Microreactor Microfluidic Systems with Human Microsomes and Hepatocytes for use
Microfluidics and Microtechnology for Microreactor Systems
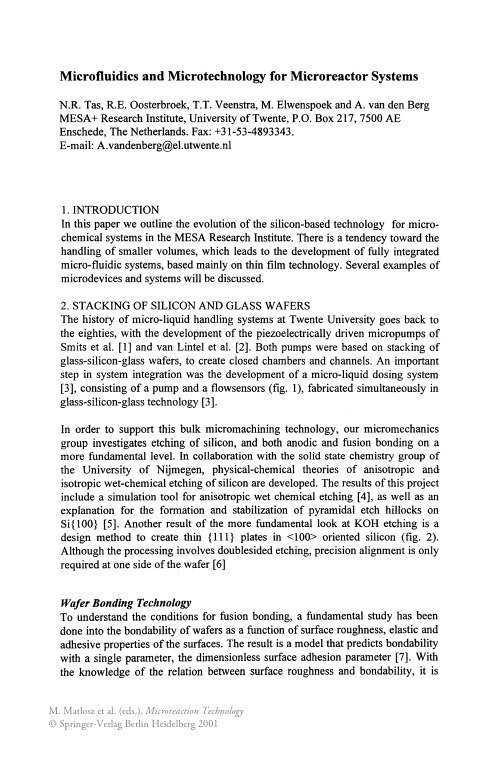
M. Matlosz et al. (eds.), Microreaction Technology © Springer-Verlag Berlin Heidelberg 2001
26
a)
Heater resistor Air chamber Flow sensor
Pump Chamber
Valve 2
Piezos Pyrex Silicon
Figure 3: Peristaltic micropump. From left to right: top view and cross section showing the basic design, and photograph of a realized pump. In the cross section the pump membranes are shown in the activated upward position.
Microfluidics and Microtechnology for Microreactor Systems
N.R. Tas, R.E. Oosterbroek, T.T. Veenstra, M. Elwenspoek and A. van den Berg MESA+ Research Institute, University of Twente, P.O. Box 217,7500 AE Enschede, The Netherlands. Fax: +31-53-4893343. E-mail: A.vandenberg@el.utwente.nl
Wafer Bonding Technology
To understand the conditions for fusion bonding, a fundamental study has been done into the bondability of wafers as a function of surface roughness, elastic and adhesive properties of the surfaces. The result is a model that predicts bondability with a single parameter, the dimensionless surface adhesion parameter [7]. With the knowledge of the relation between surface roughness and bondability, it is
Development of a Microreactor for Solid Phase Synthesis
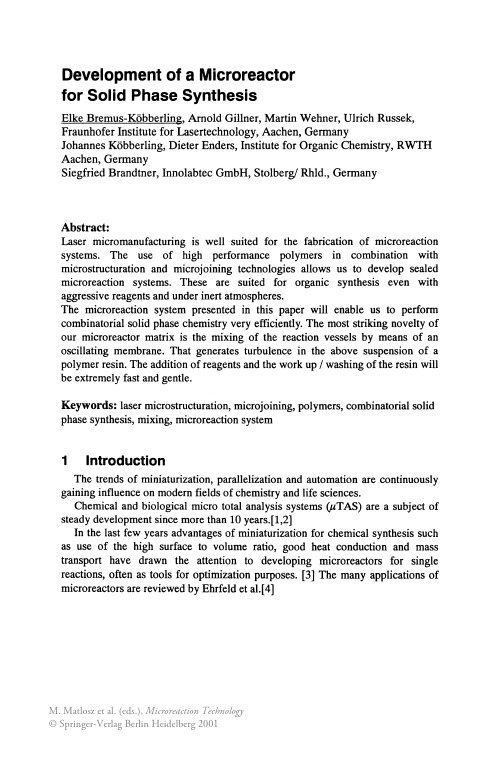
1.2
Solid Phase Organic Chemistry
One of today's most effective and reliable methods for the preparation of large libraries is based on the use of solid phase organic synthesis due to the ease of its automation and the good purities of the final products. This technology - the build up of a molecule on a template which is covalently bonded via a linker to a polymer support, followed by cleavage of the bond to the linker - has been developed to a very reliable tool in parallel synthesis [5]. The main advantages are: • The ease of driving a reaction to completion by employing excess reagent which can be washed off after the reaction. • The avoidance of difficult purification steps such as chromatography, distillation or crystallization. SPOS needs only few repetitive unit operations which have to be realized under inert conditions: • Addition of liquid reagents / solvents • Agitation • Filtration • Heating / cooling
Investigation, Analysis and Optimization of Exothermic Nitrations in Microreactor Processes
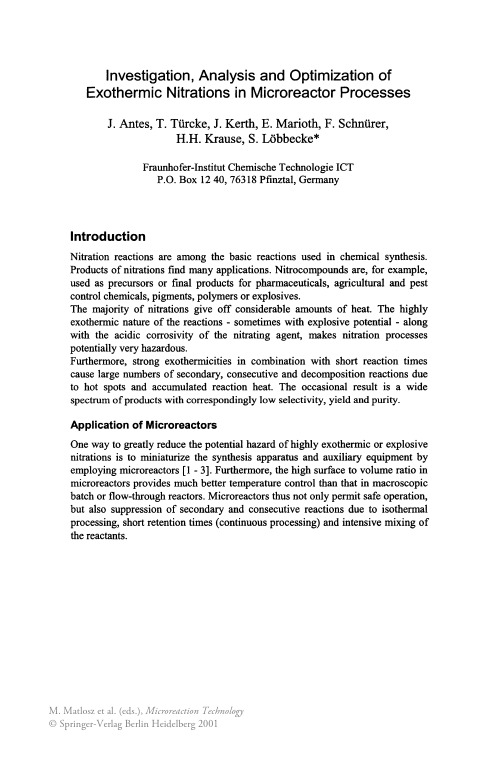
Two different types of microreactors were used for the nitration experiments. A commercially available silicon micromixer based on a microfluidic split-andrecombine structure (MiMoCo GmbH, IlmenauiGermany) and a microreactor array made of glass containing 20 reaction channels in parallel with integrated educt mixing zones and cooling structure (development in cooperation with mgt mikroglas technik AG, Mainz/Germany) [2, 3]. Since nitrating agents are highly corrosive media only resistant materials can be applied for such reactions. Silicon, glass and titanium were proved to be suitable for nitration processes. Microstructured devices made of stainless steels did not withstand such corrosion processes. Different nitrating agents were used for the microreaction experiments. Besides concentrated and fuming nitric acid (65% resp. conc. RN0 3), nitrating acid ("mixed acid": RN0 31H2S04 = 1: 1 - 6: 1) and dinitrogen pentoxide (N205) as a less acid nitrating agent were used. N20 5 was either dissolved in CH2Cl 2 or used as a gaseous reactant (in-situ production: N20 4 + 0 3). Nitrating agents were used in up to 6-fold excess. Ureas (dissolved in dichloromethane) and nitrating agents (liquid or dissolved) were supplied by pulsation-free pumps, mixed inside the microreactors (resp. micromixers) and then passed through reaction capillaries (e.g. PTFE tubes) of different lengths representing different retention times (0.6 s - 82 s). Nitrations were carried out in a continuous mode at defined temperatures between O°C and 20°C. The reaction mixture eluting out of the microreaction system was quenched in ice water and/or cold dichloromethane, extracted and passed to NMR, MS, IR, HPLC or GC-MS for analysis. For a more effective investigation and optimization of nitrations analytical devices and sensors for process control (like temperature monitoring, flow control, etc.) were adapted to the microreaction processes. FTIR microscopy was applied for the online monitoring of nitrations in silicon microreactors. Due to a high spatial resolution of this analytical method (~ 10 flm) educts and products of nitrations can be identified and distinguished at different positions inside the microreactor [5]. Both intermediates and final products of nitrations can be IR spectroscopically detected by focusing the IR
Xona microfluidics llc神经元突触轴突连接微流体培养板共8页
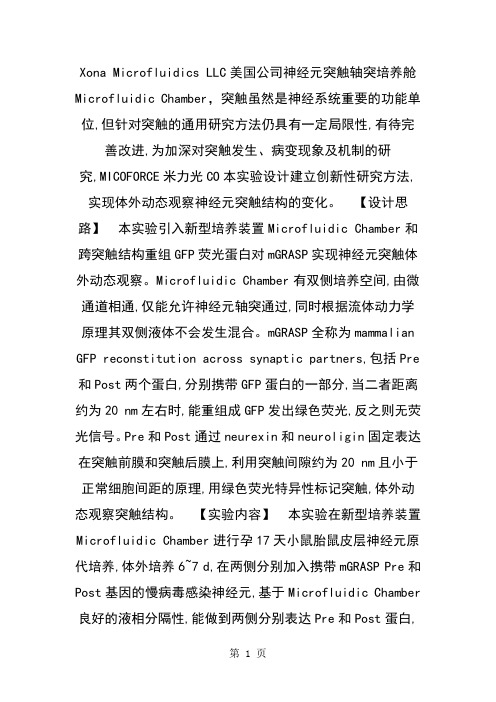
Xona Microfluidics LLC美国公司神经元突触轴突培养舱Microfluidic Chamber,突触虽然是神经系统重要的功能单位,但针对突触的通用研究方法仍具有一定局限性,有待完善改进,为加深对突触发生、病变现象及机制的研究,MICOFORCE米力光CO本实验设计建立创新性研究方法,实现体外动态观察神经元突触结构的变化。
