双金属氢氧化物
铁系层状双金属氢氧化物非均相类芬顿反应及其机理研究

铁系层状双金属氢氧化物非均相类芬顿反应及其机理研究随着现代工农业飞速发展,水体污染越来越严重,其中尤以水中有机物的污染最为严重。
在水中有机污染物的处理方法中,芬顿技术以处理效率高、反应迅速快的特点被广泛应用,因为均相芬顿必须在酸性的反应条件下才能达到较好的处理效果,非均相芬顿法以其较宽的p H值范围逐渐被重视,在水处理方面得到了广泛的应用。
层状双金属氢氧化物(Layered double hydroxides,简称LDHs)是一种带有永久正电荷的具有水滑石层状晶体结构的功能材料。
由于其独特的性质,层状结构以及层间阴离子的可交换性,在材料、化工、工业催化、医药和环境科学等领域具有潜在的应用价值。
本研究以含铁LDH(包括Fe(Ⅱ)/Fe(Ⅲ)-LDH和Mg/Fe-LDH)的非均相芬顿反应为主要研究背景,以有机染料甲基橙为目标对象,通过合成含有不同层间阴离子以及不同铁元素的LDH,利用结构中的铁与双氧水发生芬顿反应对甲基橙进行氧化降解,通过调节材料结构中铁的比例和种类以及层间阴离子的种类,探讨LDH中层间阴离子以及铁元素在非均相芬顿反应中的作用,并考察层间阴离子对LDH结构的影响。
本文得的主要研究结果和结论如下所示:(1)通过共沉淀法在常温下合成十二烷基苯磺酸根(DBS-)为层间阴离子的Fe(Ⅱ)/Fe(Ⅲ)-LDH,即有机绿锈(DBS-GR),并通过XRD,FT-IR,SEM等方法对其进行结构表征,表明成功通过此种简单快速的方法合成了DBS-GR,其中有机阴离子的以单分子层平行倾斜的方式排列在金属层板间。
有机绿锈在第一次芬顿降解反应中对甲基橙的去除效果都很好,速率很快,反应15 min去除率达到96%。
去除原理为有机绿锈在降解甲基橙的过程中产生羟基自由基,进攻甲基橙分子的偶氮键,使其断裂分解,羟基自由基进一步分解小分子有机物,直到完全氧化成CO2和H2O分子。
有机绿锈非均相芬顿体系能将芬顿反应的初始p H值扩展到中性左右进行反应,能达到和酸性条件一样的结果。
层状双金属氢氧化物

包装工程第44卷第19期·104·PACKAGING ENGINEERING2023年10月层状双金属氢氧化物/聚乙烯醇气体阻隔薄膜材料制备及性能研究张子怡,李梦冉,薛程,范婷婷,李欢欢,李中波*(安徽农业大学轻纺工程与艺术学院,安徽合肥230036)摘要:目的研发出一种具有优异氧气阻隔性能的柔性薄膜,其在食品包装领域具有良好的应用前景。
方法以具有生物降解性能的聚乙烯醇(PVA)为成膜基材,镁铝层状双金属氢氧化物(MgAl-LDH)为改性剂,柠檬酸为交联剂,采用流延法制备出具有优异气体阻隔性能的PVA/MgAl-LDH复合薄膜。
结果随着柠檬酸的含量的增加,复合薄膜的亲水性能逐渐增加,阻隔性能逐渐下降;随着复合薄膜中MgAl-LDH的含量的增加,复合薄膜的疏水性能和阻隔性能逐渐提高。
当复合薄膜中MgAl-LDH的质量分数为1.5%时,薄膜的力学性能最好,抗拉强度为42 MPa,断裂伸长率为16.7%,此MgAl-LDH质量分数下薄膜的气体阻隔性能也最优异,气体透过量为16 mL/(m2·24 h·0.1 MPa)。
结论柠檬酸的引入增加了薄膜内部亲水基团的数量,提升了复合薄膜的亲水性能。
MgAl-LDH可以减少PVA薄膜内部自由体积,提升PVA薄膜的力学性能和阻隔性能。
关键词:聚乙烯醇;镁铝层状双金属氢氧化物;柠檬酸;复合薄膜;阻隔性能中图分类号:TS206.4 文献标识码:A 文章编号:1001-3563(2023)19-0104-08DOI:10.19554/ki.1001-3563.2023.19.014Preparation and Properties of PVA/MgAl-LDH Gas Barrier FilmsZHANG Zi-yi, LI Meng-ran, XUE Cheng, FAN Ting-ting, LI Huan-huan, LI Zhong-bo*(College of Light Textile Engineering and Art, Anhui Agricultural University, Hefei 230036, China)ABSTRACT: The work aims to develop a flexible film with excellent oxygen barrier performance and good application prospects in the field of food packaging. The PVA/MgAl-LDH composite film with excellent gas barrier performance was prepared by the casting method with biodegradable polyvinyl alcohol (PVA) as the film-forming substrate, mag-nesium-aluminum layered bimetallic hydroxide (MgAl-LDH) as the modifier, and citric acid as the cross-linking agent.The experimental results showed that with the increase of citric acid content, the hydrophilic property of the composite film gradually increased and the barrier performance decreased gradually. With the increase of MgAl-LDH content in the composite film, the hydrophobic property and barrier property of the composite film gradually increased. When the content of MgAl-LDH in the composite film was 1.5%, the mechanical properties of the film were the best, with a ten-sile strength of 42 MPa and an elongation at break of 16.7%. The gas barrier performance of the film with this MgAl-LDH content was also the best, with a gas permeability of 16 mL/(m2·24 h·0.1 MPa). In addition, the introduc-tion of citric acid increases the number of hydrophilic groups inside the film, and the hydrophilic properties of the composite film are enhanced. MgAl-LDH can reduce the free volume inside the PVA film and enhance the mechanical收稿日期:2023-04-27基金项目:安徽省教育厅自然科学重点项目(2022AH050875);安徽省科技重大专项(202103a06020005);安徽省大学生创新创业项目(S202120364214)第44卷第19期张子怡,等:层状双金属氢氧化物/聚乙烯醇气体阻隔薄膜材料制备及性能研究·105·and barrier properties of the PVA film.KEY WORDS: polyvinyl alcohol; magnesium-aluminum layered bimetallic hydroxide; citric acid; composite film; bar-rier performance阻隔性薄膜指对气体、有机化合物等低分子量的化学物质具有非常低的透过性的薄膜。
潜力无限的层状双金属氢氧化物电极材料
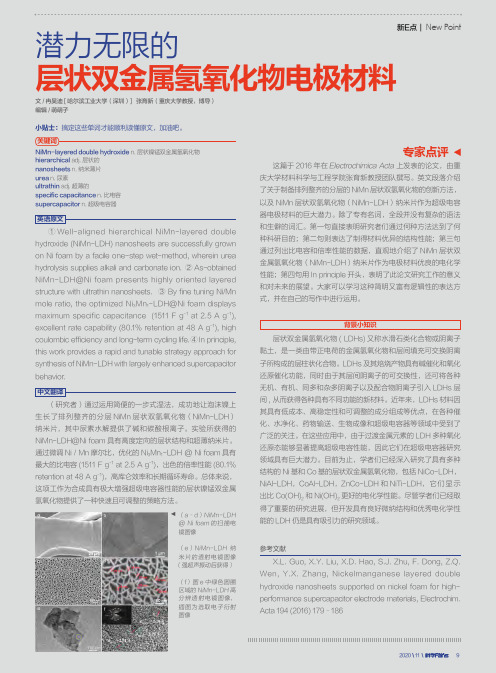
(研究者)通过运用简便的一步式湿法,成功地让泡沫镍上生长了排列整齐的分层NiMn层状双氢氧化物(NiMn-LDH)纳米片,其中尿素水解提供了碱和碳酸根离子。
实验所获得的NiMn-LDH@Ni foam具有高度定向的层状结构和超薄纳米片。
通过微调Ni / Mn摩尔比,优化的Ni3Mn1-LDH @ Ni foam具有最大的比电容(1511 F g-1 at 2.5 A g-1),出色的倍率性能(80.1%retention at 48 A g-1),高库仑效率和长期循环寿命。
总体来说,这项工作为合成具有极大增强超级电容器性能的层状镍锰双金属氢氧化物提供了一种快速且可调整的策略方法。
X.L. Guo, X.Y. Liu, X.D. Hao, S.J. Zhu, F. Dong, Z.Q.Wen, Y.X. Zhang, Nickelmanganese layered doublehydroxide nanosheets supported on nickel foam for high-performance supercapacitor electrode materials, Electrochim.Acta 194 (2016) 179–186①Well-aligned hierarchical NiMn-layered doublehydroxide (NiMn-LDH) nanosheets are successfully grownon Ni foam by a facile one-step wet-method, wherein ureahydrolysis supplies alkali and carbonate ion.②As-obtainedNiMn-LDH@Ni foam presents highly oriented layeredstructure with ultrathin nanosheets. ③By fine tuning Ni/Mnmole ratio, the optimized Ni3Mn1-LDH@Ni foam displaysmaximum specific capacitance (1511 F g-1 at 2.5 A g-1),excellent rate capability (80.1% retention at 48 A g-1), highcoulombic efficiency and long-term cycling life. ④In principle,this work provides a rapid and tunable strategy approach forsynthesis of NiMn-LDH with largely enhanced supercapacitorbehavior.这篇于2016年在Electrochimica Acta上发表的论文,由重庆大学材料科学与工程学院张育新教授团队撰写。
层状双氢氧化物的制备性质及应用
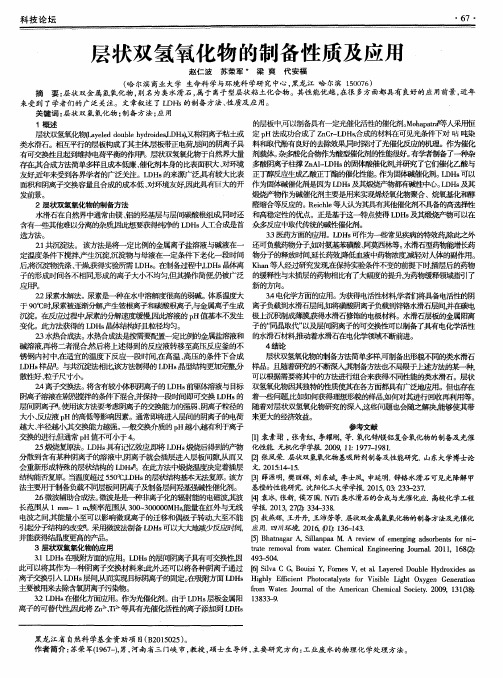
・ 6 7・
层状双氢氧化物 的制备性质及应用
赵仁波 苏荣军 梁 爽 代安福 ( 哈 尔滨 商业 大学 生命科 学与环境科学研 究 中心 , 黑龙江 哈 尔滨 1 5 0 0 7 6 ) 摘 要: 层状双金属 氢氧化物 , 别名为类水滑石 , 属 于离子型层状粘土化合物 。其性能优越 , 在很 多方面都 具有 良好的应用前景 , 近年 来受到 了学者们的广泛关注。文章叙述 了 L DH s 的制备 方法、 性质及应 用。 关键词 : 层 状 双 氢氧 化 物 ; 制备方法 ; 应用 的层板中’ 可以制各具有— 性的催 l 。 Mo h a p a t r 等^ 采用恒 1概 述 层状双氢氧化! I . a y e l e d d o u b l e h y d mi d e D Hs ) 叉称阴离子粘土或 定 p H法成功合成了z n c 卜L D H s , 合成的材料在可见光条件下对 咕 吨染 类水滑石。相互平行的层扳构成了其主体. 层板带正电荷层 问的阴离子具 料和取代酚有良好的去除效果 同时探讨了光催化反应的机理。作为催化 有可交换性目起到维持电荷平衡的作用 层状双氢氧化物于 自 然界大量 剂载体。 杂多 酸化合 物作为 酸型催 J 的性能很 好。 有学者制备了 —种杂 存在萁合成方法简单多样 目成本低廉、 催化剂本身的比表面积大、 对环境 多酸阴离子柱撑 Z n A 1 一 L D H s 的固体酸催化剂拼研 究了它们催化乙酸与 友好近年来受到各界学者的广泛关注。L D H s 因为 L D Hs 及其煅烷严 睹 责 性中心。 L D H s 2  ̄ 面积和阴离子重换 容量且合成 的成本低、 对环境友好周 I 比 _ 恩有巨大的开 作为固体确 煅烧产物作为碱催化剂主要是用来实现烯陉氧f { 二 物聚合、烷氧基化和醇 发前景。 2层状双氢氧化物的制备方法 醛缩合等 反应的。 R e i c h l e 等^ 认为其具有其他催化剂不具备的高选择性 水滑石在自然界中通常由镁、 铝的羟基层与层间碳酸根组成 同时还 和高稳定 l 生的优 。正是基于这—特 点使得 L D H s 及其煅烧产物可以在 含有— 以分离的杂烦 因此想要获得纯 争的 L D H s人工合成是首 玲雪坂 应 中取代传统的碱 陛催仪剂 。 选方法 。 3 3医药方面的应用。L D H s 可作为—些常见疾病的特效药, 除此之外 2 1 共沉淀法。该方法是将一定比例的金属离子盐溶液与碱液在一 还可负载药物分子加对氨基苯磺酸、 阿莫西林等。 水滑石型药物自 增长药 定温度条件下搅拌产 生沉淀抗 淀物与母液在一定条件下老化一段时间 物分子的释放时间, 延长药效, 降低血液中药物浓度, 减轻对 ^ 体的副作用。 后 磺物洗涤、 干燥荔得实验所需 L D H s 。在制备过程中, L D H s 晶体离 K h a n 等^ 经过研究发现在保持实验条件不变的前提下时插 层后的药物 子的形成时间各不相同形成的离子大小不均匀饵 其操作简便 撤 广泛 的缓释性与未括i 层的药物相比有了大幅度的提升' 为药物缓释领域指引了 应胛 。 新的方向。 2 2 尿素7 J } } 去 。尿素是 — 种 在水中溶 度彳 艮 高的弱碱。 体系温良大 3 . 4 电化学方面的应用。 为获得电活I 生 材料 = 丁 牛 电活f 生 的阴 于9 0 ℃时尿素被逐渐分解产生铵根离子和碳酸根离子, 与金属离子生成 离子负载至 l 冰 滑石层间如将磺酸阴 离子负载到锌铬水滑石层间拼在碳电 沉淀。