XMM-Newton and Broad Iron Lines
Comparing Suzaku and XMM-Newton Observations of the Soft X-ray Background Evidence for Sola
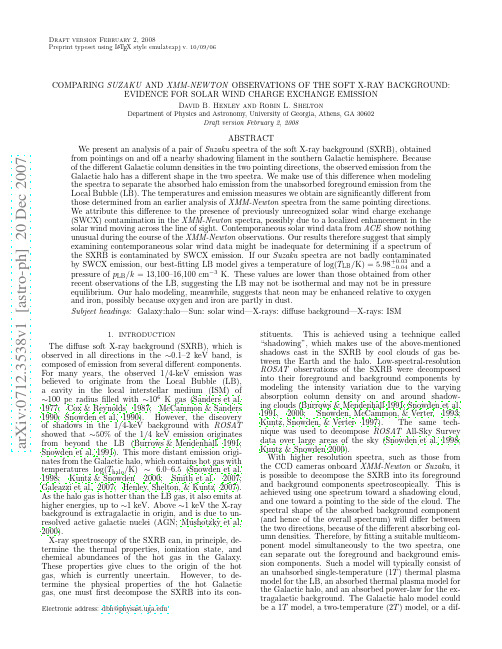
a r X i v :0712.3538v 1 [a s t r o -p h ] 20 D e c 2007Draft version February 2,2008Preprint typeset using L A T E X style emulateapj v.10/09/06COMPARING SUZAKU AND XMM-NEWTON OBSERVATIONS OF THE SOFT X-RAY BACKGROUND:EVIDENCE FOR SOLAR WIND CHARGE EXCHANGE EMISSIONDavid B.Henley and Robin L.SheltonDepartment of Physics and Astronomy,University of Georgia,Athens,GA 30602Draft version February 2,2008ABSTRACTWe present an analysis of a pair of Suzaku spectra of the soft X-ray background (SXRB),obtained from pointings on and offa nearby shadowing filament in the southern Galactic hemisphere.Because of the different Galactic column densities in the two pointing directions,the observed emission from the Galactic halo has a different shape in the two spectra.We make use of this difference when modeling the spectra to separate the absorbed halo emission from the unabsorbed foreground emission from the Local Bubble (LB).The temperatures and emission measures we obtain are significantly different from those determined from an earlier analysis of XMM-Newton spectra from the same pointing directions.We attribute this difference to the presence of previously unrecognized solar wind charge exchange (SWCX)contamination in the XMM-Newton spectra,possibly due to a localized enhancement in the solar wind moving across the line of sight.Contemporaneous solar wind data from ACE show nothing unusual during the course of the XMM-Newton observations.Our results therefore suggest that simply examining contemporaneous solar wind data might be inadequate for determining if a spectrum of the SXRB is contaminated by SWCX emission.If our Suzaku spectra are not badly contaminatedby SWCX emission,our best-fitting LB model gives a temperature of log(T LB /K)=5.98+0.03−0.04and a pressure of p LB /k =13,100–16,100cm −3K.These values are lower than those obtained from other recent observations of the LB,suggesting the LB may not be isothermal and may not be in pressure equilibrium.Our halo modeling,meanwhile,suggests that neon may be enhanced relative to oxygen and iron,possibly because oxygen and iron are partly in dust.Subject headings:Galaxy:halo—Sun:solar wind—X-rays:diffuse background—X-rays:ISM1.INTRODUCTIONThe diffuse soft X-ray background (SXRB),which is observed in all directions in the ∼0.1–2keV band,is composed of emission from several different components.For many years,the observed 1/4-keV emission was believed to originate from the Local Bubble (LB),a cavity in the local interstellar medium (ISM)of ∼100pc radius filled with ∼106K gas (Sanders et al.1977;Cox &Reynolds 1987;McCammon &Sanders 1990;Snowden et al.1990).However,the discovery of shadows in the 1/4-keV background with ROSAT showed that ∼50%of the 1/4keV emission originates from beyond the LB (Burrows &Mendenhall 1991;Snowden et al.1991).This more distant emission origi-nates from the Galactic halo,which contains hot gas with temperatures log(T halo /K)∼6.0–6.5(Snowden et al.1998;Kuntz &Snowden 2000;Smith et al.2007;Galeazzi et al.2007;Henley,Shelton,&Kuntz 2007).As the halo gas is hotter than the LB gas,it also emits at higher energies,up to ∼1keV.Above ∼1keV the X-ray background is extragalactic in origin,and is due to un-resolved active galactic nuclei (AGN;Mushotzky et al.2000).X-ray spectroscopy of the SXRB can,in principle,de-termine the thermal properties,ionization state,and chemical abundances of the hot gas in the Galaxy.These properties give clues to the origin of the hot gas,which is currently uncertain.However,to de-termine the physical properties of the hot Galactic gas,one must first decompose the SXRB into its con-Electronic address:dbh@stituents.This is achieved using a technique called “shadowing”,which makes use of the above-mentioned shadows cast in the SXRB by cool clouds of gas be-tween the Earth and the halo.Low-spectral-resolution ROSAT observations of the SXRB were decomposed into their foreground and background components by modeling the intensity variation due to the varying absorption column density on and around shadow-ing clouds (Burrows &Mendenhall 1991;Snowden et al.1991,2000;Snowden,McCammon,&Verter 1993;Kuntz,Snowden,&Verter 1997).The same tech-nique was used to decompose ROSAT All-Sky Survey data over large areas of the sky (Snowden et al.1998;Kuntz &Snowden 2000).With higher resolution spectra,such as those from the CCD cameras onboard XMM-Newton or Suzaku ,it is possible to decompose the SXRB into its foreground and background components spectroscopically.This is achieved using one spectrum toward a shadowing cloud,and one toward a pointing to the side of the cloud.The spectral shape of the absorbed background component (and hence of the overall spectrum)will differ between the two directions,because of the different absorbing col-umn densities.Therefore,by fitting a suitable multicom-ponent model simultaneously to the two spectra,one can separate out the foreground and background emis-sion components.Such a model will typically consist of an unabsorbed single-temperature (1T )thermal plasma model for the LB,an absorbed thermal plasma model for the Galactic halo,and an absorbed power-law for the ex-tragalactic background.The Galactic halo model could be a 1T model,a two-temperature (2T )model,or a dif-2HENLEY AND SHELTONferential emission measure (DEM)model (Galeazzi et al.2007;Henley et al.2007;S.J.Lei et al.,in preparation).Recent work has shown that there is an additional complication,as X-ray emission can originate within the solar system,via solar wind charge exchange (SWCX;Cox 1998;Cravens 2000).In this process,highly ion-ized species in the solar wind interact with neutral atoms within the solar system.An electron transfers from a neutral atom into an excited energy level of a solar wind ion,which then decays radiatively,emitting an X-ray photon.The neutral atoms may be in the outer reaches of the Earth’s atmosphere (giving rise to geo-coronal emission),or they may be in interstellar ma-terial flowing through the solar system (giving rise to heliospheric emission).It has been estimated that the heliospheric emission may contribute up to ∼50%of the observed soft X-ray flux (Cravens 2000).The geocoro-nal emission is typically an order of magnitude fainter,but during solar wind enhancements it can be of similar brightness to the heliospheric emission (Wargelin et al.2004).SWCX line emission has been observed with Chandra ,XMM-Newton ,and Suzaku (Wargelin et al.2004;Snowden,Collier,&Kuntz 2004;Fujimoto et al.2007).As the SWCX emission is time varying,it cannot easily be modeled out of a spectrum of the SXRB.If SWCX contamination is not taken into account,analyses of SXRB spectra will yield incorrect results for the LB and halo gas.This paper contains a demonstra-tion of this fact.Henley et al.(2007)analyzed a pair of XMM-Newton spectra of the SXRB using the pre-viously described shadowing technique.One spectrum was from a direction toward a nearby shadowing filament in the southern Galactic hemisphere (d =230±30pc;Penprase et al.1998),while the other was from a direc-tion ∼2◦away.The filament and the shadow it casts in the 1/4-keV background are shown in Figure 1.Contem-poraneous solar wind data from the Advanced Composi-tion Explorer (ACE )showed that the solar wind was steady during the XMM-Newton observations,without any flares or spikes.The proton flux was slightly lower than average,and the oxygen ion ratios were fairly typ-ical.These observations led Henley et al.(2007)to con-clude that their spectra were unlikely to be severely con-taminated by SWCX ing a 2T halo model,they obtained a LB temperature of log(T LB /K)=6.06and halo temperatures of log(T halo /K)=5.93and 6.43.The LB temperature and the hotter halo temperature are in good agreement with other recent measurements of the SXRB using XMM-Newton and Suzaku (Galeazzi et al.2007;Smith et al.2007),and with analysis of the ROSAT All-Sky Survey (Kuntz &Snowden 2000).We have obtained spectra of the SXRB from the same directions as Henley et al.’s (2007)XMM-Newton spectra with the X-ray Imaging Spectrometer (XIS;Koyama et al.2007)onboard the Suzaku X-ray obser-vatory (Mitsuda et al.2007).The XIS is an excellent tool for studying the SXRB,due to its low non-X-ray background and good spectral resolution.Our Suzaku pointing directions are shown in Figure 1.We analyze our Suzaku spectra using the same shadowing technique used by Henley et al.