Hydrogen sensing using titania nanotubes
以硅氧烷溶胶为介质制备纳米TiO2光致超亲水涂层
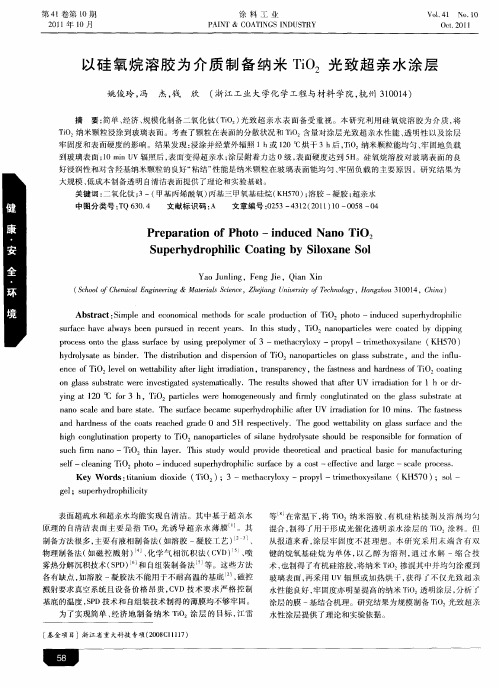
( col)C e i l n ier g& Maeas c ne Z eag l i rt o eho g , aghu30 1 ,C ia Sho f hmc gne n 厂 aE i t ilSi c , h i nv sy fTcnl y H n zo 10 4 hn ) r e j n / ei o
n n c l n ae sae.Th u a eb c me s p r y r p ii t rUV ra ito r1 n .Th a t e s a o s a e a d b r t t e s r c e a u eh d o hlc a e f f ir d ai n f 0 mi s o ef sn s a d had e so h o t e c e r d n n r n s ft e c a sr a h d g a e 0 a d 5H e p ciey.Th o d wetb lt n g a s s ra e a d t e r s e tv l e g o ta ii o ls u c n h y f h g o g ui ai n p o e y t O2n n p ri ls o ia e h d o y ae s o l e r s o sb e fr fr a in o ih c n ltn t rp r o Ti a o a t e fsln y r ls t h u d b e p n il m t f o t c o o o
GR5,TC4钛合金十大性能
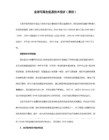
This property of titanium shows that its yield ratio (tensile strength / yield strength) is soaring, indicating that the metallic titanium material has poor plastic deformation upon forming. Due to the titanium yield strength and elastic modulus of the ratio of fat, the resilience of titanium molding large.
Titanium is a chemically very reactive metal that reacts with many elements and compounds at elevated temperatures. Titanium suction mainly refers to elevated temperature and carbon, hydrogen, nitrogen, oxygen reaction.
6, non-magnetic, non-toxic
Titanium is non-magnetic metal that cannot be magnetized in a large magnetic field. It is non-toxic and has excellent compatibility with human tissues and blood. Therefore, titanium is employed in the medical field.
二氧化钛纳米管的氢敏特性(英文)
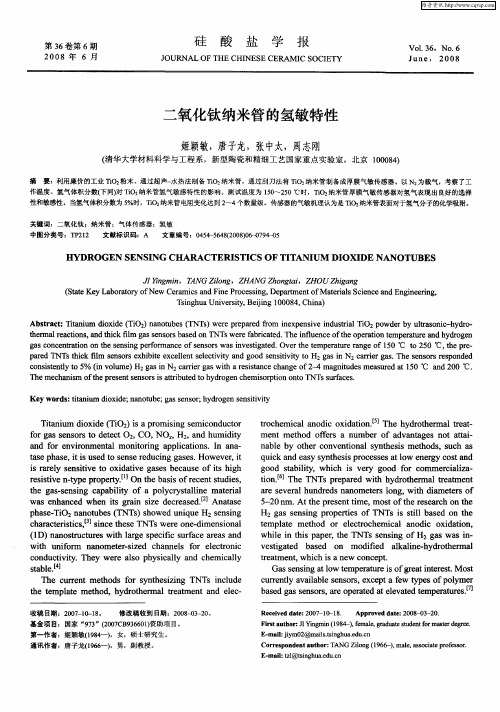
cnie t % ( ou ) 2 a a i a t s tn e h g f _ g i ds aue 5 ℃ a d2 0℃. o s t l t 5 i v lme H snN2 re gs ha eia c a e 4ma nt e srda 10 sn yo n g i crr wi r s cn o 2 u me t n 0
姬 颖敏, 唐子龙, 张中太, 周志刚
( 华大学材料科学与工程系 ,新型陶瓷和精细工艺国家重点实验室,北京 10 8 ) 清 0 0 4
摘 要 :利用 廉价 的工业 TO 粉末 、通过 超声一 热法 制备 TO 纳 米管 ,通过 刮刀 法将 TO 纳 米管制 备 成厚膜 气 敏传感 器 。以 N2 i2 水 i2 i2 为载 气 ,考察 了工
维普资讯
第 3 6卷第 6期 2 0 年 6 月 0 8
硅
酸
盐
学
报
Vo . 6,No 6 13 .
J une, 2008
J URNA L O 0F THE CHI ES CERAM I S CI N E C o ETY
二氧化钛纳米管 的氢敏特性
f rg ss n o st ee tO 2 o a e s r o d tc ,CO , N , ,a d h m i i H2 n u dt y a d f r e vr n e tlm o io ig a p i ain .I n . n o n io m n a n trn p lc t s n a a o ts h s ,ti s dt e s e ucn a e . w e e ,t a ep a e i su e o s n er d i gg s s Ho v r i i a ey s n i v o o d tv a e e a e o shih sr r l e st e t xiai e g s sb c us fi g i t r ssi tpepr p ry Ont eb sso c n t de . e it n— ve y o e t. “ h a i fr e tsu is e
基于纳米金标记的适体传感器的研制
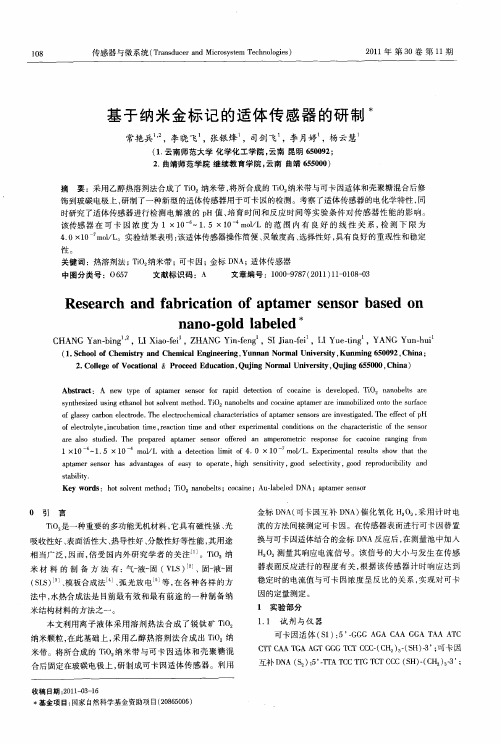
基金项 目: 国家 自然科学基 金资助项 目(0 6 0 6 2850)
第l l期
常艳兵 , : 等 基于纳米金标记 的适体传感器 的研制
19 0
壳聚糖( H T 购 于美 国 Sg 公 司 ; C I) i ma 四氯化钛 ( i1) 氯 TC 和
(/) W V 的壳聚糖醋酸溶液。将制备好的 TO 纳米带按质量 i
a e as s d e .T e r p rd p a r e s r o fr d n mp r merc e p n e o a on rn i g r m r lo t id h p e ae a t me s n o f e a a e o t r s o s f r c c ie a gn f u e i o l×1 . ×1 mo/ t a d tc in l t o .0 × 1 mo/ .E p rme tl r s l h w t a h 0一 一1 5 0一 l L wi h ee t i f4 o mi 0一 l L x ei n a e ut s o h t t e s
sa iiy t blt .
