Biochar from Biomass and Waste
生物炭的详细制备工艺流程
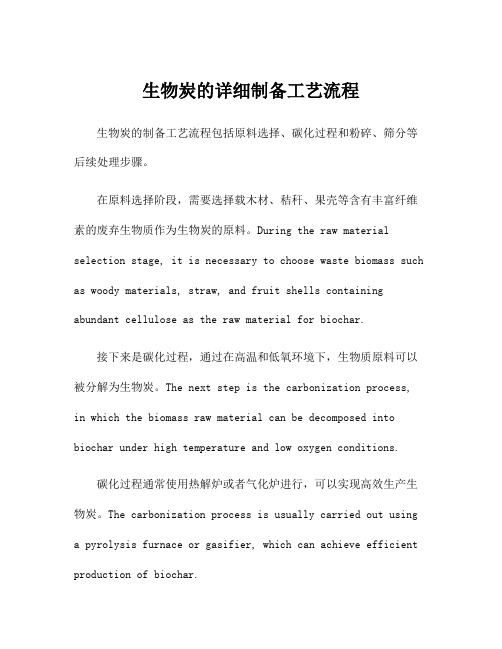
生物炭的详细制备工艺流程生物炭的制备工艺流程包括原料选择、碳化过程和粉碎、筛分等后续处理步骤。
在原料选择阶段,需要选择载木材、秸秆、果壳等含有丰富纤维素的废弃生物质作为生物炭的原料。
During the raw material selection stage, it is necessary to choose waste biomass such as woody materials, straw, and fruit shells containing abundant cellulose as the raw material for biochar.接下来是碳化过程,通过在高温和低氧环境下,生物质原料可以被分解为生物炭。
The next step is the carbonization process, in which the biomass raw material can be decomposed into biochar under high temperature and low oxygen conditions.碳化过程通常使用热解炉或者气化炉进行,可以实现高效生产生物炭。
The carbonization process is usually carried out using a pyrolysis furnace or gasifier, which can achieve efficient production of biochar.经过碳化后,生物炭需要进行粉碎、筛分等后续处理,以获得符合要求的生物炭产品。
After carbonization, biochar needs to undergo subsequent processes such as crushing and screening to obtain biochar products that meet the requirements.在整个生物炭制备工艺中,需要控制碳化温度、时间、气氛等参数,以确保生物炭产品的质量。
CHEMICAL REMOVAL OF BIOMASS FROM WASTE AIR
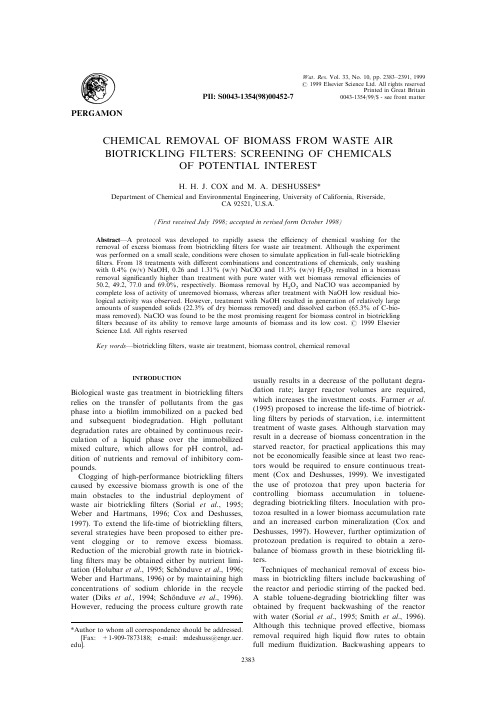
CHEMICAL REMOVAL OF BIOMASS FROM WASTE AIR BIOTRICKLING FILTERS:SCREENING OF CHEMICALSOF POTENTIAL INTERESTH.H.J.COX and M.A.DESHUSSES*Department of Chemical and Environmental Engineering,University of California,Riverside,CA 92521,U.S.A.(First received July 1998;accepted in revised form October 1998)Abstract ÐA protocol was developed to rapidly assess the e ciency of chemical washing for the removal of excess biomass from biotrickling ®lters for waste air treatment.Although the experiment was performed on a small scale,conditions were chosen to simulate application in full-scale biotrickling ®lters.From 18treatments with di erent combinations and concentrations of chemicals,only washing with 0.4%(w/v)NaOH,0.26and 1.31%(w/v)NaClO and 11.3%(w/v)H 2O 2resulted in a biomass removal signi®cantly higher than treatment with pure water with wet biomass removal e ciencies of 50.2,49.2,77.0and 69.0%,respectively.Biomass removal by H 2O 2and NaClO was accompanied by complete loss of activity of unremoved biomass,whereas after treatment with NaOH low residual bio-logical activity was observed.However,treatment with NaOH resulted in generation of relatively large amounts of suspended solids (22.3%of dry biomass removed)and dissolved carbon (65.3%of C-bio-mass removed).NaClO was found to be the most promising reagent for biomass control in biotrickling ®lters because of its ability to remove large amounts of biomass and its low cost.#1999Elsevier Science Ltd.All rights reservedKey words Ðbiotrickling ®lters,waste air treatment,biomass control,chemical removalINTRODUCTIONBiological waste gas treatment in biotrickling ®lters relies on the transfer of pollutants from the gas phase into a bio®lm immobilized on a packed bed and subsequent biodegradation.High pollutant degradation rates are obtained by continuous recir-culation of a liquid phase over the immobilized mixed culture,which allows for pH control,ad-dition of nutrients and removal of inhibitory com-pounds.Clogging of high-performance biotrickling ®lters caused by excessive biomass growth is one of the main obstacles to the industrial deployment of waste air biotrickling ®lters (Sorial et al.,1995;Weber and Hartmans,1996;Cox and Deshusses,1997).To extend the life-time of biotrickling ®lters,several strategies have been proposed to either pre-vent clogging or to remove excess biomass.Reduction of the microbial growth rate in biotrick-ling ®lters may be obtained either by nutrient limi-tation (Holubar et al.,1995;Scho nduve et al.,1996;Weber and Hartmans,1996)or by maintaining high concentrations of sodium chloride in the recycle water (Diks et al.,1994;Scho nduve et al.,1996).However,reducing the process culture growth rateusually results in a decrease of the pollutant degra-dation rate;larger reactor volumes are required,which increases the investment costs.Farmer et al.(1995)proposed to increase the life-time of biotrick-ling ®lters by periods of starvation,i.e.intermittent treatment of waste gases.Although starvation may result in a decrease of biomass concentration in the starved reactor,for practical applications this may not be economically feasible since at least two reac-tors would be required to ensure continuous treat-ment (Cox and Deshusses,1999).We investigated the use of protozoa that prey upon bacteria for controlling biomass accumulation in toluene-degrading biotrickling ®lters.Inoculation with pro-tozoa resulted in a lower biomass accumulation rate and an increased carbon mineralization (Cox and Deshusses,1997).However,further optimization of protozoan predation is required to obtain a zero-balance of biomass growth in these biotrickling ®l-ters.Techniques of mechanical removal of excess bio-mass in biotrickling ®lters include backwashing of the reactor and periodic stirring of the packed bed.A stable toluene-degrading biotrickling ®lter was obtained by frequent backwashing of the reactor with water (Sorial et al.,1995;Smith et al.,1996).Although this technique proved e ective,biomass removal required high liquid ¯ow rates to obtain full medium ¯uidization.Backwashing appears toWat.Res.Vol.33,No.10,pp.2383±2391,1999#1999Elsevier Science Ltd.All rights reservedPrinted in Great Britain0043-1354/99/$-see front matterPII:S0043-1354(98)00452-7*Author to whom all correspondence should be addressed.[Fax:+1-909-7873188;e-mail:mdeshuss@].2383be limited to those biotrickling®lters containing support materials that are easily¯uidized and a 40%larger reactor volume is required to allow for bed expansion during¯uidization(Sorial et al., 1997).A proposed alternative to backwashing is to periodically stir the packed bed to shear o excess biomass(Wu bker et al.,1997).It is unclear how the latter proposal can be implemented at an industrial scale.Chemical removal of biomass is an attractive alternative for biomass control in biotrickling®lters but it remains a relatively unexplored area.So far, biomass removal from biotrickling®lters by chemi-cal washing has only been reported by Weber and Hartmans(1996),who used a sodium hydroxide solution.In the present contribution,we discuss results on the screening of various chemicals(oxi-dants,surfactants,bactericidal and hydrolyzing agents)to remove biomass from samples taken from a toluene-degrading biotrickling®lter. Experimental conditions were chosen in order to simulate future industrial treatment in biotrickling ®lters.As an inhibitory action on bacteria by the selected chemicals was expected,the biological ac-tivity of unremoved biomass was also measured.MATERIALS AND METHODSBiomass samplesBiomass was grown on the surface of2.5cm polypropy-lene Pall rings(Flexirings1,Koch Engineering,Wichita, KS)in a source biotrickling®lter(1.3m in height,0.152m in diameter)degrading toluene.The inoculum was a bac-terial species isolated from sludge using toluene as the sole carbon and energy pressed air with a metered amount of toluene resulting in an average inlet concen-tration of1.03g mÀ3was passed through the reactor from top to bottom at a volumetric load of64m3mÀ3hÀ1, resulting in a toluene load of65.9g mÀ3hÀ1.Liquid was recirculated concurrently with the air¯ow at a super®cial velocity of7.9m hÀ1and a mineral medium(Cox and Deshusses,1997)was continuously fed at a¯ow rate of 0.24l hÀ1.Pall rings with biomass were randomly taken from the upper part of the source biotrickling®lter after 79to147days of operation for the experiments described below.Experimental set-upRemoval of biomass was investigated in a small test bio-trickling®lter(0.3m in height,diameter0.152m)stacked with clean Pall rings.A schematic of the experimental set-up is shown in Fig.1and a picture of biomass attached on the Pall rings is shown in Fig.2.For each treatment,®ve rings with biomass were taken from the source bio-trickling®lter,weighed after10min of drying in air(wet biomass weight plus ring before treatment)and placed at regular intervals along the longitudinal axis of the test bio-trickling®lter.In most experiments,0.75l of liquid con-taining the tested chemical was recirculated for3h over the packed bed at a super®cial liquid velocity of7.9m hÀ1 using a centrifugal pump(model1P677A from Dayton Electric Mfg.Co.,Chicago,IL)and a needle valve and ¯owmeter combination.At the top of the test biotrickling ®lter,a liquid distribution system made of a container with equally spaced holes ensured homogenous distri-bution of the recycle liquid over the packed bed.All treat-ments lasted for3h(except for a treatment with hot water which lasted30min)and was ended by stopping the liquid recirculation.The liquid was then allowed to drain from the test biotrickling®lter for10min and collected for analysis of suspended solids and total dissolved carbon. The rings with unremoved biomass were carefully removed from the test biotrickling®lter,air-dried for10min and