(2007).We find that there is poor agreement between the results of our Suzaku analysis and the results of the XMM-Newton analysis in Henley et al.(2007).We attribute this discrepancy to previously un-Fig. 1.—The shadowing filament used for our observations,shown in Galactic coordinates.Grayscale :ROSAT All-Sky Sur-vey R1+R2intensity (Snowden et al.1997).Contours :IRAS 100-micron intensity (Schlegel et al.1998).Yellow squares :Our Suzaku pointing directions.recognized SWCX contamination in the XMM-Newton spectra,which means that SWCX contamination can oc-cur at times when the solar wind flux measured by ACE is low and does not show flares.This paper is organized as follows.The Suzaku obser-vations and data reduction are described in §2.The anal-ysis of the spectra using multicomponent spectral models is described in §3.The discrepancy between the Suzaku results and the XMM-Newton results is discussed in §4.This discrepancy is due to the presence of an additional emission component in the XMM-Newton spectra,which we also describe in §4.In §5we measure the total intensi-ties of the oxygen lines in our Suzaku and XMM-Newton spectra.We concentrate on these lines because they are the brightest in our spectra,and are a major component of the 3/4-keV SXRB (McCammon et al.2002).In §6we present a simple model for estimating the intensity of the oxygen lines due to SWCX,which we compare with our observations.We discuss our results in §7,and con-clude with a summary in §8.Throughout this paper we quote 1σerrors.2.OBSERVATIONS AND DATA REDUCTIONBoth of our observations were carried out in early 2006March.The details of the observations are shown in Ta-ble 1.In the following,we just analyze data from the back-illuminated XIS1chip,as it is more sensitive at lower energies than the three front-illuminated chips.Our data were initially processed at NASA Goddard Space Flight Center (GSFC)using processing version 1.2.2.3.We have carried out further processing and fil-tering,using HEAsoft 1v6.1.2and CIAO 2v3.4.We first combined the data taken in the 3×3and 5×5observa-tion modes.We then selected events with grades 0,2,3,4,and 6,and cleaned the data using the standard data selection criteria given in the Suzaku Data Reduc-tion Guide 3.We excluded the times that Suzaku passed through the South Atlantic Anomaly (SAA),and also times up to 436s after passage through the SAA.We also excluded times when Suzaku ’s line of sight was el-evated less than 10◦above the Earth’s limb and/or was1/lheasoft 2/ciao3/docs/suzaku/analysis/abc/abc.htmlOBSERVING CHARGE EXCHANGE WITH SUZAKU AND XMM-NEWTON3TABLE1Details of Our Suzaku ObservationsObservation l b Start time End time Usable exposureID(deg)(deg)(UT)(UT)(ks)Offfilament501001010278.71−47.072006-03-0116:56:012006-03-0222:29:1455.6Onfilament501002010278.65−45.302006-03-0320:52:002006-03-0608:01:1969.0less than20◦from the bright-Earth terminator.Finally,we excluded times when the cut-offrigidity(COR)wasless than8GV.This is a stricter criterion than that inthe Data Reduction Guide,which recommends exclud-ing times with COR<6GV.However,the higher CORthreshold helps reduce the particle background,and forobservations of the SXRB one desires as low a particlebackground as possible.The COR threshold that weuse has been used for other Suzaku observations of theSXRB(Fujimoto et al.2007;Smith et al.2007).Finally,we binned the2.5–8.5keV data into256-s time bins,andused the CIAO script analyzepublic4HENLEY AND SHELTON34:00.03:33:00.032:00.031:00.015:00.020:00.025:00.0-63:30:00.035:00.040:00.0Right ascensionD e c l i n a t i o nOn filament22:00.021:00.03:20:00.019:00.015:00.0-62:20:00.025:00.030:00.035:00.0Right ascensionD e c l i n a t i o nOff filamentFig.2.—Cleaned and smoothed Suzaku XIS1images in the 0.3–5keV band for our on-filament (left )and off-filament (right )observations.The data have been binned up by a factor of 4in the detector’s x and y directions,and then smoothed with a Gaussian whose standard deviation is equal to 1.5times the binned pixel size.The particle background has not been subtracted from the data.The red circles outline the regions that were excluded from the analysis (see text for details).in our fit (Snowden et al.1997).The R1and R2count-rates help constrain the model al lower energies (below ∼0.3keV),while the higher channels overlap in energy with our Suzaku spectra.We extracted the ROSAT spec-tra from 0.5◦radius circles centered on our two Suzaku pointing directions using the HEASARC X-ray Back-ground Tool 8v2.3.The spectral analysis was carried out using XSPEC 9v11.3.2(Arnaud 1996).For the thermal plasma com-ponents,we used the Astrophysical Plasma Emission Code (APEC)v1.3.1(Smith et al.2001)for the Suzaku data and the ROSAT R4–7bands,and the Raymond-Smith code (Raymond &Smith 1977and updates)for the ROSAT R1–3bands.For a given model component (i.e.,the LB or one of the two halo components),the tem-perature and normalization of the ROSAT Raymond-Smith model are tied to those of the corresponding Suzaku APEC model.We chose to use the Raymond-Smith code for the lower-energy ROSAT channels be-cause APEC’s spectral calculations below 0.25keV are inaccurate,due to a lack of data on transitions from L-shell ions of Ne,Mg,Al,Si,S,Ar,and Ca 10.As the upper-limit of the ROSAT R1and R2bands is 0.284keV,and the R3band also includes such low-energy photons (Snowden et al.1997),APEC is not ideal for fitting to these energy bands.For the absorption,we used the XSPEC phabs model,which uses cross-sections from Ba l uci´n ska-Church &McCammon (1992),with an updated He cross-section from Yan,Sadeghpour,&Dalgarno (1998).Following Henley et al.(2007),we used the interstellar chemical abundance table from Wilms,Allen,&McCray (2000).For many astrophysically abundant elements,these abundances are lower than those in the widely used solar abundance table of Anders &Grevesse (1989).However,recently several elements’solar photospheric abundances have been revised downwards (Asplund et al.2005),and are in good agreement with the Wilms et al.(2000)8/cgi-bin/Tools/xraybg/xraybg.pl9/docs/xanadu/xspec/xspec1110/atomdb/issuesOBSERVING CHARGE EXCHANGE WITH SUZAKU AND XMM-NEWTON5-4-2 0 2 40.3135channel energy (keV)(d a t a -m o d e l )/σ0.001 0.010.11c o u n t s s -1k e V-1On filamentLocal Bubble Halo (cold)Halo (hot)ExtragalacticInstrumental lines TotalO V I I O V I I IN e I XM g X I ?A l KS i KA u M -4-2 0 2 40.3135channel energy (keV)(d a t a -m o d e l )/σ0.0010.010.11c o u n t s s -1k e V-1Off filamentLocal Bubble Halo (cold)Halo (hot)ExtragalacticInstrumental lines TotalN V I I ?O V I I O V I I IN e I XA l KS i KA uMFig.3.—Our observed on-filament (left )and off-filament (right )Suzaku spectra,with the best-fitting model obtained by fitting jointly to the Suzaku and ROSAT data (Model 1in Table 7).The gap in the Si K instrumental line is where channels 500–504have been removed from the data (see §2).10100100010-6 c o u n t s s -1 a r c m i n-2-4-2 0 2 4R1R2R3R4R5R6R7ROSAT band(d a t a - m o d e l ) / σFig. 4.—The on-filament (dashed )and off-filament (solid )ROSAT All-Sky Survey spectra,compared with Model 1from Ta-ble 7.For clarity the individual model components have not been plotted.CALDB.This model component attenuated the emis-sion from the LB,halo,and extragalactic background for the Suzaku spectra only.We adjusted the model oxygen abundance to give C /O =6(Koyama et al.2007),and set the abundances of all other elements to zero.The results of this model are given as Model 2in Table 7.Figures 5and 6show this model com-pared with the Suzaku and ROSAT spectra,respec-tively.One can see from Figure 6that the fit to the ROSAT data is greatly improved.The model implies a column density of carbon atoms,N C =(0.28±0.04)×1018cm −2,in addition to the amount of contamina-tion given by the CALDB contamination model,which is N C =3.1×1018cm −2at the center of the XIS1chip(from the CALDB file aecontami xrtxis08.html6HENLEY AND SHELTON-4-2 0 2 40.3135channel energy (keV)(d a t a -m o d e l )/σ0.0010.010.1c o u n t s s -1k e V-1On filamentLocal Bubble Halo (cold)Halo (hot)ExtragalacticInstrumental lines Total-4-2 0 2 40.3135channel energy (keV)(d a t a -m o d e l )/σ0.0010.010.1c o u n t s s -1k e V-1Off filamentLocal Bubble Halo (cold)Halo (hot)ExtragalacticInstrumental linesTotalFig. 5.—As Figure 3,but with a vphabs component included for the Suzaku spectra to model XIS contamination above that included in the CALDB (Model 2in Table 7;see §3.2for details).10 100100010-6 c o u n t s s -1 a r c m i n-2-4-2 0 2 4R1R2R3R4R5R6R7ROSAT band(d a t a - m o d e l ) / σFig.6.