明书
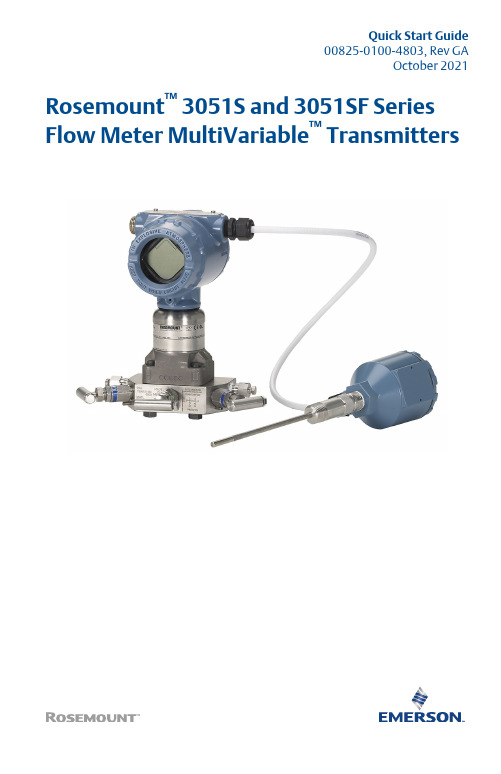
Quick Start Guide00825-0100-4803, Rev GAOctober 2021 Rosemount™ 3051S and 3051SF Series Flow Meter MultiVariable™ TransmittersQuick Start Guide October 2021 ContentsAbout this guide (3)Mount the transmitter (5)Consider housing rotation (11)Set the switches (12)Connect wiring and power up (13)Engineering Assistant installation (17)Flow configuration (19)Verifying device configuration (28)Trimming the transmitter (32)Safety instrumented systems installation (33)Product certifications (34)2Rosemount 3051SMVOctober 2021Quick Start Guide1About this guideThis guide provides basic guidelines to install the Rosemount 3051SMultiVariable Transmitter. It also provides the basic Rosemount 3051SMVconfiguration guidelines for the Rosemount 3051SFA, Rosemount 3051SFC,and Rosemount 3051SFP. It does not provide instructions for detailedconfiguration, diagnostics, maintenance, service, troubleshooting, orinstallations. Refer to the Rosemount 3051SMV Reference Manual for moreinstruction. The manual and this guide are also available electronically at/Rosemount.1.1Safety messagesWARNINGFailure to follow these installation guidelines could result in death orserious injury.Ensure only qualified personnel perform the installation.ExplosionsExplosions could result in death or serious injury.Installation of device in an explosive environment must be in accordancewith appropriate local, national, and international standards, codes, andpractices.Review the Hazardous Locations Certifications for any restrictionsassociated with a safe installation.Process leaksProcess leaks could result in death or serious injury.Install and tighten thermowells and sensors before applying pressure.Do not remove the thermowell while in operation.Conduit/cable entriesUnless marked, the conduit/cable entries in the transmitter housing usea ½–14 NPT thread form. Entries marked “M20” are M20 × 1.5 threadform. On devices with multiple conduit entries, all entries will have thesame thread form. Only use plugs, adapters, glands, or conduit with acompatible thread form when closing these entries.When installing in a hazardous location, use only appropriately listed orEx certified plugs, glands, or adapters in cable/conduit entries.Quick Start Guide3Quick Start Guide October 2021 WARNINGElectrical shockElectrical shock could cause death or serious injury.Avoid contact with the leads and terminals. High voltage that may bepresent on leads can cause electrical shock.Unless marked, the conduit/cable entries in the housing use a ½–14 NPTthread form. Entries marked “M20” are M20 × 1.5 thread form. Ondevices with multiple conduit entries, all entries will have the samethread form. Only use plugs, adapters, glands, or conduit with acompatible thread form when closing these entries.When installing in a hazardous location, use only appropriately listed orEx certified plugs, glands, or adapters in cable/conduit entries.Physical accessUnauthorized personnel may potentially cause significant damage to and/ormisconfiguration of end users’ equipment. This could be intentional orunintentional and needs to be protected against.Physical security is an important part of any security program andfundamental to protecting your system. Restrict physical access byunauthorized personnel to protect end users’ assets. This is true for allsystems used within the facility.4Rosemount 3051SMVOctober 2021Quick Start Guide 2Mount the transmitter2.1Liquid flow applicationsProcedure1.Place taps to the side of the line.2.Mount beside or below the taps.3.Mount the transmitter so that the drain/vent valves are orientedupward.AA. Direction of flow2.2Gas flow applicationsProcedure1.Place taps in the top or side of the line.Mount beside or above the taps.2.A. Direction of flowQuick Start Guide52.3Steam flow applicationsProcedure1.Place taps to the side of the line.2.Mount beside or below the taps.3.Fill impulse lines with water.AA. Direction of flow2.4Mounting bracketsFigure 2-1: Mounting Bracket – Coplanar FlangePanel mountPipe mountQuick Start Guide October 2021 6Rosemount 3051SMVFigure 2-2: Mounting Brackets – Traditional FlangePanel mountPipe mountFigure 2-3: Mounting Brackets – In-linePanel mountPipe mount2.5Bolting considerationsIf the transmitter installation requires assembly of a process flange,manifold, or flange adapters, follow these assembly guidelines to ensure atight seal for optimal performance characteristics of the transmitter. Onlyuse bolts supplied with the transmitter or sold by Emerson as spare parts.Figure 2-4 illustrates common transmitter assemblies with the bolt lengthrequired for proper transmitter assembly.October 2021Quick Start Guide Quick Start Guide7Figure 2-4: Common Transmitter AssembliesA 4 × 1.75-in.(44 mm)D4 × 1.75-in.(44 mm)4 × 2.25-in.(57 mm)C4 × 1.75-in. (44 mm) 4 × 1.50-in.(38 mm)4 × 2.88-in.(73 mm)A.Transmitter with coplanar flange B.Transmitter with coplanar flange and optional flange adapters C.Transmitter with traditional flange and optional flange adapters D.Transmitter with coplanar flange and optional Rosemount Conventional Manifold and flange adaptersNoteFor all other manifolds, contact Customer Central technical support.Bolts are typically carbon steel or stainless steel. Confirm the material byviewing the markings on the head of the bolt and referencing Table 2-1 . Ifbolt material is not shown in Table 2-1, contact the local Emersonrepresentative for more information.Use the following bolt installation procedure:Procedure1.Carbon steel bolts do not require lubrication and the stainless steelbolts are coated with a lubricant to ease installation. However, noadditional lubricant should be applied when installing either type ofbolt.2.Finger-tighten the bolts.3.Torque the bolts to the initial torque value using a crossing pattern.See Table 2-1 for initial torque value.4.Torque the bolts to the final torque value using the same crossing pattern. See Table 2-1 for final torque value.Quick Start Guide October 20218Rosemount 3051SMVOctober 2021Quick Start Guide5.Verify the flange bolts are protruding through the sensor modulebefore applying pressure (see Figure 2-5).ExampleTable 2-1: Torque Values for the Flange and Flange Adapter BoltsFigure 2-5: Proper Bolt InstallationA.BoltB.Sensor moduleQuick Start Guide92.6O-rings with flange adaptersWARNINGFailure to install proper flange adapter O-rings may cause process leaks,which can result in death or serious injury. Only use the O-ring that isdesigned for its specific flange adapter.DA.Flange adapterB.O-ring C.PTFE-based profile (square)D.Elastomer profile (round)Whenever the flange or adapters are removed, visually inspect the O-rings.Replace them if there are any signs of damage, such as nicks or cuts. If the O-rings are replaced, re-torque the flange bolts and alignment screws afterinstallation to compensate for seating of the O-rings.Quick Start Guide October 202110Rosemount 3051SMV3Consider housing rotationTo improve field access to wiring or to better view the optional LCD display:Procedure1.Loosen the housing rotation set screw.2.Turn the housing up to 180° left or right of its original (as shipped)position.3.Re-tighten the housing rotation set screw.Figure 3-1: Transmitter Housing Set ScrewA.LCD displayB.Housing rotation set screw (3/32-in.)CAUTIONDo not rotate the housing more than 180° without first performing adisassembly procedure. Over-rotation may sever the electricalconnection between the sensor module and the electronics.4Set the switchesThe transmitter’s default configuration sets the alarm condition to high (HI)and the security to off.Procedure1.If the transmitter is installed, secure the bus and remove power.2.Required: Remove the transmitter cover opposite the field terminalside. Do not remove the instrument covers in explosiveenvironments when the circuit is live.3.Slide the Security and Alarm switches into the preferred position byusing a small screwdriver.NoteThe Security switch will need to be in the off position in order to makeany configuration changes.4.Required: In order to meet explosion-proof requirements, reinstallthe housing cover and tighten so the cover is fully seated with metalto metal contact between the housing and cover. After the cover isseated properly, replace the flathead screw located on the bottom ofthe housing cover.Figure 4-1: Transmitter Switch ConfigurationA BA.SecurityB.AC Termination5Connect wiring and power upCAUTIONDo not connect the power across the test terminals. Power could damagethe test diode in the test connection. Twisted pairs yield best results. Use 24to 14 AWG wire and do not exceed 5,000 ft. (1500 m).Use the following steps to wire the transmitter:Procedure1.Remove the cover on the field terminals side of the housing.2.Connect the positive lead to the “PWR/COMM +” terminal, and thenegative lead to the “PWR/COMM –” terminal.3.If the optional process temperature input is not installed, plug andseal the unused conduit connection. If the input is being utilized, seeInstall optional process temperature input (Pt 100 RTD sensor) formore information.When the enclosed pipe plug is utilized in the conduit opening, itmust be installed with a minimum engagement of five threads tocomply with explosion-proof requirements. Refer to the Rosemount™3051SMV Reference Manual for more information.4.If applicable, install wiring with a drip loop. Arrange the drip loopso the bottom is lower than the conduit connections and thetransmitter