Self Assembly of three New Copper coordination Polymers Based on N
22版:Unit 5 Poems(创新设计)

目录
6._s_h_e_l_f_______ n. 架子;搁板 7._b_l_a_n_k_______ adj. 空白的;没表情的
n. 空白;空格 8.__so_w_________ vt.&vi. 种;播种 9.__d_a_w_n_______ n. 黎明;开端;萌芽 10.__t_ea_s_e_______ vi.&vt. 取笑(某人);揶揄;逗弄 11.__d_e_a_d_li_n_e____ n. 最后期限;截止日期 12.__s_tr_i_n_g______ n. 细绳;线;一串
目录
4.The poems may not make sense and even seem contradictory , but _____________th_e_y__a_r_e_e_a_s_y_t_o_l_e_a_r_n_a_n_d__r_e_c_it_e_______________________. 这些诗歌不一定有意义,有的甚至看起来前后矛盾,但它们很容易学习和背 诵。
5.____p_o_li_s_h____ vt.修改;润色;抛光 n.上光剂;抛光;擦亮→___p_o_l_is_h_e_d___
adj.磨光的;擦亮的
目录
6.__c_o_m_p_l_ic_a_t_e__ vt.使复杂化→_c_o_m__p_l_ic_a_t_e_d_ adj.复杂的;难懂的 7.____r_a_c_e_____ n.种族→____r_a_c_ia_l____ adj.种族的;人种的 8.__c_o_r_r_es_p_o_n_d__ vi.相一致;符合;相当于;通信→__c_o_rr_e_s_p_o_n_d_e_n_c_e_ n.来往信
SELF-ASSEMBLY OF MACROMOLECULES ON MULTILAYERED PO
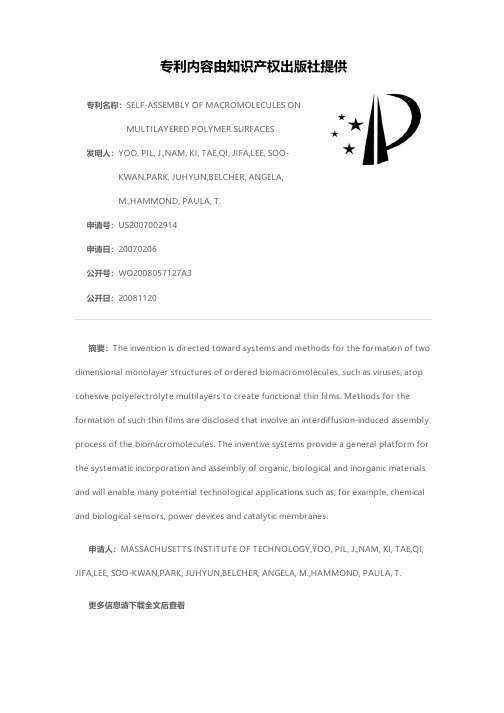
专利名称:SELF-ASSEMBLY OF MACROMOLECULES ON MULTILAYERED POLYMER SURFACES发明人:YOO, PIL, J.,NAM, KI, TAE,QI, JIFA,LEE, SOO-KWAN,PARK, JUHYUN,BELCHER, ANGELA,M.,HAMMOND, PAULA, T.申请号:US2007002914申请日:20070206公开号:WO2008057127A3公开日:20081120专利内容由知识产权出版社提供摘要:The invention is directed toward systems and methods for the formation of two dimensional monolayer structures of ordered biomacromolecules, such as viruses, atop cohesive polyelectrolyte multilayers to create functional thin films. Methods for the formation of such thin films are disclosed that involve an interdiffusion-induced assembly process of the biomacromolecules. The inventive systems provide a general platform for the systematic incorporation and assembly of organic, biological and inorganic materials and will enable many potential technological applications such as, for example, chemical and biological sensors, power devices and catalytic membranes.申请人:MASSACHUSETTS INSTITUTE OF TECHNOLOGY,YOO, PIL, J.,NAM, KI, TAE,QI, JIFA,LEE, SOO-KWAN,PARK, JUHYUN,BELCHER, ANGELA, M.,HAMMOND, PAULA, T.更多信息请下载全文后查看。
一个典型铜萃取三相物处理技术应用实践研究

世界有色金属 2020年 7月下152化学化工Chemical Engineering一个典型铜萃取三相物处理技术应用实践研究何 建(中国中铁资源集团有限公司,北京 100039)摘 要:文章以刚果(金)某铜钴矿为研究对象。
通过对铜萃取三相物产生的主要原因和处理方法进行分析,提出三种参考方法旨在促进整体萃取效果,帮助企业合理控制成本。
关键词:铜钴矿;萃取;三相物处理;实践研究中图分类号:TF111 文献标志码:A 文章编号:1002-5065(2020)14-0152-2Study on the application of a typical copper extraction three-phase material treatment technologyHE Jian(China Railway Resources Group Co., Ltd,Beijing 100039,China)Abstract: This paper takes a copper cobalt deposit in Congo (Kinshasa) as the research object. Based on the analysis of the main causes and treatment methods of copper extraction of three-phase substances, three reference methods are proposed to promote the overall extraction effect and help enterprises reasonably control the cost.Keywords: copper cobalt ore; extraction; three phase material treatment; practical research刚果(金)近年来铜钴矿湿法冶炼企业绝大多数采用浸出(L)-萃取(SX)-电积工艺(EW)生产铜,其萃取系统经长期运转都必然产生一些絮凝状的污物(俗称三相物[1-4])当其量逐渐增加后,可能影响系统的稳定运行,如果随水相或是有机相进入下一工序,不仅浪费有机相还可能会导致富铜液质量下降,进而影响最终产品质量。
氨基酸的结晶
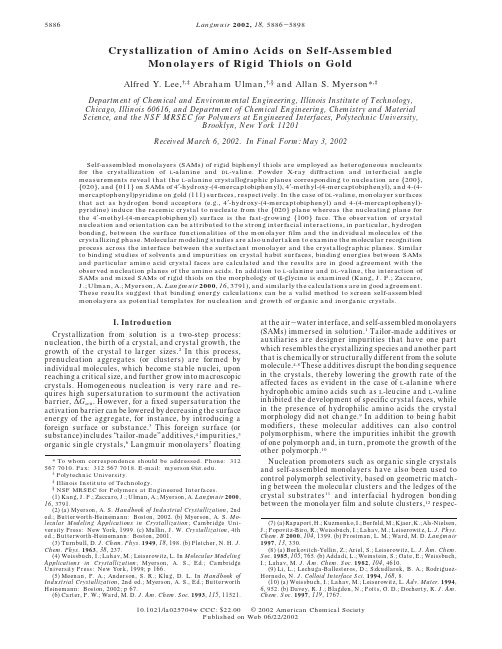
Crystallization of Amino Acids on Self-AssembledMonolayers of Rigid Thiols on GoldAlfred Y.Lee,†,‡Abraham Ulman,†,§and Allan S.Myerson*,‡Department of Chemical and Environmental Engineering,Illinois Institute of Technology, Chicago,Illinois60616,and Department of Chemical Engineering,Chemistry and Material Science,and the NSF MRSEC for Polymers at Engineered Interfaces,Polytechnic University,Brooklyn,New York11201Received March6,2002.In Final Form:May3,2002Self-assembled monolayers(SAMs)of rigid biphenyl thiols are employed as heterogeneous nucleants for the crystallization of L-alanine and DL-valine.Powder X-ray diffraction and interfacial angle measurements reveal that the L-alanine crystallographic planes corresponding to nucleation are{200}, {020},and{011}on SAMs of4′-hydroxy-(4-mercaptobiphenyl),4′-methyl-(4-mercaptobiphenyl),and4-(4-mercaptophenyl)pyridine on gold(111)surfaces,respectively.In the case of DL-valine,monolayer surfaces that act as hydrogen bond acceptors(e.g.,4′-hydroxy-(4-mercaptobiphenyl)and4-(4-mercaptophenyl)-pyridine)induce the racemic crystal to nucleate from the{020}plane whereas the nucleating plane for the4′-methyl-(4-mercaptobiphenyl)surface is the fast-growing{100}face.The observation of crystal nucleation and orientation can be attributed to the strong interfacial interactions,in particular,hydrogen bonding,between the surface functionalities of the monolayer film and the individual molecules of the crystallizing phase.Molecular modeling studies are also undertaken to examine the molecular recognition process across the interface between the surfactant monolayer and the crystallographic planes.Similar to binding studies of solvents and impurities on crystal habit surfaces,binding energies between SAMs and particular amino acid crystal faces are calculated and the results are in good agreement with the observed nucleation planes of the amino acids.In addition to L-alanine and DL-valine,the interaction of SAMs and mixed SAMs of rigid thiols on the morphology of R-glycine is examined(Kang,J.F.;Zaccaro, J.;Ulman,A.;Myerson,ngmuir2000,16,3791),and similarly the calculations are in good agreement. These results suggest that binding energy calculations can be a valid method to screen self-assembled monolayers as potential templates for nucleation and growth of organic and inorganic crystals.I.IntroductionCrystallization from solution is a two-step process: nucleation,the birth of a crystal,and crystal growth,the growth of the crystal to larger sizes.2In this process, prenucleation aggregates(or clusters)are formed by individual molecules,which become stable nuclei,upon reaching a critical size,and further grow into macroscopic crystals.Homogeneous nucleation is very rare and re-quires high supersaturation to surmount the activation barrier,∆G crit.However,for a fixed supersaturation the activation barrier can be lowered by decreasing the surface energy of the aggregate,for instance,by introducing a foreign surface or substance.3This foreign surface(or substance)includes“tailor-made”additives,4impurities,5 organic single crystals,6Langmuir monolayers7floating at the air-water interface,and self-assembled monolayers (SAMs)immersed in solution.1Tailor-made additives or auxiliaries are designer impurities that have one part which resembles