High Thermoelectric Figure of Merit and Nanostructuring in Bulk p-type Ge-x(SnyPb1-y)(1-x)Te Alloys
结构功能一体化热电材料

结构功能一体化热电材料英文回答:Structural-functional integrated thermoelectricmaterials are a type of material that can convert wasteheat into electricity. These materials have gainedsignificant attention in recent years due to theirpotential applications in various fields such as energy harvesting, waste heat recovery, and solid-state cooling. The integration of structural and functional properties in these materials allows for enhanced thermoelectric performance.One example of a structural-functional integrated thermoelectric material is a composite material consistingof a semiconductor matrix embedded with nanostructured inclusions. The semiconductor matrix provides theelectrical conductivity necessary for the flow of electrons, while the nanostructured inclusions scatter phonons, which are responsible for heat transfer. This scattering effectreduces the thermal conductivity of the material, leadingto an increased thermoelectric efficiency.Another example is the use of layered materials with a high thermoelectric figure of merit (ZT). These materials have a unique crystal structure that allows for efficient charge transport while maintaining low thermal conductivity. The layered structure provides a pathway for electrons to move through the material, while the weak interlayerbonding hinders phonon transport. This combination of properties results in a high ZT value, indicating a high thermoelectric performance.Structural-functional integrated thermoelectricmaterials can also be designed by controlling the composition and microstructure of the material. For instance, by introducing dopants or defects into the material, the electrical conductivity can be enhanced,while the thermal conductivity can be reduced. This control over the material's properties allows for the optimizationof thermoelectric performance.In addition to their thermoelectric properties,structural-functional integrated thermoelectric materials can also possess other desirable characteristics. For example, some materials exhibit mechanical flexibility, making them suitable for applications in wearable devicesor flexible electronics. Others may have good chemical stability, enabling their use in harsh environments. The integration of these additional functionalities further expands the potential applications of these materials.In conclusion, structural-functional integrated thermoelectric materials are a promising class of materials that can efficiently convert waste heat into electricity.By integrating structural and functional properties, these materials exhibit enhanced thermoelectric performance. The design and control of composition, microstructure, and additional functionalities contribute to their potential applications in various fields.中文回答:结构功能一体化热电材料是一种能够将废热转化为电能的材料。
fundamentals of thermoelectricity oxford 2015
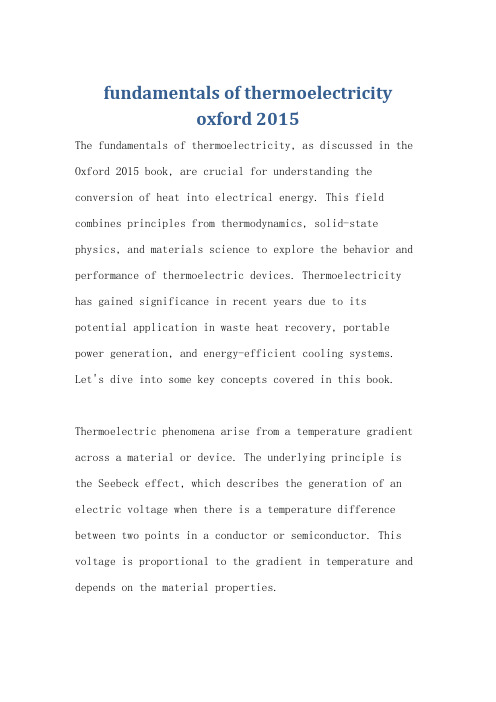
fundamentals of thermoelectricityoxford 2015The fundamentals of thermoelectricity, as discussed in the Oxford 2015 book, are crucial for understanding the conversion of heat into electrical energy. This field combines principles from thermodynamics, solid-state physics, and materials science to explore the behavior and performance of thermoelectric devices. Thermoelectricity has gained significance in recent years due to its potential application in waste heat recovery, portable power generation, and energy-efficient cooling systems. Let's dive into some key concepts covered in this book.Thermoelectric phenomena arise from a temperature gradient across a material or device. The underlying principle is the Seebeck effect, which describes the generation of an electric voltage when there is a temperature difference between two points in a conductor or semiconductor. This voltage is proportional to the gradient in temperature and depends on the material properties.热电现象是在材料或器件中存在温度梯度时产生的。
