2010_Chemistry_H2_P3__question_paper
A_review_of_advanced_and_practical_lithium_battery_materials
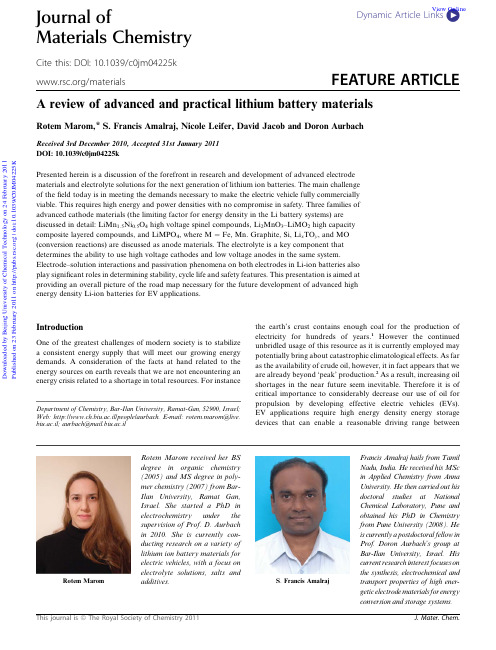
A review of advanced and practical lithium battery materialsRotem Marom,*S.Francis Amalraj,Nicole Leifer,David Jacob and Doron AurbachReceived 3rd December 2010,Accepted 31st January 2011DOI:10.1039/c0jm04225kPresented herein is a discussion of the forefront in research and development of advanced electrode materials and electrolyte solutions for the next generation of lithium ion batteries.The main challenge of the field today is in meeting the demands necessary to make the electric vehicle fully commercially viable.This requires high energy and power densities with no compromise in safety.Three families of advanced cathode materials (the limiting factor for energy density in the Li battery systems)are discussed in detail:LiMn 1.5Ni 0.5O 4high voltage spinel compounds,Li 2MnO 3–LiMO 2high capacity composite layered compounds,and LiMPO 4,where M ¼Fe,Mn.Graphite,Si,Li x TO y ,and MO (conversion reactions)are discussed as anode materials.The electrolyte is a key component that determines the ability to use high voltage cathodes and low voltage anodes in the same system.Electrode–solution interactions and passivation phenomena on both electrodes in Li-ion batteries also play significant roles in determining stability,cycle life and safety features.This presentation is aimed at providing an overall picture of the road map necessary for the future development of advanced high energy density Li-ion batteries for EV applications.IntroductionOne of the greatest challenges of modern society is to stabilize a consistent energy supply that will meet our growing energy demands.A consideration of the facts at hand related to the energy sources on earth reveals that we are not encountering an energy crisis related to a shortage in total resources.For instancethe earth’s crust contains enough coal for the production of electricity for hundreds of years.1However the continued unbridled usage of this resource as it is currently employed may potentially bring about catastrophic climatological effects.As far as the availability of crude oil,however,it in fact appears that we are already beyond ‘peak’production.2As a result,increasing oil shortages in the near future seem inevitable.Therefore it is of critical importance to considerably decrease our use of oil for propulsion by developing effective electric vehicles (EVs).EV applications require high energy density energy storage devices that can enable a reasonable driving range betweenDepartment of Chemistry,Bar-Ilan University,Ramat-Gan,52900,Israel;Web:http://www.ch.biu.ac.il/people/aurbach.E-mail:rotem.marom@live.biu.ac.il;aurbach@mail.biu.ac.ilRotem MaromRotem Marom received her BS degree in organic chemistry (2005)and MS degree in poly-mer chemistry (2007)from Bar-Ilan University,Ramat Gan,Israel.She started a PhD in electrochemistry under the supervision of Prof.D.Aurbach in 2010.She is currently con-ducting research on a variety of lithium ion battery materials for electric vehicles,with a focus on electrolyte solutions,salts andadditives.S :Francis AmalrajFrancis Amalraj hails from Tamil Nadu,India.He received his MSc in Applied Chemistry from Anna University.He then carried out his doctoral studies at National Chemical Laboratory,Pune and obtained his PhD in Chemistry from Pune University (2008).He is currently a postdoctoral fellow in Prof.Doron Aurbach’s group at Bar-Ilan University,Israel.His current research interest focuses on the synthesis,electrochemical and transport properties of high ener-getic electrode materials for energy conversion and storage systems.Dynamic Article Links CJournal ofMaterials ChemistryCite this:DOI:10.1039/c0jm04225k /materialsFEATURE ARTICLED o w n l o a d e d b y B e i j i n g U n i v e r s i t y o f C h e m i c a l T e c h n o l o g y o n 24 F e b r u a r y 2011P u b l i s h e d o n 23 F e b r u a r y 2011 o n h t t p ://p u b s .r s c .o r g | d o i :10.1039/C 0J M 04225KView Onlinecharges and maintain acceptable speeds.3Other important requirements are high power density and acceptable safety features.The energy storage field faces a second critical chal-lenge:namely,the development of rechargeable systems for load leveling applications (e.g.storing solar and wind energy,and reducing the massive wasted electricity from conventional fossil fuel combustion plants).4Here the main requirements are a very prolonged cycle life,components (i.e.,relevant elements)abun-dant in high quantities in the earth’s crust,and environmentally friendly systems.Since it is not clear whether Li-ion battery technology can contribute significantly to this application,battery-centered solutions for this application are not discussedherein.In fact,even for electrical propulsion,the non-petroleum power source with the highest energy density is the H 2/O 2fuel cell (FC).5However,despite impressive developments in recent years in the field,there are intrinsic problems related to electrocatalysis in the FCs and the storage of hydrogen 6that will need many years of R&D to solve.Hence,for the foreseeable future,rechargeable batteries appear to be the most practically viable power source for EVs.Among the available battery technologies to date,only Li-ion batteries may possess the power and energy densities necessary for EV applications.The commonly used Li-ion batteries that power almost all portable electronic equipment today are comprised of a graphite anode and a LiCoO 2cathode (3.6V system)and can reach a practical energy density of 150W h kg À1in single cells.This battery technology is not very useful for EV application due to its limited cycle life (especially at elevated temperatures)and prob-lematic safety features (especially for large,multi-cell modules).7While there are ongoing developments in the hybrid EV field,including practical ones in which only part of the propulsion of the car is driven by an electrical motor and batteries,8the main goal of the battery community is to be able to develop full EV applications.This necessitates the