Ke r s y wo d :h ts le t t o o o v n h d;T O2n n b l ;c c i e me i a o et s o an ;Au lb l d DNA;a t me e s r — ee a p a rs n o
本文利用离 子液体 采用 溶 剂热 法合成 了锐 钛矿 T0 i。
纳米颗粒 , 在此基础上, 采用乙醇热溶剂法合成出T0 纳 i。
米带 。将 所合成的 TO 纳米带 与可 卡 因适体 和壳 聚糖 混 i 合后 固定在玻碳电极 上 , 研制 成可卡 因适体传 感器 。利 用
定向原位生长TiO2纳米条涂层及其固相微萃取性能研究
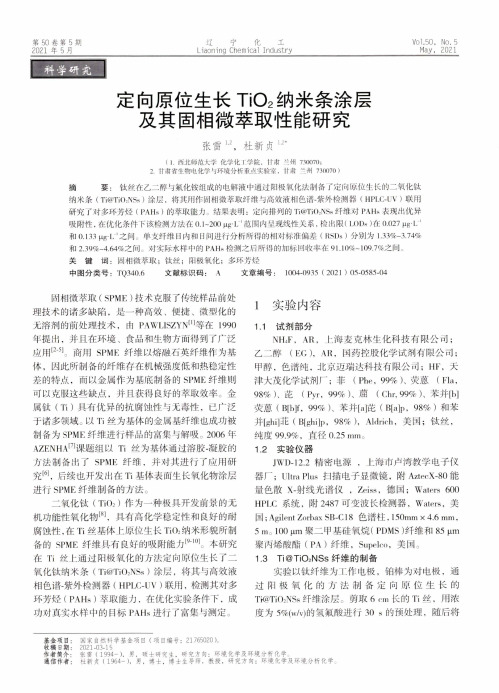
辽 宁化工 Liaoning Chemical Industry
Vol.50, No. 5 May, 2021
定向原位生长Ti〇2纳米条涂层 及其固相微萃取性能研究
张雷1' 杜新贞0
( 1 . 西 北 师 范 大 学 化 学 化 工 学 院 ,甘 肃 兰 州 730070; 2 . 甘肃省生物电化学与环境分析重点实验室,甘 肃 兰 州 730070 >
JWD-12.2精 密 电 源 ,上海市卢湾教学电子仪 器 厂 ; Ultra P lu s扫描电子显微镜,附 AztecX-80能 量 色 散 X-射 线 光 谱 仪 ,Zeiss, 德 国 ; Waters 600 HPLC系 统 ,附 2487可变波长检测器,Waters,美 国 ; Agilent Zorbax SB-C18 色谱 柱 ,150mm x 4_6 mm, 5 m。100 nm 聚二甲基硅氧烷( PDMS )纤 维 和 85 nm 聚 丙 烯 酸 酯 (PA ) 纤 维 ,Supelfo, 美 国 。 1.3 Ti@ Ti0 2N S s 纤维的制备
二 氧 化 钛 (Ti0 2) 作为一种极具开发前景的无 机 功 能 性 氧 化 物 [8],具 有 高 化 学 稳 定 性 和 良 好 的 耐 腐蚀性,在 T i丝基体上原位生长Ti0 2纳米形貌所制 备 的 SPME纤 维 具 有 良 好 的 吸 附 能 力 本 研 究 在 T i丝上通过阳极氧化的方法定向原位生长了二 氧 化 钛 纳 米 条 (Ti@ TiO:NSs) 涂 层 ,将其与高效液 相色谱-紫外检测器(HPLC-UV )联 用 ,检测其对多 环 芳 烃 ( PAHs ) 萃 取 能 力 ,在优化实验条件下,成 功 对 真 实 水 样 中 的 目 标 PAHs进 行 了 富 集 与 测 定 。
镍钛类水滑石衍生物富氧选择性催化还原NO
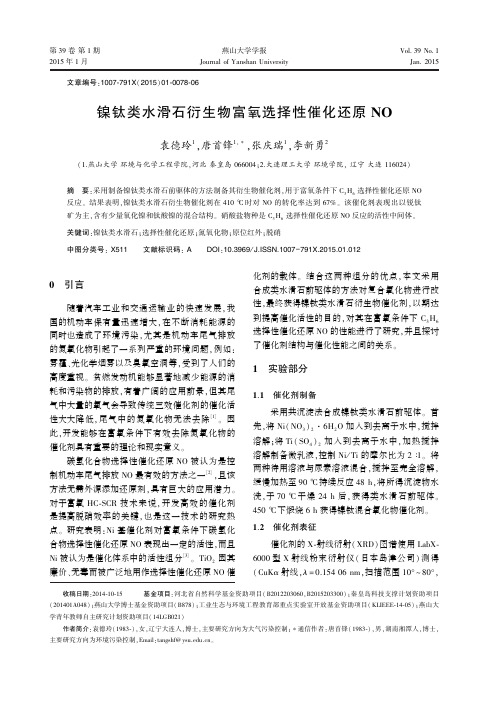
第39卷第1期燕山大学学报Vol 39No 12015年1月JournalofYanshanUniversityJan.2015㊀㊀文章编号:1007⁃791X(2015)01⁃0078⁃06镍钛类水滑石衍生物富氧选择性催化还原NO袁德玲1,唐首锋1,∗,张庆瑞1,李新勇2(1.燕山大学环境与化学工程学院,河北秦皇岛066004;2.大连理工大学环境学院,辽宁大连116024)收稿日期:2014⁃10⁃15㊀㊀㊀基金项目:河北省自然科学基金资助项目(B2012203060,B2015203300);秦皇岛科技支撑计划资助项目(201401A048);燕山大学博士基金资助项目(B878);工业生态与环境工程教育部重点实验室开放基金资助项目(KLIEEE⁃14⁃05);燕山大学青年教师自主研究计划资助项目(14LGB021)作者简介:袁德玲(1983⁃),女,辽宁大连人,博士,主要研究方向为大气污染控制;∗通信作者:唐首锋(1983⁃),男,湖南湘潭人,博士,主要研究方向为环境污染控制,Email:tangshf@ysu.edu.cn㊂摘㊀要:采用制备镍钛类水滑石前驱体的方法制备其衍生物催化剂,用于富氧条件下C3H6选择性催化还原NO反应㊂结果表明,镍钛类水滑石衍生物催化剂在410ħ时对NO的转化率达到67%㊂该催化剂表现出以锐钛矿为主,含有少量氧化镍和钛酸镍的混合结构㊂硝酸盐物种是C3H6选择性催化还原NO反应的活性中间体㊂关键词:镍钛类水滑石;选择性催化还原;氮氧化物;原位红外;脱硝中图分类号:X511㊀㊀文献标识码:A㊀㊀DOI:10.3969/J.ISSN.1007-791X.2015.01.0120㊀引言随着汽车工业和交通运输业的快速发展,我国的机动车保有量迅速增大,在不断消耗能源的同时也造成了环境污染,尤其是机动车尾气排放的氮氧化物引起了一系列严重的环境问题,例如:雾霾㊁光化学烟雾以及臭氧空洞等,受到了人们的高度重视㊂贫燃发动机能够显著地减少能源的消耗和污染物的排放,有着广阔的应用前景,但其尾气中大量的氧气会导致传统三效催化剂的催化活性大大降低,尾气中的氮氧化物无法去除[1]㊂因此,开发能够在富氧条件下有效去除氮氧化物的催化剂具有重要的理论和现实意义㊂碳氢化合物选择性催化还原NO被认为是控制机动车尾气排放NO最有效的方法之一[2],且该方法无需外源添加还原剂,具有巨大的应用潜力㊂对于富氧HC⁃SCR技术来说,开发高效的催化剂是提高脱硝效率的关键,也是这一技术的研究热点㊂研究表明:Ni基催化剂对富氧条件下碳氢化合物选择性催化还原NO表现出一定的活性,而且Ni被认为是催化体系中的活性组分[3]㊂TiO2因其廉价㊁无毒而被广泛地用作选择性催化还原NO催化剂的载体㊂结合这两种组分的优点,本文采用合成类水滑石前驱体的方法对复合氧化物进行改性,最终获得镍钛类水滑石衍生物催化剂,以期达到提高催化活性的目的,对其在富氧条件下C3H6选择性催化还原NO的性能进行了研究,并且探讨了催化剂结构与催化性能之间的关系㊂1㊀实验部分1.1㊀催化剂制备采用共沉淀法合成镍钛类水滑石前驱体㊂首先,将Ni(NO3)2㊃6H2O加入到去离子水中,搅拌溶解;将Ti(SO4)2加入到去离子水中,加热搅拌溶解制备微乳液,控制Ni/Ti的摩尔比为2ʒ1㊂将两种待用溶液与尿素溶液混合,搅拌至完全溶解,缓慢加热至90ħ持续反应48h,将所得沉淀物水洗,于70ħ干燥24h后,获得类水滑石前驱体㊂450ħ下煅烧6h获得镍钛混合氧化物催化剂㊂1.2㊀催化剂表征催化剂的X⁃射线衍射(XRD)图谱使用LabX⁃6000型X射线粉末衍射仪(日本岛津公司)测得(CuKα射线,λ=0.15406nm,扫描范围10ʎ 80ʎ,第1期袁德玲等㊀镍钛类水滑石衍生物富氧选择性催化还原NO79㊀扫描速度5ʎ/min)㊂催化剂的物理结构使用NOVA4200e型气体吸附分析仪(美国康塔仪器公司)测定,N2吸附⁃脱附实验在-198ħ(液氮)条件下进行㊂X⁃射线电子能谱(XPS)表征采用Perkin⁃ElmerPHI5600X⁃射线光电子能谱仪进行㊂辐照光源采用AlKα,能量为1486.6eV,电压15kV,电流为20mA㊂全谱扫描2次(步长0.5eV),分谱扫描15次(步长0.2eV)㊂图谱校正采用C1s1/2=284.6㊂电子自旋共振(ESR)表征采用德国布鲁克有限公司的EPR/ESR320型电子顺磁共振波谱仪检测㊂测定参数为:有心磁场为4100G,微波频率为9.8GHz(X带),扫频宽度为8000,调制带宽为10.25G,时间常数为40.96ms,时间为2ˑ103ms㊂1.3㊀催化活性测试催化剂的活性测试在固定床气态反应装置上进行㊂石英反应管的直径为6mm,催化剂用量为0.2g㊂反应气成分为1000ppmNO+1000ppmC3H6+10%O2+He(平衡气)㊂反应气流量为100mL/min㊂尾气中的C3H6组分采用气相色谱(天美7980Ⅱ)进行在线分析,采用6DX⁃104色谱柱和氢火焰检测器(FID)㊂NO的测定采用化学传感器多组分气体分析仪Testo350㊂1.4㊀原位红外研究原位FTIR的实验在VERTEX70红外光谱仪(布鲁克公司)上进行,取0.02g样品压成圆片,400ħHe气预处理1h㊂入口气体的流速为40mL/min㊂2㊀结果与讨论2.1㊀催化剂的表征图1为镍钛类水滑石前驱体及其衍生物催化剂的XRD谱图㊂煅烧前的催化剂前驱体表现出明显的层状材料特征,即低角度区的衍射峰强且对称,高角度区的峰弱且对称性差㊂2θʈ11ʎ,24ʎ,34ʎ,37ʎ,47ʎ和61ʎ处的衍射峰被认为是水滑石(003),(006),(101),(012),(015)和(116)晶面的衍射峰㊂说明本研究合成的催化剂前驱体具有水滑石的结构㊂煅烧后得到的催化剂层状结构消失,表现出氧化物的结构,2θʈ25.28ʎ㊁37.80ʎ㊁48.05ʎ㊁55.06ʎ㊁62.69ʎ和68.76ʎ处的衍射峰均对应锐钛矿结构㊂此外,2θʈ43.36ʎ㊁63.01ʎ衍射峰对应于钛酸镍,37.24ʎ对应于氧化镍㊂由XRD的谱图可知镍钛类水滑石衍生物催化剂表现为以锐钛矿为主,同时含有少量的钛酸镍和氧化镍的混合结构㊂A标记锐钛矿相,N标记氧化镍相,S标记钛酸镍相图1㊀镍钛类水滑石衍生物催化剂的XRD图谱Fig.1㊀XRDpatternsofNiTihydortalcite⁃derivedcatalyst㊀㊀采用氮气吸附的方法对催化剂的比表面积以及孔径分布进行表征㊂图2为镍钛类水滑石衍生物催化剂氮气吸附⁃脱附曲线及孔径分布情况㊂其吸附曲线为Ⅳ型,回滞环为H2型,说明该催化剂具有复杂的孔结构,孔的形状主要是圆柱形和球形㊂根据BET公式计算催化剂的比表面积是171m2/g,BJH公式计算催化剂的平均孔径是3.82nm㊂根据XRD的表征结果说明镍钛类水滑石衍生物催化剂的主要晶相为锐钛矿,而商品锐钛矿比表面积为47m2/g[4],与之相比,本研究中合成的镍钛类水滑石衍生物催化剂具有较大的比表面积,可以提供更多的吸附位点,有利于提高催化反应活性㊂采用XPS的手段对NiTi类水滑石衍生物催化剂的表面元素价态进行表征,结果如图3所示㊂图3(a)为镍钛类水滑石衍生物催化剂的XPS全谱,从图中可以看出所有样品都主要含有Ni㊁Ti和O元素㊂图3(b)给出了催化剂中Ni元素2p轨道的结合能图谱,催化剂的Ni2p3/2和Ni2p1/2轨道在856ʃ0.4eV和853ʃ0.4eV处出现结合能谱峰,表明镍以正二价形式存在[5]㊂显然,正二价的镍比80㊀燕山大学学报2015正一价的镍和零价的镍具有更高的正电,即更高的受电子性㊂NO是典型的带有负电的物种,因此,正二价的镍更容易和NO发生化学吸附,有利于催化反应的发生㊂图2㊀镍钛类水滑石衍生物催化剂的N2吸附脱附曲线及孔径分布图谱Fig.2㊀N2adsorption⁃desorptionisothermsandBJHporesizedistribution(inset)ofNiTihydortalcite⁃derivedcatalyst㊀㊀图3(c)给出了O1s轨道的结合能图谱㊂O1s的结合能谱峰宽且明显不对称,表明催化剂表面存在多个氧物种㊂其中,530eV处较为明显的结合能峰是与金属离子相连的晶格氧的特征峰,结合能稍高的峰对应的是表面化学吸附氧㊁缺陷氧以及低配位的氧物种㊂说明催化剂中的氧的主要以氧化物中的晶格氧形式存在,并含有少量表面化学吸附氧[5⁃6]㊂已有的研究表明,这些表面化学吸附氧物种对催化反应有着积极的贡献[6]㊂图3(d)中是Ti2p轨道的结合能图谱,Ti2p3/2和Ti2p1/2轨道的分别在大约458ʃ0.4eV和464ʃ0.4eV处出现结合能谱峰,说明催化剂中表面的Ti以正四价形式存在[7]㊂㊀㊀图4为镍钛类水滑石衍生物催化剂的ESR图谱㊂Ni元素在ESR检测中只有Ni2+有ESR信号,其他形式的Ni均无信号㊂图中显示样品中存在正二价的Ni,且并无超精细结构存在,这一结果与XPS表征结果一致㊂镍钛类水滑石衍生物催化剂表现出了各向同性的