硒化锡合成反应方程式

硒化锡合成反应方程式1. 引言硒化锡(SnSe)是一种重要的半导体材料,具有优异的热电性能和光学性能。
它在太阳能电池、热电材料、光电器件等领域具有广泛的应用前景。
硒化锡的合成方法有多种,其中最常用的是化学气相沉积(CVD)和溶剂热法。
本文将重点介绍硒化锡的溶剂热法合成反应方程式。
2. 实验原理溶剂热法合成硒化锡主要是利用一种含有硒源和金属锡源的溶剂,在高温下进行反应生成硒化锡。
常用的硒源有硒粉、硒酸等,金属锡源可以是金属锡粉、氧化锡等。
在反应过程中,首先将硒源和金属锡源加入适当的溶剂中,并进行搅拌混合,形成均匀的混合物。
然后将混合物转移到高温容器中,在一定温度下进行反应一段时间。
最后,通过冷却和过滤等步骤,得到硒化锡产物。
3. 实验步骤1.准备硒源、金属锡源和溶剂。
硒源可以选择硒粉,金属锡源可以选择金属锡粉,溶剂可以选择有机溶剂如乙二醇。
2.将硒粉和金属锡粉按一定比例加入乙二醇中,并进行搅拌混合,使其均匀分散。
3.将混合物转移到高温容器中,并加热至一定温度(通常为200-300摄氏度)。
4.在一定时间内保持恒定温度下反应,搅拌反应体系以促进反应进行。
5.反应结束后,将反应体系冷却至室温。
6.用滤纸或其他适当的方法过滤产物,将硒化锡分离出来。
7.对得到的硒化锡进行干燥处理,得到最终产物。
4. 反应方程式根据实验原理和步骤,可以写出硒化锡的合成反应方程式:Sn + Se → SnSe其中,Sn表示金属锡,Se表示硒。
5. 实验条件合成硒化锡的实验条件包括温度、时间和溶剂选择等。
通常情况下,温度在200-300摄氏度之间,反应时间可以根据需要进行调整。
乙二醇是常用的溶剂选择,也可以根据实际情况选择其他有机溶剂。
6. 结果与讨论通过溶剂热法合成的硒化锡具有良好的结晶性和纯度,可以用于进一步的物理性质测试和材料应用研究。
此外,通过调节反应条件和原料比例等因素,还可以得到不同形貌和尺寸的硒化锡纳米材料。
7. 结论本文介绍了硒化锡的溶剂热法合成反应方程式及实验步骤。
2019年武汉理工大学理学院博士研究生招生入围考试考生信息公示汇总表
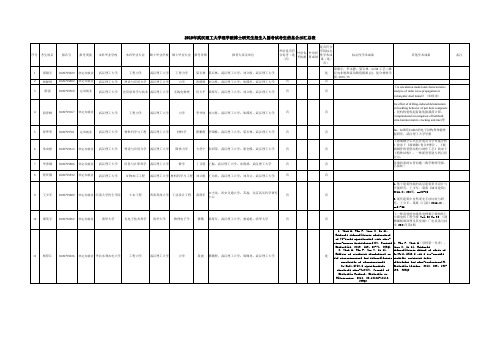
《一种高效的非线性电网数字锁相环》
否
中国电机工程学报 Vol.32 No.33 《调 频激励器原理及其发展》广电设备与技
术 2004年第4期
1. Yang H, Wen P, Zhou X, et al.
Enhanced thermoelectric performance
of Te-doped skutterudite with nano-
/否)
外语免 考依据
外语折 算成绩
学院标志 性学术成 果(是/
否)
标志性学术成果
其他学术成果
谢翔宇,李永静,晏石林.LCM 工艺二维
是 径向非饱和流动数值模拟 [J].复合材料学
报, 2018, 35.
否
否
否
否
《A calculation model and characteristics analysis of radio wave propagation in rectangular shed tunnel》(EI收录)
5 舒翠翠 1049799701 定向就业 武汉理工大学 材料科学与工程 武汉理工大学
材料学
翟鹏程 李国栋,武汉理工大学;晏石林,武汉理工大学
否
6 朱央炫 1049799612 非定向就业 武汉理工大学 理论与应用力学 武汉理工大学 固体力学
方岱宁 朱四荣,武汉理工大学;谢官模,武汉理工大学
否
7 华香颖 1049799806 非定向就业 武汉理工大学 信息与计算科学 武汉理工大学
是
Effects of sintering temperature on Co4Sb11.5Te0.5 via a two-pronged the microstructure and thermoelectric strategy combining grain
【国家自然科学基金】_热电性质_基金支持热词逐年推荐_【万方软件创新助手】_20140802
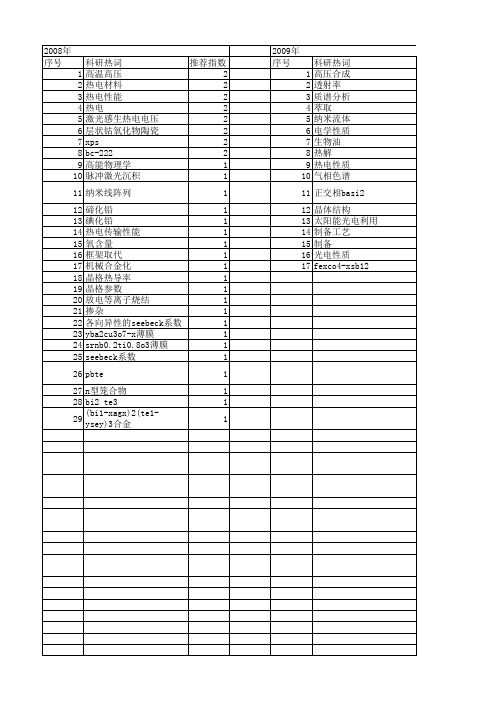
2014年 序号 1 2 3 4 5 6 7 8
2014年 科研热词 热电材料 纳米复合材料 硫属化合物 热电性能 层状材料 导电聚苯胺 原子薄膜 协同调控 推荐指数 2 1 1 1 1 1 1 1
2013年 序号 1 2 3 4 5 6 7 8 9 10 11 12 13 14 15 16 17 18
科研热词 推荐指数 电输运 2 热电性能 2 高温氧化物 1 电磁学 1 热电材料 1 热电势测量 1 氧化物 1 教学改革 1 拓扑绝缘体 1 建设措施 1 低温 1 介观体系 1 viii型笼合物 1 n型传导 1 bi2srca2co2oy晶体 1 bi2se3 1
2012年 序号 1 2 3 4 5 6 7 8 9 10 11 12 13 14 15 16 17 18 19 20 21 22 23 24 25 26 27 28 29 30 31 32 33 34 35 36 37 38 39
科研热词 推荐指数 热电材料 3 高压制备 1 高压 1 铁原子链 1 退火处理 1 赛贝克系数 1 自加热效应 1 红外探测器 1 稀土硫化物 1 石墨条带 1 电流崩塌 1 电导率 1 玻尔兹曼输运 1 热电输运 1 热电性质 1 热电子效应 1 热电优值 1 热电 1 热导率 1 形变势 1 弛豫时间 1 应用 1 太阳能电池 1 固相反应 1 吸附 1 双异质结高电子迁移率晶体管 1 压力 1 制备方法 1 制备技术 1 β -fesi2 1 x射线衍射 1 thermoelectric figure of merit1 seebeck系数 1 relaxation time 1 deformational potential theory1 cosb3 1 ca3co4o9 1 boltzmann transport 1 bi0.5sb1.5te3薄膜 1
热电经济指标释义与计算
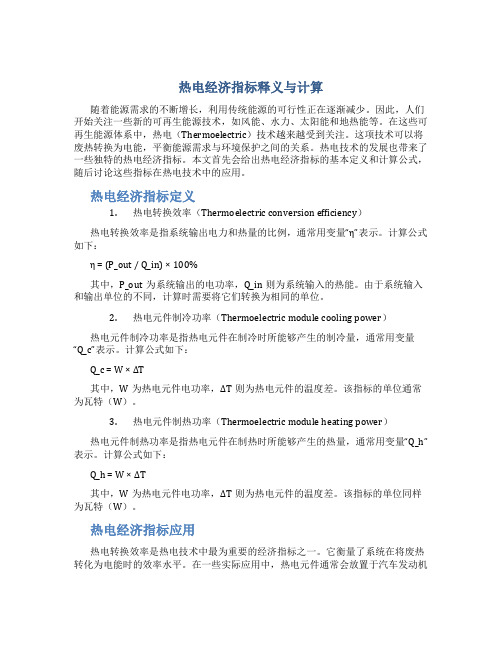
热电经济指标释义与计算随着能源需求的不断增长,利用传统能源的可行性正在逐渐减少。
因此,人们开始关注一些新的可再生能源技术,如风能、水力、太阳能和地热能等。
在这些可再生能源体系中,热电(Thermoelectric)技术越来越受到关注。
这项技术可以将废热转换为电能,平衡能源需求与环境保护之间的关系。
热电技术的发展也带来了一些独特的热电经济指标。
本文首先会给出热电经济指标的基本定义和计算公式,随后讨论这些指标在热电技术中的应用。
热电经济指标定义1.热电转换效率(Thermoelectric conversion efficiency)热电转换效率是指系统输出电力和热量的比例,通常用变量“η”表示。
计算公式如下:η = (P_out / Q_in) × 100%其中,P_out为系统输出的电功率,Q_in则为系统输入的热能。
由于系统输入和输出单位的不同,计算时需要将它们转换为相同的单位。
2.热电元件制冷功率(Thermoelectric module cooling power)热电元件制冷功率是指热电元件在制冷时所能够产生的制冷量,通常用变量“Q_c”表示。
计算公式如下:Q_c = W × ΔT其中,W为热电元件电功率,ΔT则为热电元件的温度差。
该指标的单位通常为瓦特(W)。
3.热电元件制热功率(Thermoelectric module heating power)热电元件制热功率是指热电元件在制热时所能够产生的热量,通常用变量“Q_h”表示。
计算公式如下:Q_h = W × ΔT其中,W为热电元件电功率,ΔT则为热电元件的温度差。
该指标的单位同样为瓦特(W)。
热电经济指标应用热电转换效率是热电技术中最为重要的经济指标之一。
它衡量了系统在将废热转化为电能时的效率水平。
在一些实际应用中,热电元件通常会放置于汽车发动机或煤炭电力站等需要大量能源的系统中。
通过将废热转化为电能,电力系统的效率可以得到显著的提升。
热电材料的研究现状与未来展望
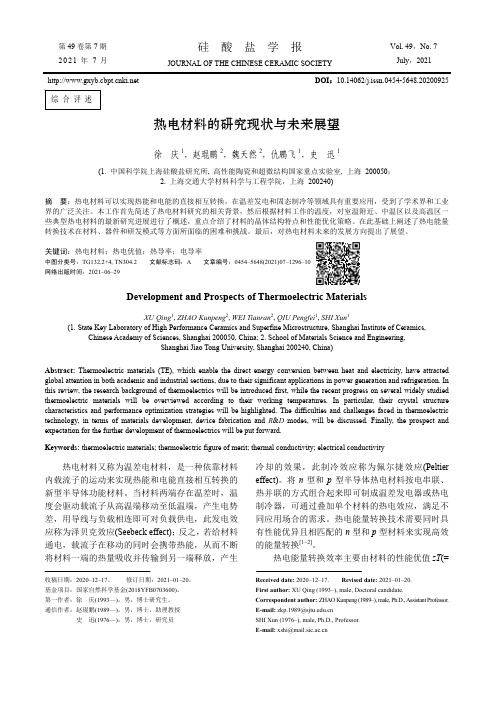
