Simulation of Fluid Flow, Heat Transfer and Micro-Segregation in Twin-roll Strip Casting of Stai
学习使用SolidWorksFlowSimulation进行流体分析
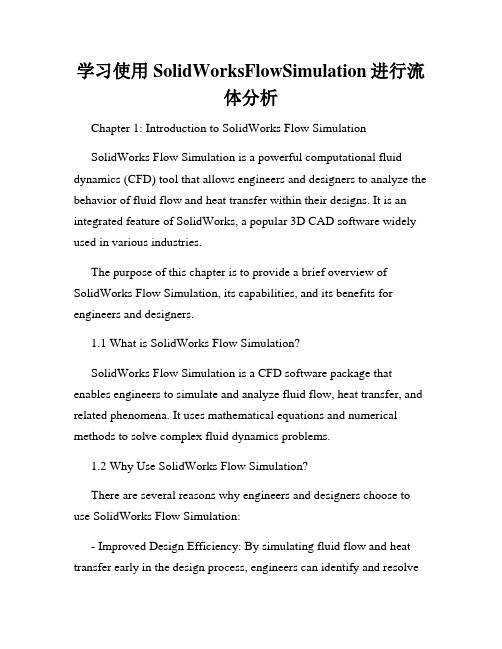
学习使用SolidWorksFlowSimulation进行流体分析Chapter 1: Introduction to SolidWorks Flow SimulationSolidWorks Flow Simulation is a powerful computational fluid dynamics (CFD) tool that allows engineers and designers to analyze the behavior of fluid flow and heat transfer within their designs. It is an integrated feature of SolidWorks, a popular 3D CAD software widely used in various industries.The purpose of this chapter is to provide a brief overview of SolidWorks Flow Simulation, its capabilities, and its benefits for engineers and designers.1.1 What is SolidWorks Flow Simulation?SolidWorks Flow Simulation is a CFD software package that enables engineers to simulate and analyze fluid flow, heat transfer, and related phenomena. It uses mathematical equations and numerical methods to solve complex fluid dynamics problems.1.2 Why Use SolidWorks Flow Simulation?There are several reasons why engineers and designers choose to use SolidWorks Flow Simulation:- Improved Design Efficiency: By simulating fluid flow and heat transfer early in the design process, engineers can identify and resolvepotential issues before physical prototypes are built. This saves time and reduces costs.- Accurate Results: SolidWorks Flow Simulation uses validated numerical algorithms to provide accurate results. It considers factors such as turbulence, flow rates, pressure drops, and thermal effects.- Visualization: SolidWorks Flow Simulation provides interactive 3D visualizations of fluid flow patterns, velocity vectors, temperature distributions, and other parameters. This helps engineers better understand the behavior of their designs.- Optimization: SolidWorks Flow Simulation offers optimization capabilities, allowing engineers to automatically find the best design parameters for their fluid systems. This can lead to improved performance and efficiency.1.3 Applications of SolidWorks Flow SimulationSolidWorks Flow Simulation has a wide range of applications in various industries, including:- Automotive: Analyzing airflow around vehicles, optimizing cooling systems, and improving aerodynamics.- Aerospace: Evaluating aircraft wing designs, studying fluid flow in jet engines, and optimizing heat transfer in spacecraft.- HVAC: Simulating air conditioning systems, optimizing ventilation design, and analyzing thermal comfort.- Electronics: Studying the cooling of electronic components, evaluating heat sink designs, and analyzing airflow in computer servers.Chapter 2: Getting Started with SolidWorks Flow SimulationChapter 2 provides a step-by-step guide on how to get started with SolidWorks Flow Simulation. It covers the basic workflow, setup, and analysis of a typical fluid flow problem.2.1 Creating a StudyThe first step in using SolidWorks Flow Simulation is to create a new study. This involves defining the