缺血和再灌注损伤的机制
脑缺血再灌注损伤机制PPT课件
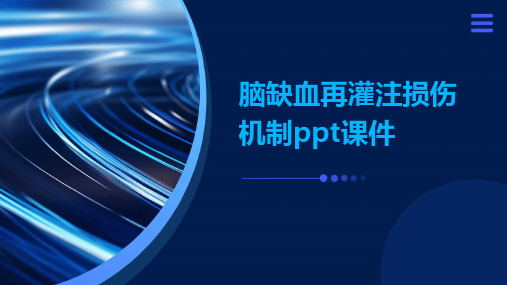
其他治疗方式
控制危险因素
如高血压、糖尿病、高血脂等,降低脑缺血的发生风险。
康复治疗
针对脑缺血后遗留的功能障碍,进行康复训练,提高生活 质量。
健康宣教
提高公众对脑缺血的认识,加强预防措施的宣传和教育。
05
CATALOGUE
脑缺血再灌注损伤的研究进展与展望
研究进展
01
脑缺血再灌注损伤的病理生理机制
深入探讨了脑缺血再灌注损伤过程中炎症反应、氧化应激、细胞凋亡等
关键环节的作用机制,为治疗提供了理论基础。
02
脑缺血再灌注损伤的药物治疗
研究发现了多种具有神经保护作用的药物治疗方法,如抗血小板聚集药
物、溶栓药物、抗炎药物等,为临床治疗提供了新的选择。
03
脑缺血再灌注损伤的基因治疗
通过基因敲除或基因转染技术,调控关键基因的表达,以达到治疗脑缺
分类
根据缺血时间和再灌注时间的不 同,脑缺血再灌注损伤可分为急 性期、亚急性期和慢性期。
发生机制
能量代谢障碍
缺血时,脑组织能量生成不足, 导致细胞内ATP耗竭,细胞膜离 子泵功能受损,细胞内钠离子和 钙离子浓度升高,引发细胞毒性
水肿和细胞死亡。
炎症反应
再灌注后,炎症细胞因子和趋化 因子被激活,引发炎症反应,导
细胞信号转导异常
信号转导通路紊乱
脑缺血再灌注损伤过程中,细胞内信号转导通路发生紊乱,导致 细胞功能异常。
信号分子异常
参与信号转导的分子在脑缺血再灌注损伤后出现异常,影响信号转 导过程。
信号转导抑制剂的作用
某些物质在脑缺血再灌注损伤后发挥信号转导抑制剂的作用,干扰 信号转导过程。
细胞内蛋白质合成异常
致白细胞浸润和组织损伤。
肾脏缺血再灌注损伤机制
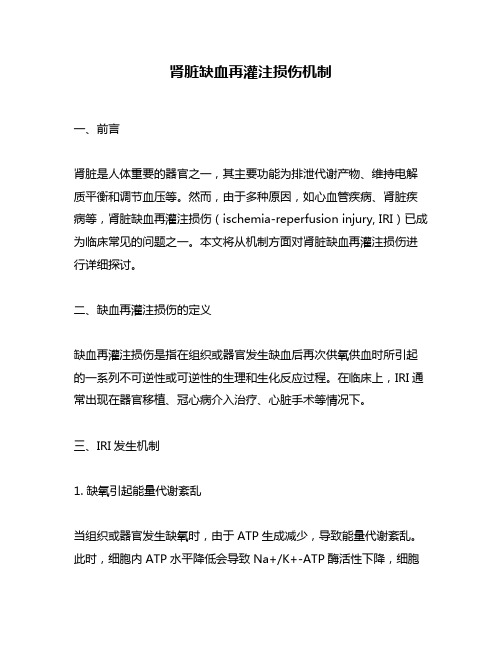
肾脏缺血再灌注损伤机制一、前言肾脏是人体重要的器官之一,其主要功能为排泄代谢产物、维持电解质平衡和调节血压等。
然而,由于多种原因,如心血管疾病、肾脏疾病等,肾脏缺血再灌注损伤(ischemia-reperfusion injury, IRI)已成为临床常见的问题之一。
本文将从机制方面对肾脏缺血再灌注损伤进行详细探讨。
二、缺血再灌注损伤的定义缺血再灌注损伤是指在组织或器官发生缺血后再次供氧供血时所引起的一系列不可逆性或可逆性的生理和生化反应过程。
在临床上,IRI通常出现在器官移植、冠心病介入治疗、心脏手术等情况下。
三、IRI发生机制1. 缺氧引起能量代谢紊乱当组织或器官发生缺氧时,由于ATP生成减少,导致能量代谢紊乱。
此时,细胞内ATP水平降低会导致Na+/K+-ATP酶活性下降,细胞内钠离子增加,钙离子内流,从而引起细胞肿胀和膜损伤。
此外,缺氧还会导致线粒体功能障碍和ROS生成增加。
2. 再灌注引起氧化应激反应再灌注时,组织或器官会受到一系列的氧化应激反应影响。
再灌注后,由于氧供应恢复,线粒体内的呼吸链会被激活,从而产生一系列自由基(ROS)和活性氮(RNS)。
这些自由基和RNS可造成脂质过氧化、蛋白质氧化、DNA损伤等。
3. 炎症反应IRI也会导致炎症反应的发生。
在缺血时,组织或器官受到严重的缺血和低氧环境的影响,导致细胞死亡和坏死。
当再灌注时,坏死细胞释放出许多危险信号分子(DAMPs),如高迁移率族蛋白-1(HMGB-1)、热休克蛋白(HSPs)等,这些信号分子会激活免疫系统,从而引起炎症反应。
4. 凋亡和坏死IRI还会导致细胞凋亡和坏死。
在缺血时,细胞内ATP水平下降,导致凋亡抑制因子(IAPs)失活,从而导致凋亡的发生。
同时,在再灌注时,由于氧化应激和炎症反应的作用,细胞也会发生坏死。
四、IRI的影响因素1. 缺血时间缺血时间是影响IRI严重程度的重要因素。
一般来说,缺血时间越长,IRI越严重。
骨骼肌缺血再灌注损伤机制
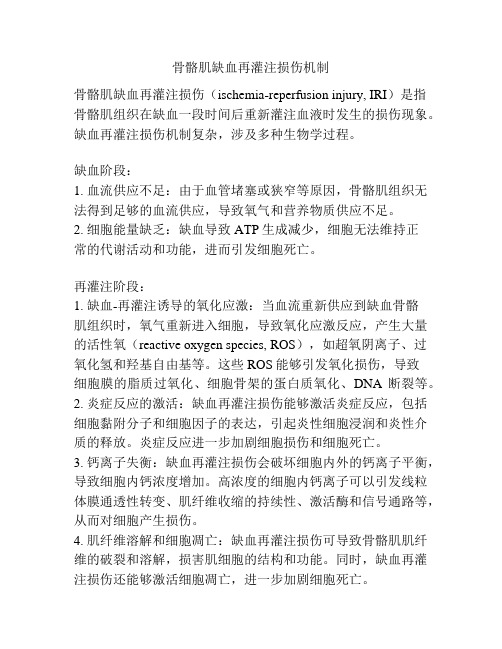
骨骼肌缺血再灌注损伤机制骨骼肌缺血再灌注损伤(ischemia-reperfusion injury, IRI)是指骨骼肌组织在缺血一段时间后重新灌注血液时发生的损伤现象。
缺血再灌注损伤机制复杂,涉及多种生物学过程。
缺血阶段:1. 血流供应不足:由于血管堵塞或狭窄等原因,骨骼肌组织无法得到足够的血流供应,导致氧气和营养物质供应不足。
2. 细胞能量缺乏:缺血导致ATP生成减少,细胞无法维持正常的代谢活动和功能,进而引发细胞死亡。
再灌注阶段:1. 缺血-再灌注诱导的氧化应激:当血流重新供应到缺血骨骼肌组织时,氧气重新进入细胞,导致氧化应激反应,产生大量的活性氧(reactive oxygen species, ROS),如超氧阴离子、过氧化氢和羟基自由基等。
这些ROS能够引发氧化损伤,导致细胞膜的脂质过氧化、细胞骨架的蛋白质氧化、DNA断裂等。
2. 炎症反应的激活:缺血再灌注损伤能够激活炎症反应,包括细胞黏附分子和细胞因子的表达,引起炎性细胞浸润和炎性介质的释放。
炎症反应进一步加剧细胞损伤和细胞死亡。
3. 钙离子失衡:缺血再灌注损伤会破坏细胞内外的钙离子平衡,导致细胞内钙浓度增加。
高浓度的细胞内钙离子可以引发线粒体膜通透性转变、肌纤维收缩的持续性、激活酶和信号通路等,从而对细胞产生损伤。
4. 肌纤维溶解和细胞凋亡:缺血再灌注损伤可导致骨骼肌肌纤维的破裂和溶解,损害肌细胞的结构和功能。
同时,缺血再灌注损伤还能够激活细胞凋亡,进一步加剧细胞死亡。
总的来说,骨骼肌缺血再灌注损伤机制是一个复杂的过程,涉及氧化应激、炎症反应、钙离子失衡、肌纤维溶解和细胞凋亡等多个方面,这些因素相互作用,共同促使骨骼肌细胞发生损伤和死亡。
了解这些机制,有助于针对性地预防和治疗骨骼肌缺血再灌注损伤。
细胞内钙超载引起缺血-再灌注损伤的机制

细胞内钙超载引起缺血-再灌注损伤的机制
细胞内钙超载引起缺血-再灌注损伤的机制是一个复杂的过程,涉及多种细胞和分子机制。
以下是可能的机制:
1. 预充填性钙释放:在缺血条件下,细胞内储存的钙离子增加。
当血液再次流经缺血区域时,血管扩张导致细胞内钙离子的迅速释放,这种预充填性钙释放可能会超过细胞负荷能力。
2. 氧化应激:缺血-再灌注过程产生大量活性氧化物(如超氧
化物自由基、过氧酸等),导致细胞内的氧化应激增加。
氧化应激会破坏细胞的膜完整性和离子通道功能,导致细胞内钙超载。
3. 炎症反应:缺血-再灌注过程会引起炎症反应,释放多种炎
症介质。
这些炎症介质可以促进细胞内钙离子的释放和进入,进一步增加细胞内钙浓度。
4. mitrochondrial钙超载:缺血-再灌注过程还会导致线粒体功
能障碍,包括线粒体钙离子的增加。
线粒体钙超载导致线粒体内能量产生减少,细胞凋亡通路的激活,进一步加剧细胞损伤。
所有这些机制共同作用,导致细胞内钙超载,细胞功能损害和细胞凋亡。
这些过程可以进一步导致局部组织坏死和全身炎症反应,加重缺血-再灌注损伤。
心肌缺血-再灌注损伤的发病机制概述

心肌缺血-再灌注损伤的发病机制概述心肌缺血-再灌注损伤是一种常见的心脏疾病,可以导致心肌死亡、心力衰竭等严重后果。
其发病机制十分复杂,包括氧化应激反应、炎症反应、细胞凋亡等多种生理和分子机制。
本文将对其主要发病机制进行概述。
1. 氧化应激反应:缺血导致心肌细胞缺氧,使得细胞无法正常进行氧化磷酸化反应,从而降低ATP的合成,导致细胞能量代谢失衡。
同时,缺血还可引起一系列氧自由基及其他活性氧的释放和生成,继而形成氧化应激反应。
氧化应激反应会引发一系列信号传递通路的改变,如c-Jun N-末端激酶、p38-MAPK等信号通路的激活,从而导致心肌细胞的损伤。
2. 炎症反应:缺血再灌注过程中,炎症细胞及其激活因子的释放是重要的病理生理过程。
再灌注会刺激各种炎性细胞的激活,特别是中性粒细胞激活,产生一系列炎症介质。
这些炎症介质一方面可以直接破坏心肌细胞,另一方面也能诱导心肌细胞和免疫细胞分泌炎性介质,形成炎症反应。
炎症反应可引起心肌细胞凋亡、坏死和细胞外基质降解等病理变化。
3. 细胞凋亡:缺血再灌注损伤引起心肌细胞凋亡的机制可能与缺血引起线粒体功能障碍和细胞内Ca2+过载有关。
缺血冲击过程中线粒体内膜通透性的增加可导致细胞死亡,而Ca2+的过载也是细胞死亡的重要因素。
Bcl-2家族的蛋白激活是载线粒体膜通透性和细胞凋亡的重要因素;P53对心肌细胞凋亡发挥着重要作用。
心肌细胞凋亡的细胞死亡类型表明其可通过积极调控诱导凋亡途径减轻心肌损伤。
针对心肌缺血-再灌注损伤的发病机制,目前已有不少针对性的治疗策略。
例如,抗氧化剂、抗炎药物和ATP敏感性钾通道开放剂等可用于减轻氧化应激和炎症反应;缩短再灌注时间、预处理干细胞等可用于减轻心肌细胞凋亡等。
尽管这些治疗策略在临床上有着一定的成效,但其对病因机制的理解和干预仍有待进一步探讨。
急性心肌梗死缺血再灌注损伤的机制及其药物防治进展
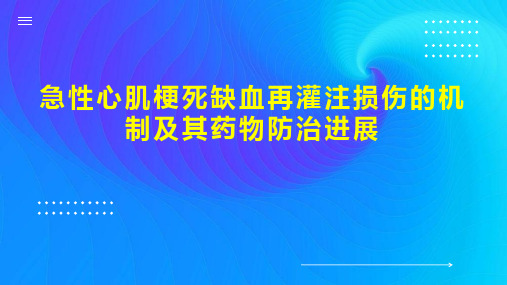
三、药物防治进展
1、抗氧化损伤药物
抗氧化损伤药物是一类能够清除氧自由基、抑制氧化应激反应的药物,如维 生素C、维生素E、硫辛酸等。这些药物可减轻IRI引起的氧化应激反应,保护心 肌细胞。
2、血管扩张药物
血管扩张药物可以扩张冠状动脉,增加心肌血流量,减轻心肌缺血。其中, 硝酸酯类药物是常用的血管扩张药物,可减轻IRI,保护心脏功能。
背景
心肌缺血再灌注损伤的发病机制涉及多个方面,其中自由基损伤、钙离子超 载、炎症反应和细胞凋亡等是关键因素。在心肌缺血期间,能量代谢障碍导致自 由基大量产生,当血液重新流经缺血心肌时,自由基引发氧化应激反应,进而导 致心肌细胞损伤和凋亡。此外,钙离子超载也在心肌缺血再灌注损伤中起到重要 作用,细胞内钙离子浓度异常升高,导致心肌细胞收缩功能障碍,最终引发心肌 损伤。
参考内容
引言
心肌缺血再灌注损伤是指心肌在短暂缺血后重新获得血液供应时所导致的进 一步损伤。这种损伤往往比单纯缺血所造成的损伤更加严重,因此对其发病机制 和防治策略进行深入探讨具有重要意义。本次演示将详细阐述心肌缺血再灌注损 伤的发病机制、症状及危害、实验研究、药物治疗以及其他防治策略,以期为临 床提供有力参考。
1、自由基清除剂:如维生素C、E等,可清除体内自由基,减轻氧化应激反 应,保护心肌细胞。
2、钙离子通道阻滞剂:如硝苯地平、维拉帕米等,可抑制钙离子内流,减 轻钙离子超载引起的细胞损伤。
3、抗炎药物:如阿司匹林、氯吡格雷等,可抑制炎症反应,减轻心肌细胞 的进一步损伤。
4、细胞凋亡抑制剂:如某些抗肿瘤药物,可抑制心肌细胞凋亡,延长细胞 生命。
急性心肌梗死缺血再灌注损伤的机 制及其药物防治进展
目录
01 一、缺血再灌注损伤 机制
病生-第13章缺血-再灌注损伤PPT课件

案例三:肢体缺血-再灌注损伤
总结词
肢体缺血-再灌注损伤可导致肢体肌肉和 骨骼损伤,影响患者的运动功能和生活 能力。
VS
详细描述
肢体缺血-再灌注损伤通常发生在肢体动 脉阻塞后,血流重新恢复时。由于缺血期 间肌肉和骨骼受损,再灌注时会引起炎症 反应和氧化应激,导致肢体肌肉和骨骼损 伤。患者可能出现肢体疼痛、肿胀、活动 受限等症状,严重时可导致截肢或残疾。
药物治疗
使用抗氧化剂
使用细胞保护剂
抗氧化剂可以清除自由基,减少组织 损伤。
细胞保护剂可以保护细胞免受缺血和 再灌注损伤的影响。
使用抗炎药物
抗炎药物可以减轻炎症反应,减少组 织损伤。
其他治疗方法
手术治疗
对于严重的缺血-再灌注损伤,可 能需要手术治疗。
细胞移植
细胞移植可以促进组织再生和修复, 减少组织损伤。
02
优化治疗方案,根据患 者的具体情况制定个性 化的治疗方案,提高治 疗效果。
03
加强医护人员的培训和 教育,提高他们对缺血再灌注损伤的认识和救 治能力。
04
加强多学科协作,整合 医疗资源,为患者提供 全方位、高效的救治服 务。
THANKS
感谢观看
炎症介质释放
