模板试剂影响
不同模板剂对纳米氧化铁形貌及硫化性能的影响

2018年第37卷第5期 CHEMICAL INDUSTRY AND ENGINEERING PROGRESS·1831·化 工 进展不同模板剂对纳米氧化铁形貌及硫化性能的影响史磊,冯宇,李阳,张帅国,武蒙蒙,米杰(太原理工大学煤科学与技术教育部与山西省重点实验室,山西 太原 030024)摘要:以Fe(NO 3)3·9H 2O 和CO(NH 2)2为原料,添加不同模板剂,即丙三醇、十六烷基三甲基溴化铵(CTAB )和酒石酸,由水热反应制得纯相α-Fe 2O 3。
采用X 射线衍射分析(XRD )、扫描电子显微镜(SEM )、氮吸附(BET )表征手段对产物的物相组成、微观形貌及孔结构进行了分析与对比。
结果表明,添加丙三醇、CTAB 及酒石酸为模板剂后,分别得到具有棒状、球状及长方体形貌的α-Fe 2O 3。
利用微型固定床硫化装置对不同模板剂制备的纳米α-Fe 2O 3分别进行脱除H 2S 的实验,结果表明,添加3种模板剂后得到的脱硫剂都具有较高的硫化性能。
其中,添加酒石酸得到的α-Fe 2O 3的孔隙结构更加丰富,其硫化性能要优于另外两种,穿透时间可达到350min ,硫容为11.5g/100g 。
本文通过添加不同的模板剂,制备得到了具有不同形貌的脱硫剂活性组分,提高了硫化性能,为实现脱硫剂高效脱硫提供了思路。
关键词:纳米氧化铁;水热;表面活性剂;硫化;吸附剂;形貌中图分类号:TQ02 文献标志码:A 文章编号:1000–6613(2018)05–1831–06 DOI :10.16085/j.issn.1000–6613.2017-1340Impacts of different templates on the morphologies and sulfurationperformances of nano ferric oxidesSHI Lei ,FENG Yu ,LI Yang ,ZHANG Shuaiguo ,WU Mengmeng ,MI Jie(Key Laboratory of Coal Science and Technology of Shanxi Province and Ministry of Education ,Taiyuan University ofTechnology ,Taiyuan 030024,Shanxi ,China )Abstract :Nano ferric oxides with different morphologies were successfully prepared via a facile hydrothermal method. Analytical grade Fe(NO 3)3·9H 2O ,CO(NH 2)2 and different template agent including glycerol ,cetyltrimethyl ammonium bromide (CTAB )and tartaric acid were employed as raw materials. X-ray diffraction (XRD ),scanning electron microscope (SEM )and nitrogen adsorption-desorption isotherms (BET ) were used to characterize the phase composition ,morphologies and pore structure of as-prepared products. The results revealed that the obtained pure Fe 2O 3 crystals were spherical ,rod-like or cuboid structures. Moreover ,the template agent played an important role in controlling the shape and size of the products. The desulfurization behaviors were also investigated in a micro reactor. The three sorbents with different morphologies all showed excellent H 2S capture ability. Specifically ,the sorbent used tartaric acid as template agent showed enhanced desulfurization performance than others ,of which ,the breakthrough time reached 350min ,and the corresponding sulfur capacity was 11.5g/100g sorbent. In this paper ,we prepared desulfurizer active group with different morphologies by adding template agent ,which could increase the sulfuration properties ,and provide new thoughts for high efficiency desulfurization.Key words :nano ferric oxide ;hydrothermal ;surfactants ;sulfuration ;sorbents ;morphology 。
试剂风险报告分析模板
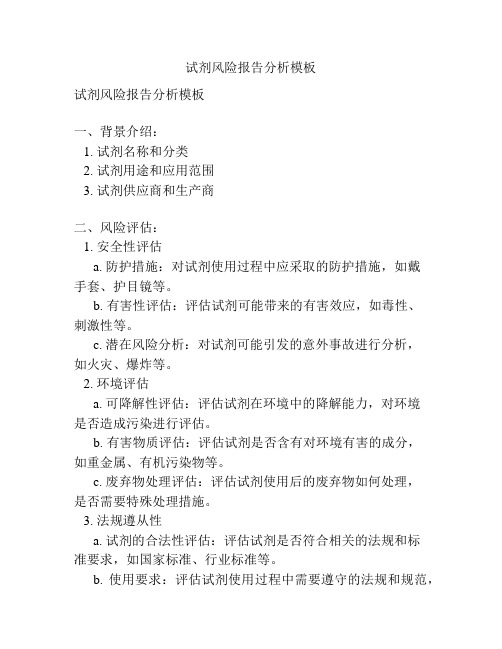
试剂风险报告分析模板试剂风险报告分析模板一、背景介绍:1. 试剂名称和分类2. 试剂用途和应用范围3. 试剂供应商和生产商二、风险评估:1. 安全性评估a. 防护措施:对试剂使用过程中应采取的防护措施,如戴手套、护目镜等。
b. 有害性评估:评估试剂可能带来的有害效应,如毒性、刺激性等。
c. 潜在风险分析:对试剂可能引发的意外事故进行分析,如火灾、爆炸等。
2. 环境评估a. 可降解性评估:评估试剂在环境中的降解能力,对环境是否造成污染进行评估。
b. 有害物质评估:评估试剂是否含有对环境有害的成分,如重金属、有机污染物等。
c. 废弃物处理评估:评估试剂使用后的废弃物如何处理,是否需要特殊处理措施。
3. 法规遵从性a. 试剂的合法性评估:评估试剂是否符合相关的法规和标准要求,如国家标准、行业标准等。
b. 使用要求:评估试剂使用过程中需要遵守的法规和规范,如使用许可、报备等要求。
c. 监管机构指导建议:评估监管机构对试剂使用的指导建议,如安全操作手册、使用指南等。
三、风险控制:1. 个人防护:详细列出试剂使用过程中需要采取的个人防护措施,如穿防护服、戴口罩等。
2. 应急预案:制定试剂使用过程中的应急预案,包括事故发生时的处置方法、紧急联系方式等。
3. 废弃物处理:制定试剂使用后废弃物的处理方法和要求,以确保环境不受污染。
4. 培训和教育:对试剂使用人员进行培训和教育,提高其对试剂风险的认识和防护意识。
四、结论:根据以上评估和控制措施,评估出试剂的风险程度,并给出相应的建议和说明。
五、参考文献:列出用于评估和分析的相关资料和法规。
以上为试剂风险报告分析模板,仅供参考,具体报告内容和格式需根据实际情况进行调整。
PCR失败原因分析
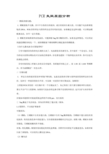
PCR失败原因分析一模板质量问题:1. 模板提取不完整,其中不含你的目的基因,或目的基因含量太低。
可以跑个电泳看看提取的DNA,PCR阳性样品与阴性样品是否有明显差别。
如果确定是这种问题,可以增加模板量试试,但不一定行得通。
2. 模板里面残留某种试剂成份,可能抑制Taq聚合酶的活性,如果是这种情况,可以用试剂盒把模板再纯化一下,或将模板做个梯度稀释以确定最佳的模板量。
3.为什么跑电泳会出现拖带呢?①有可能的因为你的电压调的太高了。
电泳跟很多因素有关,其中就有一个是电压,在适当的电压范围内增加是可以加快迁移速率,但是要是超到一个临界值反而有害。
你可以适当的调低点看看。
②有时候发现上样量太多的话会有拖带。
你看能否回收之后,按1:50 或1:100 等稀释后,再当成模板扩一次怎么样。
二引物问题1. 样品自身的基因型差异导致扩增失败。
也就是说你的引物与某些基因型的样品结合的好,而和另一些基因型结合不好。
可以换一对更保守的引物试试。
祝顺利!2.普通PCR所用的一对引物中有一个引物加多了,为正常的三倍只要引物特异性比较好,那么不会产生大的影响。
如果恰巧是加多的这条引物不是很特异的话,也许会扩出来非特异带。
在制备单链探针时候就采取这样的不对称pcr,没关系的三 Taq酶处于失活状态。
因为活性降低了能出现二聚体。
1.1假阴性,不出现扩增条带可能原因:(1)模板:①模板中含有杂蛋白质;②模板中含有Taq酶抑制剂;③模板中蛋白质没有消化除净,特别是染色体中的组蛋白;④在提取制备模板时丢失过多,或吸入酚,模板本身拷贝数低;⑤模板核酸变性不彻底。
对策:纯化模板;配制有效而稳定的消化处理液,其程序亦应固定不宜随意更改;如果怀疑污染了抑制剂,可以使用乙醇沉淀DNA。
(2)酶失活对策:需更换新酶,或新旧两种酶同时使用,以分析是否因酶的活性丧失或不够而导致假阴性。
需注意的是有时忘加Taq酶或溴乙锭。
(3)引物:引物质量、引物的浓度、两条引物的浓度是否对称,是PCR失败或扩增条带不理想、容易弥散的常见原因。
PCR反应体系模板浓度

PCR反应体系模板浓度引言PCR(聚合酶链式反应)是一种重要的分子生物学技术,广泛应用于生物医学研究、基因工程和临床诊断中。
PCR反应体系中的模板浓度是影响PCR反应效果的重要因素之一。
本文将介绍PCR反应体系模板浓度的相关知识,并探讨其在PCR实验中的影响和优化方法。
PCR反应体系模板浓度的意义PCR反应的目的是通过反复复制DNA模板,快速扩增特定DNA序列。
模板浓度直接影响PCR反应的效率和特异性。
若模板浓度过低,PCR反应可能无法得到充分扩增;而模板浓度过高,则可能导致非特异扩增和产物过多。
因此,合理调控PCR反应体系中的模板浓度非常重要。
模板浓度的确定方法常用的确定模板浓度的方法包括比色法、浓度计测定法和凝胶电泳法等。
其中,比色法是一种快速估测模板浓度的方法,通过观察DNA溶液的颜色深浅来判断浓度。
浓度计测定法可以精确测定DNA的浓度,但需要专业仪器。
凝胶电泳法是一种常用的手段,可以根据DNA在凝胶中的行迹快慢来判断模板浓度。
模板浓度对PCR反应的影响扩增效率模板浓度直接影响PCR反应的效率。
一般而言,适中的模板浓度能够提高PCR反应的扩增效率。
若模板浓度过低,反应产物过少,无法被有效检测;若模板浓度过高,反应产物过多,可能导致假阳性和非特异扩增。
特异性合适的模板浓度可以提高PCR反应的特异性。
当模板浓度适宜时,扩增产物主要来自于目标序列,特异性较高。
然而,模板浓度过高可能导致非特异性扩增,产生非特异性产物,影响稳定性和解析性。
模板浓度的优化为了获得更好的PCR反应结果,需要对PCR反应体系中的模板浓度进行优化。
以下是一些建议:适当稀释若已确定的模板浓度较高,可以通过适当稀释来降低模板浓度。
稀释后的模板浓度应能够保证PCR反应产物的特异性,同时减少非特异性扩增的可能性。
优化试剂浓度优化PCR反应体系中其他试剂的浓度,如聚合酶、引物、缓冲液等。
