SERS表面增强拉曼
表面增强拉曼光谱
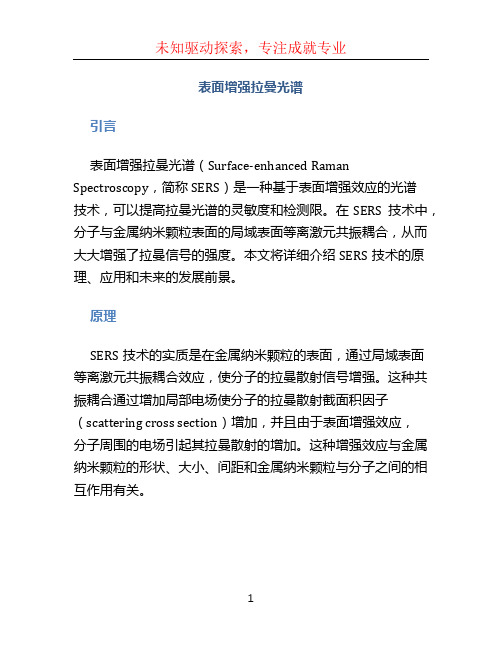
表面增强拉曼光谱引言表面增强拉曼光谱(Surface-enhanced Raman Spectroscopy,简称SERS)是一种基于表面增强效应的光谱技术,可以提高拉曼光谱的灵敏度和检测限。
在SERS技术中,分子与金属纳米颗粒表面的局域表面等离激元共振耦合,从而大大增强了拉曼信号的强度。
本文将详细介绍SERS技术的原理、应用和未来的发展前景。
原理SERS技术的实质是在金属纳米颗粒的表面,通过局域表面等离激元共振耦合效应,使分子的拉曼散射信号增强。
这种共振耦合通过增加局部电场使分子的拉曼散射截面积因子(scattering cross section)增加,并且由于表面增强效应,分子周围的电场引起其拉曼散射的增加。
这种增强效应与金属纳米颗粒的形状、大小、间距和金属纳米颗粒与分子之间的相互作用有关。
实验方法SERS实验通常使用激光作为光源,经过一个光栅或者光束分离镜,使得激光聚焦到样品表面。
此外,还需使用金属纳米颗粒作为增敏基质。
在实验过程中,样品可以是液体、固体或气体。
SERS光谱测量通常使用拉曼散射光谱仪进行。
与普通的拉曼光谱仪相比,SERS光谱仪需要更高的灵敏度和稳定性。
常用的金属纳米颗粒包括银、金、铜等,具体的选择取决于实验所需的增强效果和波长。
应用SERS技术在许多领域有着广泛的应用,包括化学分析、生物医学、环境监测等。
在化学分析领域,SERS能够提供准确的分子结构信息,可用于表征和鉴定化合物。
对于非常低浓度的物质,SERS技术是一种极其敏感的检测方法。
在生物医学领域,SERS被广泛用于生物分子的检测、肿瘤标记物的检测以及药物递送系统的研究。
由于SERS技术具有高灵敏度和高特异性,可以用于早期癌症诊断和治疗过程中药物的监测。
在环境监测领域,SERS技术可用于检测和监测环境中的微量有毒物质,例如水中的重金属离子或化学污染物。
发展前景虽然SERS技术已经取得了巨大的成功,并在许多领域得到了广泛应用,但仍然存在一些挑战需要克服。
sers表面增强拉曼光谱

sers表面增强拉曼光谱的基本原理和应用SERS(Surface-enhanced Raman Spectroscopy)表面增强拉曼光谱是一种功能强大的分析技术,用于增强和检测分子的拉曼散射信号。
它结合了拉曼光谱和表面增强效应(SERS效应),可以实现对微量样品的高灵敏度分析。
以下是SERS表面增强拉曼光谱的基本原理和应用:基本原理:1.SERS效应:SERS效应是指当分子或化合物置于具有纳米结构表面(如金属纳米颗粒)上时,它们的拉曼散射信号被显著增强的现象。
这种增强主要是由于局域表面等离激元共振的产生和电荷转移效应。
2.Raman散射:拉曼散射是一种基于光与物质相互作用的光谱技术,通过激发分子中的振动和旋转能级,从而产生特征性的散射光谱。
每种分子都有独特的拉曼散射光谱,可以用于研究分子结构、分析化学组成等。
应用:1.化学分析:SERS可以用于分析和鉴别化学物质,包括有机分子、无机化合物和生物分子等。
因其高灵敏度和选择性,可以应用于环境监测、食品安全和药品分析等领域。
2.生物医学研究:SERS在生物医学领域中具有广泛应用,如细胞成像、分子诊断、药物传递等。
可以通过利用SERS标记剂将其与生物分子(如蛋白质、核酸)结合来实现对生物分子的探测和定量。
3.表面分析:使用SERS技术可以研究材料的表面特性,包括表面催化反应、电化学过程和表面吸附等。
通过吸附在金属纳米颗粒上的分子的拉曼散射,可以获得有关表面化学反应和动力学的信息。
总之,SERS表面增强拉曼光谱是一种强大的分析技术,可用于高灵敏度和选择性的分子分析。
它在化学、生物医学和材料科学等领域中有广泛的应用前景。
SERS(表面增强拉曼散射)理论
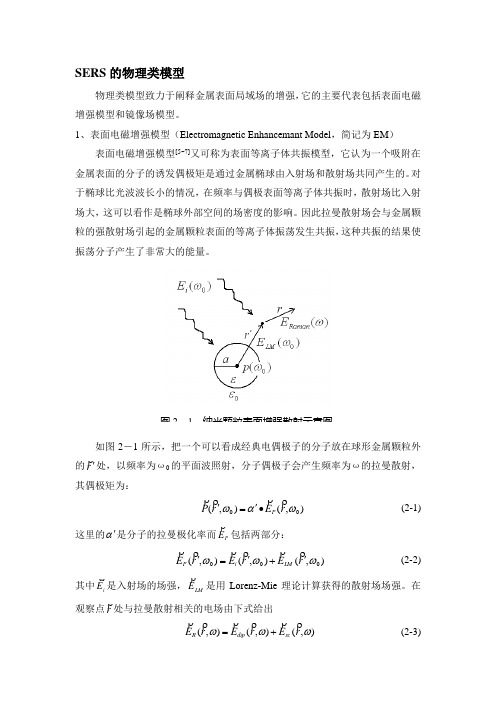
SERS 的物理类模型物理类模型致力于阐释金属表面局域场的增强,它的主要代表包括表面电磁增强模型和镜像场模型。
1、表面电磁增强模型(Electromagnetic Enhancemant Model ,简记为EM )表面电磁增强模型[5~7]又可称为表面等离子体共振模型,它认为一个吸附在金属表面的分子的诱发偶极矩是通过金属椭球由入射场和散射场共同产生的。