fluid domain, selecting the appropriate fluid type, and specifying the boundary conditions.2.2 Meshing the GeometryAfter creating a study, the next step is to generate a mesh for the geometry. Meshing is the process of dividing the fluid domain into a network of small cells or elements. A finer mesh provides higher accuracy but requires more computational resources.2.3 Defining Boundary ConditionsBoundary conditions define the inputs and outputs of the fluid flow problem. This includes specifying the inlet velocity or pressure, outlet conditions, wall properties, and any additional constraints or assumptions.2.4 Solving the Flow ProblemOnce the geometry is meshed and the boundary conditions are set, SolidWorks Flow Simulation can solve the flow problem. This involves solving the Navier-Stokes equations, which describe the conservation of mass, momentum, and energy.2.5 Analyzing the ResultsAfter the flow problem is solved, engineers can analyze the results using various post-processing tools. This includes visualizing flow patterns, velocity profiles, pressure distributions, temperature gradients, and other relevant parameters.Chapter 3: Advanced Features and TechniquesChapter 3 explores some of the advanced features and techniques available in SolidWorks Flow Simulation. These features allow engineers to handle more complex fluid flow problems and obtain more detailed insights.3.1 Turbulence ModelingTurbulence modeling is crucial in accurately simulating turbulent flow, which is common in many real-world scenarios. SolidWorks Flow Simulation provides various turbulence models, such as the k-epsilon model and Reynolds stress model, to capture turbulence effects.3.2 Multiphase FlowMultiphase flow refers to the simultaneous flow of multiple fluid phases, such as gas-liquid, liquid-solid, or gas-liquid-solid. SolidWorksFlow Simulation offers tools to model and analyze multiphase flow phenomena, such as cavitation, droplet dynamics, and particle transport.3.3 Heat Transfer AnalysisSolidWorks Flow Simulation includes comprehensive heat transfer analysis capabilities. Engineers can analyze conduction, convection, and radiation heat transfer within their designs. This is particularly useful in evaluating thermal management solutions and optimizing heat dissipation.3.4 Fluid-Structure Interaction (FSI)Fluid-structure interaction occurs when the fluid flow affects the structural behavior of a design, and vice versa. SolidWorks Flow Simulation allows engineers to perform FSI analysis, enabling them to study the interaction between fluid forces and structural responses.Chapter 4: Case Studies and Real-Life ExamplesChapter 4 presents several case studies and real-life examples that demonstrate the practical applications of SolidWorks Flow Simulation. These examples cover a diverse range of industries and showcase the capabilities and benefits of the software.4.1 Automotive Cooling System OptimizationThis case study focuses on optimizing the cooling