ESR相应信号,表明Ni2+在正八面体结构的中心位置,且正八面体结构晶体结构的周期性良好[8]㊂ESR表征结果进一步说明正二价的镍位于正八面体结构的中心,且晶体结构良好㊁分散均匀㊂分散均匀被普遍认为是有利于催化反应活性的提高㊂图3㊀镍钛类水滑石衍生物催化剂的XPS图谱Fig.3㊀XPSprofileofNiTihydortalcite⁃derivedcatalyst2.2㊀催化活性评价图5显示了镍钛类水滑石衍生物催化剂在富氧条件下选择性催化还原NO的催化性能㊂随着反应温度的升高,NO的转化率先增加后下降,410ħ时N2收率达到最大值67%㊂C3H6的转化率随着反应温度的升高而增加,430ħ时完全转化㊂NO的转化率达到最大值时的410ħ对应C3H6的转化率接近100%,由此说明反应温度超过410ħ时,NO的转化率的下降是由缺少还原剂引起的㊂作为一种廉价的混合氧化物催化剂,该催化剂的富氧条件下的催化活性表现出一定的优势[9]㊂原因可能是催化剂中正二价的镍均匀地分散在正八面体晶格结构的中心,为含氮物种提供了良好的化学吸附位点㊂此外,表面表化学吸附第1期袁德玲等㊀镍钛类水滑石衍生物富氧选择性催化还原NO81㊀O物种对催化反应有着积极的贡献,也可能是较高催化活性的原因㊂为了进一步探讨化学吸附物种的形成及催化机理,采用原位红外的手段对反应过程进行详细的研究㊂图4㊀镍钛类水滑石衍生物催化剂的ESR图谱Fig.4㊀ESRprofileofNiTihydortalcite⁃derivedcatalyst图5㊀镍钛类水滑石衍生物催化剂上NO转化率和C3H6转化率Fig.5㊀CatalyticperformanceofNiTihydortalcite⁃derivedcatalystasafunctionoftemperature2.3㊀原位红外研究原位红外的手段能够研究反应中间体及反应机理,能够在线的给出反应过程中催化剂表面形成的吸附物种的种类及相对含量的变化情况,是目前研究催化反应机理较为先进的手段㊂图6(a)给出了镍钛类水滑石衍生物催化剂最佳反应温度条件下共吸附NO和O2的红外图谱,图中1905cm-1和1845cm-1处的红外谱峰归属为NO的气态吸附(NO-),1627cm-1和1599cm-1归属于二齿桥式酸盐,1577cm-1和1504cm-1处的红外谱峰归属于单齿酸盐,1360 1390cm-1处的红外谱峰归属离子态的硝酸盐(NO3-)2[10]㊂图中单齿硝酸盐随吸附时间的延长逐渐分解,表明单齿硝酸盐在反应条件下不稳定㊂试验结果表明:随着反应过程中NO和O2通过镍钛类水滑石衍生物催化剂,催化剂表面可能形成的含氮物种为气态吸附NO㊁二齿桥式酸盐以及不稳定的单齿硝酸盐㊂图6(b)给出了镍钛类水滑石衍生物催化剂最佳反应温度条件下吸附C3H6的红外图谱,图中1660cm-1处的红外吸收谱峰归属为表面吸附的C3H6,1577cm-1处的红外峰归属为乙酸盐,1440cm-1处的红外峰归属为碳酸盐,1370cm-1和1328cm-1处的红外峰归属为甲酸盐[11]㊂图中1630 1450cm-1范围内出现的较宽红外吸收峰是有机分子上不同形式的振动重叠引起的,说明催化剂表面形成了大量的C3H6不完全氧化产物㊂试验结果表明:在反应气氛条件下,催化剂表面可能形成了表面吸附的C3H6㊁乙酸盐㊁碳酸盐㊁甲酸盐以及大量的C3H6不完全氧化产物㊂为了进一步研究反应条件下中间体的变化情况以及反应机理,图6(c)给出了镍钛类水滑石衍生物催化剂最佳反应温度㊁反应气氛条件下及关闭反应系统后的红外图谱㊂在反应气氛条件下检测到气态吸附-NO(1905cm-1和1845cm-1)㊁二齿硝酸盐(1627cm-1和1600cm-1)㊁单齿硝酸盐(1577cm-1和1504cm-1)㊁C3H6(1664cm-1)㊁乙酸盐(1577cm-1)㊁甲酸盐(1370cm-1和1328cm-1)以及碳酸盐(1440cm-1)㊂该结果说明:在反应过程中,催化剂表面形成了上述含氮物种及C3H6不完全氧化产物㊂㊀㊀关闭红外反应池的进气口和出气口后继续反应并采集红外图谱(6(c)Ⅰ和Ⅱ)Ⅰ和Ⅱ为反应气氛30min后关闭系统继续反应30min和90min,能够反应在封闭体系内物种的反应特性㊂关闭反应系统后,硝酸盐的吸收峰消失,乙酸盐㊁甲酸盐和碳酸盐的吸收峰强度明显增强㊂表明硝酸盐和未完全氧化的碳氢化合物发生反应被消耗,因而吸收峰消失,说明硝酸盐是该反应的活性物种㊂在没有气体吹扫的条件下,红外池中的残留的C3H6在催化剂表面不断发生化学吸附,且没有足够的含氮吸附物种与之反应,导致乙酸盐㊁甲82㊀燕山大学学报2015酸盐和碳酸盐的吸收峰强度明显增强,这一结果说明该催化剂具有非常强的C3H6不完全氧化能力㊂原位红外的研究结果说明,NO在富氧条件下能够在镍钛类水滑石衍生物催化剂表面生成硝酸盐,这些硝酸盐可以和C3H6的不完全氧化物种发生反应,硝酸盐是C3H6选择性催化还原反应的重要中间体㊂图6㊀镍钛类水滑石衍生物催化剂的原位FTIR图谱Fig.6㊀InsituFTIRspectraoverNiTihydortalcite⁃derivedcatalyst3 结论1)本文合成了镍钛类水滑石前驱体,其衍生物催化剂用于富氧条件下C3H6选择性催化还原NO反应表现出了良好的催化活性,410ħ时NO的转化率达67%㊂2)镍钛类水滑石衍生物催化剂的结构以锐钛矿为主,含有少量氧化镍和钛酸镍的混合结构,镍元素以正二价的形式存在㊂3)硝酸盐是C3H6选择性催化还原NO反应的活性中间体,提高反应中硝酸盐的生成量可以提高反应的催化活性㊂参考文献1 WuY ZhangSJ LiML etal.ThechallengetoNOxemissioncontrolforheavy⁃dutydieselvehiclesinChina J .AtmosphericChemistryAndPhysics 2012 12 19 9365⁃9379.2 ThirupathiB SmirniotisPG.Nickel⁃dopedMn/TiO2asaneff⁃cientcatalystforthelow⁃temperatureSCRofNOwithNH3 Cata⁃lyticevaluationandcharacterizations J .JournalofCatalysis2012 288ʒ74⁃83.3 BaranR KaminskaII SrebowataA etal.Selectivehydrodechlo⁃rinationof1 2⁃dichloroethaneonNiSiBEAzeolitecatalyst InfluenceofthepreparationprocedureonahighdispersionofNicenters J .MicroporousandMesoporousMaterials 2013 169ʒ120⁃127.第1期袁德玲等㊀镍钛类水滑石衍生物富氧选择性催化还原NO83㊀4 邹学军李新勇.肇启东等.可见光响应的LaVO4/TiO2纳米管的合成㊁表征及对气相甲苯的光催化性能 J .高等学校化学学报2012 335 1046⁃1049.5 GuanB LinH ZhuL etal.SelectivecatalyticreductionofNOxwithNH3overMn CesubstitutionTi0.9V0.1O2⁃deltananocompositescatalystspreparedbyself⁃propagatinghigh⁃temper⁃aturesynthesismethod J .JournalofPhysicalChemistryC 2011 115 26 12850⁃12863.6 ChenZH WangFR LiH etal.Low⁃temperatureselectivecat⁃alyticreductionofNOxwithNH3overFe⁃Mnmixed⁃oxidecatalystscontainingFe3Mn3O8Phase J .Industrial&EngineeringChemis⁃tryResearch 2012 51 1 202⁃212.7 JiL SreekanthPM SmirniotisPG etal.Manganeseoxide/ti⁃taniamaterialsforremovalofNOxandelementalmercuryfromfluegas J .Energy&Fuels 2008 22 4 2299⁃2306.8 DorettaC MarcellaB VincenzoM etal.CrandNiDopingofLi4Ti5O12CationDistributionandFunctionalProperties J .JournalofPhysicalChemistryC 2009 113 45 19664⁃19671. 9 KlingstedtF ArveK EranenK etal.Towardimprovedcatalyticlow⁃temperatureNOxremovalindiesel⁃poweredvehicles J .AccountsofChemicalResearch 2006 39 4 273⁃282.10 YuanD LiX Zhaoj etal.AnovelCu⁃containingcatalystde⁃rivedfromTi⁃embeddedhydrotalcite⁃likecompoundsforselectivecatalyticreductionofNOwithC3H6underlean⁃burnconditions J .JournalofCatalysis 2014 309 268⁃279.11 ShanW LiuF HeH etal.AsuperiorCe⁃W⁃TimixedoxidecatalystfortheselectivecatalyticreductionofNOxwithNH3 J .AppliedCatalysisB⁃Environmental 2012 115⁃116 100⁃106.NiTihydrotalcite⁃derivedcatalystforselectivecatalyticreductionofNOunderlean⁃burnconditionsYUANDe⁃ling1TANGShou⁃feng1ZHANGQing⁃rui1LIXin⁃yong21.SchoolofEnvironmentalandChemicalEngineering YanshanUniversity Qinhuangdao Hebei066004 China 2.SchoolofEnvironmentalScienceandTechnology DalianUniversityofTechnolgy Dalian Liaoning116024 ChinaAbstract AderivativecatalystwasderivedfromtheNiTihydrotalcite⁃likecompound andtestedintheselectivecatalyticreductionofNObyC3H6inthepresenceofexcessO2.TheresultshowedthattheNOconversionofNiTihydrotalcite⁃derivedcatalyswasa⁃chieved67%at410ħ.Amajoranatasewithlessnickeloxideandnickeltitanatemixedstructurewasdisplayedinthiscatalyst