【设计思路】本实验引入新型培养装置Microfluidic Chamber和跨突触结构重组GFP荧光蛋白对mGRASP实现神经元突触体外动态观察。
Microfluidic Chamber有双侧培养空间,由微通道相通,仅能允许神经元轴突通过,同时根据流体动力学原理其双侧液体不会发生混合。
mGRASP全称为mammalian GFP reconstitution across synaptic partners,包括Pre 和Post两个蛋白,分别携带GFP蛋白的一部分,当二者距离约为20 nm左右时,能重组成GFP发出绿色荧光,反之则无荧光信号。
Pre和Post通过neurexin和neuroligin固定表达在突触前膜和突触后膜上,利用突触间隙约为20 nm且小于正常细胞间距的原理,用绿色荧光特异性标记突触,体外动态观察突触结构。
【实验内容】本实验在新型培养装置Microfluidic Chamber进行孕17天小鼠胎鼠皮层神经元原代培养,体外培养6~7 d,在两侧分别加入携带mGRASP Pre和Post基因的慢病毒感染神经元,基于Microfluidic Chamber 良好的液相分隔性,能做到两侧分别表达Pre和Post蛋白,而不会出现二者同一细胞中共表达的情况。
培养4周左右,表达Pre的神经元轴突穿过微通道与另一侧表达Post的神经元接触,形成突触后即可在突触间隙发出绿色荧光。
可在培养基中加入神经营养因子或药物,体外动态观察生理、病理刺激对突触结构的影响。
微生物燃料电池英语

微生物燃料电池英语Microbial Fuel Cells (MFCs) are innovative bioelectrochemical systems that harness the metabolicactivity of microorganisms to convert organic or inorganic substrates directly into electrical energy. These systems are gaining attention for their potential applications in wastewater treatment, energy recovery from waste, and remote power generation.The core of an MFC consists of an anode and a cathode, separated by a proton exchange membrane or a cation exchange membrane. Microorganisms, typically bacteria, are present at the anode, where they oxidize the substrate, releasing electrons and protons. These electrons are then transferred through an external circuit to the cathode, where they are accepted by an electron acceptor, such as oxygen, which is reduced to water.One of the key advantages of MFCs is their ability to utilize a wide range of substrates, including domestic sewage, agricultural waste, and even industrial effluents. This not only makes them environmentally friendly but alsoeconomically viable, as they can convert waste into avaluable resource.However, there are challenges associated with MFCs. The efficiency of energy conversion is currently relatively low, and the design of the MFCs needs to be optimized to enhancetheir performance. Moreover, the selection and cultivation of microorganisms that can efficiently transfer electrons to the anode is a critical aspect of MFC research.Recent advancements in MFC technology have focused on improving the biofilm formation on the anode, enhancing the electron transfer rate, and developing novel materials for the construction of the anode and cathode. The integration of MFCs with other technologies, such as solar cells or wind turbines, is also being explored to create hybrid systemsthat can provide a more consistent power output.In conclusion, microbial fuel cells represent a promising and sustainable approach to energy production and waste management. With continued research and development, these systems could play a significant role in meeting the growing global demand for clean and renewable energy.。
微纳米流动和核磁共振技术
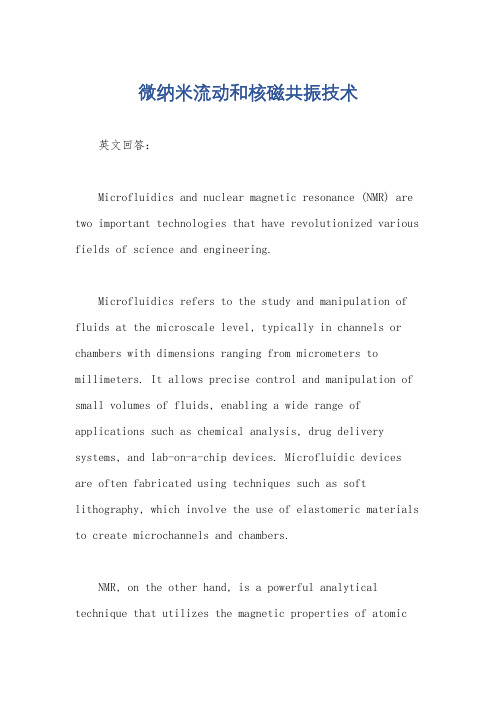
微纳米流动和核磁共振技术英文回答:Microfluidics and nuclear magnetic resonance (NMR) are two important technologies that have revolutionized various fields of science and engineering.Microfluidics refers to the study and manipulation of fluids at the microscale level, typically in channels or chambers with dimensions ranging from micrometers to millimeters. It allows precise control and manipulation of small volumes of fluids, enabling a wide range of applications such as chemical analysis, drug delivery systems, and lab-on-a-chip devices. Microfluidic devices are often fabricated using techniques such as soft lithography, which involve the use of elastomeric materials to create microchannels and chambers.NMR, on the other hand, is a powerful analytical technique that utilizes the magnetic properties of atomicnuclei to study the structure and dynamics of molecules. It is based on the principle of nuclear spin, which is the intrinsic angular momentum possessed by atomic nuclei. By subjecting a sample to a strong magnetic field and applying radiofrequency pulses, NMR can provide information about the chemical composition, molecular structure, and molecular interactions of the sample. NMR has diverse applications in fields such as chemistry, biochemistry, medicine, and materials science.Microfluidics and NMR can be combined to create powerful analytical tools for studying various biological and chemical systems. For example, microfluidic devices can be used to precisely control the flow of samples and reagents, while NMR can provide detailed information about the composition and structure of the samples. This combination has been used in the development ofmicrofluidic NMR systems, which allow rapid and sensitive analysis of small sample volumes. These systems have been applied in areas such as metabolomics, drug discovery, and environmental monitoring.中文回答:微纳米流体力学和核磁共振技术是两种重要的技术,已经在科学和工程的各个领域引起了革命性的变化。
Microreactors
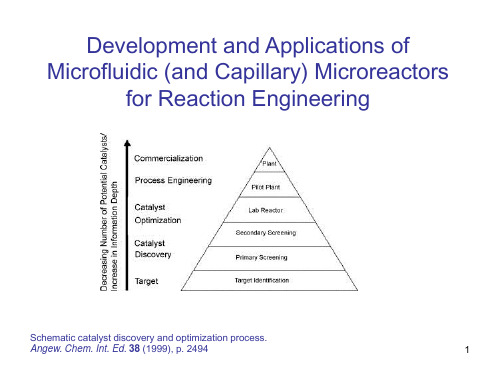
Unequal height causes pressure drop and non-uniform velocity – non-uniform zeta potential of the channel
Non-uniform zeta potential may give rise to adsorption of analytes on the channel
Schematic diagram of the microchip system for sample introduction and detection.
