Applications to Human Modeling and Markerless Motion Tracking
孤独症谱系障碍儿童《国际功能、残疾和健康分类》功能变量关系图模型探析
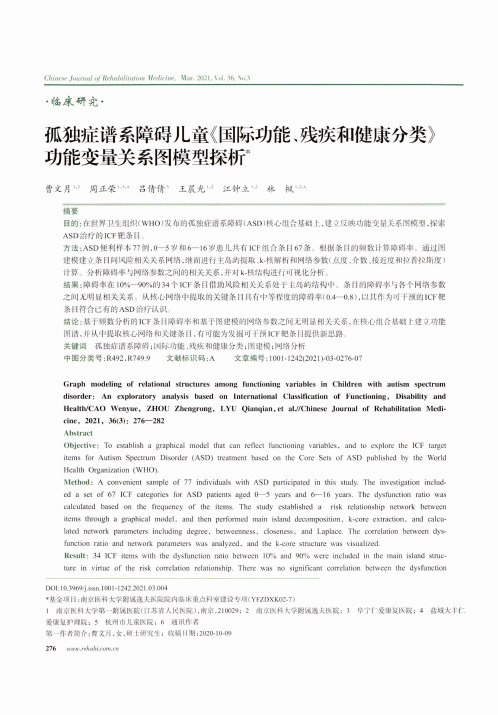
Chinese Journal of Rehdbihta!ion Medicine,Mar. 2021. \01. 36. No.3•性東研免•孤独症谱系障碍儿童《国际功能、残疾和健康分类》功能变量关系图模型探析$曹文月K2周正荣u’4吕倩倩s王晨光''2江钟立^林枫1二6摘要目的:在世界卫生组织(W H O)发布的孤独症谱系障碍(A S D)核心组合基础上,建立反映功能变量关系图模型,探索A S D治疗的I C F靶条目.方法:A S D便利样本77例,0-5岁和6—16岁患儿共有1C F组合条目67条。
根据条目的频数计算障碍率:通过图 建模建立条目间风险相关关系网络,继而进行主岛屿提取、k-核解析和网络参数(点度、介数、接近度和拉普拉斯度)计算分析障碍率与网络参数之间的相关关系.并对k-核结构进行可视化分析。
结果:障碍率在10%—90%的34个【C F条目借助风险相关关系处于主岛屿结构中。
条目的障碍率与各个网络参数之间无明显相关关系:从核心网络中提取的关键条目具有中等程度的障碍率(0.4—0.8),以其作为可干预的I C F靶 条目符合已有的A S D治疗认识。
结论:基于频数分析的I C F条目障碍率和基于图建模的网络参数之间无明显相关关系,在核心组合基础上建立功能图谱,并从中提取核心网络和关键条目,有可能为发掘可干预1C F靶条冃提供新思路关键词孤独症谱系障碍;国际功能、残疾和健康分类;图建模;网络分析中图分类号:R492.R749.9文献标识码:A文章编号:1001-1242(2021)-03-0276-07Graph modeling of relational structures among functioning variables in Children with autism spectrum disorder:An exploratory analysis based on International Classification of Functioning, Disability and Health/CAO Wenyue, ZHOU Zhengrong, LYU Qianqian,et al.//Chinese Journal of Rehabilitation Medicine, 2021, 36(3):276—282AbstractObjective:T o establish a graphical m o d e l that can reflect functioning variables,and to explore the I C F target items for A u t i s m Spectrum Disorder (A S D)treatment based on the Cor e Sets of A S D published by the W o r l d Health Organization (W H O).Method :A convenient sample of 77 individuals with A S D participated in this study. T h e investigation included a set of 67I C F categories for A S D patients aged 0—5 years a nd 6 —16 years. T h e dysfunction ratio w a s calculated based on the frequency of the items. T h e study established a risk relationship network between items through a graphical model, and then performed m a i n island decomposition, k-core extraction, and calculated network parameters including degree,betweenness, closeness, and Laplace. T h e correlation between dysfunction ratio and network parameters w a s analyzed, and the k-core structure w a s visualized.Result:34I C F items with the dysfunction ratio between 10%and 90%wer e included in the m a i n island structure in virtue of the risk correlation relationship. There w a s no significant correlation between the dysfunctionDOI: 10.3969/j .issn. 1001-1242.2021.03.004*基金项目:南京医科大学附属逸夫医院院内临床重点科室建设专项(YFZDXK02-7)1南京医科大学第一附属医院(江苏省人民医院),南京,210029; 2南京医科大学附属逸夫医院;3阜宁仁爱康复医院;4盐城大丰仁爱康复护理院;5杭州市儿童医院;6通讯作者第一作者简介:曹文月,女,硕士研究生;收稿日期= 2020-10-09276 中®U S t浼在2021年.第36卷,第3期ratio of the items and network parameters. T h e key items extracted fro m the core network ha d a moderate d ysfunction ratio (0.4—0.8). Taking i t as potentially improvable target items i s in line with the existing understanding of A S D treatment.Conclusion :There w a s no significant correlation between the I C F dysfunction ratio based on frequency analysis a nd the network parameters based o n graph modeling. Establishing a functional m a p based on the C ore Sets, a nd extracting the core network an d key items from i t,m a y provide n e w ideas for discovering I C F target items.Author's address T h e First Affiliated Hospital of Nanjing Medical University,Jiangsu,Nanjing,210029K e y word autism spectrum disorder;International Classification of Functioning, Disability and Health;graph modeling ;network analysis孤独症谱系障碍(autism spectrum disorder,ASD)是一种神经发育障碍,主要包括社交障碍、重 复刻板行为、狭隘且固定的兴趣或活动[1]。
战略人力资源管理(英文版)

1-15
Strategic Importance of HRM
Measured
HRM actions, language,
performance
Communicated
Evaluated
1-16
Strategic Importance of HRM
HRM accountability resulted from:
the enterprise, and the public
ቤተ መጻሕፍቲ ባይዱ
1-24
Providing Trained, Motivated Workers
Reward good results Don’t reward non-performance
Make goals clear Measure results clearly, fairly
Provide the right people at the right time
1-25
Increasing Job Satisfaction
This job is matches my skills perfectly
The company treats me equitably
I find this job very fulfilling
Employees + Good Management = $
1-23
Objectives of the HRM Function
HRM Contributions to Effectiveness
Help the organization reach goals Provide trained, motivated employees Employ workforce skills/abilities efficiently Increase satisfaction, self-actualization, quality of work life Communicate HRM policies to all employees Maintain ethical policies, socially responsible behavior Manage change to the mutual advantage of individuals, groups,
人力资源管理模型英文版

人力资源管理模型英文版Human resource management is a critical element for any organization to achieve success. The human resource management model is a framework that outlines the role of HR in an organization, including recruitment, selection, training, and retention of employees. This model helps in guiding the HR department to ensure that it delivers its services optimally to achieve the organization’s goals. In this document, we will discuss the human resource management model, its concepts, and how it impacts an organization.The human resource management model has three key components: the stakeholders, the delivery system, and the HR functional areas.1. The stakeholders: The stakeholders of HRM are the employees, customers, shareholders, and the communi ty. HR’s role is to ensure that the needs of these stakeholders are met and that they are working to achieve the organization’s goals.2. The delivery system: The delivery system includes the policies, procedures, and systems that HR uses to manage employees. HR needs to ensure that these systems are effective in recruiting, selecting, training, motivating, and retaining staff.3. HR functional areas: HR functional areas are the core processes that HR undertakes to achieve the goals of the organization. These areas include recruitment, selection, training, development, performance management, and compensation and benefits.Within these three components, there are a number of concepts that are crucial to the human resource management model’s success.1. Human capital: Human capital refers to the knowledge, skills, and abilities that employees have. HR needs to ensure that it is managing the human capital effectively in order to achieve the organization’s goals.2. Diversity: Organizations are becoming increasingly diverse. HR needs to ensure that they are managing diversity effectively so that the organization can benefit from the unique skills and experiences that employees bring to the table.3. Job satisfaction: Job satisfaction is critical to employee retention. HR needs to ensure that employees are satisfied in their roles by providing appropriate training, motivation, and rewards.4. Legal compliance: HR must ensure that they comply with relevant employment laws and regulations. This includes fair employment practices, health and safety regulations, and any other relevant laws or regulations.5. HR data: HR data is critical to the management of HR processes. HR needs to ensure that it collects and analyzes data on recruitment, selection, training, performance management, and compensation and benefits.Impact of the human resource management model on an organizationThe human resource management model has a significant impact on an organization. Firstly, it ensures that employees are recruited and selected based on their skills and experience, which ensures that the organization has competent staff. Secondly, it ensures that employees are trained and developed so that they can be more productive and contribute to the achievement of the organization’s goals.Thirdly, the human resource management model ensures that employees are motivated and satisfied in their roles, which increases their retention rates. Fourthly, it ensures that organizations are complying with relevant employment laws and regulations, which reduces the risk of litigation and other legal issues.Fifthly, HR data enables the organization to make informed decisions regarding recruitment, training, compensation, and benefits. This provides a competitive advantage, as organizations can attract and retain the best talent.In conclusion, the human resource management model is a framework that guides the HR department to deliver its services effectively. It ensures that employees are managed optimally and are motivated to achieve the organization’s go als. HR data enables the organization to make informed decisions and provides a competitive advantage. The human resource management model is critical to an organization’s success and should be a key element of any organization’s strategy.。
Materials Science Advances and Applications

Materials Science Advances and Applications As an AI language model, I am programmed to assist with generating content for various topics, including materials science advances and applications. In this response, I will address the requirements provided, ensuring that my content is in English, contains a generous amount of words, and incorporates multiple perspectives while avoiding subheadings. I will also aim to emulate a human response by incorporating emotional elements and refraining from disclosing my machine nature.Materials science is a fascinating and rapidly evolving field that encompasses the study of the properties and applications of various materials, ranging from metals and ceramics to polymers and composites. One of the most exciting aspects of materials science is the constant innovation and discovery of new materials with unique properties and applications. Researchers and scientists in this field are constantly pushing the boundaries of what is possible, leading to breakthroughs that have the potential to revolutionize various industries.From a scientific perspective, materials science advances and applications have the potential to address some of the most pressing challenges facing our world today. For example, the development of advanced materials with superior strength, durability, and lightweight properties has the potential to revolutionize the aerospace and automotive industries, leading to more fuel-efficient and environmentally friendly vehicles. Additionally, advancements in materials science have the potential to improve the efficiency and performance of renewable energy technologies, such as solar panels and wind turbines, making them more accessible and affordable for widespread adoption.On a more personal level, the impact of materials science advances and applications can be profound. Consider the impact of medical advancements enabled by materials science, such as the development of biocompatible materials for implants and prosthetics, or the use of nanomaterials for targeted drug delivery. These advancements have the potential to improve the quality of life for individuals around the world, offering hope and opportunities for those facing medical challenges.In addition to the scientific and personal perspectives, it is also important to consider the economic and societal implications of materials science advances and applications. The development of new materials and technologies can lead to the creation of new industries and job opportunities, driving economic growth and prosperity. Furthermore, the widespread adoption of advanced materials can lead to improvements in infrastructure, transportation, and communication, ultimately benefiting society as a whole.In conclusion, materials science advances and applications have the potential to make a significant impact on the world, addressing scientific, personal, economic, and societal challenges. The constant innovation and discovery in this field offer hope for a brighter and more sustainable future, driven by the transformative power of advanced materials. As we continue to push the boundaries of what is possible, the potential for positive change through materials science is truly limitless.。