炎症细胞释放的炎症介质如TNF-α、 IL-1β等可诱导细胞凋亡和组织损伤。
03
缺血-再灌注损伤的防治
早期治疗
快速恢复血流
在发生缺血后,应尽快恢 复血流,以减少组织损伤。
减轻缺血程度
在血流恢复之前,应采取 措施减轻缺血程度,如使 用血管扩张剂等。
控制再灌注时间
在血流恢复后,应控制再 灌注时间,避免长时间缺 血和再灌注损伤。
案例二:脑缺血-再灌注损伤
缺血再灌注损伤机制

缺血再灌注损伤机制缺血再灌注损伤(ischemia-reperfusion injury)是一种普遍存在的生理现象,常见于心血管外科手术、心肌梗死、脑中风等各种临床情况中。
本文将以缺血再灌注损伤机制为主题,从深度和广度两个方面探讨该主题的各个方面,以帮助读者更全面地理解这一现象。
一、缺血再灌注损伤的基本概念缺血再灌注损伤指的是当组织或器官遭受缺血(血液供应中断)一段时间后,再次供血恢复时所引发的损伤反应。
尽管再灌注的目的是恢复局部供血,但却可能对组织或器官造成更严重的伤害,导致细胞坏死、炎症反应和功能丧失等不良后果。
二、缺血再灌注损伤的机制1. 氧化应激和自由基产生在缺血时,组织或器官缺乏氧气和能量供应,导致线粒体功能障碍和ATP合成降低。
当再灌注发生时,由于血液中大量的氧气重新供应,导致活化的线粒体释放更多反应性氧种和自由基,从而引发氧化应激反应,破坏细胞膜和细胞器功能。
2. 炎症反应激活缺血再灌注损伤可引发炎症反应,释放细胞因子、趋化因子和炎症介质,进一步导致炎症细胞浸润、血管扩张和血小板聚集等炎症反应。
这些炎症反应激活了免疫细胞和炎性细胞,进一步加剧了组织损伤。
3. 钙离子紊乱缺血再灌注损伤会导致细胞内和细胞外钙离子浓度失衡,破坏细胞内钙离子平衡和细胞外钙离子浓度梯度。
这种钙离子紊乱会引发线粒体功能失调、细胞凋亡和细胞死亡等多种病理生理过程。
4. 血管内皮功能损伤缺血再灌注损伤可导致血管内皮细胞的受损和功能异常,进而引发血管扩张、血小板聚集和血管渗透性增加等现象。
这些改变会进一步造成血管内皮功能的破坏,加重缺血再灌注损伤。
三、缺血再灌注损伤防治策略1. 保护组织氧供在缺血再灌注过程中,保持良好的氧供对减轻损伤非常重要。
提前做好血液输注、氧气供应和改善心血管循环等措施,可以有效预防缺血再灌注损伤的发生。
2. 抗氧化治疗应用抗氧化剂,如维生素C、维生素E和谷胱甘肽等,可以减轻缺血再灌注引起的氧化应激反应。
- 1、下载文档前请自行甄别文档内容的完整性,平台不提供额外的编辑、内容补充、找答案等附加服务。
- 2、"仅部分预览"的文档,不可在线预览部分如存在完整性等问题,可反馈申请退款(可完整预览的文档不适用该条件!)。
- 3、如文档侵犯您的权益,请联系客服反馈,我们会尽快为您处理(人工客服工作时间:9:00-18:30)。
F o c u s o n va s c u l a r d i s e a s ereviewIschemia and reperfusion—from mechanism to translationHolger K Eltzschig & Tobias EckleIschemia and reperfusion–elicited tissue injury contributes to morbidity and mortality in a wide range of pathologies, including myocardial infarction, ischemic stroke, acute kidney injury, trauma, circulatory arrest, sickle cell disease and sleep apnea. Ischemia-reperfusion injury is also a major challenge during organ transplantation and cardiothoracic, vascular and general surgery. An imbalance in metabolic supply and demand within the ischemic organ results in profound tissue hypoxia and microvascular dysfunction. Subsequent reperfusion further enhances the activation of innate and adaptive immune responses and cell death programs. Recent advances in understanding the molecular and immunological consequences of ischemia and reperfusion may lead to innovative therapeutic strategies for treating patients with ischemia and reperfusion–associated tissue inflammation and organ dysfunction. Ischemia and reperfusion is a pathological condition characterized by an initial restriction of blood supply to an organ followed by the subsequent restoration of perfusion and concomitant reoxygenation. In its classic manifestation, occlusion of the arterial blood supply is caused by an embolus and results in a severe imbalance of metabolic supply and demand, causing tissue hypoxia. Perhaps surprisingly, restoration of blood flow and reoxygenation is frequently associated with an exacerbation of tissue injury and a profound inflammatory response1 (called ‘reperfusion injury’). Ischemia and reperfusion injury contributes to pathology in a wide range of conditions (Table 1). For example, cardiac arrest and other forms of trauma are associated with ischemia of multiple organs and subsequent reperfusion injury when blood flow is restored. Cyclic episodes of airway obstruction during obstructive sleep apnea also lead to hypoxia with subsequent reoxygenation on arousal2. Similarly, individuals with sickle cell disease have periodic episodes of painful vaso-occlusion and subsequent reperfusion with many characteristics that resemble ischemia and reperfusion3. Exposure of a single organ to ischemia and reperfusion (for example, the liver) may subsequently cause inflammatory activation in other organs (for example, the intestine), eventually leading to multiorgan failure4. However, it is important to point out that ischemic syndromes are a heterogeneous group of conditions. Although there are some similarities in the biological responses among these syndromes, there are important differences between a systemic reduction in perfusion (for example, during shock) compared to regional ischemia and reperfusion of a single organ (or differences between warm ischemia—as occurs, for example, during myocardial ischemia and reperfusion—and cold ischemic conditions— such as those that occur during organ transplantation when the organ is cooled with a cold perfusion solution following procurement).Department of Anesthesiology, Mucosal Inflammation Program, University of Colorado, Aurora, Colorado, USA. Correspondence should be addressed to H.K.E. (holger.eltzschig@). Published online 7 November 2011; doi:10.1038/nm.2507© 2011 Nature America, Inc. All rights reserved.Indeed, a wide range of pathological processes contribute to ischemia and reperfusion associated tissue injury (Fig. 1). For example, limited oxygen availability (hypoxia) as occurs during the ischemic period is associated with impaired endothelial cell barrier function5 due to decreases in adenylate cyclase activity and intracellular cAMP levels and a concomitant increase in vascular permeability and leakage6. In addition, ischemia and reperfusion leads to the activation of cell death programs, including apoptosis (nuclear fragmentation, plasma