在反应过程中尿 素的分解速度缓慢, 因此溶液的 p H值基本不发生 极 E 沉积制成薄膜获得水滑石修饰的电极材料。水滑石层板的金属阳离 变化。此方法获得的L D Hs 晶体结构好 目 粒径均匀。 子的“ 同晶取代” 以及层间阴离子的可交换性可以制备了具有电化学活性 2 3水热合盛法。 水热合成法是按需要配置—定比例的金属盐溶液和 的水滑石材料推 动着水滑石在电化学领域不断前进。 碱溶液, 再将二者混合然后将上述得到的反应液转移至高压反应釜的不 4结论 锈钢内衬 中, 在适宜 的温度下反应一段时间, 在高温 、 高压 的条件下合成 层状双氢氧化物的制备方法简单多样, 可制备出形貌不同的类水滑石 L D Hs 样品日 。与共沉淀法相比场 粒制 得的 L D H s 晶型结构更加完整, 分 样品。且随着研究的不断深 入其制备方法也不局限于 E 述方法的某—张 散l 好、 粒子尺寸小。 可以根据需要将其中的力诖逆 行 组合来获得不同性能 的类水l 捐石 。层状 2 , 4离子交换法。 将含有较, J 懒 阴离子的 L D H s 前驱体溶液与目标 双氢氧化物因其独特的陛质 各方面都具有广泛地应用。但也 芷 阴离子溶液在剧烈搅拌的条件下混合 铂 争一 段时间即可交换 L D Hs 的 着—些问题 E 匕 虫 Ⅱ 女 昨耐 陇 貌的样品, 如何对其进行回收再利用等。 层间阴离子 使用该力法要考虑阴离子的交换能力的强弱、 阴离子粒径的 随着对层状双氢氧化物研究的深入这 些问题也会随之解决, 能够使其带 大小、 反应液 p H的高低等影响因素。通常即将进 ^层间的阴离子的电荷 来更- 大的经济效益。 越大 、 半径越小其 交换能力越强。 —般交换介质的 p H越小越有利于离子 参考文献 交换自 过 } j 亍 ' f 旦 通常 p H 呵, J 、 于4 。 [ 1 】 袁素瑁 , 张青红, 李耀刚, 等. 氧化铺镁铝复合氧化物的制备及光催 2 5 煅烧复原法。L D Hs 具有记忆效廊’ 即将 L 2 0 0 9 , l 1 : 1 9 7 7 - 1 9 8 1 . 分散到含有某种阴离子的溶液中朋 离子就会插层进 入层板间隙从 而又 [ 2 1张凤 采 层状双氢氧化物基吸附剂制备及t } 生 能研宄 山东大学博士论 0 1 5 : 1 4 - - 1 5 . 会耍新 形成特殊 的层状结构的 L D H  ̄ o在此力怯 中煅烧温度决定着插层 丈 2 结构能否复原。 当温度超_ 过5 5 0  ̄ C , L D H s 的层状结构基本无法复原。 该方 [ 3 ] 薛源明, 樊丽辉, 刘东斌 李士风 申延明. 锌铬水滑石可见光降解甲 法主要用于制备负载不同层板间阴离子及制备层间羟基强碱 陆催化剂。 基橙 的性能研 究. 沈F B 4  ̄ - T - 大学学报 2 0 1 5 , 0 3 : 2 3 3 - 2 3 7 . 2 . 6 铹波辅 叻合成法。 嫩提提_种 非离子化的辐射能的电磁波0 波 [ 4 ] 袁如 张新, 侯万国. N i f r i 类水滑石的合成与光催化应. 高校化 学工程 长范围从 1 m m ~1 n l ’ 频率范围从 3 0 0 ~ 3 0 0 0 0 0 MH  ̄ 能量在红外与无线 学报 2 0 1 3 , 2 7 3 3 4 - 3 3 8 . 电波之间 旨 量小至可以影响微观离子的迁移和偶极子转动, 大至不能 圈 敖燕辉, 王丹丹, 王沛芳等. 层状双金属氢氧化物的制备方法及光催化 引起分子结构的改变 采用微波法 备L D H s 可以大大地减少反应时间 应 用. 四川环境 2 O 1 6 p1 1 3 6 - - 1 4 3 . 并台 晶度更高的产品。 圈B h a t n a g a r S i l l a n p a a M A r e v i e w o f e me r g i n g a d s o r b e n t s f o r n i — 3层状双氢氧化物的应用 t r a t e r e mo v a l f r o m wa t e r .Ch e mi c l a En g i n e e i r n g J o u na r l 2 0 1 1 ,1 6 8 93 _ _ 5 0 4 . 3 . 1 L D H s 在吸附方面的应用。L D Hs 的层间阴离子具有可交换 因 4 此可以将其作为一种阴离子交换材料来此 外, 还可以将各种阴离子通过 同 S i l v a C G B o u i z i F o me s e t a k L a y e r e d D o u b l e H y d r o x i d e s a s l y Ef f i c i e n t P h o t o c a t a l y s t s or f Vi s i b l e L i g h t Ox y g e n Ge n e r a t i o n 离子交换引入 L D H s 层间 而实现 目 标阴离子的固定。 在吸附方面 L D H s Hi 子污染物。 f r o m Wa t e r . J o u r n a l o f t h e A m e i r c a n C h e mi c a l S o c i e t y . 2 0 �
金属氢氧化物 电解海水 析氧反应 氢氧化钴 nife-ldh
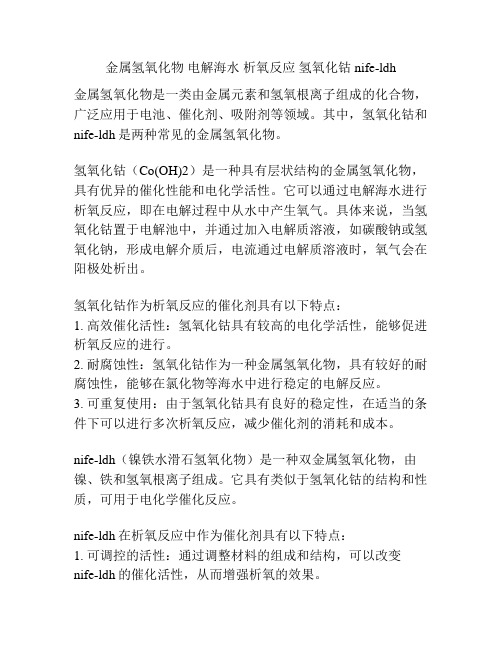
金属氢氧化物电解海水析氧反应氢氧化钴 nife-ldh金属氢氧化物是一类由金属元素和氢氧根离子组成的化合物,广泛应用于电池、催化剂、吸附剂等领域。
其中,氢氧化钴和nife-ldh是两种常见的金属氢氧化物。
氢氧化钴(Co(OH)2)是一种具有层状结构的金属氢氧化物,具有优异的催化性能和电化学活性。
它可以通过电解海水进行析氧反应,即在电解过程中从水中产生氧气。
具体来说,当氢氧化钴置于电解池中,并通过加入电解质溶液,如碳酸钠或氢氧化钠,形成电解介质后,电流通过电解质溶液时,氧气会在阳极处析出。
氢氧化钴作为析氧反应的催化剂具有以下特点:1. 高效催化活性:氢氧化钴具有较高的电化学活性,能够促进析氧反应的进行。
2. 耐腐蚀性:氢氧化钴作为一种金属氢氧化物,具有较好的耐腐蚀性,能够在氯化物等海水中进行稳定的电解反应。
3. 可重复使用:由于氢氧化钴具有良好的稳定性,在适当的条件下可以进行多次析氧反应,减少催化剂的消耗和成本。
nife-ldh(镍铁水滑石氢氧化物)是一种双金属氢氧化物,由镍、铁和氢氧根离子组成。
它具有类似于氢氧化钴的结构和性质,可用于电化学催化反应。
nife-ldh在析氧反应中作为催化剂具有以下特点:1. 可调控的活性:通过调整材料的组成和结构,可以改变nife-ldh的催化活性,从而增强析氧的效果。
2. 高电化学稳定性:nife-ldh在电解海水中具有较高的稳定性,能够长时间地进行析氧反应而不易被腐蚀。
3. 可再生性:nife-ldh在合适的条件下可以进行再生和重复使用,减少催化剂的消耗和成本。
需要注意的是,金属氢氧化物作为导体材料,可以通过导电剂的添加来提高其电导率,从而提高电解反应的效率。
此外,在实际应用中,还需要考虑如何降低电解过程中的能量损耗、提高氧气的生成速率等方面的问题。
综上所述,金属氢氧化物可以作为电解海水析氧的催化剂,其中氢氧化钴和nife-ldh是两种常见的金属氢氧化物。
通过调整催化剂的活性、稳定性和可再生性等特点,可以实现更高效、更经济的电解海水析氧反应。
层状双金属氢氧化物微观结构与性质的理论研究进展

层状双金属氢氧化物微观结构与性质的理论研究进展倪哲明1,*胥倩1潘国祥1,2毛江洪1(1浙江工业大学化学工程与材料学院,催化新材料研究室,杭州310032;2湖州师范学院化学系,浙江湖州313000)摘要:总结了近年来理论计算方法在研究层状双金属氢氧化物(LDHs)结构与功能方面的应用现状.结合LDHs 材料的结构特点,归纳了量子力学、分子力学、几何建模及物理静电模型相结合对LDHs 材料进行结构模拟的思路,比较了各种方法在LDHs 结构模拟上的优势及存在的不足.量子力学方法能够精确获得水滑石材料的层板构成及作用机制、简单阴离子插层水滑石主客体间的超分子作用实质以及电子性质、反应机理等方面的信息.与量子力学相比较,分子力学方法可以快速得到插层水滑石材料的层间阴离子排布及取向、水合膨胀特性及宏观力学性质等.几何模型和物理静电模型能构建直观、形象的数学模型,大大简化了计算量,因此能计算接近实际LDHs 尺寸的体系,为推测LDHs 结构信息提供了可能性.随着理论方法和计算机硬件水平的发展,使得计算机模拟技术逐渐成为获得LDHs 材料微观结构参数、电子性质和动力学性质的一种有效手段.关键词:层状双金属氢氧化物;结构与性质的关系;理论研究进展中图分类号:O641Theoretical Processing in Understanding the Structures and Properties of Layered Double HydroxidesNI Zhe -Ming 1,*XU Qian 1PAN Guo -Xiang 1,2MAO Jiang -Hong 1(1Laboratory of Advanced Catalytic Materials,College of Chemical Engineering and Materials Science,Zhejiang University of Technology,Hangzhou 310032,P.R.China ;2Department of Chemistry,Huzhou Teachers College,Huzhou 313000,ZhejiangProvince,P.R.China )Abstract :We review the techniques,applications,characteristics,and insights gained from the use of theoretical calculations that were applied to the study of layer double hydroxides (LDHs)materials by using a series of typical case studies.The advantages and shortcomings of different theoretical calculation methods (quantum mechanics,molecular mechanics,geometric model,and electrostatic potential energy model)for the study of the properties of LDHs minerals are compared.Based on quantum mechanics calculations,we obtained information about template effects on the construction of layered double hydroxides,super molecular interactions in LDHs containing simple anions,electronic properties,and reaction pathways pared with quantum mechanics,molecular mechanics is quicker in obtaining information about the interlayer structure,arrangement,orientation,hydration,and the swelling trajectory as well as elastic constants etc of LDHs intercalated with various anions.The geometric model and electrostatic potential energy model offer a more intuitive and visual mathematical model of LDHs minerals.The calculations were done on the verge of full size LDHs,which may allow the prediction of the crystal structure.Along with the development of theoretical methods and computer techniques,computational simulation method has become an effective adjust to experimental techniques for obtaining the microscopic structures,electronic and dynamic properties of LDHs minerals.Key Words :Layered double hydroxide;Structure -property relationship;Theoretical processing[Review]物理化学学报(Wuli Huaxue Xuebao )Acta Phys.-Chim.Sin .,2009,25(4):792-805Received:November 9,2008;Revised:January 16,2009;Published on Web:February 23,2009.*Corresponding author.Email:jchx@;Tel:+86571-88320373.浙江省自然科学基金(Y406069)资助项目鬁Editorial office of Acta Physico -Chimica SinicaApril792No.4倪哲明等:层状双金属氢氧化物微观结构与性质的理论研究进展层状双金属氢氧化物(layer double hydroxides,简称LDHs)是一类重要的新型无机功能材料,可作为制备无机-无机、无机-有机纳米复合材料的母体[1].因其具有特殊的层状结构及物理化学性质,在催化[2-5]、离子交换和吸附[6-8]、医药[9-13]等诸多领域具有广阔的应用前景.目前,粉末X射线衍射(XRD)、红外(IR)、热重差热分析(TG-DTA)、核磁共振(NMR)、X射线光电子能谱技术(XPS)、透射电镜(TEM)、中子衍射等实验手段已被应用于LDHs微观结构研究中,并取得了一些重要的结构数据[14-17].比如,LDHs微观结构研究中最为重要的XRD表征技术,能够得到合成材料是否具有层状构型,以及层间距、层板中金属离子的平均间距等重要结构参数[14].