科学文献
科技文献的定义
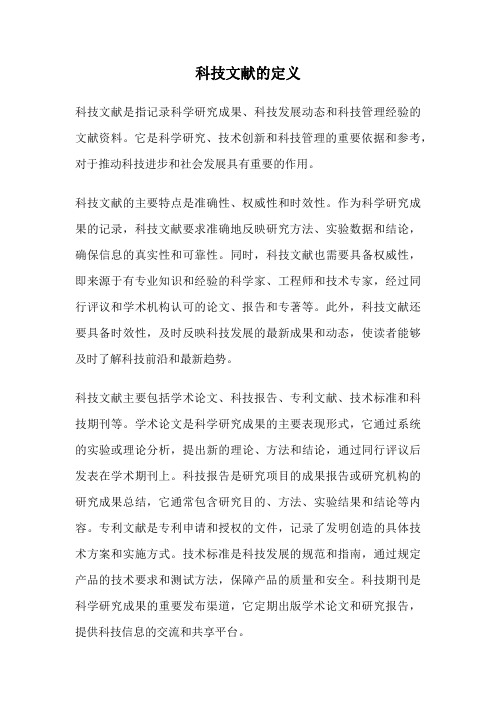
科技文献的定义科技文献是指记录科学研究成果、科技发展动态和科技管理经验的文献资料。
它是科学研究、技术创新和科技管理的重要依据和参考,对于推动科技进步和社会发展具有重要的作用。
科技文献的主要特点是准确性、权威性和时效性。
作为科学研究成果的记录,科技文献要求准确地反映研究方法、实验数据和结论,确保信息的真实性和可靠性。
同时,科技文献也需要具备权威性,即来源于有专业知识和经验的科学家、工程师和技术专家,经过同行评议和学术机构认可的论文、报告和专著等。
此外,科技文献还要具备时效性,及时反映科技发展的最新成果和动态,使读者能够及时了解科技前沿和最新趋势。
科技文献主要包括学术论文、科技报告、专利文献、技术标准和科技期刊等。
学术论文是科学研究成果的主要表现形式,它通过系统的实验或理论分析,提出新的理论、方法和结论,通过同行评议后发表在学术期刊上。
科技报告是研究项目的成果报告或研究机构的研究成果总结,它通常包含研究目的、方法、实验结果和结论等内容。
专利文献是专利申请和授权的文件,记录了发明创造的具体技术方案和实施方式。
技术标准是科技发展的规范和指南,通过规定产品的技术要求和测试方法,保障产品的质量和安全。
科技期刊是科学研究成果的重要发布渠道,它定期出版学术论文和研究报告,提供科技信息的交流和共享平台。
科技文献的利用可以促进科学研究的发展和技术创新的进步。
科学家和工程师可以通过查阅和分析科技文献,了解前人的研究成果和经验,避免重复劳动和错误,为自己的研究工作奠定基础。
科技管理人员可以通过研究科技文献,掌握科技发展的动态和趋势,制定科技政策和计划,引导科技创新和产业升级。
工程师和技术人员可以通过科技文献,学习新的技术方法和应用案例,提高自己的专业能力和技术水平。
科技文献是科学研究、技术创新和科技管理的重要资源和工具。
科技工作者应该重视科技文献的收集和利用,不断更新自己的知识和技能,推动科技进步和社会发展。
同时,科技出版机构和科技管理部门也应该加强对科技文献的管理和服务,提高科技文献的质量和影响力,为科技创新提供有力支持。
高中生如何有效阅读科学文献

高中生如何有效阅读科学文献科学文献是高中生学习科学知识的重要资源,它们包含了前沿的研究成果和学术观点。
然而,对于许多高中生来说,阅读科学文献可能是一项具有挑战性的任务。
本文将介绍一些帮助高中生有效阅读科学文献的方法和技巧。
一、选择适合的文献在开始阅读科学文献之前,高中生应该学会选择适合自己的文献。
首先,他们可以通过在学校图书馆或在线数据库中搜索关键词来找到相关的文献。
其次,他们应该根据自己的学习目标和兴趣选择文献。
例如,如果他们对生物学感兴趣,那么可以选择与生物学相关的研究论文。
二、了解文献的结构科学文献通常由摘要、引言、方法、结果和讨论等部分组成。
高中生在阅读文献之前,应该先了解这些部分的作用和内容。
摘要通常是文献的概要,可以帮助读者快速了解研究的目的和主要结果。
引言部分介绍了研究的背景和目的,方法部分描述了研究的实验设计和数据采集方法,结果部分展示了实验结果,讨论部分对结果进行解释和分析。
三、注意关键词和术语科学文献中常常使用一些专业的术语和关键词,高中生应该学会识别和理解这些术语。
他们可以通过查阅词典或在线资源来解释这些术语的含义。
此外,高中生还可以将这些术语和关键词记录下来,以便在阅读过程中进行参考和复习。
四、提问和思考在阅读科学文献时,高中生应该保持积极的思考和提问的态度。
他们可以思考文献中的研究问题、实验设计和结果,并提出自己的疑问和观点。