对于椭球比光波波长小的情况,在频率与偶极表面等离子体共振时,散射场比入射场大,这可以看作是椭球外部空间的场密度的影响。
因此拉曼散射场会与金属颗粒的强散射场引起的金属颗粒表面的等离子体振荡发生共振,这种共振的结果使振荡分子产生了非常大的能量。
如图2-1所示,把一个可以看成经典电偶极子的分子放在球形金属颗粒外的r 'ρ处,以频率为ω0的平面波照射,分子偶极子会产生频率为ω的拉曼散射,其偶极矩为:),(),(00ωαωr E r P P ρρρρ•'=' (2-1)这里的α'是分子的拉曼极化率而P E ρ包括两部分:),(),(),(000ωωωr E r E r E LM i P '+'='ρρρρρρ (2-2)其中i E ϖ是入射场的场强,LM E ρ是用Lorenz-Mie 理论计算获得的散射场场强。
在观察点r ρ处与拉曼散射相关的电场由下式给出),(),(),(ωωωr E r E r E sc dip R ρρρρρρ+=(2-3)图2-1 纳米颗粒表面增强散射示意图其中,dip E ρ是球形颗粒不存在时振荡偶极子P ρ发射的场,sc E ρ是由球形颗粒产生的必须满足频率ω的边值问题的散射场。
拉曼散射的强度R I 是远场振幅R E ρ的平方:2/)ex p(),(lim r ikr r E I R kr R ωϖϖ∞→=,增强因子G 定义为0R R I I G =,其中0R I 是在金属球形颗粒不存在时的拉曼强度。
SERS(表面增强拉曼散射)理论

SERS 的物理类模型物理类模型致力于阐释金属表面局域场的增强,它的主要代表包括表面电磁增强模型和镜像场模型。
1、表面电磁增强模型(Electromagnetic Enhancemant Model ,简记为EM )表面电磁增强模型[5~7]又可称为表面等离子体共振模型,它认为一个吸附在金属表面的分子的诱发偶极矩是通过金属椭球由入射场和散射场共同产生的。
对于椭球比光波波长小的情况,在频率与偶极表面等离子体共振时,散射场比入射场大,这可以看作是椭球外部空间的场密度的影响。
因此拉曼散射场会与金属颗粒的强散射场引起的金属颗粒表面的等离子体振荡发生共振,这种共振的结果使振荡分子产生了非常大的能量。
如图2-1所示,把一个可以看成经典电偶极子的分子放在球形金属颗粒外的r ' 处,以频率为ω0的平面波照射,分子偶极子会产生频率为ω的拉曼散射,其偶极矩为:),(),(00ωαωr E r P P ∙'=' (2-1)这里的α'是分子的拉曼极化率而P E包括两部分: ),(),(),(000ωωωr E r E r E LM i P '+'=' (2-2)其中i E 是入射场的场强,LM E 是用Lorenz-Mie 理论计算获得的散射场场强。
在观察点r 处与拉曼散射相关的电场由下式给出),(),(),(ωωωr E r E r E sc dip R +=(2-3)图2-1 纳米颗粒表面增强散射示意图其中,dip E 是球形颗粒不存在时振荡偶极子P 发射的场,sc E 是由球形颗粒产生的必须满足频率ω的边值问题的散射场。
拉曼散射的强度R I 是远场振幅R E 的平方:2/)exp(),(lim r ikr r E I R kr R ω ∞→=,增强因子G 定义为0R R I I G =,其中0R I 是在金属球形颗粒不存在时的拉曼强度。
那么在小颗粒的限制下,增强因子可由下式给出:[]230333033303)(3)1/()1/()(3i n n r g a r i r g a g a r i i n n g a i G ⋅+'+'-'+'-⋅+= (2-4) 这里的i 指入射场在r '处的偏振态,也就是()i E r E i 00,='ω,r r n ''=/ ,g和g 0是表达式()()21+-εε在ω和ω0处的值,其中ε是胶体颗粒与周围物质的复合介电函数的比值。
表面增强拉曼光谱的基本原理和应用
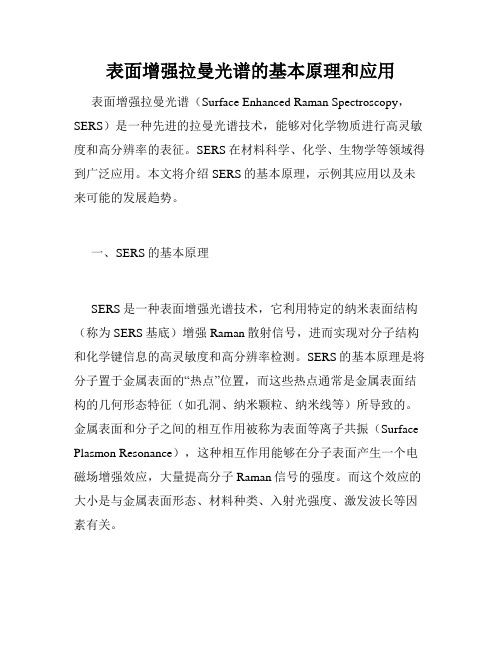
表面增强拉曼光谱的基本原理和应用表面增强拉曼光谱(Surface Enhanced Raman Spectroscopy,SERS)是一种先进的拉曼光谱技术,能够对化学物质进行高灵敏度和高分辨率的表征。