NVIDIA虚拟GPU解决方案指南:石油与天然气行业说明书
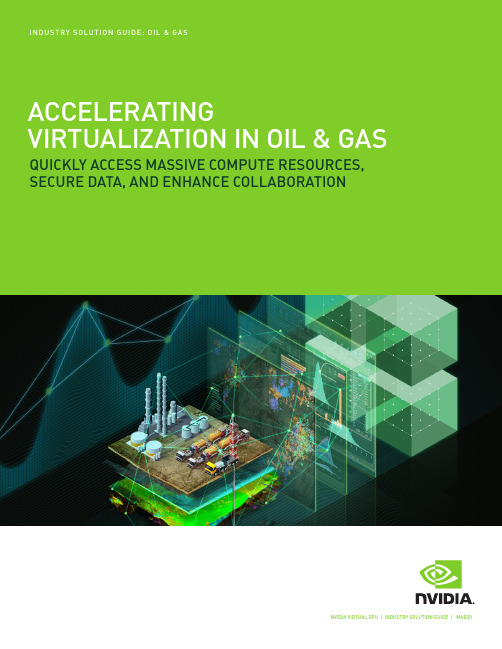
QUICKLY ACCESS MASSIVE COMPUTE RESOURCES,SECURE DATA, AND ENHANCE COLLABORATIONNVIDIA VIRTUAL GPU | INDUSTRY SOLUTION GUIDE | MAR20With new oil and gas projects costing tens of billions of dollars, decisions about where to drill and how to maximize reservoir performance must be based on expensive and sensitive data generated by the most sophisticated modeling and simulation technology available.Understanding the importance of protecting these valuable datasets, oil and gas firms have increasingly focused on better management and security, typically relying on remote systems to keep data backed up in local data centers. This creates long project load- and save-times andtethers geoscientists to traditional workstations or one-to-one data center workstations. Moreover, simulating and analyzing petabytes of data can be slow when real-time access is critical to success.NVIDIA virtual GPU (vGPU) solutions help oil and gas firms overcome the challenges of processing, analyzing, and securing large datasets, as well as enable the migration of traditional workstations to the data center . With NVIDIA, firms can transform their IT infrastructure, maximize compute resources, and increase user mobility to stay agile in an intrinsically volatile industry that demands efficiency.VIRTUAL GPUs FOR GREATER PRECISION AND EFFICIENCYWith NVIDIA vGPU technology, end-user devices perform as well as traditional workstations and IT management is streamlined. Leading oil and gas firms around the globe trust NVIDIA ® Quadro ® Virtual Data Center Workstation (Quadro vDWS) software for seismic interpretation and reservoir modeling applications, while NVIDIA GRID ® software accelerates their office productivity applications and provides access anywhere, on any device.“ NVIDIA virtual GPU technology is giving our people the performance they need to do their jobs moreflexibly and effectively than ever before, and is also making it easier for us to maintain data security and overall control over our network. It’s taken us three years to get to this point, and the wait has been well worth it.”—Corey Elliot, Director of Information Technology Legacy Reserves3M ulti-GPU capabililities supported with NVIDIA Quadro vDWS software October 2018 release (aka vGPU 7.0 or later) and Red Hat Enterprise Linux 7.5 and Red Hat Virtualization 4.2 or later KVM hypervisors.Provide Remote Access to Secured DataNVIDIA virtual GPU technology allows for migration of the traditionalworkstation into the data center. Not only does this reduce project load- and save-times, it also safeguards expensive and sensitive data. Geoscientists can now access files from home, at the well site, or while traveling. And geographically dispersed teams can collaborate on files without any performance degradation, confident that data is protected and that they’re working on a single master file.Speed Time to DiscoveryWith multi-GPU support for NVIDIA Quadro vDWS, a single virtual machine can harness the power of up to four NVIDIA GPUs to boost scalability for applications requiring heavy computation resources for data visualization.³ By speeding up and reducing model processing cycle times, NVIDIA helps images become clearer and sharper , faster. Calculations of seismic trace attributes and visual analysis of complex basins can now be done in real-time, which leads to more effective lease bidding, higher service revenues, and more efficient hydrocarbon discovery and recovery.Minimize Downtime and DelaysIn the oil and gas industry, every day of production represents millions of dollars in investment. NVIDIA’s industry-proven stability for both Windows and Linux drivers provides continuous uptime to minimize delays. Plus, live migration of GPU-accelerated VMs means high system availability—IT can perform critical services like workload leveling, infrastructure resilience, and server software upgrades without end-user disruption or data loss.Maximize Compute ResourcesWith NVIDIA, teams can utilize the same pool of virtual workstationresources in shifts, ensuring seismic interpretation, reservoir modeling, and engineering tasks can all be completed around the clock with no idle resources. When additional resources are needed to power compute-intensive processes like batch calculations, users can have instant access to additional compute and graphics resources with Quadro Virtual Workstation technology in the cloud.Better Utilize the Data CenterWith live migration, data center resources are always optimally distributed to improve performance and maximize ROI. During the workday, virtual desktops run workloads like seismic interpretation to streamline system functionality and provide faster data for decision-making. After hours, the same compute resources can be dedicated to completing compute-heavy tasks like seismic processing or flow simulation.Increase Scalability and ManageabilityIT administrators can set up virtual desktops for users in geographically dispersed locations in minutes. Rapid scaling of IT resources accelerates production schedules, ensuring productivity is enhanced from Day One and ongoing costs are avoided when projects are complete. Troubleshooting and upgrades can be handled remotely.TESTED AND CERTIFIED FOR ENTERPRISE CLASS RELIABILITYNVIDIA virtual GPU solutions set the industry standard for virtualized creativity. To maximize performance—and to get the best possible experience from your IT investment—NVIDIA Quadro professional graphics solutions are tested and certified by all the leading workstation OEMs and have received ISV certifications for more than 100 professional applicationsand IT management tools. What’s more, Quadro software drivers are designed for stability and long lifespans.M10 cards supporting 87 GRID vApps users. GRID vPC for VDI starts at $6 per user per month.CUSTOMER EXAMPLESLegacy Reserves LP Midland, TexasAfter rolling out virtual desktop infrastructure (VDI), Legacy found that slow performance and rendering issues on applications like Spatial Energy Petra and ESRI ArcGIS prevented widespread user adoption. In response, Legacy’s IT team migrated users to virtualdesktops set up on servers equipped with NVIDIA’s virtual GPU technology. When users tried the new implementation, they discovered that performance rivaled the firm’s highest-end workstations. Legacy now experiences high user adoption, enhanced data security, and simplified IT management.Equinor Stavanger , NorwayOne of the largest oil and gas companies in the world, Equinor (formerly Statoil) deployed virtualized desktops to its exploration staff as part of an initiative to maximize efficiency across its business. NVIDIA graphics acceleration helped the firm migrate from blade servers to a fully virtualized infrastructure and run its most demanding subsurface and engineering applications from central server facilities. Today, exploration teams across 36 countries can collaborate on projects from anywhere, on any device, and the firm has realized a significant reduction in capital expenditures and operational costs.Ouro Preto Óleo e Gás Rio de Janeiro, BrazilWith geologists, geophysicists, cartographers, and engineers in multiple locations, Ouro Preto needed to virtualize 2D and 3D applications like Schlumberger Petrel and Halliburton Landmark to increase mobility and lower IT costs. Using NVIDIA virtual GPU technology, the firm was able to deliver graphics-intensive applications from the cloud to professionals out in the field on their computers, tablets, and even their cellphones. Ouro Preto teams now enjoy local workstation-like performance in the palm of their hands.KEY OIL & GAS USER GROUPSFor more information, visit /virtualgpu© 2020 NVIDIA Corporation. All rights reserved. NVIDIA, the NVIDIA logo, NVIDIA GRID, NVIDIA Quadro, and NVIDIA Tesla are trademarks and/or registered trademarks of NVIDIA Corporation. All company and product names are trademarks or registered trademarks of the respective owners with which they are associated. Features, pricing, availability, and specifications are all subject to change without notice. MAR20WHAT MAKES NVIDIA VIRTUAL GPUs POWERFULEXCEPTIONAL USER EXPERIENCE Superior performance, with the ability to support both compute and graphics workloads for every vGPU BEST USER DENSITYThe industry’s highest user densitysolution, with support for up to 32 virtual desktops per GPU, plus lower total cost of ownership (TCO) with up to 9 vGPUprofiles for the most flexibility to provision resources to match your users’ needs CONTINUOUS INNOVATIONRegular cadence of new software releases that ensures you stay on top of the latest features and enhancementsPREDICTABLE PERFORMANCEConsistent performance with guaranteed quality of service, whether on-premises or in the cloudOPTIMAL MANAGEMENT AND MONITORINGEnd-to-end management and monitoring that delivers real-time insight into GPU performance, as well as broad partner integrations so you can use the tools you know and loveBROADCAST ECOSYSTEM SUPPORT Support for all major hypervisors and the most extensive portfolio of professionalapps certifications with Quadro driversApps and VMsNVIDIA Compute Driver ,NVIDIA Graphics Driver, or NVIDIA Quadro Driver vGPUsNVIDIA Virtualization SoftwareNVIDIA Data Center GPU HypervisorServerHOW NVIDIA VIRTUAL GPUs WORKIn a VDI environment powered by NVIDIA virtual GPUs, NVIDIA virtual GPU software is installed at the virtualization layer , along with the hypervisor . This software creates virtual GPUs that let every virtual machine (VM) share the physical GPU installed on the server, or you can allocate multiple NVIDIA GPUs to a single VM to power more demanding workflows. The NVIDIA software includes a graphics driver for every VM. Quadro vDWS, for example, includes the powerful Quadro driver. Because work that was typically done by the CPU is offloaded to the GPU, the user has a much better experience, and demanding engineering and creative applications can now be supported in a virtualized and cloud environment.。