第49卷第7期 2021年7月硅 酸 盐 学 报Vol. 49,No. 7 July ,2021JOURNAL OF THE CHINESE CERAMIC SOCIETY DOI :10.14062/j.issn.0454-5648.20200925热电材料的研究现状与未来展望徐 庆1,赵琨鹏2,魏天然2,仇鹏飞1,史 迅1(1. 中国科学院上海硅酸盐研究所, 高性能陶瓷和超微结构国家重点实验室, 上海 200050;2. 上海交通大学材料科学与工程学院,上海 200240)摘 要:热电材料可以实现热能和电能的直接相互转换,在温差发电和固态制冷等领域具有重要应用,受到了学术界和工业界的广泛关注。
本工作首先简述了热电材料研究的相关背景,然后根据材料工作的温度,对室温附近、中温区以及高温区一些典型热电材料的最新研究进展进行了概述,重点介绍了材料的晶体结构特点和性能优化策略。
在此基础上阐述了热电能量转换技术在材料、器件和研发模式等方面所面临的困难和挑战。
最后,对热电材料未来的发展方向提出了展望。
关键词:热电材料;热电优值;热导率;电导率中图分类号:TG132.2+4, TN304.2 文献标志码:A 文章编号:0454–5648(2021)07–1296–10 网络出版时间:2021–06–29Development and Prospects of Thermoelectric MaterialsXU Qing 1, ZHAO Kunpeng 2, WEI Tianran 2, QIU Pengfei 1, SHI Xun 1(1. State Key Laboratory of High Performance Ceramics and Superfine Microstructure, Shanghai Institute of Ceramics,Chinese Academy of Sciences, Shanghai 200050, China; 2. School of Materials Science and Engineering,Shanghai Jiao Tong University, Shanghai 200240, China)Abstract: Thermoelectric materials (TE), which enable the direct energy conversion between heat and electricity, have attracted global attention in both academic and industrial sections, due to their significant applications in power generation and refrigeration. In this review, the research background of thermoelectrics will be introduced first, while the recent progress on several widely studied thermoelectric materials will be overviewed according to their working temperatures. In particular, their crystal structure characteristics and performance optimization strategies will be highlighted. The difficulties and challenges faced in thermoelectric technology, in terms of materials development, device fabrication and R &D modes, will be discussed. Finally, the prospect and expectation for the further development of thermoelectrics will be put forward.Keywords: thermoelectric materials; thermoelectric figure of merit; thermal conductivity; electrical conductivity热电材料又称为温差电材料,是一种依靠材料内载流子的运动来实现热能和电能直接相互转换的新型半导体功能材料。
氧化物热电材料研究进展

氧化物热电材料研究进展徐飞;李安敏;程晓鹏;孔德明【摘要】由于能源危机正在到来,废热回收已经成为解决能源短缺问题的有效途径之一,热电材料在废热收集环节中占有举足轻重的地位.其中,氧化物热电材料拥有抗氧化能力强、热稳定性好、原料相对低廉、制备工艺相对简单、无毒、无污染、使用寿命长等传统合金材料不具备的优点,但由于低的电导率因而限制了其在热电性能方面的表现.已经有大量研究发现,可以通过元素掺杂,改善氧化物热电材料的热电性能,氧化物热电材料再次受到广大研究者的关注.综述了氧化物热电材料的研究进展与今后的发展方向,着重阐述了以BiCuSeO为代表的氧化物热电材料的基本结构、性能特征与研究进展;评述了BiCuSeO材料Bi位、Cu位和O位掺杂研究以及BiCuSeO的结构优化;并简单介绍了NaCo2 O 4、Ca3 Co4 O 9、SrTiO 3、ZnO、In2 O 3热电材料的研究情况.【期刊名称】《功能材料》【年(卷),期】2019(050)004【总页数】11页(P4038-4048)【关键词】废热回收;热电材料;氧化物热电材料;BiCuSeO;元素掺杂【作者】徐飞;李安敏;程晓鹏;孔德明【作者单位】广西大学资源环境与材料学院,广西有色金属及特色材料加工重点实验室,南宁 530004;广西大学资源环境与材料学院,广西有色金属及特色材料加工重点实验室,南宁 530004;广西大学资源环境与材料学院,广西有色金属及特色材料加工重点实验室,南宁 530004;广西大学资源环境与材料学院,广西有色金属及特色材料加工重点实验室,南宁 530004【正文语种】中文【中图分类】TB340 引言对于热电材料研究,早在1822年,塞贝克(Seebeck)就在《普鲁士科学院报》中描述了一个这样的现象,在相互连接的不同导体中, 由于温度差就会出现自由磁子。
将两种不同金属材料连接,将连线一端处于较高温度下,温度为T1(热端),而另一端处于开路且较低温度下,温度为T2(冷端),这时冷端存在一个开路电压ΔV,这个现象被称为Seebeck效应,ΔV被称为Seebeck电压,ΔV与热冷两端的温差ΔT成正比,即ΔV=SΔT=S(T1-T2)(1)其中,S为Seebeck系数,只与材料自身的电子能带结构相关。
- 1、下载文档前请自行甄别文档内容的完整性,平台不提供额外的编辑、内容补充、找答案等附加服务。
- 2、"仅部分预览"的文档,不可在线预览部分如存在完整性等问题,可反馈申请退款(可完整预览的文档不适用该条件!)。
- 3、如文档侵犯您的权益,请联系客服反馈,我们会尽快为您处理(人工客服工作时间:9:00-18:30)。
1054Chem.Mater.2010,22,1054–1058DOI:10.1021/cm902009tHigh Thermoelectric Figure of Merit and Nanostructuring in Bulk p-type Ge x(Sn y Pb1-y)1-x Te Alloys Following a SpinodalDecomposition Reaction†Yaniv Gelbstein,*Boaz Dado,Ohad Ben-Yehuda,Yatir Sadia,Zinovy Dashevsky,andMoshe P.DarielDepartment of Materials Engineering,Ben-Gurion University of the Negev,Beer-Sheva84105,IsraelReceived July6,2009.Revised Manuscript Received August6,2009The use of thermoelectricity for direct energy conversion from thermal to electrical energy has a long-standing history.We report that the complex p-type Ge(Sn,Pb,Te)Te alloys upon suitable alloying with SnTe can be designed to obtain very high ZT values of up to1.2because of both optimal electronic transport properties and minimal lattice thermal conductivity.Such alloys follow a