the crystallizing species and another part that is chemically or structurally different from the solute molecule.4,8These additives disrupt the bonding sequence in the crystals,thereby lowering the growth rate of the affected faces as evident in the case of L-alanine where hydrophobic amino acids such as L-leucine and L-valine inhibited the development of specific crystal faces,while in the presence of hydrophilic amino acids the crystal morphology did not change.9In addition to being habit modifiers,these molecular additives can also control polymorphism,where the impurities inhibit the growth of one polymorph and,in turn,promote the growth of the other polymorph.10Nucleation promoters such as organic single crystals and self-assembled monolayers have also been used to control polymorph selectivity,based on geometric match-ing between the molecular clusters and the ledges of the crystal substrates11and interfacial hydrogen bonding between the monolayer film and solute clusters,12respec-*To whom correspondence should be addressed.Phone:312 5677010.Fax:3125677018.E-mail:myerson@.†Polytechnic University.‡Illinois Institute of Technology.§NSF MRSEC for Polymers at Engineered Interfaces.(1)Kang,J.F.;Zaccaro,J.;Ulman,A.;Myerson,ngmuir2000, 16,3791.(2)(a)Myerson,A.S.Handbook of Industrial Crystallization,2nd ed.;Butterworth-Heinemann:Boston,2002.(b)Myerson,A.S.Mo-lecular Modeling Applications in Crystallization;Cambridge Uni-versity Press:New York,1999.(c)Mullin,J.W.Crystallization,4th ed.;Butterworth-Heinemann:Boston,2001.(3)Turnbull,D.J.Chem.Phys.1949,18,198.(b)Fletcher,N.H.J. Chem.Phys.1963,38,237.(4)Weissbuch,I.;Lahav,M.;Leiserowitz,L.In Molecular Modeling Applications in Crystallization;Myerson, A.S.,Ed.;Cambridge University Press:New York,1999;p166.(5)Meenan,P.A.;Anderson,S.R.;Klug,D.L.In Handbook of Industrial Crystallization,2nd ed.;Myerson,A.S.,Ed.;Butterworth Heinemann:Boston,2002;p67.(6)Carter,P.W.;Ward,M.D.J.Am.Chem.Soc.1993,115,11521.(7)(a)Rapaport,H.;Kuzmenko,I.;Berfeld,M.;Kjaer,K.;Als-Nielsen, J.;Popovitz-Biro,R.;Weissbuch,I.;Lahav,M.;Leiserowitz,L.J.Phys. Chem.B2000,104,1399.(b)Frostman,L.M.;Ward,ngmuir 1997,13,330.(8)(a)Berkovitch-Yellin,Z.;Ariel,S.;Leiserowitz,L.J.Am.Chem. Soc.1985,105,765.(b)Addadi,L.;Weinstein,S.;Gate,E.;Weissbuch,I.;Lahav,M.J.Am.Chem.Soc.1982,104,4610.(9)Li,L.;Lechuga-Ballesteros,D.;Szkudlarek,B.A.;Rodriguez-Hornedo,N.J.Colloid Interface Sci.1994,168,8.(10)(a)Weissbuch,I.;Lahav,M.;Leiserowitz,L.Adv.Mater.1994, 6,952.(b)Davey,R.J.;Blagden,N.;Potts,G.D.;Docherty,R.J.Am. Chem.Soc.1997,119,1767.5886Langmuir2002,18,5886-589810.1021/la025704w CCC:$22.00©2002American Chemical SocietyPublished on Web06/22/2002tively.Similar to Langmuir monolayers,self-assembled monolayers can be used as an interface across which stereochemical matching13and hydrogen bonding14in-teraction can transfer order and symmetry from the monolayer surface to a growing crystal.However,SAMs and mixed SAMs15lack the mobility of molecules at an air-water interface and hence the possibility to adjust lateral positions to match a face of a nucleating crystal. This is clearly evident in the case of the SAMs of rigid biphenyl thiols,where even conformational adjustment is not possible.Recently,SAMs of4-mercaptobiphenyl have been shown to be more superior to those of al-kanethiolates and are stable model surfaces.16Further-more,the ability to engineer surface functionalities at the molecular level makes SAMs of rigid thiols very attractiveas templates for heterogeneous nucleation. Organosilane monolayer films have been used to promote nucleation and growth of calcium oxalate mono-hydrate crystals17and have been employed in“biomimetic”synthesis as observed in the oriented growth of CaCO318 and iron hydroxide crystals.19Functionalized SAMs of alkanethiols have also been shown to control the oriented growth of CaCO3.20This was also evident in the hetero-geneous nucleation and growth of malonic acid crystals21 on alkanethiolate SAMs on gold where the monolayer composition strongly influenced the orientation of the malonic acid crystals.Additionally,functionalized alkane-thiolate SAMs have enhanced the growth of protein crystals.22More recently,SAMs and mixed SAMs of rigid thiols served as templates.1It was observed that glycine nucleated in the R-form independent of the hydroxyl and pyridine surface concentration and the morphology of the glycine crystal was very sensitive to the OH and pyridine site densities.Self-assembled monolayers on solid surfaces offer many advantages for enhanced crystal nucleation.In this work, SAMs of rigid thiols on gold are employed to investigate the effects of interfacial molecular recognition on nucle-ation and growth of L-alanine and DL-valine crystals.In addition,molecular modeling techniques are employed to examine the affinity between monolayer surfaces and particular amino acid crystal faces and to gain a better understanding of the molecular recognition events oc-curring.The modeling techniques employed are similar to studies of solvent and additive interactions on crystal habit23but have never been applied to organic monolayer films as templates for nucleation.II.Experimental SectionMaterials.Anhydrous ethanol was obtained from Pharmco (Brookfield,CT).L-Alanine(CH3CH(NH2)CO2H),and DL-valine ((CH3)2CHCH(NH2)CO2H)were purchased from Aldrich and used without further purification.Distilled water purified with a Milli-Q water system(Millipore)was used.Details of the synthesis of the4′-substituted4-mercaptobiphenyl(see Figure1)are described elsewhere.24Gold Substrate and Monolayer Preparation.Glass slides were cleaned in ethanol in an ultrasonic bath at40°C for10min. The slides were next treated in a plasma chamber at an argon pressure of0.1Torr for30min.Afterward,they were mounted in the vacuum evaporator(Key High Vacuum)on a substrate holder,approximately15cm above the gold cluster.The slides were baked overnight in a vacuum(10-7Torr)at300°C.Gold (purity>99.99%)was evaporated at a rate of3-5Å/s until the film thickness reached1000Å;the evaporation rate and film thickness were monitored with a quartz crystal microbalance (TM100model from Maxtek Inc.).The gold substrates were annealed in a vacuum at300°C for18h.After cooling to room temperature,the chamber was filled with high-purity nitrogen and the gold