将插层阴离子的尺寸与层间距大小相关联,能推测阴离子在层间大致的排布情况(比如单层垂直、双层垂直交替、倾斜交替等)[14].IR、TG-DTA、NMR、中子衍射技术可作为辅助手段来佐证XRD推测所得LDHs微观结构的正确性[15-17].然而,对于复杂阴离子插层LDHs体系,其层间阴离子的排布形态确认仍存在困难,层间结构水分子的排布信息,从实验手段进行表征更是无法得知的.LDHs单晶合成非常有限(迄今为止只合成了2、3个LDHs单晶[1,18]),因而要从实验角度精确探求LDHs微观结构还存在相当大的困难.同时,要探求LDHs层板内部金属离子与羟基之间、主体层板与客体阴离子及水分子之间的超分子作用实质,从实验角度也是很难解决的.近年来,理论模拟计算作为获得LDHs材料微观结构信息和动力学性质的一种有效手段,为LDHs材料的分子设计提供了理论指导.本文总结了近年来理论计算方法在LDHs材料结构与功能方面的研究进展.结合LDHs 材料的结构特点,归纳了量子力学、分子力学、几何建模及物理静电模型相结合对LDHs材料进行结构模拟的思路,比较了各种方法在LDHs结构模拟上的优势及存在的不足.1层状LDHs材料微观结构与功能的关系LDHs是一类具有主体氢氧化物层板、客体阴离子柱撑的无机功能材料,其结构与水镁石结构类似[19,20].LDHs由水滑石(Mg6Al2(OH)16CO3·4H2O)经层板金属阳离子与层间阴离子调变而形成,其结构如图1所示,组成通式为[M II1-x M III x(OH)2]x+(A n-)x/n·m H2O,其中M II和M III分别代表二价和三价金属离子;x 是摩尔比n(M3+)/(n(M2+)+n(M3+));A n-是层间阴离子;m为层间结构水分子数目.LDHs独特的层状构型和结构的可调变性决定了其功能的多样性和应用的广泛性,研究LDHs材料微观结构与功能间的关系,必须对材料的精细结构取得足够的了解.LDHs材料的功能特性按照其微观和亚微观结构(三级结构:主体氢氧化物的层板、层间客体阴离子、结构水分子)进行划分,大致可分为以下三个方面:(1)层板有序结构主要由金属离子与羟基通过配位键形成,主体层板金属离子具有同晶可取代性.LDHs层状材料经合适温度焙烧,能够形成具有高表面积、高分散性、酸碱性可调的复合氧化物.焙烧产物中某些过渡金属经还原,便可形成性能优良的纳米金属负载型催化剂.由于LDHs层板金属离子具有可调变性(如Mg2+、Zn2+、Co2+、Ni2+、Cu2+和Al3+、Fe3+、Cr3+等),所以LDHs的焙烧/还原衍生物在催化、吸附等领域得到广泛应用.(2)层间客体阴离子具有可组装性.LDHs具有客体阴离子可插层性,主体层板与层间客体阴离子间存在着静电和氢键等分子间作用力,很多功能性阴离子能通过这些分子间相互作用而被引入LDHs 层间,形成一类新型LDHs基复合材料.比如:(a)杂多、同多酸阴离子、金属配离子;(b)羧酸、磺酸类有机阴离子;(c)布洛芬、萘普生等医药分子及草甘膦等农药分子;(d)氨基酸、DNA、核苷酸、ATP、酶等生物分子.这些分子/离子与LDHs组装后,能应用于催化、PVC改性剂、药物缓释、无机/生物复合材料、紫外阻隔材料、油田化工品、环境修复等领域.(3)层间水合膨胀及剥离特性.实验证明层间水分子具有稳定LDHs结构的作用,层间水分子与层板及阴离子间主要以氢键作用相结合,并能在一定温度下可逆脱除/吸收,所以在湿度调节方面得图1LDHs材料的结构示意图Fig.1Scheme of microstructure for LDHs793Acta Phys.-Chim.Sin.,2009Vol.25到应用.其他有机中性分子通过克服主客体间超分子作用力被引入LDHs层间,能使LDHs材料层间距膨胀乃至剥离,形成纳米LDHs层片,应用于LDHs/高分子复合材料的合成中.根据Lehn对超分子化学的阐述[21],LDHs主体层板形成、层板中M2+(M3+)-OH-(H2O)作用机制、层板与层间阴离子及水分子间相互作用都可纳入超分子化学研究范畴.从超分子化学角度,使用实验表征与理论模拟计算方法研究LDHs层板内部金属离子与羟基之间、层板与客体阴离子及水分子之间的相互作用,可取得LDHs材料结构调变与其功能间的关系.LDHs超分子结构与功能的基础性研究工作的进展,将有助于LDHs功能的强化以及应用领域的拓展.2理论方法研究LDHs材料的结构与性质近年来,理论模拟计算作为有效的研究方法在LDHs材料的微观结构、力学、热学、电磁学等性能的研究中卓有成效.其中,计算机模拟研究根据模拟尺度、理论依据、研究性质的不同,可分为量子力学(quantum mechanics,QM)和分子力学方法(molecular mechanics,MM)两大类,它被认为是本世纪以来除理论分析和实验观察之外的第三种科学研究手段,称之为“计算机实验”[22].2.1量子力学方法量子力学方法主要包括:从头算法(ab initio method)、分子轨道半经验计算法(semi-empirical molecular orbital method)和密度泛函理论(density functional theory,DFT)[22,23].它以分子中电子的非定域化(delocalization)为基础,电子的行为以其波函数表示.根据海森堡(Heisenberg)的测不准原理,量子力学仅能计算区间内电子出现的概率,其概率正比于波函数绝对值的平方.通过求解由核和电子组成的多电子体系的薛定谔方程,从而获得LDHs材料结构与功能方面的信息[22-24].它建立在原子核与核外电子作用基础上,计算精度高.量子力学方法适用于简单的分子或电子数量较少的体系,能够精确计算分子的性质、结构、构象、偶极矩、电离能、电子亲和力、电子密度等,以及了解分子间相互作用的电子性质.2.1.1从头算法从头算法利用变分原理(variation principle),将系统电子的波函数展开为原子轨道波函数的组合,而原子轨道的波函数又为一些特定的数学函数(如高斯函数)的组合[22,23].这种方法虽然精确,却甚为缓慢,所能计算的系统亦极为有限,通常不超过100个原子.目前,该方法主要应用于LDHs材料精确结构参数[25]、金属离子同晶取代对层板结构的影响[26]、酸碱性等物化性质的影响[26]、催化反应机理、催化剂活性[26]以及电子相关性质(态密度、能带结构)[26]方面的计算.Masini等[25]运用ab initio Car-Parrinello模拟了水镁石(brucite)表面的羟基化和脱羟基作用反应,在获得水镁石(0001),(1100)和(1000)晶面结构特性的同时,通过比较水镁石表面和内部的脱羟基反应所需的能量,指出水镁石分解生成MgO和H2O的反应首先在材料表面发生.Trave等[26]计算了含有不同层板阳离子和层间阴离子的LDHs体系的晶格参数、自由度等结构信息,探讨了层板Al3+的排列方式和Mg/Al摩尔比两个参数对Mg/Al-LDHs结构的影响,当R(M II/M III 摩尔比)约为3时,体系的结合能最低,而层间通道高度最大,建立了结构参数与化学组成和结合能之间的关系.另一方面,通过对态密度等电子性质的研究,发现层间阴离子的性质对化合物的电子性质有很大的影响.与LDHs-Cl体系相比,LDHs-OH体系最高占据轨道(HOMO)-最低空轨道(LUMO)间的禁带宽度较小,且层间的LUMO轨道主要由层间的阴离子提供(如图2),导致了LDHs-OH具有更强的接受电子的能力,所以LDHs-OH较LDHs-Cl在羟醛缩合碱催化反应中具有更高的碱催化活性.李蕾等[27]在制备表征磺基水杨酸、4-羟基-3-甲氧基肉桂酸和2-羟基-4-甲氧基二苯甲酮-5-磺酸等紫外吸收剂插层锌铝水滑石的基础上,运用(a)(b)图2(a)LDHs-Cl和(b)LDHs-OH的HOMO和LUMO轨道分布图[26]Fig.2Spatial distributions of the HOMO and LUMO for(a)LDHs-Cl and(b)LDHs-OH [26]794No.4倪哲明等:层状双金属氢氧化物微观结构与性质的理论研究进展Gaussian98[28]软件中的ab initio分子轨道法(HF/6-31G)计算了LDHs层间有机紫外吸收剂的分子结构和电荷分布,提出了合理的客体阴离子在主体层间的排列方式,并分析了其结构与光化学行为的关系.结果表明,层板间的限域空间有利于主客体间的静电和氢键相互作用,插层产物的紫外吸收范围和能力显著增强,是一类具有潜在应用价值的无机-有机超分子复合结构的紫外吸收材料.2.1.2分子轨道半经验方法分子轨道半经验方法在从头算法的基础上,多引用了一些实验值为参数,求解Hartree-Fock-Roothaan 方程,以取代计算真正的积分项[22,23].采用此方法计算LDHs材料时,相关的结构参数如键长、键角等,往往不是通过几何优化获得,而是来源于实验文献.目前常用的分子轨道半经验方法有休克尔方法(EHMO),全略微分重叠(CNDO),间略微分重叠(INDO),改进的间略微分重叠(MINDO),忽略双原子微分重叠(MNDO),以及在忽略双原子微分重叠(MNDO)基础上发展起来的AM1方法和PM3方法等[22,23].与从头算法相比,利用半经验分子轨道方法可计算较大的分子,但需以大量的实验数据为基础.Pu等[29,30]采用MNDO/d、PM3法优化了不同尺寸水滑石层板结构,优化所得的晶体团簇模型呈六边形结构,且层板的Mg/Al摩尔比为可变参数,但当层板直径变得比较大时,Mg/Al摩尔比的极限值为3.在插层反应过程中,层板的边缘较容易吸附阳离子,而层板中心则易接受阴离子.在此基础上,他们采用B3PW91方法在Lanl2dz基组水平上进一步研究了Mg/Al摩尔比为3的水滑石层板结构[31],通过将结构参数和模拟得到的XRD图谱分别与实验观测结果相比较,确定了水滑石层板符合空间群R3m(166),沿第三维方向有序堆积.李蕾等[32]采用以含原子对排斥的EHMO法ASED(atom superposition and electron delocalization) -MO法为基础的结构自动优化EHTOPT程序,优化计算了Mg6Al2(OH)16X·H2O主体层板与不同简单客体阴离子稳定结构的能量变化、成键状况及电荷转移情况,揭示了LDHs层板与层间阴离子间存在静电吸引、氢键等非共价键弱相互作用.其中氢键作用为主,且强弱与阴离子电荷分布、空间排布方式密切相关,层间阴离子电荷分布对层板酸碱性变化也有影响.2.1.3密度泛函理论密度泛函理论是一种研究多电子体系电子结构的量子力学方法,即为非常精确的量子计算方法.在Kohn-Sham DFT的框架中,最难处理的多体问题(由于处在一个外部静电势中的电子相互作用而产生的)被简化成了一个没有相互作用的电子在有效势场中运动的问题[23].密度泛函理论是目前多种领域中电子结构计算的领先方法,但是用它来恰当地描述分子间相互作用,特别是范德华力(van der Waals),或者计算半导体的能隙还存在一定的困难.目前该方法主要被应用于研究LDHs材料层板金属离子同晶取代对层板结构的影响[31]、层板内部金属离子与羟基之间配位作用[33]、简单阴离子插层LDHs材料的微观结构性质和主客体间超分子作用实质[34-36]、成键规律、热稳定性、酸碱性等物化性质、离子交换性能、催化性能[32-37]、反应机理[38,39]等相关领域的研究.Wei等[33]采用混合密度泛函B3LYP方法对构建LDHs时产生的模板效应进行了研究.根据计算所得的结构畸变角θ的不同,可将层板金属离子分为三类(如图3):类型I(canonical structure,θ:0°-1°),类型II(slightly distorted structure,θ:1°-10°)和类型III(heavily distorted structure,θ>10°).计算了与LDHs 结构相关的键长、O—M—O键弯曲角、键能、价电图3[M(H2O)6]n+的三种几何优化结构[33]Fig.3Three types of optimized structures of the[M(H2O)6]n +[33]795Acta Phys.-Chim.Sin.,2009Vol.25子构型、配位场以及Jahn-Teller效应和自然键轨道(NBO).指出同晶取代Mg2+进入LDHs层板的金属阳离子,其与氧原子形成的八面体六配位的畸变角较离子半径对LDHs的层板结构有着更大的影响,且影响程度为类型I>类型II>类型III.倪哲明等[34,35]建立了LDHs与卤素阴离子(F-、Cl-)的单层簇模型,运用Gaussian03[40]程序,采用混合密度泛函B3LYP方法,在6-31G(d)基组水平上进行结构优化和频率分析,然后分别用6-31G(d)和6-311++G(d,p)计算主客体相互作用能,分析了LDHs 主体层板与卤素阴离子的超分子作用(静电作用和氢键作用),并对F-、Cl-超分子作用的强弱进行了比较.然后又建立了LDHs与CO2-3、H2O的双层簇模型,采用B3LYP/6-31G(d)//B3LYP/3-21G方法计算类水滑石(LDHs-CO3-n H2O)的结构与能量,探讨了主客体间的超分子作用,并对LDHs层间存在的三种不同类型氢键的强度进行了比较.在此基础上,还构建LDHs-Cl-n H2O周期性模型(如图4),采用了CASTEP[41]优化计算其微观结构,从结构参数、Mulliken电荷布居、态密度(DOS)、能量等角度研究层间Cl-和不同数目水分子的分布形态以及与LDHs层间的超分子作用[36].随着水分子数的增加,层间距逐渐增大后趋于平衡.水合过程中氢键作用比静电作用更占优势,layer-water型氢键要略强于anion-water型氢键.当n=1、2时,Cl-与水分子所在平面以平行层板的方式存在于LDHs层间,并且与两层板的距离基本相等;当n=3、4时,Cl-与水分子则以偏向某一层的方式随机地存在于LDHs 层板间,并且得出LDHs-Cl的水合具有饱和量.Anderson等[37]在实验合成sulfonato-salen-M III (M=Mn,Fe,Co)配合物插层Zn/Al-LDHs的基础上,在分子氧和常温、常压条件下,测试其对生成环己烯和二聚环戊二烯的环氧化反应的催化性能.采用混合密度泛函B3LYP方法,计算了sulfonato-salen-M III (M=Mn,Fe,Co)的结构参数(如图5),发现不同插层产物的层间通道高度和催化行为是有区别的,中心金属离子的性质较大地修饰了催化活性位附近的化学环境,从而导致了不同配合物的催化活性不同,催化性能大小为LDH-[Fe(Cl)(salen)]<LDH-[Co(Cl) (salen)]<LDH-[Mn(Cl)(salen)].Greenwell等[38]采用平面波密度泛函理论(PW-DFT)研究了镁铝水滑石催化叔丁醇酯交换反应的机理.通过计算反应机理中涉及的过渡态,指出Choudary等提出的机理(如图6(a))中催化剂再生的步骤无法实现.