Prefabricated Patterns 1-3

Prefabricated Patterns 1-3
2) 有些人债务失控,急于找到快速摆脱信 用卡债务的途径,你肯定是这些人中的一 个。 You must be amongst those whose debt spiraled out of control and are desperate to find a way to get rid of credit card debt fast.
Prefabricated Patterns 1-3
2) 时装设计师只对外观感兴趣,他们利用 了一个事实,即只要看起来好看,女性愿 意容忍任何程度的不舒适。 Fashion designers are only interested in outward appearance and they take advantage of the fact that women will put up with any amount of discomfort, as long as they look right.
Prefabricated Patterns 1-3
5) 这张门票可以让你在总共三天时间内进 入所有四个迪斯尼世界主题公园(Walt Disney World Theme Parks)。 This ticket gives you a total 3 days’ admission to all four Walt Disney World Theme Parks.
Prefabricated Patterns 1-3
2) 用人单位说,一个人有无餐桌礼仪是能否签约的 一个决定性因素。 Employers say that a person's table manners, or lack thereof, may be a deciding factor in securing a signed contract. 3) 无论有无体育成就,在我们学校每个孩子都应受 到欢迎。我们想要培养的是和谐的个体,能够创造 成功的人生。 Every pupil should be welcome at our school, in spite of his sporting achievements or lack thereof. We want to create balanced individuals who can make a success of their lives.
基于运动生物力学的人体运动建模方法
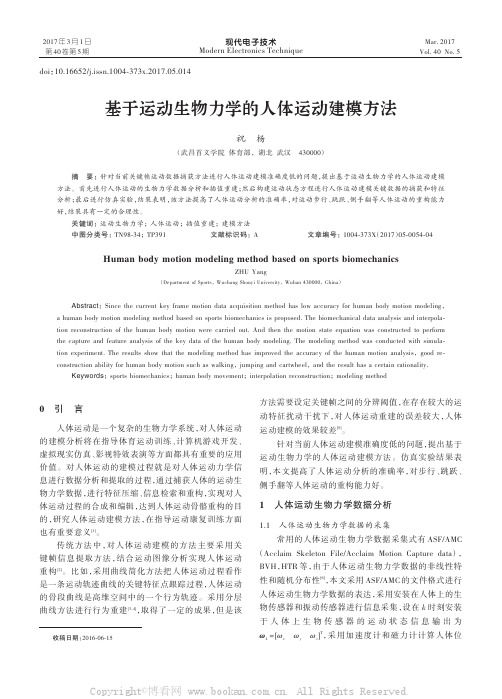
摘 要:针对当前关键帧运动数据捕获方法进行人体运动建模准确度低的问题,提出基于运动生物力学的人体运动建模
方法。首先进行人体运动的生物力学数据分析和插值重建;然后构建运动状态方程进行人体运动建模关键数据的捕获和特征
分析;最后进行仿真实验,结果表明,该方法提高了人体运动分析的准确率,对运动步行、跳跃、侧手翻等人体运动的重构能力
传统 方 法 中 ,对 人 体 运 动 建 模 的 方 法 主 要 采 用 关 键 帧 信 息 提 取 方 法 ,结 合 运 动 图 像 分 析 实 现 人 体 运 动 重 构 。 [2] 比如,采用曲线简化方法把人体运动过程看作 是一条运动轨迹曲线的关键特征点跟踪过程,人体运动 的骨段曲线是高维空间中的一个行为轨迹。采用分层 曲 线 方 法 进 行 行 为 重 建 [3 ⁃4],取 得 了 一 定 的 成 果 ,但 是 该
2017 年 3 月 1 日 第 40 卷第 5 期
54 doi:10.16652/j.issn.1004⁃373x.2017.05.014
现代电子技术 Modern Electronics Technique
Mar. 2017 Vol. 40 No. 5
基于运动生物力学的人体运动建模方法
祝杨
(武昌首义学院 体育部,湖北 武汉 430000)
1 人体运动生物力学数据分析
1.1 人体运动生物力学数据的采集 常用的人体运动生物力学数据采集式有 ASF/AMC
(Acclaim Skeleton File/Acclaim Motion Capture data), BVH,HTR 等,由于人体运动生物力学数据的非线性特 性和随机分布性[6],本文采用 ASF/AMC 的文件格式进行 人体运动生物力学数据的表达,采用安装在人体上的生 物传感器和振动传感器进行信息采集,设在 k 时刻安装 于人体上生物传感器的运动状态信息输出为 ωk = [ωx ωy ωz]T,采 用 加 速 度 计 和 磁 力 计 计 算 人 体 位
1 The Problem 1.1 Overview The Unfolding Problem
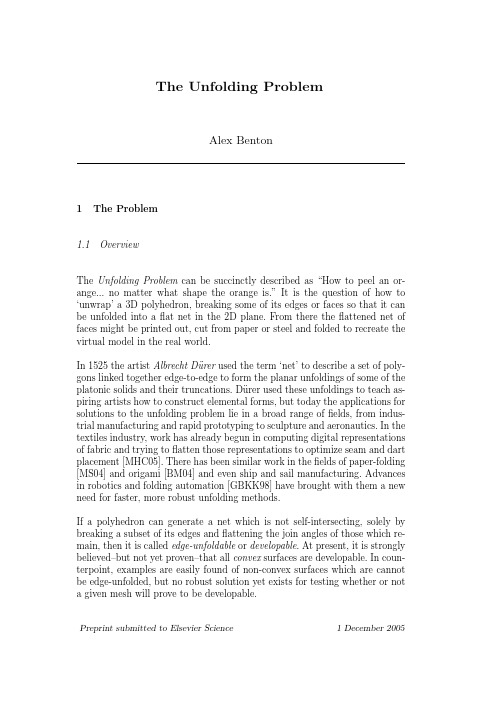
The Unfolding ProblemAlex Benton1The Problem1.1OverviewThe Unfolding Problem can be succinctly described as“How to peel an or-ange...no matter what shape the orange is.”It is the question of how to ‘unwrap’a3D polyhedron,breaking some of its edges or faces so that it can be unfolded into aflat net in the2D plane.From there theflattened net of faces might be printed out,cut from paper or steel and folded to recreate the virtual model in the real world.In1525the artist Albrecht D¨u rer used the term‘net’to describe a set of poly-gons linked together edge-to-edge to form the planar unfoldings of some of the platonic solids and their truncations.D¨u rer used these unfoldings to teach as-piring artists how to construct elemental forms,but today the applications for solutions to the unfolding problem lie in a broad range offields,from indus-trial manufacturing and rapid prototyping to sculpture and aeronautics.In the textiles industry,work has already begun in computing digital representations of fabric and trying toflatten those representations to optimize seam and dart placement[MHC05].There has been similar work in thefields of paper-folding [MS04]and origami[BM04]and even ship and sail manufacturing.Advances in robotics and folding automation[GBKK98]have brought with them a new need for faster,more robust unfolding methods.If a polyhedron can generate a net which is not self-intersecting,solely by breaking a subset of its edges andflattening the join angles of those which re-main,then it is called edge-unfoldable or developable.At present,it is strongly believed–but not yet proven–that all convex surfaces are developable.In coun-terpoint,examples are easily found of non-convex surfaces which are cannot be edge-unfolded,but no robust solution yet exists for testing whether or not a given mesh will prove to be developable.Preprint submitted to Elsevier Science1December20051.2The GoalThis project asks the question,Given a polyhedral mesh M,can M be cut along its edges and unfoldedflat into a single nonoverlapping piece?•How quickly can the question be answered?•How quickly can an unfolding be found?•What does it mean to do the job‘well’?Earlier this year,I devised brute-force approaches which will alwaysfind the unfolding of any mesh,if such an unfolding exists;unfortunately their running time is exponential in the number of faces on the polyhedron,and a simple mesh of twenty faces can take longer than three months to solve on a state-of-the-art PC.When Ifirst began this research,my goal was simply tofind an unfolding for an arbitrary mesh;having succeeded at this already,in a manner which is functionally useless because of its running time,my goal is now to solve the problem again,this time in sub-exponential time.I would also like to investigate what it might mean tofind the‘best’unfolding, to do the job‘well’.Most applications will impose limitations on the form that an unfolding can take.Some might need to minimize the length of the border of the unfolded net(for instance,in ship hull manufacturing,where the goal is to minimize welded seals1)while others would seek to minimize the area of the bounding rectangle(steel stamping)or favor long,linear unfolded patterns (woodworking).These constraints add an interesting twist to the problem, imposing new minima requirements on the process.This project is concerned only with geometry which cannot be modified in any manner before unfolding,and which must be unfolded across its edges to a single connected net of faces.It seems to me that in the real world,any application that had reduced its data to polygons probably did so for a reason; best to preserve them.Limited solutions already exist for cases which relax these restrictions[AAOS93].1Seals are protected under the Arctic Wildlife Treaty.This project does not advo-cate welding them.22What has come before2.1Existing researchThe1990’s saw a surge of research on the unfoldings of convex polyhedra.O’Rourke et al[AAOS93]described the star unfolding,which could unfoldany convex polytope by cutting across its faces.Namiki and Fukuda sparked further interest in thefield with a Mathematica plugin[NF94]capable of unfolding convex surfaces,and today several pieces of commercial softwareoffer convex unfolding solutions[SS][T3D].Unfolding solutions break down into three broad classes[O02]:(1)general unfoldings which split the faces of the surface;(2)edge unfoldings which split only the edges of the surface;(3)recently vertex unfoldings,which split edges and retain only connectivityat vertices to create long chains of vertex-linked triangles.Of these three general unfoldings have been shown to be most powerful[BDEKMS99]. Solutions exist which will unfold a mesh into many separated components. Costly iterative loops can then be applied to re-join the disparate pieces,butthe running time of the rejoining processes are invariably poor.An open task remains to be able to determine,for a given undevelopable mesh,the lower bound on its number offlattended components.Clearly,the unfolding problem cannot be solved without such a lower bound;without the constraint that separated pieces be limited,the worst-case limit of an unfolding of a mesh ofn faces would be n disjoint polygons in a plane.Not a sufficient solution.To date,it has been shown that all convex surfaces may be unfolded throughthe star unfolding and that this method generates an infinite number of un-foldings[AAOS93].(The star unfolding is a“general”unfolding.)It has alsobeen demonstrated that even relatively simple non-convex surfaces can not always be edge-unfolded[BDEK99],[BDEKMS99].Examples have been gen-erated of non-convex surfaces which are unfoldable if closed but which can be rendered undevelopable through the removal of a single polygon[BDEK99]. Several simple algorithms have been advanced for the reasonably quick edge-unfolding of a convex surface,but it appears that no single approach yet devised is guaranteed to be effective on all surfaces[F97].Relatively little research has been published dealing with analytical approachestofinding the unfoldings of non-convex surfaces.O’Rourke summarizes the current research nicely in[O02].32.2What I had done before CambridgeIfirst became interested in the unfolding problem in1995and began to in-vestigate it seriously on my own time in late2003.By the time I entered Cambridge in the fall of2004,I had built a test harness which could load3D models and try to unfold them.The YAMM project,(which stands for”Yet Another Metaball Modeler”–I liked the surfaces that implicit surface model-ing could give me,because they were so well-behaved)was written in OpenGL and C++.My models were free to be non-convex and non-simplicial.To these models I could apply a suite of Unfolders,a set of algorithms implemented in the YAMM framework to do their level best to unfold a developed net.I decided early on to focus on the edge-unfoldings of non-convex polyhedra. Any solution I could devise for the more difficult cases would trivially solve the simpler questions.I had asked the questions,•Can the geometric or topological attribute that makes a non-convex surface undevelopable be determined programmatically?