housing.5.Reinstall the housing cover and tighten so that metal contacts metalto meet explosion-proof requirements.Figure 5-1 shows the wiring connections necessary to power aRosemount 3051SMV and enable communications with a hand-heldField Communicator.Figure 5-1: Transmitter WiringA. Power supplyNoteInstallation of the transient protection terminal block does notprovide transient protection unless the Rosemount 3051SMVhousing is properly grounded.5.1Conduit electrical connector wiring (option GE or GM)For Rosemount 3051SMV with conduit electrical connectors GE or GM, referto the cordset manufacturer’s installation instructions for wiring details. ForFM Intrinsically Safe, Division 2 hazardous locations, install in accordancewith Rosemount drawing 03151-1009 to maintain outdoor rating (NEMA®4X and IP66). See the Rosemount 3051SMV Reference Manual.5.2Power supplyThe dc power supply should provide power with less than two percent ripple.The total resistance load is the sum of the resistance of the signal leads andthe load resistance of the controller, indicator, intrinsic safety barriers, andrelated components.Figure 5-2: Load Limitation5.3Install optional process temperature input (Pt 100 RTDsensor)NoteTo meet ATEX/IECEx Flameproof certification, only ATEX/IECEx Flameproofcables (temperature input code C30, C32, C33, or C34) may be used.Procedure1.Mount the Pt 100 RTD sensor in the appropriate location.NoteUse shielded four-wire cable for the process temperatureconnection.2.Connect the RTD cable to the Rosemount 3051SMV by inserting thecable wires through the unused housing conduit and connect to thefour screws on the transmitter terminal block. An appropriate cablegland should be used to seal the conduit opening around the cable.3.Connect the RTD cable shield wire to the ground lug in the housing.Figure 5-3: RTD Wiring ConnectionA.Ground lugB.RTD cable assembly wiresC.Pt 100 RTD sensor6Engineering Assistant installationEngineering Assistant 6.1 or laterThe Rosemount 3051SMV Engineering Assistant 6.1 or later is PC-basedsoftware that performs configuration, maintenance, diagnostic functions,and serves as the primary communication interface to the transmitter withthe fully compensated mass and energy flow feature board.The Rosemount 3051SMV Engineering Assistant software is required tocomplete the flow configuration.To ensure correct operation, download the most current version of theEngineering Assistant software at /Rosemount-Engineering-Assistant.6.1System requirementsThe following are the minimum system requirements to install theRosemount 3051SMV Engineering Assistant software:•Pentium®-grade processor: 500 MHz or faster•Operating system: Windows™ XP Professional (32-bit), or Windows 7 (32-bit or 64-bit)•256 MB RAM•100 MB free hard disk space•RS232 serial port or USB port (for use with HART® modem)•CD-ROM6.2Install Rosemount 3051SMV Engineering Assistant 6.1 orlaterProcedure1.Uninstall any existing versions of Engineering Assistant 6.2.Insert the new Engineering Assistant disk into the CD-ROM.3.Windows should detect the presence of a CD and start theinstallation program. Follow the on-screen prompts to finish theinstallation. If Windows does not detect the CD, use WindowsExplorer or My Computer to view the contents of the CD-ROM, andthen double click the SETUP.EXE program.4. A series of screens (Installation Wizard) will appear and assist in theinstallation process. Follow the on-screen prompts. It isrecommended to use the default installation settings.NoteEngineering Assistant versions 6.1 or later require the use ofMicrosoft®.NET Framework version 4.0 or later. If .NET version 4.0 isnot currently installed, the software will be automatically installedduring the Engineering Assistant installation. Microsoft .NET version4.0 requires an additional 200 MB of disk space.6.3Connect to a personal computerProcedure1.Remove the cover from the field terminals side of the housing.2.Power the device as outlined in Connect wiring and power up.3.Connect the HART modem cable to the PC.4.On the side of the transmitter marked “Field Terminals,” connect themodem mini-grabbers to the two terminals marked “PWR/COMM.”unch the Engineering Assistant software. For more information onlaunching software, see Launch Engineering Assistant 6.1 or later.6.Once the configuration is complete, replace cover and tighten untilmetal contacts metal to meet explosion-proof requirements.Figure 6-1 shows how to connect a computer to a Rosemount3051SMV.Figure 6-1: Connecting a PC to the TransmitterA.Power supplyB.Modem7Flow configurationRosemount 3051SMV Engineering Assistant 6.1 or laterThe Rosemount 3051SMV Engineering Assistant is designed to guide theuser through the setup of the flow configuration for a Rosemount 3051SMV.The flow configuration screens allow the user to specify the fluid, operatingconditions, and information about the primary element, including insidepipe diameter. This information will be used by the Rosemount 3051SMVEngineering Assistant software to create flow configuration parameters thatwill be sent to the transmitter or saved for future use.Online and offline modesThe Engineering Assistant software can be used in two modes: Online andOffline. In Online mode, the user can receive the configuration from thetransmitter, edit the configuration, send the changed configuration to thetransmitter, or save the configuration to a file. In offline mode, the user cancreate a new flow configuration and save the configuration to a file or openand modify an existing file.The following pages provide instructions on creating a new flowconfiguration in offline mode. For more information on other functionality,see the Rosemount 3051SMV Reference Manual.7.1Basic navigation overviewFigure 7-1: Engineering Assistant Basic Navigation OverviewAFGHB C D E7.2Launch Engineering Assistant 6.1 or laterFlow configuration for the Rosemount 3051SMV is achieved by launchingthe Engineering Assistant software from the Start menu.Procedure1.Select the Start menu→All Programs→Engineering Assistant.Engineering Assistant will open to the screen shown in Figure 7-2.2.Select Offline button located in the lower right hand corner of thescreen shown in Figure 7-2.Figure 7-2: Engineering Assistant Device Connection Screen7.3Use Preferences tabThe Preferences tab, shown in Figure 7-3, allows you to select the preferredengineering units to display.Procedure1.Select the preferred engineering units.2.If Custom Units are selected, configure the Individual Parameters.3.Check the box if unit preferences should be retained for futureEngineering Assistant sessions.Figure 7-3: Preferences Tab7.4Select fluid for database liquid/gasThe Fluid Selection tab shown in Figure 7-4 allows the user to choose theprocess fluid.Figure 7-4: Fluid Selection TabNoteThe following example will show a flow configuration for the database gas air used with a Rosemount 405C Conditioning Orifice Plate as the primary element. The procedure to set up any other fluid with any other primary element will be similar to this example. Natural gases, custom liquids, and custom gases require additional steps during the configuration. See the Rosemount 3051SMV Reference Manual for more information. Procedure1.Engineering Assistant may open to the Preferences tab. Using the tabsat the top of the screen, navigate to the Fluid Selection tab.2.Expand the Gas category (click on the + icon).3.Expand the Database Gas category.4.Select Air from the list of database fluids.5.Enter the Nominal Operating Pressure, select the Enter or Tab key.6.Enter the Nominal Operating Temperature, select the Enter or Tab key.Engineering Assistant will automatically fill in suggested operatingranges, as shown in Figure 7-4. These values may be edited asneeded by the user.7.Verify the Reference/Atmospheric Conditions are correct for theapplication. These values may be edited as needed.NoteReference pressure and temperature values are used by EngineeringAssistant to convert the flow rate from mass units to mass unitsexpressed as standard or normal volumetric units.8.Select Next to proceed to the Fluid Properties tab.7.5Fluid propertiesNoteThe Fluid Properties tab is an optional step and is not required to complete aflow configuration.The Fluid Properties tab for the database gas air is shown in Figure 7-5. This isused to verify the properties of the chosen fluid are acceptable.To check density, compressibility, and viscosity of the selected fluid at otherpressure and temperature values, enter a Pressure and Temperature andselect Calculate.NoteChanging the pressure and temperature values on the Fluid Properties tabdoes not affect the fluid configuration.Figure 7-5: Fluid Properties Tab7.6Select primary elementThe Primary Element Selection tab shown in Figure 7-6 allows the user tochoose the primary element.Figure 7-6: Primary Element Selection TabContinuing with the example configuration:Procedure1.Expand the Conditioning Orifice Plate category.2.Select 405C/3051SFC.3.Enter the measured Meter Tube Diameter (pipe ID) at a referencetemperature. If the meter tube diameter cannot be measured, selecta Nominal Pipe Size and Pipe Schedule to input an estimated valuefor the meter tube diameter (English units only).4.If necessary, edit the Meter Tube Material.5.Enter the Line Size and select the Beta of the conditioning orificeplate. The required primary element sizing parameters will bedifferent depending on what primary element was selected.6.If necessary, select a