适当调整这些试剂的浓度,可以进一步提高PCR反应的特异性和扩增效率。
金属有机骨架材料的合成与应用文献综述
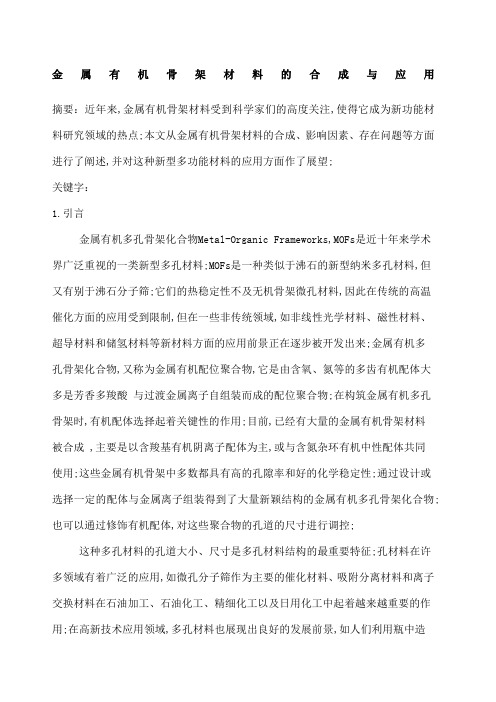
金属有机骨架材料的合成与应用摘要:近年来,金属有机骨架材料受到科学家们的高度关注,使得它成为新功能材料研究领域的热点;本文从金属有机骨架材料的合成、影响因素、存在问题等方面进行了阐述,并对这种新型多功能材料的应用方面作了展望;关键字:1.引言金属有机多孔骨架化合物Metal-Organic Frameworks,MOFs是近十年来学术界广泛重视的一类新型多孔材料;MOFs是一种类似于沸石的新型纳米多孔材料,但又有别于沸石分子筛;它们的热稳定性不及无机骨架微孔材料,因此在传统的高温催化方面的应用受到限制,但在一些非传统领域,如非线性光学材料、磁性材料、超导材料和储氢材料等新材料方面的应用前景正在逐步被开发出来;金属有机多孔骨架化合物,又称为金属有机配位聚合物,它是由含氧、氮等的多齿有机配体大多是芳香多羧酸与过渡金属离子自组装而成的配位聚合物;在构筑金属有机多孔骨架时,有机配体选择起着关键性的作用;目前,已经有大量的金属有机骨架材料被合成 ,主要是以含羧基有机阴离子配体为主,或与含氮杂环有机中性配体共同使用;这些金属有机骨架中多数都具有高的孔隙率和好的化学稳定性;通过设计或选择一定的配体与金属离子组装得到了大量新颖结构的金属有机多孔骨架化合物;也可以通过修饰有机配体,对这些聚合物的孔道的尺寸进行调控;这种多孔材料的孔道大小、尺寸是多孔材料结构的最重要特征;孔材料在许多领域有着广泛的应用,如微孔分子筛作为主要的催化材料、吸附分离材料和离子交换材料在石油加工、石油化工、精细化工以及日用化工中起着越来越重要的作用;在高新技术应用领域,多孔材料也展现出良好的发展前景,如人们利用瓶中造船路线,在微孔分子筛孔道中制备染料复合体,为进一步研究固体微激光器提供基础;通过纳米化学反应路线技术,在微孔分子筛笼中制备Cd4S4 纳米团簇或通过“嫁接”或“锚装”等方法组装具有特定功能与性质的复杂分子、配合物、簇合物、金属有机化合物、超分子、纳米态、齐聚体与高聚物等;半个世纪以来,随着多孔材料类型与品种的不断扩充与发展,应用领域的拓宽与需求的增加,研究领域和学科间交叉与渗透的日益加强及深化,研究方法与现代试验技术的进步,大大推动了多孔材料化学内涵的深入与学科面的拓宽;2、合成合成一种新的MOFs时,除了要考虑的几何因素外,最重要的是维持骨架结构的完整性;因此,需要找到足够温和的条件以维持有机配位体的功能和构造,而又有足够的反应性建立金属与有机物之间的配位键;原料的选择首先,金属组分主要为过渡金属离子,使用得较多的是Zn2+、Cu2+、Ni2+、Pd2+、Pt2+、Ru2+和Co2+ 等,所使用的价态多为二价;其次,有机配体应至少含有一个多齿型官能团,如CO2 H、CS2H 、NO2、SO3H、PO3H 等;多齿型官能团使用较多的为CO2H ,如对苯二甲酸BDC 、均三苯甲酸BTC 、草酸、琥珀酸等;选择合适的有机配体不仅可以形成新颖结构的MOFs ,而且也可能产生特殊的物理性质;另外,溶剂在合成过程中可以起溶解和对配体去质子化的作用;金属盐和多数配体都是固体,所以需要溶剂对其进行溶解;在金属离子和配体配位之前,配体如羧酸需要去质子化,因此多选用碱性溶剂;目前,使用较多的去质子化碱为有机胺类物质,如三乙胺TEA 、N , N-2-二甲基甲酰胺DMF 、N , N-2-二乙基甲酰胺DEF 、N-2-甲基吡咯烷酮等,同时它们又是好的溶剂;近年来,逐渐有了用氢氧化钠等强碱去质子化;有时,溶剂也能作为配体与金属离子进行配位,或与其它配体形成氢键等弱的相互作用,这种弱结合的分子可以通过加热和真空的方法排除;最后,为了使合成的金属有机骨架具有理想的孔隙,就需要选择适宜的模板试剂;模板试剂有时为单独的物质,有时就是所使用的溶剂;合成方法MOFs 的合成一般分为两种方法:扩散法和溶剂热法;扩散法:将金属盐、有机配体和溶剂按一定的比例混合成溶液,放入一个小玻璃瓶中,将此小瓶置于一个加入去质子化溶剂的大瓶中,封住大瓶的瓶口,然后静置一段时间即可有晶体生成;这种方法条件比较温和,易获得高质量的单晶用于结构分析,但是比较耗时,而且要求反应物的溶解性要比较好,室温下能溶解;溶剂热法:与原来的水热法原理是一样的,只是溶剂不再局限于水;溶剂热法MOFs 的合成,一般是将反应物与有机胺、去离子水、乙醇和甲醇等溶剂混合,放入密封容器如带有聚四氟衬里的不锈钢反应器或玻璃试管中加热,温度一般在100 —200 ℃,在自生压力下反应;随着温度的升高反应物就会逐渐溶解;这种方法反应时间较短,而且解决了反应物在室温下不能溶解的问题;合成中所使用的溶剂尤其是有机溶剂带有不同的官能团、不同的极性、不同的介电常数以及不同的沸点和粘度等,从而可以大大地增加合成路线和合成产物结构的多样性;溶剂热生长技术具有晶体生长完美、设备简单、节省能量等优点,从而成为近年来使用的热点;3. 影响因素合成MOFs时,分子间作用力是很难预测的;因此,就需要努力辨识和修正合成条件,使金属和配体之间能按意想的方式作用并结合;金属离子和配体的浓度、溶剂的极性、pH值和温度的微小的变化都可能导致晶体质量和产率的变化,或产生全新的骨架结构;但是,只要在适的条件下,合成MOFs具有产率高,耗能低,节省时间及溶剂;目前,已有一些高度多孔MOFs被合成;金属离子与配体的摩尔比金属离子与配体的比例再大程度上影响着骨架的性能;一般地,金属离子与配体的摩尔比在1:10到10:1之间;当金属的比例超过配位的化学计量比时,配体能充分地以多齿型配位,而且金属会有不饱和位存在,对于骨架性能的研究尤其是催化作用是非常有利的;反之,配体就可能会全部或部分以单齿型配位,形成的骨架可能没有前者稳定,而且没有或仅有很少量的金属不饱和位;模板试剂和溶剂在骨架的合成中,模板试剂的选择是非常重要的;使用不同的模板试剂可能会产生完全不同结构的骨架;模板试剂的量并不是很关键,能相应较大量地使用,不会影响反应和微孔材料的制备;混合溶剂经常用于调节体系的极性和溶剂-配体交换动力学,影响晶体生长速率;溶剂分子与骨架发生的弱相互作用是稳定骨架的一种有效的方法;通过增加溶剂浓度和搅拌以降低反应时间,可提高产率;去质子化溶剂的选择也是非常重要的,它可能会使酸性配体完全或部分地去质子化,从而使得配体与金属离子的配位方式不同,生成不同的骨架结构;温度和pH值温度:在高温的水热条件和在室温的温和条件下,羧基的配位能力有所不同, 因而生成的骨架结构就不同;在高温条件下羧基是以多齿型配位的,易形成多维结构;而在室温条件下是以单齿型配位的,易形成一维结构;PH值:反应溶液的pH 值不同,生成的骨架结构不同;随着反应的pH值的增大,金属离子桥接氧或羟基的数量就增加,可使骨架单元增大;4.存在问题5.应用由于MOFs材料具有不饱和配位的金属位和比表面积大的特点,使得其在化学工业上有大量的应用,如传感器、催化剂、分离、气体的储存、过滤、膜分离等;催化剂一些MOFs 可以作为催化剂,用于许多类型的反应,如氧化、环氧化、甲氧基化、酰化、羰基化、水合、烷氧基化、脱氢、加氢、异构化、低聚、多聚和光催化等方面;对于MOFs 在催化剂方面的应用已经有不少研究,如环氧丙烷的合成60 ,用MOF25 作为催化剂,混合物氧、氦和丙烯反应10h 后,产率为413 % ,选择性为812 %;Muler 等61 用MOF25 作烷氧基化催化剂,由一缩二丙二醇和环氧丙烷合成了多羟基化合物;用环氧乙烷对一缩甲基丙二醇进行了烷氧基化,也得到了多羟基化合物;他们还由42叔丁基苯甲酸和乙炔合成了乙烯基242叔丁基苯甲酸酯59 ,其中酸的转化率达到94 % ,选择性为83 %;Schlichte 等62 改善了Cu3 BTC 2 的合成,开发了这种材料在醛类和酮类化合物的氰硅烷氧基化合成氰醇中的催化作用,在不同溶剂中,对苯甲醛进行了氰硅烷氧基化,最高产率为55 %;Seo 等63 合成了手性的MOFs 材料,开发了其手性催化作用;气体储存在MOFs 的微孔功能的研究中,结构的稳定性是一个很重要的因素;MOFs 的孔隙是稳定的,当移走客体分子后骨架结构不会改变;在加热的情况下,也能在高于300 ℃以上保持结构不变;由于MOFs材料大部分具有孔隙结构和特殊的构造,在气体的存储方面有潜在的应用;对于MOFs 的存储应用,主要集中在甲烷24 ,64 和氢25 ,65 等燃料气上;Yaghi等22 对有MOF25 骨架结构的16 种MOFs 进行了甲烷吸附性能的研究,这些骨架的孔是均匀、周期性排列的,孔隙率达到9111 %;在36 个大气压、室温下,对甲烷的吸附量可以达到240cm3 STP ·g- 1 ;Yaghi等66 也对几种骨架的吸附氢气的能力做了比较,说明了MOFs 材料对于氢气的存储有较大的潜力,同时也说明了表面积不是吸附量大小的唯一决定因素,功能性基团的存在也起很重要的作用;分离由于多孔材料特有的骨架结构和表面性质,使得其对不同的气体的吸附作用不一样,从而可以对某些混合气体体系进行分离;Kim 等67 合成了甲酸锰化合物,其表面积不是很大,约为240m2Pg ,但是这种金属有机骨架对氮气、氢气、氩气、二氧化碳、甲烷等具有选择性的吸附;对氢气和二氧化碳的吸附能力很强,但对氮气、氩气、甲烷的吸附能力却很弱;这种材料就可以作为一种选择性分子筛,可能会有很重要的工业应用,如从天然气中脱除二氧化碳,从含有氮气、一氧化碳或甲烷的混合气中回收氢气;Bülow 等68 对Cu2BTC 的吸附性能进行了评价,实验表明其吸附性能要好于沸石13X ,并且能分离一氧化碳、二氧化碳和乙烷等混合气体;6.结果与展望综上所述,金属有机骨架材料存在着巨大的潜在应用价值,因此合成具有大孔径、高比表面积的金属有机骨架结构已成为多孔材料研究领域的一个热点;目前主要挑战是如何合成稳定的金属有机骨架材料,因为合成金属有机骨架材料主要借助以下几种非共价键的作用力:氢键、配位共价键、静电和电荷转移引力以及芳香π堆积作用135 ,上述这些非共价键的作用强度远不能与共价键相比;金属有机骨架材料不稳定的另一个原因是由于客体溶剂分子直接与金属配位,当客体脱附后很容易引起配位结构发生改变,所以获得稳定的金属有机多孔材料的一个策略是阻止溶剂分子直接配位到金属中心上;因此现在虽已报道合成了大量的金属有机骨架材料,但它们中的绝大多数除掉客体分子后其骨架结构会发生改变甚至坍塌;这就要求合理选择次级建筑单元、有机配体和活性金属中心来获得有价值的孔材料;此外,改变金属有机骨架材料结构中的配体可以调控孔结构的柔性,可获得不同的吸附性质;这些材料的工业化合成方法的研究及其在工业应用方面的开发将是科学家们面临的另一个挑战;。
输血科试剂确认记录模板

输血科试剂确认记录模板1. 背景介绍输血科试剂确认记录模板是为了确保输血操作的安全性和准确性而设计的一项重要工具。
在输血过程中,涉及到多个环节,包括试剂准备和使用等。
为了提高工作效率和确保操作准确,需要有一个规范化的模板来记录试剂的确认情况。
2. 模板结构2.1 试剂信息试剂名称生产日期有效期至批号2.2 确认记录2.2.1 试剂准备确认试剂名称操作人员准备日期时间2.2.2 试剂使用确认试剂名称操作人员使用日期时间2.2.3 试剂废弃确认试剂名称操作人员废弃日期时间3. 使用指南3.1 填写试剂信息填写试剂的名称、生产日期、有效期至和批号等信息,确保信息的准确性和完整性。
3.2 试剂准备确认当准备试剂时,操作人员需填写试剂名称、操作人员和准备日期时间等信息,并确认准备的试剂和信息是否与模板相符。
3.3 试剂使用确认操作人员在使用试剂前,应填写试剂名称、操作人员和使用日期时间等信息,并确认试剂的使用是否符合要求。