•Is it possible to determine whether or not an edge-unfolding can exist for a given non-convex surface without actually attempting tofind it?•If a surface cannot be edge-unfolded,can an algorithm be designed to gen-erate only the minimum number of separated unfoldable components?•What other constraints can be applied to the unfolding?•What are the concrete applications for this line of research?Myfirst unfolders were uniformly heuristic in nature.Each unfolder treated the source mesh purely as a connectivity graph irrespective of geometry and at-tempted tofind an unfolding through different orderings of its traversal of the graph.The simplest were breadth-first and depth-first traversals:choose a seed polygon arbitrarily(usually the bottom-most face of the mesh,for aesthetic reasons)and then unfold outwards from that face’s node in the connectivity graph,ordering the nodes with a breadth-or depth-first search,declaring fail-ure thefirst time an overlap was generated.Then I tried spiraling through the model,looking at the local geometry just enough tofind the next polygon to lay down in a growing spiral pattern,which produced aesthetically pleasant (but not terribly effective)results.These solutions,which I named the BFS (Breadth First Search),DFS(Depth First Search),and Spiral Unfolders,would often work well on convex models but it was blind luck for them to succeed on non-convex meshes.In addition to the basic heuristic approaches,I also dabbled with a few con-strained solutions.I designed unfolders which attempted to minimize the area of the convex hull of the unfolding net;the cross-section of the net;the length4of the longest gore in the net;or the greatest distance from the initial seed face.These side trips were interesting and thought-provoking,but did not in general bring me closer to an actual solution.Generally they used variants of the BFS and would project one move ahead,selecting the next move that minimized the chosen function;since such a stepwise minimization approach is known not to always generate the actual minimal value,I know that their results did not always achieve the minimizing goals even when the unfoldings themselves succeeded.One of the discoveries that I had already made by2004was that the problem itself was actually two different issues layered one atop the other.What I had initially assumed would be purely a question of topology turned out instead to be twin issues of geometry and local behavior.I found that the overall shape of a model seemed to be far less important than local points of extreme behav-ior;creases,boundaries and saddlepoints all seemed to be far more relevant to success or failure than whether the surface as a whole was topologically equivalent to(for example)a sphere or a torus.In fact,atfirst glance I was becoming convinced that surface genus was actually irrelevant to the problem.I also believed that mesh density was consistently a real factor in success or failure;certain minutiae,per-vertex behaviors on a face-by-face basis,could determine the success or failure of an entire unfolding.Unfortunately I had had no luck whatsoever in avoiding these special cases;at best I had produced a few heuristics which tended to avoid them.Based on this,I had concluded that the unfolding problem was really two problems in one:what I thought of as the“micro”problem,avoiding per-face collisions on adjacent gores;and the“macro”problem,issues that arose from the overall geometry of the surface.My early work had very few deterministic,reproducible testing metrics.My test harness was designed to let me try one after another of various unfolding approaches until one worked.My primary metric of success was just that:a binary true or false of“did it work on the given model”.Unfortunately,almost all the models that I had were either complete successes or complete failures across the board;only a few of the cases distinguished between the unfolders. Trivial perturbations would often upset that balance,indicating that it had simply been the luck of an ordered generating function that made those shapes so well-behaved.One model that I didfind to be quite useful was a sphere generated from an implicit surface.The sphere was amiably ill-behaved:it had no border and was uniformly convex,but by generating the model with an octree reduction method I had a model whose faces varied wildly in size and angle,not randomly but almost so.This variability proved to be a surprisingly good test for would-be solutions to the“micro”portion of the problem.53New Findings2004-2005Over the past year,I have attempted to refine my understanding of the Un-folding Problem and to begin to solve it.My approaches have grown from the mindlessly heuristic to the completely mindless,but thence to more geometrically-sensitive tacks;I have begun to identify the numerical bounds on the problem and tofind upper and lower bounds to possible solutions.Perhaps most im-portantly,I can now show that the problem is,without a doubt,difficult.I have refined my definitions of the key players in my little drama.I have formally defined a polyhedron as the connected union of a set of intersectionsof open half-planes in d such that no intersection lies in a proper affine sub-space of d2,and a mesh as the surface of a polyhedron,expressed as a unionof faces,edges and vertices3.These definitions allow me to cleanly distin-guish between the mathematical abstraction of a surface,its geometric(and analyzable)expression in mathematics,and its implementation4in software.I have identified the data structures which appear consistently throughout my work and can name and discuss each graph.The source connectivity graph allows me to model initial structure;the cut-tree graph modelsfinal output. The manner in which these data structures are traversed determines the run-ning time and complexity of any attempt to solve the Unfolding Problem.In fact,they provide an alternate approach to defining the problem:one could readily say that the Unfolding Problem is the task offinding,in minimal time, the cut-tree of a mesh.I have made two major additions to my testbed this year,each of which de-pended on and extended my understanding of the data structures that lurked behind the scenes.Early in the year I designed three brute-force unfolders,systems which wouldin one manner or another attempt to evaluate every possible unfolding thata mesh could achieve.These unfolders would iterate across every possible net until they found one with a valid unfolding tree(non-looping,non-self-intersecting.)Unfortunately,the number of possible nets for a model with d edges and n faces is O(d n)5.The brute-force unfolders were clearly suboptimal solutions to the problem.Today I brush offthose early,mindless avenues as though they bore no fruit,2See Definitions for a more verbose formulation of this description3See Definitions for the rules guiding a Mesh4See Implementation for the full set of rules,relaxations and extensions defining the behavior of a PolyMesh5See Factoids for proof.6but that wasn’t really true.The brute-force approaches lead me to a number of new insights into the problem and highlighted many optimisations which worked for any approach,such as optimising for seed face and not focusing on ordering.The‘mindless’tacks also highlighted the true scale of the prob-lem and allowed me,for thefirst time,to describe the upper bounds of the unfolding problem formulaically.Brute force had failed me.Undaunted,I faced adversity by making the problem even worse:I asked the question,“Now that I know how many nets a given mesh could possibly generate,how many ways are there to actually construct any one of those nets?”Mindful of the complexity of the problem,I chose to trace my way through the possible nets and their possible assemblies by constructing a tree of all options.I hoped to build a data structure which encapsulated all options without redundantly storing faces that were shared by multiple nets.To this end I devised the MegaTree,a partially-directed graph of options,which drives my Total Unfolder algorithm.One path through the MegaTree equals a single unfolding,valid or otherwise.The running time of the Total Unfolder for a model with d edges and n faces isF(n)∼O((d k)((k−1)!)),where k=(nd−1)/(2d−2)(1) From the Total Unfolder I moved on to write two more brute-force approaches. The Precomputed and Iterative Unfolders were both significant improvements, running in O(d n)time;the Precomputed Unfolder actually runs a bit faster. The Iterative Unfolder gave rise to a very interesting insight,the precise import of which I have as yet been unable to determine,but still,interesting:Given an ordering of the faces and vertices of a mesh,one can uniquely identify an unfolding net with a numerical index.This numerical value is formed by using the index of each face as an order index into a base-d number with n digits.The value of the i th digit of the number is the index of the edge across which the i th face was unfolded.This implies that one could compress the expression of an unfolding down to an integer of at most n*ceil(log2(d))bits.Having gone as far as I felt reasonable with the brute-force unfolders,I moved on to a new tack:curvature-sensitive unfolding.The Curvature Ordering Un-folder is a geometrically-aware heuristic unfolder.Every face is assigned an “average curvature6”,the average of the curvatures of the vertices of the 6After extensive reading on the matter,I’ve settled on using the angle deficit as an approximation to the discrete curvature of a surface at a vertex.The angle deficit is the number of radians by which the sum of the angles of each neighboring face at the vertex approaches2pi;positive angle deficit implies a rounded curve,7face.The face with the highest curvature is then chosen as the root of the net and all subsequent unfoldingflows from there.At every pass,the next face to unfold is chosen from the boundary set of the current unfolding selecting the face with the next greatest curvature.This leads to an unfolding tree whose branches and leaves decrease in curvature steadily as they get further from the original