slides were either placed into the adsorbing solution right after the ellipsometric measurement was performed or stored in a vacuum desiccator for later use.25Atomic force microscopy(AFM)studies24revealed terraces of Au(111)with typical crystalline sizes of0.5-1µm2.Monolayers were formed by overnight(∼18h)immersion of clean substrates in10µm ethanol solutions of the thiols.The substrates were removed from the solution,rinsed with copious amounts of absolute ethanol to remove unbound thiols,and blown dry with a jet of nitrogen. Contact angle measurements,IR spectroscopy,and ellipsometry showed that after1h,90%or more of the SAMs are formed.26 Thus,to ensure equilibrium SAMs,the gold substrates were left overnight in the dipping solution.Crystal Growth.Nucleation and growth experiments were carried out in Quartex jars(1oz.)at25°C.Supersaturated solutions(25%)of L-alanine and DL-valine were obtained by dissolving 4.58g and 1.95g in22.0g of Millipore water, respectively.The solutions were heated to65°C for90min in an ultrasonic bath to obtain complete dissolution.The solutions were cooled to room temperature for90min before the SAMs were carefully introduced and aligned vertically to the wall. Macrocrystals of L-alanine and DL-valine nucleated at the surfaces(11)(a)Bonafede,S.J.;Ward,M.D.J.Am.Chem.Soc.1995,117, 7853.(b)Mitchell,C.A.;Yu,L.;Ward,M.D.J.Am.Chem.Soc.2001, 123,10830.(12)Carter,P.W.;Ward,M.D.J.Am.Chem.Soc.1994,116,769.(13)(a)Landau,E.M.;Levanon,M.;Leiserowitz,L.;Lahav,M.;Sagiv, J.Nature1985,318,353.(b)Weissbuch,I.;Berfeld,M.;Bouwman,W.; Kjaer,K.;Als,J.;Lahav,M.;Leiserowitz,L.J.Am.Chem.Soc.1997, 119,933.(14)Weissbuch,I.;Popvitz,R.;Lahav,M.;Leiserowitz,L.Acta Crystallogr.1995,B51,115.(15)For a review on SAMs of thiols on gold see:(a)Ulman,A.An Introduction to Ultrathin Organic Films:From Langmuir-Blodgett to Self-Assembly;Academic Press:Boston,1991.(b)Ulman,A.Chem. Rev.1996,96,1533.(16)(a)Kang,J.F.;Ulman,A.;Liao,S.;Jordan,R.J.Am.Chem.Soc. 1998,120,9662.(b)Kang,J.F.;Jordan,R.;Ulman,ngmuir1998,14,3983.(17)Campbell,A.A.;Fryxell,G.E.;Graff,G.L.;Rieke,P.C.; Tarasevich,B.J.Scanning Microsc.1993,7(1),423.(18)Archibald,D.D.;Qadri,S.B.;Gaber,ngmuir1996,12, 538.(19)Tarasevich,B.J.;Rieke,P.C.;Liu,J.Chem.Mater.1996,8,292.(20)Aizenberg,J.;Black,A.J.;Whitesides,G.M.J.Am.Chem.Soc. 1999,121,4500.(21)Frostman,L.M.;Bader,M.M.;Ward,ngmuir1994, 10,576.(22)Ji,D.;Arnold,C.M.;Graupe,M.;Beadle,E.;Dunn,R.V.;Phan, M.N.;Villazana,R.J.;Benson,R.;Colorado,R.,Jr.;Lee,T.R.;Friedman, J.M.J.Cryst.Growth2000,218,390.(23)(a)Docherty,R.;Meenan,P.In Molecular Modeling Applications in Crystallization;Myerson,A.S.,Ed.;Cambridge University Press: New York,1999;p106.(b)Myerson,A.S.;Jang,S.M.J.Cryst.Growth 1995,156,459.(c)Walker,E.M.;Roberts,K.J.;Maginn,ngmuir 1998,14,5620.(d)Evans,J.;Lee,A.Y.;Myerson,A.S.In Crystallization and Solidification Properties of Lipids;Widlak,N.,Hartel,R.W.,Narine, S.,Eds.;AOCS Press:Champaign,IL,2001;p17.(24)Kang,J.F.;Ulman,A.;Liao,S.;Jordan,R.;Yang,G.;Liu,G. Langmuir2001,17,95.(25)(a)Jordan,R.;Ulman,A.J.Am.Chem.Soc.1998,120,243.(b) Jordan,R.;Ulman,A.;Kang,J.F.;Rafailovich,M.;Sokolov,J.J.Am. Chem.Soc.1999,121,1016.(26)Ulman,A.Acc.Chem.Res.2001,34,855.Figure1.Rigid4′-substituted4-mercaptobiphenyls.Crystallization of Amino Acids on Thiol SAMs Langmuir,Vol.18,No.15,20025887and near the edge of the substrates.Only crystals having visible SAM area around them were considered,and the rest were discarded.The chosen crystals attached to the substrates were removed from the solution and stored in a vacuum desiccator for later analysis.Due to the strong adhesion of the crystal face to the SAM surface,gold marks were often observed on the crystal face that nucleated on the SAM surface.Characterization.A Rudolph Research AutoEL ellipsometer was used to measure the thickness of the monolayer surface.The He -Ne laser (632.8nm)light fell at 70°on the sample and reflected into the analyzer.Data were taken over five to seven spots on each sample.The measured thickness of the SAMs of biphenyl thiols ranged from 12to 14Å,assuming a refractive index of 1.462for all films.Powder X-ray diffraction patterns of crystalline L -alanine and DL -valine were obtained with a Rigaku Miniflex diffractometer with Cu K R radiation (λ)1.5418Å).All samples were manually ground into fine powder and packed in glass slides for analysis.Data were collected from 5°to 50°with a step size of 0.1°.Crystal habits of L -alanine and DL -valine were indexed by measuring the interfacial angles using a two-circle optical goniometer.All possible measured interfacial angles were compared with the theoretical values derived from the unit cell parameters of L -alanine and DL -valine crystals.27,28III.Modeling SectionIII.1.General.All of the binding energy calculations,including molecular mechanics and dynamics simulations,are carried out with the program Cerius 2.The overall methodology and procedures are summarized in Figure 2.The crystal structures of each amino acid are obtained from the Cambridge Crystallographic Database (ref codes GLYCIN17,LALNIN12,and VALIDL for R -glycine,L -alanine,and DL -valine,respectively).To accurately predict the crystal morphology,molecular mechanics simulations using a suitable potential function (or force field)are performed.In this work,molecular simulations are carried out using the DREIDING 2.21force field.29The van der Waals forces are approximated with the Lennard-Jones 12-6expression,and hydrogen bonding energy is modeled using a Lennard-Jones-like 12-10expression.The Ewald summation technique is employed for the summation of long-range van der Waals and electrostatic interactions under the periodic boundary conditions,and the charge distribution within the molecule is calculated using the Gasteiger method.30ttice Energy Calculation.The lattice energy E lat,also known as the cohesive or crystal binding energy,is calculated by summing all the atom -atom interactions between a central molecule and all the surrounding molecules in the crystal.If the central molecule and the n surrounding molecules each have n ′atoms,thenwhere V kij is the interaction between atom i in the central molecule and atom j in the k th surrounding parison to the “experimental”lattice energy,V exp ,allows us to assess the accuracy of the intermolecular interactions between the molecules