Stability enhancement of ZnTPPS in acidic aqueous solutions by polymeric micelles
稳定性和一致性是中国铸造业亟待解决的问题
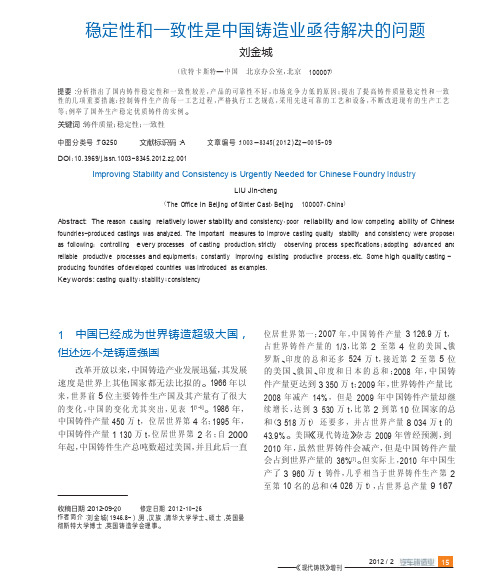
稳定性和一致性是中国铸造业亟待解决的问题刘金城(欣特卡斯特—中国 北京办公室,北京 100007)提要:分析指出了国内铸件稳定性和一致性较差,产品的可靠性不好,市场竞争力低的原因;提出了提高铸件质量稳定性和一致性的几项重要措施:控制铸件生产的每一工艺过程,严格执行工艺规范,采用先进可靠的工艺和设备,不断改进现有的生产工艺 等;例举了国外生产稳定优质铸件的实例。
关键词:铸件质量;稳定性;一致性 中图分类号:TG250文献标识码:A文章编号:1003-8345(2012)Z 2-0015-09DOI :10.3969/j.issn.1003-8345.2012.z 2.001 Improving Stability and Consistency is Urgently Needed for Chinese Foundry IndustryLIU Jin-cheng(The Office in Beijing of Sinter Cast ,Beijing 100007,Chin a )Abstract: The reason causing relatively lower stability and consistency ,poor reliability and low competing ability of Chinese foundrie s -produced castings was analyzed. The important measures to improve casting quality stability and consistency were proposed as following :controlling every processes of casting production ;strictly observing process specifications ;adopting advanced and reliable productive processes and equipments ;constantly improving existing productive process ,etc. Some high quality casting - producing foundries of developed countries was introduced as examples. Key words: casting quality ;stabilit y ;consistency位居世界第一;2007 年,中国铸件产量 3 126.9 万 t ,占世界铸件产量的 1/3,比第 2 至第 4 位的美国、俄 罗斯、印度的总和还多 524 万 t ,接近第 2 至第 5 位 的美国、俄国、印度和日本的总和;2008 年,中国铸 件产量更达到 3 350 万 t ;2009 年,世界铸件产量比 2008 年减产 14%,但是 2009 年中国铸件产量却继 续增长,达到 3 530 万 t ,比第 2 到第10 位国家的总 和(3 518 万t ) 还要多,并占世界产量 8 034 万 t 的 43.9%。
Enhanced Photocatalytic Hydrogen Evolution over Cu-Doped ZnIn2S4 under Visible Light Irradiation
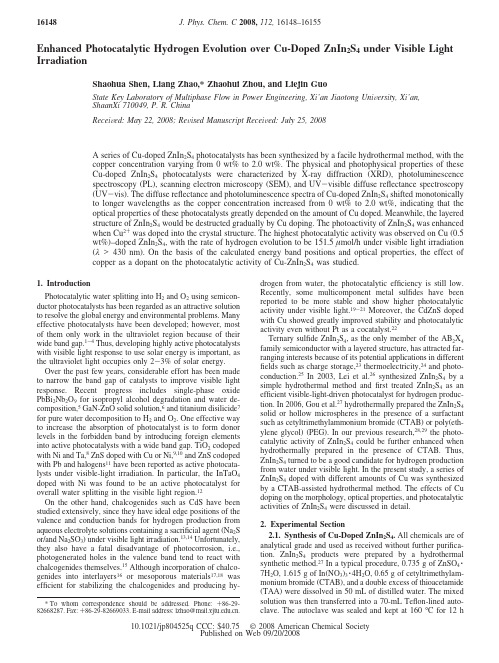
Enhanced Photocatalytic Hydrogen Evolution over Cu-Doped ZnIn 2S 4under Visible Light IrradiationShaohua Shen,Liang Zhao,*Zhaohui Zhou,and Liejin GuoState Key Laboratory of Multiphase Flow in Power Engineering,Xi’an Jiaotong Uni V ersity,Xi’an,ShaanXi 710049,P.R.ChinaRecei V ed:May 22,2008;Re V ised Manuscript Recei V ed:July 25,2008A series of Cu-doped ZnIn 2S 4photocatalysts has been synthesized by a facile hydrothermal method,with the copper concentration varying from 0wt%to 2.0wt%.The physical and photophysical properties of these Cu-doped ZnIn 2S 4photocatalysts were characterized by X-ray diffraction (XRD),photoluminescence spectroscopy (PL),scanning electron microscopy (SEM),and UV -visible diffuse reflectance spectroscopy (UV -vis).The diffuse reflectance and photoluminescence spectra of Cu-doped ZnIn 2S 4shifted monotonically to longer wavelengths as the copper concentration increased from 0wt%to 2.0wt%,indicating that the optical properties of these photocatalysts greatly depended on the amount of Cu doped.Meanwhile,the layered structure of ZnIn 2S 4would be destructed gradually by Cu doping.The photoactivity of ZnIn 2S 4was enhanced when Cu 2+was doped into the crystal structure.The highest photocatalytic activity was observed on Cu (0.5wt%)–doped ZnIn 2S 4,with the rate of hydrogen evolution to be 151.5µmol/h under visible light irradiation (λ>430nm).On the basis of the calculated energy band positions and optical properties,the effect of copper as a dopant on the photocatalytic activity of Cu-ZnIn 2S 4was studied.1.IntroductionPhotocatalytic water splitting into H 2and O 2using semicon-ductor photocatalysts has been regarded as an attractive solution to resolve the global energy and environmental problems.Many effective photocatalysts have been developed;however,most of them only work in the ultraviolet region because of their wide band gap.1-4Thus,developing highly active photocatalysts with visible light response to use solar energy is important,as the ultraviolet light occupies only 2-3%of solar energy.Over the past few years,considerable effort has been made to narrow the band gap of catalysts to improve visible light response.Recent progress includes single-phase oxide PbBi 2Nb 2O 9for isopropyl alcohol degradation and water de-composition,5GaN-ZnO solid solution,6and titanium disilicide 7for pure water decomposition to H 2and O 2.One effective way to increase the absorption of photocatalyst is to form donor levels in the forbidden band by introducing foreign elements into active photocatalysts with a wide band gap.TiO 2codoped with Ni and Ta,8ZnS doped with Cu or Ni,9,10and ZnS codoped with Pb and halogens 11have been reported as active photocata-lysts under visible-light irradiation.In particular,the InTaO 4doped with Ni was found to be an active photocatalyst for overall water splitting in the visible light region.12On the other hand,chalcogenides such as CdS have been studied extensively,since they have ideal edge positions of the valence and conduction bands for hydrogen production from aqueous electrolyte solutions containing a sacrificial agent (Na 2S or/and Na 2SO 3)under visible light irradiation.13,14Unfortunately,they also have a fatal disadvantage of photocorrosion,i.e.,photogenerated holes in the valence band tend to react with chalcogenides themselves.15Although incorporation of chalco-genides into interlayers 16or mesoporous materials 17,18was efficient for stabilizing the chalcogenides and producing hy-drogen from water,the photocatalytic efficiency is still low.Recently,some multicomponent metal sulfides have been reported to be more stable and show higher photocatalytic activity under visible light.19-21Moreover,the CdZnS doped with Cu showed greatly improved stability and photocatalytic activity even without Pt as a cocatalyst.22Ternary sulfide ZnIn 2S 4,as the only member of the AB 2X 4family semiconductor with a layered structure,has attracted far-ranging interests because of its potential applications in different fields such as charge storage,23thermoelectricity,24and photo-conduction.25In 2003,Lei et al.26synthesized ZnIn 2S 4by a simple hydrothermal method and first treated ZnIn 2S 4as an efficient visible-light-driven photocatalyst for hydrogen produc-tion.In 2006,Gou et al.27hydrothermally prepared the ZnIn 2S 4solid or hollow microspheres in the presence of a surfactant such as cetyltrimethylammonium bromide (CTAB)or poly(eth-ylene glycol)(PEG).In our previous research,28,29the photo-catalytic activity of ZnIn 2S 4could be further enhanced when hydrothermally prepared in the presence of CTAB.Thus,ZnIn 2S 4turned to be a good candidate for hydrogen production from water under visible light.In the present study,a series of ZnIn 2S 4doped with different amounts of Cu was synthesized by a CTAB-assisted hydrothermal method.The effects of Cu doping on the morphology,optical properties,and photocatalytic activities of ZnIn 2S 4were discussed in detail.2.Experimental Section2.1.Synthesis of Cu-Doped ZnIn 2S 4.All chemicals are of analytical grade and used as received without further purifica-tion.ZnIn 2S 4products were prepared by a hydrothermal synthetic method.27In a typical procedure,0.735g of ZnSO 4·7H 2O,1.615g of In(NO 3)3·4H 2O,0.65g of cetyltrimethylam-monium bromide (CTAB),and a double excess of thioacetamide (TAA)were dissolved in 50mL of distilled water.The mixed solution was then transferred into a 70-mL Teflon-lined auto-clave.The autoclave was sealed and kept at 160°C for 12h*To whom correspondence should be addressed.Phone:+86-29-82668287.Fax:+86-29-82669033.E-mail address:lzhao@.J.Phys.Chem.C 2008,112,16148–161551614810.1021/jp804525q CCC:$40.75 2008American Chemical SocietyPublished on Web 09/20/2008and then cooled to room temperature naturally.A yellow precipitate was obtained,which was then filtered and washed with absolute ethanol and distilled water for several times.After being dried in a vacuum at 80°C,ZnIn 2S 4was obtained.For the synthesis of Cu-doped ZnIn 2S 4,a desired amount of 0.1M Cu(NO 3)2solution was added into the mixed solution before transferring into the autoclave.ZnIn 2S 4doped with different amounts of Cu is denoted as Cu(X )-ZnIn 2S 4;the value of X ,from 0wt%to 2.0wt%,is used to describe the weight content of Cu.2.2.Characterization.The X-ray diffraction (XRD)patterns were obtained from a PANalytical X’pert MPD Pro diffracto-meter using Ni-filtered Cu K R irradiation (Wavelength 1.5406Å).The diffuse reflection of the samples was determined by a Hitachi U-4100UV -vis -near-IR spectrophotometer.The analysis of photoluminescence spectra (PL)was carried out at room temperature using a PTI QM-4fluorescence spectropho-tometer.The sample morphology was observed by a JEOL JSM-6700FE scanning electron microscope.2.3.Evaluation of Photocatalytic Activity.Photocatalytic hydrogen evolution was performed in a gas-closed circulation system with a top window Pyrex cell.A 300W Xe lamp was used as the light source,and the UV part of the light was removed by a cutoff filter (λ>430nm).Hydrogen evolved was analyzed by an online thermal conductivity detector (TCD)gas chromatograph (NaX zeolite column,nitrogen as a carrier gas)every 10min.In all experiments,the photocatalyst powder (0.2g)was dispersed by a magnetic stirrer in 150mL of 0.25M Na 2SO 3/0.35M Na 2S aqueous solution.Here,Na 2SO 3/Na 2S mixed sacrificial agent was used to scavenge photogenerated holes.Nitrogen was purged through the cell before reaction to remove oxygen.Pt (1wt%)as a cocatalyst for the promotion of hydrogen evolution was photodeposited in situ on the photocatalysts from the precursor of H 2PtCl 6·6H 2O.The tem-perature for all the photocatalytic reactions was kept at 25(0.5°C.The blank experiments showed no appreciable H 2evolution in the absence of irradiation or photocatalyst.Apparent quantum yield defined by the eq 1was measured using a 420nm band-pass filter and an irradiatometer.A.Q.Y.(%))the number of reacted electronsthe number of incident photons×100)the number of evolved H 2molecules ×2the number of incident photons×100(1)2.4.Band Structure Calculation.The plane-wave-based density functional theory (DFT)calculation was carried out for ZnIn 2S 4with hexagonal structure by employing the CASTEPprogram to obtain further information about the energy structure of the Cu-ZnIn 2S 4photocatalysts.30The core electrons were treated using ultrasoft pseudopotentials,31and the valence electronic configurations for Zn,In,and S are 3d 104s 2,4d 105s 25p 1,and 3s 23p 4,respectively.The kinetic energy cutoff was taken to be 400eV for ZnIn 2S 4.The exchange and correlation interactions were modeled using the generalized gradient approximation (GGA).The calculation was carried out using the primitive unit cell of [ZnIn 2S 4]2,which had 31occupied orbitals.The atomic coordinates of ZnIn 2S 4were obtained from Gou et al.273.Results and Discussion3.1.Crystal Structure.Figure 1A shows X-ray diffraction patterns of Cu(X )-ZnIn 2S 4photocatalysts (X )0wt%to 2.0wt%).Without Cu doping,the diffraction peaks of the obtained ZnIn 2S 4sample can be readily indexed as a pure phase hexagonal ZnIn 2S 4(ICSD-JCPDS card No.01-072-0773,a )3.85Å,c )24.68Å),and no other impurities such as ZnS,In 2S 3,oxides,or organic compounds related to reactants were detected.When ZnIn 2S 4was doped with Cu,the XRD patterns of the obtained Cu(X )-ZnIn 2S 4were almost the same as that of the hexagonal ZnIn 2S 4,except that the diffraction peaks were slightly shifted to higher angles with the amount of Cu doped increasing,as shown in Figure 1B.This observation suggested that Cu 2+was homogeneously incorporated into the lattice of ZnIn 2S 4,because the ionic radius of Cu 2+(0.72Å)is smaller than the radius of Zn 2+(0.74Å)and In 3+(0.81Å).32Furthermore,Cu 2+may occupy the Zn 2+site,since charge compensation was very easy in this case,as deduced by Shionoya et al.33An XRD pattern of CuS around 2θ)30°could be easily observed while the amount of Cu doped was close to 2.0wt%.This was due to the limitation of doping amount in the ZnIn 2S 4lattice.113.2.Morphology.Figure 2shows SEM images for Cu(X )-ZnIn 2S 4with X )0wt%to 2.0wt%.During the hydrothermal process,addition of Cu ion directly affects the morphology of Cu-ZnIn 2S4.When none of the Cu was doped,ZnIn 2S 4exhibited a lot of separate microspheres with diameters of 1-2µm,as shown in Figure 2a.Further observation showed that all the microspheres were composed of numerous petals/sheets.This growth tendency of lamellar structures might be related to the layered feature of hexagonal ZnIn 2S 4.27When X )0.1wt%to 0.5wt %,ZnIn 2S 4products still appeared in the shape of microspheres;however,increasing the amount of Cu doped led to gradual decrease in the diameter of Cu(X )-ZnIn 2S 4micro-spheres.The diameter of microsphere was about 0.5-1µmforFigure 1.X-ray diffraction patterns of Cu(X )-ZnIn 2S 4;the values of X were (a)0.0wt%,(b)0.1wt%,(c)0.3wt%,(d)0.5wt%,(e)0.7wt%;(f)0.9wt%,(g)1.2wt%,(h)1.6wt%,(i)2.0wt%.Enhanced Photocatalytic Hydrogen Evolution J.Phys.Chem.C,Vol.112,No.41,200816149Figure 2.Part 1of 2.16150J.Phys.Chem.C,Vol.112,No.41,2008Shen et al.Cu(0.5wt%)-ZnIn 2S 4.As X increased further,the shape of microspheres for Cu(X )-ZnIn 2S 4was destructed partially and even throughly,as shown in Figure 2e -g,as well as the petals/sheets presented to be more irregular.When X )1.6wt%to 2.0wt %,instead of microspheres and petals/sheets,Cu(X )-ZnIn 2S 4turned out to be a bulky conglomeration with a rough surface,and petals/sheets could be hardly observed.The morphology of the Cu(X )-ZnIn 2S 4samples obtained in the same synthetic condition varies regularly with the increasing amount of Cu doped.That is to say,the layered structure of hexagonal ZnIn 2S 4,as well as the shape of microspheres,could be destructed gradually by the increasing Cu dopant.3.3.Optical Properties.Figure 3shows the UV -vis diffuse reflectance spectra of Cu(X )-ZnIn 2S 4with X )0wt%to 2.0wt%.The onset of the absorption edge of ZnIn 2S 4was at about 495nm,corresponding to the band gap of 2.51eV.Moreover,ZnIn 2S 4had an intense absorption band with a steep edge in the visible-light region.This shape indicated that the UV -vis absorption was due to the band gap transition but not due to the transition from impurity levels to the conduction band of ZnIn 2S4.As X increased,a new absorption shoulder around 500nm was observed,and the absorption edge of Cu(X )-ZnIn 2S 4shifted monotonously from 575to 650nm with an increase inX from 0.1wt%to 1.2wt%,corresponding to the narrowing of the band gap from 2.16to 1.91eV.The absorption shoulder with a long tail on the low energy side,which was characteristic of doped photocatalysts 34,35and indicated that intraband gap states were formed by the dopants in the forbidden band,couldFigure 2.Part 2of 2.Scanning electron microscope images of Cu(X )-ZnIn 2S 4;the values of X were (a)0.0wt%,(b)0.1wt%,(c)0.3wt%,(d)0.5wt%,(e)0.7wt%;(f)0.9wt%,(g)1.2wt%,(h)1.6wt%,(i)2.0wt%.Figure 3.Diffuse reflectance spectra of Cu(X )-ZnIn 2S 4;the values of X were (a)0.0wt%,(b)0.1wt%,(c)0.3wt%,(d)0.5wt%,(e)0.7wt%;(f)0.9wt%,(g)1.2wt%,(h)1.6wt%,(i)2.0wt%.Enhanced Photocatalytic Hydrogen Evolution J.Phys.Chem.C,Vol.112,No.41,200816151be assigned to the transition from the Cu3d level above the valence band to the conduction band of ZnIn 2S 4.In addition,a broad band (λ>750nm)was observed for Cu (1.6wt%to 2.0wt%)-ZnIn 2S 4.This was due to the impurity CuS phase,as indicated by X-ray diffraction.The PL spectra of Cu(X )-ZnIn 2S 4,with X )0wt%to 2.0wt%,were obtained using an excitation wavelength of 330nm,as shown in Figure 4.The PL intensity and response range of Cu(X )-ZnIn 2S 4were influenced by Cu doping.The PL spectra,in company with the diffuse reflect spectra,shifted successively to longer wavelength with the change in the amount of Cu doped,indicating that the energy structure of Cu(X )-ZnIn 2S 4depended on the value of X.Similar phenomena has been reported by Kudo et al.in the (AgIn)x Zn 2(1-x )S 2solid solutions.19However,as shown in Table 1,the emission spectra of Cu(X )-ZnIn 2S 4(X )0.0wt%to 0.7wt%)was somewhat red-shifted vis-a-vis the absorption edge.This is because the PL