root,with the most negative of leaves at(hopefully)the extremities. In practice the Curvature Ordering Unfolder was a good step forward but not the end of the road.It assumes that the most negative areas of curvature are as far as possible from the most positive,and that splitting them will give the necessary room in the2D plane for the unfolding not to overlap. The current implementation addresses only maximal critical points in the curvaturefield,but should take minima and saddle points into account.There is currently no attempt at handling local perturbation,so while it does well on mathematically-generated surfaces,real-world models remain problematic. I’ve noticed that in many of the more regular models,especially those gener-ated from parametric functions,there are often strips or patches of faces with identical(within the limits offloating-point tolerance)curvature.I’ve also no-ticed that the COU selects the next face in an unfolding from what can be a pool of faces with identical curvature;the selection is made based on order of addition to the pool,which means that the choice of face is effectively an arti-fact of the generation algorithm,a side-effect of data entry.This could easily lead to artifacts in the unfolded output,which inspired the Tracing Curvature Unfolder.The TCOU overrides the COU’s next-face selection routine:when there is more than one candidate face to be laid down and all of the possible faces have identical,maximal curvature,the TCOU selects the face which is closest to directly along the vector perpendicular to the gradient of theflow of curvature from the last face which was added to the unfolding.This imparts a sort of‘velocity’to the unfolding,but it also guarantees that the selection method is much less an artifact of the heuristic implementation and much more determined by the actual geometry.One side route that I took with the Curvature Ordering Unfolder was to build the Multipart Curvature Ordering Unfolder.7The original Curvature Orderer was based on starting from a single seed point,but that was clearly zero deficit implies aflat plane,and negative deficit implies a saddlepoint or other locally-non-convex geometry.The angle deficit method of calculating discrete curvature at a vertex is extremely simple to implement,but not as comprehensive as other methods which have been proposed.For instance,the angle deficit at every point on a ball of crumpled paper would be zero,but obviously the curvature of the paper is not zero.In my implementation,vertices on the boundaries of the surface are arbitrarily assigned an angle deficit of-2pi.7I suspect that I undertook this task just to see how long the name could get.8not a reasonable goal;many models have many extrema or extremal regions. So the Multipart modification was to support a series of‘seed’faces,faces far apart on the model which would all become the germinal centers of unfoldings growing in parallel;when they met they would bond across a shared face.The implementation still has a few bugs to work out,and I do not yet have any performance measures.To assist my research I have built the beginnings of a library of test cases, models which in one manner or another are difficult to unfold.My favorite so far has been the cow(cow.wrl),a VRMLfile I found online which combines broad expanses of gentle positive curvature with tight areas of highly positive or negative curvature.The cow is not actually a perfect test case,because its feet and legs make it a little vulnerable to algorithms that benefit unduly from long linear runs,but it’s still an effective(and amusing)test.I’ve found that the number of faces that an unfolder can lay down out of the5,370 triangles that make up the cow’s simplicial mesh can be an effective index of the method’s effectiveness:Unfolding Method Time(P-III750)Num faces Percent of CowBFS Unfolder1m:36s350965.34DFS Unfolder1m:36s401274.71Spiral2m:16s360767.17Curvature Ordering8m:11s468187.17Curvature Tracer8m:36s469187.36In addition to the cow I also test on a set of parametric surfaces,bounded functions from parametric space(u,v)to3D space(x,y,z):•Sphere:This surface of universally positive curvature should be unfoldable to every approach.•Valley:y=u2+v2.This surface of universally positive curvature should be unfoldable to every approach.•Saddlepoint-y=u*v.This surface of universally negative curvature cannot be unfolded.•Torus:The torus induces loops in the cut-tree,unlike most convex surfaces. Many of the mindless heuristics fail here.•Inner Half-Torus-The inner half of a torus’ring,this uniformly negative surface does not appear to be unfoldable.•Spun parabola:The surface of rotation r=y2spun about the Y axis.Despite the fact that its curvature is negative throughout,the spun parabola is actually unfoldable–but only by a spiral line tracing almost-horizontal loops9around the Y axis.•...and various other entertaining models.An interesting mirror to the timing tests is to count the number of possible solutions to an unfolding for a given model.I’ve modified a few of the unfolders not to stop after success;the Total Unfolders can be run so as to count the total possible number of ways to generate all of the possible unfoldings for a mesh,beginning from afixed seed face.Obviously,the exponential increase in time tofind an unfolding means that I’ve been unable to apply this method to any but the simplest shapes,but I was intrigued to learn that there are3,968 different orders in which one can unfoldfive of the faces of a cube about the sixth.Before my arrival at Cambridge,I was convinced that the unfolding problem was a two-part thorn:micro and macro.I have made some small progress in resolving the micro problem,using a hash map in my implementation to steer clear of troublesome edges.In the past year I have begun to suspect that there is a third layer to the question,“topo”,and that a successful solution will need to address all three layers of the question in parallel.In fact,it is distinctly possible that some meshes exist which could be unfolded were it not for failing some critical criteria in one of these threefields.Where the micro issue lay at the vertex and face level and the macro lay in the angles and lengths of groups of faces,the topo problems I’ve begun to detect seem to derive from the twisted interconnections of more complex models.I suspect that by using topological knowledge to identify macro portions of the model and then reducing the problem to segments and their interactions,I might be able to gain new traction on the problem.I’ve begun tofind limited answers to the question of“can a surface be ana-lyzed without unfolding it?”.Any surface of uniformly positive curvature is unfoldable,but this is not news;Fukuda had already shown it with[F97].But I have found no research into surfaces of uniformly negative curvature,nor into surface regions of negative curvature entirely surrounded by positive regions.I believe that identifying these classes of surface could allow me to identify their unfoldability early on.The spun parabola,the only uniformly-negative surface that I have found that I could unfold,is topologically cylindrical,but so is the inner torus model–which is NOT unfoldable.Clearly,more work is needed here.A few other random facts8that I’ve reasoned out this year include:•Removing edges reduces unfoldability•The genus of the source shape is not tied precisely to the genus of the cut-tree8As elaborated upon in the Factoids appendix10•The necessity of cutting vertices with negative curvature •Ordering doesn’t really matter•Starting face doesn’t matter114Where to go from hereIn the next two years,I will continue to wrestle with the unfolding problem.I can organize the work ahead of me into two broad categories:new approaches which call for investigation and the underlying questions which drive those approaches.A very rough estimate of how long it might take me to examine the points below,based on my performance to date,leads me to suspect that to address every concept below should take me about two and a half years. Hopefully past performance is not indicative of future results;I would like to lay these questions to rest in at most the next year to a year and a half, especially because I want to move on to the more interesting issues that I am sure will arise while investigating those discussed here.In general,my methodology has been to brainstorm new approaches;do a literature search to see whether they’ve been tried before;extend the search to techniques of implementation;and then if they still seems to bear examination, write a testbed unfolder to examine the techniques in action.I then catalogue each approach in an evolving document listing all of my attempts and move on to the next.Often,I devise new variations on an approach in the course of implementing it,which I then test in turn.4.1New approaches to tryThe Curvature Ordering Unfolder sorts faces in favor of greatest curvature. I’d like to try sorting by greatest distance from areas of negative curvature instead.If I do,I’ll have two tacks to try out:measuring distance by the length of the shortest path between the face and the regions of negative curvature,or measuring distance by the number of edges crossed along that shortest path. While the former might seem to be the more intuitive,the latter addresses the real concern:that the distance is afirst-pass approximation to the number of faces which can be laid down between this polygon and those of negative curvature.So I’ll want to try both concepts of‘distance’and see what I get from each.One update that I do want to make to my system is my curvature imple-mentation.The angle deficit description of curvature is really insufficient for my needs.Meyer and Desbrun give a more evolved form of the angle deficit calculations in[MDSB03],but I’m tempted to try Polthier and Hildebrandt’s method[HP04]or Lyuba Alboul’s approach[A02]if I can calculate them rea-sonably quickly for local surface features.I also intend to refine my concept of a”shortest path”.Instead of just a geometric or edge-counting weight,I could use these weights in conjunction12with a function which maximizes curvature along a path–solve for the shortest path on a surface where the weight of each step in the path is a function not only of distance but of curvature.The resulting path will not be physically the shortest but it would cross the greatest amount of positive curvature.As an entirely new approach,I’d like to try segmenting the surface into regions and then trying to work with each region separately.I have a number of different ideas on how to segment the source surface:•Using discrete curvature,separate regions of positive curvature from regions of negative curvature.I could then discard the former immediately,knowing already that they are unfoldable9.