weighed(wet biomass weight plus ring after treatment). Unremoved biomass was separated from the Pall rings and immediately analyzed for biological activity in oxygen uptake rate(OUR)experiments.The Pall rings were cleaned,dried and weighed in order to calculate the amount of wet biomass before and after treatment.The e ciency of biomass removal was calculated as the percen-tage removal of wet biomass.In total,18di erent treatments were investigated.The principal action mechanism of each chemical is listed in Table1.The general procedure as outlined above was fol-lowed for the treatment with demineralized water(control experiment,H2O),0.04and0.4%(w/v)sodium hydroxide (NaOH),0.1and0.5%(w/v)sodium dodecylsulfate (SDS),0.01%(w/v)NaOH with0.1%(w/v)SDS,0.05Fig.1.Schematic of the experimental set-up.H.H.J.Cox and M.A.Deshusses2384and 0.2%(w/v)sodium azide (NaN 3),0.011,0.26and 1.31%(w/v)sodium hypochlorite (NaClO),1.1and 11.3%(w/v)hydrogen peroxide (H 2O 2),saturated iodine in 4%(v/v)ethanol [0.02%(w/v)I 2as determined by titration with Na 2S 2O 3],0.9%(w/v)ammonia (NH 3)and 0.9%(w/v)NH 3in combination with 0.22%(w/v)formaldehyde (HCO).For the treatment with demineralized water at el-evated temperature (H 2O,T =638C),a heating plate was used to maintain 1.2l of recycle liquid at 658C.This treat-ment lasted for only 30min and the average temperature inside the column was 638C.For the treatment with ozone,demineralized water was circulated over the column for 3h while ozone-containing air was passed counter-cur-rently at a ¯ow rate of 1.9l min À1.Ozone was prepared with an ozone generator model 100-O2from California Acrylic Industries (Pomona,CA).The ozone concen-tration in the gas phase was 0.5g m À3as determined by ti-tration with Na 2S 2O 3after trapping of ozone in 2%KI (APHA et al.,1985).Analytical proceduresThe dry matter content of the biomass was determined in quadruplicate by drying,at 958C overnight,known amounts of wet biomass,taken from the source biotrick-ling ®lter after 162days of operation.Analyses of the dried biomass for C,H and N content were done by Desert Analytics Laboratory (Tucson,AZ).Suspended solids in the liquid after treatment were determined bycentrifugation at 17,000g for 10min and by drying the pel-let at 958C overnight.Total dissolved carbon in the liquid after treatment was determined in triplicate by analyzing the supernatant using a model TOC-5050total carbon analyzer (Shimadzu,Kyoto,Japan).The activity of the biomass remaining on the rings after the treatment was determined in OUR experiments.Each of the 5rings was placed into 100ml of mineral medium and shaken vigor-ously to separate the biomass from the ring.OURs with and without 0.19mM toluene were determined in single experiments at room temperature in a custom made 57ml glass vial and by using an oxygen electrode and meter (Orion model 860,Boston,MA).OURs were related to the amount of unremoved,wet biomass and toluene-induced OURs were corrected for endogenous respiration.RESULTSCharacterization of biomass samplesBiomass sampling from the source biotrickling ®l-ter started after 79days of toluene supply,at which time the reactor contained 14.065kg wet biomass (approximately 60%of the reactor volume).The average amount of wet biomass on the Pall rings was 11.2g (average of 90rings,a range of 7.1to 16.5g with a standard deviation of 1.9g).Since the biomass content of the bioreactor was high,bio-mass was present both inside and on the outer sur-face of the rings.Assuming a bio®lm density of 1kg l À1and that Pall rings can be represented by empty cylinders,the maximum amount of wet bio-mass needed to ®ll the ring would be 12.3g.The average amount of biomass on the rings (11.2g)in-dicates that the amount of biomass on the outer surface was small compared to the amount ofbio-Fig.2.Picture of a clean Pall ring (left)and one covered with biomass (right)before cleaning.Since the biomass content was very high,a large portion of the inner volume of the ring was ®lled with biomass.Table 1.Principal action mechanisms of the chemicals tested toremove excess biomass in biotrickling ®lters CompoundMechanism H 2O none,control NaOHhydrolysis NaClO,H 2O 2,O 3,I 2oxidation SDS surfactant NaN 3toxic/reductionWater (638C),NH 3,NH 3+HCOotherRemoval of biomass from biotrickling ®lters 2385mass inside the ring.This was con®rmed by visual observation (Fig.2).The average dry matter con-tent of the biomass was 4.86%.Elemental analysis of the dried biomass showed a C:H:N ratio of 43.4:6.2:7.8%on a dry weight basis.Chemical removal of biomassThe control experiment with recirculation of demineralized water resulted in an average loss of wet biomass of 12.9%.Visual observation indicated that mainly biomass from the outer surface of the rings was removed,whereas biomass inside the rings was still present.Since no chemical reaction between water and biomass is expected,selective removal of biomass from the outer surface of the rings in this case was due to shear stresses caused by trickling of liquid on the rings in the column and/or to losses during handling of the rings.Table 2summarizes the wet biomass removal e -ciencies of the treatments investigated as well as pH measurements of the liquid before and after treat-ment.Most treatments had about the same removal e ciency as the control treatment with water.Only treatments with 0.4%(w/v)NaOH,0.26and 1.31%(w/v)NaClO and 11.3%(w/v)H 2O 2resulted in statistically signi®cant higher removal e ciencies (Student t -test,p >0.95).Biomass in the treatments using 0.26and 1.31%(w/v)NaClO and 11.3%(w/v)H 2O 2turned from an initial brown color to yel-lowish-white at the end of the treatment.This was not observed in treatments with 0.011%(w/v)NaClO nor with 1.1%(w/v)H 2O 2.In treatments using NaOH,only the 0.4%(w/v)NaOH appli-cation resulted in the remaining biomass becoming a highly viscous liquid.All treatments with SDS resulted in signi®cant parison of the pH during treatment and removal e ciencies at0.04and 0.4%NaOH (w/v)(Table 2)indicates that biomass removal induced by an alkaline environ-ment may be expected at a pH of about 12.4and higher.Analysis of the liquid after treatmentBiomass reduction may either be due to bio®lm detachment (cells and ¯ocs),to solubilization of biomass by chemical reaction or to oxidation of biomass to volatile compounds.In order to quan-tify these processes,the amounts of suspended solids and total dissolved carbon in the liquid after treatment were determined.It should be noted that larger ¯ocs tended to be captured on clean Pall rings below the test rings in the test biotrickling ®l-ter and thus determination of suspended solids in the liquid is an underestimation of bio®lm detach-ment.The liquid after treatment contained small amounts of suspended solids and dissolved carbon in cases of treatments with low biomass removal e ciencies,whereas the highest amounts were found in treatments with high biomass removal e -ciencies (Table 3).Since the total amounts of wet biomass on ®ve rings before and after treatment were known and biomass dry matter and carbon content were deter-mined,mass balances were calculated for the treat-ments with high removal e ciencies.As reported in Table 4,treatment with 0.4%(w/v)NaOH resulted in a slightly higher recovery of removed biomass as suspended solids,which may indicate that detach-ment of cells and bio®lm ¯ocs was a more import-ant process in this treatment as compared to the treatments with NaClO and H 2O 2.Marked di er-ences were found in the recovery of removed C-bio-mass as dissolved carbon,with values ranging from 18.5%for the treatment with 11.3%(w/v)H 2O 2toTable 2.Wet biomass removal e ciency from Pall rings during 3h treatment in a test biotrickling ®lterTreatment No.TreatmentWet biomass removal e ciency (%)a pH liquidstart end 1H 2O12.9(11.0)ND c 7.62H 2O,T =638C b 14.6(11.5)ND 8.030.4%(w/v)NaOH 50.2(22.4)12.812.440.04%(w/v)NaOH 10.3(9.8)12.09.550.5%(w/v)SDS 10.9(7.0) 6.78.060.1%(w/v)SDS10.4(12.0) 6.27.470.04%(w/v)NaOH +0.1%(w/v)SDS9.6(5.1)11.89.280.2%(w/v)NaN 320.4(8.0)7.87.990.05%(w/v)NaN 3 2.8(6.7)7.48.310 1.31%(w/v)NaClO 77.0(16.1)12.19.1110.26%(w/v)NaClO 49.2(13.2)11.49.0120.011%(w/v)NaClO 14.3(5.6)ND 8.01311.3%(w/v)H 2O 269.0(19.4) 4.3 6.514 1.1%(w/v)H 2O 228.2(16.0) 5.17.3150.02%(w/v)I 210.8(3.7) 5.47.4160.5g O 3m À3air 5.9(3.2)ND ND 170.9%(w/v)NH 36.5(6.4)11.59.3180.9%(w/w)NH 3+0.22%(w/v)HCO9.9(11.8)11.210.2aAverage of ®ve determinations,standard deviation (s n À1)in parentheses.b 30min treatment.c Not determined.H.H.J.Cox and M.A.Deshusses238665.3%for the treatment with0.4%(w/v)NaOH. No attempts were made to close dry matter and carbon balances,which would have required analy-sis of the liquid for dissolved solids and analysis of the gas phase for production of CO2and volatile organic compounds.However,assuming that the carbon content in suspended solids was