通过一系列计算模拟,提出层间水的图4LDHs-Cl-n H2O(n=1-4)主客体作用模型[36]Fig.4Host-guest interaction models of LDHs-Cl-n H2O(n=1-4)[36]图5Sulfonato-salen-M III配合物的几何结构[37]Fig.5Computed structures of sulfonato-salen-M III [37] 796No.4倪哲明等:层状双金属氢氧化物微观结构与性质的理论研究进展存在是催化剂再生的必要条件(如图6(b)),叔丁基阳离子在层间不可能单独存在,活性部分叔丁醇的羟基—OH与LDHs层板间存在着比较强的相互作用.LDHs层板的亲水性与t-Bu-LDH的层间区域的疏水性,导致有机物分子的极化基团排列于LDHs 表面,增强了材料的催化活性,促进整个催化反应的进行.Wei等[39]采用混合密度泛函B3PW91方法在6-31G(d,p)基组水平上,对手性药物左旋多巴(L-dopa)插层前后的外消旋现象进行了研究.对反应中涉及的过渡态的模拟结果表明,插层前左旋多巴单体手性碳上的H会迁移至羧基,构成烯醇式的活性中间体,从而外消旋化.插层后,左旋多巴的羧基会和LDHs层板发生强的主客体相互作用(相互作用能为-1100kJ·mol-1),而无法成为质子接受体,外消旋化得到了抑制.从而证明了LDHs材料是一种很好的用来贮存和运载手性药物的载体.2.2分子力学方法分子力学方法起源于1970年左右,是依据经典力学(classical mechanics)的计算方法.此种方法主要依据Born-Oppenheimer近似原理,计算中将电子的运动忽略,而将系统的能量视为原子核位置的函数[23,24].以原子间相互作用势为基础,主要依据分子的力场计算分子的各种特性.该方法主要包括:能量最小化(energy minimization)、蒙持卡罗计算法(Monte Carlo method,MC)和分子动力学模拟(molecular dynamics simulation,MD).分子力场中的关于LDHs材料的结构参数通常可经由量子力学计算或实验方法得到.与量子力学相比较,此方法可以快速地得到分子的各种性质,但无法得到有关体系电子性质方面的结果.分子力学方法常被用于药物、团簇体、生化大分子的研究、复杂的有机阴离子(药物、氨基酸、DNA)插层LDHs复合材料超分子结构的优化计算.2.2.1能量最小化法能量最小化方法是将最初的结构进行模型修饰(model refinement)的过程,藉由分子力学的能量最小化来修正不利的非共价碰触及达到理想的键结合和能量最低的构型.分子力学方法主要计算包括键长、键角、二面角、静电作用力和范德华作用力等位能参数,它的能量计算公式:E total=E stretching+E bending+E dihedral+E out-of-plane+E cross terms+E van der Waals+E coulombic在分子力学方法中常用于能量最小化的力场有CHARMM[42],AMBER[43],CVFF[44],CFF91[45]或GROMOS[46]等.Fogg等[47]采用GULP程序(general utility lattice program)计算了Li/Al-LDHs-Cl、Li/Al-LDHs-Br和Li/ Al-LDHs-NO3体系的最优结构.模拟结果表明,能量最低时所得的结构与实验表征的结果相一致,能够较好地解释NO-3在Li/Al-LDHs-NO3层板间的无规律排列现象.这种计算方法同时也被认为是研究Li/ Al-LDHs-CO3、Li/Al-LDHs-SO4和Li/Al-LDHs-C2O4等体系层间间距和层间客体排布取向的有效手段. 2.2.2蒙特卡罗计算法蒙特卡罗计算法借由系统中质点(原子或分子)的随机运动,结合统计力学的概率分配原理,以得到体系的统计及热力学资料.此方法多用以研究复杂体系及金属的结构及其相变性质.蒙特卡罗计算法的弱点在于只能计算统计的平均值,不能追踪势能变化的路径,无法得到系统的动态信息[24].此计算所依据的随机运动并不适合物理学的运动原理,与其他的非量子计算方法相较亦非特别经济快速.因此,自分子动力学计算逐渐盛行后,蒙特卡罗计算方(a)Choudary et al.′s proposed mechanism(b)Greenwell et al.′s proposed mechanism图6两种可能的酯交换反应机理[38]Fig.6Two possible reaction mechanism for the transesterification reaction [38]797Acta Phys.-Chim.Sin.,2009Vol.25法已较少为人所采用.Kirkpatrick等[48]采用NMR与Monte Carlo模拟相结合的方法,观察模拟了SO2-3、SeO2-4、PO2-4、HPO3-4、MoO2-4、ClO-4、SeO2-3、CO2-3、F-、Cl-、Br-、I-、OH-和NO-3阴离子进入Li/Al和Mg/Al-LDHs后与层间水相互影响可能发生的三种膨胀情况,并分析了相对湿度(RH)对该体系膨胀行为的影响.类型I,明显膨胀行为,膨胀尺度为0.15-0.30nm,通过XRD和NMR 测定发现,层间水从单层排列转变为双层排列,与吸附水等温曲线相一致.类型II,轻微膨胀行为,膨胀尺度<0.05nm,伴随着大量的层间水交换行为;层间水在层间只呈单层排列,且随着RH的增加,层间阴离子会经历一个动力学无序化阶段.类型III,基本不产生膨胀行为,膨胀尺度为0-0.02nm,只有微量的层间水交换行为;由于层间客体的紧密排列,只有极少量的水分子被吸收进入层间.此外,由于阴离子的体积较小,使其和层板羟基之间存在着强烈的静电作用和氢键作用,导致RH对环境结构和阴离子的动态特征几乎没有影响.2.2.3分子动力学模拟分子动力学模拟是应用力场及根据牛顿运动力学原理所发展的计算方法[23],在物理学、化学、生物学和材料科学等许多领域中得到广泛地应用.与蒙特卡罗计算方法比较,分子动力学模拟时系统中粒子的运动有正确的物理依据.优点是精准性高,可同时获得系统的动态与热力学统计资料,并可广泛地适用于各种系统及各类特性的探讨.分子动力学模拟方法本身亦有一定的限制,由于此计算需要引用数理积分方法,因此仅能研究系统短时间范围内的运动,而无法模拟一些时间较长的运动问题[24].MD方法主要用于软性层状粘土材料的模拟,在可变层间空间条件下,计算LDHs层间客体(简单阴离子、有机大分子、生物分子)的排布方式及取向[49-55]、层间阴离子与主体层板的成键情况、吸附、水合膨胀特性模拟[50-59]以及氢键结构分析[57-60],预测化学反应的活性及产物分析[55],LDHs材料XRD图谱模拟计算[61,62],药物分子在层板间的存在形式、结合情况[63-65]、热效应[62]等方面的计算模拟研究.Ma等[50]对包含水分子和不同客体阴离子(CO2-3、SO2-4、OH-、F-、Cl-、Br-、NO-3)的α-Ni(OH)2(1)、β-Ni(OH)2 (2)和Ni/Al-LDHs(3)三个体系的结构进行了分子动力学模拟,发现对于α-Ni(OH)2和Ni/Al-LDHs体系,当层间阴离子为F-、Cl-、Br-、OH-、NO-3、CO2-3时,层间水分子的堆积模式为紧密堆积(如图7(a));当层间阴离子为SO2-4时,层间水分子的排列方式相对松散(如图7(b));且对于Ni/Al-LDHs-SO2-4体系,由于层间含水量较高,水分子在层间为双层排布(如图7(c)).同时,对β-Ni(OH)2体系水合能以及无水β-Ni(OH)2的相对结合能进行了有关计算,为不同阴离子在离子交换反应的相对亲和力提供了理论依据,并指出库仑引力是影响势能的主要因素,层间阴离子的净电荷和范德华半径以及层间水的含量都对结合能有显著的影响,尤其当层间为有机阴离子时,效果更为显著.Lombardo等[62]首次以实验XRD和MD相结合的方法应用于获取热处理过程中低晶状[Zn0.65Al0.35 (OH)2]Cl0.35·0.35H2O(I)和[Zn0.65Al0.35(OH)2](CO3)0.175·0.69H2O(II)的结构、动力学等性质.他们工作的亮点是MD模拟所得到的XRD谱图是通过计算一系列晶胞参数不同的结构模型拟合得到的,而不是传图7层间水分子的堆积方式[50]Fig.7Schematic to show possible packing styles of interlayer water molecules [50] 798。
金属氢氧化物 电解海水 析氧反应 氢氧化钴 nife-ldh
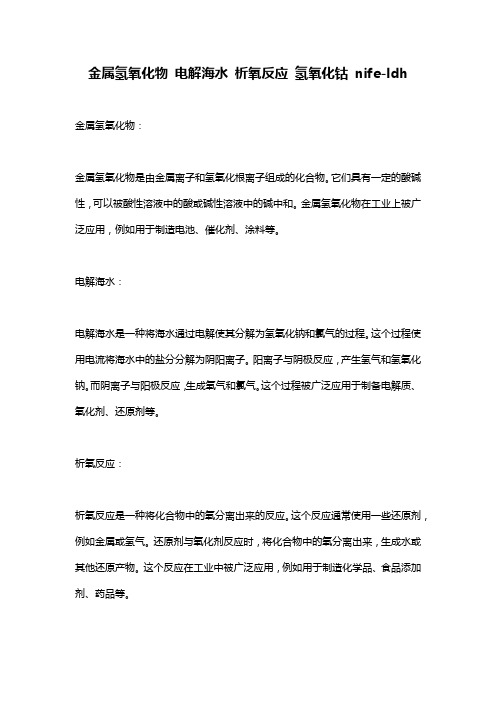
金属氢氧化物电解海水析氧反应氢氧化钴nife-ldh
金属氢氧化物:
金属氢氧化物是由金属离子和氢氧化根离子组成的化合物。
它们具有一定的酸碱性,可以被酸性溶液中的酸或碱性溶液中的碱中和。
金属氢氧化物在工业上被广泛应用,例如用于制造电池、催化剂、涂料等。
电解海水:
电解海水是一种将海水通过电解使其分解为氢氧化钠和氯气的过程。
这个过程使用电流将海水中的盐分分解为阴阳离子。
阳离子与阴极反应,产生氢气和氢氧化钠。
而阴离子与阳极反应,生成氧气和氯气。
这个过程被广泛应用于制备电解质、氧化剂、还原剂等。
析氧反应:
析氧反应是一种将化合物中的氧分离出来的反应。
这个反应通常使用一些还原剂,例如金属或氢气。
还原剂与氧化剂反应时,将化合物中的氧分离出来,生成水或其他还原产物。
这个反应在工业中被广泛应用,例如用于制造化学品、食品添加剂、药品等。
氢氧化钴:
氢氧化钴是一种由钴离子和氢氧化根离子组成的化合物。
它具有一定的酸碱性,可以被酸性溶液中的酸或碱性溶液中的碱中和。
氢氧化钴在工业上被广泛应用,例如用于制造电池、催化剂、涂料等。
NiFe-LDH:
NiFe-LDH是一种由镍离子和铁离子组成的层状双金属氢氧化物。
它具有很高的比表面积和电化学活性,因此被广泛应用于电化学能源转换、催化剂、吸附剂等领域。
该化合物被认为是一种有潜力的电化学材料,可以用于制备高效的电极材料和催化剂。
三价金属和四价双金属层状氢氧化物

三价金属和四价双金属层状氢氧化物哎呀,今天咱们聊聊一个听起来有点高大上的话题——三价金属和四价双金属层状氢氧化物。
听上去好像有点晦涩难懂,其实就是一些化学物质,跟我们生活中的很多东西息息相关,别急,咱们慢慢来,轻松聊聊。
什么是三价金属呢?简单来说,这种金属在化学反应中最喜欢丢掉三个电子。
想象一下,一个小孩拿着糖果,他有五颗糖,结果他和朋友们玩游戏,决定把三颗糖送出去。
剩下的两颗糖,嘿,就是他的“金属状态”。
像铬、铁、铝这些小家伙们,都喜欢以三价形式出现,它们可不是省油的灯,能和其他元素玩得热火朝天。
说到四价双金属,那就更有意思了。
它们可是一对双胞胎!简单来说,就是两种金属在一起,每个金属都丢掉四个电子。
听起来很复杂,但其实就像两个人一起共享一辆自行车,骑得飞快。
比如,钛和锰这两个家伙,凑到一起就能形成强壮的四价双金属氢氧化物。
它们的化学活性超强,做事效率那叫一个高!想想看,搭档一起工作,总比单打独斗强吧。
层状氢氧化物,哎哟,这个词一听就让人觉得高深。
其实就是一种像蛋糕一样的结构,一层一层叠加。
就像奶油蛋糕上面撒的巧克力屑,三价金属和四价双金属就在这个结构中各自占据一层。
你想啊,这样的结构,不仅美观,还能把不同的性质结合在一起,真是让人眼前一亮。
这就像调料一样,把不同的味道混合在一起,创造出新鲜的口感。
在实际应用中,这些层状氢氧化物可是大有用处。
想象一下,科学家们用它们来制造超级电池,能量储存能力杠杠的!有了它们,电动车的续航能力就能大幅提升。
简直就像给小汽车加了个大油箱,出门再也不怕找不到充电桩了。
这些材料在环境保护上也能发挥大作用。
比如,有些金属能吸附水中的重金属离子,就像在水里放了一个小过滤器,把脏东西统统抓住,保护咱们的水源。
这可真是科技的力量啊,真想给它们竖个大拇指。
不过,要实现这些好处,研究人员可是费了不少脑筋。
他们不断实验,寻找最佳的组合方式。
就像调配一瓶鸡尾酒,得小心翼翼,才能调出完美的口感。
- 1、下载文档前请自行甄别文档内容的完整性,平台不提供额外的编辑、内容补充、找答案等附加服务。
- 2、"仅部分预览"的文档,不可在线预览部分如存在完整性等问题,可反馈申请退款(可完整预览的文档不适用该条件!)。
- 3、如文档侵犯您的权益,请联系客服反馈,我们会尽快为您处理(人工客服工作时间:9:00-18:30)。
Hexagonal nanosheets from the exfoliation of Ni 2+-Fe 3+LDHs:a route towards layered multifunctional materials †Gonzalo Abell a n,a Eugenio Coronado,*a Carlos Mart ı-Gastaldo,a Elena Pinilla-Cienfuegos a and Antonio Ribera abReceived 13th May 2010,Accepted 20th June 2010DOI:10.1039/c0jm01447hHere we report the synthesis of a crystalline micrometric-sized hexagonal-shaped Ni 2+-Fe 3+LDH by following a modified homogeneous precipitation method.The exfoliation of the material in formamide leads to stable suspensions of hexagonal nanometric sheets,which have been extensively characterized.Our data confirm that the intrinsic properties of the bulk material are retained by these segregated nanosheets,thus opening the door for their use in the development of layered multifunctional materials.Exfoliation of layered hydroxides,defined as the segregation of these lamellar inorganic solids into single entities through soft chemistry methods,1can be regarded as a versatile synthetic route to prepare stable