development of Li-ion batteries with much higher energy densities compared to the practical state-of-the-art.The biggest challenge is that Li-ion batteries are complicated devices whose components never reach thermodynamic stability.The surface chemistry that occurs within these systems is very complicated,as described briefly below,and continues to be the main factor that determines their performance.9Nicole Leifer Nicole Leifer received a BS degree in chemistry from MIT in 1998.After teaching high school chemistry and physics for several years at Stuyvesant High School in New York City,she began work towards her PhD in solid state physics from the City University of New York Grad-uate Center.Her research con-sisted primarily of employing solid state NMR in the study of lithium ion electrode materialsand electrode surfacephenomena with Prof.Green-baum at Hunter College andProf.Grey at Stony Brook University.After completing her PhD she joined Prof.Doron Aurbach for a postdoctorate at Bar-Ilan University to continue work in lithium ion battery research.There she continues her work in using NMR to study lithium materials in addition to new forays into carbon materials’research for super-capacitor applications with a focus on enhancement of electro-chemical performance through the incorporation of carbonnanotubes.David Jacob David Jacob earned a BSc from Amravati University in 1998,an MSc from Pune University in 2000,and completed his PhD at Bar-Ilan University in 2007under the tutelage of Professor Aharon Gedanken.As part of his PhD research,he developed novel methods of synthesizing metal fluoride nano-material structures in ionic liquids.Upon finishing his PhD he joined Prof.Doron Aurbach’s lithium ionbattery group at Bar-Ilan in2007as a post-doctorate and during that time developed newformulations of electrolyte solutions for Li-ion batteries.He has a great interest in nanotechnology and as of 2011,has become the CEO of IsraZion Ltd.,a company dedicated to the manufacturing of novelnano-materials.Doron Aurbach Dr Doron Aurbach is a full Professor in the Department of Chemistry at Bar-Ilan Univer-sity (BIU)in Ramat Gan,Israel and a senate member at BIU since 1996.He chaired the chemistry department there during the years 2001–2005.He is also the chairman of the Israeli Labs Accreditation Authority.He founded the elec-trochemistry group at BIU at the end of 1985.His groupconducts research in thefollowing fields:Li ion batteries for electric vehicles and for otherportable uses (new cathodes,anodes,electrolyte solutions,elec-trodes–solution interactions,practical systems),rechargeable magnesium batteries,electronically conducting polymers,super-capacitors,engineering of new carbonaceous materials,develop-ment of devices for storage and conversion of sustainable energy (solar,wind)sensors and water desalination.The group currently collaborates with several prominent research groups in Europe and the US and with several commercial companies in Israel and abroad.He is also a fellow of the ECS and ISE as well as an associate editor of Electrochemical and Solid State Letters and the Journal of Solid State Electrochemistry.Prof.Aurbach has more than 350journals publications.D o w n l o a d e d b y B e i j i n g U n i v e r s i t y o f C h e m i c a l T e c h n o l o g y o n 24 F e b r u a r y 2011P u b l i s h e d o n 23 F e b r u a r y 2011 o n h t t p ://p u b s .r s c .o r g | d o i :10.1039/C 0J M 04225KAll electrodes,excluding 1.5V systems such as LiTiO x anodes,are surface-film controlled (SFC)systems.At the anode side,all conventional electrolyte systems can be reduced in the presence of Li ions below 1.5V,thus forming insoluble Li-ion salts that comprise a passivating surface layer of particles referred to as the solid electrolyte interphase (SEI).10The cathode side is less trivial.Alkyl carbonates can be oxidized at potentials below 4V.11These reactions are inhibited on the passivated aluminium current collectors (Al CC)and on the composite cathodes.There is a rich surface chemistry on the cathode surface as well.In their lithiated state,nucleophilic oxygen anions in the surface layer of the cathode particles attack electrophilic RO(CO)OR solvents,forming different combinations of surface components (e.g.ROCO 2Li,ROCO 2M,ROLi,ROM etc.)depending on the electrolytes used.12The polymerization of solvent molecules such as EC by cationic stimulation results in the formation of poly-carbonates.13The dissolution of transition metal cations forms surface inactive Li x MO y phases.14Their precipitation on the anode side destroys the passivation of the negative electrodes.15Red-ox reactions with solution species form inactive LiMO y with the transition metal M at a lower oxidation state.14LiMO y compounds are spontaneously delithiated in air due to reactions with CO 2.16Acid–base reactions occur in the LiPF 6solutions (trace HF,water)that are commonly used in Li-ion batteries.Finally,LiCoO 2itself has a rich surface chemistry that influences its performance:4LiCo III O 2 !Co IV O 2þCo II Co III 2O 4þ2Li 2O !4HF4LiF þ2H 2O Co III compounds oxidize alkyl carbonates;CO 2is one of the products,Co III /Co II /Co 2+dissolution.14Interestingly,this process seems to be self-limiting,as the presence of Co 2+ions in solution itself stabilizes the LiCoO 2electrodes,17However,Co metal in turn appears to deposit on the negative electrodes,destroying their passivation.Hence the performance of many types of electrodes depends on their surface chemistry.Unfortunately surface studies provide more ambiguous results than bulk studies,therefore there are still many open questions related to the surface chemistry of Li-ion battery systems.It is for these reasons that proper R&D of advanced materials for Li-ion batteries has to include bulk structural and perfor-mance studies,electrode–solution interactions,and possible reflections between the anode and cathode.These studies require the use of the most advanced electrochemical,18structural (XRD,HR microscopy),spectroscopic and surface sensitive analytical techniques (SS NMR,19FTIR,20XPS,21Raman,22X-ray based spectroscopies 