emission of Cu(X )-ZnIn 2S 4presented here is broadband,36or donor -acceptor defect (such as sulfur vacancy)based,37in nature.In constrast,the absorption edge of Cu(X )-ZnIn 2S 4(X )0.9wt%to 2.0wt%)shifted to longer wavelength when compared to the emission spectra.This may be due to the excess of Cu doping,resulting in the formation of CuS,which has a total absorption in the visible-light region.Thus,as reported by Peng et al.on Cu-ZnS,38the PL emission of Cu(X )-ZnIn 2S 4may arise from the recombination between the shallow donor level (sulfur vacancy)and the t 2level of Cu 2+(splitting from Cu3d).As the energy level of sulfur vacancy relative to the valence band nearly stays constant in these samples despite the variation in Cu 2+concentration,it can be concluded that the t 2energy level ofCu 2+ions is farther from the valence band with increasing Cu 2+concentration.In addition,it could be found from Figure 4that the PL intensity of Cu(X )-ZnIn 2S 4gradually increased as the Cu content increased and arrived at the highest degree when the Cu content was 0.5wt%.While the Cu content continued to increase,namely more than 0.5wt%,the PL intensity began to decrease.In Mn-ZnS 39and Cu-ZnS,38a similar photolumi-nescence phenomenon was also observed,which can be explained by the effect of ion doping.As the foregoing analysis shows,these PL spectra were related to native defects (e.g.,sulfur vacancy).When Cu 2+was doped into ZnIn 2S 4,more defect states would be introduced.Therefore,it is reasonable that the defect-related PL intensities were enhanced for the Cu 2+-doped samples compared with the undoped sample.As for the decrease of the PL intensity with the Cu 2+concentration above 0.5wt%,it may be caused by the formation of CuS,though the XRD measurement did not detect the existence of the copper sulfide phase.In Eu-doped GaN,40a similar concentration quenching phenomenon was also observed,which was mainly attributed to the formation of EuN compound.3.4.Band Structures.Figure 5A shows the band structure and the density of state (DOS)of ZnIn 2S 4.The density contour maps for the lowest unoccupied molecular orbitals (LUMO)and highest occupied molecular orbitals (HOMO)of ZnIn 2S 4are shown in Figure 5B.We can observe clearly that both the top of valence band and the bottom of the conduct band lay at the G point of the Brillouin zone from Figure 5A.So the pure ZnIn 2S 4crystal is a direct gap band semiconductor.The theoretical value of the direct gap at G is 0.4eV,which is less than the experimental value of about 2.51eV.Such an underestimation of the band gap is a well-known artifact of GGA.The density of states (Figure 5A)indicates that the S3p orbitals make a significant contribution to the valence band top of ZnIn 2S 4and that the highest occupied molecular orbital (HOMO)levels are composed mainly of the hybridized S3p and Zn3d orbitals.The In5s orbitals locate at more negative energy levels than that of S3p orbitals and do not contribute much to the valence band top.The lowest unoccupied molecular orbital (LUMO)levels are composed mainly of the In5s5p and S3p orbitals.The Zn4s4p orbitals locate at more positive energy levels and do not contribute much to the conduction band bottom.Based on the optical properties and DFT calculations,the schematic energy level diagram of Cu(X )-ZnIn 2S 4is shown in Figure 6.As for ZnIn 2S 4,upon photoexcitation,electrons would transfer from the valence band (hybridized S3p and Zn3d orbitals)to the conduction band (hybridized In5s5p and S3p orbitals),leaving photogenerated holes in the valence band.As aforementioned,the PL emission has been known to be due to the photoluminescence transition from the sulfur-vacancy-related donor level or the conduction band to the valence band.Therefore,the energy difference between the absorption edge and the emission spectra of ZnIn 2S 4can be explained.Taking the Cu doping into account,the t 2state,splitting from discrete Cu3d levels,will form above the edge of valence band of ZnIn 2S 4,which is consistent with those reported by Peng 38and Xu 41in Cu-ZnS.A similar orbital-splitting phenomenon has been observed by Ye 42and Zou 43for Cr-3d and Ni-3d orbtials in In 12NiCr 2Ti 10O 42and Ca 2NiWO 6.The t 2(Cu3d)level,pro-duced by doping Cu 2+into ZnIn 2S 4,works as the donor level and acceptor level for photoexcitation and PL emission,respec-tively,44as shown in Figure 6.With the amount of Cu doped increasing,the t 2(Cu3d)level will be elevated farther fromtheFigure 4.Photoluminescence spectra of Cu(X )-ZnIn 2S 4;the values of X were (a)0.0wt%,(b)0.1wt%,(c)0.3wt%,(d)0.5wt%,(e)0.7wt%;(f)0.9wt%,(g)1.2wt%,(h)1.6wt%,(i)2.0wt%,excited at 330nm.TABLE 1:Band Gap,Absorption Edge,PL Emission and Emission Intensity of Cu(X )-ZnIn 2S 4,with X )0.0wt%to 2.0wt%X (wt%)band gap a (eV)absorption edge (nm)PL emission (nm)emission intensity(normalized,a.u.)0.0 2.514955600.330.1 2.165755970.720.3 2.115876110.760.5 2.05604617 1.000.7 2.026146260.740.9 1.946396320.561.2 1.916506390.421.6 1.737156550.202.01.657506660.19aCalculated from absorption edge.16152J.Phys.Chem.C,Vol.112,No.41,2008Shen et al.valence band,which results in the redshift of absorption edge and PL emission for Cu(X )-ZnIn 2S 4.3.5.Photocatalytic Activities of Cu(X )-ZnIn 2S4.Figure 7shows the dependence of photocatalytic H 2evolution from an aqueous Na 2SO 3/Na 2S solution over Cu(X )-ZnIn 2S 4under visible-light irradiation (λ>430nm).Either nondoped orCu-Figure 5.(A)Band structure and density of states for ZnIn 2S 4calculated by the density functional method.(B)Density contour maps for the LUMO and HOMO of ZnIn 2S 4.Figure 6.Schematic energy level diagram of Cu(X )-ZnIn 2S 4.Vs stands for sulfurvacancy.Figure 7.Photocatalytic H 2evolution under visible-light irradiation over Cu(X )-ZnIn 2S 4;the values of X were (a)0.0wt%,(b)0.1wt%,(c)0.3wt%,(d)0.5wt%,(e)0.7wt%,(f)0.9wt%,(g)1.2wt%,(h)1.6wt%,(i)2.0wt%.Enhanced Photocatalytic Hydrogen Evolution J.Phys.Chem.C,Vol.112,No.41,200816153doped ZnIn 2S 4was active and stable for splitting water to hydrogen.The rate of H 2evolution over nondoped ZnIn 2S 4was 26.1µmol ·h -1.The apparent quantum yield at 420nm was calculated to be 9.6%by eq 1.As the amount of doped Cu was increased,the photocatalytic activity of Cu(X )-ZnIn 2S 4was increased,because the visible-light absorption band of Cu(X )-ZnIn 2S 4grew.11The highest activity was obtained when 0.5wt %of Cu was doped,the rate of hydrogen evolution reached at 151.5µmol ·h -1,with the apparent quantum yield determined to be 14.2%.As the concentration of Cu doped was above 0.5wt%,the activity was decreased,though the visible-light absorption band grew further.Such a similar dependence of photocatalytic H 2evolution upon the amount of dopant has been observed for several other photocatalysts.11,44,45These observa-tions indicated that the photocatalytic activites depended upon not only the visible-light absorption (i.e.,band gap)but also some other factors.One of the reasons for the decrease in photocatalytic activity may be due to the impurity phase of CuS,resulted from the excess of Cu doping,as proved by XRD results.The CuS impurity may work as recombination sites between photogenerated electrons and holes.Another possible inactivation factor for the ZnIn 2S 4doped with excessive Cu is that the layered structure could be destructed gradually with the amount of Cu increased further,as revealed by SEM images.Some perovskite photocatalysts with layered structure have also shown good photocatalytic activity for splitting water to hydrogen,as the dipole moment along layers seems to enhance the charge separation,resulting in high activity.46,47Figure 8shows the photocatalytic activity and photolumi-nescence intensity as a function of Cu content in the Cu(X )-ZnIn 2S 4.The change in photoluminescence intensity was concurrent to that in photocatalytic activity,as both photolu-minescence intensity and photocatalytic activity reached the highest level when 0.5wt %of Cu was doped.Photolumines-cence depends on various factors such as the densities of photoexcited charges and recombination centers,the extent of nonradiation process,and the mobility of photoexcited charges.44It is difficult to determine which process is mainly responsible for changes in photoluminescence intensity with Cu content.However,in association with the change in photocatalytic activity,a plausible explanation can be made as follows.During the PL process,vacancies (such as sulfur vacancy)and defects can easily bind photoinduced electrons to form excitons,so that the PL signal can easily occur.48When Cu 2+ions were doped into ZnIn 2S 4,more vacancies (such as sulfur vacancy)or defect states would be introduced.38The larger the content of vacancy or defect,the stronger the PL signal.Meanwhile,an increasein Cu content not only raised the t 2(Cu3d)level to narrow the band gap of Cu(X )-ZnIn 2S 4,which has been demonstrated by the UV -vis diffuse reflectance spectra,but also enhanced the density of the t 2(Cu3d)levels and increased the concentration and the mobility of the photoexcited electrons.44These could be related to the enhancement of photocatalytic activity.However,further increase in the amount of Cu doped would result in the increased absorption to longer wavelength,which was likely due to the formation of CuS,as discussed previously.Thus,the CuS impurity weakened the photoluminescence,40as well as lowered photocatalytic activity of Cu-doped ZnIn 2S 4.114.ConclusionsIn summary,we have synthesized a series of Cu-doped ZnIn 2S 4photocatalysts by a simple hydrothermal method.The photocatalytic activity of ZnIn 2S 4was remarkably enhanced by Cu doping.The optimized Cu (0.5wt%)-doped ZnIn 2S 4photocatalyst showed the highest activity for splitting water into H 2,with the rate of hydrogen evolution to be 151.5µmol ·h -1.With increasing concentration of Cu doped,the UV -vis spectra and PL emission peak were systematically shifted to longer wavelength.Moreover,there were certain intrinsic relationships between the PL emission intensity and photocatalytic activity of Cu-doped ZnIn 2S 4.That is to say,the change in photolumi-nescence intensity was concurrent to that in photocatalytic activity,both depending on the increasing concentration of Cu doped.Acknowledgment.The authors gratefully acknowledge the financial support of the National Natural Science Foundation of China (No.50521604)and National Basic Research Program of China (No.2003CB214500).The authors also would like to thank the molecular simulating platform and National High Performance Computing Center (Xi’an)at Xi’an Jiaotong University for the calculation resource support.References and Notes(1)Domen,K.;Kudo,A.;Ohnishi,T.J.Catal.1986,102,92.(2)Hwang,D.W.;Kim,H.G.;Kim,J.;Cha,K.Y.;Kim,Y.G.;Lee,J.S.J.Catal.2000,193,40.(3)Kato,H.;Asakura,K.;Kudo,A.J.Am.Chem.Soc.2003,125,3082.(4)Sato,J.;Saito,N.;Yamada,Y.;Maeda,K.;Takata,T.;Kondo,J.N.;Hara,M.;Kobayashi,H.;Domen,K.J.Am.Chem.Soc.2005,127,4150.(5)Kim,H.G.;Hwang,D.W.;Lee,J.S.J.Am.Chem.Soc.2004,126,8912.(6)Maeda,K.;Teramura,K.;Lu,D.;Takata,T.;Saito,N.;Inoue,Y.;Domen,K.Nature 2006,440,295.(7)Ritterskamp,P.;Kuklya,A.;Wu ¨stkamp,M.;Kerpen,K.;Weidentha-ler,C.;Demuth,M.Angew.Chem.,Int.Ed.2007,46,7770.(8)Niishiro,R.;Kato,H.;Kudo,A.Phys.Chem.Chem.Phys.2005,7,2241.(9)Kudo,A.;Sekizawa,M.Catal.Lett.1999,58,241.(10)Kudo,A.;Sekizawa,mun.2000,15,1371.(11)Tsuji,I.;Kudo,A.J.Photochem.Photobiol.A 2003,156,249.(12)Zou,Z.G.;Ye,J.H.;Sayama,K.;Arakawa,H.Nature 2001,414,625.(13)Bubler,N.;Meier,K.;Reber,J.F.J.Phys.Chem.1984,88,3261.(14)Naman,S.A.;Gra ¨tzel,M.J.Photochem.Photobiol.A 1994,77,249.(15)Inoue,T.;Watanabe,T.;Fujishima,A.;Honda,K.J.Electrochem.Soc.1977,124,719.(16)Shangguan,W.;Yoshida,A.J.Phys.Chem.B 2002,106,12227.(17)Shen,S.;Guo,L.J.Solid State Chem.2006,179,2629.(18)Shen,S.;Guo,L.Mater.Res.Bull.2008,43,437.(19)Tsuji,I.;Kato,H.;Kobayashi,H.;Kudo,A.J.Am.Chem.Soc.2004,126,13406.(20)Tsuji,I.;Kato,H.;Kudo,A.Angew.Chem.,Int.Ed.2005,44,3565.(21)Jang,J.S.;Choi,S.H.;Shin,N.;Yub,C.;Lee,J.S.J.Solid State Chem.2007,180,1110.Figure 8.Dependence of photocatalytic activity for H 2evolution and PL emission intensity over Cu(X )-ZnIn 2S 4,with X )0.0wt%to 2.0wt%.16154J.Phys.Chem.C,Vol.112,No.41,2008Shen et al.(22)Liu,G.;Zhao,L.;Ma,L.;Guo,mun.2008,9,126.(23)Romeo,N.;Dallaturca,A.;Braglia,R.;Sberveglieri,G.Appl.Phys. Lett.1973,22,21.(24)Seo,W.S.;Otsuka,R.;Okuno,H.;Ohta,M.;Koumoto,K.J.Mater. Res.1999,14,4176.(25)Romeo,N.;Tarricone,L.;Zanotti,L.Il Nuo V o Cim.D1983,2, 2007.(26)Lei,Z.;You,W.;Liu,M.;Zhou,G.;Takata,T.;Hara,M.;Domen, K.;Li,mun.2003,17,2142.(27)Gou,X.;Cheng,F.;Shi,Y.;Zhang,L.;Peng,S.;Chen,J.;Shen, P.J.Am.Chem.Soc.2006,128,7222.(28)Shen S.;Zhao L.;Guo L.Mater.Res.Bull.,2008,doi:10.1016/ j.materresbull.2008.03.027.(29)Shen S.;Zhao L.;Guo L.Int.J.Hydrogen Energy,2008, doi:10.1016/j.ijhydene.2008.05.043.(30)Segall,M.D.;Lindan,P.J.D.;Probert,M.J.;Pickard,C.J.;Hasnip, P.J.;Clark,S.J.;Payne,M.C.J.Phys.:Condens.Matter2002,14,2717.(31)Vanderbilt,D.Phys.Re V.B1990,41,7892.(32)Lei,Z.;Ma,G.;Liu,M.;You,W.;Yan,H.;Wu,G.;Takata,T.; Hara,M.;Domen,K.;Li,C.J.Catal.2006,237,322.(33)Shionoya,S.;Tamoto,Y.J.Phys.Soc.Jpn.1964,19,1142.(34)Konta,R.;Ishii,T.;Kato,H.;Kudo,A.J.Phys.Chem.B2004, 108,8992.(35)Miyauchi,M.;Takashio,M.;Tobimatsu,ngmuir2004,20, 232.(36)Spanhel,I.;Anderson,M.A.J.Am.Chem.Soc.1991,113,2826.(37)Castro,S.L.;Bailey,S.G.;Raffaelle,R.P.;Banger,K.K.;Hepp,A.F.J.Phys.Chem.B2004,108,12429.(38)Peng,W.Q.;Cong,G.W.;Qu,S.C.;Wang,Z.G.Opt.Mater. 2006,29,313.(39)Ghosh,P.K.;Ahmed,Sk.F.;Jana,S.;Chattopadhyay,K.K.Opt. Mater.2007,29,1584.(40)Bang,H.;Morishima,S.;Sawahata,J.;Seo,J.;Takiguchi,M.; Tsunemi,M.;Akimoto,K.;Nomura,M.Appl.Phys.Lett.2004,85,227.(41)Xu,S.J.;Chua,S.J.;Liu,B.;Gan,L.M.;Chew,C.H.;Xu,G.Q. Appl.Phys.Lett.1998,73,478.(42)Wang,D.;Zou,Z.;Ye,J.Chem.Phys.Lett.2005,411,285.(43)Li,D.;Zheng,J.;Zou,Z.J.Phys.Chem.Solids2006,67,801.(44)Arai,N.;Saito,N.;Nishiyama,H.;Domen,K.;Kobayashi,H.;Sato, K.;Inoue,Y.Catal.Today2007,129,407.(45)Nishimoto,S.;Matsuda,M.;Miyake,M.Chem.Lett.2006,35, 308.(46)Kudo,A.;Kato,H.;Nakagawa,S.J.Phys.Chem.B2000,104, 571.(47)Hwang,D.W.;Kim,H.G.;Lee,J.S.;Kim,J.;Li,W.;Oh,S.H. J.Phys.Chem.B2005,109,2093.(48)Jing,L.;Qu,Y.;Wang,B.;Li,S.;Jiang,B.;Yang,L.;Fu,W.;Fu,H.;Sun,J.Sol.Energy Mater.Sol.Cells2006,90,1773.JP804525QEnhanced Photocatalytic Hydrogen Evolution J.Phys.Chem.C,Vol.112,No.41,200816155。
油水分离英文文献
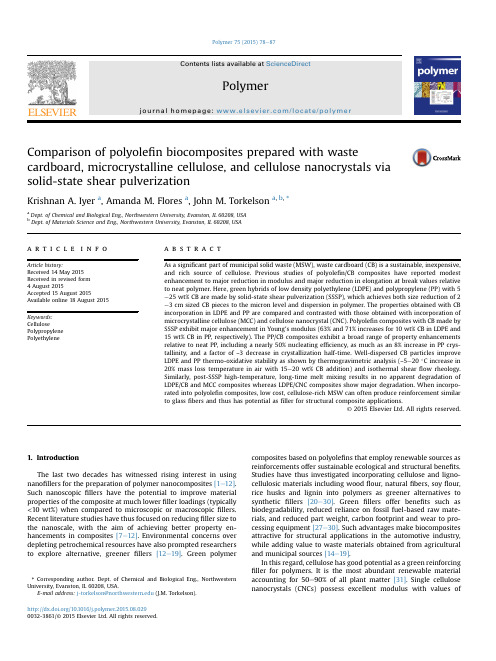