—As Figure 5,but for Model 2from Table 7.In Model 5we fit exactly the same model to the Suzaku data alone.Without the ROSAT data,we cannot con-strain the LB temperature T LB .We therefore fix it at the value determined in Model 2:T LB =105.98K.We also fix N C for the vphabs contamination component at the Model 2value:N C =0.28×1018cm −2.The best-fitting model parameters are in very good agree-ment with those obtained by fitting jointly to the Suzaku and ROSAT spectra (compare Models 2and 5).We can only get consistent results between the Suzaku -ROSAT joint fit and the fit to just the Suzaku data by using a two-temperature model for the halo.However,note that the Model 5LB emission measure is consistent (within its errorbar)with zero.This is not to say that our data imply that there is no LB at all:as noted above,we need a LB component and two halo components to get a good joint fit to the Suzaku and ROSAT data.Instead,the Model 5results imply that our Suzaku data are consis-tent with the LB not producing significant emission in the Suzaku band (i.e.,E 0.3keV).Also shown in Table 7are the results of fitting our model (without any LB component)to the on-and off-filament Suzaku spectra individually (Models 6and 7,respectively).As has already been noted,the normaliza-tion of the extragalactic background differs significantly between the two spectra.However,the plasma model pa-rameters for the individual spectra are in good agreement with each other.These results seem to justify allowing the extragalactic normalization to differ between the two spectra while keeping all other model parameters equal for the two spectra.3.3.Chemical Abundances in the HaloWe investigated the chemical abundances of the X-ray-emissive halo gas by repeating the above-described mod-eling,but allowing the abundances of certain elements in the halo components to vary.In particular we wished to investigate whether or not varying the neon and magne-sium halo abundances improved the fits to the Ne ix and Mg xi features noted above.We also allowed the abun-dance of iron to vary,as iron is an important contributor of halo line emission to the Suzaku band.For this investigation we just fit to the Suzaku data,fixing the LB temperature at log(T LB /K)=5.98,and fixing the carbon column density of the vphabs contam-ination component at N C =0.28×1018cm −2.As we could not accurately determine the level of the contin-uum due to hydrogen,we could not measure absolute abundances.Instead,we estimated the abundances rel-ative to oxygen by holding the oxygen abundance at its Wilms et al.(2000)value,and allowing the abundances of neon,magnesium,and iron to vary.Both halo com-ponents were constrained to have the same abundances.The best-fitting temperatures and emission measures of the various model components are presented as Model 8in Table 7,and the abundances are presented in Table 2.The best-fitting model parameters are not significantly affected by allowing certain elements’abun-dances to vary (compare Model 8with Model 5).Iron does not seem to be enhanced or depleted relative to oxy-gen in the halo.Neon and magnesium both appear to be enhanced in the halo relative to oxygen,which is what one would expect from Figures 3and 5,as the modelsOBSERVING CHARGE EXCHANGE WITH SUZAKU AND XMM-NEWTON7 TABLE2Halo AbundancesElement AbundanceO1(fixed)Ne a1.8±0.4Mg a4.6+3.5−2.8Fe1.2+0.4−0.5Note.—Abun-dances are relative tothe Wilms et al.(2000)interstellar abundances:Ne/O=0.178,Mg/O=0.051,Fe/O=0.055.a These enhanced abun-dances may be an arte-fact of SWCX contami-nation;see§7.2.shown in thosefigures underpredict the neon and mag-nesium emission.We discuss these results in§§7.2and§7.5.In§7.2we discuss the possibility that the enhanced neon and mag-nesium emission is in fact due to SWCX contamination of these lines,rather than being due to these elements being enhanced in the halo.On the other hand,in§7.5 we discuss the implications of neon really being enhanced in the halo with respect to oxygen and iron.PARING THE SUZAKU AND XMM-NEWTONSPECTRAFor comparison,Table7also contains the results of the analysis of the XMM-Newton spectra from the same ob-servation directions by Henley et al.(2007).The Model9 results are taken directly from their“standard”model. However,it should be noted that Henley et al.(2007) used APEC to model all of their data,whereas in the analysis described above we used the Raymond-Smith code to model the ROSAT R1–3bands.We have therefore reanalyzed the XMM-Newton+ROSAT spec-tra,this time using the Raymond-Smith code to model the ROSAT R1–3bands,and using APEC to model the ROSAT R4–7bands and the XMM-Newton spec-tra.This new analysis allows a fairer comparison of our XMM-Newton results with our Suzaku results.The XMM-Newton spectra we analyzed are identical to those used by Henley et al.(2007)–see that paper for details of the data reduction.We added a broken power-law to the model to take into account soft-proton contamination in the XMM-Newton spectra.This broken power-law was not folded through XMM-Newton’s effective area, and was allowed to differ for the on-and off-filament datasets(see Henley et al.2007).The presence of this contamination means we cannot independently constrain the normalization of the extragalactic background.We therefore freeze the on-and off-filament normalizations at the Suzaku-determined values.The results of this new analysis are presented as Model10in Table7.As can be seen,there is poor agreement between the best-fit parameters of the Suzaku +ROSAT model(Model2)and the XMM-Newton+ ROSAT model(Model10).We believe this discrepancy is due to an extra emission component in the XMM-Newton spectra.In Figure7we plot the differences between the XMM-Newton spectra and our best-fitting0.10.20.30.40.5data-model(ctss-1keV-1)On filament MOS 1MOS 2 00.10.20.30.40.50.5125channel energy (keV)Off filament MOS 1MOS 2Fig.7.—The excesses in our on-filament(top)and off-filament (bottom)XMM-Newton spectra over our best-fitting model to the Suzaku+ROSAT data(Table7,Model2).The gap in the data between1.4and1.9keV is where two bright instrumental lines have been removed.Suzaku+ROSAT model(Model2from Table7).To our best-fitting Suzaku+ROSAT model we have added a broken power-law to model the soft-proton contami-nation in the XMM-Newton spectra.The parameters of this broken power-law are frozen at the values de-termined from thefitting to the XMM-Newton spectra described in the previous paragraph.The on-filament XMM-Newton spectra show excess line emission at∼0.57 and∼0.65keV,most likely due to O vii and O viii,re-spectively.The features in the off-filament spectra are not as clear.However,there appears to be excess O vii emission in the MOS1spectrum,and excess emission at ∼0.7keV(of uncertain origin)and∼0.9keV(proba-bly Ne ix)in the MOS2spectrum.We can estimate the significance of the excess emission by calculatingχ2 for the XMM-Newton data compared with the Suzaku +ROSAT model.We concentrate on the excess oxy-gen emission and calculateχ2for the0.5–0.7keV energy range.Wefindχ2=106.28for24degrees of freedom for the on-filament spectra,andχ2=43.03for22degrees of freedom for the off-filament spectra.These correspond toχ2probabilities of2.5×10−12and0.0047,respectively, implying that the excesses are significant in both sets of spectra at the1%level.We measure the intensities of the extra oxygen emis-sion byfittingδ-functions at E=0.570keV and 0.654keV to the excess spectra in Figure7.Wefit theseδ-functions simultaneously to the on-and off-filament XMM-Newton excess spectra.The intensities of the excess oxygen emission in the XMM-Newton spec-tra over the best-fitting Suzaku+ROSAT model are 3.8±0.5L.U.(O vii)and1.4±0.3L.U.(O viii).We believe that this excess oxygen emission is due to SWCX contamination in our XMM-Newton spectra.As noted in the Introduction,this was not taken into ac-count in the original analysis of the XMM-Newton spec-tra.This is because the solar windflux was steady and slightly below average during the XMM-Newton obser-vations,leading Henley et al.