Specific heat study of spin-structural change in pyrochlore Nd$_2$Mo$_2$O$_7$
质子交换膜燃料电池-磷酸掺杂PBI高温质子交换膜传导机理研究
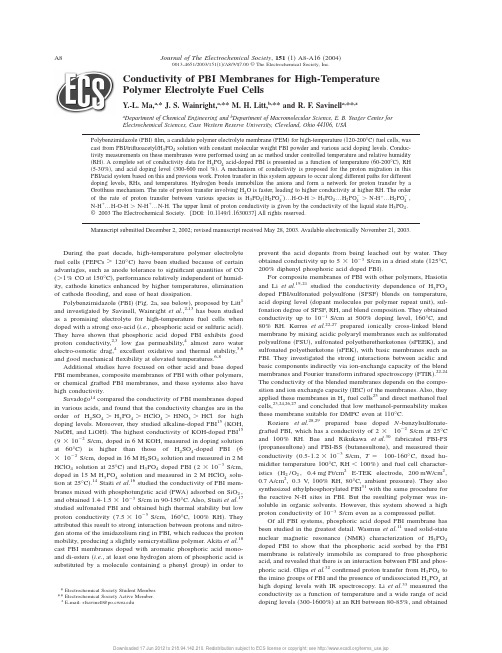
Conductivity of PBI Membranes for High-TemperaturePolymer Electrolyte Fuel CellsY.-L.Ma,a,*J.S.Wainright,a,**M.H.Litt,b,**and R.F.Savinell a,**,za Department of Chemical Engineering andb Department of Macromolecular Science,E.B.Yeager Center forElectrochemical Sciences,Case Western Reserve University,Cleveland,Ohio44106,USAPolybenzimidazole͑PBI͒film,a candidate polymer electrolyte membrane͑PEM͒for high-temperature͑120-200°C͒fuel cells,was cast from PBI/trifluoacetyl/H3PO4solution with constant molecular weight PBI powder and various acid doping levels.Conduc-tivity measurements on these membranes were performed using an ac method under controlled temperature and relative humidity ͑RH͒.A complete set of conductivity data for H3PO4acid-doped PBI is presented as a function of temperature͑60-200°C͒,RH ͑5-30%͒,and acid doping level͑300-600mol%͒.A mechanism of conductivity is proposed for the proton migration in this PBI/acid system based on this and previous work.Proton transfer in this system appears to occur along different paths for different doping levels,RHs,and temperatures.Hydrogen bonds immobilize the anions and form a network for proton transfer by a Grotthuss mechanism.The rate of proton transfer involving H2O is faster,leading to higher conductivity at higher RH.The order of the rate of proton transfer between various species is H3PO4(H2PO4Ϫ)...H-O-HϾH3PO4...H2PO4ϪϾN-Hϩ...H2PO4Ϫ, N-Hϩ...H-O-HϾN-Hϩ...N-H.The upper limit of proton conductivity is given by the conductivity of the liquid state H3PO4.©2003The Electrochemical Society.͓DOI:10.1149/1.1630037͔All rights reserved.Manuscript submitted December2,2002;revised manuscript received May28,2003.Available electronically November21,2003.During the past decade,high-temperature polymer electrolyte fuel cells(PEFCsϾ120°C)have been studied because of certain advantages,such as anode tolerance to significant quantities of CO (Ͼ1%CO at150°C͒,performance relatively independent of humid-ity,cathode kinetics enhanced by higher temperatures,elimination of cathodeflooding,and ease of heat dissipation.Polybenzimidazole͑PBI͒͑Fig.2a,see below͒,proposed by Litt1 and investigated by Savinell,Wainright et al.,2-13has been studied as a promising electrolyte for high-temperature fuel cells when doped with a strong oxo-acid͑i.e.,phosphoric acid or sulfuric acid͒. They have shown that phosphoric acid doped PBI exhibits good proton conductivity,2,3low gas permeability,4almost zero water electro-osmotic drag,4excellent oxidative and thermal stability,5,6 and good mechanicalflexibility at elevated temperatures.6-8 Additional studies have focused on other acid and base doped PBI membranes,composite membranes of PBI with other polymers, or chemical grafted PBI membranes,and these systems also have high conductivity.Savadogo14compared the conductivity of PBI membranes doped in various acids,and found that the conductivity changes are in the order of H2SO4ϾH3PO4ϾHClO4ϾHNO3ϾHCl for high doping levels.Moreover,they studied alkaline-doped PBI15͑KOH,NaOH,and LiOH͒.The highest conductivity of KOH-doped PBI15 (9ϫ10Ϫ2S/cm,doped in6M KOH,measured in doping solution at60°C͒is higher than those of H2SO4-doped PBI(6ϫ10Ϫ2S/cm,doped in16M H2SO4solution and measured in2M HClO4solution at25°C͒and H3PO4doped PBI(2ϫ10Ϫ3S/cm, doped in15M H3PO4solution and measured in2M HClO4solu-tion at25°C͒.14Staiti et al.16studied the conductivity of PBI mem-branes mixed with phosphotungstic acid͑PWA͒adsorbed on SiO2, and obtained1.4-1.5ϫ10Ϫ3S/cm in90-150°C.Also,Staiti et al.17 studied sulfonated PBI and obtained high thermal stability but low proton conductivity(7.5ϫ10Ϫ5S/cm,160°C,100%RH͒.They attributed this result to strong interaction between protons and nitro-gen atoms of the imidazolium ring in PBI,which reduces the proton mobility,producing a slightly semicrystalline polymer.Akita et al.18 cast PBI membranes doped with aromatic phosphoric acid mono-and di-esters͑i.e.,at least one hydrogen atom of phosphoric acid is substituted by a molecule containing a phenyl group͒in order to prevent the acid dopants from being leached out by water.They obtained conductivity up to5ϫ10Ϫ3S/cm in a dried state͑125°C, 200%diphenyl phosphoric acid doped PBI͒.For composite membranes of PBI with other polymers,Hasiotis and Li et al.19-21studied the conductivity dependence of H3PO4 doped PBI/sulfonated polysulfone͑SPSF͒blends on temperature, acid doping level͑dopant molecules per polymer repeat unit͒,sul-fonation degree of SPSF,RH,and blend composition.They obtained conductivity up to10Ϫ1S/cm at500%doping level,160°C,and 80%RH.Kerres et al.22-27prepared ionically cross-linked blend membrane by mixing acidic polyaryl membranes such as sulfonated polysulfone͑PSU͒,sulfonated polyetheretherketones͑sPEEK͒,and sulfonated polyetherketone͑sPEK͒,with basic membranes such as PBI.They investigated the strong interactions between acidic and basic components indirectly via ion-exchange capacity of the blend membranes and Fourier transform infrared spectroscopy͑FTIR͒.22-24 The conductivity of the blended membranes depends on the compo-sition and ion exchange capacity͑IEC͒of the membranes.Also,they applied these membranes in H2fuel cells25and direct methanol fuel cells,23,24,26,27and concluded that low methanol-permeability makes these membrane suitable for DMFC even at110°C.Roziere et al.28,29prepared base doped N-benzylsulfonate-grafted PBI,which has a conductivity of2ϫ10Ϫ2S/cm at25°C and100%RH.Bae and Rikukawa et al.30fabricated PBI-PS ͑propanesultone͒and PBI-BS͑butanesultone͒,and measured theirconductivity(0.5-1.2ϫ10Ϫ3S/cm,Tϭ100-160°C,fixed hu-midifier temperature100°C,RHϽ100%)and fuel cell character-istics(H2/O2,0.4mg Pt/cm2E-TEK electrode,200mW/cm2, 0.7A/cm2,0.3V,100%RH,80°C,ambient pressure͒.They also synthesized ethylphosphorylated PBI31with the same procedure for the reactive N-H sites in PBI.But the resulting polymer was in-soluble in organic solvents.However,this system showed a high proton conductivity of10Ϫ3S/cm even as a compressed pellet.Of all PBI systems,phosphoric acid doped PBI membrane has been studied in the greatest detail.Wasmus et al.11used solid-state nuclear magnetic resonance͑NMR͒characterization of H3PO4 doped PBI to show that the phosphoric acid sorbed by the PBI membrane is relatively immobile as compared to free phosphoric acid,and revealed that there is an interaction between PBI and phos-phoric acid.Glipa et al.32confirmed proton transfer from H3PO4to the imino groups of PBI and the presence of undissociated H3PO4at high doping levels with IR spectroscopy.Li et al.33measured the conductivity as a function of temperature and a wide range of acid doping levels͑300-1600%͒at an RH between80-85%,and obtained*Electrochemical Society Student Member. **Electrochemical Society Active Member. z E-mail:rfsavinell@ 0013-4651/2003/151͑1͒/A8/9/$7.00©The Electrochemical Society,Inc.a conductivity of 4.6ϫ10Ϫ2S/cm at 165°C.They suggested a use-ful H 3PO 4doping level between 350-750%,considering both con-ductivity and mechanical strength.Kawahara et al.34studied PBI complexes with strong acids (H 3PO 4,H 2SO 4,CH 3SO 3H,or C 2H 5SO 3H).They immersed the PBI membranes into a mixed so-lution of strong acid and methanol,and the highest doping level of 2.9mol/repeat unit was observed for PBI/H 3PO 4complexes.The FTIR data 34indicated that acid molecules,except H 3PO 4,protonate the N atom in the imidazole ring.They concluded that H 3PO 4does not protonate imidazole groups of PBI but interacts by hydrogenbonding between the OH and N groups.The presence of HPO 42Ϫand H 2PO 4Ϫanions,based on FTIR,implies that the proton conduction occurs according to the Grotthuss mechanism.The conductivity of the anhydrous PBI/H 3PO 4complex reached 10Ϫ5S/cm at 160°C.The influences of pressure on the conductivity and activation volume at various temperatures were also studied.Fontanella et al.3measured the isobar conductivity data of 60050,75°C.Based on they proposed that by segmental motions of the polymer.Bouchet et al.35proposed an activated mechanism ͑Grotthuss mechanism ͒for the proton migration from conductivity data as a function of temperature ͑30-90°C ͒and isostatic pressure ͑1-4000bars ͒,and determined the activation volume ⌬V *(4-10cm 3/mol),⌬H *͑0.6-1.1eV ͒,and ⌬S *͓40-190J/͑mol K ͔͒from isobar and isothermal conductivity data.Based on IR spectroscopic study of PBI-acid complexes,36a microscopic model was developed,sug-gesting proton transfer from one imide site to another in which the anionic species participate by Grotthuss mechanism.The nitrogen of the imide is protonated by the acids.The anions are linked to the polymer by rather strong hydrogen bonding.Pu et al.37proposed that proton transport in phosphoric acid blended PBI is the conse-quences of the two contributions,one is based on rapid proton ex-change ͑hopping ͒via hydrogen bonds between solvent molecules,which could be the phosphate,N -heterocycles of PBI and water molecules;and the other is based on the self-diffusion of phosphate moieties and water molecules ͑vehicle mechanism ͒.They studied the temperature and pressure dependence of the conductivity of 180-380%H 3PO 4doped PBI ͑activation volume of 3.8-6cm 3/mol).From their conductivity data,the activation energy ͑70-85kJ/mol ͒,obtained using the Arrhenius equation,is approximately independent of acid concentration.Although the proton conduction mechanisms proposed by the various authors discussed here are different,the values of the activation volume reported are consistent.They all showed that activation volume decreases with the increasing tem-perature.At 75°C,Fontanella 3even obtained a negative activation volume for 85%phosphoric acid.This result suggests that only a small charge carrier,a solvent-free proton,transports through the membrane.The results of Hittorf measurements by Weng 13to mea-sure the transference number of 500%H 3PO 4-doped PBI at 150°C are also compatible with the above results.They obtained an anion (H 2PO 4Ϫ)transference number of 0.01-0.02,and a proton transfer-ence number of 0.98-0.99,indicating that the vast majority of the charge is carried by the proton in the acid doped PBI membranes.All of the above work was done based on PBI membranes,but the results vary from author to author due to various preparation processes for the membranes and various testing conditions.Since PBI membranes were proposed for utilization in a high-temperature fuel cell,it is important that all of the measurements be performed at conditions similar to those of an operating fuel cell.Phosphoric acid doped PBI membranes have generally been pre-pared by three methods,8(i )cast from a solution of polymer in NaOH/ethanol solution under N 2environment,and washed by water until pH 7,then doped by immersion in phosphoric acid solution;(ii )cast from a solution of 3-5%polymer in N ,N -dimethylacetamide ͑DMAc ͒with 1-2%LiCl,evaporated DMAc,and washed by boiling water to remove the LiCl,then dopedby immersion in phosphoric acid solution.The final acid loading for method (i )and (ii )is calculated from the weight difference of the membranes before and after the immersion.(iii )PBI and acid di-rectly cast from a solution of PBI and H 3PO 4in a suitable solvent such as trifluoroacetic acid,TFA.The solvent is evaporated and the film is ready for use.Most of membranes reported in the literatures 2-7,9-11,32,33,36were prepared by the DMAc method.The TFA method was used in this work as it allows for direct control of the acid doping level.Even though the doping level is similar,the properties of the film formed by the various methods are substantially different.Films cast using the DMAc method are normally stronger and tougher than those cast from TFA.The TFA films require a polymer of higher inherent viscosity ͑IV ͒in order to generate films of reasonable strength.7͑I.V .is a measurement often used to characterize the mo-lecular weight of a polymer,the relationship of the I.V .and the weight-average molecular weight of PBI is shown in Ref.38͒.Com-Figure 1.Weight loss of PBI on TGA,N 2flowing,ramping rate °C/min,630%H 3PO 4doping level.mercial PBI powder can be extracted with DMAc to increase aver-age molecular weight for good mechanical properties.The TFA cast film has much more crystallinity as comparable to a similarly acid loaded DMAc castfilm,and the surface texture is different.The TFA films are more rubbery and softer,and the conductivity of TFA cast film is higher.7Although phosphoric acid doped PBI has been studied for ten years as a polymer electrolyte membrane for fuel cells,the conduc-tivity mechanism is still unclear.In this work,a complete set of conductivity data based on phosphoric acid doped PBI were ob-tained as a function of temperature,RH,and doping level under the conditions closer to anticipated fuel cell operation conditions.The paper also presents an attempt to better understand the nature of the proton conduction in the system based on previous reports and re-sults of this work.ExperimentalPreparation of acid doped PBI membranes.—High molecular weight͑HMw͒PBI with I.V.equal to1.2͑see below for I.V.mea-surement,this I.V.corresponds to the Mw of230,000based on Ref. 38͒was prepared by extracting low molecular weight components from PBI powder͑supplied by Hoechst-Celanese͒with DMAc͑see Ref.7for details of the extraction͒.The HMw PBI was dissolved in TFA and then85wt%H3PO4was added to prepare solutions cor-responding to different doping levels.Films were cast with the PBI/TFA/H3PO4solution on a glass plate with a Gardner knife and dry N2blowing over the surface.The I.V.is found by measuring the viscosity of a0.5g PBI/100 mL solution of the polymer dissolved in a solvent͑96wt%sulfuric acid for PBI͒at30°C.The equation for calculating the I.V.is given belowI.V.ϭlog e͓flow time of the solution/flow time of the solvent͔solution concentration in g/dlwhere,flow time is measured in a Ubbelohde calibrated viscometer tube from Fisher Scientific.Thermogravimetric analysis(TGA)measurement.