membrane blebbing, cell shrinkage and loss of mitochondrial membrane potential and integrity), autophagy-associated cell death (cytoplasmic vacuolization, loss of organelles and accumulation of vacuoles with membrane whorls) and necrosis (progressive cell and organelle swelling, plasma membrane rupture and leakage of proteases and lysosomes into the extracellular compartment)7. The ischemic period in particular is associated with significant alterations in the transcriptional control of gene expression (transcriptional reprogramming). For example, ischemia is associated with an inhibition of oxygen-sensing prolylhydroxylase (PHD) enzymes because they require oxygen as a cofactor. Hypoxia-associated inhibition of PHD enzymes leads to the post-translational activation of hypoxia and inflammatory signaling cascades, which control the stability of the transcription factors hypoxia-inducible factor (HIF) and nuclear factor-κB (NF-κB), respectively8. Despite successful reopening of the vascular supply system, an ischemic organ may not immediately regain its perfusion (no reflow phenomenon). Moreover, reperfusion injury is characterized by autoimmune responses, including natural antibody recognition of neoantigens and subsequent activation of the complement system (autoimmunity)9. Despite the fact that ischemia and reperfusion typically occurs in a sterile environment, activation of innate and adaptive immune responses occurs and contributes to injury, including activation of pattern-recognition receptors such as TLRs and inflammatory cell trafficking into the diseased organ (innate and adaptive immune activation)10. In this review, we highlight recent studies that provide new insight into the molecular and immunological pathways of ischemia and1391nature medicine volume 17 | number 11 | november 2011reviewTable 1 Examples of ischemia and reperfusion injuryAffected organ Heart Kidney Intestine Brain Trauma and resuscitation Circulatory arrest Sickle cell disease Sleep apnea Cardiac surgery Thoracic surgery Example of clinical manifestation Acute coronary syndrome Acute kidney injury Intestinal ischemia and reperfusion; multiorgan failure Stroke Multiple organ failure; acute kidney injury; intestinal injury Hypoxic brain injury; multiple organ failure; acute kidney injury Acute chest syndrome; pulmonary hypertension, priapism, acute kidney injury Hypertension; diabetes Acute heart failure after cardiopulmonary bypass Acute lung injury Compartment syndrome of extremity Acute kidney injury Acute graft failure; early graft rejection Single-organ ischemia and reperfusionMultiple-organ ischemia and reperfusionIschemia and reperfusion during major surgeryPeripheral vascular surgery Major vascular surgery Solid organ transplantationreperfusion, as well as discuss examples of innovative therapeutic approaches based on these mechanistic findings (Table 2). Ischemia and reperfusion causes sterile inflammation With a few exceptions, such as bacterial translocation after intestinal injury, ischemia and reperfusion typically occurs in a sterile environment. Nevertheless, the consequences of ischemia and reperfusion share many phenotypic parallels with activation of a host immune response directed toward invading microorganisms10. This sterile immune response involves signaling events through pattern-recognition molecules such as Toll-like receptors (TLRs), recruitment and activation of immune cells of the innate and adaptive immune system and activation of the complement system (Fig. 2). As these responses can have adverse consequences, targeting immune activation is an emerging therapeutic concept in the treatment of ischemia and reperfusion. In contrast, some aspects of the adaptive immune response—particularly the recruitment and expansion of regulatory T cells (Treg cells)—may be beneficial11. Innate immune responses. The inflammatory response to sterile cell death or injury has many similarities to that observed during microbial infections. In particular, host receptors that mediate the response to microorganisms have been implicated in the activation of sterile inflammation during ischemia and reperfusion10. For example, ligand binding to TLRs leads to the activation of downstream signaling pathways, including NF-kB, mitogen-activated protein kinase (MAPK) and type I interferon pathways, resulting in the induction of proinflammatory cytokines and chemokines10. These receptors can also be activated by endogenous molecules in the absence of microbial compounds, particularly in the context of cell damage or death, as occurs during ischemia and reperfusion10. Such ligands have been termed ‘damage-associated molecular patterns’ (DAMPs). Many of these ligands (for example, high-mobility group box 1 (HMGB1) protein or ATP) are normally sequestered intracellularly; upon tissue damage, they are released into the extracellular compartment where they can activate an immune response12,13. There is also evidence that extracellularly located damage-associated molecular patterns