3.4 试剂废弃确认试剂使用完毕后,操作人员需填写试剂名称、操作人员和废弃日期时间等信息,并确认试剂废弃的过程是否安全、规范。
4. 观点和理解4.1 试剂确认的重要性试剂确认记录模板在输血科工作中具有重要意义。
通过对试剂信息的准确记录和确认,可以避免因试剂错误使用而引发不必要的风险。
试剂确认可以提高输血操作的可靠性,保障病患的安全。
4.2 规范化管理的必要性实施规范化的试剂确认记录可以帮助提高运行效率和工作质量,减少操作失误的可能性。
通过制定统一的模板,有利于减少个体差异和主观判断,确保操作的一致性和准确性。
4.3 持续改进的重要性试剂确认记录模板是一个不断完善和改进的过程。
输血科应不断总结经验,根据实际情况对模板进行调整和优化,以适应输血操作的不断变化和发展。
结语输血科试剂确认记录模板对于维护输血操作的安全和标准化具有重要作用。
通过试剂信息的准确记录和确认,可以确保输血过程的可靠性和精确性。
Pcr生物安全风险评估

Pcr生物安全风险评估
PCR技术是一种常用的生物技术方法,用于复制和扩增DNA
片段。
在进行PCR实验时,需要注意以下生物安全风险:
1. 污染风险:PCR实验需要使用DNA模板和引物,以及其他
试剂和缓冲液。
如果这些试剂受到细菌、真菌或病毒的污染,可能导致PCR产物的假阳性结果或其他偏差。
2. 散播风险:PCR实验中产生的PCR产物是经过扩增的DNA
片段,如果没有妥善的处理和处置,可能会导致DNA的散播
和外溢。
这可能会对环境和人类健康产生潜在影响。
3. 感染风险:PCR实验需要处理和操作生物材料,如DNA和
细菌菌株。
如果操作不当或无适当的安全操作措施,可能会导致实验人员受到感染的风险。
为了降低PCR实验的生物安全风险,应采取以下措施:
1. 严格的实验室管理:确保实验室符合相关的生物安全规范和标准,如戴上实验室制服、使用个人防护装备、定期检查和清洁实验室设施等。
2. 生物材料处理:正确处理和处置PCR产物和废弃物,如使
用严密封闭的容器储存和运输PCR产物,遵循正确的废物处
理程序,以防止散播和外溢。
3. 操作技术:严格遵循PCR实验的操作规程和标准操作程序,
掌握正确的技术和操作方法,避免污染和感染风险。
4. 定期检查和监测:定期检查实验室设施和操作程序,确保其符合最新的生物安全要求。
定期进行实验室内环境和生物材料的检测和监测,以及实验人员的健康状况检查。
总结起来,PCR实验存在生物安全风险,但通过严格的实验室管理、生物材料处理、操作技术和定期检查和监测,可以降低这些风险。
pcr模板浓度过高

pcr模板浓度过高PCR(聚合酶链式反应)是一种在分子生物学和遗传学中常用的技术,它可以扩增DNA序列。
但在实际操作中,我们可能会遇到PCR模板浓度过高的问题。
本文将探讨PCR模板浓度过高的原因及解决方法。
一、PCR模板浓度过高的原因1.模板DNA浓度过高PCR模板DNA浓度是进行PCR反应的一个重要指标。
如果模板DNA浓度过高,将导致PCR反应中模板DNA扩增数量过多,容易导致PCR产物的数量过多和杂交效率低下。
2.反应体积不合适PCR反应体积小,则模板DNA的原始浓度相对较高,使扩增反应的特异性和功效都会受到影响,而PCR反应体积大,则模板DNA的原始浓度相对较低,可能会导致PCR反应产物过少。
3.反应条件不恰当PCR反应温度、时间和放大产物的循环次数等参数不当,均有可能导致PCR模板浓度过高的问题。
二、PCR模板浓度过高的危害1.影响PCR扩增效率当PCR模板浓度过高时,PCR混合液中的模板DNA过多,增加了PCR扩增的难度,降低了PCR效率,从而导致PCR扩增效果不佳。
2.影响PCR检测结果PCR模板浓度过高会导致PCR反应过程中,模板DNA扩增过多,PCR产物的数量较多,产生“混带”的效果,影响PCR检测结果的准确性。
三、如何解决PCR模板浓度过高的问题1. 通过稀释样品解决。
将高浓度模板DNA进行适当的稀释,稀释倍数的选择主要取决于试验目的和所需条件。
试验目的不同,需要稀释的倍数也不一样。
2.优化PCR反应条件解决。
通过优化PCR反应温度、时间和放大产物的循环次数等参数,控制PCR反应体系中模板DNA的扩增数量,从而解决PCR模板浓度过高的问题。
3.使用内部控制解决。
添加适当量的内部控制试剂,以稳定PCR反应体系中的模板DNA含量,使其在一定范围内不发生变化,从而避免PCR模板浓度过高的问题。
总之,PCR模板浓度过高是进行PCR反应过程中常见的问题。
我们可以通过稀释、优化反应条件和使用内部控制等方法解决此问题。
- 1、下载文档前请自行甄别文档内容的完整性,平台不提供额外的编辑、内容补充、找答案等附加服务。
- 2、"仅部分预览"的文档,不可在线预览部分如存在完整性等问题,可反馈申请退款(可完整预览的文档不适用该条件!)。
- 3、如文档侵犯您的权益,请联系客服反馈,我们会尽快为您处理(人工客服工作时间:9:00-18:30)。
A Versatile Family of Interconvertible Microporous Chiral Molecular Frameworks:The First Example of Ligand Control of Network ChiralityC.J.Kepert,†T.J.Prior,and M.J.Rosseinsky*Contribution from the Department of Chemistry,Uni V ersity of Li V erpool,Li V erpool L697ZD,United Kingdom,and School of Chemistry,Uni V ersity of Sydney,NSW2006,AustraliaRecei V ed October26,1999.Re V ised Manuscript Recei V ed February1,2000Abstract:Two families of molecular frameworks which grow as homochiral single crystals are described. Both consist of multiple interpenetration of the three-connected chiral(10,3)-a(Y*)network and result from the tridentate coordination of the1,3,5-benzenetricarboxylate(btc)ligand to octahedral metal centers which act as linear connectors.The nature of the interpenetration is controlled by the auxiliary ligands bound in the equatorial plane of the metal center.Ethylene glycol(eg)binds in a unidentate fashion to form phase A which has28%accessible solvent volume and contains four interpenetrating(10,3)-a networks.1,2-Propanediol(1,2-pd)coordinates as a bidentate ligand to yield a phase B with a greatly enhanced51%of solvent accessible volume,because only two(distorted)(10,3)-a′networks interpenetrate.Ligands in the void space and bound to the metal center can both be liberated thermally:the kinetics of this process allow isolation of microporous desolvated crystalline A and B.The porous phases lose crystallinity reversibly upon further loss of ligands bound to the equatorial metal:crystallinity is restored upon exposure to the vapors of simple alcohols,which can also effect conversion of B to A.Both phases present interpenetrating network topologies that are unique to chemistry and adopt space groups that are new for molecular solids:A crystallizes in P4232and B adopts I4132.B can be grown homochirally from enantiomerically pure diol template.The stereochemistry of the alcohol bound to the metal controls the helicity of the chiral framework.The structure determination of the 1,2-propanediol phase represents the first demonstration that chiral molecules can specifically template helix handedness in a chiral porous framework solid.IntroductionMolecular framework solids formed by inorganic coordination polymer networks1are currently attracting considerable attention in view of possible applications in selective sorption and catalysis.2Frameworks which are robust to guest exchange,3 are microporous,4and display zeolite-like structural stability to guest removal5are now known.The extent to which the network structure and chemical nature of the pores can be manipulated to prepare porous solids with structures and sorptive/exchange properties different from those of the well-known zeolite and ALPO framework hosts is as yet uncertain.One area in which traditional frameworks have not made considerable impact is enantioselective sorption and catalysis due to the difficulty in preparing such phases in chiral structures without enantiomer intergrowth within single crystallites and with void volume accessible either by template removal or exchange.6Chiral functionalization of achiral zeolitic frame-works has been demonstrated.7The aluminosilicate zeolite 8 and the titanosilicate ETS-109are multiphasic and retain enantiopurity only over a few crystallographic layers.Single-crystal growth has allowed resolution of chiral zincophos-*Address correspondence to this author at the University of Liverpool. Phone:44(0)1517943499.Fax:44(0)1517943587.E-mail: m.j.rosseinsky@.