Land Use Changes of an Aeolian-Loessial Soil Area in Northwest China: Implications for Ecologic
LAND USE AND LAND COVER CHANGE IN CHINA
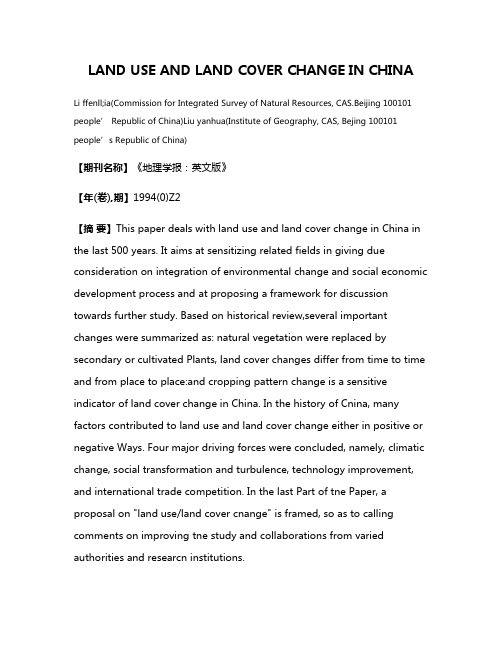
LAND USE AND LAND COVER CHANGE IN CHINALi ffenll;ia(Commission for Integrated Survey of Natural Resources, CAS.Beijing 100101 people’ Republic of China)Liu yanhua(Institute of Geography, CAS, Bejing 100101 people’s Republic of China)【期刊名称】《地理学报:英文版》【年(卷),期】1994(0)Z2【摘要】This paper deals with land use and land cover change in China in the last 500 years. It aims at sensitizing related fields in giving due consideration on integration of environmental change and social economic development process and at proposing a framework for discussion towards further study. Based on historical review,several important changes were summarized as: natural vegetation were replaced by secondary or cultivated Plants, land cover changes differ from time to time and from place to place:and cropping pattern change is a sensitive indicator of land cover change in China. In the history of Cnina, many factors contributed to land use and land cover change either in positive or negative Ways. Four major driving forces were concluded, namely, climatic change, social transformation and turbulence, technology improvement, and international trade competition. In the last Part of tne Paper, a proposal on "land use/land cover cnange" is framed, so as to calling comments on improving tne study and collaborations from varied authorities and researcn institutions.【总页数】16页(P25-40)【关键词】land;use;land;cover;change;global;change;driving;force;impacts;framework 【作者】Li ffenll;ia(Commission for Integrated Survey of Natural Resources, CAS.Beij ing 100101 people’ Republic of China)Liu yanhua(Institute of Geography, CAS, Bejing 100101 people’s Republic of China)【作者单位】【正文语种】中文【中图分类】F129.9【相关文献】nd use and land cover change and its driving forces in Maqu County, China in the past 25 years [J], JunFeng Lu;ZhiBao Dong;GuangYinHu;WenJin Li;WanYin Luo;MingLiang Tan;nd use and land cover change and its driving forces in Maqu County, China in the past 25 years [J], JunFeng Lu ;ZhiBao Dong;GuangYinHu;WenJin Li;WanYin Luo;MingLiang Tan3.A review of land use/land cover change mapping in the China-Central Asia-West Asia economic corridor countries [J], Amin Naboureh;Jinhu Bian;Guangbin Lei;Ainong Li4.Effects of land use and land cover change on soil physical, chemical and mineralogical properties under grassland in Mu Us sand land of Inner Mongolia, China [J], JIN Zhaond use/land cover change responses to ecological water conveyance in the lower reaches of Tarim River,China [J], WANG Shanshan;ZHOU Kefa;ZUO Qiting;WANG Jinlin;WANG Wei因版权原因,仅展示原文概要,查看原文内容请购买。
临海的变化六级英语作文
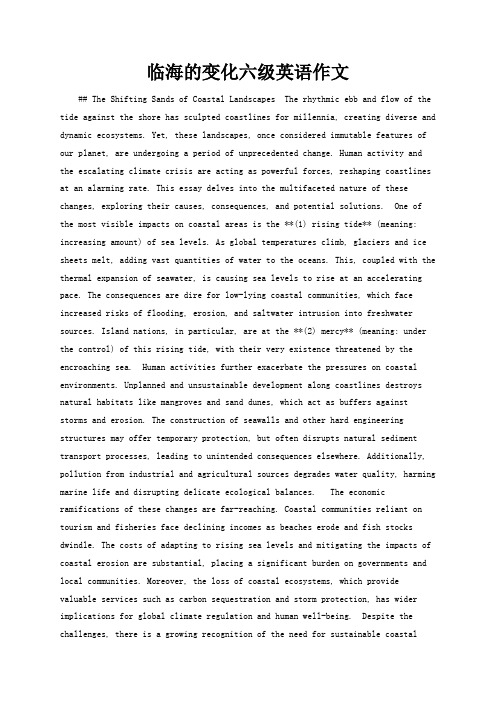
临海的变化六级英语作文## The Shifting Sands of Coastal Landscapes The rhythmic ebb and flow of the tide against the shore has sculpted coastlines for millennia, creating diverse and dynamic ecosystems. Yet, these landscapes, once considered immutable features of our planet, are undergoing a period of unprecedented change. Human activity and the escalating climate crisis are acting as powerful forces, reshaping coastlines at an alarming rate. This essay delves into the multifaceted nature of these changes, exploring their causes, consequences, and potential solutions. One of the most visible impacts on coastal areas is the **(1) rising tide** (meaning: increasing amount) of sea levels. As global temperatures climb, glaciers and ice sheets melt, adding vast quantities of water to the oceans. This, coupled with the thermal expansion of seawater, is causing sea levels to rise at an accelerating pace. The consequences are dire for low-lying coastal communities, which face increased risks of flooding, erosion, and saltwater intrusion into freshwater sources. Island nations, in particular, are at the **(2) mercy** (meaning: under the control) of this rising tide, with their very existence threatened by the encroaching sea. Human activities further exacerbate the pressures on coastal environments. Unplanned and unsustainable development along coastlines destroys natural habitats like mangroves and sand dunes, which act as buffers against storms and erosion. The construction of seawalls and other hard engineering structures may offer temporary protection, but often disrupts natural sediment transport processes, leading to unintended consequences elsewhere. Additionally, pollution from industrial and agricultural sources degrades water quality, harming marine life and disrupting delicate ecological balances. The economic ramifications of these changes are far-reaching. Coastal communities reliant on tourism and fisheries face declining incomes as beaches erode and fish stocks dwindle. The costs of adapting to rising sea levels and mitigating the impacts of coastal erosion are substantial, placing a significant burden on governments and local communities. Moreover, the loss of coastal ecosystems, which provide valuable services such as carbon sequestration and storm protection, has wider implications for global climate regulation and human well-being. Despite the challenges, there is a growing recognition of the need for sustainable coastalmanagement practices. Implementing nature-based solutions, such as restoring wetlands and planting mangroves, can help to protect coastlines while enhancing biodiversity. Embracing renewable energy sources and reducing greenhouse gas emissions are crucial to mitigating climate change and slowing the rate of sea-level rise. Additionally, investing in early warning systems and disaster preparedness measures can help communities adapt to the changing coastal environment and build resilience. Ultimately, protecting our coastlines requires a **(3) paradigm shift** (meaning: a fundamental change in approach) in how we interact with these dynamic environments. We must move away from short-term, exploitative practices and embrace a more holistic and sustainable approach that recognizes the interconnectedness of human activities and the natural world. By working together, we can ensure that future generations can continue to enjoy the beauty and bounty of our coastlines.。
Assessing the consequence of land use change on agricultural productivity in China

Assessing the consequence of land use change on agricultural productivity in ChinaHuimin Yan ⁎,Jiyuan Liu,He Qing Huang,Bo Tao,Mingkui CaoInstitute of Geographic Sciences and Natural Resources Research,Chinese Academy of Sciences,11A Datun Road,Anwai,100101,Beijing,Chinaa b s t r a c ta r t i c l e i n f o Article history:Accepted 8February 2008Available online 3January 2009Keywords:cultivated land land use changeagricultural productivity remote sensing food security ChinaChina's cultivated land has been undergoing dramatic changes along with its rapidly growing economy and population.The impacts of land use transformation on food production at the national scale,however,have been poorly understood due to the lack of detailed spatially explicit agricultural productivity information on cropland change and crop productivity.This study evaluates the effect of the cropland transformation on agricultural productivity by combining the land use data of China for the period of 1990–2000from TM images and a satellite-based NPP (net primary production)model driven with NOAA/AVHRR data.The cropland area of China has a net increase of 2.79Mha in the study period,which causes a slightly increased agricultural productivity (6.96Mt C)at the national level.Although the newly cultivated lands compensated for the loss from urban expansion,but the contribution to production is insigni ficant because of the low productivity.The decrease in crop production resulting from urban expansion is about twice of that from abandonment of arable lands to forests and grasslands.The productivity of arable lands occupied by urban expansion was 80%higher than that of the newly cultivated lands in the regions with unfavorable natural conditions.Signi ficance of cropland transformation impacts is spatially diverse with the differences in land use change intensity and land productivity across China.The increase in arable land area and yet decline in land quality may reduce the production potential and sustainability of China's agro-ecosystems.©2008Elsevier B.V.All rights reserved.1.IntroductionLand use activities,primarily for agricultural expansion and economic growth,have transformed one third to one half of our planet's land surface in the form of forest clearance,agricultural practice and urban expansion,which made profound impacts on ecosystem service,food production and environment (GLP,2005;Vitousek et al.,1997;Foley et al.,2005).Being home to one out of every five people in the world,China has been undergoing dramatic modi fications due to the unprecedented combination of economic and population growth since the early 1980s (Liu et al.,2003;Chen,1999).The loss of cultivated land due to widespread construction activities was considered to be one of the most serious problems affecting China's food security (FAO,2002).In the recent years,a good number of studies have highlighted impacts of land use change on China's potential to maintain food self-suf ficiency (Brown,1995;Rozelle and Rosegrant,1997;Heilig,1999;Feng et al.,2005).Some previous studies have analyzed and assessed China's cropland area change and its in fluences on crop yield using census data at both national and provincial levels (Yang and Li,2000).Such aggregated assessments,however,lack a detailed analysis of the variation of agricultural productivity caused by various forms of land transformation (such as urbanization and reclamation)and regional variability.Owing to the spatial heterogeneity of land use change caused by highly diverse natural and socio-economic conditions across China,accurate information on both cropland area and spatially explicit quanti fication of agricultural productivity are of critical importance for policy makers to assess the impacts of land use modi fication on national food security (Fischer et al.,1998;Verburg et al.,2000).Satellite remote sensing is a signi ficant data source for quantifying vegetation productivity,as it is able to detect actual vegetation dynamics top-down over large areas directly.It is helpful in avoiding the problems associated with “scaling up ”in process-based modeling which extra-polates data from point measurements since the detailed information on factors such as climate,soil,and especially management of agro-ecosystems are not always available at the national scale (Schimel,1995;Field et al.,1995;Reeves et al.,2005).Moreover,the measurement of photosynthetic production from remote sensing using net primary productivity (NPP)provides a common unit of production among the different crop types and hence it is a more useful approach in quantifying the impacts of land transformation (Bounoua et al.,2004).Furthermore,satellite remote sensing has been used signi ficantly for monitoring and assessing land use change at large scales in the last two decades.Liu et al.(2002,2005a),for instance,used LANDSAT TM/ETM to construct 1:100,000scale China's National Land-Use/Land-Cover Dataset (NLCD hereafter)and to detect land use information across China for the period of 1990–2000at a spatial resolution of 30m.From the result of their study,for the first time,an unambiguous and spatially explicit understanding of the degree to which the whole nation's cropland has been converted was obtained.In this study,we have quanti fied the in fluences of cropland transformation on the agricultural productivity of China during theGlobal and Planetary Change 67(2009)13–19⁎Corresponding author.Tel.:+861064889808;fax:+861064889399.E-mail address:yanhm@ (H.Yan).0921-8181/$–see front matter ©2008Elsevier B.V.All rights reserved.doi:10.1016/j.gloplacha.2008.12.012Contents lists available at ScienceDirectGlobal and Planetary Changej o u rn a l h o m e p a g e :w ww.e l s ev i e r.c o m /l o c a t e /g l o p l a c h a1990s in a spatially explicit form.By integrating land use change data from NLCD and agricultural productivity data derived from satellite remote sensing images at a resolution of8km,the impacts of cropland transformation on agricultural productivity from the impacts of climate change and management measures for the period of1991–2000were distinguished.In addition,the spatial heterogeneity of agricultural productivity variation caused by various types of cropland transformation was differentiated in detail,and its implications for food security and agro-ecosystem sustainability were examined.In the analysis,our focus was particularly onfive widely occurring land transformation types,namely: conversions of cropland to urban,cropland to forest and grassland, grassland to cropland,and forest to cropland.NPP is taken as the measure of agricultural productivity in this study and defined as the production per unit crop area,with a unit of g C m−2yr−1,while P(total productivity) refers to the summed NPP over the crop area in each geographic unit and has a unit of g C for pixels or Mt C(1012g C)for regions.2.Materials and methodsnd use change dataTo characterize the spatial and temporal patterns of land use/land cover changes across China during the1990s,the Chinese Academy of Sciences initiated a nationwide land cover/land use project in the late nd use datasets for the periods of the end of the1980s(NLCD80) and the end of the1990s(NLCD90)with a mapping scale of1:100,000 were developed.NLCD80was derived from LANDSAT TM images at the end of1980s and/or the beginning of1990s,and NLCD90was produced from remotely sensed images taken in1999/2000.According to the land-cover classification system for NLCD,the land cover was categorized into 6types:cropland,forest,grassland,water bodies,unused land,and built-up land including urban areas(Liu et al.,2002,2003,2005a).The accuracy of NLCD dataset was evaluated with about8000pictures which were taken by cameras equipped with global position system(GPS) from an extensivefield survey along transects of more than75,000km across China.The accuracy of TM images derived cropland was94.9%, while that of built-up area had the highest accuracy of96.3%.The accuracy for forest and grassland was90.1%and88.1%,respectively.A more detailed description of the data sources and methods used for developing NLCD dataset and for evaluating the accuracy of the dataset have been reported by Liu et al.