SERS在材料科学、化学、生物学等领域得到广泛应用。
本文将介绍SERS的基本原理,示例其应用以及未来可能的发展趋势。
一、SERS的基本原理SERS是一种表面增强光谱技术,它利用特定的纳米表面结构(称为SERS基底)增强Raman散射信号,进而实现对分子结构和化学键信息的高灵敏度和高分辨率检测。
SERS的基本原理是将分子置于金属表面的“热点”位置,而这些热点通常是金属表面结构的几何形态特征(如孔洞、纳米颗粒、纳米线等)所导致的。
金属表面和分子之间的相互作用被称为表面等离子共振(Surface Plasmon Resonance),这种相互作用能够在分子表面产生一个电磁场增强效应,大量提高分子Raman信号的强度。
而这个效应的大小是与金属表面形态、材料种类、入射光强度、激发波长等因素有关。
SERS的显著优势是能够检测微量分子,因此被广泛应用于化学物质检测。
例如,SERS可以检测到化学物质的痕量,包括药物、污染物和微生物等。
此外,SERS还可以对分子在空间和时间尺度上的行为进行分析,帮助科学家了解化学反应、催化机制等问题。
二、SERS的应用1. 化学物质检测SERS已被广泛应用于化学物质检测。
例如,SERS可以检测到二氧化碳、二硫化碳、氯仿、水中的有机物等化学物质。
由于SERS技术能够在极低浓度下检测到目标物质,因此非常适用于环境监测、食品检测和生物诊断等领域。
2. 生物医学检测SERS技术可以用于生物医学检测,例如检测癌症标志物、病原体和细胞等。
SERS还可以帮助科学家研究生物分子在细胞膜和基质中的相互作用。
SERS在肿瘤、心血管、神经学等领域的研究也有很大的发展空间。
3. 材料表征SERS技术也可以用于材料表征,例如检测材料中的缺陷、化学键、晶格结构等。
表面增强拉曼光谱综述

表面增强拉曼光谱综述表面增强拉曼光谱(Surface-Enhanced Raman Spectroscopy, SERS)是一种强大的分析技术,用于提高拉曼散射的灵敏度。
这种技术自1974年被发现以来,已经成为化学、物理、生物学和材料科学领域的重要工具。
以下是对SERS的一个综述:1. 基本原理●拉曼散射:基于分子振动能级变化的非弹性散射过程,可提供分子结构信息。
●表面增强机制:将样品放置在特殊的金属表面(通常是纳米结构的银或金)上,可以显著增强拉曼信号。
2. 增强机制●电磁机制:最主要的机制,涉及金属纳米结构上的局域表面等离子体共振(LSPR),导致拉曼散射信号的强烈增强。
●化学机制:与样品和金属表面间的化学作用有关,可能导致电子转移,影响拉曼散射的强度。
3. 材料和方法●金属纳米结构:银和金是最常用的材料,但也有使用铜、铂等其他金属。
●制备方法:包括化学还原法、电化学沉积、纳米刻蚀技术等。
4. 应用●化学分析:用于检测极低浓度的化学物质,包括环境污染物、食品添加剂、药物成分等。
●生物医学:在细胞成像、疾病诊断、生物标记物检测等方面的应用。
●材料科学:用于研究纳米材料、催化剂、能源材料等。
5. 发展趋势和挑战●灵敏度和选择性的提高:研究人员致力于提高SERS的灵敏度,以检测更低浓度的样品。
●标准化和可重复性:由于SERS受到许多因素的影响,实验结果的可重复性是一个挑战。
●新材料和新技术:包括二维材料、异质结构的探索等。
6. 未来展望SERS作为一种高度灵敏的分析技术,有望在环境监测、疾病早期诊断、新材料开发等领域发挥更大作用。
随着纳米技术和光谱学的不断发展,SERS技术的应用范围和效率都有望进一步提升。
表面增强拉曼光谱
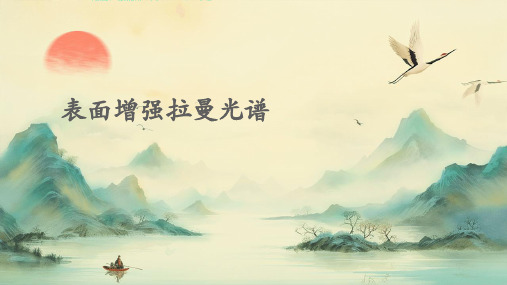
在生物分子检测中的应用
蛋白质结构分析
表面增强拉曼光谱可以用于蛋白质二级结构的分析,有助于理解 蛋白质的功能和生物学意义。
生物分子相互作用研究
通过观察生物分子间的拉曼光谱变化,可以研究生物分子间的相互 作用和识别,有助于发现新的药物靶点和生物标记物。
生物分子定量分析
表面增强拉曼光谱可以实现生物分子的高灵敏度检测和定量分析, 有助于疾病诊断和治疗监测。
表面增强拉曼光谱
• 介绍 • 表面增强拉曼光谱的实验方法 • 表面增强拉曼光谱在生物医学中的应
用 • 表面增强拉曼光谱在环境科学中的应
用 • 表面增强拉曼光谱的未来发展
01
介绍
什么是表面增强拉曼光谱?
