Greenhouse gas emissions in Chicago

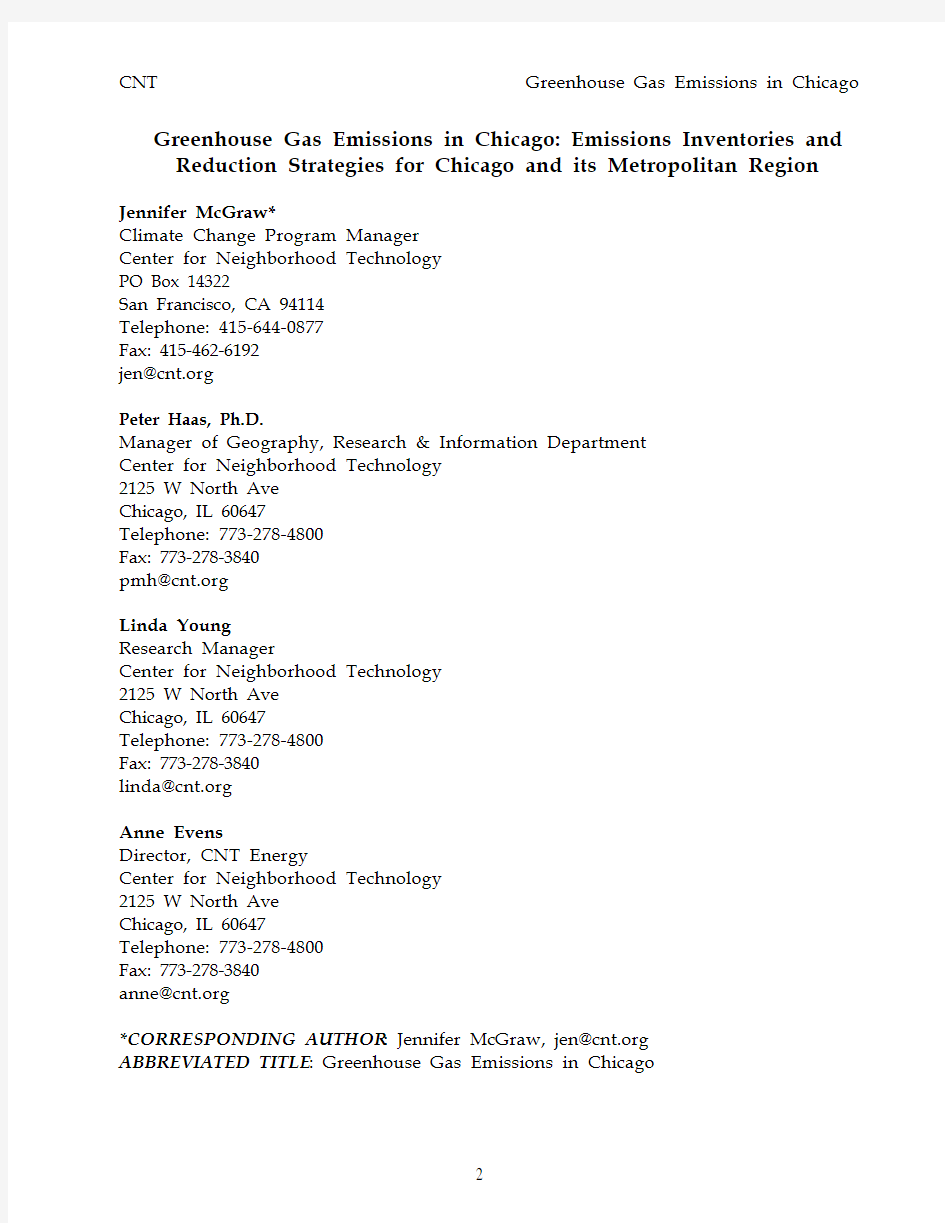
Greenhouse Gas Emissions in Chicago: Emissions Inventories and Reduction Strategies for Chicago and its Metropolitan Region
Jennifer McGraw, Peter Haas, Linda Young, and Anne Evens
February 2010
NOTICE: this is the author’s version of a work that was accepted for publication in Journal of Great Lakes Research. Changes resulting from the publishing process, such as peer review, editing, corrections, structural formatting, and other quality control mechanisms may not be reflected in this document. Changes may have been made to this work since it was submitted for publication. A definitive version was subsequently published in Journal of Great Lakes Research (2009), doi:10.1016/j.jglr.2009.11.010 .
Center for Neighborhood Technology
2125 W. North Ave.
Chicago, IL 60647
773-278-4800
https://www.360docs.net/doc/446774262.html,
Greenhouse Gas Emissions in Chicago: Emissions Inventories and Reduction Strategies for Chicago and its Metropolitan Region Jennifer McGraw*
Climate Change Program Manager
Center for Neighborhood Technology
PO Box 14322
San Francisco, CA 94114
Telephone: 415-644-0877
Fax: 415-462-6192
jen@https://www.360docs.net/doc/446774262.html,
Peter Haas, Ph.D.
Manager of Geography, Research & Information Department
Center for Neighborhood Technology
2125 W North Ave
Chicago, IL 60647
Telephone: 773-278-4800
Fax: 773-278-3840
pmh@https://www.360docs.net/doc/446774262.html,
Linda Young
Research Manager
Center for Neighborhood Technology
2125 W North Ave
Chicago, IL 60647
Telephone: 773-278-4800
Fax: 773-278-3840
linda@https://www.360docs.net/doc/446774262.html,
Anne Evens
Director, CNT Energy
Center for Neighborhood Technology
2125 W North Ave
Chicago, IL 60647
Telephone: 773-278-4800
Fax: 773-278-3840
anne@https://www.360docs.net/doc/446774262.html,
*CORRESPONDING AUTHOR: Jennifer McGraw, jen@https://www.360docs.net/doc/446774262.html, ABBREVIATED TITLE: Greenhouse Gas Emissions in Chicago
ABSTRACT
A greenhouse gas emissions inventory was conducted for Chicago and its metropolitan region for the years 2000 and 2005. Emissions of carbon dioxide, methane, nitrous oxide, hydrofluorocarbons, perfluorocarbons, and sulfur hexafluoride totaled 34.7 million metric tons of carbon dioxide equivalents (MMTCO2e) in Chicago in 2000 with 91 percent of emissions attributable to the indirect emissions associated with electricity consumption, the direct emissions of natural gas use, and the direct emissions of the transportation sector. A portfolio of 33 potential emissions reduction strategies was analyzed that, implemented together, could meet Chicago’s target of reducing greenhouse gas emissions to 25 percent below 1990 levels by 2020. The largest potential for reduction is found in the areas with the largest emissions—energy use in buildings and transport. Compared to its metropolitan region, Chicago is found to have existing transportation efficiencies on a per household basis that can be an example for other communities.
INDEX WORDS: Climate Change, Chicago, GHG, Inventory, Mitigation
INTRODUCTION
Global climate change presents a challenge of epic proportions for Chicago and the world. To address climate change, its origins must be understood. The primary sources of anthropogenic greenhouse gas (GHG) emissions contributing to global warming have been well documented at a large scale through global studies and national inventory reports. The global standard for such national inventories is the Intergovernmental Panel on Climate Change’s (IPCC) “2006 IPCC Guidelines for National Greenhouse Gas Inventories” (IPCC 2006). But, as smaller entities such as cities seek to take action on global warming they must understand their GHG emissions sources at a finer grain of detail.
Creating an emissions inventory at the city level poses unique challenges; for example, many cities are net importers of electricity—meaning that less electricity is generated within the city boundaries than is consumed within the city. If only electricity generation emissions were inventoried, the city would undercount the impact of its electricity use and miss many important opportunities to reduce electricity demand, and perhaps its associated emissions, with energy efficiency and conservation. The IPCC 2006 Guidelines provide no method for calculating GHG emissions based on electricity consumption, because on a national level it is sufficient to calculate the emissions associated with the total fuel consumed for electricity generation. Such issues were addressed in large part for business inventories with the introduction of the World Business Council for Sustainable Development and World Resource Institute’s “The Greenhouse Gas Protocol: A Corporate Accounting and Reporting Standard” in 2001. Many cities have used this method to document the GHG emissions associated with their local government operations—their buildings, fleets and other assets—but this standard is not fully applicable to the challenges of emissions inventories for cities as geographic entities, which are too small in geography to accurately follow national inventory methods, yet are more like nations than businesses in that cities do not own or operate all of the facilities in their boundaries. As a result, cities have needed to innovate on these methods to document their contribution to global warming in a complete and accurate way. The challenges of this evolving methodology and limited data have meant that few cities know their total GHG emissions or the sources of those emissions. This situation is changing rapidly as cities seek to address their climate change impact. This article documents the first ever inventory of GHG emissions for Chicago, Illinois.
The primary reason for creating an emissions inventory is to generate an understanding of the sources of emissions and identify where the opportunities for emissions reductions lie. Before taking action, many cities choose to set an emissions reduction target to shape their planning and benchmark their progress. Climate scientists estimate that a 50-85 percent reduction below 2000 global GHG emissions by 2050 is required to achieve an atmospheric concentration of GHGs at 445-490 ppm and stabilize the climate at 2.0-2.4 degrees Celsius above pre-industrial temperatures (Metz et. al. 2007). A thousand cities in the United States have signed on to the U.S. Mayor’s Climate Protection Agreement, which encourages cities to reduce emissions to 7 percent
below 1990 levels by 2012—the national emissions reductions commitment the U.S. would have made if it had ratified the Kyoto Protocol. Chicago has signed on to this Agreement, but Chicago’s Climate Change Task Force used the Chicago emissions inventories for 2000 and 2005 and estimates of future and past emissions to set a more aggressive target of reducing emissions to 25 percent below 1990 levels by 2020, which would put the city on the path to an 80 percent reduction by 2050.
With this target set, a portfolio of emission reduction strategies was developed for Chicago. The emissions reduction potential of each strategy was estimated and quantitative and qualitative analysis was conducted to determine emission reduction potentials, the nature and scale of the programs and policies necessary, similar activities underway in Chicago and the region that could be built upon, examples of successful programs from other areas, and implementation opportunities and barriers. To understand the scale of the reductions needed a business as usual scenario was developed based on historical and national trends for each emissions sector. This projection is discussed further in the research report prepared for the project (McGraw et. al. 2008). This research was done with the goal of providing the Chicago Climate Change Task Force with the information necessary to create a feasible and measurable emissions reduction plan for Chicago.
METHODS
Chicago’s GHG emissions inventory was calculated for the years 2000 and 2005 using IPCC and GHG Protocol (IPCC 2006, WBCSD and WRI 2004) methods and local data sources in combination with modeling of national data to local demographics. The emissions inventory for 2000 was prepared because it was the earliest year for which necessary data were readily available. An emissions inventory for 2005 was also created as the most recent year with complete data.
As discussed above, 1990 is one of the standard baseline years for setting emissions reductions targets and it was the year the City of Chicago wanted to use as a reference point to make comparisons with other communities and programs; however relevant activity data for Chicago in 1990 were unavailable from local energy utilities and others. Therefore an estimated emissions value based on historic Chicago area and national growth trends was used as a reference point for 1990. This point is just an estimate and should be viewed as such; its primary use is to enable a reduction target to be set. Reaching that target is the focus of the Chicago Climate Action Plan.
Emissions in the years 2000 and 2005 were calculated for all direct sources within the geographical boundaries of the city of Chicago—natural gas use, transportation, non-energy industrial processes, and the use of GHGs in products. Indirect emissions from the consumption of electricity and disposal of waste to waste treatment facilities outside of city boundaries were also calculated. Finally, emission sequestration associated with Chicago’s trees was estimated. Emissions were calculated for the six major categories of GHGs regulated under the Kyoto Protocol: carbon dioxide (CO2), methane (CH4), nitrous oxide (N2O), hydrofluorocarbons (HFCs), perfluorocarbons (PFCs), and sulfur hexafluoride (SF6). Emissions were converted into carbon dioxide
equivalents (CO2e) by multiplying emissions in mass by global warming potentials (GWPs) from the IPCC Third Annual Assessment Report (Houghton et. al. 2001) and summing the resulting weighted emissions (Table 1).
TABLE 1. Summary of Chicago GHG emissions inventories including primary activity data type by emissions source.