24
Catalytic reactions - Suzuki
Байду номын сангаас
Reaction scheme for the coupling of 4-bromobenzonitrile and phenylboronic acid in a microreactor under EOF
Daniel et.al Chem comm 2005
23
Interface-syringe flow and EOF
This had an error percentage ranging from 1 to 20%. Because the hydrodynamic flow is insensitive to electrophoretic mobility, this electrophoresisbased microchip device was free of injection bias due to different ionic strength and electrophoretic mobility in the sample.
麻省理工学院微电子实验室简介
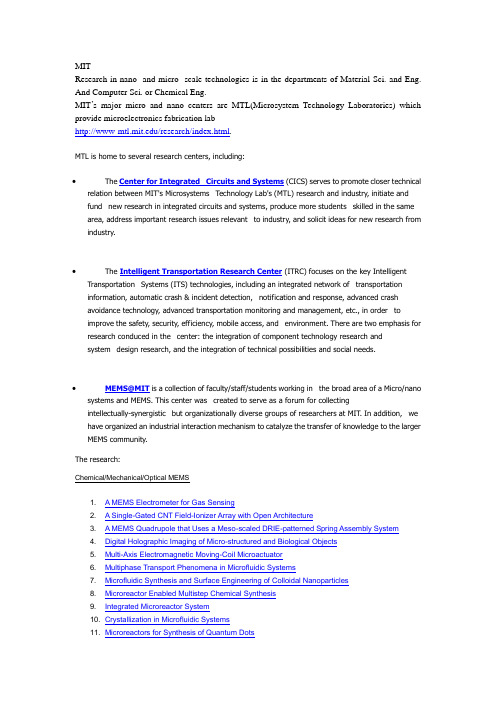
MITResearch in nano- and micro- scale technologies is in the departments of Material Sci. and Eng. And Computer Sci. or Chemical Eng.MIT’s major micro and nano centers are MTL(Microsystem Technology Laboratories) which provide microelectronics fabrication lab/research/index.html.MTL is home to several research centers, including:∙The Center for Integrated Circuits and Systems (CICS) serves to promote closer technical relation between MIT's Microsystems Technology Lab's (MTL) research and industry, initiate and fund new research in integrated circuits and systems, produce more students skilled in the same area, address important research issues relevant to industry, and solicit ideas for new research from industry.∙The Intelligent Transportation Research Center (ITRC) focuses on the key Intelligent Transportation Systems (ITS) technologies, including an integrated network of transportation information, automatic crash & incident detection, notification and response, advanced crashavoidance technology, advanced transportation monitoring and management, etc., in order toimprove the safety, security, efficiency, mobile access, and environment. There are two emphasis for research conduced in the center: the integration of component technology research andsystem design research, and the integration of technical possibilities and social needs.∙MEMS@MIT is a collection of faculty/staff/students working in the broad area of a Micro/nano systems and MEMS. This center was created to serve as a forum for collectingintellectually-synergistic but organizationally diverse groups of researchers at MIT. In addition, we have organized an industrial interaction mechanism to catalyze the transfer of knowledge to the larger MEMS community.The research:Chemical/Mechanical/Optical MEMS1. A MEMS Electrometer for Gas Sensing2. A Single-Gated CNT Field-Ionizer Array with Open Architecture3. A MEMS Quadrupole that Uses a Meso-scaled DRIE-patterned Spring Assembly System4. Digital Holographic Imaging of Micro-structured and Biological Objects5. Multi-Axis Electromagnetic Moving-Coil Microactuator6. Multiphase Transport Phenomena in Microfluidic Systems7. Microfluidic Synthesis and Surface Engineering of Colloidal Nanoparticles8. Microreactor Enabled Multistep Chemical Synthesis9. Integrated Microreactor System10. Crystallization in Microfluidic Systems11. Microreactors for Synthesis of Quantum Dots12. A Large Strain, Arrayable Piezoelectric Microcellular Actuator13. MEMS Pressure-sensor Arrays for Passive Underwater Navigation14. A Low Contact Resistance MEMS-Relay15. "Fast Three-Dimensional Electrokinetic Pumps for Microfluidics16. Carbon Nanotube - CMOS Chemical Sensor Integration17. An Energy Efficient Transceiver for Wireless Micro-Sensor Applications18. Combinatorial Sensing Arrays of Phthalocyanine-based Field-effect Transistors19. Nanoelectromechanical Switches and Memories20. Integrated Carbon Nanotube Sensors21. Organic Photovoltaics with External Antennas22. Integrated Optical-wavelength-dependent Switching and Tuning by Use of Titanium Nitride (TiN)MEMS Technology23. Four Dimensional Volume Holographic Imaging with Natural Illumination24. White Light QD-LEDs25. Organic Optoelectronic Devices Printed by the Molecular Jet Printe26. Design and Measurement of Thermo-optics on SiliconBioMEMS1. A Microfabricated Platform for Investigating Multicellular Organization in 3-D Microenvironments2. Microfluidic Hepatocyte Bioreactor3. Micromechanical Control of Cell-Cell Interaction4. A MEMS Drug Delivery Device for the Prevention of Hemorrhagic Shock5. Multiwell Cell Culture Plate Format with Integrated Microfluidic Perfusion System6. Characterization of Nanofilter Arrays for Biomolecule Separation7. Patterned Periodic Potential-energy Landscape for Fast Continuous-flow BiomoleculeSeparation8. Continuous-flow pI-based Sorting of Proteins and Peptides in a Microfluidic Chip Using DiffusionPotential9. Cell Stimulation, Lysis, and Separation in Microdevices10. Polymer-based Microbioreactors for High Throughput Bioprocessing11. Micro-fluidic Bioreactors for Studying Cell-Matrix Interactions12. A Nanoscanning Platform for Biological Assays13. Label-free Microelectronic PCR Quantification14. Vacuum-Packaged Suspended Microchannel Resonant Mass Sensor for BiomolecularDetection15. Microbial Growth in Parallel Integrated Bioreactor Arrays16. BioMEMS for Control of the Stem-cell Microenvironment17. Microfluidic/Dielectrophoretic Approaches to Selective Microorganism Concentration18. Microfabricated Approaches for Sorting Cells Using Complex Phenotypes19. A Continuous, Conductivity-Specific Micro-organism Separator20. Polymer Waveguides for Integrated BiosensorsEnabling Technology1. A Double-gated CNF Tip Array for Electron-impact Ionization and Field Ionization2. A Double-gated Silicon Tip, Electron-Impact Ionization Array3. A Single-Gated CNT Field-Ionizer Array with Open Architecture4. Aligning and Latching Nano-structured Membranes in 3D Micro-Structures5. Characterization and Modeling of Non-uniformities in DRIE6. Understanding Uniformity and Manufacturability in MEMS Embossing7. Atomic Force Microscopy with Inherent Disturbance Suppression for Nanostructure Imaging8. Vacuum-Sealing Technologies for Micro-chemical Reactors9. Direct Patterning of Organic Materials and Metals Using Micromachined Printheads10. MEMS Vacuum Pump11. Rapid and Shape-Controlled Growth of Aligned Carbon Nanotube Structures12. Prediction of Variation in Advanced Process Technology Nodes13. Parameterized Model Order Reduction of Nonlinear Circuits and MEMS14. Development of Specialized Basis Functions and Efficient Substrate Integration Techniques forElectromagnetic Analysis of Interconnect and RF Inductors15. A Quasi-convex Optimization Approach to Parameterized Model-order Reduction16. Amorphous Zinc-Oxide-Based Thin-film Transistors17. Magnetic Rings for Memory and Logic Devices18. Studies of Field Ionization Using PECVD-grown CNT Tips19. Growth of Carbon Nanotubes for Use in Origami Supercapacitors20. Self-Alignment of Folded, Thin-Membranes via Nanomagnet Attractive Forces21. Control System Design for the Nanostructured Origami™ 3D Nanofabrication Process22. Measuring Thermal and Thermoelectric Properties of Single Nanowires and Carbon Nanotubes23. Nanocomposites as Thermoelectric Materials24. CNT Assembly by Nanopelleting25. Templated Assembly by Selective Removal26. Building Three-dimensional Nanostructures via Membrane FoldingPower MEMS1. Hand-assembly of an Electrospray Thruster Electrode Using Microfabricated Clips2. A Fully Microfabricated Planar Array of Electrospray Ridge Emitters for Space PropulsionApplications3. Thermal Management in Devices for Portable Hydrogen Generation4. Autothermal Catalytic Micromembrane Devices for Portable High-Purity Hydrogen Generation5. Self-powered Wireless Monitoring System Using MEMS Piezoelectric Micro Power Generator6. An Integrated Multiwatt Permanent Magnet Turbine Generator7. Micro-scale Singlet Oxygen Generator for MEMS-based COIL Lasers8. A Thermophotovoltaic (TPV) MEMS Power Generator9. MEMS Vibration Harvesting for Wireless Sensors10. Fabrication and Structural Design of Ultra-thin MEMS Solid Oxide Fuel Cells11. Tomographic Interferometry for Detection of Nafion® Membrane Degradation in PEM Fuel Cells∙The Center for Integrated Photonic Systems (CIPS) mission is to