(2002,2003,2005a).2.2.NPP estimationFor establishing the link between satellite data and NPP,a production efficiency model(GLO-PEM)has been developed to estimate plant PAR absorption,light use efficiency,and autotrophic respiration(Prince, 1991;Prince and Goward,1995;Cao et al.,2004).GLO-PEM was designed to run with both biological and environmental variables derived from satellite images(Prince and Goward,1995;Goetz and Prince,2000).Hence,it is a suitable tool to incorporate the effects of both climate change and crop management measures on crop growth activity into NPP estimation at the same resolution as satellite data.GLO-PEM consists of linked components that describe the processes of canopy radiation absorption,utilization,autotrophic respiration,and the regulation of these processes by environmental factors such as temperature,water vapor pressure deficit,and soil moisture(Prince and Goward,1995;Goetz et al.,2000;Cao et al.,2004).In the model,the following relationship is used to determine NPP:NPP=XtS t·N tðÞe g−Rh ið1Þwhere S t is the incident PAR in time t,N t is the fraction of incident, photosynthetically active radiation(PAR)absorbed by vegetation canopy(Fapar)at time t(as demonstrated by Prince and Goward (1995),N t can be calculated as a linear function of NDVI),εg is the light utilization efficiency of PAR absorbed by vegetation within the context of gross primary production,and R is the autotrophic respiration calculated as a function of standing aboveground biomass, air temperature,and photosynthetic rate(Prince and Goward,1995; Goetz et al.,2000).Plant photosynthesis depends on both the capacity of photosynthetic enzyme to assimilate CO2(Farquhar et al.,1980; Collatz et al.,1991)and the stomatal conductance to CO2from the atmosphere into the intercellular spaces(Harley et al.,1992;Gollan et al.,1992).The two factors are affected by environmental factors, such as air temperature,water vapor pressure deficit(VPD),soil moisture,and atmospheric CO2concentration.For this,the following relationship can be used to determineεg:e g=e g T·σð2Þwhereεg⁎is the maximum possible light utilization efficiency of PAR absorbed by vegetation determined by photosynthetic enzyme kinetics,which is a function of photosynthetic pathway,temperature and CO2/O2ratio,andσis the reduction ofεg⁎caused by environ-mental factors that control stomatal conductance and can be determined from the following relationship:σ=f TðÞfδqðÞfδθðÞð3Þwhere f(T),f(δq)and f(δθ)represent the stresses of air temperature, VPD,and soil moisture to stomatal conductance,respectively.GLO-PEM is driven with the Pathfinder AVHRR(Advanced Very High Resolution Radiometers)data at a spatial resolution of8km and temporal of10days.These were obtained from AVHRR sensors aboard the National Ocean and Atmosphere Administration(NOAA)satellites. The algorithms to calculate NDVI,Fapar,biomass,air temperature,and VPD from the AVHRR data are included in the model(Prince and Goward,1995;Goetz and Prince,1999).Detailed descriptions of the algorithms for determining the interrelationship among model variables and satellite data preprocessing were given in the studies of Prince and Goward(1995),Goetz et al.(2000),and Cao et al. (2004).In this study,we use GLO-PEM and the NOAA/NASA Pathfinder AVHRR Land(PAL)data at resolutions of8km and10 days to estimate NPP for the end of the1990s(1998and1999)and that of the1980s(1988and1989)for the purpose of matching with land use change data in temporal dimension.The validation of the results yielded from GLO-PEM over a large scale was difficult becausefield measurements are not available to evaluate remotely sensed measures.The county-level census data for production and planted area of major crops over China was obtained from the national natural resource database(www.naturalresources. ).These provided a comprehensive,independent measure of carbon uptake throughout the growing season of plants for compar-ison with the satellite-derived estimates(Malmstrom et al.,1997). Based on the statistic data,the method proposed by Lobell and Hicke (2002)was employed to estimate the cropland NPP for all counties across China.In this study remote sensing data for1996were used due to the availability of agricultural census data for that year.In order to minimize errors in regions with sparse agriculture,comparison of our result was limited to those of counties where cropland area accounts for more than30%of the total county area according to1996statistic data.For the same reason,selection of crops was limited to those types growing on more than one million ha according to1996statistic data. Hence,a total of1171counties were sampled for comparisons.The results of correlation analyses showed that the estimates of crop NPP at county level were consistent with the NPP derived from county-level statistics(correlation coefficient R=0.68).Although the crop yield from agricultural statistics are likely biased due to uncertainty in cropland area,the use of data at the county level still provided a large number of samples for evaluating remote sensing estimates of NPP.14H.Yan et al./Global and Planetary Change67(2009)13–19We also compared NPP from provincial statistics data against GLO-PEM model derived NPP over the1981–1996years for29provinces of China.According to Table1,the correlation coefficient(R,measuring interannual variation similarity)between modeled-NPP and statistic-derived NPP are high in those provinces suitable for intensive cropping due to favorable temperature and rainfall conditions.The large plain areas,the central part of China,had highest correlation coefficient(such as,R=0.86in Hunan Province and R=0.83in Jiangxi Province);while correlation coefficient was low at hilly and arid area,especially at north-west part of China(such as Gansu and Ningxia provinces)where model usually did not take into account a significant amount of NPP contributed by residue and weeds on abandoned land which eventually influenced the NDVI signals(Lobell and Hicke,2002).Moreover,differences between estimates were also attributed to potential shortcomings in the statistic based estimate.It is also clear from Table1that16provinces had R N0.6while23out of 29provinces were with R N0.35for model evaluation.The high correlation coefficients in most areas suggest that GLO-PEM was able to estimate NPP at an acceptable level.2.3.Data fusion between land use change and NPPTo combine the cropland area data from NLCD in a vector format with the NPP estimated from GLO-PEM in a raster format,the cropland data in vector format were converted to raster format with8km resolution using a data fusion technique developed by Liu et al. (2002).The converted raster format data recorded the percentage of cropland area or converted area between cropland and other land cover types in each8km×8km pixel,the area of each type in the 8×8km grid is therefore consistent with the counterpart in the original,high-resolution vector data.Such data fusion technique enables the conversion of vector data into raster data without destroying the acreage information.The impacts of land use change on agricultural productivity were further analyzed based on the spatially matched land use change and NPP data.2.4.Discrimination of agricultural productivity change caused by cropland transformationIt is known that changes in agricultural production often originate from either change in cropland area or in the crop production per unit land area.In this study,the total agricultural production is defined as the product of crop area and NPP in the form of:P=A·NPP=c·A g·NPPð4Þwhere P is the total agricultural production,NPP is the production per unit crop area(g C m−2yr−1),A is cropland area that equals to the product of the proportion of cropland in each pixel(c)and pixel area (A g=64km2).In the calculation of the changes of P,we took the value of NPP in1988and1989as the NPP level at the end of the1990s (NPP1)so as to maintain consistency with the land cover change data; similarly,the average value of NPP in1998and1999was regarded as the NPP level at the end of the1980s(NPP2).Each pixel has one NPP value derived from GLO-PEM model,while the heterogeneous land-scape induced errors were neglected,because the fraction of non-vegetated area in8km×8km pixel was very little within the land transformation types we focused in this study.To evaluate the effects of cropland area changes on agricultural production,we applied the following method proposed by Hicke et al. (2004)to determine the fractional contributions of cropland area(A) and/or NPP to P:ΔA=A2−A1ð5ÞΔNPP=NPP2−NPP1ð6ÞΔP=P2−P1=A2·NPP2−A1·NPP1=A1+ΔAðÞ·NPP1+ΔNPPðÞ−A1·NPP1=ΔNPP·A1+ΔA·NPP1+ΔA·ΔNPPð7Þwhere,A1and A2represent the cropland area at the end of the1980s and 1990s,respectively.Rearranging Eq.(7)and dividingΔP by P1result in:ΔP=P1=ΔA=A1+ΔNPP=NPP1+ΔA·ΔNPP=P1:ð8ÞThus,the fractional increase in P is the sum of the fractional increases in area A,NPP and an interaction term between A and NPP.3.Results and analysesAccording to the land use change data,China's cropland had a net increase of2.79Mha during the1990s since the newly cultivated land was 5.97Mha while3.18Mha out of the total cropland was modified for other uses especially for urban expansion,grassland and forest.The result calculated from Eq.(8)shows that China's total agricultural productivity had an increase of150.16Mt yr−1in the1990s,within which6.96Mt yr−1 was attributed to cropland transformation due to increased cropland area, while142.99Mt yr−1to an increased NPP,and a negligible fraction to the area–NPP interaction(Table2).In next two sub-sections,spatial distribution and extent of effects of land use changes on agricultural productivity are analyzed and described.3.1.Contributions of cropland area on agricultural productivity contrasting to NPP changeChina's newly cultivated cropland,as presented in Fig.1,is mainly distributed in the Northeast Region(Zone I),the Inner Mongolia Region (Zone II)and the Gan-Xin Region(Zone VIII).Croplands that were modified for other uses were found largely in Southern China.Spatial variation patterns of the total agricultural productivity and its two fractions(area-induced and NPP-induced)across China in the1990sTable1Correlation coefficients between modeled-NPP and statistic-derived NPP during1981–1996 in29provinces of China.Region Province Correlationcoefficient Percentageof croplandPercentageof plainNorth-East Inner Mongolia0.769.945.7 Liaoning0.5544.442.4Jilin0.3739.366.2Heilongjiang0.6335.364.8 North Beijing0.6229.769.8 Tianjin0.6360.7100Hebei0.6551.959.3Shanxi0.2239.013.1Shandong0.7766.765.2Henan0.6865.367.8 South-East Shanghai0.0867.0100 Jiangsu0.6268.693.2Zhejiang0.5726.735.2Fujian0.7717.98.2Guangdong0.7325.148.6 Central Anhui0.8057.474.5 Jiangxi0.8327.140.0Hubei0.7537.657.4Hunan0.8628.938.6 South-West Guangxi0.6821.930.8 Sichuan0.6625.012.5Guizhou0.5228.2 2.3Yunnan0.4118.0 2.7Tibet0.230.4 2.0 North-West Shannxi0.2334.910.3 Gansu−0.4417.510.7Qinghai−0.21 1.2 4.6Ningxia−0.0535.326.9Xinjiang0.11 3.671.215H.Yan et al./Global and Planetary Change67(2009)13–19are presented in Fig.2.Although land conversion was responsible for crop production increase in northern China and decrease in South China, its effects on the total agricultural production change were spatially scattered due to NPP-induced total agricultural production change.Most of the cropland with reduced total agricultural production were found in northwestern China,except for some areas in the Yangtze River and the Pearl River deltas(parts of Zone V and VII)(Fig.2A).By comparing Fig.2B and C,the relative contributions of cropland area and NPP to change in agriculture productivity can be determined.In the Yangtze River delta and the Pearl River delta,land conversion had a strong negative impact causing the total production to decrease significantly, while it resulted in a positive impact on the western side of the Song-Nen Plain(part of Zone I).The negative effects of land conversion in most southern regions have been cancelled out by the increase in NPP.In the Loess Plateau Region(Zone IV),however,the newly cultivated cropland could not compensate for the loss of productivity caused by a reduction in NPP.It can also be observed from Fig.2that the area-induced decrease in agricultural production occurred largely in the northern and eastern regions of China where NPP showed an increasing trend,mainly in the Huang-Huai-Hai Plain region(Zone III)and the Yangtze River drainage basin(Zone V).In the western part of the Song-Nen Plain(part of Zone1),however,NPP had a decreasing trend although a large amount of land has been newly cultivated.The shrinking of cropland occurred widely in the Huang-Huai-Hai Plain region(Zone III),the lower and middle areas of the Yangtze River drainage basin(Zone V),the Southwest Region(Zone VI)and the Southern China Region(Zone VII).The cropland area in the above mentioned four regions has decreased by4684km2,5174km2, 1963km2and587km2,respectively,which reduce the total production by2.32,3.24,1.33and0.26Mt C,correspondingly.However,the increased NPP in the four regions has not only offset the negative effects of cropland transformation but has also enhanced the crop production,resulting in improved total production by18.6%,23.7%, 15.2%and12.7%,respectively.In the Loess Plateau Region(Zone IV)and the Gan-Xin Region(Zone VIII),the land resources are generally of poor quality with heavy soil erosion,lower NPP and susceptibility to degradation.Although there were1376km2and3389km2of newly cultivated cropland respectively in the two regions,the reduced NPP still made the total production decrease slightly.In the Northeast Region(Zone I)and the Inner Mongolia Region(Zone II),both cropland area and NPP had a positive influence on productivity,but the influence from newly cultivated cropland were more significant than that from the increase in NPP in the Northeast Region(Zone I).3.2.Agricultural productivity variations caused byfive major cropland conversion formsAs stated earlier,the increase in crop production gained from land use transformation,6.96×106ton,is the net result of the positive effects of new cultivation and the negative effects of urbanization or natural vegetation