primary element Material from the dropdownmenu.7.Select Next > to advance to the Save/Send Configuration tab.NoteTo be in compliance with appropriate national or internationalstandards, beta ratios and differential producer diameters should bewithin the limits as listed in the applicable standards. TheEngineering Assistant software will alert the user if a primary element value exceeds these limits, but will allow the user to proceed with the flow configuration.7.7Save/send configurationThe Save/Send Configuration tab shown in Figure 7-7 allows you to verify,save, and send the configuration information to the transmitter with thefully compensated mass and energy flow feature board.Procedure1.Review the information under the Flow Configuration and DeviceConfiguration headings.NoteFor more information, see Verifying device configuration.Figure 7-7: Save/Send Configuration Tab2.Select the icon above each window to edit the configurationinformation in these windows.NoteThe user will be notified if the configuration has been modified sinceit was last sent to the transmitter. A warning message will be shownto the right of the Send Flow Data and/or Send Transmitter Data checkboxes.3.To send the configuration, select the Send To button.NoteThe Send Flow Data and Send Transmitter Data check boxes can beused to select what configuration data is sent to the transmitter. Ifeither check box is unselected, the corresponding data will not besent.4.The Engineering Assistant Device Connection screen will appear, seeFigure 7-8.Figure 7-8: Engineering Assistant Device Connection Screen5.Select the Search button located in the lower right hand corner ofthe screen. Engineering Assistant will begin to search for connected devices.6.When the search is completed, select the device to communicatewith and select Send Configuration button.NoteAfter the configuration is sent to the device, saving the configuration file is recommended. The user can select the Save button on theSave/Send screen or select Save from the program menu.Once the configuration is finished being sent to the device, the user will be notified by a pop-up dialog box.7.If finished with the configuration process, close EngineeringAssistant.8Verifying device configurationUse Rosemount 3051SMV Engineering Assistant or any HART-compliantmaster to communicate with and verify configuration of the Rosemount3051SMV.Table 8-1 shows the Field Communicator fast keys for the fully compensatedmass and energy flow. Table 8-2 shows the Fast Keys for the direct processvariable output.NoteDevice configuration procedures are given for Rosemount 3051SMVEngineering Assistant 6.1 or later and AMS Device Manager 9.0 or later in theRosemount 3051SMV Reference Manual.A check (✓) indicates the basic configuration parameters. At a minimum,these parameters should be verified as part of the configuration and startupprocedure.Table 8-1: Fast Keys for Fully Compensated Mass and Energy FlowTable 8-2: Fast Keys for Direct Process Variable Output9Trimming the transmitterTransmitters are shipped fully calibrated per request or by the factorydefault of full scale.9.1Zero trimA zero trim is a single-point adjustment used for compensating mountingposition and line pressure effects on static and differential pressure sensors.When performing a zero trim, ensure that the equalizing valve is open andall wet legs are filled to the correct level.The transmitter will only allow up to five percent of URL zero error to betrimmed.9.1.1Perform a zero trim using the Field CommunicatorProcedure1.Equalize or vent the transmitter and connect the FieldCommunicator (for more information on connecting,see Figure 5-1).2.If the device is equipped with a static pressure sensor, zero the sensorby inputting the following Fast Key sequence at the Rosemount3051SMV menu:e the zero trim (selection 1) for a transmitter equipped with a gagestatic pressure sensor or lower sensor trim (selection 2) for atransmitted equipped with an absolute static pressure sensor.NoteWhen performing a lower sensor trim on an absolute pressuresensor, it is possible to degrade the performance of the sensor ifinaccurate calibration equipment is used. Use a barometer that is atleast three times as accurate as the absolute sensor of thetransmitter.4.Zero the differential pressure sensor by inputting the following FastKey sequence at the Rosemount 3051SMV menu:10Safety instrumented systems installation For safety certified installations, refer to the appropriate reference manualfor the installation procedure and system requirements:•For DP only measurements (measurement type D) refer to theRosemount 3051S Reference Manual.•For MultiVariable measurements (measurement type 1–7) refer to the Rosemount 3051SMV Reference Manual.11Product certifications11.1Rosemount 3051SMV/3051SFxRev 2.10European directive informationA copy of the EC Declaration of Conformity can be found at the end of theQuick Start Guide. The most recent revision of the EC Declaration ofConformity can be found at /Rosemount.Ordinary location certificationAs standard, the transmitter has been examined and tested to determinethat the design meets the basic electrical, mechanical, and fire protectionrequirements by a nationally recognized test laboratory (NRTL) as accreditedby the Federal Occupational Safety and Health Administration (OSHA).Installing Equipment in North AmericaThe US National Electrical Code (NEC) and the Canadian Electrical Code(CEC) permit the use of Division marked equipment in Zones and Zonemarked equipment in Divisions. The markings must be suitable for the areaclassification, gas, and temperature class. This information is clearly definedin the respective codes.11.1.1USAE5 US Explosionproof (XP) and Dust-Ignitionproof (DIP)Certificate FM16US0089XStandards FM Class 3600 – 2011, FM Class 3615 – 2006, FM Class 3616 –2011, FM Class 3810 – 2005, ANSI/NEMA 250 – 2003 Markings XP CL I, DIV 1, GP B, C, D; T5; DIP CL II, DIV 1, GP E, F, G; CL III;T5(–50 °C ≤ T a ≤ +85 °C); Factory Sealed; Type 4XI5 US Intrinsically Safe (IS) and Nonincendive (NI)Certificate FM16US0233Standards FM Class 3600 –2011, FM Class 3610 – 2007, FM Class 3611 –2004, FM Class 3616 – 2006, FM Class 3810 – 2005, NEMA250 – 1991Markings IS CL I, DIV 1, GP A, B, C, D; CL II, DIV 1, GP E, F, G; Class III; Class1, Zone 0 AEx ia IIC T4; NI CL 1, DIV 2, GP A, B, C, D; T4(–50 °C ≤T a ≤ +70 °C) when connected per Rosemount drawing03151-1206; Type 4XNoteTransmitters marked with NI CL 1, DIV 2 can be installed in Division 2locations using general Division 2 wiring methods or Nonincendive FieldWiring (NIFW). See Drawing 03151-1206.US Intrinsic Safety (IS) and Nonincendive (NI)Certificate:1143113Standards:FM Class 3600:2011, FM Class 3610:2010, FM Class3611:2004, FM Class 3810:2005, UL50E (1st Ed.)Markings:IS Class I/II/III, Division 1, Groups A, B, C, D, T4/ E, F, and GT135 °C; Class I, Zone 0 AEx ia IIC T4 Ga;T4 (-50 °C ≤ T a ≤ +70 °C) [HART];T4 (-50 °C ≤ T a ≤ +60 °C) [Fieldbus];when connected per Rosemount drawing 03151-1207; Type4XIE US FISCO Intrinsically SafeCertificate FM16US0233Standards FM Class 3600 – 2011, FM Class 3610 – 2010, FM Class 3611 –2004, FM Class 3616 – 2006, FM Class 3810 – 2005, NEMA250 – 1991Markings IS CL I, DIV 1, GP A, B, C, D; T4(–50 °C ≤ Ta ≤ +70 °C); whenconnected per Rosemount drawing 03151-1006; Type 4X US FISCO Intrinsically SafeCertificate:1143113Standards:FM Class 3600:2011, FM Class 3610:2010, FM Class3611:2004, FM Class 3810:2005, UL50E (1st Ed.)Markings:IS Class I/II/III, Division 1, Groups A, B, C, D, T4/ E, F, and GT135 °C; Class I, Zone 0 AEx ia IIC T4 Ga;T4 (-50 °C ≤ T a ≤ +70 °C) [HART];T4 (-50 °C ≤ T a ≤ +60 °C) [Fieldbus];when connected per Rosemount drawing 03151-1207; Type4X11.1.2CanadaE6 Canada Explosionproof, Dust Ignition-proof, Division 2Certificate1143113。
数字无线通信系统中的调制(英文)
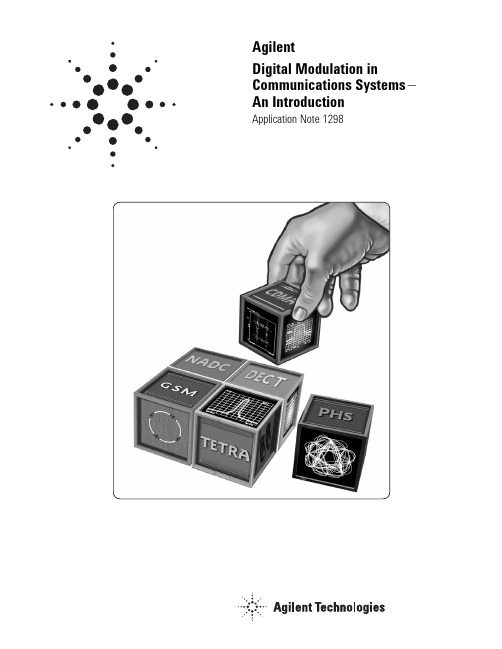
AgilentDigital Modulation in Communications Systems—An IntroductionApplication Note 1298This application note introduces the concepts of digital modulation used in many communications systems today. Emphasis is placed on explaining the tradeoffs that are made to optimize efficiencies in system design.Most communications systems fall into one of three categories: bandwidth efficient, power efficient, or cost efficient. Bandwidth efficiency describes the ability of a modulation scheme to accommodate data within a limited bandwidth. Power efficiency describes the ability of the system to reliably send information at the lowest practical power level.In most systems, there is a high priority on band-width efficiency. The parameter to be optimized depends on the demands of the particular system, as can be seen in the following two examples.For designers of digital terrestrial microwave radios, their highest priority is good bandwidth efficiency with low bit-error-rate. They have plenty of power available and are not concerned with power efficiency. They are not especially con-cerned with receiver cost or complexity because they do not have to build large numbers of them. On the other hand, designers of hand-held cellular phones put a high priority on power efficiency because these phones need to run on a battery. Cost is also a high priority because cellular phones must be low-cost to encourage more users. Accord-ingly, these systems sacrifice some bandwidth efficiency to get power and cost efficiency. Every time one of these efficiency parameters (bandwidth, power, or cost) is