†University of Sydney.(1)(a)Hoskins,B.F.;Robson,R.J.Am.Chem.Soc.1990,112,1546.(b)Batten,S.R.;Robson,R.Angew.Chem.,Int.Ed.Engl.1998,37,1461.(c)Blake,A.J.;Champness,N.R.;Hubberstey,P.;Li,W.-S.;Withersby, M.A.;Schro¨der,M.Coord.Chem.Re V.1999,183,117-138.(d)Choi,H. J.;Suh,M.P.J.Am.Chem.Soc.1998,120,10628.(2)(a)Barton,J.B.;Bull,L.M.;Klemperer,W.G.;Loy,D.A.; McEnaney,B.;Misono,M.;Monson,P.A.;Pez,G.;Scherer,G.W.;Vartuli, J.C.;Yaghi,O.M.Chem.Mater.1999,11,2633-2656.(b)Yaghi,O.M.; Li,H.L.;Davis,C.;Richardson,D.;Groy,T.L.Acc.Chem.Res.1998, 31,474.(c)Yaghi,O.M.;Li,G.M.;Li,H.L.Nature1995,378,703-706.(d)Fujita,M.;Kwon,Y.J.;Washizu,S.;Ogura,K.J.Am.Chem. Soc.1994,116,1151-1152.(3)(a)Chui,S.S.Y.;Lo,S.M.F.;Charmant,J.P.H.;Orpen,A.G.; Williams,I.D.Science1999,283,1148-1150.(b)Kondo,M.;Yoshitomi, T.;Seki,K.;Matsuzaka,H.;Kitagawa,S.Angew.Chem.,Int.Ed.Engl. 1997,36,1725-1727.(4)(a)Reineke,T.M.;Eddaoudi,M.;O’Keeffe,M.;Yaghi,O.M.Angew. Chem.,Int.Ed.Engl.1999,38,2590-2594.(b)Li,H.;Eddaoudi,M.;Groy, T.L.;Yaghi,O.M.J.Am.Chem.Soc.1998,120,8571-8572.(c)Kondo, M.;Okubo,T.;Asami,A.;Noro,S.;Yoshitomi,T.;Kitagawa,S.;Ishii,T.; Matsuzaka,H.;Seki,K.Angew.Chem.,Int.Ed.Engl.1999,38,140-143.(d)Eddaoudi,M.;Li,H.L.;Reineke,T.;Fehr,M.;Kelley,D.;Groy,T. L.;Yaghi,O.M.Top.Catal.1999,9,105-111.(5)(a)Kepert,C.J.;Rosseinsky,mun.1999,375.(b) Venkataraman,D.;Gardner,G.B.;Lee,S.;Moore,J.S.J.Am.Chem. Soc.1995,117,11600-11601.(c)Li,H.;Eddaoudi,M.;O’Keeffe,M.; Yaghi,O.M.Nature1999,402,276-279.(6)(a)Baiker,A.Curr.Opin.Solid State Sci.1998,3,86.(b)Akporiaye,D.E.J.Chem.Soc.,mun.1994,1711.(c)Davis,M.E.;Lobo R.F.Chem.Mater.1992,4,756.(7)(a)Feast,S.;Bethell,D.;Page,P.C.B.;King,F.;Rochester,C.H.; Siddiqui,M.R.H.;Willock,D.J.;Hutchings,G.J.J.Chem.Soc.,Chem. Commun.1995,2409-2411.(b)Ogunwumi,S. B.;Bein,T.Chem. Commun.1997,901.(c)Johnson,B.F.G.;Raynor,S.A.;Shephard,D. S.;Maschmeyer,T.;Thomas,J.M.;Sankar,G.;Bromley,S.;Oldroyd,R.; Gladden,L.;Mantle,mun.1999,1167-1168.(8)Newsam,J.M.;Treacy,M.M.J.;Koetsier,W.T.;de Gruyter,C.B. Proc.R.Soc.London,Ser.A1988,420,375.(9)Anderson,M.W.;Terasaki,O.;Ohsuna,T.;Philippou,A.;MacKay, S.P.;Ferreira,A.;Rocha,J.;Lidin,S.Nature1994,367,347-351.5158J.Am.Chem.Soc.2000,122,5158-516810.1021/ja993814s CCC:$19.00©2000American Chemical SocietyPublished on Web05/16/2000phates,10,11the aluminophosphates Co(tn)3‚[Al3P4O16]‚2H2O (GTex-2)12and trans-Co(dien)2‚Al3P4O16‚3H2O(GTex-3),13and the tin(II)phosphate[CN3H6][Sn4P3O12](GUAN-SnPO).14The gallophosphate d-Co(en)3‚[H3Ga2P4O16]15and aluminophosphate d-Co(en)3‚[Al2P4O16]‚3H2O16were grown in enantiomerically pure form using a resolved template;however,all these examples collapse irreversibly upon calcination.A series of chiral but dense cobalt phosphates XCoPO4(X)NH4,Na,K, Rb)have been reported recently.17Atom site ordering leads to chirality in the porous gallium germanate(KGaGeO4)6‚7H2O (UCSB-7K).18Chiral templation of zincophosphate growth has recently resulted in two mesoporous phases of unknown structure.19Of particular note in the quest for organic-based chiral frameworks has been the recent observation of porosity in cross-linked protein crystals.20The molecular frameworks Cd(tcm)[B(OMe)4]‚x MeOH,21Ni(tpt)(NO3)2,22[{Ni(bpy)(Ar-COO)2(MeOH)2}n],23[{Zn(sala)(H2O)2}2]‚2H2O,24Zn2(btc)-(NO3)‚H2O‚5EtOH,25and Ag(hat)ClO4‚3CH3NO226are all chiral,the last two displaying the helical(10,3)-a network27and retaining crystallinity on solvent removal.A high degree of robustness to solvent removal has been reported recently in coordination frameworks incorporating chirally functionalized ligands.28In this paper the combination of alcohol and pyridine equatorial ligands at an octahedral metal center connecting two trans diaxial btc ligands is shown to be a flexible recipe to prepare a wide range of chiral porous solids with large extraframework void regions.The neutral M3(btc)2framework adopts the(10,3)-a network(also known as Y*,SrSi2,or“Laves Graph”,one of the seven networks of equivalent three-connected points that repeat regularly in space and have the property that the shortest circuit from any point and including any two of the links meeting at the point is a decagon).27The extent of network interpenetration is controlled by whether the alcohol ligands bound equatorially to the metal are uni-or bidentate.This network