andnitrateNO3-wasthereactiveintermediateproducedduringtheselectivecatalyticreductionofNOwithC3H6.Keywords NiTihydrotalcite⁃likecompound selectivecatalyticreduction nitrogenoxide InsituFTIR DeNOx上接第67页ResearchonprofessorLISai⁃meiᶄsexperienceoftreatingdiabetesmellitusbasedonpartialorderedstructuretheoryXUSun⁃jing1LISai⁃mei1ZHUZhang⁃zhi2LIYat⁃tung1LIUYu⁃zhou1LIUChao⁃nan11.FirstSchoolofClinicalMedicine GuangzhouUniversityofChineseMedicine Guangzhou Guangdong510405 China2.DepartmentofEndocrinology TheFirstaffiliatedHospitalofGuangzhouUniversityofChineseMedicine Guangzhou Guangdong510405 ChinaAbstract SyndromedifferentiationandtreatmentisacharacteristicoftraditionalChinesemedicine.Sincetheaccuracyofsyndromedifferentiationandtreatmentreliesondoctorsᶄwitsandsavvy theclinicalskillislimitedindevelopment.ThemassiveclinicaldataofthetraditionalChinesemedicinehasthecharacteristicofbigdata.Intheageofbigdata thefocusofpresentresearchistoestab⁃lishabigdatamodelforTCM soastoprovideanewthinkingforinheritingandpreservingthesufficientclinicalexperienceofTCM.Basedontheoryofformalconceptanalysis apreliminaryanalysisismadeonprofessorLIᶄsrulesofSyndromedifferentiationintreatingdiabetesmellitus.ThefeaturesofsymptomandsignforShaoyangsyndromearediscussedfromtwelveaspects.Itisconclu⁃dedthatthemethodbasedonthetheoryofformalconceptanalysisprovideanewresearchideaforbigdataofTCM whichhassig⁃nificantvalueinacademicresearchandapplication.Keywords formalconceptanalysis diabetesmellitus Shaoyang LISai⁃mei attributepartialorderedstructurediagram。
卟啉敏化的钛酸盐纳米管的可见光催化活性

关 键 词 : 酸 盐 纳 米 管 ;卟啉 ;敏 化 ; 催 化 钛 光
中图分类号 : 6 1 0 1. 3
文献标识码 : A
文章编号 :10 -8 1 0 1l一9 90 0 14 6 ( t)o1 9 .6 2
P o o a a y i tvt fTi n t n t b sS n iie t o p y i h t c t l t Aci i o t a e Na o u e e st d wi P r h rn c y a z h
活性 很 高 在 它 的催化 作 用下 . 4 n紫 外光 照 经 0mi
罗丹 明 B的降解 率 可 达 到 9 %左 右 。 目前 , 0 主要 采
用 以下几 种办 法来 获得 在可 见光 区具 有催 化 活性 的 钛 酸 盐 纳 米 管 :1通 过 阳离 子 交 换 , 金 属 离 子 引 f1 将
fA P ) 负 电荷 的钛 酸盐 纳 米管 (N s 间 的静 T PI 与带 T T)  ̄
电 吸 引 作 用 制 备 了 卟 啉 敏 化 的 钛 酸 盐 纳 米 管 (A P.N s. 可 见 光 照射 下考 察 了 T P I N s T P I T 1在 T A P . T T 对 甲基橙 光 降解 的催 化 活 性 .并 对 T P I N s A P. T 光 T
第 2 7卷 第 1 0期 21 年 1 01 0月
无
机
化
学
学
报
Vo .7 No1 1 .0 2 19 .0 4 9 92 0
C N EJ HI ES OURNAL 0FI NORGANI C CHEMI T S RY
二氧化钛胶粒嵌入超细层状钛酸盐制备金红石型氧化钛纳米纤维(中英文混合版)
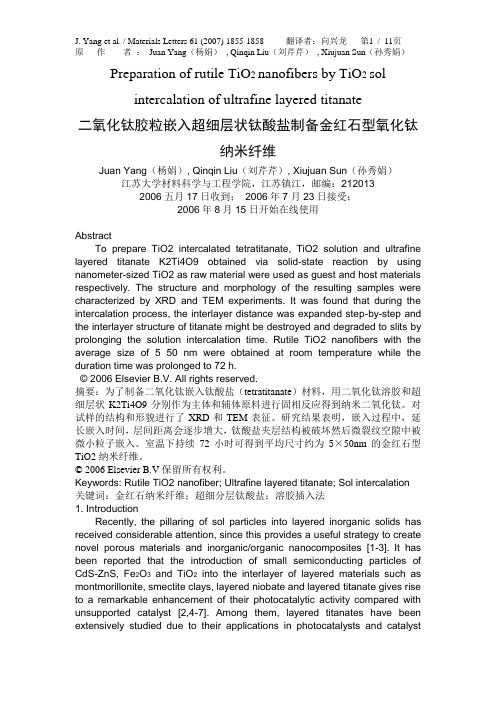
Preparation of rutile TiO2 nanofibers by TiO2 solintercalation of ultrafine layered titanate二氧化钛胶粒嵌入超细层状钛酸盐制备金红石型氧化钛纳米纤维Juan Yang(杨娟), Qinqin Liu(刘芹芹), Xiujuan Sun(孙秀娟)江苏大学材料科学与工程学院,江苏镇江,邮编:2120132006五月17日收到;2006年7月23日接受;2006年8月15日开始在线使用AbstractTo prepare TiO2 intercalated tetratitanate, TiO2 solution and ultrafine layered titanate K2Ti4O9 obtained via solid-state reaction by using nanometer-sized TiO2 as raw material were used as guest and host materials respectively. The structure and morphology of the resulting samples were characterized by XRD and TEM experiments. It was found that during the intercalation process, the interlayer distance was expanded step-by-step and the interlayer structure of titanate might be destroyed and degraded to slits by prolonging the solution intercalation time. Rutile TiO2 nanofibers with the average size of 5×50 nm were obtained at room temperature while the duration time was prolonged to 72 h.© 2006 Elsevier B.V. All rights reserved.摘要:为了制备二氧化钛嵌入钛酸盐(tetratitanate)材料,用二氧化钛溶胶和超细层状K2Ti4O9分别作为主体和辅体原料进行固相反应得到纳米二氧化钛。
- 1、下载文档前请自行甄别文档内容的完整性,平台不提供额外的编辑、内容补充、找答案等附加服务。
- 2、"仅部分预览"的文档,不可在线预览部分如存在完整性等问题,可反馈申请退款(可完整预览的文档不适用该条件!)。
- 3、如文档侵犯您的权益,请联系客服反馈,我们会尽快为您处理(人工客服工作时间:9:00-18:30)。
Hydrogen sensing using titania nanotubesOomman K.Varghese,Dawei Gong,Maggie Paulose,Keat G.Ong,Craig A.Grimes *Department of Electrical Engineering and Materials Research Institute,217Materials Research Laboratory,The Pennsylvania State University,University Park,PA 16802,USAAbstractTitanium dioxide nanotubes,made by anodization,are highly sensitive to hydrogen;for example,cycling between nitrogen atmosphere and 1000ppm hydrogen a variation in measured resistance of 103is seen for 46nm diameter nanotubes at 2908C.The hydrogen sensors are completely reversible and have response times of approximately 150s.Field emission scanning electron microscopy and Glancing angle X-ray diffraction (GAXRD)are used to study the surface morphology and crystal structure of the nanotubes.