the same as that in the biomass before treatment,the ratio of C-liquid produced to C-biomass removed in the treatments with0.4%(w/v)NaOH,0.26and1.31% (w/v)NaClO and11.3%(w/v)H2O2was88,60,63 and33%,respectively(Table4).These results may indicate that oxidation of biomass to gaseous or volatile compounds was signi®cant in the treatments using oxidants,such as NaClO and especially H2O2. Biological activity of unremoved biomass Endogenous and toluene-induced OURs of fresh biomass analyzed before chemical treatment were on average1.31and1.46m g O2gÀ1wet biomass-minÀ1,respectively.In the control experiment with water,unremoved biomass had approximately the same activity(Table5).This indicates that3h of trickling of a liquid on the test rings in the test bio-trickling®lter is in itself not a cause for loss of bio-logical activity.The data reported in Table5showsTable3.Removal of biomass from Pall rings as suspended solids and total dissolved carbon Treatment Suspended solids(SS)Dissolved carbon(C)total (mg)biomass recovered as SS(%)atotal(mg)biomass recovered as dissolved C(%)bH2O45 2.310 1.2H2O,T=638C128 6.240 4.50.4%(w/v)NaOH19411.224732.80.04%(w/v)NaOH81 3.311010.50.5%(w/v)SDS54 2.5132d14.30.1%(w/v)SDS70 3.30d00.04%(w/v)NaOH+0.1%(w/v)SDS31 1.5110d12.00.2%(w/v)NaN351 1.915 1.30.05%(w/v)NaN328 1.611 1.41.31%(w/v)NaClO24511.235337.30.26%(w/v)NaClO155 6.822422.60.011%(w/v)NaClO82 3.937 4.111.3%(w/v)H2O21879.910512.81.1%(w/v)H2O21727.739 4.00.02%(w/v)I277 4.1ND ND0.5g O3mÀ3air ND c ND ND ND0.9%(w/v)NH339 1.512511.10.9%(w/w)NH3+0.22%(w/v)HCO54 2.121d 1.9a Dry suspended solids in the liquid after treatment,as percentage of the total amount of dry biomass present on®ve Pall rings before treatment(dry biomass=0.0486Âwet biomass).b Total dissolved carbon in the liquid after treatment,as percentage of the total amount of C-biomass present on®ve Pall rings before treatment(C-biomass=0.4339Âdry biomass).c Not determined.d Values corrected for the presence of carbon containing reagents,assuming that the reagent concentration at the end of the treatment was the same as initially pre-sent.Table4.Mass balances for four treatments with the highest biomass removal e ciencyParameter Treatment0.4%(w/v) NaOH 0.26%(w/v)NaClO1.31%(w/v)NaClO11.3%(w/v)H2O2Dry biomassBiomass before treatment(g) 1.733 2.282 2.182 1.892 Biomass removed a(g)0.870 1.123 1.680 1.306 Recovered as suspended solids(g)0.1940.1550.2450.187 Recovery of removed biomass as suspended solids(%)22.313.814.614.3CarbonC-biomass before treatment(g)0.7520.9900.9470.821 C-biomass removed b(g)0.3780.4870.7290.567 Recovered as dissolved C(g)0.2470.2240.3530.105 Recovery of removed C-biomass as dissolved C(%)65.346.048.418.5 Total carbon recovery c(%)88606333a Assuming that the dry matter content in biomass before and after treatment is the same(0.0486g dry biomass gÀ1wet biomass).b Assuming that the carbon content of dry biomass before and after treatment is the same(0.4339g C gÀ1dry biomass).c(Suspended solidsÂ0.4339+dissolved carbon)/C-biomass removed.Removal of biomass from biotrickling®lters2387that chemical treatments caused various levels of inhibition of biological activity of the remaining biomass,depending on the chemical used.Interestingly,toluene oxidation generally appeared to be more inhibited than endogenous respiration,but this was not further investigated.From the four treatments with high biomass removal e ciencies,only unremoved biomass in the treatment with 0.4%(w/v)NaOH showed some level of biologicalactivity.In fact,toluene oxidation activity was found in only one sample out of the ®ve examined.Treatments with 0.26and 1.31%(w/v)NaClO and 11.3%(w/v)H 2O 2resulted in a complete loss of respiration activity of the unremoved biomass.In these cases it was found that residual H 2O 2and NaClO concentrations in suspensions for OUR ex-periments (i.e.after ten-fold dilution of unremoved biomass in mineral medium and assuming that the biomass was saturated with the reagent in a concen-tration as initially present at the start of the treat-ment)were su ciently high to cause complete inhibition of respiration activity of fresh and untreated biomass.DISCUSSIONThe e ciency of biomass removal of biomass from Pall rings was investigated in a laboratory test biotrickling ®lter at a super®cial liquid velocity commonly used in biotrickling ®lter operation.Experimental conditions were chosen to simulate chemical removal of biomass in full-scale biotrick-ling ®lters,with the exception being that the Pall rings with biomass in the test biotrickling ®lter were surrounded by clean Pall rings.The high ratio of re-agent to biomass may have facilitated reaction in the test biotrickling ®lter and,consequently,removal e ciencies as reported in Table 2may be higher than with actual treatment in biotrickling ®l-ters under otherwise identical conditions.Asindi-Fig.3.Correlation between toluene-induced oxygen uptake rate in the biomass remaining after treat-ment and wet biomass removal e ciency of the various treatments (see Table 2for identi®cation ofeach treatment).The inset shows target characteristics for various treatments.Table 5.Biological activity of unremoved biomass measured as the oxygen uptake rate (m g O 2g À1wet biomass min À1;average of ®ve determinations with standard deviation (s n À1)in parentheses)TreatmentEndogenous Toluene-induced H 2O1.21(0.27)1.92(0.54)H 2O,T =638C 00.4%(w/v)NaOH 0.76(0.52)0.12(0.26)0.04%(w/v)NaOH 1.27(0.22)0.06(0.11)0.5%(w/v)SDS 1.54(0.51)0.59(0.37)0.1%(w/v)SDS2.54(0.59)0.59(0.70)0.04%(w/v)NaOH +0.1%(w/v)SDS1.69(0.78)0.15(0.18)0.2%(w/v)NaN 30.55(0.11)0.27(0.21)0.05%(w/v)NaN 3 1.45(0.39)1.13(0.70)1.31%(w/v)NaClO 000.26%(w/v)NaClO 00.011%(w/v)NaClO 0.93(0.15)0.78(0.43)11.3%(w/v)H 2O 201.1%(w/v)H 2O 20.24(0.21)0.09(0.15)0.02%(w/v)I 2 1.63(0.41)0.64(0.32)0.5g O 3m À3air 1.21(0.58)0.82(0.38)0.9%(w/v)NH 30.67(0.33)0.05(0.09)0.9%(w/w)NH 3+0.22%(w/v)HCOH.H.J.Cox and M.A.Deshusses2388cated by the high standard deviation,removal e -ciencies per treatment sometimes varied consider-ably(Table2).Since the distribution of biomass on the ring was di erent for each sample,access of liquid to the biomass may have di ered as well. This may also have resulted in di erent ratios of inactive to active biomass in samples within each treatment,causing large¯uctuations in the biologi-cal activity of unremoved biomass as observed in OUR experiments(Table5).Chemical treatment of clogged biotrickling®lters should result in a high e ciency of biomass removal to reduce the frequency of cleaning. Ideally,chemical treatment should also have mini-mum adverse e ects on the activity of biomass remaining on the rings in order to reduce the time of recovery of the biotrickling®lter after treatment (Fig.3,Inset).In Fig.3,the toluene oxidation ac-tivity of the unremoved biomass after the treatment is plotted vs biomass removal e ciencies.Many treatments resulted in partial or complete inacti-vation of the biomass without signi®cant removal of biomass.These include the treatments with SDS (a surfactant commonly used with NaOH in an alkali lysis procedure to disrupt cell membranes; Sambrook et al.,1989),I2(an alternative for NaClO/Cl2in wastewater disinfection;White, 1972),NaN3(an inhibitor of the respiratory chain at concentrations of0.0065±0.065%;Heinen,1971) or water at638C.Biomass removal e ciencies could have been better for these treatments if higher reagent concentrations were tested.OUR exper-iments however show that these treatments should probably not be considered because of their toxicity at low concentration.The low biomass removal e -ciency in these and other treatments is most prob-ably due to the fact that cells in a bio®lm are embedded in a polymer matrix.This may have served as a means to increase cell detention but did not o er much protection against the di usion of aggressive chemicals.It further suggests that suc-cessful development of biomass washing strategies should consider chemicals that have a proven e ect on the components of the polymer matrix. Treatment with ozone did not result in biomass removal,although ozone is a stronger oxidant than NaClO(E0=1.24and0.90mV,respectively;Lide, 1996).Apparently the ozone concentration in the gas phase(0.5g mÀ3)and the corresponding con-centration in the liquid phase(0.1mg lÀ1,dimen-sionless Henry coe cient of0.2;White,1972)were too low to e ectively oxidize or remove biomass. This is further supported by Siddiqui et al.