Comparison of polyole fin biocomposites prepared with wastecardboard,microcrystalline cellulose,and cellulose nanocrystals via solid-state shear pulverizationKrishnan A.Iyer a ,Amanda M.Flores a ,John M.Torkelson a ,b ,*a Dept.of Chemical and Biological Eng.,Northwestern University,Evanston,IL 60208,USA bDept.of Materials Science and Eng.,Northwestern University,Evanston,IL 60208,USAa r t i c l e i n f oArticle history:Received 14May 2015Received in revised form 4August 2015Accepted 15August 2015Available online 18August 2015Keywords:CellulosePolypropylene Polyethylenea b s t r a c tAs a signi ficant part of municipal solid waste (MSW),waste cardboard (CB)is a sustainable,inexpensive,and rich source of cellulose.Previous studies of polyole fin/CB composites have reported modest enhancement to major reduction in modulus and major reduction in elongation at break values relative to neat polymer.Here,green hybrids of low density polyethylene (LDPE)and polypropylene (PP)with 5e 25wt%CB are made by solid-state shear pulverization (SSSP),which achieves both size reduction of 2e 3cm sized CB pieces to the micron level and dispersion in polymer.The properties obtained with CB incorporation in LDPE and PP are compared and contrasted with those obtained with incorporation of microcrystalline cellulose (MCC)and cellulose nanocrystal (CNC).Polyole fin composites with CB made by SSSP exhibit major enhancement in Young's modulus (63%and 71%increases for 10wt%CB in LDPE and 15wt%CB in PP,respectively).The PP/CB composites exhibit a broad range of property enhancements relative to neat PP,including a nearly 50%nucleating ef ficiency,as much as an 8%increase in PP crys-tallinity,and a factor of ~3decrease in crystallization half-time.Well-dispersed CB particles improve LDPE and PP thermo-oxidative stability as shown by thermogravimetric analysis (~5e 20 C increase in 20%mass loss temperature in air with 15e 20wt%CB addition)and isothermal shear flow rheology.Similarly,post-SSSP high-temperature,long-time melt mixing results in no apparent degradation of LDPE/CB and MCC composites whereas LDPE/CNC composites show major degradation.When incorpo-rated into polyole fin composites,low cost,cellulose-rich MSW can often produce reinforcement similar to glass fibers and thus has potential as filler for structural composite applications.©2015Elsevier Ltd.All rights reserved.1.IntroductionThe last two decades has witnessed rising interest in using nano fillers for the preparation of polymer nanocomposites [1e 12].Such nanoscopic fillers have the potential to improve material properties of the composite at much lower filler loadings (typically <10wt%)when compared to microscopic or macroscopic fillers.Recent literature studies have thus focused on reducing filler size to the nanoscale,with the aim of achieving better property en-hancements in composites [7e 12].Environmental concerns over depleting petrochemical resources have also prompted researchers to explore alternative,greener fillers [12e 19].Green polymercomposites based on polyole fins that employ renewable sources as reinforcements offer sustainable ecological and structural bene fits.Studies have thus investigated incorporating cellulose and ligno-cellulosic materials including wood flour,natural fibers,soy flour,rice husks and lignin into polymers as greener alternatives to synthetic fillers [20e 30].Green fillers offer bene fits such as biodegradability,reduced reliance on fossil fuel-based raw mate-rials,and reduced part weight,carbon footprint and wear to pro-cessing equipment [27e 30].Such advantages make biocomposites attractive for structural applications in the automotive industry,while adding value to waste materials obtained from agricultural and municipal sources [14e 19].In this regard,cellulose has good potential as a green reinforcing filler for polymers.It is the most abundant renewable material accounting for 50e 90%of all plant matter [31].Single cellulose nanocrystals (CNCs)possess excellent modulus with values of*Corresponding author.Dept.of Chemical and Biological Eng.,Northwestern University,Evanston,IL 60208,USA.E-mail address:j-torkelson@ (J.M.Torkelson).Contents lists available at ScienceDirectPolymerjournal homepage:www.elsev /locate/polymer/10.1016/j.polymer.2015.08.0290032-3861/©2015Elsevier Ltd.All rights reserved.Polymer 75(2015)78e 87137GPa or higher[32e34].The presence of amorphous domains and defects reduces the modulus of cellulose to29e36GPa [32e34].Discoveries of these excellent properties have led to re-ports of polymer composites incorporating cellulosicfillers ranging from nanocellulose to macroscale cellulose-rich municipal solid waste(MSW)materials[32e58].Corrugated cardboard(CB)and waste paper account for almost one-third of the total MSW generated in the United States[59].Kraft pulping of wood employed for the production of cardboard results in the partial elimination of hemicellulose and lignin[58,60,61].Corrugated cardboard is chemically made up of~75%cellulosics,~15%lignin, and the rest being binders/adhesives such as starch and acrylam-ides[60,61].The hemicellulosic part is primarily made up of mannose,galactose and xylose.Despite advancements in recycling technologies,with increasing number of recycling operations the quality of cellulose fibers in CB decreases.As a result,almost25million tons of CB waste are discarded annually into landfills in the United States[59]. Lack of effective processing techniques results in extremely low valuation of~$95/ton for paper scrap[62];in contrast,the highly refined form of cellulose known as microcrystalline cellulose(MCC) that is used in the pharmaceutical industry is priced at~$2500/ton [63].Cardboardfibers are known to possess other attractive prop-erties like high specific strength and high aspect ratio(~100)[44]. Similar to wood-based polymer composites,thermoplastic com-posites with waste CB are speculated to be useful in load-bearing applications,frames,car interiors,etc[44].Despite their potential,the majority of studies producing such composites via melt processing have reported poor or mixed re-sults.Yuan et al.[45]noted,“Because of the poor compatibility of polypropylene(PP)and paperflour,it is nearly impossible to pre-pare…PP/paperflour blends with good mechanical properties.”(The presence of hydroxyl groups on cellulose makes it inherently incompatible with the hydrophobic polyolefin matrices.)Thus,all literature reports attempting to produce such composites have utilized surface modification,particle size reduction,extraction and/or compatibilizer addition for the preparation of polyolefin/CB composites[42e58].Chibani et al.[46]reported no improvement in tensile modulus with incorporation of up to28wt%unmodified CB in PP composites prepared via melt processing.They further suggested the addition of maleated PP as a compatibilizer to improve material properties.Salmah et al.[47]utilized intensive grinding to obtain waste paper particles that were an average of 31m m in size.Incorporating20wt%of thisfiller in low density polyethylene(LDPE)resulted in a125%increase in modulus but a 90%decrease in elongation at break compared to neat LDPE.Ashori and Nourbakhsh[56]incorporated10e40wt%of old corrugated cardboardfiber into PP by melt mixing;all composites exhibited tensile modulus values well below(as much as50%below)that of neat PP.Drzal and coworkers incorporated recycled cellulosefiber (average20m m diameter)extracted from waste paper into PP via melt processing and observed a64%increase in modulus for30wt%filler content[49e51].The extra steps employed in these studies such as particle size reduction,extraction and compatibilization not only add to the time and cost of processing but also reduce the green aspect of thefiller.We previously reported on property enhancements achieved by incorporating CNC in polyolefins via solid-state shear pulverization (SSSP)[39].For example,in90/10wt%LDPE/CNC composites made by SSSP,there was a70%increase in tensile modulus and no reduction within error in elongation at break relative to neat LDPE [39].The ambient conditions and solid-state nature of SSSP enabled high shear and compressive forces to be imparted to the materials, resulting in excellent CNC dispersion and strong suppression of filler degradation that accompanies high-temperature melt processing of cellulose-basedfillers[39].Past studies have shown the ability of SSSP to produce excellent dispersion of pristinefillers ranging from bundled carbon nanotubes and graphite to waste materials from natural sources[27e30,39,64e69].Excellent dispersion of immiscible blends has also been achieved by SSSP [70e72].Studies have also taken advantage of ambient conditions in SSSP to achieve functionalization and long-chain branching of PP without major molecular weight reduction[73e75].Here,we compare the reinforcing ability of well-dispersed cel-lulose-rich CB waste to well-dispersed,synthetic sources of cellu-lose such as MCC and CNC in polyolefin composites.The CB is fed to the SSSP apparatus in several-centimeter-sized pieces with SSSP achieving both major particle size reduction and dispersion.For commercial viability of such composites as well as for sustainability considerations,it is important to compare low cost,cellulose-rich waste materials with much higher cost cellulosefillers.(At pre-sent,CNC production is at the pilot-plant scale rather than involving large-scale,commercial production.)We show that well-dispersed,cellulose-rich CB from MSW can often provide property enhancements similar to those achieved by highly refined,micro-crystalline and nanocrystalline cellulose.2.Experimental section2.1.MaterialsTwo PP samples,PP1(Total3276)and PP2(Total3270)were used as received.As reported by the supplier(Total Petrochemi-cals),both samples had the same density(0.905g/cm3)and melt flow index(MFI,2g/10min).Low density polyethylene(Exxon-Mobil LD103)with density of0.919g/cm3and MFI of1.1g/10min (190 C and2.2kg load)was supplied by ExxonMobil.Randomly chopped cardboard pieces(average size of~2e3cm)procured locally were used as-received without deinking.Microcrystalline cellulose(lattice NT with average particle size of~50m m and aspect ratio of~1.4as reported by the supplier)was supplied by FMC Biopolymers and used without modification.Unmodified cellulose nanocrystals were produced at the Forest Products Laboratory in Madison,WI as described in Ref.[76].(Data related to polyolefin/ CNC nanocrystals and used for comparative purposes are taken from Ref.[39].)2.2.Preparation of polyolefin/cellulose compositesPolyolefin pellets were fed to a Berstorff ZE-25pulverizer with a K-tron S-60feeder at a~100g/h feed rate.(A commercial-scale apparatus has processed polyolefins at rates exceeding150kg/h [27,28].)The CB pieces(~2e3cm)were fed to the pulverizer via the K-tron S-60feeder whereas powdery MCC and CNC were added with a powder feeder(Brabender Technologies Inc.DDSR12-1 volumetric feeder)at different feed rates to obtain desiredfiller content.The composites were produced using a200rpm screw speed and a screw design that yielded high specific energy(see Ref.[77]for a detailed description of screw design and energy in-puts).The pulverizer barrels were cooled by a recirculating ethylene glycol/water mix(À7 C,Budzar Industries WC-3chiller). Other details and process conditions are available in Refs.[27e30,39].2.3.Characterization of polyolefin/cellulose compositesField-emission scanning electron microscopy(FE-SEM)samples were prepared by melting and extruding material obtained from SSSP with an Atlas Electronic Devices MiniMax molder(cup-and-rotor mixer).Morphologies of cryo-fractured sections wereK.A.Iyer et al./Polymer75(2015)78e8779obtained via an SU8030instrument after sputtering with gold/ palladium(Denton DeskIII).The FE-SEM images of as-received MCC were acquired after casting from water onto Si wafers.The mor-phologies of pristine CNC and polyolefin/CNC composites produced by SSSP have been reported[39].Uniaxial tensile test samples of~0.7mm thickness were pre-pared by compression molding using a PHI(Model0230C-X1)press at180 C or140 C depending on the matrix polymer for5min with 5ton ram force.Dog-bone shaped specimens were cut using a Dewes-Gumbs die and tested using an MTS Sintech2S tensile tester according to ASTM D1708with a5kN load cell using a crosshead speed of50mm/min.Polymer crystallization was characterized by differential scan-ning calorimetry(DSC;Mettler-Toledo822e).After heating samples above the melt temperature,a10 C/min cooling rate was used to determine the non-isothermal crystallization onset temperature (T c).The percent polymer crystallinity was obtained by dividing the specific enthalpy from the area associated with the crystallization part of the nonisothermal cooling curve by the polymer mass fraction;this value was divided by the theoretical heat of fusion for neat polyethylene or PP of285.9and207.1J/g,respectively[78,79]. Since the PP matrices used for the preparation of CB and synthetic cellulose(MCC and CNC)composites are different,a nucleating efficiency(NE)was calculated based on the calorimetric efficiency scale proposed by Lotz and coworkers[65,80]:NEð%Þ¼ÀT cÀT c;lowÁ ÀT c;maxÀT c;lowÁÂ100(1)where T c,low is the onset crystallization temperature for a non-nucleated PP(equal to T c for neat PP1and PP2,117.1and121.4 C, respectively),T c,max is the onset crystallization temperature for a self-nucleated PP(both PP in this study had T c,max of140.2 C),and T c is the non-isothermal onset temperature of crystallization.(All onset temperatures were recorded at a cooling rate of10 C/min.) Isothermal crystallization half-times for the PP-based composites were determined at140 C after cooling from the melt at40 C/min.Thermo-oxidative degradation was monitored via thermo-gravimetric analysis(TGA;Mettler Toledo851e)under air.~5mg samples were heated from25 C to700 C at a10 C/min heating rate.At least three samples were run to show reproducibility.(Neat PP leaves behind no residue within error at700 C.)In order to understand the effect of the differentfillers on the thermal stability of polyolefins under isothermal shear meltflow,shear storage modulus was monitored as a function of time at200 C using small amplitude oscillatory shear measurements at10rad/s(TA In-struments ARES rheometer;25mm parallel platefixture).Water absorption measurements were performed on compres-sion molded discs(thickness¼~0.125inch and diameter¼2inch) following ASTM D570.Specimens were dried at80 C for24h, cooled in a desiccator,and immediately weighed(w1).Specimens were then immersed in distilled water at~23 C.Samples were periodically removed,dried with absorbent paper,and weighed (w2).The percentage water absorption(WA)is calculated as follows:WAð%Þ¼ðw2Àw1Þ=w1Â100(2) 3.Results and discussion3.1.Dispersion and morphology of polyolefin/cellulose compositesFig.1a shows~2e3cm sized CB pieces that were employed in the present study.The large shear and compressive forces imparted to the material during solid-state processing leads to majorfiller size reduction in the polymer composites.Fig.1b and c shows SEM images of fractured surfaces of LDPE/CB and PP/CB composites with 10wt%CB.The originally centimeter-sized CB pieces are reduced via a single-step SSSP process to particles in the range of1e10m m in size that are wetted into the polymer matrix.In addition,the fractured surfaces show no signs of pull-out,indicating the absence of majorfiller agglomeration.Fig.1d shows SEM images of as-received MCC.Agglomerates of MCC can be as large as 100e200m m due to the strong interparticle affinity arising from the numerous hydroxyl groups on the cellulose surface.Similar to polyolefin/CB composites,SEM images of LDPE/MCC and PP/MCC composites(Fig.1e and f)show very good dispersion.The highly agglomerated MCC particles underwent major size reduction to particles no larger than1m m.In an earlier study,excellentfiller dispersion was obtained in polyolefin/CNC composites made by SSSP[39].Previous studies have reported challenges in achieving excellent dispersion of cellulosicfillers in polyolefins via melt mixing.Chi-bani et al.[46]observed severefiller agglomeration,debonding and fiber pull-out,indicating poor adhesion between the CBfibers and PP matrix.In their study,5wt%PP grafted with maleic anhydride was used as compatibilizer to improve the interfacial adhesion which seemingly enhanced thefiller dispersion.Ganster et al.