#2003Elsevier Science B.V .All rights reserved.Keywords:Titania;Nanotube;Hydrogen;Nanoporous;Gas sensor1.IntroductionWe recently reported [1]the fabrication of self organized titania nanotube arrays using an anodization technique.Although the as prepared nanotubes are amorphous,they crystallize on annealing at elevated temperatures and are structurally stable to at least 6008C.This stability of structure,which is one of the essential criteria of a gas sensing material,prompted us to study the gas sensing behavior of these nanotubes to technologically important gases,such as oxygen,carbon monoxide,ammonia,carbon dioxide and hydrogen.Titania has earned much attention for its oxygen sensing capabilities [2–7].Furthermore with proper manipulation of the microstructure,crystalline phase and/or addition of proper impurities or surface functionalization titania can also be used as a reducing gas sensor [8–19].The interaction of a gas with a metal oxide semiconductor is primarily a surface phenomenon,therefore nanoporous metal oxides [14,15,20,21]offer the advantage of providing large sensing surface areas.Hydrogen sensing is needed for industrial process control,combustion control,and in medical applications with hydro-gen indicating certain types of bacterial infection.In this work we report on the hydrogen sensing properties of titania nanotubes made via anodization [1].Room temperature metal oxide hydrogen sensors are generally based on Schottky barrier modulation of devices like Pd/TiO 2orPt/TiO 2by hydrogen [22–24].Elevated temperature hydro-gen sensors examine the electrical resistance with hydrogen concentration;for example,Birkefeld et al.[25]observed that the resistance of anatase phase of titania varies in presence of carbon monoxide and hydrogen at temperatures above 5008C,but on doping with 10%alumina it becomes selective to hydrogen.2.ExperimentalTitania nanotubes [1]were grown from titanium foil (99.5%pure from Alfa Aesar,Ward Hill,MA,USA)of thickness 0.25mm.The anodization was performed in an electrolyte medium of 0.5%hydrofluoric acid (J.T.Baker-Phillipsburg,NJ,USA)in water,using a platinum foil cathode.Well-defined nanotube arrays were grown using anodizing potentials ranging from 12to 20V .Nanotube length increases with anodization time,reaching a length of 400nm in approximately 20min,and then remains constant.For the present study the samples were anodized for 25min.The samples were then annealed at 5008C in a pure oxygen ambient for 6h,with a heating and cooling rate of 18C/min.A field emission scanning electron microscope (FESEM)from JEOL (model JSM6300),Peabody,MA,USA was used to study the surface morphology of the nanotubes.A glan-cing angle X-ray diffractometer (GAXRD)from Philips (model X’pert MRD PRO),The Netherlands was used to determine the crystalline phase.The electrode geometry of the titania nanotube sensors is shown in Fig.1a .The sensor consists of a basetitaniumSensors and Actuators B 93(2003)338–344*Corresponding author.E-mail address:cgrimes@ (C.A.Grimes).0925-4005/03/$–see front matter #2003Elsevier Science B.V .All rights reserved.doi:10.1016/S0925-4005(03)00222-3metal foil with a nanotube array grown on the top.An insulating barrier layer separates the nanotubes from the conducting titanium foil.Preliminary studies using evapo-rated gold films as well as silver paste as interdigital electrodes showed that these materials diffuse into the titania nanotubes at elevated temperatures resulting in sensor con-tamination.Hence a pressure contact was used to electrically contact the nanotubes,with two spring-loaded parallel 10mm by 2mm platinum contact pads (100m m thickness).A schematic diagram of the experimental set up used for the gas sensing studies is shown in Fig.1b .The test chamber consists of a 1.3l quartz tube,with stainless steel end caps,placed inside a furnace (Thermolyne,USA model 21100tubular furnace).The electrical contact was formed by attaching the platinum pads to the ends of a spring-loaded ‘U ’shaped quartz rod.Gas flow through the test chamber was controlled via a computer-controlled mass flow con-troller (MKS instruments,Austin,TX,USA).The electrical resistance of the titania sensors were measured using a computer-controlled digital multimeter (Keithley,USA model 2000).Prior to data collection the test chamber was initially evacuated using a mechanical pump,where-upon nitrogen (99.999%pure)was passed while the sensorunder test was heated to the desired temperature.The test gases examined,oxygen,carbon dioxide,ammonia,carbon monoxide or hydrogen,were mixed in appropriate ratios with nitrogen to create the necessary test gas ambient.3.Results and discussionThe surface morphology of nanotube arrays prepared using an anodization potential of 20V and annealed at 5008C for 6h in a pure oxygen ambient is shown in Fig.2a and b .It can be seen from these images that the nanotubes are uniform over the surface.The nanotubes are approximately 400nm in length and have a barrier layer [1]thickness of %50nm.For the nanotubes fabricated using 20V anodization the average pore diameter,as determined from FESEM images,is 76nm (standard deviation 15nm),with a wall thickness of 27nm (standard deviation 6nm).The sample anodized at 12V was found to have an average pore diameter of 46nm (standard deviation 8nm)with a wall thickness 17nm (standard deviation 2nm).The por-osities of the 20and 12V samples were calculated as 45and 61%,respectively.Fig.1.Schematic representation of (a)the electrode geometry,and (b)the experimental apparatus.O.K.Varghese et al./Sensors and Actuators B 93(2003)338–344339Glancing