suspensions of sheets,typically exhibiting homogeneous micrometric lateral size and nanometric thickness.Concerning materials science,these 2D nanosheets offer very interesting features:(a)owing to the nanometric thickness of these layers,they may exhibit new physical properties different from the bulk;(b)compared to other low dimensional nano-materials (0D or 1D),their two-dimensional structure facilitates their processability.In fact,these layers can be re-assembled into highly oriented films by employing Langmuir–Blodgett (LB)or Layer-by-Layer (LbL)techniques;2(c)they can lead to the design of new multifunctional materials combining sophisticated phys-ical properties through the rational choice of the constituting building blocks and the precise control of their arrangement in the solid state.3Among these layered materials,we were particularly interested in the layered double hydroxides (LDHs)compounds.The struc-ture of these anionic clays is closely related to that of the brucite,Mg(OH)2,with partial substitution of some of the divalent posi-tions with M 3+cations.This fact generates an excess of positive charge in the layers which must be balanced by the presence of anions and water molecules interleaved between the layers according to the formula:[M II 1Àx M III x (OH)2](A n À)x/n $yH 2O.In this way,their exfoliation in organic solvents produces steady colloidal suspensions of positively charged nanosheets,4which in the presence of anionic components,can be further re-assembled into sandwich-like layered materials as result of the presence of attractive Coulombic interactions.5In addition,LDHs offer a wide plethora of intrinsic magnetic,optical or catalytic proper-ties,6which ultimately will be retained by the segregated layers andtransferred to these layered super-structures.Concerning magne-tism,we recently reported the presence of spontaneous magneti-zation below 15K in Ni 2+-Fe 3+LDHs prepared by the traditional coprecipitation method,as result of the combination of strong antiferromagnetic superexchange interactions between the in-plane ions mediated by the hydroxyl bridge and much weaker dipolar interactions between the layers.7Unfortunately,the synthesis of crystalline Fe 3+-based LDHs with hexagonal morphology in an extendable controlled manner has been elusive so far.More generally,the synthesis of non-Al 3+-based LDHs is very disfavoured mainly due to the specific amphoteric behaviour of Al(OH)3.Except for remarkable efforts,8the use of traditional coprecipitation methods,based on the direct combination of the constituting ions in a basic medium,has just led to the isolation of amorphous phases.Although these materials can indirectly enhance its crystallinity after an additional hydrothermal treat-ment,they cannot be effectively exfoliated.9Here we report how,by following a modified homogeneous precipitation method,whose novelty resides in the use of the chelating agent triethanolamine (TEA;C 6H 15NO 3)as auxiliary reagent,permits the synthesis of a highly crystalline micrometric-sized hexagonal-shaped [Ni 1-x Fe x (OH)2](CO 3)x/2(x ¼0.25)LDH.Besides,this material can be readily ion-exchanged with nitrate anions through the so-called ‘‘acid-salt treatment’’,10permitting its quantitative exfoliation in formamide.Regarding the potential magnetic functionality of these Ni 2+Fe 3+-LDH nanosheets,7this work paves the way for their use in the design of advanced multifunctional materials.ExperimentalSynthesisAll chemical reagents,Ni(NO 3)2$6H 2O,Fe(NO 3)3$9H 2O,Al(NO 3)3$9H 2O,NaNO 3,HNO 3,C 6H 15NO 3(triethanolamine,TEA)and CO(NH 2)2(urea)were used as received from commercial suppliers (Sigma-Aldrich and Fluka)without further purification.Synthesis of NiFe-CO 3LDH (1):LDHs were synthesized following a modified homogeneous precipitation method by using urea and TEA as ammonium releasing reagent (ARR)and chelating agent,respectively.aUniversidad de Valencia (ICMol),Catedr atico Jos e Beltr a n n 2,46980Paterna,Spain.E-mail:eugenio.coronado@uv.es;Fax:+34963543273;Tel:+34963544415bFundaci on General de la Universidad de Valencia (FGUV),Amadeu de Savoia n 4,46010Valencia,Spain†Electronic Supplementary Information (ESI)available:Further details concerning the experimental procedure and physical characterization of the materials here reported including X-ray diffraction,SEM images,EDAX metallic analysis,FT-IR and TG/DTA studies.See DOI:10.1039/c0jm01447h/PAPER /materials |Journal of Materials ChemistryD o w n l o a d e d b y H e F e i U n i v e r s i t y o f T e c h n o l o g y o n 10 J a n u a r y 2012P u b l i s h e d o n 02 A u g u s t 2010 o n h t t p ://p u b s .r s c .o r g | d o i :10.1039/C 0J M 01447HView Online / Journal Homepage / Table of Contents for this issueIn a typical procedure,the nitrate salts of the metals were dissolved in 50mL of deionized purged water together with TEA,in order to reach a total metal cation concentration of 20mM in the final solution,with x ¼0.25and a concentration of TEA equimolar with iron concentration,being x ¼[Fe 3+]/([Fe 3+]+[Ni 2+]).Next,50mL of an aqueous solution of urea (35mM)were added.The amount of urea was fixed to be 1.75times the total metal concentration.The resulting dark brown mixture was placed in a 125cm 3stainless steel Teflon-lined autoclave and heated up to 150 C in a preheated oven.After 48h,the auto-clave was left to cool down at room temperature and the resulting yellowish fine powder was filtered and dried in a vacuum.The pH value of the remaining solution was found to be around 8.IR (KBr pellet)n /cm À1:3446(br,st);1637.0(m);1358(sh,m);735(st);492(sh,m).EDAX (calculated for Ni 0.75Fe 0.25):Found Ni ¼75.2%(75.0%);Fe ¼24.8%(25.0%).Water content (TG):12.9%.Anal.calc.for [Ni 0.75Fe 0.25(OH)2](CO 3)0.125$0.8H 2O (F w ¼113.35):C,1.32;H,3.19%.Found:C,1.72;H,2.89%.Preparation of NiFe-NO 3LDH (2):Nitrate-exchange was carried in an excess of nitrate anion.In a typical procedure,1g of 1was immersed in a round-bottom flask containing 1000mL of an aqueous solution of NaNO 3(1.5M)and HNO 3(0.005M).This mixture was mechanically stirred under inert atmosphere during 48h.Afterwards,the resulting yellowish product was filtered,washed thoroughly with deionized water and dried in vacuum at room temperature.IR (KBr pellet)n /cm À1:3448(br,st);1637.0(m);1385(sh,m);1352(w);654(st);486(sh,m).EDAX (calculated for Ni 0.75Fe 0.25):Found