spinodal decomposition reaction,leading to an ordered periodic nano-structure that is effective in the scattering of phonons and reduction of the lattice thermal conductivity.IntroductionThermoelectricity is concerned with the interactionbetween thermal and electrical phenomena.The mostcommon applications are concerned with the conver-sion of thermal energy(or heat)into electrical powerand with the use of electrical current for cooling.Thedimensionless thermoelectric figure of merit(ZT),ex-pressed in eq1,is widely used as a measure of thethermoelectric efficiency with respect to the material’sproperties.ZT¼R2σK Tð1Þwhere Z is the thermoelectric figure of merit,R theSeebeck coefficient,σthe electrical conductivity,κthethermal conductivity,and T the absolute temperature.Materials with high ZT values are known as thermo-electric materials.Today’s commercial materials have aZT=1,leading to conversion efficiency on the order ofseveral percent(∼6%).Increasing ZT can be achievedby either increasing the numerator of ZT,P=R2σ, known as the power factor,or decreasing the thermalconductivity,κ(the denominator of ZT in eq1).κis asum of two major contributions,the lattice thermalconductivityκL(due to phonons flow)and the electronicthermal conductivityκe(due to electronic flow),as de-scribed in eq2.K¼K LþK eð2ÞCurrently,PbTe-based thermocouples,with an n-type leg (doped with PbI2for achieving maximal ZT values of about 1.1in the temperature range of400-600°C for carrier concentrations of2-6Â1019cm-3,respectively)and a p-type leg(alloyed with SnTe)have been employed in real applications.1,2The latter,in the form of Pb1-x Sn x-Te,unfortunately display much lower ZT values(e0.5) than those for the n-type PbTe component.Higher ZT values(∼0.8at450°C)for p-type legs were found in Na-doped PbTe;3however,because of the brittle nature of this material and the correspondent poor mechanical pro-perties,its usage in practical applications was eliminated.3,4 Therefore,further improvement of the ZT values of the p-type legs is a significant challenge that carries substantial benefit.Recently,optimally doped pseudobinary Pb1-x Ge x Te compounds were proposed as potential candidates to im-prove the thermoelectric performance of p-type legs.5,6 However,the ZT values of these pseudobinary alloys can be further increased by decreasing the lattice thermal con-ductivity due to the presence of additional structural defects. Recently,very low thermal conductivity values were ob-served in several nanostructured PbTe-based materials.7-12 One mechanism for nanopattern generation is based on the†Accepted as part of the2010“Materials Chemistry of Energy Conversion Special Issue”.*Corresponding author.E-mail:yanivge@bgu.ac.il.(1)Gelbstein,Y.;Dashevsky,Z.;Dariel,M.P.Physica B2005,363,196–205.(2)Gelbstein,Y.;Dashevsky,Z.;Dariel,M.P.Physica B2007,391,256–265.(3)Gelbstein,Y.;Gotesman,G.;Lishzinker,Y.;Dashevsky,Z.;Dariel,M.P.Scr.Mater.2008,58,251–254.(4)Gelbstein,Y.;Dashevsky,Z.;Dariel,M.P.J.Appl.Phys.2008,104,033702.(5)Gelbstein,Yaniv;Dashevsky,Zinovi;Dariel,Moshe P.Phys.Status Solidi2007,1(No.6),232–234.(6)Gelbstein,Y.;Ben-Yehuda,O.;Pinhas,E.;Edrei,T.;Sadia,Y.;Dashevsky,Z.;Dariel,M.P.J.Electron.Mater.2009,38(7),1478.(7)Poudeu,P.F.P.;D’Angelo,J.;Downey,A.D.;Short,J.L.;Hogan,T.P.;Kanatzidis,M.G.Angew.Chem.,Int.Ed.2006,45,1–5. (8)Sootsman,J.R.;Pcionek,R.J.;Kong,H.;Uher,C.;Kanatzidis,M.G.Chem.Mater.2006,18,4993–4995./cm Published on Web08/21/2009r2009American Chemical SocietyArticle Chem.Mater.,Vol.22,No.3,20101055spinodal decomposition.Spinodal decomposition is a me-chanism for phase separation that leads to a characteristicmodulated structure that can be exploited to control themicrostructure at the nanometer scale.13Nanostructures for spinodal decomposed systems werereported for