create a meaningful vision of the future, a framework for understanding how technology, industry and business interact and evolve together in the future is required. Models provide us with a process for analyzing the many complex factors that shape this industry and the progress of related technologies.The materials processing center .Making matter meet human needsResearchThe Center brings together MIT faculty and research staff from diverse specialties to collaborate on interdisciplinary materials problems. Center research involves over 150 faculty, research staff, visiting scientists, and graduate and undergraduate students.MPC researchers cover the full range of advanced materials, processes, and technologies, including∙electronic materials∙batteries & fuel cells∙polymers∙advanced ceramics∙materials joining∙composites of all types∙photonics∙electrochemical processing ∙traditional metallurgy∙environmental degradation∙materials modeling- many scale ∙materials systems analysis∙nanostructured materials∙magnetic materials and processes ∙biomaterials∙materials economicsFaculty ProfilesA.I. AkinwandeFlat panel displays,Vacuum Microelectronics and its application to flat panel displays, RF power sources, and sensors. Wide bandgap semiconductors and applications to flat panel displays, UV emitters and RF power sourcesView current research abstracts (pdf)G. BarbastathisBiomedical design instrumentation; precision engineering robotics; volume holographic architectures for data storage, color-selective tomographic imaging, and super-resolving confocal microscopy; interferometric surface characterization; and adaptive micro-opto-mechanics. Optical MEMS.View current research abstracts (pdf)View group web siteM. BazantResearch focuses on transport phenomena in materials and engineering systems, especially diffusion coupled to fluid flow. My group is currently studying granular flow in pebble-bed nuclear reactors, nonlinear electrokinetic flows in microfludic devices, ion transport in thin-film lithium batteries, and advection-diffusion-limited aggregation.View current research abstracts (pdf)View group web siteS. BhatiaResearch focuses on applications of micro- and nanotechnology to tissue repair and regeneration. Emphasis on development of microfabrication tools to improve cellular therapies for liver disease, living cell arrays to study stem cell biology, and nanoparticles for cancer diagnosis and treatment.View current research abstracts (pdf)View group web siteD. BoningSemiconductor manufacturing. Modeling and control of chemical mechanical polishing. Variation modeling and reduction in fabrication processes, devices, and interconnects. Run by run and feedback control for quality and environment in semiconductor fabrication. Software systems for distributed and collaborative computer aided design and fabrication.View current research abstracts (pdf)View group web siteA.P. ChandrakasanDesign of digital integrated circuits and systems. Emphasis on the energy efficient implementation of distributed microsensor and signal processing systems. Protocols and Algorithms for Wireless Systems. Circuits techniques for deep sub-micron technologies.View current research abstracts (pdf)View group web siteG. ChenMicro- and nanoscale heat transfer and energy conversion with applications in thermoelectrics, photonics, and microelectronics; nano-mechanical devices and micro-electro-mechanical systems; radiation and electromagnetic metamaterials.View current research abstracts (pdf)View group web siteM. CulpepperResearch focuses on precision interfaces, precision manufacturing, design for manufacturing, applying precision principles as enabling technologies in multi-disciplinary product design: electronic test equipment, automotive systems, precision compliant mechanisms.View current research abstracts (pdf)View group web siteL. DanielResearch focuses on engineering design applications to drive research in simulation and optimization algorithms and software, design of microfabricated inductors.View current research abstracts (pdf)View group web siteP. DoyleUnderstanding the dynamics of single polymers and biomolecules under forces and fields; lab-on-chip separations, polymer rheology. DNA electrophoresis in microdevices. Superparamagnetic colloids. Brownian Dynamics simulations of complex molecules. Microheology of biopolymers.View current research abstracts (pdf)View group web siteA. EpsteinSmart engines, turbine heat transfer and aerodynamics, advanced diagnostic instrumentation, turbomachinery noise, environmental impact of aircraft.View current research abstracts (pdf)View group web siteD. FreemanBiological micromechanics, MEMS, light microscopy and computer microvision.View current research abstracts (pdf)牋牋牋牋牋牋牋牋牋牋牋?牋View group web siteM. GrayMicrofabricated devices for use in diagnostic medicine and biological research. Particle and fuid analysis of flowing media using absorbance and fluorescence techniques as a means for understanding cell or organism metabolism and phenotypic expression.View group web siteJ. HanBioMEMS, biomolecule analysis, micro/nanofluidics, micro-analysis systems.View current research abstracts (pdf)View group web siteJ. JacobsonDevelopment of processes for directly and continuously printing communication, computation, and displays onto arbitrary substrates. Electronic control of biomolecules.View group web siteK. JensenMicrofabrication and characterization of devices and systems for chemical synthesis and detection, hydrocarbon fuel conversion to electrical energy, bioprocessing and bioanalytics. Multiscale simulation of transport and reaction processes. Chemical vapor deposition of polymer, metal, and semiconductor thin films. Synthesis and characterization of quantum dot composite materials.View current research abstracts (pdf)View group web siteR. KarnikMicro- and nanofluidic systems. Application of transport phenomena in nanofluidics for flow control, separation, sensing. Microfluidic devices for studying chemical kinetics and nanoparticle synthesis.View group web siteS.G. KimSystems Design and Manufacturing, MEMS for optical beam steering, microphotonic packaging and active alignment, micro power generation, massive parallel positional assembly of nanostructures, and nano actuator array.View current research abstracts (pdf)View group web siteJ.H. LangAnalysis, design and control of electromechanical systems. Application to traditional electromagnetic actuators, micron scale actuators and sensors, and flexible structures.View current research abstracts (pdf)View group web siteC. LivermoreMicroElectroMechanical Systems (MEMS). Design and fabrication of high power microsystems. Nanoscale self-assembly and manufacturing.View current research abstracts (pdf)View group web siteS. ManalisApplication of micro- and nanofabrication technologies towards the development of novel methods for probing biological systems. Current projects focus on electrical and mechanical detection schemes for analyzing DNA, proteins, and cells.View current research abstracts (pdf)View group web siteD.J. PerreaultAnalysis, design, and control of cellular power converter architectures. DC/DC Converters fordual-voltage electrical systems. Electrical system transient investigation. Exploration of non-conventional electricity sources for motor vehicles.View group web siteM.A. SchmidtMicroElectroMechanical Systems (MEMS). Microfabrication technologies for integrated circuits, sensors, and actuators. Design of microsensor and microactuator systems.View current research abstracts (pdf)A. SlocumPrecision Engineering; Machine Design; Product Design.View current research abstracts (pdf)View group web siteC.V. ThompsonProcessing, structure, properties, performance, and reliability of thin films and structures for micro- and nano-devices and systems. Reliability and Interconnect.View current research abstracts (pdf)View group web