are generated or released in the process of catabolism10. Such catabolic DAMPs can either activate an immune response12 or function as a safety signal to restrain potentially harmful immune responses and1392promote tissue integrity during ischemia and reperfusion (examples of the latter type of catabolic DAMP are adenosine and the fibrinogenderived peptide Bb15–42)14,15. One of the most widely studied pattern recognition receptors is TLR4, which is known to mediate inflammatory responses to Gram-negative bacteria through its activation by lipopolysaccharide. Mice with targeted gene deletion of Tlr4 are hyporesponsive to lipopolysaccharide, as are humans with missense mutations in TLR4 (ref. 16). TLR4 activation may be enhanced by oxidative stress17, which is generated by ischemia and reperfusion and is known to prime inflammatory cells for increased responsiveness to subsequent stimuli. Alveolar macrophages from rodents subjected to hemorrhagic shock and resuscitation express increased surface levels of TLR4, an effect that was inhibited by adding the antioxidant N-acetylcysteine to the resuscitation fluid17. Moreover, H2O2 treatment of cultured macrophages similarly caused an increase in surface TLR4 expression17. Fluorescent resonance energy transfer between TLR4 and the raft marker GM1, as well as biochemical analysis of raft components, showed that oxidative stress redistributes TLR4 to lipid rafts in the plasma membrane, consistent with the idea that oxidative stress primes the responsiveness of cells of the innate immune system17. Other studies have implicated TLR4 signaling in renal ischemia and reperfusion. Mice with a genetic deletion of Tlr4 are protected from kidney ischemia, and experiments using bone-marrow chimeric mice suggest that kidney-intrinsic Tlr4 signaling has the predominant role in mediating kidney injury18. In addition, a study of patients undergoing kidney transplantation revealed a detrimental role of TLR4 signaling in early graft failure19. Indeed, endogenous TLR4 ligands such as HMGB1 and biglycan were induced during human kidney transplantation, thereby supporting a role for TLR4 in sterile inflammation in the kidney19. Kidneys from individuals with a TLR4 loss-of-function allele (as assessed by diminished affinity of TLR4 for its ligand HMGB1) contained lower levels of proinflammatory cytokines in association with higher rates of immediate graft function after transplantation19. Other TLRs may also have deleterious effects. TLR3, implicated in sensing viral RNA, was proposed to sense RNA released from necrotic cells independent of viral activation, and treatment with a neutralizing antibody to TLR3 was protective in studies of intestinal ischemia and reperfusion in vivo20. TLR2 expression on epithelia is induced by hypoxia21 or inflammation22, and renal TLR2 signaling contributes to acute kidney injury and inflammation during ischemia and reperfusion23. Taken together, these studies suggest that inhibitors of TLR signaling could be effective for the treatment of sterile inflammation induced by ischemia and reperfusion. Accordingly, antagonists for TLR receptors are currently under development24 (Table 2). These antagonists are structural analogs of TLR agonists and likely act by binding the receptor without inducing signal transduction24. For example, experimental studies of TAK-242, a small-molecule inhibitor of TLR4, in large animals have shown efficacy in the treatment of acute kidney injury25. A recent randomized clinical© 2011 Nature America, Inc. All rights reserved.Vascular leakageNo reflow phenomenonCell death programsApoptosis, necrosis, autophagy-associated cell deathIschemia and reperfusionAutoantibody and complement activationAutoimmunityTranscriptional reprogrammingInnate and adaptive immune activationFigure 1 Biological processes implicated in ischemia and reperfusion.volume 17 | number 11 | november 2011 nature medicinereviewTable 2 Examples of promising therapeutic approaches targeting ischemia and reperfusionIntervention TAK-242 Target Inhibition of TLR4 Potential downside Immune suppression, worsening of bacterial infections Unclear Unclear Immune suppression; worsening of bacterial infection Stage Phase 2 clinical trial in acute respiratory failure; preclinical studies in ischemia and reperfusion Preclinical Phase 2 clinical trial Phase 2 clinical trial Reference 25,26T cell–based approaches Fibrinogen split product Bb15–42 Cyclosporine Chloramphenicol PHD inhibitorsSuppression of gd T cells; expansion of Treg cells Unclear Inhibition of apoptosis Activation of autophagy37,39,40,42 15,56,57 66 69 79,92–94Bone marrow toxicity (bone marrow Preclinical (large animal study) suppression or aplastic anemia) Phase 2 clinical trial in renal anemia; preclinical studies in ischemia and reperfusion Phase 2 clinical trialInhibition of the oxygen sensing PHD Unclear enzymes resulting in HIF stabilization Multiple (for example, adenosine signaling, HIF stabilization and attenuation of inflammation) Multiple Multiple Multiple