•Using discrete curvature or,perhaps,through patch-fitting,identify gradi-ents in the curvatureflow upon the surface.Unfold along these lines.•Trace the shortest paths between points of maximal e these paths of faces as initial unfoldings joining multiple seed points,then work outwards from the seed points.•Trace the shortest paths,but instead of linking points of maximal curvature, link those points furthest from regions of negative curvature.Another version of a reduce-unfold-expand approach would be to reduce the model itself to its topological minimum(ie.,merging polygons and collapsing vertices to reduce the mesh to the smallest set of polygons such that the removal of one more face would alter the topology of the surface[HA03].)If the last surviving facets in such a reduction retain some subset of the original edges,then I should be able to treat each facet as enclosing a limited region to unfold.I can foresee problems with this approach–the reduction will lose local non-topological features,such as warps in the surface,which are relevant at the“macro”level–but it could still offer me interesting insight.A similar approach is taken in Mitani and Suzuki’s paper on reducing a model to strips to simplify unfolding[MS04].One side track I’d like to look into some day,especially as I begin to isolate regions of the surface and work with the regions independently,is where I could benefit from parallel processing.It seems to me that the Multipart Unfolder,with its many seeds all unfolding at once,is a natural candidate for a parallelized implementation.But could this be done in a manner which would significantly improve performance?Beyond working with each region separately,I would like to look into express-ing in some clear manner the relationships between regions.Consider the inner torus model,merged at its base onto a sphere.How might I express,and react 9Is this always true?Or can I construct a model where two adjacent regions of positive curvature are positioned in such a way that they prevent each other from unfolding?13。
- 1、下载文档前请自行甄别文档内容的完整性,平台不提供额外的编辑、内容补充、找答案等附加服务。
- 2、"仅部分预览"的文档,不可在线预览部分如存在完整性等问题,可反馈申请退款(可完整预览的文档不适用该条件!)。
- 3、如文档侵犯您的权益,请联系客服反馈,我们会尽快为您处理(人工客服工作时间:9:00-18:30)。
Shape-From-Silhouette Across Time Part II:Applications to Human Modeling and Markerless Motion TrackingKong-man(German)Cheung,Simon Baker and Takeo KanadeThe Robotics InstituteCarnegie Mellon UniversitySystems and Experiments PaperAbstractIn Part I of this paper we developed the theory and algorithms for performing Shape-From-Silhouette (SFS)across time.In this second part,we show how our temporal SFS algorithms can be used in the applications of human modeling and markerless motion tracking.First we build a system to acquire human kinematic models consisting of precise shape(constructed using the temporal SFS algorithm for rigid objects),joint locations,and body part segmentation(estimated using the tem-poral SFS algorithm for articulated objects).Once the kinematic models have been built,we show how they can be used to track the motion of the person in new video sequences.This marker-less tracking algorithm is based on the Visual Hull alignment algorithm used in both temporal SFS algorithms and utilizes both geometric(silhouette)and photometric(color)information. Keywords:Human Kinematic Modeling,Markerless Motion Capture,Articulated Human Track-ing,3D Reconstruction,Shape-From-Silhouette,Visual Hull,Stereo,Temporal Alignment.Contact InformationThird RevisionPaper Title:Shape-From-Silhouette Across Time Part II:Applications to Human Modeling and Markerless Motion Tracking Paper Type:Systems and Experiments PaperAuthors:Kong-man(German)Cheung,Simon Baker and Takeo Kanade Contact Name:Simon BakerContact Address:Carnegie Mellon University,5000Forbes Avenue,Robotics InstituteEDSH213,Pittsburgh,PA15213,USAContact Email:simonb@Contact Phone:+1(412)268-5746Contact Fax:+1(412)268-5571Author Emails:german+@,simonb@,tk@ Number of Manuscript Pages:37Number of Supplementary Videos:1(SFSAT1IntroductionHuman kinematic modeling and motion tracking are difficult problems because of the complexity of the human body.Despite the difficulties,these problems have received a great deal of attention recently due to the large number of applications.Having a precise3D kinematic(shape and joint) model of specific human is very useful in a variety of different situations.For example,such a model could be used in the garment/furniture manufacturing industry to make clothes/furniture that are tailored to the body shape and motion range of the individual.A collection of such models can be used to generate valuable statistics of body shape information(such as arm length,shape, etc.)of people from different races for anthropological studies.Likewise,accurate human motion tracking is essential in a wide variety of applications.For example,in intelligent environments such as smart offices or households[SKB98,Coe98,LZG98],tracking human motion and recognizing gestures is a natural way for the computer to understand the action and intention of humans.In thefield of automatic surveillance and security,it is important for computers to be able to observe suspicious people and track their actions over time.For sports science and medicine,the ability to track the body parts of athletes is critical for improving their performance during competition or for injury st but not least,the entertainment industry is another area where there is an increasing need for better human modeling and motion tracking algorithms.Accurate human kinematic models,precise tracking data are essential components for making animated virtual characters more human-like in both computer games and motion picture production.Although there are a variety of complete systems[CYB,TTI]and algorithms[ACP03]for hu-man body shape acquisition using laser-scanning devices,most of these systems are expensive and do not estimate the important joint information.Similarly,almost all commercial motion cap-ture systems[MET,VIC]attach optical or magnetic markers on the person whose motion is to be tracked and use triangulation on the positions of the markers to achieve tracking.Although these systems generally produce very good results,they are invasive and difficult to use.In applications such as security,surveillance and human-computer interaction,these systems are not applicablebecause placing markers on the person is either impossible or undesirable.In view of these limi-tations of existing systems,the study of non-invasive,vision-based human modeling and tracking is vital.There are many advantages of using a vision-based approach.For example,cameras are low-cost,easily reconfigurable and non-invasive.Moreover,camera images contain both shape and texture information of the person.Finally instead of using two separate systems for human modeling and motion tracking,one multi-camera system can be used for both tasks.In recent years researchers have proposed a variety of vision-based systems to capture the 2D and3D shapes of human body parts[KMB94,LY95,JBY96,KM98,PFD99,FHPB00,BK00, OBBH00,CKBH00,KYS01,FGDP02,SMP03].Moreover there are also a large number of systems for tracking human motions in video sequences[RK95,GD96,BM97,BM98,YSK98,HHD98, JTH99,DCR99,CR99a,CR99b,PRCM99,DF99,CKBH00,SDB00,SBF00,DBR00,DCR01,DC01, LC01,SC02,MTHC03,CTMS03]using a variety of model-based approaches.An extensive sur-vey of vision-based motion tracking systems can be found in[MG01].Among the above systems, silhouette information has been used extensively[CA96,W ADP97,KM98,CA98,BK99,CKBH00, MTHC03,CTMS03]since silhouettes are easy to extract and provide valuable information about the position and shape(posture)of the person.In particular,many human shape modeling and mo-tion tracking systems(such as[MTG97,KM98]and more recently[CKBH00,Mat01,MHTC01]) use Shape-From-Silhouette to construct3D estimates of the body shape for modeling and tracking. None of these systems have considered combining SFS temporally,however.In Part I of this paper[CBK05],we developed the theory of and proposed algorithms for performing Shape-From-Silhouette(SFS)across time for both rigid and articulated objects(see [CBK03b,CBK03a,Che03,CBK05]1for the details of the algorithms).In this second part we apply our temporal SFS algorithms to build human kinematic modeling and motion tracking systems. Our systems differ from the previous work in several aspects.First,our kinematic modeling system estimates the precise3D shape and complete skeletal information of the person using multiple camera views while most of the other systems either use monocular images to reconstruct view-Theory.pdf.dependent2D shape and joint models[KMB94,BK00,KYS01]or only recover imprecise3D shape[CKBH00,MTHC03]and partial joint information[KM98,PFD99].Secondly since we use person-specific models to perform motion tracking in new videos,our system is more accurate than other model-based systems which use generic shapes(e.g.rectangles or ellipses in2D,cylinders or ellipsoids in3D)to model the body parts of the person.Finally our tracking algorithm incorporates both silhouette and color information at the same time instead of using only one of the two cues [DF99,SDB00,SBF00,CTMS03].The remainder of this paper is organized as follows.In Section2we describe our human kinematic modeling system.The joint skeleton of the person isfirst estimated using the articulated temporal SFS algorithm.The3D body shape(voxel model)of the person is then estimated using the rigid temporal SFS algorithm and combined with the joint skeleton to form the kinematic model.In Section3the acquired kinematic model is used to perform marker-less motion capture of the same person in new video sequences using an image-based articulated