(1997) who observed that removal of biodegradable dis-solved organic carbon(BDOC)from drinking water sources was optimal at an applied ozone to DOC ratio of2:1(g gÀ1).Although ozone treatment of BDOC and immobilized biomass may not be directly comparable,the results of Siddiqui et al. (1997)indicate that the ozone to carbon ratio applied in our experiment was too low(0.25g gÀ1). The economical and ecological impacts of using a 2:1ratio to remove excess biomass in biotrickling ®lters is not yet known.No treatment was found that can combine a high biomass removal e ciency and high toluene oxi-dation activity of the biomass remaining after treat-ment(Fig.3).OUR experiments did not allow for distinction between loss of activity due to cell death during chemical treatment and permanent or tem-porary inhibition of still viable cells.Also,since unremoved biomass was not washed after treatment (to simulate industrial operation),residual reagents may have a ected OUR experiments.In control ex-periments with H2O2and NaClO,calculated re-sidual reagent concentrations were su ciently high to completely inhibit respiration of fresh biomass, even after a ten-fold dilution to suspend the bio-mass for OUR experiments.H2O2and NaClO are well-known disinfectants and concentrations used in this study were several orders of magnitude higher than that required for disinfection(White,1972). The present results indicate that rapid recovery of biotrickling®lters after treatment with NaClO or H2O2may be hindered by both cell death during treatment as well as the presence of residual reagent in unremoved biomass after treatment.This suggests that post treatments using a reducing agent in the case of a chemical wash with an oxidant or using an acid or pH bu er after an alkali treatment might be bene®cial.Such a post treatment would neutralize the active chemical and eliminate the e ect of any residual chemicals.This could poten-tially speed up the recovery of the process culture ®lter after chemical treatment.From the four treat-ments with signi®cantly higher biomass removal e ciencies,only biomass after treatment with0.4% (w/v)NaOH showed some biological activity in OUR experiments.This speaks in favor of the use of NaOH to control biomass accumulation in bio-trickling®lters.Weber and Hartmans(1996)indeed observed that the toluene elimination capacity of a biotrickling®lter was fully restored within one day after treatment with0.4%(w/v)NaOH.They also found that the NaOH washes resulted in an average removal of230g dry biomass from a71l reactor, i.e.3.2kg dry biomass mÀ3.The percentage biomass removal in the case of Weber and Hartmans(1996) could not be calculated and compared to the results of the present research,because the amount of bio-mass in their reactor was not determined.However, a removal of3.2kg dry biomass mÀ3reactor volume is insu cient for controlling biomass in high per-formance biotrickling®lters that are subject to rapid clogging.Our source-biotrickling®lter gained approximately0.37kg dry biomass mÀ3reactor-dayÀ1,which would require treatment with0.4% (w/v)NaOH once every8.7days to maintain a con-stant biomass concentration in the reactor.A more e ective reagent may be desired to obtain a higherRemoval of biomass from biotrickling®lters2389。
生物炭促进针铁矿类芬顿氧化降解氧氟沙星研究
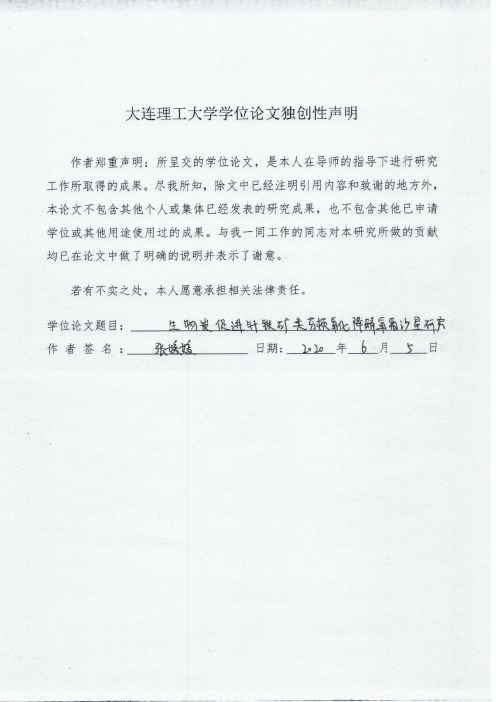
大连理工大学硕士学位论文摘要氧氟沙星(OFX) 是广泛应用于医疗、养殖和畜牧等行业中的喹诺酮类抗生素,其在生产和使用过程中进入环境会造成潜在的生态风险。
研究表明,以含铁矿物等作为催化剂的非均相类芬顿氧化技术能够用于抗生素的降解。
然而,含铁矿物催化的非均相类芬顿体系中Fe(III)/Fe(II) 循环缓慢,提高Fe(III)/Fe(II) 转化效率成为改善非均相类芬顿体系催化活性的关键。
研究表明,向类芬顿体系中添加某些还原剂和配合剂能够促进Fe(III)/Fe(II) 转化效率,提高类芬顿降解效果。
作为限氧条件下热解生物质的产物,生物炭(biocahr, BC) 来源广泛、成本低廉,且具有良好的吸附及配合金属离子能力和氧化还原活性。
有鉴于此,本研究探索利用BC强化针铁矿(Gt) 催化类芬顿反应降解OFX。
利用小麦秸秆在300 °C或600 °C条件下分别热解制备生物炭样品BC300和BC600。
将上述BC样品加入Gt类芬顿体系可显著促进体系氧化降解OFX。
在4 h内,Gt/H2O2和BC600/H2O2体系中只有38.4%和48.4%的OFX (20 mg/L) 被去除,而在Gt/BC600/H2O2体系中,OFX去除效率大于94.0%。
Gt/H2O2、BC600/H2O2和Gt/BC600/H2O2体系的准一级动力学速率常数分别为0.12、0.16和0.72 h-1,表明Gt-BC 共存类芬顿体系中发生了协同催化降解。
与BC300相比,在较高的热解温度下产生的BC600可以更好地促进OFX的降解。
在Gt-BC共存类芬顿体系中,当Gt浓度为0.2 g/L,BC600浓度为0.3 g/L,H2O2浓度为2 mM,pH为3时,OFX的降解效果最佳,4 h内可达94.2%。
在连续四次重复使用过程中,Gt/BC600/H2O2体系对OFX的去除效率分别为94.2%,87.8%,82.4%和75.5%,表明其具有较好的催化降解稳定性。
生物炭
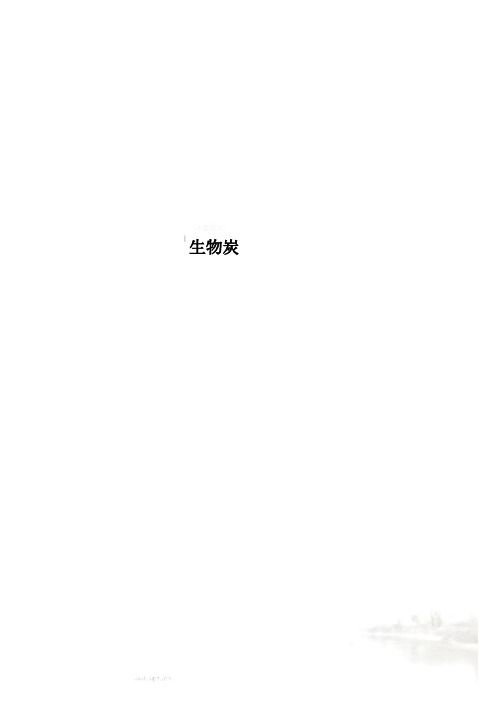
生物炭化学前沿论文论文题目:生物炭的应用(综述)课程名称:化学前沿学院:化学与化工学院学生姓名:**学号:**********指导老师:**2015年7 月28 日生物炭的应用(综述)摘要近年来,随着南美亚马逊流域考古发现黑土(black earths)及对其进行深入的研究,了解到生物炭不仅能改变土壤的pH、改变土壤的有机质及土壤的水分,还能改变不同农作物的形态特征,并且能提高全球粮食安全保障,它更能减缓全球气候变化。
本文将从生物炭的元素组成及其物理化学性质;生物炭的制备和对土壤理化性质的影响生,物炭对作物物态特征和养分吸收的影响等方面进行综述,以便人们对生物炭有更进一步的了解和重视,从而减少因对生物炭的生产及农用重视程度不够而造成资源的大量浪费。
并且用废弃生物质生产生物炭,实现可持续发展道路。
关键词:生物炭、物化特征、影响、展望。
Application of biological carbon (review)AbstractIn recent years, with the South American Amazon River Basin earths black (black earths) and its in-depth study,To understand the biological carbon can not only change the soil pH, soil organic matter and soil moisture, it can also change the morphological characteristics of different crops, and can improve global food security, it can mitigate global climate change. The from the biological carbon elemental composition and physical and chemical properties; biological activated carbon prepared and on soil physical and chemical properties of effects, carbon on state characteristics of crops and nutrient absorption effect were reviewed, so that people of biochar has further understanding and recognition, thereby reducing the result of biochar production and agricultural utilization degree of attention is not enough to cause resources wasting. And the use of waste biomass production of biological carbon, to achieve sustainable development path.Key words: biological carbon, physical and chemical characteristics, influence and Prospect.引言自从进入21 世纪以来,人们逐渐遭到“环境、能源危机、粮食”等各种因素的影响,并且情况越来越变得严重,因此,不同国家的政府、专家、学者等都在探索着各种能够解决这些危机的办法,但效果却不是很明显。
生物炭对植物土传病害的影响与作用
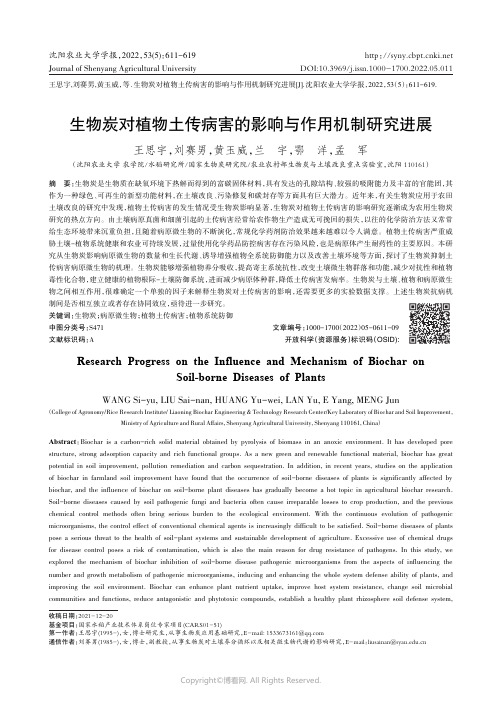
王思宇,刘赛男,黄玉威,等.生物炭对植物土传病害的影响与作用机制研究进展[J].沈阳农业大学学报,2022,53(5):611-619.沈阳农业大学学报,2022,53(5):611-619Journal of Shenyang Agricultural University http :// DOI:10.3969/j.issn.1000-1700.2022.05.011收稿日期:2021-12-20基金项目:国家水稻产业技术体系岗位专家项目(CARS01-51)第一作者:王思宇(1995-),女,博士研究生,从事生物炭应用基础研究,E-mail:*****************通信作者:刘赛男(1985-),女,博士,副教授,从事生物炭对土壤养分循环以及相关微生物代谢的影响研究,E-mail :******************.cn 生物炭对植物土传病害的影响与作用机制研究进展王思宇,刘赛男,黄玉威,兰宇,鄂洋,孟军(沈阳农业大学农学院/水稻研究所/国家生物炭研究院/农业农村部生物炭与土壤改良重点实验室,沈阳110161)摘要:生物炭是生物质在缺氧环境下热解而得到的富碳固体材料,具有发达的孔隙结构、较强的吸附能力及丰富的官能团,其作为一种绿色、可再生的新型功能材料,在土壤改良、污染修复和碳封存等方面具有巨大潜力。
近年来,有关生物炭应用于农田土壤改良的研究中发现,植物土传病害的发生情况受生物炭影响显著,生物炭对植物土传病害的影响研究逐渐成为农用生物炭研究的热点方向。
由土壤病原真菌和细菌引起的土传病害经常给农作物生产造成无可挽回的损失,以往的化学防治方法又常常给生态环境带来沉重负担,且随着病原微生物的不断演化,常规化学药剂防治效果越来越难以令人满意。
植物土传病害严重威胁土壤-植物系统健康和农业可持续发展,过量使用化学药品防控病害存在污染风险,也是病原体产生耐药性的主要原因。
本研究从生物炭影响病原微生物的数量和生长代谢、诱导增强植物全系统防御能力以及改善土壤环境等方面,探讨了生物炭抑制土传病害病原微生物的机理。
生物炭制备及其吸附应用研究进展
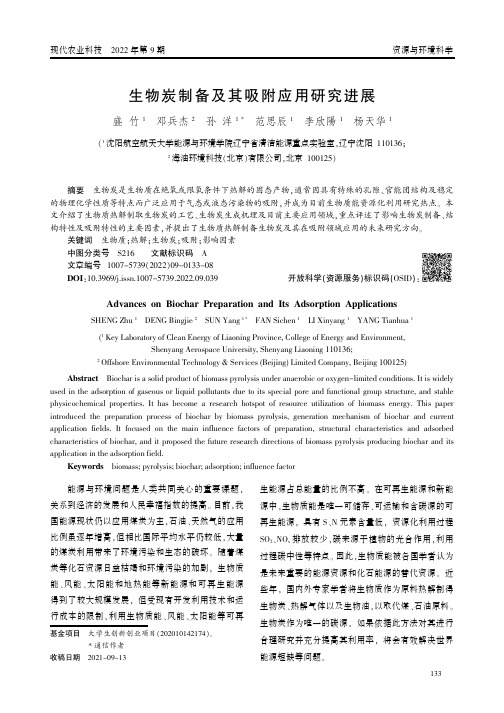
基金项目大学生创新创业项目(202010142174)。
*通信作者收稿日期2021-09-13摘要生物炭是生物质在绝氧或限氧条件下热解的固态产物,通常因具有特殊的孔隙、官能团结构及稳定的物理化学性质等特点而广泛应用于气态或液态污染物的吸附,并成为目前生物质能资源化利用研究热点。
本文介绍了生物质热解制取生物炭的工艺、生物炭生成机理及目前主要应用领域,重点评述了影响生物炭制备、结构特性及吸附特性的主要因素,并提出了生物质热解制备生物炭及其在吸附领域应用的未来研究方向。
关键词生物质;热解;生物炭;吸附;影响因素中图分类号S216文献标识码A 文章编号1007-5739(2022)09-0133-08DOI :10.3969/j.issn.1007-5739.2022.09.039开放科学(资源服务)标识码(OSID ):Advances on Biochar Preparation and Its Adsorption ApplicationsSHENG Zhu 1DENG Bingjie 2SUN Yang 1*FAN Sichen 1LI Xinyang 1YANG Tianhua 1(1Key Laboratory of Clean Energy of Liaoning Province,College of Energy and Environment,Shenyang Aerospace University,Shenyang Liaoning 110136;2Offshore Environmental Technology &Services (Beijing)Limited Company,Beijing 100125)Abstract Biochar is a solid product of biomass pyrolysis under anaerobic or oxygen-limited conditions.It is widely used in the adsorption of gaseous or liquid pollutants due to its special pore and functional group structure,and stable physicochemical properties.It has become a research hotspot of resource utilization of biomass energy.This paper introduced the preparation process of biochar by biomass pyrolysis,generation mechanism of biochar and current application fields.It focused on the main influence factors of preparation,structural characteristics and adsorbed characteristics of biochar,and it proposed the future research directions of biomass pyrolysis producing biochar and its application in the adsorption field.Keywords biomass;pyrolysis;biochar;adsorption;influence factor生物炭制备及其吸附应用研究进展盛竹1邓兵杰2孙洋1*范思辰1李欣陽1杨天华1(1沈阳航空航天大学能源与环境学院辽宁省清洁能源重点实验室,辽宁沈阳110136;2海油环境科技(北京)有限公司,北京100125)能源与环境问题是人类共同关心的重要课题,关系到经济的发展和人民幸福指数的提高。
奖种2019年度环境技术进步奖
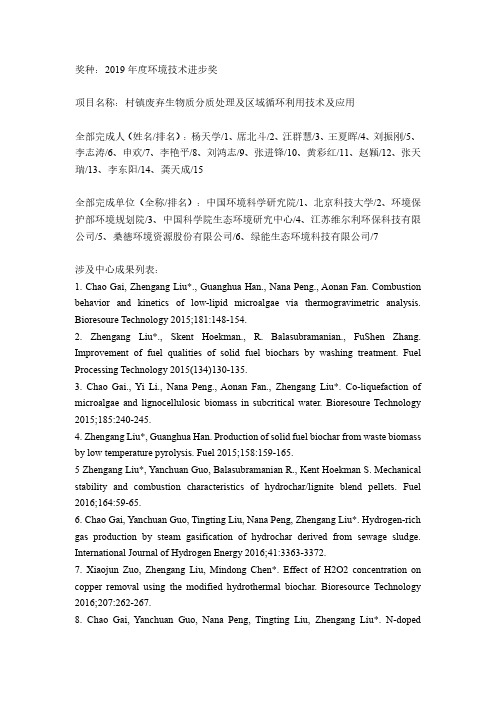