通过提问和思考,他们可以更好地理解文献的内容,并培养批判性思维能力。
五、扩展阅读阅读科学文献不仅限于一篇文章,高中生可以通过扩展阅读来深入了解某个主题。
例如,他们可以查阅相关的综述文章、书籍或其他研究论文,以获取更全面的知识。
扩展阅读还可以帮助高中生了解当前研究领域的热点问题和最新进展。
六、记录和总结在阅读科学文献时,高中生应该养成记录和总结的习惯。
他们可以使用笔记本或电子工具来记录重要的观点、关键词和疑问。
此外,他们还可以将阅读的内容进行总结和归纳,以便后续的学习和复习。
如何阅读科学文献
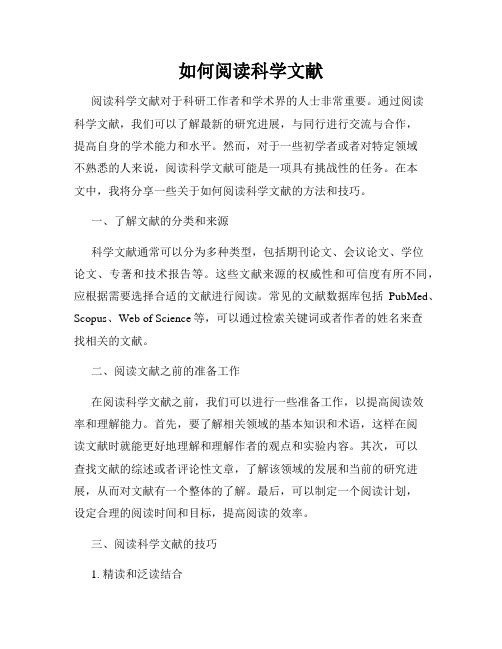
如何阅读科学文献阅读科学文献对于科研工作者和学术界的人士非常重要。
通过阅读科学文献,我们可以了解最新的研究进展,与同行进行交流与合作,提高自身的学术能力和水平。
然而,对于一些初学者或者对特定领域不熟悉的人来说,阅读科学文献可能是一项具有挑战性的任务。
在本文中,我将分享一些关于如何阅读科学文献的方法和技巧。
一、了解文献的分类和来源科学文献通常可以分为多种类型,包括期刊论文、会议论文、学位论文、专著和技术报告等。
这些文献来源的权威性和可信度有所不同,应根据需要选择合适的文献进行阅读。
常见的文献数据库包括PubMed、Scopus、Web of Science等,可以通过检索关键词或者作者的姓名来查找相关的文献。
二、阅读文献之前的准备工作在阅读科学文献之前,我们可以进行一些准备工作,以提高阅读效率和理解能力。
首先,要了解相关领域的基本知识和术语,这样在阅读文献时就能更好地理解和理解作者的观点和实验内容。
其次,可以查找文献的综述或者评论性文章,了解该领域的发展和当前的研究进展,从而对文献有一个整体的了解。
最后,可以制定一个阅读计划,设定合理的阅读时间和目标,提高阅读的效率。
三、阅读科学文献的技巧1. 精读和泛读结合对于篇幅较长或者对自己比较重要的文献,可以进行精读。
在精读时,要认真阅读摘要和介绍部分,了解研究的背景和目的,然后逐段、逐句进行仔细阅读,理解作者的实验设计和研究结果。
在阅读过程中,可以做一些标记或者写下关键点,以便于后续的回顾和整理。
对于篇幅较长或者对自己不是很重要的文献,可以进行泛读。
在泛读时,可以关注文献的结构、图表和重点段落,了解作者的主要观点和研究结果。
泛读可以帮助快速获取信息,筛选出对自己研究有用的文献。
2. 多角度阅读在阅读科学文献时,要注意从多个角度进行思考和分析。
可以思考文献的创新点、实验设计、结果解释以及与其他相关文献的联系。
可以尝试用自己的话总结和表达作者的观点和结论,以帮助更好地理解文献内容。
科学文献的名词解释

科学文献的名词解释
文献:
1. Abstract
2. Bibliographic Database
3. Editor
4. Index
一、Abstract:
抽象是科学文献中概述性段落的内容,即文章的摘要,它在文献的开头,能简要介绍文献的内容和目的,是科学文献查找、分析和利用的重要基础。
Abstract由一般性问题、技术方法、重要结果、结论和指出事实的综述性的评论组成,概述文献的内容及方法,是检索文献信息的重要依据。
二、Bibliographic Database:
文献数据库是以文献数据为基础建立起来的文献信息体系,其主要内容是存储文献信息(如书籍、期刊、报纸、图书、报告摘要、摘录、贴文等)的元数据,并提供跨文献的快速检索的功能。
一般而言,文献数据库由代表文献的描述性元数据(如题名、作者、出版社、出版日期等)和用于检索所建立的全文索引组成。
三、Editor:
编辑是指组织、审查和整理文献内容,以便发表的这类编辑服务活动。
编辑可以编排、修改、撰写、组织文献,编辑从文献撰写、组织和修订等方面起着协调作用,以确保出版物的质量。
四、Index:
索引是科学文献检索的一个重要技术工具,它的主要目的是使读者能够轻松找到所需要的信息。
索引包括有关文献的词汇表,能够提供有用的参考资料,而无需检查整个文献的文本内容。
另外,索引还可以帮助读者了解文献的整体框架,有利于从文献中快速获取信息。
十大科技文献源

十大科技文献源科技的发展日新月异,不断推动着人类社会的进步。
以下是十大科技文献源,它们记录了人类在不同领域的探索和创新。
1.《自然》(Nature)作为世界上最古老的科学杂志之一,《自然》杂志为读者提供了丰富的科学研究成果和前沿的科技进展。
它既包括基础科学领域的研究,也关注应用科学的发展。
2.《科学》(Science)《科学》杂志是世界上最有影响力的综合性科学杂志之一,涵盖了各个学科领域的最新研究成果。
它以其高质量的科学报道和严谨的学术评审而闻名,是科学界的权威之一。
3.《人工智能》(Artificial Intelligence)《人工智能》期刊聚焦于人工智能领域的研究和应用,包括机器学习、自然语言处理、计算机视觉等。
它发布的论文对于推动人工智能技术的发展具有重要意义。
4.《物理评论快报》(Physical Review Letters)《物理评论快报》是物理学领域最具影响力的学术期刊之一,发表了许多重要的物理学突破性研究。
它以其简洁、精确和具有启发性的论文而受到广泛关注。
5.《细胞》(Cell)《细胞》杂志是细胞生物学和分子生物学领域的顶级期刊之一,报道了该领域的最新研究成果和突破性发现。
它对于理解生命的基本机制和疾病的发生机理具有重要意义。
6.《计算机视觉国际会议》(Conference on Computer Vision and Pattern Recognition)计算机视觉是人工智能领域的一个重要分支,该会议是该领域最重要的学术会议之一。
它汇集了来自全球的顶尖研究人员,分享了最新的计算机视觉技术和应用。
7.《美国国家科学院院刊》(Proceedings of the National Academy of Sciences)《美国国家科学院院刊》是美国国家科学院的官方期刊,发表了各个学科领域的重要研究成果。
它是一本跨学科的期刊,涵盖了自然科学、社会科学和工程技术等领域。
8.《医学》(The Lancet)《医学》杂志是世界上最具影响力的医学期刊之一,发表了许多重要的医学研究。
科学阅读的方法和技巧
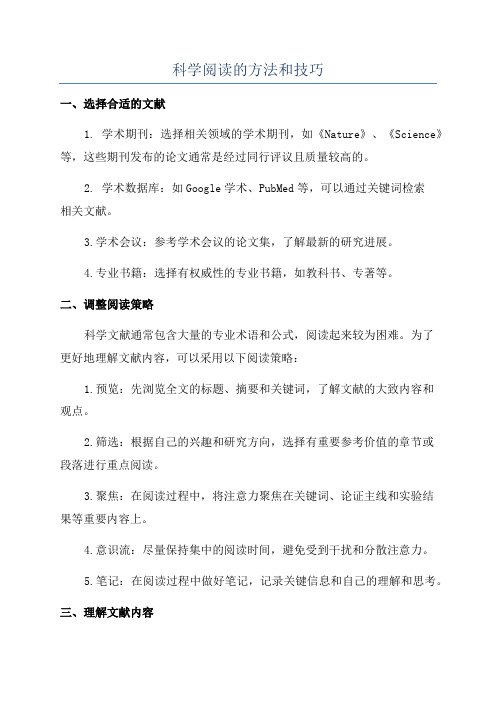
科学阅读的方法和技巧一、选择合适的文献1. 学术期刊:选择相关领域的学术期刊,如《Nature》、《Science》等,这些期刊发布的论文通常是经过同行评议且质量较高的。
2. 学术数据库:如Google学术、PubMed等,可以通过关键词检索相关文献。
3.学术会议:参考学术会议的论文集,了解最新的研究进展。
4.专业书籍:选择有权威性的专业书籍,如教科书、专著等。
二、调整阅读策略科学文献通常包含大量的专业术语和公式,阅读起来较为困难。
为了更好地理解文献内容,可以采用以下阅读策略:1.预览:先浏览全文的标题、摘要和关键词,了解文献的大致内容和观点。
2.筛选:根据自己的兴趣和研究方向,选择有重要参考价值的章节或段落进行重点阅读。
3.聚焦:在阅读过程中,将注意力聚焦在关键词、论证主线和实验结果等重要内容上。
4.意识流:尽量保持集中的阅读时间,避免受到干扰和分散注意力。