ATP breakdown (attenuation of ATP signaling and promotion of adenosine generation and signaling) AMP conversion to adenosine; enhanced adenosine generation and signaling Specific adenosine receptor agonists targeting Adora2a Specific adenosine receptor agonist targeting Adora2b Promotion of angiogenesis Inhibition of apoptosis UnclearIschemic preconditioning79–83Ischemic postconditioning Remote ischemic conditioning Nitric oxide (NO) ApyraseUnclear Unclear Elevation of methemoglobin UnclearPhase 2 clinical trial Phase 2 clinical trial Phase 2 clinical trial Preclinical87,88 89 106,107 81,120© 2011 Nature America, Inc. All rights reserved.NucleotidaseUnclearPreclinical80,122,123Regadenoson, ATL146e Bay 60-6583 Inhibitors of miR-92a Activators of miR-499 or miR-24Unclear Sickling of red blood cells in individuals with sickle cell disease Unclear UnclearPhase 1 trial ongoing Preclinical Preclinical Preclinical3,131 80,129,130 137 138,139trial of TAK-242 in patients with sepsis and shock or with respiratory failure showed a trend toward improved survival with TAK-242 treatment26, but the findings were not statistically significant. Nevertheless, translational approaches using TLR inhibitors remain promising. Sterile inflammation during ischemia and reperfusion is also characterized by the accumulation of inflammatory cells. Particularly during the early phase of reperfusion, innate immune cells dominate the cellular composition of the infiltrates. The functional contributions of these cells are not clear: they may contribute to a pathological activation of inflammation and promote collateral tissue injury, or conversely to the resolution of injury. Notably, a recent study showed that monocytes can be recruited from a splenic reservoir to injured tissue after myocardial ischemia and reperfusion to participate in wound healing27. Other studies have found that depletion of conventional dendritic cells increases sterile inflammation and tissue injury in the context of hepatic ischemia and reperfusion injury28. The protection afforded by dendritic cells depends on their production of the anti-inflammatory cytokine interleukin-10 (IL-10), resulting in attenuated levels of tumor necrosis factor-a, IL-6 and reactive oxygen species (ROS). ROS, implicated in the tissue damage that occurs during ischemia and reperfusion11, are toxic molecules that alter cellular proteins, lipids and ribonucleic acids, leading to cell dysfunction or death. NADPH oxidase, an enzyme expressed in virtually all inflammatory cells, contributes to the formation of one such cytotoxic ROS, peroxynitrite. In addition, H2O2 derived from O2– dismutation gives rise to highly toxic hydroxyl radicals through the Haber-Weiss reaction, facilitated by the increased availability of free iron in ischemia11. Peroxynitrite and other reactive species induce oxidative DNA damage and consequent activation of the nuclear enzymenature medicine volume 17 | number 11 | november 2011poly (ADP-ribose) polymerase 1 (PARP-1), the most abundant isoform of the PARP enzyme family. Accordingly, PARP inhibitors are in clinical development for the treatment of ischemia and reperfusion injury29. At sites of sterile inflammation, the accumulation of granulocytes has to be tightly controlled, as too few granulocytes may not allow for adequate tissue repair, whereas too many granulocytes can promote uncontrolled inflammation and tissue injury30. In a clinically relevant mouse model for transplant-mediated lung ischemia and reperfusion, a recent study showed that expression of the Bcl3 protein by the recipient led to inhibition of emergency granulopoiesis and limited acute graft injury30. Inhibition of myeloid progenitor cell differentiation may therefore have promise as a therapeutic strategy for the prevention of tissue injury in the context of sterile inflammation. Adaptive immune response. Ischemia and reperfusion elicits a robust adaptive immune response that involves, among other cell types, T lymphocytes. The mechanisms by which antigen-specific T cells are activated during sterile inflammation are not well understood, but emerging evidence indicates a contribution of both antigen-specific and antigenindependent mechanisms of activation31,32. Several studies have shown that T cells accumulate during ischemia and reperfusion. For example, T cells are localized to the infarction boundary zone within 24 h of reperfusion of the ischemic brain, accumulate further at 3 and 7 d after reperfusion and are decreased in number after 14 d33. Studies of mouse lines deficient in specific populations of lymphocytes showed that both CD4+ and CD8+ T cells have a detrimental role in ischemia and reperfusion of the brain34, the heart35 and the kidneys36. Further, a recent study suggested a pivotal role for IL-17 produced by gd T cells in ischemia and reperfusion injury of the brain37. Elevated levels of IL-17 were found1393review aNecrotic cell ICAM-1 Poly P β2 integrinInjuryTissue injury PlateletsICAM-1 Kindlin-3 Platelets Neutrophil recruitment