by the defined po-tential function.where the term 2RT represents a compensation factor for the difference between the vibrational contribution to the crystal enthalpy and gas-phase enthalpy 31and ∆H sub is the experimental sublimation energy.III.3.Morphological Predictions.The morphology of each amino acid crystal is predicted using the attach-ment energy (AE)32calculation and the Bravais -Friedel -Donnay -Harker (BFDH)law.33The habit or shape of the crystal depends on the growth rate of the faces present.Faces that are slow growing have the greatest morpho-logical importance,and conversely,faces that are fast growing have the least morphological importance and are the smallest faces on the grown crystal.The simplest morphological simulation is the BFDH law which assumes that the linear growth rate of a given crystal face is inversely proportional to the corresponding interplanar distance after taking into account the extinction conditions of the crystal space group.The attachment energy of a crystal face is the difference between the crystal energy and the slice energy.Hartman and Bennema 32found that the relative growth rate of a face is directly proportional to the attachment energy and as a result,the more negative the attachment energy (or more energy released)for a particular face,the less prominent that face is on the crystal.Conversely,faces with the lowest attachment energies are the slowest growing faces and thus have the greatest morphological importance .III.4.Molecular Modeling of SAMs of 4-Mercapto-biphenyls on a Au(111)Surface.Molecular dynamics (MD)simulations are useful techniques in gaining insights on the structural and dynamical properties of self-assembled monolayers.In contrast to molecular mechan-ics,molecular dynamics computes the forces and moves the atom in response to forces,while molecular mechanics computes the forces on the atoms and changes their position to minimize the interaction energy.Recently,MD simulations have been used to investigate the packing order and orientation of rigid 4-mercaptobiphenyl thiol monolayers on gold surfaces.Results show that hydrogen-terminated biphenylmercaptan packs in the herringbone conformation 34and suggest average tilt angles of 8°.(27)Simpson,H.J.;Marsh,R.E.Acta Crystallogr .1966,20,550.(28)Mallikarjunan,M.;Rao,S.T.Acta Crystallogr .1969,B25,296.(29)Mayo,S.L.;Olafson,B.D.;Goddard,W.A.,III J.Phys.Chem .1990,94,8897.(30)Gasteiger,J.;Marsili,M.Tetrahedron 1980,36,3219.(31)Williams,D.E.J.Phys.Chem .1966,45,3370.(32)(a)Hartman,P.;Bennema,P.J.Cryst.Growth 1980,49,145.(33)(a)Bravais,A.Etudes Crystallographiques ;Gauthier-Villars:Paris,1866.(b)Friedel,M.G.Bulletin de la Societe Francaise de Mineralogie 1907,30,326.(c)Donnay,J.D.;Harker,D.Am.Mineral.1937,22,446.Figure 2.Overall scheme showing the computational meth-odology adopted when calculating the binding energy betweenthe crystallographic plane and the monolayer surface.Elat)∑k )1n ∑i )1n ′∑j )1n ′V kij (1)V exp )-∆H sub -2RT(2)5888Langmuir,Vol.18,No.15,2002Lee et al.Based on this work,molecular mechanics simulations are performed for hydroxy-and methyl-terminated 4-mer-captobiphenyl along with 4-(4-mercaptophenyl)pyridine for binding studies with different crystallographic planes.In the periodic model,each unit cell contains four biphenyl molecules and the geometric parameters are a )10.02Å,b )42.25Å,c )10.11Åand R )138.3°, )119.9°,γ)95.7°.The length in the y -direction is set to ∼42Åto ensure two-dimensional periodicity.Also,the gold atoms are arranged in a hexagonal lattice along the XY plane with a nearest neighbor atom of 2.88Å,and the biphenyl occupied a ( 3× 3)R30°Au(111)lattice.To simulate different 4′-substituted 4-mercaptobiphenyls,minimiza-tion was carried out by fixing the biphenyl moiety and varying the substituents at the 4′-position.As a result,the simulated models yielded uniform ordered SAMs of 4′-substituted 4-mercaptobiphenyls and 4-(4-mercapto-phenyl)pyridine with identical packing structure and dynamics to those of a hydrogen-terminated monolayer of biphenylmercaptan (Figure 3).However,this is not true experimentally since adsorption of different 4′-substituted 4-mercaptobiphenyls on gold surfaces results in different monolayer structures and thus one of the main assump-tions made in this work.III.5.Binding of Crystal Habit Faces to SAMs of 4-Mercaptobiphenyls on a Au(111)Surface.Based on BFDH and attachment energy morphology prediction,crystal habit faces with the highest morphological im-portance are chosen for binding studies.The crystal surfaces of interest are cleaved and extended to a 3×3unit cell and partially fixed,allowing flexibility in the tail atoms of the amino acid molecules and a more accurate representation of the effects of SAMs of rigid thiols on the crystallographic plane in the calculation of binding energies.The crystal surface is then docked onto a 3×1×3partially fixed nonperiodic monolayer surface,and the conjugate gradient energy minimization technique is performed.Next,the crystal surface is moved to another site on the monolayer surface and the minimization calculations are again performed.This process was repeated 15-20times to obtain the global minimum.For each monolayer surface,numerous calculations are carried out with different crystallographic planes of each amino acid.The binding energy (φBE )of each crystallographic surface with the monolayer surface iswhere φIE is the minimum interaction energy of the monolayer and crystal surfaces,φM is the minimum energy of the monolayer surface in the absence of the crystal face but in the same conformation as it adopts on the surface,and φS is the minimum energy of the crystal surface with no monolayer surface present and in the same molecular conformation in which it docks on the surface.Negative values of binding energies indicate preferential binding of the crystallographic surfaces with SAMs of 4-mercaptobiphenyl.In cases where the binding energy is positive,there is a less likely chance that the particular crystal face will interact and nucleate on the monolayer surface.Thus,using this approach it is possible to screen self-assembled monolayers as possible templates for nucleation and growth of crystals.IV.Results and DiscussionIV.1.Crystallization of Amino Acids on SAMs on Gold.L -Alanine crystallizes from water in