restoration.The loss from the reduced cropland area has been compensated by the wide range of new cultivation in northern China.Among various forms of land transformation,it was found that there arefive types that play a dominant role.They are the conversions of cropland to urban,forest,and grassland,grassland to cropland,and forest to cropland(Liu et al.,2005b).During the ten-year period,urbanization has caused a decrease of7.84Mt in crop production,while the cropland transformed into forest and grassland has reduced crop production by2.64Mt C and1.69Mt,respectively. Conversely,the increase in crop production from newly cultivated cropland transformed from forest and grassland was8.34Mt C and 10.13Mt,respectively.Among the above mentionedfive major types of land use changes, the transformation of grassland to cropland exhibited the most significant positive effects on food production,and it was followed by the transformation of forest to cropland.Meanwhile,urban sprawl appears to be the most influencing human activity on crop production decrease,whose negative impact on crop production was almost double the sum of the loss of crop production resulting from the transformation of cropland to forest and grassland(Table3).Furthermore,most of the area losses in cropland due to urbanization were of good quality and with high productivity,while the newly developed cropland was generally from area of poor land quality and with low productivity. This can be seen through comparing the changes in cropland area and the resulting productivity with the changes in urban sprawl and the transformation of grassland to cropland(Fig.3).Of the resulting productivity change by cropland transformation,there was22.8%lost to urbanization,while29.5%was gained from the newly cultivated land from grassland.However,all the cropland lost to urbanizationaccountedFig.1.Cropland transformation in China during the1990s.A is spatial distribution of cropland transformation,where the pixel value is the proportion of transformed cropland area within each8km×8km pixel,positive value means cropland area increased and negative value means cropland area decreased.B presents the sum of cropland area of newly cultivated and modified for other uses within the9agricultural regions.The corresponding agricultural regions'names are presented in Table2.Table2Effects of cropland area change and NPP on total production(Unit:Mt).Zone Agricultural region Croplandarea NPP NPP–areainteractionI Northeast Region11.789.780.91II Inner Mongolia and the Great Wall Region 2.057.200.28III Huang-Huai-Hai Region−2.3132.09−0.38IV Loess Plateau Region0.12−0.45−0.003V Middle and lower reaches of the YangtzeRiver drainage basin−3.2357.35−0.54VI Southwest Region−0.2521.33−0.03VII South China Region−1.33 1.59−0.09VIII Gan-Xin Region0.08−0.220.08IX Tibet Region0.08−0.002−0.003Whole China 6.96142.990.2116H.Yan et al./Global and Planetary Change67(2009)13–19for only 16.6%of the total transformed area,while the total cropland transformed from newly cultivated grassland was 38.8%of the total transformed cropland area.That is to say,an occupation of 1ha cropland by urban requires cultivating 1.8ha grassland to compensate the resulted production loss.Land use change is highly diverse across the whole country,so are its impacts on agricultural productivity due to natural and socio-economic differences in different geographical regions (Fig.4).In the Northeast Region (Zone I),the agricultural production resulting from cropland transformation was as high as 11.8Mt C,to which the most contributed land use change types were grassland and forestland cultivation,including 5.8Mt C resulted from an increase in forest area and 5.2Mt C from an increase in grassland cultivation.The Inner-Mongolia and the Great Wall Region (Zone II)is a typical farm and pasture interleaving area,where intensive land conversions ofcropland to grassland and grassland to cropland occurred simulta-neously.In this region,the agricultural productivity has gained a net increase of 2.1Mt C,to which the most signi ficant contributions were 2.9Mt C gain from grassland cultivation and 0.9Mt C loss from converting cropland into grassland.Cropland transformation has caused a loss of 2.3Mt C in agricultural productivity in the Huang-Huai-Hai Plain Region (Zone III),within which the rapid urbanization has caused a loss of 2.63Mt,mainly happened in the Beijing –Tianjin –Tangshan economic area,while the new cultivation in the region only has had limited potential to compensate the loss.In the Loess Plateau Region (Zone IV),the large area of newly cultivatedcroplandFig.2.Effects of land use change and NPP on total productivity in China during the 1990s (Unit:g C),A:total productivity change,B:land use transformation induced change,and C:NPP-inducedchange.Fig.3.Proportions of 5major forms of cropland transformation and the induced agricultural productivity change in China during the 1990s.Table 3Impacts of the different forms of land use change on the total agricultural productivity of China during the nd use changes Area induced productivity variation (%)Cropland to forest land −7.7Cropland to grassland −4.9Cropland to built-up land −22.8Forest land to cropland 24.3Grassland to cropland 29.5Others11.0Positive values indicate land use changes make agricultural productivity increase.Negative values indicate land use changes make agricultural productivity decrease.17H.Yan et al./Global and Planetary Change 67(2009)13–19。
Land-use and land-cover change

Land-use and land-cover changeErle Ellis;Robert Pontius1 Introduction1.1 Land-change science2 Causes and Consequences2.1 Biodiversity loss2.2 Climate Change2.3 Pollution2.4 Other impacts3 Methods3.1 Remote sensing3.2 Geospatial analysis3.3 Driving forces3.4 Modeling4 Sustainable land management5 Further ReadingIntroductionLand-use and land-cover change (LULCC); also known as land change) is a general term for the human modification of Earth's terrestrial surface. Though humans have been modifying land to obtain food and other essentials for thousands of years, current rates, extents and intensities of LULCC are far greater than ever in history, driving unprecedented changes in ecosystems and environmental processes at local, regional and global scales. These changes encompass the greatest environmental concerns of human populations today, including climate change, biodiversity loss and the pollution of water, soils and air. Monitoring and mediating the negative consequences of LULCC while sustaining the production of essential resources has therefore become a major priority of researchers and policymakers around the world.Land-change scienceSatellite image of deforestation in the Amazon region, taken from the Brazilian state of Para on July 15, 1986. The dark areas are forest, the white is deforested areas, and the gray is re-growth. The pattern of deforestation spreading along roads is obvious in the lower half of the image. Scattered larger clearings can be seen near the center of the image. (Source: NASA)Land cover refers to the physical and biological cover over the surface of land, including water, vegetation, bare soil, and/or artificial structures. Land use is a more complicated term. Natural scientists define land use in terms of syndromes of human activities such as agriculture, forestry and building construction that alter land surface processes including biogeochemistry, hydrology and biodiversity. Social scientists and land managers define land use more broadly to include the social and economic purposes and contexts for and within which lands are managed (or left unmanaged), such as subsistence versus commercial agriculture, rented vs. owned, or private vs. public land. While land cover may be observed directly in the field or by remote sensing, observations of land use and its changes generally require the integration of natural and social scientific methods (expert knowledge, interviews with land managers) to determine which human activities are occurring in different parts of the landscape, even when land cover appears to be the same. For example, areas covered by woody vegetation may represent an undisturbed natural shrubland, a forest preserve recovering from a fire (use = conservation), regrowth following tree harvest (forestry), a plantation of immature rubber trees (plantation agriculture), swidden agriculture plots that are in between periods of clearing for annual crop production, or an irrigated tea plantation. As a result, scientific investigation of the causes and consequences of LULCC requires an interdisciplinary approach integrating both natural and social scientific methods, which has emerged as the new discipline of land-change science.Causes and ConsequencesChanges in land use and land cover date to prehistory and are the direct and indirect consequence of human actions to secure essential resources. This may first have occurred with the burning of areas to enhance the availability of wild game and accelerated dramatically with the birth of agriculture, resulting in the extensive clearing (deforestation) and manag ement of Earth’s terrestrial surface that continues today. More recently, industrialization has encouraged the concentration of human populations within urban areas (urbanization) and the depopulation of rural areas, accompanied by the intensification of agriculture in the most productive lands and the abandonment of marginal lands. All of these causes and their consequences are observable simultaneously around the world today.Biodiversity lossBiodiversity is often reduced dramatically by LULCC. When land is transformed from a primary forest to a farm, the loss of forest species within deforested areas is immediate and complete. Even when unaccompanied by apparent changes in land cover, similar effects are observed whenever relatively undisturbed lands are transformed to more intensive uses, including livestock grazing, selective tree harvest and even fire prevention. The habitat suitability of forests and other ecosystems surrounding those under intensive use are also impacted by the fragmenting of existing habitat into smaller pieces (habitat fragmentation), which exposes forest edges to external influences and decreases core habitat area. Smaller habitat areas generally support fewer species (island biogeography), and for species requiring undisturbed core habitat, fragmentation can cause local and even general extinction. Research also demonstrates that species invasions bynon-native plants, animals and diseases may occur more readily in areas exposed by LULCC, especially in proximity to human settlements.Climate ChangeLULCC plays a major role in climate change at global, regional and local scales. At global scale, LULCC is responsible for releasing greenhouse gases to the atmosphere, thereby driving global warming. LULCC can increase the release of carbon dioxide to the atmosphere by disturbance of terrestrial soils and vegetation, and the major driver of this change is deforestation, especially when followed by agriculture, which causesthe further release of soil carbon in response to disturbance by tillage. Changes in land use and land cover are also behind major changes in terrestrial emissions of other greenhouse gases, especially methane (altered surface hydrology: wetland drainage and rice paddies; cattle grazing), and nitrous oxide (agriculture: input of inorganic nitrogen fertilizers; irrigation; cultivation of nitrogen fixing plants; biomass combustion).Though LULCC certainly plays a critical role in greenhouse gas emissions, the complexity and dynamic interplay of land use processes favoring net accumulation versus net release of carbon dioxide and other greenhouse gases makes it a poorly constrained component of our global budgets for these gases; an active area of current research. A further source of uncertainty in estimating the climate changes caused by LULCC is the release of sulfur dioxide and particulates by biomass combustion associated with agriculture, land clearing and human settlements. These emissions are believed to cause regional and global cooling by the reflection of sunlight from particulates and aerosols, and by their effects on cloud cover.Land cover changes that alter the reflection of sunlight from land surfaces (albedo) are another major driver of global climate change. The precise contribution of this effect to global climate change remains a controversial but growing concern. The impact of albedo changes on regional and local climates is also an active area of research, especially changes in climate in response to changes in cover by dense vegetation and built structures. These changes alter surface heat balance not only by changing surface albedo, but also by altering evaporative heat transfer caused by evapotranspiration from vegetation (highest in closed canopy forest), and by changes in surface roughness, which alter heat transfer between the relatively stagnant layer of air at Earth’s surface (the boundary layer) and the troposphere. An example of this is the warmer temperatures observed within urban areas versus rural areas, known as the urban heat island effect.PollutionChanges in land use and land cover are important drivers of water, soil and air pollution. Perhaps the oldest of these is land clearing for agriculture and the harvest of trees and other biomass. Vegetation removal leaves soils vulnerable to massive increases in soil erosion by wind and water, especially on steep terrain, and when accompanied by fire, also releases pollutants to the atmosphere. This not only degrades soil fertility over time, reducing the suitability of land for futureagricultural use, but also releases huge quantities of phosphorus, nitrogen, and sediments to streams and other aquatic ecosystems, causing a variety of negative impacts (increased sedimentation, turbidity, eutrophication and coastal hypoxia). Mining can produce even greater impacts, including pollution by toxic metals exposed in the process. Modern agricultural practices, which include intensive inputs of nitrogen and phosphorus fertilizers and the concentration of livestock and their manures within small areas, have substantially increased the pollution of surface water by runoff and erosion and the pollution of groundwater by leaching of excess nitrogen (as nitrate). Other