5.笔记:在阅读过程中做好笔记,记录关键信息和自己的理解和思考。
三、理解文献内容科学文献通常采用科学语言和专业术语,为了更好地理解文献内容,可以采用以下方法:1.查阅词典和参考书:查阅相关课本、词典、参考书籍等,弄清楚不熟悉的术语和概念。
2.加深背景知识:扩大自己的科学背景知识,了解相关领域的基本原理和理论框架。
3.多角度理解:通过阅读多个文献,了解不同研究观点和方法,从不同角度思考和分析问题。
4.精确解释:将复杂的概念或内容用自己的语言重新解释一遍,以确保自己理解透彻。
四、分析论证逻辑科学文献通常有一定的逻辑结构,分析论证逻辑有助于深入理解文献内容。
可以采用以下方法:1.总结主旨:通过阅读摘要、引言和结论等部分,总结文献的主旨和观点。
4.反思批判:对文献的内容进行批判性思考,发现可能存在的问题和不足之处。
五、扩展思考和应用1.感知科学思维:思考作者是如何发现问题、提出假设、设计实验和得出结论的,借鉴科学思维方法。
2.拓展思考:将文献中的观点与自己的观点进行比较和对比,思考存在的差异和原因,并以此为基础进行拓展思考。
科学文献阅读技巧详解

科学文献阅读技巧详解科学文献阅读技巧详解在科学研究的道路上,掌握有效的文献阅读技巧至关重要。
想象一下,文献就像是一座座深奥的宝库,里面珍藏着无数宝贵的知识和经验。
然而,要想从这些宝库中获取有用的信息,需要具备一定的技巧和策略。
首先,当你面对一篇新的科学文献时,它可能会显得有些“冷漠”。
不过,不要担心,这只是因为它还没有“认识”你。
开始阅读前,先浏览摘要部分,这就像与文献“打个招呼”,让它知道你对它感兴趣。
接下来,进入文献的正文部分,你会发现它有如一位导游,带领你探索未知的领域。
要有耐心,不要急于求成。
有时候,文献会使用复杂的术语和句子,就像在说一门外语一样。
这时,不妨反复阅读,逐步理解每一个词语背后的含义,就像与文献进行一场深入的交流。
在阅读过程中,可以时常停下来思考,并做好记录。
文献常常会提出问题或者让你有新的启发,这就像它在与你进行互动,促使你深入思考。
记得,做好笔记非常重要,这有助于你将碎片化的信息整理成有条理的知识体系。
此外,不要忽视文献中的图表和数据。
它们就像文献的“视觉演示”,通过直观的方式展示研究结果。
深入理解图表背后的数据,有助于你更全面地把握文献的核心内容。
最后,要保持批判性思维。
就像与一位智者交谈一样,不要轻易接受文献中的每一个观点。
要学会提出问题,评估实验设计的有效性,并思考研究结果的可能局限性。
这样,你才能更好地理解文献,甚至为未来的研究提供新的思路和方法。
总结来说,科学文献阅读并非一项简单的任务,它需要技巧和耐心。
通过与文献建立良好的互动关系,你将能够开启一段充满发现和启发的学术之旅。
不断地练习和改进阅读技巧,相信你定能在科学研究的道路上越走越远。
中国科学引用文献格式
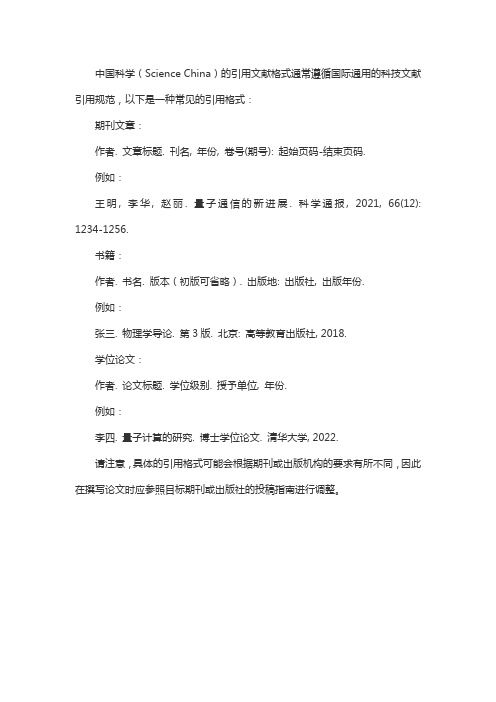
中国科学(Science China)的引用文献格式通常遵循国际通用的科技文献引用规范,以下是一种常见的引用格式:
期刊文章:
作者. 文章标题. 刊名, 年份, 卷号(期号): 起始页码-结束页码.
例如:
王明, 李华, 赵丽. 量子通信的新进展. 科学通报, 2021, 66(12): 1234-1256.
书籍:
作者. 书名. 版本(初版可省略). 出版地: 出版社, 出版年份.
例如:
张三. 物理学导论. 第3版. 北京: 高等教育出版社, 2018.
学位论文:
作者. 论文标题. 学位级别. 授予单位, 年份.
例如:
李四. 量子计算的研究. 博士学位论文. 清华大学, 2022.