Emissions Source Primary Activity Data
Sources for Chicago
Inventory
2000
Chicago
GHG
Emissions
MMTCO2e
2005
Chicago
GHG
Emissions
MMTCO2e
Percent of
2005 Total
Chicago
Emissions
Energy: Electricity Use Electricity use from utility billing
data 12.916.0
44%
Energy; Natural Gas Use Natural gas use from utility
billing data 11.59.9 27%
Transportation: On-Road Vehicle travel statistics for city
from state department of
transportation 6.6 6.5
18%
Transportation: Off-Road National transit database
commuter rail fuel use, Amtrak
passenger rail energy use,
regional air quality monitoring
agency cargo rail fuel use,
utility electric use data for
transit system 0.70.7 2%
Aviation City aviation fuel use from tax
data 8.77.5
Not
included in
total
Industrial Processes National emissions inventory,
U.S. Census 0.40.0 0%
Product Use National emissions inventory,
U.S. Census 1.2 1.5 4%
Waste City annual solid waste
disposal data 1.1 1.2 3%
Wastewater Emissions report from regional
wastewater treatment agency 0.40.3 1%
Total 34.736.2
100%
Total Including Aviation 43.543.7 121%
Agriculture, Forestry, and Other Land Use Tree crown cover calculated
using GIS analysis -0.1-0.1 0% Energy
Energy emissions in this study include emissions associated with electricity and natural gas consumption. Other non-transport energy sources (for example, kerosene and propane) were investigated and data for Chicago were unavailable. However, electricity and natural gas are 96 percent of the energy use in the area (Energy Information Administration 2004).
Electricity emissions were calculated by gathering electricity consumption data from the local utility, Commonwealth Edison, and applying CO2 emissions factors associated with the local North American Electric Reliability Council region from the U.S. Environmental Protection Agency (U.S. EPA) Emissions & Generation Resource Integrated Database (eGRID) (U.S. EPA. 2006) and CH4 and N2O emissions factors from the California Climate Action Registry General Reporting Protocol (California Climate Action Registry 2006). The regional power pool emissions factors were used rather than emissions factors associated with specific generation plants because electricity is traded across a grid and at any given point electricity could be supplied by any of the grid-connected plants in operation. Hence, a regional average of GHG emissions per kilowatt hour (kWh) is the best representative of the GHG impacts of electricity consumption. Electricity consumption, in terms of kWh, was measured based on user account data, and transmission and distribution losses were not included.
Natural gas emissions were calculated by gathering natural gas consumption data from People’s Energy and from the Illinois Commerce Commission for the Nicor Gas service territory (Illinois Commerce Commission 2002 and 2006), and applying natural gas emissions factors from the U.S. EPA’s Inventory of U.S. Greenhouse Gas Emissions and Sinks (U.S. EPA 2007a) and the IPCC 2006 Guidelines for National Greenhouse Gas Inventories (IPCC 2006). For energy consumption the calculation of emissions is a simple equation of energy consumed * GHG emissions per unit of energy = GHG emissions.
Transportation
Transportation emissions were developed using vehicle miles traveled (VMT) data from the Illinois Department of Transportation (Illinois Department of Transportation 2001 and 2006) for on-road vehicles combined with fleet mix data from the Lake Michigan Air Directors Consortium (Lake Michigan Air Directors Consortium 2007) and vehicle efficiency data from the Federal Highway Administration (Federal Highway Administration 2001 and 2006) to create a profile of fuel consumption and VMT in Chicago by vehicle type. Fuel use and VMT data from Amtrak, Lake Michigan Air Directors Consortium, Energy Information Administration (Energy Information Administration 2007b) and National Transit Database (Federal Transit Administration 2001 and 2006) were used to calculate passenger rail, cargo rail, and commuter rail emissions. In addition, the emissions associated with electricity used for Chicago’s transit system was categorized as part of transportation emissions.
Fuel sales from the City of Chicago Department of Aviation were used to calculate emissions associated with flights originating at Chicago’s two airports. A recent publication from the Airport Cooperative Research Program (ACRP) recommends the use of modeled fuel use data by flight to calculate emissions from air travel, but those modeled data are not yet publicly available by airport from the Federal Aviation Administration. The ACRP report recommends aviation fuel sales data as an alternative method (Kim et. al. 2009.).
Emissions from flights originating in Chicago, though calculated, are not included in Chicago’s total inventory, as there is not yet a standard for allocating responsibility for air travel emissions to specific cities. The Kyoto protocol excludes international shipping and aviation from national GHG reduction commitments (United Nations 1997). The transnational nature of these modes of transport complicates the accounting of, and designation of responsibility for, their resulting GHG emissions. Similar accounting questions arise for domestic and international aviation when examining GHG emissions on the city scale, as airports generally serve an entire region rather than a city, and at major aviation hubs like O’Hare many passengers are simply transferring and are not originating nor terminating their trip in Chicago.
There are other off-road transportation emissions sources that were not captured in this inventory because data were unavailable. These sources include fuel consumed by marine transportation, construction equipment, business equipment (i.e. forklifts), recreational equipment (i.e. golf carts), and lawn and gardening equipment. None of these is likely to be significant for Chicago; marine transportation uses 5 percent of transportation energy nationally, and other off-road sources not addressed here, including agricultural equipment, use 8 percent of the national total (U.S. EPA 2007a). Moreover, one would expect these off road uses of petroleum to be included in fuel sales data, which was cross checked with VMT for on-road transportation and fond to be within the same range. Future research should investigate the off-road uses of fossil fuels in Chicago in further detail through fuel sales, activity surveys, and vehicle sales.
Emissions factors for transportation are from the U.S. EPA Inventory of U.S. Greenhouse Gas Emissions and Sinks (U.S. EPA 2007a) and the U.S. EPA State Inventory Tool (U.S. EPA 2007b). Fuel consumption data in the transport sector was multiplied by the fuel emission factor to calculate emissions. For on-road transport, CH4 and N2O emissions factors are expressed as emissions per mile, because the value is dependent on emissions control technology, as opposed to the straight fuel combustion-based calculation for CO2 (IPCC 2006). The emissions from on-road transportation in Chicago were cross checked with fuel sale data from the City of Chicago Department of Revenue.
Industrial Processes and Product Use
Industrial processes and the use of GHGs in products contributed 5 percent of U.S. GHG emissions in 2007 (U.S. EPA 2009). Data on emissions from non-energy industrial processes and the use of GHGs in products are very difficult to find at the city level, so the emissions are estimated as a proportion of national emissions as reported in U.S. EPA 2007a. Inventories of local industrial emitters and data on the sales and use of products that result in GHG emissions would be preferable, and further research in this area is encouraged. If Chicago was a smaller city, or home to a major industrial emitter, such a as a large cement producer, industrial process emissions might be a significant share of the community’s emissions, but industries using GHG emitting processes are no longer major employers in Chicago, and given the share of the city’s emissions from industrial processes and product use, this rough estimation
method was deemed to introduce an acceptably small level of error into the study. Understanding the scale of likely emissions from these sources for Chicago and discussing the emission reduction potential presented in this sector make it worthwhile to include these sources in the community’s emissions profile.
The industrial processes sector includes non-energy related GHG emissions, such as those generated in the process of cement manufacturing. The US Census Bureau’s Economic Census and Annual Survey of Manufacturers (U.S. Census Bureau 2001a and 2005) was used to determine the proportion of U.S. GHG producing industrial activity in Chicago. First, GHG producing industries located in Chicago were identified by North American Industry Classification System (NAICS) code. The relevant industries in Chicago were found to be Iron and Steel Production and Integrated Circuit or Semiconductor manufacturing. The employment in these sectors in Chicago was then calculated as a percentage of national employment by sector and used to prorate the national GHG emissions in that sector. The potential for error in this method is substantial, but until better data are available for industrial processes at the city scale, it is a fair approximation.
In addition to these industrial activities, there are a number of products used in Chicago that contain GHGs. These include the sulfur hexafluoride (SF6) used as an insulator in electrical equipment and the nitrous oxide (N2O) used as an anesthetic by dentists. Again, local data on these emissions were unavailable, so a similar method as the industrial process emissions was employed—national emissions were prorated by Chicago’s share of the national population using the U.S. EPA National Inventory and U.S. Census data (U.S. EPA 2007a and U.S. Census Bureau 2001b).
Waste and Wastewater
Annual waste disposal and waste composition data provided by the City of Chicago were used with degradable organic content values by waste type from IPCC 2006 to enable the calculation of the total degradable organic content (DOC) of landfilled waste at 16 percent. This was used to calculate methane generation by using the following IPCC 2006 default values: a methane correction factor (MCF) of 1, 10 percent oxidation (OX) of CH4, 50 percent CH4 content in landfill gas (F), and a conversion of carbon to CH4 of 16/12. The U.S. EPA National Inventory value of 77 percent of degradable organic content decomposed (DOCf) was used along with the City of Chicago’s data on 75 percent methane recovery (R). The equation to calculate emissions from waste disposal was: Mass of Waste Disposed (net of recycling and other diversion) * DOC * MCF * DOCf * F * OX * 16/12 * R = CH4 from landfilled waste.
Only CH4 associated with waste decomposition was calculated. Solid waste also produces CO2 as it decomposes, but as the carbon stored in decomposing food, paper, and paper products is biogenic in origin—it was absorbed from the atmosphere by plants in recent history—its release does not contribute to global warming, and therefore is not counted in this inventory. All of the landfills used by Chicago in 2000 and 2005 were located outside of the city (Illinois Environmental Protection Agency 2006), so the emissions associated with waste disposal are considered indirect
emissions. Chicago has a number of closed landfills within its city boundaries. Solid waste takes decades to decompose, so closed landfills can continue to generate CH4 emissions for 50 years or more. IPCC 2006 uses a first order decay method to account for current year emissions from historic waste disposal, but data were unavailable at the time of this study to estimate these emissions for Chicago.
Emissions associated with wastewater treatment were based on an estimate conducted by the Metropolitan Water Reclamation District (MWRD) using the methodology detailed in IPCC 2006. It was assumed that all sewer discharge was delivered to the MWRD plants via a covered sewer collection system. The MWRD estimate of the fugitive methane emissions for the entire district (which is larger than Chicago) was then scaled to represent the water treatment associated only with the Chicago population. IPCC 2006 methods calculate wastewater emissions as a function of wastewater treatment technology and total organic waste (TOW). TOW is calculated based on population and biological oxygen demand (BOD) per capita, so prorating emissions by population is a reasonable estimation method for this sector., although further study in this area would be useful, because Chicago is both an economic center and a tourist destination, so its share of regional wastewater generation may be higher than its proportion of the regional population. Chicago accounted for 57 percent of the MWRD population in 2000 and 54 percent of the MWRD population in 2005. As with solid waste, the CO2 emissions associated with wastewater are considered biogenic and were not included in the inventory.
Water reclamation plants recover methane during the water treatment process. This recovered methane is used on site for heating and/or electricity generation. There are no data available on the amount of methane that is recovered by MWRD annually. This is an area for further research.
Agriculture, Forestry, and Other Land Use
IPCC 2006 provides an estimated annual carbon accumulation value per hectare of tree crown cover in settled areas of 2.9 tons carbon per hectare (10.6 tons CO2). This value was multiplied by the total crown cover in Chicago to estimate the CO2 sequestered by Chicago’s urban forest annually. Chicago’s tree canopy was calculated using GIS analysis from aerial photos and shapefiles provided by the Chicago Department of Environment. Chicago may have benefited from additional carbon uptake from the growth of other plants such as shrubbery and grasses, but this would be negligible at the city scale and was not measured.
Agriculture in the U.S. emitted 547.4 MMTCO2e in 2000 through livestock, crop, and soil activities. Some of these agricultural emissions are associated with the food and goods Chicagoans consume, however, from a direct emissions accounting perspective there is little agricultural activity within Chicago’s borders, so no agricultural emissions are included in Chicago’s emissions inventory. The lifecycle and indirect emissions associated with goods and services is an issue of importance to long term climate stability and should be further studied.
Regional GHG Emissions
Inventories of GHG emissions in 2000 and 2005 for the six-county Chicago Metropolitan Region were also conducted in order to examine the role of Chicago in the region, and the emissions reduction potential of regional strategies such as transit and transit-oriented development. While most of this article discusses the emissions inventory and mitigation strategies developed for Chicago, the regional inventory is also discussed as a point of comparison.
