马尔文激光粒度仪简介教学资料
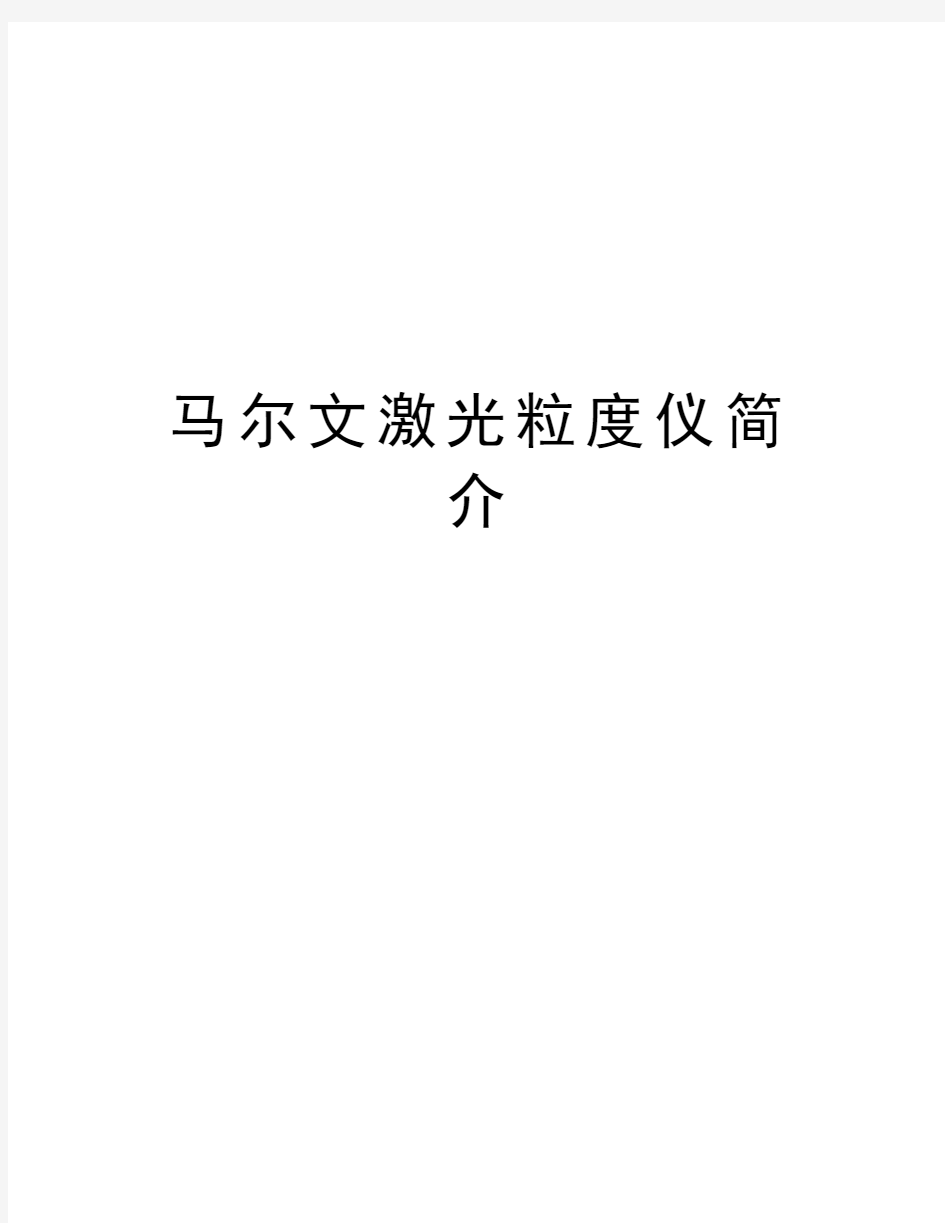

马尔文激光粒度仪简
介
laParticle size analysis-Laser diffraction methods
(ISO-13320-1)
Introduction
Laser diffraction methods are nowadays widely used for particle sizing in many different applications. The success of the technique is based on the tact that it can be applied to various kinds of particulate systems, is fast and can be automated and that a variety of commercial instruments is available. Nevertheless, the proper use of the instrument and the interpretation of the results require the necessary caution.
Therefore, there is a need for establishing an international standard for particle size analysis by laser diffraction methods. Its purpose is to provide a methodology for adequate quality control in particle size analysis.
Historically, the laser diffraction technique started by taking only scattering at small angles into consideration and, thus, has been known by the following names:
-fraunhofer diffraction;
-(near-) forward light scattering;
-low-angle laser light scattering (LALLS).
However, the technique has been broadened to include light scattering in a wider angular range and application of the Mie theory in addition to approximating theories such as Fraunhofer and anomalous diffraction.
The laser diffraction technique is based on the phenomenon that particles scatter light in all directions with an intensity pattern that is dependent on particle size. All present instruments assume a spherical shape for the particle. Figure 1 illustrates the characteristics of single particle scattering patterns: alternation of high and low intensities, with patterns that extend for smaller particles to wider angles than for larger particles[2-7,10,15 in the bibliography].
Within certain limits the scattering pattern of an ensemble of particles is identical to the sum of the individual scattering patterns of all particles present. By using an optical model to compute scattering for unit volumes of particles in selected size classes and a mathematical deconvolution procedure, a volumetric particle size distribution is calculated, the scattering pattern of which fits best with the measured pattern (see also annex A).
A typical diffraction instrument consists of a light beam (usually a laser), a particulate dispersing device, a detector for measuring the scattering pattern and a computer for both control of the instrument and calculation of the particle size distribution. Note that the laser diffraction technique cannot distinguish between scattering by single particles and
scattering by clusters of primary particles forming an agglomerate or an aggregate. Usually, the resulting particle size for agglomerates is related to the cluster size, but sometimes the size of the primary particles is reflected in the particle size distribution as well. As most particulate samples contain agglomerates or aggregates and one is generally interested in the size distribution of the primary particles, the clusters are usually dispersed into primary particles before measurement.
Historically, instruments only used scattering angles smaller than 14°,which limited the application to a lower size of about 1μm. The reason for this limitation is that smaller particles show most of their distinctive scattering at larger angles (see also annex Z).Many recent instruments allow measurement at larger scattering angles, some up to about 150°,for example through application of a converging beam, more or larger lenses, a second laser beam or more detectors. Thus smaller particles down to about 0.1μm can be sized. Some instruments incorporate additional information from scattering intensities and intensity differences at various wavelengths and polarization planes in order to improve the characterization of particle sizes in the submicrometre range.
Particle size analysis – Laser diffraction methods-
Part 1:
General principles
1 scope
This part of ISO 13320 provides guidance on the measurement of size distributions of particles in any two-phase system, for example powders, sprays, aerosols, suspensions, emulsions and gas bubbles in liquids, through analysis of their angular light scattering patterns. It does not address the specific requirements of particle size measurement of specific products. This part of ISO13320 is applicable to particle sizes ranging from approximately 0.1μm to 3μm.
For non-spherical particles, an equivalent-sphere size distribution is obtained because the technique uses the assumption of spherical particles in its optical model. The resulting particle size distribution may be different from those obtained by methods based on other physical principles (e.g. Sedimentation, sieving).
3,terms, definitions and symbols
For the purposes of this part of ISO 13320, the following terms, definitions and symbols apply.
3.1 terms, definitions
3.1.1 absorption
introduction of intensity of a light beam traversing a medium through energy conversion in the medium
3.1.2 coefficient of variation (变异系数)
Noative measure(%) for precision: standard deviation divided by mean value of population and multiplied by 100 or normal distributions of data the median is equal to the mean
3.1.3complex refractive index(Np)
Refractive index of a particle, consisting of a real and an imaginary (absorption) part.
Np=n p-ik p
3.1.4 relative refractive index (m)
complex refractive index of a particle, relative to that the medium。
3.1.5 deconvolution
Mathematical procedure whereby the size distribution of a particle ensemble is inferred from measurements of their scattering pattern.
3.1.6 diffraction
Spreading of light around the contour of a particle beyond the limits of its geometrical shadow with a small deviation from rectilinear propagation
3.1.7 extinction
Attenuation of a light beam traversing a medium through absorption and scattering .
3.1.8 model matrix
Matrix containing light scattering vectors for unit volumes of different size classes, scaled to the detector’s geometry, as derived from model computation.
3.1.9multiple scattering
Subsequent scattering of light at more than one particle, causing a scattering pattern that is no longer the sum of the patterns from all individual particles (in contrast to single scattering )
3.1.10 obscuration
Optical concentration
Percentage or fraction of incident light that is attenuated due to extinction (scattering and/or absorption) by the particles
3.1.11 optical model
Theoretical model used for computing the model matrix for optically homogeneous spheres with, if necessary, a specified complex refractive index, e.g. Frauhofer diffraction, anomalous diffraction, Mie scattering
3.1.12 reflection
Return of radiation by a surface, without change in wavelength
3.1.13 refraction
Change of the direction of propagation of light determined by change in the velocity of propagation in passing from one medium to another, in accordance with Snell’s law
N m sinθm=n p sinθp
3.1.14 scattering
General term describing the change in propagation of light at the interface of two media
3.1.15 scattering pattern
Angular or spatial pattern of light intensities[I(θ) and I(r) respectively] originating from scattering ,or the related energy values taking into account the sensitivity and the geometry of the detector elements
3.1.16 single scattering
Scattering whereby the contribution of a single member of variation (relative percentage) of the size distribution
NOTE for normal distributions about 95% of the population falls within ±2 standard deviations from the mean value and about 99.7% within ±3 standard deviations from the mean value.