请注意,具体的引用格式可能会根据期刊或出版机构的要求有所不同,因此在撰写论文时应参照目标期刊或出版社的投稿指南进行调整。
- 1、下载文档前请自行甄别文档内容的完整性,平台不提供额外的编辑、内容补充、找答案等附加服务。
- 2、"仅部分预览"的文档,不可在线预览部分如存在完整性等问题,可反馈申请退款(可完整预览的文档不适用该条件!)。
- 3、如文档侵犯您的权益,请联系客服反馈,我们会尽快为您处理(人工客服工作时间:9:00-18:30)。
Submitted to Geophysical Research Letters March,1996t o Observations of the Lunar Plasma Wake from the WIND Spacecraf n December 27,1994K.W.Ogilvie 1,J.T.Steinberg 2,R.J.Fitzenreiter 1,C.J.Owen 1,zarus 2,W.1M.Farrell 1,and R.B.Torbert 3Laboratory for Extraterrestrial Physics,NASA/Goddard Space Flight Center,Green-2belt,Maryland 20771,USACenter for Space Research,Massachusetts Institute of Technology,Cambridge,MA 302139Institute for Earth,Oceans and Space,Department of Physics,University of New A Hampshire,Durham,NH 03824bstract.On December 27,1994,the WIND spacecraft crossed the lunar wake at a e distance of 6.5lunar radii (R )behind the moon.The observations made were th L first employing modern instruments and a high data rate.The SWE plasma instrument n on WIND observed new aspects of the interaction between the solar wind and unmag-etized dielectric bodies.The plasma density decreased exponentially from the peri-c phery of the wake towards its center as predicted by simple theory.Behind the moon old ion beams were observed incident on the lunar cavity from its periphery and mov-e ing along the interplanetary magnetic field direction.The ions were accelerated by an lectric field of order 2×10volts/m.The region of plasma depletion was observed t −4o extend beyond the light shadow,consistent with a rarefaction wave moving out I from the wake into the undisturbed solar wind.ntroductionThe first orbital measurements of the plasma and magnetic field signatures of the -s moon were made between 1967and 1972by Explorer 35and by the Apollo sub atellites (see Schubert and Lichtenstein,[1974]and many references therein).They t a showed conclusively that the moon behaves as a non-conducting dielectric sphere tha bsorbs the solar wind falling upon its surface.Minor magnetic disturbances close to -m the limb were thought to result from the abrupt interruption in the flow [Krall and Tid an,1969;Ness and Schatten,1969]or from local weakly magnetized regions in themoon [Sonett and Mihalov,1972;Ness and Whang,1972].-s Numerous close flybys have shown the moon to possess a plasma wake,with a den ity decrease on the anti-sunward side.This paper presents the first high resolution ion n i and electron measurements in and near the wake of the moon made with moder nstrumentation [Lepping et al.,1995;Ogilvie et al.,1995].-g The WIND spacecraft is destined for a halo orbit surrounding the forward Lagran ian point,some 200Earth radii (R )upstream of Earth in the solar wind.Prior to its h e alo orbit insertion,the spacecraft follows double lunar swing-by orbits which require ,1that it approach close to the moon for gravity-assisted orbit changes [Acuna et al.995].During these approaches the spacecraft passes behind the moon.We report in r r this paper observations on December 27,1994at a downstream distance of 6.5luna adii,R (R ∼∼1738km).Earlier wake crossings by other spacecraft discussed by L L L e m Schubert and Lichtenstein [1974]occurred at smaller distances (∼∼2R )from th oon.Instrumentation and DataThe SWE plasma instrument on the WIND spacecraft has been described in detail u by Ogilvie et al.[1995].In brief,it consists of a Faraday cup subsystem,which meas-res the vector velocity,number density,and temperature of proton and helium com-r (ponents of the solar wind,together with a tri-axial ion and electron spectromete VEIS),which performs 3-D measurements of electrons,and in some modes,of the e subsonic ion population.In this paper we discuss observations of the complete 3-D lectron velocity distribution function made in one 3second spacecraft rotation and n f repeated every 6seconds by the VEIS in its electron mode.The electron distributio unction is obtained by measuring the electron energy spectrum,consisting of 16ener-t gies,6times per rotation for each of the 6detectors.The velocity distributions are hus approximated by 576points.Moments calculated from these distributions give a ,t time series,at 6s.spacing,for bulk flow velocity components,density,temperature emperature anisotropy,etc.For the results reported here,each measurement of the s r ion velocity distribution requires about 30seconds to accumulate,with measurement epeated every 87seconds (29spacecraft spins).,1The early trajectory of the WIND spacecraft,following its launch on November 1994until the end of January 1995,consisted of four phasing orbits about the Earth r w with apogees beyond the lunar orbit,after which the spacecraft passed across the luna ake at a distance of 6.5lunar radii,R from the center of the moon on December 27,O 1994.L bservations and InterpretationShown in Figure 1are some of the plasma parameters derived from the measure-m ments made by SWE as well as the magnetic field magnitude and longitude from easurements made by the WIND MFI instrument (R.P.Lepping principal investiga-s tor)as WIND crossed the lunar wake.The ion and electron number densities both how reductions clearly associated with the moon.The minimum densities measured o t are about 20times lower than the solar wind densities measured just before entry int he wake.While the electron temperature increases in the wake by a factor of aboutfour,the ion temperature remains fairly constant.The magnetic