the ortho-rhombic space group P 21212(a )6.025Å,b )12.324Å,and c )5.783Å),27and the morphology of the crystals is bipyramidal,dominated by the {020},{120},{110},and {011}growth forms,35as shown in Figure 4.The crystal grown in aqueous solution is indexed by comparing the interfacial angles measured by optical goniometry and theoretical values based on the unit cell of L -alanine.Powder X-ray diffraction patterns (Figure 5)and inter-facial angle measurements reveal that L -alanine crystals nucleating on SAM surfaces crystallize in the ortho-rhombic space group with similar unit cell dimensions.However,functionalized SAMs induce the formation of L -alanine crystals in different crystallographic directions.L -Alanine crystals display the normal bipyramidal habit but are randomly oriented with the different surfaces.In methyl-terminated SAMs,L -alanine selectively nucle-ated on the {020}plane on the surface (Figure 6),whereas in 100%OH SAM surfaces,L -alanine nucleated on an unobserved {200}side face.The crystal exhibits a similar morphology as observed in aqueous solution with an appearance of a {200}face adjacent to the {110}planes (Figure 6).In both cases,the area of each crystal face is substantially larger than those of the other faces on the crystal.The SAM surfaces almost act as an additive or impurity molecule specifically interacting with the crystal face and consequently reducing the relative growth rate and modifying the habit.Crystallization of L -alanine on 4-(4-mercaptophenyl)pyridine surfaces resulted in the {011}face as the plane corresponding to nucleation (Figure 6).The preferential interaction of the monolayer with the {011}face can be attributed to hydrogen bonding at the crystal -monolayer interface.Unlike the other two sur-faces where they can serve as both hydrogen bond donors and acceptors (4′-hydroxy-4-mercaptobiphenyl)or solely as H-bond donors (4′-methyl-4-mercaptobiphenyl),the pyridine electron pair at the surface only serve as hydrogen bond acceptors.The binding of the pyridine surface and the {011}plane can be explained by the amino and methyl groups protruding out perpendicular to the plane (Figure 7)and forming N -H ‚‚‚N and C -H ‚‚‚N hydrogen bonds with the SAM surface,respectively.In contrast,the 100%(34)Ulman,A.;Kang,J.F.;Shnidman,Y.;Liao,S.;Jordan,R.;Choi,G.Y.;Zaccaro,J.;Myerson,A.S.;Rafailovich,M.;Sokolov,J.;Flesicher,C.Rev.Mol.Biotech .2000,74,175.(35)Lehmann,M.S.;Koetzle,T.F.;Hamilton,W.C.J.Am.Chem.Soc .1972.101,2657.Figure 3.Snapshots of (a)4′-methyl-4-mercaptobiphenyl,(b)4′-hydroxy-4-mercaptobiphenyl,(c)4-(4-mercaptophenyl)pyri-dine,and (d)mixed SAMs of 4′-methyl-4-mercaptobiphenyl and 4′-hydroxy-4-mercaptobiphenyl (top view).φBE )φIE -(φM +φS )(3)Crystallization of Amino Acids on Thiol SAMs Langmuir,Vol.18,No.15,20025889CH 3and 100%OH SAM surfaces do not interact as strongly with the hydrogen bond donating plane.In a similar manner,the appearance of an unobserved {200}face of L -alanine grown in aqueous solution on [Au]-S -C 6H 4-C 6H 4-OH can be attributed to hydrogen bonds forming between the two surfaces.The {200}surface contains alternating methyl (CH 3)and carboxylic groups (COO -)that form N -H ‚‚‚O and O ‚‚‚H -O with the hydroxide group of the monolayer film (Figure 7),ideal for binding with surfaces that can serve as both hydrogen bond donors and acceptors.As a result,the preferential interaction leads to the stabilization and appearance of the {200}face.The oriented nucleation of L -alanine crystals on func-tionalized SAMs arises due to the different molecular structures of each crystal face.Similar to the adsorption of additive onto a crystal face,the interaction (or binding)with the monolayer surface depends on the functional group that each crystal face possesses.As a result of preferential interactions with specific crystal faces,in-terfacial molecular recognition directs nucleation and subsequently influences the crystal growth.In addition to L -alanine,SAMs of rigid thiols are employed to investigate the possibility of inhibiting the racemic crystal and inducing the formation of one of its enantiomers.The powder X-ray diffraction pattern (Figure 8)reveals that DL -valine nucleates in the monoclinic form independent of the hydroxyl,methyl,or pyridine surface concentration and that there was no trace of conglomer-ates.DL -Valine crystallizes in the monoclinic space group P 21/c with a unit cell of dimensions a )5.21Å,b )22.10Å,c )5.41Å,and )109.2°.28Although the structural literature reports three separate space group assignments,Leiserowitz and co-workers 36have shown that two of the three space groups (P 21and P 1)are highly improbable for racemic crystals.Interfacial angle measurements and powder X-ray diffraction undertaken in this work agreed much better with the theoretical values and simulated pattern based on the unit cell of the monoclinic space group(36)Wolf,S.G.;Berkovitch-Yellin,Z.;Lahav,M.;Leiserowitz,L.Mol.Cryst.Liq.Cryst .1990,186,3.Figure 4.Crystallographic image (a)and morphology (b)of L -alanine crystal grown from aqueoussolution.Figure 5.X-ray diffractograms of L -alanine nucleated on functionalized SAMs,compared with L -alanine crystallized from aqueous solution (bottom).Indices of the crystallographic planes corresponding to the diffraction intensities of major peaks are indicated at the top.5890Langmuir,Vol.18,No.15,2002Lee et al.。
电感耦合等离子体发射光谱法(ICP-OES)测定三元前驱体中的硫含量
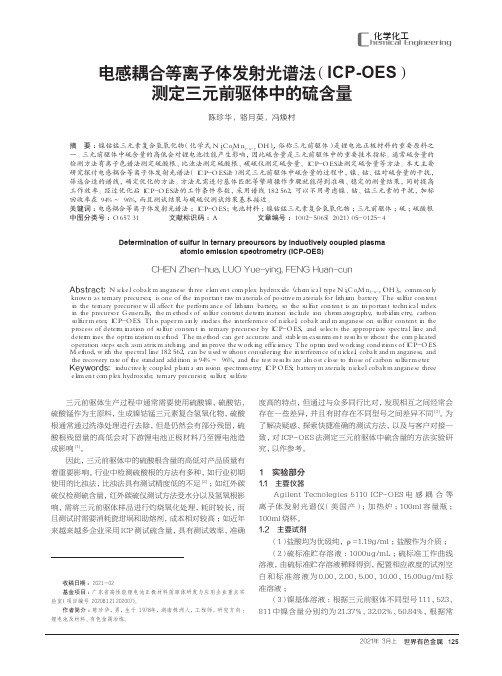
125化学化工C hemical Engineering电感耦合等离子体发射光谱法(ICP-OES )测定三元前驱体中的硫含量陈珍华,骆月英,冯焕村(广东佳纳能源科技有限公司,广东 英德 513056)摘 要:镍钴锰三元素复合氢氧化物(化学式NixCoyMn1-x-y(OH)2,俗称三元前驱体)是锂电池正极材料的重要原料之一。
三元前驱体中硫含量的高低会对锂电池性能产生影响,因此硫含量是三元前驱体中的重要技术指标。
通常硫含量的检测方法有离子色谱法测定硫酸根、比浊法测定硫酸根、碳硫仪测定硫含量、ICP-OES法测定硫含量等方法。
本文主要研究探讨电感耦合等离子体发射光谱法(ICP-OES法)测定三元前驱体中硫含量的过程中,镍、钴、锰对硫含量的干扰,筛选合适的谱线,确定优化的方法。
方法无需进行基体匹配等繁琐操作步骤就能得到准确、稳定的测量结果,同时提高工作效率。
经过优化后ICP-OES法的工作条件参数,采用谱线182.562,可以不用考虑镍、钴、锰三元素的干扰,加标回收率在94%~96%,而且测试结果与碳硫仪测试结果基本接近。