3.2 symbols
4 principle
A representative sample, dispersed at an adequate concentration in a suitable liquid or gas, is passed through the beam of a monochromatic light source, usually a laser. The light scattered by the particles at various angles it measured by a multi-element detector and numerical values relating to the scattering pattern are then recorded for subsequent analysis. These numerical scattering values are then transformed, using an appropriate optical model and mathematical procedure, to yield the proportion of total volume to a discrete number of size classes forming volumetric particle size distribution.
5 Laser diffraction instrument
In the conventional set-up, a light source(typically a laser) is used to generate a monochromatic, coherent, parallel beam. This is followed by a beam processing unit, usually a beam expander with integrated filter, producing a extended and nearly ideal beam to illuminate the dispersed particles.
A representative sample, dispersed at an adequate concentration is passed through the light beam in a measuring zone by a transporting medium (gas or liquid); this measuring zone should be within the working distance of the lens used. Sometimes, the particle stream in a process is illuminated directly by the laser beam for measurement, as in
the case of sprays, aerosols and air bubbles in liquids. In other cases (such as emulsions, pastes and powders), representative samples can be dispersed in suitable liquids (see annex C). Often dispersants (wetting agents: stabilizers) and /or mechanical forces (agitation; ultrasonication) are applied for deagglomeration of particles and stabilization of the dispersion. For these liquid dispersions a recirculating system is most commonly used, consisting of an optical measuring cell, a dispersion bath usually equipped with stirrer and ultrasonic elements, a pump and tubing.
Dry powders can also be converted into aerosols through application of dry powder dispersers, which apply mechanical forces for deagglomeration. Here a dosing device feeds the disperser with a constant mass flow of sample. The disperser uses the energy of a compressed gas or the differential pressure to a vacuum to disperse the particles It outputs an aerosol that is blown through the measuring zone, usually into the inlet of a vacuum pipe that collects the particles.
There are two positions in which the particles can enter the laser beam. In the conventional case the particles enter the parallel beam before and within the working distance of the collecting lens [see Figure 3-a]. In the so-called reversed Fourier optics case the particles are entered behind the collecting lens and, thus, in a converging beam [see Figure 3-b].
The advantage of the conventional set-up is that a reasonable path length for the sample is allowed within the working distance of the lens. The second set-up allows only small path lengths but enables measurement of scattered light at larger angles, which is useful when submicrometre particles are present.
The interaction of the incident light beam and the ensemble of dispersed particles results in a scattering pattern with different light intensities at various angles (see annex a for theoretical background of laser diffraction), the total angular intensity distribution I(θ),consisting of both direct and scattered light, is then focused by a positive lens or an ensemble of lenses onto a multi-element detector. The lens(es) provide(s) for a scattering pattern which, within limits, is not dependent upon the location of the particles in the light beam. So, the continuous angular intensity distribution I(θ) is converted into a discrete spatial intensity distribution I(r) on a set of detector elements.
It is assumed that the recorded scattering pattern of the particle ensemble is identical to the sum of the patterns from all individual single scattering particles presented in random relative positions. Note that only a limited angular range of scattered light is collected by the lens(es) and, thus, by the detector.
The detector generally consists of a number of photodiodes; some instruments apple one photodiode in combination with moving slits. The
photodiodes convert the spatial intensity distribution I(r) into a set of photocurrents I n, subsequent electronics then convert and digitize the photocurrents into a set of intensity or energy vectors L n. Representing the scattering pattern. A central element measures the intensity of the non-scattered light and, thus, with a calculation, provides a measure of optical concentration or obscuration. Some instruments provide special geometries of the central element in order to automatically re-centre or re-focus the detector by moving the detector or the lens. It is desirable that the detector elements are positioned so as to prevent the light reflected from the surface from re-traversing the optical system.
A computer controls the measurement and is used for storage and manipulation of the detected signals, for storage and/or calculation of a proper form of the optical model (usually as a model matrix containing light scattering vectors per unit of volume per size class, scaled to the detector’s geometry and sensitivity) and calculation of the particle size distribution (see annex a for theoretical background of laser diffraction). Also it may provide automated instrument operation.
Several significant differences exist, both in hardware and software, not only between instruments from different manufacturers but also between different types from one company. The instrument specifications should give adequate information for proper judgement of these differences. In annex B recommendations are presented for the specifications of laser diffraction instruments.
6 operational procedures
6.1 requirements
6.1.1 instrument location
The instrument should be located in a clean environment that is free from excessive electrical noise, mechanical vibration, and temperature fluctuations and is out of direct sunlight. The operating area should be well ventilated. The instrument should either contain a rigid internal optical bench or be installed on a rigid table or bench to avoid realignment of the optical system at frequent intervals.
Warning:the radiation of instruments equipped with a low power laser can cause permanent eye damage. Never look into the direct path of the laser beam or its reflections. Avid cutting the laser beam with reflecting surfaces. Observe the local laser radiation safety regulations.
6.1.2 dispersion liquids
Any optically transparent liquid of known refractive index may be used. Thus, a variety of liquids is available for preparation of liquid dispersions of powders. Annex c provides requirements for the dispersion liquids.
If an organic liquid is used for dispersion, observe the local health and safety regulations. Use a cover for the ultrasonic bath when using liquids with a high vapour pressure in order to prevent the formation of hazardous vapour concentrations above the bath and/or the generation of low-temperature zones with fluctuating refractive indices in the fluid by evaporation.
6.1.3 dispersion gases
For dry dispersion and spray applications a compressed gas is sometimes used. If used, it is essential that it is free from oil, water and particles. To achieve this, a dryer with a filter is required. Any vacuum unit should be located apart from the measurement zone, so that the output of the hot air does not reach the measuring zone, draught should be avoided in order to avoid unstable particulate streams.
6.2 Sample inspection, preparation, dispersion and concentration
6.2.1 sample inspection
Inspect the material to be analysed, visually or with the aid of a microscope, firstly to estimate its size range and particle shape and later to check whether the particles have been dispersed adequately.
The size distribution measured in a sample is only valid for a batch of material if the sample is representative for that batch and dispersed adequately.
6.2.2 preparation
For dry powders, prepare a representative sample of suitable volume for the measurement by an adequate sample splitting technique, for instance a rotating riffler. If very small samples are required, or in the case of wet powders, it is also possible to take fractional samples out of a well-mixed sample paste. The consistency of the paste then avoids segregation errors. The pastes are formed by adding dispersant to the sample drop by drop while mixing it with a spatula. As long as the mixture forms lumps, single drops should be added while continuing the mixing after each drop. A good consistency for the paste is one like honey or toothpaste. If the paste becomes too fluid by mistake, it shall not be used, and a new preparation should be initiated.
If the maximum size exceeds the measuring range, remove the material that is too coarse, e.g. by presieving. In this case determine and report the amount/percentage removed.
Sprays, aerosols and gas bubbles in liquid should be measured directly, provided that their concentration is at an adequate level (see
6.2.3 and 6.2.4) ,since sampling or dilution is generally impossible without altering the particle size distribution.
6.2.3 Dispersion
6.2.3.1 Dry powders can be dispersed either in air or in liquid. The dispersion procedure shall be adjusted to the purpose of the measurement,
e.g. It has to be decided whether agglomerates should be detected or broken down to the primary particles
6.2.3.2 An adequate dry disperser should be applied; here, generally compressed air or vacuum is applied for dispersion by shear stress with the assistance of mechanical de-agglomeration by particle-particle or particle-wall collisions(see figure 4). For dry dispersion, the complete fractional sample shall be used for the measurement. Note that the use of large sample quantities can overcome the poor statistical representation of coarse particles in a wide size distribution. It is necessary to check that comminution of the particles does not occur and conversely that a good dispersion has been achieved. This is usually done by direct comparison of dry dispersion with a liquid one: ideally, the results should be the same. Another possibility for checking the degree of dispersion or comminution is by changing the dispersing energy (e.g. The primary air pressure) and monitoring the change of the size distribution. Usually upon increasing the dispersing energy the amount of fines is increased at first, due to improved dispersion, until a plateau is reached, where the size distribution is nearly constant with increasing energy. At still higher energies the amount of fines may rise again as a result of comminution. On some occasions, agglomeration has been found at high flow rates through a cascade. The centre of the plateau define the optimum dispersing energy. Note, however, that a plateau is not always found (for instance for highly aggregated or fragile particles).
6.2.3.3 For the preparation of liquid dispersions a variety of liquids is available. Annex C presents requirements and some advice. Generally, pasting, stirring and ultrasonication can be used to facilitate proper dispersion of particles in the liquid. A preliminary check on the dispersion quality can be made by visual/microscopic inspection of the suspension. Also, it is possible to perform some measurements of the suspension in the laser diffraction instrument, with intermediate ultrasonication: the measured size distribution should not change significantly if the sample is well dispersed and the particles are neither fragile nor soluble.
The minimum volume of sample , required for repeatable measurement, increases as the width of the size distribution becomes greater in order to allow a sufficient number of large particles to be present. Accordingly, the volume of the dispersion fluid required to suspend these samples also increases if the limits of optical concentration are to be observed.