The methods described above for the Chicago city inventory were also used for most sections of the regional inventory. Data were obtained for activities in the six-county Chicago region from the sources described above for energy, transportation, and industrial processes and product use, and the same methods were used to estimate these emissions at the regional scale. Regional solid waste disposal data (IL EPA 2006) were combined with the solid waste emissions estimation methods used for Chicago. The wastewater emissions from MWRD were prorated based on regional population, as the six-county area extends beyond the MWRD service area, but wastewater data for those other areas were not available. Unlike Chicago, the region contains both agriculture and livestock that contribute to the area’s GHG emissions. These emissions were estimated as a share of national emissions based on agricultural land area and livestock counts by type (U.S. EPA 2007a and U.S. Department of Agriculture 2004). A more specific inventory of regional agricultural emissions using IPCC 2006 methods would be informative.
Mitigation Strategies
A broad survey of projects and programs that can reduce GHG emissions was conducted including solicitation of input from stakeholders and research into best practices in communities around the world to identify feasible solutions that suit Chicago. After review of all mitigation ideas, 33 were selected for in-depth research based on their feasibility, potential for GHG reductions, and capacity for rapid implementation at the city and regional level. Many programs with smaller emission reduction potentials were combined into larger strategies that met the scale of the reductions needed. It should be noted that nearly all of these strategies utilize currently available technology, and therefore, from a technical standpoint, could begin to be implemented immediately. Two of the strategies, Cap and Trade and Carbon Tax, were researched as umbrella strategies that could enable all of the others, thus their emissions savings are equal to the size of the whole.
Estimating the emissions reduction potential of each strategy required assuming a specific scale of adoption and a potential emission reduction per unit of activity. The scale of adoption for these illustrative purposes was set at an achievable, but aggressive, level. For strategies that expand programs that already exist, the current level of activity was assumed to be part of business as usual, because it is represented in Chicago’s emissions inventory, and savings were calculated only for program expansion.
In many cases the potential emissions reductions per unit of activity were developed based on data in the 2000 emissions inventory, such as annual emissions per
household associated with electricity and natural gas consumption. For example, consider the residential energy retrofit strategy which assumes a program that targets 400,000 homes by 2020 and saves an estimated 30 percent of energy use per building on average. Using the 2000 inventory, residential energy use emitted 11.4 MMTCO2e and there were 1.06 million occupied housing units (U.S. Census 2001b), so each unit’s energy use contributed 10.7 metric tons of CO2e on average. A 30 percent savings would therefore result in an annual 3.2 metric ton CO2e reduction per unit, or 1.3 MMTCO2e per year for 400,000 homes. This is just an estimate of the scale of such a program. As an emissions reduction program is planned the assumptions inherent in such a calculation should be vetted and actual savings should be tracked over time. The methodologies for the United Nations Framework Convention on Climate Change Clean Development Mechanism are a good resource for information on how to measure emissions savings from particular projects as they are planned and implemented.
For other strategies, such as the adoption of car sharing, the activity savings or associated emissions reduction were developed based on literature. In the case of car sharing programs, Cervero et. al. 2007 shows that the average car share user saves 0.28 gallons (1.06 L) of fuel per day as compared to a non-user. Over the course of a year, that results in 102 gallons (386 L) of fuel and 0.88 metric tons CO2e saved per car share user based on emissions rates from the 2000 Chicago inventory. The addition of 183,000 car share users therefore results in a total annual savings of 0.16 MMTCO2e.
The actual emission reduction impacts of a given policy or program will depend on dozens of implementation details, so the emission reduction potentials of each strategy the City of Chicago puts into place will need to continue to be refined and actual savings achieved should be studied, but the research discussed here is meant to give a sense of the scale of the reduction potential of each strategy for planning purposes. Additionally, the GHG impacts of some strategies, such as high speed rail, are more aptly measured at a regional scale. A discussion of regional savings and more details on the methods for each strategy can be found in McGraw et. al. 2008.
Many of the emission reduction strategies overlap in their impacts. An energy efficiency strategy that reduces electricity use has a different emissions reduction potential when combined with a renewable power strategy that decarbonizes the electricity supply. As a result, after the emissions reduction potential of each strategy was documented separately the strategies were analyzed together as a whole with their interactions accounted for. This ensured that the potential savings of the portfolio of strategies would meet Chicago’s emissions reduction target, because even though the sum total of the individual savings of each emissions strategy is much greater than the reduction target, taken together they offset each other in many places. This often overlooked issue added a level of complexity to the mitigation analysis, but was essential to ensure enough reduction options were developed to meet Chicago’s goals.
RESULTS
In the year 2000, Chicago emitted 34.7 million metric tons of carbon dioxide equivalents (MMTCO2e) of GHGs—12 tons for each of Chicago’s 2.9 million residents,
or 32 tons per household (U.S. Census Bureau 2001b). The majority—91 percent—of these emissions came from the consumption of electricity, natural gas, and transportation. Chicago’s GHG emissions grew in 2005 to 36.2 MMTCO2e, 4.2 percent higher than 2000 emissions levels. Comparatively, U.S. national emissions grew 1.6 percent 2000 to 2005 from 7,146.1 to 7,260.4 MMTCO2e (U.S. EPA 2007a) (Fig 1).
FIG. 1. Chicago’s greenhouse gas emissions in the year 2000 were 34.7 MMTCO2e and rose to 36.3 MMTCO2e in 2005.
Energy
Chicago’s non-transportation energy use emitted 24.4 MMTCO2e in 2000, which was 71 percent of the total citywide emissions. By 2005, energy emissions grew by 6 percent to 25.9 MMTCO2e. Chicago’s GHG emissions from energy use were nearly evenly split between electricity and natural gas in 2000, but in 2005 electricity emissions grew while natural gas emissions shrank.
The 25 percent growth in electricity emissions from 2000 to 2005 was due in part to a 14 percent growth in electricity consumption from 21 billion kWh to 24 billion kWh. The growth in emissions was also attributable to an increase in electricity emissions per kWh. The emissions from electricity consumption are calculated based on the average emissions from all power plants in the North American Electric Reliability Council region, or regional power pool. In addition to any real changes within the electric
supply, the boundaries of the power pool that includes Chicago changed between 2000 and 2005. The resulting emissions factor for electricity grew nine percent from 2000 to 2005; in 2000, it was 0.609 kg per kWh and in 2005, it was 0.664 kg per kWh (U.S. EPA 2002 and 2006).
Electricity emissions in Chicago’s residential sector grew 34 percent and electricity consumption grew from 5.5 to 6.8 billion kWh from 2000 to 2005. Non-residential electricity consumption emissions grew 21 percent as electricity use increased from 15 to 17 billion kWh from 2000 to 2005.
While this study measured electricity emissions based on consumption, there are two large coal-fired electricity generation facilities in Chicago. These two plants create approximately 1 percent of the electricity generated in the regional power pool (U.S. EPA 2002 and 2006), so their emissions impact is already included in this research. Taken together, these two plants produced the equivalent of 21 percent of the electricity consumed in Chicago, but their emissions were equivalent to 35 percent of the CO2 emissions from Chicago’s electricity consumption (U.S. EPA 2002 and 2006).
Natural gas use in Chicago fell 14 percent from 2000 to 2005 with the largest drop—34 percent—in the industrial sector from 300 million to 200 million Therms. The residential sector fell 13 percent from 1.5 to 1.3 billion Therms, while the commercial sector stayed level at 350 million Therms. The emissions factors used for natural gas were the same in 2000 and 2005 at 5.31 kg CO2, 0.527 g CH4, and 0.0105 g N2O per Therm (U.S. EPA 2007a).
Transportation
Transportation is the second largest source of GHG emissions in Chicago. Excluding the airports, transportation emitted 7.3 MMTCO2e in 2000 and 7.1 MMTCO2e in 2005. In 2000, transportation was 21 percent of Chicago’s GHG emissions.
On-road vehicles, including cars, trucks, and motorcycles, generated the majority of transportation GHGs in Chicago in 2000 and 2005—91 percent. The 3 percent decrease in total GHGs in this sector from 7.12 MMTCO2e in 2000 to 6.85 MMTCO2e in 2005 was largely due to an increase in the weighted average fuel economy for the vehicles on the road in Chicago from 16.5 miles per gallon (mpg) to 18.7 mpg (7.01 to 7.95 km/L) as vehicle efficiency in the U.S. increased from 2000 and 2005 for passenger cars and heavy-duty trucks (Federal Highway Administration 2001 and 2006).
Emissions associated with off-road transportation accounted for nine percent of Chicago’s transportation emissions in 2000 and 2005—0.687 MMTCO2e and 0.657 MMTCO2e respectively. Metra, the Chicago regional commuter rail system, consumed 90.5 million L of diesel fuel in 2000, generating 0.244 MMTCO2e. In 2005, Metra’s fuel consumption increased to 91.2 million L and its emissions increased slightly to 0.247 MMTCO2e. At 1.6 billion reported passenger miles (2.6 billion km), Metra’s GHG emissions were 0.15 kg CO2e per passenger mile(0.093 kg/km) in 2000 (Federal Transit Administration 2001 and 2006).
Electricity consumed by transportation in Chicago was 331 million kWh in 2000, generating 0.202 MMTCO2e. Most of this can be attributed to the Chicago Transit
Authority (CTA), operators of Chicago’s “L”, elevated electric train system. In 2005, electricity use associated with transportation increased 23 percent to 406 million kWh and the associated emissions increased to 0.271 MMTCO2e. At a reported 1 billion passenger miles per year (1.6 billion km), the CTA’s GHG emissions were approximately 0.20 kg CO2e per passenger mile (0.12 kg/km) in 2000 (Federal Transit Administration 2001 and 2006).
Emissions for Amtrak regional and long-distance rail emissions in Chicago were estimated at 0.01 MMTCO2e in 2000 and 2005 based on VMT and vehicle efficiency data in Chicago provided by Amtrak. Chicago was one of the nation’s busiest Amtrak locations with 2.5 million passengers riding Amtrak to or from Chicago in 2005 (Amtrak 2005). As with air travel and cargo, the total emissions associated with Chicago Amtrak passengers is much greater than what is emitted within Chicago boundaries, and for most purposes regional and long distance rail emissions should be examined at a geographic scale larger than a city.
Chicago is a major shipping hub, and cargo rail emissions in the city were 0.23 MMTCO2e in 2000 based on 83 million L of diesel fuel consumed as reported by the Lake Michigan Air Directors Consortium. Cargo rail emissions fell to 0.13 MMTCO2e in 2005, as reported fuel consumption fell to 49 million L. It is not clear that this decrease is a trend however, as multimodal shipping using rail is gaining popularity in the U.S. (Davis and Diegel 2007).
With 77 million passengers traveling through O’Hare airport and 18 million passengers using Midway airport in 2005, Chicago is one of the busiest aviation hubs in the world (City of Chicago 2006). In the year 2000, air travel GHG emissions in Chicago were 8.74 MMTCO2e based on domestic aviation fuel sale data provided by the Chicago Department of Revenue and international aviation fuel information from the Chicago Department of Aviation. Aviation emissions in Chicago were lower in 2005 at 7.53 MMTCO2e, though passengers and aircraft traffic increased between 2000 and 2005. This may be due to efficiency improvements, changes in refueling practices, or inconsistencies in the way the taxed fuel data were recorded. More detailed study of aviation GHG emissions in Chicago may help answer this uncertainty.
Industrial Processes and Product Use
Industrial processes and product use generated 1.6 MMTCO2e in 2000, or 5 percent of the city total GHG emissions, and 1.5 MMTCO2e in 2005, or 4 percent of the total. The activity data in this sector are very difficult to find on at the city level, so the emissions of this sector are estimated as a proportion of national emissions as reported in U.S. EPA 2007a. Many of the emissions in this sector are compounds with high GWPs, so relatively small emissions in this area can have large comparative climate change impact. Industrial processes were found to emit 0.433 MMTCO2e in 2000 and 0.0443 MMMTCO2e in 2005. Emissions from industrial processes decreased because of lower employment levels in GHG emitting industries due to the continuing deindustrialization of Chicago. The use of GHGs in products generated emissions of 1.19 MMTCO2e in 2000 and 1.53 MMTCO2e in 2005 in Chicago. The growth in this area
is largely due to increasing use of high GWP compounds as substitutes for ozone depleting substances, such as refrigerants.