siteT. ThorsenIntegrating microfluidic design and fabrication techniques, electronics and optics with biochemical applications. Optimizing channel dimensions, geometry, and layout to generate 3-D fluidic networks that are functional and scalable. Interface development to combine microfluidic technologies with pneumatic valves, MEMS-based detector systems, and software-based data acquisition and interpretation, creating devices for fundamental research and diagnostic applications.View current research abstracts (pdf)View group web siteH.L. TullerCharacterize and understand key electronic, microstructural, and optical properties of advanced ceramic materials. Fabrication andcharacterization of crystals, ceramics and glasses for electronic devices, lasers, electrochemical energy conversion, sensors and actuators.View current research abstracts (pdf)View group web siteJ. VoldmanBiological applications of microsystem technology. Engineering and use of microsystems for analysis and engineering of single cells. Physical and electrical cell manipulation. Design, modeling, microfabrication, and testing of microfluidic biological devices employing unconventional materials and fabrication processes. Electromechanics at the microscale.View current research abstracts (pdf)View group web siteE. N. WangDevelopment of MEMS/NEMS for: Biochemical sensing and detection; Thermal management of high power density and high performance systems; Diagnostics for biological systems and bio-functionality View group web siteB. WardlePower MEMS microyhydraulics, structural health monitoring, nanocomposites, damageresistance/tolerance of advanced composite materials, cost modeling in the structural design process, conversion of technology to value.View current research abstracts (pdf)View group web siteJ. WhiteTheoretical and practical aspects of numberical algorithms for problems in circuit, device, interconnect, packaging, and micromechanical system design; parallel numerical algorithms; interaction between numerical algorithms and computer architecture.View current research abstracts (pdf)View group web siteLaser-cooling brings large object near absolute zeroAnne Trafton, News OfficeApril 5, 2007Using a laser-cooling technique that could one day allow scientists to observe quantum behavior in large objects, MIT researchers have cooled a coin-sized object to within one degree of absolute zero.Fig.1Assistant professor Nergis Mavalvala, left, and Ph.D. student Thomas Corbitt are part of an international team that has devised a way to cool large objects to near absolute zero. Enlarge image (no JavaScript)Fig.Super-mirrorMIT researchers have developed a technique to cool this dime-sized mirror (small circle suspended in the center of large metal ring) to within one degree of absolute zero. Enlarge image (no JavaScript)Fig.2Assistant professor Nergis Mavalvala, right, and Ph.D. student Thomas Corbitt look over the laser system they use to cool a coin-sized mirror to within one degree of absolute zero. Enlarge image (no JavaScript)。
- 1、下载文档前请自行甄别文档内容的完整性,平台不提供额外的编辑、内容补充、找答案等附加服务。
- 2、"仅部分预览"的文档,不可在线预览部分如存在完整性等问题,可反馈申请退款(可完整预览的文档不适用该条件!)。
- 3、如文档侵犯您的权益,请联系客服反馈,我们会尽快为您处理(人工客服工作时间:9:00-18:30)。
Biomedical Microdevices7:2,117–125,2005C 2005Springer Science+Business Media,Inc.Manufactured in TheNetherlands.Microreactor Microfluidic Systems with Human Microsomes and Hepatocytes for use in Metabolite StudiesJeanna C.Zguris,1Laura J.Itle,2Daniel Hayes,3andMichael V.Pishko4,∗1Department of Chemical Engineering,2Department of Chemical Engineering and The Huck Institute for theLife Sciences,3Department of Material Science,and4Departments of Chemical Engineering,Chemistry,and MaterialsScience&Engineering,The Pennsylvania State University,UniversityPark,PA16802-4420,USAE-mail:mpishko@Abstract.In the area of drug discovery,high-speed synthesis has increased the number of drug candidates produced.These poten-tial drugs need to be evaluated for their adsorption,distribution, metabolism,elimination,and toxicology(ADMET)properties as early in the drug development stage as possible.Previously,a poten-tial drug’s ADMET properties have been found out by using mono-layer cell cultures and live animals.These methods can be costly, time-intensive,and impractical for screening the large amount of potential drugs created by combinatorial chemistry.A quick,small, inexpensive,and highly parallel device would be desirable to deter-mine a drug candidate’s properties(i.e.,metabolism of the drug). Here we fabricate a microfluidic device entrapping human micro-somes within poly(ethylene)glycol hydrogels thereby generating an in situ microreactor to assess a drug candidate’s metabolic proper-ties that can be coupled to analysis equipment.We show that mi-crosomes can be entrapped without the loss of enzymatic activity during photopolymerization.Additionally,a microreactor utilizing hepatocytes was also created for comparison with the microsome microreactor.Key Words.microsomes,hydrogels,microreactor,cytochrome P450IntroductionAdsorption,distribution,metabolism,elimination,and toxicology(ADMET)studies are a key area of drug development in which potential drugs are evaluated in an attempt to determine adverse in vivo reactions prior to human trials.Despite rigorous testing,approximately 75,000–135,000deaths due to adverse drug reactions oc-cur annually in the United States,making it the sixth lead-ing cause of death(Hodgson,2001).In addition to limit-ing the number of deaths due to adverse drug reactions, a distinct economic advantage exists for pharmaceutical companies that determine ADMET properties early in the development process(Myers and Baker,2001).An esti-mated90%of drugs are dropped from development in the late clinical stage;eliminating any number of these dead-end drug candidates earlier in the process would allow for the reinvestment of research moneys into more productive avenues(Contag,2002).We have devised a microreactor with microsomes en-trapped in a polymer matrix of poly(ethylene)glycol that can be used to determine the metabolic products of potential drug candidates.Derived from the ultrasonic homogenization of human liver,microsomes retain the metabolic properties of the liver,the organ largely re-sponsible for drug metabolism(Pearce,et al.,1996). Microsomes contain a high percentage of cytochrome P450,group of hemeproteins(Khan,2003)that com-pose an enzyme superfamily subdivided by enzyme struc-ture(Chauret et al.,1999).The cytochrome P450family plays a major role in the oxidative metabolism of xeno-biotics,including toxins,exogenous chemicals,and drugs (Pearce et al.,1996;Chauret et al.,1999;V ondracek et al., 2001).Cytochrome P450metabolism of potential drug can-didates is becoming a routine aspect of high through-put drug screening.For example,the inhibition of Cy-tochrome P450activity can indicate an adverse drug-drug interaction(Crespi et al.,1997).In vitro drug metabolism and cytochrome P450activity related to drug exposure is measured in a variety of biological systems with in-creasing orders of structure and organization.These sys-tems include microsomes,supersomes,hepatocytes,and liver slices(Brandon et al.,2003).The production of drug metabolites can vary depending on the system used,with microsomes and supersomes producing Phase I metabo-lites due to Cytochrome P450,while hepatocytes and liver slices can produce Phase I(hydrolysis,oxidation, and reduction)and Phase II(conjugation)metabolites in in vitro environments(Brandon,2003;DeGraaf et al.,2003).Despite incomplete metabolism of some drug candidates,microsomes are an inexpensive,easy to use *Corresponding author.117118Zguris et al.alternative for initial testing of a drug’s metabolism.In this case human microsomes are used,which will pro-vide us with information regarding the metabolites that will be produced in humans(Sivapathasundaram et al., 2003).The immobilization of microsomes in a