关键词:电感耦合等离子体发射光谱法;ICP-OES;电池材料;镍钴锰三元素复合氢氧化物;三元前驱体;硫;硫酸根中图分类号:O657.31 文献标识码:A 文章编号:1002-5065(2021)05-0125-4Determination of sulfur in ternary precursors by inductively coupled plasmaatomic emission spectrometry (ICP-OES)CHEN Zhen-hua, LUO Yue-ying, FENG Huan-cun(Guangdong Jiana Energy Technology Co., Ltd,Yingde 513056,China)Abstract: Nickel cobalt manganese three element complex hydroxide (chemical type NixCoyMn1-x-y(OH)2, commonly known as ternary precursor, is one of the important raw materials of positive materials for lithium battery. The sulfur content in the ternary precursor will affect the performance of lithium battery, so the sulfur content is an important technical index in the precursor. Generally, the methods of sulfur content determination include ion chromatography, turbidimetry, carbon sulfur meter, ICP-OES. This paper mainly studies the interference of nickel, cobalt and manganese on sulfur content in the process of determination of sulfur content in ternary precursor by ICP-OES, and selects the appropriate spectral line and determines the optimization method. The method can get accurate and stable measurement results without the complicated operation steps such as matrix matching, and improve the working efficiency. The optimized working conditions of ICP-OES Method, with the spectral line 182.562, can be used without considering the interference of nickel, cobalt and manganese, and the recovery rate of the standard addition is 94%~96%, and the test results are almost close to those of carbon sulfur meter.Keywords: inductively coupled plasma emission spectrometry; ICP OES; battery materials; nickel cobalt manganese three element complex hydroxide; ternary precursor; sulfur; sulfate收稿日期:2021-02基金项目:广东省高性能锂电池正极材料前驱体研发与应用企业重点实验室(项目编号2020B121202007)。
电感耦合等离子体法(ICP法)测定镍钴锰三元复合氢氧化物中的微量磷元素
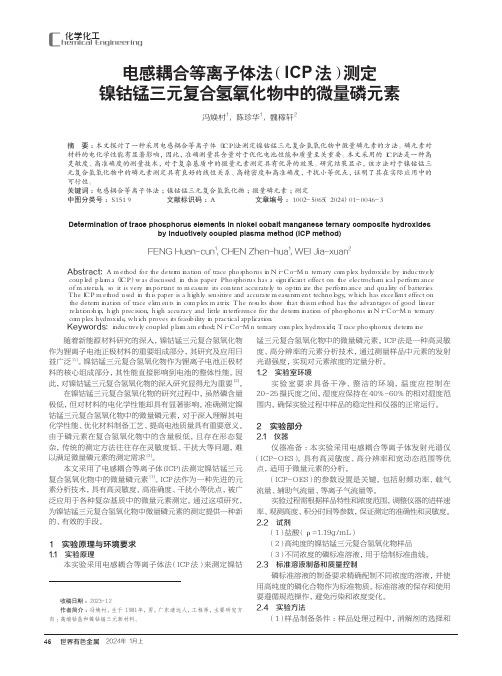
46化学化工C hemical Engineering电感耦合等离子体法(ICP 法)测定镍钴锰三元复合氢氧化物中的微量磷元素冯焕村1,陈珍华1,魏稼轩21.广东佳纳能源科技有限公司,广东 英德 513056;2.江西佳纳能源科技有限公司,江西 龙南 341700摘 要:本文探讨了一种采用电感耦合等离子体(ICP)法测定镍钴锰三元复合氢氧化物中微量磷元素的方法。
磷元素对材料的电化学性能有显著影响,因此,准确测量其含量对于优化电池性能和质量至关重要。
本文采用的ICP法是一种高灵敏度、高准确度的测量技术,对于复杂基质中的微量元素测定具有优异的效果。
研究结果显示,该方法对于镍钴锰三元复合氢氧化物中的磷元素测定具有良好的线性关系、高精密度和高准确度,干扰小等优点,证明了其在实际应用中的可行性。
关键词:电感耦合等离子体法;镍钴锰三元复合氢氧化物;微量磷元素;测定中图分类号:S151.9 文献标识码:A 文章编号:1002-5065(2024)01-0046-3Determination of trace phosphorus elements in nickel cobalt manganese ternary composite hydroxidesby inductively coupled plasma method (ICP method)FENG Huan-cun 1, CHEN Zhen-hua 1, WEI Jia-xuan 21.Guangdong Jiana Energy Technology Co., Ltd.,Yingde 513056,China; 2. Jiangxi Jiana Energy Technology Co., Ltd., Longnan 341700,ChinaAbstract: A method for the determination of trace phosphorus in Ni-Co-Mn ternary complex hydroxide by inductively coupled plasma (ICP) was discussed in this paper. Phosphorus has a significant effect on the electrochemical performance of materials, so it is very important to measure its content accurately to optimize the performance and quality of batteries. The ICP method used in this paper is a highly sensitive and accurate measurement technology, which has excellent effect on the determination of trace elements in complex matrix. The results show that this method has the advantages of good linear relationship, high precision, high accuracy and little interference for the determination of phosphorus in Ni-Co-Mn ternary complex hydroxide, which proves its feasibility in practical application. Keywords: inductively coupled plasma method; Ni-Co-Mn ternary complex hydroxide; Trace phosphorus; determine收稿日期:2023-12作者简介:冯焕村,生于1981年,男,广东清远人,工程师,主要研究方向:高端钴盐和镍钴锰三元新材料。
天然高分子材料之胶原

从生物医学角度,胶原在发育、伤口愈合、血小板活化和 血管生成中具有重要作用。另外很多遗传病的起因也被证 明是由于胶原发生了突变,而胶原在其合成、降解或免疫 学方面的微小变化也可以引起许多疾病。
通常把50%胶原分子发生变形的温度称为变性温 度Td(denaturation temperature)。由于这 一现象和结晶的熔解相类似,故又称为熔点或熔 解温度。胶原的变性温度与羟脯氨酸的含量呈正 相关。
其它生化特性
天然胶原除非先经尿素处理使之变性,否则不易 溶于碱、弱酸及一般浓的中性盐类。
戊二醛是到目前为止应用最广泛的蛋白质交联剂,其水溶液在室 温下就能高效地与胶原缩氨酸链上的氨基反应,形成稳定交联。
是一种同型双功能交联剂。其两个醛基可分别与两个相同或不同 分子的伯氨基形成Schiff 碱, 将两个分子以五碳桥连接起来。 高浓度的GTA 与胶原分子赖氨酸或羟赖氨酸残基E2氨基反应, 形成分子间交联。
ECM是细胞外大分子构成的网络。包括:胶原、 非胶原糖蛋白、氨基聚糖与蛋白聚糖、弹性蛋白 等。
结缔组织中含量较高。 影响细胞的存活、死亡、增殖和分化。
Collagen 胶原
胶原是动物中的主要纤维蛋白,是所有多细胞动物,包括 海绵体、无脊椎动物和脊椎动物的细胞外基质的主要结构 成分。
胶原原纤维占人和高等动物总蛋白量可高达30%,其作用 是保持组织完整以及提供每一个结缔组织所需要的特殊力 学性能。
原胶原分子二级结构
原胶原形成 胶原纤维
胶原纤维电镜图
胶原类型
在原纤维中发现了20种胶原,其中5种含量最丰 富的胶原类型是: I 型胶原是骨、腱、角膜和韧带中的主要胶原, 最高可达体内所有胶原的90%。 II 型胶原在软骨、玻璃体和脊索中形成原纤维。 III 型胶原与I 型胶原并存于结缔组织。 V型和VI型为异三聚体,与其他三种胶原共存 于原纤维中。
手工制作铜器英语作文
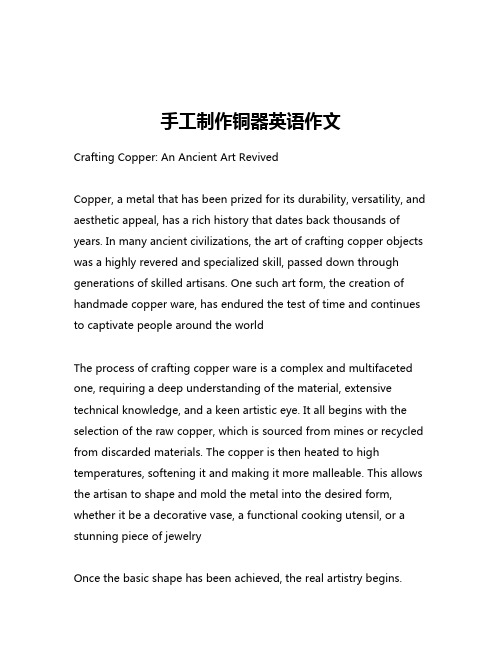
手工制作铜器英语作文Crafting Copper: An Ancient Art RevivedCopper, a metal that has been prized for its durability, versatility, and aesthetic appeal, has a rich history that dates back thousands of years. In many ancient civilizations, the art of crafting copper objects was a highly revered and specialized skill, passed down through generations of skilled artisans. One such art form, the creation of handmade copper ware, has endured the test of time and continues to captivate people around the worldThe process of crafting copper ware is a complex and multifaceted one, requiring a deep understanding of the material, extensive technical knowledge, and a keen artistic eye. It all begins with the selection of the raw copper, which is sourced from mines or recycled from discarded materials. The copper is then heated to high temperatures, softening it and making it more malleable. This allows the artisan to shape and mold the metal into the desired form, whether it be a decorative vase, a functional cooking utensil, or a stunning piece of jewelryOnce the basic shape has been achieved, the real artistry begins.Using a variety of specialized tools, the artisan carefully hammers, bends, and sculpts the copper, coaxing it into the desired form. This requires a delicate touch and years of experience, as the metal can be temperamental and easily damaged if not handled with the utmost care. The artisan must also be mindful of the unique properties of copper, such as its tendency to oxidize and change color over time, and incorporate these qualities into the final designAs the piece takes shape, the artisan may also incorporate additional decorative elements, such as intricate engravings, embossed patterns, or the addition of other materials like enamel or gemstones. These embellishments not only enhance the visual appeal of the piece but also imbue it with a sense of history and cultural significance. Many copper ware artisans draw inspiration from the rich cultural traditions of their respective regions, incorporating motifs and designs that reflect the unique aesthetic sensibilities of their peopleThe final stage of the copper crafting process is the finishing touches. This may involve a series of polishing and buffing steps to ensure a smooth, lustrous surface, or