TiO2-SnO2,13Mn-Cu,14and Cu-Ni-Sn15 alloys.Recently,a reduction of the lattice thermal con-ductivity and a corresponding ZT enhancement inPb1-x Sn x Te-PbS system was attributed to nano structuresresulting from the spinodal decomposition.12Alloying of(GeTe)x(PbTe)1-x compounds with SnTecan result in a spinodal decomposition with changedperiodicity that depends on the heat treatment.16To thebest of our knowledge,the nanostructure and the transportproperties of these alloys have not yet been investigated.The present communication is concerned with develop-ment of highly efficient nanostructured p-type Ge x-(Sn y Pb1-y)1-x Te alloys for thermoelectric applications,using spark plasma sintering(SPS).The thermoelectricfigure of merit was optimized by means of both alloyingand doping methods.Two alloys in this family,Ge0.5Sn0.25Pb0.25Te(undoped,Ag-doped and Bi2Te3-doped)and Ge0.6Sn0.1Pb0.3Te,were prepared and inves-tigated.The results were compared to a GeTe referencesample.Experimental DetailsSynthesis.GeTe,Ge0.5Sn0.25Pb0.25Te(undoped,3mol%Ag-doped,and3mol%Bi2Te3-doped),and Ge0.6Sn0.1Pb0.3Te alloyswere prepared according to the following procedure:(a)sealingthe source materials(purity of5N)at appropriate concentrationsin a quartz ampule under a vacuum of1Â10-5Torr,(b)meltingthe alloys in a rocking furnace at800°C for1h followed by waterquenching,(c)milling the compound to a maximal particle size of60mesh powder,and(d)Spark Plasma Sintering(SPS)(type HPD5/1FCT Systeme GmbH)at450°C for30min under amechanical pressure of32MPa for GeTe and at550°C for60min under a mechanical pressure of25MPa for the Ge x(Sn y-Pb1-y)1-x Te alloys.The different SPS parameters were optimizedin order to obtain dense samples(>96%)without any lateralcracks for the various investigated alloys.Electrical Properties.Seebeck coefficient and the electricalconductivity measurements were performed in a self-con-structed apparatus under an argon atmosphere up to∼450°Cat a heating rate of3°C/min.For Seebeck coefficient measure-ments,an auxiliary heater was used to maintain a temperature difference of10°C between the extremities of the samples. Electrical conductivity was measured by the“four-probe”method using an alternating power source of1V/50Hz.Thermal Conductivity.The thermal conductivity(κ)was determined as a function of temperature from room tempera-ture to500°C using the flash diffusivity method(LFA457, Netzsch).The front face of a disk-shaped sample(L=12mm, thickness≈1-2mm)is irradiated by a short laser burst and the resulting rear face temperature is recorded and analyzed.Ther-mal conductivity values were calculated using the equation κ=R3C P3F,where R is the thermal diffusivity,C P is the specific heat(measured using differential scanning calorimetry,STA 449,Netzsch),and F is the bulk density of the sample(calculated from the sample’s geometry and mass).Microscopy.The microstructure of Ge0.5Sn0.25Pb0.25Te (undoped and3mol%Bi2Te3-doped)was investigated by optical microscopy(Zeiss Axiovert25.Etching prior to optical microscopy by immersion for few seconds in a60mL solution composed of10mL of alcohol,10g of potassium hydroxide, 17.5mL of glycerol,and22.5mL of distilled water),high-resolution scanning electron microscopy(Jeol-7400F HRSEM), transmission electron microscopy(TEM,FEI TECNAI,G2), and high-resolution transmission electron microscopy(Jeol-2010HRTEM).Specimens used for the TEM and HRTEM were prepared as follows.Small square shape pieces with approximate sizes of 5mmÂ5mmÂ2mm were first cut from the spark plasma sintered disk using a low speed diamond wheel saw.The samples were then mounted inside a copper tube(L=3mm)and hand-polished with subsequently increasing grit(1000-1500)sand paper to about200-300μm in thickness.Samples were then thinned using a Gatan precision dimple