Complement activationRNAHMGB1Factor XII Coagulation IgM NMHC-IITLR2TLR3TLR4 ROSEC VSMCNF-kBCytokines ChemokinesInflammation IL-17 γδ T cell CD8+ T cell CD4+ T cellb© 2011 Nature America, Inc. All rights reserved.DC IL-10ResolutionTNF IL-6 ROS VE-cadherin Wound healingTregFibrinogen TF Fibrin A2B Apoptosis NFNF -k κB NF-Bβ15–42 Adenosine Ad HIF MacrophageSpleen reservoirMarina CorralPHDFigure 2 Injury and resolution during ischemia and reperfusion. (a) Ischemia and reperfusion is associated with a pathological activation of the immune system. Tissue hypoxia during the ischemic period results in TLR-dependent stabilization of the transcription factor NF-kB, leading to transcriptional activation of inflammatory gene programs. TLR4 expression can be increased by ROS and can be activated by endogenous ligands such as HMGB1. TLR3 can be activated by RNA released from necrotic cells. After reperfusion, granulocytes such as neutrophils adhere to the vasculature and infiltrate the tissue, and platelets can ‘piggyback’ on neutrophils. Activated platelets can interact with vascular endothelia at the site of injury; this interaction depends on a shift of platelet integrins from a low-affinity state to a highaffinity state (integrin activation or priming), which requires Kindlin-3. Activated platelets release inorganic polyphosphate that directly binds to and activates the plasma protease factor XII, contributing to proinflammatory and procoagulant activation during ischemia and reperfusion. CD4+, CD8+ and gd T cells contribute to tissue injury; for example, by the release of IL-17 from gd T cells. (b) Ischemia and reperfusion activates endogenous mechanisms of injury resolution. Tissue hypoxia results in the inhibition of oxygen-sensing PHD enzymes and stabilization of the HIF transcription factor, activating a wide range of transcriptional programs involved in injury resolution, including the production of extracellular adenosine that signals through receptors such as ADORA2B (A2B). In addition, hypoxia-elicited inhibition of PHDs results in NF-kB activation, which contributes to the resolution phase by preventing apoptosis. Regulatory T cells and dendritic cells are important sources of IL-10, which has a crucial role in dampening inflammation and attenuating reactive oxygen production. Splenic reservoir monocytes are recruited from the spleen to the site of tissue energy where they participate in wound healing. Breakdown products from fibrinogen, such as fibrin-derived peptide Bb15–42, protect the myocardium from injury. NMHC-II, non-muscle myosin heavy chain type II; DC, dendritic cell; EC, endothelial cell; Poly P, polyphosphate; TF, tissue factor; VSMC, vascular smooth muscle cell.Hypoxiaboth in individuals suffering from stroke38 as well as in mice exposed to brain ischemia and reperfusion37. Notably, subsequent studies identified gd T cells (as opposed to CD4+ T helper cells) as the main source of IL-17 (ref. 37). In addition, genetic and pharmacologic approaches targeting IL-17 or gd T cells led to reduced inflammation and robust neuroprotection, indicating that gd T cells that produce IL-17 are an attractive therapeutic target for ischemic stroke (Table 2). In contrast, Treg cells appear to have a protective role of in ischemia and reperfusion. For example, a recent study using an experimental stroke model showed that depletion of Treg cells substantially increased delayed brain damage and caused a deterioration in functional outcome39. Based on results including the finding that transfer of wildtype but not IL-10–deficient Treg cells attenuated ischemic brain injury, the authors proposed that Treg cell–dependent production of IL-10 decreases tumor necrosis factor a abundance at early time points and delays interferon g accumulation39. Although not tested in the setting of ischemia and reperfusion, administration of ex vivo expanded human Treg cells had beneficial effects in a model of transplant atherosclerosis, providing evidence that such an approach is feasible40. Other strategies to enhance Treg cell function following ischemia and reperfusion could involve boosting expression of FOXP3, the key transcription factor for Treg cell differentiation. Previous studies have shown that Foxp3 levels are subject to epigenetic regulation41. Indeed, pharmacological inhibitors of histone/protein deacetylases are effective in treating experimentally induced inflammatory bowel disease and in improving cardiac and islet graft survival in mouse transplantation models through increasing Treg cell numbers and function42. Innate autoimmunity, complement, platelets and coagulation. During ischemia and reperfusion, innate recognition proteins can be self reactive and initiate inflammation against self tissue in a manner similar1394to the response triggered by pathogens (known as ‘innate autoimmunity’)9. A series of studies has linked reperfusion injury to the occurrence of so-called ‘natural’ antibodies, leading to activation of the complement system. Natural antibodies are