system of a car engine. By simulating the airflow around the radiator, fan, and othercomponents, engineers can identify design improvements that enhance cooling efficiency and reduce engine overheating.4.2 HVAC System Design OptimizationIn this example, SolidWorks Flow Simulation is used to optimize the design of an HVAC system in a commercial building. By analyzing air distribution, temperature gradients, and ventilation effectiveness, engineers can improve occupant comfort and energy efficiency.4.3 Electronics Cooling AnalysisThis case study demonstrates the thermal analysis of an electronic circuit board. SolidWorks Flow Simulation helps engineers evaluate heat sink designs, optimize airflow through the components, and ensure proper cooling of sensitive electronic devices.Chapter 5: ConclusionIn conclusion, SolidWorks Flow Simulation is a valuable tool for engineers and designers involved in fluid flow and heat transfer analysis. It provides a comprehensive set of features and capabilities that enable users to simulate, analyze, and optimize their designs.By integrating SolidWorks Flow Simulation into the design process, engineers can gain valuable insights, improve design efficiency, and make informed decisions that lead to better performing and more reliable products.Whether in automotive, aerospace, HVAC, or electronics industries, SolidWorks Flow Simulation offers a powerful solution for fluid analysis, contributing to safer, more efficient, and more innovative designs.。
Polyflow在双螺杆挤出模拟中的应用

Polyflow在双螺杆挤出模拟中的应用彭炯,胡冬冬,陈晋南(北京理工大学化工与材料学院,北京100081,中国)摘要:建立了同向双螺杆挤出机捏合段三维非等温流动的数学模型,利用Polyflow软件包对其进行了数值求解,给出了流道内压力、速度的分布,以及进出口压差对流量、温度及压力的影响规律。
数值结果对捏合盘的优化设计和工艺参数的选择有一定的指导意义。
关键词:双螺杆挤出机,捏合元件,数值模拟,Polyflow中图分类号:TQ320.66Polyflow in the Simulation of Twin-Screw ExtrusionPeng Jiong, Hu Dong-dong and Chen Jin-nan(School of Chemical Engineering and Materials Science, Beijing Institute of Technology, Beijing100081)Abstract: A 3-D numerical model of the non-isothermal flow in the kneading zone of a co-rotating twin-screw extruder was presented. The numerical results were obtained by a software package Polyflow. The distributions of the pressure and velocity, and the influences of the pressure difference between the inlet and outlet on the flow rates, temperature and pressure were also presented. Numerical results are of great significance for guiding of the optimization of kneading disc design and the selection of processing parameters.Keywords: Twin-screw extruders, kneading element, numerical simulation, Polyflow双螺杆挤出机作为连续混合设备,已广泛应用于聚合物加工、食品加工以及医药工业。
电渣重熔过程渣池流场数值模拟