grinder and ion milled to electron transparency using a Gatan precision ion polishing system(PIPS).Results and DiscussionAll the investigated Ge x(Sn y Pb1-y)1-x Te alloys dis-played a microscale quasi-ordered periodic fishbone structure,the orientation of which depended on the original high temperature cubic phase grain in which the demixing process took place.A typical structure of these alloys as observed in Ge0.5Sn0.25Pb0.25Te is shown in Figure1.From the optical microscope(a)and HRSEM (b)micrographs of this figure,the wavelength of the dissociation as determined from the side branches of the fishbone was about10μm.High-magnification TEM micrograph(c)revealed a finer periodic nanostructure with a wavelength of about100nm.In addition,electron-dispersive spectroscopy(EDS)analysis showed that the fishbone structure is Pb-rich,whereas the matrix is Ge-rich.The bright aspect of the Pb-rich regions(Pb is heavier than Ge)is obtained in the backscattered electron (BSE)examination mode of the HRSEM(b).Line scan EDS analysis crossing the fishbone patterns and the surrounding matrix revealed continuous and complemen-tary compositional modulations of Pb and Ge when scanning across the side-branches of the fishbone pattern (Figure1).It is noteworthy that the Sn composition remained constant while crossing the Pb-rich fishbone lamellas through the surrounding Ge-rich matrix.Higher magnification of HRTEM revealed that the Pb-rich(9)Poudeu,P.F.P.;D’Angelo,J.;Kong,H.;Downey,A.D.;Short,J.L.;Pcionek,R.;Hogan,T.P.;Uher,C.;Kanatzidis,M.G.J.Am.Chem.Soc.2006,126,14347–14355.(10)Androulakis,J.;Hsu,K.F.;Pcionek,R.J.;Kong,H.;Uher,C.;D’Angelo,J.;Downey,A.D.;Hogan,T.P.;Kanatzidis,M.G.Adv.Mater.2006,18,1170–1173.(11)Hsu,K.F.;Loo,S.;Guo,F.;Chen,W.;Dyck,J.S.;Uher,C.;Hogan,Tim;Polychroniadis,E.K.;Kanatzidis,M.G.Science 2004,303,818–820.(12)Androulakis,J.;Lin,C.-H.;Kong,H.;Uher,C.;Wu,C.-I.;Hogan,T.;Cook,B.A.;Caillat,T.;Paraskevopoulos,K.M.;Kanatzidis, M.G.J.Am.Chem.Soc.2007,129,9780–9788.(13)Chaisan,W.;Yimnirun,R.;Ananta,S.;Cann,D.P.J.Solid StateChem.2005,178,613–620.(14)Yin,F.;Ohsawa,Y.;Sato,A.;Kawahara,K.Acta Mater.2000,48,1273–1282.(15)Zhao,J.-C.;Notis,M.R.Acta Mater.1998,46,4203–4218.(16)Yashina,L.V.;Leute,V.;Shtanov,V.I.;Schmidtke,H.M.;Neudachina,V.S.J.Alloys Compd.2006,413,133–143.1056Chem.Mater.,Vol.22,No.3,2010Gelbstein et al. fishbone structure by itself composed from smaller scalemodulations in the10nm range(Figure2).These con-tinuous modulations without any sharp borders,as inprecipitates,are possibly consistent with the continuousnature of the spinodal decomposition generated struc-ture.The electrons diffraction patterns in this figureshow a doublet pattern(two nonconcentric periodicreflections),indicating the presence of two similar struc-tures with closely related lattice parameters providingadditional evidence to the decomposition of Ge x(Sn y-Pb1-y)1-x Te into Ge and Pb rich phases.In addition to the micro and nanoperiodic Pb-richmodulations,the3mol%Bi2Te3doped Ge0.5Sn0.25-Pb0.25Te sample showed Birich nano precipitates with atypical size of50nm(Figure3),which were not observedat any of the other investigated compositions.The effectof these precipitates will be discussed later.The temperature dependence of the Seebeck coeffi-cient,electrical conductivity and thermal conductivity of Ge0.5Sn0.25Pb0.25Te(undoped,3mol%Ag-doped, and3mol%Bi2Te3-doped)and Ge0.6Sn0.1Pb0.3Te alloys compared to GeTe are presented in Figures4-6.We discuss first the doping influence of Ge0.5Sn0.25-Pb0.25Te by Ag and Bi2Te3on the thermoelectric proper-ties.By comparing curves2,3,and5for Ag doping, undoped,and Bi2Te3doping,respectively,in Figures4-6,a consistent trend of decreasing Seebeck coefficient and increasing electrical and thermal conductivity values forFigure1.