Ni ¼75.13%(75.0%);Fe ¼24.87(25.0%).Water content (TG):6.7%.Anal.calc.for [Ni 0.75Fe 0.25(OH)2](NO 3)0.250$0.5H 2O (F w ¼125.51):N,2.79;H,3.21%.Found:N,2.95;H,2.39;C,0.44%.Exfoliation of the NiFe-NO 3LDH:2was finely powdered and dispersed in degassed formamide at a concentration of 1g L À1.Then,the mixture was vigorously stirred at 600rpm for three days.To facilitate the exfoliation,the dispersion was successively sonicated in an ultrasonic water bath (Branson 5510)during three intervals of 20min periodically during the three days,until the turbidity of the dispersion remained constant.Finally,the yellowish translucent colloidal suspension was centrifuged at 2000rpm for 10min to remove the remaining non-exfoliated material.The resulting emulsion clearly exhibited the Tyndall effect when irradiated with a laser beam,thus confirming the presence of exfoliated LDH layers (see Fig.2in the main text).Restacked NiFe-CO 3LDH (R-NiFe-CO 3;3):This sample was isolated by adding an aqueous solution of Na 2CO 3(250mL,1M)to 250mL of a freshly prepared suspension of exfoliated layers of 2in formamide.The mixture was mechanically stirred;with immediate appearance of the yellowish precipitate.Mechanical stirring was maintained for 5additional minutes and finally the suspension was kept static,without stirring during 12h,until no sign of turbidity was detected.Afterwards,the resulting finely divided powder was filtered,washed thoroughly with deionized water and dried in vacuum at room temperature.IR (KBr pellet)n /cm À1:3485(br,st);1637.0(m);1358(w);1360(st);727(st);521(sh,m);494(sh,m).EDAX (calculated for Ni 0.75Fe 0.25):Found Ni ¼74.2%(75.0%);Fe ¼25.8%(25.0%).Water content (TG):14.4%.Anal.calc.for [Ni 0.74Fe 0.26(OH)2](CO 3)0.125$0.7H 2O (F w ¼112.08):C,1.34;H,3.06%.Found:C,1.95;H,2.65;N,0.28%.Physical characterizationMetallic atomic composition of bulk samples was determined by means of electron probe microanalysis (EPMA)performed in a Philips SEM-XL30equipped with an EDAX microprobe.Carbon,nitrogen and hydrogen contents were determined by microanalytical procedures by using a LECO CHNS-932in the Universidad Complutense de Madrid.Particle morphologies and dimensions were studied with a Hitachi S-4100scanning electron microscope at an accelerating voltage of 20keV,over metalized samples with a mixture of gold and palladium during 30s.Infrared spectra were recorded in a FT-IR Nicolet 5700spec-trometer in the 4000–400cm À1range with a nominal resolution of 0.4cm À1,using powdered samples diluted in KBr pellets.Ther-mogravimetric analysis of all compounds were carried out with a Mettler Toledo TGA/SDTA 851apparatus in the 25–800 C temperature range under a 10 C min À1scan rate and an air flow of 30mL min À1.X-ray powder diffraction patterns were collected with a Siemens d-500X-ray diffractometer (Cu-K a radiation;l a ¼1.5418 A)equipped with a rotating anode D-max Rigaku operating at 80mA and 45kV.Samples were mounted on a flat sample plate.Profiles were collected in the 2.5 <2q <70 range with a step size of 0.05 .Dynamic light scattering (DLS)measurements were carried out at 25 C with a Zetasizer Nano ZS instrument (Malvern Instruments Ltd.)on a freshly prepared emulsion of exfoliated LDH in formamide (1g L À1).Mean hydrodynamic diameter and correlation functions were deter-mined by accumulative analysis.HRTEM studies of exfoliated nanosheets were carried out on a JEM-2010microscope (JEOL,Japan)operating at 200kV.Samples were prepared by dropping a suspension of the fresh exfoliated sample in formamide on a carbon-coated copper grid.The digital analysis of the HRTEM micrographs was done using DigitalMicrographTM 1.80.70for GMS 1.8.0by Gatan.Atomic Force Microscopy (AFM)measurements were collected in a Multimode atomic force microscope from Veeco.Typically,a fresh diluted emulsion resulting from the exfoliation of 2in formamide was deposited onto a clean Si wafer by dip coating.The images were obtained with a Si tip (fr and K of ca.300kHz and 42N m À1,respectively)using tapping-mode in air at room temperature.Images were recorded with 512Â512pixels and 0.5–1Hz scan rate.Pro-cessing and analysis of the images were carried out using the Nanotec WSxM software.11Results and discussionNiFe-CO 3LDH (1)was synthesized from aqueous solutions of the nitrate salts of Fe 3+and Ni 2+,TEA and urea under hydro-thermal conditions,according to the homogeneous precipitation method (see the Experimental section).TEA has been widely employed in the synthesis of mesoporous materials according to the so called ‘‘atrane route’’,in view of its ability to chelate metallic ions forming complexes which remain inert towards hydrolysis.12This feature pushed us to employ it as an auxiliary reagent in the synthesis of LDHs,since it could prevent the formation of non-soluble metallic oxides,maintaining a constant ratio of the LDH constituting metals in solution.On the other hand,urea,acting as an ammonia releasing reagent (ARR),isD o w n l o a d e d b y H e F e i U n i v e r s i t y o f T e c h n o l o g y o n 10 J a n u a r y 2012P u b l i s h e d o n 02 A u g u s t 2010 o n h t t p ://p u b s .r s c .o r g | d o i :10.1039/C 0J M 01447Hresponsible for gradually increasing the pH of the synthetic gel in a controlled manner under the reaction conditions.13Fig.1shows the X-ray diffraction pattern of 1.It exhibits the typical profile for LDH materials with sharp intense peaks at low theta values,whereas they become weaker and less defined at higher angular values.No residual peaks were observed thus confirming the absence of contaminant phases.By assuming rhombohedral symmetry,the (00l)peaks,related to the stacking periodicity along the c axis,and the (110)in-plane peak were indexed and permitted calculating the unit cell parameters of 1(Table 1).From these parameters,we estimated a basal spacing(BS)of 7.8 Ain excellent agreement with that reported for other carbonate-intercalated LDHs.13These data,together with the metal analysis,FT-IR and TG/DTA studies (SI4,SI5(ESI†)and Table 2)permit estimating a molecular formula of [Ni 0.75Fe 0.25(OH)2](CO 3)0.125$0.8H 2O for 1.According to scan-ning electron microscopy (SEM)images,this material exhibits an homogeneous distribution of well-defined hexagonal-shaped particles with lateral sizes of ca.1m m (SI1).Exfoliation of carbonate-intercalated LDHs is far unfav-ourable due to the divalent