奖种:2019年度环境技术进步奖项目名称:村镇废弃生物质分质处理及区域循环利用技术及应用全部完成人(姓名/排名):杨天学/1、席北斗/2、汪群慧/3、王夏晖/4、刘振刚/5、李志涛/6、申欢/7、李艳平/8、刘鸿志/9、张进锋/10、黄彩红/11、赵颖/12、张天瑞/13、李东阳/14、龚天成/15全部完成单位(全称/排名):中国环境科学研究院/1、北京科技大学/2、环境保护部环境规划院/3、中国科学院生态环境研究中心/4、江苏维尔利环保科技有限公司/5、桑德环境资源股份有限公司/6、绿能生态环境科技有限公司/7涉及中心成果列表:1. Chao Gai, Zhengang Liu*., Guanghua Han., Nana Peng., Aonan Fan. Combustion behavior and kinetics of low-lipid microalgae via thermogravimetric analysis. Bioresoure Technology 2015;181:148-154.2. Zhengang Liu*., Skent Hoekman., R. Balasubramanian., FuShen Zhang. Improvement of fuel qualities of solid fuel biochars by washing treatment. Fuel Processing Technology 2015(134)130-135.3. Chao Gai., Yi Li., Nana Peng., Aonan Fan., Zhengang Liu*. Co-liquefaction of microalgae and lignocellulosic biomass in subcritical water. Bioresoure Technology 2015;185:240-245.4. Zhengang Liu*, Guanghua Han. Production of solid fuel biochar from waste biomass by low temperature pyrolysis. Fuel 2015;158:159-165.5 Zhengang Liu*, Yanchuan Guo, Balasubramanian R., Kent Hoekman S. Mechanical stability and combustion characteristics of hydrochar/lignite blend pellets. Fuel 2016;164:59-65.6. Chao Gai, Yanchuan Guo, Tingting Liu, Nana Peng, Zhengang Liu*. Hydrogen-rich gas production by steam gasification of hydrochar derived from sewage sludge. International Journal of Hydrogen Energy 2016;41:3363-3372.7. Xiaojun Zuo, Zhengang Liu, Mindong Chen*. Effect of H2O2 concentration on copper removal using the modified hydrothermal biochar. Bioresource Technology 2016;207:262-267.8. Chao Gai, Yanchuan Guo, Nana Peng, Tingting Liu, Zhengang Liu*. N-dopedbiochar derived from co-hydrotermal carbonization of rice husk and chlorella pyrenoidosa for enhancing copper ions adsorption. RSC Adv 2016;6:53713-53722. 9. Nana Peng, Yi Li, Tingting Liu, Chao Gai, Zhengang Liu*. Emission, distribution and toxicity of polycyclic aromatic hydrocarbons (PAHs) during municipal solid waste (MSW) and coal co-combustion. Science of The Total Environment 2016;565:1201-1207.10. Nana Peng, Zhengang Liu*, Tingting Liu, Chao Gai. Emissions of polycyclic aromatic hydrocarbons (PAHs) during hydrothermally treated municipal solid waste combustion for energy generation. Applied Energy 2016;184:396-403.11. Chao Gai, Mengjun Chen., Zhengang Liu*. Gasification characteristics of hydrochar and pyrochar derived from sewage sludge. Energy 2016;113:957-965. 12. Chao Gai, Fang Zhang, Qianqian Lang., Tingting Liu., Nana Peng., Zhengang Liu*. Facile one-pot synthesis of iron nanoparticles immobilized into the porous hydrochar for catalytic decomposition of phenol. Applied Catalysis B: Environment 2017;204:566-76.13. Nana Peng, Yi Li, Tingting Liu, Qianqian Lang, Chao Gai, Zhengang Liu*. Polycyclic aromatic hydrocarbons and toxic heavy metals in municipal solid waste and corresponding hydrochars. Energy and Fuel 2017;31:1665-1671.14. Chao Gai, Fang Zhang, Yanchuan Guo, Nana Peng, Tingting Liu, Qianqian Lang, Yu Xia, Zhengang Liu*. Hydrochar-supported, in-situ generated nickel nanoparticles for sorption-enhanced catalytic gasification of sewage sludge. ACS Sustainable Chemistry&Engineering 2017;5:7613-7622.15. Chao Gai,Fang Zhang, Tianxue Yang, Zhengang Liu*., Wentao Jiao, Nana Peng, Tingting Liu, Qianqian Lang, Yu Xia. Hydrochar supported bimetalliuc Ni-Fe nanocatalysts with tailored composition, size and shape for improved biomass steam reforming performance. Green Chemistry 2018,20,2788-2800.16. Pin Ma, Wenli Lu, Xiaoying Yan, Wendan Li, Li Li, Yanyan Fang, Xiong Yin*, Zhengang* Liu, Yuan lin.Heteroatom tri-doped porous carbon derived from waste biomass as Pt-free counter electrode in dye-sensitized solar cells. RSC Adv. 2018,8,18427-18433.17. Jing Ma, Mengjun Chen, Tianxue Yang, Zhengang Liu*, Wentao Jiao, Dong Li, Chao Gai. Gasification performance of the hydrochar derived from co-hydrothermal carbonization of sewage sludge and sawdust. Energy 2019,173:732-739.18. Chao Gai, Nengmin Zhu, S.K. Hoekman, Zhengang Liu*, Wentao Jiao, Nana Peng.Highly dispersed nickel nanoparticles supported on hydrochar for hydrogen-rich syngas production from catalytic reforming of biomass. Energy Conversion and Management 2019,183:474-484.。
农机购置与应用补贴产品自主投档典型错误解析
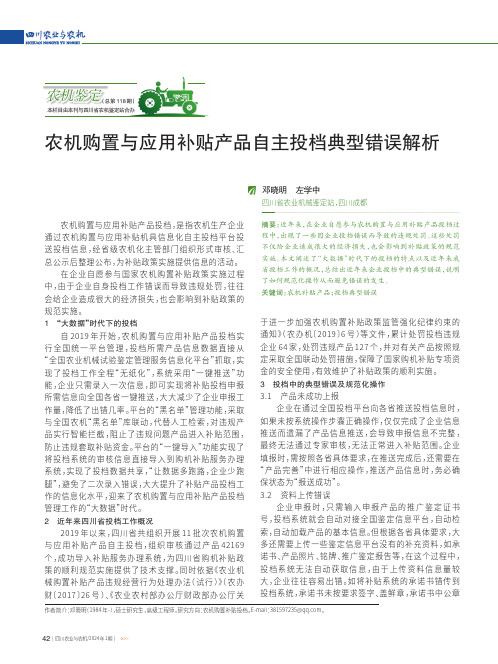
|四川农业与农机/2024年1期|>>>NONGYE YU NONGJI(总第118期)本栏目由本刊与四川省农机鉴定站合办农机鉴定农机购置与应用补贴产品自主投档典型错误解析邓晓明左学中四川省农业机械鉴定站,四川成都农机购置与应用补贴产品投档,是指农机生产企业通过农机购置与应用补贴机具信息化自主投档平台投送投档信息,经省级农机化主管部门组织形式审核、汇总公示后整理公布,为补贴政策实施提供信息的活动。
在企业自愿参与国家农机购置补贴政策实施过程中,由于企业自身投档工作错误而导致违规处罚,往往会给企业造成很大的经济损失,也会影响到补贴政策的规范实施。
1“大数据”时代下的投档自2019年开始,农机购置与应用补贴产品投档实行全国统一平台管理,投档所需产品信息数据直接从“全国农业机械试验鉴定管理服务信息化平台”抓取,实现了投档工作全程“无纸化”,系统采用“一键推送”功能,企业只需录入一次信息,即可实现将补贴投档申报所需信息向全国各省一键推送,大大减少了企业申报工作量,降低了出错几率。
平台的“黑名单”管理功能,采取与全国农机“黑名单”库联动,代替人工检索,对违规产品实行智能拦截,阻止了违规问题产品进入补贴范围,防止违规套取补贴资金。
平台的“一键导入”功能实现了将投档系统的审核信息直接导入到购机补贴服务办理系统,实现了投档数据共享,“让数据多跑路,企业少跑腿”,避免了二次录入错误,大大提升了补贴产品投档工作的信息化水平,迎来了农机购置与应用补贴产品投档管理工作的“大数据”时代。
2近年来四川省投档工作概况2019年以来,四川省共组织开展11批次农机购置与应用补贴产品自主投档,组织审核通过产品42169个,成功导入补贴服务办理系统,为四川省购机补贴政策的顺利规范实施提供了技术支撑。
同时依据《农业机械购置补贴产品违规经营行为处理办法(试行)》(农办财〔2017〕26号)、《农业农村部办公厅财政部办公厅关于进一步加强农机购置补贴政策监管强化纪律约束的通知》(农办机〔2019〕6号)等文件,累计处罚投档违规企业64家,处罚违规产品127个,并对有关产品按照规定采取全国联动处罚措施,保障了国家购机补贴专项资金的安全使用,有效维护了补贴政策的顺利实施。
- 1、下载文档前请自行甄别文档内容的完整性,平台不提供额外的编辑、内容补充、找答案等附加服务。
- 2、"仅部分预览"的文档,不可在线预览部分如存在完整性等问题,可反馈申请退款(可完整预览的文档不适用该条件!)。
- 3、如文档侵犯您的权益,请联系客服反馈,我们会尽快为您处理(人工客服工作时间:9:00-18:30)。
Biochar from Biomass and WasteW.Kwapinski •C.M.P.Byrne •E.Kryachko •P.Wolfram •C.Adley •J.J.Leahy •E.H.Novotny •M.H.B.HayesReceived:3March 2010/Accepted:28May 2010/Published online:12June 2010ÓSpringer Science+Business Media B.V.2010Abstract There is an increasing realisation that biomass and organic wastes are valuable feedstocks for second generation biorefining processes that give rise to platform chemicals to substitute for dwindling petrochemical resources,and for pyrolysis processes that produce syngas,bio-oil,and biochar from biomass,organic wastes,and the biorefining residuals of the future.The experimental work described has focused on physical properties and compo-sitions of biochars produced from miscanthus (Miscan-thus 9giganteus ),willow (Salix spp)and pine (Pinus sylvestris )at 500°C and at 400,500,and 600°C in the case of the miscanthus.Although the morphologies of the cell structures were maintained in the pyrolysis,the surface area of the miscanthus biochar was greatly increased by heating at 600°C for 60min.Nuclear magnetic resonance spectra showed the disappearance of evidence for the car-bohydrate and lignin plant components as the pyrolysis temperature was raised,and the compositions of miscan-thus biochars after heating for 10and for 60min at 600°C were very similar and composed of fused aromatic struc-tures and with no traces of the aliphatic components in the starting materials.In greenhouse and growth chamberexperiments the growth of maize (Zea mays L)seedlings was found to be inhibited by soil amendments with biochar from miscanthus formed at 400°C for 10min,but stimu-lated by miscanthus char formed at 600°C for 60min.In the course of discussion the relevance of the results obtained is related to the roles that soil amendments with biochar can have on soil fertility,carbon sequestration,on the emissions of greenhouse gases from soil,on fertilizer requirements,and on waste management.It is clear that biochar soil amendments can have definite agronomic and environmental benefits,but it will be essential to have clear guidelines for biochar production from various feedstocks and under varying pyrolysis parameters.It will be equally important to have a classification system for biochars that clearly indicate the product compositions that will meet acceptable standards.A case can be made for sets of standard biochars from different substrates that meet the required criteria.Keywords Biochar ÁBiomass ÁWaste ÁPyrolysis ÁThermal conversion ÁPlant growth ÁCarbon sequestrationIntroductionSoil amendment with biochar has attracted widespread attention because it increases the sequestration of carbon in soils and thereby decreases the amounts of CO 2that enters the atmosphere [1–3].Biochar is the carbon rich product obtained when biomass is thermally decomposed (pyroly-sed)in restricted air conditions at temperatures between 350and 700°C [4].The use of biochar as a soil amender is not a new concept.The Terra Preta da indio (TP),or the dark earth soils of the Amazon region are areas of very fertileW.Kwapinski ÁC.M.P.Byrne (&)ÁE.Kryachko ÁP.Wolfram ÁC.Adley ÁJ.J.Leahy ÁM.H.B.Hayes Department of Chemical and Environmental Sciences,University of Limerick,Limerick,Ireland e-mail:corinna.byrne@ul.ie W.Kwapinskie-mail:witold.kwapinski@ul.ie M.H.B.Hayese-mail:michael.h.hayes@ul.ieE.H.NovotnyEmbrapa Solos,Rua Jardim Botaˆnico,Rio de Janerio 1024,BrazilWaste Biomass Valor (2010)1:177–189DOI 10.1007/s12649-010-9024-8anthropogenic soils interspersed with relatively infertile ferrisols close to rivers.There are several theories regard-ing the processes that led to their formation.It is not clear whether the soils resulted from intentional soil improve-ment processes,or were the products of the agricultural and household activities of indigenous populations[5].The dwelling places in the pre-Columbian past gave rise to accumulations of plant and animal debris,as well as to large amounts of ashes and of bonfire residues(charcoal), and several chemical elements,such as P,Mg,Zn,Cu,Ca, Sr,and Ba,which represent a geochemical signature of human occupation[6].The accumulation of such nutrient residues is likely to be a major driver of the formation of the fertile soils.Large amounts of biochar derived carbon stocks remain in these soils centuries and millennia after they were abandoned.Typical Amazonian