For example, for a sample with particles in the approximate size range of 2μm to 200μm, a sample volume of at least 0.3 ml is needed. This will require at least 500ml of suspension fluid for its dispersion. Also, the measurement time or the number of detector readings within
one measurement should be sufficient to reach a reasonable precision. Appropriate measurement conditions should be established experimentally, in relation to the desired precision.
6.2.4 Concentration
The particle concentration in the dispersion should be above a minimum level, which for many instruments will correspond to about 5% obscuration, in order to produce an acceptable signal-to-noise ratio in the detector. Likewise, it should be below a maximum level, which for many instruments will correspond to about 35% obscuration for particles larger than about 20μm, in order to avoid multiple scattering (where light is scattered subsequently at more than one particle).
For particles smaller than about 20μm, the obscuration value should be kept below about 15% for the same reason. In general, multiple scattering appears at larger scattering angles. Without multiple scattering correction, the amount of fines calculated will exceed the true value. If work at higher concentrations is required, it should be possible to correct for multiple scattering or systematic errors will arise. A first estimate for the concentration can be reserved from figure 5.
Figure 5, though only an example, shows that the optimum particulate concentration is nearly proportional to the circle size: smaller particles require lower concentrations. For instance, particles with a diameter of about 1μm fire volumetric concentrations during measurement of about 0.002%, whereas the concentration for 100μm circles should be about 0.2%, in a cell with a 2mm path length. As a consequence, the width of the particle size distribution influences the optimum sample concentration for measurement. Moreover, the range of concentrations, shown in figure 5, is influenced by the laser beam width, the path length of the measurement zone, the optical perties of the particles and the sensitivity of the detector elements.
New of the above, measurements should be performed at different particulate concentrations in order to decide be optimum concentration range for any typical sample of material.
6.3 measurement
6.3.1 procedure
Optical measurement of a particle size distribution by laser diffraction comprises the following steps:
a ) setting up instrument and blank measurement
After selection of the appropriate particle size range and proper alignment of the optical part of the instrument. The blank measurement is performed in which a particle-free dispersion medium is used. Detector data are saved in order to subtract them later from the data obtained with that sample in order to obtain net sample signals.
b ) measurement the scattering pattern of dispersed sample(s)
Generally, a measuring time allowing for a large number of detector scans or sweeps at short time intervals is used: typically some 2 seconds or 1000 sweeps. For each detector element an average signal is calculated, sometimes together with its standard deviation. Data are store in the computer memory. The magnitude of the signal from each detector element depends upon the detection area, the light intensity and the quantum efficiency. The coordinates (size and position) of the detector elements together with the focal distance of the lens determine the region of scattering angles for each element. Generally all these factors are factory determined and stored in the computer.
Most instruments also measure the intensity of the central laser beam. The fractional difference between a dispersed sample and a blank experiment is given as an obscuration value, which is indicative of the total amount of scattered light and the particle concentration.
c ) selection of an appropriate optical model
Most often either the fraunhofer or the Mie theory is used.
Sometimes other approximating theories are applied for calculation of the scattering matrix. When using the Mie theory, the refractive indices of particulate and medium, or their ratio, should be brought into the instrument in order to allow calculation of the model matrix (see annex D for the refractive indexes of liquids and solids). Often , small values of the imaginary part of the refractive index (about 0.01-0.1) are applied to cope with the surface roughness of the particles.
NOTE: small differences in the assumed complex refractive index may cause significant differences in resulting particle size distributions..
In order to obtain traceable results it is essential that the refractive index values used are reported
d ) conversion of scattering pattern into particl
e size distribution
This deconvolution step is the inverse of the calculation of a scattering pattern for a given particle size distribution. The fact that short measured data always contain some random and systematic errors, may cause erroneous size distribution results. Several mathematical procedures have been developed for use in the different instruments available [4, 6, 7, 10, 12, 14]. They contain some weighting of deviations between measured and calculated scattering patterns (e.g. least squares), some constraints (e.g. Non-negativity for amounts of particles) and/or some smoothing of the size distribution curve. A new procedure [5] uses the observed fluctuations of the detector signals to introduce proper weighting of these data and to calculate confidence intervals for the particle size distribution.
6.3.2 Precautions
Before starting, and during any measurement, the instructions given in the instrument manual should be followed. The following precautions should be taken.
a ) Before switching on the power to the instrument make sure that all components of the system are properly grounded. It is essential that all the particle dispersing and transporting devices, such as the ultrasonic bath, the dry disperser, the vacuum inlets and vacuum hoses, are earthed to prevent ignition of organic solvents or dust explosions caused by electrostatic discharges.
b ) After switching the power on, allow sufficient time for the instrument to stabilize. Gas lasers such as the He-Ne laser usually have a warm-up time of more than half an hour.
c) Check the instrument status and , if necessary, set up the required measuring range and lens. Ensure, by watching the intensities on the detector, that the detector is properly centred and positioned in the focal plane of the lens. Without particles. the background signal should be below the specified thresholds for that instrument set-up and dispersing device. If this is not the case, inspect and, if necessary, clean the optical components to ensure proper performance.
d ) Mak
e sure that the particles are only introduced into the laser beam within the specified working distance o
f the lens, so that all relevant scatterin
g radiation leaving the particles strikes within the clear aperture of the lens that focuses it on the detector (and thus, vignetting is avoided).
Validate the instrument operation with respect to both precision and accuracy at regular time intervals by measuring a control sample of known size distribution (see 6.4 and 6.5.2).
e ) In the case o
f wet dispersion, check that air bubbles are absent in the dispersion liquid. Foamin
g detergents should be avoided.
In the case of dry dispersion, check, visually or by inspection of subsequent obscuration values, that the dosing unit for the disperser generates a steady mass flow.
f ) For aerosols and sprays: make sure that no bright daylight is allowed, either directly or via scatterin
g by particles, into the detector and that the flow of particles/droplets is even.
Investigate, if possible, the influence of the optical model (relative refractive index) on the resulting particle size distribution, especially if a significant fraction of the particles is smaller than about 10μm.
NOTE occasionally a strong dependency of the results on the refractive index has been found whereby even slightly different values resulted in major systematic errors (see further annexes A and D ).
6.4 repeatability
For samples where the coefficient of variation of the particle size distribution is equal to or less than about 50%(or fluo of diameter of largest to smallest particle about 10:1(或者最大最小颗粒直径之比为10:1)) and for measurements performed on at least five different samples from the same batch in the mid-range of any instrument setting, the repeatability of characteristic particle –es in size distributions should be as follows: for any chosen central value of the distribution, e.g. The median size (x0), the coefficient of variation should be smaller than 3%. Values at the sides of the distribution, e.g. X10 and x90, should have a coefficient of variation not 5%. Below 10μm, these maximum values should be doubled.
6.5 accuracy
6.5.1 calibration
User diffraction systems are based on first principles, though with idealized properties of the particles(cf. Annex Z). Thus, calibration in the strict sense is not required. However, it is still necessary and desirable to confirm the correct –ration of the instrument by a validation procedure (see 6.5.2).
6.5.2 validation
6.5.2.1 primary validation can be made with any certified or standard reference material, acceptable to the practice zone end-users’ industries. Here, the total measurement procedure is being examined, including sampling, sample dispersion ,sample transport through the measuring zone, measurement and deconvolution procedure [13]. It is essential that the total operational procedure is adequately described in full detail.
Citified or standard reference materials consisting of a known distribution having a range of spherical particles – one decade of size are preferred. They should be certified to mass percentage by an absolute technique, if –lable, and used in conjunction with an agreed, detailed operation procedure. It is essential that the real and –rinary part of the complex refractive index are precisely specified for the material if the Mie theory is applied in the analysis.
Response of a laser diffraction instrument is considered to meet this standard if the mean value of the x50–ing from at least three independent measurements deviates less than 3% from the certified range of values of the Certified or Standard Reference Material, i.e. The mean value together with its standard deviation; the mean –es for the x10 and x90 should deviate less than 5% from the certified range of values.
Through use of spherical reference materials is preferable, non-spherical ones may also be used. Preferably, these should have certified or typical values coming from laser diffraction analyses according to an agreed, detailed –ational procedure. If the Reference values come from
other methods than laser diffraction, a significant bias may –t. The reason for this bias is that the different principles applied in the various methods may lead to different sensitivity to the properties of the particles and, thus, to different equivalent-sphere diameters for the same non-rical particle.
Addition to the certified reference materials mentioned above, product samples of typical composition and particle distribution for a specified class of products can also be applied for validation of instrument behaviour and operational procedures, provided that their particle size distribution has been proven to be stable over time. Here, the results should comply with previously determined data with the same precision and bias as those for the certified reference materials.
Mixtures in ratios of volume of two or more reference materials having the same properties can be applied to test the accuracy of the reported fractional quantities, the size resolution and the sensitivity to fines or coarse material. Representative sampling from the various materials is here, however, even more important than in the normal case, since the fractional quantities can be very small.