nature of carbonate,which intro-duces stronger electrostatic interactions than other monovalent anions.4For this reason,the starting NiFe-CO 3(1)was trans-formed into NiFe-NO 3(2)through an ion exchange reaction byimmersing it into an aqueous salt-acid mixed solution of NaNO 3and HNO 3.According to Fig.1,the X-ray diffraction of 2showsa small increase in the BS of 0.1 A(Table 1),slightly lower than that expected for nitrate-intercalated LDHs.14This fact together with the presence of low intensity CO 32Àvibration modes in the FT-IR data (SI4)seems to indicate the presence of residual carbonate anions in the interlamellar space.The presence of sharp intense diffraction peaks,with no signature of contami-nant phases,indicates that the ion exchange step proceed with no remarkable loss of crystallinity.In view of the SEM studies (SI2),the micrometric size and hexagonal morphology of the starting material is also maintained after decarbonation.Besides,the constant values of the metal ratio and a parameter permits dis-carding any acid-induced chemical change affecting the LDH layers.According to all these data and the TG/DTA analysis (SI4,SI5and Table 2),2can be formulated as [Ni 0.75Fe 0.25(OH)2](NO 3)0.250$0.5H 2O.Steady yellowish colloidal emulsions of [Ni 0.75Fe 0.25(OH)2]0.25+layers were easily obtained by mechanically stirring and soni-cating a suspension of 2in formamide.The experiments here reported were carried out with concentrations of 1g L À1,but preliminary results indicate that this system can undergo quan-titative exfoliation even at higher concentrations (ca.10g L À1).The presence of exfoliated LDH layers was firstly confirmed by the appearance of Tyndall light scattering when irradiating these emulsions with a laser beam (Fig.2).Direct information con-cerning the size of the exfoliated layers in the formamide dispersion was obtained from Dynamic Light Scattering (DLS)studies (Fig.3a).Even though the average hydrodynamic diameter of LDH dispersions is commonly biased towards the larger particles,15in this case we observe a homogeneous Gaussian distribution of the size of the particles centeredaroundFig.1X-Ray powder diffraction patterns and peaks indexation of 1(black),2(red)and 3(blue).The graphical scheme in the right side shows the structural model for these layered compounds.Table 1X-Ray diffraction data and unit cell parameters for 1–32Q [ ](hkl )Calc.param.a / A(003)(006)(009)(110)a c BS 111.4022.9034.4559.95 3.0823.347.78Æ0.02211.2722.6034.3559.75 3.1023.557.85Æ0.01311.4022.9034.4059.903.0823.347.78Æ0.03aa ¼2d 110;c ¼d 003+2d 006+3d 009;BS ¼c /3.Table 2Metal ratio of the synthetic gels (calculated)and the isolated compounds (experimental)as estimated from Electronic Probe Micro-analysis (EPMA),water content deduced from the thermogravimetric analysis and molecular formulae calculated for 1–3x a Water contentMolecular formula Calc.Exp.Wt.[%]Mol.10.250.2512.90.8[Ni 0.75Fe 0.25(OH)2](CO 3)0.125$0.8H 2O 20.250.25 6.70.5[Ni 0.75Fe 0.25(OH)2](NO 3)0.250$0.5H 2O 30.250.2610.70.7[Ni 0.74Fe 0.26(OH)2](CO 3)0.125$0.7H 2Oax ¼[Fe 3+]/([Ni 2+]+[Fe 3+]).D o w n l o a d e d b y H e F e i U n i v e r s i t y o f T e c h n o l o g y o n 10 J a n u a r y 2012P u b l i s h e d o n 02 A u g u s t 2010 o n h t t p ://p u b s .r s c .o r g | d o i :10.1039/C 0J M 01447H960nm.Fig.3b,c shows high-resolution transmission electron microscopy (HRTEM)images of the NiFe-LDH nanosheets obtained from dropping a freshly prepared emulsion of 2on a carbon-coated copper grid.The exfoliated nanosheets show generally hexagonal morphologies with weak homogeneous contrast,revealing their uniform nanometric thickness,and lateral sizes coincident with those extracted from DLS studies.Fig.3d shows a selected area electron diffraction (SAED)pattern acquired from one of the exfoliated nanosheets.It exhibits a hexagonal arrangement of diffraction peaks as expected for the in-plane symmetry intrinsic to LDH layers.Indexation of the SAED pattern under a hexagonal closed packed structure (hcp)yielded a ¼2.9 A,in good agreement with the values estimated from the indexation of the powder diffraction patterns of thebulk samples (3.08and 3.10 A,for 1and 2,respectively).This point seems to confirm that these nanosheets do not experience any chemical change during the exfoliation process but retain theinternal atomic structure of the LDH layers from the pristine layered bulk material.Fig.4displays atomic force microscopy (AFM)images collected by dip coating a clean Si wafer in a fresh emulsion of 2.The image covering a 6Â6m m area (Fig.4a)shows the presence of particles with lateral sizes coincident once again with the DLS experiments.In some cases,the presence of regular shapes with internal angles close to 120 ,reminiscent of the hexagonal morphologies of the non-exfoliated material,can be observed.The thickness of these nanosheets was studied with more detail in several areas.According to Fig.4b,we observe the presence of an average height profile of 0.8nm corresponding to the presence of a single unilamellar nanosheet.The observed deviation with respect to the crystallographic thickness of a single brucite layer (0.48nm),has been already observed in several AFM studies of exfoliated layered hydroxides and generally attributed to the adsorption of solvent molecules and residual counterions present in the medium.2b,5,15,16Finally,to demonstrate the ability of these single layers to act as macromolecular cations,RNiFe-CO 3(3;where R accounts for restacked)was obtained by adding an excess of Na 2CO 3to the exfoliated NiFe-LDH nanosheets.The presence of intense elec-trostatic interactions between the positively charged layers and the anionic moieties mediates the re-assembling of the original layered structure.On basis of the X-ray diffraction data (Fig.1),the restacked material exhibits the typical pattern for LDHs,with the same unit cell parameters and BS exhibited by 1(Table 1).Besides,the barely broader profile and less intense reflections observed denote a crystallinity quite similar to the original material (1).The observed decrease of the crystallinity must be probably related to the presence of a bigger amount of stacking faults and overall disorder intrinsic to the exfoliation/restacking route.Additionally,a minor impurity related to the presence of a Ni,Fe spinel-like oxide was detected.This fact is not surprising and similar features have been previously observed in the synthesis of related NiFe LDHs through co-precipitation.17Nevertheless,the chemical nature of the LDH layers remains mainly unaffected as confirmed by the constant metallic compo-sition,FT-IR and TG/DTA characterization (SI4,5and Table 2).ConclusionsIn summary,we have successfully overcome the synthetic prob-lems inherent to the preparation of non-Al 3+-containingLDHsFig.2Picture of the colloidal emulsion obtained from the exfoliation of NiFe-NO 3LDH in formamide.The Tyndall effect,resulting from the scattering of the irradiated beam by the particles in solution,confirms the presence of exfoliated LDHnanosheets.Fig.3(a)Distribution of sizes as extracted from DLS studies.