23).This presentation provides a review of the forefront of the study of advanced materials—electrolyte systems,current collectors,anode materials,and finally advanced cathodes materials used in Li-ion batteries,with the emphasis on contributions from the authors’group.ExperimentalMany of the materials reviewed were studied in this laboratory,therefore the experimental details have been provided as follows.The LiMO 2compounds studied were prepared via self-combus-tion reactions (SCRs).24Li[MnNiCo]O 2and Li 2MnO 3$Li/MnNiCo]O 2materials were produced in nano-andsubmicrometric particles both produced by SCR with different annealing stages (700 C for 1hour in air,900 C or 1000 C for 22hours in air,respectively).LiMn 1.5Ni 0.5O 4spinel particles were also synthesized using SCR.Li 4T 5O 12nanoparticles were obtained from NEI Inc.,USA.Graphitic material was obtained from Superior Graphite (USA),Timcal (Switzerland),and Conoco-Philips.LiMn 0.8Fe 0.2PO 4was obtained from HPL Switzerland.Standard electrolyte solutions (alkyl carbonates/LiPF 6),ready to use,were obtained from UBE,Japan.Ionic liquids were obtained from Merck KGaA (Germany and Toyo Gosie Ltd.,(Japan)).The surface chemistry of the various electrodes was charac-terized by the following techniques:Fourier transform infrared (FTIR)spectroscopy using a Magna 860Spectrometer from Nicolet Inc.,placed in a homemade glove box purged with H 2O and CO 2(Balson Inc.air purification system)and carried out in diffuse reflectance mode;high-resolution transmission electron microscopy (HR-TEM)and scanning electron microscopy (SEM),using a JEOL-JEM-2011(200kV)and JEOL-JSM-7000F electron microscopes,respectively,both equipped with an energy dispersive X-ray microanalysis system from Oxford Inc.;X-ray photoelectron spectroscopy (XPS)using an HX Axis spectrom-eter from Kratos,Inc.(England)with monochromic Al K a (1486.6eV)X-ray beam radiation;solid state 7Li magic angle spinning (MAS)NMR performed at 194.34MHz on a Bruker Avance 500MHz spectrometer in 3.2mm rotors at spinning speeds of 18–22kHz;single pulse and rotor synchronized Hahn echo sequences were used,and the spectra were referenced to 1M LiCl at 0ppm;MicroRaman spectroscopy with a spectrometerfrom Jobin-Yvon Inc.,France.We also used M €ossbauer spec-troscopy for studying the stability of LiMPO 4compounds (conventional constant-acceleration spectrometer,room temperature,50mC:57Co:Rh source,the absorbers were put in Perspex holders.In situ AFM measurements were carried out using the system described in ref.25.The following electrochemical measurements were posite electrodes were prepared by spreading slurries comprising the active mass,carbon powder and poly-vinylidene difluoride (PVdF)binder (ratio of 75%:15%:10%by weight,mixed into N -methyl pyrrolidone (NMP),and deposited onto aluminium foil current collectors,followed by drying in a vacuum oven.The average load was around 2.5mg active mass per cm 2.These electrodes were tested in two-electrode,coin-type cells (Model 2032from NRC Canada)with Li foil serving as the counter electrode,and various electrolyte puter-ized multi-channel battery analyzers from Maccor Inc.(USA)and Arbin Inc.were used for galvanostatic measurements (voltage vs.time/capacity,measured at constant currents).Results and discussionOur road map for materials developmentFig.1indicates a suggested road map for the direction of Li-ion research.The axes are voltage and capacity,and a variety of electrode materials are marked therein according to their respective values.As is clear,the main limiting factor is the cathode material (in voltage and capacity).The electrode mate-rials currently used in today’s practical batteries allow forD o w n l o a d e d b y B e i j i n g U n i v e r s i t y o f C h e m i c a l T e c h n o l o g y o n 24 F e b r u a r y 2011P u b l i s h e d o n 23 F e b r u a r y 2011 o n h t t p ://p u b s .r s c .o r g | d o i :10.1039/C 0J M 04225Ka nominal voltage of below 4V.The lower limit of the electro-chemical window of the currently used electrolyte solutions (alkyl carbonates/LiPF 6)is approximately 1.5V vs.Li 26(see later discussion about the passivation phenomena that allow for the operation of lower voltage electrodes,such as Li and Li–graphite).The anodic limit of the electrochemical window of the alkyl carbonate/LiPF 6solutions has not been specifically determined but practical accepted values are between 4.2and 5V vs.Li 26(see further discussion).With some systems which will be discussed later,meta-stability up to 4.9V can be achieved in these standard electrolyte solutions.Electrolyte solutionsThe anodic stability limits of electrolyte solutions for Li-ion batteries (and those of polar aprotic solutions in general)demand ongoing research in this subfield as well.It is hard to define the onset of oxidation reactions of nonaqueous electrolyte solutions because these strongly depend on the level of purity,the presence of contaminants,and the types of electrodes used.Alkyl carbonates are still the solutions of choice with little competition (except by ionic liquids,as discussed below)because of the high oxidation state of their central carbon (+4).Within this class of compounds EC and DMC have the highest anodic stability,due to their small alkyl groups.An additional benefit is that,as discussed above,all kinds of negative electrodes,Li,Li–graphite,Li–Si,etc.,develop excellent passivation in these solutions at low potentials.The potentiodynamic behavior of polar aprotic solutions based on alkyl carbonates and inert electrodes (Pt,glassy carbon,Au)shows an impressive anodic stability and an irreversible cathodic wave whose onset is $1.5vs.Li,which does not appear in consequent cycles due to passivation of the anode surface bythe SEI.The onset of these oxidation reactions is not well defined (>4/5V vs.Li).An important discovery was the fact that in the presence of Li salts,EC,one of the most reactive alkyl carbonates (in terms of reduction),forms a variety of semi-organic Li-con-taining salts that serve as passivation agents on Li,Li–carbon,Li–Si,and inert metal electrodes polarized to low