increased, another one decreases, becomes more complex, or does not perform well in a poor environment. Cost is a dom-inant system priority. Low-cost radios will always be in demand. In the past, it was possible to make a radio low-cost by sacrificing power and band-width efficiency. This is no longer possible. The radio spectrum is very valuable and operators who do not use the spectrum efficiently could lose their existing licenses or lose out in the competition for new ones. These are the tradeoffs that must be considered in digital RF communications design. This application note covers•the reasons for the move to digital modulation;•how information is modulated onto in-phase (I) and quadrature (Q) signals;•different types of digital modulation;•filtering techniques to conserve bandwidth; •ways of looking at digitally modulated signals;•multiplexing techniques used to share the transmission channel;•how a digital transmitter and receiver work;•measurements on digital RF communications systems;•an overview table with key specifications for the major digital communications systems; and •a glossary of terms used in digital RF communi-cations.These concepts form the building blocks of any communications system. If you understand the building blocks, then you will be able to under-stand how any communications system, present or future, works.Introduction25 5 677 7 8 8 9 10 10 1112 12 12 13 14 14 15 15 16 17 18 19 20 21 22 22 23 23 24 25 26 27 28 29 29 30 311. Why Digital Modulation?1.1 Trading off simplicity and bandwidth1.2 Industry trends2. Using I/Q Modulation (Amplitude and Phase Control) to Convey Information2.1 Transmitting information2.2 Signal characteristics that can be modified2.3 Polar display—magnitude and phase representedtogether2.4 Signal changes or modifications in polar form2.5 I/Q formats2.6 I and Q in a radio transmitter2.7 I and Q in a radio receiver2.8 Why use I and Q?3. Digital Modulation Types and Relative Efficiencies3.1 Applications3.1.1 Bit rate and symbol rate3.1.2 Spectrum (bandwidth) requirements3.1.3 Symbol clock3.2 Phase Shift Keying (PSK)3.3 Frequency Shift Keying3.4 Minimum Shift Keying (MSK)3.5 Quadrature Amplitude Modulation (QAM)3.6 Theoretical bandwidth efficiency limits3.7 Spectral efficiency examples in practical radios3.8 I/Q offset modulation3.9 Differential modulation3.10 Constant amplitude modulation4. Filtering4.1 Nyquist or raised cosine filter4.2 Transmitter-receiver matched filters4.3 Gaussian filter4.4 Filter bandwidth parameter alpha4.5 Filter bandwidth effects4.6 Chebyshev equiripple FIR (finite impulse response) filter4.7 Spectral efficiency versus power consumption5. Different Ways of Looking at a Digitally Modulated Signal Time and Frequency Domain View5.1 Power and frequency view5.2 Constellation diagrams5.3 Eye diagrams5.4 Trellis diagramsTable of Contents332 32 32 33 33 34 3435 35 3637 37 37 38 38 39 39 39 40 41 41 42 434344466. Sharing the Channel6.1 Multiplexing—frequency6.2 Multiplexing—time6.3 Multiplexing—code6.4 Multiplexing—geography6.5 Combining multiplexing modes6.6 Penetration versus efficiency7. How Digital Transmitters and Receivers Work7.1 A digital communications transmitter7.2 A digital communications receiver8. Measurements on Digital RF Communications Systems 8.1 Power measurements8.1.1 Adjacent Channel Power8.2 Frequency measurements8.2.1 Occupied bandwidth8.3 Timing measurements8.4 Modulation accuracy8.5 Understanding Error Vector Magnitude (EVM)8.6 Troubleshooting with error vector measurements8.7 Magnitude versus phase error8.8 I/Q phase error versus time8.9 Error Vector Magnitude versus time8.10 Error spectrum (EVM versus frequency)9. Summary10. Overview of Communications Systems11. Glossary of TermsTable of Contents (continued)4The move to digital modulation provides more information capacity, compatibility with digital data services, higher data security, better quality communications, and quicker system availability. Developers of communications systems face these constraints:•available bandwidth•permissible power•inherent noise level of the systemThe RF spectrum must be shared, yet every day there are more users for that spectrum as demand for communications services increases. Digital modulation schemes have greater capacity to con-vey large amounts of information than analog mod-ulation schemes. 1.1 Trading off simplicity and bandwidthThere is a fundamental tradeoff in communication systems. Simple hardware can be used in transmit-ters and receivers to communicate information. However, this uses a lot of spectrum which limits the number of users. Alternatively, more complex transmitters and receivers can be used to transmit the same information over less bandwidth. The transition to more and more spectrally efficient transmission techniques requires more and more complex hardware. Complex hardware is difficult to design, test, and build. This tradeoff exists whether communication is over air or wire, analog or digital.Figure 1. The Fundamental Tradeoff1. Why Digital Modulation?51.2 Industry trendsOver the past few years a major transition has occurred from simple analog Amplitude Mod-ulation (AM) and Frequency/Phase Modulation (FM/PM) to new digital modulation techniques. Examples of digital modulation include•QPSK (Quadrature Phase Shift Keying)•FSK (Frequency Shift Keying)•MSK (Minimum Shift Keying)•QAM (Quadrature Amplitude Modulation) Another layer of complexity in many new systems is multiplexing. Two principal types of multiplex-ing (or “multiple access”) are TDMA (Time Division Multiple Access) and CDMA (Code Division Multiple Access). These are two different ways to add diversity to signals allowing different signals to be separated from one another.Figure 2. Trends in the Industry62.1 Transmitting informationTo transmit a signal over the air, there are three main steps:1.A pure carrier is generated at the transmitter.2.The carrier is modulated with the informationto be transmitted. Any reliably detectablechange in signal characteristics can carryinformation.3.At the receiver the signal modifications orchanges are detected and demodulated.2.2 Signal characteristics that can be modified There are only three characteristics of a signal that can be changed over time: amplitude, phase, or fre-quency. However, phase and frequency are just dif-ferent ways to view or measure the same signal change. In AM, the amplitude of a high-frequency carrier signal is varied in proportion to the instantaneous amplitude of the modulating message signal.Frequency Modulation (FM) is the most popular analog modulation technique used in mobile com-munications systems. In FM, the amplitude of the modulating carrier is kept constant while its fre-quency is varied by the modulating message signal.Amplitude and phase can be modulated simultane-ously and separately, but this is difficult to gener-ate, and especially difficult to detect. Instead, in practical systems the signal is separated into another set of independent components: I(In-phase) and Q(Quadrature). These components are orthogonal and do not interfere with each other.Figure 3. Transmitting Information (Analog or Digital)Figure 4. Signal Characteristics to Modify2. Using I/Q Modulation to Convey Information72.3 Polar display—magnitude and phase repre-sented togetherA simple way to view amplitude and phase is with the polar diagram. The carrier becomes a frequency and phase reference and the signal is interpreted relative to the carrier. The signal can be expressed in polar form as a magnitude and a phase. The phase is relative to a reference signal, the carrier in most communication systems. The magnitude is either an absolute or relative value. Both are used in digital communication systems. Polar diagrams are the basis of many displays used in digital com-munications, although it is common to describe the signal vector by its rectangular coordinates of I (In-phase) and Q(Quadrature).2.4 Signal changes or modifications inpolar formFigure 6 shows different forms of modulation in polar form. Magnitude is represented as the dis-tance from the center and phase is represented as the angle.Amplitude modulation (AM) changes only the magnitude of the signal. Phase modulation (PM) changes only the phase of the signal. Amplitude and phase modulation can be used together. Frequency modulation (FM) looks similar to phase modulation, though frequency is the controlled parameter, rather than relative phase.Figure 6. Signal Changes or Modifications8One example of the difficulties in RF design can be illustrated with simple amplitude modulation. Generating AM with no associated angular modula-tion should result in a straight line on a polar display. This line should run from the origin to some peak radius or amplitude value. In practice, however, the line is not straight. The amplitude modulation itself often can cause a small amount of unwanted phase modulation. The result is a curved line. It could also be a loop if there is any hysteresis in the system transfer function. Some amount of this distortion is inevitable in any sys-tem where modulation causes amplitude changes. Therefore, the degree of effective amplitude modu-lation in a system will affect some distortion parameters.2.5 I/Q formatsIn digital communications, modulation is often expressed in terms of I and Q. This is a rectangular representation of the polar diagram. On a polar diagram, the I axis lies on the zero degree phase reference, and the Q axis is rotated by 90 degrees. The signal vector’s projection onto the I axis is its “I” component and the projection onto the Q axisis its “Q” component.Figure 7. “I-Q” Format92.6 I and Q in a radio transmitterI/Q diagrams are particularly useful because they mirror the way most digital communications sig-nals are created using an I/Q modulator. In the transmitter, I and Q signals are mixed with the same local oscillator (LO). A 90 degree phase shifter is placed in one of the LO paths. Signals that are separated by 90 degrees are also known as being orthogonal to each other or in quadrature. Signals that are in quadrature do not interfere with each other. They are two independent compo-nents of the signal. When recombined, they are summed to a composite output signal. There are two independent signals in I and Q that can be sent and received with simple circuits. This simpli-fies the design of digital radios. The main advan-tage of I/Q modulation is the symmetric ease of combining independent signal components into a single composite signal and later splitting such a composite signal into its independent component parts. 