6.5.2.2 For secondary validation of a laser diffraction instrument a suitable reference reticle [1, 8, 9] can be used. Thus, only the quality of instrument optics and software is being examined, leaving out the effects of sample dispersion and handling. For instruments with a focal length above 300mm, the application of reticles is not reliable due to speckle effects. Of course, the proper use of a reticle includes the requirement that the illuminating beam diameter allows measurement of the full circular area of the dots deposited. Note that some reverse Fourier applications, where the reticle must be placed close to the detector, may fall within this restriction. The response of a laser diffraction instrument is considered to meet the requirements of this part of ISO13320 if the mean value for the x50 coming from at least three measurements deviates less than 2% from the quoted value and for x10 and x90 less than 3%.
6.6 Error sources; diagnosis
6.6.1 Systematic measurement errors (bias) may arise from improper sample preparation, departure from the theoretical assumptions for the particulate mater and/or improper operation or functioning of the instrument.
6.6.2 Errors made in sample preparation are often a main part of the total error. They can be attributed to the following causes:
-Improper sampling technique, leading to a non-representative sample in the measurement zone; this type of error is especially
significant when using an inadequate sample splitting technique
in the case of a large batch of free flowing material having a
wide size distribution, but errors can also be due to selective
transport within the instrument, for example, application of too
low a pumping speed may lead to sedimentation of the larger
particles in the pumping circuit;
-Incomplete deagglomeration of particles, due to an improper dispersion procedure (liquid; dispersant; ultrasonication);
-Comminution of particles by mechanical forces during
dispersion (e.g. Ultrasonication);
-Swelling, re-agglomeration, dissolution or evaporation of particles/droplets before or during measurement;
-Inclusion of air bubbles due to foaming dispersants and/or vigorous stirring;
-Scattering from differences in refractive index in the dispersing liquid or gas due to temperature fluctuations generated by, for
example, evaporation of the dispersing liquid or presence of a
flame.
6.6.3 Another main source for bias arises from the departure from the theoretical assumptions for the particulate material. Again, the errors can come from different sources.
-Firstly, most particles in real life do not fulfil the assumption of sphericity. Non-sphericity of particles leads to different cross-
sections in different orientations. since particles are generally
measured in all possible orientations, this leads to some
broadening of the particle size distribution as compared to the
equivalent volume distribution. Moreover, the median and
mean diameter may be shifted, often to a larger size.
-Secondly, the particle surface may be rough instead of smooth.
This causes diffuse light scattering at the boundary, which often
has a similar influence as absorption of light within the particle.
-Thirdly, the particles may be optically heterogeneous, as is the case for porous particles. This may lead to an apparent presence
of significant amounts of very small particles, which are non-
existent.
-Last but not least, the wrong optical model or parameters may have been chosen. For instance, if the Fraunhofer
approximation is applied for samples containing an appreciable
amount of small, transparent particles a significantly larger
amount of small particles may be calculated (see also annex A ).
Generally, the choice of a wrong model also results in a large
difference between particulate concentration as calculated by
the instrument and from the mass of sample and the volume of
dispersion medium (see also 6.6.6).
6.6.4 errors in the operational procedure or in the functioning of the
instrument can be specified as follows:
-particles with diameters outside the measuring range are present [in this case, adapt the measuring range (change the lens) and/or remove too coarse material, e.g. by presieving ];
-the sample is introduced into the laser beam outside the working distance of the lens;
-lens(es) or windows of the measurement cell are dirty and, thus, should be cleaned;
-measurements are conducted with excessive levels of background;
-the optical system has not been aligned properly;
-the particle concentration is too high, causing multiple scattering;
-the mathematical procedure for deconvolution of light intensity values to particle size distribution is inadequate (check with the instrument manufacturer).
6.6.5 the absence of maintaining good control on the above points may also lead to errors of a random nature. Moreover, random errors may result from
-using insufficient measurement time, or readouts of each detector output
-working at too low a concentration, and
-instrument imperfections, e.g. fluctuating laser intensity, or noisy detector elements.
6.6.6 errors of specific parts of the procedure can be diagnosed by the following operations.
-Measuring the intensity of the laser beam for at least 1h during
a blank experiment: it should be stable within the limits given
in the instruction manual.
-Observing the signals from all detector elements during a blank measurement: the background signal should show a smooth
behaviour with only small positive or zero values. Negative or
overload (100%) readings indicate faulty detector elements,
defects in the electronics or scratched cell windows or lenses.
Significant intensities on only localized detector elements are
often caused by reflections at damaged optical surfaces of the
lens, the cuvette or other parts illuminated by the laser beam;
-Observing the detector signals from repeated sample measurements, calculating both mean values for each element
and their standard deviations and comparing the measured
signals for all detector elements with previously measured ones.
Thus, an impression is gained of the precision and accuracy of
these signals: large systematic differences or zero values for the signals usually indicate a faulty detector element, a defect in the
electronics, dirty windows or lenses, bad alignment, presence of air bubbles or a problem in the sampling and/or dispersion
procedure. Moreover, these data enable, after conversion to
light intensity values, comparison of the measured light
scattering patterns coming from different instruments, to some
extent irrespective of the geometry of the detector elements in
these instruments (provided that the geometry is known);
-Comparing the actual particulate concentration, as calculated from the sample mass and the volume of the dispersion medium, with a calculated one from the obscuration and particle size
distribution, while using the same optical model for calculating
the extinction coefficients of the particle size fractions as for
the deconvolution; a large difference indicates an inadequate
optical model or bad sample handling [1];
-Comparing for all detector elements the measured and calculated signals (for the resulting, best-fitting particle size
distribution); large systematic differences indicate either a
faulty element or an inadequate optical model.
6.7 resolution; sensitivity
The resolution of the particle size distribution, i.e. the capability to differentiate between different particle sizes, and the sensitivity for small(extra) amounts of particles of certain size are restricted by the following factors:
-number, position and geometry of the detector elements;