(2007)to conclude that SWCX contamination was unlikely to be significant.We discuss the SWCX contamination in our spectra further in§6.5.MEASURING THE OXYGEN LINES8HENLEY AND SHELTONAs well as using the above-described method to sep-arate the LB emission from the halo emission,we mea-sured the total intensities of the O vii complex and O viii line at∼0.57and∼0.65keV in each spectrum.These lines are a major component of the SXRB,accounting for the majority of the observed ROSAT R4diffuse back-ground that is not due to resolved extragalactic discrete sources(McCammon et al.2002),and are easily the most prominent lines in our Suzaku spectra.To measure the oxygen line intensities,we used a model consisting of an absorbed power-law,an absorbed APEC model whose oxygen abundance is set to zero,and two δ-functions to model the oxygen lines.As in the pre-vious section,the power-law models the extragalactic background,and its photon index was frozen at1.46 (Chen et al.1997).The APEC model,meanwhile,mod-els the line emission from elements other than oxygen, and the thermal continuum emission.The absorbing columns used were the same as those used in the ear-lier Suzaku analysis.As with our earlier analysis,we multiplied the whole model by a vphabs component to model the contamination on the optical blockingfilter which is in addition to that included in the CALDB con-tamination model(see§3.2).Wefix the carbon column density of this component at0.28×1018cm−2(Table7, Model2).Wefit this model simultaneously to our on-and off-filament spectra.However,all the model param-eters were independent for the two directions,except for the oxygen line energies–these were free parameters in thefit,but were constrained to be the same in the on-and off-filament spectra.We used essentially the same method to measure the oxygen line intensities in our XMM-Newton spectra.However,we did not use a vphabs contamination model,and,as before,we added a broken power-law to model the soft-proton contami-nation.Table3gives the energies and total observed intensities of the O vii and O viii emission measured from our Suzaku and XMM-Newton spectra.We can use the difference in the absorbing column for the on-and off-filament directions to decompose the ob-served line intensities into foreground(LB+SWCX)and background(halo)intensities.If I fg and I halo are the in-trinsic foreground and halo line intensities,respectively, then the observed on-filament intensity I on is given byI on=I fg+e−τon I halo,(1) whereτon is the on-filament optical depth at the energy of the line.There is a similar expression for the observed off-filament intensity I off,involving the off-filament op-tical depthτoff.These expressions can be rearranged to giveI fg=eτon I on−eτoff I offe−τon−e−τoff.(3) For the purposes of this decomposition,we use the Ba l uci´n ska-Church&McCammon(1992)cross-sections (with an updated He cross-section;Yan et al.1998)with the Wilms et al.(2000)interstellar abundances.We use the cross-sections at the measured energies of the lines. For the Suzaku measurements,the cross-sections we use are7.17×10−22cm2for O vii(E=0.564keV)and 4.66×10−22cm2for O viii(E=0.658keV).For the XMM-Newton O vii emission we use a cross-section of 7.03×10−22cm2(E=0.568keV).We cannot decom-pose the XMM-Newton O viii emission because the on-filament O viii line is brighter than the off-filament line. This gives rise to a negative halo intensity,which is un-physical.The results of this decomposition are presented in Ta-ble4.Note that the foreground oxygen intensities mea-sured from the Suzaku spectra are consistent with zero. This is consistent with our earlierfinding that the Suzaku spectra are consistent with there being no local emis-sion in the Suzaku band(see§3.2).The difference be-tween the foreground O vii intensities measured from our XMM-Newton and Suzaku spectra is5.1±3.1L.U.. This is consistent with the O vii intensity measured from the excess XMM-Newton emission over the best-fitting Suzaku+ROSAT model(3.8±0.5L.U.;see§4).The halo O vii intensities measured from our XMM-Newton and Suzaku spectra are consistent with each other.This is as expected,as we would not expect the halo intensity to significantly change in∼4years.6.MODELING THE SOLAR WIND CHARGE EXCHANGEEMISSIONIn§§4and5we presented evidence that our XMM-Newton spectra contain an extra emission component,in addition to the components needed to explain the Suzaku spectra.In particular,the O vii and O viii emission are enhanced in the XMM-Newton spectra.We attribute this extra component to SWCX emission,as it seems unlikely to be due to a change in the Local Bubble or halo emission.This extra component helps explain why our XMM-Newton and Suzaku analyses give such different results in Table7.Previous observations of SWCX have found that in-creases in the SWCX emission are associated with en-hancements in the solar wind,as measured by ACE. These enhancements consist of an increase in the pro-tonflux,and may also include a shift in the ionization balance to higher ionization stages(Snowden et al.2004; Fujimoto et al.2007).In§6.1,we present a simple model for heliospheric and geocoronal SWCX emission,and use contemporaneous solar wind data from the ACE and WIND satellites to determine whether or not the ob-served enhancement of the oxygen lines in the XMM-Newton spectra is due to differences in the solar wind between our two sets of observations.In addition to the variability associated with solar wind enhancements,the heliospheric SWCX intensity is also expected to vary during the solar cycle,due to the dif-ferent states of the solar wind at solar maximum and solar minimum(Koutroumpa et al.2006).As our two sets of observations were taken∼4years apart,at differ-ent points in the solar cycle,in§6.2we examine whether the SWCX intensity variation during the solar cycle can explain our observations.6.1.A Simple Model for Heliospheric and GeocoronalSWCX Emission6.1.1.The BasicsA SWCX line from a X+n ion of element X results from a charge exchange interaction between a X+(n+1) ion in the solar wind and a neutral atom.The intensity of that line therefore depends on the density of X+(n+1)。
First Light Measurements with the XMM-Newton Reflection Grating Spectrometers Evidence for

a r X i v :a s t r o -p h /0011018v 1 1 N o v 2000Astronomy &Astrophysics manuscript no.(will be inserted by hand later)First Light Measurements with the XMM-Newton Reflection Grating Spectrometers:Evidence for an Inverse First Ionisation Potential Effect and Anomalous Ne Abundance inthe Coronae of HR 1099A.C.Brinkman 1,E.Behar 2,M.G¨u del 3,M.Audard 3,A.J.F.den Boggende 1,G.Branduardi-Raymont 4,J.Cottam 2,C.Erd 5,J.W.den Herder 1,F.Jansen 5,J.S.Kaastra 1,S.M.Kahn 2,R.Mewe 1,F.B.S.Paerels 2,J.R.Peterson 2,A.P.Rasmussen 2,I.Sakelliou 4,and C.de Vries 11Space Research Organization of the Netherlands,Sorbonnelaan 2,3584CA Utrecht,The Netherlands2Columbia Astrophysics Laboratory,Columbia University,550West 120th Street,New York,NY 10027,USA 3Paul Scherrer Institute,W¨u renlingen and Villigen,CH-5235Switzerland4Mullard Space Science Laboratory,University College London,Dorking RH56NS,United Kingdom5Astrophysics Division,Space Science Department of ESA,ESTEC,2200AG Noordwijk,The NetherlandsReceived –;accepted –Abstract.The RS CVn binary system HR 1099was extensively observed by the XMM-Newton observatory in February 2000as its first-light target.A total of 570ks of exposure time was accumulated with the Reflection Grating Spectrometers(RGS).The integrated X-ray spectrum between 5–35˚Ais of unprecedented quality and shows numerous features attributed to transitions of the elements C,N,O,Ne,Mg,Si,S,Fe,and Ni.We perform an in-depth study of the elemental composition of the average corona of this system,and find that the elemental abundances strongly depend on the first ionisation potential (FIP)of the elements.But different from the solar coronal case,we find an inverse FIP effect ,i.e.,the abundances (relative to oxygen)increase with increasing FIP .Possible scenarios,e.g.,selective enrichment due to Ne-rich flare-like events,are discussed.Key words.atomic processes —line:formation —nuclear reactions,nucleosynthesis,abundances —stars:coronae —stars:individual (HR 1099)—X-rays:stars1.IntroductionThe outer atmospheres of stars are of fundamental importance to the chemical enrichment of interstellar space through stel-lar winds and the ejection of energetic particles (Meyer 1985).Interestingly,the elemental composition of the hot magnetic so-lar corona and the solar wind are markedly different from the Sun’s surface composition,which suggests that some fraction-ation process in the denser layers of the solar atmosphere must selectively enrich the corona with particular elements.Various observations of the solar corona,the solar wind,solar energetic particles,and cosmic rays have established that elements with a first ionisation potential (FIP)below 10eV are overabundant by factors of up to 4with respect to the solar photosphere,unlike elements with a high FIP that maintain their photospheric abun-dance (Feldman 1992,see also Meyer 1985).This is usually re-ferred to as the “FIP effect”.However,the physics of this frac-tionation mechanism is poorly parative abun-dance studies of stars other than the Sun may shed light on the underlying physics.2 A.C.Brinkman et al.:XMM-Newton RGS observations of HR1099Fig.1.First order spectrum of HR1099as measured by the RGS instruments onboard XMM-Newton.The most promi-nent spectral features are indicated justabove the spectrum by the correspond-ing emitting ion.present observation is HR1099,one of the X-ray brightest and most magnetically active stellar systems,which was observed early in the programme.HR1099has been the target of previ-ous EUVE and low-resolution X-ray spectroscopic investiga-tions(e.g.Pasquini et al.1989,Griffith&Jordan1998).Ayres et al.