2.7 I and Q in a radio receiverThe composite signal with magnitude and phase (or I and Q) information arrives at the receiver input. The input signal is mixed with the local oscillator signal at the carrier frequency in two forms. One is at an arbitrary zero phase. The other has a 90 degree phase shift. The composite input signal (in terms of magnitude and phase) is thus broken into an in-phase, I, and a quadrature, Q, component. These two components of the signal are independent and orthogonal. One can be changed without affecting the other. Normally, information cannot be plotted in a polar format and reinterpreted as rectangular values without doing a polar-to-rectangular conversion. This con-version is exactly what is done by the in-phase and quadrature mixing processes in a digital radio. A local oscillator, phase shifter, and two mixers can perform the conversion accurately and efficiently.Figure 8. I and Q in a Practical Radio Transmitter Figure 9. I and Q in a Radio Receiver102.8 Why use I and Q?Digital modulation is easy to accomplish with I/Q modulators. Most digital modulation maps the data to a number of discrete points on the I/Q plane. These are known as constellation points. As the sig-nal moves from one point to another, simultaneous amplitude and phase modulation usually results. To accomplish this with an amplitude modulator and a phase modulator is difficult and complex. It is also impossible with a conventional phase modu-lator. The signal may, in principle, circle the origin in one direction forever, necessitating infinite phase shifting capability. Alternatively, simultaneous AM and Phase Modulation is easy with an I/Q modulator. The I and Q control signals are bounded, but infi-nite phase wrap is possible by properly phasing the I and Q signals.This section covers the main digital modulation formats, their main applications, relative spectral efficiencies, and some variations of the main modulation types as used in practical systems. Fortunately, there are a limited number of modula-tion types which form the building blocks of any system.3.1 ApplicationsThe table below covers the applications for differ-ent modulation formats in both wireless communi-cations and video. Although this note focuses on wireless communica-tions, video applications have also been included in the table for completeness and because of their similarity to other wireless communications.3.1.1 Bit rate and symbol rateTo understand and compare different modulation format efficiencies, it is important to first under-stand the difference between bit rate and symbol rate. The signal bandwidth for the communications channel needed depends on the symbol rate, not on the bit rate.Symbol rate =bit ratethe number of bits transmitted with each symbol 3. Digital Modulation Types and Relative EfficienciesBit rate is the frequency of a system bit stream. Take, for example, a radio with an 8 bit sampler, sampling at 10 kHz for voice. The bit rate, the basic bit stream rate in the radio, would be eight bits multiplied by 10K samples per second, or 80 Kbits per second. (For the moment we will ignore the extra bits required for synchronization, error correction, etc.)Figure 10 is an example of a state diagram of a Quadrature Phase Shift Keying (QPSK) signal. The states can be mapped to zeros and ones. This is a common mapping, but it is not the only one. Any mapping can be used.The symbol rate is the bit rate divided by the num-ber of bits that can be transmitted with each sym-bol. If one bit is transmitted per symbol, as with BPSK, then the symbol rate would be the same as the bit rate of 80 Kbits per second. If two bits are transmitted per symbol, as in QPSK, then the sym-bol rate would be half of the bit rate or 40 Kbits per second. Symbol rate is sometimes called baud rate. Note that baud rate is not the same as bit rate. These terms are often confused. If more bits can be sent with each symbol, then the same amount of data can be sent in a narrower spec-trum. This is why modulation formats that are more complex and use a higher number of states can send the same information over a narrower piece of the RF spectrum.3.1.2 Spectrum (bandwidth) requirementsAn example of how symbol rate influences spec-trum requirements can be seen in eight-state Phase Shift Keying (8PSK). It is a variation of PSK. There are eight possible states that the signal can transi-tion to at any time. The phase of the signal can take any of eight values at any symbol time. Since 23= 8, there are three bits per symbol. This means the symbol rate is one third of the bit rate. This is relatively easy to decode.Figure 10. Bit Rate and Symbol Rate Figure 11. Spectrum Requirements3.1.3 Symbol ClockThe symbol clock represents the frequency and exact timing of the transmission of the individual symbols. At the symbol clock transitions, the trans-mitted carrier is at the correct I/Q(or magnitude/ phase) value to represent a specific symbol (a specific point in the constellation).3.2 Phase Shift KeyingOne of the simplest forms of digital modulation is binary or Bi-Phase Shift Keying (BPSK). One appli-cation where this is used is for deep space teleme-try. The phase of a constant amplitude carrier sig-nal moves between zero and 180 degrees. On an I and Q diagram, the I state has two different values. There are two possible locations in the state dia-gram, so a binary one or zero can be sent. The symbol rate is one bit per symbol.A more common type of phase modulation is Quadrature Phase Shift Keying (QPSK). It is used extensively in applications including CDMA (Code Division Multiple Access) cellular service, wireless local loop, Iridium (a voice/data satellite system) and DVB-S (Digital Video Broadcasting — Satellite). Quadrature means that the signal shifts between phase states which are separated by 90 degrees. The signal shifts in increments of 90 degrees from 45 to 135, –45, or –135 degrees. These points are chosen as they can be easily implemented using an I/Q modulator. Only two I values and two Q values are needed and this gives two bits per symbol. There are four states because 22= 4. It is therefore a more bandwidth-efficient type of modulation than BPSK, potentially twice as efficient.Figure 12. Phase Shift Keying3.3 Frequency Shift KeyingFrequency modulation and phase modulation are closely related. A static frequency shift of +1 Hz means that the phase is constantly advancing at the rate of 360 degrees per second (2 πrad/sec), relative to the phase of the unshifted signal.FSK (Frequency Shift Keying) is used in many applications including cordless and paging sys-tems. Some of the cordless systems include DECT (Digital Enhanced Cordless Telephone) and CT2 (Cordless Telephone 2).In FSK, the frequency of the carrier is changed as a function of the modulating signal (data) being transmitted. Amplitude remains unchanged. In binary FSK (BFSK or 2FSK), a “1” is represented by one frequency and a “0” is represented by another frequency.3.4 Minimum Shift KeyingSince a frequency shift produces an advancing or retarding phase, frequency shifts can be detected by sampling phase at each symbol period. Phase shifts of (2N + 1) π/2radians are easily detected with an I/Q demodulator. At even numbered sym-bols, the polarity of the I channel conveys the transmitted data, while at odd numbered symbols the polarity of the Q channel conveys the data. This orthogonality between I and Q simplifies detection algorithms and hence reduces power con-sumption in a mobile receiver. The minimum fre-quency shift which yields orthogonality of I and Q is that which results in a phase shift of ±π/2radi-ans per symbol (90 degrees per symbol). FSK with this deviation is called MSK (Minimum Shift Keying). The deviation must be accurate in order to generate repeatable 90 degree phase shifts. MSK is used in the GSM (Global System for Mobile Communications) cellular standard. A phase shift of +90 degrees represents a data bit equal to “1,”while –90 degrees represents a “0.” The peak-to-peak frequency shift of an MSK signal is equal to one-half of the bit rate.FSK and MSK produce constant envelope carrier signals, which have no amplitude variations. This is a desirable characteristic for improving the power efficiency of transmitters. Amplitude varia-tions can exercise nonlinearities in an amplifier’s