is intrinsically chiral due to the helices from which it is constructed,making the large void volume of this structure of particular interest.Bulk homochiral samples of enantiopure networks can be prepared from resolved template molecules with the hand of the helix directly controlled by that of the diol bound to the metal.Careful desolvation below145°C affords crystalline samples with both empty chiral pores and vacant coordination sites around the metal centers.Experimental SectionSynthesis:Ethylene Glycol(1,2-Ethanediol),Methanol,Ethanol, and1-Propanol Frameworks.Single crystals of phase A(ethylene glycol)and phase A1(other alcohols)were grown by slow diffusion of pyridine into a stoichiometric2:3solution of H3(btc)(trimesic acid) and M(NO3)2‚6H2O(M)Ni,Co)in the desired alcohol.Three milliliters of this mixture(0.1mmol of H3(btc),0.15mmol of Ni(NO3)2‚6H2O)was placed in one arm of the H-cell(height90mm,crossbar 60mm,circular section diameter15mm)and in the other0.6mL(7.42 mmol)of pyridine.Pure solvent was carefully layered on to each side until the crossbar was full.The cell was then stoppered and left to stand.Crystals of up to100mm3were grown over a1month period.1,2-Propanediol(1,2-pd),2,3-Butanediol(2,3-bd),1,2-Butanediol (1,2-bd),1,2,4-Butanetriol,1,2-Pentanediol,and3-Chloro-1,2-pro-panediol.Diffusion of pyridine vapor into a stoichiometric2:3solution of H3(btc)and Ni(NO3)2‚6H2O in the desired diol/triol yielded octahedral single crystals of phase B up to1mm in length within2 weeks.Two milliliters of alcoholic solutions containing10µmol of H3(btc)and15µmol of Ni(NO3)2‚6H2O were placed in cylindrical specimen jars,dimensions75×25mm.Approximately0.2mL(2.47 mmol)of pyridine was placed in a stoppered vial.The vial was placed inside the jar and its stopper pierced with a single pin prick.The jar was then sealed and left to stand.The different methods for growing crystals of phases A and B are entirely complementary.Either method may be used to produce crystals of each phase.Structure Determination.Single-crystal X-ray diffraction data were recorded on an Enraf-Nonius DIP2000image plate diffractometer with Mo K R radiation at150K.Crystals were quench-cooled in the nitrogen gas cryostream.In the case of the ethylene glycol salt,90images were recorded with successive2°rotations inφand reduced with the HKL suite of programs.29The293K structure of this phase was refined against Cu K R radiation data obtained on an Enraf-Nonius MACH3 four circle diffractometer.Structure solution of the ethylene glycol and methanol salts(phases A and A1,respectively)was by a combination of geometric considerations and Fourier techniques using SHELXL-93.30Structures of the1,2-propanediol and2,3-butanediol salts(phaseB)were solved using Patterson methods in SHELXS-8631and subsequent Fourier syntheses within SHELXL-93.30Details of crystal-lographic work are given in Table1and the accompanying CIF files. Two separate crystals of the1,2-propanediol phase B were examined and gave identical results.Thermogravimetric analyses were performed on a Rheometric Scientific STA1500instrument to study the loss of encapsulated solvent as a function of time and temperature.Samples were heated under flowing nitrogen to600°C ramped at2°C min-1.An atmosphere of laboratory air was used for samples heated at40°C for periods greater than6h.Powder X-ray Diffraction Study of Sorption and Desorption.Data were recorded on a Siemens D5000diffractometer operating in transmission geometry with capillary samples,Cu K R1radiation from a Ge monochromator,and a6°linear position sensitive detector.(10)(a)Rajic,N.;Logar,N.Z.;Kaucic,V.Zeolites1995,15,672-678.(b)Harrison,W.T.A.;Gier,T.E.;Stucky,G.D.;Broach,R.W.; Bedard,R.A.Chem.Mater.1996,8,145-151.(11)Neeraj,S.;Natarajan,S.;Rao,mun.1999,165.(12)Bruce,D.A.;Wilkinson,A.P.;White,M.G.;Bertrand,J.A.J. Chem.Soc.,mun.1995,2059-2060.(13)Bruce,D.A.;Wilkinson,A.P.;White,M.G.;Bertrand,J.A.J. Solid State Chem.1996,125,228.(14)Ayyappan,S.;Bu,X.;Cheetham,A.K.;Rao,C.N.R.Chem.Mater. 1998,10,3308.(15)Stalder,S.M.;Wilkinson,A.P.Chem.Mater.1997,9,2168-2173.(16)Gray,M.J.;Jasper,J.D.;Wilkinson,A.P.;Hanson,J.C.Chem. Mater.1997,9,976.(17)Feng,P.Y.;Bu,X.H.;Tolbert,S.H.;Stucky,G.D.J.Am.Chem. Soc.1997,119,2497-2504.(18)Gier,T.E.;Bu,X.;Feng,P.;Stucky,G.D.Nature1998,395,154.(19)Nenoff,T.M.;Thoma,S.G.;Provencio,P.;Maxwell,R.S.Chem. Mater.1998,10,3077-3080.(20)Vilenchik,L.Z.;Griffith,J.P.;St Clair,N.;Navia,M.A.;Margolin,A.L.J.Am.Chem.Soc.1998,120,4290-4294.(21)Batten,S.R.;Hoskins,B.F.;Robson,R.Angew.Chem.,Int.Ed. Engl.1997,36,636-637.(22)Abrahams,B.F.;Batten,S.R.;Granna,M.J.;Hamit,H.;Hoskins,B.F.;Robson,R.Angew.Chem.,Int.Ed.Engl.1999,38,1475-1477.(23)Biradha,K.;Seward,C.;Zaworotko,M.J.Angew.Chem.,Int.Ed. Engl.1999,38,492-495.(24)(a)Ranford,J.D.;Vittal,J.J.;Wu,D.Angew.Chem.,Int.Ed.Engl. 1998,37,1114.(b)Zaworotko,M.J.Angew.Chem.,Int.Ed.Engl.1998, 37,1211.(25)Yaghi,O.M.;Davis,C.E.;Li,G.M.;Li,H.L.J.Am.Chem.Soc. 1997,119,2861-2868.