—The weight loss and gain of acid-doped PBI samples,showing dehydration and hydration,respectively,was recorded by TGA measurement͑TGA2950thermogravimetric analyzer,TA Instruments͒.First,the sample weight was measured from30to200°C with dry N2flowing,at aramping rate of1°C/min and then held at200°C for4h to check thechange of the weight with time.Then,the sample was put into astainless steel vessel with controlled RH and temperature.The en-vironment for recovering the weight is the same as that for conduc-tivity measurement͑see next section͒.The vessel was kept in an oven at100°C,where it was held isothermally at RHϭ10%for4h or longer to rehydrate the sample.Several cycles were run tocheck the reversibility with time of the weight loss and weight gain.Conductivity.—Conductivity measurements were made using thefour-probe techniqueas reported elsewhere.2,39The apparatus wascontained within a sealed stainless steel vessel,which was placedinside an oven and connected to a vacuum pump.The RH at varioustemperatures was obtained by injecting deionized water into thesealed vacuumed chamber after40min under vacuum.The pressurein the chamber is not larger than1atm͓except for160°C,RH30%͑1.58atm͒;200°C,RH10%͑1.22atm͔͒.In this manner the tem-perature,pressure,and water vapor pressure in contact with thesample could be controlled.AC impedance measurements between1Hz and20kHz were made using a Solartron1287/1260potentiostatand frequency response analyzer using Zplot software.Films wereheld at the desired conditions for4h to ensure a steady state beforemeasurements were taken.The conductivity of H3PO4acid-doped PBI membrane is knownto vary with RH,temperature,and doping level.2The temperatureswere controlled from60to200°C,RH from5to30%,doping levelat300,420,558,and630%,using PBI with an I.V.within the rangeof1.20-1.22.These ranges cover the conditions anticipated for anoperational fuel cell.Results and DiscussionTGA results.—Previous TGA measurements7indicate that thereare two dramatic weight loss ranges with increasing temperature,one appears at40-100°C caused by the loss of free water,and theother appears at130-200°C caused by the loss of water produced byaciddimerization Figure2.Chemical structure of͑a͒po-lybenzimidazole͑PBI͒,͑b͒H3PO4proto-nated PBI,͑c͒proton transfer along acid-BI-acid,͑d͒proton transfer along acid-acid,͑e͒proton transfer along acid-H2O.2H3PO4→H4P2O7ϩH2O↑͓1͔Similarly,two dramatic weight loss ranges were shown in Fig. 1b,one͑100-96%͒represents the loss of the free water͑4-5wt% loss͒within30-100°C;the other͑96-89%͒represents the loss of water mainly produced by acid dimerization as Reaction1͑6-7wt %loss͒.As the sample was held at200°C for4h,there is another slight weight loss͑89-87%͒,which is the loss of water produced by continuance of Reaction1͑Fig.1b͒or the formation of triphospho-ric acid͑Reaction2͒,or even the formation of higher polyphospho-ric acidH4P2O7ϩH3PO4→H5P3O10ϩH2O↑͓2͔In630%phosphoric acid doped PBI membrane͑66.7wt%phos-phoric acid and33.3wt%PBI͒,two phosphoric acid molecules can protonate the N atom in the imidazole group of PBI to form H2PO4Ϫ͑Fig.2b͒.The excess acids act as concentrated phosphoric acid so-lution held by the PBI matrix.When equilibrated at100°C and10% RH,based on the water vapor pressure data of phosphoric acid ͑Table I͒,the concentration of the phosphoric acid is about88.7wt %.Thus,the free water in the membrane is about5.0wt%.This is consistent with thefirst dramatic weight loss.Between120and 200°C,6.1wt%water is formed if all of phosphoric acids dehydrate to pyrophosphoric acid via Reaction1.It is possible that further dehydration to triphosphoric acid at a high temperature and very dry condition,9.2wt%water is formed if all of phosphoric acids de-hydrate to triphosphoric acid͑Reaction2͒.However,it is well known that several phosphorus species always coexist in concen-trated phosphoric acid solution.Therefore,the second weight loss can be considered as the dehydration of H3PO4.The third one͑Fig. 1a͒is due to the further slow dehydration as held at200°C.This weight loss can be recovered by equilibrating the sample in the closed cell at controlled temperature͑100°C͒and RH͑10%͒. The recovery reaches above99%after each cycle͑Table II͒.This indicates that the weight loss is due to the dehydration,and the processes of dehydration and hydration are reversible in this tem-perature range.Conductivity of acid-doped PBI membrane.—Temperature dependence of conductivity of acid-doped PBI membrane.—In Fig. 3to5,the variations in conductivities with temperature at constant RH are shown for various doping levels.The conductivities increase with increasing temperature and RH.The temperature dependence of conductivity could be accurately described by an Arrhenius equa-tion͓Tϭ0exp(ϪEa/RT)͔͑Fig.3-5͒.The activation energy (Ea)and the pre-exponential factor(0)of conductivity were de-rived͑listed in Table III and IV͒.The data of RHϭ0%was mea-sured before injecting water.The condition of P H2Oϭ0(RHϭ0%)cannot be reliably obtained in H3PO4system as pyrophos-phoric acid is formed.The abnormal behavior of conductivity at RHϭ0%is discussed later.Doping level dependence of conductivity.—It has been shown that the acid doping level affects not only the conductivity,but also the mechanical properties of the membrane,such as modulus and elongation.8At low doping levels,the modulus rises with the acid content because a crystalline phase develops,and then the mem-brane becomes softer as more phosphoric acid(Ͼ300%)is added. In this work,membranes with a reasonable doping level͑300-630%͒were used.Li et al.33also suggest a useful H3PO4doping levelbetween300-750%,taking into consideration both factors of con-ductivity and mechanical strength.From Fig.6,it can be seen that the conductivity increases withthe doping level for a given temperature and constant RH.The ac-tivation energy decreases with increasing doping level͑Table III͒.The activation energy at high doping levels͑630%͒is close to that of H3PO4͑concentrated aqueous solution͒͑Table V͒.42,43These data indicate that proton movement becomes easier at higher doping lev-els.This can be expected as there is excess phosphoric acid in themembrane after maximum protonation of PBI by acid.The excess H3PO4acid works similarly to concentrated H3PO4solution.This is consistent with the conclusion of Glipa et al.32and Bouchet36based on IR analysis that suggests the maximum degree of protonation of PBI is reached when there are two H3PO4per polymer repeat units (xϭ2)and that in the highly doped systems,even in the presence of water,undissociated phosphoric acid is in equilibrium with ionic species,and the mechanism of proton transfer should be expected to be close to that of concentrated phosphoric acid solutions.Based on previous results,32,36at lower doping levels(x ϭ0-2,before the maximum protonation is reached͒,H3PO4proto-nates the nitrogen atom of the imino group of the PBI structure͑Fig. 2b͒.H2PO4Ϫseems to be the predominant anion over the entire acid concentration range with the appearance of HPO42Ϫfor small values of x(Ͻ0.2)and H3PO4for the highest values of x(Ͼ1.2).Proton exchange mainly happens between protonated and nonprotonated imino nitrogen groups(N-Hϩ...N-H)͑Fig.2b͒on neighboring poly-mer chains,considering the distance of N atoms in one repeat unit of PBI.The T g increases with increasing concentration of H3PO4in the polymer.Membranes with phosphoric acid in the range of doping levels of0ϽxϽ2have too low a conductivity to be used as electrolytes in fuel cells.After the maximum degree of protonation is reached(xϭ2), excess acid exists in the membranes.However,for xϽ3,there is not much excess H3PO4,and the average P-P distance is larger than the N-N distance and too large to allow proton jumps between the anions.36Therefore,it can be suggested that the proton conductivity in acid-doped PBI in this doping range would rather result from a cooperative motion of two protons along the polymer-anion chain by the Grotthuss mechanism͑Fig.2c͒.With increasing doping level,there is more excess H3PO4.Now, protons migrate along the mixed H2PO4Ϫ...H3PO4and N-Hϩ...H2PO4Ϫanionic chains by successive proton transfer and anion reorientation steps͑Fig.2d͒.Table I.The relationship of neutral H3PO4species concentration…wt%…with temperature and RH.40,41Temperature͑°C͒6080100130140150170H3PO4•x H2O RH5%93.9594.0794.5594.980.25-0.5 10%86.4787.4389.9889.9790.77910.5-0.75 20%79.6280.6481.5683.6883.6684.391-1.25 30%74.2475.1875.9378.17 1.5-1.75Table II.630%H3PO4doped PBI sample weight variation withTGA cycling.Cycle no.Sample weight͑mg͒Weight,initialand gainedWeight whenTϭ100°CWeight whenTϭ200°CWeight after4h at200°C155.04652.6248.82347.653 254.5352.348.57347.456 354.15851.8448.17747.243 453.65851.6948.07147.008For x ϭ4.2-6,the further addition of H 3PO 4leads to excess acid in the polymer,which has an NMR spectrum very similar to that given by pure phosphoric acid.32Proton migration happensmainly along the acid and anion chain (H 2PO 4Ϫ–H ϩ...H 2PO 4Ϫ)͑Fig.2d ͒or the acid and H 2O chain ͑Fig.2e ͒depending on the water content.Conductivity is increasing with doping level.In this case,the conductivity mechanism is more like a concentrated H 3PO 4so-lution ͑Grotthuss mechanism ͒.With these doping levels,the mem-branes have high conductivity and are the most suitable for fuel cells.However,the conductivity is still one order lower than that of the concentrated acid,even 100wt %H 3PO 4.Figure 7compares the conductivities of acid-doped PBI with different doping levels,phos-phoric acid aqueous solution equilibrium at RH 20%43͑concentra-tion is based on data of Table I ͒,and pure phosphoric acid.43Thereasons of the lower conductivity can be considered from the con-ductivity mechanism.As we mentioned above,for the polymer-acid system,the conductivity is mainly attributed from H 3PO 4domains,where proton transfer follows the proton jump mechanism ͑Grot-thuss mechanism ͒.The mechanism of proton jump conduction con-sists of two steps,the first is the orientation of the solvent molecules so that hydrogen bonds are formed through which a proton jump may occur.The second step,which is generally considered to be the fast process in water and probably involves a proton transfer be-tween proton donor and proton acceptor,is the proton movement within the hydrogen bridge.In this system,H 3PO 4molecules are bonded to PBI chains by H bond when protonate PBI.Even excess acids in a PBI matrix,hin-dered by PBI chains,are very difficult to rotate and move,compared to excess acids in pure phosphoric acid.PBI chains act as a frame for immobilizing phosphoric acid molecules.Also,the PBI-acid sys-tem is much more viscous than pure acid so that the mobility is much less than the latter.Furthermore,the acid domain is not so continuous as in pure phosphoric acid even if the doping level is very high.It is possible that the PBI chain interrupts the H bond for proton transfer between phosphoric molecules,causing lower con-ductivity.Actually,even when small molecules such as imidazole 44and 1-methyl imidazole 44KH 2PO 4,45BF 3,46K 2HPO 4,47and K 2SO 447were added into concentrated phosphoric acid instead of water,the conductivity decreases with the increase of the amount of the small molecules due to increase of viscosity and interruption ofacid-chainFigure 3.Temperature dependence of ionic conductivity of acid-doped PBI,300%dopinglevel.Figure 4.Temperature dependence of ionic conductivity of acid-doped PBI,420%dopinglevel.Figure 5.Temperature dependence of ionic conductivity of acid-doped PBI,600%doping level.Table III.Activation energy of conductivity Ea …kJ Õmol …from Arrhenius equation.T ϭ0exp(ϪEa /RT )Ea ͑kJ/mol ͒RH Doping level 5%10%20%30%300%41Ϯ144Ϯ147Ϯ249Ϯ2T ͑°C ͒range 80-19070-20070-15070-140420%34Ϯ334Ϯ234Ϯ332Ϯ3T ͑°C ͒range 80-16060-18060-15060-150630%28Ϯ228Ϯ226Ϯ224Ϯ2T ͑°C ͒range80-20070-20060-16050-160for proton transfer.Munson and Lazarus48introduced various ioniz-ing solutes other than water such as H2SO4,HClO4,(NH4)2HPO4, NH4HSO4,KH2PO4,LiH2PO4,Mg(H2PO4)2,NH4ClO4, KHSO4,LiClO4into phosphoric acid,and found that these solutes resulted in a linear decrease in the conductivity of the phosphoric acid.The conductivity decrease was also ascribed to a breaking of the H-bonded structure of the phosphoric acid by the ions,which inhibits the formation of the structures necessary for proton jumps.Relative humidity dependence of conductivity.—Figure8shows the RH dependence of the conductivity of acid-doped PBI at con-stant temperature and doping level.The conductivity increases with increase of RH.As reported by Wainright et al.2at a given tempera-ture,an increase of RH leads to higher water content in the electro-lyte,which presumably lowers the viscosity within the membrane, leading to higher mobility and conductivity.As discussed above,the excess H3PO4in the polymer behaves like a concentrated H3PO4solution.PBI molecule can be regarded as a solvent and the equilibrium of the species in the H3PO4/PBI system is derived.Basically,species of H3PO4,H4P2O7,PBI,and H2O exist in this system.The dissociation constants of these species are shown in Table VI.49In pure phosphoric acid͑100wt%͒,self-dissociation of anhy-drous phosphoric acid is represented by the following reactions452H3PO4 H4PO4ϩϩH2PO4Ϫfast͓3͔2H3PO4 H4P2O7ϩH2O↑͓4͔The pyrophosphoric acid H4P2O7is a very strong acid.It has two strong dissociations to H3P2O7Ϫand H2P2O72Ϫ,thus Eq.͑4͒is al-ways written as2H3PO4 H3OϩϩH3P2O7Ϫslow͓5͔Thefirst equilibrium͑Eq.3͒is labeled fast because the conduc-tivity of freshly melted phosphoric acid is high(7.68ϫ10Ϫ2S/cm).The conductivity decreases slowly on standing toan equilibrium value(6.52ϫ10Ϫ2S/cm),the probable mechanism being the slow reaction represented above.45In the studied system conditions(RHϭ5-30%,doping level ϭ300-600%),if one assumes that H3PO4is in equilibrium with the H2O partial pressure above the membrane as in the concentrated H3PO4system,then one can derive the concentration of H3PO4in the membrane based on the data shown in Table I.40,41This is viable since Savinell et al.showed that the water content is controlled by the water vapor activity and is independent of temperature in H3PO4 equilibrated Nafion membrane,50and the amount of water sorbed is consistent with known vapor liquid equilibrium data for phosphoric acid and water.In this concentration range,the equilibrium fraction of H4P2O7is sufficiently small as to be ignored based on the com-position data of concentrated phosphoric acids from Jameson.51 From Table I,the concentration of H3PO4at constant RH is nearly independent of temperature.On the other hand,the neutral acid concentration varies with RH dramatically.Higher RH decreases the concentration of neutral H3PO4and increases the proton conductiv-ity.From the dissociation constant data͑Table VI͒,since H3PO4is the most acidic species and PBI is the most basic species,H3PO4 protonates PBIfirst as follows͑assuming the presence of H4P2O7 can be ignored͒H3PO4ϩPBI H2PO4ϪϩPBI•Hϩ͓6͔The excess H3PO4and H2O have another simultaneous equilibrium H3PO4ϩH2O H2PO4ϪϩH3Oϩ͓7͔The equilibrium constant of Reaction͑6͒can be estimated as fol-lowsFigure 6.Conductivity of phosphoric acid doped PBIfilms,cast from PBI/TFA/H3PO4solution,at Tϭ140°C.Table IV.Pre-exponential factor ln(0)from Arrhenius equation.Tϭ0exp(ϪEa/RT)ln(0)͑S/K cm͒RH Doping level5%10%20%30%300%12.0313.1015.2515.29T͑°C͒range80-19070-20070-15070-140420%11.7111.9812.2111.87T͑°C͒range80-16060-18060-15060-150630%10.4310.7410.419.80T͑°C͒range80-20070-20060-16050-160Table parison of conductivity and activation energy.Ea͑eV͒Ea͑kJ/mol͒͑S/cm͒Nafion420.2221.21 5.00ϫ10Ϫ2͑100%͒H3PO4͑85wt%,80-170°C͒430.148a14.29a0.568͑150°C͒PBI/H3PO4͑This work͒͑630%,50-160°C͒0.29Ϯ0.020.24Ϯ0.0228Ϯ2(RHϭ5%)24Ϯ2(RHϭ30%)4.70ϫ10Ϫ3͑150°C,RHϭ5%)5.90ϫ10Ϫ2͑150°C,RHϭ30%)a Ea isfitted with Arrhenius equation from the data of Ref.43.。