amplitude-transfer function, generating spectral regrowth, a component of adjacent channel power. Therefore, more efficient amplifiers (which tend to be less linear) can be used with constant-envelope signals, reducing power consumption.Figure 13. Frequency Shift KeyingMSK has a narrower spectrum than wider devia-tion forms of FSK. The width of the spectrum is also influenced by the waveforms causing the fre-quency shift. If those waveforms have fast transi-tions or a high slew rate, then the spectrumof the transmitter will be broad. In practice, the waveforms are filtered with a Gaussian filter, resulting in a narrow spectrum. In addition, the Gaussian filter has no time-domain overshoot, which would broaden the spectrum by increasing the peak deviation. MSK with a Gaussian filter is termed GMSK (Gaussian MSK).3.5 Quadrature Amplitude ModulationAnother member of the digital modulation family is Quadrature Amplitude Modulation (QAM). QAM is used in applications including microwave digital radio, DVB-C (Digital Video Broadcasting—Cable), and modems.In 16-state Quadrature Amplitude Modulation (16QAM), there are four I values and four Q values. This results in a total of 16 possible states for the signal. It can transition from any state to any other state at every symbol time. Since 16 = 24, four bits per symbol can be sent. This consists of two bits for I and two bits for Q. The symbol rate is one fourth of the bit rate. So this modulation format produces a more spectrally efficient transmission. It is more efficient than BPSK, QPSK, or 8PSK. Note that QPSK is the same as 4QAM.Another variation is 32QAM. In this case there are six I values and six Q values resulting in a total of 36 possible states (6x6=36). This is too many states for a power of two (the closest power of two is 32). So the four corner symbol states, which take the most power to transmit, are omitted. This reduces the amount of peak power the transmitter has to generate. Since 25= 32, there are five bits per sym-bol and the symbol rate is one fifth of the bit rate. The current practical limits are approximately256QAM, though work is underway to extend the limits to 512 or 1024 QAM. A 256QAM system uses 16 I-values and 16 Q-values, giving 256 possible states. Since 28= 256, each symbol can represent eight bits. A 256QAM signal that can send eight bits per symbol is very spectrally efficient. However, the symbols are very close together and are thus more subject to errors due to noise and distortion. Such a signal may have to be transmit-ted with extra power (to effectively spread the symbols out more) and this reduces power efficiency as compared to simpler schemes.Figure 14. Quadrature Amplitude ModulationCompare the bandwidth efficiency when using256QAM versus BPSK modulation in the radio example in section 3.1.1 (which uses an eight-bit sampler sampling at 10 kHz for voice). BPSK uses80 Ksymbols-per-second sending 1 bit per symbol.A system using 256QAM sends eight bits per sym-bol so the symbol rate would be 10 Ksymbols per second. A 256QAM system enables the same amount of information to be sent as BPSK using only one eighth of the bandwidth. It is eight times more bandwidth efficient. However, there is a tradeoff. The radio becomes more complex and is more susceptible to errors caused by noise and dis-tortion. Error rates of higher-order QAM systems such as this degrade more rapidly than QPSK as noise or interference is introduced. A measureof this degradation would be a higher Bit Error Rate (BER).In any digital modulation system, if the input sig-nal is distorted or severely attenuated the receiver will eventually lose symbol lock completely. If the receiver can no longer recover the symbol clock, it cannot demodulate the signal or recover any infor-mation. With less degradation, the symbol clock can be recovered, but it is noisy, and the symbol locations themselves are noisy. In some cases, a symbol will fall far enough away from its intended position that it will cross over to an adjacent posi-tion. The I and Q level detectors used in the demodulator would misinterpret such a symbol as being in the wrong location, causing bit errors. QPSK is not as efficient, but the states are much farther apart and the system can tolerate a lot more noise before suffering symbol errors. QPSK has no intermediate states between the four corner-symbol locations, so there is less opportunity for the demodulator to misinterpret symbols. QPSK requires less transmitter power than QAM to achieve the same bit error rate.3.6 Theoretical bandwidth efficiency limits Bandwidth efficiency describes how efficiently the allocated bandwidth is utilized or the ability of a modulation scheme to accommodate data, within a limited bandwidth. The table below shows the theoretical bandwidth efficiency limits for the main modulation types. Note that these figures cannot actually be achieved in practical radios since they require perfect modulators, demodula-tors, filter, and transmission paths.If the radio had a perfect (rectangular in the fre-quency domain) filter, then the occupied band-width could be made equal to the symbol rate.Techniques for maximizing spectral efficiency include the following:•Relate the data rate to the frequency shift (as in GSM).•Use premodulation filtering to reduce the occupied bandwidth. Raised cosine filters,as used in NADC, PDC, and PHS, give thebest spectral efficiency.•Restrict the types of transitions.Modulation Theoretical bandwidthformat efficiencylimitsMSK 1bit/second/HzBPSK 1bit/second/HzQPSK 2bits/second/Hz8PSK 3bits/second/Hz16 QAM 4 bits/second/Hz32 QAM 5 bits/second/Hz64 QAM 6 bits/second/Hz256 QAM 8 bits/second/HzEffects of going through the originTake, for example, a QPSK signal where the normalized value changes from 1, 1 to –1, –1. When changing simulta-neously from I and Q values of +1 to I and Q values of –1, the signal trajectory goes through the origin (the I/Q value of 0,0). The origin represents 0 carrier magnitude. A value of 0 magnitude indicates that the carrier amplitude is 0 for a moment.Not all transitions in QPSK result in a trajectory that goes through the origin. If I changes value but Q does not (or vice-versa) the carrier amplitude changes a little, but it does not go through zero. Therefore some symbol transi-tions will result in a small amplitude variation, while others will result in a very large amplitude variation. The clock-recovery circuit in the receiver must deal with this ampli-tude variation uncertainty if it uses amplitude variations to align the receiver clock with the transmitter clock. Spectral regrowth does not automatically result from these trajectories that pass through or near the origin. If the amplifier and associated circuits are perfectly linear, the spectrum (spectral occupancy or occupied bandwidth) will be unchanged. The problem lies in nonlinearities in the circuits.A signal which changes amplitude over a very large range will exercise these nonlinearities to the fullest extent. These nonlinearities will cause distortion products. In con-tinuously modulated systems they will cause “spectral regrowth” or wider modulation sidebands (a phenomenon related to intermodulation distortion). Another term which is sometimes used in this context is “spectral splatter.”However this is a term that is more correctly used in asso-ciation with the increase in the bandwidth of a signal caused by pulsing on and off.3.7 Spectral efficiency examples inpractical radiosThe following examples indicate spectral efficien-cies that are achieved in some practical radio systems.The TDMA version of the North American Digital Cellular (NADC) system, achieves a 48 Kbits-per-second data rate over a 30 kHz bandwidth or 1.6 bits per second per Hz. It is a π/4 DQPSK based system and transmits two bits per symbol. The theoretical efficiency would be two bits per second per Hz and in practice it is 1.6 bits per second per Hz.Another example is a microwave digital radio using 16QAM. This kind of signal is more susceptible to noise and distortion than something simpler such as QPSK. This type of signal is usually sent over a direct line-of-sight microwave link or over a wire where there is very little noise and interference. In this microwave-digital-radio example the bit rate is 140 Mbits per second over a very wide bandwidth of 52.5 MHz. The spectral efficiency is 2.7 bits per second per Hz. To implement this, it takes a very clear line-of-sight transmission path and a precise and optimized high-power transceiver.。
外文翻译无线局域网

无线局域网概览无线局域网(WLAN )一般是一个有线局域网的延伸。
无线局域网组件将数据包转换成无线电波或红外线(IR )光脉冲,并传送到其他无线装置,或到一个作为通往有线局域网效劳的接入点。
现今大多数无线局域网是基于IEEE 和标准之间的无线通信设备和网络。
这些标准予诺数据传输别离在1到2 Mbps 或5至11 Mbps。
并指定一个一起的架构,传输方式,及其他方面的无线数据传输,以改善互操作性的产品。
技术在设计一个无线局域网解决方案时,制成品无线局域网有多种技术选择。
每种技术来都有自身的优势和局限性。
窄带技术窄带无线电系统在一个特定的无线电频率传输和接收用户信息。
窄带无线电频率维持其频带为可能方才通过信息的大小。
在不同的频道频率认真和谐不同用户可幸免通信渠道之间产生不良的串音。
私人线很像一个电台频率。
当每一个家庭在一个居委会有自己的私人线,人们在一个主页不能听其他家的。
在无线电系统,隐私和不干与是完成所要求作出其他的家园。
无线电接收器过滤掉所有的无线电信号,除那些指定的频率。
从客户角度来看,有一个缺点,窄带技术是最终用户,针对每一个招聘网站的地址,必需取得FCC的许可。
扩频技术大多数无线局域网系统利用扩频技术,军事开发的宽带射频技术使用在靠得住,平安,关键任务通信系统。
扩展频谱是旨在提供高的带宽利用率,靠得住性,完整性和平安性。
换言之,在窄带传输中更多的带宽被消耗,但衡量所产生的信号,在成效,声音方面,更易侦测,只要接收机知悉对扩频信号播出的参数。
若是接收机没有调整在正确的频率,扩频信号看起来像背景噪音。
有两种类型的无线扩频:跳频和直接序列。
跳频扩频技术跳频扩谱(fhss )不管发射机和接收机,在一个众所周知的模式利用窄频载波转变的频率。
妥帖同步,净成效是要维持一个单一的逻辑通道。
关于一个意想不到的接收器,fhss似乎是短时脉冲噪声。
直接序列扩频技术直接序列扩展频谱(扩频)为每个位元生成一个多余的比特模式,以转交。
机器人英语翻译
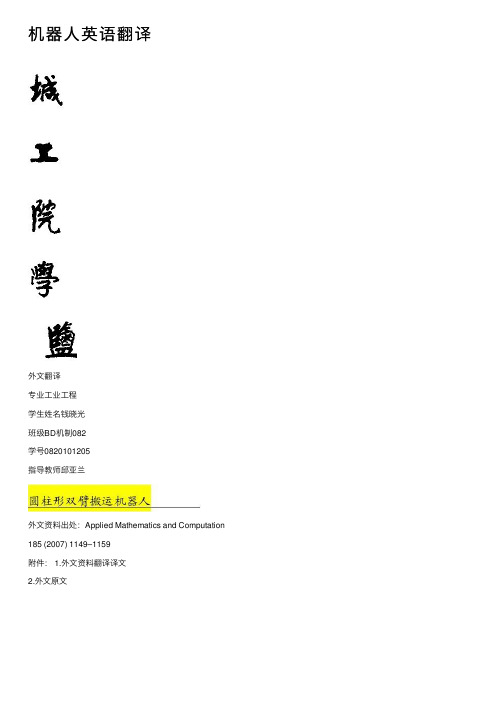