the application of a protective coating to preserve the metal's color and luster. The attention to detail and the dedication of the artisan are evident in every aspect of the finished piece, making each one a true work of artBut the charm of handmade copper ware extends beyond itsaesthetic appeal. These objects are often imbued with a sense of history and tradition, serving as tangible links to the rich cultural heritage of the societies that produced them. For many, the act of using or displaying a handcrafted copper piece is a way to honor the craftsmanship and artistry of the past, while also celebrating the enduring appeal of this versatile and beautiful materialIn recent years, there has been a growing resurgence of interest in the art of copper crafting, as people around the world rediscover the unique charm and timeless appeal of these handmade objects. Artisans in various parts of the world are reviving ancient techniques and incorporating modern innovations to create stunning new pieces that capture the essence of this timeless art formWhether it's a simple yet elegant copper vase, a beautifully engraved serving tray, or a meticulously crafted copper sculpture, these handmade creations have the power to transport us to a different time and place, to connect us with the rich cultural heritage of the past, and to inspire us with the boundless creativity and skill of the human spirit. As we continue to explore and appreciate the art of copper crafting, we are not just celebrating the beauty of the material itself, but also the enduring legacy of the artisans who have dedicated their lives to mastering this ancient and revered craft。
- 1、下载文档前请自行甄别文档内容的完整性,平台不提供额外的编辑、内容补充、找答案等附加服务。
- 2、"仅部分预览"的文档,不可在线预览部分如存在完整性等问题,可反馈申请退款(可完整预览的文档不适用该条件!)。
- 3、如文档侵犯您的权益,请联系客服反馈,我们会尽快为您处理(人工客服工作时间:9:00-18:30)。
of 1 were obtained, yield 46% (based on Cu). Anal. Calcd for C9H12CuN2O8S (%): C, 24.38; H, 2.76; N, 6.28; S, 7.33. Found: C, 24.33; H, 2.70; N, 6.30; S, 7.20.2.3.2 Synthesis of [Cu2(L)2]n (2).The procedures were similar to the synthesis of 1 except that the reaction temperature was 120︒C, green block crystals of 2 were obtained with a yield of 23% (based on Cu), Anal. Calcd for C9H8Cu2N2O6S (%): C, 27.12; H, 2.05; N, 7.13; S, 8.06. Found: C, 27.04; H, 2.00; N, 7.01; S, 8.01.2.3.3 Synthesis of [Cu(HL)2]n (3). The procedures were similar to the synthesis of 1 except that the reaction temperature was 90︒C. Yield 55% (based on Cu). Anal. Calcd for C18H18CuN4O12S2 (%): C, 35.47; H, 2.87; N, 9.20; S, 10.45. Found: C, 35.41; H, 2.95; N, 9.18; S, 10.49.2.4 X-ray crystallographyDiffraction intensities were collected on a Bruker Apex CCD area-detector diffractometer (Mo-Ka, r=0.71073 A˚) at 293 K. The structure was solved with direct methods and reEned with a full-matrix least-squares technique with the SHELXTL program package[21]. The hydrogen atoms were placed at calculated positions and refined as riding atoms with isotropic displacement parameters. Crystallographic crystal data and structure processing parameters for 1-3are summarized in Table 1. Selected bond lengths and bond angles for complexe 1-3are listed in table 2, Hydrogen bonds for complexes 1-3 are listed in table 3.3. Results and Discussion3.1. Description of structure3.1.1 Crystal structure of [Cu(L)]·6H2O]n (1).A single-crystal X-ray diffraction study reveals that 1adopts a 3-D coordination polymer which crystallizes in the orthorhombic, space group P212121. The asymmetric unit contains one Cu(II) cation, one ligand and six water molecules.As illustrated in figure 1a, Cu(II) adopts a distorted tetrahedron coordination environment, which is completed by two nitrogen atoms from pyridine (N1A) and sulfamine (N2) of two ligands as well as two oxygen atoms(O1, O4B) from two carboxylates located at the equatorial plane, and one oxygen (O3) from carboxylate at the axial positions. Carboxylic acid using both cis-trans-Bridged bidentate and monodentate coordination of the way. Because of Jahn-Teller effect the bonds, so the Cu-N range from 1.964 (13) to 2.055 (13) Å (Cu(1)–N(2) = 2.1.064 and Cu(1)–N(1)A = 2.055Å), Cu-O bond length range from 1.9066 (11) to 2.422 (1) Å, which similar to the reported bond length[22].Three Cu(II) cations are bridged by one ligand to construct 2-D sheet( figure 1b). Each threeof Cu(II) cations is bridged by two carboxylate groups from two L2- anions in cis-trans-Bridged bidentate mode . The further link by ligand bridging the Cu3 unit with 21 helicity along the c-axisdirection, in which the helical pitch of 9.834 Å is equal to the length of the c-axis (figure 1c). As shown in Figure 1d, the extension of the structure into a 3D framework is accomplished by one ligand bearing two Cu from the different 2D layers along c-axis.3.1.2 Crystal structure of [Cu2(L)2]n (2). A single-crystal X-ray diffraction analysis reveals that crystal 2crystallizes in the monoclinic system, space group P21/c. The asymmetric unit contains two crystallographically independent Cu(II) cations and one ligand. As illustrated in Figure 2a, Cu1(II) cations adopts a distorted tetrahedral coordination environment, which is completed by Cu2(II) cations (Cu(1)–Cu(2)=2.619(13)) and two bridging carboxylate oxygen atoms (O2, O3A) from two different ligands as well as one nitrogen atom (N2) from sulfamine at the axial position. Cu2(II) cations is completed by Cu1(II) cations and bridging carboxylate oxygen atoms (O1, O4) from two different ligands。