-their signal to noise ratio;
-fine structure in the measured scattering pattern;
-difference in scattering pattern between size classes;
-actual size range of the particulate material;
-adequacy of the optical model;
-smoothing applied in the deconvolution procedure.
These factors prevent the laser diffraction technique in its usual design from being a high resolution technique: the minimum width of each size class is usually about 1.1 to 2 (ratio of upper to lower limit of the size class). Actual values for resolution and/or sensitivity for quality control reasons should be determined by using mixtures of known composition.
7 reporting of results
Results should be reported in accordance with ISO 9276-1. Moreover, the following information should be reported in order that the measurements can be readily repeated by different operators in different laboratories. Some of items may be optional for some materials.
激光粒度测定仪
目的:建立马尔文Mastersizer2000湿法激光粒度仪及Mastersizer2000工作站操作规程,以便更好的使用该仪器。 范围:用于马尔文Mastersizer2000湿法激光粒度仪的使用、维护和保养。 依据: 责任:药分部 内容: 1.操作规程 1.1仪器组成 马尔文Mastersizer2000激光粒度仪主机,湿法进样器1(Hydro 2000SM,油相)、湿法进样器2(Hydro 2000MU,水相)联想电脑、打印机组成,各部件相互联接,各有开关。 1.2 开机 先开仪器主机和湿法进样器,再开电脑,仪器需要预热15到30分钟。 1.3 SOP设置(湿法) 1.3.1点击配置菜单,选择新建SOP,会弹出一个新窗口。 1.3.2点击下一步,在样品处理单元找到所使用的进样器类型,如水相选择Hydro MU2000A,油相选择Hydro 2000SM(A)),然后点击下一步。 1.3.3在物质名称、分散剂名称选择要测试的样品和分散剂,设置光学参数值。模型处选择通用模式,然后确定,再点击下一步。 1.3.4标记处的样品名称下留空白,然后操作者说明和注解处,选择测量前输入。 1.3.5报告/保存处不选择任何设置 1.3.6样品测量时间和背景测量时间分别设置6秒和8秒。在高级选项测量中的遮光度界限设置中使用默认限制(10~20),再点击下一步。 1.3.7进样器设置,转速设置,通过手动调节转速(湿法设置)。 1.3.8重复测量循环设置3~5次,然后完成,保存时输入新的SOP名称。 1.4测量 1.4.1湿法SOP测量 点击测量项下的启动SOP,选择所需的SOP名称;进入测量窗口,然后测量窗口上的点击“对光”,对光好后,如果背景状态正常,就不需要换分散剂了(如果是第一次打开软件的话,对光按键是隐藏在测量背景下面的,只要点击“开始”键,仪器就会对光接着测量背景的)。 1.4.2手动测量
激光粒度仪讲解
激光粒度仪测定粒度分布组成 一、试验目的 本实验目的是测定粒子尺寸及粒度大小分布,通过试验了解激光粒度仪的工作原理及组成,学习激光粒度仪的使用及操作;掌握分布曲线所显示的粒度大小及分布情况。颗粒及颗粒行为是无机非金属材科研究的基础。因此,颗粒的表征和颗粒的测试具有同样的重要性。粉体的粒度是颗粒在空间范围所占大小的线性 尺度。粒度越小,粒度的微细程度越大。颗粒群是指含有许多颗粒的粉体或分散体系中的分散相。若颗粒进度都相等或近似相等,称为单进度或单分散的体系或颗粒群。实际颗粒所含颗粒的粒度大都有一个分散范围,常称为多进度的、多谱的或多分散的体系或颗粒群。粒度分布是表征多分散体系中颗粒大小不均一程度的。粒度分布范围越窄,其分布的分散程度就越小,集中度也就越高。 粒度分布测量中分为频率分布和累积分布。累积分布横坐标表示各粒级的粒度;纵坐标表示在某Df以下的颗粒所占总颗粒的个数或质量百分数。通过粒度 分布曲线分析所显示的粒度大小和粒度大小分布,了解材料的研磨情况,推断出材料粒度不同其性能不同。同时可以反映出材料性能不同与材料颗粒粒径的大小 有关系。 二、试验仪器 RISE—2008型激光粒度分析仪,1000ml烧杯二只,试样若干种类 三、试验原理 根据光学衍射和散射的原理,从激光器发出的激光束经显微物镜聚集,针孔滤波和准直后,变成直径约10mm的平行光束,该光束照射到待测的颗粒上,就 发生了散射,散射光经傅立叶透镜后,照射到光电探测器上的任一点都对应于某一确定的散射角,光电探测器阵列由一系列同心环带组成,每个环带是一个独立的探测器,能将投射到上面的散射光线形地转换成电压,然后送给数据采集卡, 该卡将电信号放大,再进行AID转化后送入计算机。Rise-2008型激光粒度仪依据全量程米氏散射理论,充分考虑到被测颗粒和分散介质的折射率等光学性质, 根据激光照射在颗粒上产生的散射光能量反演出颗粒群的粒度大小和粒度分布 规律。
马尔文激光粒度仪(MS2000)操作规程-干湿法
马尔文MS2000操作规程 一.开机顺序:先开仪器主机和湿法或干法进样器,再开电脑,仪器需要预热15到30分钟。 关机顺序:先关电脑软件,再关湿法或干法进样器和仪器主机。 二.湿法测量程序: a)手指轻轻按键控制面板第一个显示中间的on/off键盘,让水循环起来。 b)在桌面上双击Mastersizer2000操作软件,进入操作软件,输入操作者姓 名,然后鼠标左键点击确定。 c)在文件那里点击打开,打开已有的文件或新建一个文件,确保记录存放 在你所需要的文件名下。 d)单击“测量”菜单中的“手动”按钮,进入测量窗口。 e)然后点击“对光”,对光好后,如果背景状态正常,就不需要换水了(如 果是第一次打开软件的话,对光按键是隐藏在测量背景下面的,只要点 击“开始”键,仪器就会对光接着测量背景的)。 f)然后进入“选项”菜单,选择合适的光学参数,在“物质”那里选择好 催化剂,或者新鲜催化剂,再进入“文档”菜单,输入样品名称,然后 “确定”退出。 g)然后单击“开始”按钮,系统开始测量背景,当背景测量完成以后并提 示“加入样品”后,开始加入样品到遮光度10%,到控制的遮光度范围 内,然后单击“开始”或按“测量样品”仪器会进行测量样品。每测量 一次,结果会按记录编号和时间存在已经指定的文件里。 h)测量结束后,抬起烧杯上方盖子到两个黑线中间,附近会自动把样品池 的水排除,然后换新鲜的水并清洗两到三次(以背景正常为准)。三.干法测量步骤:干法测量可用SOP(标准操作规程)来进行测量。 1.把样品放入干法进样器的样品盘中 2.点击测量窗口中的“启用SOP” 3.选择已经设置好的SOP 4.根据仪器运行SOP提示输入样品的编号
欧美克LS-POP激光粒度分析仪作业指导书
1. 目的: 为了规范对激光粒度分析仪的操作使用,从而确保产品粒度检验结果的正确性、真实性、可靠性,特制定本文件。 2. 内容: 2.1 工作原理 利用颗粒对光的散射现象,根据散射光能的分布推算被测颗粒的粒度分布。 2.2 技术指标 测试范围:0.2~500μm 进样方式:湿法,循环进样器和静态样品池 重复性误差:<3% 测试时间:1-2分钟 独立探测单元数:32 光源种类:氦-氖激光 功率:2.0 mW 波长:0.6328 μm 2.3工作环境 2.3.1 仪器应安装在洁净、少尘、无烟、带空调的环境中。仪器的组件中含有激光管、光学镜头、针孔和测量窗口等。这些光学部件如果受到灰尘、油脂、石油产品或其他有害物质的侵蚀,将会造成光洁度下降、腐蚀、堵塞、功率下降等损害。 2.3.2 室温要稳定,没有明显的气流,没有直射阳光,否则会引起激光功率不稳,光束准直欠佳和外界杂散光的干扰,从而造成测量的重复性下降。 2.3.3 ,仪器的工作环境要求温度在5-35℃之间,空气湿度不可高于85% ,否则光学镜头表面可能会结露,致使光线不能聚焦,时间长了还会使镜头发霉。 2.3.4 地面不能有明显的震动,否则会导致光路系统偏移,引起测量结果异常。 2.3.5 电源电压220V,50/60HZ,有三头插座且接地线良好。 2.3.6严禁将零线和地线合接。 2.3.7本仪器的接地线不可与其他地线专用。 2.4 输出项目 粒度分布表、粒度分布曲线、平均粒径、中位径、比表面积等。
2.5 相关名词解释 2.5.1 粒径:又称颗粒尺寸,用以表征颗粒的大小。除了球形颗粒这一特例外,粒径并不是真实的物理尺寸,而是会随测量原理变化的等效尺寸。在激光散射法技术中,粒径是指与待测颗粒有相同的化学性质并有最相近的光散射特性的球形颗粒(组合)的直径(分布)。 2.5.2 粒度分布:是指一个粉体样品中各种粒径的颗粒所占的比例。因为任何一个粉体样品都是由大小不同的颗粒组成的,所以用粒度分布才能确切地描述其粗细情况。 2.5.3 悬浮介质:测量粒度时需要把样品分散在液体或气体中。这里的液体或气体就称为悬浮介质。合适的悬浮介质应该是既能让样品在其中分散,又不让样品在其中分解或发生化学反应的。 2.5.4 光能分布:即散射光的能量分布,就是照射到粒度仪各光电探测器上的散射光的能量。背景光能代表被光路上的尘埃粒子或各光学镜面的疵点散射的光能分布;而样品颗粒的散射光能是被待测样品的颗粒散射的光能,其分布与样品颗粒的粒度相对应,但不等于粒度分布。 2.5.5 遮光比:指测量用的照明光束被测量的样品颗粒阻挡的部分与照明光的比值。颗粒在测量介质中的浓度越高,则遮光比越大。 2.5.6 平均粒径:是指样品中所有颗粒的粒径的平均值,可以根据粒度分布计算而得。 2.5.7粒度分布宽度:用以表征样品粒径的均匀程度。粒度分布宽,表示样品颗粒的粗细不均匀;反之,则表示均匀。 2.6 准备阶段 2.6.1系统开机 打开电源开关 测量单元(预热半小时后进行下面步骤) 循环进样器 打印机 显示器 计算机主机 2.6.2 测量单元预热 2.6.2.1如关机超过半小时再重新开机,必须预热半小时。 2.6.2.2打开测量单元电源,半小时后,激光率才能稳定。如果环境温度较低,等待时间还要延长。 2.6.2.3判断激光功率是否达到稳定的依据是,背景光能分布的零环高度是否稳定。正常
激光粒度仪
激光粒度分析仪 摘要:激光粒度分析仪是根据光的散射原理测量粉颗粒大小的,是一种比较通用的粒度仪。其特点是测量的动态范围宽、测量速度快、操作方便,尤其适合测量粒度分布范围宽的粉体和液体雾滴。对粒度均匀的粉体,比如磨料微粉,要慎重选用。 激光粒度仪集成了激光技术、现代光电技术、电子技术、精密机械和计算机技术,具有测量速度快、动态范围大、操作简便、重复性好等优点,现已成为全世界流行的粒度测试仪器。 关键字:工作原理系统构成参数 引言:在工农业生产和研究中,很多原材料和产品都以粉体的形态存在着,粉体在生产中占有举足轻重的地位。粉体的粒度分布可以影响到产品的质量和性能,因此,在粉体行业,有效控制与测量粉体的粒度分布,对提高产品质量、降低能源消耗、控制环境污染、保护人类的健康具有重要意义。 粒度测试仪器是用物理的方法测试固体颗粒的大小和分布的一种仪器,在建筑、涂料、石油、医药、环保、食品等领域有广泛地应用。粒度仪根据测试原理的不同分为沉降式粒度仪、沉降天平、激光粒度仪、光学颗粒计数器、电阻式颗粒计数器、颗粒图像分析仪等。其中,激光粒度仪作为一种以激光作为探测光源的新型粒度测试仪器,因其测试速度快、测试范围宽、重复性和真实性好、操作简单等特点,已经在粉体加工、应用与研究领域得到广泛应用。 激光粒度分析仪是根据光的散射原理测量粉颗粒大小的,是一种比较通用的粒度仪。其特点是测量的动态范围宽、测量速度快、操作方便,尤其适合测量粒度分布范围宽的粉体和液体雾滴。对粒度均匀的粉体,比如磨料微粉,要慎重选用。 激光粒度仪集成了激光技术、现代光电技术、电子技术、精密机械和计算机技术,具有测量速度快、动态范围大、操作简便、重复性好等优点,现已成为全世界流行的粒度测试仪器。
马尔文激光粒度仪简介
laParticle size analysis-Laser diffraction methods (ISO-13320-1) Introduction Laser diffraction methods are nowadays widely used for particle sizing in many different applications. The success of the technique is based on the tact that it can be applied to various kinds of particulate systems, is fast and can be automated and that a variety of commercial instruments is available. Nevertheless, the proper use of the instrument and the interpretation of the results require the necessary caution. Therefore, there is a need for establishing an international standard for particle size analysis by laser diffraction methods. Its purpose is to provide a methodology for adequate quality control in particle size analysis. Historically, the laser diffraction technique started by taking only scattering at small angles into consideration and, thus, has been known by the following names: -fraunhofer diffraction; -(near-) forward light scattering; -low-angle laser light scattering (LALLS). However, the technique has been broadened to include light scattering in a wider angular range and application of the Mie theory in addition to approximating theories such as Fraunhofer and anomalous diffraction. The laser diffraction technique is based on the phenomenon that particles scatter light in all directions with an intensity pattern that is dependent on particle size. All present instruments assume a spherical shape for the particle. Figure 1 illustrates the characteristics of single particle scattering patterns: alternation of high and low intensities, with patterns that extend for smaller particles to wider angles than for larger particles[2-7,10,15 in the bibliography]. Within certain limits the scattering pattern of an ensemble of particles is identical to the sum of the individual scattering patterns of all particles present. By using an optical model to compute scattering for unit volumes of particles in selected size classes and a mathematical deconvolution procedure, a volumetric particle size distribution is calculated, the scattering pattern of which fits best with the measured pattern (see also annex A).