机器⼈英语翻译外⽂翻译专业⼯业⼯程学⽣姓名钱晓光班级BD机制082学号0820101205指导教师邱亚兰外⽂资料出处:Applied Mathematics and Computation 185 (2007) 1149–1159附件: 1.外⽂资料翻译译⽂2.外⽂原⽂灵活的双臂空间机器⼈捕捉物体的控制动⼒学译者:钱晓光⽂摘:在本⽂中,我们提出有效载荷的影响,来控制⼀个双臂空间机器⼈灵活的获取⼀个物体。
该拉格朗⽇公式动⼒学模型推导出了机器⼈系统原理。
源⾃初始条件的动⼒学模型模拟了整个系统的获取过程。
⼀个PD控制器设计,其⽬的是为了稳定机器⼈来捕捉对象,动态模拟执⾏例⼦:例:1.机器⼈系统不受控制发⽣撞击,仿真结果表明影响效果。
2.空间机器⼈捕获物体的成功是伟⼤的。
仿真结果表明,该机器⼈关节⾓和机械⼿的迅速程度已经达到稳定。
关键词:柔性臂;空间机器⼈;冲击;动⼒学;PD控制⽅案:圆柱型机器⼈;技能训练1.介绍空间机器⼈将成为⼈类未来在太空检验、装配和检索故障等⽇常⼯作的主要元素。
空间机器⼈满⾜宇航员额外的活动,对这些来说是很有价值的。
然⽽,⼈类⽣活配套设施的成本和时间对航员是有限制的,⾼度风险使空间机器⼈成为宇航员助⼿的选择。
增加设备的流动性, ⾃由飞⾏系统中⼀个或多个臂安装在⼀艘装有推进器⾥,然⽽,扩展推进器的使⽤却得到了极⼤的限制。
⼀个⾃由浮动的操作模式能增加系统的可操作性。
有很多的研究成果对刚性臂空间机器⼈做了研究。
考虑到空间机器⼈以下的特点:轻质量、长臂、重载荷、灵活、有效性等,切应考虑到良好的控制精度和性能。
与此同时,也存在着许多研究动态建模和单臂空间机器⼈灵活控制的成果。
作者描述了碰撞动⼒学建模⽅案的空间机器⼈和研究了多⼿臂灵活空间机器⼈。
吴中书使⽤假设模态⽅法描述了弹性变形,建⽴了动态模型,研究了拉格朗⽇公式和仿真的柔性双臂空间机械臂。
由两个特定操作阶段:影响阶段和撞击阶段。
影响阶段确定了初始条件的对象。
UWB超宽带通信系统的设计与仿真
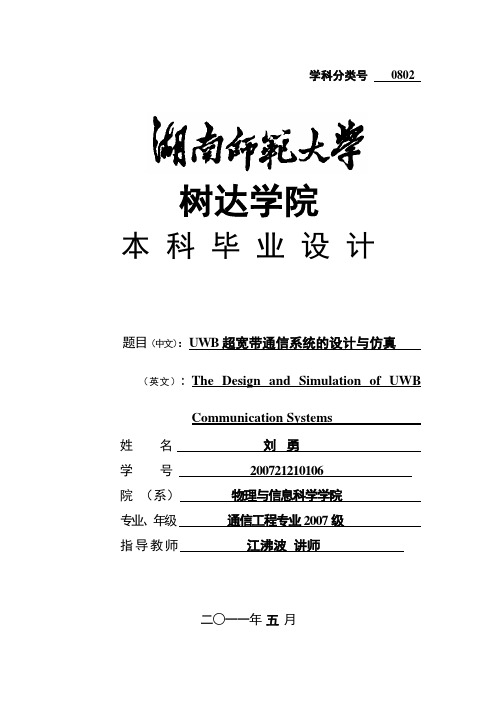
学科分类号0802树达学院本科毕业设计题目(中文):UWB超宽带通信系统的设计与仿真(英文):The Design and Simulation of UWB Communication Systems姓名刘勇学号200721210106院(系)物理与信息科学学院专业、年级通信工程专业2007级指导教师江沸波讲师二○一一年五月湖南师范大学树达学院本科毕业设计诚信声明本人郑重声明:所呈交的本科毕业设计,是本人在指导老师的指导下,独立进行研究工作所取得的成果,成果不存在知识产权争议,除设计中已经注明引用的内容外,本设计不含任何其他个人或集体已经发表或撰写过的作品成果。
对本设计的研究做出重要贡献的个人和集体均已在文中以明确方式标明。
本人完全意识到本声明的法律结果由本人承担。
本科毕业设计作者签名:年月日湖南师范大学本科毕业设计任务书二、湖南师范大学树达学院本科毕业设计评审表绩满分为10分。
总成绩=正文部分成绩+外文资料译文成绩。
评定成绩分为优秀、良好、中等、及格、不及格五个等级,总成绩90—100分记为优秀,80—89分记为良好,70—79分记为中等,60—69分记为及格,60分以下记为不及格。
若译文成绩为零,则不计总成绩,评定等级记为不及格。
三、湖南师范大学树达学院本科毕业设计答辩记录表目录摘要 (1)Abstract (1)1绪论 (2)1.1超宽带无线通信的概述 (3)1.2超宽带无线通信技术的研究历史及现状 (4)1.3本文所做的工作 (5)1.4本文的结构 (5)2超宽带无线通信系统发射机设计 (6)2.1超宽带脉冲的产生 (7)2.2高斯脉冲信号 (9)2.3超宽带无线通信的调制技术 (10)2.3.1单脉冲调制 (11)2.3.2多脉冲调制 (12)3超宽带无线信道建模 (13)3.1超宽带信道的基本概念 (13)3.2超宽带信道模块设计 (15)4超宽带相关接收模块设计 (16)4.1自相关模块 (16)4.2互相关模块 (17)5超宽带(UWB)无线通信系统的simulink建模 (18)5.1系统的总体结构 (18)5.2仿真模型的发射机 (19)5.3仿真模型的接收机 (20)5.4仿真模型的信道结构 (21)6 系统的仿真分析 (22)6.1发射端信号仿真分析 (23)6.2接收端信号仿真分析 (23)6.3接收信道仿真 (24)6.4误码率分析 (24)总结 (25)参考文献 (26)致谢 (27)外文文献翻译........................................... 1-6UWB超宽带通信系统的设计与仿真通信工程专业2007级刘勇摘要: 超宽带无线通信技术由于其较高的数据传输速率、较低的功率衰耗和强大的抗多径干扰能力等优点,视为下一代无线通信领域的关键技术。
脉冲无线电技术

脉冲无线电技术王婷婷陈雁摘要:脉冲无线电是一种超宽频(UWB)的扩频(SS)信号传输方式。
它所具有的属性使它成为在高密度多信道环境下近程通信的可行候选方式。
连续时间脉冲无线电多址联接模型利用脉冲位置调制(pulse position modulation)和随机跳时代码(random time-hopping code )以减轻多路径效应及抑制多用户之间的干扰。
本文使用目前已有的脉冲信号技术可支持的调制格式来描述脉冲无线电的特征,并解析估计它在理想多址联接信道(MAC)条件下多址联接的能力。
关键词:脉冲无线电、扩频多址联接、时跳、超宽带无线电、脉冲位置调制、脉冲正交调制、伪随机序列导言:利用超短脉冲来传播数字信息的想法最早提出于1992年,并被称为脉冲无线电(Impulse Radio)。
它的实现依赖于脉冲位置调制(Plus-Position Modulation)和时间分集(time diversity)。
而时间分集通过依照一随机代码多次(≥1000)重复同一码元获得,而这又使脉冲无线电具有很高的处理增益。
现在,脉冲无线电已进入多用户通信的研究领域,即所说的脉冲无线电多址联接(impulse radio multiple-access,IRMA)。
它以异步用户传输和依赖于能量控制的统计多用户(MUI)干扰抑制为理论基础。
脉冲无线电利用持续时间极短(通常为几纳秒)的基带脉冲交换信息,因此它所传播的无线电能量以很小的幅度分布在很宽的频带上(从接近直流到几吉赫兹)。
当这样的脉冲作用于设计恰当的天线时,在传播过程中会发生畸变。
天线起到滤波器的作用,即使在自由空间,波的辐射也伴随着脉冲的分化和演变。
脉冲无线电工作在几吉赫兹下的使用频率密度很高的范围中,因而必须要与各种不同的干扰信号竞争,同时也必须确保不影响在其他特定频带上工作的窄带无线电波。
要满足这些要求,就必须使用扩频技术(spread-spectrum techniques)。
雷尼希亚RMP60机械工具接触测试探头系统说明书

• 2.4 GHz radio transmission, allows single system forworldwide use.• Interference-free channel hopping transmission.• No channel selection required. • RMP60 meets the radio regulations of:Europe: CE 0536! USA: FCC ID: KQGRMP60, FCC ID: KQGRMP60V2 FCC ID: KQGRMP60MV2 Japan: RMP60: 004NYCA0042, RMP60: 004NYCA0406 RMP60M: 004NYCA0407Canada: IC: 3928A-RMP60, IC: 3928A-RMP60V2Australia, China, Israel, New Zealand, Russia, Switzerlandand India.• Partner RMP60 and RMI systems allow interference-freemultiple probe installations.• The RMP60 is suitable for use with Renishaw single anddouble touch probing cycles.• User adjustable trigger force for long/cranked styli. • A weak link is included in each kit to protect the probein the event of excessive stylus overtravel, when using steel styli.• The RMP60 is a compact 3D touch-trigger probe(±X, ±Y , +Z sense directions) with radio transmission, used for workpiece set-up and inspection on small to large CNC machining centres and vertical turret lathes.• The RMP60 transmits omnidirectionally with a range of15 m (49.2 ft).• Ease of installation.• A standard battery life of 140 hours continuous use, orthe equivalent of approximately 100 days at 5 % usage is achievable. For applications requiring greater battery life, certain high capacity lithium thionyl chloride batteries can be used.• Repeatability, 1.0 µm (40 µin) is certified at480 mm/min (1.57 ft/min) with 50 mm stylus.• Probe switch on is user configurable between M code, spinor shank.• Probe switch off is user configurable between M code,time, spin or shank switch dependant on turn on method.StylusData sheet H-2000-2122-03-A A Tech Authoirty, Inc3857 Schaefer Ave, Ste C Chino, CA. 91710 (909)972-7520Operating envelope - RMP60/RMIThe RMP60 transmission envelope and range is shown below.The probe system should be positioned so that the optimum range can be achieved over the full travel of the machine’s axes including the tool magazine. Always face the RMI in the direction of the machine spindle and tool magazine. If the probe is not in range when in the tool magazine use spin or shank turn on.The RMP60 and RMI must be within a mutual operating envelope. The operating envelope shows line-of-sight performance. However, radio transmission does not require line-of-sight as long as any reflected radio path is less than the 15 m (49.2 ft) system operating range.RMP60 probeRange metres (feet)OPERATING AND SWITCH ON/OFF75°0°15°90°75°60°75°0°19 (0.75)50 (1.97)RMP60 dimensionsdimensions mm (in)ZData sheetRMP60 - radio probeBattery dead - at this stage probe status is forced open and the probe cycle will stop.System operationPrior to probe operation, it is imperative that the program selected to ‘drive’ the probe has been verified. Incorrect programming could result in damage to the machine, workpiece and probe system.The RMP60 probe operates in one of three modes:1. Stand-by mode - The RMP60 uses a small current,while waiting for a switch-on signal to be received.2. Operating mode - Activated by one of the methodsdescribed below. Signals are only transmitted by the probe in this mode and the probe is now ready for use.3. Configuration mode - Trigger Logic™ allows a numberof probe set-up options to be programmed, by triggering the probe when the batteries are inserted. Programmableoptions are described on the next page.Probe environmentPrimary application Inspection probe for machiningcentres Sense directions 5 way ±X ±Y +ZWeight (without a shank) with batteries without batteries901 g (31.79 oz) 855 g (30.16 oz)Trigger force using 50 mm (1.97 in) stylus low force direction factory settingX Y 0.75 N / 75 gf (2.65 ozf)Z 5.30 N / 530 gf (18.69 ozf)Trigger force using 50 mm (1.97 in) stylus high force directionX Y 1.4 N / 140 gf (4.94 ozf)Z 5.30 N / 530 gf (18.69 ozf)Max. spin speed 1000 rev/minOvertravel X Y 18°Z 11 mm (0.43 in)Sealing IPX8 (BS 5490, IEC 529)1 atmosphereRepeatability maximum 2σ value in any direction 1.0 µm (0.00004 in) is valid fortest velocity of 480 mm/min(1.57 ft/min) at stylus tip, usingstylus 50 mm (1.97 in) long.Probe specificationProbe status LEDsWhen operating the probe status LEDs give a visual indication of the probe state (triggered or seated) and battery condition.Multiple probe modeRMP60 can be user configured using T rigger Logic™ to allow multiple RMP60s to be used with a single RMI.Notes:Radio turn on cannot be used in multiple probe mode. RMP60s set to ‘mode-on’ can coexist alongside any number of RMP60’s set to ‘mode-off’.To allow multiple probes/single RMI in close proximity, 16 choices of ‘mode-on’ colours are available – each representing a different machine tool installation.Only one of the multiple probes per machine will need partnering as, by configuring multiple probes to a single‘mode-on’ choice, all probes have the same identification. The probe to be partnered is partnered after selection of multiple probe on mode.There is no limit to the number of probes that can be used with a single RMI as long as they all have the same ‘mode-on’ colour choice.All RMP60s are factory-set to ‘mode off’.The addition of further probe(s) into a single probe installation requires all probes to be re-configured to the same multiple probe ‘mode-on’ choice and the repartnering of one of the probes to the installed RMI.The addition of further probes (or replacements) into a multi probe installation is achieved simply by reconfiguration to the same ‘mode-on’ colour choice.Comprehesive details of how to set-up and change mutiple probe settings are included in the RMP60 installation and user's guide, H-2000-5219.RMP60/RMI Temperature Storage -10 °C to 70 °C (14 °F to 158 °F)Normal operating5 °C to 50 °C (41 °F to 122 °F)Notes:The RMP60 will be turned on after 1 sec in all modes.)After being turned on, the RMP60 must be on for a minimum of 1 sec (7 seconds for spin option) before being turned off. In radio on configuration (either radio on/radio off or radio on/time off) the RMP60 has a built-in hibernate mode. This saves battery life when the RMP60 is in stand-by and the RMI is un-powered (or out of range).The RMP60 goes into hibernate mode 30 sec after the RMI is un-powered (or out of range). When in this mode, the RMP60 checks for a powered RMI every 30 secs, if the RMI is found, the RMP60 goes from the hibernate mode to stand-by, ready for radio turn on.Probe switch on and offThe probe is switched on by one of the following options.All options are user configurable.RMP60 switch-on method.Switch-on options are configurable.RMP60 switch-off method.Switch-off options are