表面增强拉曼光谱(Surface Enhanced Raman Spectroscopy,简称SERS)是一种先进的分子光谱技术,通 过在金属表面上的特定结构或粗糙表面上的金属纳米结构,实 现对拉曼散射的显著增强。
在环境科学领域,它可以用于 污染物和环境毒物的检测和分 析。
02
表面增强拉曼光谱的实验方法
实验设备与材料
01
02
03
拉曼光谱仪
用于检测拉曼散射信号, 通常配备有激光光源和光 谱检测系统。
表面增强剂
如金属纳米颗粒或金属薄 膜,用于增强拉曼散射信 号。
样品
需要进行表面增强拉曼光 谱测定的物质,可以是分 子、纳米材料或生物样品 等。
实验结果分析
信号增强效果评估
通过对比增强前后的拉曼光谱 信号强度,评估表面增强剂的
增强效果。
分子结构分析
根据拉曼光谱的特征峰位置和 峰形,分析待测样品的分子结 构。
分子相互作用研究
通过分析拉曼光谱的变化,研 究分子与表面增强剂之间的相 互作用。
表面增强拉曼光谱的原理与应用
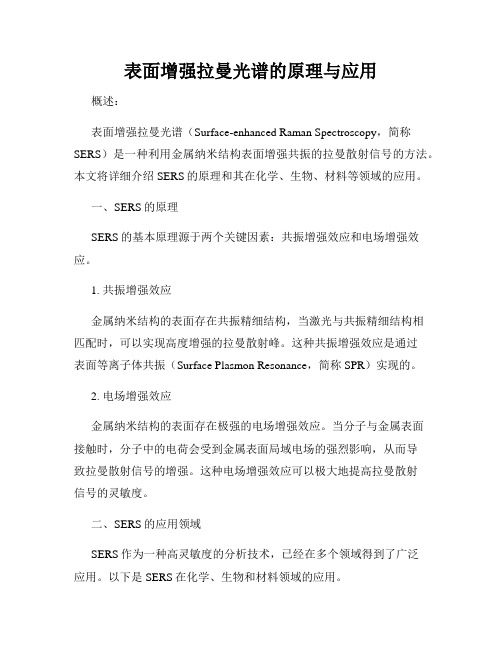
表面增强拉曼光谱的原理与应用概述:表面增强拉曼光谱(Surface-enhanced Raman Spectroscopy,简称SERS)是一种利用金属纳米结构表面增强共振的拉曼散射信号的方法。
本文将详细介绍SERS的原理和其在化学、生物、材料等领域的应用。
一、SERS的原理SERS的基本原理源于两个关键因素:共振增强效应和电场增强效应。
1. 共振增强效应金属纳米结构的表面存在共振精细结构,当激光与共振精细结构相匹配时,可以实现高度增强的拉曼散射峰。
这种共振增强效应是通过表面等离子体共振(Surface Plasmon Resonance,简称SPR)实现的。
2. 电场增强效应金属纳米结构的表面存在极强的电场增强效应。
当分子与金属表面接触时,分子中的电荷会受到金属表面局域电场的强烈影响,从而导致拉曼散射信号的增强。
这种电场增强效应可以极大地提高拉曼散射信号的灵敏度。
二、SERS的应用领域SERS作为一种高灵敏度的分析技术,已经在多个领域得到了广泛应用。
以下是SERS在化学、生物和材料领域的应用。
1. 化学领域SERS可以用于分子结构鉴定、化学反应动力学研究和分子吸附等方面。
通过SERS技术,可以获得很高的分子识别能力,从而在化学反应的机理研究中发挥重要作用。
2. 生物领域SERS广泛应用于生物分子的检测、生物传感和生物成像等方面。
由于SERS技术对生物分子的高灵敏度,可以用于检测低浓度的蛋白质、DNA和药物等生物分子,有助于生物医学研究和临床诊断。
3. 材料领域在材料科学领域,SERS可以用于表面增强光催化、纳米材料的表征和表面等离子体共振等方面的研究。
SERS技术不仅可以提供材料的化学组成信息,还可以揭示材料的结构和光学性质,对材料的表征提供了有力的手段。
三、SERS的发展前景与挑战虽然SERS在分析领域具有广泛的应用前景,但仍然面临着一些挑战。
首先,SERS在实际应用中需要制备高度可重复和稳定的金属纳米结构,这对技术的推广应用提出了要求。
- 1、下载文档前请自行甄别文档内容的完整性,平台不提供额外的编辑、内容补充、找答案等附加服务。
- 2、"仅部分预览"的文档,不可在线预览部分如存在完整性等问题,可反馈申请退款(可完整预览的文档不适用该条件!)。
- 3、如文档侵犯您的权益,请联系客服反馈,我们会尽快为您处理(人工客服工作时间:9:00-18:30)。
Surface-enhanced Raman spectroscopyFrom Wikipedia, the free encyclopedia(Redirected from Surface-enhanced Raman scattering)Raman spectrum of liquid 2-mercaptoethanol (below) and SERS spectrum of 2-mercaptoethanol monolayer formed on roughened silver (above). Spectra are scaled and shifted for clarity. A difference in selection rules is visible: Some bands appear only in the bulk-phase Raman spectrum or only in the SERS spectrum.Surface-enhanced Raman spectroscopy or surface-enhanced Raman scattering (SERS) is a surface-sensitive technique that enhances Ramanscattering by molecules adsorbed on rough metal surfaces or by nanostructures such as plasmonic-magnetic silica nanotubes.[1] The enhancement factor can be as much as 1010 to 1011,[2][3] which means the technique may detect single molecules.[4][5]Contents[hide]•1History•2Mechanismso 2.1Electromagnetic theoryo 2.2Chemical theory•3Surfaces•4Applicationso 4.1Oligonucleotide targeting•5Selection rules•6ReferencesHistory[edit]SERS from pyridine adsorbed on electrochemically roughened silver was first observed by Martin Fleischmann, Patrick J. Hendra and A. James McQuillan at the Department of Chemistry at the University of Southampton, Southampton, UK in 1973.[6] This initial publication has been cited over 4000 times. The 40th Anniversary of the first observation of the SERS effect has been marked by the Royal Society of Chemistry by the award of a National Chemical Landmark plaque to the University of Southampton. In 1977, two groups independently noted that the concentration of scattering species could not account for the enhanced signal and each proposed a mechanism for the observed enhancement. Their theories are still accepted as explaining the SERS effect. Jeanmaire and Van Duyne[7] proposed an electromagnetic effect, while Albrecht and Creighton[8] proposed a charge-transfer effect. Rufus Ritchie, of Oak Ridge National Laboratory's Health Sciences Research Division, predicted the existence of the surface plasmon.[9]Mechanisms[edit]The exact mechanism of the enhancement effect of SERS is still a matter of debate in the literature. There are two primary theories and while their mechanisms differ substantially, distinguishing them experimentally has not been straightforward.The electromagnetic theory proposes the excitation of localized surface plasmons, while the chemical theory proposes the formation of charge-transfer complexes. The chemical theory applies only for species that have formed a chemical bond with the surface, so it cannot explain the observed signal enhancement in all cases, whereas the electromagnetic theory can apply even in those cases where the specimenis physisorbed only to the surface. It has been shown recently that SERS enhancement can occur even when an excited molecule is relatively far apart from the surface which hosts metallic nanoparticles enabling surface plasmon phenomena.[10] This observation provides a strong support for the electromagnetic theory of SERS. Research in 2015 on a more powerful extension of the SERS technique called SLIPSERS (SlipperyLiquid-Infused Porous SERS)[11] has further supported the EM theory.[12] Electromagnetic theory[edit]The increase in intensity of the Raman signal for adsorbates on particular surfaces occurs because of an enhancement in the electric field provided by the surface. When the incident light in the experiment strikes the surface, localized surface plasmons are excited. The field enhancement is greatest when the plasmon frequency, ωp, is in resonance with the radiation. In order for scattering to occur, the plasmon oscillations must be perpendicular to the surface; if they are in-plane with the surface, no scattering will occur. It is because of this requirement that roughened surfaces or arrangementsof nanoparticles are typically employed in SERS experiments as these surfaces provide an area on which these localized collective oscillations can occur.