ferrisols have low fertility and contain about100Mg carbon ha-1m-1.The fertile TP soils,though derived from similar mineral parent materials,contain around2.7±0.5times more organic carbon(approximately250Mg ha-1m-1)than the corre-sponding ferrisols[4].Carbon stored in soils in this way far exceeds the potential sequestration of C in plant biomass should bare soil be restocked with primary forest that could,at maturity,be expected to have about 110Mg C ha-1above ground[7,8].There is increasing evidence indicating that biochar not only increases the stable carbon stocks in a soil but also increases nutrient availability beyond a fertiliser effect[9], and is much more efficient at enhancing soil quality than any other organic soil amendment[10].Based on these considerations the concept of Terra Nova has emerged,or of soils whose properties and fertility would be enhanced by modern variants of the management practises that cre-ated the TP[11].This communication reviews procedures for the pro-duction and characterisation of biochars,and describes, with some results,procedures that were utilised for the preparation and characterisation of biochars.It reviews aspects of how applications of biochar can influence pro-cesses causing climate change,decrease emissions from waste and of NO x from soil,and provides evidence to show that although biochar can enhance seed germination and plant growth there must be careful control of the prepara-tion processes because some preparations can inhibit plant growth.Biochar Production and CharacterisationPyrolysis is one of the many technologies that produce energy from biomass and waste.The production of biochar, a carbon-rich,solid,by-product[1]distinguishes pyrolysis from the other technologies.The major pyrolysis products are pyrolytic oil(bio-oil),synthesis gas with differing energy values(syngas),and biochar[12,13].A range of process conditions,such as the composition of the feed-stock,temperature and heating rate can be optimized to provide different amounts and properties of products. Volatile products can be captured to provide energy,or upgraded to specific chemical products(e.g.wood pre-servative,meat browning,foodflavouring,adhesives,etc.) [1,14].Depending on the operational time and temperature, there are three subclasses of pyrolysis.These are conven-tional slow pyrolysis,fast pyrolysis,andflash pyrolysis [15].Yields of liquid products are maximised in conditions of low temperature,high heating rate,and a short gas residence time,whereas a high temperature,low heating rate and long gas residence time would maximise yields of fuel gas.Low operational temperatures and low heating rates give maximum yields of biochar[16].Feedstocks currently used on a commercial-scale,or in research facilities include wood chip and wood pellets,tree bark,crop residues(straw,nut shells and rice hulls),switch grass,organic wastes including distillers’grain,bagasse from the sugarcane industry,olive mill waste,chicken lit-ter,dairy manure,sewage sludge and paper sludge[11]. The particle sizes and moisture contents of feedstock are important as wet feedstocks with large particle sizes will require more energy for pyrolysis.Some of the carbon in the feedstock may need to be burned to supply the energy needed,and so less feedstock is converted to biochar and to the other products.Maximising the production of biochar relative to the mass of the initial feedstock is always at the expense of bio-oil and gas production.The studies described here have involved the pyrolysis of willow(Salix species),miscanthus(9giganteus)and pine(Pinus sylvestris)biomass and the characterisation of the biochars formed.Materials and MethodsIn thefirst set of experiments250g samples of the miscanthus,pine and willow were chipped(maximum size 1cm)and heated in the absence of air to500°C for10min in a1dm3capacity pyrolyser.The pyrolysed materials were then rapidly moved to an ambient temperature zone, still in a nitrogen atmosphere,and allowed to cool.In the second set of experiments250g samples of the miscanthus chips were heated for10min at400°C and for60min at 600°C.The data for the yields of biochar obtained(on a dry weight basis)from the three substrates;the elemental analyses(Elementar);BET surface area(Gemini Microm-eritics apparatus);and high heating value(HHV)deter-mined using a Paar calorimeter(CEN TS14918)are givenin Table1.Morphologies of biochars were observed using Scanning Electron Microscopy(SEM)(CaryScope Jcm5700).Solid-state13C NMR experiments were carried out using a VARIAN INOVA spectrometer at13C and1H frequen-cies of100.5and400.0MHz,respectively.A JACKOB-SEN5mm magic-angle spinning double-resonance probe head was used for Varied-Amplitude Cross-Polarisation (CP)experiments at spinning frequencies of13kHz. Typical cross-polarisation times of1ms,acquisition times of13ms,and recycle delays of500ms were used.The cross-polarisation time was chosen after variable contact time experiments,and the recycle delays in CP experi-ments were chosen to befive times longer than the longest 1H spin–lattice relaxation time(T1H)as determined by inversion-recovery experiments.ResultsThe yields of biochar obtained from the different sources are given in Table1.The pyrolysis temperature typically changes the yield and properties of biochar.With increasing temperature,the yield of biochar commonly decreases but the carbon concentration increases[17].That trend is evident for the data in Table1.The data for Miscanthus(Table1)show the very great increase in surface area for the Miscanthus biochar as the temperature and time of heating were increased.The SEM pictures(Fig.1)show that the plant cellular morphologies were maintained in the biochars following heating of the miscanthus and willow feedstocks for10min at500°C.The HHV data indicate the relative values as fuels of thedifferent biochar products.There were little differences in the values for willow,miscanthus and pine biochars pro-duced under the same conditions(at500°C for10min).The data for miscanthus indicate that the more‘mature’biochar formed at600°C for60min was a slightly better fuel.The NMR spectrum of the miscanthus used as a feed-stock for biochar production in this study is shown in Fig.2.This spectrum is similar to that obtained for miscanthus by Brosse et al.[18]and is dominated by car-bohydrate signals in the resonance region60–110ppm, representing the contributions from cellulose and hemi-celluloses,and the resonance at105ppm is assigned to the anomeric C.Contributions from lignin are indicated by the resonance at56ppm(methoxyl)and at142–164ppm (O-aromatic)[5].There are also some aliphatic contributions with signals from alkyl C groups(0–46ppm),probablyTable1Yields and some properties of biochars from different substrates and reaction conditionsBiochar source Willow Pine Miscanthus Miscanthus MiscanthusPyrolysis time,min1010101060 Temperature,°C500500500400600Yield of char,wg.%25.0–26.222.2–22.525.9–26.229.4–31.219.8–20.2 Surface area,m2g-1 1.41–1.55 1.93–2.15 1.65–1.95 1.4–1.750.9–51.1 HHV,MJ kg-131.0–31.530.4–31.329.9–30.729.4–30.331.5–32.5 C,wt.%79.981.476.374.885.1H,wt.% 3.34 3.38 4.26 4.33 2.40N,wt.%0.970.470.400.390.55Fig.1SEM of A miscanthus biochar and B willow biocharattributable to cutin and waxes,and the20–25ppm can be attributed to terminal methyl groups.13C NMR is one of the most promising techniques for the determination and characterisation of biochar in soils [19].The13C NMR spectra for the biochars formed from miscanthus by heating at different temperatures and time intervals(Fig.3)are vastly different from that for the feedstock.The spectral patterns show that the structural compositions of the biochars change during pyrolysis.The spectra for the miscanthus biochar at400,500and600°C are directly comparable because the pyrolysis time was the same(10min)for both reactions.Both spectra show significant aliphatic composition[3]at the25–35ppm resonance,and for terminal methyl at*15ppm.Raising the pyrolysis temperature to greater than400°C gave rise to a loss of aliphatic-C moieties and a transformation of C compounds to mostly poly-condensed aromatic-C type structures dominated by a peak at130ppm[3].There is still some evidence in the spectrum at400°C,and to a lesser extent in that at500°C for contributions from lignin with a peak at56ppm indicative of methoxyl and a shoulder at*150ppm typical of the O-aromatic func-tionalities in lignin.When the temperature was raised to 600°C the lignin signals disappeared.Increased heating also resulted in a significant peak shift in the aryl region from131to126ppm[3].An upfield shift of the aryl peak to126ppm is typical of charred residues attributable to polycyclic aromatic structures[5].That indicates that fused aromatic structures were formed with low H(see Table1) and O substituents[20].These changes signify the