field strength rose in e p the wake as reported previously [Ness et al.1968;1969].Within the region where th lasma is significantly depleted,two distinct proton distributions are simultaneously h a detected.Each appears as a cold beam,narrow in velocity space,one convecting wit speed slightly faster than the ambient solar wind,and the other convecting slightly slower.The appearance of inter-penetrating proton beams in the lunar wake is illustrated in -t Figure 2,which presents a time sequence showing observed current (which is propor ional to particle flux)versus energy/charge scans from a SWE Faraday cup.The e u detector is pointing in roughly the same near-sunward direction for each scan.Th pper (earliest)panel shows a large peak associated with solar wind protons,and a e a smaller peak at higher energy/charge due to alpha particles.In succeeding panels,th lpha signal disappears,and solar wind proton peak becomes lower in amplitude while -t moving to higher energies.By the time of the fourth panel (14:58UT),a second pro on signal at lower energy/charge is clearly present.As time proceeds,the first proton r e signal diminishes,while the second proton peak grows in amplitude,moves to highe nergy/charge,and appears to evolve into what is the unperturbed solar wind proton peak by the time of the last panel,when WIND has moved beyond the wake.The measurements show that ambient solar wind conditions changed somewhat dur-s ing WIND’s lunar encounter:when WIND emerged from the wake,the solar wind peed was higher and the density lower (see Figure 1)and the alpha particle flux was u much lower (see Figure 2).Thus,one must keep in mind that the wake may have ndergone some slight temporal changes due to solar wind variations during the space-m craft crossing.Nonetheless,these observations show important overall features that are ost probably characteristic of a steady-state wake structure.;t The moon absorbs solar wind,carving out a plasma cavity in the downstream wake he solar wind acts to refill that cavity.The physical processes and phenomena S involved in the expansion of plasma into a vacuum are described in the review by amir et al.[1983]and references therein.Initially,because they are lighter,more e i mobile,and have a greater thermal speed,the electrons attempt to fill in ahead of th ons.The resulting ambipolar electric field accelerates the ions into the cavity.t Because the solar wind is collisionless,particles filling in the plasma cavity are res-ricted to move only along magnetic field lines.The ambipolar electric field and the .T ion acceleration resulting from that field are directed along the magnetic field lines hus,the plasma does not fill the wake from all directions at once,but instead fills it t from two opposite "sides"whose locations are determined by the magnetic field orien-ation.The variations of the plasma parameters observed as WIND crossed the lunar -s wake are similar in character to the one-dimensional analytical solutions for the expan ion of plasma into vacuum discussed by Samir et al.[1983].Because the direction of n the filling motion is restricted by the field,a model which is one-dimensional is onetheless quite useful.The principal characteristics of plasma expansion into vacuum are demonstrated in .G the solution for the simplest case:a single ion species and Maxwellian electrons iven a plasma at time t =0of density N in the region x <0,and a vacuum in the o i e eregion x >0,the solutions for the ion and electron densities,N and N ,and for thi i on speed V are as follows:(1) +1)]x tN =N =N exp[−(S e i o o (2)+1)x t V =S (S i o o e o e i √ -t where S is the sound speed,approximated here as T /M ;T is the electron tempera ure and M is the ion mass.The solutions are valid for times t >0and x >−S t.T i o hese equations describe several important features.First,a region of decreased ndensity propagates back into the plasma as a rarefaction wave with speed S (Equatio o 1o ).Second,at any fixed time t =t >0,the density decreases exponentially as a func-,tion of x +S t,the distance from the rarefaction wave front at x =−S t (Equation 1)o o w i hile the ion filling-in speed V increases linearly with distance from the wave front r (Equation 2).The region of decreased plasma density is referred to as the expansion egion.The exponential decrease in density,the rarefaction wave,and the linearly e e increasing ion speed are all evident in the WIND lunar wake measurements,as will b xplained below.As the solar wind encounters and flows past the moon,plasma begins to expand into e s the lunar wake cavity,while at the same time continuing to convect away from th un.There is a rough correspondence between the distance downstream from the e w moon,and variable t in the above equations -the time for plasma to expand into th ake.Moreover,a spacecraft which crosses the wake at a fixed distance downstream,t is scanning the plasma as a function of the position variable x,while the expansion ime t is kept fixed.WIND crossed the wake at a distance of ∼∼6.5R from the moon,L 4s while the solar wind flow speed was ∼∼475km/s,giving a fixed expansion time of ∼∼2econds.It follows that there is a correspondence between the measurement time e m shown in Figure 1,and the variable x in Equations 1and 2,so the variation of th easured density and speed versus time can be compared to the dependence on x of N and V in Equations 1and 2.Since the expansion is