Study of the kinetics of nucleation and growth
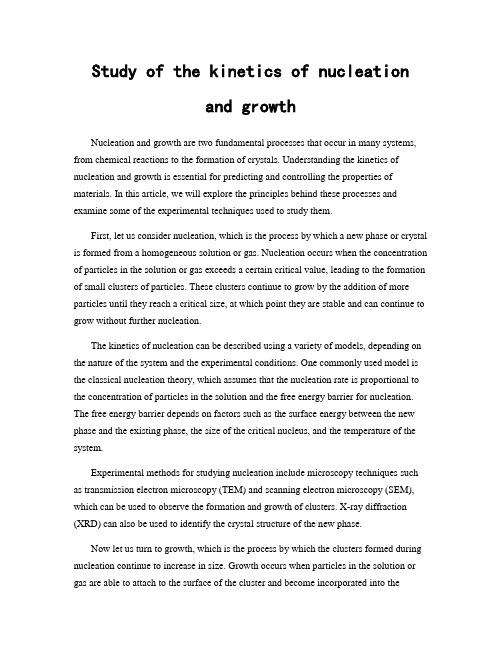
Study of the kinetics of nucleationand growthNucleation and growth are two fundamental processes that occur in many systems, from chemical reactions to the formation of crystals. Understanding the kinetics of nucleation and growth is essential for predicting and controlling the properties of materials. In this article, we will explore the principles behind these processes and examine some of the experimental techniques used to study them.First, let us consider nucleation, which is the process by which a new phase or crystal is formed from a homogeneous solution or gas. Nucleation occurs when the concentration of particles in the solution or gas exceeds a certain critical value, leading to the formation of small clusters of particles. These clusters continue to grow by the addition of more particles until they reach a critical size, at which point they are stable and can continue to grow without further nucleation.The kinetics of nucleation can be described using a variety of models, depending on the nature of the system and the experimental conditions. One commonly used model is the classical nucleation theory, which assumes that the nucleation rate is proportional to the concentration of particles in the solution and the free energy barrier for nucleation. The free energy barrier depends on factors such as the surface energy between the new phase and the existing phase, the size of the critical nucleus, and the temperature of the system.Experimental methods for studying nucleation include microscopy techniques such as transmission electron microscopy (TEM) and scanning electron microscopy (SEM), which can be used to observe the formation and growth of clusters. X-ray diffraction (XRD) can also be used to identify the crystal structure of the new phase.Now let us turn to growth, which is the process by which the clusters formed during nucleation continue to increase in size. Growth occurs when particles in the solution or gas are able to attach to the surface of the cluster and become incorporated into thecrystal lattice. The rate of crystal growth is determined by the concentration of particles in the solution or gas, the surface area of the crystal, and the diffusion coefficient of the particles.The kinetics of growth can be described using the Lifshitz-Slyozov-Wagner (LSW) theory, which assumes that the rate of crystal growth is inversely proportional to the cube of the particle size. This means that smaller particles grow faster than larger particles, leading to a decrease in the overall particle size distribution over time.Experimental methods for studying crystal growth include techniques such as time-resolved XRD, which can be used to monitor the evolution of the crystal structure over time. In situ optical microscopy can also be used to observe the growth of individual crystals in real time.In summary, the study of the kinetics of nucleation and growth is essential for understanding the behavior of materials in a wide range of applications. The principles behind these processes are complex, but can be described using mathematical models such as classical nucleation theory and the LSW theory. A variety of experimental techniques are available to study these processes, including microscopy, XRD, and optical techniques. By combining theoretical models with experimental data, researchers can gain a detailed understanding of the mechanisms behind nucleation and growth, and develop new materials with tailored properties.。
磁学 径向克尔 英文 kerr effect
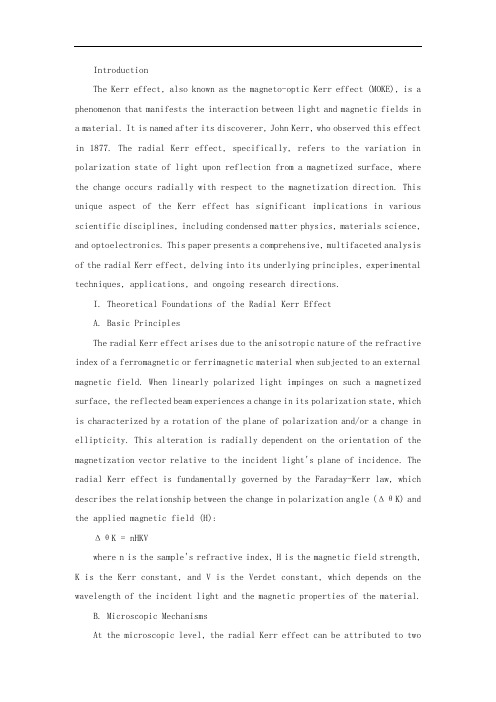
IntroductionThe Kerr effect, also known as the magneto-optic Kerr effect (MOKE), is a phenomenon that manifests the interaction between light and magnetic fields in a material. It is named after its discoverer, John Kerr, who observed this effect in 1877. The radial Kerr effect, specifically, refers to the variation in polarization state of light upon reflection from a magnetized surface, where the change occurs radially with respect to the magnetization direction. This unique aspect of the Kerr effect has significant implications in various scientific disciplines, including condensed matter physics, materials science, and optoelectronics. This paper presents a comprehensive, multifaceted analysis of the radial Kerr effect, delving into its underlying principles, experimental techniques, applications, and ongoing research directions.I. Theoretical Foundations of the Radial Kerr EffectA. Basic PrinciplesThe radial Kerr effect arises due to the anisotropic nature of the refractive index of a ferromagnetic or ferrimagnetic material when subjected to an external magnetic field. When linearly polarized light impinges on such a magnetized surface, the reflected beam experiences a change in its polarization state, which is characterized by a rotation of the plane of polarization and/or a change in ellipticity. This alteration is radially dependent on the orientation of the magnetization vector relative to the incident light's plane of incidence. The radial Kerr effect is fundamentally governed by the Faraday-Kerr law, which describes the relationship between the change in polarization angle (ΔθK) and the applied magnetic field (H):ΔθK = nHKVwhere n is the sample's refractive index, H is the magnetic field strength, K is the Kerr constant, and V is the Verdet constant, which depends on the wavelength of the incident light and the magnetic properties of the material.B. Microscopic MechanismsAt the microscopic level, the radial Kerr effect can be attributed to twoprimary mechanisms: the spin-orbit interaction and the exchange interaction. The spin-orbit interaction arises from the coupling between the electron's spin and its orbital motion in the presence of an electric field gradient, leading to a magnetic-field-dependent modification of the electron density distribution and, consequently, the refractive index. The exchange interaction, on the other hand, influences the Kerr effect through its role in determining the magnetic structure and the alignment of magnetic moments within the material.C. Material DependenceThe magnitude and sign of the radial Kerr effect are highly dependent on the magnetic and optical properties of the material under investigation. Ferromagnetic and ferrimagnetic materials generally exhibit larger Kerr rotations due to their strong net magnetization. Additionally, the effect is sensitive to factors such as crystal structure, chemical composition, and doping levels, making it a valuable tool for studying the magnetic and electronic structure of complex materials.II. Experimental Techniques for Measuring the Radial Kerr EffectA. MOKE SetupA typical MOKE setup consists of a light source, polarizers, a magnetized sample, and a detector. In the case of radial Kerr measurements, the sample is usually magnetized along a radial direction, and the incident light is either p-polarized (electric field parallel to the plane of incidence) or s-polarized (electric field perpendicular to the plane of incidence). By monitoring the change in the polarization state of the reflected light as a function of the applied magnetic field, the radial Kerr effect can be quantified.B. Advanced MOKE TechniquesSeveral advanced MOKE techniques have been developed to enhance the sensitivity and specificity of radial Kerr effect measurements. These include polar MOKE, longitudinal MOKE, and polarizing neutron reflectometry, each tailored to probe different aspects of the magnetic structure and dynamics. Moreover, time-resolved MOKE setups enable the study of ultrafast magneticphenomena, such as spin dynamics and all-optical switching, by employing pulsed laser sources and high-speed detection systems.III. Applications of the Radial Kerr EffectA. Magnetic Domain Imaging and CharacterizationThe radial Kerr effect plays a crucial role in visualizing and analyzing magnetic domains in ferromagnetic and ferrimagnetic materials. By raster-scanning a focused laser beam over the sample surface while monitoring the Kerr signal, high-resolution maps of domain patterns, domain wall structures, and magnetic domain evolution can be obtained. This information is vital for understanding the fundamental mechanisms governing magnetic behavior and optimizing the performance of magnetic devices.B. Magnetometry and SensingDue to its sensitivity to both the magnitude and direction of the magnetic field, the radial Kerr effect finds applications in magnetometry and sensing technologies. MOKE-based sensors offer high spatial resolution, non-destructive testing capabilities, and compatibility with various sample geometries, making them suitable for applications ranging from magnetic storage media characterization to biomedical imaging.C. Spintronics and MagnonicsThe radial Kerr effect is instrumental in investigating spintronic and magnonic phenomena, where the manipulation and control of spin degrees of freedom in solids are exploited for novel device concepts. For instance, it can be used to study spin-wave propagation, spin-transfer torque effects, and all-optical magnetic switching, which are key elements in the development of spintronic memory, logic devices, and magnonic circuits.IV. Current Research Directions and Future PerspectivesA. Advanced Materials and NanostructuresOngoing research in the field focuses on exploring the radial Kerr effect in novel magnetic materials, such as multiferroics, topological magnets, and magnetic thin films and nanostructures. These studies aim to uncover newmagnetooptical phenomena, understand the interplay between magnetic, electric, and structural order parameters, and develop materials with tailored Kerr responses for next-generation optoelectronic and spintronic applications.B. Ultrafast Magnetism and Spin DynamicsThe advent of femtosecond laser technology has enabled researchers to investigate the radial Kerr effect on ultrafast timescales, revealing fascinating insights into the fundamental processes governing magnetic relaxation, spin precession, and all-optical manipulation of magnetic order. Future work in this area promises to deepen our understanding of ultrafast magnetism and pave the way for the development of ultrafast magnetic switches and memories.C. Quantum Information ProcessingRecent studies have demonstrated the potential of the radial Kerr effect in quantum information processing applications. For example, the manipulation of single spins in solid-state systems using the radial Kerr effect could lead to the realization of scalable, robust quantum bits (qubits) and quantum communication protocols. Further exploration in this direction may open up new avenues for quantum computing and cryptography.ConclusionThe radial Kerr effect, a manifestation of the intricate interplay between light and magnetism, offers a powerful and versatile platform for probing the magnetic properties and dynamics of materials. Its profound impact on various scientific disciplines, coupled with ongoing advancements in experimental techniques and materials engineering, underscores the continued importance of this phenomenon in shaping our understanding of magnetism and driving technological innovations in optoelectronics, spintronics, and quantum information processing. As research in these fields progresses, the radial Kerr effect will undoubtedly continue to serve as a cornerstone for unraveling the mysteries of magnetic materials and harnessing their potential for transformative technologies.。
氢谱和质谱英文
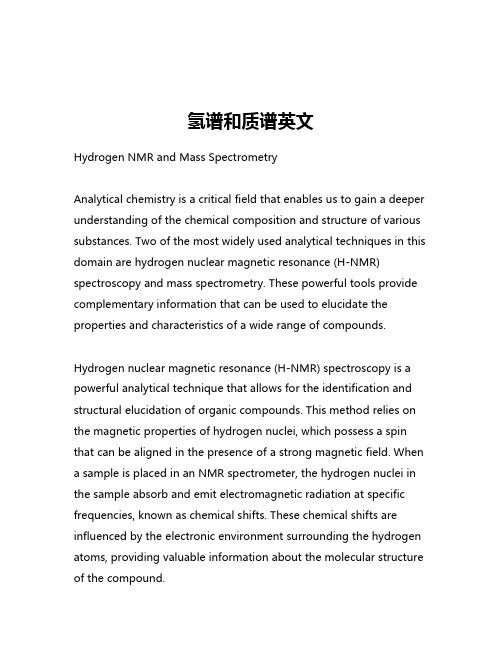