(2001)qualitatively compare the Chandra HETG spec-tra of HR1099and Capella,the latter being a somewhat cooler and less active coronal source.A companion paper(Audard et al.2001)to the present work discusses spectral variability in HR1099,based on selected XMM-Newton RGS data sets. 2.Observations and AnalysisThe XMM-Newton X-ray observatory incorporates a pay-load with two identical high-resolution Reflection Grating Spectrometers(RGS;den Herder et al.2001)with a spectral resolution of about0.06˚A FWHM.The spectral band cov-ered by the RGS ranges from5to35˚A.This range contains strong lines of C,N,O,Ne,Mg,Si,S,Ca,Fe,and Ni.The clearly detected eight consecutive charge states of Fe are of crucial importance for the present analysis.HR1099was ob-served in January and February2000for a total of570ksec yielding approximately1.3million source counts.The spec-trum was extracted by counting events inside a narrow spatial window along the dispersed CCD image,and then by using the CCD energy resolution to separate the spectral orders.The ex-tracted totalfirst-order spectrum between7and35˚A is shown in Fig.1.3.ResultsIn optically thin coronal plasmas,the power of a particular spectral line depends on the collisional and radiative transition rates.In this work,these quantities are calculated by means of the relativistic Hebrew University Lawrence Livermore Atomic Code(Bar-Shalom et al.1998).Owing to the high spectral resolution,we can directly scale the contribution of each ion species to match the observation.The atomic-level models are each calculated for a single electron temperature,T max, at which the relevant ion has its maximum abundance.Fig.2 shows the theoretical spectrum from7–18˚A(red curve),which also includes a phenomenological continuum component(blue) corresponding to a bremsstrahlung spectrum(which dominates over any other continuum components at the temperatures in question),compared with the HR1099data points(black).As can be seen,the agreement is very good.The majority of lines in this region are due to highly ionised Fe as demonstrated by the individual ion curves at the bottom of thefigure.The con-tribution of each ion species yields a direct measurement of the amount of coronal gas in the conditions appropriate for that ion.With the distance d(=29pc)to HR1099,the calculated line power and the measured lineflux can be used to ob-tain the emission measure EM(in cm−3),which is a product of the electron density and the H-ion density,integrated over the emitting volume.We assume that each ion emits mostly around the temperature T max.This leads to afirst approxi-mation of the emission measure distribution.This distribution is sufficiently accurate for our purposes if no steep gradients are present.Finally,in order to plot the EM,we use the ionic abundances from ionisation balance calculations(Arnaud& Raymond1992,Mazotta1998)and assume,as afirst step,ele-mental abundances of the solar photosphere.The resulting EM as a function of the electron temperature T e is shown in Fig.3 for ions of C,N,O,Ne,Mg,S,Fe,and Ni.These ions sample the HR1099hot coronae in the T e range from kT e=100eV to1500eV and illustrate the monotonic increase of the EM in this range.The errors on the temperature for each ion represent the range of T e for which the ion fraction exceeds50%of its maximum value,except for the He-like ions O6+,Ne8+,and Mg10+,for which line ratio methods(Gabriel&Jordan1969) are used to determine T e more precisely and the bars reflect a 25%uncertainty.HR10993Fig.2.Observed HR1099flux spec-trum(black dots)compared with thetotal calculated spectrum(red curve).The inferred thermal bremsstrahlungcontinuum is represented by the bluecurve.The eight separate plots at thebottom show the relative individual-ioncontributions of Fe16+to Fe23+(not toscale).The non-Fe lines(O,Ne,Mg,and Ni)are indicated explicitly.Table1.Abundance estimates a of the HR1099coronae,Solar photosphere b and Solar corona c relative to ONe21.560.570.150.15 1.0 3.8N14.530.170.120.13 1.08 1.4O13.61 1.0 1.0 1.0 1.0 1.0C11.260.280.470.50 1.060.59S10.360.00850.0190.024 1.260.45Fe7.870.00940.0380.16 4.210.25Mg7.640.0430.0450.18 4.00.95Ni7.630.000700.00210.0089 4.240.334 A.C.Brinkman et al.:XMM-Newton RGS observations of HR 10993.The empirical emission measure O 6+,O 7+,Ne 8+,Ne 9+,Mg 10+,11+,Fe 16+through Fe 23+,Ni 18+,5+,N 6+and S 13+(in order from left right for each element).The straight is the best-fit power-law for the Fealone.Fig.4.Enrichment of elemental abun-dances in the HR 1099coronae rela-tive to (solar)photospheric values,plot-ted as a function of the first ionisation potential (FIP).All values are given error bars of 20%.Note the increase with FIP,exactly opposite the trend ob-served in the average solar corona.the respective ratios of the solar corona,the contrast is even sharper.In fact,HR 1099reveals systematically a reversed FIP effect,which is illustrated in Fig.4;the coronal enrichment in-creases monotonically with the FIP.The only exception may be Mg (low FIP).Ne is strongly enhanced,by a factor of 3.8compared to the solar photosphere.This enhancement is rem-iniscent of a similar anomaly observed in a subset of solar flares (Schmelz 1993,Murphy et al.1991).Interestingly,flares are thought to be of great importance to the heating of ap-parently quiet coronae of active stars (Doyle &Butler 1985,G¨u del 1994,Audard et al.2000)and have been suggested to be pivotal for the elemental fractionation process as well (Wang1996,G¨u del et al.1999,Mewe et al.1997).Continuous mix-ing of photospheric and coronal material in low-lying loops through frequent flares could suppress the solar-like FIP effect (Feldman &Widing 1990)in these stars altogether.It is generally believed that the fractionation is the result of diffusive processes across magnetic field lines somewhere in the chromosphere,where the ionisation fraction differs sub-stantially among elements of high or low FIP (von Steiger &Geiss 1989).Low FIP elements can then be accelerated into the quiescent corona,leaving an excess of high FIP elements in the chromosphere (Schmelz 1993).If this chromospheric layer can be accessed by flares (Schmelz 1993),then a high-FIP-A.C.Brinkman et al.:XMM-Newton RGS observations of HR10995rich plasma may be heated and lifted into dense coronal X-ray loops giving rise to the reversed coronal FIP effect observed in HR1099.In any case,it is clear that the simple mechanism in-volving steady-state diffusion,which has been proposed for the quiet solar corona,does not operate in more active stars such as HR1099.Since cosmic rays do show a low FIP enhancement, it is unlikely that such active stars can be major contributors to the cosmic rayflux.The wide survey of stellar coronae planned with the RGS on XMM-Newton will provide more general an-swers to the questions raised by these observations. Acknowledgements.We thank the many teams in industry and at the scientific institutions and ESA,for building such an excellent space-craft and payload-and operating it.We are particularly grateful to Mr.Robert Lain´e and his project team at ESA for leading the project.We thank the XMM-Newton SOC staff for making the com-missioning phase data available to us.SRON isfinancially supported by the Netherlands Organization for Scientific Research(NWO). The Columbia University team acknowledges generous support from the National Aeronautics and Space Administration.The PSI group is supported by the Swiss Academy of Natural Sciences and the Swiss National Science Foundation(grants2100-049343and2000-058827).MSSL acknowledges support from the Particle Physics and Astronomy Research Council.This work is based on observations ob-tained with XMM-Newton,an ESA science mission with instruments and contributions directly funded by ESA member states and the USA (NASA).ReferencesAntunes,A.,Nagase,F.,White,N.E.1994,ApJ,436,L83 Arnaud,M.,Raymond,J.1992,ApJ,398,394Audard,M.,G¨u del,M.,Drake,J.J.,Kashyap,V.2000,ApJ,in press Audard,M.,G¨u del,M.,Mewe,R.2001,A&A,365(this issue) Ayres,T.R.,Brown,A.,Osten,R.A.,Huenemoerder,D.P.,Drake,J.J., Brickhouse,N.S.,Linsky,J.L.2001,ApJ,in pressBar-Shalom,A.,Klapisch,M.,Goldstein,W.H.,Oreg,J.1998,The HULLAC Code for Atomic Physics,unpublishedden Herder,J.W.,Brinkman,A.C.,Kahn,S.M.,et al.2001,A&A,365 (this issue)Doyle,J.G.,Butler,J.1985,Nature,318,378Drake,J.J.,Laming,J.M.,Widing,K.G.1995,ApJ,443,393 Drake,J.J.,Laming,J.M.,Widing,K.G.1997,ApJ,478,403 Drake,S.A.1996,in:X-ray Measurements of Coronal Abundances, 6th Ann.Astrophysics Conference,ASP Conf.Proc.,S.S.Holt,G.Sonneborn(eds.),San Francisco:ASP,p.215Feldman,U.1992,Phys.Scripta46,202Feldman,U.,Mandelbaum,P.,Seely,J.F.,Doschek,G.A.,Gursky,H.1992,ApJS,81,387Feldman,U.,Widing,K.G.1990,ApJ,363,292Gabriel,A.H.,Jordan,C.1969,MNRAS,145,241G¨u del,M.1994,ApJS,90,743G¨u del,M.,Linsky,J.L.,Brown,A.,Nagase,F.1999,ApJ,511,405 H´e noux J.