[35] observed similarfiber pull-out and poorfiller dispersion when MCC was incorporated in polyethylene by melt mixing.Wetting and dispersion similar to that achieved in our study were obtained only after the addition of maleated PP.In short,solid-state processes are able to achieve excellentfiller dispersion and particle size reduc-tion;this leads to the production of polyolefin/CB composites with morphologies similar to those observed by employing MCC or CNC fillers.3.2.Mechanical properties of polyolefin/cellulose composites3.2.1.Tensile properties of polyolefin/CB compositesTable1summarizes the effect of CB on the mechanical proper-ties of LDPE/CB composites made by SSSP.Excellent dispersion and particle size reduction led to major improvements in Young's modulus.For example,relative to neat LDPE,incorporation of5wt% CB results in a~38%increase in modulus and20wt%CB results in a 190%increase.The yield strength of LDPE/CB composites shows a 22%increase relative to neat polymer for5wt%CB and values unchanged from that of neat LDPE at other CB loadings.These re-sults compare well with those obtained by incorporating MCC and CNC into LDPE.The63%increase in modulus seen with90/10wt% LDPE/CB is within error the same as that obtained with90/10wt% LDPE/MCC and LDPE/CNC(Fig.2a and Table1).Similarly,the90/ 10wt%LDPE/CB composites retain yield strength that is within error invariant from those of neat LDPE and the90/10wt%LDPE/ MCC composite and only slightly lower than that of90/10wt% LDPE/CNC.In addition,the excellentfiller dispersion and particle size reduction achieved by SSSP processing led to unparalleled reten-tion of elongation at break values in LDPE composites.As shown in Fig.2b,90/10wt%LDPE/MCC and LDPE/CNC composites have elongation at break values that are within error unchanged from that of neat LDPE.Due to the slightly larger size scales of CB par-ticles in the polymer matrix(yet less than10m m in size),the95/5 and90/10wt%LDPE/CB composites exhibit reductions in elonga-tion from500%for neat LDPE to390%and260%,respectively. Notably,LDPE/CB composites produced by SSSP retain sufficient elongation at break to be viable for commercial applications atfiller loadings as high as20wt%.As shown in Table2,relative to neat PP1with a modulus of 910MPa,PP1composites with5and25wt%CB exhibit24and92% increases in Young's modulus,respectively.In addition,all PP1/CBK.A.Iyer et al./Polymer75(2015)78e87 80composites produced here show yield strength similar to neat PP1.Due to minor degradation of CB during compression molding,the 95/5wt%PP1/CB composite retains an elongation at break of 40%while the 75/25wt%PP1/CB composite has a value of 8%.None-theless,all composites exhibit ductile behavior except the 75/25wt %PP1/CB composite with a barely ductile fracture.These results are comparable to those obtained with MCC and CNC composites;relative to neat PP2with a 1200MPa modulus,90/10wt%PP2/MCC and 90/10wt%PP2/CNC composites show ~33%and ~53%increases in modulus,respectively.parison with literature data for polyole fin/cellulose based compositesFew literature studies have investigated the fabrication of polyole fin/CB composites via melt mixing,all of which have employed particle size reduction steps prior to melt processing in order to achieve CB particles that are less than 50m m in size [46,47].Salmah et al.[47]utilized intensive grinding to produce,on average,31-m m-sized waste paper particles,that were subsequently incor-porated into LDPE via melt mixing.The LDPE composites with 23wt%filler showed a ~100%increase in modulus relative to neat LDPE (modulus of 80MPa).In contrast,the 80/20wt%LDPE/CB composite produced via SSSP shows a 190%increase in modulus.The severe filler agglomeration and void formation during melt processing result in dramatically reduced elongation at break values in the composite.Salmah et al.[47]reported major reduction in elongation at break from 400%for neat LDPE to only 50%for composites with 9wt%filler produced via melt processing.Dramatically superior performance is observed with an SSSP pro-cessed composite of similar filler content,showing a 260%elon-gation at break (neat LDPE has 500%elongation).Chibani et al.[46]incorporated up to 15wt%CB fibers in PP via melt mixing and observed no improvement in tensile modulus.In comparison,in the present study,an 85/15wt%PP/CB composite shows a ~71%increase in Young's modulus relative to neat PP.Poor outcomes have also been reported when synthetic cellu-lose fillers were incorporated in composites prepared via melt processing or solution mixing.Spoljaric et al.[36]produced a 90/10wt%PP/MCC composite using ultrasonication in solution and reported a ~30%increase in modulus relative to neat PP.OurstudyFig.1.a)Photographs of ~2e 3cm size CB pieces employed in preparing polyole fin/CB composites.Field-emission scanning electron micrographs of b)90/10wt%LDPE/CB,c)85/15wt%PP/CB,d)as-received MCC,e)90/10wt%LDPE/MCC and f)90/10wt%PP/MCC composites prepared via SSSP.Table 1Mechanical properties of LDPE/cellulosic filler composites produced by SSSP.SampleYoung's modulus E (MPa)Yield strength s (MPa)Elongation at break ε(%)Neat LDPE160±510.0±0.3500±3095/5wt%LDPE/CB 220±1012.2±1.0390±3090/10wt%LDPE/CB 260±2010.7±0.6260±1580/20wt%LDPE/CB 465±3510.1±0.233±1090/10wt%LDPE/MCC 260±2011.0±0.1485±2090/10wt%LDPE/CNC270±1013.0±1.0460±30Note:Data for LDPE/CNC composites are from Ref.[39].K.A.Iyer et al./Polymer 75(2015)78e 8781reports a similar 33%increase for the same MCC loading using the industrially scalable,solventless,continuous SSSP process.As shown in Ref.[39],polyole fin/CNC composites produced by SSSP have the highest reported enhancement in modulus relative to similar composites produced via melt or solution mixing in litera-ture studies.Moreover,no study has produced PP/CNC composites using industrially scalable,melt processing techniques due to concerns with CNC thermal degradation.Alternatively,such com-posites can be manufactured by using ambient temperature,solid-state processes that are able to achieve very good dispersion.We further demonstrate here that effective agglomerate breakup and superior dispersion of macroscopic cellulose rich MSW achieved by SSSP give rise to property enhancements that are comparable to those seen with microscale or nanoscale cellulose.Well-dispersed cellulosic fillers thus provide excellent reinforcement to poly-ole fins,making the plastic stiffer and more durable.3.3.Water uptake of polyole fin/cellulose based compositesWater absorption behavior is important in applications related to structural materials for outdoor use.The overall water absorp-tion of a composite depends on moisture content of the filler,void fraction,quality of dispersion,permeability,filler hydrophilicity,etc [46].Fig.3shows the percentage weight gain as a function of the square root of time with increasing filler content in composite samples (thickness ¼0.125inch and diameter ¼2.0inch,following ASTM D570).Neat LDPE and PP show no appreciable water ab-sorption after 300h immersion.Hence,water uptake in these composites is a result of filler incorporation.Under the same conditions,LDPE composites show marginal water absorption.For example,after 24h immersion,LDPE com-posites with 10wt%CNC,MCC,and CB show 0.12,0.15,and 0.19wt%water gain,respectively;none of the composites reaches a constant,equilibrium sorption value after 300h immersion.Similarly,after 24h immersion,PP composites with 10wt%CNC,MCC and 15wt%CB show 0.09,0.13,and 0.08wt%water absorption;none of the PP composites reaches equilibrium after 300h immersion.Via melt mixing,Tajvidi et al.[54]incorporated 15wt%waste paper in PP and observed ~0.50%water absorption after 24h,a factor of six greater than that observed with 85/15wt%PP1/CB produced via SSSP (samples were tested according to ASTM D570in their study).The very low water absorption reported in the current study is consis-tent with excellent filler dispersion and absence of voids within the composites.In addition,increasing the cellulose crystallinity by extraction of microscopic and nanoscopic crystallite does not signi ficantly reduce water absorption in the composite.Overall,the very low moisture absorption with cellulose-based composites makes them appealing in load bearing and outdoorapplications.Fig.2.a)Young's modulus values of LDPE/CB (blue dots),LDPE/MCC (red lines)and LDPE/CNC (green checkered)composites prepared by SSSP,and b)Elongation at break values of LDPE/CB (blue dots),LDPE/MCC (red lines)and LDPE/CNC (green checkered)composites prepared by SSSP.(For interpretation of the references to color in this figure legend,the reader is referred to the web version of this article.)Table 2Mechanical properties of PP/cellulosic filler composites produced by SSSP.SampleYoung's modulus E (MPa)Yield strength s (MPa)Elongation at break ε(%)Neat PP1910±5032.0±0.7740±4095/5wt%PP1/CB 1130±9031.2±1.040±1085/15wt%PP1/CB 1560±8032.6±1.710±275/25wt%PP1/CB 1750±9032.7±0.98±3Neat PP21200±2036.0±1.0700±4090/10wt%PP1/MCC 1600±5035.6±1.110±290/10wt%PP1/CNC a1830±7038.0±1.012±3Note.PP1and PP2samples employed in this study had different tensile properties despite having the same MFI values (as reported by suppliers).aData for PP/CNC composites are from Ref.[39].K.A.Iyer et al./Polymer 75(2015)78e 87823.4.Nucleating ef ficiency of polyole fin/CB compositesFig.4and Table 3summarize the effects of well-dispersed CB,MCC and CNC on the crystallization of LDPE and PP.Cellulosic fillers are often good nucleating agents for polymer crystallization.However,the extent of enhancement in crystallization depends strongly on filler dispersion and size reduction and the inherent crystallizability of the polymer.The LDPE used in this study has a very fast crystallization rate,and hence incorporating cellulosic fillers provides no additional bene fit.Within error,all LDPE/CB composites retain crystallization behavior similar to that of neat LDPE.On the other hand,excellent particle size reduction and very good dispersion of CB pieces lead to major increases in PP crystal-lization rate.Relative to neat PP1with on onset temperature of crystallization (T c )of 117.1 C,incorporating 5wt%and 15wt%CB results in 10.2 C and 11.4 C increases in T c ,respectively.En-hancements are also seen in overall crystallinity of PP in the com-posite;neat PP1is 44.7%crystalline whereas in a 75/25wt%PP1/CBFig.3.Water uptake of neat polyole fin and SSSP-processed composites plotted as a function of square root of time for a)LDPE,LDPE/CB,LDPE/MCC,and LDPE/CNC and b)PP1,PP1/CB,PP2/MCC and PP2/CNC (Note.PP1and PP2had similar water absorptionbehavior).Fig.4.a)Nonisothermal crystallization curves for neat PP1and PP1/CB composites prepared via SSSP.b)Isothermal crystallization curves (140 C)for neat PP1and PP1/CB composites prepared via SSSP.K.A.Iyer et al./Polymer 75(2015)78e 8783。
拉曼光谱分析(二)

Semi-quantitati v e analysis of indigo by surface enhanced resonance Raman spectroscopy (SERRS)using sil v er colloidsI.T.Shadi,B.Z.Chowdhry,M.J.Snowden,R.Withnall *Vibrational Spectroscopy Centre,School of Chemical and Life Sciences,Uni v ersity of Greenwich,Pembroke,Chatham Maritime CampusChatham,Kent ME44TB,UKRecei v ed 13June 2002;accepted 2September 2002AbstractIn this paper we report for the first time semi-quantitati v e analysis of indigo using surface enhanced Raman spectroscopy (SERS)and surface enhance resonance Raman spectroscopy (SERRS).Indigo,a dye widely used today in the textile industry,has been used,historically,both as a dye and as a pigment;the latter in both paintings and in printed material.The molecule is uncharged and largely insoluble in most sol v ents.The application of SERS/SERRS to the semi-quantitati v e analysis of indigo has been examined using aggregated citrate-reduced sil v er colloids with appropriate modifications to experimental protocols to both obtain and maximise SERRS signal intensities.Good linear correlations are obser v ed for the dependence of the intensities of the SERRS band at 1151cm (1using laser exciting wa v elengths of 514.5nm (R 00.9985)and 632.8nm (R 00.9963)on the indigo concentration o v er the range 10(7Á10(5and 10(8Á10(5mol dm (3,respecti v ely.Band intensities were normalised against an internal standard (sil v er sol band at 243cm (1).Resonance Raman spectra (RRS)of aqueous solutions of indigo could not be collected because of its low solubility and the presence of strong fluorescence.It was,howe v er,possible to obtain RS and RRS spectra of the solid at each laser excitation wa v elength.The limits of detection (L.O.D.)of indigo by SERS and SERRS using 514.5and 632.8nm were 9ppm at both exciting wa v elengths.Signal enhancement by SERS and SERRS was highly pH dependent due to the formation of singly protonated and possibly doubly protonated forms of the molecule at acidic pH.The SERS and SERRS data pro v ide e v idence to suggest that an excess of monolayer co v erage of the dye at the surface of sil v er colloids is obser v ed at concentrations greater than 7.85)10(6mol dm (3for each exciting wa v elength.The data reported herein also strongly suggest the presence of multiple species of the indigo molecule.#2003Else v ier B.V.All rights reserved.Keywords:Indigo;Colloids;Sil v er sol;Surface enhanced resonance Raman spectroscopy (SERRS);Resonance Raman spectroscopy (RRS);Semi-quantitati v e analysis;Internal standard1.IntroductionIndigo,a dye widely used today in the textile industry [1],is also of archaeological and historical importance [2]ha v ing been used as a dye and a pigment,the latter in both paintings and printed*Corresponding author.Tel.:'44-208-331-8691;fax:'44-208-331-9983.E-mail address:r.withnall@ (R.Withnall).Spectrochimica Acta Part A 59(2003)2213Á2220www.else v /locate/saa1386-1425/03/$-see front matter #2003Else v ier B.V.All rights reserved.doi:10.1016/S1386-1425(03)00065-9material.A v ailable e v idence suggests the use of the dye pre-dates the Christian era by at least4000 years[3,4].The molecule is uncharged and rela-ti v ely insoluble in most sol v ents.To our knowl-edge a surface enhanced Raman spectroscopy (SERS)/surface enhanced resonance Raman spec-troscopy(SERRS)in v estigation of this molecule using sil v er colloids has not been reported in the literature.Howe v er,analytical in v estigations of this molecule ha v e largely been carried out using HPLC,resonance Raman scattering(RRS)[2]and FT Raman[5],the last two techniques ha v ing been applied to solid material.In this study SERS and SERRS of indigo ha v e been obtained by adding methanolic solutions to aqueous sil v er sols.Spec-tra were obtained through modification of a pre v iously used experimental protocol and the SERS/SERRS signal output optimised.SERRS signal enhancement arises from a com-bination of signal intensification,v ia RRS and surface enhanced Raman scattering mechanisms, which can increase the efficiency of the Raman scattering process by10-fold[10]or greater[6]. For maximum sensiti v ity,SERRS requires con-trolled aggregation of the colloidal sol used[7]. Surface enhancement of the Raman signals is dependent on the size of the colloidal particles as well as the exciting wa v elength employed.This is because the surface plasmon absorption bands of metals such as sil v er and gold show wa v elength dependent shifts with metal particle size,and surface enhancement is achie v ed by choosing the Raman exciting wa v elength to coincide with the plasmon band[8].Spectra were subsequently collected using a LabRam spectrometer,equipped with argon ion and heliumÁneon lasers which pro v ided exciting radiation of wa v elengths equal to514.5and632.8 nm,respecti v ely.The exciting wa v elength of632.8 nm lies within the electronic absorption band of solutions of indigo in methanol which peaks at611 nm,con v ersely the exciting wa v elength of514.5 nm lies in the short wa v elength wing.SERRS has been shown to ha v e significant potential for the quantitati v e determination of analytes,e.g.SERRS studies,using citrate reduced and borohydride reduced sil v er sols,in an in v es-tigation of alcian blue8GX,re v ealed different properties for each sol.Furthermore,it was demonstrated that it was possible to combine the linear regions obser v ed in SERRS with that of RRS(upon normalisation against an internal standard)extending the quantifiable linear con-centration range[9].SERRS has also been applied to a study of the detection and identification of specific sequences of labelled DNA suggesting a potential approach towards detecting specific sequences of DNA,which could ultimately replace the need to amplify DNA using polymerase