氢谱和质谱英文Hydrogen NMR and Mass SpectrometryAnalytical chemistry is a critical field that enables us to gain a deeper understanding of the chemical composition and structure of various substances. Two of the most widely used analytical techniques in this domain are hydrogen nuclear magnetic resonance (H-NMR) spectroscopy and mass spectrometry. These powerful tools provide complementary information that can be used to elucidate the properties and characteristics of a wide range of compounds.Hydrogen nuclear magnetic resonance (H-NMR) spectroscopy is a powerful analytical technique that allows for the identification and structural elucidation of organic compounds. This method relies on the magnetic properties of hydrogen nuclei, which possess a spin that can be aligned in the presence of a strong magnetic field. When a sample is placed in an NMR spectrometer, the hydrogen nuclei in the sample absorb and emit electromagnetic radiation at specific frequencies, known as chemical shifts. These chemical shifts are influenced by the electronic environment surrounding the hydrogen atoms, providing valuable information about the molecular structure of the compound.The H-NMR spectrum of a compound can reveal the number and type of hydrogen atoms present, as well as their relative positions within the molecule. By analyzing the chemical shifts, signal intensities, and coupling patterns observed in the spectrum, researchers can determine the connectivity and arrangement of the hydrogen atoms, which in turn provides insights into the overall structure of the molecule.One of the key advantages of H-NMR spectroscopy is its ability to provide detailed structural information without the need for extensive sample preparation. The technique is non-destructive, allowing for the recovery of the sample after analysis. Additionally, H-NMR spectroscopy is a quantitative method, meaning that the intensity of the signals in the spectrum is directly proportional to the amount of the corresponding hydrogen atoms in the sample.Mass spectrometry, on the other hand, is an analytical technique that measures the mass-to-charge ratio (m/z) of ions in a sample. This information can be used to determine the molecular weight and elemental composition of a compound. In a mass spectrometer, the sample is first ionized, typically by bombarding it with high-energy electrons or other ionization methods. The resulting ions are then accelerated and separated based on their mass-to-charge ratios using various types of mass analyzers, such as quadrupole, time-of-flight, or ion trap analyzers.The mass spectrum generated by a mass spectrometer provides a wealth of information about the sample. The molecular ion peak, which corresponds to the intact molecular ion, can be used to determine the molecular weight of the compound. Additionally, the fragmentation pattern observed in the mass spectrum can provide valuable structural information about the molecule. By analyzing the different fragment ions and their relative abundances, researchers can gain insights into the connectivity and functional groups present in the compound.One of the key advantages of mass spectrometry is its high sensitivity and selectivity. Modern mass spectrometers can detect and analyze trace amounts of compounds, making it a powerful tool for the identification and quantification of even complex and low-abundance analytes. Additionally, mass spectrometry can be coupled with various separation techniques, such as gas chromatography (GC-MS) or liquid chromatography (LC-MS), to provide even more detailed and comprehensive analysis of complex mixtures.The combination of H-NMR spectroscopy and mass spectrometry provides a powerful analytical toolkit for the structural elucidation and characterization of organic compounds. While H-NMR spectroscopy provides information about the connectivity andarrangement of hydrogen atoms within a molecule, mass spectrometry can provide insights into the overall molecular weight and elemental composition of the compound.In many cases, the complementary information obtained from these two techniques can be used to confirm the identity and purity of a compound, as well as to elucidate its structural features. For example, H-NMR spectroscopy can be used to determine the connectivity and relative positions of hydrogen atoms, while mass spectrometry can provide information about the molecular weight and the presence of specific functional groups or elements.Furthermore, the combination of H-NMR spectroscopy and mass spectrometry can be particularly useful in the identification and characterization of complex organic molecules, such as natural products, pharmaceuticals, and polymers. By leveraging the strengths of both techniques, researchers can gain a comprehensive understanding of the chemical structure and properties of these compounds, which is essential for a wide range of applications, including drug discovery, materials science, and environmental analysis.In conclusion, hydrogen nuclear magnetic resonance (H-NMR) spectroscopy and mass spectrometry are two powerful analytical techniques that play a crucial role in the field of analytical chemistry.While H-NMR spectroscopy provides detailed information about the connectivity and arrangement of hydrogen atoms within a molecule, mass spectrometry can reveal the molecular weight and elemental composition of a compound. The complementary nature of these techniques makes them invaluable tools for the structural elucidation and characterization of a wide range of organic compounds, with applications spanning diverse fields of research and industry.。
交流散热风扇内部结构
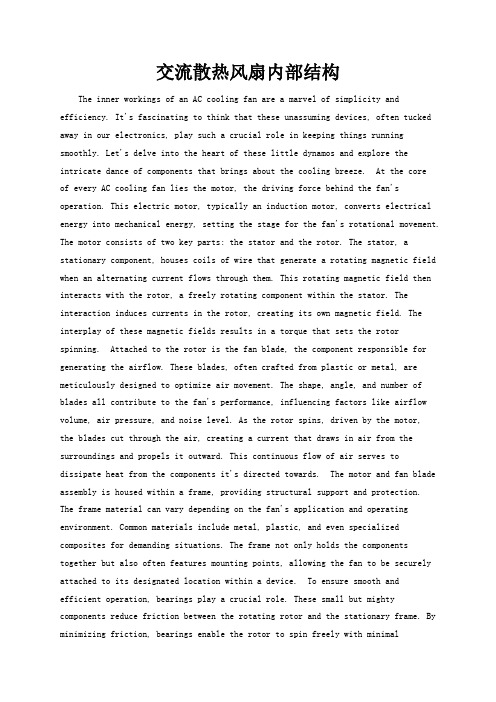
交流散热风扇内部结构The inner workings of an AC cooling fan are a marvel of simplicity and efficiency. It's fascinating to think that these unassuming devices, often tucked away in our electronics, play such a crucial role in keeping things running smoothly. Let's delve into the heart of these little dynamos and explore the intricate dance of components that brings about the cooling breeze. At the coreof every AC cooling fan lies the motor, the driving force behind the fan's operation. This electric motor, typically an induction motor, converts electrical energy into mechanical energy, setting the stage for the fan's rotational movement. The motor consists of two key parts: the stator and the rotor. The stator, a stationary component, houses coils of wire that generate a rotating magnetic field when an alternating current flows through them. This rotating magnetic field then interacts with the rotor, a freely rotating component within the stator. The interaction induces currents in the rotor, creating its own magnetic field. The interplay of these magnetic fields results in a torque that sets the rotor spinning. Attached to the rotor is the fan blade, the component responsible for generating the airflow. These blades, often crafted from plastic or metal, are meticulously designed to optimize air movement. The shape, angle, and number of blades all contribute to the fan's performance, influencing factors like airflow volume, air pressure, and noise level. As the rotor spins, driven by the motor,the blades cut through the air, creating a current that draws in air from the surroundings and propels it outward. This continuous flow of air serves todissipate heat from the components it's directed towards. The motor and fan blade assembly is housed within a frame, providing structural support and protection. The frame material can vary depending on the fan's application and operating environment. Common materials include metal, plastic, and even specialized composites for demanding situations. The frame not only holds the components together but also often features mounting points, allowing the fan to be securely attached to its designated location within a device. To ensure smooth andefficient operation, bearings play a crucial role. These small but mighty components reduce friction between the rotating rotor and the stationary frame. By minimizing friction, bearings enable the rotor to spin freely with minimalresistance, enhancing the fan's longevity and reducing noise generation. Various types of bearings are employed in AC cooling fans, each with its own set of characteristics. Sleeve bearings, known for their simplicity and cost-effectiveness, are often found in less demanding applications. Ball bearings, on the other hand, offer greater durability and smoother operation, making them suitable for high-performance scenarios. The electrical connection to the AC cooling fan is established through wires that supply power to the motor. These wires are typically color-coded to indicate their function, facilitating proper connection and ensuring safe operation. The voltage and current requirements of the fan are carefully matched to the power source, ensuring compatibility and preventing damage. Beyond these core components, AC cooling fans may incorporate additional features tailored to specific applications. Some fans include speed control mechanisms, allowing for adjustment of the airflow based on cooling needs. Others may integrate temperature sensors, enabling the fan to automatically regulate its speed in response to temperature fluctuations. These advanced features enhance the fan's versatility and efficiency, making them adaptable to a wide range of operating conditions. The seemingly simple act of generating a cooling breeze is, in reality, a testament to the ingenuity of engineering. The interplay of electrical and mechanical components, meticulously designed and assembled, results in a device that plays a vital role in countless applications. From keeping our computers humming along to ensuring the smooth operation of industrial equipment, AC cooling fans stand as unsung heroes, quietly and effectively dissipating heat and maintaining the delicate balance of temperature that keeps our world running.。
核磁共振中自旋裂分或J偶合[精心整理]
![核磁共振中自旋裂分或J偶合[精心整理]](https://img.taocdn.com/s3/m/e0a152e09f3143323968011ca300a6c30c22f1b7.png)
核磁共振中自旋裂分或J偶合Spin-spin splitting or J couplingCoupling in 1H NMR spectraWe have discussed how the chemical shift of an NMR absorption is affected by the magnetic field B e produced by the circulation of neighboring electrons. Now we wish to examine how the magnetic field produced by neighboring nuclei B n affects the appearance of the 1H NMR absorption. The effect occurs through the interaction of nuclear spins with bonding electron spins rather than through space. Let's first consider the absorption of a hydrogen nucleus labeled A with only one neighboring hydrogen nucleus in a vicinal position labeled X. Let's also assume that H A and H X have significantly different chemical shifts.H X will have approximately equal probability of existing in either the low energy alpha state or high energy beta state. Again because of the small energy difference between the low and high energy states, the high energy state is easily populated from thermal energy. For those molecules in which H X exists in the low energy state, about half the molecules in the sample, its magnetic field B n will subtract from the magnetic field B o-B e and for those molecules in which H X exists in the higher energy state, again about half the molecules, its magnetic field B n will add to B o-B e.Note: whether B n for a particular spin state adds to or subtracts from B o is a function of the number of intervening bonds; this phenomenon doesn't usually affect the appearance of the signal and will not be explained here but results from the mechanism of coupling involving interaction of nuclear spins with electron spins. For the example of vicinal coupling (3 intervening bonds), the B n field is negative for H X in the alpha spin state; for geminal coupling B n is positive for H X in the alpha spin state. Geminal coupling occurs between protons of different chemical shift bonded to the same carbon (2 intervening bonds); it will be discussed later.As a consequence of the B n field in a vicinal system, at fixed external magnetic field B o, a lower frequency will be required to achieveresonance for those molecules which have H X in the state than for those molecules which have H X in the state. The NMR signal for H A will appear as a two line pattern as shown in Figure 16. We say the H X splits the absorption H A into a doublet and the two protons are coupled to each other. The intensity of the two lines will be equal since the probability of H X existing in the or states is approximately equal. The chemical shift, which is defined as the position of resonance in the absence of coupling, is the center of the doublet. Just as H X splits the signal of H A into a doublet, H A splits the signal of H X into a doublet. The overall splitting pattern consisting of two doublets is call an A X pattern. The splitting of H A by H X is diagramed in Figure 16.When the molecule bears two equivalent vicinal protons, four possibilities exist for their combined magnetic fields: both are in spin states, one is in the spin state and one in the spin state, andvice versa, or both in the spin state. These four possibilities have about equal probability, and the appearance of the NMR signal is a 3-line pattern, a triplet(Figure 17), with intensities 1:2:1 because the effect of and are the same. With one adjacent proton in the spin state andthe other in the spin state, the effect of the B n field becomes zero, and the center line of the triplet is the position of the chemical shift. The two H X protons split the H A signal into a triplet and the H A proton splits the two H X protons into a doublet. The overall splitting pattern consisting of a triplet and a doublet is called an A X2 pattern.Three chemical shift equivalent vicinal protons H X split the absorption of H A into a quartet with intensity pattern 1:3:3:1 as shown in Figure 10. The chemical shift is the center of the quartet. The three H X protons split the H A signal into a quartet and the H A proton splits the signal for the three H X protons into a doublet. The overall splitting pattern consisting of a quartet and a doublet is called an A X3 pattern.The spacing between the lines of a doublet, triplet or quartet is called the coupling constant. It is given the symbol J and is measured in units of Hertz (cycles per second). The magnitude of the coupling constant can be calculated by multiplying the separation of the lines in units (ppm) by the resonance frequency of the spectrometer in megaHertz.J Hz = ppm x MHz (typically 300, 400, or 500 MHz)In general, N neighboring protons with the same coupling constant J will split the absorbance of a proton or set of equivalent protons into N+1 lines. Note that the splitting pattern observed for a particular proton or set of equivalent protons is not due to anything inherent to that nucleus but due to the influence of the neighboring protons. The relative intensity ratios are given by Pascal's triangle as shown in Figure 18.Because of the mechanism of J coupling, the magnitude is field independent: coupling constants in Hertz will be the same whether the spectrum is measured at 300 MHz or 500 MHz. Coupling constants range in magnitude from 0 to 20 Hz. Observable coupling will generally occur between hydrogen nuclei that are separated by no more than