激光粒度仪实验报告
实验一LS230/VSM+激光粒度仪测定果汁饮料粒度 1实验目的 了解激光粒度仪的基本操作; 了解激光粒度仪测定的基本原理。 2实验原理 激光粒度分析仪的原理是基于激光的散射或衍射,颗粒的大小可直接通过散射角的大小表现出来,小颗粒对激光的散射角大,大颗粒对激光的散射角小,通过对颗粒角向散射光强的测量(不同颗粒散射的叠加),再运用矩阵反演分解角向散射光强即可获得样品的粒度分布。 激光粒度仪原理图如图1所示,来自固体激光器的一束窄光束经扩充系统扩充后,平行地照射在样品池中的被测颗粒群上,由颗粒群产生的衍射光或散射光经会聚透镜会聚后,利用光电探测器进行信号的光电转换,并通过信号放大、A/D 变换、数据采集送到计算机中,通过预先编制的优化程序,即可快速求出颗粒群的尺寸分布。 3实验试剂与仪器 实验样品:果汁饮料。 实验仪器:LS230/VSM+激光粒度仪。 4实验步骤 按照粒度仪、计算机、打印机的顺序将电源打开,并使样品台里充满蒸馏水,开泵,仪器预热10分钟。
进入LS230的操作程序,建立连接,再进行相应的参数设置: 启动Run-run cycle(运行信息) (1)选择measure offset(测量补偿),Alignment(光路校正),measure background(测量空白),loading(加样浓度),Start 1 run(开始测量(2)输入样品的基本信息,并将分析时间设为60秒,点击start(开始)。 如需要测量小于μm以下的颗粒,选择Include PIDS,并将分析时间改 为90秒后,点击start(开始) (3)泵速的设定根据样品的大小来定,一般设在50,颗粒越大,泵速越高,反之亦然。 在测量补偿,光路校正,测量空白的工作通过后,根据软件的提示,加入样品控制好浓度,Obscuration应稳定在8-12%:假如选择了PIDS,则要把PIDS稳定在40-50%,待软件出现ok提示后,点击Done(完成)。 分析结束后,排液,并加水清洗样品台,准备下一次分析。 作平行试验,保存好结果,根据要求打印报告。 退出程序,关电源,样品台里加满水,防止残余颗粒附着在镜片上。 5实验结果与讨论 实验结果 由实验结果显示: 平均粒径:μm
马尔文粒度仪MS2000使用心得
马尔文粒度仪MS2000使用心得(湿法进样) 今年4月下旬,我们安装了马尔文激光粒度仪,并进行了现场培训。经过几个月的粒度测定实际操作,现将感悟出来的一些心得总结如下: 1、取样 当然了,样品必须具有代表性、取样要按四分法或其他适宜方法缩分取样,这些化验员都知道,就不细说,注意细节就行;之所以再次提出取样问题只是强调取样是个重要而又容易被忽略的问题。 2、样品制备 由于我们采用的是湿法进样,样品的制备就存在一个分散问题,样品应制备成具有相对分散稳定性的样品。记得安装培训的时候,我们请安装工程师给我们做了一个样品,由于没有现成的方法、时间有限,工程师给我们简单的做了个演示,结果,样品分散情况不大好,容易产生凝聚、沉淀(遮光度在下降),样品也容易吸附在样品窗、硅胶管、烧杯、搅拌桨等附件上,激光强度下降很快。后来,我们做了分散剂浓度及样品浓度筛选实验,选择样品分散稳定的分散剂浓度及样品浓度后,样品不容易凝聚、沉淀了,激光强度下降慢了。 3、背景确定 好的背景是获得正确、准确结果的基础。影响背景测量的主要因素有:分散介质、气泡、灰尘、温度差、搅拌速度、超声、分散剂量、样品窗等。分散介质(包括分散剂)的选择:依据产品性能参照相关手册选择。比如说选择水吧,也不是越纯越好,越纯净的越容易脏嘛,也更容易溶进更多的气体,搅拌时容易产生气泡。新鲜蒸馏水就好。气泡:气泡源于分散介质,如果分散介质能脱一下气,效果会比较好,常用的脱气方法有减压抽滤、超声脱气、加热沸腾驱赶。湿法进样器配有超声处理器,方便,但是超声脱气效果最差,超声产生的气泡容易附着在搅拌桨、烧杯壁、进样管道及样品池窗上;建议用新蒸馏放冷到室温的蒸馏水。灰尘:灰尘无处不在,尽量避免在仪器室称量样品,以免产生更多粉尘、尽可能保持仪器室洁净,湿拖地、擦桌椅等;条件允许的话换鞋、用洁净室。温度差:仪器预热15~30分钟,分散介质、分散剂提前放进仪器室温度平衡,建议装个空调(空调要用起来喔)。搅拌速度:以能使样品不沉淀,又不产生气泡为准;若搅拌产生气泡,可以先停止搅拌,待气泡逸出后再启动搅拌。超声:建议用外置超声处理后再加到进样烧杯里。测量时不使用超声。分散介质的量:
激光粒度分析结果在形貌分析中的应用讲解
实验技术与方法 激光粒度分析结果在形貌分析中的应用 胡汉祥1,2,丘克强1 (1.中南大学化学化工学院,长沙410083; 2.湖南建材高等专科学校化学化工系,衡阳421008) 摘要:激光粒度分析仪通常只用于颗粒大小与分布的测定。通过比较粉体颗粒的激光粒度分 析与扫描电镜分析的结果,发现,激光粒度分析仪所测定的粒度分布函数同时包含了一些形貌分析信息。利用这些信息可为试样进一步作SEM测定创造了条件。关键词:粒度分布;形貌;分析方法 中图分类号:TB302.1文献标识码:A文章编 号:100124012(2006)THEIMAGINGINFORMAEGRAPH OFPARTICLTION 2,,QIUKe2qiang1 (1.SchoolofEngineering,CentralSouthUniversity,Changsha410083,China; 2.DepartmentofEngineering,HunanBuildingMaterialsCollege,Hengyang421008,China) Abstract:TheLaserParticlesSizersareoftenemployedtodeterminetheaverageparticlediamet erandthe particlesizedistribution.TherelationsbetweentheparticlesdistributiongraphandSEMimage softhepowdersweredescribedinthispaper.Authorproposedthatthedoublemodesofthepartic lesdistributionmayimplythetwo2dimensionalconstructionoftheparticle.ItisusefulforSEM ditermination. Keywords:Particlesizedistribution;Pattern;Analysismethod 1引言 常用于粒度测定的方法有X射线衍射法、BET测定法、激光粒度分布仪测定法及透射电镜与扫描电镜测定法。能直观提供形貌分析信息只有透射电镜与扫描电镜
如何判断和选择激光粒度分析仪
如何判断和选择激光粒度分析仪 阅读次数:535 文章日期:2003-5-12 22:03:13 以往的粒度分析方法通常采用筛分或沉降法。常用的沉降法存在着检测速度慢(尤其对小粒子)、重复性差、对非球型粒子误差大、不适用于混合物料(即粒子比重必须一致才能较准确)、动态范围窄等缺点。随着激光衍射法的发明,粒度测量完全克服了沉降法所带来的弊端,大大减轻了劳动强度及加快了样品检测速度(从半小时缩短到了1分钟)。 激光衍射法测量粒度大小基于以下事实:即小粒子对激光的散射角大,大粒子对激光的散射角小。通过散射角的大小测量即可换算出粒子大小。其依据的光学理论为米氏理论和弗朗霍夫理论。其中弗朗霍夫理论为大颗粒米氏理论的近似,即忽略了米氏理论的虚数子集,并且假定颗粒不透明;并忽略光散射系数和吸收系数,即设定所有分散剂和分散质的光学参数均为1,因此数学处理上要简单得多,对有色物质和小粒子误差也大得多。同样,近似的米氏理论对乳化液也不适用。 另外,根据瑞利散射定律,散射光的光强与颗粒直径的六次方成正比,与散射光的光源波长的四次方成反比。这意味着颗粒直径减少10倍,散射光强减弱100万倍!而光源波长越短,散射光强度越高。 再者,由于小粒子散射角大,而主检测器面积有限,一般只能接受到最多45度角的散射光(即大于0.5微米的粒子)。那么,如何检测小粒子,如何克服小粒子光散射能量低,超出主检测器范围的问题,就成为评价激光粒度分析技术的关键。 所以,判断激光粒度分析仪的优劣,主要看其以下几个方面: 1 粒度测量范围粒度范围宽,适合的应用广。不仅要看其仪器所报出的范围,而是看超出主检测器面积的小粒子散射(〈0.5μm〉如何检测。 最好的途径是全范围直接检测,这样才能保证本底扣除的一致性。不同方法的混合测试,再用计算机拟合成一张图谱,肯定带来误差。
英国马尔文激光粒度仪
英国马尔文激光粒度仪 仪器简介: Mastersizer 2000 粒度仪是马尔文仪器公司的最新激光衍射系统,技术先进,操作既简单又直观。采用模块化设计,配备一系列测量干湿样品的自动样品分散装置。采用内置的 SOP 系统进行控制,提供简便的开发和传输方法Mastersizer 系列激光粒度仪经过不断的发展,能够满足工业和学术界用户粒度测量的需要。Mastersizer 创造性地使用激光衍射技术,已成为世界上实验室粒度分析的首选产品。它可以精确、无损伤地测量从亚微米到几毫米的范围广泛的颗粒粒度,湿法和干法分散均可使用。 主要特点: 1,准确性和重复性 精度:根据马尔文质量审核标准, Dv50具有± 1% 的精度。仪器到仪器的重复性:根据马尔文质量审核标准, Dv50的重复性优于 1% RSD。 2,重复性保证 由软件驱动的 SOP 消除了用户间的差异,并且可以全面共享。所有测量参数自动嵌入结果文件,并可以通过电子邮件使收件人审阅。测量可以通过遵循同样的 SOP而重复出来。 3,广泛的测量范围 测量物质从0.02μm 到2000μm。 4,广泛的样品类型 适用于乳化液、悬浮液和干粉的测量。 5,简单易用 全自动,使用简单。消除了不同用户间的的可变性。减少对新用户的培训要求,并充分发挥熟练人员的潜力。 6,灵活性 多种样品分散装置。通过自动配置,快速地切换样品分散装置。"即插即用"盒式系统允许同时连接两个样品分散装置。 7,规范符合性 完整的 QSpec 验证文档,并符合 21 CFR 第 11 部分的规定要求。 8,界面友好的软件 由软件驱动的标准操作规程 (SOP) 消除了用户间的差异。