chain reaction(PCR)procedures[10].Vibrational spec-tra of LH2complex isolated from two photosyn-thetic bacteria were obtained using SERRS[11]. Metallation kinetics of a free base porphyrin, where the SERRS sil v er colloid system has been employed as a probe,has been reported for the in v estigation of porphyrinÁnucleic acids interac-tion[12].The SERRS technique has also been applied successfully to measure Raman spectra from an oxygenic photosynthetic pigmentÁprotein complex by excitation within the Q(y)transition [13].and SERRS spectra of porphyrin and metal-loporphyrin species in systems ha v e been obtained using sil v er nanoparticles modified by anionic organosulfur spacers[14].These examples illus-trate some of the di v erse applications of the SERRS technique.The in v estigation reported herein was under-taken with the specific aim of de v eloping appro-priate experimental protocols for optimization of signal intensities,with subsequent determination and comparison of the extent of the linearity of the signal dependence on concentration and the limits of detection(L.O.D.)for the semi-quantitati v e analysis of indigo by SERS and SERRS.2.Experimental2.1.ReagentsIndigo(Aldrich),poly(L-lysine)hydrobromide M r4000Á15000(Sigma),sil v er nitrate(BDH), methanol(Fisher),tri-sodium citrate(Fisher), ascorbic acid(Fisher),sodium hydroxide(Fisher) and hydrochloric acid(Fisher)were of analytical grade.The dye was used without further purifica-I.T.Shadi et al./Spectrochimica Acta Part A59(2003)2213Á2220 2214tion.Double de-ionised water was used for all experiments.2.2.InstrumentationSERS/SERRS and RS spectra were obtained using a Labram Raman spectrometer(Instruments S.A.,Ltd.)equipped with an1800g mm(1 holographic grating,a holographic super-notch filter(Kaiser),an Olympus BX40microscope,and a Peltier-cooled CCD(MPP1chip)detector.A heliumÁneon laser and an argon ion laser pro v ided 632.8and514.5nm exciting radiation,respecti v ely which was attenuated by a10%neutral density filter,resulting in a laser power of0.8mW at the static sol.All SERS/SERRS and RRS spectra were collected by using a1808back-scattering geome-try.An Olympus microscope objecti v e,ha v ing a magnification of)10and a numerical aperture of 0.25,was used both to focus the incident laser light and to collect the back-scattered Raman light.2.3.Colloid preparationA sil v er colloid was prepared according to a modified LeeÁMeisel procedure[7,15].All glass-ware was acid washed with aqua regia[HNO3ÁHCl(1:3,v/v)]followed by gentle scrubbing with a soap solution.Sil v er nitrate(90mg)was suspended in500ml of de-ionised water at458C and rapidly heated to boiling before a1%solution of tri-sodium citrate(10ml)was added under v igorous stirring.The solution was held at boiling for90min with continuous stirring upon cooling; the v olume was made up to500ml with de-ionised water.The quality of the resulting colloid was checked by determining the wa v elength of the absorption maximum in the v isible region on a PerkinÁElmer Lambda-2UVÁVis spectrometer. Good quality sil v er colloids for SERS apparently ha v e an absorption maximum at approximately 404nm and full width half height(FWHH)ofB 60nm[6].The nature of the LeeÁMeisel colloid [15,16],often used for SERS,has been examined using v isible absorption,photon correlation and NMR spectroscopic techniques which confirm that the surface of the sil v er particles are co v ered with a layer of citrate with pendent negati v ely charged groups.Howe v er,the subsequent addition of poly(L-lysine)again coats the surface resulting in pendent positi v ely charged groups on the colloidal surface[17].2.4.Indigo solutionsFor SERS/SERRS in v estigation solutions,ha v-ing a final indigo concentration in the range of 10(8Á10(5mol dm(3were prepared in methanol. For RRS in v estigation,a10(5mol dm(3dye concentration(maximum solubility)was used. Samples were always made up fresh,immediately before analysis was carried out.The suppliers of the indigo(structure shown in Fig.1)confirm it has a purity of95%.2.5.RS of solidFor RRS in v estigation of the solid the dye was used,directly from the suppliers bottle without further purification.2.6.Sample preparationAggregation of the sil v er colloid particles was induced by poly(L-lysine).One hundred and fifty microlitres of a0.01%aqueous solution of poly(L-lysine)was added to1ml of sil v er colloid which had been diluted with1ml of de-ionised water, followed by150m l of the methanolic indigo solution and35m l of a1mol dm(3aqueous solution of ascorbic acid.In subsequent experi-ments poly(L-lysine)was not used.Instead aggre-gation of the sol was induced with35m l of1mol dm(3HCl before adding150m l of the methanolic indigosolution.Fig.1.Schematic structure of indigo.I.T.Shadi et al./Spectrochimica Acta Part A59(2003)2213Á222022152.7.ReproducibilitySERRS spectra were collected approximately5 min after mixing the indigo solution with the sil v er sol.2.8.Concentration dependence of indigo (normalization)The concentration dependence of indigo was determined by plotting the log intensity of the 514.5and632.8nm excited SERRS bands at582, 986and1151cm(1of indigo v s log indigo concentration.The same bands were normalized against the internal standard(sil v er sol band at 243cm(1).The intensities of the Raman bands were measured as the peak area after baseline correction.2.9.SERRS pH dependenceA pH profile of a10(5mol dm(3indigo dye concentration was obtained o v er the pH range of 0.5Á6.5using an exciting wa v elength of514.5nm.2.10.Packing effects at colloidal surface Packing effects at the colloidal surface were determined by plotting wa v enumber shifts for the band at1717cm(1v s log dye concentration.3.Results and discussion3.1.SERRSThe theory of SERS enhancement of analytes is well known[8,10,16].In the current study it was found that aggregation of the colloidal particles, using poly(L-lysine),pre v ented collection of SERS/ SERRS spectra.In a subsequent series of experi-ments a modified protocol was applied in which poly(L-lysine)was not used.It was also apparent that adsorption of indigo molecules to sil v er colloids was highly pH dependent.We were able to further optimise signals in a subsequent set of experiments by substituting ascorbic acid with1 mol dm(3HCl.Aggregation of the sol was induced by addition of35m l of1mol dm(3HCl to the diluted sol to which150m l of the aqueous dye was added.SERS/SERRS pH profiles were obtained for each exciting wa v elength and opti-mum signal intensification for this molecule was found to be at approximately pH1.75.SERS spectra of the aqueous dye solutions using 514.5nm excitation(Fig.2a)show strong SERS bands at242,582,986,1151,1366,1624and1717 cm(1.For SERRS in v estigations using632.8nm Fig.2.(a)Representati v e514.5nm excited SERS spectra of indigo in the signal v s concentration range examined.Concen-trations of the dye,from top to bottom are:7.85)10(5, 3.95)10(5, 1.98)10(5,7.85)10(6, 3.95)10(6, 1.98) 10(6,7.85)10(7and3.95)10(7mol dm(3.SERS v ibra-tional bands used for analysis are indicated by solid arrows, dashed arrow represents the sil v er sol band used as internal standard.(b)Log concentration dye v s log signal intensity (peak area)o v er the concentration range examined for the bands at582j(I),986m(II)and1151cm(1'(III).(c) Bands at582j(I)and986m(II)and1151cm(1'(III) normalised against the sil v er sol band at242cm(1.I.T.Shadi et al./Spectrochimica Acta Part A59(2003)2213Á2220 2216excitation (Fig.3a)strong bands were obser v ed at 243,583,806,988,1151,1238,1323,1464,1626and 1717cm (1.It is worth noting that the same bands were obser v ed for each exciting wa v elength with two exceptions,the profile of both the spectra and the relati v e intensities of bands for each exciting wa v elength differed significantly (Fig.2a and Fig.3a).3.2.Linear regionsFor each exciting wa v elength fluorescence was completely quenched with good linear correlations [(R 00.9985and 0.9963)]pro v iding L.O.D.s of 9ppm using the band at 1151cm (1for the dye concentrations of 3.95)10(7and 1.98)10(7mol dm (3using 514.5and 632.8nm exciting wa v elengths,respecti v ely.A linear concentration range of 3orders of magnitude was obtained for each exciting wa v elength (Fig.2b and Fig.3c).This v alue reflects the plots that pro v ided the best linear correlations.It was obser v ed that there was no marked impro v ement in linear correlations for 514.5nm excited bands,upon normalisation using the 243cm (1sil v er sol band as internal standard.The re v erse is true for 632.8nm excited bands,where a significant impro v ement to linear correla-tions was obser v ed for all bands examined upon normalisation (see Table 1).This appears to be due to the resonance effect obser v ed in 632.8nm excited SERRS spectra.3.3.General profileIn pre v ious studies [9]of dyes it has been obser v ed that the highest concentration of dye gi v es a comparati v ely low Raman intensity signal (due to the surface of the colloidal sil v er particles being in excess of a full monolayer co v erage).When compared to subsequent samples of lower concentration,where Raman intensities increase and peak,thereafter signal intensities decrease as a function of concentration down to the L.O.D.;this region shows a linear dependence of the SERS/SERRS signal with concentration.Indigo does not follow this profile most probably due to its low solubility.Spectra appear to be obtained as a direct consequence of protonation of the dye and subsequent adsorption directly to the colloidal sil v er surface resulting in what appears to be monolayer co v erage.The phenomenon of self-absorption of scattered radiation is not obser v ed for the dye concentrations used in this study,instead,it appears spectra for the highest concen-tration of indigo examined (maximum solubility)are obtained in what would be considered the upper linear region of a SERRSconcentrationFig.3.(a)Representati v e 632.8nm excited SERRS spectra of indigo in the signal v s concentration range examined.Concen-trations of dye from top to bottom are:7.85)10(5,3.95)10(5, 1.98)10(5,7.85)10(6, 3.95)10(6, 1.98)10(6,7.85)10(7,3.95)10(7and 1.98)10(7mol dm (3.SERRS v ibrational bands used for analysis are indicated by solid arrows,dashed arrow represents the sil v er sol band used as internal standard.(b)Log concentration of dye v s log signal intensity (peak area)o v er the concentration range examined for the bands at 583j (I)and 986m (II)and 1151cm (1'(III).(c)Bands at 583j (I),986m (II)and 1151cm (1'(III)normalised against the sil v er sol band at 242cm (1.I.T.Shadi et al./Spectrochimica Acta Part A 59(2003)2213Á22202217study (profile)as obser v ed with other dyes.For 514.5and 632.8nm exciting wa v elengths max-imum signals were obser v ed for a dye concentra-tion of 7.85)10(5mol dm (3(Fig.2b and Fig.3b),thereafter,band intensities decreased as a function of concentration,and linearity for the signal dependence on dye concentration is ob-ser v ed down to 3.95)10(7and 1.98)10(7mol dm (3for each exciting wa v elength,respecti v ely.3.4.Packing effectsThe data (Fig.2b and c,Fig.3b and c)appear to suggest a monolayer co v erage of the dye on the colloidal surfaces.Howe v er,closer examination of the wa v enumber shifts for the v ibrational band at 1717cm (1(due to C ÄO)as a function of log dye concentration seems to suggest that an excess of monolayer co v erage is in fact obser v ed in the concentration range 10(6Á10(5mol dm (3(Fig.4c)for each exciting wa v elength.3.5.Solution RRSThe low solubility of the dye,in methanol,together with strong fluorescence did not re v eal dye bands (only methanol bands were obser v ed)using 514.5(Fig.4a (II))and 632.8nm (not shown)excitation.3.6.RRS of solidGood spectra were obtained for each exciting wa v elength.The spectrum obtained using 514.5nm excitation is shown in Fig.4a (I).3.7.SERRS pH dependencepH profiles were obtained,using a dye concen-tration of 10(4mol dm (3,for each exciting wa v elength re v ealing an optimum pH at approxi-mately 1.75(the pH profile obtained using 514.5nm excitation is shown in Fig.4b).3.8.Identification of multiple species of the dye Spectra collected across the pH range examined (0.5Á6.5)strongly suggest the presence of twoT a b l e 1P a r a m e t e r s o b t a i n e d f r o m m u l t i l i n e a r r e g r e s s i o n f o r a n a l y s i s o f i n d i g oT e c h n i q u e (n m )B a n d (c m (1)S l o p e I n t e r c e p tC o r r e l a t i o n c o e f f i c i e n tC o n c e n t r a t i o n r a n g e (m o l d m (3)R .S .D .(9)L .O .D a (p p m )O r d e r s o f M a g n i t u d e bS E R S 514.55820.475.370.991810(7Á10(50.053193S E R S 514.5c5820.600.210.990510(7Á10(50.073833S E R S 514.59861.278.570.980010(6Á10(50.1808112S E R S 514.5c9861.483.780.984010(6Á10(50.1760152S E R S 514.511510.535.760.998510(7Á10(50.025693S E R S 514.5c11510.660.580.993210(7Á10(50.067663S E R R S 632.85830.888.820.992010(7Á10(50.1108103S E R R S 632.8c5830.863.200.9956710(7Á10(50.077673S E R R S 632.89860.979.030.973010(6Á10(50.1560172S E R R S 632.8c9860.923.330.982410(6Á10(50.1196102S E R R S 632.811510.446.540.980610(7Á10(50.0844133S E R R S 632.8c 11510.430.970.996310(7Á10(50.035793aT h r e e t i m e s s t a n d a r d d e v i a t i o n o f i n t e r c e p t /s l o p e .bF o r l i n e a r r e g i o n s .cN o r m a l i s e d a g a i n s t t h e v i b r a t i o n a l b a n d a t 243c m (1.I.T.Shadi et al./Spectrochimica Acta Part A 59(2003)2213Á22202218forms of the same dye.It was apparent that the ratios of se v eral bands differed significantly,as a function of pH.This was further substantiated on closer examination of the dye bands in spectra from the SERS/SERRS concentration study where it was clear that the intensities of se v eral bands decreased at a faster rate than other bands.Further e v idence for this can be seen when the slopes using 514.5and 632.8nm exciting wa v e-lengths are compared for the v ibrational bands at 582and 984cm (1(see Table 1and Fig.2c and Fig.3c).4.ConclusionIn this study it has been shown that SERS/SERRS o v ercomes the difficulties associated with obtaining RRS spectra of aqueous solutions of indigo for semi-quantitati v e analysis.Both 514.5and 632.8nm exciting wa v elengths re v ealed simi-lar quantifiable linear concentration ranges of 3orders of magnitude in the concentration range 10(8Á10(5mol dm (3.In this study it was possible to normalise dye bands against an internal standard,resulting in significant enhancement of RSD fits for 632.8nm excited SERRS spectra but made little difference to 514.5nm excited SERS spectra.The molecule was shown to be highly pH sensiti v e,the data re v ealing the presence of pro-tonated forms of the molecule.AcknowledgementsR.W.and B.Z.C.wish to acknowledge the EPSRC (ref.GR/L85176)and Instruments S.A.,Ltd.for jointly funding the purchase of the Labram Raman Spectrometer.References[1]H.A.Lubs,The chemistry of synthetic dyes and pigments,A.C.S.Monograph Series,Malabar (1955).[2]R.Withnall,A.Derbyshire,S.Thiel,M.J.Hughes,Proc.SPIE 4098(2000)217.[3]M.R.Fox,J.H.Pierce,Textile Chemist Colorist 22(1990)13.[4]A.S.Tra v is,Textile Chemist Colorist 22(1990)18.[5]E.Tatsch,B.Schrader,J.Raman Spectrosc.26(1995)467Á473.[6]C.Rodger,V.Rutherford,P.C.White,W.E.Smith,J.Raman Spectrosc.29(1998)601.[7]C.Rodger,W.E.Smith,G.Dent,M.Edmondson,J.Chem.Soc.,Dalton Trans.5(1996)791.[8]J.A.Creighton,C.G.Blatchford,M.G.Albrecht,J.Chem.Soc.,Faraday Trans.75(2)(1979)790Á798.[9]I.T.Shadi,B.Z.Chowdhry,M.J.Snowden,R.Withnall,Appl.Spectrosc.54(2000)384Á389.[10]D.Graham,B.J.Mallinder,W.E.Smith,Biopolymers 57(2000)85Á91.[11]G.Chumano v ,R.Picorel,I.O.deZarate,T.M.Cotton,M.Seibert,Photochem.Photobiol.71(2000)589Á595.[12]M.Prochazka,P.Y.Turpin,J.Stepanek,J.Bok,J.Molec.Struct.483(1999)221Á224.Fig.4.(a)Raman scattering (RS)spectra for solid indigo (I)and a 2)10(4mol dm (3methanolic solution (II)of indigo using 514.5nm excitation.(b)514.5nm excited SERS pH profile in the pH range 0Á7,for the v ibrational band at 582cm (1,of indigo at a dye concentration 7.85)10(5mol dm (3.(c)Plot of wa v enumber shift for the C ÄO band around 1717cm (1across the concentration range examined for 514.5and 632.8nm exciting wa v elengths.I.T.Shadi et al./Spectrochimica Acta Part A 59(2003)2213Á22202219[13]R.Picorel,G.Chumano v,E.Torrado,T.M.Cotton,M.Selbert,J.Phys.Chem.102(1998)2609Á2613.[14]B.Vicko v a,P.Smejkal,P.Michl,M.Prochazka,P.Mojzes,F.Lednicky,J.Pfleger,J.Inorg.Biochem.79 (2000)295Á300.[15]P.C.Lee,D.Meisel,J.Phys.Chem.86(1982)3391.[16]C.H.Munro,W.E.Smith,M.Garner,J.Clarkson,P.C.White,Langmuir11(1995)3712Á3720.[17]C.H.Munro,W.E.Smith,D.R.Armstrong,P.C.White,J.Phys.Chem.99(1995)879.I.T.Shadi et al./Spectrochimica Acta Part A59(2003)2213Á2220 2220。