three sigma bonds.H-C-H, two sigma bonds or geminal couplingH-C-C-H, three sigma bonds or vicinal couplingCoupling is never observed between chemical shift equivalent nuclei, be it from symmetry or by accident, not because the B n field disappears but because spin transitions that would reveal the coupling are forbidden by symmetry. The role of symmetry in forbidding spectral transitions is of general importance in spectroscopy but is beyond the scope of this discussion. The magnitude of the coupling constant also provides structural information; for example, trans-alkenes show larger vicinal coupling than cis-alkenes. Sometimes, coupling is not observed betweenprotons on heteroatoms such as the OH proton of an alcohol and adjacent protons on carbon. In this case the absence of coupling results from rapid exchange of the OH protons via an acid base mechanism; because of rapid exchange the identity of the spin state, or , of the acidic proton is lost. Examples of coupling constants J are shown in Figure12.The example of geminal coupling of protons on a saturated carbon requires a structure in which the protons have different chemical shifts. This commonly occurs in a chiral molecule with a tetrahedral stereocenter adjacent to the methylene group as shown in the following compounds with stereocenters labeled with an asterisk. The geminal protons are labeled H A and H B rather than H A and H X because they have similar chemical shifts (A and B are close in the alphabet). Coupling between the geminal protons is independent of optical activity and rotation about single bonds. The hydrogens H A and H B are said to be diastereotopic hydrogens because if alternately each one is replaced with a deuterium atom, the resulting two structures are diastereomers (stereoisomers that aren't mirror images).Now let's examine the 1H NMR spectrum of methyl propanoate (methyl propionate). Notice that hydrogen atoms of the methyl group bonded to oxygen appear as a singlet at 3.6 ppm. They are chemical shift equivalent and hence, do not couple with each other. The chemical shift results from the deshielding effect of the strongly electronegative oxygen atom. The resonance for the methylene protons appear as a quartet at 2.3 ppm. Thesplitting is caused by the three chemical shift equivalent protons on the adjacent methyl group. The methylene protons do not split each other since they are also chemical shift equivalent. The methyl protons appear at 1.1 ppm and are split into a triplet by the adjacent methylene protons.The coupling constant for the methyl triplet and the methylene quartet is 7 Hz. The overall splitting pattern consisting of a three-proton triplet and a two-proton quartet is called an A3X2 pattern.next section: Spin-spin splitting and coupling - More complex 1H NMR splitting© University of Colorado, Boulder, Chemistry and Biochemistry Department, 2003Spin-spin splitting or J couplingMore complex splitting patterns1H NMR patterns are more complex than predicted by the N+1 coupling rule when coupling of one proton or set of equivalent protons occurs to two different sets of protons with different size coupling constants or when coupling occurs between protons with similar but not identical chemical shifts. The former situation can still be analyzed in terms of overlapping N+1 patterns using stick diagrams. This is shown for the spectrum of phenyloxirane which has three oxirane protons of different chemical shift all coupled to each other. The protons are labeled H A, H M, and H X to reflect that they are not close to each other in chemical shift. Each resonance appears as a doublet of doublets, and the overall pattern of three doublets of doublets is called an A M X pattern.The situation of protons with close chemical shifts coupled to each other is more complex. If only two protons are coupled to each other, the pattern still appears as two doublets but the intensities are no longer 1:1 and the chemical shifts are not the centers of the doublets; the separation between the lines of each doublet is still the coupling constant J. The chemical shifts are closer to the larger peaks of each doublet and can be calculated using a simple equation as shown below.If more than two protons of close chemical shift are coupled to each other, more complex patterns, often described as complex multiplets, are observed. Multiplets still provide useful structural information because they indicate the presence of coupled protons of similar chemical shift. The AB pattern and complex multiplet patterns result from what is called second order effects. Second order effects occur when the ratio of the chemical shift separation in Hz to the coupling constant is less than approximately 10 or /J < 10. Even when this ratio is greater than 10,slight intensity perturbation is evident in first order patterns as shown by the spectrum for 2-butanone. In fact, if we draw an arrow over the pattern showing the slight tilt (blue arrows in Figure 25), the arrowspoint toward each other. So we say the patterns for coupled protons point towards each other.Spin-spin splitting and couplingCoupling in 13C NMR spectraBecause the 13C isotope is present at only 1.1% natural abundance, the probability of finding two adjacent 13C carbons in the same molecule of a compound is very low. As a result spin-spin splitting between adjacent non-equivalent carbons is not observed. However, splitting of the carbon signal by directly bonded protons is observed, and the coupling constants are large, ranging from 125 to 250 Hz. Methyl groups appear as quartets, methylenes as triplets, methines as doublets, and unprotonated carbons as singlets. Commonly, splitting of the signal by protons is eliminated by a decoupling technique which involves simultaneous irradiation of the proton resonances at 300 MHz while observing the carbon resonances at 75 MHz. The decoupling is accomplished with a second broad band, continuous, oscillating magnetic field B2(as opposed to the pulsed B1field), and the decoupling is continued during data collection. The B2field causes rapid proton spin transitions such that the 13C nuclei lose track of the spin states of the protons. Figure 26 shows a proton decoupled 13C spectrum of ethyl acetate. The purpose of proton decoupling is to eliminate overlapping signal patterns and to increase the signal to noise ratio. Decoupling of the protons increases the signal to noise ratio by causing the collapse of quartets, triplets, and doublets to singlets and bycausing a favorable increase in the number of carbons in the -spin state relative to the -spin state. The latter effect is called the Nuclear Overhauser Effect (NOE); how it causes this change in spin state populations will not be discussed here.Integration of 1H NMR spectraThe area under each pattern is obtained from integration of the signal (or better the function that defines the signal) and is proportional to the number of hydrogen nuclei whose resonance is giving rise to the pattern. The integration is sometimes shown as a step function on top of the peak with the height of the step function proportional to the area. The integration of the patterns at 1.1, 2.4, and 3.7 ppm for methyl propanoate is approximately 3:2:3 (see figure 22). Note, the error in integration can be as high as 10% and depends upon instrument optimization. The integration of an 1H NMR spectrum gives a measure of the proton count adjusted for the molecular symmetry. Methyl propanoate has no relevant molecular symmetry and so, the integration gives the actual proton count: 3+2+3=8 protons. In contrast diethyl ether (Et-OEt) has a plane of symmetry which makes the two ethyl groups equivalent, and so, only two signal are observed, a triplet and a quartet, with integration 3:2.The areas represented by the integration step function is usually integrated by the instrument and displayed as numerical values under the scale. For instance, the normalized integration values for 2-butanoneare shown in Figure 27. Note that these values are not exact integers andneed to be rounded to the nearest integer to obtain the proper value.Integration of 13C NMR SpectraIn a 1H NMR spectrum, the area under the signals is proportional to the number of hydrogens giving rise to the signal. As a result the integration of the spectrum is a measure of the proton count. In a 13C NMR spectrum the area under the signal is not simply proportional to the number of carbons giving rise to the signal because the NOE from proton decoupling is not equal for all the carbons. In particular, unprotonated carbons receive very little NOE, and their signals are always weak, only about 10% as strong as signals from protonated carbons.Because the resolution in 13C NMR is excellent, the number of peaks in the spectrum is a measure of the carbon count adjusted for the symmetry of the molecule. For example, hexane gives three peaks: the two methyls are equivalent as are two sets of methylenes. Several examples are analyzed as follows; the chemical shifts shown are not the observed values but calculated values from empirical rules:Hexane shows three peaks, two methyls and two sets of methylenes.Acetone shows two peaks, one for the methyls and one for the carbonyl carbon.Ethyl benzoate shows 7 peaks; the benzene ring shows only 4 peaks because of two sets of equivalent carbons.Ethyl 3-chlorobenzoate, however, shows 9 peaks, a separate signal for each carbon because it has no symmetry.Cis-1,2-dimethylcyclohexane shows 4 peaks; because of rapid chair-chair interconversion, we can analyze the NMR spectrum in terms of a flat structure; hence, the methyls are equivalent, as are the methines, and there are two sets of equivalent methylenes.Solvents for NMR spectroscopyA common solvent for dissolving compounds for 1H and 13C NMR spectroscopy is deuteriochloroform, DCCl3. In 1H NMR spectra, the impurity of HCCl3 in DCCl3gives a small signal at 7.2 ppm (see spectrum of methyl propanoate). In 13C spectroscopy 1.1% of the deuteriochloroform has a 13C isotope and it is bonded to a deuterium atom. The nucleus of the deuterium atom, the deuteron, has a more complicated nuclear spin than does the proton, and it has a gyromagnetic ratio () 1/6 as large. This more complicated nuclearspin gives rise to three spin states instead of the two spin states for the proton, and the deuteron undergoes resonance at a different frequency than either the proton or 13C nucleus. These spin states are approximately all equally populated. Because the spin-spin coupling between the 13C and the deuterium is not eliminated during proton decoupling, the DCCl3shows three equal peaks of low to moderate intensity at about 77 ppm (see Figure 13). The separation is the carbon-deuterium coupling constant JCD. The intensity is low to moderate because the 13C receives no Nuclear Overhauser Enhancement from the proton decoupling.。
化学及化工专业词汇英语翻译(P-Z)4
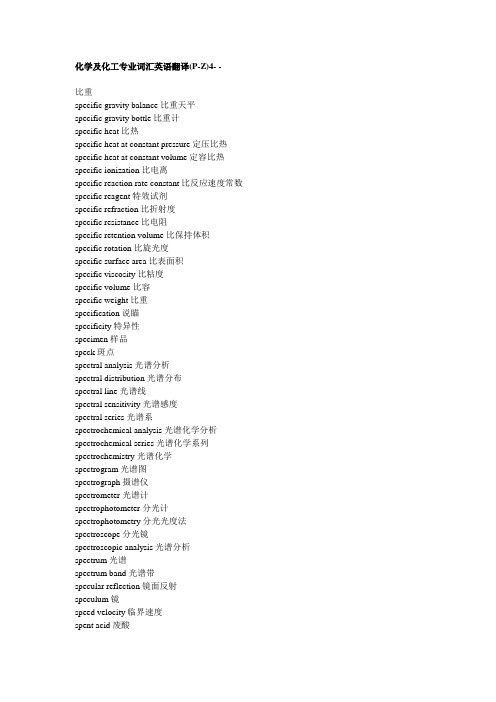
化学及化工专业词汇英语翻译(P-Z)4- -比重specific gravity balance 比重天平specific gravity bottle 比重计specific heat 比热specific heat at constant pressure 定压比热specific heat at constant volume 定容比热specific ionization 比电离specific reaction rate constant 比反应速度常数specific reagent 特效试剂specific refraction 比折射度specific resistance 比电阻specific retention volume 比保持体积specific rotation 比旋光度specific surface area 比表面积specific viscosity 比粘度specific volume 比容specific weight 比重specification 说瞄specificity 特异性specimen 样品speck 斑点spectral analysis 光谱分析spectral distribution 光谱分布spectral line 光谱线spectral sensitivity 光谱感度spectral series 光谱系spectrochemical analysis 光谱化学分析spectrochemical series 光谱化学系列spectrochemistry 光谱化学spectrogram 光谱图spectrograph 摄谱仪spectrometer 光谱计spectrophotometer 分光计spectrophotometry 分光光度法spectroscope 分光镜spectroscopic analysis 光谱分析spectrum 光谱spectrum band 光谱带specular reflection 镜面反射speculum 镜speed velocity 临界速度spent acid 废酸spent lye 废碱液sperm oil 抹香鲸脑油spermaceti 鲸蜡spermidine 精脒spermine 精胺sphalerite 闪锌矿sphere of action 酌球spherical condenser 球形冷凝器spherical flask 球形长颈瓶spherulite 球晶sphingolipid 鞘脂类sphingomyelin 鞘髓磷脂sphingosine 鞘氨醇spin 自旋spin label 自旋标记物spin orbit interaction 自旋轨道相互酌spin quantum number 自旋量子数spin wave 自旋波spinacene 鲨鱼烯spindle 液体比重计;轴spindle oil 锭子油spinel 尖晶石spinnability 可纺性spinneret 喷丝头spinning 纺丝spinning bath 纺丝浴spinning machine 纺丝机spinning property 可纺性spinning pump 纺丝泵spinning solution 纺丝液spinodal decomposition 旋节线分解spiral condencer 旋管冷却器spiral condenser 旋管冷凝器spiral cooler 旋管冷却器spiral heat exchanger 螺旋型换热器spiral plate exchanger 旋板换热器spirane 螺环化合物spirit 酒精spirit color 醇溶染料spirit lamp 酒精灯spirit stain 酒精着色剂spirit varnish 醇溶清漆spiro compound 螺环化合物split product 裂解产物splitting 分解spodumene 锂辉石sponge 海绵sponge rubber 海绵状橡皮spongin 海绵硬朊sponginess 海绵质spontaneous combustion 自然燃烧spontaneous crystallization 自发结晶spontaneous emission 自发发射spontaneous heating 自然加热spontaneous ignition 自发着火spontaneous ignition temperature 自燃温度spontaneous process 自发过程spot 斑点spot analysis 点滴分析spot plate 滴试板spot reaction 点滴反应spot test 点滴试验spray coating 喷雾涂布spray dryer 喷雾干燥器spray drying 喷雾干燥spray dyeing 喷雾染色spray gun 喷射枪spray lacquer 喷漆spray nozzle 喷嘴spray polymerization 喷雾聚合spray sizing 喷雾上浆spray tower 喷雾塔spray water proofing 喷雾防水sprayer 喷雾器spraying 喷雾spraying burner 喷射燃烧器spring balance 弹簧秤spring manometer 弹黄压力计spun fiber 纺成纤维squalene 鲨鱼烯square error 平方误差square hole sieve 方孔筛ss acid ss 酸stab culture 穿刺培养stability 稳定性stability constant 稳定常数stability test 稳定性试验stabilization 稳定化stabilized gasoline 稳定汽油stabilizer 稳定剂stable equilibrium 稳定平衡stable isotope 稳定同位素stactometer 滴量计stain 着色剂stained glass 彩色玻璃staining method 着色法stainless steel 不锈钢stalactite 钟乳石stalagmite 石笋stalagmometer 滴重计stand 架台stand oil 熟油standard 标准standard cell 标准电池standard cellulose 标准纤维素standard deviation 标准偏差standard electrode 标准电极standard electrode potential 标准电极势standard hydrogen electrode 标准氢电极standard illuminant 标准光源standard pressure 标准压力standard sample 标准试样standard sand 标准砂standard solution 标准溶液standard state 标准状态standard temperature 标准温度standardization 标定stannate 锡酸盐stannic acid 锡酸stannic chloride 氯化锡stannic oxide 二氧化锡stannic salt 正锡盐stannite 黄锡矿stannous chloride 二氯化锡stannous hydroxide 氢氧化亚锡stannous oxide 氧化亚锡stannous salt 亚锡盐staphylococcus 葡萄球菌staple fiber 切断纤维staple fiber spinning 短纤维纺纱starch 淀粉starch granule 淀粉粒starch iodine paper 碘化物淀粉纸starch