angle X-ray diffraction patterns of a 20V sample annealed at 5008C for 6h in oxygen ambient is shown in Fig.3.It can be seen that both anatase and rutile phases of titania are present in the sample.A detailed study [26]of these structures using high resolution transmission electron microscope (HRTEM)showed that the anatase crystallites were concentrated on the walls of the nanotubes and rutile on the barrier layer.Nanotubes annealed in a pure oxygen ambient were found to be stable (intact)to temperatures of approximately 5808C.Above this temperature protrusions were seen com-ing out through the nanotubes,an effect which spread with increasing temperature.These protrusions,which are due to oxidation of the titanium substrate,collapse the nanotubes.Fig.4shows the response of the 20V sample as a function of ambient temperature,as it is switched from a nitrogen environment to one containing 1000ppm hydrogen,and then back to nitrogen.The plot was made using (R g /R 0)À1versus time where R 0is the base resistance of the sensor,i.e.the sensor resistance before introducing the test gas,and R g the measured resistance in the presence of test gas.The sensor shows increasing hydrogen sensitivity with tempera-ture,with a three order of magnitude change in resistance at temperatures above %3008C.At all the temperatures the original resistance it recovered without hysteresis.The sensitivity S is de fined by the formula S ¼R 0ÀR gsR gswhere R 0is the resistance of the sensor before passing the gas and R gs that after passing gas and reaching the saturation value.The sensitivity of a 20V sample with temperature,to 1000ppm hydrogen,is shown in Fig.5.Sensitivity is seen to increase with temperature to approximately 3808C where the increase in sensitivity with temperature is beginning to saturate.The response time,de fined as the time needed for the sensor to reach 90%of the final signal for a given concen-tration of gas,is plotted against temperature in Fig.6(the time includes that required for the gas to equilibrateinsideFig.2.The surface morphology of the titania nanotubes after annealing at 5008C:(a)high,and (b)low magnification images of a 20V sample,and (c)a high magnification image of a 12Vsample.Fig.3.Glancing angle X-ray diffraction pattern of a 20V sample (glancing angle ¼28)annealed at 5008C in oxygen ambient.A ,R and T represent the reflections from anatase crystallites,rutile crystallites,and the titanium substrate,respectively.340O.K.Varghese et al./Sensors and Actuators B 93(2003)338–344the measurement chamber,estimated to be %30s).The response time reduces exponentially with temperature.To check the behavior of the sensor on repeated hydrogen exposure,the hydrogen concentration was varied in discrete steps of 100ppm from 0to 500ppm while keeping the temperature constant at 2908C;the chamber was flushed with nitrogen after each exposure to hydrogen.The responseof the 20V prepared nanotube sensors,kept at 2908C,is shown in Fig.7.The behavior of the sensor is consistent,recovering its original resistance after repeated exposure to varying hydrogen gas concentrations.The sensitivity of the sensor in this concentration range is plotted in Fig.8a ;there is a linear increase in sensitivity at low concentrations.The sensitivity of the hydrogen sensor over 100ppm to 4%(the explosive limit in the presence of oxygen)is shown in Fig.8b .Fig.9shows the hydrogen sensitivities,at 2908C,of nanotube sensors having a pore diameter of 76nm,and a pore diameter of 46nm.While smaller diameter nanotubes had greater sensitivity to hydrogen the samples made at lower anodizing voltages tended to be more brittle,and harder to mechanically handle without breaking.The 20V sample was exposed to oxygen,carbon mon-oxide,ammonia and carbon dioxide at 2908C.The sensor was found to have no detectable variation in resistance on exposure to carbon dioxide.The sensitivities of thetitaniaFig.4.Variation of resistance R g ,normalized with respect to baseline resistance R 0,of a 20V sample with time on exposure to 1000ppm hydrogen at different temperatures.It may be noted that the inverse of R g /R 0was used in the plot for representing data in positive y-direction.Fig. 5.The sensitivity temperature dependence of a 20V sample to 1000ppmhydrogen.Fig.6.Response time variation of a 20V sample to temperature.The dots represent measureddata.Fig.7.Resistance of a 20V sample when exposed to different concentrations of hydrogen at 2908C.The nitrogen –hydrogen mixture was passed for 1500s;the chamber was then flushed with nitrogen for 3000s before passing the nitrogen –hydrogen mixture again.O.K.Varghese et al./Sensors and Actuators B 93(2003)338–344341nanotubes to the other gases is shown in Fig.10.The sensitivity of the nanotubes to carbon monoxide and ammo-nia are negligible compared to that of hydrogen.The resis-tance of the nanotubes increased in presence of oxygen,and did not regain their original electrical conductivity