agricultural chemicals, including herbicides and pesticides are also released to ground and surface waters by agriculture, and in some cases remain as contaminants in the soil. The burning of vegetation biomass to clear agricultural fields (crop residues, weeds) remains a potent contributor to regional air pollution wherever it occurs, and has now been banned in many areas.Other impactsOther environmental impacts of LULCC include the destruction of stratospheric ozone by nitrous oxide release from agricultural land and altered regional and local hydrology (dam construction, wetland drainage, irrigation projects, increased impervious surfaces in urban areas). Perhaps the most important issue for most of Earth’s human population is the long-term threat to future production of food and other essentials by the transformation of productive land to nonproductive uses, such as the conversion of agricultural land to residential use and the degradation of rangeland by overgrazing.MethodsThe methods of land-change science include remote sensing and geospatial analysis and modeling, together with the interdisciplinary assortment of natural and social scientific methods needed to investigate the causes and consequences of LULCC across a range of spatial and temporal scales.Remote sensingRemote sensing is an essential tool of land-change science because it facilitates observations across larger extents of Earth’s surface than is possible by ground-based observations. This is accomplished by use of cameras, multi-spectral scanners, RADAR and LiDAR sensors mounted on air- and space-borne platforms, yielding aerial photographs, satelliteimagery, RADAR and LiDAR datasets. Data available from remote sensing vary from the very high-resolution datasets produced irregularly over extents no larger than a single state or province (by aerial photography, imaging, LiDAR, and by high resolution satellite sensors such as IKONOS and Quickbird), to regional datasets produced at regular intervals from satellites (e.g., Landsat, SPOT), to the lower-resolution (> 250 m) datasets now produced across the entire Earth on a daily basis (e.g., MODIS).Geospatial analysisMaps and measurements of land cover can be derived directly from remotely sensed data by a variety of analytical procedures, including statistical methods and human interpretation. Maps of land use and land cover (LULC) are produced from remotely sensed data by inferring land use from land cover (e.g., urban = barren, agriculture = herbaceous vegetation). Conventional LULC maps are categorical, dividing land into categories of land use and land cover (thematic mapping; land classification), while recent techniques allow the mapping of LULC or other properties of land as continuous variables or as fractional cover of the land by different LULC categories, such as tree canopy, herbaceous vegetation, and barren (continuous fields mapping). Both types of LULC datasets may be compared between time periods using geographic information systems (GIS) to map and measure LULCC at local, regional, and global scales.Driving forcesAssessing the driving forces behind LULCC is necessary if past patterns are to be explained and used in forecasting future patterns. Driving forces on LULCC can include almost any factor that influences human activity, including local culture (food preference, etc.), economics (demand for specific products, financial incentives), environmental conditions (soil quality, terrain, moisture availability), land policy & development programs (agricultural programs, road building, zoning), and feedbacks between these factors, including past human activity on the land (land degradation, irrigation and roads). Investigation of these drivers of LULCC requires a full range of methods from the natural and social sciences, including climatology, soil science, ecology, environmental science, hydrology, geography, information systems, computer science, anthropology, sociology, and policy science.ModelingSpatially-explicit models of the social and environmental causes and consequences of LULCC is made possible by GIS and other computer-based techniques which can define and test relationships between environmental and social variables using a combination of existing data (census data, soil maps, LULC maps), observations on the ground (ecological measurements, household surveys and interviews with land managers) and data from remote sensing. These spatial models of LULCC drivers and their impacts can be used to establish cause and effect in LULCC observed in the past and are also extremely useful tools for land mangers and policymakers, offering forecasts of future land use changes and their effects. Models of LULCC dependence on political, economic, environmental and other changes can then be used to explore the impacts of policy decisions and other factors using scenario analysis and other computer modeling techniques, guiding policymakers and land managers toward sustainable land management decisions.Sustainable land managementSustainable land management is a central challenge in the sustainable management of earth systems and resources. On the one hand, land management must ensure a growing supply of food and other resources to human populations, which are expected to grow for decades to come. On the other hand, management of land to procure these resources is linked with potentially negative consequences in the form of climate change, biodiversity loss and pollution. Moreover, local alteration of land use and land cover can have global consequences, requiring local and regional solutions to global problems and the cooperation of the w orld’s policymakers, land managers, and other stakeholders in land management at local, regional and global scales.At the global scale, the Kyoto Protocol offers an example of international efforts to reduce climate change caused by greenhouse gas emissions from land. It offers incentives, such as a trade in carbon credits, that encourage land use practices which promote the storage of carbon on land, including the planting of trees, perennial crops, the return of crop residues to soils, and no-till agriculture,. The Protocol also promotes practices that reduce emissions of methane and nitrous oxide from agricultural land.Regional efforts to modify land use practices to reduce nonpoint pollution of air and water are already in place in many areas of the world, includingthe USA (Chesapeake Bay Program) and China (Tai Lake Program). In developed areas, including cities and suburbs, there are nowwell-developed land use policies and practices to protect streams and other aquatic ecosystems from the excessive runoff and flooding produced by the construction of impervious surfaces (buildings and roads).Management of land in support of biodiversity covers a wide range of policies and practices. The most basic of these is to set-aside existing biodiverse habitats as conservation reserves from which humans are excluded. Another is the establishment of preserves and parks in which local human populations and tourists participate in the less harmful economic use and preservation of biodiverse lands. More recently, efforts are being made to restore biodiverse habitats on lands stripped of their original habitat, and to manage existing agricultural and urban landscapes to enhance their suitability as habitat by practices including the planting of native plants and the restoration of habitat patches within intensively managed landscapes. Another new land use practice is the establishment of corridors of habitat between existing patches of habitat distributed across landscapes, creating larger effective habitats by connecting smaller patches together and enhancing species migrations. This will be an especially important practice in response to future changes in climate that will cause the habitat ranges of many species to migrate, mostly northward, requiring species migration through managed areas.Protection of productive agricultural land has become a major priority in many regions of the world. Land degradation by overgrazing and intensive agriculture on marginal lands is a major driver of land loss;a number of national and international programs have responded with land reforms and incentive programs to avoid this outcome. In rapidly industrializing nations with dense populations such as China, and in the past, Korea, Japan and Western European nations, demand for land for industry and residential use is driving the transformation of some of the most productive agricultural land in the world out of production. Policy efforts to avoid this loss of production are also in place, but their effectiveness in the face of economic demand is often limited. Another threat is the wide adoption of automobile transportation in some developed nations, which has transformed large areas of agricultural land to relatively low density residential uses around cities and along highways (urban sprawl). “Smart growth” and other programs have been developed in these areas to encourage more efficient and desirable land use and to protect agricultural land.The examples above demonstrate the variety of solutions to environmental harm by LULCC that are in progress. The effectiveness of these and otherregional and national efforts to reduce the negative impacts of LULCC remain to be seen. The need for greater efforts and new methods to monitor and mediate the negative consequences of LULCC remains acute, if we are to sustain current and future human populations under desirable conditions.Further Reading∙DeFries, R. S., G. P. Asner, and R. A. Houghton, editors. 2004. Ecosystems and Land Use Change. American Geophysical Union, Washington, DC.∙Foley, J. A., R. DeFries, G. P. Asner, C. Barford, G. Bonan, S. R. Carpenter, F. S. Chapin, M. T. Coe, G. C. Daily, H. K. Gibbs, J. H. Helkowski, T. Holloway, E. A. Howard, C. J.Kucharik, C. Monfreda, J. A. Patz, I. C. Prentice, N. Ramankutty, and P. K. Snyder. 2005.Global consequences of land use. Science 309:570-574.∙Global Land Project. 2005. Science Plan and Implementation Strategy. IGBP Report No.53/IHDP Report No. 19, IGBP Secretariat, Stockholm.∙Meyer, W. B., and B. L. Turner. 1994. Changes in Land Use and Land Cover: A Global Perspective. Cambridge University Press, Cambridge England; New York, NY, USA.∙Ruddiman, W. F. 2003. The anthropogenic greenhouse era began thousands of years ago.Climatic Change 61:261-293.∙Turner II, B. L., W. C. Clark, R. W. Kates, J. F. Richards, J. T. Mathews, and W. B. Meyer.1990. The Earth as Transformed by Human Action: Global and Regional Changes in theBiosphere Over the Past 300 Years. Cambridge University Press with Clark University,Cambridge; New York.CitationErle Ellis (Lead Author);Robert Pontius (Topic Editor) "Land-use and land-cover change". In: Encyclopedia of Earth. Eds. Cutler J. Cleveland (Washington, D.C.: Environmental Information Coalition, National Council for Science and the Environment). [First published in the Encyclopedia of Earth April 18, 2010; Last revised Date April 18, 2010; Retrieved March 7, 2011The AuthorDr. Erle Ellis is Associate Professor of Geography and Environmental Systems at the University of Maryland, Baltimore County,where he teaches Environmental Science, Landscape Ecology and Biogeochemistry. His research focuses on ecological processes in anthropogenic landscapes at local, regional and global scales, and their transformation by population growth and industrially-based technologies. He has studied long-term changes in nitrogen balance in village ecosystems of China's Tai Lake Re ... (Full Bio)。
Changes in land uses and management in two Nature Reserves in Spain
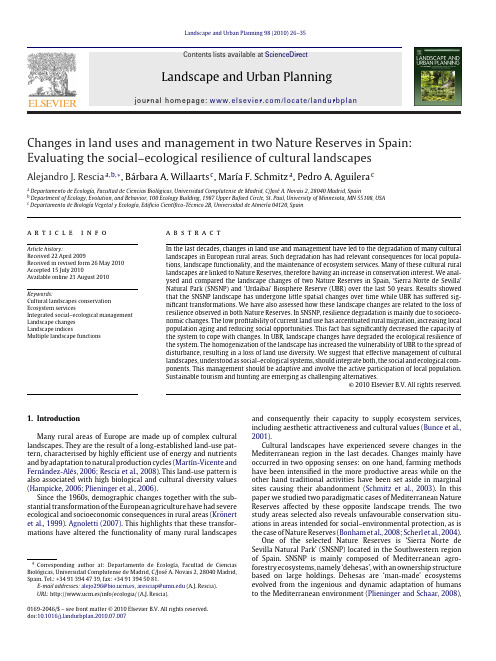
Landscape and Urban Planning 98 (2010) 26–35Contents lists available at ScienceDirectLandscape and UrbanPlanningj o u r n a l h o m e p a g e :w w w.e l s e v i e r.c o m /l o c a t e /l a n d u r b p l anChanges in land uses and management in two Nature Reserves in Spain:Evaluating the social–ecological resilience of cultural landscapesAlejandro J.Rescia a ,b ,∗,Bárbara A.Willaarts c ,María F.Schmitz a ,Pedro A.Aguilera caDepartamento de Ecología,Facultad de Ciencias Biológicas,Universidad Complutense de Madrid,C/JoséA.Novais 2,28040Madrid,SpainbDepartment of Ecology,Evolution,and Behavior,100Ecology Building,1987Upper Buford Circle,St.Paul,University of Minnesota,MN 55108,USA cDepartamento de Biología Vegetal y Ecología,Edificio Científico-Técnico 2B,Universidad de Almería 04120,Spaina r t i c l e i n f o Article history:Received 22April 2009Received in revised form 26May 2010Accepted 15July 2010Available online 21 August 2010Keywords:Cultural landscapes conservation Ecosystem servicesIntegrated social–ecological management Landscape changes Landscape indicesMultiple landscape functionsa b s t r a c tIn the last decades,changes in land use and management have led to the degradation of many cultural landscapes in European rural areas.Such degradation has had relevant consequences for local popula-tions,landscape functionality,and the maintenance of ecosystem services.Many of these cultural rural landscapes are linked to Nature Reserves,therefore having an increase in conservation interest.We anal-ysed and compared the landscape changes of two Nature Reserves in Spain,‘Sierra Norte de Sevilla’Natural Park (SNSNP)and ‘Urdaibai’Biosphere Reserve (UBR)over the last 50years.Results showed that the SNSNP landscape has undergone little spatial changes over time while UBR has suffered sig-nificant transformations.We have also assessed how these landscape changes are related to the loss of resilience observed in both Nature Reserves.In SNSNP,resilience degradation is mainly due to socioeco-nomic changes.The low profitability of current land use has accentuated rural migration,increasing local population aging and reducing social opportunities.This fact has significantly decreased the capacity of the system to cope with changes.In UBR,landscape changes have degraded the ecological resilience of the system.The homogenization of the landscape has increased the vulnerability of UBR to the spread of disturbance,resulting in a loss of land use diversity.We suggest that effective management of cultural landscapes,understood as social–ecological systems,should integrate both,the social and ecological com-ponents.This management should be adaptive and involve the active participation of local population.Sustainable tourism and hunting are emerging as challenging alternatives.