three-dimensional matrix provides several advantages over co-valent linkage or physical adsorption including increased storage stability,the ability to separate products from the incubation step,and the ability to reuse and recycle the microsomes for later tests(Sakai-Kato et al.,2002).It has been shown by Sakai-Kato and co-workers that micro-somes can be entrapped within a sol-gel matrix(Sakai-Kato et al.,2002).Microsomes can also be entrapped in a poly(ethylene)glycol matrix,which has several advan-tages over sol-gel technology.These advantages include biocompatibility of the matrix,non-toxic crosslinking so-lutions,faster polymerization time,and ease of patterning (Honiger et al.,1995;Cruise et al.,1998;Alcantar et al., 2000;Elisseeff et al.,2000;Revzin et al.,2001;Burdick and Anseth,2002;Koh et al.,2002,2003;Liu,2002;Liu et al.,2002;Revzin,2003).Previously,we have encap-sulated and patterned enzymes and whole cells for use in sensing applications(Revzin et al.,2001;Koh et al., 2002,2003).However,microsomes harness the advan-tages of both enzyme and whole cell encapsulation.Mi-crosomes contain multiple enzymes,leading to a variety of products,but without needing a tightly regulated external environment.Here we describe the fabrication of a poly(ethylene) glycol microreactor containing microsomes in microflu-idic channels for easy sample delivery.Because it is con-tained within a microfluidic device,this microreactor has the ability to be coupled with a variety of downstream de-tection devices for drug metabolites.However,this method relies on the ability of drug candidates to be transferred through the hydrogel matrix and for drug products to be returned to the process stream.To this end,we charac-terized the mass transfer by calculating the mesh size of PEG hydrogels.Additionally,we compare the activ-ity of encapsulated microsomes with microsomes in so-lution,hepatocytes in solution,and encapsulated hepa-tocytes by utilizing afluorescent substrate,EFC and its fluorescent product HFC(Roberts et al.,1995;Kent et al., 2004).Experimental SectionMicrosomesGLP human liver microsomes(CellzDirect,Austin TX) were used.For imaging,the microsome membrane was stained with3,3 -dioctadecyloxacarbocyanine perchlorate (DiOC)(Molecular Probes,Eugene,OR)dissolved in dimethyl sulfoxide(DMSO)(VWR International,West Chester,PA).Cell cultureSV-40transformed murine hepatocytes were obtained from American Type Culture Collection(Manassas, V A).Cells were incubated at37◦C in5%CO2and 95%humidified air in Dulbecco’s Modified Eagle’s Medium(DMEM,Sigma,St.Louis,MO)with4.5g/L glucose,200nM dexamethasone,4%fetal bovine serum(FBS),and1%antibiotic/antimycotic solution (Sigma,St.Louis,MO).Hepatocytes were grown to confluence in75cm2polystyrene tissue cultureflasks and confluent cells were subcultured every2to3days by trypsinization with0.25%(w/v)trypsin and0.13%(w/v) EDTA.Forfluorescent whole cell imaging,cells were stained with Cell Tracker Orange CMTMR(5-(and-6)-(((4-chloromethyl)benzyl)amino)tetramethylrhodamine) (Molecular Probes,Eugene,OR).Preparation of PEG hydrogel spheresHydrogel spheres were prepared by following a pre-viously described procedure(Russell et al.,1999).A poly(ethylene)glycol diacrylate(PEG-DA,Sigma,St. Louis,MO)precursor solution was extruded through a21-gauge needle into a graduated cylinder of mineral oil.The precursor droplets were photopolymerized with a365nm, 300mW/cm2light source(EFOS Ultracure100ss Plus, UV spot lamp,Mississauga,Ontario).The spheres were collected and washed repeatedly with water.For mass transfer experiments,spheres containing 100nmfluorescently particles(Molecular Probes,Eu-gene,OR)and spheres that did not contain any particles with the composition of700µL of PEG-DA,300µL of phosphate buffer saline solution(0.1M,pH7.3)and 10µL of Darocur1173.For spheres that were created mi-crosome activity measurements,a precursor solution with 700µL of PEG-DA,200µL of phosphate buffered saline solution(0.1M,pH7.4),100µL of glucose-6-phosphate dehydrogenase at0.07KU/mL,20µL of microsomes at 20mg protein/mL and10µL of2-Hydroxy-2-methyl-1-phenyl-1-propanone(Darocur1173,Ciba,Tarrytown, NY)was prepared.To entrap hepatocytes in PEG spheres, a cell suspension of1.0×106to1.5×106cells/mL in90%phosphate buffered saline,10%PEG-DA(MW 575),and1%2-hydroxy-2-methyl-1-phenyl-1-propanone (Darocur1173,Ciba,Tarrytown,NY)photoinitiator was used.Microreactor Microfluidic Systems with Human Microsomes and Hepatocytes119Cytochrome P450activity in microsomes and mammalian cellsFor the determination of cytochrome P450in microsomes, suspension or entrapped microsomes were used.For sus-pension studies,solutions were made with3000µL of phosphate buffer saline solution(0.1M,pH7.4),100µL of glucose-6-phosphate dehydrogenase at0.07KU/mL, 20µL of microsomes at20mg protein/mL,100µL of glucose-6-phosphate(0.57M,Sigma,Sigma,St.Louis, MO),and100µL of nicotinamide adenine dinucleotide phosphate oxidized sodium salt(NADPH)(VWR Inter-national,West Chester,PA).The NADPH,glucose-6-phosphate and glucose-6-phosphate dehydrogenase were added for the NADPH regenerating system that is needed with the use of microsomes.Microsome containing spheres were generated as previously described and sus-pended in2mL PBS with100µL of glucose-6-phosphate (0.57M,Sigma,Sigma,St.Louis,MO)and100µL of NADPH.7-ethoxy-4-trifluoromethylcoumarin(1g/L, EFC)(Molecular Probes,Eugene,OR)were added to microsomes or sphere suspensions in varied amounts. Fluorescent emission spectra of the EFC or the prod-uct of the reaction,7-hydroxy-4-trifluoromethyl coumarin (HFC)(DeLuca et al.,1988;Buters et al.,1993)were ob-tained using afluorescence spectrometer(QM-1,Photon Technology International).For the determination of cytochrome P450activity in mammalian hepatocytes,cell suspensions or entrapped hepatocytes were used.For suspension studies,adherent cultures were detached via trypsinization,pelleted,and re-suspended at a density of1.0×106to1.5×106cells/mL in sterile0.1M phosphate buffer saline solution(PBS),pH 7.4.Cell containing spheres were generated as previously described and suspended in PBS.10µL of1g/L7-ethoxy-4-trifluoromethylcoumarin(EFC)(Molecular Probes,Eu-gene,OR)were added to cell or sphere suspensions.Flu-orescent emission spectra of the EFC or the HFC were obtained using afluorescence spectrometer(QM-1,Pho-ton Technology International).Preparation of PEG hydrogel microstructuresThe hydrogel microstructures were made using a simi-lar method as previously reported(Revzin et al.,2001).In short,the hydrogel arrays were patterned photolithograph-ically with poly(ethylene)glycol diacrylate(PEG-DA) (MW575,Sigma,Milwaukee,WI)on glass substrates.To prepare the substrates for the arrays,an oxidized surface was created by an acid wash(6N sulfuric acid,Aldrich Chemical Co.,Milwaukee,WI)followed by a base wash (0.1M sodium hydroxide,Sigma,St.Louis,MO)of the glass followed by treatment with3-(trichlorosilyl)propyl methacrylate(Sigma,St.Louis,MO)to form a self as-sembled monolayer(SAM)with pendant methacrylate groups.A solution containing PEG-DA(MW575,Sigma, St.Louis,MO),0.1M phosphate buffered saline, and a photoinitiator,2-Hydroxy-2-methyl-1-phenyl-1-propanone(Darocur1173,Ciba,Tarrytown,NY)was prepared.For microsome containing arrays,the con-centration of components was700µL of PEG-DA, 200µL of phosphate buffer saline solution(0.1M, pH7.3),100µL of glucose-6-phosphate dehydrogenase at0.07KU/mL,20µL of microsomes at20mg pro-tein/mL that were previously exposed to DIOC and10µL of2-Hydroxy-2-methyl-1-phenyl-1-propanone.To gen-erate cell-containing hydrogel arrays,a cell suspension of1.0×106to1.5×106cells/mL in PBS was ing the cell suspension,a10%PEG-DA solution with1%photoinitiator,2-Hydroxy-2-methyl-1-phenyl-1-propanone(Darocur1173,Ciba,Tarrytown,NY),was prepared.The microsome or cell-containing precursor solution was subsequently placed onto the methacrylated substrate. The