[13]The light incident on the surface can excite a variety of phenomena in the surface, yet the complexity of this situation can be minimized by surfaces with features much smaller thanthe wavelength of the light, as only the dipolar contribution will be recognized by the system. The dipolar term contributes to the plasmon oscillations, which leads to the enhancement. The SERS effect is so pronounced because the field enhancement occurs twice. First, the field enhancement magnifies the intensity of incident light, which will excite the Raman modes of the molecule being studied, therefore increasing the signal of the Raman scattering. The Raman signal is then further magnified by the surface due to the same mechanism that excited the incident light, resulting in a greater increase in the total output. At each stage the electric field is enhanced as E2, for a total enhancement of E4.[14]The enhancement is not equal for all frequencies. For those frequencies for which the Raman signal is only slightly shifted from the incident light, both the incident laser light and the Raman signal can be near resonance with the plasmon frequency, leading to the E4 enhancement. When the frequency shift is large, the incident light and the Raman signal cannot both be on resonance with ωp, thus the enhancement at both stages cannot be maximal.[15]The choice of surface metal is also dictated by the plasmon resonancefrequency. Visible and near-infrared radiation (NIR) are used to excite Ramanmodes. Silver and gold are typical metals for SERS experiments because their plasmon resonance frequencies fall within these wavelength ranges, providing maximal enhancement for visible and NIR light. Copper's absorption spectrum also falls within the range acceptable for SERS experiments.[16] Platinum and palladium nanostructures also display plasmon resonance within visible and NIR frequencies.[17]Chemical theory[edit]While the electromagnetic theory of enhancement can be applied regardless of the molecule being studied, it does not fully explain the magnitude of the enhancement observed in many systems. For many molecules, often those with a lone pair of electrons, in which the molecules can bond to the surface, a different enhancement mechanism that does not involve surface plasmons has been described. This chemical mechanism involves charge transfer between the chemisorbed species and the metal surface. The chemical mechanism only applies in specific cases and probably occurs in concert with the electromagnetic mechanism.[18][19]The HOMO to LUMO transition for many molecules requires much more energy than the infrared or visible light typically involved in Raman experiments. When the HOMO and LUMO of the adsorbate fall symmetrically about the Fermi level of the metal surface, light of half the energy can be employed to make the transition, where the metal acts as a charge-transfer intermediate.[18] Thus a spectroscopic transition that might normally take place in the UV can be excited by visible light.[15]Surfaces[edit]While SERS can be performed in colloidal solutions, today the most common method for performing SERS measurements is by depositing a liquid sample onto a silicon or glasssurface with a nanostructured noble metal surface. While the first experiments were performed on electrochemically roughened silver,[20] now surfaces are often prepared using a distribution of metal nanoparticles on the surface[21] as well as usinglithography[22] or porous silicon as a support.[23][24]Applying a thin film of silver onto wafers of silicon, through a day-long immersion in a saturated solution of silver nitrate in n-octanol, is a widely accepted method to prepare a surface-enhanced Raman scattering substrate.[25] The most common metals used for plasmonic surfaces are silver and gold, however aluminum has recently been explored as an alternative plasmonic material, because its plasmon band is in the UV region, contrary to silver and gold.[26] Hence, there is great interest in using aluminum for UV SERS. It has, however, surprisingly also been shown to have a large enhancement in the infrared, which is not fully understood.[27] In the current decade, it has been recognized that the cost of SERS substrates must be reduced in order to become a commonly used analytical chemistry measurement technique.[28] To meet this need, plasmonic paper has experienced widespread attention in the field, with highly sensitive SERS substrates being formed through approaches such assoaking,[29][30][31] in-situ synthesis,[32][33] screen printing[34] and inkjet printing.[35][36][37]The shape and size of the metal nanoparticles strongly affect the strength of the enhancement because these factors influence the ratio of absorption and scattering events.[38][39] There is an ideal size for these particles, and an ideal surface thickness for each experiment.