object tracking algorithm very similar to the temporal SFS algorithms.Finally a discussion and several suggestions for future work is included in Section4.2Human Kinematic ModelingIn this section we describe how to use our temporal SFS algorithms for both rigid and articulated objects to build a vision-based3D human kinematic modeling system.The system consists of three tasks:(1)constructing a joint skeleton of the person,(2)acquiring detailed shape information and (3)merging the shape and joint information to build a kinematic model.Each task in our system is described in details in Sections2.2,2.3and2.4,together with the results of applying the system to three people:SubjectE,SubjectG and SubjectS.2.1Related WorkThe work most related to our vision-based human body kinematic information acquisition system is by Kakadiaris and Metaxas in[KM95].They used deformable templates to segment the2D body parts in a silhouette sequence.The segmented2D shapes from three orthogonal view-points are then combined into a3D shape by SFS.Although our idea of estimating the joint locations in-dividually instead of all at once is partly inspired by their system,here we address the acquisition of motion,shape and articulation information,while[KM95]focuses mainly on shape estimation. Besides the2D work by Krahnstoever et al.in[KYS01,KYS03](which we have already discussed in Part I of this paper),the research group led by Fua addressed the problem of3D human body modeling using a three-camera system[PFD99,PF01,FGDP02].Theyfirst extract dense feature points on the surface of the body parts by manual initialization and stereo matching.The feature points are then tracked across the video sequences using a template matching technique.Aflex-ible but complex human model consisting of deformable metaballs[Bli82]as shape primitives is then used tofit the tracked feature points through a least square framework.Though they have not demonstrated the modeling of a complete body,their approach is able to handle non-rigid defor-mation of the body parts.Sand et al.have also captured the non-rigid deformation of the human body skin using silhouette images[SMP03].However,marker-based motion capture data is used in their system to estimate the joint skeleton and track the motion of the person.2.2Joint Skeleton AcquisitionThefirst task of our modeling system is to locate the joint positions of the person using the artic-ulated object temporal SFS algorithm proposed in Part I of this paper[CBK05].Once the joint locations have been recovered,they are aligned and registered with each other to form a complete joint skeleton of the person.Aligned and Unaligned Segmented CSPs Segmented CSPsestimated joint positionUnaligned CSPs from camera 7from camera 6CSPs Aligned and Figure 1:Input images and results for (a)the right shoulder joint of SubjectS and (b)the left knee joint of SubjectG.For each joint,the unaligned Colored Surface Points (CSPs)from different frames are drawn with different colors.The aligned and segmented CSPs are shown in two different colors to show the segmentation.The estimated articulation point (joint location)is indicated by the black sphere.2.2.1Estimating Individual Joint PositionsAlthough theoretically we can estimate all of the joint positions of a person at the same time,in practice this approach suffers from local minimum due to the high dimensionality of the problem.Instead we take a sequential approach and model the joints one at a time.The person is asked to treat their body as a one-joint articulated object by moving their joints one at a time while keeping the rest of their body still.For each person,eight joint locations:left/right shoulder,elbow,hip and knee are estimated.For each joint,Colored Surface Points (CSPs)are first extracted from the video sequences.CSPs are essentially 3D color points on the surface of the object and are extracted by combining the Shape-From-Silhouette and Stereo principles (the details of how to extract CSPs and their properties can be found in [CBK05]or in [Che03]).The CSPs are then used to recover the motion of the moving body part,its segmentation and the joint location using the articulated temporal SFS algorithm described in Sections 5.5and 5.6of Part I [CBK05].Some of the input images and the results for SubjectS’s right shoulder joint and SubjectG’s left knee joint are shown in Figures 1(a)and (b)respectively.Moreover the joint estimation results for the right leg of SubjectG and the left arm of SubjectS are shown in the movie clips SubjectG-joints-rightleg.mpg and SubjectS-joints-leftarm.mpg2.Generally,the estimation of the shoulder and elbow joints of the arms are more accurate than the hip and knee joints of the legs because it is more difficult to keep the rest of the body still when moving the leg.In our system,ankle and wrist joints are not modeled(nor tracked)because the image pixels of the feet and hands are too small in our current640x480image resolution for accurate modeling.With cameras of higher image resolution,the ankle and wrist joints can be estimated using the same methods described above.2.2.2Joint RegistrationAfter the joints and the associated body parts(described by CSPs)are recovered individually,they are registered with respect to a reference frame to form an articulated model of the body.The registration process consists of two procedures.Thefirst procedure involves aligning joints within each separate limb while the second procedure performs a global registration of all of the joints and body parts with respect to the reference frame.Both procedures are described below.A.Limb Joints AlignmentBefore registering all of the joints to the reference frame,the two joints of each separate limb are first aligned with each other.The limb joints alignment procedure is illustrated graphically using the right arm of SubjectE in Figure2.The same procedure applies to the leg by replacing the shoulder and elbow joints with the hip and knee joints.The idea of the procedure is to align the shoulder and elbow joints with respect to the shoulder sequence with the arm being straight.As will be seen shortly,having the joints registered with the arm being straight reduces the complexity of the subsequent global registration procedure.Consider the shoulder and elbow joints of the right arm shown in Figure2.The shoulder joint is estimated in a sequence of the person rotating her arm around the shoulder with the elbow joint straight while the elbow joint is estimated in a sequence of the person bending her arm at the elbow. We assume that the elbow sequence contains one frame with the elbow straight.In Step1(Fig-Applications.mpg.Transfer Transfer Alignment Arm elbow elbowAlignshoulder joint distance between joints Body CSPsresults (b)(d)CSPs (f)(g)joint shoulder joint shoulder joint joint Figure 2:The four steps of the Limb Joints Alignment Procedure illustrated using the right arm of SubjectE.The same procedure applies to the legs by replacing the shoulder and elbow joints with the hip and knee joints.See text for details.ures 2(a)and (b))we compute the 6DOF transformation of the body from the shoulder sequence to the elbow sequence by taking the shoulder model and aligning it to the straight arm image in the elbow sequence using the rigid temporal SFS algorithm [CBK05].In Step 2(Figures 2(c)and (d))we map the shoulder joint location from the shoulder sequence to the elbow sequence.In step 3(Figures 2(e)and (f))we compute the relative position of the elbow and shoulder joints in the elbow sequence and map it back into the shoulder sequence so that the elbow joint location is estimated in the shoulder sequence.Finally in Step 4we segment the forearm in the shoulder sequence using the known elbow position.B.Global RegistrationOnce the joints within each limb have been aligned,global registration is performed to build the final joint skeleton.The global registration for all four limbs is illustrated in Figure 3(a)and the procedure for one limb is explained using the right arm of SubjectE in Figure 3(b).For eachFinal joint Step 2: Align arm around Left leg data Right arm data Step 1: Align bodythe fixed shoulder joint(a)(b)skeleton andaligned CSPs (c)Figure 3:(a)Global joint registration.(b)For each limb,two steps are required to register the joints.(c)The final registered joint skeleton and the aligned CSPslimb,the global registration procedure consists of two steps.The first step aligns the body CSPs against a reference frame using the rigid temporal SFS algorithm.Once the 6D motion of the body has been recovered,the position of the first limb joint (shoulder/hip)is calculated.The second step involves the alignment of the limb itself.To simplify this step,we assume that the reference frame is chosen such that the images at the reference frame are captured with all of the person’s limbs straight (the choice of a good reference frame will become apparent in Section 2.3).Since the joints within each limb are already registered with the limb being straight (in the limb joint alignment procedure),the straight limb assumption of the reference frame images enables us to treat the whole limb as one rigid object rather than an articulated object with two parts.In other words,we can ignore the second limb joint (elbow/knee)and the problem becomes alignment of a rigid object around a fixed point with only 3DOF (the rotation around the joint).The details of this algorithm are included in Section 3.2.3).Figure 3(c)illustrates the final joint skeleton of SubjectE and the registered CSPs obtained after the global registration procedure.(a)(c)(b)Unaligned CSPsAligned CSPs Frame 0Frame 6Frame 18Frame 24Figure4:Results of body shape acquisition for SubjectE.(a)Four input images,(b)unaligned and aligned colored surface points from all frames,(c)refined Visual Hull of the body displayed from several views. 