even after several hours in a nitrogen environment.Since the sensor measurements were conducted in atmo-spheres without oxygen,the increase in conductivity cannot be due to hydrogen removing oxygen from the lattice [27–29]or the removal of chemisorbed oxygen [30–32].It is likely that the hydrogen molecules get dissociated at the defects on the titania surface.These molecules can diffuse into the titania lattice,and act as electron donors [25,33,34].But this process would lead to very slow response and recovery times and complete recovery would be dif ficult.Since the sensor completely regains its original resistance with hydrogen cycling it appears that this is not the domi-nant mechanism behind high hydrogen sensitivity.Hence,we believe that the major process behind the interaction between the nanotubes and hydrogen is the chemisorption of the dissociated hydrogen on the titania surface [32].During chemisorption hydrogen acts as a surface state and a partial charge transfer takes place from hydrogen to the conduction band of titania.This creates an electron accumulation layer on the nanotube surface that enhances its electrical conductance.On removing the hydrogen ambient,electron transfer takes place back to hydrogen and it desorbs,thus restoring the original resistance of the nanotubes.Another factor that may play a role in the hydrogen sensitivity (and selectivity)is the platinum electrodes.It is possible that platinum is acting as a catalyst for interaction of hydrogen with titania.At elevated temperatures hydrogen dissociation can occur on platinum surfaces.These disso-ciated hydrogen atoms may spill [31,35]onto the nanotube surface where they diffuse into the nanotube surface.From the present study it was not clear how signi ficant a role the platinum electrodes play.Anatase,the polymorph of titania has been reported to be of high sensitivity towards reducing gases like hydrogen and carbon monoxide [13,16,25].Our nanotube samples contain anatase phase mainly on the walls and rutile in the barrier layer.As the diffusing hydrogen atoms go to the interstitial sites [25,33]and as the c /a ratio of anatase is almost four times compared to that of rutile,it appears that anatase lattice accommodates hydrogen easily and hence has a higher contribution to hydrogen sensitivity.The effect of chemisorption can be neglected in the oxygen sensing experiments.As the recovery requires several hours it appears that the nanotubes contain oxygen vacancies or titanium interstitial defects in presence of nitrogen.On exposing the sensor to oxygen ambient,the lattice reoxidizes and hence the conductivity of the sensor decreases.On removing oxygen,the reduction of thelatticeFig.8.The sensitivity variation of a 20V sample at 2908C for (a)low hydrogen concentrations,and (b)0.01to 4%hydrogen concentra-tions.Fig.9.A comparison of the variation in resistance of samples having pore diameters of 46and 76nm,vs.time,upon exposure to 1000ppm of hydrogen at 2908C.Fig.10.Variation of resistance of a 20V sample when exposed to 1000ppm carbon monoxide,5%ammonia and 1%oxygen at 2908C.342O.K.Varghese et al./Sensors and Actuators B 93(2003)338–344will not immediately occur hence the sensor requires several hours to regain its original conductivity.It should be noted that the conducting titanium foil beneath the nanotubes ultimately limits the sensitivity by reducing the baseline resistance of the sensor.4.ConclusionsTitania nanotubes prepared using anodization and annealed in an oxygen atmosphere at a temperature of 5008C were found highly sensitive to hydrogen.The nano-tube sensors contain both anatase and rutile phases of titania, and showed appreciable sensitivity towards hydrogen at temperatures as low as1808C.The sensitivity increased drastically with temperature showing a variation of three orders in magnitude of resistance to1000ppm of hydrogen at4008C.The response time decreased with increasing temperature;at2908C full switching of the sensor took approximately3min.Results were highly reproducible with no indication of hysteresis.Our results showed these sensors are capable of monitoring hydrogen levels from100ppm to 4%.At2908C nanotubes with smaller pore diameter (46nm)showed higher sensitivity to hydrogen compared to larger pore diameter samples(76nm).The sensors showed high selectivity to hydrogen compared to carbon monoxide,ammonia and carbon dioxide.Although the sensor was sensitive to high concentrations of oxygen,the response time was high and the sensor did not completely regain the original condition.We believe that the hydrogen sensitivity of the nanotubes is due to hydrogen chemisorp-tion onto the titania surface where they act as electron donors.In summary,it was demonstrated that sensors com-prised of titania nanotubes prepared using anodization can successfully be used as hydrogen sensors. AcknowledgementsPartial support of this work by the National Science Foundation through grant ECS-9875104is gratefully acknowledged.References[1] D.Gong,C.A.Grimes,O.K.Varghese,W.Hu,R.S.Singh,Z.Chen,E.C.Dickey,Titanium oxide nanotube arrays prepared by anodicoxidation,J.Mater.Res.16(2001)3331.