configurable.1. Radio onRadio switch on is commanded by M code. (factory setting).1. Radio offRadio switch-off is commanded by M code. (factory setting).A timer automatically switches the probe off after 90 min from the last trigger, if not turned off by M code.2. Timer off (time out)The RMP60 will time out (12, 33 or 134 sec - user configurable) after the last probe trigger or reseat.2. Spin startSpin at 650 rev/min for 1 sec minimum (6 sec maximum).3. Spin stopSpin at 650 rev/min for 1 sec minimum (6 sec maximum).A timer automatically switches the probe off after 90 min from last trigger off.4. Timer off (time out)The RMP60 will time out (12, 33 or 134 sec - userconfigurable) after the last probe trigger or reseat.3. Shank switch5. Shank switch offBattery life expectancyTypical battery reserve lifeUsing the standard alkaline battery at 5% usage, typically the probe will continue to operate for approximately 1 week after a low battery warning is first indicated.Replace the batteries as soon as is practicable.Rechargeable batteries: either nickel metal hydride (NiMh) or nickel cadnium (NiCd) can be used, but expect a battery life of approximately 50% of the alkaline figures given in the table below.To achieve stated radio stand-by life, the RMP60 must be in-range of a powered partner RMI.Battery Shank/spin turn onRadio turn on Continuous useTwo AA type Stand-by life (days - typical)5% usage 72 minutes/day (days - typical)Stand-by life (days - typical)5% usage 72 minutes/day (days - typical)(hours - typical)Alkaline65010013065140Lithium ThionylChloride1300200260130280For applications requiring greater battery life, certain high capacity lithium thionyl chloride batteries can be used.Data sheetRMP60 - radio probe(Ø2.48)RMP60M is a special modular version of RMP60. It enables probe inspection of part features inaccessible to RMP60, by fitting selected adaptors and extensions as shown.RMP60M modular systemRMP60M dimensions40.75 50.00 / 100.00 / 150.00 66.25 (Ø2.48)100.00 / 150.00 / 200.00 50.50 (Ø2.48)66.25Parts list - Please quote the Part no. when ordering equipment.TypePart no.DescriptionRMP60A-4113-0001RMP60 probe with batteries, tool kit and user’s guide (factory set to radio on/radio off).RMP60M module A-4113-1003RMP60M probe with batteries, tool kit and user’s guide (factory set to radio on/radio off).Battery P-BT03-0005AA battery - Alkaline type supplied as standard with probe (two required).Battery P-BT03-0008AA battery - Lithium thionyl chloride (two required).Stylus A-5000-3709PS3-1C ceramic stylus 50 mm long with Ø6 mm ball.Weak link kit A-2085-0068Weak link (Part no. M-2085-0069 x 2) and 5 mm AF spanner.Tool kit A-4038-0304Probe tool kit comprising: Ø1.98 mm stylus tool, 2.0 mm AF hexagon key,2.5 mm AF hexagon key (x 2), 4 mm AF hexagon key, and shank grub screws (x 2).Diaphragm kit A-4038-0302RMP60 outer diaphragm.Battery cassette A-4038-0300RMP60 battery cassette assembly.Cassette seal A-4038-0301Battery cassette housing seal.Bobbin kit A-4038-0303Bobbin for shank switch (supplied with shank).RMI A-4113-0050RMI, side exit, with 15 m (49.2 ft) cable, tool kit and user’s guide.Mtg brkt A-2033-0830Mounting bracket with fixing screws, washers and nuts.Extension L100A-4038-1010RMP60M extension - 100 mm long.Extension L150A-4038-1027RMP60M extension - 150 mm long.Extension L200A-4038-1028RMP60M extension - 200 mm long.Probe module A-4038-1002RMP60M probe module assembly.RMP60/LP2 adaptor A-4038-0212RMP60M LP2 adaptor assembly.LPE1A-2063-7001LPE1 extension bar - 50 mm long.LPE2A-2063-7002LPE2 extension bar - 100 mm long.LPE3A-2063-7003LPE3 extension bar - 150 mm long.MA4A-2063-7600MA4 90° adaptor assembly.RMP60 user’s guide H-2000-5219RMP60 user’s guide.Styli –See brochure H-1000-3200 Styli and accessories.Software –See data sheet H-2000-2289 Probe software for machine tools.Shanks –See data sheet H-2000-2011 Shanks.RMI–See data sheet H-2000-2123 RMI.Renishaw plcNew Mills, Wotton-under-Edge, Gloucestershire GL12 8JR United KingdomT +44 (0)1453 524524F +44 (0)1453 524901E ***************© 2006 Renishaw plc. All rights reserved. Renishaw reserves the right to change specifications without noticeIssued 11.06 Part no. H-2000-2122-03-AFor worldwide contact details, please visit ourmain web site at /contact*H-2000-2122-03*。
Ultra WideBand (UWB) 系统介绍
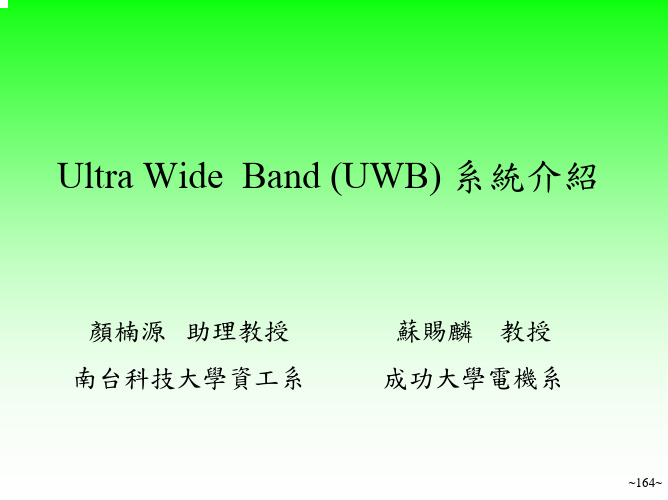
~172~Βιβλιοθήκη PPM + THSS
Str(t)
transmitting 0 pulse wtr(t)
Tc Tf Ts : data symbol time
t
Str(t)
transmitting 1
δ
δ Tc Tf
δ
δ t
Ts
codeword C = [1 0 0 2] , N h = 3 code period N p = 4 Ts = N s ⋅ T f i.e. , Ts = 4 ⋅ T f N s : number of pulses per data symbol T f ≥ N h ⋅ Tc i.e., T f = 3 ⋅ Tc
~171~
Impulse Radio UWB Techniques (1)
Time-Modulated (Hopping) UWB (TM(H)-UWB) — low duty cycle (Impulse radio) — data modulation by pulse position (time dithering) or signal polarity — multiaccess channelization by time coding (Time- Hopping, TH) — for precise location, tracking, radar sensing (through wall), data communications
- 1、下载文档前请自行甄别文档内容的完整性,平台不提供额外的编辑、内容补充、找答案等附加服务。
- 2、"仅部分预览"的文档,不可在线预览部分如存在完整性等问题,可反馈申请退款(可完整预览的文档不适用该条件!)。
- 3、如文档侵犯您的权益,请联系客服反馈,我们会尽快为您处理(人工客服工作时间:9:00-18:30)。
arXiv:cs/0502083v1 [cs.IT] 22 Feb 2005
Abstract — Spectral properties and performance of multi-pulse impulse radio ultra-wideband systems with pulse-based polarity randomization are analyzed. Instead of a single type of pulse transmitted in each frame, multiple types of pulses are considered, which is shown to reduce the effects of multiple-access interference. First, the spectral properties of a multipulse impulse radio system is investigated. It is shown that the power spectral density is the average of spectral contents of different pulse shapes. Then, approximate closed-form expressions for bit error probability of a multi-pulse impulse radio system are derived for RAKE receivers in asynchronous multiuser environments. The theoretical and simulation results indicate that impulse radio systems that are more robust against multiple-access interference than a “classical” impulse radio system can be designed with multiple types of ultra-wideband pulses. Index Terms— Ultra-wideband (UWB), multi-pulse impulse radio (IR), RAKE receivers, multiple-access interference (MAI), asynchronous systems.
2005 Conference on Information Sciences and Systems, The Johns Hopkins University, March 16–18, 2005
Impulse Radio Systems with Multiple Types of Ultra-Wideband Pulses 0
0 This research was supported by Mitsubishi Electric Research Laboratories, Cambridge, MA, in part by the National Science Foundation under grant ANI-03-38807, and in part by the New Jersey Center for Wireless Telecommunications.
(k ) (k )
(k )
(k )
where pj (t) is the UWB pulse transmif ⌋ ∈ {+1, −1} is the equiprobable information bit, Tf is the frame time, Tc is the chip interval, and Nf is the number of frames/pulses per information symbol. The (k ) time hopping (TH) code, denoted by cj , is modelled by a uniform distribution in {0, 1, ..., Nc − 1}, with Nc = Tf /Tc (k ) (l) being the number of chips per frame, and cj and ci are independent for (j, k) = (i, l). Random polarity codes, dj , are binary random variables taking values ±1 with equal proba(k ) (l) bility, and dj and di are independent for (j, k) = (i, l) [10]. Use of random polarity codes helps reduce the spectral lines in the power spectral density of the transmitted signal [11] and mitigate the effects of MAI [10]. The receiver for user k is assumed to know its polarity code. Note the difference of the signal model in (1) from a classical IR system, in which the same pulse is used in all the frames. In other words, the signal model in (1) is a more general formulation of an IR system, which reduces to the original (k ) proposal in [1] when pj (t) = p(t) ∀j, k. We assume that there are Np different types of pulses employed in the system and (k ) (k ) pj +iNp (t) = pj (t) for any integer i. Also, for simplicity of
Sinan Gezici1 , Zafer Sahinoglu2 , Hisashi Kobayashi1, and H. Vincent Poor1
1 2
Department of Electrical Engineering, Princeton University, Princeton, NJ 08544, USA Mitsubishi Electric Research Laboratories, 201 Broadway, Cambridge, MA 02139, USA of different types of UWB pulses with good cross-correlation properties. Multi-pulse IR systems have recently been proposed in [9]. However, there has been no theoretical analysis of those systems, in terms of their spectral properties and bit error probability (BEP) performance, and no quantitative investigation of the gains that can be obtained by multiple types of UWB pulses. In this paper, we consider an asynchronous multiuser environment and analyze the BEP performance of a generic RAKE receiver over frequency-selective channels. The results are valid for different number of UWB pulse types, hence cover the single-pulse system as a special case. We also analyze average power spectrum density (PSD) of multi-pulse IR signals, and obtain a simple relation between the Fourier transforms of the UWB pulses and the average PSD of the transmitted signal. The remainder of the paper is organized as follows. Section II describes the transmitted signal model and Section III analyzes its spectral properties. In Section IV, the performance analysis of multi-pulse IR RAKE receivers is presented. The simulation results are given in Section V, which is followed by the concluding remarks in Section VI.
II. Signal Model
Consider a K -user environment with the following transmitted signal from user k: s (k ) ( t ) =