paste 淀粉糊starch sirup 淀粉糖汁starch sugar 淀粉糖starch value 淀粉值starting material 起始物料state analysis 状态分析state of aggregation 聚集状态static bed 固定床static friction 静摩擦static pressure 静压stationary flow 定常流stationary furnace 固定炉stationary mercury electrode 固定汞电极stationary phase 固定相stationary process 定常过程stationary state 定常状态statistic 统计量statistic data 统计资料statistic mechanics 统计力学statistical error 统计误差statistical quality control 统计质量控制statistical test 统计检验statistical thermodynamics 统计热力学statoscope 微动气压器steady flow 定常流steady state 定常状态steam 水蒸汽steam bath 蒸汽浴steam boiler 蒸汽锅炉steam calorimeter 蒸汽热量计steam coil 蒸汽旋管steam consumption 蒸汽消耗steam distillation 蒸汽蒸馏steam drier 蒸汽干燥器steam ejector 蒸汽喷射器steam emulsion number 蒸汽乳化值steam funnel 蒸汽漏斗steam generator 蒸汽发生器steam heating 蒸汽加热steam jacket 蒸汽套steam jet 蒸汽喷射steam sterilizer 蒸汽杀菌器steam trap 汽水阀steam valve 蒸汽阀steam vulcanization 蒸汽硫化steaming 蒸汽加工stearate 硬脂酸盐stearic acid 硬脂酸stearic acid nitril 硬脂腈stearin candle 硬脂蜡stearin pitch 硬脂沥青stearonitril 硬脂腈stearyl alcohol 硬脂醇stearyl chloride 硬脂酰氯steatite 块滑石stechiometer 化学计量仪stechiometry 化学计量法steel 钢steeping 浸渍stefan boltzmann's law 斯蒂芬玻尔兹曼定律stephanite 脆银矿stereochemical formula 立体化学式stereochemistry 立体化学stereocopolymer 立体共聚物stereoelectronic effect 立体电子效应stereoformula 立体化学式stereoisomer 立体异构体stereoisomerism 立体异构stereopolymerization 立体聚合stereoregular polymer 立体定向聚合物stereoselective reaction 立体选择反应stereoselectivity 立体选择性stereospecific catalyst 立体定向催化剂stereospecific polymer 立体定向聚合物stereospecific polymerization 立体定向聚合stereospecific synthesis 立体定向合成stereospecificity 立体特异性stereostructure 立体结构steric effect 位阻效应steric factor 位阻因素steric strain 空间应变sterile cooling 杀菌冷却sterile solution 无菌溶液sterilization 杀菌sterilizer 杀菌器steroide 甾族化合物sterol 甾醇stibiconite 黄锑矿stibine 锑化氢stibonium salt 四氢锑盐stick lac 师虫胶stick sulphur 棒状硫磺stickiness 粘滞性sticking probability 粘着概率stigmasterol 豆甾醇stilbene 芪均二苯代乙烯stilbene dye 芪染料stilbite 束沸石still 蒸馏锅still head 分馏头still residue 蒸馏余液stirred tank reactor 搅拌釜反应器stirrer 搅拌机stirring 搅拌stochastic differential equation 随机微分方程式stochastic process 随机过程stochastic variable 随机变数stock solution 储液stoichiometric calculation 化学计算stoichiometry 化学计量学stoneware 缸器stop solution 停止液stopping power 制动能力storage 储藏storage battery 蓄电池storage life 储存寿命storage loss 贮存损失storage tank 储蓄槽storax 安息香straight chain 直链straight chain molecule 直链分子straight run distillation 直接蒸馏straight run gasoline 直馏汽油strain 应变strainer 滤网stratcold process 斯特拉柯尔特法stratosphere 平零straw pulp 草纸浆streaming birefringence 怜双折射streaming potential 怜电位streamline 吝streamlined shape 吝型strecker's synthesis 斯特雷克尔合成strength 强度strength of solution 溶液浓度streptococcus 链球菌streptodornase 链道酶streptokinase 链激酶streptolin 黄链丝菌素streptomycin 链霉素streptothricin 链丝菌素stress 应力stress concentration 应力集中stress corrosion cracking 应力腐蚀裂纹stress relaxation 应力松弛stress strain diagram 应力应变图stretch spinning process 伸纺丝法stretching test 拉伸试验stretching vibration 伸缩振动striae 条纹stripped atom 裸原子stripping 汽提stripping agent 退色剂strong acid 强酸strong base 强碱strong electrolyte 强电解质strontium 锶strontium carbonate 碳酸锶strontium chlorate 氯酸锶strontium hydroxide 氢氧化锶strontium nitrate 硝酸锶strontium oxide 氧化锶strontium sulfide 硫化锶strontium yellow 锶黄strophanthin 毒毛旋花素strophanthus 毒毛旋花属structural chemistry 结构化学structural foam 构造泡沫structural formula 结构式structural isomer 结构同分异构体structural isomerism 结构同分异构structural optimization 构架最佳化structural phase transition 结构相变structural viscosity 结构粘度structure analysis 结构分析structure design 结构设计structure factor 结构因子strychnine 士的宁strychnine nitrate 硝酸士的宁stuffing 加脂styphnic acid 收敛酸styrax 安息香styrenated oil 苯乙烯化油styrene 苯乙烯styrene butadiene rubber 丁苯橡胶styrene oxide 氧化苯乙烯styrene resin 苯乙烯尸styrol 苯乙烯styrone 肉桂醇styryl alcohol 肉桂醇sub bituminous coal 亚烟煤sub reference fuel 副标准燃料subchloride 低氯化物suberane 环庚烷suberate 辛二酸盐suberene 环庚烯suberic acid 辛二酸suberin 软木脂subgroup 副族sublimate 升华物sublimation 升华sublimation cooling 升华冷却sublimation curve 升华曲线sublimation point 升华点sublimed sulfur 硫黄华submerged combustion 沉没燃烧submicro analysis 半微分析submicron 亚微细粒suboxide 低氧化物subsidiary valence 副价subsiding tank 沉淀槽subsoil water 地下水substance 物质substantive color 直接染料substantive dye 直接染料substantivity 直接性substituent 取代基substitute 代用品substitution 取代substitution compound 取代化合物substitution product 取代产物substitution reaction 取代反应substrate 基质subtractive color process 减色法successive reaction 逐次反应succharose 蔗糖succinaldehyde 琥珀醛succinate 琥珀酸盐succinic acid 琥珀酸succinic anhydride 琥珀酐succinimide 琥珀酸亚胺succinyl 琥珀酰sucker 吸管sucking 抽吸sucrose 蔗糖suction 抽吸suction bottle 吸滤瓶suction drier 吸引式干燥器suction filter 吸滤器suction flask 吸滤瓶suction funnel 吸滤器suction gas 吸气suction pump 抽吸泵suffocation 窒息sugar 糖sugar carbon 糖炭sugar charcoal 糖炭sugar of lead 铅糖sugar syrup 糖浆suint 羊色粗脂sulfa drug 磺胺剂sulfacetamide 乙酰磺胺sulfadiazine 磺胺嘧啶sulfaguanidine 磺胺胍sulfamic acid 氨基磺酸sulfamide 硫酰胺sulfanilamide 对氨基苯磺酰胺sulfanilic acid 磺胺酸sulfatase 硫酸酯酶sulfate 硫酸盐sulfate oil 磺化油sulfate process 硫酸盐法sulfathiazole 磺胺噻唑sulfation 硫酸化sulfatizing roasting 硫酸盐焙烧sulfide 硫化物sulfinic acid 亚磺酸sulfitation 亚硫酸盐化sulfite 亚硫酸盐sulfite process 亚硫酸盐法sulfite pulp 亚硫酸盐纸桨sulfite waste liquor 亚硫酸盐废液sulfo group 磺基sulfobenzoic acid 磺基苯酸sulfonal 损那sulfonamide 磺酰胺sulfonamide drug 磺胺剂sulfonate 磺酸盐sulfonated oil 磺化油sulfonation 磺化sulfonator 磺化器sulfone 砜sulfonic acid 磺酸sulfonium base 锍碱sulfonium salt 锍盐sulfophthalic acid 磺基酞酸sulfosalicylic acid 磺基水杨酸sulfoxide 亚砜sulfoxylate 次硫酸盐sulfoxylic acid 次硫酸sulfur 硫磺sulfur black 硫化黑sulfur blue 硫化蓝sulfur burner 烧硫炉sulfur chloride 氯化硫sulfur dioxide 二氧化硫sulfur dye 硫化染料sulfur fluoride 氟化硫sulfur liver 硫磺肝sulfur oxide 氧化硫sulfur soap 硫化皂sulfur trioxide 三氧化硫sulfuration 硫化sulfuretted hydrogen 硫化氢sulfuric acid 硫酸sulfuric acid anhydride 硫酐sulfurous acid 亚硫酸sulfurous acid gas 二氧化硫sulfurous anhydride 亚硫酐sulfurous ester 亚硫酯sulfuryl chloride 硫酰氯sulphur 硫磺sulphuric acid 硫酸sultone 磺内酯sum over states 状态和sun bleaching 曝晒漂白sunflower oil 向日葵油superacid 过酸superconducting material 超导材料superconductivity 超导率supercooled liquid 过冷液体supercooling 过冷superexchange interaction 超交换相互酌superexcited state 超激发态superficial velocity 空塔速度superfluidity 超猎superfusion 过熔superheated steam 过热蒸汽superheater 过热器superinsulation 超绝热superionic conductor 超离子导体superlattice 超晶格结构supermicro analysis 超微分析supernatant liquid 上层液体superoxide 过氧化物superphosphate of lime 过磷酸钙supersaturated solution 过饱和溶液supersaturated steam 过饱和蒸气supersaturation 过饱和supersonic fuel 超声燃料supersonic wave 超声波superstructure 超晶格结构supersymmetry 超对称性supplementary unit 辅助单位supporting electrolyte 支持电解质surface 表面surface active agent 表面活性剂surface activity 表面活性surface chemistry 表面化学surface combustion 表面燃烧surface condenser 表面冷凝器surface denaturation 表面变性surface density 表面密度surface diffusion 表面扩散surface drying 表面干燥surface elasticity 表面弹性surface energy 表面能surface integral 面积分surface layer 表面层surface level 表面能级surface phenomenon 界面现象surface potential 表面电势surface preparation 表面处理surface pressure 表面压力surface reaction 界面反应surface renewal theory 表面更新理论surface resistance 表面阻力surface tension 表面张力surface treatment 表面处理surface viscosity 表面粘度surfactant 表面活性剂susceptibility 磁化率suspended matter 浮游物质suspended solid 浮游物质suspending agent 悬浮剂suspension 悬浮液suspension colloid 悬胶质suspension insulator 悬垂碍子suspension polymerization 悬浮聚合suspensoid 悬胶质sweet basil 罗勒sweet gasoline 脱硫汽油sweet oil 橄榄油sweetened condensed milk 甜炼乳sweetening 脱硫swelling 膨润swelling agent 膨润剂swelling heat 膨润热swelling pressure 膨润压力switch oil 开关油syenite 正长石sylvite 钾石盐symbiosis 共生symbol of element 元素符号symmetric compound 对称化合物symmetric matrix 对称矩阵symmetrical molecule 对称分子symmetry 对称symmetry axis 对称轴symmetry classes 对称类sympathetic ink 隐显墨水sympathetic reaction 交感反应synchronism 同时性synchroscope 同步示波器synchrotron 同步加速器syndet 合成洗涤剂syndiotactic polymer 间同立构聚合物syneresis 脱水收缩synergism 协同酌synergist 协琢synergistic effect 协同效应synthesis 合成synthesis gas 合成气synthetase 合成酶synthetic ammonia 合成氨synthetic chemistry 合成化学synthetic detergent 合成洗涤剂synthetic dyes 合成染料synthetic fiber 合成纤维synthetic fuel 合成燃料synthetic gypsum 合成石膏synthetic latex 合成胶乳synthetic mica 合成云母synthetic perfume 合成香料synthetic resin 合成尸synthetic rubber 合成橡胶synthetic tannin 合成丹宁synthetic zeolite 合成沸石synthetics 合成品synthol 辛托合成过程system engineering 系统工程systematic analysis 系统分析systematic error 系统误差systemic insecticide 内吸杀虫剂t distribution t 分布table feeder 平板给料机table salt 食盐tabular crystal 平片状结晶tac 碳化钽tachometer 转数计tachysterin 环裂甾醇tackifier 胶粘剂tackiness 粘着性tackiness agent 胶粘剂tacky 胶粘的tactoid 类晶团聚体tactosol 凝聚溶胶tafel rearrangement 塔菲尔换位tag closed tester 泰格密闭闪点试验器tagged molecule 标记分子tailings 尾矿talc 滑石tall oil 妥尔油tallow 脂talose 塔洛糖tank development 槽显影tank furnace 槽炉tannase 丹宁酶tannic acid 丹宁酸tannin 丹宁tannin black dyeing 酸黑染料tannin extract 丹宁提取物tannin mordanting 丹宁媒染tanning 革tanning agent 剂tannoform 亚甲丹宁tantalate 钽酸盐tantalic acid 钽酸tantalite 钽铁矿tantalum 钽tantalum carbide 碳化钽tantalum chloride 氯化钽tantalum oxide 氧化钽tap aspirator 龙头廉泵tap funnel 分液漏斗tar 焦油tar acid 焦油酸tar base 焦油碱tar distillation 焦油蒸馏tar heavy oil 沥青重油tar light oil 沥青轻油tar middle oil 沥青中油tar oil 焦油tar separator 焦油分离器tar value 焦油值tar water 焦油下水tare 皮重target 标志tarnish 变暗tarragon oil 龙蒿油tarry material 煤焦油材料tartar 酒石tartar emetic 吐酒石tartaric acid 酒石酸tartrate 酒石酸盐tartronic acid 丙醇二酸tasimeter 微压计taurine 牛磺酸taurocholic acid 牛磺胆酸tautomer 互变体tautomeric change 互变异构变化tautomerism 互变现象taylor's series 泰勒级数tea oil 茶油tear gas 催泪性毒气tear resistance 撕破强度tearing property 催泪性technetium 锝technical analysis 工业分析technical atmosphere 工业大气压technical chemistry 工业化学technical reagent 工业试药teclu burner 特克卢燃烧器teflon 特弗隆tellurate 碲酸盐telluric acid 碲酸telluride 碲化物tellurite 亚碲酸盐tellurium 碲tellurium dioxide 二氧化碲tellurium tetrachloride 四氯化碲tellurous acid 亚碲酸telomer 帝物telomerization 第聚合酌temper brittleness 回火脆性temper carbon 回火碳temper hardening 回火硬化temperature coefficient 温度系数temperature coefficient of resistance 电阻温度系数temperature color scale 色温标temperature control 温度第temperature correction 温度校正temperature dependency 温度依存性temperature difference 温差temperature drop 温降temperature gradient 温度梯度temperature indicating compound 指示温度化合物temperature indicator 温度指示器temperature jump method 温度突变法temperature regulator 温度第器temperature scale 温标temperature stress 温差应力tempering 回火tempering oil 淬火用油template reaction 模板反应temporary hardness 暂时硬度temporary plankton 暂时性浮游生物tenacity 韧性tennantite 砷铜矿tenorite 黑铜矿tensile strain 受拉应变tensile strength 抗拉强度tensile test 拉力试验tensiometer 表面张力计tension 张力terbium 铽terebene 松节油精terephthalaldehyde 对酞醛terephthalic acid 对酞酸term of multiplet 多重项terminal group 末端基termination reaction 终止反应ternary alloy 三元合金ternary compound 三元化合物ternary point 三重点ternary system 三元系terpene alcohol 萜烯醇terpenoid 萜烯酯terphenyl 三联苯terpinene 萜品烯terpineol 萜品醇terpinolene 萜品油烯terpinyl acetate 乙酸萜品酯terpolymer 三元共聚物terra alba 白土terra cotta 混合陶器;赤陶土terramycin 土霉素tert butyl alcohol 叔丁醇tertiary alcohol 叔醇tertiary amine 叔胺tertiary calcium phosphate 磷酸三钙tertiary carbon atom 叔碳原子test of hypothesis 假设检验test paper 试纸test plant 试验车间test tube 试管test tube clamp 试管夹test tube holder 试管夹test tube stand 试管架testosterone 睾酮tetraborane 丁硼烷tetrabromoethylene 四溴代乙烯tetracaine 丁卡因tetracarboxylic acid 四羧tetrachlorobenzene 四氯代苯tetrachloroethane 四氯乙烷tetracosane 廿四碳烷tetracycline 四环素tetradecane 十四烷tetradecyl alcohol 十四醇tetraethylene glycol 四甘醇tetraethyllead 四乙基铅tetrafluoromethane 四氟甲烷tetragonal system 正方系tetrahydrofuran 四氢呋喃tetrahydronaphthalene 四氢化葵tetraiodoethylene 四碘乙烯tetralin 四氢化葵tetraline 四氢化萘tetramethyl lead 四甲基铅tetramethylene 环丁烷tetramethylenediamine 四甲撑二胺tetramethyleneimine 吡咯烷tetranitromethane 四硝基甲烷tetrasaccharide 四糖tetrasilane 四硅烷tetrose 丁糖tetroxide 四氧化物tetryl 特屈儿textile 织物textile assistant 纺织助剂textile auxiliary agent 纺织助剂textile chemistry 纺织化学textile fiber 纺织用纤维textile finishing 织物完成加工textile industry 纺织工业textile oil 纺织用油textile printing 织物印花textile soap 丝光皂texture 构造组织thalline 沙啉thallium 铊thallium acetate 醋酸亚铊thallium chloride 氯化铊thallium monoxide 一氧化二铊thallium nitrate 硝酸亚铊thallium sulfate 硫酸亚铊thallium sulfide 硫化亚铊thallous compound 一价铊化合物thebaine 蒂巴因theine 咖啡碱thenard's blue 藤氏蓝thenardite 天然无水芒硝theobromine 可可碱theophylline 茶碱theorem of minimum entropy production 最小熵产生定理theoretical air requirement 理论空气量theoretical chemistry 理论化学theoretical organic chemistry 理论有机化学theoretical plate 理论板theoretical porosity 理论空隙率theoretical reaction temperature 理论反应温度theoretical yield 理论收率theory of electrolytic dissociation 电离理论theory of high polymer solution 高聚物溶液论theory of reaction rate 反应速率理论theory of similarity 相似理论theory of valence 原子价理论thermal absorption 热吸收thermal activation 热活化thermal analysis 热分析thermal black 热炭黑thermal conductivity 导热系数thermal cracking 热分解thermal decomposition 热分解thermal diffusion 热扩散thermal diffusivity 热扩散系数thermal dissociation 热力离解thermal efficiency 热效率thermal equilibrium 热平衡thermal equivalent 热当量thermal expansion 热膨胀thermal glass 耐热玻璃thermal neutron 热中子thermal plasticity 热塑性thermal polymerization 热聚合thermal radiation 热辐射thermal reforming 热重整thermal resistance 耐热性thermal resistor 热敏电阻器thermal shock 热冲击thermal stability 热稳定性thermal stress 热应力thermal treatment 热处理thermally stable polymer 耐热聚合物thermion 热离子thermionic current 热离子电流thermistor 热敏电阻器thermit 铝热剂thermite process 铝热法thermobalance 热天平thermochemical equation 热化学方程式thermochemistry 热化学thermochromism 热色现象thermocolor 示温涂料thermocouple 温差电偶thermocouple vacuum gage 热电偶式真空计thermodiffusion 热扩散thermodynamic equilibrium 热力平衡thermodynamical potential 热力势thermodynamics 热力学thermoelasticity 热弹性thermoelectric cell 热元件thermoelectric converter 热元件thermoelectric current 热电流thermoelectric pyrometer 热电偶高温计thermoelectric refrigeration 热电致冷thermoelectromotive force 温差电动势thermoelectron 热电子thermoelectronic emission 热电子放射thermograph 温度记录器thermogravimetric analysis 热重量分析thermoluminescence 热发光thermolysis 热分解thermolysis curve 热解曲线thermometer 温度计thermometric analysis 测温分析thermometric conductivity 温度传导率thermometric titration 测温滴定thermonuclear reaction 热核反应thermophile bacteria 耐热细菌thermopile 温差电堆thermoplastic resin 热塑尸thermoplasticity 热塑性thermoplastics 热塑塑料thermoregulator 温度第器thermoselective aromatization 热选择的芳化thermosetting adhesive 热硬化胶着剂thermosetting resin 热固性尸thermostat 恒温器thermostatic bimetal 恒温双金属thermotropic liquid crystal 热熔液晶thiamin 硫胺素thiamine diphosphate 二磷酸硫胺thiazine 噻嗪thiazine dyes 噻嗪染料thiazole 噻唑thiazole dye 噻唑染料thickener 浓缩器thin film 薄膜thin layer chromatography 薄层色谱法thinner 稀释剂thinning 稀释thinning agent 稀释剂thio acid 硫代酸thioacetal 硫缩醛thioacetamide 硫代乙酰胺thioacetazone 胺苯硫脲thioacetic acid 硫代乙酸thioalcohol 硫醇thioaldehyde 乙硫醛thioamide 硫代酰胺thiocarbamide 硫脲thiocarbanilide 均二苯硫脲thiocarbonate 硫代碳酸盐thiocarbonic acid 硫代碳酸thiocarbonyl chloride 二氯硫化碳thiochrome 硫色素thiocol 愈创木酚磺酸钾thiocresol 硫代甲酚thioctic acid 硫辛酸thiocyanate 硫氰酸盐thiocyanic acid 硫氰酸thiocyanic ester 硫氰酸酯thiocyanogen 硫化氰thiocyanogen value 硫氰值thiodan 硫丹thioether 硫醚thiofuran 噻吩thioindigo 硫靛thiokol 聚硫橡胶thiol 硫羟thiolic acid 硫羟酸thionic acid 硫羰酸thionine 亚氨嗪thionthiolic acid 硫羰代硫羰酸thionyl chloride 亚硫酰二氯thiophene 噻吩thiophenol 苯硫酚thiophosgene 硫光气thioplast 硫塑料thiosalicylic acid 硫代水杨酸thiosugar 硫糖thiosulfate 硫代硫酸盐thiosulfuric acid 硫代硫酸thiourea 硫脲素thioxene 二甲基噻吩thioxine dyes 硫化染料thiram 福美双third order reaction 三级反应thiuram 秋兰姆thixotropy 触变性thomas converter 托马斯转炉thomson's atom model 汤姆逊原子模型thomsonite 杆沸石thoria 氧化钍thorite 钍石thorium 钍thorium chloride 氯化钍thorium nitrate 硝酸钍thorium oxide 氧化钍thorium series 钍系thorium sulfate 硫酸钍thorpe reaction 苏反应thought experiment 思考实验thread like molecule 线型分子three color process 三原色印刷three dimensional structure 三维结构three high mill 三辊式轧机three way cock 三通阀threonine 苏氨酸threshold dose 临界剂量threshold treatment 极限处理threshold value 临界值throat 炉顶throat opening 炉口throat platform 炉顶操专thrombese 凝血酶thrombin 凝血酶thrombokinase 促凝血酶throughput 生产能力;量thujane 桧烷thujone 侧柏酮thulium 铥thymidine 胸腺嘧啶thymidylic acid 胸苷酸thymine 胸腺嘧啶thymol 百里酚thymol blue 百里酚蓝thymolphthalein 百里酚酞thymoquinone 百里香醌thyrocalcitonin 降钙素thyroid hormone 甲状腺激素thyrotropin 促甲状腺激素thyroxine。