produced in the absence of deliberate immunization and are a major component of the repertoire of B1 cells, which produce IgM and, in some cases, IgG43. For example, a single type of natural antibody prepared from a panel of B1 cell hybridomas (IgMCM-22) restored reperfusion injury in antibody-deficient mice9, suggesting that reperfusion injury can be considered to be an autoimmune type of disorder. Using mouse models of skeletal muscle and intestinal reperfusion injury, a highly conserved region within nonmuscle myosin heavy chain type II A and C was subsequently identified as a self target for natural IgM in the initiation of reperfusion injury44. More recently, additional neoepitopes have been identified, for example, the soluble cytosolic protein annexin IV43. Together, these studies indicate that neoepitopes expressed on ischemic tissues are targets for natural antibody binding during the reperfusion phase with subsequent complement activation, neutrophil recruitment and tissue injury43. The complement system acts as an immune surveillance system to discriminate among healthy host tissue, cellular debris, apoptotic cells and foreign intruders, varying its response accordingly45. Locally produced and activated, the complement system yields cleavage products that function as intermediaries, amplifying sterile inflammation during ischemia and reperfusion through complement-mediated recognition of damaged cells and anaphylatoxin release, thereby fueling inflammation and the recruitment of immune cells45. Studies in animal models have indicated that inhibition of the complement system might effectively treat ischemia and reperfusion injury; however, results from clinical studies have largely been disappointing46–49. A limitation of the clinical studies could be that one of the inhibitors used, an antibody targetingvolume 17 | number 11 | november 2011 nature medicinereviewthe complement protein C5, would not affect C3b, which is “upstream” of C5 in the complement cascade and is a key mediator of bacterial opsonization and immune complex solubilization and clearance48. In addition, the complexity of the complement system and incomplete mechanistic insight into the functional consequences of manipulating individual components of the cascade may contribute to difficulties in therapeutic targeting of complement pathways. A recent study of hepatic ischemia and reperfusion injury in mice indicated a dual role of the complement system50: although excessive complement activation is detrimental, a threshold of complement activation is crucial for liver regeneration, and impaired regeneration due to inadequate complement activation can lead to acute liver failure following hepatic resection or liver transplantation. Excessive platelet aggregation and release of platelet-derived mediators can exacerbate tissue injury following ischemia and reperfusion. Platelet activation can occur through integrin-mediated endothelial interactions51. In addition, platelets can be transported by inflammatory cells across epithelial barriers (by ‘piggybacking’ on polymorphonuclear leukocytes) to sites of injury or inflammation52. A recent study showed a central role for a FERM domain-containing protein (Fermt3, also known as Kindlin-3) in mediating integrin-dependent platelet activation and aggregation51. Fermt3–/– mice were protected in a model of ischemia and infarction after mesenteric arteriole injury with virtually no firm adhesion of platelets to the injured vessel wall51. Other studies have shown that platelets release inorganic polyphosphates, polymers of 60–100 phosphate residues that directly activate plasma protease factor XII and thereby function as proinflammatory and procoagulant mediators in vivo53. Ischemia and reperfusion triggers coagulation by inflammatory mediators and platelet activation in many ways, but several natural anticoagulant mechanisms can inhibit clot formation following ischemia and reperfusion, such as those mediated by antithrombinheparin, tissue factor inhibitor and protein C54,55. Furthermore, fibrin degradation following ischemia and reperfusion, resulting in the formation of fibrin D fragments, including the peptide Bb15–42, has been implicated in attenuating inflammation and preserving vascular barrier function during shock56 and in dampening ischemia-reperfusion injury15. Administration of an intravenous bolus of Bb15–42 attenuated myocardial injury in mice17, and a subsequent randomized clinical trial of patients with acute myocardial infarction with ST-segment elevation showed that treatment with intravenously-administered Bb15–42 upon reperfusion reduced the size of the necrotic core zone, as assessed using magnetic resonance imaging 5 days after infarction (ref. 57, Table 2). Cell death during ischemia and reperfusion Ischemia and reperfusion activates various programs of cell death, which can be categorized as necrosis, apoptosis or autophagy-associated cell death7. Necrosis, characterized by cell and organelle swelling with subsequent rupture of