potentials.Fig.2and Scheme 1indicates the most significant reduction schemes for EC,as elucidated through spectroscopic measure-ments (FTIR,XPS,NMR,Raman).27–29It is important to note (as reflected in Scheme 1)that the nature of the Li salts present greatly affects the electrode surface chemistry.When the pres-ence of the salt does not induce the formation of acidic species in solutions (e.g.,LiClO 4,LiN(SO 2CF 3)2),alkyl carbonates are reduced to ROCO 2Li and ROLi compounds,as presented in Fig. 2.In LiPF 6solutions acidic species are formed:LiPF 6decomposes thermally to LiF and PF 5.The latter moiety is a Lewis acid which further reacts with any protic contaminants (e.g.unavoidably present traces of water)to form HF.The presence of such acidic species in solution strongly affects the surface chemistry in two ways.One way is that PF 5interactswithFig.1The road map for R&D of new electrode materials,compared to today’s state-of-the-art.The y and x axes are voltage and specific capacity,respectively.Fig.2A schematic presentation of the CV behavior of inert (Pt)elec-trodes in various families of polar aprotic solvents with Li salts.26D o w n l o a d e d b y B e i j i n g U n i v e r s i t y o f C h e m i c a l T e c h n o l o g y o n 24 F e b r u a r y 2011P u b l i s h e d o n 23 F e b r u a r y 2011 o n h t t p ://p u b s .r s c .o r g | d o i :10.1039/C 0J M 04225Kthe carbonyl group and channels the reduction process of EC to form ethylene di-alkoxide species along with more complicated alkoxy compounds such as binary and tertiary ethers,rather than Li-ethylene dicarbonates (see schemes in Fig.2);the other way is that HF reacts with ROLi and ROCO 2Li to form ROH,ROCO 2H (which further decomposes to ROH and CO 2),and surface LiF.Other species formed from the reduction of EC are Li-oxalate and moieties with Li–C and C–F bonds (see Scheme 1).27–31Efforts have been made to enhance the formation of the passivation layer (on graphite electrodes in particular)in the presence of these solutions through the use of surface-active additives such as vinylene carbonate (VC)and lithium bi-oxalato borate (LiBOB).27At this point there are hundreds of publica-tions and patents on various passivating agents,particularly for graphite electrodes;their further discussion is beyond the scope of this paper.Readers may instead be referred to the excellent review by Xu 32on this subject.Ionic liquids (ILs)have excellent qualities that could render them very relevant for use in advanced Li-ion batteries,including high anodic stability,low volatility and low flammability.Their main drawbacks are their high viscosities,problems in wetting particle pores in composite structures,and low ionic conductivity at low temperatures.Recent years have seen increasing efforts to test ILs as solvents or additives in Li-ion battery systems.33Fig.3shows the cyclic voltammetric response (Pt working electrodes)of imidazolium-,piperidinium-,and pyrrolidinium-based ILs with N(SO 2CF 3)2Àanions containing LiN(SO 2CF 3)2salt.34This figure reflects the very wide electrochemical window and impressive anodic stability (>5V)of piperidium-and pyr-rolidium-based ILs.Imidazolium-based IL solutions have a much lower cathodic stability than the above cyclic quaternary ammonium cation-based IL solutions,as demonstrated in Fig.3.The cyclic voltammograms of several common electrode mate-rials measured in IL-based solutions are also included in the figure.It is clearly demonstrated that the Li,Li–Si,LiCoO 2,andLiMn 1.5Ni 0.5O 4electrodes behave reversibly in piperidium-and pyrrolidium-based ILs with N(SO 2CF 3)2Àand LiN(SO 2CF 3)2salts.This figure demonstrates the main advantage of the above IL systems:namely,the wide electrochemical window with exceptionally high anodic stability.It was demonstrated that aluminium electrodes are fully passivated in solutions based on derivatives of pyrrolidium with a N(SO 2CF 3)2Àanion and LiN(SO 2CF 3)2.35Hence,in contrast to alkyl carbonate-based solutions in which LiN(SO 2CF 3)2has limited usefulness as a salt due to the poor passivation of aluminium in its solutions in the above IL-based systems,the use of N(SO 2CF 3)2Àas the anion doesn’t limit their anodic stability at all.In fact it was possible to demonstrate prototype graphite/LiMn 1.5Ni 0.5O 4and Li/L-iMn 1.5Ni 0.5O 4cells operating even at 60 C insolutionsScheme 1A reaction scheme for all possible reduction paths of EC that form passivating surface species (detected by FTIR,XPS,Raman,and SSNMR 28–31,49).Fig.3Steady-state CV response of a Pt electrode in three IL solutions,as indicated.(See structure formulae presented therein.)The CV presentations include insets of steady-state CVs of four electrodes,as indicated:Li,Li–Si,LiCoO 2,and LiMn 1.5Ni 0.5O 4.34D o w n l o a d e d b y B e i j i n g U n i v e r s i t y o f C h e m i c a l T e c h n o l o g y o n 24 F e b r u a r y 2011P u b l i s h e d o n 23 F e b r u a r y 2011 o n h t t p ://p u b s .r s c .o r g | d o i :10.1039/C 0J M 04225Kcomprising alkyl piperidium-N(SO2CF3)2as the IL solvent and Li(SO2CF3)2as the electrolyte.34Challenges remain in as far as the use of these IL-based solutions with graphite electrodes.22Fig.4shows the typical steady state of the CV of graphite electrodes in the IL without Li salts.The response in this graph reflects the reversible behavior of these electrodes which involves the insertion of the IL cations into the graphite lattice and their subsequent reduction at very low potentials.However when the IL contains Li salt,the nature of the reduction processes drastically changes.It was recently found that in the presence of Li ions the N(SO2CF3)2Àanion is reduced to insoluble ionic compounds such as LiF,LiCF3, LiSO2CF3,Li2S2O4etc.,which passivate graphite electrodes to different extents,depending on their morphology(Fig.4).22 Fig.4b shows a typical SEM image of a natural graphite(NG) particle with a schematic view of its edge planes.Fig.4c shows thefirst CVs of composite electrodes comprising NG particles in the Li(SO2CF3)2/IL solution.These voltammograms reflect an irreversible cathodic wave at thefirst cycle that belongs to