(2021年整理)材料专业常用术语英语单词表

材料专业常用术语英语单词表编辑整理:尊敬的读者朋友们:这里是精品文档编辑中心,本文档内容是由我和我的同事精心编辑整理后发布的,发布之前我们对文中内容进行仔细校对,但是难免会有疏漏的地方,但是任然希望(材料专业常用术语英语单词表)的内容能够给您的工作和学习带来便利。
同时也真诚的希望收到您的建议和反馈,这将是我们进步的源泉,前进的动力。
本文可编辑可修改,如果觉得对您有帮助请收藏以便随时查阅,最后祝您生活愉快业绩进步,以下为材料专业常用术语英语单词表的全部内容。
Unit 1property (材料的)性质heat treatment 热处理metal 金属glass 玻璃plastics 塑料fiber 纤维electronic devices 电子器件component 组元,组分semiconducting materials 半导体材料materials science and engineering 材料科学与工程materials science 材料科学materials engineering 材料工程materials scientist 材料科学家materials engineer 材料工程师synthesize 合成synthesissubatomic structure 亚原子结构electron 电子atom 原子nuclei 原子核nucleusmolecule 分子microscopic 微观的microscope 显微镜naked eye 裸眼macroscopic 宏观的specimen 试样deformation 变形polished 抛光的reflect 反射magnitude 量级solid materials 固体材料mechanical properties 力学性质force 力elastic modulus 弹性模量strength 强度electrical properties 电学性质electrical conductivity 导电性dielectric constant 介电常数electric field 电场thermal behavior 热学行为heat capacity 热容thermal conductivity 热传导(导热性)magnetic properties 磁学性质magnetic field 磁场optical properties 光学性质electromagnetic radiation 电磁辐射light radiation 光辐射index of refraction 折射率reflectivity 反射率deteriorative characteristics 劣化特性processing 加工performance 性能linear 线性的integrated circuit chip 集成电路芯片strength 强度ductility 延展性deterioration 恶化,劣化mechanical strength 机械强度elevated temperature 高温corrosive 腐蚀性的fabrication 制造Unit 2chemical makeup 化学组成atomic structure 原子结构advanced materials 先进材料high-technology 高技术smart materials 智能材料nanoengineered materials 纳米工程材料metallic materials 金属材料nonlocalized electrons 游离电子conductor 导体electricity 电heat 热transparent 透明的visible light 可见光polished 抛光的surface 表面lustrous 有光泽的aluminum 铝silicon 硅alumina 氧化铝silica 二氧化硅oxide 氧化物carbide 碳化物nitride 氮化物dioxide 二氧化物clay minerals 黏土矿物porcelain 瓷器cement 水泥mechanical behavior 力学行为ceramic materials 陶瓷材料stiffness 劲度strength 强度hard 坚硬brittle 脆的fracture 破裂insulative 绝缘的resistant 耐……的resistance 耐力,阻力,电阻molecular structures 分子结构chain-like 链状backbone 骨架carbon atoms 碳原子low densities 低密度mechanical characteristics 力学特性inert 隋性synthetic (人工)合成的fiberglass 玻璃纤维polymeric 聚合物的epoxy 环氧树脂polyester 聚酯纤维carbon fiber—reinforced polymer composite 碳纤维增强聚合物复合材料glass fiber-reinforced materials 玻璃纤维增强材料high-strength, low-density structural materials 高强度低密度结构材料solar cell 太阳能电池hydrogen fuel cell 氢燃料电池catalyst 催化剂nonrenewable resource 不可再生资源Unit 3periodic table (元素)周期表atomic structure 原子结构magnetic 磁学的optical 光学的microstructure 微观结构macrostructure 宏观结构positively charged nucleus 带正电的原子核atomic number 原子序数proton 质子atomic weight 原子量neutron 中子negatively charged electrons 带负电的电子shell 壳层magnesium 镁chemical bonds 化学键partially-filled electron shells 未满电子壳层bond 成键metallic bond 金属键nonmetal atoms 非金属原子covalent bond 共价键ionic bond 离子键Unit 4physical properties 物理性质chemical properties 化学性质flammability 易燃性corrosion 腐蚀oxidation 氧化oxidation resistance 抗氧化性vapor (vapour)蒸汽,蒸气,汽melt 熔化solidify 凝固vaporize 汽化,蒸发condense 凝聚sublime 升华state 态plasma 等离子体phase transformation temperatures 相变温度density 密度specific gravity 比重thermal conductivity 热导linear coefficient of thermal expansion 线性热膨胀系数electrical conductivity and resistivity 电导和电阻corrosion resistance 抗腐蚀性magnetic permeability 磁导率phase transformations 相变phase transitions 相变crystal forms 晶型melting point 熔点boiling point 沸腾点vapor pressure 蒸气压atm 大气压glass transition temperature 玻璃化转变温度mass 质量volume 体积per unit of volume 每单位体积the acceleration of gravity 重力加速度temperature dependent 随温度而变的,与温度有关的grams/cubic centimeter 克每立方厘米kilograms/cubic meter 千克每立方米grams/milliliter 克每毫升grams/liter 克每升pounds per cubic inch 磅每立方英寸pounds per cubic foot 磅每立方英尺alcohol 酒精benzene 苯magnetize 磁化magnetic induction 磁感应强度magnetic field intensity 磁场强度constant 常数vacuum 真空magnetic flux density 磁通密度diamagnetic 反磁性的factor 因数paramagnetic 顺磁性的ferromagnetic 铁磁性的non-ferrous metals 非铁金属,有色金属brass 黄铜ferrous 含铁的ferrous metals 含铁金属,黑色金属relative permeability 相对磁导率transformer 变压器,变换器eddy current probe 涡流探针Unit 5hardness 硬度impact resistance 耐冲击性fracture toughness 断裂韧度,断裂韧性structural materials 结构材料anisotropic 各向异性orientation 取向texture 织构fiber reinforcement 纤维增强longitudinal 纵向transverse direction 横向short transverse direction 短横向a function of temperature 温度的函数,温度条件room temperature 室温elongation 伸长率tension 张力,拉力compression 压缩bending 弯曲shear 剪切torsion 扭转static loading 静负荷dynamic loading 动态载荷cyclic loading 循环载荷,周期载荷cross-sectional area 横截面stress 应力stress distribution 应力分布strain 应变engineering strain 工程应变perpendicular 垂直normal axis 垂直轴elastic deformation 弹性形变plastic deformation 塑性形变quality control 质量控制nondestructive tests 无损检测tensile property 抗张性能,拉伸性能Unit 6lattice 晶格positive ions 正离子a cloud of delocalized electrons 离域电子云ionization 电离,离子化metalloid 准金属,类金属nonmetal 非金属diagonal line 对角线polonium 钋semi—metal 半金属lower left 左下方upper right 右上方conduction band 导带valence band 价带electronic structure 电子结构synthetic materials (人工)合成材料oxygen 氧oxide 氧化物rust 生锈potassium 钾alkali metals 碱金属alkaline earth metals 碱土金属volatile 活泼的transition metals 过渡金属oxidize 氧化barrier layer 阻挡层basic 碱性的acidic 酸性的electrochemical series 电化序electrochemical cell 电化电池cleave 解理,劈开elemental 元素的,单质的metallic form 金属形态tightly-packed crystal lattice 密排晶格,密堆积晶格atomic radius 原子半径nuclear charge 核电荷number of bonding orbitals 成键轨道数overlap of orbital energies 轨道能重叠crystal form 晶型planes of atoms 原子面a gas of nearly free electrons 近自由电子气free electron model 自由电子模型an electron gas 电子气band structure 能带结构binding energy 键能positive potential 正势periodic potential 周期性势能band gap 能隙Brillouin zone 布里渊区nearly-free electron model 近自由电子模型solid solution 固溶体pure metals 纯金属duralumin 硬铝,杜拉铝Unit 9purification 提纯,净化raw materials 原材料discrete 离散的,分散的iodine 碘long—chain 长链alkane 烷烃,链烃oxide 氧化物nitride 氮化物carbide 碳化物diamond 金刚石graphite 石墨inorganic 无机的mixed ionic—covalent bonding 离子-共价混合键constituent atoms 组成原子conduction mechanism 传导机制phonon 声子photon 光子sapphire 蓝宝石visible light 可见光computer-assisted process control 计算机辅助过程控制solid—oxide fuel cell 固体氧化物燃料电池spark plug insulator 火花塞绝缘材料capacitor 电容electrode 电极electrolyte 电解质electron microscope 电子显微镜surface analytical methods 表面分析方法Unit 12macromolecule 高分子repeating structural units 重复结构单元covalent bond 共价键polymer chemistry 高分子化学polymer physics 高分子物理polymer science 高分子科学molecular structure 分子结构molecular weights 分子量long chains 长链chain—like structure 链状结构monomer 单体plastics 塑料rubbers 橡胶thermoplastic 热塑性thermoset 热固性vulcanized rubbers 硫化橡胶thermoplastic elastomer 热塑弹性体natural rubbers 天然橡胶synthetic rubbers 合成橡胶thermoplastic 热塑性thermoset 热固性resin 树脂polyethylene 聚乙烯polypropylene 聚丙烯polystyrene 聚苯乙烯polyvinyl—chloride 聚氯乙烯polyvinyl 聚乙烯的chloride 氯化物polyester 聚酯polyurethane 聚氨酯polycarbonate 聚碳酸酯nylon 尼龙acrylics 丙烯酸树脂acrylonitrile-butadiene—styrene ABS树脂polymerization 聚合(作用)condensation polymerization 缩聚addition polymerization 加聚homopolymer 均聚物copolymer 共聚物chemical modification 化学改性terminology 术语nomenclature 命名法chemist 化学家the Noble Prize in Chemistry 诺贝尔化学奖catalyst 催化剂atomic force microscope 原子力显微镜(AFM) Unit 15composite 复合材料multiphase 多相bulk phase 体相matrix 基体matrix material 基质材料reinforcement 增强体reinforcing phase 增强相reinforcing material 加强材料metal—matrix composite 金属基复合材料ceramic—matrix composite 陶瓷基复合材料resin—matrix composite 树脂基复合材料strengthening mechanism 增强机理dispersion strengthened composite 弥散强化复合材料particle reinforced composites 颗粒增强复合材料fiber—reinforced composites 纤维增强复合材料Unit 18nanotechnology 纳米技术nanostructured materials 纳米结构材料nanometer 纳米nanoscale 纳米尺度nanoparticle 纳米颗粒nanotube 纳米管nanowire 纳米线nanorod 纳米棒nanoonion 纳米葱nanobulb 纳米泡fullerene 富勒烯size parameters 尺寸参数size effect 尺寸效应critical length 临界长度mesoscopic 介观的quantum mechanics 量子力学quantum effects 量子效应surface area per unit mass 单位质量的表面积surface physics and chemistry 表面物理化学substrate 衬底,基底graphene 石墨烯chemical analysis 化学分析chemical composition 化学成分analytical techniques 分析技术scanning tunneling microscope 扫描隧道显微镜spatial resolution 空间分辨率de Brogile wavelength 德布罗意波长mean free path of electrons (电子)平均自由程quantum dot 量子点band gap 带隙continuous density of states 连续态密度discrete energy level 离散能级absorption 吸收infrared 红外ultraviolet 紫外visible 可见quantum confinement (effect) 量子限域效应quantum well 量子势阱optoelectronic device 光电子器件energy spectrum 能谱electron mean free path 电子平均自由程spin relaxation length 自旋弛豫长度Unit 21biomaterial 生物材料implant materials 植入材料biocompatibility 生物相容性in vivo 在活体内in vitro 在活体外organ transplant 器管移植calcium phosphate 磷酸钙hydroxyapatite 羟基磷灰石research and development 研发 R&D Preparation & Characterizationprocessing techniques 加工技术casting 铸造rolling 轧制,压延welding 焊接ion implantation 离子注入thin—film deposition 薄膜沉积crystal growth 晶体生长sintering 烧结glassblowing 玻璃吹制analytical techniques 分析技术characterization techniques 表征技术electron microscopy 电子显微术X—ray diffraction X射线衍射calorimetry 量热法Rutherford backscattering 卢瑟福背散射neutron diffraction 中子衍射nuclear microscopy 核子微探针。
- 1、下载文档前请自行甄别文档内容的完整性,平台不提供额外的编辑、内容补充、找答案等附加服务。
- 2、"仅部分预览"的文档,不可在线预览部分如存在完整性等问题,可反馈申请退款(可完整预览的文档不适用该条件!)。
- 3、如文档侵犯您的权益,请联系客服反馈,我们会尽快为您处理(人工客服工作时间:9:00-18:30)。
a r X i v :c o n d -m a t /0402234v 2 [c o n d -m a t .s t r -e l ] 13 F eb 2004Specific heat study of spin-structural change in pyrochlore Nd 2Mo 2O 7Y.Onose 1,Y.Taguchi 2∗,T.Ito 3,and Y.Tokura 1,2,31Spin Superstructure Project,ERATO,Japan Science and Technology Agency (JST),Tsukuba 305-8562,Japan2Department of Applied Physics,University of Tokyo,Tokyo 113-8656,Japan 3Correlated Electron Research Center (CERC),National Institute of Advanced Industrial Science and Technology (AIST),Tsukuba 305-8562,JapanBy measurements of specific heat,we have investigated the magnetic field (H )induced spin-structural change in Nd 2Mo 2O 7that shows spin-chirality-related magneto-transport phenomena.A broad peak around 2K caused by the ordering of 2-in 2-out structure of the Nd moments at zero H shifts to the lower temperature (T )up to around 3T and then to the higher T above around 3T with increasing H for all the direction of H .This is due to the crossover from antiferromagnetic to ferromagnetic arrangement between the Nd and Mo moments.While the peak T increases monotonically above 3T for H //[100],another peak emerges around 0.9K at 12T for H //[111],which is ascribed to the ordering of 3-in 1-out structure.For H //[110],a spike like peak is observed at around 3T,which is caused perhaps by some spin flip transition.PACS numbers:,75.30.Mb,75.30.KzNovel magnetic phenomena are frequently observed due to geometrical frustration in pyrochlore oxides where the magnetic ions reside on the vertices of linked tetrahe-dra.One such example is the so-called “spin ice”state in R 2Ti 2O 7(R =Ho,Dy).1In Dy 2Ti 2O 7,for example,the ferromagnetic interaction among Ising-like Dy moments induces the 2-in 2-out structure,in which the two Dy mo-ments in a tetrahedron point inward and the other two moments point outward.However,there are macroscop-ically large numbers of spin structures satisfying the 2-in 2-out rule.Consequently,the macroscopically degener-ate ground state is realized in this material as shown by Ramirez et al .2In Nd 2Mo 2O 7,the Nd moments show the 2-in 2-out structure similarly to the spin ice materials.3,4In this ma-terial,however,the spin-polarized itinerant Mo 4d elec-trons coexist with the Nd moments.The Mo and Nd sub-lattices have the same structure,but are displaced from each other by half a unit cell.Although the anisotropy of the Mo 4d moments is small,the Mo moments are slightly (at most by several degrees 3,4)tilted from the direction of the net magnetization due to the antiferromagnetic in-teraction with the Ising-like Nd moments.Recently,an unusual behavior of anomalous Hall effect was observed in Nd 2Mo 2O 7and the origin was proposed to be the spin chirality induced by such a non-coplanar spin structure of Mo moments.3In this paper,we investigate the spin-structural change in Nd 2Mo 2O 7by means of specific heat measurements.Most of the arguments are concerning the Ising-like Nd moments.We find many behaviors similar to those of the spin ice materials although zero point entropy is not observed in this material.Some of these results provide a supporting evidence of the spin chirality scenario for the unusual anomalous Hall effect.2magnetization curves at1.7K for the H//[100],[111], and[110]axes in Figs.1(a),(b),and(c),respectively.5 For all thefield directions,enough high-H reverses the Nd moments,resulting in the saturate moments that are much larger than the Mo spin moment(≈1.4µB/Mo). For H//[100]and[110],the saturate moments are in ac-cord with those expected for the2-in2-out structure of the Nd moments(see the insets of Figs.1(a)and(c)) with the magnitude being g effJ≈2.3µB.These ex-pected values are indicated by the dashed arrows.On the other hand,in the case of H//[111],the saturate moment coincides with that expected for the3-in1-out structure (see the inset of Fig.1(b)),which is also indicated by the dashed arrow.In the canonical case of Dy2Ti2O7, the metamagnetic transition from the2-in2-out to3-in 1-out structure