© 2010 Elsevier B.V. All rights reserved.1.IntroductionMany rural areas of Europe are made up of complex cultural landscapes.They are the result of a long-established land-use pat-tern,characterised by highly efficient use of energy and nutrients and by adaptation to natural production cycles (Martín-Vicente and Fernández-Alés,2006;Rescia et al.,2008).This land-use pattern is also associated with high biological and cultural diversity values (Hampicke,2006;Plieninger et al.,2006).Since the 1960s,demographic changes together with the sub-stantial transformation of the European agriculture have had severe ecological and socioeconomic consequences in rural areas (Krönert et al.,1999).Agnoletti (2007).This highlights that these transfor-mations have altered the functionality of many rural landscapes∗Corresponding author at:Departamento de Ecología,Facultad de Ciencias Biológicas,Universidad Complutense de Madrid,C/JoséA.Novais 2,28040Madrid,Spain.Tel.:+34913944739,fax:+34913945081.E-mail addresses:alejo296@bio.ucm.es ,aresciap@ (A.J.Rescia).URL:http://www.ucm.es/info/ecologia/(A.J.Rescia).and consequently their capacity to supply ecosystem services,including aesthetic attractiveness and cultural values (Bunce et al.,2001).Cultural landscapes have experienced severe changes in the Mediterranean region in the last decades.Changes mainly have occurred in two opposing senses:on one hand,farming methods have been intensified in the more productive areas while on the other hand traditional activities have been set aside in marginal sites causing their abandonment (Schmitz et al.,2003).In this paper we studied two paradigmatic cases of Mediterranean Nature Reserves affected by these opposite landscape trends.The two study areas selected also reveals unfavourable conservation situ-ations in areas intended for social–environmental protection,as is the case of Nature Reserves (Bonham et al.,2008;Scherl et al.,2004).One of the selected Nature Reserves is ‘Sierra Norte de Sevilla Natural Park’(SNSNP)located in the Southwestern region of Spain.SNSNP is mainly composed of Mediterranean agro-forestry ecosystems,namely ‘dehesas’,with an ownership structure based on large holdings.Dehesas are ‘man-made’ecosystems evolved from the ingenious and dynamic adaptation of humans to the Mediterranean environment (Plieninger and Schaar,2008),0169-2046/$–see front matter © 2010 Elsevier B.V. All rights reserved.doi:10.1016/ndurbplan.2010.07.007A.J.Rescia et al./Landscape and Urban Planning98 (2010) 26–3527Fig.1.Location of the Nature Reserves studied corresponding to‘Sierra Norte de Sevilla’Natural Park—SNSNP(A)and to‘Urdaibai’Biosphere Reserve—UBR(B).SNSNP presents a landscape characterised by dehesas.UBR presents a landscape characterised by abundant forestry plantations.representing perfect examples of how the rational use of natu-ral resources ensures the long-term ecological sustainability of Mediterranean ecosystems and provides a wide range of ecosystem services.The other Nature Reserve is‘Urdaibai Biosphere Reserve’(UBR) in the Northern region of Spain.UBR is a typical agrarian mosaic landscape based on the‘caserío’(farm).This type of land use consti-tutes a farming system based upon smallholdings and multiple land uses.Over time,Such systems have maintained a high level of land-scape diversity together with a viable and ecologically sustainable production system(Atauri et al.,2004;Rescia et al.,1994).Both,SNSNP and UBR Nature Reserves,are clear examples of social–ecological systems(SES).They are complex adaptive sys-tems characterised by cross-scale interactions and feedback loops between the ecological and socioeconomic components(Berkes and Folke,2000;Walker et al.,2006).The persistence of these SES relies on their ecological and socioeconomic resilience,that is, on their capacity for transformation and adaptation in the face of change(Berkes and Folke,2000;Holling,2001;Walker et al.,2006).The social–ecological resilience of SNSNP and UBR is threatened by rapid and drastic economic and environmental changes occur-ring over the last decades.These changes are affecting the resilience28 A.J.Rescia et al./Landscape and Urban Planning98 (2010) 26–35Fig.2.Geomorphic Units map of ‘Sierra Norte de Sevilla’Natural Park and distribution of the area occupied by the five Geomorphic Units (GU)identified.A mountainous relief predominates (56.5%)and transforming this area in a marginal zone.of both systems beyond the thresholds of their adaptive capacity.This situation increases their degree of vulnerability to the adverse effects of changes (Adger,2000,2006).There are relatively few studies of SES associated with Nature Reserves that have examined landscape changes and their social–environmental consequences (Caillon and Degeorges,2007;Wittemyer et al.,2008).Therefore,in our study we analyse the vul-nerability of SES presenting different signs of resilience degradation together with a loss of ecosystem services.To this end,our first objective is to evaluate the changes in land use and land cover over the last 50years in SNSNP and UBR.The second objective of the paper involves comparing the resilience of the SES of both Nature parison of these systems,which present certain sim-ilarities in their land use but show different tendencies of changes,are considered to constitute a resilience surrogate (Carpenter et al.,2005).This type of comparative study is crucial because the resilience of an SES cannot always be directly observed and different situ-ations require different approaches and solutions.Based on our results,we attempt to establish criteria for the integrated man-agement of both SES;highlighting the role of public participation in the design and implementation of a successful management plan.The management should seek to ensure the socioeconomic development of both SES while integrating the maintenance of key ecosystem services in its objectives.2.Materials and methods 2.1.Study areas2.1.1.Sierra Norte de Sevilla Natural Park (SNSNP)SNSNP was set up as a Nature Reserve in 1989and has an area of approximately 170,000ha.It occupies the central part of ‘Sierra Morena’mountains in the Andalusia Autonomous Region.The land-scape in SNSNP is conditioned by a long history of forestry and livestock farming.The mountain areas have traditionally been used for forestry and are covered by autochthonous Holm oak forests (Quercus ilex L.),frequently mixed with formations of cork oak (Quercus suber L .).Olive plantations (Olea europaea L.)are also com-mon in the mountain areas.On the peneplains,the landscape ischaracterised by formations of dehesa containing most of the live-stock farming,together with small areas of cereal crops (Fig.1).The beginning of the industrialisation process occurred between the 1940s and the 1960s in Spain.This had important effects on rural areas.In SNSNP,it has had very negative demographic repercussions and a serious impact on the local economy.These socioeconomic changes led to a new form of dehesa management,modifying its original production capacity and land-use system.The very few initiatives promoted by the Administration aimed at recovering the economic profitability of these systems have not succeeded and have only served to exacerbate the socioeconomic degradation (CMA,2004;INE,2006).2.1.2.Urdaibai Biosphere Reserve (UBR)UBR was also established as a Nature Reserve in 1989and it encompasses an area of approximately 23,000ha in the Basque Country Region.It occupies a river basin characterised by having a very rugged relief,with narrow valleys and a flat valley bottom draining into an estuary.The vegetation of the mature ecosystems has been relegated to relictic enclaves as a result of human activity.There are noteworthy formations of marshland vegetation,reed beds,forests of Quercus ilex L.and many woodlands of decidu-ous species (Fagus sylvatica L.,Quercus robur L.,Quercus pyrenaica Willd.)following the layout of streams or of tectonic or geomor-phological features (Fig.1).The Socioeconomic changes over the last few decades also had relevant effects in UBR.An important part of the local popula-tion migrated to the urban environments,changing the traditional production systems.This process involved the plantations of fast-growth forest species,which radically changed the spatial structure of the landscape in UBR.Such changes gave rise to a marked reduc-tion of the landscape diversity (nd uses and vegetation)with severe ecological consequences (Rescia et al.,1994)nd classification of SNSNPConsidering the large area SNSNP comprises and its environ-mental variability,prior to our landscape analysis we performed a land classification to subdivide the study area in different homo-geneous Geomorphic Units (GU)(Appendix A ).The resulting fiveA.J.Rescia et al./Landscape and Urban Planning98 (2010) 26–3529Table1Landscape indices used to evaluate changes in the spatial pattern in‘Sierra Norte de Sevilla’Natural Park and‘Urdaibai’Biosphere Reserve.They are based on theory of communication(Shannon and Weaver,1989.See the text for formulae).Landscape Indices Definition Spatial interpretationN Total number of boundaries between land uses(N≥1)Indicates the grain size of land uses and suggests the degree of fragmentation of the landscapen Richness.Total number of types of boundariesbetween land uses(1≤n≤N)Indicates the variety(different boundaries)of land uses of the landscapeE(b)Evenness.Relative abundance of the differentboundaries between land uses(0≤E(b)≤1)Indicates the spatial configuration of landscape.The maximum E(b)indicates a landscape with an even distribution of boundaries between uses(inversely to dominance)H(b)Diversity of boundaries.It takes into account nand E(b).Strictly speaking,measures the degreeof uncertainty offinding at random determinedboundary between land-uses(0≤H(b)≤log2n)Indicates the variety and distribution of the boundaries between land uses.It is a measure of information(organization and history) contained in the landscapeH(l)Landscape heterogeneity.It takes into account,N,and H(b).When n=N,H(l)=1(0≤H(l)≤1)Indicates the spatial variability or structural heterogeneity of the landscape but does not express landscape functionality(Two equal H(l) landscapes do not imply two equal spatial complexity landscapes)C(s)Spatial complexity.Measurement of thecombination of spatial neighbours found in aspace with a given N,H(b)and H(l)Indicates landscape functionality(possibility of flows of matter,energy and species).It enables the number of interactions between land uses and the degree of landscape connectivity to be inferred(the highest H(b)implies the greatest C(s))GUs were:(1)Calcareous mountains;(2)Metamorphic mountains;(3)Metamorphic peneplains;(4)Peneplains with granite outcrops; and(5)Metamorphic inland mountain valleys(Fig.2).2.3.Spatial analysis of SNSNP and UBR using landscape indicesLandscape indices(or metrics)can be useful for quantifying spa-tial patterns,ecological processes and for assessing the integrity of the landscape under study.Their usefulness for landscape analy-sis and environmental planning has been examined and confirmed in several studies(O’Neill et al.,1988;Peng et al.,2009).The use of these metrics for analyzing spatial patterns has become quite popular(Botequilha Leitão et al.,2006).However,with the abun-dance of indices available,it is imperative to address several issues when using landscape metrics in assessment efforts.These issues are,for example,the study objectives,the type of spatial configura-tion of the landscape,the effects of scale on the indices employed and the appropriate selection of indices to be applied in order to avoid redundancy in the information they provide.The quality of information contained in the indices will depend upon considera-tion of these aspects(Gustafson,1998;Li and Wu,2004).Keeping in mind these requirements,in our paper we selected suitable land-scape indices to evaluate changes in the spatial pattern of both study areas(Table1).2.4.Data acquisitionWe took randomly distributed circles with a radius of1km(83 in SNSNP and24in UBR,in proportion with the size of the area of each site).The land uses in each circle for each year(1956and 2003in SNSNP;1946and2001in UBR)were identified from aerial photographs(Table2).In each circle,the frequency and type of existing boundaries between each pair of adjacent land uses were recorded.The term‘boundary’refers to two adjoining patches of different land uses presenting a common or shared perimeter.In the1km-radius circles,for the different years in each study area,we calculated different landscape indices(see Table1).Using the Shannon-Wiener formula(Shannon and Weaver,1949),we cal-culated the diversity of the boundaries,H(b):H(b)=−[p i/j log2(p i/j)]where p i/j is the proportion of the boundary between adjacent patches i and j considering the total number of boundaries(N) present in the series of circles sampled for each year(p i/j=1). Thus,the elements of the Shannon–Wiener formula were not patches,but rather boundaries,considered as a more informative parameter which includes patches and their neighbouring patches as a stronger measure of spatial diversity.Subsequently,the richness of boundaries(n)between differ-ent patches and evenness(E(b))was also calculated(Pielou,1975) according to the formula:E(b)=H(b)log2nThe H(b)index expresses boundary diversity in a space and the landscape heterogeneity that these boundaries generate,H(l),isTable2Land use units identified in‘Sierra Norte de Sevilla’Natural Park and‘Urdaibai’Biosphere Reserve.SNSNP UBRRiparian forests Riparian forestsHolm oak forests Holm oak forestsForest plantations Mixed forestsShrublands Old Pine plantationsDehesa Young Pine plantationsDehesa with shrublands Very young Pine plantations Meadows Clear-cutsWoody crops(olives)ShrublandsHerbaceous crops Pastures and TreesWithout vegetation(urban,dams)PasturesMeadows and HedgerowsMarshCropsWithout vegetation(urban,industrial nuclei,quarry)30 A.J.Rescia et al./Landscape and Urban Planning98 (2010) 26–35Table3Temporal evolution and variation in the landscape indices used to analyse the spatial pattern,at local scale,of‘Sierra Norte de Sevilla’Natural Park