restricted to occur along the i i magnetic field,a correction is made to take into account the fact that the field makes an angle of about 45°to the solar wind flow direction.As WIND enters the wake,the log of the density decreases approximately linearly y d (see Figure 1),in agreement with the exponential behavior of Equation 1.The densit ecrease matches Equation 1reasonably well for S ∼∼40km/s,while T /M using the o e i m e √ easured T is somewhat less (35km/s).The density recovery on exit from the wake a l is less smooth,and does not follow an exponential dependence so well over such arge spatial distance,possibly because of solar wind temporal changes during lunar t 1wake crossing.If only the final portion of the recovery is considered,between abou 5:22and 15:32,the density variation appears somewhat less steep than that seen on t wake entry,consistent with a somewhat higher sound speed at that time.T /M a √ i wake exit was 40km/s,a value greater than that seen at entry.e Evidence for a rarefaction wave is found by comparing the time period during which w the density was below ambient solar wind values,to the time period for which WIND as within the light shadow of the moon.Figure 1shows the times of entry and exitfor the plasma depletion region,and for the light shadow;the density depleted region e a is larger than the geometric shadow.These four times can be used to determine th ngle between the rarefaction wave and the light shadow (the sunward direction)on o t each side of the wake.As WIND entered the lunar wake,that angle was about 5°t he sunward direction,while on the exit side the angle was about 4.4°.At the time of f t entry the solar wind was flowing from a direction approximately 2.5°to the west o he solar direction (in a moon-fixed reference frame).Correcting for the solar wind h i flow,the angle of the rarefaction wave to the aberrated wake axis is about 2.5°,whic s somewhat less than the 3.1°expected based on S ∼∼T /M.At the time of exit,no o e i √ .T angle correction is required;the solar wind flow direction was nearly anti-sunward he observed exit-side wave angle is somewhat larger than the 3.1°expected from w T /M on that side.Taking into account any aberration due to the solar wind flo e i √ direction,we find on both sides rarefactions consistent with waves propagating out-ward from the lunar wake at roughly the expected angles.Next we examine the velocity profiles for the two proton streams:the first stream d s which is related to solar wind filling in the wake from the entry side,and the secon tream which is related to solar wind filling in the wake from the exit side.The i speeds and flow angles for each are shown in Figure 1.The speed of the first stream ncreases linearly as WIND moves into the expansion region.From Equation 2we see :that by knowing the rate of change of V with x one can estimate the expansion time t i ∆i V /∆x =1/t.Determining the rate of change of the speed profile for the first proton e g stream we obtain an expansion time of 22seconds,a value similar to our estimat iven above.The acceleration of ions into the wake occurs along the direction of the d magnetic field,which is at an angle of about 45°to the undisturbed solar wind flow irection (see Figure 3).This field angle requires the ion acceleration to have a com--t ponent parallel to the initial solar wind flow,causing ions to speed up.The accelera ion deflects the flow into the wake from the entry side.,a In the middle of the wake,the second stream is slower than that from the entry side nd it increases in speed rather than decreases as WIND moves away from the middle s f of the wake (see Figure 1)!The velocity of the second stream can be understood a ollows.As on the entry side,the ion acceleration into the wake must occur along the e a magnetic field on the exit side.However,because the field is 45°to the flow,th cceleration on the exit side has a component anti-parallel to the initial solar wind flow,causing the ions to slow down as they move into the wake (see Figure 3).The description presented of ions accelerated into the wake electrostatically is con-o sistent with the fact that the ion temperature remains steady across the wake.The bserved velocity change from solar wind to wake center corresponds to an electros-f 2tatic potential change of about 400volts over an expansion distance on the order o 000km.From these rough figures,we get a crude estimate of 2×10volts/m for the −4d c time-averaged electric field acting on the ions.The oblique angle of the magnetic fiel auses the wake to refill asymmetrically.In the wake center,where two streams of different velocity are seen simultaneously,the velocity difference (V V −V )between the 12e o two must lie along the magnetic field B .In order to verify that alignment,the cosin f the angle between (V V −V )and B was determined,and found to be ≥0.96for every case.12F w In an accompanying paper by Farrell et al.[1996]in this issue,the presence of UL aves observed by the magnetometer just outside the wake is reported and interpreted.t These waves coincided with the observation by the VEIS of counter streaming elec-rons with velocities between 5×10and 2×10km/s.Though no effects were seen in t 34he moment plots,the electron distribution functions,shown by Farrell et al.