电渣重熔过程渣池流场数值模拟王晓花;厉英【摘要】采用商业软件ANSYS和FLUENT建立了电渣重熔过程渣池流场数学模型,分析了电渣重熔过程电磁力和热浮力共同作用下渣池流动行为,以及典型电渣重熔工艺参数(电极形貌、插入深度、填充比和电流强度)对电渣重熔过程渣池内流场的影响规律.结果表明:电磁力有利于渣池内产生逆时针涡流,浮力有利于渣池产生顺时针涡流.电极端部形貌对渣池流动影响较大,当电渣重熔电流均为5000A,频率为50 Hz时,平头电极所在渣池内同时存在逆时针涡流和顺时针涡流,锥形电极所在渣池内只存在逆时针涡流.电极填充比和电流都对渣池内流动行为影响较大,减小电极填充比和增大电流强度都会使渣池内逆时针涡流增加.【期刊名称】《材料与冶金学报》【年(卷),期】2014(013)002【总页数】6页(P133-137,151)【关键词】电渣重熔;渣池;流场;数值模拟【作者】王晓花;厉英【作者单位】东北大学材料与冶金学院,沈阳110819;东北大学材料与冶金学院,沈阳110819【正文语种】中文【中图分类】TG142.4电渣重熔获得的最终产品具有成分均匀、杂质含量低、凝固组织致密等优点.因此,电渣重熔被广泛应用于高附加值特殊钢和镍基超级合金生产[1~4].渣池在电渣重熔电流作用下产生大量焦耳热熔化自耗电极,并在金属熔滴形成和下落过程中去除金属熔滴中的夹杂物和有害元素,从而达到净化金属溶液的目的.电渣重熔过程电磁力和热浮力作用使得渣池内熔渣产生复杂的湍流流动,影响金属熔滴流动和温度以及金属熔滴净化效果,从而影响最终重熔钢锭成分和组织均匀性.因此,电渣重熔过程渣池流动行为研究对于制定合理的电渣重熔工艺,生产高质量钢锭至关重要.鉴于电渣重熔过程渣池内发生复杂的物理化学变化,且渣池为非透明材料,很难由物理方法直接观察渣池内部情况,而数值模拟对于高温冶金过程传输现象研究是一种行之有效的方法,因此越来越受到冶金工作者的重视.以Choudhary和Sezekely[1~3]为代表的研究者首先开始采用耦合Maxwell方程、湍流Navier-Stokes方程和能量守恒方程的方法,研究了电渣重熔过程渣池内熔渣流动行为.随后以Ferng、Jardy和Weber为代表的研究者[4~6]进一步分析了供电模式、填充比和熔渣物性参数对电渣重熔过程渣池流动行为的影响,但大多忽略了渣池温度分布不均产生的热浮力对渣池流动行为的影响.魏季和任永莉[7,8]研究了电渣重熔过程电磁场对渣池流动行为的影响,但忽略了电渣重熔过程渣池内部温度分布不均产生的热浮力对渣池流动行为的影响.尧军平、刘福斌、董艳伍等[9~12]为代表的研究者采用ANSYS分析了电渣重熔过程操作参数对渣池流动行为的影响.然而要充分了解电渣重熔过程传输现象,还需要进一步完善其他因素的影响,特别是电磁力和热浮力共同作用下渣池的流动行为[13,14].本研究在前期建立的电渣重熔电磁场数学模型[15]基础上,耦合电渣重熔过程流场和温度场数学模型,分析了电磁力和热浮力共同作用下电渣重熔渣池内熔渣流动行为,以及电渣重熔操作参数(电极形貌、电流强度和填充比)对渣池流场的影响规律.电渣重熔过程中电磁场、温度场和流场相互作用,对渣池内熔渣流动行为影响较为复杂.为了简化计算,本研究作如下假设:(1)电渣重熔达到稳定后,在较短时间内处于准稳态过程;(2)电极端部与渣池接触处温度为重熔金属的液相线温度;(3)结晶器绝缘,没有电流通过结晶器;(4)渣的密度随温度变化.连续性方程:动量方程:式中电磁力为:根据Boussinesq假设,与温度相关的密度变化仅出现在动量方程的浮力项.因此,电渣重熔过程渣池内熔渣温度分布不均所引起的热浮力对渣池运动的影响,可将浮力项直接包含在动量方程.能量方程:采用k-ε双方程模型[16]描述渣池内湍流流动:湍动能方程:湍动能耗散率方程:式中湍动能产生项:以上各式中:u为速度矢量,m/s;p为压强,Pa;ρ为熔体密度,kg/m3;cp为等压比热容,J/(kg·℃);λeff为有效导热系数,W/(m·℃);QJ为体积焦耳热,W/m3;t为时间,s;μ为层流黏度系数,P a·s;μt为湍流黏度系数,Pa·s;k为湍动能,m2/s2;μ0为真空磁导率,1.26×10-6H/m; J为感应电流密度,A/m2;H为磁场强度,A/m; T和T0分别为熔渣温度和参考温度,℃;k-ε双方程模型中通用系数均采用Launder和Spalding推荐数值[16].温度场边界条件:渣/电极界面为常温度边界条件,为了简化取自耗电极液相线温度;渣/空气界面为辐射边界条件;渣/结晶器和渣金界面为对流换热边界条件.流场边界条件:渣/电极界面和渣/结晶器界面采用无滑移边界条件;渣金界面和渣自由表面采用零剪切力边界条件.本研究针对单电极电渣重熔系统,结晶器直径为 0.36 m,电极直径为 0.10 m,渣池厚度0.20 m,渣黏度0.03 Pa·s[12].图1为电渣重熔模型计算区域示意图.采用大型有限元商业软件ANSYS对电渣重熔过程电磁场进行分析,具体的计算方法可详见笔者前期工作[15],在此不再赘述.将电磁场分析得到的体积电磁力和体积焦耳热存储成一定格式的数据文件,并采用FLUENT的UDF二次开发接口函数读入到Fluent所建立的电渣重熔渣池模型,从而耦合求解电磁力和热浮力共同作用下电渣重熔过程渣池流动行为.图2为电渣重熔电流5 000 A,频率50 Hz时,电极端部形貌对渣池流场的影响. 从图2中可以看出电极端部为平面时,渣池内部同时存在一个逆时针涡流和顺时针涡流,液渣流动速度介于0~0.05 m/s之间且最大速度位于渣池中心轴线处附近.电极与结晶器壁之间的逆时针涡流是电磁力和浮力共同作用的结果,这是因为电磁力驱动的逆时针流动强于位于结晶器壁和电极冷面之间的热流体浮力驱动的顺时针流动.由于渣池内靠近结晶器壁径向上存在较大的温度梯度,使得浮力占据主导地位,因而渣池内靠近结晶器壁存在顺时针方向的涡流.电极端头为平面时,渣池内流动特征与Choudhary和Szekely的研究结果[1]基本一致,同时说明了模型准确可靠.当电极端头为锥形时,渣池内仅存在逆时针涡流,这是由于电磁力较浮力强,使得渣池内产生逆时针流动,且流速介于0~0.20 m/s之间,最大流速同样位于中心渣池轴线处附近.图3为不同电极形貌对渣池内温度场的影响.从图中可以看出当电极端部为平面时,渣池内最高温度区域位于电极下方,电极/渣池界面和渣池/结晶器界面处温度梯度最大.渣池内电磁力和热浮力共同驱动下产生的逆时针涡流和顺时针涡流使得渣池内流动剧烈并使渣池内高温区扩大,温度趋于均匀.当电极端部为锥形时,由于电渣重熔过程产生的电磁力驱动熔渣成逆时针流动,促使熔渣温度逐渐均匀,并在涡流中心出现温度最高值.图4为电渣重熔电流5 000 A,频率50 Hz时,平头电极插入渣池深度对渣池流动行为的影响.从图4中可以看出随着电极插入深度的减少,渣池内部电极与结晶器壁之间,由于电磁力占主导作用所产生的逆时针回旋区逐渐缩小,而由于热浮力占主导作用而在渣池内靠近结晶器壁处所产生的顺时针回旋区逐渐扩大.当平头电极插入深度减少到0.02 m时,渣池内部电极与结晶器壁间的逆时针回旋区无限趋近消失,但在渣池内部电极下方出现逆时针回旋区.图5为电渣重熔电流5 000 A,频率50 Hz时,填充比(电极半径/结晶器半径)对渣池流场行为的影响.从图5中可以看出随着填充比的增加,由电磁力所引起的位于电极与结晶器壁之间的逆时针涡流逐渐减弱.相反,顺时针涡流逐渐增强.这是由于随着填充比的增加,渣池内部电流分布发生变化,体积电磁力减小,最大焦耳热也相应减小,但位置更加靠近结晶器壁,从而使得渣池内部电磁力的主导地位逐渐降低,靠近结晶器壁处热浮力作用逐渐增强.图6为填充比0.56和插入深度0.04 m时,不同电流强度对电渣重熔渣池内流场的影响.从图6中可以看出随着电流强度的降低,渣池内部的体积电磁力减小,从而使渣池内由电磁力占主导作用引起的位于电极与结晶器壁之间的逆时针涡流逐渐减小.