landscape.N:total number of boundaries;n:number of boundary types;E(b):boundary evenness;H(l):landscape heterogeneity;C(s):spatial complexity.The unit of E(b),H(l)and C(s)is bits.GeoUnits Landscape indices50years ago Present time Variation(%)GU1N560513−8.39 n30300.00 E(b)0.880.880.00 H(l)0.470.48+2.13 C(s) 2.05 2.07+0.98GU2N958903−5.74 n3337+12.12 E(b)0.790.790.00 H(l)0.400.42+5.00 C(s) 1.62 1.73+6.79GU3N485545+12.37 n2836+28.57 E(b)0.840.64−23.81 H(l)0.450.37−17.78 C(s) 1.82 1.22−32.97GU4N182212+16.48 n2331+34.78 E(b)0.820.81−1.22 H(l)0.500.52+4.00 C(s) 1.85 2.10+13.51GU5N402424+5.47 n2326+13.04 E(b)0.810.810.00 H(l)0.430.44+2.33 C(s) 1.57 1.68+7.01defined by:H(l)=H(b) log2NBut spatial complexity,C(s),is different from H(l),and can be expressed as:C(s)=H(b)·H(l)=H2(b) log2NThe measurement unit of the indices is bits(for more details,see Rescia et al.,1994).3.Resultsndscape change in SNSNPBy typifying the SNSNP we calculated landscape indices for each GU identified in order to perform a more detailed analysis of the changes.In doing so,we can infer the landscape variations according to the different local spatial dynamics.The values for the landscape indices show that the SNSNP landscape has undergone spatial variation of a certain relevance at the local scale(Table3). Mountains units encompass the part of the protected area pre-senting fewest changes.The peneplains units occupy the part of the territory presenting the biggest spatial changes.The inland mountain valleys present moderate changes in their metric val-ues.At the regional scale,SNSNP presents a considerable increase in the richness of the boundaries(variation:≈+19%)and less even-ness in the distribution of the boundaries between uses(variation:≈−5%).These changes have given rise to a certain spatial repe-tition of boundaries of uses,principally between the agriculture, livestock farming and forestry uses.Spatially,this repetition of boundaries involves a relative increase in the dominance of these types of intensive use over traditional extensive uses.Nonetheless, this variation is quite insignificant in the values of the indices at the landscape scale.The spatial complexity and heterogeneity of the landscape have remained practically the same(variation≈−1% and0%,respectively)(Table4).Thus,Table4shows that at the landscape scale,a quite simi-lar spatial pattern was maintained throughout the50-year study period.The general trend of the spatial changes in the SNSNP is a stagnant one.The values of the indices show that this landscape presents relatively low change dynamics.On average,over80%of the area remains unchanged,most of the changes responding to sit-uations of abandonment(≈8%)or of intensification of uses(≈10%) (Fig.3).ndscape change in UBRRescia et al.(1994)studied the changes in the spatial struc-ture of the landscape of UBR over the last50years.Temporal analysis shows a noteworthy increase in boundary types(n) (variation:+75%).At the same time,one can observe a loss of evenness of the boundaries(E(b))between uses(variation:≈−7%).In turn,the N number of boundaries exhibits a con-siderable increase,giving rise to fragmentation of the space through reduction of patch size and increased richness of bound-aries.At the landscape scale,this brings about a less heterogeneous and less complex landscape than the original ndscape het-erogeneity shows a decrease(variation:≈−12%)within a recurringTable4Temporal evolution and variation in the landscape indices used to analyse the spatial pattern,at regional scale,of‘Sierra Norte de Sevilla’Natural Park landscape.N:total number of boundaries;n:number of boundary types;E(b):boundary evenness;H(l): landscape heterogeneity;C(s):spatial complexity.The unit of E(b),H(l)and C(s)is bits.Landscape indices50years ago Present time Variation(%)N517519+0.39n2732+18.52E(b)0.830.79−4.82H(l)0.450.450.00C(s) 1.78 1.76−1.12A.J.Rescia et al./Landscape and Urban Planning98 (2010) 26–3531Fig.3.Aerial photographs of‘Sierra Norte de Sevilla’Natural Park—SNSNP(left)and‘Urdaibai’Biosphere Reserve—UBR(right)taken during thefifties(above)and currently (below)showing the typology of spatial change in each Natural Reserve.In SNSNP has dominated the continuity of the same type of land uses(S).In UBR has prevailed the change of type of land uses(Ch).S:stable;A:abandonment;I:intensification;Ch:land-use change.pattern.Furthermore,the spatial complexity of the landscape has been seen to decrease over the last50years and current spatial complexity is lower than initially recorded(variation:≈−7%).The information obtained from the landscape indices applied is shown in Table5.It indicates a significant change in the landscape of UBR.There are clear differences between the current landscape of UBR and that of50years ago(Fig.3).4.Discussionndscape changes and ecological consequencesThe historical process of changes in land uses in both areas had quite different connotations.In SNSNP,a coarse-grain landscape was maintained but with variation in intensity of land-use.That is, there was a change in land management but practically no changes in land uses.On the contrary,in UBR there were changes in land uses,that is,one type of use was replaced by another.Essentially,aTable5Temporal evolution and variation in the landscape indices used to analyse the spa-tial pattern,at regional scale,of‘Urdaibai’Biosphere Reserve landscape.N:total number of boundaries;n:number of boundary types;E(b):boundary evenness;H(l): landscape heterogeneity;C(s):spatial complexity.The unit of E(b),H(l)and C(s)is bits.Landscape indices50years ago Present time Variation(%)N15286677+336.98n64112+75.00E(b)0.900.84−6.67H(l)0.510.45−11.76C(s) 2.76 2.57−6.88medium-grain agricultural landscape changed to afine-grain tree-plantation landscape,with a clearly intensive use.In SNSNP no new land uses have appeared and there has been no retreat or expansion of any land use in particular.The landscape continues to be dominated by dehesas in different states of conser-vation.As already stated,however,there has been a change in use intensity.Intensification involves an associated loss of biodiversity (Plieninger and Wilbrand,2001),contamination of soils and loss of water quality(Matson et al.,1997),and alteration of biogeochem-ical cycles(Vitousek et al.,1997).Abandonment or extensification leads to the processes of shrub encroachment(Tárrega et al.,2009) and tree death in over-aged stands of the dehesa(Moreno and Pulido,2009).In UBR,the multiple-use farmland is now almost exclusively based on forestry(monoculture of Pinus radiata D Don.).Homogeni-sation of the landscape increases the risk offires,increase the risk of pathogen outbreaks(Lloret et al.,2002),and alters runoff regimes and soil moisture contents(Huxman et al.,2005).Likewise,loss of heterogeneity in the landscape reduces territorial biological diver-sity(Díaz et al.,2006),and,in many cases,causes a loss of aesthetic quality(Gómez-Limón and de Lucio-Fernández,1999).4.2.Degradation of the social–environmental resilience4.2.1.Socioeconomic and ecological vulnerabilityResilience degradation has led to an increased vulnerability of ecosystems and local populations in different cultural landscapes (Chapin et al.,2004;Eakin and Wehbe,2009).In the case of SNSNP, the local population has become more vulnerable as a result of socioeconomic changes over the last few decades.The main socioe-32 A.J.Rescia et al./Landscape and Urban Planning 98 (2010) 26–35Table 6Driving forces of changes of the social–ecological system of both Nature Reserves studied.Changes,drastic and rapid,affected their adaptability and transformability increasing their vulnerability.Resilience of the SES studied had been consolidated with gradual changes over time.DriversSocial–ecological SystemsResilience Degradation Perceived in •Loss of profitability of local products (low market competitiveness)‘Sierra Norte de Sevilla’Natural Park•Economic viability of traditional use •Rural migration•Cultural diversity•Ecosystem services (cultural)•Social structure (fabric)•Aging and decreasing population •Traditional ecological knowledge•Land-use changes (intensification and spatial homogenisation)‘Urdaibai’Biosphere Reserve•Landscape diversity •Rural migration•Biological diversity •Cultural diversity•Ecosystem services (ecological)•Traditional multiple-use structure (‘caserío’)•Traditional ecological knowledgeconomic transformation has been associated with the fall in price of typical dehesa products on the market,especially due to the production objectives of the Common Agricultural Policy (CAP).Meanwhile,together with industrialisation of the farms,there has been a 70%decrease in cattle and a 300%increase in pig production.(IEA-SIMA,2008).There has also been a 50%drop in the population (INE,2006)over the last 50years,along with a weakened busi-ness sector and deficient qualifications in the job market.All this has contributed to the greater vulnerability of the local population (Table 6).These observed changes are common places in different dehesa systems.Plieninger and Wilbrand (2001)conducted a detailed eco-nomic analysis of dehesas in the Southwestern region of Spain and perceived a similar situation to that of the SNSNP.Moreover,in other cases,the socioeconomic changes were accompanied by a radical transformation of the landscape,thus threatening the con-tinuity of the SES even further (Fernández-Alés et al.,1992).The loss of resilience in UBR is due to its drastic changes in land use.These changes have led to increased ecological vulnerability resulting from homogenisation of the landscape (Metzger et al.,2006).This process has had significant ecological repercussions (see Section 4.1and Table 6).Specifically,in UBR,it has been observed that there has been an important loss of biological diver-sity (Rescia et al.,1995),serious soil erosion problems (Schmitz et al.,1998),and alterations in the species richness of the underbrush due to intensive forest management (Atauri et al.,2004).Both environmental and economic changes in land-use systems in SNSNP and UBR are threatening the population’s traditional ecological knowledge and contributing to the degradation of the resilience therein.The adaptation and resilience of cultural land-scapes has been strengthened by the transmission of an empirical knowledge of resource use.This traditional ecological knowledge is one aspect of cultural diversity which represents knowledge of the conservation and sustainable use of an environment (Heckenberger et al.,2007;Loh and Harmon,2005;Rescia et al.,2009).Loss thereof would therefore affect the conservation of cultural diversity.4.3.Managing to restore the social–environmental resilience 4.3.1.Sustainable uses:hunting and rural tourismThe restoration of resilience in the SNSNP should be based upon measures aimed especially towards economic and social aspects.Its traditional economic and social structure was based on an acceptable level of animal production (especially extensive pig pro-duction,with sheep,cattle and goats),olive growing and otherTable 7Driving forces and management that contribute to reducing the vulnerability of the social–ecological system of both Nature Reserves studied.These would recover their capacity for adaptation and transformation and the resilience they had consolidated over a long period of time would be restored.DriversSocial–ecologicalSystemsResilience Restoration Management on •Sustainable hunting ‘Sierra Norte de Sevilla’Natural Park•Multiple-use landscape •Rural tourism•Landscape functionality•Valuing local products (denomination of origin)•Social re-activation (association,cooperation)•Subsidies on Dehesa-landscape •Economic competiveness of local products •Paying for bio-cultural services•Modernization of farms•Sustainable forestry ‘Urdaibai’Biosphere Reserve•Rural re-population plans•Rural tourism•Economic incentives to settle rural population•Valuing local products (forest certification)•Cultural re-valuation of traditional ecological knowledge•Subsidies on ‘Caserío’-landscape •Paying for bio-cultural services。
印度提议禁止使用27种农药

64现代农药第19卷第4期菌酯•戊%醇悬浮剂与0.01%芸苔素内酯可溶液剂、10%3虫5可湿性粉剂进行桶混施药,以期达到“一喷多防”和增产的效果。
结果表明,于小麦齐穗初花期用48%\烯菌酯•戊%醇悬浮剂施一次药,盛花期用\烯菌酯组合物即48%\烯菌酯•戊%醇悬浮剂与0.01%芸苔素内酯可溶液剂、10%3虫5可湿性粉剂进行桶混施药后,\烯菌酯组合物对小麦赤霉病、白粉病、锈病和k虫的防治效果均较好,对三大病害的防效分别为91.92%、84.31%、77.95%。
药后1、3、7d对k虫的防效分别为52.50%289.56%和95.30%;通过理论测产知,此组合物产能最高,为7251.75kg/hm2,增产作用明显,增产率为38.53%。
说明用此组合物不仅可以效小麦病虫害的,还可以到增产的作用,实现“一喷多效”、小麦丰产增收的目的。
因此,在生产上应进行大面积的推广应用。
参考文献[1]杜宇笑,李鑫格,王雪,等•不同产量水平稻茬小麦氮素需求特征研究[J].作物学报,2020,网络首发https:///kcms/de-tail/11.1809.S.20200713.1406.006.html.[2]张承启•新型杀菌剂氧烯菌酯对禾谷镰刀菌的作用机制研究[D].杭州:浙江大学:2016.[3]VALIPOUR nd use policy and agricultural water managementof the previous half of century in AWrica[J].Applied Water Science, 2015,5:367-395.[4]YIN Y,LIU X,LI B,et al.Characterization of sterol demethylationinhibitor-resistant isolates of Fusarium asiaticum and F.graminearum collected from wheat in China[J].Disease Control Pest Management,2009,99:487-497.[5]ZHANG C Q,CHEN Y,YIN Y N,et al.A small molecule speciesspecifically inhibits Fusarium myosin I[J].Environmentalogy Microbiology,2015,17:2735-2746.[6]ZHANG X X,SUN H Y,SHEN C M,et al.Survey of Fusarium spp.causing wheat crown rot in major winter wheat growing regions of China[J].Plant Disease,2015,99:1610.[7],,,.的小麦白粉病因Pm57抗性丧失突变体的筛选与鉴定[J].植物遗传资源学报, 2020,21(2):386-393.[8]韦刚.45%烯•苯•嗓FS种子包衣防治小麦病虫害效果试验[J].安通,2020,26(4):91-93.[9].菌剂\烯菌酯菌Fusarium asiaticum单端抱霉烯族毒素合成的机制研究[D].南京:南京农业大学:2017.[10]李艳朋,李猛,李秀(•植保无人机与飞防助剂在小麦赤霉病防治上的应用效果[J].浙江农业科学:2020,61(3):445-447.[11]赵帅,李艳朋,李猛,等•不同药剂防治小麦白粉病药效试验[J].大麦与谷类科学:2020,37(3):53-55.[12]张冬梅,付文君•19%7氧•丙环%悬浮剂防治小麦锈病药效试验[J].新疆农业科技,2020(1):33-34.[13]江彦军,孙明清,张红芹,等•纳米农药制剂对小麦k虫的防效试[J].通,2020,26(12):74-75.[14]马书芳•不同药剂应用植保无人机防治小麦赤霉病田间药效试验[J].现代农业科技,2017(6):124-125.[15]蒲乐凡,任慧,欧杨晨,等•小麦茎基腐病和赤霉病抗源筛选及关联SNP位点分析[J].麦类作物学报,2020,40(7):521-529. [16]HOU Y P,MAO X W,WANG J X,et al.Sensitivity of Fusariumasiaticum to a novel succinate dehydrogenase inhibitor fungicide pydiflumetofen[J].Crop Protection,2017,96:237-244.[17]向礼波,杨立军,薛敏峰,等•禾谷镰抱菌对氟%菌酰羟胺敏感性基线的建立及药剂田间防效[J]•农药学学报,2018,20(4):445-451.[18]CHEN Y,ZHOU M G.Characterization of Fusarium graminearum isolates resistant to both carbendazim and a new fungicide JS399-19[J].Phytopathology,2009,99:441-446.[19]ZHANG Y,CHEN W C,SHAO W Y,et al.Molecular,biologicaland physiological characterizations of resistance to phenamacril in Fusarium graminearum[J].Plant Pathology,2017,66(9):1404-1412.[20]LI M X,LI T,DUAN Y B,et al.Evaluation of phenamacril and ip-conazole for control of rice bakanae disease caused by Fusarium fujikuroi[J~].Plant Disease,2018,102:1234-1239.[21]卢中健,张杰峰,沈田辉,等.48%氤烯菌酯•戊%醇SC防治小麦赤霉病药效试验研究[J].上海农业科技,2020(3):121-122. [22]张春云,张桥,吴庭友,等.几种杀菌剂对小麦赤霉病及真菌毒素的控制效果[J].安徽农业科学:2019,47(20):155-158.(责任编辑:高蕾) 27种*+I近日,印度农业部提出禁止使用27种农药,以及禁售农药活性成分。