[40] Particles that are too large allow the excitation of multipoles, which are nonradiative. As only the dipole transition leads to Raman scattering, the higher-order transitions will cause a decrease in the overall efficiency of the enhancement. Particles that are too small lose their electrical conductance and cannot enhance the field. When the particle size approaches a few atoms, the definition of a plasmon does not hold, as there must be a large collection of electrons to oscillate together.[14] An ideal SERS substrate must possess high uniformity and high field enhancement. Such substrates can be fabricated on a wafer scale and label-free superresolution microscopy has also been demonstrated using the fluctuations of surface enhanced Raman scattering signal on such highly uniform, high-performance plasmonic metasurfaces. [41]Applications[edit]SERS substrates prepared with silver nano rods are used to detect the presence of low abundance biomolecules, and can therefore detect proteins in body fluids.[42][43][44] This technology has been utilized to detect urea and blood plasma label free in human serum and may become the next generation in cancer detection and screening.[43][44] The ability to analyze the composition of a mixture on the nano scale makes the use of SERS substrates beneficial for environmental analysis, pharmaceuticals, material sciences, art and archeological research, forensic science, drug and explosives detection, food quality analysis, and single algal cell detection.[45][46][47] SERS combined with plasmonic sensing can be used for high-sensitivity and quantitative detection of biomolecular interaction.[48]Oligonucleotide targeting[edit]SERS can be used to target specific DNA and RNA sequences using a combination of gold and silver nanoparticles and Raman-active dyes, such as Cy3. Specific single nucleotide polymorphisms (SNP) can be identified using this technique. The gold nanoparticles facilitate the formation of a silver coating on the dye-labeled regions of DNA or RNA, allowing SERS to be performed. This has several potential applications: For example, Cao et al. report that gene sequences for HIV, Ebola, Hepatitis, and Bacillus Anthracis can be uniquely identified using this technique. Each spectrum was specific, which is advantageous over fluorescence detection; some fluorescent markers overlap and interfere with other gene markers. The advantage of this technique to identify gene sequences is that several Raman dyes are commercially available, which could lead to the development of non-overlapping probes for gene detection.[49]Selection rules[edit]The term surface enhanced Raman spectroscopy implies that it provides the same information that traditional Raman spectroscopy does, simply with a greatly enhanced signal. While the spectra of most SERS experiments are similar to the non-surface enhanced spectra, there are often differences in the number of modes present. Additional modes not found in the traditional Raman spectrum can be present in the SERS spectrum, while other modes can disappear. The modes observed in any spectroscopic experiment are dictated by the symmetry of the molecules and are usually summarized by selection rules. When molecules are adsorbed to a surface, the symmetry of the system can change, slightly modifying the symmetry of the molecule, which can lead to differences in mode selection.[50]One common way in which selection rules are modified arises from the fact that many molecules that have a center of symmetry lose that feature when adsorbed to a surface. The loss of a center of symmetry eliminates the requirements of the mutual exclusion rule, which dictates that modes can only be either Raman or Infrared active. Thus modes that would normally appear only in the infrared spectrum of the free molecule can appear in the SERS spectrum.[13]A molecule's symmetry can be changed in different ways depending on the orientation in which the molecule is attached to the surface. In some experiments, it is possible to determine the orientation of adsorption to the surface from the SERS spectrum, as different modes will be present depending on how the symmetry is modified.[51]References[edit]1. Jump up^ Xu, X., Li, H., Hasan, D., Ruoff, R. S., Wang, A. X. and Fan, D. L. (2013), Near-Field EnhancedPlasmonic-Magnetic Bifunctional Nanotubes for Single Cell Bioanalysis. Adv. Funct.Mater.. doi:10.1002/adfm.2012038222. Jump up^Blackie, Evan J.; Le Ru, Eric C.; Etchegoin, Pablo G. (2009). "Single-Molecule Surface-EnhancedRaman Spectroscopy of Nonresonant Molecules". J. Am. Chem. Soc.131 (40):14466–14472. doi:10.1021/ja905319w.PMID19807188.3. Jump up^Blackie, Evan J.; Le Ru, Eric C.; Meyer, Matthias; Etchegoin, Pablo G. (2007). "Surface EnhancedRaman Scattering Enhancement Factors: A Comprehensive Study". J. Phys. Chem. C. 111 (37):13794–13803.doi:10.1021/jp0687908.4. Jump up^Nie, S; Emory, SR (1997). "Probing Single Molecules and Single Nanoparticles by Surface-EnhancedRaman Scattering". Science. 275 (5303): 1102–6.doi:10.1126/science.275.5303.1102. PMID9027306.5. Jump up^Le Ru, Eric C.; Meyer, Matthias; Etchegoin, Pablo G. (2006). "Proof of Single-Molecule Sensitivity inSurface Enhanced Raman Scattering (SERS) by Means of a Two-Analyte Technique". J. Phys. Chem. B. 110 (4): 1944–1948.doi:10.1021/jp054732v. PMID16471765.6. Jump up^Fleischmann, M.; PJ Hendra & AJ McQuillan (15 May 1974). "Raman Spectra of Pyridine Adsorbed ata Silver Electrode". Chemical Physics Letters. 