相反,由于电磁力逐渐减小,渣池内热浮力逐渐占主导地位,从而使渣池内部由热浮力占主导地位产生的靠近结晶器壁的顺时针涡流逐渐增强.通过计算定量分析可知随着电渣重熔电流强度从6 000 A降低到4 000 A,渣池内部最大流速从0.06 m/s降低到0.04 m/s.本文建立了耦合电渣重熔过程渣池内电磁场、温度场和流场的数学模型,分析了电磁力和热浮力作用下,不同电渣重熔工艺参数(电极形貌、插入深度、填充比和电流强度)对渣池流场的影响规律.(1)电渣重熔过程渣池内熔渣流动行为受电磁力和热浮力共同作用.电磁力有利于渣池内产生逆时针涡流,热浮力有利于渣池产生顺时针涡流.(2)电渣重熔过程渣池流场受电极形貌影响较大.电渣重熔电流5 000 A,频率50 Hz时,电极端部为平面,渣池内部同时存在逆时针涡流和顺时针涡流;电极端部为锥形,渣池内部只存在逆时针涡流.(3)电极插入深度、填充比和电流强度都会影响电渣重熔过程渣池内熔渣流动行为.增加电极插入深度、减小填充比和增大电流强度都会使渣池内部逆时针涡流增强.【相关文献】[1]Dilawari A H,Szekely J.Heat transfer and fluid flow phenomena in electroslag refining[J]. Metallurgical Transaction B,1978,9B(1):77-87.[2]Choudhary M,Szekely J.The modeling of pool profiles,temperature profiles and velocity fields in ESR systems[J].Metallurgical Transaction B,1980,11B(3):439-452. [3]Choudhary M,Szekely J,Medovar B I,et al.The velocity field in the molten slag region of ESR systems:a comparison of measurements in a model system with theoretical predictions[J].Metallurgical Transaction B,1982,13B(1):35-43.[4]Ferng Y M,Chieng C C,Pan C.Numerical simulation of electro-slag remelting process[J].Numerical Heat Transfer A,1989,16(4):429-449.[5]Jardy A,Ablitzer D,Wadier J F.Magnetohydrodynamic and thermalbehavior of electroslag remelting slags[J].Metallurgical and Materials Transactions B,1991,22B(1): 111-120.[6]Weber V,Jardy A,Dussoubs B,et al.A comprehensive model of the electroslag remelting process:description and validation[J].Metallurgical and Materials Transactions B,2009,40B(3):271-280.[7]魏季和,任永莉.电渣重熔体系内磁场的数学模拟[J].金属学报,1995,31(2):51-60. (Wei Jihe,Ren Yongli.Mathematical simulation of magnetic field in ESR system[J].Acta Metallurgica Sinica,1995,31 (2):51-60.)[8]魏季和,任永莉.电渣重熔体系内熔渣流场的数学模拟[J].金属学报,1994,30(11):481-490.(Wei Jihe,Ren Yongli.Mathematical modelling of slag flow field in ESR system[J].Acta Metallurgica Sinica,1994,30 (11):481-490.)[9]尧军平,徐俊杰.电渣熔铸过程渣池流场的模拟研究[J].铸造,2007,56(7):712-715. (Yao Junping,Xu Junjie.Simulation analysis of the slag pool flow field in the electroslag casting process[J].Foundry,2007,56(7):712-715.)[10]刘福斌,姜周华,藏喜民,等.电渣重熔过程渣池流场的数学模拟[J].东北大学学报(自然科学版),2009,30(7): 1013-1017.(Liu Fubin,Jiang Zhouhua,Zang Ximin,et al.Mathematical modelling of flow field in slag bath during electroslag remelting[J].Journal of northeastern university(natural science),2009,30(7):1013-1017.)[11]Dong Y W,Jiang Z H,Li Z B.Mathematical model for electroslag remelting process[J].Journal of Iron and Steel Reasearch,2007,14(5):7-12.[12]Dong Y W,Jiang Z H,Liu H,et al.Simulation of multielectrode ESR process for manufacturing large ingot[J].ISIJ International,2012,52(12):2226-2234.[13]Hernandez-Morales B,Mitchell A.Review of mathematical models of fluid flow,heat transfer,and mass transfer in electroslag remelting process[J].Ironmaking and Steelmaking,1999,26(6):423-438.[14]Mitchell A.Solidification in remelting process[J].Materials Science and Engineering A,2005,413-414:10-18.[15]王晓花,厉英.电渣重熔过程电磁场和温度场数值模拟[J].东北大学学报(自然科学版),2014,35(6).(Wang Xiaohua, LiYing. Numericalsimulation of electromagnetic field and temperature field of ESR[J].Journal of northeastern university(natural science),2014,35(6).) [16]Launder B E,Spalding D B.The numerical computation of turbulent flows [J].Computer Methods in Applied Mechanics and Engineering,1974,3(2):269-289.。