(26)Abrahams,B.F.;Jackson,P.A.;Robson,R.Angew.Chem.,Int.Ed.Engl.1999,37,2656.(27)(a)Wells,A.F.Three-Dimensional Nets and Polyhedra;Wiley-Interscience:New York,1977.(b)O’Keeffe,M.;Hyde,B.G.Crystal Structures.1.Patterns and Symmetry;American Mineralogical Society Monograph,1996.(28)Kiang,Y.-H.;Gardner,G.B.;Lee,S.;Xu,Z.;Lobkovsky,E.B.J. Am.Chem.Soc.1999,121,8204.(29)Otwinowski,Z.;Minor,W.Processing of X-ray Diffraction Data Collected in Oscillation Mode;Otwinowski,Z.,Minor,W.,Eds.;Academic Press:New York,1996;p276.(30)Sheldrick,G.M.SHELXL-93Program for the refinement of crystal structures;Universita¨t Gottingen,1993.(31)Sheldrick,G.M.SHELXS-86;Universita¨t Gottingen,1986.Intercon V ertible Microporous Chiral Molecular Frameworks J.Am.Chem.Soc.,Vol.122,No.21,20005159Resolvation was studied by allowing open capillaries to equilibrate with solvent vapor in closed containers.Resolvation,as seen by changes to the XRD pattern,is not seen to occur when samples are left to stand in air,ruling out the possibility that water is responsible for the resolvation behaviors observed.Vibrational Spectroscopy.Infrared data were collected on samples prepared as KBr disks in airtight cells over the range400to4000cm-1 using a Mattson Instruments Galaxy Series FTIR6020spectrometer. Raman data were collected with a Dilor Labram14/23IM spectrometer using a20mW HeNe laser(632nm)and the sample placed on a clean microscope slide in air.Gas Chromatography.Single crystals of phase B were removed from the vessel in which they were grown from racemic1,2-propanediol and dried on filter paper.Individual crystals(ca.1.5mg,0.7µmol) were dissolved in a minimum volume,ca.2mL(35mmol),of glacial acetic acid and the resulting solution diluted100times by ethyl acetate. For each crystal five2µL portions of this solution were injected into a SUPELCO R-DEX120GC column held at a steady80°C.Detection of eluted components was carried out by a Fisons GC8000series gas chromatogram.An80°C isotherm and a helium carrier pressure of 100kPa gave near baseline resolution of(R)-and(S)-1,2-propanediol. This experiment was repeated on three different crystals.Solution NMR.Solid samples(ca.5mg)were dried briefly on filter paper and dissolved in the minimum volume of dilute HNO3in D2O. Solution1H NMR spectra in D2O were recorded on a Varian Mercury 300MHz spectrometer.ResultsThe diffusion crystal growth outlined above produces three new but related structural families.The ethylene glycol(A)and methanol/ethanol/1-propanol(A1)structures contain four inter-penetrating(10,3)-a networks with cubic and tetragonal crystal symmetry,respectively,while the1,2-propanediol family have a much more open structure with only two interpenetrating, distorted networks.The structure of the tetragonal phase A1is massively disordered and will be described in detail in a separate publication.The yields are difficult to determine precisely due to wetting of the product by the solvent,but are typically of the order of75%based on the metal.Fourfold Interpenetration of the(10,3)-a Net in A(Eth-ylene Glycol).Ni3(btc)2(py)6(eg)6‚(eg)x‚(H2O)y(x≈3,y≈4) and an analogous Co II phase grow as octahedral blue and red crystals,respectively,both of which are insoluble in water and common organic solvents.The systematic absences(00l:l) 2n+1),m3h m Laue symmetry,and structural modelings defined the space group as P4232(No.208),making these the first molecular materials to crystallize with this symmetry.A brief description of this phase has been given in a previous com-munication.32Here we concentrate on demonstrating how the pores form around the templating unit,and how these pores are linked into channels despite the network interpenetration. The tridentate btc anions are connected linearly by the Ni II cations.The two btc ligands per metal occupy axial positions trans to each other in the octahedral coordination sphere of Ni II, with the equatorial positions being occupied in a disordered manner by50%ethylene glycol and50%pyridine.(Figure1a). The trans btc groups are orientated approximately orthogonal to each other due to a hydrogen bonding interaction between the noncoordinated oxygen atom of the btc carbonyl group and the equatorial alcohol group(O11‚‚‚O2)2.60Å),as shown in Figure1a:the alcohol ligands are locally cis,generating the perpendicular arrangement of the btc planes.This interaction thus generates the three-dimensional(10,3)-a rather than a planar (6,3)network structure.Framework Structure of A.Four equivalent(10,3)-a networks,each related by unit translation or2-fold rotation, interpenetrate in a manner foreseen by Wells27a but are seen here chemically,we believe,for the first time(Figure1b).The four btc anions per unit cell define a body-centered cubic array of pseudotetrahedral cavities,centered on the2a0,0,0and 1/2,1/2,1/2special positions,which are linked by channels running parallel and diagonal to the unit cell edges(Figure2a,b).The pores occupy28%of the