Waste and Wastewater
Chicago’s waste and wastewater treatment emitted 1.37 MMTCO2e in 2000 growing 15 percent to 1.58 MMTCO2e in 2005. Emissions in this sector were 4 percent of Chicago’s total GHG inventory.
Chicagoans generated 4.3 million metric tons of solid waste in 2000—1.5 metric tons per person according to data provided by the Chicago Department of the Environment. Waste generation grew by 16 percent to 5.0 million tons in 2005—1.77 metric tons per person. According to the City of Chicago, 56 percent of the waste generated was sent to landfills in 2000 and 2005. The result was emissions of 1.06 MMTCO2e in 2000 and 1.23 MMTCO2e in 2005. Solid waste made up 77 percent of the emissions in this sector in 2000.
Fugitive methane emissions from water reclamation plants were estimated to be 0.352 MMTCO2e in 2000 and 0.346 MMTCO2e in 2005 according to calculations performed by MWRD using IPCC 2006 methods.
Agriculture, Forestry, and Other Land Use
Chicago’s trees had a crown cover of 8,350 hectares in 2000. Thus, trees covered 14.2 percent of Chicago’s land area—greater tree cover than the average desert (10 percent), but less than the average grassland (20 percent) (IPCC 2006). The result is that Chicago’s trees absorbed 0.0888 MMTCO2e in 2000. This was 0.3 percent of the total citywide emissions.
Reduction Target
A reduction target of 25 percent below 1990 levels by 2020 was set. Based on an estimate of 1990 emissions this target would be equivalent to 24.2 MMTCO2e in 2020, which is 30% below 2000 emissions levels and 33% below 2005 emissions levels. Several other interim and longer-term targets were also set in relation to the 1990 reference point to guide short and long-term climate action planning (Fig 2).
FIG. 2. The Chicago Climate Action Plan calls for a reduction to 25 percent below 1990 emissions levels by 2020 and sets interim and future targets as well. The 1990 value is an estimate because activity data required for an emissions inventory were not available for 1990.
Regional GHG Emissions
The six-county Chicago metro area—Cook, Will, DuPage, Kane, McHenry and Lake counties—had a population of 8.1 million in 2000 (U.S. Census Bureau 2001b). Chicago’s 2.9 million residents made up 36 percent of the region (U.S. Census Bureau 2001b). The Chicago region emitted 105 MMTCO2e in 2000, or 12.9 tons per capita. As in Chicago, energy and transportation accounted for 91 percent of the regional emissions. However, transportation was a larger share of emissions in the region—31 percent— than in Chicago—20 percent. Emissions in all sectors grew at a faster rate in the region than in Chicago, resulting in ten percent growth between 2000 and 2005 to 116 MMTCO2e, or 13.8 tons per capita (U.S. Census Bureau. 2006). The two main sources of this growth in GHG emissions were electricity use and solid waste generation.
Mitigation Strategies
The emission reduction potentials of 33 strategies were analyzed for Chicago to use in developing its Climate Action Plan. The estimated annual emissions savings of each strategy at full deployment were modeled for 2020—the year of Chicago’s emission reduction target—and range from 0.01 to 3.0 MMTCO2e. Due to the impacts of the mitigation strategies on each other they are not simply additive, but when modeled together to account for overlapping savings the total emission reductions can meet the goal of reducing Chicago’s emissions to 24.2 MMTCO2e by 2020 if implemented aggressively.
The quantitative and qualitative analysis of each mitigation measure is discussed in further detail in McGraw et. al. 2008. Not all strategies analyzed were adopted by Chicago in its final plan, and some were altered in scale or scope for adoption (City of
Chicago 2008). Moreover, these strategies were analyzed to understand the scale of their potential mitigation impact for planning purposes, and as Chicago works to implement its Climate Action Plan the emission reduction strategies will evolve and actual program results should be tracked.
The strategies developed for Chicago’s emission reduction portfolio fall into nine categories:
Framing/Leadership: Climate action in Chicago should be framed by ongoing City and civic leadership; early action; measurement and evaluation mechanisms; and education and promotion of behavior change. These framing strategies influence the implementation of all other strategies. While they may not be individually measurable, deploying them effectively is essential for the success of the overall portfolio.
Energy Demand: Reducing the amount of energy used in buildings, both existing and new, is one of the largest potential sources of emissions savings for Chicago. Savings can begin to be achieved quickly in this area and provide additional benefits that include lower utility bills.
Energy Supply: Decarbonizing Chicago’s energy sources by expanding the supply of renewable energy and reducing the GHG emissions of conventional energy sources will lower the emissions of the remaining energy Chicago needs after demand reductions.
Transportation Demand: Reducing the miles traveled by vehicles in Chicago through promotion of transit-oriented development; alternative means of transportation, including walking, bicycling, car sharing, and public transportation; and efficient freight movement will reduce the emissions from transportation fuel use and can lower costs for households and organizations.
Transportation Petroleum Use: Transportation demand reductions will not eliminate on-road vehicle travel in Chicago altogether, so lowering petroleum use by increasing the number of fuel-efficient vehicles and utilizing alternative fuels will further cut Chicago’s transportation emissions.
Waste and Water: Improving water efficiency, reducing waste generation, and increasing reuse and recycling can reduce waste methane emissions as well as associated energy emissions from transport and treatment.
Industrial Processes and Product Use: Reducing the use of high GWP GHGs in products, such as refrigerants, may be the best source of emissions reduction potential for Chicago in this sector, but additional research and development is needed on these technologies.
Land Cover and Forestry: Additional trees, green spaces, and green roofs can improve the carbon sequestration potential of Chicago’s urban forest as well as lower heating and cooling emissions in buildings.
Cross-cutting Strategies: Two overarching strategies were analyzed: creation of a carbon tax, and creation of a cap and trade system. In both cases, the role of the City could be to advocate for implementation of the strategy at a national level and then to use the strategy as a tool to help implement the other reduction measures. Because of
DISCUSSION
Electricity consumption, natural gas use, and transportation are the main sources of Chicago’s global warming impact—91 percent of Chicago’s emissions come from these three sectors, therefore most emission reductions opportunities come from these areas as well. There is no one single cure for Chicago’s climate impact, but many actions that must be taken together. A portfolio of 33 climate change mitigation strategies was developed that will allow Chicago to contribute its share to climate stabilization. With early, continuous, and aggressive action, these strategies will reduce Chicago’s GHG emissions and bring additional environmental and economic benefits to Chicago.
While they range widely in scale and scope, each of the strategies analyzed can make a significant contribution to Chicago’s GHG reduction effort. In some cases, such as building retrofits, the potential reductions are large and the value of implementation is clear. Some smaller strategies, however, such as the planting of trees or promotion of walking, are valuable components of a broader sustainability strategy, because they
bring significant additional benefits, add to public awareness of the issues, or can be relatively easily deployed.
Chicago’s emission reduction goal of 25 percent below 1990 GHG levels by 2020 is ambitious in terms of the action required, but essential from a global climate standpoint. All the strategies framed here, taken together and deployed at scale, could reach Chicago’s overall reduction goal. Getting there is attainable, but will require significant action by every sector of Chicago. It will also require Chicago to work regionally, nationally, and in concert with other cites. Climate analysis and planning at the city-scale is important, because many of the actual changes that must occur to reduce global GHG emissions will be implemented at the local level, but some reduction strategies that benefit Chicago, such as high speed rail or fuel economy standards, will only be feasible at larger geographies of implementation.
Not all emissions reductions are identical. Some programs are more cost effective than others, some programs deliver significant benefits in addition to GHG savings, and programs can vary widely in their financial, political, and technical feasibility. Moreover, in the race to curb global warming emissions reductions achieved now may be of more value than those implemented at a later time. All of these elements should be considered before choosing to implement one mitigation strategy over another. Due to space constraints, such quantitative and qualitative discussion for each strategy is not presented here but can be found in McGraw et. al. 2008.
Some of the strategies with the biggest reductions are also those that will bring the biggest economic benefits to Chicago residents and businesses. For example, energy and transportation efficiencies could save Chicago households hundreds, if not thousands, of dollars a year, and will bring substantial savings to Chicago businesses as well.
Energy Strategies
Demand side strategies are as critical as supply side strategies for GHG reductions at the city and regional level. The energy saved in buildings and the miles not driven can together account for nearly half of the targeted reductions. They can take advantage of the inherent efficiency of urban areas and the extraordinary resources represented by the public transportation network. Having implemented efficiency measures wherever possible, renewable sources of energy can ensure that the energy we do use is as clean as possible.
Improving the energy efficiency of buildings is the biggest single opportunity for GHG reduction in Chicago. With 70 percent of Chicago’s GHG emissions generated by electricity and natural gas use, energy efficiency is a critical strategy. Because 80 percent of buildings that will exist in 2020 are already built, these strategies must focus on both existing and new buildings. Taken together, strategies to reduce energy use in buildings accounts for approximately 30 percent of GHG reductions analyzed.