26 (2):163–166.Bibcode:1974CPL....26..163F. doi:10.1016/0009-2614(74)85388-1.7. Jump up^Jeanmaire, David L.; Richard P. van Duyne (1977). "Surface Raman Electrochemistry Part I.Heterocyclic, Aromatic and Aliphatic Amines Adsorbed on the Anodized Silver Electrode". Journal ofElectroanalytical Chemistry.84: 1–20. doi:10.1016/S0022-0728(77)80224-6.8. Jump up^Albrecht, M. Grant; J. Alan Creighton (1977). "Anomalously Intense Raman Spectra of Pyridine at aSilver Electrode". Journal of the American Chemical Society. 99(15): 5215–5217. doi:10.1021/ja00457a071.9. Jump up^"Technical Highlights. New Probe Detects Trace Pollutants in Groundwater". Oak Ridge NationalLaboratory Review. 26 (2).10. Jump up^Kukushkin, V. I.; Van’kov, A. B.; Kukushkin, I. V. (2013). "Long-range manifestation ofsurface-enhanced Raman scattering". JETP Letters. 98 (2):64–69.doi:10.1134/S0021364013150113. ISSN0021-3640.11. Jump up^/content/early/2015/12/29/151898011312. Jumpup^/single-molecule-detection-of-contaminants-explosives-or-diseases-now-possible 13. ^ Jump up to:a b Smith, E.; Dent, G., Modern Raman Spectroscopy: A Practical Approach. John Wiley and Sons:2005 ISBN 0-471-49794-014. ^ Jump up to:a b Moskovits, M., Surface-Enhanced Raman Spectroscopy: a Brief Perspective. InSurface-Enhanced Raman Scattering – Physics and Applications, 2006; pp. 1–18ISBN 3-540-33566-815. ^ Jump up to:a b Campion, Alan; Kambhampati, Patanjali (1998). "Surface-enhanced Raman scattering". ChemicalSociety Reviews. 27 (4): 241. doi:10.1039/A827241Z.16. Jump up^Creighton, J. Alan; Eadon, Desmond G. (1991). "Ultraviolet?visible absorption spectra of the colloidalmetallic elements". Journal of the Chemical Society, Faraday Transactions. 87 (24):3881.doi:10.1039/FT9918703881.17. Jump up^Langhammer, Christoph; Yuan, Zhe; Zorić, Igor; Kasemo, Bengt (2006). "Plasmonic Properties ofSupported Pt and Pd Nanostructures". Nano Letters. 6 (4):833–838.Bibcode:2006NanoL...6..833L. doi:10.1021/nl060219x.PMID16608293.18. ^ Jump up to:a b Lombardi, John R.; Birke, Ronald L.; Lu, Tianhong; Xu, Jia (1986). "Charge-transfer theory ofsurface enhanced Raman spectroscopy: Herzberg–Teller contributions". The Journal of Chemical Physics. 84 (8): 4174. Bibcode:1986JChPh..84.4174L.doi:10.1063/1.450037.19. Jump up^Lombardi, J.R.; Birke, R.L. (2008). "A Unified Approach to Surface-Enhanced RamanSpectroscopy". Journal of Physical Chemistry C. 112 (14): 5605–5617.doi:10.1021/jp800167v.20. Jump up^Fleischmann, M.; Hendra, P.J.; McQuillan, A.J. (1974). "Raman spectra of pyridine adsorbed at a silverelectrode". Chemical Physics Letters. 26 (2):163–166.Bibcode:1974CPL....26..163F. doi:10.1016/0009-2614(74)85388-1.21. Jump up^Mock, J. J.; Barbic, M.; Smith, D. R.; Schultz, D. A.; Schultz, S. (2002). "Shape effects in plasmonresonance of individual colloidal silver nanoparticles". The Journal of Chemical Physics. 116 (15):6755.Bibcode:2002JChPh.116.6755M. doi:10.1063/1.1462610.22. Jump up^Witlicki, Edward H.; et al. (2011). "Molecular Logic Gates Using Surface-Enhanced Raman-ScatteredLight". J. Am. Chem. Soc.133 (19): 7288–7291.doi:10.1021/ja200992x.23. Jump up^Lin, Haohao; Mock, Jack; Smith, David; Gao, Ting; Sailor, Michael J. (August 2004)."Surface-Enhanced Raman Scattering from Silver-Plated Porous Silicon". The Journal of Physical ChemistryB. 108 (31): 11654–11659.doi:10.1021/jp049008b.24. Jump up^Talian, Ivan; Mogensen, Klaus Bo; Oriňák, Andrej; Kaniansky, Dušan; Hübner, Jörg (August 2009)."Surface-enhanced Raman spectroscopy on novel black silicon-based nanostructured surfaces". Journal ofRaman Spectroscopy.40 (8): 982–986. doi:10.1002/jrs.2213.25. Jump up^Shrestha, LK; Wi JS; Williams J; Akada M; Ariga K (March 2014). "Facile fabrication of silvernanoclusters as promising surface-enhanced Raman scattering substrates".Journal of Nanoscience andNanotechnology. 14 (3): 2245–51. doi:10.1166/jnn.2014.8538. PMID24745219.26. Jump up^Dörfer, Thomas; Schmitt, Michael; Popp, Jürgen (November 2007). "Deep-UV surface-enhancedRaman scattering".Journal of Raman Spectroscopy. 38 (11): 1379–1382.doi:10.1002/jrs.1831.27. Jump up^Mogensen, Klaus Bo; Gühlke, Marina; Kneipp, Janina; Kadkhodazadeh, Shima; Wagner, Jakob B.;Espina Palanco, Marta; Kneipp, Harald; Kneipp, Katrin (2014). "Surface-enhanced Raman scattering on aluminum using near infrared and visible excitation". Chemical Communications. 50 (28): 3744. doi:10.1039/c4cc00010b. 28. Jump up^Hoppmann, Eric P.; Yu, Wei W.; White, Ian M. (2014)."Inkjet-Printed Fluidic Paper Devices forChemical and Biological Analytics Using Surface Enhanced Raman spectroscopy"(PDF). IEEE. IEEE. 20 (3): 195–204.doi:10.1109/jstqe.2013.2286076.29. Jump up^Lee, Chang H.; Tian, Limei; Singamaneni, Srikanth (2010). "Paper-Based SERS". ACS. AmericanChemical Society. 2 (12): 3429–3435. doi:10.1021/am1009875. Retrieved 2015-01-16.30. Jump up^Ngo, Ying Hui; Li, Dan; Simon, George P.; Garnier, Gil (2012). "Gold Nanoparticle". Langmuir.American Chemical Society. 