crystal volume,as calculated by summing voxels more than1.2Åaway from the van der Waals surface of the framework.33Each has a volume of ca.600Å3 and a maximum diameter of12Å(calculated using VDW radii). The highly diffuse electron density in the pores was modeled as arising from a3-fold disordered,pseudotetrahedral cluster of four ethylene glycol molecules linked together by hydrogen bonding(denoted by a network of 2.80Åintermolecular O‚‚‚O distances),which is illustrated in Figure2c.Each eg is located over the centroid of a btc unit from each of the four (10,3)-a nets and thus this(eg)4supramolecular assembly templates the quadruply interpenetrating network structure. The(10,3)-a network is inherently chiral due to the〈100〉4-fold(e.g.,42helices parallel to the cubic cell vectors in Figure 1b)and〈111〉3-fold helices.The four sets of helices in A have(32)Kepert,C.J.;Rosseinsky,mun.1998,31-32.(33)Spek,A.L.Acta Crystallogr.,Sect.A1990,46,C34.Table1.Crystal Data and Refinement Summaries for All Structures Discussedsolvent:ethylene glycol32 phase:A(4×(10,3)-a)solvent:1,2-propanediolphase:B(2×(10,3)-a′)solvent:2,3-butanediolphase:B(2×(10,3)-a′)formula C66H98N6O34Ni3C66H98N6O34Ni3C81H124N6O34Ni3C84H126N6O30Ni3 FW/g mol-11695.631695.631901.991876.04T/K150(2)293(2)150(2)150(2)λ/Å0.71073 1.541800.710730.71073 description blue octahedra blue octahedra blue octahedra blue octahedra size/mm30.40×0.375×0.350.40×0.375×0.350.22×0.22×0.220.15×0.15×0.15 crystal system Cubic Cubic cubic cubicspace group P4232P4232I4132I4132a/Å15.922(1)16.025(1)28.471(1)28.457(1)V/Å34036.4(4)4115.2(4)23078.5(14)23044.5(14)Z2288F calc /Mg m-3 1.395 1.368 1.095 1.081µ/mm-10.781 1.5380.5530.5502θmax/deg53.46149.3852.8245.28data/restraints/parameters1445/37/1241347/37/1243947/10/1472562/44/114R(F)/%{I>2σ(I),all data}0.0965,0.12330.0697,0.09450.0817,0.12660.1719,0.1740R w(F2)/%{I>2σ(I),all data}0.2426,0.25990.1889,0.20460.2072,0.23660.4277,0.4291GOF 1.220 1.0560.951 1.384Flack parameter0.03(11)-0.04(15)-0.02(4)0.13(11)5160J.Am.Chem.Soc.,Vol.122,No.21,2000Kepert et al.the same handedness and the refined value of the Flack parameter (0.03(11))shows that each crystal of the chiral framework phase grows as one single enantiomer,with homo-chiral pores and channels.This spontaneous resolution originates in the inability of helices of opposite handedness to intergrow due to the inter-btc repeat.As no part of the initial reaction mixture is intrinsically chiral,bulk samples will be 50:50mixtures of left-and right-handed helical crystals.Twofold Interpenetration of a Distorted (10,3)-a Net in B (1,2-Propanediol and 2,3-Butanediol).The search for a suitable template to grow homochiral bulk samples of A led to the use of the methyl-substituted chiral glycol 1,2-propanediol (1,2-pd)as solvent.This apparently insignificant change leads to the synthesis of a yet more expanded chiral phase,B ,derived from a network that is a pronounced distortion of the aristotype (10,3)-a net.The distortion arises from the intercentroid vectors no longer being coplanar,with the angles between the inter-btc vectors changing from 120°in (10,3)-a to 114.27°in phase B .Phase B ,which is insoluble in water and common organic solvents,is shown for the 1,2-pd solvate to have the composition Ni 3(btc)2(py)6(1,2-pd)3‚11(1,2-pd)‚8(H 2O)by chemical analysis (calcd:C 47.52,H 7.28,N 3.69,Ni 7.74.Found for two distinct samples:C 47.49,H 6.31,N 3.67,Ni 7.76,and C 47.56,HabFigure 1.(a)Coordination at the nickel cation in A .The axial positions are occupied by two trans -1,3,5-benzenetricarboxylate (btc)ligands which each coordinate to three nickel cations.The orientations of these btc units are controlled by hydrogen bonding (dashed lines)to the two cis unidentate ethylene glycol (eg)molecules.The coordination is completed by two pyridine molecules.(b)Quadruple interpenetration of the (10,3)-a net (denoted 4×(10,3)-a)in A :3-fold sites (representing btc)are linked together by linear rods (representing nickel).One of the four equivalent nets has been highlighted forclarity.cFigure 2.(a)Structure of A viewed along the [100]direction.Cavity centers are marked with large spheres and are occupied by the units displayed in panel c.The channels between the cavities along this direction link next-nearest neighbor cavities separated by a .(b)Structure of A viewed along the [111]direction showing the pseudohexagonal channels defined by pyridine and ethylene glycol ligands.