precursor solution was then covered with a photomask and exposed to ultraviolet light at365nm,300mW/cm2 (EFOS Ultracure100ss Plus,UV spot lamp,Mississauga, Ontario)region for1–2seconds.Areas exposed to light cross-linked via a free radical mechanism and the result-ing hydrogel network isfixed in place while the remaining unreacted monomer is washed away with water.Arrays were imaged on an Axiovert Zeiss200Mfluorescent mi-croscope with a mercury light source(Zeiss,Thornwood, NY).Preparation of microfluidic channelsThe masters had negative patterns that were prepared us-ing photolithography with SU-850negative photoresist (Microlithography Chemical Corp,Newton MA).The patterns were made on glass slides and silica wafers. The pattern was placed on the middle of the substrate utilizing a chrome sodalime photomask made by Ad-vanced Reproductions(Andover,MA).To create the poly(dimethylsiloxane)(PDMS)channels for the genera-tion of microreactors within microfluidic channels,mas-ters were placed in petri dishes and covered with a10:1 mixture of PDMS prepolymer to curing agent(Dow Corn-ing Sylgard184,Midland,MI).The resulting degassed mixture was poured into the Teflon mold with the master and cured at60◦C for at least2hours.After curing,the PDMS replica was removed from the master and the in-let and outlet ports were placed by piercing the replica with a blunted syringe needle through the backside of the network.PDMS channels and glass coverslips were treated with a115V high frequency generator model120Zguris et al.BD-10AS(Electro-Technic Products,Inc,Chicago,IL) to irreversibly seal the channel to the glass.To create microreactors within the microfluidic net-work,polymer precursor solution previously described containing either microsomes or hepatocytes were pumped into the microfluidic channels.A photomask was positioned over the microfluidic channel,and the polymer precursor solution was exposed to ultraviolet light as de-scribed above.Unreacted polymer precursor was rinsed from the channel using water.Arrays within microfluidic networks were imaged using an Axiovert Zeiss200Mflu-orescent microscope with a mercury light source(Zeiss, Thornwood,NY).Results and DiscussionFabrication of microsome containing hydrogel arrays The process of making hydrogel microstructures with mi-crosomes has been developed.The microsomes are bought with a20mg/mL protein,which includes a large amount of tissue debris.The large debris can be seen in Fig-ure1(a).The large tissue debris could block the microflu-idic channel and alter the mass transfer of the analyte to the microsomes in the hydrogel.Depending on the chan-nel width it could be critical that the larger tissue debris was removed.This was accomplished by centrifuging the solution,removing and keeping thefluid and throwing out the pellet.After thisfiltering technique most of the large debris is removed.This can be shown by Figure1(b), there is sufficiently less debris after thisfiltering technique.Thefiltered or unfiltered solution of microsomes can be entrapped in hydrogel microstructures.We have shown that the microsomes in the unfiltered state can be en-trapped with a polymer matrix and stay intact.This is shown in Figure2,where the lipid membranes are stained with DiOC remainingfluorescently active.The hydrogels contain a7:3ratio of PEG:PBS precursor solution.Mass transfer to microsomes in PEG hydrogels Microsomes must be permanentlyfixed within the hy-drogel or free microsomes could disrupt any analysis of products.PEG hydrogels containingfluorescently tagged nanoparticles the same size as microsomes were moni-tored for2.5hours to insure that no particles leached into the surroundingfluid.In Figure3,thefluorescent inten-sity of the hydrogels is shown as a function of time.No substantial decrease influorescent intensity was shown, indicating that no particles leached from the hydrogels.To substantiate this result and to verify that drug candidates could reach microsomes entrapped in a hydrogel matrix, the mesh size of the gels was calculated.Additionally,the mesh size of the gel can be used to find the effective diffusivity(Peppas et al.,2000)of the potential drug;the effective diffusivity would allow the incubation time of the microsomes with the drug candi-date to be calculated.Mesh size was estimated by using the Peppas-Merrill equation.Though the Peppas-Merrill equation corrected the Flory-Rehner model(Peppas et al., 2000)for solvated systems,it still contains some limita-tions(Wu et al.,2004).A key limitation precludes the cal-culation of mesh size for polymer networks formed with dilute precursor solutions;in this case,the mesh size can-not be calculated mathematically for hydrogels entrapping hepatocytes.The determination of the mesh size has been deter-mined for other polymer systems(Mellott et al.,2001; Russell et al.,2001;Kim and Peppas,2002;Berger et al., 2003;Wang et al.,2003)by using the Peppas-Merrill equation(Peppas and Merrill,1976)(equation(1)).In the equation,v denotes the volume of the polymer af-ter crosslinking in either the relaxed state(subscript r) and in the swollen state(subscript s).V is the molar vol-ume of the medium,M N is the molecular weight of lin-ear polymer chains polymerized in the same conditions without crosslinks,andχis the Flory-Huggins interaction parameter.1c=2N−(v/V1)ln(1−v2,s)+v2,s+χ1v22,sv2,s(v2,s/v2,r)1/3−12(v2,s/v2,r)(1) N is the number of links between crosslinks,which is calculated by equation(2).In this equation,M r is the average molecular weight of the repeating unit.n=2M cM r(2)Equation(3)is used to determine r,the root mean squared end to end distance in the freely jointed state.In this equa-tion,l is the carbon-carbon bond length(1.54angstroms) and n is obtained from equation(2).(r2)1/2=l√n(3) Equation(4)is used to calculate the root mean square end to end distance of the polymer chain in the unperturbed state,where C n is the Flory characteristic ratio or rigidity factor of polymer.r o1/2=C n(r)1/2(4)The above value can be used to calculate the mesh size (ξ)in equation(5).ξ=υ−1/32,sr2o1/2(5)Microreactor Microfluidic Systems with Human Microsomes and Hepatocytes121Fig.1.Microsomes with lipids stained with DiOC(a)unfiltered microsome mixture originally purchased from CellzDirect at10×(b) Filtered microsome mixture at10×(c)Filtered microsome mixture at 40×magnification.Fig.2.Microsomes entrapped in PEG hydrogel microstructures(2.5×magnification).To calculate mesh size,hydrogel spheres were fabri-cated with and without nanoparticles.They are investi-gated in the relaxed,hydrated,and dry states.The av-erage mesh size was found to be11.4±1.6Angstroms (standard deviation,n=22)for a precursor solution of 700µL of PEG,300µL of water,10µL of Darocur 1173,and nanoparticles.The average mesh size of hy-drogels without nanoparticles was10.0±2.5(standard deviation,n=71)for a precursor solution of700µL of PEG,300µL of water,and10µL of Darocur1173. There is no significant difference between these two val-ues,indicating that100nm microsomes will notleach Fig.3.Leaching of the100nmfluorescently tagged particles from a hydrogel over time.122Zguris etal.Fig.4.Investigation of activity of microsomes of the average values between the wavelengths of 468.5±2.5nm (A)product emission of microsomes in solution over time and (B)product emission of microsomes in PEG sphere microstructures over time.from the gel and that the inclusion of nanoparticles in the hydrogels does not significantly alter hydrogel mesh size.Cytochrome P450activityThe cytochrome P450activity of microsomes in solu-tion was compared to the activity of the microsomes entrapped in the hydrogel microstructures,to ensure that encapsulation did not disrupt enzymatic activity.As shown in Figures 4(A)and (B),microsomes en-trapped in PEG maintained cytochrome P450activity de-spite undergoing the gelation process.The EFC emission peak increased over time in both microsomes in