28 (23): 8782–8790. doi:10.1021/la3012734. Retrieved 2015-01-16.31. Jump up^Ngo, Ying Hui; Li, Dan; Simon, George P.; Garnier, Gil (2013). "Effect of cationic polyacrylamides onthe aggregation and SERS". Journal of Colloid and Interface Science. Elsevier. 392:237–246.doi:10.1016/j.jcis.2012.09.080.32. Jump up^Laserna, J. J.; Campiglia, A. D.; Winefordner, J. D. (1989). "Mixture analysis and quantitativedetermination of nitrogen-containing organic molecules by surface-enhanced Raman spectrometry". Anal. Chem.American Chemical Society. 61 (15): 1697–1701.doi:10.1021/ac00190a022.33. Jump up^Chang, Yung; Yandi, Wetra; Chen, Wen-Yih; Shih, Yu-Ju; Yang, Chang-Chung; Chang, Yu; Ling,Qing-Dong; Higuchi, Akon (2010). "Tunable Bioadhesive Copolymer Hydrogels of Thermoresponsive Poly( N -isopropyl acrylamide) Containing Zwitterionic Polysulfobetaine". Biomacromolecules. American ChemicalSociety. 11 (4): 1101–1110.doi:10.1021/bm100093g.34. Jump up^Qu, Lu-Lu; Li, Da-Wei; Xue, Jin-Qun; Zhai, Wen-Lei; Fossey, John S.; Long, Yi-Tao(2012-02-07). "Batch fabrication of disposable screen printed SERS arrays".Lab Chip. 12 (5):876–881. doi:10.1039/C2LC20926H.ISSN1473-0189.35. Jump up^Yu, Wei W.; White, Ian M. (2013). "Inkjet-printed paper-based SERS". Analyst. Royal Society ofChemistry. 138(4): 1020. doi:10.1039/c2an36116g.36. Jump up^Hoppmann, Eric P.; Yu, Wei W.; White, Ian M. (2013)."Highly sensitive and flexible inkjet printedSERS"(PDF). Methods. Elsevier. 63 (3): 219–224.doi:10.1016/j.ymeth.2013.07.010.37. Jump up^Fierro-Mercado, Pedro M.; Hern, Samuel P. (2012)."Highly Sensitive Filter Paper Substrate forSERS".International Journal of Spectroscopy. Hindawi Publishing Corporation. 2012:1–7. doi:10.1155/2012/716527.38. Jump up^H. Lu; Zhang, Haixi; Yu, Xia; Zeng, Shuwen; Yong, Ken-Tye; Ho, Ho-Pui (2011). "Seed-mediatedPlasmon-driven Regrowth of Silver Nanodecahedrons (NDs)"(PDF).Plasmonics. 7 (1):167–173. doi:10.1007/s11468-011-9290-8.39. Jump up^ Aroca, R., Surface-enhanced Vibrational Spectroscopy. John Wiley & Sons (2006) ISBN0-471-60731-240. Jump up^Bao, Li-Li; Mahurin, Shannon M.; Liang, Cheng-Du; Dai, Sheng (2003). "Study of silver films over silicabeads as a surface-enhanced Raman scattering (SERS) substrate for detection of benzoic acid". Journal of Raman Spectroscopy.34 (5): 394–398. Bibcode:2003JRSp...34..394B.doi:10.1002/jrs.993.41. Jump up^Ayas, S. (2013). "Label-Free Nanometer-Resolution Imaging of Biological Architectures throughSurface Enhanced Raman Scattering". Scientific Reports. 3: 2624.doi:10.1038/srep02624.doi:10.1038/srep02624 42. Jump up^Yang, J; et al. (May 2013). "Surface-Enhanced Raman Spectroscopy Based Quantitative Bioassay onAptamer-Functionalized Nanopillars Using Large-Area Raman Mapping"(PDF). ACS Nano. 7 (6):5350–5359.doi:10.1021/nn401199k.43. ^ Jump up to:a b Han, YA; Ju J; Yoon Y; Kim SM (May 2014)."Fabrication of cost-effective surface enhancedRaman spectroscopy substrate using glancing angle deposition for the detection of urea in body fluid". Journal of Nanoscience and Nanotechnology. 14 (5): 3797–9.doi:10.1166/jnn.2014.8184. PMID24734638.44. ^ Jump up to:a b Li, D; Feng S; Huang H; Chen W; Shi H; Liu N; Chen L; Chen W; Yu Y; Chen R (March2014). "Label-free detection of blood plasma using silver nanoparticle based surface-enhanced Ramanspectroscopy for esophageal cancer screening". Journal of Nanoscience and Nanotechnology.10 (3):478–84. doi:10.1166/jbn.2014.1750.PMID24730243.45. Jump up^Deng, Y; Juang Y (March 2014). "Black silicon SERS substrate: Effect of surface morphology on SERSdetection and application of single algal cell analysis".Biosensors and Bioelectronics. 53:37–42.doi:10.1016/j.bios.2013.09.032.46. Jump up^Hoppmann, Eric; et al. (2013). Trace detection overcoming the cost and usability limitations oftraditional SERS technology(PDF) (Technical report). Diagnostic anSERS.line feed characterin |title= at position 50 (help)47. Jump up^Wackerbarth H; Salb C; Gundrum L; Niederkrüger M; Christou K; Beushausen V; Viöl W(2010). "Detection of explosives based on surface-enhanced Raman spectroscopy". Applied Optics. 49 (23): 4362–4366.doi:10.1364/AO.49.004362.48. Jump up^Xu, Zhida; Jiang, Jing; Wang, Xinhao; Han, Kevin; Ameen, Abid; Khan, Ibrahim; Chang, Te-Wei; Liu,Logan (2016)."Large-area, uniform and low-cost dual-mode plasmonic naked-eye colorimetry and SERS sensor with handheld Raman spectrometer". Nanoscale. 8: 6162–6172.doi:10.1039/C5NR08357E.49. Jump up^Cao, Y. C.; Jin, R; Mirkin, CA (2002). "Nanoparticles with Raman Spectroscopic Fingerprints for DNAand RNA Detection". Science. 297 (5586):1536–1540.Bibcode:2002Sci...297.1536C.doi:10.1126/science.297.5586.1536. PMID12202825.50. Jump up^Moskovits, M.; Suh, J. S. (1984). "Surface selection rules for surface-enhanced Raman spectroscopy:calculations and application to the surface-enhanced Raman spectrum of phthalazine on silver". The Journal of Physical Chemistry. 88 (23): 5526–5530.doi:10.1021/j150667a013.51. Jump up^Brolo, A.G.; Jiang, Z.; Irish, D.E. (2003). "The orientation of 2,2′-bipyridine adsorbed at a SERS-activeAu(111) electrode surface"(PDF). Journal of Electroanalytical Chemistry. 547 (2):163–172.doi:10.1016/S0022-0728(03)00215-8.。