-C.1995,Adv.Space Res.,15,23Mazzotta,P.,Mazzitelli,G.,Colafrancesco,S.,Vittorio,N.1998, A&ASS,133,403Meyer,J.P.1985,ApJ,57,173Mewe,R.,Kaastra,J.S.,van den Oord,G.H.J.,Vink,J.,Tawara,Y.1997,A&A,320,147Murphy,R.J.,Ramaty,R.,Kozlovsky,B.,Reames,D.V.1991,ApJ, 371,793Pasquini,L.,Schmitt,J.H.M.M.,Pallavicini,R.1989,A&A,226,225Randich,S.,Giampapa,M.S.,Pallavicini,R.1994,A&A,283,893 Schmelz,J.T.1993,ApJ,408,373von Steiger,R.,Geiss,J.1989,A&A,225,222Wang,Y.-M.1996,ApJ,464,L91White,N.E.1996,in:Cool stars,Stellar Systems and the Sun,ASP Conf.Series,109,p.193。
脉冲星测量技术用于深空探测器自主导航的原理及方法

脉冲星测量技术用于深空探测 器自主导航的原理及方法
1/76
脉冲星的发现
2/76
脉冲星机制
当一颗恒星变成超新星时,经过激烈变化后,留下满天膨胀的气体和微 小物质,余下的核心直径只有几十到十几公里。超新星的内爆非常强烈,恒 星原子里的质子和电子被紧紧地压缩 在一起,抵消了它们的电荷,形成 中子。这种中子星可以达到水密度 的1014倍,有着极强的磁场,可以 非常快速地旋转。因为磁轴不与旋 转轴重合,二者一般具有一定的 夹角,当脉冲星高速旋转时,辐 射束将沿着磁场两极方向被抛出 ,随着脉冲星的自转,该辐射束 周期性扫过探测器的视界,形成 脉冲。
11/76
脉冲星导航技术研究历程
12/76
研究历程
a) 脉冲星导航思想的萌芽阶段 1. 脉冲星导航思想最早于 20 世纪 70 年代提出。 2. 1971 年,Reichley,Downs 和Morris 首次描述了把射电脉冲星作为时钟的思 想。 3. 1974 年,Downs 在文献《Interplanetary Navigation Using Pulsation Radio Source》中提出一种基于射电脉冲星信号进行行星际导航的思想,标志着脉 冲星导航思想的初步形成。但由于脉冲星的射电信号强度较弱,宇宙中的射 电信号噪声强度大,导航中需要至少 25m 口径的天线接收信号,因此该方法 很难在工程中实现。 4. 20 世纪 70 年代后期,天文观测在 X 射线波段能量范围 1~20keV、频率范围 2.5×1017 ~ 4.8×1017Hz的进展,促进了对 X 射线脉冲星特性的研究。 5. 1980 年 Downs 和 Reichley 提出测量脉冲星脉冲到达时间的技术。 1981 年 Chester 和Butman 在国际上第一次正式提出利用 X 射线脉冲星进行航天器导 航的思想。
MCG-6-30-15的铁Kα线的研究
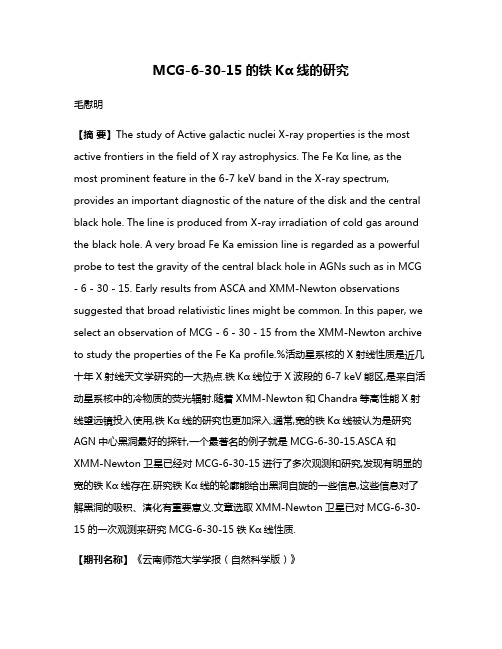
我们用XSPEC软件拟合观测能谱。为了应用Ⅹ2检验,我们首先使能谱的每一个能量 bin里至少包含有20个光子。以下的拟合包含了银河系的吸收(各个源在视线方向上的中性氢柱密度可由 Heasoft软件包中全天中性氢分布得到[8]),还加上了源本征的吸收。
射电宁静AGNs的X射线能谱通常包含一个或多个连续谱成分以及一些吸收和发射的特征。MCG-6-30-15也同样如此。由于软 X射线(0.3-2ke V)波段的能谱比较复杂,我们首先拟合2-10 Ke V波段的能谱,通常一个简单的幂律谱能较好地拟合此能段的数据,如图1所示。从图1可看出,在6—7 Ke V能段有明显的的“线状”的剩余,即铁Kα线。拟合得到的参数分别为:幂律谱指数 T=1.84±0.04,Reduced chi-squared(Ⅹ2)=1.72(大于1.2,拟合不能接受)。当把幂律模型外推到0.3-2.0Ke V能段时,我们发现在软X射线能段有很强的超出,就是我们通常说的“软X射线超”或“软X射线过剩(soft X-ray excess)”,如图2所示。我们用一个黑体成分来代表“软X射线超”。拟合结果如图3所示,从图上可以看出,在软X射线波段,有明显的吸收。拟合得到黑体温度k T=0.09±0.02 Ke V,幂律谱指数T=1.69±0.02,Reduced chi-squared(Ⅹ2)=1.30。接下来的拟合中,我们加了几条吸收边以及一个高斯成分来代表铁Kα线。拟合结果如图4所示。从图4可看出,此拟合效果较好。拟合得到幂律谱指数T=1.85±0.04,黑体温度k T=0.13±0.005Ke V,铁线的线心能量(LineE)为6.37±0.02Ke V,线 宽 (Sig ma)为 0.18±0.02 Ke V。Reduced chi-squared(Ⅹ2)=1.15。
The XMM-Newton view of radio-quiet and X-ray dim isolated neutron stars
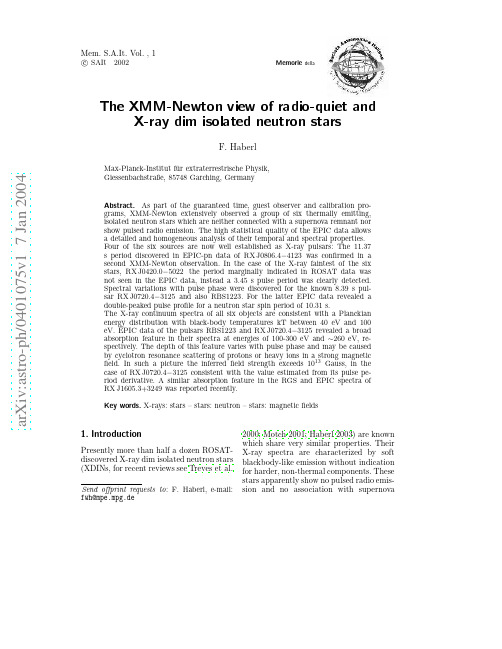
a r X i v :a s t r o -p h /0401075v 1 7 J a n 2004Mem.S.A.It.Vol.,1cSAIt 2002MemoriedellaF.HaberlMax-Planck-Institut f¨u r extraterrestrische Physik,Giessenbachstraße,85748Garching,GermanyAbstract.As part of the guaranteed time,guest observer and calibration pro-grams,XMM-Newton extensively observed a group of six thermally emitting,isolated neutron stars which are neither connected with a supernova remnant nor show pulsed radio emission.The high statistical quality of the EPIC data allows a detailed and homogeneous analysis of their temporal and spectral properties.Four of the six sources are now well established as X-ray pulsars:The 11.37s period discovered in EPIC-pn data of RX J0806.4−4123was confirmed in a second XMM-Newton observation.In the case of the X-ray faintest of the six stars,RX J0420.0−5022the period marginally indicated in ROSAT data was not seen in the EPIC data,instead a 3.45s pulse period was clearly detected.Spectral variations with pulse phase were discovered for the known 8.39s pul-sar RX J0720.4−3125and also RBS1223.For the latter EPIC data revealed a double-peaked pulse profile for a neutron star spin period of 10.31s.The X-ray continuum spectra of all six objects are consistent with a Planckian energy distribution with black-body temperatures kT between 40eV and 100eV.EPIC data of the pulsars RBS1223and RX J0720.4−3125revealed a broad absorption feature in their spectra at energies of 100-300eV and ∼260eV,re-spectively.The depth of this feature varies with pulse phase and may be caused by cyclotron resonance scattering of protons or heavy ions in a strong magnetic field.In such a picture the inferred field strength exceeds 1013Gauss,in the case of RX J0720.4−3125consistent with the value estimated from its pulse pe-riod derivative.A similar absorption feature in the RGS and EPIC spectra of RX J1605.3+3249was reported recently.Key words.X-rays:stars –stars:neutron –stars:magnetic fields1.IntroductionPresently more than half a dozen ROSAT-discovered X-ray dim isolated neutron stars (XDINs,for recent reviews see Treves et al.2 F.Haberl:Radio-quiet and X-ray dim isolated neutronstarsFig.1.EPIC-pn light curves folded on the pulse period for the four pulsars among the XDINs.remnants.Some of them exhibit pulsationsin their X-rayflux indicating the neutronstar rotation period.Although there is littledoubt that the soft X-rays are of thermalorigin from the surface of an isolated neu-tron star,the details for the formation ofthe spectrum are not clear.XMM-Newtonobserved six XDINs as part of the guaran-teed time,guest observer and calibrationprograms.Here I summarize some of thefirst results of a homogeneous analysis oftheir temporal and spectral properties.2.X-ray pulsationsThe second brightest of the known XDINsis RX J0720.4−3125and was thefirstdiscovered as X-ray pulsar(Haberl et al.1997)with a period of8.39s.XMM-Newton observed RX J0720.4−3125sixtimes.As example the folded EPIC-pnlight curve from satellite revolution534is drawn in Fig.1which shows a pulsedfraction of about11%.In Chandra dataof RBS1223=1RXS J130848.6+212708Hambaryan et al.(2002)discovered pulsa-tions with a period of5.16s and XMM-Newton observations revealed that the trueneutron star spin period is more likely10.31s.This is supported by pulse phase de-pendent hardness ratio variations whichare different for the two intensity max-ima(Haberl et al.2003).RBS1223ex-hibits the deepest modulation of the knownXDINs in its double peaked pulse profileof18%(Fig.1).Thefirst XMM-Newtonobservation of RX J0806.4−4123revealeda6%modulation(Fig.1)with a periodof11.37s(Haberl&Zavlin2002)whichwas confirmed in a second observation(Haberl et al.2004a).Four XMM-Newtonobservations of RX J0420.0−5022did notconfirm the22.7s pulsations originally in-dicated in ROSAT data,but clearly re-F.Haberl:Radio-quiet and X-ray dim isolated neutron stars3veal a3.45s period.The pulsed fraction is about12%(Fig.1).In Table1the de-rived pulse periods for the four pulsars are summarized together with pulse period derivatives assuming linear period changes between multiple XMM-Newton observa-tions.In most cases the time base line is too short to determine precise˙P values, only for RX J0720.4−3125observations by different satellites cover already more than 10years(Zane et al.2002;Kaplan et al. 2002).3.X-ray spectraThe X-ray spectra of XDINs obtained by the ROSAT PSPC were all consistent with black-body emission little attenuated by interstellar absorption suggesting that the objects are close-by.To look for ab-sorption features which may be created by heavy chemical elements in the stel-lar atmosphere high resolution spectra of RX J1856.4−3754were obtained by the LETGS on Chandra(Burwitz et al.2001, 2003).Surprisingly,no significant narrow features were detected.A spectral analysis(in a homogenous way using the latest calibration data)of the EPIC-pn spectra,which are of unprece-dented statistical quality,shows that in sev-eral cases a black-body model yields un-satisfactoryfits.The strongest deviations are seen from RBS1223and Haberl et al. (2003)demonstrate that a non-magnetic atmosphere models can neither explain the observed spectrum.However,it was found that adding an absorption feature mod-eled by a broad(100eV)Gaussian line at an energy between100and300eV to the Planckian continuum yields accept-ablefits.Similarly,but at a higher en-ergy of450eV and therefore inside the