solution and in hydrogel spheres;no such increase was notice-able in a control sample containing only PEG spheres (Figure 4(C)).Similar experiments were conducted to determine the cytochrome P450activity of hepatocytes both in solution and in spheres (Figures 5(A)and (B)).These graphs show that for the same activity a larger amount ofcellsFig.5.Cytochrome P450activity of hepatocytes in free solution (A)and in PEG hydrogels (B)expressed in counts/second.or total protein are needed.This is due to the concen-trated nature of cytochrome P450present in microsomes and the lack of competitive pathways (Brandon,Raap et al.,2003).This indicates that the microreactor can be tai-lored to the needs of the user whether they want phase I metabolites (microsomes)or a combination of Phase I &II (hepatocytes).Fabrication of microreactors within PDMS microchannelsTo illustrate the feasibility of placing microsome or hep-atocyte microreactors within microfluidic channels for easy sample delivery,we generated arrays microsome and hepatocyte microreactors in microfluidic networks.Poly-mer precursor solutions containing either microsomes or hepatocytes were placed in the microfluidic networks.A chrome mask was positioned over the reactor chamber to generate patterns of hydrogels within the microfluidic channel.Arrays of microsomes are shown in Figure 6,while hepatocytes are shown in Figure 7.Microreactor Microfluidic Systems with Human Microsomes and Hepatocytes123(A)Fig.6.Images of microsomes in microfluidic channels.(A)Schematic drawing of hydrogel structures within in the microfluidic chamber.(B)2.5×Fluorescent image of the channel with hydrogel structures with microsomes stained with DIOC lipid stain.(C)20×Fluorescent image of one hydrogel structure in the channel with microsomes stained with DIOClipid.(A)(C)Fig.7.Array of hydrogels containing hepatocytes:(A)schematic drawing,(B)fluorescent,and (C)an enlarged image of one array element.124Zguris et al.ConclusionWe have demonstrated the ability to entrap microsomes in a poly(ethylene)glycol hydrogel microstructures without loss of activity during the gelation process.The activ-ity of cytochrome P450in microsomes in PEG structures was compared with the activity of hepatocytes in simi-lar microstructures.It was shown that fewer microsomes are needed than hepatocytes to generate the same level of activity in this system.Hydrogel arrays containing mi-crosomes and hepatocytes were created in microfluidic channels for easy sample delivery.Additionally,these de-vices can be coupled to mass spectrometers to create a device that requires little of the operator.Previously it has been shown that microfluidic devices have been cou-pled to MALDI-TOF MS(Brivio et al.,2002;Gustafsson et al.,2004)and electrospray ionization-mass spectrome-try(Kim and Knapp,2001;Chiou et al.,2002;Benetton et al.,2003;LeGac et al.,2004),which are both used to detect metabolite products currently. AcknowledgmentWe thank NASA(contract BIOTECH-01-0023-0131)for their kind support.ReferencesN.A.Alcantar,E.S.Aydil et al.,“Polyethylene glycol-coated biocom-patible surfaces,”J Biomed Mater Res51(3),343–351(2000).S.Benetton,J.Kameoka et al.,“Chip-based P450drug metabolism cou-pled to electrospray ionization-mass spectrometry detection,”Analyt-ical Chemistry:A-G.(2003).J.Berger,M.Reist et al.,“Structure and interactions in covalently and ionically crosslinked chitosan hydrogels for biomedical applications,”European Journal of Pharmaceutics and Biopharmaceutics(57),19–34(2003).E.F.A.Brandon,C.D.Raap et al.,“An update on in vitro test methods in human hepatic drug biotransformation research:Pros and cons.”Toxicology and Applied Pharmacology(189),233–246(2003).E.F.A.Brandon,C.D.Raap,I.B.Meijerman,H.Jos,and Jan H.M. Schellens,“An update on in vitro test methods in human hepatic drug biotransformation research:Pros and cons,”Toxicology and Applied Pharmacology189,233–246(2003).M.Brivio,R.H.Fokkens et al.,“Integrated microfluidic systems enabling (Bio)chemical reactions with on-line MALDI-TOF mass spectrome-try,”Anal Chem74(16),3972–3976(2002).J.A.Burdick and K.S.Anseth,“Photoencapsulation of osteoblasts in injectable RGD-modified PEG hydrogels for bone tissue engineering,”Biomaterials23(22),4315–4323(2002).J.T.Buters,C.D.Schiller et al.,“A highly sensitive tool for the assay of cyctochrome P450enzyme activity in rat,dog and man,”Biochemical Pharmacology46(9),1577–1584(1993).N.Chauret,N.Tremblay et al.,“Description of a96-well plate assay to measure cytochrome p4503a inhibition in human liver microsomesusing a selectivefluorescent probe,”Analytical Biochemistry276, 215–226(1999).C.-H.Chiou,G.-B.Lee et al.,“Micro devices integrated with microchan-nels and electrospray nozzles using PDMS casting techniques,”Sen-sors and Actuators B86,280–286(2002).P.R.Contag,“Whole-animal cellular and molecular imaging to acceler-ate drug development,”Drug Discovery Today7(10),555–562(2002).C.L.Crespi,ler et al.,“Microtiter plate assays for inhibition of human,drug-metabolizing cyctochromes P450,”Analytical Biochem-istry248,188–190(1997).G.M.Cruise,D.S.Scharp et al.,“Characterization of permeability and network structure of interfacially photopolymerized poly(ethylene glycol)diacrylate hydrogels,”Biomaterials19(14),1287–1294 (1998).I.A.M.DeGraaf,C.E.Van Meijeren et al.,“Comparison of in vitro prepa-rations for semi-quantitative prediction of in vivo drug metabolism,”Drug Metabolism and Disposition30(10),1129–1136(2003).J.G.DeLuca,G.R.Dysart et al.,“A direct,highly sensitive assay for cytochrome P-450catalyzed O-Deethylation using a novel coumarin analog,”Biochemical Pharmacology37(9),1731–1739(1988).J.Elisseeff,W.McIntosh et al.,“Photoencapsulation of chondrocytes in poly(ethylene oxide)-based semi-interpenetrating networks,”J Biomed Mater Res51(2),164–171(2000).M.Gustafsson,D.Hirschberg et al.,“Integrated sample prepartation and MALDI mass spectrometry on a microfluidic compact disk,”Anal Chem76,345–350(2004).J.Hodgson,“ADMET—Turning chemicals into drugs,”Nature Biotech-nology19,722–726(2001).J.Honiger,P.Balladur et al.,“Permeability and biocompatibility of a new hydrogel used for encapsulation of hepatocytes,”Biomaterials 16(10),753–759(1995).U.M.Kent,L.Pascual et al.,“Mechanistic studies with N-benzyl-1-aminobenzotriazole-inactivated CYP2B1:Differential effects on the metabolism of7-ethoxy-4-(trifluoromethyl)coumarin,testosterone and benzphetamine,”Archives of Biochemistry and Biophysics423, 277–287(2004).K.K.Khan,“Homotropic versus heterotopic cooperativity of cytochrome P450eryF:A substrate oxidation and spectral titration study,”Drug Metabolism and Disposition31(4),356–359(2003).B.Kim and N.A.Peppas,“Synthesis and characterization of pH-sensitive glycopolymer for oral drug delivery systems,”J.Biomater.Sci.Poly-mer Edn13(11),1271–1281(2002).J.-S.Kim and D.R.Knapp,“Microfabricated PDMS multichannel emit-ter for electrospray ionization mass spectrometry,”J Am Soc Mass Spectrom12,463–469(2001).W.G.Koh,L.J.Itle et al.,“Molding of hydrogel microstructures to cre-ate multiphenotype cell microarrays,”Anal Chem75(21),5783–5789 (2003).W.G.Koh, A.Revzin et al.,“Poly(ethylene glycol)hydrogel mi-crostructures encapsulating living cells,”Langmuir18(7),2459–2462 (2002).S.LeGac,J.Carlier et al.,“Monoliths for microfluidic devices in pro-teomics,”Journal of Chromatography B808,3–14(2004).V.Liu and S.N.Bhatia,“Three-dimensional photopatterning of hydro-gels containing living cells,”Biomedical Microdevices4(4),257–266 (2002).V.A.Liu,W.E.Jastromb et al.,“Engineering protein and cell adhesivity using PEO-terminated triblock polymers,”J Biomed Mater Res60(1), 126–134(2002).M.B.,Mellott,K.Searcy et al.,“Release of protein from highly cross-linked hydrogels of poly(ethylene glycol)diacrylate fabricated by UV polymerization,”Biomaterials22,929–941(2001).S.Myers and A.Baker”Drug discovery—an operating model for a new era,”Nature Biotechnology19,727–730(2001).。