the reduction and passivation processes and their highly reversible repeated Li insertion into the electrodes comprising NG. Reversible capacities close to the theoretical ones have been measured.Fig.4d and e reflect the structure and behavior of synthetic graphiteflakes.The edge planes of these particles are assumed to be much rougher than those of the NG particles,and so their passivation in the same IL solutions is not reached easily. Their voltammetric response reflects the co-insertion of the IL cations(peaks at0.5V vs.Li)together with Li insertion at the lower potentials(<0.3V vs.Li).Passivation of this type of graphite is obtained gradually upon repeated cycling(Fig.4e), and the steady-state capacity that can be obtained is much lower than the theoretical one(372mA h gÀ1).Hence it seems that using graphite particles with suitable morphologies can enable their highly reversible and stable operation in cyclic ammonium-based ILs.This would make it possible to operate high voltage Li-ion batteries even at elevated temperatures(e.g. 4.7–4.8V graphite/LiMn1.5Ni0.5O4cells).34 The main challenge in thisfield is to demonstrate the reasonable performance of cells with IL-based electrolytes at high rates and low temperatures.To this end,the use of different blends of ILs may lead to future breakthroughs.Current collectorsThe current collectors used in Li-ion systems for the cathodes can also affect the anodic stability of the electrolyte solutions.Many common metals will dissolve in aprotic solutions in the potential ranges used with advanced cathode materials(up to5V vs.Li). Inert metals such as Pt and Au are also irrelevant due to cost considerations.Aluminium,however,is both abundantand Fig.4A collection of data related to the behavior of graphite electrodes in butyl,methyl piperidinium IL solutions.22(a)The behavior of natural graphite electrodes in pure IL without Li salt(steady-state CV is presented).(b)The schematic morphology and a SEM image of natural graphite(NG)flakes.(c)The CV response(3first consecutive cycles)of NG electrodes in IL/0.5lithium trifluoromethanesulfonimide(LiTFSI)solution.(d and e)Same as(b and c)but for synthetic graphiteflakes.DownloadedbyBeijingUniversityofChemicalTechnologyon24February211Publishedon23February211onhttp://pubs.rsc.org|doi:1.139/CJM4225Kcheap and functions very well as a current collector due to its excellent passivation properties which allow it a high anodic stability.The question remains as to what extent Al surfaces can maintain the stability required for advanced cathode materials (up to 5V vs.Li),especially at elevated temperatures.Fig.5presents the potentiodynamic response of Al electrodes in various EC–DMC solutions,considered the alkyl carbonate solvent mixture with the highest anodic stability,at 30and 60 C.37The inset to this picture shows several images in which it is demonstrated that Al surfaces are indeed active and develop unique morphologies in the various solutions due to their obvious anodic processes in solutions,some of which lead to their effective passivation.The electrolyte used has a critical impact on the anodic stability of the Al.In general,LiPF 6solutions demonstrate the highest stability even at elevated temperatures due to the formation of surface AlF 3and even Al(PF 6)3.Al CCs in EC–DMC/LiPF 6solutions provide the highest anodic stability possible for conventional electrode/solution systems.This was demonstrated for Li/LiMn 1.5Ni 0.5O 4spinel (4.8V)cells,even at 60 C.36This was also confirmed using bare Al electrodes polarized up to 5V at 60 C;the anodic currents were seen to decay to negligible values due to passivation,mostly by surface AlF 3.37Passivation can also be reached in Li(SO 2CF 3),LiClO 4and LiBOB solutions (Fig.5).Above 4V (vs.Li),the formation of a successful passivation layer on Al CCs is highly dependent on the electrolyte formula used.The anodic stability of EC–DMC/LiPF 6solutions and Al current collectors may be further enhanced by the use of additives,but a review of additives in itself deserves an article of its own and for this readers are again referred to the review by Xu.32When discussing the topic of current collectors for Li ion battery electrodes,it is important to note the highly innovative work on (particularly anodic)current collectors by Taberna et al.on nano-architectured Cu CCs 47and Hu et al.who assembled CCs based on carbon nano-tubes for flexible paper-like batteries,38both of whom demonstrated suberb rate capabilities.39AnodesThe anode section in Fig.1indicates four of the most promising groups of materials whose Li-ion chemistry is elaborated as follows:1.Carbonaceous materials/graphite:Li ++e À+C 6#LiC 62.Sn and Si-based alloys and composites:40,41Si(Sn)+x Li ++x e À#Li x Si(Sn),X max ¼4.4.3.Metal oxides (i.e.conversion reactions):nano-MO +2Li ++2e À#nano-MO +Li 2O(in a composite structure).424.Li x TiO y electrodes (most importantly,the Li 4Ti 5O 12spinel structure).43Li 4Ti 5O 12+x Li ++x e À#Li 4+x Ti 5O 12(where x is between 2and 3).Conversion reactions,while they demonstrate capacities much higher than that of graphite,are,practically speaking,not very well-suited for use as anodes in Li-ion batteries as they generally take place below the thermodynamic limit of most developed electrolyte solutions.42In addition,as the reactions require a nanostructuring of the materials,their stability at elevated temperatures will necessarily be an issue because of the higher reactivity (due to the 1000-fold increase in surface area).As per the published research on this topic,only a limited meta-stability has been demonstrated.Practically speaking,it does not seem likely that Li batteries comprising nano-MO anodes will ever reach the prolonged cycle life and stability required for EV applications.Tin and silicon behave similarly upon alloying with Li,with similar stoichiometries and >300%volume changes upon lith-iation,44but the latter remain more popular,as Si is much more abundant than Sn,and Li–Si electrodes indicate a 4-fold higher capacity.The main approaches for attaining a workable revers-ibility in the Si(Sn)–Li alloying