Cu1(II) cations is four-coordinated,Cu2(II) cations is three-coordinated. Both carboxylate groups of the ligand adopt cis-cis-Bridged bidentate mode coordinating with two Cu1(II) cations and two Cu2(II) cations. The Cu–Cu bond lengths is 2.619 Å, which is in the normal range[22].Three Cu1–Cu2 units are bridged by one ligand to contruct an 1-D ladder coordination polymer in which Cu–Cu unit take as handraill and pyridine is foodboard in the equatorial direction. From the crystal packing of the coordination polymer, the pyridine are parallel to each other with a centroid-to-centroid distance of 3.4084 Å, which supports the presence of π-πstacking interactions (figure 2b). The non-coordinated sulfamine and sulfonic acid groups participate in N–H···O hydrogen bonds, which defind 3D hydrogen-bonded network and support the supramolecular architecture (figure 2c).3.1.3 Crystal structure of [Cu(HL)2]n (3).A single-crystal X-ray diffraction study reveals that 3 adopts a 1-D coordination polymer which crystallizes in the space group P21/c. The asymmetric unit contains one Cu(II) cation and two ligands. As illustrated in figure 1a, the Cu(II) cation is four-coordinated by two nitrogen atoms from pyridine of two ligands(Cu(1)–N(1) = 2.0140 and Cu(1)–N(1)A = 2.0140Å) and two carboxylate oxygen atoms of two ligands(Cu(1)–O(2)B = 1.9435 and Cu(1)–O(2)C = 1.9435Å). Four coordination sites of Cu(II) are coplanar (N(1)A–Cu(1)–N(1) = 180.00 and O(2)C–Cu(1)–O(2)B = 180.00°) in a perfectly symmetric and form an approximate spuare that Cu(II) cation is located in the center and coordinated atoms are occupied four vertex position of the spuare. The bond lengths and bond angles are in the normalrange[22].Two Cu(II) cations are bridged by one ligand to contruct an 1-D coordination polymer in the equatorial direction(figure 3b). Notably, the presence of sulfonic acid groups and sulfamine leads to the formation of N–H···O and C–H···O hydrogen bonds interactions, which defines a 3D hydrogen-bonded network and, thus, supports the supramolecular architecture (figure 3c).3.2 Powder X-Ray diffractionIn order to check the purity of complex 1, powder X-ray diffraction (XRD) of the as-synthesized sample was measured at room temperature. As shown in Figure 4, the peak positions of experimental patterns are in good agreement with the simulated ones, which clearly indicates good purity of the complex.3.2 Magnetic PropertieThe magnetic property of the complex 1 was investigated over the temperature range 2.0-300.0 K, and the resulting data is shown in Figure 5. For 1, the χm T value at 300 K is 0.51 cm3 mol-1 K, which is much higher than the expected value (0.16 cm3mol-1K ) of two isolated spin-only Cu(II) ions (S=1/2, g =2.0). As T is lowered, χm T decreases continuously to a value of 3.22 cm3mol-1K at 2 K. This behavior in dicatesa dominant antiferromagnetic interaction between the Cu(II) is in correspondence with the Curie-Weiss law in the range of 45-300 K with C = 0.53 cm3mol-1K and θ = -17.43 K.4. ConclusionIn this paper, Cu(NO3)2 ·3H2O, N-[(3-Pyridine)-sulfonyl]aspartate as raw material, in the same ratio material、solvent and pH, under different reaction temperatures , three copper coordination polymers based on N-[(3-Pyridine)-sulfonyl]aspartate ligand were synthesized. Single-crystal X-ray diffraction study reveals that 1 adopts a 3-D coordination polymer which crystallizes in the orthorhombic, space group P212121; 2 crystallizes in the monoclinic system, space group P21/c; 3 adopts a 1-D coordination polymer which crystallizes in the space groupP21/c. A structural comparison of these polymers suggests that different reaction conditions play important role in the construction of resulting architectures for 1-3. It seems more conducive to the synthesis of 3D complexes at high temperature conditions.AcknowledgmentsThe authors acknowledge the financial support from the Scientific Research and Technological Development Project of Guilin (No. 20110330);.The project of Synthesis, Properties of crystal structure and Applied research of 2,4,6 - pyridyl amide(NO. 200807MS090); The project of education department of Guangxi province(NO.YB2014331); Guangxi Natural Science Foundation2014GXNSFAA118035.References[1] Aijaz A., Saudo E.C., Bharadwaj P.K.. Cryst. Growth. Des., 2011,11, p. 1122.[2] Murray L.J., Dinca M., Long J.R.. Chem. Soc. Rev., 2009,38, p. 1294.[3] Rosi N.L., Eckert J., Eddaoudi M., Yaghi O.M., et al. Science., 2003, 300, p. 1127.[4] Huang F.P., Li H.Y., Yu Q., et al.. CrystEngComm.,2012,14, p. 4756;[5] Seo J.S., Whang D., Lee H., et al. Nature., 2000, 404, p. 982.[6] Armelaoa L., Quici S., Barigelletti F., et al. Coord. Chem. Rev., 2010, 254, p. 487.[7] Ma S.Q., Simmons J.M., Yuan D.Q., et al. Chem. Commun., 2009, 27, p. 4049.[8] Zeng M.H., Yao M.X., Liang H., et al. Angew. Chem., 2007, 46, p. 1832.[9] Wu C.D., Lu C.Z., Zhuang H.H., Huang J.S.. Inorg. Chem., 2002, 41, p. 5636.[10] Lu J., Shen E.H., Li Y.G., et al. Cryst. Growth. Des., 2005, 5, p. 65.[11] Xu C.Y., Li L.K., Wang Y.P., et al. Cryst. Growth. Des., 2011, 11, p. 4667.[12] Anokhina E.V., Jacobson A.J. J. Am. Chem. Soc., 2004, 126, p. 3044.[13] Vaidhyanathan R., Bradshaw D., Rebilly J.N.,et al. Angew.Chem.,2006, 45, p. 6495.[14] Jiang Y.M., Wang X.J., Ying X.J., et al. Inorg. Chem. Commun., 2006, 9, p. 1063.[15] Hu S., He K.H., Zeng M.H., et al. Inorg. Chem., 2008, 47, p. 5218.[16] Li J.X., Jiang Y.M., Lian B.R. J. Chem. Crystallogr., 2008, 38, p. 711.[17] Zhang S.H., Jiang Y.M., Liu Z.M. J. Coord. Chem., 2008,61, p. 1927.[18] Li J.X., Jiang Y.M., Chen M.J.. J. Coord. Chem., 2008, 61, p. 1765.[19] Qin X.Y., Zhang S.H., Jiang Y.M., et al. J. Coord. Chem., 2009, 62, p. 427.[20] Liang Y.J., Meng X.J., Huang F.P., et al. J. Coord. Chem., 2011, 64, p. 3751.[21] G.M. Sheldrick. SHELXS-97, University of Göttingen,Germany 1997.[22] Abdel-nasser, Alaghaz M. A. J. Mole. Stru., 2014 1072, p. 103.[23] Chowdhuri D.S., Rana A., Bera M., et al. Polyhedron., 2009,28, p. 2131.[24] Deng Z.P., Huo L.H., Li M.S., et al. Cryst. Growth. Des., 2011,11, p. 3090.Figure CaptionsFigure 1. (a) Coordination environment of Cu(II) ions in 1 with hydrogen atoms omitted for clarity with thermal ellipsoids at 50% level.(b) 2-D sheet along the c-axis of ploymer 1. (c) the left- and right-handed helical chains along c-axis. (d) Extended 3D network viewed along the c-axis.Figure 2. (a) Coordination environment of Cu(II) ions in 2 with hydrogen atoms omitted for clarity with thermal ellipsoids at 60% level. (b) 1-D ladder of ploymer 2 and distance of centroid-to-centroid of pyrazine rings. (c) Hydrogen bonds that make up a 3D network polymer.Figure 3. (a) Coordination environment of Cu(II) ions in 3 with hydrogen atoms omitted for clarity with thermal ellipsoids at 50% level. (b) 1-D chain of ploymer 3. (c) Hydrogen bonds that make up a 3D network polymer.Figure 4 XRD pattern of complex 10501001502002503000.0000.0050.0100.0150.0200.0250.0300.0350501001502002503000100200300400500600700χM / (m o l c m -3)T /K0.050.100.150.200.250.300.350.400.45χM T /( c m 3 K m o l -1)1/χM / (m o l c m -3)T /KFigure 5. Temperature dependence of χM , χ M T vs T and χm -1 vs T (inset) for 11.948(5)。