微纳金属结构光吸收增强英文

微纳金属结构光吸收增强英文Enhanced Light Absorption in Micro-Nano Metal Structures.The field of photonics has witnessed remarkable advancements in recent years, with a particular focus on enhancing light absorption in micro-nano metal structures. This enhancement is crucial for various applications ranging from solar cells, photodetection, and sensing to plasmonic devices. The unique optical properties of metals at the nanoscale offer opportunities for manipulatinglight-matter interactions, leading to improved performance in these areas.1. Plasmonic Resonance in Metal Nanostructures.The key to understanding light absorption enhancement in micro-nano metal structures lies in the concept of plasmonic resonance. Plasmons are collective oscillations of electrons in a metal that can be excited by incidentlight. When the frequency of incident light matches the natural frequency of these oscillations, a resonance condition is achieved, leading to a significant enhancement of the electromagnetic field around the metal structure. This enhanced field in turn increases the light absorption by the metal.2. Nanostructuring for Enhanced Absorption.Nanostructuring metals offers a powerful means to control plasmonic resonances and thereby enhance light absorption. By reducing the dimensions of metal structures to the nanoscale, it becomes possible to tune the plasmonic resonances to match the desired wavelength of light. This tuning can be achieved by varying the size, shape, and composition of the nanostructures.3. Materials Considerations.The choice of metal material is also crucial for light absorption enhancement. Noble metals such as gold andsilver are commonly used due to their strong plasmonicresponse. However, these metals often suffer from high ohmic losses that limit their performance. Alternatively, alternative metals with lower losses, such as aluminum and magnesium, have been explored. Furthermore, the use of alloys and composite materials can further optimize the plasmonic response and absorption properties.4. Applications of Enhanced Light Absorption.Enhanced light absorption in micro-nano metal structures finds applications in diverse fields. In solar cells, for example, plasmonic nanostructures can increase the absorption of sunlight, leading to improved conversion efficiencies. Similarly, in photodetection and sensing applications, the enhanced absorption can enhance the sensitivity and response speed. In plasmonic devices, the strong localization of light at the nanoscale offers opportunities for nanoscale imaging, spectroscopy, and manipulation of light.5. Challenges and Future Directions.Despite the significant progress made in enhancinglight absorption in micro-nano metal structures, several challenges remain. One of the primary challenges is the limited stability of plasmonic nanostructures, especially under harsh environmental conditions. Additionally, the integration of these structures into practical devices requires further research and development. Futuredirections include exploring new materials and design strategies to overcome these challenges and further improve light absorption enhancement.In conclusion, enhanced light absorption in micro-nano metal structures holds promise for revolutionizing various photonic applications. By harnessing the unique optical properties of metals at the nanoscale, it is possible to manipulate light-matter interactions and achieve remarkable improvements in light absorption. While challenges remain, ongoing research and development in this field are expected to lead to transformative advancements in the near future.。
Effect of valence electron concentration on stability of fcc or bcc phase in high entropy alloys

See discussions, stats, and author profiles for this publication at: /publication/229595062 Effect of valence electron concentration on stability of fcc or bcc phase in high entropy alloysARTICLE in JOURNAL OF APPLIED PHYSICS · MAY 2011Impact Factor: 2.19 · DOI: 10.1063/1.3587228CITATIONS 80DOWNLOADS143VIEWS4104 AUTHORS, INCLUDING:Sheng GuoChalmers University of Technology29 PUBLICATIONS 329 CITATIONSSEE PROFILEAvailable from: Sheng GuoRetrieved on: 16 September 2015Effect of valence electron concentration on stability of fcc or bcc phase in high entropy alloysSheng Guo,1Chun Ng,1Jian Lu,2and C.T.Liu1,a)1Department of Mechanical Engineering,The Hong Kong Polytechnic University,Hung Hom,Kowloon,Hong Kong,People’s Republic of China2College of Science and Engineering,City University of Hong Kong,Kowloon,Hong Kong(Received8March2011;accepted3April2011;published online16May2011)Phase stability is an important topic for high entropy alloys(HEAs),but the understanding to it isvery limited.The capability to predict phase stability from fundamental properties of constituentelements would benefit the alloy design greatly.The relationship between phase stability andphysicochemical/thermodynamic properties of alloying components in HEAs was studiedsystematically.The mixing enthalpy is found to be the key factor controlling the formation of solidsolutions or compounds.The stability of fcc and bcc solid solutions is well delineated by thevalance electron concentration(VEC).The revealing of the effect of the VEC on the phase stabilityis vitally important for alloy design and for controlling the mechanical behavior of HEAs.V C2011American Institute of Physics.[doi:10.1063/1.3587228]I.INTRODUCTIONHigh entropy alloys(HEAs)constitute a new type of metallic alloys for structural and particularly high-tempera-ture applications,due to their high hardness,wear resistance, high-temperature softening resistance and oxidation resist-ance.1–3HEAs are typically composed of more thanfive me-tallic elements in equal or near-equal atomic ratios,and interestingly they tend to form solid solution structure (mainly fcc and/or bcc)rather than multiple intermetallic compounds as expected from general physical metallurgy principles.They were termed as HEAs because the entropy of mixing is high when the alloying elements are in equia-tomic ratio,and it was initially believed that the high entropy of mixing leads to the formation of the solid solution struc-ture rather than intermetallic compounds.The generally used alloying elements in HEAs are fcc-type Cu,Al,Ni,bcc-type Fe,Cr,Mo,V and hcp-type Ti,Co (crystal structure at ambient temperature).When these alloy-ing elements are mixed with different combination,or with same combination but different amount of certain elements, fcc,bcc or mixed fcc and bcc structures may form.For example,cast CoCrCuFeNi(in atomic ratio,same after-wards)has the fcc structure while AlCoCrCuFeNi has the fccþbcc structure;4and the amount of Al in the Al x CoCrCu-FeNi system can tune the crystal structure from fcc to fccþbcc and to fully bcc4.The structure directly affects the mechanical properties,and to take again the Al x CoCrCuFeNi system as an example:with increasing x,the structure changes from fcc to fccþbcc andfinally to bcc;the hardness and strength increase with the increasing amount of bcc phases but the alloys get brittle.4,5Although the embrittle-ment mechanism by bcc phases still needs further explora-tion,it is certainly important to be able to control the formation of bcc phases.The target of this work is hence to find out the physical parameters that control the stability for the fcc and bcc phases in HEAs.II.ANALYSISWang et al.briefly discussed the reason of addition of Al in the Al x CoCrCu1Àx FeNiTi0.5system causing the struc-tural transition from fcc to bcc.6They claimed that the alloy-ing of larger Al atoms introduces the lattice distortion energy,and the formation of a lower atomic-packing-effi-ciency structure,such as bcc,can decrease this distortion energy.This does make sense but is far away from being sat-isfactory;besides,it can not quantitatively predict when the bcc structure will form as a function of Al additions.Ke et al.claimed that,in the Al x Co y Cr z Cu0.5Fe v Ni w system,Ni and Co are fcc stabilizers and Al and Cr are bcc stablizers;1.11Co is equivalent to Ni as the fcc stablizers and2.23Cr is equivalent to Al for the bcc stablizers.Furthermore,if the equivalent Co%is greater than45at.%,the alloy has an fcc structure,and the alloy has a bcc structure if the equivalent Cr%is greater than55at.%.7This empirical rule is useful but it has no scientific merits and is valid only for the specific alloy systems.The establishment of scientific principles to control the crystal structures in HEAs can also contribute to the alloy design of HEAs with desirable properties.For example,we can use less expensive Ni to partially or com-pletely replace more expensive Co;or we can reduce the amount of Cu which is known to cause segregation issue because the mainly positive enthalpy of mixing between Cu and other alloying elements.4As a test to the equivalency of Ni and Co as fcc stabil-izers,we prepared a series of Al x CrCuFeNi2(0.2x 1.2) alloys to study the effect of Al amount on the phase stability in this alloy system,in comparison to the well studied Al x CoCrCuFeNi system.The alloys were prepared by arc-melting a mixture of the constituent elements with purity better than99.9%in a Ti-gettered high-purity argon atmos-phere.Repeated melting was carried out at leastfive times toa)Author to whom correspondence should be addressed.Electronic mail:mmct8tc@.hk.0021-8979/2011/109(10)/103505/5/$30.00V C2011American Institute of Physics109,103505-1JOURNAL OF APPLIED PHYSICS109,103505(2011)improve the chemical homogeneity of the alloy.The molten alloy was drop-cast into a10mm diameter copper mold.The phase constitution of the alloy was examined by X-ray dif-fractometer using Co radiation(Bruker AXS D8Discover). The X-ray diffraction patterns are shown in Fig.1where it is clear that at x0.8,the alloys have a single fcc structure and the bcc phase starts to appear at x¼1.0.In the Al x CoCrCu-FeNi system,fully fcc structure is obtained at x0.5and bcc phase starts to appear at x>0.8.4The experimental results indicate that Co is not necessarily required in obtain-ing the solid solution structure in HEAs,which is good foralloy design from economy concerns.This new alloy systemalso provides more data to study the phase stability in HEAs,ideally from the consideration of the fundamental propertiesof constituent alloying elements.Zhang et al.studied the relationship between the phasestability and the atomic size difference,d(¼100ffiffiffiffiffiffiffiffiffiffiffiffiffiffiffiffiffiffiffiffiffiffiffiffiffiffiffiffiffiffiffiffiffiffiffiffiP Ni¼1c ið1Àr i=rÞ2q,r¼P ni¼1c i r i,where c i and r i areatomic percentage and atomic radius of the i th component),and also the mixing enthalpy,D H mix(¼P ni¼1;i¼jX ij c i c j,X ij¼4D AB mix,where D AB mix is the mixing enthalpy of binaryliquid AB alloys)for multi-component alloys.8They foundthat the solid solution tends to form in the region delineatedbyÀ15KJ/mol D H mix5KJ/mol and1d 6.Therequirement of atomic size difference for formation of thesolid solution structure is not surprising as basically it is inline with the well established Hume-Rothery rule.9Othertwo requirements from the Hume-Rothery rule to form thesolid solution are electronegativity and electron concentra-tion.Fang et al.defined the electronegativity difference in amulti-component alloy system as D v(¼ffiffiffiffiffiffiffiffiffiffiffiffiffiffiffiffiffiffiffiffiffiffiffiffiffiffiffiffiffiffiffiffiffiP ni¼1c iðv iÀvÞ2q,v¼P ni¼1c i v i,where v i is the Pauling electronegativity forthe i th component).10The effect of electron concentration isa little bit more complex.There are basically two definitionsof the electron concentration:average number of itinerantelectrons per atom,e/a,and the number of total electronsincluding the d-electrons accommodated in the valenceband,valence electron concentration or VEC.11,12e/a orVEC for a multi-component alloy can be defined as theweighted average from e/a or VEC of the constituent compo-nents:e/a¼P ni¼1c iðe=aÞi or VEC¼P ni¼1c iðVECÞi,where(e/a)i and(VEC)i are the e/a and VEC for the individual ele-ment.Hume-Rothery rule works with the e/a definition ande/a has clear effect on the crystal structure for the so-calledelectron compounds or Hume-Rothery compounds.9How-ever,the HEAs comprise mainly transition metals(TMs)ande/a for TMs are very controversial.11Very recently,Mizutanireviewed the various definitions of e/a for TMs and con-cluded that e/a for TMs are small positive numbers.11Unfortunately,not all e/a for TMs have been determined ande/a for a TM element even varies in different environment.For convenience,VEC was used here to study the electronconcentration effect on the phase stability in HEAs.III.RESULTSFollowing Zhang et al.’s method,8the atomic size dif-ference,d,and the mixing enthalpy,D H mix for the Al x CoCr-CuFeNi4and Al x CrCuFeNi2systems are plotted in Fig.2.The electronegativity difference,D v,and VEC are also plot-ted to show how these factors referred in the Hume-Rotheryrule affect the solid solution formation.D H mix,D v and VECare all plotted as a function of d in Fig.2for convenience,and this does not indicate these parameters are mutually de-pendent.For comparison,d,D H mix,D v and VEC for threeadditional systems of HEAs[CoCrCuFeNiTi x(see Ref.13),Al0.5CoCrCuFeNiTi x(see Ref.14),Al0.5CoCrCuFeNiV x(see Ref.15)],where compounds will form in the originallyfcc-typed alloy by increasingly doping the amount of onealloying element(Ti or V),are also plotted in Fig.2.The cal-culation required physicochemical and thermodynamicFIG.1.(Color online)X-ray diffraction patterns for Al x CrCuFeNi2alloys(x¼0.2$1.2).FIG.2.