reactions have been through the use of both nanoparticles (e.g.,a Si–C nanocomposites 45)and composite structures (Si/Sn–M1–M2inter-metalliccompounds 44),both of which can better accommodate these huge volume changes.The type of binder used in composite electrodes containing Si particles is very important.Extensive work has been conducted to determine suitable binders for these systems that can improve the stability and cycle life of composite silicon electrodes.46As the practical usage of these systems for EV applications is far from maturity,these electrodes are not dis-cussed in depth in this paper.However it is important to note that there have been several recent demonstrations of how silica wires and carpets of Si nano-rods can act as much improved anode materials for Li battery systems in that they can serveasFig.5The potentiodynamic behavior of Al electrodes (current density measured vs.E during linear potential scanning)in various solutions at 30and 60 C,as indicated.The inset shows SEM micrographs of passivated Al surfaces by the anodic polarization to 5V in the solutions indicated therein.37D o w n l o a d e d b y B e i j i n g U n i v e r s i t y o f C h e m i c a l T e c h n o l o g y o n 24 F e b r u a r y 2011P u b l i s h e d o n 23 F e b r u a r y 2011 o n h t t p ://p u b s .r s c .o r g | d o i :10.1039/C 0J M 04225K。
chemicalreactionengineering3ededition作者octavelevenspiel课后习题答案
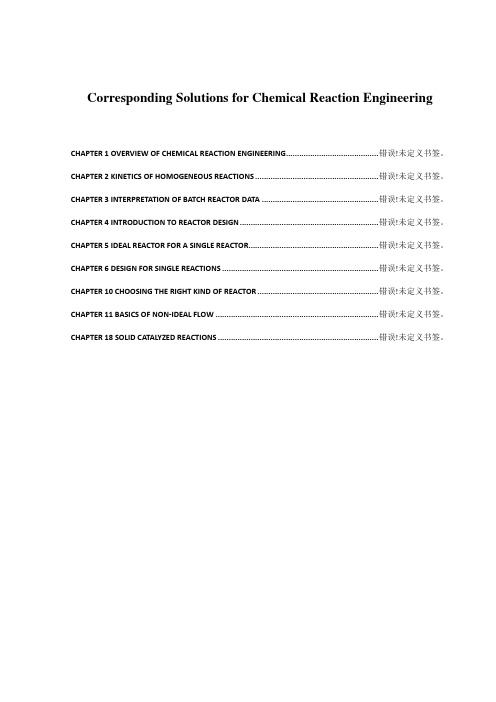
Corresponding Solutions for Chemical Reaction EngineeringCHAPTER 1 OVERVIEW OF CHEMICAL REACTION ENGINEERING .......................................... 错误!未定义书签。
CHAPTER 2 KINETICS OF HOMOGENEOUS REACTIONS ........................................................ 错误!未定义书签。
CHAPTER 3 INTERPRETATION OF BATCH REACTOR DATA ..................................................... 错误!未定义书签。
CHAPTER 4 INTRODUCTION TO REACTOR DESIGN ............................................................... 错误!未定义书签。
CHAPTER 5 IDEAL REACTOR FOR A SINGLE REACTOR........................................................... 错误!未定义书签。
CHAPTER 6 DESIGN FOR SINGLE REACTIONS ....................................................................... 错误!未定义书签。
CHAPTER 10 CHOOSING THE RIGHT KIND OF REACTOR ....................................................... 错误!未定义书签。
2012-HCI-MA-H2-P1-Prelim
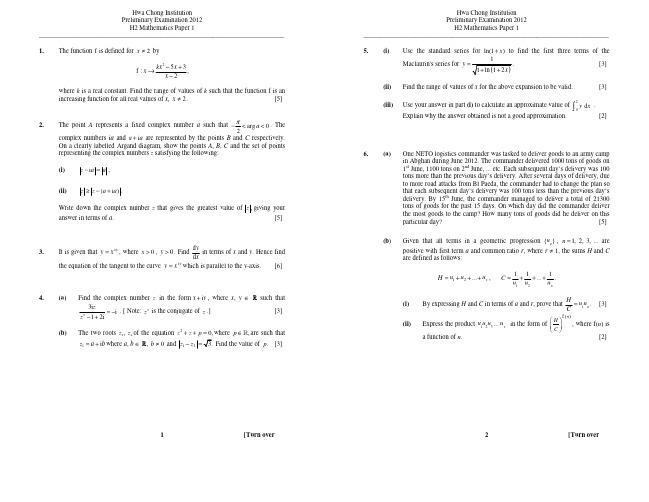
(i)
f :x→
kx 2 − 5 x + 3 , x−2
(ii) (iii)
Use the standard series for ln(1 + x ) to find the first three terms of the 1 Maclaurin's series for y = . [3] 1 + ln (1 + 2 x )
One NETO logistics commander was tasked to deliver goods to an army camp in Abghan during June 2012. The commander delivered 1000 tons of goods on 1st June, 1100 tons on 2nd June, ... etc. Each subsequent day’s delivery was 100 tons more than the previous day’s delivery. After several days of delivery, due to more road attacks from Bl Paeda, the commander had to change the plan so that each subsequent day’s delivery was 100 tons less than the previous day’s delivery. By 15th June, the commander managed to deliver a total of 21300 tons of goods for the past 15 days. On which day did the commander deliver the most goods to the camp? How many tons of goods did he deliver on this particular day? [5] Given that all terms in a geometric progression {un } , n = 1, 2, 3, ... are positive with first term a and common ratio r, where r ≠ 1, the sums H and C are defined as follows:
2010年高考化学真题(Word版)——全国1卷(试题+答案解析)
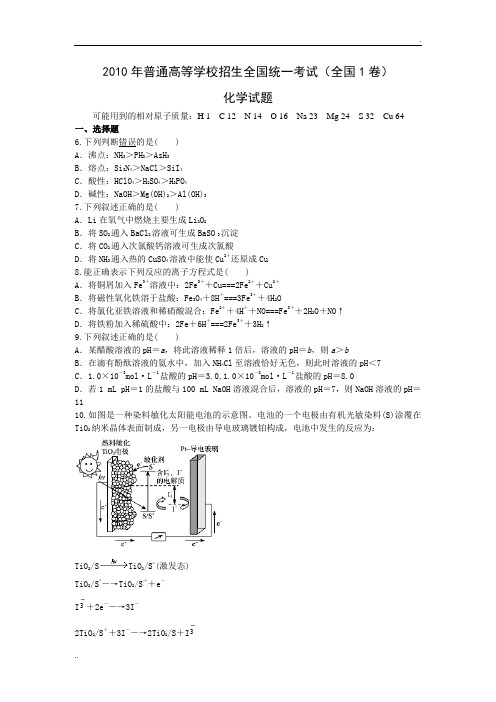
2010年普通高等学校招生全国统一考试(全国1卷)化学试题可能用到的相对原子质量:H 1 C 12N 14O 16Na 23Mg 24S 32Cu 64一、选择题6.下列判断错误的是( )A.沸点:NH3>PH3>AsH3B.熔点:Si3N4>NaCl>SiI4C.酸性:HClO4>H2SO4>H3PO4D.碱性:NaOH>Mg(OH)2>Al(OH)37.下列叙述正确的是( )A.Li在氧气中燃烧主要生成Li2O2B.将SO2通入BaCl2溶液可生成BaSO 3沉淀C.将CO2通入次氯酸钙溶液可生成次氯酸D.将NH3通入热的CuSO4溶液中能使Cu2+还原成Cu8.能正确表示下列反应的离子方程式是( )A.将铜屑加入Fe3+溶液中:2Fe3++Cu===2Fe2++Cu2+B.将磁性氧化铁溶于盐酸:Fe3O4+8H+===3Fe3++4H2OC.将氯化亚铁溶液和稀硝酸混合:Fe2++4H++NO===Fe3++2H2O+NO↑D.将铁粉加入稀硫酸中:2Fe+6H+===2Fe3++3H2↑9.下列叙述正确的是( )A.某醋酸溶液的pH=a,将此溶液稀释1倍后,溶液的pH=b,则a>bB.在滴有酚酞溶液的氨水中,加入NH4Cl至溶液恰好无色,则此时溶液的pH<7C.1.0×10-3mol·L-1盐酸的pH=3.0,1.0×10-8mol·L-1盐酸的pH=8.0D.若1 mL pH=1的盐酸与100 mL NaOH溶液混合后,溶液的pH=7,则NaOH溶液的pH=1110.如图是一种染料敏化太阳能电池的示意图。
电池的一个电极由有机光敏染料(S)涂覆在TiO2纳米晶体表面制成,另一电极由导电玻璃镀铂构成,电池中发生的反应为:TiO2/S TiO2/S*(激发态)TiO2/S*―→TiO2/S++e-I+2e-―→3I-2TiO2/S++3I-―→2TiO2/S+I下列关于该电池叙述错误的是( )A.电池工作时,是将太阳能转化为电能B.电池工作时,I-在镀铂导电玻璃电极上放电C.电池中镀铂导电玻璃为正极D.电池的电解质溶液中I-和I的浓度不会减少11.下图表示4溴环己烯所发生的4个不同反应。
Nature Materials 9, 904-907(2010)--np-NiPt for ORR

PUBLISHED ONLINE: 17 OCTOBER 2010 | DOI: 10.1038/NMAT2878
Oxygen reduction in nanoporous metal–ionic liquid composite electrocatalysts
J. Snyder1, T. Fujita2, M. W. Chen2 and J. Erlebacher1*
The improvement of catalysts for the four-electron oxygenreduction reaction (ORR; O2 + 4H+ + 4e− → 2H2O) remains a critical challenge for fuel cells and other electrochemicalenergy technologies. Recent attention in this area has centred on the development of metal alloys with nanostructured compositional gradients (for example, core–shell structure) that exhibit higher activity than supported Pt nanoparticles (Pt–C; refs 1–7). For instance, with a Pt outer surface and Ni-rich second atomic layer, Pt3Ni(111) is one of the most active surfaces for the ORR (ref. 8), owing to a shift in the d-band centre of the surface Pt atoms that results in a weakened interaction between Pt and intermediate oxide species, freeing more active sites for O2 adsorption2,9. However, enhancements due solely to alloy structure and composition may not be sufficient to reduce the mass activity enough to satisfy the requirements for fuel-cell commercialization10, especially as the high activity of particular crystal surface facets may not easily translate to polyfaceted particles. Here we show that a tailored geometric and chemical materials architecture can further improve ORR catalysis by demonstrating that a composite nanoporous Ni–Pt alloy impregnated with a hydrophobic, high-oxygen-solubility and protic ionic liquid has extremely high mass activity. The results are consistent with an engineered chemical bias within a catalytically active nanoporous framework that pushes the ORR towards completion.