粒度分析仪简介及使用
实验7、粒度分析仪简介及使用 纯牛奶粒度分布的测定(激光粒度法) 一、实验目的: 1.掌握粒度分析仪的测定原理及操作方法。 2.测定纳米粒子的粒度尺径及分布和Zeta电位性质。 二、实验原理: 2.1 激光粒度仪介绍 激光粒度分析仪仪是利用粒子的布朗运动,根据光的散射原理测量粉颗粒大小的,是一种比较通用的粒度仪。其特点是测量的动态范围宽、测量速度快、操作方便,尤其适合测量粒度分布范围宽的粉体和液体雾滴。对粒度均匀的粉体,比如磨料微粉,要慎重选用。 激光粒度仪集成了激光技术、现代光电技术、电子技术、精密机械和计算机技术,具有测量速度快、动态范围大、操作简便、重复性好等优点,现已成为全世界最流行的粒度测试仪器。 激光粒度仪作为一种新型的粒度测试仪器,已经在其它粉体加工与应用领域得到广泛的应用。它的特点是测试速度快、重复性好、准确性好、操作简便。对提高产品质量、降低能源消耗有着重要的意义。 2.2激光粒度仪的原理 激光粒度仪是根据颗粒能使激光产生散射这一物理现象测试粒度分布的。由于激光具有很好的单色性和极强的方向性,所以在没有阻碍的无限空间中激光将会照射到无穷远的地方,并且在传播过程中很少有发散的现象。如图1所示。 图1,激光束在无阻碍状态下的传播示意图 米氏散射理论表明,当光束遇到颗粒阻挡时,一部分光将发生散射现象,散射光的传播方向将与主光束的传播方向形成一个夹角θ,θ角的大小与颗粒的大小有关,颗粒越大,产生的散射光的θ角就越小;颗粒越小,产生的散射光的θ角就越大。即小角度(θ)的散射光是有大颗粒引起的;大角度(θ1)的散射光是由小颗粒引起的,如图2所示。进一步研究表明,散射光的强度代表该粒径颗粒的数量。这样,测量不同角度上的散射光的强度,就可以得到样品的粒度分布了。
激光粒度仪主要品牌分析(报告精选)
北京先略投资咨询有限公司
激光粒度仪主要品牌分析 (最新版报告请登陆我司官方网站联系) 公司网址: https://www.360docs.net/doc/c611600789.html, 1
目录 (最新版报告请登陆我司官方网站联系) (1) 公司网址: https://www.360docs.net/doc/c611600789.html, (1) 激光粒度仪主要品牌分析 (3) 第一节激光粒度仪品牌构成 (3) 第二节主要品牌区域市场占有率分析 (4) 第三节品牌满意度分析 (4) 2
激光粒度仪主要品牌分析 第一节激光粒度仪品牌构成 品牌知名度以及市场占有率是评价品牌竞争实力的重要指标。产业结构低,缺乏品牌是导致企业缺乏讨价还价能力的深层次原因。我国激光粒度仪行业的产品结构还不太合理,高科技含量、高附加值的产品不多,企业在市场竞争中拼资源、拼价格的现象比较严重。面对资源的制约和其他同类产品的激烈竞争,必须加强技术创新和产品创新,提升产品品牌才行。 从消费者正在使用产品的分布情况可以看出不同品牌的市场占有情况。因激光粒度仪产业进入门槛较高,生产企业较为集中,因此目前我国共有各种类型的颗粒测试仪器生产厂家二十余家,在国产粒度仪生产厂家中,丹东市百特仪器有限公司、珠海欧美克仪器有限公司、济南微纳颗粒仪器股份有限公司、成都精新粉体测试设备有限公司等几个厂商已经形成一定的生产规模,是国内颗粒测试仪器的主要生产商及出口商。 与此同时,国产品牌的激光粒度仪性能逐渐提高,新产品不断推出,如济南微纳颗粒仪器股份有限公司的Winner2005、Winner2008系列智能激光粒度仪测试范围下限0.05微米,2009年研制成功的Winner800系列光子相关纳米激光粒度仪测试范围下限更是达到了1纳米(0.001微米)。与进口颗粒测试仪器相比,国产仪器在价格、成本与售后服务上具有明显优势。首先,在产品价格上,进口颗粒测试仪器的平均价格约4万美元/台,价格是国内同类型仪器的数倍。其次,在售后服务上,国产颗粒测试仪器具有天然优势,能做到收费低廉、反应迅速、零配件及时供应;而进口仪器则受人员、授权、地理、语言等方面限制,面临时间长、费用高等问题。与进口激光粒度仪相比,正是由于国产粒度仪的技术水平不断创新发展使国产仪器在价格和成本控制上具有明显优势。 3
WJL激光粒度仪使用说明书
WJL激光粒度仪使用说明书 上海精密科学仪器有限公司 目录 1. 用途及特点 (2) 2. 规格及主要技术参数 (2) 3. 工作原理 (2) 4. 仪器结构 (3) 5. 操作步骤 (4) 6. 主要技术问题 (7) 7. 仪器成套性 (8) 8. 常见故障及其处理方法 (9) 9. 售后服务事项和生产者责任 (9) 1、用途及特点 WJL激光粒度仪是利用激光所特有的单色性、准直性等特点,根据颗粒对光的散射现象,按照Mie 散射理论作为仪器的测量基础而设计的实验室测试仪器。激光粒度仪广泛应用于化工、机械、冶金、电子、建筑及环保等行业的各种粉沙、微珠等原材料颗粒以及高分子乳胶物料等各类粉体材料颗粒的大小测定。 目前国内外同类粒度仪有一个共同的特点,即光学系统复杂,光路调整烦琐,因而仪器的造价一般较高;同时衍射式粒度仪由于在测量原理上的限制,必然使它在小粒径范围内很难达到令人满意的测量精度。 WJL激光粒度仪结构简单,操作方便。整个测量过程不需调节仪器中的任何部件。同时仪器还具有自标定、自校正功能,从而保证仪器有较高的测量精度。 2、规格及主要技术参数 可测粒径范围:0.5-200μm; 测量时间:采样时间0.5秒,计算时间一般为几秒种; 准确度:±6%(D50); 重复性:±6%(D50); 测量对象:粉末状颗粒、液-液和液-固系统中的液滴或固体颗粒; 粒径分布模式:自由分布及函数限定(R-R)分布; 电源:220V±22V,50Hz; 主机重量:12kg; 尺寸:608×228×168mm; 10.消耗功率:小于50W(不包括计算机)。 3、工作原理 激光粒度仪主要由激光器、样品池、光电探测器和计算机系统等部分组成,其结构如下图所示。被测颗粒放入样品池使之成为悬浮状态,当He-Ne激光器发出的激光束通过样品池时将会产生散射光,散射光的分布与被测颗粒的直径D、颗粒的相对折射率m和散射角θ有关。散射光由光电探测器接收,并经放大和A/D转换后经RS232或USB接口送入计算机,经数据处理和计算后就可以显示或打印出被测颗粒的粒径分布,各种平均直径及比表面积等参数。 4、仪器结构 仪器外视图
马尔文激光粒度仪操作流程
马尔文激光粒度仪 型号:Malvern Nano ZS 操作步骤: 一、开机 1、打开电脑主机 2、打开仪器开关(开关在仪器的后面),状态指示灯由棕黄色变为绿色,预 热30min。 二、 粒度样品装样 1、选择干净的样品池,缓慢注入溶液以避免气泡,使用一次性滴液管,同时 倾斜样品池装样至10‐15mm之间,用盖子将样品池封住。 2、将样品放入进样槽,至其停止.抛光的光学表面必须面向仪器前面 3、关闭样品池区盖子。 三、 Zeta电位样品装样 1、用滴管取至少1ml样品,将滴管与样品池一端连接。 2、将样品缓慢注射入样品池,检查是否除去所有气泡。 3、移去溅在外部电极上的任何液体
!!! 注意:进行测量之前必须塞住塞子 4、持样品池顶部,远离下部测量区,将样品池推入样品槽至其停止。 5、关闭样品池区盖子。 !!! 注意:虚线方向为正面 四、 测试 1、粒度测试 1)双击桌面上的DTS(nano)打开软件 2)选择File>New>Measurement File创建新的测量记录文件,保存在D盘 ‐data of DLS‐组内/组外。
对于已经存在测试文件,请选择Open打开文件 3)打开测试文件后,选择File>New>SOP或者File>Open>SOP来新建或者调出 标准测量程序。对于不常测试的样品,可以选择Measure>Manual来进行手动测 量设置。 3)新建SOP,选择File>New>SOP或者手动创建测量程序是会跳出如下对话框
4)左键点击? Measurement type,然后从下拉菜单中选择测量类型,粒径测量 我们选择Size。 4)按右向箭头,依次输入样品的概述 (编号和样品名称,样品的折光指数和吸收率)
马尔文MS3000粒度仪
超高速智能粒度分析仪 粒度引领粒度分析技术新时代 Mastersizer 3000 马尔文仪器(中国)
Mastersizer 3000不仅仅是一台新仪器—它是全新的粒度测量大师 马尔文仪器公司于1970年推出世界第一台商用激光粒度仪。随后第一套Mastersizer 系统在1988年诞生,自此,马尔文仪器一直引领着激光衍射粒度分析领域的发展。我们投身于这一技术,并为世界各地超过10,000家的Mastersizer 用户带来最新的技术、应用和各种粒度分析解决方案。 The Mastersizer 3000 最高性能、最小体积 Mastersizer 3000—最先进的系统 快速、可靠且高度自动化的激光衍射技术已经成为了世界上使用最广泛的粒度分析技术。现在,Mastersizer 3000将引领激光衍射技术步入全新的高速智能时代。 2
3 Mastersizer 3000的优势 Mastersizer 3000仪器及其分散系统的创新设计和革新工艺体现了马尔文公司的热诚和专业。我们根据市场需求开发了在最小的空间中包含最全面性能的仪器。快速而准确,Mastersizer 3000让所有人都能得心应手,无论是新用户还是粒度分析专家。 The Mastersizer 3000