(Color online)Relationship between the mixing enthalpy,D H mix(a),the Electronegativity,D v(b)and the valence electron concentration,VEC,(c),and the atomic size difference,d,forfive HEA systems:Al x CoCrCu-FeNi,CoCrCuFeNiTi x,Al0.5CoCrCuFeNiTi x,Al0.5CoCrCuFeNiV x,andAl x CrCuFeNi2.Note on the legend:fully closed symbols for sole fcc phases;fully open symbols for sole bcc phase;top-half closed symbols for mixes fccand bcc phases;left or right-half closed symbols for phases containing atleast one compound phase(left or right half simply indicates different typesof compounds).parameters for the constituent alloying elements are from Refs.16–19and some of them are listed in Table I .As seen from Fig.2,using the definitions of atomic size difference,mixing enthalpy,valence electron concentration and electronegativity defined here,D H mix is the only effec-tive parameter that can predict the formation of sole solid solutions (hence no formation of compounds)in HEAs.Solid solution form when À5KJ/mol D H mix 5KJ/mol,and compounds would form once D H mix is more negative.On the other hand,d ,D v and VEC all fail to effectively predict the formation of solid solution phases or compounds.Figure 2provides some clues to obtain the solely solid solution struc-ture in HEAs based simply on the fundamental properties of constituent elements.This is certainly useful but from Fig.2it is still unclear when the bcc phase will form and what is the determining factor controlling the bcc phase formation.A careful examination of Fig.2,however,suggests that bcc phases start to form when VEC reaches $8.0[Fig.2(c)].The other three parameters,D H mix ,d ,and D v do not behave such a clear indicator function.To make the point clearer,VEC for three HEA systems,Al x CoCrCuFeNi (see Ref.4),Al x CoCrCu 0.5FeNi (see Ref.7),and Al x CoCrCuFeNi 2(this work)in which increasingly doping of the same element Al would cause phase constitution from sole fcc to mixed fccand bcc,are plotted in Fig.3.Figure 3clearly shows that VEC can be used to quantitatively predict the phase stability for fcc and bcc phases in HEAs:at VEC !8.0,sole fcc phase exists;at 6.87 VEC <8.0,mixed fcc and bcc phases will co-exist and sole bcc phase exists at VEC <6.87.Note that at the boundary VEC ¼8.0,sometimes bcc phases also form but they are minor phases (see Fig.1and Ref.4).Although there is one exception for the Al x CoCrCu 0.5FeNi alloy where 6.87 VEC <8.0but the stable phase is sole bcc (not fcc þbcc),we suspect this VEC -defined phase stability shall work effectively for most cases.To prove this,VEC for more HEA systems with fcc,fcc þbcc,or bcc structure containing other alloying elements like Ti,V,Mn,Nb,Mo,Ta,W even metalloid B and C,are plotted in Fig.4(data are from litera-tures in Ref.7and 20–24).Although there are still some exceptions,the fcc/bcc phase boundary can clearly be delineated by VEC .With a note to those exceptions,the VEC -defined fcc/bcc phase boundary seems to work unsatis-factorily for Mn-containing HEA systems.TABLE I.Physiochemical properties for commonly used elements in HEAs.Element Atom radius (A˚)Pauling electronegativityVEC Al 1.4321.613B 0.8202.043C 0.773 2.554Co 1.251 1.889Cr 1.249 1.666Fe 1.241 1.838Mn 1.350 1.557Mo 1.363 2.166Nb 1.429 1.65Ni 1.246 1.9110Ta 1.430 1.505Ti 1.462 1.544V 1.316 1.635W1.3672.366FIG.3.(Color online)Relationship between VEC and the fcc,bcc phase sta-bility for three HEA systems:Al x CoCrCuFeNi,Al x CrCuFeNi 2and Al x CoCr-Cu 0.5FeNi.Note on the legend:fully closed symbols for sole fcc phases;fully open symbols for sole bcc phase;top-half closed symbols for mixes fcc and bccphases.FIG. 4.(Color online)Relationship between VEC and the fcc,bcc phase sta-bility for more HEA systems further to Fig.3.Note on the legend:fully closed symbols for sole fcc phases;fully open symbols for sole bcc phase;top-half closed symbols for mixes fcc and bcc phases.IV.DISCUSSIONThe effect of the VEC on the phase stability has been studied before by the current authors for the intermetallic compounds only.One example is on the(Fe,Co,Ni)3V inter-metallic alloys with long-range-ordered(LRO)structures.25 We found that these LRO alloys are characterized by specific sequences of stacked close-packed ordered layers and the stacking character can be altered systematically by control-ling the VEC of these alloys.With the decreasing VEC by partial substitution of Co and Ni by Fe,the LRO changes from purely hexagonal,to L12-type cubic ordered structure, through different ordered mixtures of hexagonal and cubic layers.As the hexagonal structure exhibits brittle fracture while the cubic ordered structures are ductile,the control of VEC can hence be used to tune the mechanical properties of LRO alloys.We also investigated the role of VEC in the phase stability of NbCr2-based transition-metal Laves phase alloys.26It was found that when the atomic size ratios were kept nearly identical,the VEC is the dominant factor in con-trolling the phase stability(C14,hexagonal or C15,cubic)in this type of high-temperature structural alloys.Our results in the present work prove that VEC also plays a decisive role in the stability of fcc and bcc solid solution phases in the multi-component HEAs.Mizutani has shown that VEC is crucial in determining the Fermi level whenfirst-principles band calcu-lations are carried out to study the band structure.11In the first-principles band calculations,the integration of the den-sity of states(DOS)actually results in VEC,which includes not only s-and p-electrons but also d-electrons forming the valence band.11Mizutani emphasized that the parameter VEC,instead of e/a,should be used in realistic electronic structure calculations to take into account the d-electron con-tribution.11More theoretical work needs to be carried out to understand the physical basis for the mechanism behind the VEC rule on the phase stability,for example from the VEC-electronic structure-bind structure energy perspective.27 Two issues need to be emphasized here for the discussion of the VEC rule on the phase stability.First,the VEC ranges for different phases to be stable might overlap and these ranges also vary depending on the specific alloy systems.A very close example is in some ternary Mg alloys that possess typical Laves structures.9It was found that the electron con-centration(e/a though)ranges for MgCu2-type(cubic,with packing ABCABCABC),MgNi2-type(hexagonal,with pack-ing ABACABAC)and MgZn2-type(hexagonal,with packing ABABAB)structures differ in various alloy systems,and the e/a ranges for MgNi2-type structure and MgZn2-type struc-ture overlap for the Mg-Cu-Al system.This can probably explain the exceptions that appear in Figs.3and4.Second, the phases referred in this work are all identified in the as-cast state and they are hence very possibly in the metastable state. However,evidences have shown that these metastable phases have quite good thermal stability28–31and can hence be regarded as very close to the stable phases.This gives confi-dence to the general applicability of the VEC rule,consider-ing it works so well for such an extended series of various alloy systems.More work is certainly needed to further verify this.Admitting these two issues mentioned above,one solid result out of our study is that,in HEAs the bcc phase is stable at lower VEC while the fcc phase is stable at higher VEC. This trend already sheds some light on the alloy design,and the fact that most HEA alloy systems satisfying the VEC (<6.87,bcc;!8,fcc)rule even simplifies the process.As the VEC rule on the phase stability between the fcc and bcc phases is tested only for the HEAs in this work,its applicabil-ity to other alloy systems other than the nearly equiatomic HEAs(i.e.,in the traditional alloys where only one or two pri-mary elements dominate),awaits further analysis.In addition, it would be interesting to know whether this VEC rule can be used to predict the phase stability for other structured phases, like the hcp-typed phases.More work along these directions is under way.V.CONCLUSIONSIn summary,the phase stability in HEAs and its relation-ship to the physicochemical and thermodynamic properties of constituent alloying elements are systematically studied. The mixing enthalpy determines whether the solid solution phases or compounds form in the nearly equiatomic multi-component alloy systems.Most importantly the VEC is found to be the physical parameter to control the phase sta-bility for fcc or bcc solid solutions.Fcc phases are found to be stable at higher VEC(!8)and instead bcc phases are sta-ble at lower VEC(<6.87).This work provides valuable input for understanding of the phase stability and to design ductile crystal structures in HEAs. ACKNOWLEDGMENTSThis research was supported by the internal funding from HKPU.1B.Cantor,I.T.H.Chang,P.Knight,and A.J.B.Vincent,Mater.Sci. Eng.A375,213(2004).2W.H.Wu,C.C.Yang,and J.W.Yeh,Ann.Chim.Sci.Mater.31,737(2006). 3J.W.Yeh,Ann.Chim.Sci.Mater.31,633(2006).4C.J.Tong,Y.L.Chen,S.K.Chen,J.W.Yeh,T.T.Shun,C.H.Tsau, S.J.Lin,and S.Y.Chang,Metall.Mater.Trans.36A,881(2005).5C.W.Tsai,M.H.Tsai,J.W.Yeh,and C.C.Yang,J.Alloys Compd.490, 160(2010).6F.J.Wang,Y.Zhang,and G.L.Chen,J.Alloys Compd.478,321(2009). 7G.Y.Ke,S.K.Chen,T.Hsu,and J.W.Yeh,Ann.Chim.Sci.Mater.31, 669(2006).8Y.Zhang,Y.J.Zhou,J.P.Lin,G.L.Chen,and P.K.Liaw,Adv.Eng. Mater.10,534(2008).9R.W.Cahn and P.Hassen,Physical Metallurgy,4th ed.(North Holland, Amsterdam,1996),Vol.1.10S.S.Fang,X.Xiao,X.Lei,W.H.Li,and Y.D.Dong,J.Non-Cryst.Sol-ids321,120(2003).11U.Mizutani,Hume-Rothery Rules for Structurally Complex Alloy Phases (CRC Press,Boca Raton,2011).12T.B.Massalski,Mater.Trans51,583(2010).13X.F.Wang,Y.Zhang,Y.Qiao,and G.L.Chen,Intermetallics15,357 (2007).14M.R.Chen,S.J.Lin,J.W.Yeh,S.K.Chen,Y.S.Huang,and C.P.Tu, Mater.Trans.47,1395(2006).15M.R.Chen,S.J.Lin,J.W.Yeh,S.K.Chen,Y.S.Huang,and M.H. Chuang,Metall.Mater.Trans.37A,1363(2006).16A.Takeuchi and A.Inoue,Mater.Trans.41,1372(2000).17A.Takeuchi and A.Inoue,Mater.Trans.46,2817(2005).18O.N.Senkov and D.B.Miracle,Mater.Res.Bull.36,2183(2001).19WebElements:the periodic table on the web,available at:http://www. /.20C.C.Tung,J.W.Yeh,T.T.Shun,S.K.Chen,Y.S.Huang,and H.C. Chen,Mater.Lett.61,1(2007).21H.Y.Chen,C.W.Tsai,C.C.Tung,J.W.Yeh,T.T.Shun,C.C.Yang, and S.K.Chen,Ann.Chim.Sci.Mater.31,685(2006).22O.N.Senkov,G.B.Wilks,D.B.Miracle,C.P.Chuang,and P.K.Liaw, Intermetallics18,1758(2010).23C.Li,J.C.Li,M.Zhao,L.Zhang,and Q.Jiang,Mater.Sci.Technol.24, 376(2008).24Y.F.Li,L.J.Kong,Z.H.Gan,and Z.X.Yuan,J.Wuhan,Univ.Sci. Technol.32,60(2009).25C.T.Liu,Inter.Met.Rev.29,168(1984).26J.H.Zhu,P.K.Liaw,and C.T.Liu,Mater.Sci.Eng.A239–240,260 (1997).27D.Nguyen-Manh and D.G.Pettifor,Intermetallics7,1095(1999).28L.H.Wen,H.C.Kou,J.S.Li,H.Chang,X.Y.Xue and L.Zhou,Interme-tallics17,266(2009).29C.M.Lin,H.L.Tsai,and H.Y.Bor,Intermetallics18,1244(2010).30C.M.Lin and H.L.Tsai,pd.489,30(2010).31O.N.Senkov,G.B.Wilks,J.M.Scott,and D.B.Miracle,Intermetallics 19,698(2011).。
Enhancement of CO2 Adsorption and CO2_N2 Selectivity on ZIF-8 via Postsynthetic Modi

Enhancement of CO2Adsorption and CO2/N2Selectivity on ZIF-8via Postsynthetic ModificationZhijuan ZhangSchool of Chemistry and Chemical Engineering,South China University of Technology,Guangzhou,510640,P.R.ChinaDept.of Chemistry and Chemical Biology,Rutgers University,Piscataway,New Jersey,08854Shikai Xian,Qibin Xia,Haihui Wang,and Zhong LiSchool of Chemistry and Chemical Engineering,South China University of Technology,Guangzhou,510640,P.R.ChinaJing LiDept.of Chemistry and Chemical Biology,Rutgers University,Piscataway,New Jersey,08854DOI10.1002/aic.13970Published online January11,2013in Wiley Online Library()Imidazolate framework ZIF-8is modified via postsynthetic method using etheylenediamine to improve its adsorption per-formance toward CO2.Results show that the BET surface area of the modified ZIF-8(ED-ZIF-8)increases by39%,and its adsorption capacity of CO2per surface area is almost two times of that on ZIF-8at298K and25bar.H2O uptake on the ED-ZIF-8become obviously lower compared to the ZIF-8.The ED-ZIF-8selectivity for CO2/N2adsorption gets significantly improved,and is up to23and13.9separately at0.1and0.5bar,being almost twice of those of the ZIF-8. The isosteric heat of CO2adsorption(Q st)on the ED-ZIF-8becomes higher,while Q st of N2gets slightly lower com-pared to those on the ZIF-8Furthermore,it suggests that the postsynthetic modification of the ZIF-8not only improves its adsorption capacity of CO2greatly,but also enhances its adsorption selectivity for CO2/N2/H2O significantly. V C2013American Institute of Chemical Engineers AIChE J,59:2195–2206,2013Keywords:ZIF-8,modification,adsorption/gas,isosteric heat of adsorption,selectivityIntroductionCO2has often been cited as the primary anthropogenicgreenhouse gas(GHG)as well as the leading culprit inglobal climate change.The development of a viable carboncapture and sequestration technology(CCS),is therefore,ascientific challenge of the highest order.1–4Currently,a vari-ety of methods,such as membrane separation,chemicalabsorption with solvents,and adsorption with solid adsorb-ents,have been proposed to sequester CO2from thefluegases of power plant.Thereinto,the adsorption is consideredto be one of the most promising technologies for capturingCO2fromflue gases because of their easy control,low oper-ating and capital costs,and superior energy efficiency.5–7Many adsorbents have been investigated for CO2adsorptionincluding activated carbons,zeolites,hydrotalcites and metaloxides.8–14However,although some zeolite materials havebeen claimed to be most adequate for CO2separation fromflue streams,it is difficult to regenerate them without signifi-cant heating which leads to low productivity and greatexpense.15,16Recently,metal-organic frameworks(MOFs)haveattracted great attention and present a promising platform forthe development of next-generation capture materialsbecause of their high capacity for gas adsorption and tunablepore surfaces that can facilitate highly selective binding ofCO2.17–26To optimize a MOF for a particular application,itis important to be able to tailor its pore metrics and function-ality in a straightforward fashion.However,tailoring MOFsmaterials by modifying their textural properties(e.g.,surfacearea and pore volume)and surface chemistry(acid–baseproperties,functional groups)for adsorption application isstill a difficult task.27Many researchers have given insightinto modification of the MOF materials so as to develop newand better adsorbents.Strategies reported include ligandfunctionalization,24,28–39framework interpenetration,22,23introduction of alkali-metal cations,40–42control of poresize32,43–47and incorporation of open metal sites(OMSs).39,48–51However,because of the instability underconditions for the synthesis of MOFs or the competitivereaction with some framework components,it may be diffi-cult for certain functional groups to incorporate into MOFsusing aforementioned strategies.Another strategy for gener-ating desired functionalities in MOFs is the postsynthesis Additional Supporting Information may be found in the online version of thisarticle.Correspondence concerning this article should be addressed to Z.Li atcezhli@.V C2013American Institute of Chemical EngineersAIChE Journal2195June2013Vol.59,No.6modification of preconstructed,robust precursor MOFs.52–55 For example,An et al.33demonstrated that postsynthetic exchange of extra-framework cations within anionic bio-MOF-156can be used as a means to systematically modify its pore dimensions and metrics.Farha et al.57synthesized a series of cavity modified MOFs by replacing coordinated sol-vents with several different pyridine ligands.They found that a p-(CF3)NC5H4-modified MOF showed considerable improvements in the CO2/N2selectivities compared to the parent framework.46Long and his coworkers58previously reported the grafting of ethylenediamine(en)within a water-stable MOF H3[(Cu4Cl)3(BTTri)8](CuBTTri),and found that the en modified sample had more greater attraction of CO2 at low pressures and the CO2/N2selectivity also increased over the entire pressure range measured.More recently, Long and coworkers59incorporated the N,N0-dimethylethyle-nediamine(mmen)into the CuBTTri MOF,and showed that the CO2uptake was drastically enhanced.Zhang et al.60 reported that after ZIF-8was modified by ammonia impreg-nation,the surface basicity was greatly increased and there-fore the CO2uptake was enhanced.Park et al.61reported a postsynthetic reversible incorporation of organic linkers3,6-di(4-pyridyl)-1,2,4,5-tetrazine(bpta)into SNU-30 [Zn2(TCPBDA)(H2O)2]Á30DMFÁ6H2O through single-crystal-to-single-crystal transformations,and found that the desol-vated SNU-310exhibited enhanced selective adsorption of CO2over N2.Xiang et al.62incorporated the CNTs into HKUST-1,and then modified it with Li1.The results showed that the hybrid Li@CNT@[Cu3(btc)2],which is formed by the combination of Li doping and CNT incorpora-tion,having an enhancement of CO2uptakes by about 305%.However,to this date,no work has been reported out on the postsynthetic modification of ZIF-8to enhance its functionality.In this work,the