Since such a large portion of electricity and natural gas use in Chicago heats and cools our buildings, the use is very dependent on the weather. This trend is seen in the residential sector: the number of cooling degree days—a measure of how hot weather is
50万吨年煤气化生产工艺
咸阳职业技术学院生化工程系毕业论文(设计) 50wt/年煤气化工艺设计 1.引言 煤是由古代植物转变而来的大分子有机化合物。我国煤炭储量丰富,分布面广,品种齐全。据中国第二次煤田预测资料,埋深在1000m以浅的煤炭总资源量为2.6万亿t。其中大别山—秦岭—昆仑山一线以北地区资源量约2.45万亿t,占全国总资源量的94%;其余的广大地区仅占6%左右。其中新疆、内蒙古、山西和陕西等四省区占全国资源总量的81.3%,东北三省占 1.6%,华东七省占2.8%,江南九省占1.6%。 煤气化是煤炭的一个热化学加工过程,它是以煤或煤焦原料,以氧气(空气或富氧)、水蒸气或氢气等作气化剂,在高温条件下通过化学反应将煤或煤焦中的可燃部分转化为可燃性的气体的过程。气化时所得的可燃性气体称为煤气,所用的设备称为煤气发生炉。 煤气化技术开发较早,在20世纪20年代,世界上就有了常压固定层煤气发生炉。20世纪30年代至50年代,用于煤气化的加压固定床鲁奇炉、常压温克勒沸腾炉和常压气流床K-T炉先后实现了工业化,这批煤气化炉型一般称为第一代煤气化技术。第二代煤气化技术开发始于20世纪60年代,由于当时国际上石油和天然气资源开采及利用于制取合成气技术进步很快,大大降低了制造合成
气的投资和生产成本,导致世界上制取合成气的原料转向了天然气和石油为主,使煤气化新技术开发的进程受阻,20世纪70年代全球出现石油危机后,又促进了煤气化新技术开发工作的进程,到20世纪80年代,开发的煤气化新技术,有的实现了工业化,有的完成了示范厂的试验,具有代表性的炉型有德士古加压水煤浆气化炉、熔渣鲁奇炉、高温温克勒炉(ETIW)及干粉煤加压气化炉等。 近年来国外煤气化技术的开发和发展,有倾向于以煤粉和水煤浆为原料、以高温高压操作的气流床和流化床炉型为主的趋势。 2.煤气化过程 2.1煤气化的定义 煤与氧气或(富氧空气)发生不完全燃烧反应,生成一氧化碳和氢气的过程称为煤气化。煤气化按气化剂可分为水蒸气气化、空气(富氧空气)气化、空气—水蒸气气化和氢气气化;按操作压力分为:常压气化和加压气化。由于加压气化具有生产强度高,对燃气输配和后续化学加工具有明显的经济性等优点。所以近代气化技术十分注重加压气化技术的开发。目前,将气化压力在P>2MPa 情况下的气化,统称为加压气化技术;按残渣排出形式可分为固态排渣和液态排渣。气化残渣以固体形态排出气化炉外的称固态排渣。气化残渣以液态方式排出经急冷后变成熔渣排出气化炉外的称液态排渣;按加热方式、原料粒度、汽化程度等还有多种分类方法。常用的是按气化炉内煤料与气化剂的接触方式区分,主要有固定床气化、流化床气化、气流床气化和熔浴床床气化。 2.2 主要反应 煤的气化包括煤的热解和煤的气化反应两部分。煤在加热时会发生一系列的物理变化和化学变化。气化炉中的气化反应,是一个十分复杂的体系,这里所讨论的气化反应主要是指煤中的碳与气化剂中的氧气、水蒸汽和氢气的反应,也包括碳与反应产物之间进行的反应。 习惯上将气化反应分为三种类型:碳—氧之间的反应、水蒸汽分解反应和甲烷生产反应。 2.2.1碳—氧间的反应 碳与氧之间的反应有: C+O2=CO2(1)
煤气化工艺流程
煤气化工艺流程 1、主要产品生产工艺煤气化是以煤炭为主要原料的综合性大型化工企业,主要工艺围绕着煤的洁净气化、综合利用,形成了以城市煤气为主线联产甲醇的工艺主线。 主要产品城市煤气和甲醇。城市燃气是城市公用事业的一项重要基础设施,是城市现代化的重要标志之一,用煤气代替煤炭是提高燃料热能利用率,减少煤烟型大气污染,改善大气质量行之有效的方法之一,同时也方便群众生活,节约时间,提高整个城市的社会效率和经济效益。作为一项环保工程,(其一期工程)每年还可减少向大气排放烟尘万吨、二氧化硫万吨、一氧化碳万吨,对改善河南西部地区城市大气质量将起到重要作用。 甲醇是一种重要的基本有机化工原料,除用作溶剂外,还可用于制造甲醛、醋酸、氯甲烷、甲胺、硫酸二甲酯、对苯二甲酸二甲酯、丙烯酸甲酯等一系列有机化工产品,此外,还可掺入汽油或代替汽油作为动力燃料,或进一步合成汽油,在燃料方面的应用,甲醇是一种易燃液体,燃烧性能良好,抗爆性能好,被称为新一代燃料。甲醇掺烧汽油,在国外一般向汽油中掺混甲醇5?15勉高汽油的辛烷值,避免了添加四乙基酮对大气的污染。 河南省煤气(集团)有限责任公司义马气化厂围绕义马至洛阳、洛阳至郑州煤气管线及豫西地区工业及居民用气需求输出清洁能源,对循环经济建设,把煤化工打造成河南省支柱产业起到重要作用。 2、工艺总流程简介: 原煤经破碎、筛分后,将其中5?50mm级块煤送入鲁奇加压气化炉,在炉内与氧气和水蒸气反应生成粗煤气,粗煤气经冷却后,进入低温甲醇洗净化装置,除去煤气中的CO2和H2S净化后的煤气分为两大部分,一部分去甲醇合成系统,合成气再经压缩机加压至,进入甲醇反应器生成粗甲醇,粗甲醇再送入甲醇精馏系统,制得精甲醇产品存入贮罐;另一部分去净煤气变换装置。合成甲醇尾气及变换气混合后,与剩余部分出低温甲醇洗净煤气混合后,进入煤气冷却干燥装置,将露点降至-25 C后,作为合格城市煤气经长输管线送往各用气城市。生产过程中产生的煤气水进入煤气水分离装置,分离出其中的焦油、中油。分离后煤气水去酚回收和氨回收,回收酚氨后的煤气水经污水生化处理装置处理,达标后排放。低温甲醇洗净化装置排出的H2S到硫回收装置回收硫。空分
煤气化工艺流程
精心整理 煤气化工艺流程 1、主要产品生产工艺 煤气化是以煤炭为主要原料的综合性大型化工企业,主要工艺围绕着煤的洁净气化、综合利用,形成了以城市煤气为主线联产甲醇的工艺主线。 主要产品城市煤气和甲醇。城市燃气是城市公用事业的一项重要基础设施,是城市现代化的重要标志之一,用煤气代替煤炭是提高燃料热能利用率,减少煤烟型大气污染,改善大气质量行之 化碳 15%提 作用。 2 。净化 装置。合成甲醇尾气及变换气混合后,与剩余部分出低温甲醇洗净煤气混合后,进入煤气冷却干燥装置,将露点降至-25℃后,作为合格城市煤气经长输管线送往各用气城市。生产过程中产生的煤气水进入煤气水分离装置,分离出其中的焦油、中油。分离后煤气水去酚回收和氨回收,回收酚氨后的煤气水经污水生化处理装置处理,达标后排放。低温甲醇洗净化装置排出的H2S到硫回收装置回收硫。空分装置提供气化用氧气和全厂公用氮气。仪表空压站为全厂仪表提供合格的仪表空气。 小于5mm粉煤,作为锅炉燃料,送至锅炉装置生产蒸汽,产出的蒸汽一部分供工艺装置用汽
,一部分供发电站发电。 3、主要装置工艺流程 3.1备煤装置工艺流程简述 备煤工艺流程分为三个系统: (1)原煤破碎筛分贮存系统,汽运原煤至受煤坑经1#、2#、3#皮带转载至筛分楼、经节肢筛、破碎机、驰张筛加工后,6~50mm块煤由7#皮带运至块煤仓,小于6mm末煤经6#、11#皮带近至末煤仓。 缓 可 能周期性地加至气化炉中。 当煤锁法兰温度超过350℃时,气化炉将联锁停车,这种情况仅发生在供煤短缺时。在供煤短缺时,气化炉应在煤锁法兰温度到停车温度之前手动停车。 气化炉:鲁奇加压气化炉可归入移动床气化炉,并配有旋转炉篦排灰装置。气化炉为双层压力容器,内表层为水夹套,外表面为承压壁,在正常情况下,外表面设计压力为3600KPa(g),内夹套与气化炉之间压差只有50KPa(g)。 在正常操作下,中压锅炉给水冷却气化炉壁,并产生中压饱和蒸汽经夹套蒸汽气液分离器1
煤化工工艺流程
煤化工工艺流程 典型的焦化厂一般有备煤车间、炼焦车间、回收车间、焦油加工车间、苯加工车间、脱硫车间和废水处理车间等。 焦化厂生产工艺流程 1.备煤与洗煤 原煤一般含有较高的灰分和硫分,洗选加工的目的是降低煤的灰分,使混杂在煤中的矸石、煤矸共生的夹矸煤与煤炭按照其相对密度、外形及物理性状方面的差异加以分离,同时,降低原煤中的无机硫含量,以满足不同用户对煤炭质量的指标要求。 由于洗煤厂动力设备繁多,控制过程复杂,用分散型控制系统DCS改造传统洗煤工艺,这对于提高洗煤过程的自动化,减轻工人的劳动强度,提高产品产量和质量以及安全生产都具有重要意义。
洗煤厂工艺流程图 控制方案 洗煤厂电机顺序启动/停止控制流程框图 联锁/解锁方案:在运行解锁状态下,允许对每台设备进行单独启动或停止;当设置为联锁状态时,按下启动按纽,设备顺序启动,后一设备的启动以前一设备的启动为条件(设备间的延时启动时间可设置),如果前一设备未启动成功,后一设备不能启动,按停止键,则设备顺序停止,在运行过程中,如果其中一台设备故障停止,例如设备2停止,则系统会把设备3和设备4停止,但设备1保持运行。
2.焦炉与冷鼓 以100万吨/年-144孔-双炉-4集气管-1个大回流炼焦装置为例,其工艺流程简介如下:
100万吨/年焦炉_冷鼓工艺流程图 控制方案 典型的炼焦过程可分为焦炉和冷鼓两个工段。这两个工段既有分工又相互联系,两者在地理位置上也距离较远,为了避免仪表的长距离走线,设置一个冷鼓远程站及给水远程站,以使仪表线能现场就近进入DCS控制柜,更重要的是,在集气管压力调节中,两个站之间有着重要的联锁及其排队关系,这样的网络结构形式便于可以实现复杂的控制算法。
煤气化工艺流程
煤气化工艺流程 1、主要产品生产工艺 煤气化是以煤炭为主要原料的综合性大型化工企业,主要工艺围绕着煤的洁净气化、综合利用,形成了以城市煤气为主线联产甲醇的工艺主线。 主要产品城市煤气和甲醇。城市燃气是城市公用事业的一项重要基础设施,是城市现代化的重要标志之一,用煤气代替煤炭是提高燃料热能利用率,减少煤烟型大气污染,改善大气质量行之有效的方法之一,同时也方便群众生活,节约时间,提高整个城市的社会效率和经济效益。作为一项环保工程,(其一期工程)每年还可减少向大气排放烟尘1.86万吨、二氧化硫3.05万吨、一氧化碳0.46万吨,对改善河南西部地区城市大气质量将起到重要作用。 甲醇是一种重要的基本有机化工原料,除用作溶剂外,还可用于制造甲醛、醋酸、氯甲烷、甲胺、硫酸二甲酯、对苯二甲酸二甲酯、丙烯酸甲酯等一系列有机化工产品,此外,还可掺入汽油或代替汽油作为动力燃料,或进一步合成汽油,在燃料方面的应用,甲醇是一种易燃液体,燃烧性能良好,抗爆性能好,被称为新一代燃料。甲醇掺烧汽油,在国外一般向汽油中掺混甲醇5~15%提高汽油的辛烷值,避免了添加四乙基酮对大气的污染。 河南省煤气(集团)有限责任公司义马气化厂围绕义马至洛阳、洛阳至郑州煤气管线及豫西地区工业及居民用气需求输出清洁能源,对循环经济建设,把煤化工打造成河南省支柱产业起到重要作用。 2、工艺总流程简介: 原煤经破碎、筛分后,将其中5~50mm级块煤送入鲁奇加压气化炉,在炉内与氧气和水蒸气反应生成粗煤气,粗煤气经冷却后,进入低温甲醇洗净化装置