革新的光学核心 Mastersizer 3000利用经过验证的激光衍射技术测定颗粒粒径。根据测定样品产生的在不同角度上的衍射光强度分布来计算粒度分布。采集这些数据所需的光学系统是本仪器的核心。 在新型的折叠光路设计中,Mastersizer 3000中的蓝光固态光源保证了亚微米级的分辨率,使粒径分析下限达到10nm。高速的数据采集速率大大增加了测试中的信号采集次数,提高了分析的重现性,即使是分布最宽的样品也能精确测定。同时也显著提高了测试速度。总之,依靠该独特的光学系统,仅一台仪器即可在整个极宽的动态范围内获得值得信赖的粒径数据。 The Mastersizer 3000 2 46 6 7 3 4
马尔文MS2000SM 激光粒度仪 操作程序-标准版
马尔文MS2000操作程序 一.开机顺序:先开仪器主机和湿法进样器,再开电脑软件。 关机顺序:先关电脑软件,再关湿法进样器和主机。二.湿法测量程序: a)往进样器加水,调节控制盒,让水循环起来,搅拌速度调到2500。 b)在桌面上双击Mastersizer2000操作软件,进入操作软件,输入操作者姓名,然后鼠标左键点击确定,开机预热30分钟以后才能进行测量样品。 c)在“文件File”那里点击打开,打开已有的文件或新建一个文件,确保测量记录存放在你所需要的文件名下。 d)单击“测量Measure”菜单中的“手动Manual”按钮,进入测量窗口。 e)然后点击“对光Align”,对光好后,如果背景Background状态正常,(如果Detector Number那边的信号基本成左边高右边低的态势就是比较正常的背景)就不需要换水了,如果换了几次水以后,背景还是不正常,就需要打开样品池窗口进行清洁样品窗口。(如果是第一次打开软件的话,“对光Align”按键是隐藏在“测量背景Measure Background”下面的,只要点击“开始Start”键盘,仪器就会对光接着测量背景的)。 f)然后进入“选项Options”菜单,选择合适的光学参数,在“物质Material”那的“物质名称Sample material name”那里选择好
样品的物质名称,如果测量的是仪器配的标准样品,要求选择“Glass Beads”,在“分散介质Dispersant name”选择Water,“模型Models”选择“通用模式General purpose”,如果是测量标准样品要求选择“single mode”,“颗粒形貌Particle shape”选择“不规则Irregular”,标准样品要求选择“spherical”,“测量选项Measurement”那里选择“样品测量时间Sample measurement”8秒,“背景测量时间Background measurement”10秒,在“测量循环Measurement Cycles”选择“测量次数Number of measurement cycles”3次,“延时时间Delay between measurement”选择0秒,下面的“创建平均结果Average Result”选择创建。再进入“文档Documentation”菜单,输入样品名称,然后确定退出。 g)然后单击“开始Start”按钮,系统开始测量背景,当背景测量完成以后并提示“加入样品Add Sample”后,开始加入样品到“遮光度Laser Obscuration”到10%左右,等待样品分散10秒,然后单击“开始Start”或按“测量样品Measure Sample”进行测量样品。每测量一次,结果会按照记录编号顺序自动存在文件里。 h)测量结束后,调节控制盒速度到0,打开排水手柄,让水排完以后,然后换新鲜的水循环清洗两到三次(以对光后背景正常为准)。
应用马尔文激光粒度仪分析泥沙数据处理模板
Journal of Water Resources Research 水资源研究, 2015, 4(6), 513-521 Published Online December 2015 in Hans. https://www.360docs.net/doc/c611600789.html,/journal/jwrr https://www.360docs.net/doc/c611600789.html,/10.12677/jwrr.2015.46064 文章引用: 田岳明, 沙剑. 应用马尔文激光粒度仪分析泥沙数据处理模板[J]. 水资源研究, 2015, 4(6): 513-521. A Sediment Data Processing Template for Malvern Laser Particle Size Analyzer Yueming Tian 1, Jian Sha 2 1Hydrological Bureau of the Yangtze River Water Resources Commission, Wuhan Hubei 2 Hydrology and Water Resources Survey Bureau of the Yangtze River Estuary in Shanghai, Shanghai Received: Nov. 8th , 2015; accepted: Nov. 27th , 2015; published: Dec. 10th , 2015 Copyright ? 2015 by authors and Hans Publishers Inc. This work is licensed under the Creative Commons Attribution International License (CC BY). https://www.360docs.net/doc/c611600789.html,/licenses/by/4.0/ Abstract Malvern laser particle size analyzer has been applied in the particle analysis of river sediments since the beginning of this century, and has been highly praised by users for simple operation, stable data from analysis, high automation of processing and quick achievements, etc. regardless of its significant devia-tion from the principles of traditional sedimentation analysis approach [1]. Now, it has been applied and popularized in the particle analysis of river sediments. Nevertheless, users may run into some problems in the use of this equipment, such as, inconvenient setting and check of fundamental parameters, lack of automatic detection and warning about quality of test data, troublesome selection of characteristic and extreme values in the data of tested particle grading, inconsistent form of analysis report, and cumber-some reading and output of data, etc. To solve these problems and unify the principles and requirements for operation of laser analyzer at the sediment monitoring stations, a “sediment data processing tem-plate for Malvern laser particle size analyzer” is developed while making full use of the existing functions of laser analyzer. This template is able to configure the fundamental parameters automatically, check the quality of analytic data and give warnings, print the reports in standard form according to the code of each monitoring station, and generate the textual output of analytic data in batches, etc., which can be directly sorted out and edited in the Southern Hydrological Data Processing (SHDP) software. The opera-tion for several years has achieved favorable effects, improved working efficiency, reduced error rate and ensured the quality of achievements. Keywords Laser Particle Size Analyzer, Sediment Analysis, Data Processing, Research Template 作者简介:田岳明(1959-),男,长江水利委员会水文局,高级工程师,主要从事河流泥沙粒度分析技术管理与研究。