surface membranes and the spilling of their intracellular contents7, is a frequent outcome of ischemia and reperfusion. Necrotic cells are highly immunostimulatory and lead to inflammatorycell infiltration and cytokine production. In contrast, apoptosis involves an orchestrated caspase signaling cascade that induces a self-contained program of cell death, characterized by the shrinkage of the cell and its nucleus, with plasma membrane integrity persisting until late in the process7. Although this process has traditionally been viewed as less immunostimulatory than necrosis10, recent studies have shown that extracellular release of ATP from apoptotic cells through pannexin hemichannels acts as a ‘find-me’ signal that attracts phagocytes58,59. Inhibition of apoptosis may have promise as a therapeutic strategy for ischemiareperfusion injury. For example, a study in a mouse model of acute kidney injury identified the matricellullar protein thrombospondin 1nature medicine volume 17 | number 11 | november 2011(THBS1, also known as TSP-1), produced by injured proximal tubular cells, as an inducer of apoptosis and found that Thbs1–/– mice are protected from injury60. Other studies have focused on platelet-derived growth factor CC (PDGF-CC), a potent neuro-protective factor that acts by modulating glycogen synthase kinase 3b (GSK-3b) activity61. PDGF-CC gene or protein delivery protected neurons from apoptosis in both the retina and brain in various animal models of neuronal injury, including ischemia-induced stroke. PDGF-CC treatment resulted in increased levels of GSK-3b Ser9 phosphorylation and decreased levels of Tyr216 phosphorylation, consistent with previous findings that Ser9 phosphorylation inhibits and Tyr216 phosphorylation promotes apoptosis61,62. The transcription factor NF-kB may also modulate apoptosis during ischemia and reperfusion. Limited oxygen availability is associated with activation of NF-kB through a mechanism involving hypoxia-dependent inhibition of oxygen sensors63. Mice with disruption of the gene encoding IKK-b, the catalytic subunit of IKK that is essential for NF-kB activation, offer an opportunity to study the consequences of preventing canonical NF-kB pathway activation. This manipulation, however, results in embryonic lethality owing to massive apoptosis of the developing liver driven by tumor necrosis factor-a (ref. 64). To circumvent this difficulty, studies from the laboratory of Michael Karin examined mice with selective ablation of IKK-b. Study of intestinal ischemia and reperfusion revealed that although IKK-b deficiency in enterocytes is associated with reduced inflammation, severe apoptotic damage occurred in the reperfused mucosa65. NF-kB inhibition can therefore be viewed as a ‘double-edged sword’, in that it is associated with the prevention of systemic inflammation but increased local injury. These results underscore the need for caution in using NF-kB inhibitors for treating intestinal ischemia-reperfusion injury. The commonly used immunosuppressant cyclosporine is an inhibitor of mitochondrial permeability transition pore opening, an important step in programmed cell death7. In a randomized clinical study of 58 individuals with acute myocardial infarction with ST-segment elevation, treatment with an intravenous bolus of cyclosporine immediately before percutaneous coronary intervention was associated with smaller infarct sizes compared to the saline control (Table 2)66. Although these findings are very encouraging, given the small sample size of this trial, confirmation in a larger clinical trial will be important. In addition, the development of more specific and safer inhibitors of the mitochondrial permeability transition pore could enhance the potential of this approach67. There is strong evidence supporting the idea that autophagy is an adaptive response to sublethal stress, such as nutrient deprivation, and the deletion of key autophagic genes accelerates rather than inhibits cell death7. The transcription factor HIF, a central mediator of hypoxic responses, also seems to regulate autophagy. The process of mitochondrial autophagy is induced by hypoxia and requires HIF-dependent expression of autophagic genes68, indicating a crucial role for HIF in the metabolic adaptation of hypoxic or ischemic tissues during conditions of limited oxygen. From a therapeutic perspective, a recent study showed that chloramphenicol, traditionally used to treat bacterial infections but more recently recognized as an inducer of autophagy, is protective in a swine model of myocardial ischemia and reperfusion (Table 2)69. Microvascular dysfunction Ischemia and reperfusion is associated with a vascular phenotype that includes increased vascular permeability, endothelial cell inflammation, an imbalance between vasodilating and vasoconstricting factors and activation of coagulation and the complement system. Microvascular dysfunction following ischemia and reperfusion in humans can lead to1395© 2011 Nature America, Inc. All rights reserved.。