(c)The highly disordered,hydrogen bonded (eg)4cluster at the cavity center in A .Oxygen atoms in this cluster are shaded black.Atoms shaded gray show the two orientations of the btc units,whose disorder is coupled with that of the equatorial pyridine and ethylene glycol ligands.Each eg is located over the centroid of a btc from each of the four (10,3)-a networks,producing a templating effect.The closest C ‚‚‚C contacts of 3.73Åare between the eg and btc benzene ring carbon atoms.Intercon V ertible Microporous Chiral Molecular Frameworks J.Am.Chem.Soc.,Vol.122,No.21,200051617.43,N3.65,Ni7.72)This phase adopts the space group I4132 (No.214),determined unambiguously from the systematic absences and also unique for a molecular material.Both structures are highly disordered,with the equatorial ligands and nickel atoms in the framework disordered onto two sites.We will focus our discussions on the1,2-propanediol solvate,for which a greater degree of structural information was obtained, due to the increased framework disorder in the2,3-butanediol solvate.The low density of the new structure B arises because only two distorted(10,3)-a nets interpenetrate,related by the body-centring translation(Figure3a).This arrangement,where both networks are of the same handedness,is also new to chemistry and appears not to have been foreseen(whereas two oppositely handed regular(10,3)-a networks are known to interpenetrate highly efficiently).34The origin of the distortion away from the regular(10,3)-a net is in the fundamental modification of the binding of the vicinal diol to the metal,as the1,2-propanediol molecule is now bidentate and occupies two adjacent equatorial positions at the metal(Figure3b).The other two cis equatorial positions are occupied by symmetry-equivalent pyridine mol-ecules.The framework is again generated using octahedral Ni as a linear connector,with the trans diaxial positions occupied by two tridentate btc ligands.The refinement(absolute structure parameter for two separate crystals-0.02(4)and0.00(5))indicates that each crystal again grows from racemic1,2-propanediol as a single enantiomer,but in this case a novel templating effect is revealed due to the chirality of the equatorial diol.Refinement clearly shows that in the crystals examined only the S enantiomer of the diol((S)-1,2-pd)is bound to the metal,demonstrating that the anticlock-wise screw chirality of the43helices can be directly controlled by the stereochemistry of the equatorial ligands(see Figure S1 in Supporting Information for an ORTEP diagram).This observation has been confirmed in two separate structure refinements on different1,2-pd-templated crystals,and the same effect is evident in the structure of the2,3-butanediol analogue. The direct relationship between the hand of the diol bound to the metal and that of the resulting helical nets is thus unambigu-ously demonstrated.The diol bound to the metal now makes two hydrogen bonds to the btc ligands via each hydroxyl group(O2‚‚‚O11)2.62Å:Figure3b).This produces a distortion of the(10,3)-a network away from the ideal geometry(where the torsion angle between adjacent btc planes is the tetrahedral angle)found in the ethylene glycol salt,since the Ni II centers lie displaced out of the planes of the btc units.A similar cubic distortion of the(10,3)-a network is seen in nonporous transition metal oxalate frame-works.35The total pore volume calculated by summing voxels more than1.2Åaway from the van der Waals surface of the framework33is51%of the total crystal volume.Table2shows a range of diols and triols which give rise to this structure,and the unit cell parameters and volumes.Similarly to A,it is possible to locate solvent molecules in the extraframework space but their stereochemistry,unlike that of the diol bound directly to the metal,cannot be defined crystallographically.One1,2-pd molecule is heavily disordered about the8a1/8,1/8,1/8positions:the disorder is too extensiveto permit location of the methyl group.The other pore solvent molecule is located by hydrogen bonding to the carbonyl oxygen atom of the btc ligand(Figure3c).The data do not allow a full refinement of a model containing both enantiomers at this site: the model presented in the accompanying CIF file uses only the hand of the guest(S,i.e.,equivalent to that of the diol directly bound to the metal)which is favored by refinement, although a highly constrained competitive refinement indicates a68:32ratio of(S)-and(R)-1,2-pd at this position.(34)(a)Luzzati,V.;Spegt,P.A.Nature1967,215,701-704.(b)Hyde, S.Forma1998,13,145-178.(35)Decurtins,S.;Schmalle,H.W.;Schneuwly,P.;Ensling,J.;Gu¨tlich, P.J.Am.Chem.Soc.1994,116,9521-9528.abcFigure3.(a)Double interpenetration of the(10,3)-a′net in B(denoted 2×(10,3)-a′).These nets,one of which has been highlighted for clarity, are distorted substantially from the regular(10,3)-a network as seen in A.(b)The coordination environment at the metal center in B differs from that in A,with the diol,in this case1,2-propanediol,now bidentate. The hand of the diol bound to the metal controls that of the helices within the(10,3)-a′network.(c)Of the noncoordinating1,2-propanediol (1,2-pd)molecules occupying the chiral cavities of B,those located closest to the(10,3)-a′nets are located by hydrogen bonding to the carbonyl group of the btc anion.Refinement indicates that this position is preferentially occupied by the(S)-1,2-pd enantiomer.5162J.Am.Chem.Soc.,Vol.122,No.21,2000Kepert et al.。