sensitive band of the RGS instruments van Kerkwijk(2004)presented the detec-tion of a broad absorption feature in the spectra of RX J1605.3+3249.Haberl et al. (2004b)report a broad absorption fea-ture in the EPIC-pn spectra of the pul-sar RX J0720.4−3125at an energy of270eV andfind that the depth of the feature varies with pulse phase by a factor of∼2. Finally,also spectra of RX J0420.0−5022 indicate a possible absorption line at330 eV(Haberl et al.2004a).In Table1the spectral parameters in-ferred from thefits using the two mod-els(absorbed black-body and absorbed black-body with Gaussian-shaped absorp-tion line)are listed.Thefirst value given for column density N H and black-body tem-perature kT refers to the model without ab-sorption line and the second to the model with line added.4.DiscussionFour of the six X-ray-dim isolated neu-tron stars are now known as X-ray pul-sars with spin periods between3.45s and 11.37s.The fraction of pulsedflux in their folded X-ray light curves ranges between 6%and18%.RBS1223,RX J0720.4−3125 and RX J0420.0−5022show hardness ratio variations with pulse phase(Haberl et al. 2003,2004b,a)while for RX J0806.4−4123 the shallow modulation makes the signifi-cant detection of such an effect more diffi-cult(Haberl et al.2004a).Pulse phase re-solved spectra for thefirst three pulsars show that the temperature changes only marginally with pulse phase.Any model for the pulsed emission from this group of iso-lated neutron stars must be able to explain this behaviour.An important piece of information comes from the detection of broad ab-sorption features in the X-ray spectra of several XDINs.At least in the case of RX J0720.4−3125the depth of the feature varies strongly with pulse phase.A likely interpretation of these features is cyclotron resonance absorption which can be ex-pected in spectra from magnetized neutron stars withfield strength B in the range of 1010–1011G or2×1013–2×1014G if caused by electrons or protons,respectively.In the case of RX J0720.4−3125the measured˙P, if interpreted as magnetic dipole braking, rules out the lower range for B,leaving pro-4 F.Haberl:Radio-quiet and X-ray dim isolated neutron starsTable1.X-ray-dim isolated neutron stars observed by XMM-NewtonRX J0420.0−5022 3.453<9×10−12 1.0/2.045/45330?6? RX J0720.4−31258.391(3−6)×10−14 1.4/0.885/842705 RX J0806.4−412311.371<2×10−120.496−RBS122310.313<6×10−127.1/4.195/86100-3002-6 RX J1605.3+3249−−0.3/0.996/934509 RX J1856.5−3754−−0.960−1?F.Haberl:Radio-quiet and X-ray dim isolated neutron stars5 2002,MNRAS,334,345。
What can XMM-Newton tell us about the spin periods of Intermediate Polars

a r Xiv:as tr o-ph/49638v223Nov24The astrophysics of cataclysmic variables and related objects ASP Conference Series,Vol.?,2004J.M.Hameury &sota What can XMM-Newton tell us about the spin periods of Intermediate Polars?P.A.Evans &Coel Hellier Astrophysics Group,School of Chemistry and Physics,Keele University,Keele,Staffordshire,ST55BG Abstract.XMM-Newton ’s unprecedented combination of spectral resolution and high throughput allows us to perform the best phase-resolved X-ray analysis of intermediate polars to date.The Optical Monitor gives optical/UV photom-etry simultaneously with the X-ray data.We present a comprehensive study of X-ray spin pulses in IPs,giving spin-pulses and hardness ratios for every IP looked at with XMM-Newton to date.1.Introduction In an intermediate polar (IP)the white dwarf has a magnetic field strong enough to affect the accretion flow,but not strong enough to synchronise the white dwarf rotation with the binary period;the magnetic dipole is also inclined to the spin axis of the star.Generally accretion occurs from a magnetically truncated accretion disc;at some radius the disc material threads to the white dwarf’s field lines and is channeled towards the gravitationally preferable pole in large accretion curtains.Stand-offshocks form above each pole,resulting in hard X-ray emission.As the white dwarf rotates,our view of these regions changes and the position of the accretion curtains relative to our line of sight also varies,giving rise to spin-period modulations (e.g.Hellier,Cropper,&Mason 1991).To date eleven IPs have been observed with XMM-Newton .In this paper we present a compilation of X-ray spin-pulse profiles,hardness ratios,and UV data from XMM ’s Optical Monitor (OM)for these systems.2.AO Piscium and V1223SagittariiAO Psc and V1223Sgr show sinusoidal X-ray and UV modulations (Fig.1).The fact that their spectra get harder at pulse minimum suggests that the modulation is caused by absorption.These are thus good examples of the accretion curtain model for X-ray modulation in IPs (e.g.Hellier et al.1991).3.HT CamelopardarisHT Cam’s X-ray spin-pulse (Fig.2)appears sinusoidal with a flattened maxi-mum,and,judged from the softness ratio,appears to be energy independent.No pulse is detected in the UV,although the B -band shows sinusoidal variation.For more information,see de Martino et al.(2004b).12Evans and HellierFigure1.The spin pulses of AO Psc(left)and V1223Sgr(right).We showthe0.2–12keV X-ray data,the(0.2–4)/(6–12)keV softness ratio and(whereavailable)the UV(2050–2450˚A)data.4.RX J1548.2−4528The recently discovered IP RX J1548.2−4528shows a sinusoidal X-ray spin-pulse(Fig.2).Haberl,Motch&Zickgraf(2002)analysed this observation,and suggested that while there may be a small change in absorption with spin phase, the predominant source of modulation is a change in the visibility of the emission.5.EX HydraeEX Hya’s softness ratio has the same shape and phasing as the lightcurve (Fig.3),making it tempting to interpret this modulation as changing absorption in the accretion curtains(indeed,it was for this system that accretion curtains werefirst proposed as the cause of X-ray modulation;Rosen,Mason,&C´o rdova 1988).However,various X-ray studies(e.g.Allan,Hellier,&Beardmore1998) have suggested that the upper emitting pole is periodically occulted by the white dwarf,giving rise to the modulation.Since such occultation will affect the lower, cooler parts of the accretion column more than the higher,hotter ones,we see a deeper modulation at lower energies.6.AE AquariiAE Aqr differs from many IPs as it is a rapid rotator(P spin∼33s),and thus its magneticfield might be expelling material from the system like a propeller(e.g., Eracleous&Horne1996).It is unclear whether the X-ray emission arises fromXMM-Newton IP spin pulses3Figure2.As for Fig.1,but for HT Cam(left)and RX J1548.2−4528(right). The UV band for HT Cam is1800–2250˚A,and the B band(3900–4900˚A)is also shown.Figure3.As Fig.1,but for EX Hya(left)and AE Aqr(right).4Evans and HellierFigure4.Left:As for Fig.1,but for GK Per.Right:Spin-pulse profile ofV405Aur.The upper panel shows the0.2–0.7keV band and the lower panelshows the0.7–12keV band.accretion,as is usual in IPs,or further out in the magnetosphere,as suggested by Ikhsanov(2001).However,the XMM-Newton data shows that the pulse profile is sinusoidal and largely independent of energy.7.GK Perseii(in outburst)Watson,King,&Osborne(1985)suggested that the X-ray spin-pulse of GK Per in outburst was caused by increased absorption at spin minimum.Ishida et al.(1992)blamed changing absorption for the profile in quiescence as well,but suggested that the outburst profile may show evidence for occultation of the upper pole.Fig.4shows that the outburst softness ratio follows the lightcurve, making it more likely that absorption changes are responsible for this pulse,as Watson et al.(1985)claimed.Hellier,Harmer,&Beardmore(2004)agree with this,in analysis of an RXTE observation.8.V405AurigaeV405Aur differs from IPs such as AO Psc,first because it shows a soft blackbody component to its X-ray emission(Haberl et al.1994),and second because the blackbody is double-peaked on the spin period,while the harder emission is single-peaked but sawtoothed(Fig.4).Furthermore,de Martino et al.(2004a) and Evans&Hellier(2004)have shown that the absorption does not change with spin phase.Evans&Hellier(2004)suggest that if the angle between the magnetic and spin axes in this system is very high,then the double-peaked profile of the blackbody emission is explained.XMM-Newton IP spin pulses5Figure5.Left:as Fig.1,but for FO Aqr.Right:PQ Gem spin folds forthe blackbody(upper panel),hard X-ray(lower panel)and UV(2450–3200˚A)components.9.FO AquariiThe X-ray pulse profile of FO Aqr(Fig.5)shows X-ray minimum to occur after UV minimum.Many authors(e.g.Hellier1993;Beardmore et al.1998) have identified the‘notch’at phase0.7as the result of occultation of the upper accretion column,and the dip around phase0.5as arising from the accretion curtains intercepting our line of sight.Evans et al.