Long-term impacts of land-use change

Long-term impacts of land-use change 2011-01-18Abstract:Land-use changes, especially the conversion of native forest vegetation to cropland and plantations intropical region, can alter soil C and N pools and N availability for plant uptake. Deforestation, followed by shiftingcultivation and establishment of rubber tree plantation, is a common land-use change in Xishuangbanna, southwestChina. However the influence of this kind of land-use change on soil C and N dynamics in this region remains poorlyunderstood. This study was conducted to assess the effects of land-use change on soil C and N pools. Soil sampleswere collected on five adjacent plots, which belong to three land-use types including secondary forest-an acuminatebanana( Musa itinerans) secondary forest and a male bamboo( Dendrocalamus membranaceae) secondary forest,shifting cultivation, and rubber tree ( Hevea brasiliensis (H. B. K. ) Muell. Arg. ) plantation (one plot is 3-year-old,and another is 7-year-old). We measured soil bulk density (BP), pH value, moisture content and concentrations ofsoil organic carbon(SOC), total soilnitrogen(TSN), and inorganic N(NO-3 -N and NH~ -N) at 0-3, 3-20, 20-40and 40-60 cm depths, and calculated C and N pools in 0-20, 20-40, 40-60, and 0-60 cm soil pared with the adjacent secondary forests, shifting cultivation and establishment of rubber tree plantationsresulted in significant decline in concentrations and stocks of SOC and TSN in 0-20 and 0-60 cm soil layers, andincrease in pH and bulk density at 0-3, 3-20, and 20-40 cm depths. Soil moisture content decreased only in 0-20 cm surface soils in shifting cultivation and plantations. The dynamics of mineral N was much more complex,which had different trends among depths and ecosystems. Compared with the secondary forests, SOC stocks in 0-20 cm surface soils in shifting cultivation and rubber tree plantations(3-year-old plantation and 7-year-old plantation)decreased by 34.0%, 33%, and 23%; and TSN stocks decreased by 32.2%, 20.4%, and 20.4%, respectively,whereas the decreases of SOC and TSN stocks in 0-60 cm soil layers were much less. The results indicated that Cand N losses were mainly occurred in 0-20 cm surface soil, followed by 20-40 cm layer.作 者:YANG Jing-Cheng HUANG Jian-hui PAN Qing-min TANG Jian-Wei HAN Xing-guo ä½œè€…å •ä½ ï¼šYANG Jing-Cheng,HUANG Jian-hui,PAN Qing-min,HAN Xing-guo(Laboratory of Quantitative Vegetation Ecology, Institute of Botany, Chinese Academy of Sciences, Beijing 100093, China)TANG Jian-Wei(Xishuangbanna Tropical Botanical Garden, Chinese Academy of Sciences, Mengla 666303, China)期 刊:环境科å¦å¦æŠ¥(英文版) ISTICSCI Journal:JOURNAL OF ENVIRONMENTALSCIENCES 年,啷(期):2004, 16(2) åˆ†ç±»å ·ï¼šX14X13 Keywords:soil organic carbon (SOC) total soil nitrogen (TSN) inorganic nitrogen land- use change tropical soil Xishuangbana。
Follow-up and modeling of the land use in an intensive agricultural watershed in France
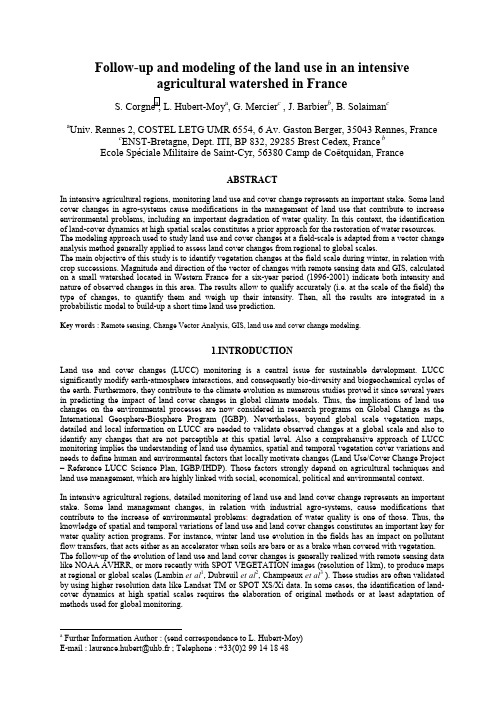
Follow-up and modeling of the land use in an intensiveagricultural watershed in FranceS. Corgne a, L. Hubert-Moy a, G. Mercier c , J. Barbier b, B. Solaiman ca Univ. Rennes 2, COSTEL LETG UMR 6554, 6 Av. Gaston Berger, 35043 Rennes, Francec ENST-Bretagne, Dept. ITI, BP 832, 29285 Brest Cedex, France bEcole Spéciale Militaire de Saint-Cyr,56380 Camp de Coëtquidan, FranceABSTRACTIn intensive agricultural regions, monitoring land use and cover change represents an important stake. Some land cover changes in agro-systems cause modifications in the management of land use that contribute to increase environmental problems, including an important degradation of water quality. In this context, the identification of land-cover dynamics at high spatial scales constitutes a prior approach for the restoration of water resources. The modeling approach used to study land use and cover changes at a field-scale is adapted from a vector change analysis method generally applied to assess land cover changes from regional to global scales.The main objective of this study is to identify vegetation changes at the field scale during winter, in relation with crop successions. Magnitude and direction of the vector of changes with remote sensing data and GIS, calculated on a small watershed located in Western France for a six-year period (1996-2001) indicate both intensity and nature of observed changes in this area. The results allow to qualify accurately (i.e.at the scale of the field) the type of changes, to quantify them and weigh up their intensity. Then, all the results are integrated in a probabilistic model to build-up a short time land use prediction.Key words : Remote sensing, Change Vector Analysis, GIS, land use and cover change modeling.1.INTRODUCTIONLand use and cover changes (LUCC) monitoring is a central issue for sustainable development. LUCC significantly modify earth-atmosphere interactions, and consequently bio-diversity and biogeochemical cycles of the earth. Furthermore, they contribute to the climate evolution as numerous studies proved it since several years in predicting the impact of land cover changes in global climate models. Thus, the implications of land use changes on the environmental processes are now considered in research programs on Global Change as the International Geosphere-Biosphere Program (IGBP). Nevertheless, beyond global scale vegetation maps, detailed and local information on LUCC are needed to validate observed changes at a global scale and also to identify any changes that are not perceptible at this spatial level. Also a comprehensive approach of LUCC monitoring implies the understanding of land use dynamics, spatial and temporal vegetation cover variations and needs to define human and environmental factors that locally motivate changes (Land Use/Cover Change Project – Reference LUCC Science Plan, IGBP/IHDP). Those factors strongly depend on agricultural techniques and land use management, which are highly linked with social, economical, political and environmental context.In intensive agricultural regions, detailed monitoring of land use and land cover change represents an important stake. Some land management changes, in relation with industrial agro-systems, cause modifications that contribute to the increase of environmental problems: degradation of water quality is one of those. Thus, the knowledge of spatial and temporal variations of land use and land cover changes constitutes an important key for water quality action programs. For instance, winter land use evolution in the fields has an impact on pollutant flow transfers, that acts either as an accelerator when soils are bare or as a brake when covered with vegetation. The follow-up of the evolution of land use and land cover changes is generally realized with remote sensing data like NOAA AVHRR, or more recently with SPOT VEGETATION images (resolution of 1km), to produce maps at regional or global scales (Lambin et al1, Dubreuil et al2, Champeaux et al3 ). These studies are often validated by using higher resolution data like Landsat TM or SPOT XS/Xi data. In some cases, the identification of land-cover dynamics at high spatial scales requires the elaboration of original methods or at least adaptation of methods used for global monitoring.a Further Information Author : (send correspondence to L. Hubert-Moy)E-mail : laurence.hubert@uhb.fr ; Telephone : +33(0)2 99 14 18 48The objective of this study is to follow-up LUCC and to model its winter behavior in an intensive agricultural region. In this paper, we focus on the Change Vector Analysis (CVA) method developed by Malila4 in 1980 and refined by Colwell and Weber, in1981, and latter by Lambin and Strahler5. This approach offers the opportunity to optimize the radiometric characteristics of each image, and then, to qualify and quantify the observed changes by calculating the magnitude and the direction of changes.The originality of this study is the adaptation of the CVA to model the land use and land cover changes at the field scale in a region characterized by high spatial and time variations of the vegetation cover. The main objective of this study is to identify vegetation changes at the field scale during several winter seasons in relation with crop successions in order to define spatial and time trajectories that will improve the knowledge of the land use and cover evolution and its impact on the water quality.2. CHANGE VECTOR ANALYSIS METHODFor three decades, a wide range of methods and techniques have been proposed to model and monitor LUCC using remotely sensed images. Most of them can be grouped in two general classes (Johnson and Kasischke6 ):by comparing raw data (method based on the exploitation of the radiometric value of analysis- Changeeach image)- Change analysis by comparing classified data (intersection between classifications).The main limitation of the classification approach lies on the increase of errors due to mis-classifications. Moreover, it gives little information about the LUCC dynamics (Malila4 ). On the other hand, the change analysis method, that uses raw data, may be applied with multiple approaches to detect the change between two images such as band differencing (Weismiller et al7 ), transformed band differencing as vegetation indices (Nelson et al8 ), regression (Singh9 ), rationing (Howarth et al10 ), CVA between multispectral data (Malila4 )…This last method offers several advantages : a more complete use of the data since all radiometric values for each pixel are taken into consideration; a more accurate result with the identification of the magnitude and direction of the land cover changes; a more adaptable method according to the objectives of the study thanks to the scalability of the CVA (Lambin11 ).“CVA is a multivariate method, which takes n bands, transforms, or spectral features as input from each pair of scene”(Johnson, Kasischke6 ). The method is based on the measure of the radiometric changes to follow-up of the LUCC. The measure of the radiometric change is evaluated with two components:- The Euclidian distance that separates radiometric values of the same pixel at two dates; it characterizes the magnitude (M) of change and measures its intensity.- The angle of the direction (D) of change that indicates the nature of the land cover change. ArrayFigure 1. Change vector representation in a two-band radiometric spaceThe figure 1 shows a naive simulation of the evolution of two pixels (Ti and Tj) represented in a two-dimension space. Bands 1and 2 may be used as a composition of bands like a vegetation index (NDVI, TSAVI…) as well as an orthogonal transformation (Principal Component Analysis), or red and near-infra red bands… The magnitude value that measures the intensity of change for the pixels Ti and Tj is calculated with the radiometric values of each pixel. Since the magnitude depends on the evolution of the two pixels over the time, the magnitude is high for [Ti-Tj] and low for [Tk-Tl]. “The direction of the change corresponds to a diagonal connecting the origin with the opposite corner of the parallelepiped defined by the vector” (Lambin et al1 ). The angle of the vector between the two dates characterises the direction of the change, illustrating for instance gain or loss of vegetation. On the figure 1, the angle of [Tn-Tm] may characterise a loss of vegetation and the angle measured on [Ti-Tj] a gain of vegetation.Since CVA consists in the comparison of radiometric values for each band, high-quality data pre-processing are required. Radiometric and geometric corrections are essential when the purposes of the image processing are: - To get physical values (by transforming numeric value to reflectance value)- To make multidate comparison between images coming from the same or any sensor.3. WINTER VEGETATION COVER MONITORING3.1 Study area and dataThe CVA was applied on a watershed, the Yar (61,5 km2) located on the western coast of Brittany (West France). Intensive farming combined with wet and warm autumns produce significant amounts of nitrogen before winter infiltration of water. For several years, high nitrogen rates are observed in rivers, mostly due to an excess in fertilization. Another effect is the euthrophization phenomenon, which occurs in the shape of algae bloom in spring on the coastal area, and that is extending from year to year. Consequences on environmental and tourist activities have lead local authorities to take decisions to restore water quality. In this area, crops cover approximately 60% of the total vegetated areas. Main crops are produced in relation with industrial breeding, principally corn, wheat and artificial meadows. During winter, which means a rainy season, most of fields remain traditionally without any vegetation cover after harvesting corn. In this area, nitrogen inputs are still too high, especially on bare soils that follow and precede corn implementation during the winter. The follow-up of LUCC is particularly difficult in this region, since spatial and time land cover variations are often high and the driven factors of changes are multiple.Change detection method must be used according to the regional context and must provide a fine change detection in order to be a powerful decision tool for the actors of the water restoration quality program. For winter trajectories, the detection of change should point out, at the field scale, the evolution between two years, at two levels: vegetation cover conversions (e.g. bare soils in T1 to cover soil in T2 and vice-versa), the rates of soil cover and their evolution between two years as far as transfer flows are concerned. To reach these objectives, CVA method has been adapted to the field scale.A set of 12 remotely sensed images (11 SPOT images and 1 IRS-LISS III -2 per year over 6 years from 1996 until 2002) is used for this study. The 5S model has been used for its relative simplicity to correct