,除去煤气中的CO2和H2S。净化后的煤气分为两大部分,一部分去甲醇合成系统,合成气再经压缩机加压至5.3MPa,进入甲醇反应器生成粗甲醇,粗甲醇再送入甲醇精馏系统,制得精甲醇产品存入贮罐;另一部分去净煤气变换装置。合成甲醇尾气及变换气混合后,与剩余部分出低温甲醇洗净煤气混合后,进入煤气冷却干燥装置,将露点降至-25℃后,作为合格城市煤气经长输管线送往各用气城市。生产过程中产生的煤气水进入煤气水分离装置,分离出其中的焦油、中油。分离后煤气水去酚回收和氨回收,回收酚氨后的煤气水经污水生化处理装置处理,达标后排放。低温甲醇洗净化装置排出的H2S到硫回收装置回收硫。空分装置提供气化用氧气和全厂公用氮气。仪表空压站为全厂仪表提供合格的仪表空气。 小于5mm粉煤,作为锅炉燃料,送至锅炉装置生产蒸汽,产出的蒸汽一部分供工艺装置用汽,一部分供发电站发电。 3、主要装置工艺流程 3.1备煤装置工艺流程简述 备煤工艺流程分为三个系统: (1)原煤破碎筛分贮存系统,汽运原煤至受煤坑经1#、2#、3#皮带转载至筛分楼、经节肢筛、破碎机、驰张筛加工后,6~50mm块煤由7#皮带运至块煤仓,小于6mm末煤经6#、11#皮带近至末煤仓。 (2)最终筛分系统:块煤仓内块煤经8#、9#皮带运至最终筛分楼驰张筛进行检查性筛分。大于6mm块煤经10#皮带送至200#煤斗,筛下小于6mm末煤经14#皮带送至缓冲仓。 (3)电厂上煤系统:末煤仓内末煤经12#、13#皮带转至5#点后经16#皮
煤气化制甲醇工艺流程
煤气化制甲醇工艺流程 1 煤制甲醇工艺 气化 a)煤浆制备 由煤运系统送来的原料煤干基(<25mm)或焦送至煤贮斗,经称重给料机控制输送量送入棒磨机,加入一定量的水,物料在棒磨机中进行湿法磨煤。为了控制煤浆粘度及保持煤浆的稳定性加入添加剂,为了调整煤浆的PH值,加入碱液。出棒磨机的煤浆浓度约65%,排入磨煤机出口槽,经出口槽泵加压后送至气化工段煤浆槽。煤浆制备首先要将煤焦磨细,再制备成约65%的煤浆。磨煤采用湿法,可防止粉尘飞扬,环境好。用于煤浆气化的磨机现在有两种,棒磨机与球磨机;棒磨机与球磨机相比,棒磨机磨出的煤浆粒度均匀,筛下物少。煤浆制备能力需和气化炉相匹配,本项目拟选用三台棒磨机,单台磨机处理干煤量43~ 53t/h,可满足60万t/a甲醇的需要。 为了降低煤浆粘度,使煤浆具有良好的流动性,需加入添加剂,初步选择木质磺酸类添加剂。 煤浆气化需调整浆的PH值在6~8,可用稀氨水或碱液,稀氨水易挥发出氨,氨气对人体有害,污染空气,故本项目拟采用碱液调整煤浆的PH值,碱液初步采用42%的浓度。 为了节约水源,净化排出的含少量甲醇的废水及甲醇精馏废水均可作为磨浆水。 b)气化 在本工段,煤浆与氧进行部分氧化反应制得粗合成气。 煤浆由煤浆槽经煤浆加压泵加压后连同空分送来的高压氧通过烧咀进入气化炉,在气化炉中煤浆与氧发生如下主要反应: CmHnSr+m/2O2—→mCO+(n/2-r)H2+rH2S CO+H2O—→H2+CO2 反应在6.5MPa(G)、1350~1400℃下进行。 气化反应在气化炉反应段瞬间完成,生成CO、H2、CO2、H2O和少量CH4、H2S等气体。 离开气化炉反应段的热气体和熔渣进入激冷室水浴,被水淬冷后温度降低并被水蒸汽饱和后出气化炉;气体经文丘里洗涤器、碳洗塔洗涤除尘冷却后送至变换工段。 气化炉反应中生成的熔渣进入激冷室水浴后被分离出来,排入锁斗,定时排入渣池,由扒渣机捞出后装车外运。 气化炉及碳洗塔等排出的洗涤水(称为黑水)送往灰水处理。 c)灰水处理 本工段将气化来的黑水进行渣水分离,处理后的水循环使用。 从气化炉和碳洗塔排出的高温黑水分别进入各自的高压闪蒸器,经高压闪蒸浓缩后的黑水混合,经低压、两级真空闪蒸被浓缩后进入澄清槽,水中加入絮凝剂使其加速沉淀。澄清槽底部的细渣浆经泵抽出送往过滤机给料槽,经由过滤机给料泵加压后送至真空过滤机脱水,渣饼由汽车拉出厂外。 闪蒸出的高压气体经过灰水加热器回收热量之后,通过气液分离器分离掉冷凝液,然后进入变换工段汽提塔。 闪蒸出的低压气体直接送至洗涤塔给料槽,澄清槽上部清水溢流至灰水槽,由灰水泵分别送至洗涤塔给料槽、气化锁斗、磨煤水槽,少量灰水作为废水排往废水处理。 洗涤塔给料槽的水经给料泵加压后与高压闪蒸器排出的高温气体换热后送碳洗塔循环
煤气化工艺资料
煤化工是以煤为原料,经过化学加工使煤转化为气体,液体,固体燃料以及化学品的过程,生产出各种化工产品的工业。 煤化工包括煤的一次化学加工、二次化学加工和深度化学加工。煤的气化、液化、焦化,煤的合成气化工、焦油化工和电石乙炔化工等,都属于煤化工的范围。而煤的气化、液化、焦化(干馏)又是煤化工中非常重要的三种加工方式。 煤的气化、液化和焦化概要流程图 一.煤炭气化
煤炭气化是指煤在特定的设备内,在一定温度及压力下使煤中有机质与气化剂(如蒸汽/空气或氧气等)发生一系列化学反应,将固体煤转化为含有CO、H2、CH4等可燃气体和CO2、N2等非可燃气体的过程。 煤的气化的一般流程图 煤炭气化包含一系列物理、化学变化。而化学变化是煤炭气化的主要方式,主要的化学反应有: 1、水蒸气转化反应C+H2O=CO+H2 2、水煤气变换反应CO+ H2O =CO2+H2 3、部分氧化反应C+0.5 O2=CO 4、完全氧化(燃烧)反应C+O2=CO2 5、甲烷化反应CO+2H2=CH4 6、Boudouard反应C+CO2=2CO 其中1、6为放热反应,2、3、4、5为吸热反应。 煤炭气化时,必须具备三个条件,即气化炉、气化剂、供给热量,三者缺一不可。 煤炭气化按气化炉内煤料与气化剂的接触方式区分,主要有: 1) 固定床气化:在气化过程中,煤由气化炉顶部加入,气化剂由气化炉底部加入,煤料与气化剂逆流接触,相对于气体的上升速度而言,煤料下降速度很慢,甚至可视为固定不动,因此称之为固定床气化;而实际上,煤料在气化过程中是以很慢的速度向下移动的,比
较准确的称其为移动床气化。 2) 流化床气化:它是以粒度为0-10mm的小颗粒煤为气化原料,在气化炉内使其悬浮分散在垂直上升的气流中,煤粒在沸腾状态进行气化反应,从而使得煤料层内温度均一,易于控制,提高气化效率。 3) 气流床气化。它是一种并流气化,用气化剂将粒度为100um以下的煤粉带入气化炉内,也可将煤粉先制成水煤浆,然后用泵打入气化炉内。煤料在高于其灰熔点的温度下与气化剂发生燃烧反应和气化反应,灰渣以液态形式排出气化炉。 4) 熔浴床气化。它是将粉煤和气化剂以切线方向高速喷入一温度较高且高度稳定的熔池内,把一部分动能传给熔渣,使池内熔融物做螺旋状的旋转运动并气化。目前此气化工艺已不再发展。 以上均为地面气化,还有地下气化工艺。 根据采用的气化剂和煤气成分的不同,可以把煤气分为四类:1.以空气作为气化剂的空气煤气;2.以空气及蒸汽作为气化剂的混合煤气,也被称为发生炉煤气;3.以水蒸气和氧气作为气化剂的水煤气;4.以蒸汽及空气作为气化剂的半水煤气,也可是空气煤气和水煤气的混合气。 几种重要的煤气化技术及其技术性能比较 1.Lurgi炉固定床加压气化法对煤质要求较高,只能用弱粘结块煤,冷煤气效率最高,气化强度高,粗煤气中甲烷含量较高,但净化系统复杂,焦油、污水等处理困难。 鲁奇煤气化工艺流程图
煤气化制甲醇工艺流程
煤气化制甲醇工艺流程 煤气化制甲醇工艺流程简述 1)气化 a)煤浆制备 由煤运系统送来的原料煤**t/h(干基)(<25mm)或焦送至煤贮斗,经称重给料机控制输送量送入棒磨机,加入一定量的水,物料在棒磨机中进行湿法磨煤。为了控制煤浆粘度及保持煤浆的稳定性加入添加剂,为了调整煤浆的PH值,加入碱液。 出棒磨机的煤浆浓度约65%,排入磨煤机出口槽,经出口槽泵加压后送至气化工段煤浆槽。 煤浆制备首先要将煤焦磨细,再制备成约65%的煤浆。磨煤采用湿法,可防止粉尘飞扬,环境好。 用于煤浆气化的磨机现在有两种,棒磨机与球磨机;棒磨机与球磨机相比,棒磨机磨出的煤浆粒度均匀,筛下物少。 煤浆制备能力需和气化炉相匹配,本项目拟选用三台棒磨机,单台磨机处理干煤量43~53t/h,可满足60万t/a甲醇的需要。 为了降低煤浆粘度,使煤浆具有良好的流动性,需加入添加剂,初步选择木质磺酸类添加剂。 煤浆气化需调整浆的PH值在6~8,可用稀氨水或碱液,稀氨水易挥发出氨,氨气对人体有害,污染空气,故本项目拟采用碱液调整煤浆的PH值,碱液初步采用42%的浓度。 为了节约水源,净化排出的含少量甲醇的废水及甲醇精馏废水均可作为磨浆水。 b)气化 在本工段,煤浆与氧进行部分氧化反应制得粗合成气。 煤浆由煤浆槽经煤浆加压泵加压后连同空分送来的高压氧通过烧咀进入气化炉,在气化炉中煤浆与氧发生如下主要反应: CmHnSr+m/2O2—→mCO+(n/2-r)H2+rH2S CO+H2O—→H2+CO2 反应在6.5MPa(G)、1350~1400℃下进行。 气化反应在气化炉反应段瞬间完成,生成CO、H2、CO2、H2O和少量CH4、H2S等气体。 离开气化炉反应段的热气体和熔渣进入激冷室水浴,被水淬冷后温度降低并被水蒸汽饱和后出气化炉;气体经文丘里洗涤器、碳洗塔洗涤除尘冷却后送至变换工段。 气化炉反应中生成的熔渣进入激冷室水浴后被分离出来,排入锁斗,定时排入渣池,由扒渣机捞出后装车外运。 气化炉及碳洗塔等排出的洗涤水(称为黑水)送往灰水处理。 c)灰水处理 本工段将气化来的黑水进行渣水分离,处理后的水循环使用。 从气化炉和碳洗塔排出的高温黑水分别进入各自的高压闪蒸器,经高压闪蒸浓缩后的黑水混合,经低压、两级真空闪蒸被浓缩后进入澄清槽,水中加入絮凝剂使其加速沉淀。澄清槽底部的细渣浆经泵抽出送往过滤机给料槽,经由过滤机给料泵加压后送至真空过滤机脱水,渣饼由汽车拉出厂外。 闪蒸出的高压气体经过灰水加热器回收热量之后,通过气液分离器分离掉冷凝液,然后进入变换工段汽提塔。
煤气化工艺流程简述
煤气化工艺流程简述 1)气化 a)煤浆制备 由煤运系统送来的原料煤**t/h(干基)(<25mm)或焦送至煤贮斗,经称重给料机控制输送量送入棒磨机,加入一定量的水,物料在棒磨机中进行湿法磨煤。为了控制煤浆粘度及保持煤浆的稳定性加入添加剂,为了调整煤浆的PH值,加入碱液。 出棒磨机的煤浆浓度约65%,排入磨煤机出口槽,经出口槽泵加压后送至气化工段煤浆槽。 煤浆制备首先要将煤焦磨细,再制备成约65%的煤浆。磨煤采用湿法,可防止粉尘飞扬,环境好。 用于煤浆气化的磨机现在有两种,棒磨机与球磨机;棒磨机与球磨机相比,棒磨机磨出的煤浆粒度均匀,筛下物少。 煤浆制备能力需和气化炉相匹配,本项目拟选用三台棒磨机,单台磨机处理干煤量43~53t/h,可满足60万t/a甲醇的需要。 为了降低煤浆粘度,使煤浆具有良好的流动性,需加入添加剂,初步选择木质磺酸类添加剂。 煤浆气化需调整浆的PH值在6~8,可用稀氨水或碱液,稀氨水易挥发出氨,氨气对人体有害,污染空气,故本项目拟采用碱液调整煤浆的PH值,碱液初步采用42%的浓度。 为了节约水源,净化排出的含少量甲醇的废水及甲醇精馏废水均可作为磨浆水。 b)气化 在本工段,煤浆与氧进行部分氧化反应制得粗合成气。 煤浆由煤浆槽经煤浆加压泵加压后连同空分送来的高压氧通过烧咀进入气化炉,在气化炉中煤浆与氧发生如下主要反应: CmHnSr+m/2O2—→mCO+(n/2-r)H2+rH2S CO+H2O—→H2+CO2 反应在6.5MPa(G)、1350~1400℃下进行。 气化反应在气化炉反应段瞬间完成,生成CO、H2、CO2、H2O和少量CH4、H2S等气体。 离开气化炉反应段的热气体和熔渣进入激冷室水浴,被水淬冷后温度降低并被水蒸汽饱和后出气化炉;气体经文丘里洗涤器、碳洗塔洗涤除尘冷却后送至变换工段。