trans-formations of labile compounds into environmentally recalcitrant forms.Recognisable fragments of feedstock biopolymers(lignin signals)in the chars produced at400 and500°C would indicate that the reaction time was insufficient for complete conversion to char.On the other hand,the char produced at600°C in10min and60min contained no recognisable feedstock components,indicat-ing complete conversion to char.Biochar as a Fertiliser and Soil AmeliorantBy improving on existing best management practices, biochar can play a major role in expanding options for sustainable soil management.Biochar amendments will not only improve soil productivity,but also decrease the environmental impact of agriculture on soil and water resources.Biochar should therefore not be seen asanalternative to existing soil management,but as a valuable addition that facilitates the development of sustainable land use[10].Biochar can act as a soil conditioner and as a fertiliser.It will enhance plant growth by supplying and retaining nutrients,and by improving soil physical and biological properties[8].However,it has been reported that biochar can have negative as well as positive effects on plant growth.Materials and MethodsGreenhouse ExperimentsThe preparation,and some properties of biochars,prepared at500°C for10min from willow,pine and miscanthus are have been described,and relevant data are given in Table1.Biochars were mixed at rates of1%and5%with a clay loam soil and cropped to maize(Zea mays L.).Six maize seeds,steeped in water for2h,were planted per pot (13cm pot diameter).Eight replications for each experi-ment were incubated in the greenhouse during May2009. Biochar was not added to the control.Pots were watered to field capacity and allowed to drain.Subsequently the pots were watered(50cm3)every fourth day.After10days the weakest three plants were removed from each pot.Whole plant and leaf growth were monitored every3days.Growth Chamber ExperimentsA shallow calcareous brown earth loam soil(20%clay, pH4.9)from the Kinvara series in the Burren area (N.Clare-S.Galway,Ireland)was amended with biochar (5wg.%).Two types of biochar were prepared from miscanthus chips(max.size1cm)in a lab-scale pyrolyser (1dm3)at:(a),400°C for10min;and(b),600°C for 60min.Ten maize(Zea mays L.)seeds were planted per pot(13cm pot diameter).Four replications for each experiment were incubated in the growth chamber.The pots were watered tofield capacity and allowed to drain. The system was incubated at25°C in a growth chamber for 21days and artificial light was provided for13h/day.The pots were watered(50cm3)every fourth day.After 10days the weakestfive plants were removed from each pot.Twenty-one days after planting all plants were cut at soil level,weighed,and oven dried at60°C until a constant weight was attained.Microbial ExperimentsTissues of plant roots were examined to determine fungal presence by staining with Chlorazol Black[21].ResultsSeedling emergence in the greenhouse wasfirst observed after15days in the cases of the controls;however,at the same time plant stems were10cm in height and had three leaves per stem in the biochar amended pots.The best growth was in pots amended with5wt%miscanthus bio-char(Fig.4and Table2).Biochar from willow and pine gave similar growth(though less than that for miscanthus). The difference in growth in the control and char-amended soils became less with time.Better growth was obtained for higher amendments in the cases of the willow and pine biochars,but even relatively small amendments(1wt.%) gave growth results similar to those at higher concentra-tions in the cases of the miscanthus biochars.The green-house experiments showed that the biochar amendments had the best effects in thefirst stages of germination and plant growth.Microbiological analyses showed greater enrichments of microorganisms on the root tissue of the plants grown in soils amended with biochar.Most of the root surface from soil amended with miscanthus biochar was covered with large fungal colonies whereas colonies were barely visible on roots from the control pots(Fig.5).When plant roots were taken from the control and char amended soils it was seen that the roots proliferated where biochar enrichments occurred(Fig.5).The data in Table3for the growth chamber experiment indicate that favourable plant responses were not obtained for all of the biochar samples.Best growth was obtained for the biochar heated at600°C for60min.The product from heating for10min at400°C suppressed plant growth measured after21days.Material from the lower temper-ature and shorter heating time had a lower surface area,and a lower C content(indicating lesser transformation to sta-ble biochar product)than for the product at the highertemperature.Fig.4Growth of plants in greenhouse experiments after21days in soil amended with1and5wg.%biochar from willow,pine,and miscanthusDiscussion and Considerations of Implications of Biochar Applications to SoilIt is important to establish the preparation criteria that give rise to biochars that will have optimum value when added to soil.Properties that will influence biochar applications for promoting plant growth and the sequestration of carbon in soil will include:–nutrients content,and composition and availability;–elemental composition,ash content,and volatility;–capacity to adsorb a wide range of organic and inorganic molecules;–surface area,porosity,and particle size;and –bulk density.A classification system for biochar is needed for potential users and researchers.Various characterisationtechniques can be utilised in order to establish a set of standards needed to develop a classification system.The two properties of biochar that are most important for carbon sequestration and for soil amendment are:(1),a high resistance to microbial degradation;and (2),an ability to retain nutrients that will equal or surpass that of other forms of soil organic matter [1].The ability of biochar to store C and improve soil fertility will depend on its physical and chemical properties.Properties of biochar relevant to soil fertility and carbon sequestration can be varied in the pyrolysis process and through the choice of feedstock [3].The major components of biomass are cellulose,hemi-cellulose and lignin.The proportions of these three com-ponents determine the ratios of volatile carbon (in bio-oil and gas)and stabilised carbon (biochar)in the pyrolysis products.Fast pyrolysis favours feedstocks with high cel-lulose and hemicellulose contents as bio-oil and gas are the main products,and with lesser yields of biochar.Feed-stocks with high lignin contents produce the highest bio-char yields when pyrolysed at moderate temperatures (approx.500°C)[17,22,23].Slow pyrolysis yields high quantities of biochar,and therefore favours feedstocks with high lignin contents.In the future,selection of feedstock may be dictated by the desired balance between pyrolysisTable 2Growth of maize seedlings (as a percentage of the controls where biochar was not added)in soils amended with biochars (5wt.%)in greenhouse experiments Biochar sourceControl Willow Pine Miscanthus Yield after 21days (as growth %of control)100(±9.12)393(±33.9)402(±32.2)437(±40.3)Yield after 28days (as growth %of control)100(±6.51)128(±9.82)135(±9.41)153(±11.9)Biochar was made for 10min in 500°C.(±standarddeviation)Fig.5Root tissues A covered with fungal colonies and B roots from control potsTable 3Mass of maize seedlings after 21days of growth (as a percentage of the control where biochar was not added)from soils amended with miscanthus biochar (3wt.%)(±standard deviation)Preparation methodControl400°C,10min 600°C,60minYield of dry matter (as wt.