[1996],flwere noticeably distorted from their form in the nearby solar wind.The bi-directional ow of electrons in this period is easily visible on the entry side,and though less pro-C nounced,was also present on the exit side.onclusionsObservations by the SWE instrument on the WIND spacecraft while crossing the lunar wake at a distance of 6.5R on December 27th,1994show the following L 1effects:.The electron temperature increases,and as predicted by a simple theory,the density s i decreases exponentially as the spacecraft moves across the wake from its edge toward ts center.2.An ambipolar electric field set up by the subsonic solar wind electrons accelerates s o the ions towards the center of the wake along the magnetic field.The electric field i f the order 2×10volts/m.This acceleration has the effect of increasing the total v −4elocity of the plasma from the entrance side of the wake,and reducing the total velo-v city of the plasma from the exit side.We thus observed two ion streams,one with elocity greater and one with velocity less,than the solar wind.The temperature of 3the ions remained unchanged,consistent with an electrostatic acceleration of the ions..The region of plasma depletion extends beyond the lunar light shadow,consistent .T with a rarefaction wave moving out from the wake into the undisturbed solar wind he entry-side and exit-side waves were slightly differently directed with respect to the e w wake because of changes in the solar wind flow direction and sound speed during th ake traversal.4.ULF waves were observed adjacent to the entrance to the wake.Although no w effects were noticed in the plot of the electron moments,bi-directional electron flow as readily apparent in the velocity distribution function plots.Bi-directional flow S also appeared on the exit side of the wake,but to a less pronounced extent.everal lunar encounters will occur during the remainder of the WIND mission.We r w intend to use data from all of these to perform a more extensive study of the luna ake,with the intent of determining the variation of the wake signature with distance from the moon.g t Acknowledgements.The authors wish to thank Dr.R.P.Lepping for the providin he magnetic field measurements,and Frank Marcoline for helping with the plasma (data analysis.Work at MIT was supported in part by NASA grant NAG5-2839GSFC),and the MIT Undergraduate Research Opportunities program.A Referencescuna,M.H,K.W.Ogilvie,D.N.Baker,S.A.Curtis,D.H,Fairfield,and W.H.,7Mish,The Global Geospace Science Program and its investigations,Space Sci.Rev.1,5-21,1995.Farrell,W.M.,R.J.Fitzenrieter,C.J.Owen,J.B.Byrnes,R.P.Lepping,K.W.Ogil-l vie,F.Neubauer,Upstream ULF waves and energetic electrons associated with the unar wake:detection of precursor activity,Geophys.Res.Lett.,(in press),1996..Krall,N.and D.A.Tidman,Magnetic field fluctuations near the moon,J.Geophys Res.,74,6439,1969.Lepping et al.,The Wind magnetic field investigation,Space Sci.Rev,71.,207-229,N 1995.ess,N.F.,and Y.C.Whang,Reply,J.Geophys.Res.,77,6924-6925,1972.d Ness,N. F.,and K.H.Schatten,Detection of interplanetary magnetic fiel fluctuations stimulated by the lunar wake,J.Geophys.Res.,74,6425-6438,1969.f Ness,N. F.,K.W.Behannon,H. E.Taylor,Y. C.Whang,Perturbations o the interplanetary magnetic field by the lunar wake,J.Geophys.Res.,73,3421-3440,O 1968.gilvie,K.W.,et al.,SWE,A comprehensive plasma instrument for the Wind space-S craft,Space Sci.Rev,71.,55-77,1995.amir,Uri,K.H.Wright,Jr.,N.H.Stone,The expansion of plasma into a vacuum:p basic phenomena and processes and applications to space plasma physics,Rev.Geo-hys.and Space Phys.,21,1631-1646,1983.y Schubert,G.,and B.R.Lichtenstein,Observations of moon-plasma interactions b orbital and surface experiments,Rev.Geophys.and Space Phys.,12,592-626,1974.Sonnett,C.P.,and J.D.Mihalov,Lunar fossil magnetism and perturbations of the solar wind,J.Geophys.Res.,77,588-603,1972.sF Figure Caption igure 1.Plasma and magnetic field parameters for lunar wake crossing."+"indi-t cates entry-side proton parameters,diamonds indicate exit-side parameters.From the op panel to the bottom:proton density,proton and electron temperatures,proton .V speed,plasma east/west flow angle,the longitude of B (0°is sunward),and B ertical lines mark the times bounding the light shadow and the region in which n e plasma density is reduced from ambient solar wind rger uncertainty i ast/west angle between 15:15and 15:37UT caused by the cumulative effect of d satellite spin rate change in the shadow:an angle offset developed between the esired optimal and the actual Faraday cup pointing directions,resulting in a less F accurate flow angle determination during that time interval.igure 2.Time sequence of Faraday cup current versus energy/charge spectra as WIND crossed the wake.At 14:32UT,signal peaks are due to solar wind H and +H e ++e ++++,with (density H )/(density H )=0.25.At 15:02UT there are two H signal peaks.At 15:43UT,peak signals due to solar wind H and a small flux of H .+e++n o Figure 3.(a)The schematic representation of lunar wake on Dec 27,1994.Regio f plasma depletion propagates out from wake as a rarefaction wave.Streamlines of i plasma flow are shown entering the wake from the satellite entry and exit side.The on acceleration is directed along magnetic field lines as shown;flow speeds up on b the entry side and slows down on the exit side.(b)Illustrative schematic to show ehavior of the ion flow vectors measured as Wind crossed the wake.Does not accurately show the actual changes in relative magnitude or direction of vectors.。