关于针对承担项目情况的解释

HR Planning System Integration and Upgrading Research ofA Suzhou Institution承担项目情况:1)国家自然科学基金“镁合金板材大变形成形机制与过程模拟研究”,编号:50405014,经费:23万元,项目起止年月:2005.1~2007.12,负责人。
2)国家自然基金项目,“辊弯成形全流程动态模拟技术研究”,编号:50375095, 经费:24万元,起止年月:2004.1~2006.12,主要参加人。
3)国家自然基金重点项目,“材料智能化近终成形加工技术的若干基础问题”,编号:50634010, 经费:180万元,起止年月:2007.01~2010.12,主要参加人。
4)国家973计划前期研究项目“材料制备新方法探索及性能研究”,编号:2006CB708600,总经费:1094万元,起止年月:2006.12~2008.11,主要参加人。
5)国家863计划重点项目,“高强高韧镁合金及其应用技术研究”,编号:002AA331120,经费:340万元,起止年月:2002.6~2005.6,主要参加人。
6)国防科工委民口配套项目,“XXX轴承的研究”,编号:MKPT-05-268,经费:165万元,起止年月:2005.1~2006.12,主要参加人。
7)上海市创新科技支撑计划项目子课题,“薄带连铸带钢力学性能及表面裂纹形成机理研究”,编号:07DZ1103,经费:80万元,起止年月:2008.2-2009.12,负责人。
8)教育部新世纪优秀人才计划项目,“镁合金板材变形机理与成形性能的宏微观研究”,编号:NCET-07-0545,经费:50万元,起止年月:2008.1-2010.12,负责人。
9)上海市重点基础研究项目,“ERW焊管排辊成形理论与工艺设计方法研究”,编号:09JC1407000,经费:30万元,起止年月:2009.9~2011.8,负责人。
Computational Fluid Dynamics and Heat Transfer
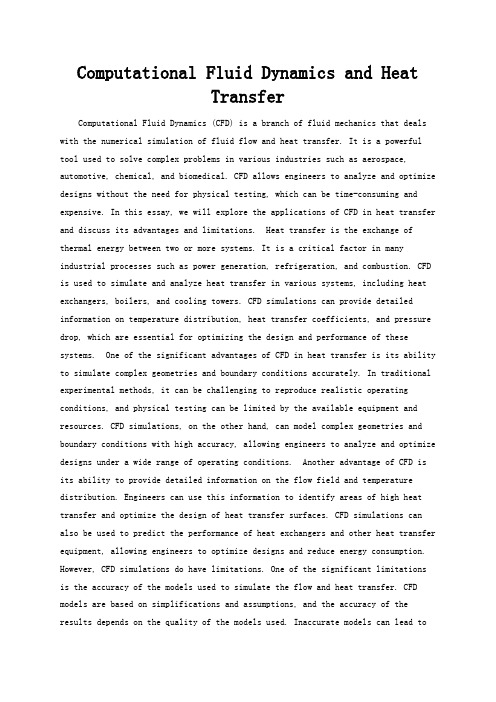
Computational Fluid Dynamics and HeatTransferComputational Fluid Dynamics (CFD) is a branch of fluid mechanics that deals with the numerical simulation of fluid flow and heat transfer. It is a powerful tool used to solve complex problems in various industries such as aerospace, automotive, chemical, and biomedical. CFD allows engineers to analyze and optimize designs without the need for physical testing, which can be time-consuming and expensive. In this essay, we will explore the applications of CFD in heat transfer and discuss its advantages and limitations. Heat transfer is the exchange of thermal energy between two or more systems. It is a critical factor in many industrial processes such as power generation, refrigeration, and combustion. CFD is used to simulate and analyze heat transfer in various systems, including heat exchangers, boilers, and cooling towers. CFD simulations can provide detailed information on temperature distribution, heat transfer coefficients, and pressure drop, which are essential for optimizing the design and performance of these systems. One of the significant advantages of CFD in heat transfer is its ability to simulate complex geometries and boundary conditions accurately. In traditional experimental methods, it can be challenging to reproduce realistic operating conditions, and physical testing can be limited by the available equipment and resources. CFD simulations, on the other hand, can model complex geometries and boundary conditions with high accuracy, allowing engineers to analyze and optimize designs under a wide range of operating conditions. Another advantage of CFD is its ability to provide detailed information on the flow field and temperature distribution. Engineers can use this information to identify areas of high heat transfer