煤气化制甲醇工艺流程
煤气化制甲醇工艺流程
煤气化制甲醇工艺流程 2008-11-08 10:11 1)气化 a)煤浆制备 由煤运系统送来的原料煤**t/h(干基)(<25mm)或焦送至煤贮斗,经称重给料机控 制输送量送入棒磨机,加入一定量的水,物料在棒磨机中进行湿法磨煤。为了控制煤 浆粘度及保持煤浆的稳定性加入添加剂,为了调整煤浆的 PH 值,加入碱液。
b5E2RGbCAP
出棒磨机的煤浆浓度约 65%,排入磨煤机出口槽,经出口槽泵加压后送至气化工 段煤浆槽。 煤浆制备首先要将煤焦磨细,再制备成约 65%的煤浆。磨煤采用湿法,可防止粉 尘飞扬,环境好。 用于煤浆气化的磨机现在有两种,棒磨机与球磨机;棒磨机与球磨机相比,棒磨机 磨出的煤浆粒度均匀,筛下物少。
p1EanqFDPw
煤浆制备能力需和气化炉相匹配,本项目拟选用三台棒磨机,单台磨机处理干煤 量 43,53t/h,可满足 60 万 t/a 甲醇的需要。
DXDiTa9E3d
为了降低煤浆粘度,使煤浆具有良好的流动性,需加入添加剂,初步选择木质磺 酸类添加剂。
1 / 10
煤浆气化需调整浆的 PH 值在 6,8,可用稀氨水或碱液,稀氨水易挥发出氨,氨气 对人体有害,污染空气,故本项目拟采用碱液调整煤浆的 PH 值,碱液初步采用 42,的 浓度。
RTCrpUDGiT
为了节约水源,净化排出的含少量甲醇的废水及甲醇精馏废水均可作为磨浆水。 b)气化 在本工段,煤浆与氧进行部分氧化反应制得粗合成气。 煤浆由煤浆槽经煤浆加压泵加压后连同空分送来的高压氧通过烧咀进入气化炉, 在气化炉中煤浆与氧发生如下主要反应:
5PCzVD7HxA
CmHnSr+m/2O2—?mCO+(n/2-r)H2+rH2S CO+H2O—?H2+CO2 反应在 6.5MPa(G)、1350,1400?下进行。 气化反应在气化炉反应段瞬间完成,生成 CO、H2、CO2、H2O 和少量 CH4、H2S 等气 体。 离开气化炉反应段的热气体和熔渣进入激冷室水浴,被水淬冷后温度降低并被水 蒸汽饱和后出气化炉;气体经文丘里洗涤器、碳洗塔洗涤除尘冷却后送至变换工段。
jLBHrnAILg
气化炉反应中生成的熔渣进入激冷室水浴后被分离出来,排入锁斗,定时排入渣 池,由扒渣机捞出后装车外运。 气化炉及碳洗塔等排出的洗涤水(称为黑水)送往灰水处理。 c)灰水处理 本工段将气化来的黑水进行渣水分离,处理后的水循环使用。 从气化炉和碳洗塔排出的高温黑水分别进入各自的高压闪蒸器,经高压闪蒸浓缩 后的黑水混合,经低压、两级真空闪蒸被浓缩后进入澄清槽,水中加入絮凝剂使其加
2 / 10
煤气化工艺流程(德士古气化炉)
煤气化工艺流程(德士古气化炉)
煤气化工艺流程 一、 制浆系统 1、系统图 2、工艺叙述 由煤贮运系统来的小于 10mm 的碎煤进入煤贮斗后, 经煤称量给料机称量送入磨 机。 30%的添加剂由人工送至添加剂溶解槽中溶解成 3%的水溶液, 由添加剂溶解槽 泵送至添加剂槽中贮存。 并由添加剂计量泵送至磨机中。在添加剂槽底部设有蒸汽盘 管,在冬季维持添加剂温度在 20--30?,以防止冻结。
b5E2RGbCAP
工艺水由研磨水泵经磨机给水阀来控制送至磨机。煤、工艺水和添加剂一同送入 磨机中研磨成一定粒度分布的浓度约 59%-62%合格的水煤浆。水煤浆经滚筒筛滤去 3mm 以上的大颗粒后溢流至磨机出料槽中,由磨机出料槽泵送至煤浆槽。磨机出料槽和煤 浆槽均设有搅拌器,使煤浆始终处于均匀悬浮状态。 二、气化炉系统 1、系统图 2、工艺叙述 来自煤浆槽浓度为 59%-62%的煤浆,由煤浆给料泵加压,投料前经煤浆循环阀循 环至煤浆槽。投料后经煤浆切断阀送至德士古烧嘴的内环隙。
DXDiTa9E3d p1EanqFDPw
空分装置送来的纯度为 99.6%的氧气经氧气缓冲罐,控制氧气压力为 6.0~6.2MPa,在准备投料前打开氧气手动阀,由氧气调节阀控制氧气流量经氧气放空 阀送至氧气消音器放空。投料后由氧气调节阀控制氧气经氧气上、下游切断阀送入德 士古烧嘴。
RTCrpUDGiT
1/9
水煤浆和氧气在德士古烧嘴中充分混合雾化后进入气化炉的燃烧室中,在约 4.0MPa、1300?条件下进行气化反应。生成以 CO 和 H 为有效成份的粗合成气。粗 25PCzVD7HxA 合成气和熔融态灰渣一起向下,经过均匀分布激冷水的激冷环沿下降管进入激冷 室的水浴中。大部分的熔渣经冷却固化后,落入激冷室底部。粗合成气从下降管和导 气管的环隙上升,出激冷室去洗涤塔。在激冷室合成气出口处设有工艺冷凝液冲洗 水,以防止灰渣在出口管累积堵塞,并增湿粗合成气。由冷凝液冲洗水调 jLBHrnAILg 3 节阀控制冲洗水量为 23m/h。 激冷水经激冷水过滤器滤去可能堵塞激冷环的大颗粒,送入位于下降管上部的激 冷环。激冷水呈螺旋状沿下降管壁流下进入激冷室。
xHAQX74J0X
激冷室底部黑水,经黑水排放阀送入黑水处理系统,激冷室液位控制在 50--55%。在开车期间,黑水经黑水开工排放阀排向真空闪蒸罐。
LDAYtRyKfE
在气化炉预热期间,激冷室出口气体由开工抽引器排入大气。开工抽引器底部通 入蒸汽,通过调节预热烧嘴风门和抽引蒸汽量来控制气化炉的真空度,气化炉配备了 预热烧嘴。
Zzz6ZB2Ltk
三、合成气洗涤系统 1、系统图 2、工艺叙述 从激冷水浴出来饱和了水汽的合成气进入文丘里洗涤器,在这里与激冷水泵 送出的黑水混合,使合成气夹带的固体颗粒完全湿润,以便在洗涤塔内能快速除 去。
2/9
煤气化工艺流程德士古气化炉
煤气化工艺流程(德士古气化炉) 煤气化工艺流程 一、制浆系统 1、系统图 二.、 ■-——一 1 ' — 輛”:牛 卜阳⑥乱一 一詡炸一小 s ------ 酬加| 2、工艺叙述 由煤贮运系统来的小于10mm 勺碎煤进入煤贮斗后, 经煤称量给料机称量送入 磨机。30%的添加剂由人工送至添加剂溶解槽中溶解成 3%勺水溶液, 由添加剂溶 解槽泵送至添加剂槽中贮存。 并由添加剂计量泵送至磨机中。在添加剂槽底部设 有蒸汽盘管,在冬季维持添加剂温度在20--30?,以防止冻结。 工艺水由研磨水泵经磨机给水阀来控制送至磨机。煤、工艺水和添加剂一同送 入磨机中研磨成一定粒度分布的浓度约 59%-62治格的水煤浆。水煤浆经滚筒筛滤 去3mm 以上的大颗粒后溢流至磨机出料槽中,由磨机出料槽泵送至煤浆槽。磨机出 料槽和煤浆槽均设有搅拌器,使煤浆始终处于均匀悬浮状态。 2、工艺叙述 来自煤浆槽浓度为59%-62%勺煤浆,由煤浆给料泵加压,投料前经煤浆循环阀 循环至煤浆槽。投料后经煤浆切断阀送至德士古烧嘴的内环隙 空分装置送来的纯度为99.6%的氧气经氧气缓冲罐,控制氧气压力为 6.0~6.2MPa ,在准 、气化炉系统 1、系统图 1滋冷重一
备投料前打开氧气手动阀,由氧气调节阀控制氧气流量经氧气放空阀送至氧气消音器放空。投料后由氧气调节阀控制氧气经氧气上、下游切断阀送入德士古烧嘴。 水煤浆和氧气在德士古烧嘴中充分混合雾化后进入气化炉的燃烧室中,在约 4.0MPa 1300?条件下进行气化反应。生成以CO和H为有效成份的粗合成气。粗2 合成气和熔融态灰渣一起向下,经过均匀分布激冷水的激冷环沿下降管进入激冷室的水浴中。大部分的熔渣经冷却固化后,落入激冷室底部。粗合成气从下降管和导气管的环隙上升,出激冷室去洗涤塔。在激冷室合成气出口处设有工艺冷凝液冲洗水,以防止灰渣在出口管累积堵塞,并增湿粗合成气。由冷凝液冲洗水调 3节阀控制冲洗水量为23m/h。 激冷水经激冷水过滤器滤去可能堵塞激冷环的大颗粒,送入位于下降管上部的激冷环。激冷水呈螺旋状沿下降管壁流下进入激冷室。 激冷室底部黑水,经黑水排放阀送入黑水处理系统,激冷室液位控制在50--55%在开车期间,黑水经黑水开工排放阀排向真空闪蒸罐。 在气化炉预热期间,激冷室出口气体由开工抽引器排入大气。开工抽引器底部通入蒸汽,通过调节预热烧嘴风门和抽引蒸汽量来控制气化炉的真空度,气化炉配备了预热烧嘴。 三、合成气洗涤系统 1、系统图 2、工艺叙述 从激冷水浴出来饱和了水汽的合成气进入文丘里洗涤器,在这里与激冷水泵 送出的黑水混合,使合成气夹带的固体颗粒完全湿润,以便在洗涤塔内能快速除去。 水蒸汽和合成气的混合物进入洗涤塔,沿下降管进入塔底的水浴中。合成气向上穿过水层,大部分固体颗粒沉降到塔底部与合成气分离。上升的合成气沿下降管和导气管的环隙向
(工艺流程)LNG气化站工艺流程图
LNG气化站工艺流程图 如图所示,LNG通过低温汽车槽车运至LNG卫星站,通过卸车台设置的卧式专用卸车增压器对汽车槽车储罐增压,利用压差将LNG送至卫星站低温LNG储罐。工作条件下,储罐增压器将储罐内的LNG增压到0.6MPa。增压后的低温LNG进入空温式气化器,与空气换热后转化为气态天然气并升高温度,出口温度比环境温度低10℃,压力为0.45-0.60 MPa,当空温式气化器出口的天然气温度达不到5℃以上时,通过水浴式加热器升温,最后经调压(调压器出口压力为0.35 MPa)、计量、加臭后进入城市输配管网,送入各类用户。 LNG液化天然气化站安全运行管理 LNG就是液化天然气(Liquefied Natural Gas)的简称,主要成分是甲烷。先将气田生产的天然气净化处理,再经超低温(-162℃)加压液化就形成液化天然气。LNG无色、无味、无毒且无腐蚀性,其体积约为同量气态天然气体积的1/600,LNG的重量仅为同体积水的45%左右。 一、LNG气化站主要设备的特性 ①LNG场站的工艺特点为“低温储存、常温使用”。储罐设计温度达到负196(摄氏度LNG常温下沸点在负162摄氏度),而出站天然气温度要求不低于环境温度10摄氏度。 ②场站低温储罐、低温液体泵绝热性能要好,阀门和管件的保冷性能要好。 ③LNG站内低温区域内的设备、管道、仪表、阀门及其配件在低温工况条件下