(a)Optical microscopy,(b)backscattered electrons HRSEM, and(c)TEM micrographs of Ge0.5Sn0.25Pb0.25Te.Figure2.HRTEM micrograph and typical electron diffraction patterns of Ge0.5Sn0.25Pb0.25Te.Figure3.HRSEM of scattered Bi-rich nanoprecipitates in3mol% Bi2Te3-doped Ge0.5Sn0.25Pb0.25Te.Figure4.Temperature dependence of the Seebeck coefficient for(1) GeTe,(2)Ge0.5Sn0.25Pb0.25Teþ3mol%Ag,(3)Ge0.5Sn0.25Pb0.25Te, (4)Ge0.6Sn0.1Pb0.3Te,and(5)Ge0.5Sn0.25Pb0.25Teþ3mol%Bi2Te3. Figure5.Temperature dependence of the electrical conductivity for the various prepared alloys.Notations are according to Figure4.Figure6.Temperature dependence of the thermal conductivity for the various prepared alloys.Notations are according to Figure4.Article Chem.Mater.,Vol.22,No.3,20101057Ag doping,as compared to the undoped condition,is apparent.This evidence indicates an Ag-acceptor behavior in the investigated alloys,leading to an increased carrier concentration.For Bi 2Te 3doping (curve 5in Figures 4-6),the opposite trend is observed,showing a donor behavior in the investigated alloys and leading to a decreased carrier concentration as compared to the undoped sample (curve 3).These trends show the potential of Bi and Ag to serve as active impurities (donors and acceptors,respectively)in Ge 0.5Sn 0.25Pb 0.25Te for tuning the optimal carrier concen-tration for thermoelectric applications.By comparing of these curves to the GeTe curve (curve 1in Figures 4-6),we observe that all the Ge 0.5Sn 0.25Pb 0.25Te curves (2,3,and 5)yield higher R and lower σand κvalues,as compared to the GeTe (curve 1),indicating a reduced carrier concentration at all the doping levels in Ge 0.5Sn 0.25Pb 0.25Te,as compared to pure GeTe.Pure GeTe shows a very high holes concen-tration of p ≈6Â1026m -3.It is well-known that for thermoelectric applications,much lower carrier concentra-tions are needed for optimal performance.This stands behind Bi doping of GeTe (p ≈2Â1026m -3),for increasing the thermoelectric performance.6Therefore,the reduced carrier concentrations obtained by using Ge 0.5Sn 0.25-Pb 0.25Te (in all of the investigated doping levels,curves 2,3,and 5)as compared to GeTe (curve 1),is desirable.Variation of the alloying of Ge x (Sn y Pb 1-y )1-x Te from undoped Ge 0.5Sn 0.25Pb 0.25Te (curve 3)to undoped Ge 0.6Sn 0.1Pb 0.3Te (curve 4)does not result in any dramatic changes of the investigated transport properties.This beha-vior can be attributed to the carrier compensation obtained by increasing the GeTe amount (from 50to 60at %)and decreasing the SnTe amount (from 25to 10at %),both p -type compounds with very high hole concentrations.The temperature dependence of the lattice thermal con-ductivity,κL ,for GeTe,Ge 0.5Sn 0.25Pb 0.25Te (undoped and 3%Bi 2Te 3doped),and Ge 0.6Sn 0.1Pb 0.3Te is presented in Figure 7.κL values were calculated as followed:1.Fermi Energy Calculation.Fermi energy (η)was calculated at low temperatures (<250°C),for assuring an extrinsic region,using eq 3and the measured Seebeck coefficient values (Figure 4)R ¼k e 52þr ÀÁF 3=2þr 32þr F1=2þr -η264375ð3ÞWhere,e ,k ,F r ,η,and r are the electron charge,Boltz-mann constant,Fermi integral,reduced Fermi energy(equal to [E F -E V ]/kT for p -type materials),and the scattering mechanism parameter (equal to -0.5for the case of scattering by acoustic phonons as the dominant scattering mechanism,as is the case of GeTe).Equation 3is valid for a quadratic dependence of the carrier energy on the crystal momentum and a power dependence of the mean free time between electron colli-sions,τon the kinetic energy ξ(τ=τo ξr ,where τo is the constant),as for the case of GeTe-based alloys.The heavy hole valence band,present in GeTe,was not taken into account,on account of its minor influence at low temperatures up to very high carrier concentrations of ∼7Â1026m -3.62.Electronic Thermal Conductivity Calculation.The electronic contribution to the thermal conductivity,κe ,was calculated using the Wiedemann -Franz relation (eq 4)and the measured electrical conductivity values (Figure 4).K e ¼L σTð4ÞWhere,L and T are Lorenz number and absolute tem-perature,respectively.Lorenz number,given by eq 5,was calculated using the Fermi energy (η)values obtained in section 1.L ¼k e2r þ72ÀÁr þ32F r þ5=2ðηÞF r þ1=2ðηÞ-r þ52ÀÁ2F 2r þ3=2ðηÞr þ32 F 2r þ1=2ðηÞ264375ð5Þttice Thermal Conductivity Calculation.Finally,the lattice contribution to thermal conductivity,κL ,was calculated by subtraction of k e from the meas-ured total thermal conductivity,κ,(Figure 6),accord-ing to eq 2.It is clear from the calculated lattice thermal conduc-tivity values of Figure 7that alloying of GeTe with both PbTe and SnTe to obtain nanostructured Ge 0.5Sn 0.25-Pb 0.25Te or Ge 0.6Sn 0.1Pb 0.3Te,which follow a spinodal decomposition reaction,results in a major reduction (∼50%)of the lattice thermal conductivity.The room temperature κL values for Ge 0.5Sn 0.25Pb 0.25Te or Ge 0.6Sn 0.1Pb 0.3Te are about 0.8W/mK,as compared to a value of ∼1.6W/mK that was found for pure GeTe.This reduction can be attributed to phonon scattering by the nano-size ordered modulations of the spinodal de-composition in Ge x (Sn y Pb 1-y )1-x Te.It can be also seen from Figure 6that Bi 2Te 3doping of Ge 0.5Sn 0.25Pb 0.25Te results in a further reduction of the lattice thermal con-ductivity (to a room temperature value of ∼0.55W/mK),which may be attributed to additional nano Bi precipita-tion in this alloy.The temperature dependence of the dimensionless thermoelectric figure of merit,ZT ,for the investigated alloys is presented in Figure 8.All the investigated alloysFigure 7.Temperature dependence of the lattice thermal conductivity for GeTe,Ge 0.5Sn 0.25Pb 0.25Te (undoped and 3%Bi 2Te 3-doped),and Ge 0.6-Sn 0.1Pb 0.3Te.1058Chem.Mater.,Vol.22,No.3,2010Gelbstein et al.display higher ZT values over most of the investigated temperatures as compared to pure GeTe.The highest ZT values were obtained for undoped Ge 0.5Sn 0.25Pb 0.25Te with a maximal value of ∼1.2at 450°C,underlining the high potential of this composition as a p -type leg in practical thermoelectric applications.ConclusionsGe 0.5Sn 0.25Pb 0.25Te (undoped,3mol %Ag-doped,and 3mol %Bi 2Te 3-doped)and Ge 0.6Sn 0.1Pb 0.3Te alloys,following a spinodal decomposition reaction,were pre-pared using spark plasma sintering,characterized by electronic microscopy and transport properties measure-ments,and compared to a reference GeTe sample.The spinodal decomposition of the matrix to both GeTe-and PbTe-rich phases was observed as periodic compositional modulations both in the micro-and nano-(down to 10nm)scales.The lattice thermal conductivity was calculated by reduction of the electronic thermal conductivity (calculated by Fermi -Dirac statistics,taking into account variations of Lorenz number with Fermi energy)from the measured total thermal conductivity.Major reduction (∼50%)of the lattice thermal conductivity was observed in undoped Ge 0.5Sn 0.25Pb 0.25Te and Ge 0.6Sn 0.1Pb 0.3Te alloys.Doping optimizations of Ge 0.5Sn 0.25Pb 0.25Te by Bi 2Te 3revealed a further reduction of the lattice thermal conductivity due to nanosized scattered Bi precipitates.Bi was found to serve as a donor,whereas Ag was found to serve as an acceptor in these alloys.Very high ZT values were obtained for un-doped Ge 0.5Sn 0.25Pb 0.25Te with a maximal value of ∼1.2at 450°C,indicating the high potential of this composition to serve as a p -type legs in practical thermoelectric applica-tions.Figure 8.Temperature dependence of the dimensionless thermoelectric figure of merit for the various prepared alloys.Notations are according to Figure4.。