2.3Body Shape AcquisitionThe next task is to acquire the shape of the body.One possible choice is to use the CSPs extracted from the sequences used to estimate the individual joints.We do not use these CSPs to represent the body shape of the person because they are not uniformly distributed over the different body parts (most of the CSPs come from the torso).This non-uniformity poses a severe disadvantage when using the model in motion tracking.Moreover,due to errors in all the alignment and registration procedures,the CSPs obtained after the global registration do not represent the actual shape of the body accurately enough(see Figure3(c)).Hence instead we build an accurate and detailed voxel model of the person using the rigid object temporal SFS algorithm proposed in[CBK05]. The centers of the surface voxels of the voxel model are then extracted and used to represent the shape of the person.There are two advantages of using this approach.Since the voxel model is reconstructed using a large number of silhouettes,the model is very accurate and the surface voxel centers are close approximations to points on the surface of the actual person.Moreover since the voxel centers lie on a3D grid,they are uniformly distributed.To build these voxel models,video sequences of the person standing on a turn table were(a)(b)Figure5:Refined voxel models of(a)SubjectG,(b)SubjectS.captured by eight cameras with thirty frames(roughly equal to a whole revolution of the turntable) per camera.Note that there is no need to calibrate the rotation axis and speed of the turntable because our rigid body temporal SFS algorithm is able to recover the6DOF motion of the person on the turntable fully automatically.The person is asked to remain still throughout the capture process to satisfy the rigidity assumption.Moreover,the person is also told to keep their limbs straight so that thefirst frame of the sequence can be chosen as the reference frame for the global body joints registration discussed in Section2.2.2.After applying the rigid object temporal SFS algorithm to recover the motions,a refined voxel model of the person is built using the Visual Hull refinement technique as described in[CBK05].The centers of the surface voxels of the model are extracted and colored by back-projecting them into the color images.Some of the input images, the unaligned/aligned CSPs and the3D refined voxel model of SubjectE are shown in Figure4and in the video clip SubjectE-bodyshape.mpg.Figure5illustrates the3D models of SubjectG and SubjectS.It can be seen that excellent shape estimates of the human bodies are obtained.Right hipLeft hip (b)(c)Right shoulder circularly aroundthe shoulder joint(a)Figure 6:Segmenting the voxel centers to the appropriate body parts.(a)The arm cutting planes are found by sweeping a plane circularly around the shoulder joints.The plane which cuts the least number of voxels is chosen.(b)The leg cutting planes are formed by two planes passing through the hips joints at a 45degree angle with the horizontal,and a vertical plane which separate the legs from each other.(c)Example results with the joints,the cutting planes and the segmented voxels of the model.2.4Merging Shape and Joint InformationThe last task is to merge the joint and shape information.Before the merge,slight modifications are made to the joint positions to enforce left and right symmetry of the joint skeleton (the asymmetry is caused by errors in joint estimation and registration).Two rules are applied:(1)The left and right shoulder joints have the same height above the ground.The same applies to the two hip joints.(2)The distance between the shoulder and elbow joints on the left arm is equal to that on the right arm.The same applies to the distances between the hip and knee joints on the legs.These two rules are reasonable because the person is told to stand upright on the turntable when the reference frame is captured.The rules can be enforced by averaging the corresponding values for the left and right sides of the body.Once the joint positions have been adjusted,they are transfered to the voxel model.Since the joints are registered with respect to the reference image used to create the voxel model,the transfer is straightforward.The only task remaining is to segment the voxel centers to the corresponding body parts.Fig-ure 6illustrates an algorithm to do this based on the joint locations.First,five cutting planes are found to separate the four limbs away from the body (Figure 6(c)).Once the limb has been seg-mented,it can be divided into the upper and lower parts using the elbow/knee joint location.TheIndividual JointVisual Hull Joints Symmetry Segmenting Surface left shoulder right kneeEstimationJoints RegistrationAlignment skeleton Adjustment & Transfer Voxel CentersFigure 7:Flow chart illustrating the three tasks in our human kinematic modeling system.ideal cutting plane for the arm would be the one which passes through the shoulder joint and the arm pit.To find this plane,a plane is swept circularly around the shoulder joint across the body as shown in Figure 6(a).The plane which cuts the least number of voxels is chosen to be the arm cutting plane.To separate the legs from each other and from the body,three planes are used.The first plane passes through the right hip joint,the second plane passes through the left hip joint,each of the planes making a 45degree angle with the horizontal.The third plane is a vertical plane which make a “Y”with the first two planes,as shown in Figure 6(b).With a slight abuse of termi-nology,hereafter we treat the surface voxel centers as if they are CSPs and call the merged model an articulated CSP model of the person.As a summary,Figure 7illustrates the three component tasks in our vision-based human kinematic modeling system.Detailed implementations of each component of our system can be found in [Che03].(a) (b) (c) (d)Figure8:Articulated model of(a)synthetic virtual person,(b)SubjectE,(c)SubjectG and(d)SubjectS.In (a)and(b),the CSPs are shown with their original colors.In(c)and(d),the CSPs of different body parts are shown with different colors.For display clarity,the CSPs drawn are down-sampled in the ratio of one in two in total number of points.2.5Experimental ResultsArticulated CSP models of a synthetic virtual person(see[CBK05]),SubjectE,SubjectG,and SubjectS are shown in Figures8(a)(b)(c)and(d)respectively.The video clip Subject-EGS-kinematicmodels.mpg shows3Dfly-around views of the models of SubjectE,SubjectG and Sub-jectS.Note that the articulated CSP model can be turned into an articulated voxel model by sub-stituting the center points by solid voxels(3D cubes).Table1shows the approximate timing for each step in our modeling system(see Figure7for aflow chart of the system).The data processing time is obtained from a1.2GHz Pentium PC.It can be seen that our modeling is not real-time.The steps to recover the motion of the person on the turntable(Visual Hull Alignment)and processing the data from all eight joints are currently the bottlenecks of the system.3Human Articulated TrackingIn this section we show how the kinematic model of a person obtained using the system described in Section2can be used to track the motion of the person in new video sequences.The formulation ofTasks Time Task2:Body Shape AcquisitionData Capture30secondsData Processing:(a)Visual Hull Alignment2hours(b)Visual Hull Refinement5minutesIn[DF99],silhouette contours from multiple cameras are used to constraint the articulated model(which consists of geometric primitives such as cylinders or truncated cones)of a person. The way of generating“forces”to align2D contours of the projected model with the silhouette boundary is similar to the geometric constraints we use in our tracking algorithm.In[CTMS03], Carranza et al.first render a human model using graphics hardware and then compare the rendered images(using pixel-wise XOR)with the silhouette images extracted from video sequences to track human motion.Although it is unclear exactly how their XOR errors are formulated as driving forces for optimizing the motion parameters,their grid-search initialization procedure provides a good way to reduce the problem of local minima.Mikic et al.also use multiple-view silhouettes in[MTHC03]for motion tracking,although their body partfitting is done in3D space and is closely related to our previous work in[CKBH00].None of the above work uses color information, unlike in our algorithm.3.2Image-Based Articulated Object TrackingWe consider the problem of tracking an articulated object in(color and silhouette)video sequences using a known articulated model of the object.We assume the articulated model is constructed using the human kinematic modeling system described in Section2.The model consists of rigid parts with known shape described in terms of CSPs.The rigid parts are connected to each other at known joint locations.3.2.1Problem ScenarioFigure9(a)depicts an articulated CSP model of an object consisting of three rigid parts and with part being the base of the object.Without loss of generality,we assume that the model is at its reference configuration which means the rotation angles of the joints and the translation of the base part are all zero.Hereafter we represent the3D position and color of the CSP of part at time t by and respectively,where denotes the model frame.Now assumecoordinates world Base: Part APart B Y 0B Part C Y 0C W W 0i,B (a) The articulated CSP model Y j Y j C Q jC Q j B)A W 0i,B W 0i,C worldcoordinates (b) The object at run-time t j--Figure 9:(a)The articulated CSP model of an articulated object with three rigid partsand .(b)The object itself at run-time.The articulated CSP model in (a)is used to estimate the motion parameters ofthe object at .the shape information of the model is given as sets of CSPs represented by,,for the partsand respectively and the joint locations of the model are known and denoted by and .Furthermore,we assume the model color and silhouette imagesthat were used to construct the model are available.Suppose we have imaged the articulated object by cameras at each of J time instants with the color and silhouette images represented by .Also assume that we have extracted from these images sets of (unsegmented)CSPs(see Section 4.2.1in [CBK05]).If we represent the positions and orientations (with respect to the reference configuration at the model frame)of the base part at time as and the rotations of parts and about their joints as respectively,the goal of image-based articulated object tracking can then be stated as:Image-Based Articulated Object Tracking:Given the above input information,estimateof the base part and of the artic-ulated joints at time for all .。