atmospheric effects (Tanré et al12 ). All the images have been then normalised with a “reference” image, the scene that has the widest radiometric dynamic range (winter 1999/00). Furthermore, geometric corrections are applied for each scene (with at least six ground control points) and data are registered in a geographical referential (Lambert 2 Conforme), with a low RMS error (<0.5 pixel). Then, all the scenes can be used for multispectral processing. Field characteristics have been regularly collected on the field to validate image processing.3.2 CVA processing procedureThe follow-up and the modelling of the land use changes have been carried out in two steps:- 1) the crop successions from winter 1996/97 to winter 2001/02 have been defined with supervised classifications (with the classical maximum likelihood method). The fields limits were updated each year from satellite data and the results were integrated into a GIS (L. Hubert-Moy et al13 ). At this step, the objectives are to quantify and localize the bare soils on the whole watershed. Though in this way, some fields may be detected as bare soils whereas it is a young crop (wheat for example); with the knowledge of the following spring and summer land cover, it is possible to know if the field was really a bare soil during all winter or if, a contrario, a winter inter-crop has been sown. Even if the classification method does not allow to quantify precisely thevegetation cover, it gives a good idea of the global winter inter-crop coverage during the winter season. On the table 1, we can note that the evolution of bare soils is very low between two winters even if during the winter 2000/01, the proportion is slightly lower (4.1%). Agricultural policies now encourage the farmers to cover their fields in winter, the results on the winter 2001/02 will confirm this evolution or will show that we were in the cycle of crop successions.Table 1. Bare soils in winter between two crops, by ha and % of the total surface (1996-2001)Multi-temporal classification allows to localize the “sensitive” fields that are not covered with vegetation during four or more winters since 1996 and to establish a diagnostic about the crop succession evolution.Nevertheless, in relation with research on pollutant transfers (like nitrogen flows), a finest study of the cover soil and its evolution is required for a more realistic modeling of LUCC in relation with the water quality.- 2) CVA proves its greatest utility when full-dimensional radiometric change information is required (Johnson et al 6 ). In this study, where all changes between winters are potentially interesting to map, CVA appears well adapted since it can capture change information through spatial and time land cover and land use trajectories. For instance, see figure 2 for the follow-up of the land use changes flowchart for the winters 2001 and 2002: Winter 1996-1997 Winter 1997-1998 Winter 1998 1999 Winter 1999-2000 Winter 2000-2001330 ha 331 ha 314 ha 331 ha 2535.4% 5.4% 5.1% 5.4% 4.1%NIR band )²3Bchange (Direction). A threshold (Table 2) is applied to each image [3]and, the two resulting images are mixed and the resulting values are affected within the fields’ boundaries into a GIS [4].Magnitude Direction1: no change [0; 5] 1: No change [-2; 5]2: low change [6; 10] 2: Gain of vegetation [6; 31]3: High change[11; 20] 3: Loss of vegetation [-27; -3]4: Very high change[21; 57]Table 2. Threshold of the Magnitude and Direction images4. RESULTSFigure 3 illustrates the CVA results. Analysis by CVA shows that the watershed is concerned by few changes between those two winters(See Table 3), 4484 ha (out of a total area of 6150 ha) are classified in the class “no change”. They correspond essentially to forest or fallow land. The surface affected to the “very high change”.Figure 3.The “very high change with loss of vegetation” class corresponds to fields that were totally covered during the winter 2000/01 and bared in 2001/02. The management of the fields depends on several factors and is very difficult to model it. However, these types of dramatic changes are generally due to the cycle of the crop successions. The “very high change with gain of vegetation” class is more important than the class 6. Climatic conditions are in this case a determinant factor: the winter 2000/01 was very rainy and most of the farmerscouldn’t sow inter-crops during the winter. On the contrary, the winter 2001/02 was dry and climatic conditions were not a restrictive factor for crop sowing.Classes 4 and 5 (“high change with gain or loss of vegetation”) represent a significant part of the watershed changes with a total of 759 ha. The changes of classes 4 and 5 are less radical than the ones of classes 6 and 7 but play a major role for the flows of nitrogen transfers since it represents 12.4% of the overall watershed surface. For the class “high change with loss of vegetation”, it can correspond for example to a field that was either covered with vegetation during the winter 2000/01 and with few vegetation during the next winter or to a field partially covered with vegetation in 2000/01 and without any vegetation cover during the winter 2001/02.The watershed upriver is characterized by little changes (presence of important forest areas). On the contrary, in the north of the watershed (more precisely the north-west), important changes can be noticed in relation with intensive agricultural practices occurring in this part of the watershed. The high rhythm of the crop successions induces strong changes between all winter seasons. However, the CVA allows to discriminate fields that have potentially a major role in the pollution flows, at a higher scale and it shows the global evolution of the land cover and its dynamics on the watershed.Table 3. Classes of change from CVA results (winter 2000/01 and 2001/02)figure 4, Figure 4. Determination of radiometric trajectories of five test fields (Yar’s watershed, 1996-2001)The fields, which have met little changes between two winters (curves 1 and 2), are characterized by short curves that illustrated short spatial-temporal trajectories. On the contrary, fields with high changes have a stretched curve due to significant radiometric changes between two successive winters.Results of the intersection of the CVA resulting map and the occurrence bare soil map produced from the multitemporal image classification [Fig.5a; Fig.5b] are shown on figure 5. It allows to spatialize and discriminate the land use trajectories and their spatial and temporal evolution.For instance, fields that are concerned with no or little changes between each winter (trajectory T2 and T2b on the Fig.5c and Fig.5d), correspond essentially to fields that belong to the category “bare soils” since the winterClasses Surface (ha)1: no change 44842: low change with loss of vegetation 1033: low change with gain of vegetation 301 4: high change with loss of vegetation 392 5: high change with gain of vegetation 367 6: very high change with loss of vegetation 81 7: very high change with gain of vegetation 1581996 or, as well, to“forest areas” and “permanent meadows” located near the river stream. The fields covered with vegetation from 1996 to 2002 totalize 3914 ha. More than 2750 ha are characterized by low changes and 1164 ha by at least one important or high change during the 6 winter-period. Among these latest, 527 ha are detected with a gain of vegetation and 637 ha with a loss of vegetation.On the other hand, some fields are concerned with high variations between each winter. For instance, the trajectory T1, shows that during the winter 98-97 and the next winter a high change with gain of vegetation has occurred, followed by a high change with loss of vegetation during the winter 99-98, followed by two winters with low changes gain and loss vegetation, and finally by a high change detected between the two last winters. In this case, 3 winters with the “bare soil” class are detected. The trajectories with high variations of land cover concern especially the fields where two to five “bare soil” situations have been detected since the winter 1996. They totalize 840 ha, 66% have met important or very important changes between the winters and only 274 ha with few or no changes. If we focus on the fields with 4 “bare soil” situations since 1996, almost 70% are concerned by trajectories where at least one winter with loss of vegetation is included and about 62 % of the changes are classified as important or very important.Thus, the combination of the classification method with the CVA provides a finest change detection. Also, the classification results quantify the land cover evolution (follow-up of the bare soil in winter since 1996).The CVA proved to be a robust method for qualifying the changes (in the determination of the land cover rates and their evolution between from a winter to another) and the combination of the two methods allows, at a field scale, to produce land use trajectories for a better understanding of the LUCC.With the help of these information, a model based on a probabilistic theory (Dempster-Shafer theory) has been successfully applied to predict a short time bare soils evolution (Hubert et al14).5. CONCLUSION AND FUTURE WORKIn this study, the follow-up of the land use and cover had to identify the land cover dynamics at field scale, in the context of a water quality restoration program. A classic approach is first applied with the determination of the crop successions issued from supervised classification of the satellite data. Then, a characterization of the land use for each winter and its evolution is achieved. Nevertheless to characterize and weigh up the evolution of observed changes in winter, a finer method is required. The Change Vector Analysis has been chosen for its ability to using the radiometric data of each image. In several studies, the CVA has already shown its advantages to capture all changes, but most of them were achieved at regional scale. The application of the CVA on the Yar’s watershed has required an adaptation of the method to manage, to qualify and identify the changes at field scale. The combination of the two techniques produces spatial-temporal trajectories for each field. All those data represent parameters to integrate in a flow nitrogen model (INCA) that predicts the water quality at the downstream of the river. Thus, the CVA provides an important source of information that can be used in multiple applications.All the data yielded are intended to be integrated in flow nitrogen’s model (INCA: Integrated in Nitrogen Catchment Area, UE, 5th Framework Program, USARQ-INRA/COSTEL). They represent one of the parameters integrated in the model to measure the evolution of the water quality in this region and elaborate predictions. Moreover, the results are integrated in a probabilistic model (that use the Dempster-Shafer theory) to perform short time predictions of the land use and cover in winter.occurrence map (b); 2] Spatialisation (c) and determination (d) of the land use trajectories at field scaleAcknowledgments:This work is funded by the PNTS (Programme National de Télédétection Spatiale) and the “Conseil Régional de Bretagne”. The authors would like to thank gratefully the “Chambre d’Agriculture des Côtes d’Armor”, the “Communauté de Communes de Lannion” the “Conseil Général des Côtes d’Armor” for their valuable help in providing database and field information on the study area.References1. E.F. Lambin, A.H. Stralher, Indicators of land cover change for change vectors analysis in multitemporal space at coarse spatial scale, International Journal of Remote Sensing, vol.15, 10, pp. 2099-2119, 1994.2. V. Dubreuil, L. Le Dû, Etude du couvert végétal (NDVI) par télédétection (NOAA-AVHRR), Participation àl'ouvrage "Le climat, l'eau et les hommes; Mélanges offerts au Professeur Jean Mounier", Presses Universitaires de Rennes, pp. 45-63, 1997.3. J.L. Champeaux, D. Arcos, E. Bazil, D. Girard, J.P. Goutorbe, J.P. Habets, J. Noilhan, J.L.Roujean, AVHRR-derived vegetation mapping over Western Europe for use in numerical weather prediction models, International Journal of Remote Sensing, 21, pp. 1235-1249, 2000.4. W. Malila, Change Vector Analysis: An approach for detecting forest changes with Landsat, Proceedings, Machine Processing of Remote Sensed Data Symposium, Purdue University, West Lafayette, Indiana, (Ann Arbor, ERIM), pp. 326-335, 1980.5. J. Colwell and F. Weber, Forest change detection, Fifteen International Symposium on Remote Sensing of Environment, 11-15 May 1981 (Ann-Arbor, ERIM), pp. 839-852, 1981.6. R.D. Johnson, E.S. Kasischke, Change vector analysis : a technique for the multi spectral monitoring of land cover and condition, International Journal of Remote Sensing, vol.19, 3, pp. 411-426, 1998.7. R.A. Weismiller, S Kristof , D.K. Scholz, P.E. Anuta, S. Momin, Change detection in coastal zone environments, Photogrammetric Engineering and Remote Sensing, 43, 1533-1539, 1977.8. R. Nelson and B. Holben, Identifying deforestation in Brazil using multiresolution satellite data, International Journal of Remote Sensing, 7, pp. 429-448, 1986.9. A. Singh, Digital change detection techniques using remotely sensed data, International Journal of Remote Sensing, 10, pp. 989-1003, 1989.10. P. Howarth, G. Wickware, Procedure for change detection using Landsat digital data, International Journal of Remote Sensing, 2, 277-291, 1981.11. E.F. Lambin, Change detection at multiple temporal scales: seasonal and annual variations in landscape variables, Photogrammetric Engineering and Remote Sensing, vol.62, 8, pp. 931-939, 1996.12. D. Tanré, C. Deroo, P. Duhaut, M. Herman, J.J. Morcrette, Description of a computer code to simulate the satellite signal in the solar spectrum : the 5S code, International Journal of Remote Sensing, vol.11, 4, pp. 659-668, 1990.13. L. Hubert-Moy, A. Cotonnec L.Le Du, A.Chardin, P. Perez,A comparison of classification procedures of remotely sensed data applied on different landscape units, Remote Sensing of Environment, ed. Elsevier, vol.75, 2, pp.174-187, 200114. L. Hubert-Moy, S. Corgne, G. Mercier, B. Solaiman, Land use and land cover change prediction with the theory of evidence : a study case in an intensive agricultural region in France, Proceedings, Fusion 2002 Conference, Annapolis, pp. 114-122, 2002.。