and optimize the design of heat transfer surfaces. CFD simulations can also be used to predict the performance of heat exchangers and other heat transfer equipment, allowing engineers to optimize designs and reduce energy consumption. However, CFD simulations do have limitations. One of the significant limitations is the accuracy of the models used to simulate the flow and heat transfer. CFD models are based on simplifications and assumptions, and the accuracy of the results depends on the quality of the models used. Inaccurate models can lead toincorrect predictions and suboptimal designs, which can be costly in terms of time and resources. Another limitation of CFD is the computational cost. CFD simulations can be computationally intensive, especially for large and complex geometries. The time required to run a simulation can be significant, and it can be challenging to optimize designs under tight deadlines. In addition, the accuracy of the results depends on the resolution of the mesh used to model the geometry. A finer mesh can provide more accurate results but can also increase the computational cost. In conclusion, CFD is a powerful tool for simulating and analyzing heat transfer in various industrial applications. It allows engineers to optimize designs and improve the performance of heat transfer equipment. However, it is essential to consider the limitations of CFD, including the accuracy of the models used and the computational cost. Overall, CFD is a valuable tool for engineers, but it should be used in conjunction with physical testing and other methods to ensure accurate and reliable results.。
cfd气流分布数值
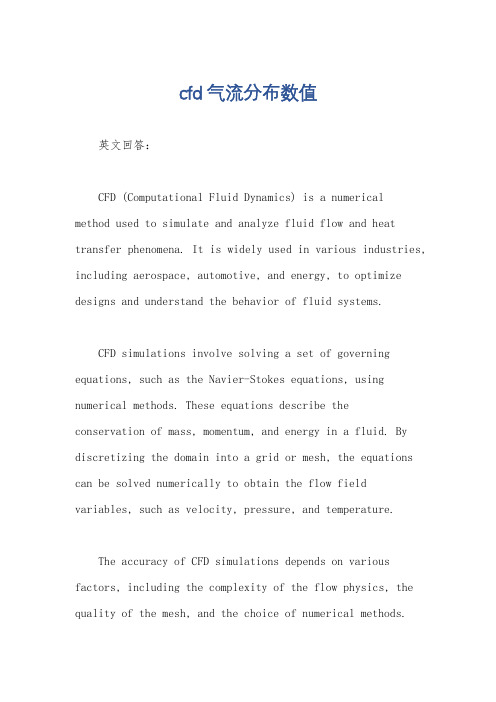
cfd气流分布数值英文回答:CFD (Computational Fluid Dynamics) is a numerical method used to simulate and analyze fluid flow and heat transfer phenomena. It is widely used in various industries, including aerospace, automotive, and energy, to optimize designs and understand the behavior of fluid systems.CFD simulations involve solving a set of governing equations, such as the Navier-Stokes equations, using numerical methods. These equations describe theconservation of mass, momentum, and energy in a fluid. By discretizing the domain into a grid or mesh, the equations can be solved numerically to obtain the flow field variables, such as velocity, pressure, and temperature.The accuracy of CFD simulations depends on various factors, including the complexity of the flow physics, the quality of the mesh, and the choice of numerical methods.In general, CFD can provide valuable insights into the flow behavior, pressure distribution, and temperaturedistribution in a system.CFD simulations can be used to study various flow phenomena, such as laminar and turbulent flows, boundary layer separation, flow around obstacles, and heat transfer. The results of CFD simulations can be visualized using contour plots, vector plots, and streamline plots, which help in understanding the flow patterns and identifying