IB 化学pastpaper,2010,paper3, TZ1

Consider the proton environments present in each of the alcohol molecules when answering the following questions. (i) Identify which alcohol gives spectrum 1 and explain your answer by stating which hydrogen atoms in the molecule are responsible for each of the two peaks. . . . . . . . . . . . . . . . . . . . . . . . . . . . . . . . . . . . . . . . . . . . . . . . . . . . . . . . . . . . . . . . . . . . . . . . . . . . . . . . . . . . . . . . . . . . . . . . . . . . . . . . . . . . . . . . . . . . . . . . . . . . . . . . . . . . . . . . . . . . . . . . . . . . . . . . . . . . . . . . . . . . . . . . . . . . . . . . . . . . . . . . . . . . . . . . . . . . . . . . . . . . . . . . . . . . . . . . . . . . . . . . . . . . . . . . . . . . . . . . . . . . . . . . . . . . . . . . . . . . . . . . . . . . . . . . . . . . . . . . . . . . . . . . . . . . . . . . . . . . . . . . . . . . . . . . . . . . . . . . . . . . . . . . . . . . . . . . . . . . . . . . . . . . . . . . . . . . . . . . . . . . . . . . . . . . . . . . . . . . . . . . . . . . . . . (ii) Deduce which alcohol gives spectrum 2. Explain which particular hydrogen atoms in the molecule are responsible for the peaks at 0.9 ppm and 3.4 ppm. . . . . . . . . . . . . . . . . . . . . . . . . . . . . . . . . . . . . . . . . . . . . . . . . . . . . . . . . . . . . . . . . . . . . . . . . . . . . . . . . . . . . . . . . . . . . . . . . . . . . . . . . . . . . . . . . . . . . . . . . . . . . . . . . . . . . . . . . . . . . . . . . . . . . . . . . . . . . . . . . . . . . . . . . . . . . . . . . . . . . . . . . . . . . . . . . . . . . . . . . . . . . . . . . . . . . . . . . . . . . . . . . . . . . . . . . . . . . . . . . . . . . . . . . . . . . . . . . . . . . . . . . . . . . . . . . . . . . . . . . . . . . . . . . . . . . . . . . . . . . . . . . . . . . . . . . . . . . . . . . . . . . . . . . . . . . . . . . . . . . . . . . . . . . . . . . . . . . . . . . . . . . . . . . . . . . . . . . . . . . . . . . . . . . . . [3] [3]
2010年硕士学位研究生入学考试物理化学试题
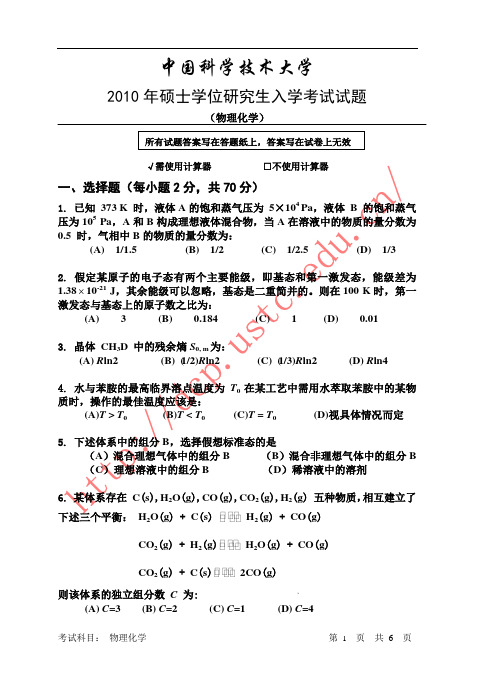
$ $ (D) Pt|H 2 (p )|H 2 SO4 ( a ) |O 2 (p )|Pt
30.电池的电动势测定中不需要用到的装置或方法是: (A) 对消法 (B) Wheatstone 电桥 (C) 温度计 (D) 标准电池
+ 31.298K 时,在 Pt | H 2 ( p = 1 atm) | H ( aH + ) CuSO4 ( aCuSO4 ) | Cu ( s ) 右边的
考试科目: 物理化学 第 3 页 共 6 页
(D) 活化熵
(A) θ = k ⋅ p1/n
a ⋅p (C) θ = 1+ a ⋅ p
= (B) θ
RT ln ( A 0 ⋅ p ) a
C⋅p ( ps − p ) 1 + ( c − 1) p / ps
(D)
V = Vm
25.对反 应 A3+ + B2- ⇔ {A⋅⋅⋅B}+ → P,当向体系中加入惰性支持电解质时,其 反应速率常数将: (A) 增大 (B)减小 (C)不变 (D)很难确定 26.当电解池的两极流过一定电流时,阴极和阳极的电势(用ϕc 和ϕa 表示) 将比平衡时的电极电势 (A) 二者均更正 (B) 二者均更负 (C) ϕc 更负,ϕa 更正 (D) ϕc 更正,ϕa 更负 27.一定浓度下,某强电解质溶液的摩尔电导率是 0.12 Ω-1⋅m2⋅mol-1, 当向该 溶液加入 2 m3 的水时,其摩尔电导率将 (A) 增大 (B)减小 (C)不变 (D)很难确定 28.通过电沉积的方式对溶液中金属离子 A2+和 B2+进行分离, 若要使[A2+] ≤ 10-7∙[B2+], 则要求ϕB2+|B − ϕA2+|A (A) > 0.21 V (B) < -0.21 V (C) >0.14 V (D) <-0.14 V 29.下列电池中,哪一个不能用来测量 H2O 的离子积 KW?