操作性能要好,并且具有良好的机械强度、密封性和抗腐蚀性。 ④因低温液体泵启动过程是靠变频器不断提高转速从而达到提高功率增大流量和提供高输出压力,所以低温液体泵要求提高频率和扩大功率要快,通常在几秒至十几秒内就能满足要求,而且保冷绝热性能要好。 ⑤气化设备在普通气候条件下要求能抗地震,耐台风和满足设计要求,达到最大的气化流量。 ⑥低温储罐和过滤器的制造及日常运行管理已纳入国家有关压力容器的制造、验收和监查的规范;气化器和低温烃泵在国内均无相关法规加以规范,在其制造过程中执行美国相关行业标准,在压力容器本体上焊接、改造、维修或移动压力容器的位置,都必须向压力容器的监查单位申报。 二、LNG气化站主要设备结构、常见故障及其维护维修方法 1.LNG低温储罐 LNG低温储罐由碳钢外壳、不锈钢内胆和工艺管道组成,内外壳之间充填珠光沙隔离。内外壳严格按照国家有关规范设计、制造和焊接。经过几十道工序制造、安装,并经检验合格后,其夹层在滚动中充填珠光沙并抽真空制成。150W低温储罐外形尺寸为中3720×22451米,空重50871Kg,满载重量123771№。 (1)储罐的结构 ①低温储罐管道的连接共有7条,上部的连接为内胆顶部,分别有气相管,上部进液管,储罐上部取压管,溢流管共4条,下部的连接为内胆下部共3条,分别是下进液管、出液管和储罐液体压力管。7条管道分别独立从储罐的下部引出。 ②储罐设有夹层抽真空管1个,测真空管1个(两者均位于储罐底部);在储罐顶部设置有爆破片(以上3个接口不得随意撬开)。 ③内胆固定于外壳内侧,顶部采用十字架角铁,底部采用槽钢支架固定。内胆于外壳间距为300毫米。储罐用地脚螺栓固定在地面上。 ④储罐外壁设有消防喷淋管、防雷避雷针、防静电接地线。 ⑤储罐设有压力表和压差液位计,他们分别配有二次表作为自控数据的采集传送终端。 (2)低温储罐的故障及维护 ①内外夹层问真空度的测定(周期一年)
煤气化工艺烧嘴
德士古煤气化工艺烧嘴的浅议 王润斌高级技工助理工程师 (鄂尔多斯金诚泰煤化工气化车间2011-9-5) (关键词:煤气化工艺烧嘴) 概述 “我国石油和化学工业在快速发展的同时,正面临着资源、能源和环境等多重压力”。由于我国石油和天然气短缺,煤炭相对丰富的资源特征,加之国际油价的持续高位运行状态,煤炭在我国的能源和化工的未来发展中所处的地位会变得越来越重要。 目前,煤炭在我国的能源消费比重不断加大,用于发电和工业锅炉及窑炉的比例大约为70%左右,其余主要是作为化工原料及民用生活。随着煤化工技术的不断发展,煤炭作为化工原料的比重将会得到不断的提高。 传统的煤化工特点是高能耗、高排放、高污染、低效益,即通常所说的“三高一低”。随着科技的不断进步,新型的煤气化技术得到了快速的发展,煤炭作为化工原料的重要性得到了普遍的认可。煤化工目前采用的方法主要有三个途径:煤的焦化、煤的气化、煤的液化。由于最终产品的不同,三种途径均有存在的市场。煤焦化的直接产品主要有焦炭、煤焦油及焦炉气,煤气化的直接产品主要有合成气、一氧化碳和氢气,煤液化后可直接得到液体燃料。 煤焦化产业相对比较成熟,煤液化存在直接液化和间接液化两种方法,由于该技术的成熟程度和投资等原因,制约了其产业化和规
模化的进一步发展。随着煤气化技术的不断成熟,特别是加压气化方法的逐步完善和下游产品的多样化,煤气化已成为我国目前煤化工的重中之重。 煤气化所产生的合成气,成为氮肥(主要是尿素)、甲醇、二甲醚、醋酸等过去主要依赖石油化工产品的主要原料,也成为国内目前煤化工所上的主要项目。煤气化除了投资比较小的常压固定床以外,粉煤加压气化(以壳牌和GSP为主要代表)、水煤浆加压气化(以德士古为主要代表)成为众多厂家引进国外节能环保的主要首选技术。国内具有自主知识产权的煤气化技术还有:①粉煤加压气化:西安热工院的干煤粉加压气化技术、陕西联合能源的灰粘聚流化床粉煤气化技术、山西煤化所的灰熔聚流化床粉煤气化技术、北京航天万源的航天炉粉煤气化技术等。②水煤浆加压气化技术:华东理工大学的四对冲烧嘴技术、北京达立科的分级气化技术、西北化工研究院的多元料浆(主要成份是水煤浆)气化技术等。 本作者近年来一直从事于水煤浆气化的工艺及设备作,水煤浆 加压气化装置(包括引进装置和国产化装置)是目前国内广泛使用的煤加压气化技术(占到国内煤加压气化装置的75%以上),气化后得到的合成气主要用于合成氨、尿素、甲醇、醋酸等的原料,也可用于城市煤气及钢铁等其他行业,气化炉烧嘴是该装置中的关键设备之一。 本作者就关于水煤浆气化炉工艺烧嘴的使用及维护方面所进行 的一些工作和思考进行简单的介绍,同时对于该烧嘴的改进方向提供一些个人看法,仅供同行参考。
Shell炉煤气化工艺介绍
Shell炉煤气化工艺介绍 目录 1.概述 1.1.发展历史 1.2. Shell炉煤气化工艺主要特点 2.工艺流程 2.1. Shell炉气化工艺流程简图 2.2.Shell炉气化工艺流程简述 3.气化原理 3.1粉煤的干燥及裂解与挥发物的燃烧气化 3.2.固体颗粒与气化剂(氧气、水蒸气)间的反应3.3.生成的气体与固体颗粒间的反应 3.4.反应生成气体彼此间进行的反应 4.操作条件下对粉煤气化性能的影响 4.1气化压力对粉煤气化性能的影响 4.2氧煤比对粉煤气化性能的影响 4.3蒸汽煤比对粉煤气化性能的影响 4.4.影响加压粉煤气化操作的主要因素 4.5煤组分变化的影响 4.6 除煤以外进料“质量”变化的影响 5.工艺指标 6.Shell炉气化工艺消耗定额及投资估算 7. 环境评价
1.概述 1.1.发展历史 Shell煤气化工艺(Shell Coal Gasfication Process)简称SCGP,是由荷兰Shell国际石油公司(Shell International Oil Products B. V.)开发的一种加压气流床粉煤气化技术。Shell煤气化工艺的发展主要经历了如下几个阶段。 (l)概念阶段20世纪70年代初期的石油危机引发了Shell公司对煤气化的兴趣,1972年Shell公司决定开发煤气化工艺时,对所开发的工艺制定了如下标准: ①对煤种有广泛的适应性,基本可气化世界上任何煤种; ②环保问题少,有利于环境保护; ③高温气化,防止焦油和酚等有机副产品的生成,并促进碳的转化; ④气化装置工艺及设备具有高度的安全性和可靠性; ⑤气化效率高,单炉生产能力大。 根据上述原则,通过固定床、流化床和气流床三种不同连续气化工艺的对比,对今后煤气化工艺的开发形成了如下基本概念: ①采用加压气化,设备结构紧凑,气化强度大; ②选用气流床气化工艺,生产能力大,气化炉结构简单; ③采用纯氧气化,气化温度高,气化效率高,合成气中有效气CO十H2含量高; ④熔渣气化、冷壁式气化炉,熔渣可以保护炉壁,并确保产生的废渣无害, ⑤对原料煤的粒度无特殊要求,干煤粉进料,有利于碳的转化。 (2)小试试验1976年Shell在荷兰阿姆斯特丹建成了规模为6t/d煤的小试装置,该装置的主要任务是进行煤种试验,验证Shell煤气化理论,为工艺模型的开发提供基础数据,并进行材料试验和煤气净化方法试验,收集基本的环保数据。在其主要试验期间(1978-1983年),先后对21个煤种进行了气化试验。目前该装置仍可根据需要进行特定煤种评价及试验。(3)中试装置在小试试验的基础上,于1978年Shell在原联邦德国的汉堡一哈尔堡(Ham- burg-Harburg)壳牌炼油厂内建设了一套日处理150t煤中试装置。其主要任务是进行不同煤种的气化试验,与小试试验结果关联并验证煤气化数据和工艺模型,进行相关的设备试验,确定煤气化的关键设备(如:气化炉、煤气冷却器、烧嘴、加料及排渣设备及阀门等)的设计原则,为工业化装置的设计提供数据,同时为生产装置积累操作经验、开发安全操作程序。中试装置累计进行了6000h(包括1000h的连续运转)的气化试验,于1983年结束运转。 (4)工业示范装置在汉堡中试的基础上,对气化和煤气冷却系统的设计进行了大幅度的改进,并在美国休斯顿郊区壳牌的Deer Park总厂建设了一套命名为SCGP-1的粉煤气化工业示范装置,该装置于1983年开始设计,1986年开始运转,气化规模为250 - 400t/d煤,气化压力2^-4MPa,约日产32. 5 X 104 m”中热值煤气和16t/h蒸汽。SCGP-1示范装置的主要任务是验证Shell煤气化工艺技术,包括工艺特性及设备可靠性,进一步开发商业化生产的操作技能和经验。SC(aP-1气化装置的示范试验装置累计运行15000h,最长连续运行1500h,气化了大约18种煤(其中包括褐煤和石油焦),获得了比期望值更好的工艺效果。该示范装置于1991年关闭。 (5)工业化应用1993年采用Shell煤气化工艺的第一套大型工业化生产装置在荷兰布根伦 (Buggenum)市的Demkolec建成,用于整体煤气化燃气一蒸汽联合循环发电,发电量为250MWo设计采用单台气化炉和单台废热锅炉,气化规模为2000t/d煤。煤电转化总(净)
煤气化工艺预案的选择
初探煤气化工艺方案的选择 1 几种煤气化工艺及特点介绍 煤气化是煤化工的龙头技术,是煤洁净利用技术的重要环节,C1化学的基础。煤气化技术是进展煤基化学品、煤基液体燃料、联合循环发电、多联产系统、制氢、燃料电池等过程工业的基础,是这些行业的共性技术、关键技术和龙头技术,对我国经济和保障国家安全具有重要的战略意义。 煤气化过程采纳的气化炉炉型,目前要紧有以下3种: 固定床﹙UGI、鲁奇﹚;
流化床﹙灰熔聚、UGAS、鲁奇CFB、温克勒、KBR、恩德等﹚; 气流床﹙Texaco、Shell、GSP、PRENFLOW、国产新型水煤浆、二段干煤粉、航天炉等﹚。 1.1固定床制气工艺 1.1.1常压固定床间歇制气工艺 工艺特点是:常压气化,固体加料10-50mm,固体排渣,间歇气化,空气和蒸汽作气化剂,吹风和制气时期交替进行,适用原料白煤和焦碳,气化温度800~1000℃。代表炉型有美国的U.G.I型和前苏联的U.G.Ⅱ型。工艺过程都比较熟悉,那个地点从略。 技术优点:历史悠久,技术成熟,设备简单,投资省,生产经验丰富。 技术缺点:技术落后,原料动力消耗高,炭转化率低70~75%,产品成本高,生产强度低,程控阀门多,维修工作量大,废气、废水排放多,污染严峻,面临淘汰。 1.1.2常压固定床连续制气 常压固定床连续制气工艺的技术特点:常压气化,固体加料,床体排渣,连续制气,富氧空气﹙氧占50%﹚或氧气加蒸汽做气化剂,无废气排放,适用煤种白煤和焦碳。
技术优点是:连续制气,炉床温度稳定,约为900~1150℃,操作简单,程控阀门少,维修费用低,生产强度大,碳转化率高,约80~84% 。 技术缺点:需要空分装置,投资比较大。 固定床连续制气工艺的技术突破在于以氧气或富氧空气加蒸汽做气化剂,由于气化剂中氧含量的增加,气化反应过程中,燃烧产生的热量与煤的气化和蒸汽分解所需要的热量能够实现平衡,能够得到稳定的反应温度和固定的反应床层,能够实现连续制气,不用专门吹风,无废气排放,生产强度和能源利用率都有了专门大的提高。 1.1.3 固定床加压气化工艺:前西德鲁奇公司(Lurgi)开发。 工艺特点:加压气化,固体加料,固体排渣,连续气化,氧气和蒸汽作气化剂,设有加压的煤锁斗和灰储斗,适用煤种:褐煤、次烟煤、活性好的弱粘结煤。 技术优点:加压气化3.1 MPa,生产强度大,碳转化率高约90%。 技术缺点:反应温度略低700~1100 ℃,甲烷含量较高,煤气当中含有焦油和酚类物质,气体净化和废水处理复杂,流程较长,投资比较大。