%of control)100(±16.1)76.6(±7.00)165(±19.8)products(gas,oil and biochar),and whether or not slow pyrolysis is being used[11].Solid state13C NMR provides an excellent tool for the characterization of biochars.The spectra in Fig.3allow determination of the optimum conditions for the prepara-tion of chars with different compositions.These spectra and the elemental analyses data(Table1)give indications of the extents to which the biomass feedstocks are con-verted to the fused aromatic structures.Such structural arrangements may be considered to be optimum for long term C sequestration,and may be an ideal biochar char-acteristic for the sequestration of soil C for millennia. Biochars produced at higher temperatures do not provide ion exchange functional groups due to dehydration and decarboxylation.That would of course limit its usefulness in retaining soil nutrients by ion exchange mechanisms.As seen in Table1,biochars produced at the higher temperatures have high surface areas,and will have high porosities.Such porosity will allow the retention of water, enhance the soil sorption properties[24–27],and provide refuge for microorganisms.Earlier studies have shown that biochar with appropriate surface areas and pore sizes will provide a refuge for Arbuscular mycorrhizal fungi(AMF)[13].The addition of biochar to soils has been found to promote higher coloni-zation rates of host plant roots by AMF[28].These fungi form a symbiotic association with plant roots,effectively extending the roots and enabling the uptake of additional plant nutrients.In return the plant provides the organic energy that the fungi need.Recent research suggests that AMF might be an important component of the soil organic pool,in addition to facilitating carbon sequestration by stabilizing soil aggregates[29].Glomalin,a glycoprotein containing30-40%carbon,is produced by all AMF[30].It has been found to be involved in the aggregation and sta-bilization of soil particles.Higher nutrient retention and nutrient availability properties have been found after charcoal additions to soil [24].These properties could arise from the oxidation of peripheral aromatic groups.However,that would be a long term effect.It is reasonable to conclude that the prolifer-ation of microbial species in the environs of biochar will give rise to significant amounts of biomass which will be transformed to humic substances with high ion exchange properties.Significance of Biochar for the Sequestration of Carbon The global carbon cycle is made up offlows and pools of carbon in the Earth’s system.The important pools of car-bon are terrestrial,atmospheric,ocean,and geological.The carbon within these pools has varying lifetimes,andflows take place between them all.Carbon in the active carbon pool moves rapidly between pools[1].In order to decrease carbon in the atmosphere,it is necessary to move it into a passive pool containing stable or inert carbon.Biochar provides a facileflow of carbon from the active pool to the passive pool.Biomass(non biochar)materials have varying degrees of resistance to biodegradation to CO2and water.The more readily biodegradable components of the biomass decom-pose quickly over one to5years,the more stable compo-nents transform over decades to centuries,and the most refractory components can last for several centuries to a few thousand years.The organic matter in long term grassland or forest reaches a steady state that will not change substantially until the management is changed. Then there will be a slow decline in the organic C content until eventually a much lower steady state is reached[31]. The carbon released in that way must be regarded as fossil carbon.Additions of organic matter during cultivation will lessen the losses of indigenous organic matter.However,it is estimated that less than10–20%of the added carbon will remain in agricultural soils after5–10years(depending on the carbon quality and the environment)[8].The net result is that the bulk of carbon added as biomass is rapidly released back to the atmosphere as CO2.Adding biochar to soils can be regarded as a means of sequestering atmospheric CO2[10].The conversion by pyrolysis of biomass organic matter to biochar significantly increases the recalcitrance of the carbon in biomass. Because biochar is inherently stable in soil,applications of biochar inevitably gives rise to far more soil C sequestra-tion than would result from applications of biomass of similar C contents[8].Biochar can thus be considered to harness the natural carbon cycle.About four times more organic carbon is stored in the Earth’s soil than in atmospheric CO2.The annual uptake of CO2by plants through photosynthesis,120Gt CO2–C [32],is8times greater than anthropogenic greenhouse gas (GHG)emissions at this time[10].Switching even a small amount of the carbon cycling between the atmosphere and plants into a much slower biochar cycle would make a worthwhile difference to atmospheric CO2concentrations. It is estimated,for example,that diverting as little as1%of net annual plant uptake into biochar would mitigate almost 10%of current anthropogenic carbon emissions[10].That does not take into consideration the additional multiplier effects that lock up additional carbon,such as increased plant growth,increased root biomass,and increased soil microbial biomass.Based on what has been stated above,for biochar to act as a carbon sink it must remain in the soil for a very long time and it must have a high level of resistance to bio-logical and chemical degradation.Radiocarbon dating of TP soils indicate origins of500to7,000years BP[33,34].Mean residence times of1300–2600years for biochar from Australian savannah soils were estimated by matching annual production of biochar from the savannah soils with measured carbon stocks for soils in Northern Australian woodlands[35].At a mean annual temperature of10°C the mean residence time of biochars was estimated to be 1,335years[36].Although there is some uncertainty about the decomposition rates of biochar in soils,the great age of biochars found in soil studies and in archaeological sites provides evidence for its stability[34].Biochar creates a real increase in terrestrial carbon stocks because it gives rise to a stable form of biomass carbon and significantly delays its decomposition[37]. Lehmann[38]estimates that20%of all the carbon in biomass could be captured by conversion to biochar. Theoretically that could amount to249109tonnes of carbon,or889109tonnes of CO2annually.However,in practical terms much of that biomass would not be avail-able for conversion to biochar and the actual potential would depend on the ability to access biomass feedstocksin an economically viable and environmentally responsible manner[37].It is estimated that10%of the annual US fossil-fuel emissions could be captured if biochar was produced using existing forest residues,or crop wastes,or fast growing biomass established on idle cropland[38].As already mentioned,the ability of biochar to store carbon and improve soil fertility will depend on its physical and chemical properties,and these can be varied in the pyrolysis process,or through the choice of feedstock. Hayes et al.[39]reviewed the potential feedstocks avail-able in Ireland for biorefining technologies.These feed-stocks include wastes and biomass residues(biodegradable municipal solid waste(MSW),poultry litter,spent mush-room compost(SMC),straws,forest residues,and sawmill residues that potentially could be utilised for biochar production.Converting biomass carbon to biochar carbon enables up to50%of the initial carbon to be sequestered in a relatively stable form.The proportion of the carbon in the biomass which is converted to biochar is highly dependent on the type of feedstock[8].Downie et al.[40]examined biochar production from different feedstocks(wastes,sludge and wood)and estimated a63%retrieval of the biomass carbon in the biochar.These values,along with measurements made in the laboratory,were used to estimate the potential carbon sequestration of biochar produced from the avail-able wastes and residues in Ireland(Fig.6).These calcu-lations do not evaluate emissions associated with the whole life cycle of biochar production but they aim to give an insight into the potential of biochar.Approximately2.4% of Ireland’s2007GHG emissions,1.67Mt of CO2,could be sequestered by the biochar produced from slow pyro-lysis of wastes and residues available under Scenario1.That uses the minimum available straw resources,the minimum collectable forest residues,all available biode-gradable MSW,poultry litter,SMC,and the available sawmill residues.In Scenario2,when all the maximum available straw resources for energy purposes and the maximum collectable forest residues are included for bio-char production,the amount of CO2–eq that can be sequestered increases to2.25Mt of CO2or3.25%of the total GHG emitted in2007.When all straw resources, including those currently used for animal bedding and soil amendments,are utilised,with the maximum collectable forest residues,Scenario3becomes operational.The resulting biochar would enable the sequestration of 2.79Mt of CO2or4.03%of the total GHG(CO2–eq) emitted in2007.Biochar for a More Appropriate Utilisation of Waste Wastes and residues from animal,crop,and forest pro-duction are valuable resources that can be used directly and indirectly for energy,including pyrolysis energy and bio-char[41].In addition to providing energy and biochar,the pyrolysis process will decrease the volume and the weight of the waste material[10].Many waste streams offer economic opportunities for energy recovery particularly when a significant reliable source of feedstock is generated at a specific location.Biochar as a waste management strategy decreases GHG emissions associated with tradi-tional ndfilling of organic waste,e.g.garden waste,results in the release of significant quantities of methane.Anaerobic digestion of animal wastes release methane and nitrous oxide,and these gases are25and298 times,respectively,more potent as GHGs than CO2.。