(2004)note that,if this is the case,the upper pole will be pointed towards the observer nearly a quarter of a cycle before the accretion curtain dip.They thus suggest that the accretion curtains are twisted,explaining the lightcurve and softness ratio.10.PQ GeminorumPQ Gem shows a soft blackbody component as well as the hard,optically thin emission characteristic of IPs(Mason et al.1992).The spin-pulse profile(Fig.5) differs greatly from that of AO Psc,with UV maximum coinciding with X-ray minimum.Potter et al.(1997)and Mason(1997)have suggested that the blackbody modulation is caused by changing views of the accretion region as the white dwarf rotates.Maximum(phase0)occurs when the upper pole is towards us.Since this occurs after the dip around phase0.8,interpreted as absorption by the accretion curtains,Potter et al.(1997)and Mason(1997)suggest that PQ Gem accretes preferentially alongfield lines preceding the magnetic pole.6Evans and HellierFigure6.Power spectrum of V2400Oph.The spin(ω)and beat(ω−Ω)frequencies are marked.11.V2400OphiuchiV2400Oph is thought to be the only discless IP,since it shows a dominant X-ray modulation on the spin-orbit beat period(Buckley et al.1995;Hellier& Beardmore2002).We have recently discovered evidence for a spin pulse during an XMM-Newton observation(Fig.6),thefirst in the X-ray band.ReferencesAllan,A.,Hellier,C.,&Beardmore,A.P.1998,MNRAS,295,167Beardmore,A.P.,Mukai,K.,Norton,A.J.,Osborne,J.P.,Hellier,C.1998,MNRAS, 297,337Buckley, D.A.H.,Sekiguchi,K.,Motch, C.,O’Donoghue, D.,Chen, A.-L., Schwarzenberg-Czerny,A.,Pietsch,W.,Harrop-Allin,M.K.1995,MNRAS,275, 1028Eracleous,M.,&Horne,K.1996,ApJ,471,427Evans,P.A.,Hellier,C.,Ramsay,G.,Cropper,M.2004,MNRAS,349,715Evans,P.A.,&Hellier,C.2004,MNRAS,353,447Haberl,F.,Thorstensen,J.R.,Motch,C.,Schwarzenberg-Czerny,A.,Pakull,M.,Sham-brook,A.,Pietsch,W.1994,A&A,291,171Haberl,F.,Motch,C.,&Zickgraf,F.-J.2002,A&A,387,201Hellier,C.,Cropper,M.,&Mason,K.O.1991,MNRAS,248,233Hellier,C.,&Beardmore,A.P.,2002,MNRAS,331,407Hellier,C.,Harmer,S.,&Beardmore,A.P.2004,MNRAS,349,710Ikhsanov,N.R.2001,A&A,374,1030Ishida,M.,Sakao,T.,Makishima,K.,Ohashi,T.,Watson,M.G.,Norton,A.J.,Kawada, M.,Koyama,K.1992,MNRAS,254,647de Martino,D.,Matt,G.,Belloni,T.,Haberl,F.,Mukai,K.2004a,A&A,415,1009 de Martino,D.,Matt,G.,Mukai,K.,Bonnet-Bidaud,J.M.,G¨a nsicke,B.T.,Haberl,F.,Mouchet,M.,2004b,in Hameury,J.M.,&Lasota,J.P.,eds.,The Physics ofCataclysmic Variables and Related Objects,ASP Conf.Ser,in pressMason,K.O.,et al.1992,MNRAS,258,749Mason,K.O.1997,MNRAS,285,493Potter,S.B.,Cropper,M.,Mason,K.O.,Hough,J.H.,Bailey,J.A.1997,MNRAS,285, 82Rosen,S.R.,Mason,K.O.,&C´o rdova,F.A.1988,MNRAS,231,549Watson,M.G.,King,A.R.,&Osborne,J.P.,1985,MNRAS,212,917。
欧空局对地观测卫星介绍综述

6 比利时Redu地面站
Redu地面站拥有多个地面终端,位于比利时 的Ardennes区,距Redu村庄约1公里,在比利时 卢森堡省内。终端提供C波段、L波段、S波段、 Ku波段和Ka波段的跟踪数据,并提供通信卫星在 轨检测(in-orbit tests,IOT)。目前S波段站用 于Integral,该站位于东经5.146 231度、北纬 50.002 685度,参考点位于海拔378.3 m。地球同 步可见仰角超过10度,在54.9度和65.1度之间。该 站装备有自己的紧急发电机系统,并通过完全重复 的光纤线路与比利时通讯网络相连接。该站全天24 小时运行。站点19公顷的面积上装备着一套自动安 全系统,还有相当大的空间做进一步扩充。它的位 置远离RF干涉,站点还有所有必需的频率清除用 2019/4/7 于运转S、C、Ku和Ka频率。
2019/4/7
15 m天线位于北纬5.25 143 694度、西经52.80 466 242度,根据WGS-84地球椭圆体参数天线位 于14.561 m。 Kourou技术设备由S波段和X波段卫星上/下链设 备组成,包括一套自动跟踪设备、一套测距系统、 一套频率和定时系统、一套监控系统和一套通讯系 统。配有不间断电源设备。 Kourou站对ESA的卫星活动起着至关重要的作用, 特别是在LEOP阶段。ESA要求地面站寻找一种灵 活、稳健和低本高效的方法进行设施的运行和维护 (包括内部和外部的通讯连接)。库鲁地面站是位 于全球8个不同地方的11个站点所组成的网络站点 之一,其主要作用是为航天器的跟踪、遥测和指挥 (Tracking, Telemetry and Command,TT&C) 提供支持。
2019/4/7
9 ESTRACK的合作站点
除欧空局自己的地面站外,ESOC还与其 他组织有着地面站服务的合作协议,必要 时还包括ESA的设备。重要的合作有: Malindi(MAL),位于肯尼亚,与罗马 大学(意大利)合作; Santiago(AGO),位于智利,与智利 大学空间研究中心合作; Svalbard(SVA),位于
基于电子束离子阱的天体X射线和极紫外辐射研究进展
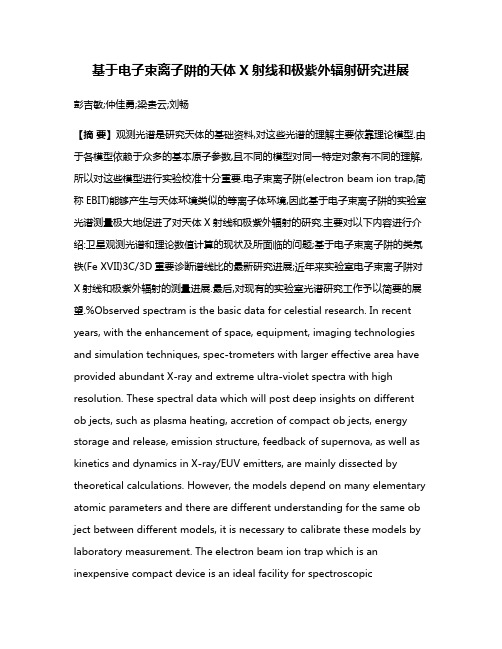
基于电子束离子阱的天体X射线和极紫外辐射研究进展彭吉敏;仲佳勇;梁贵云;刘畅【摘要】观测光谱是研究天体的基础资料,对这些光谱的理解主要依靠理论模型.由于各模型依赖于众多的基本原子参数,且不同的模型对同一特定对象有不同的理解,所以对这些模型进行实验校准十分重要.电子束离子阱(electron beam ion trap,简称EBIT)能够产生与天体环境类似的等离子体环境,因此基于电子束离子阱的实验室光谱测量极大地促进了对天体X射线和极紫外辐射的研究.主要对以下内容进行介绍:卫星观测光谱和理论数值计算的现状及所面临的问题;基于电子束离子阱的类氖铁(Fe XVII)3C/3D重要诊断谱线比的最新研究进展;近年来实验室电子束离子阱对X射线和极紫外辐射的测量进展.最后,对现有的实验室光谱研究工作予以简要的展望.%Observed spectram is the basic data for celestial research. In recent years, with the enhancement of space, equipment, imaging technologies and simulation techniques, spec-trometers with larger effective area have provided abundant X-ray and extreme ultra-violet spectra with high resolution. These spectral data which will post deep insights on different ob jects, such as plasma heating, accretion of compact ob jects, energy storage and release, emission structure, feedback of supernova, as well as kinetics and dynamics in X-ray/EUV emitters, are mainly dissected by theoretical calculations. However, the models depend on many elementary atomic parameters and there are different understanding for the same ob ject between different models, it is necessary to calibrate these models by laboratory measurement. The electron beam ion trap which is an inexpensive compact device is an ideal facility for spectroscopicbenchmark due to its overlapping electron density and temperature with as-trophysical plasma in stellar corona, supernova remnants and so on. Therefore, the spectral measurement based on the electron beam ion trap greatly promotes the study of X-ray and extreme ultraviolet radiation. This article will focus on the following: current situation of spectra of satellite observations and theoretical calculation and the problems; the latest re-search progress of the important diagnostic line ratio(Fe XVII's 3C/3D) based on EBIT;recent advances in laboratory measurement of X-rays and extreme ultraviolet radiation based on electron beam ion trap. Finally, a brief prospect for the laboratory spectroscopy research will be given.【期刊名称】《天文学进展》【年(卷),期】2017(035)004【总页数】12页(P417-428)【关键词】电子束离子阱;X射线;极紫外辐射;原子数据;高分辨率【作者】彭吉敏;仲佳勇;梁贵云;刘畅【作者单位】北京师范大学天文系,北京 100875;中国科学院光学天文重点实验室国家天文台,北京 100012;北京师范大学天文系,北京 100875;中国科学院光学天文重点实验室国家天文台,北京 100012;北京师范大学天文系,北京 100875【正文语种】中文【中图分类】P141了解天体辐射与光谱信息依赖于天体辐射成像和光谱观测,光谱获得主要基于高分辨率光谱仪观测。
- 1、下载文档前请自行甄别文档内容的完整性,平台不提供额外的编辑、内容补充、找答案等附加服务。
- 2、"仅部分预览"的文档,不可在线预览部分如存在完整性等问题,可反馈申请退款(可完整预览的文档不适用该条件!)。
- 3、如文档侵犯您的权益,请联系客服反馈,我们会尽快为您处理(人工客服工作时间:9:00-18:30)。
a
r
X
i
v
:07
1
1
.
2
9
7
6
v
1
[
a
s
t
r
o
-
p
h
]
1
9
N
o
v
2
7
Astron.Nachr./AN 999,No.88,789–795(2006)/DOI please set DOI!⋆Corresponding author:e-mail:acf@ rounding material by irradiation by the power-law.It con-sists of backscattered X-rays,fluorescence and other line photons,bremsstrahlung and other continua from the irra-diated surfaces.Examples of reflection spectra from pho-toionized slabs are shown in Fig.2.At moderate ioniza-tion parameters (ξ=F/n ∼100erg cm s −1,where F is the ionizing flux and n the density of the surface)the main components of the reflection spectrum are the Comp-ton hump peaking at ∼30keV ,the iron line at 6.4-6.9keV (depending on ionization state)and a collection of lines and reradiated continuum below 1keV .When such a spectrum is produced from the innermost parts of an accretion disk around a spinning black hole then the outside observer sees it smeared and redshifted (Fig.3)due to doppler and gravi-tational redshifts into the soft excess,the broad iron line and the Compton hump.All three main parts of the reflection spectrum have now been seen from some AGN and Galactic Black Holes (GBH).The broad iron line and reflection hump are clearly seen in the Seyfert galaxy MCG–6-30-15and in the GBH J1650-400(Fig.4).More recently it has been realised that the soft excess in many AGN can be well explained by smeared re-flection (Crummy et al 2005).The extent of the blurring of the reflection spectrum is determined by the innermost radius of the disk (Fig.4).As-suming that this is the radius of marginal stability then en-ables the spin parameter a of the hole to be measured.Ob-jects with a very broad iron line like MCG–6-30-15are in-ferred to have high spin a >0.95(Dabrowski et al 1997,Brennemann et al 2006).Some (Krolik &Hawley 2002)have argued that magnetic fields in the disk can blur the separation between innermost edge of the disk and the inner plunge region so that the above assumption is invalid.This probably makes little difference however since the reflection comes from very dense matter.Matter in the plunge region
very rapidly drops to a low density (Reynolds &Begelman
c 2006WILEY-VCH Verlag GmbH &Co.KGaA,Weinheim
790 A.C Fabian:XMM-Newton and Broad Iron Lines
Astron.Nachr./AN(2006)791
792 A.C Fabian:XMM-Newton and Broad Iron Lines
Astron.Nachr./AN(2006)793
794 A.C Fabian:XMM-Newton and Broad Iron Lines
Astron.Nachr./AN(2006)795。