[2]T.Takeuchi,Oxygen sensors,Sens.Actuators14(1988)109.[3]U.Kirner,K.D.Schierbaum,W.Gopel,Low and high temperatureTiO2oxygen sensors,Sens.Actuators B1(1990)103.[4]Y.Xu,K.Yao,X.Zhou,Q.Cao,Platinum–titania oxygen sensorsand their sensing mechanisms,Sens.Actuators B13-14(1993) 492.[5]V.Demarne,S.Balkanova, A.Grisel, D.Rosenfeld, F.Levy,Integrated gas sensor for oxygen detection,Sens.Actuators B13-14 (1993)497.[6]S.Hasegawa,Y.Sasaki,S.Matsuhara,Oxygen-sensing factor ofTiO2doped with metal ions,Sens.Actuators B13-14(1993)509.[7] A.Rothschild, F.Edelman,Y.Komem, F.Cosandey,Sensingbehavior of TiO2thing films exposed to air at low temperatures,Sens.Actuators B67(2000)282.[8]G.Sakai,N.S.Baik,N.Miura,N.Yamazoe,Gas sensing propertiesof tin oxide thin films fabricated from hydrothermally treated nanoparticles dependence of CO and H2response on film thickness, Sens.Actuators B77(2001)116.[9]V.N.Misra,R.P.Agarwal,Thick film hydrogen sensor,Sens.Actuators B21(1991)209.[10]O.K.Varghese,L.K.Malhotra,G.L.Sharma,High ethanol sensiti-vity in sol–gel derived SnO2thin films,Sens.Actuators B55(1999) 161.[11]V.A.Chaudhary,I.S.Mulla,K.Vijayamohanan,Comparative studiesof doped and surface modified tin oxide towards hydrogen sensing: synergistic effects of Pd and Ru,Sens.Actuators B50(1998)45.[12]H.Tang,K.Prasad,R.Sanjines,F.Levy,TiO2anatase thin films asgas sensors,Sens.Actuators B26-27(1995)71.[13]S.A.Akbar,L.B.Younkman,Sensing mechanism of a carbonmonoxide sensor based on anatase titania,J.Electrochem.Soc.144 (1997)1750.[14]Y.Shimizu,N.Kuwano,T.Hyodo,M.Egashira,High H2sensingperformance of anodically oxidized TiO2film contacted with Pd, Sens.Actuators B83(2002)195.[15]M.C.Carotta,M.Ferroni, D.Gnani,V.Guidi,M.Merli,G.Martinelli,M.C.Casale,M.Notaro,Nanostructured pure and Nb doped TiO2as thick film gas sensors for environmental monitoring, Sens.Actuators B58(1999)310.[16]N.O.Savage,S.A.Akbar,P.K.Dutta,Titanium dioxide based hightemperature carbon monoxide selective sensor,Sens.Actuators B72 (2001)239.[17] ini,G.Faglia,G.Sberveglieri,Y.X.Li,W.Wlodarski,M.K.Ghantasala,Sensitivity enhancement towards ethanol and methanol of TiO2films doped with Pt and Nb,Sens.Actuators B64(2000) 169.[18]I.Hayakawa,Y.Iwamoto,K.Kikuta,S.Hirano,Gas sensingproperties of platinum dispersed-TiO2thin film derived from percursor,Sens.Actuators B62(2000)55.[19]N.Savage,B.Chwieroth,A.Ginwalla,B.R.Patton,S.A.Akbar,P.K.Dutta,Composite n–p semiconducting titanium oxides as gas sensors, Sens.Actuators B79(2002)17.[20] C.C.Koch(Ed.)Nanostructured Materials:Processing,Propertiesand Applications,Noyes publications,New York,USA,2002. [21]H.-M.Lin, C.-H.Keng, C.-Y.Tung,Gas sensing properties ofnanotcrystalline TiO2,Nanostructured Mater.9(1997)747. [22]H.Kobayashi,K.Kishimoto,Y.Nakato,Reactions of hydrogen atthe interface of palladium–titanium dioxide schottky diodes as hydrogen sensors studied by work function and electrical character-istic measurements,Surf.Sci.306(1994)393.[23]L.A.Harris,A titanium dioxide hydrogen sensor,J.Electrochem.Soc.Solid State Sci.Technol.127(1980)2657.[24]K.D.Schierbaum,U.K Kirner,J.F.Geiger,W.Gopel,Schottkybarrier and conductivity gas sensors based upon Pd/SnO2and Pt/ TiO2,Sens.Actuators B4(1991)87.[25]L.D.Birkefeld, A.M.Azad,S.A.Akbar,Carbon monoxide andhydrogen detection by anatase modification of titanium dioxide,J.Am.Ceram.Soc.75(1992)2964.[26]O.K.Varghese,D.Gong,M.Paulose,C.A.Grimes,E.C.Dickey,Structural stability of titanium oxide nanotube arrays,J.Mater.Res.18(2003)156.[27]R.D.Shannon,Phase transformation studies in TiO2supportingdifferent defect mechanisms in vacuum-reduced and hydrogen-reduced rutile,J.Appl.Phys.35(1964)3414.[28]K.H.Kim,E.J.Ju,J.S.Choi,Electrical conductivity of hydrogenreduced titanium dioxide(rutile),J.Phys.Chem.Solids45(1984) 1265.O.K.Varghese et al./Sensors and Actuators B93(2003)338–344343[29]G.C.Mather, F.M.B.Marques,J.R.Frade,Detection mecha-nism of TiO2ceramic H2sensors,J.Eur.Ceram.Soc.19(1999) 887.[30]R.M.Walton,D.J.Dwyer,J.W.Schwank,J.L.Gland,Gas sensingbased on surface oxidation/reduction of platinum–titania thin films.Part2.The role of chemisorbed oxygen in film sensitisation,Appl.Surf.Sci.125(1998)199.[31]M.J.Madou,S.R.Morrison,Chemical Sensing with Solid StateDevices,Academic Press,New York,1989.[32]G.B.Raupp,J.A.Dumesic,Adsorption of CO,CO2,H2and H2O ontitania surfaces with different oxidation states,J.Phys.Chem.89 (1985)5240.[33]J.B.Bates,J.C.Wang,R.A.Perkins,Mechanisms for hydrogendiffusion in TiO2,Phys.Rev.B19(1979)4130.[34]G.J.Hill,The effect of hydrogen on the electrical properties of rutile,Br.J.Appl.Phys.1(1968)1151.[35]U.Roland,T.Braunschweig,F.Roessner,On the nature of split-overhydrogen,J.Mol.Catal.A:Chem.127(1997)61.344O.K.Varghese et al./Sensors and Actuators B93(2003)338–344。