(b)HRTEM image of the exfoliated nanosheets.(c)Zoom-in showing their hexagonal morphology.(d)SAED exhibiting a hexagonal arrangement of the diffraction peaks,as expected from the intrinsic symmetry of the LDH layers.The indexed peaks have been employed for estimating the a parameter (see maintext).Fig.4(a)Tapping-mode AFM image of the exfoliated NiFe-LDH nanosheets deposited on a silicon wafer substrate.(b)Height profile concordant with that expected for a single layer.D o w n l o a d e d b y H e F e i U n i v e r s i t y o f T e c h n o l o g y o n 10 J a n u a r y 2012P u b l i s h e d o n 02 A u g u s t 2010 o n h t t p ://p u b s .r s c .o r g | d o i :10.1039/C 0J M 01447Hby following an slightly-modified homogeneous precipitation method which employs TEA as auxiliary reagent.This procedure permits obtaining [Ni 1Àx Fe x (OH)2](CO 3)x/2(x ¼0.25)LDHs with well-defined hexagonal shapes and micrometric size in excellent yields.Preliminary results indicate that this method-ology permits also controlling the in-plane composition of the layers in the 0.2<x <0.3interval,without remarkable effects on the crystallinity,particle size or morphology of the material.In a second step,the starting precursor can be easily nitrate-exchanged in order to produce stable colloidal emulsions of NiFe-LDH nanosheets in formamide.The resulting unilamellar 2D nanometric objects have been extensively characterized by means of DLS,HRTEM and AFM experiments,demonstrating that the internal atomic structure of the LDH layers remains mainly unaffected despite the exfoliation step.Finally,we have validated the suitability of these nanosheets as cationic macro-molecular building blocks by re-assembling the carbonate-intercalated LDH in the presence of an excess of anions.In view of the magnetic functionality exhibited by NiFe-LDHs,7this work must be considered as the first step in the development of advanced multifunctional materials,which will result from the combination of the physical properties intrinsic to these cationic 2D nanosheets with those introduced by electronically active anionic discrete complexes,18or even more complex extended layers.AcknowledgementsFinancial support from the EU (MolSpinQIP)and the ERC (SPINMOL Advanced Grant to EC),the Spanish Ministeriode Ciencia e Innovaci on,with FEDER co-financing (Project Consolider-Ingenio in Molecular Nanoscience and projects MAT2007-61584and CTQ-2008-06720)and the Generalitat Valenciana (Prometeo Program)are gratefully acknowledged.We also acknowledge P.Amor o s and D.Beltr a n for helpfulscientific discussions,Efr e n Navarro-Moratalla for the aesthetic picture illustrating Fig.2and agre for his help with the experimental work.Notes and references1A.Jacobson,J.Mater.Sci.Forum ,1994,1,152–153;T.Sasaki,M.Watanabe,H.Hashizume,H.Yamada and H.Nakazawa,J.Am.Chem.Soc.,1996,118,8329–8335;R.Abe,K.Shinohara,A.Tanaka,M.Hara,J.N.Kondo and K.Domen,Chem.Mater.,1997,9,2179–2184;R. E.Schaak and T. E.Mallouk,Chem.Mater.,2000,12,2513–2516.2T.Sasaki,Y.Ebina,T.Tanaka,M.Harada and M.Watanabe,Chem.Mater.,2001,13,4661–4667;Z.Liu,R.Ma,M.Osada,N.Iyi,Y.Ebina,K.Takada and T.Sasaki,J.Am.Chem.Soc.,2006,128,4872–4880;J.X.He,K.Kobayashi,M.Takahashi,G.Villemure and A.Yamagishi,Thin Solid Films ,2001,397,255–265.3D.M.Kaschak,J.T.Lean,C.C.Waraksa,G.B.Saupe,ami and T.E.Mallouk,J.Am.Chem.Soc.,1999,121,3435–3445;M.Osada,Y.Ebina,K.Takada and T.Sasaki,Adv.Mater.,2006,18,1023–1027.4L.Li,R.Ma,Y.Ebina,N.Iyi and T.Sasaki,Chem.Mater.,2005,17,4386–4391;R.Ma,Z.Liu,L.Li,N.Iyi and T.Sasaki,J.Mater.Chem.,2006,16,3809–3813.5L.Li,R.Ma,Y.Ebina,K.Fukuda,K.Takada and T.Sasaki,J.Am.Chem.Soc.,2007,129,8000–8007.6C.Forano,T.Hibino,F.Leroux,C.Taviot-Gu e ho,Developments in Clay Science 2006,vol.1,pp.1021–1095,Eds:F.Bergaya,B.K.G.Theng,galy;Elsevier.7E.Coronado,J.R.Gal a n-Mascar o s,C.Mart ı-Gastaldo,A.Ribera,E.Palacios,M.Castro and R.Burriel,Inorg.Chem.,2008,47,9103–9110.8R.Ma,Z.Liu,K.Takada,N.Iyi,Y.Bando and T.Sasaki,J.Am.Chem.Soc.,2007,129,5257–5263;Y.Han,Z.-H.Liu,Z.Yang,Z.Wang,X.Tang,T.Wang,L.Fan and K.Ooi,Chem.Mater.,2008,20,360–363;J.Liang,R.Ma,N.Iyi,Y.Ebina,K.Takada and T.Sasaki,Chem.Mater.,2010,22,371–378.9W.Kagunya,Z.Hassan and W.Jones,Inorg.Chem.,1996,35,5970–5974;M.Del Arco,P.Malet,R.Trujillano and V.Rives,Chem.Mater.,1999,11,624–633;P.Refait,M.Abdelmousla,L.Simon and J.-M.R.G e nin,J.Phys.Chem.Solids ,2005,66,911–917;Y.Tian,G.Wang,F.Li and D.G.Evans,Mater.Lett.,2007,61,1662–1666;A.Nakahira,T.Kubo and H.Murase,IEEE Trans.Magn.,2007,43,2442–2444.10N.Iyi,T.Matsumoto,Y.Kaneko and K.Kitamura,Chem.Mater.,2004,16,2926–2932.11I.Horcas,R.Fern a ndez,J.M.G omez-Rodriguez,J.Colchero,J.G o mez-Herrero and A.M.Baro,Rev.Sci.Instrum.,2007,78,013705.12S.Cabrera,J.El Haskouri,C.Guillem,torre, A.Beltr a n,D.Beltr a n,M.D.Marcos and P.Amor os,Solid State Sci.,2000,2,405–420.13U.Costantino,F.Marmottini,M.Nocchetti and R.Vivani,Eur.J.Inorg.Chem.,1998,1439–1446;M.Ogawa and H.Kaiho,Langmuir ,2002,18,4240–4242.14F.Cavani,F.Trifir oand A.Vaccari,Catal.Today ,1991,163–301.15Q.Wu, A.Olafsen,O. B.Vistad,K. D.Knudsen,J.Roots,J.S.Pedersen and P.Norby,J.Mater.Chem.,2007,17,965–971.16G.Hu,N.Wang,D.O’Hare and J.Davis,mun.,2006,287–289;S.Ida,D.Shiga,M.Koinuma and Y.Matsumoto,J.Am.Chem.Soc.,2008,130,14038–14039.17F.B.D.Saiah,B.-L.Sub and N.Bettahara,J.Hazard.Mater.,2009,165,206–217.18E.Coronado,J.R.Gal a n-Mascar os, C.Mart ı-Gastaldo and A.Ribera,Chem.Mater.,2006,18,6112–6114.D o w n l o a d e d b y H e F e i U n i v e r s i t y o f T e c h n o l o g y o n 10 J a n u a r y 2012P u b l i s h e d o n 02 A u g u s t 2010 o n h t t p ://p u b s .r s c .o r g | d o i :10.1039/C 0J M 01447H。