is observed as a step-like increase in the magnetization curve for H//[111].6,7However,any trace of such a step is hardly observed in Nd2Mo2O7down to 70mK.5We measured the specific heat by the conventional re-laxation method.The specific heat of Nd2Mo2O7at zero H is shown in Fig.1(e).A small peak is discerned around 90K as indicated by the closed triangle.This is owing to the ferromagnetic transition of the Mo spin.In the low-T region(≤15K),the specific heat is dominated by a intense peak,which is caused by the ordering of 2-in2-out structure in the Nd sub-lattice.These fea-tures observed at zero H have already been reported in literature.8We also plot the T-dependence of the entropy S deduced from the T-integration of C(T)/T.For the analysis,we assumed the linear relation in C/T below the lowest T(≈0.4K).The S at15K is almost in ac-cord with the value of R ln2,which is expected as from the degree of freedom of Ising moments.Therefore,the entropy of Ising-like Nd moments is mostly released in the low-T(≤15K)region.In Figs.2(a)and(b),we show the T-and H-variation of specific heat of the Nd2Mo2O7crystal below15K for H//[100],in which all of the four Nd moments in a tetra-hedron make the same angle with the H(see the inset of Fig.1(a)).Whereas the peak due to the ordering of the2-in2-out structure becomes sharper with increas-ing H below3T,it becomes broader and the peak T increases as H is increased from3T.We plot the H-variation of the S at4K and the peak T in Figs.2(c) and(d),respectively.Reflecting the crossover around3 T in the specific heat,the S at4K shows down-turn and the peak T shows up-turn around3T.In the canonical case of Dy2Ti2O7,the ordering T of2-in2-out structure increases with increasing H along the[100]direction.9 In the present case,the effectivefield for the Nd mo-ments that is the sum of the applied H and the negative molecularfield from Mo spins changes its sign from neg-ative to positive at around3T.This is the reason for the non-monotonic H-dependence of S at4K and peak T. Therefore,the crossover around3T can be ascribed to the reversal of the Nd moments,which is consistent with the results of recent neutron measurements.10,11FIG.2:(a),(b):Temperature(T)and magneticfield(H) variation of specific heat for H//[100].(c):H-dependence of entropy(S)at4K and28K for H//[100].(d):H-variation of the peak T in the specific heat data shown in(a)and(b).The vertical dot-dashed line in(c)and(d)indicates the crossover H from the antiferromagnetic to ferromagnetic arrangement between Nd and Mo moments.Solid lines in(c)and(d)are merely the guide for the eyes.We plot the S at28K for H//[100]in Fig.2(c). The entropy originating from the Nd moments is almost completely released below28K.Almost H-independent S at28K indicates the absence of zero point entropy even at zero effectivefield for Nd moments,namely at around H=3T,where the decrease of S would otherwise be observed.(The slight decrease of the entropy in the high-H region corresponds to its transfer to the higher-T region above28K.)Thus,the ground state degeneracy seems to be lifted by the interaction with the itinerant Mo4d electrons even around3T.The S at28K shows a similar behavior in the cases of H//[111]and H//[110] (see Fig.3(d)and Fig.4(c)),suggesting that zero point entropy has not been observed in any configuration for Nd2Mo2O7.We show the T-and H-variation of specific heat of the Nd2Mo2O7crystal for H//[111]in Figs.3(a)-(c).In this configuration,one out of four Nd moments in a tetrahe-dron is parallel to the H as shown in the inset of Fig. 1(b).Similarly to the case of H//[100],the peak due to the ordering of the2-in2-out structure becomes sharper with increasing H up to3T owing to the decrease of the total effectivefield.The crossover around3T is also discerned in the S at4K as shown in Fig.3(d).Above 3T,the peak becomes broader with increasing H while the shift of the peak is less significant compared with the case of H//[100].Above around9T,the specific heat in the low-T region(T<1.3K)is gradually enhanced with increasing H.At11T,the specific heat shows a much broader peak,which may be viewed as composed of sev-eral peaks.Then,at12T,another clear peak emerges at low T(≈0.9K).A knee-like structure is also observed around4K in the12T data.It is worth noting hereFIG.3:(a)-(c):Temperature(T)and magneticfield(H) variation of specific heat for H//[111].(d):H-dependence of entropy(S)at1.3K,4K,and28K for H//[111].(e):Char-acteristic T obtained by the specific heat data for H//[111]. Closed and open circles show the peak T due to the ordering of the2-in2-out and3-in1-out structure,respectively.An open triangle shows the T-position of the knee-like structure in the12T data.An open square shows the T-position of the broader peak in the11T data.The vertical dot-dashed line in(d)and(e)represents the crossover H at low T from the2-in2-out to the3-in1-out structure.Solid,dashed,and hatched lines in(d)and(e)are merely the guide for the eyes. that in Dy2Ti2O7as the reference material,quite a simi-lar peak emerges at low T when the H(>∼1T)is appliedto the[111]direction.9This is ascribed to the ordering of the3-in1-out structure(see the inset of Fig.1(b)). In analogy to this,the low-T peak in the12T data for the present compound can also be ascribed to the emer-gence of the3-in1-out structure.This assignment is also supported by the fact that the saturate moment for H//[111]almost coincides with that expected by the3-in1-out structure above12T.We plot the peak T due to the ordering of the2-in2-out structure as with closed circles in Fig.3(e).An upward shift of the peak T is observed around3T similarly to the case of H//[100]. The peak T shows a kink at6T,above which the peak T slightly decreases with H.We also plot in Fig.3(e)the T positions of the peak due to the ordering of the3-in 1-out structure and the knee-like structure in the12T data with an open circle and triangle,respectively.The T-position of the broad peak at11T is also shown as an open square.The peak due to the ordering of the3-in1-out structure exists in the lower-T region compared with that of the2-in2-out structure in the lower-H data.The broad specific-heat peak at11T ranging over the wide temperature region(1.0K≤T≤2.7K),as indicated by the open square with a long vertical bar,can be as-cribed to this heavily mixed state of the2-in2-out and 3-in1-out configuration in this T-and H-region. There are several differences in the specific heat for H//[111]between the cases of Nd2Mo2O7and Dy2Ti2O7 while the3-in1-out structure is commonly observed in these materials.In the specific heat of Dy2Ti2O7,the peak due to the ordering of the Dy moments at the apical position of the tetrahedron(see the inset of Fig.1(b)) parallel to H emerges in the higher-T region than the ordering T of the2-in2-out structure when the H is ap-plied along the[111]direction.9This is because the mo-ments parallel to H are more amenable to the H than the other moments.However,for Nd2Mo2O7,no clear peak structure of the specific heat is discerned in the higher T region.Although the specific heat concerning the or-dering of the moments parallel to H may be distributed above the peak T of the2-in2-out structure in the suffi-ciently high-H region,the interaction with spin polarized itinerant carriers possibly tends to smear out the high-T peak in the case of Nd2Mo2O7.(The kink in the peak T around6T in Fig.3(e)may indicate the onset of the separation of the parallel-moment component from the main peak.)Nevertheless,there is a remnant of such a high-T peak in the specific heat of Nd2Mo2O7,such as a knee-like structure at around4K at12T.Another dif-ference is that the spin structure gradually changes from the2-in2-out to3-in1-out structure for Nd2Mo2O7in contrast to thefirst order phase transition in Dy2Ti2O7. In the specific heat,the low-T component(T<1.3K) gradually increases above around9T.These suggest that the correlation of the3-in1-out structure evolves even in the lower H region than12T.To obtain the onset H of the correlation of the3-in1-out structure,we plot the H-dependence of the S at1.3K in Fig.3(d).The quantity shows a minimum at around H=8T,which is thought to be the onset H of the correlation of the3-in1-out struc-ture.Recently,Taguchi et al.observed the sign change in the Hall resistivity for H//[111]and ascribed it to the reversal of the spin chirality caused by the spin-structural change from the2-in2-out to3-in1-out structure.5The Hall resistivity crosses zero at around H=8T,which co-incides with the onset H of the correlation of the3-in 1-out structure estimated by the present results.This is another evidence supporting the spin chirality scenario for the anomalous Hall effect.We show the T-and H-variation of the specific heat of the Nd2Mo2O7crystal for H//[110]in Figs.4(a)and (b).In this configuration,two out of four Nd moments in a tetrahedron are perpendicular to the H(see the in-set of Fig.1(c)).Similarly to the cases of H//[100]and H//[111],the peak around2K becomes sharper up to3 T and then broader above3T with increasing H.The H-variation of the peak T and S at4K is shown in Figs.4 (c)and(d),respectively.Corresponding to the crossover around3T,the S at4K shows the maximum.The peak T shows a local minimum around3T and then a kink around6T.In Dy2Ti2O7,a peak emerges in the higher-T region than the peak T due to the ordering of the2-in 2-out structure.12The higher-T peak is ascribed to the ordering of the half of Dy moments that are not perpen-dicular to the H.In Nd2Mo2O7,by contrast,the higherFIG.4:(a),(b):Temperature(T)and magneticfield(H) variation of specific heat for H//[110].Inset in(a)shows the detailed specific-heat data in the T-and H-region where the spike-like peak is observed.(c):H-dependence of entropy(S) at4K and28K for H//[110].(d):T-positions of the broad peak around2K and the spike-like peak(closed and open circles,respectively).Solid and dashed lines in(c)and(d) are merely the guide for the eyes.T peak is smeared out possibly due to the interaction with the Mo4d electrons and the kink around6T in the peak T may indicate the onset H,above which the half of the moments order ahead of the other two,similarly to the case of H//[111].Because the Nd moments perpen-dicular to the H are hardly affected by the H,the peak T is kept almost constant in the high-T region above8 T,which is similar to the Dy2Ti2O7case.12Another distinct feature for H//[110]is the presence of a spike-like peak observed only between2.5T and4.0 T,as exemplified in the inset of Fig.4(a).The peak becomes sharper and the peak T increases from1.2K to 2.7K with H in this H-region.In Fig.4(d),we plot with open circles the T where the spike-like peak emerges. The spike-like peak mainly exists below the peak T due to the ordering of the2-in2-out structure.Such a spike-like peak as releasing minimal entropy is expected in the case of a spin-flip transition.Then,the question is what kind of the spin-flip transition occurs.One possibility is the aforementioned transition from the antiferromagnetic to ferromagnetic arrangement between Nd and Mo mo-ments.As discussed above,the transition exists around 3T where the spike-like peak is observed.However,the transition is not specific for H//[110].Hence,the spike-like peak should be observed also for the other configu-rations in this case.Another possibility is the spin-flip transition concerning the Nd moments perpendicular to H.Even if the2-in2-out structure is assumed,there are two possible arrangements of the Nd moments per-pendicular to H,namely“in-out”and“out-in”,in ev-ery tetrahedron for H//[110](see the inset of Fig.1(c)). Therefore,there might be the spin-flip transition between nearly degenerate two spin-structural phases where the arrangements of the perpendicular moments are different from each other.However,the recent neutron measure-ment suggests that the net perpendicular moments does not change up to5T.10At present,we cannot draw a definite conclusion about the origin of the spin-flip transi-tion.More detailed investigations in terms of diffraction measurements would be needed.In summary,we have investigated the specific heat in Nd2Mo2O7as functions of direction and magnitude of H as well as T.A broad peak is observed around2K at zero H,which is owing to the ordering of the2-in2-out structure of the Nd moments.The peak T decreases up to around3T and then increases above around3 T with increasing H irrespective of the direction of H. This is due to the crossover from antiparallel to parallel arrangement between the net magnetizations of the Nd and Mo moments.The peak T increases monotonically above3T for H//[100].On the other hand,in the case of H//[111],another peak emerges at around0.9K at 12T,owing to the ordering of3-in1-out structure of the Nd moments.This is consistent with the sign reversal of the Hall resistivity reported by a previous study.5For H//[110],a spike-like peak with minimal entropy release is observed at around3T,which is ascribed to some spin flip transition.The authors thank N.Nagaosa,S.Onoda,H.Takagi, T.Arima,Y.Oohara,and H.Yoshizawa for enlightening discussions.The present work was in part supported by Grant-In-Aid for Scientific Research from the MEXT and by the NEDO.1S.T.Bramwell and M.J.P.Gingras,Science294,1495 (2001)and references therein.2A.P.Ramirez,A.Hayashi,R.J.Cava,R.Siddharthan, and B.S.Shastry,Nature(London)399,333(1999).3Y.Taguchi,Y.Oohara,H.Yoshizawa,N.Nagaosa,and Y. Tokura,Science291,2573(2001).4Y.Yasui,Y.Kondo,M.Kanada,M.Ito,H.Harashina,M. Sato,and K.Kakurai,J.Phys.Soc.Jpn.70,284(2001). 5Y.Taguchi,T.Sasaki,S.Awaji,Y.Iwasa,T.Tayama,T. Sakakibara,S.Iguchi,T.Ito,and Y.Tokura,Phys.Rev.Lett.90,257202(2003).6H.Fukazawa,R.G.Melko,R.Higashinaka,Y.Maeno,and M.J.P.Gingras,Phys.Rev.B65,054410(2002).7T.Sakakibara,T.Tayama,Z.Hiroi,K.Matsuhira,and S.Takagi,Phys.Rev.Lett.90,207205(2003).8S.Yoshii,K.Murata,and M.Sato,J.Phys.Soc.Jpn.71, 997(2002).9Z.Hiroi,K.Matsuhira,S.Takagi,T.Tayama,and T.Sakakibara,J.Phys.Soc.Jpn.72,411(2003).10Y.Oohara,H.Yoshizawa,Y.Taguchi,and Y.Tokura,un-published results.11Y.Yasui,S.Iikubo,H.Harashina,T.Kageyama,M.Ito, M.Sato,and K.Kakurai,J.Phys.Soc.Jpn.72,284 (2003).12Z.Hiroi,K.Matsuhira,and M.Ogata,J.Phys.Soc.Jpn.72,3045(2003).。