化学工程系毕业论文答辩PPT模板

A
L
E
N
G
I
N
E
Y
目录 CONTENTS
添加标题文字
添加标题文字
添加标题文字
添加标题文字
C
H
E
M
I
C
A
L
E
N
G
I
N
E
Y
C
H
E
M
I
C
A
L
E
N
G
I
N
E
Y
PART 01
添加标题文字
Lorem Ipsum is simply dummy text of the printing and typesetting industry. Lorem Ipsum has been the industry's standard dummy text ever since
单击此处添加您的文本或者复制您合适 的内容粘贴到此以填充文本框。单击此 处添加您的文本或者复制您合适的内容 粘贴到此以填充文本框。
单击此处添加您的文 本或者复制您
单击此处添加您的文 本或者复制您
单击此处添加您的文本或者复制您合适的内容粘贴到此以填充文本框。单击 此处添加您的文本粘贴到此以填充文本框。
请输入标题
单击此处添加标题内容,内容要与标题 相符,注意答辩顺序及文本匹配程度
添加标题文字
单击添加小标题
简单说明即可,不必过于繁琐,注意版面美观度。此处 添加具体内容,文字尽量言简意赅,简单说明即可。
简单说明即可,不必过于繁琐,注意版面美观度。此处 添加具体内容,文字尽量言简意赅,简单说明即可。
简单说明即可,不必过于繁琐,注意版面美观度。此处 添加具体内容,文字尽量言简意赅,简单说明即可。
- 1、下载文档前请自行甄别文档内容的完整性,平台不提供额外的编辑、内容补充、找答案等附加服务。
- 2、"仅部分预览"的文档,不可在线预览部分如存在完整性等问题,可反馈申请退款(可完整预览的文档不适用该条件!)。
- 3、如文档侵犯您的权益,请联系客服反馈,我们会尽快为您处理(人工客服工作时间:9:00-18:30)。
Deduce the order of the reaction with respect to I. propanone II. iodine III. hydrogen ions hence, write a rate equation for the reaction.
(ii)
A mechanism for the reaction is suggested in the scheme given below.
H+ Step 1 CH3 C CH2 OH I2 Step 2 CH3COCH2I + H+ + I-
CH3COCH3
The reaction between propanone and bromine proceeds by a similar mechanism. How would you expect the rate of this reaction to compare with that of the above reaction? Explain your answer. [6] (b) Use of the Data Booklet is relevant for this question. When a M3+/M2+ half-cell was connected to a standard hydrogen electrode, electrons flowed from the standard hydrogen electrode to the M3+/M2+ half-cell. When the M3+/M2+ half-cell was connected to the Ni2+/Ni half-cell, it was found that the electromotive force of this cell was +1.08V.
V
Pt Ni
M3+ (aq), M2+ (aq)
Ni2+(aq)
Deduce whether the M3+/M2+ half-cell is the cathode or the anode in the above set up, hence, calculate the standard reduction potential for the M3+/M2+ half-cell. [2]
9647/03, 9746/03
19 August 2010 2 hours
Write your name and class on all the work you hand in. Write in dark blue or black pen on both sides of the paper. You may use a soft pencil for any diagrams, graphs or rough work. Do not use staples, paper clips, highlighters, glue or correction fluid. Answer any four questions. A Data Booklet is provided. You are reminded of the need for good English and clear presentation in your answers The number of marks is given in the brackets [ ] at the end of each question or part question. At the end of the examination, fasten all your work securely together with the cover page provided.
(ii)
(d)
Transition metals and their compounds are used as catalysts due to their ability to exist in variable oxidation states. Peroxodisulfate(VI) ion, S2O82-, is capable of oxidising the tartrate ion, [CH(OH)(CO2-)]2, to carbon dioxide and methanoate ion: H
Explain the chemistry involved when excess aqueous ammonia is gradually added to the aqueous solution of copper (II) suphate. Explain why transition element complexes such as [Cu(NH3)4]2+ and [CuCl4]2-are coloured. [5] [Total: 20]
CH2 CH2 CH2 CH2 NH2 O H2N CH C OH
O
O H2N CH C OH H
H2N CH C OH CH2 CH2 C O OH
Lys pI (i) 9.7
Gly 6.0
Glu 3.2
Suggest a pH of the buffer solution which allows the 3 amino acids to be separated in this electrophoresis procedure. Hence, describe how the 3 amino acids will be separated. A student attempts to denature a protein consisting of Lys and Glu residues by heating to 100°C. Predict, with reasoning, whether the student’s attempt will be successful. Draw the displayed formula of a protein consisting of glu-lys-gly residue [7]
HO C CO2HO C CO2H
+ 3S2O82- + 2H2O
2CO2 + 2HCO2- + 6H+ + 6SO42-
The reaction is very slow, even when temperature is increased. However, the reaction rate can be increased by adding a suitable catalyst. (i) (ii) Suggest a reason why the reaction is slow. Given that the reduction potential for the tartrate ion is
[Turn over
3 (c) Nickel can also be electroplated onto objects to prevent corrosion. An experiment was carried out to coat a conducting object (surface area = 30 cm2) with nickel metal using NiCl2(aq). (i) Write equations to show the reactions that will occur at the anode and the cathode. In order for the object to be well coated with nickel metal, a minimum thickness of 0.20 mm must be formed on it. Calculate the minimum time needed to deposit this amount of nickel metal if a current of 0.20 A was used. [Density of nickel = 8.91 g cm-3] [3]
(ii)
[Turn over
4 2 (a) In a reaction, a tripeptide produces the following amino acids in equimolar amounts. The resulting solution was added to an excess of a buffer solution and placed at the centre of the plate. A potential difference was then applied across the plate. Amino Acid
1
SERANGOON JUNIOR COLLEGE General Certificate of Education Advanced Level Higher 2
CHEMISTRY
Preliminary Examination Paper 3 Free Response Questions
Additional Materials: Data Booklet Writing Papers READ THESE INSTRUCTIONS FIRST
This document consists of 11 printed pages and 1 blank page
[Turn over
2 1 The kinetics of the acid-catalysed reaction of propanone with iodine CH3COCH3 (aq) + I2 (aq) CH2ICOCH3 (aq) + HI (aq) can be investigated experimentally by varying the concentration of the three substances involved and determining the time for the colour of the iodine to disappear. The following results were obtained in such an experiment. Volume of propanone / cm3 8 8 4 8 (a) (i) Volume of iodine / cm3 4 4 4 2 Volume of sulphuric acid / cm3 8 4 8 8 Volume of water / cm3 0 4 4 2 Relative time for colour of iodine to disappear 1 2 2 1