areas of high velocity or high pressure gradients.In addition to steady-state simulations, CFD can also be used to perform transient simulations, which capture the time-dependent behavior of the flow. This is particularly useful in studying unsteady flows, such as flow instabilities, flow oscillations, and flow transients.CFD simulations can be validated against experimental data to ensure their accuracy. This involves comparing the numerical results with measurements obtained from physical experiments. Validation is an important step in the CFDprocess to gain confidence in the simulation results and to ensure that the simulations accurately represent the real-world flow behavior.中文回答:CFD(计算流体力学)是一种用于模拟和分析流体流动和传热现象的数值方法。
中国石油大学(华东)学术学位硕士研究生培养方案
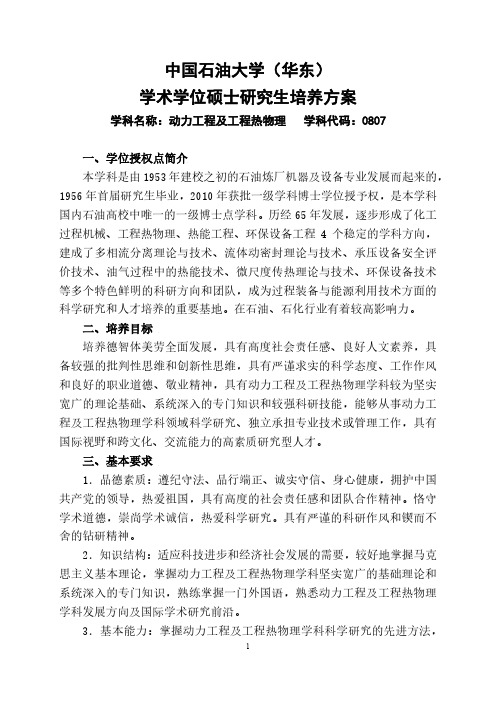
中国石油大学(华东)学术学位硕士研究生培养方案学科名称:动力工程及工程热物理学科代码:0807一、学位授权点简介本学科是由1953年建校之初的石油炼厂机器及设备专业发展而起来的,1956年首届研究生毕业,2010年获批一级学科博士学位授予权,是本学科国内石油高校中唯一的一级博士点学科。
历经65年发展,逐步形成了化工过程机械、工程热物理、热能工程、环保设备工程4个稳定的学科方向,建成了多相流分离理论与技术、流体动密封理论与技术、承压设备安全评价技术、油气过程中的热能技术、微尺度传热理论与技术、环保设备技术等多个特色鲜明的科研方向和团队,成为过程装备与能源利用技术方面的科学研究和人才培养的重要基地。
在石油、石化行业有着较高影响力。
二、培养目标培养德智体美劳全面发展,具有高度社会责任感、良好人文素养,具备较强的批判性思维和创新性思维,具有严谨求实的科学态度、工作作风和良好的职业道德、敬业精神,具有动力工程及工程热物理学科较为坚实宽广的理论基础、系统深入的专门知识和较强科研技能,能够从事动力工程及工程热物理学科领域科学研究、独立承担专业技术或管理工作,具有国际视野和跨文化、交流能力的高素质研究型人才。
三、基本要求1.品德素质:遵纪守法、品行端正、诚实守信、身心健康,拥护中国共产党的领导,热爱祖国,具有高度的社会责任感和团队合作精神。
恪守学术道德,崇尚学术诚信,热爱科学研究。
具有严谨的科研作风和锲而不舍的钻研精神。
2.知识结构:适应科技进步和经济社会发展的需要,较好地掌握马克思主义基本理论,掌握动力工程及工程热物理学科坚实宽广的基础理论和系统深入的专门知识,熟练掌握一门外国语,熟悉动力工程及工程热物理学科发展方向及国际学术研究前沿。
3.基本能力:掌握动力工程及工程热物理学科科学研究的先进方法,具备批判性思维和创新性思维,具备独立开展创造性学术研究和良好的沟通协调能力,能够运用动力工程及工程热物理学科基本理论和知识发现、分析和解决学科发展和行业技术进步过程中所遇到的科学问题和工程问题。
Mathematical Model for Fluid Flow and Heat Transfer in the Cooling Shaft of

Cpf Cp~ dp dh F ks K g h~f m n nx ny p qw q~ r r R0 RI S t t, ty Tf T~ uf Us ut vf vs v~ V Vf
general transport equation fluid specific heat, (J/(kg K)) solid specific heat, (J/(kg K)) particle diameter, (m) hydraulic diameter of the passage of gas flow, (m) inertial coefficient, dimensionless the coefficient of solid flow potential permeability, (mz) gravitational acceleration, -9.81, (m2/s) fluid-solid heat transfer coefficient, (W/(m 2 K)) mass flux through a control volume face unit outward normal vector the x -direction component of n the y -direction component of n fluid pressure, (Pa) heat flux at the wall, (W/m 2) source term the position vector radial coordinate, (m) the diameter of prechamber, (m) the diameter of the cooling chamber, (m) the surface area of cell face, (m2) unit vector tangential to the boundary. the x -direction component of t the y -direction component of t fluid temperature, (K) solid temperature, (K) Darcy velocity in x direction, (m/s) the coke descending velocity in x direction, (m/s) velocity vector component in t -direction Darcy velocity in r direction, (m/s) the coke descending velocity in r direction, (m/s) velocity vector component in n -direction, (m/s) velocity vector, (m/s) gas velocity vector, (m/s)