postsynthetic modification of the ZIF-8is proposed to prepare a novel adsorbent with higher CO2 adsorption capacity and CO2/N2selectivity.The postsyn-thetic modification of the ZIF-8crystals would be carried out by using ethylenediamine treatment.Then the surface groups of the modified ZIF-8samples(ED-ZIF-8)would be characterized.Single-component isotherms of CO2and N2 on the modified ZIF-8samples would be measured sepa-rately.Furthermore,the CO2/N2selectivity is estimated by using IAST on the basis of single-component isotherms of CO2and N2.The influence of the textural structures and sur-face chemistry of the original and modified ZIF-8samples on their adsorption capacities for CO2and selectivity of CO2/N2would be discussed and reported here.This informa-tion will be valuable for selecting appropriate adsorbents for CO2capture process.Methods and MaterialsMaterials and instrumentsZinc nitrate hexahydrate(Zn(NO3)2Á6H2O,98%,extra pu-rity)and2-methylimidazole(H A MeIM)(99%purity)were purchased from J&K Chemicals.N,N A Dimethylacetamide (DMF)was purchased from Qiangshen Chemicals Co.,Ltd. of Jiangshu(Jiangshu,China),and it was further purified by 4A molecular sieve to eliminate the water.Maganetic suspension balance RUBOTHERM was sup-plied by Germany.Its precision was0.000001g.ASAP 2010sorptometer was supplied by Micromeritics Co., Norcross,GA,USA.AdsorbentsSynthesis of ZIF-8was performed following the reported procedures63with a few modifications.First,a solid mixture of zinc nitrate hexahydrate Zn(NO3)2Á6H2O(0.956g, 3.2 mmol)and2-methylimidazole(H A MeIM)(0.24g, 3.4 mmol)was dissolved in70mL of DMF solvent.The mixture was quickly transferred to a100mL autoclave and sealed. Second,the autoclave was heated at a rate of5K/min to 413K in a programmable oven and held at this temperature for24h under autogenous pressure by solvothermal synthe-sis,followed by cooling at a rate of0.3K/min to room tem-perature.Third,after removal of mother liquor from the mix-ture,chloroform(40mL)was added to the autoclave.The as-synthesized ZIF-8crystals were then isolated byfiltration. Colorless polyhedral crystals were collected from the upper layer,washed with DMF(10mL33),and dried at383K overnight.To further remove the guest species from the framework and prepare the evacuated form of ZIF-8crystals for modifi-cation and gas-sorption analysis,the as-synthesized ZIF sam-ples were immersed in methanol at ambient temperature for 48h,and evacuated at ambient temperature for5h,and sub-sequently at an elevated temperature673K for2h. Postsynthetic modification of adsorbentsThe as-synthesized ZIF-8crystals(labeled as ZIF-8)were dried at383K for24h for postsynthetic modification.The subsequent treatment applied to the modification of ZIF-8crystals consists of the following steps:The modified ZIF-8sample(labeled as ED-ZIF-8)was synthesized using ethylenediamine as a linker.In a typical procedure,the ZIF-8sample was added to30%ethylenediamine solution and then the mixture was placed in a stainless high-pressure autoclave.The autoclave was heated in an oven at416K for 1h and then381K for6h.The light yellow product wasfil-tered and washed with deionized water.Finally,the sample was dried at383K overnight.Characterization of adsorbentsThe specific surface area and pore volume of original ZIF-8and modified ZIF-8crystals were measured on a Micrometrics gas adsorption analyzer ASAP2010instrument equipped with commercial software for calculation and analysis.Powder X-ray diffraction data were collected using a D8 advance h-2h diffractometer(Bruker)in reflectance Bragg-Brentano geometry employing Cu K a line focused radiation with40kV voltages and40mA current.The X-ray scanning speed was set at2 /min and a step size of0.02 in2h.A Jade5XRD pattern processing software(MDI,Inc.,Liver-more,CA)was used to analyze the XRD data collected on the ZIF-8samples.The surface organic molecules were analyzed by taking FTIR spectra on a Bruker550FTIR instrument equipped with a diffuse reflectance accessory that included a reaction cell.Data acquisition was performed automatically using an interfaced computer and a standard software package.The samples were dried in vacuo at423K prior to mixing with KBr powder.The samples were run in ratio mode allowing for subtraction of a pure KBr baseline.The sample chamber2196DOI10.1002/aic Published on behalf of the AIChE June2013Vol.59,No.6AIChEJournalwas kept purged with nitrogen during the entire experiment.The spectrometer collected 64spectra in the range of 400–4,000cm 21,with a resolution of 4cm 21.CO 2and N 2adsorption measurementsThe CO 2and N 2adsorption-desorption isotherms at 298K,308K,318K,and 328K were obtained on a RUBO-THERM magnetic suspension balance.The initial activa-tion of the modified sample was carried out at 423K for 12h in a vacuum environment.He (ultra-high purity,U-sung)was used as a purge gas in this study.The adsorp-tion processes were carried out using high purity CO 2and N 2(99.999%)gas.A feed flow rate of 60mL/min of CO 2,40mL/min of N 2and 30mL/min of He,respec-tively,were controlled with the mass flow controllers (MFC)to the sample chamber.Both adsorption and de-sorption experiments were conducted at the same tempera-ture.The temperature of the sorption chamber can be adjusted and maintained constant by an internal tempera-ture sensor.However,the pressure can be changed step-wise through the gas flow rate.Typically,there are four steps for finishing determination of an isotherm of CO 2or N 2by using Rubotherm magnetic suspension balance.These detail steps are shown by the operation manual of Rubotherm maganetic suspension balance.H 2O adsorption measurementsThe water adsorption measurements were conducted on a computer-controlled DuPont Model 990TGA.The partial pressure of water was varied by changing the blending ratios of water-saturated nitrogen and pure nitrogen gas streams.Before measurement,the modified ZIF-8samples were acti-vated at 423K for 6h.Results and DiscussionStructure and pore characterizationFigure 1exhibits the adsorption-desorption isotherms of N 2at 77K on the two samples ZIF-8and ED-ZIF-8.It can be seen that both samples show type-I behavior,indicating they are microporous in nature.Table 1lists structure param-eters of the two samples.These data indicate that the BET surface area and micropore volume of the ED-ZIF-8sample are significantly higher than those of the original ZIF-8sam-ple,with an increase of $39%and 35.6%,respectively.Yaghi and his coworkers reported a pore volume of 0.66cm 3/g for ZIF-8from the single crystal structure.For the ZIF-8sample,the total pore volume is calculated to be 0.54cm 3/g,because part of the pores might be blocked.However,after the postsynthetic modification,the blocked pores were reopened,and at meanwhile,some new pores were formed.64,65Thus,the total pore volume of the ED-ZIF-8sample is greatly improved.Figure 2shows the powder X-ray diffraction (PXRD)pat-tern of the modified ZIF-8sample.It can be seen that the main peaks of the modified ZIF-8sample are very clear,and similar to those of the original ZIF-8sample,indicating that the integrity of the modified ZIF-8sample maintains well af-ter the postsynthetic modification.However,for a deep look-ing,it can be found that the major peaks of ED-ZIF-8all shifted to the left side (low-angle area)a little bit,which means after modification,the lattice distance increased.In order to obtain information concerning changes in the surface groups,FTIR experiments were carried out to char-acterize the samples.Figure 3a shows the FTIR spectra of the original ZIF-8and the ED-ZIF-8sample.It is noticed that the spectra for the two samples show high similarities,and the main peaks of both ZIF-8samples match well with the published FTIR spectra for the ZIF-8.However,someTable 1.Porous Structure Parameters of the Modified ZIF-8CrystalsSample BET surface area (m 2.g 21)Langmuir surface area (m 2.g 21)Micropore volume(cm 3.g 21)Total pore volume (cm 3.g 21)Micropore diameter (nm)Mesopore diameter (nm)ZIF-8102513520.450.540.352 4.43ED-ZIF-8142818970.610.750.5444.53Figure 1.N 2adsorption-desorption isotherms of ZIF-8and ED-ZIF-8samples.[Color figure can be viewed in the online issue,which is available at .]Figure2.PXRD patterns of ZIF-8and ED-ZIF-8samples.[Color figure can be viewed in the online issue,which is available at .]AIChE Journal June 2013Vol.59,No.6Published on behalf of the AIChE DOI 10.1002/aic2197differences are also observed.For example,the spectrum of the ED-ZIF-8sample is different from that of the ZIF-8sam-ple in that (1)as shown in Figure 3b there is a new peak at 3381cm 21which is assigned to N A H group appeared on the spectrum of the ED-ZIF-8sample,suggesting some N A H groups have been introduced on the surfaces of the sample ED-ZIF-8,and (2)a peak at 3626cm 21assigned to O A H of the adsorbed H 2O is present in the spectrum of the ZIF-8sample,which is absent in the spectrum of the ED-ZIF-8sample,as shown in Figure 3b.CO 2and N 2adsorption isothermsFor comparison,Figure 4shows the isotherms of CO 2on the ZIF-8and ED-ZIF-8samples.It is visible that the amount adsorbed of CO 2increases as temperature decreases.This suggests that the adsorption of CO 2is mainly physical adsorption.More importantly,it is found that the ED-ZIF-8sample had higher CO 2adsorption capacities compared to the ZIF-8sample,indicating that the adsorption capacities of the modified ZIF-8toward CO 2are greatly improved,nearly being twice as much as the ZIF-8.One of the reasons is that the surface area (BET)of the ED-ZIF-8increases by 39%,as indicated in Table 1.The other reason is that adsorptioncapacity per unit surface area of the ED-ZIF-8for CO 2increases due to an introduction of N A H groups by postsyn-thetic modification.To further understand that,Figure 4a and 4b are separately transferred into Figure 5a and 5b in which the equilibrium uptakes of CO 2based on unit surface area (BET)of the two samples are plotted as a function of CO paring Figure 5b and Figure 5a shows that the CO 2uptake per surface area (BET)of the ED-ZIF-8is obvi-ously higher than that of the ZIF-8,which is mainly ascribed to the introduction of N A H groups,as shown in Figure 3.Figure 6a and 6b show the N 2adsorption isotherms on the two samples.It is visible that the N 2uptakes on the modified ZIF-8samples are slightly higher than that on the ZIF-8due to its larger surface area and pore volume after modification.However,after Figure 6a and 6b are converted into Figure 7a and 7b in which the equilibrium uptakes of N 2based on unit surface area of the two samples are plotted as a function of pressure,it is found from Figure 7that the equilibrium uptakes of N 2per surface area of the ED-ZIF-8are slightly lower than that of the ZIF-8,which means that ED-ZIF-8sample has less affinity toward N 2than ZIF-8sample.This will be helpful to enhance the adsorption selectivity for CO 2/N 2.Figure 3.a.FTIR spectra of the modified ZIF-8crystalsbetween 4,000–400cm 21;b.FTIR spectra of the modified ZIF-8crystals between 4000–2,400cm 21.[Color figure can be viewed in the online issue,which is available at .]Figure 4.a.Isotherms of CO 2on the ZIF-8sample withdifferent temperatures; b.isotherms of CO 2on the ED-ZIF-8sample with different temperatures.[Color figure can be viewed in the online issue,which is available at .]2198DOI 10.1002/aicPublished on behalf of the AIChE June 2013Vol.59,No.6AIChEJournalMultiple cycles of CO 2adsorption-desorption on the ED-ZIF-8To evaluate the regeneration performance of the modified sample or the reversibility of CO 2adsorption on the modi-fied sample,the experiments of multiple cycles of CO 2adsorption-desorption on the ED-ZIF-8were performed in the Rubotherm system at 298K.For adsorption process,the adsorption pressure were targeted for 25bar;while for de-sorption process the system pressure was targeted for 1mbar,and then the desorption system was quickly depressur-ized by using vacuum pumping.Figure 8shows the variation curve of the amounts adsorbed of CO 2on the ED-ZIF-8dur-ing four consecutive cycles of CO 2adsorption-desorption experiments at 298K.It was visible clearly that during the desorption,the amounts adsorbed of CO 2on the ED-ZIF-8sample decreased sharply with time,and then reached a very low content,about 2.21wt %of residual CO 2which was present on the sample after desorption at 1mbar.The effi-ciency of CO 2desorption was nearly up to 98%over the entire four circles.It indicated further that CO 2adsorption was reversible with very little accumulation of irreversible bound CO 2on the ED-ZIF-8framework.In addition,it wasalso observed from Figure 8that the curves representing the cycles of CO 2adsorption-desorption experiments were very similar,suggesting that adsorption and desorption properties of the sample ED-ZIF-8for CO 2were stable or repeatable.It also proved that the pressure swing was effective in strip-ping adsorbed CO 2from the ED-ZIF-8.H 2O adsorption isothermsFigure 9shows the water isotherms on the modified ZIF-8samples at 298K.The water uptake on the ED-ZIF-8sample is less than that on the ZIF-8sample,indicating that the sur-face of the modified sample became more hydrophobic com-pared to the ZIF-8sample.It also means that the interaction of the water molecule with the modified sample became weaker as compared to that with the ZIF-8.Ideal adsorbed solution theory (IAST)selectivity of CO 2/N 2The ideal adsorbed solution theory (IAST)developed by Myers and Praunitz 66provides an effective method to predict the adsorption selectivity and the adsorption equilibrium of gas mixtures from the isotherms of the pure components.Figure 5.a.Isotherms of CO 2on the ZIF-8samplebased on unit surface area;b.isotherms of CO 2on the ED-ZIF-8sample based on unit surface area.[Color figure can be viewed in the online issue,which is available at .]Figure 6.a.Isotherms of N 2on the ZIF-8sample atdifferent temperatures;b.isotherms of N 2on the ED-ZIF-8sample at different temperatures.[Color figure can be viewed in the online issue,which is available at .]AIChE JournalJune 2013Vol.59,No.6Published on behalf of the AIChE DOI 10.1002/aic2199Previous work reported that the IAST can accurately predict gas mixture adsorption in a number of zeolites and MOF materials.10,48,67–70The IAST assumes that the adsorbed mixture is an ideal solution at constant spreading pressure and temperature,where all the components in the mixture conform to the rule analogous to Raoult’s law,and the chemical potential of the adsorbed solution is considered equal to that of the gas phase at equilibrium.From the IAST,the spreading pressure p is given byp 0i ðp 0i Þ5RT A ðp 0iqd ln p (1)p Ã5p A 5ðp 0i 0q i dp (2)Where A is the specific surface area of the adsorbent,p andp *are the spreading pressure and the reduced spreading pres-sure,separately.p 0i is the gas pressure of component i corre-sponding to the spreading pressure p of the gas mixture.At a constant temperature,the spreading pressure of single component is the samep Ã15p Ã25…5p Ãn 5p(3)For binary adsorption of component 1and 2,the IASTrequiresy 1p t 5x 1p 1ð12y 1Þp t 5ð12x 1Þp 2(4)Where y 1and x 1denote the molar fractions of component 1in the gas phase and in the adsorbed phase,respectively.p t is the total gas pressure,p 1and p 2are the pressures of com-ponent 1and 2at the same spreading pressure as that of the mixture,respectively.Adsorption selectivity in a binary mixture of component 1and 2is defined asS 125x 1x 2 y 2y 1 (5)For the application of IAST to predict adsorption separa-tion selectivity,the following two conditions are necessary:good quality adsorption data of each single component;and excellent curve fitting model for such data.48,71,72In order to perform the integrations of Eqs.(1)and (2)required by IAST,the single-component isotherms should be fitted by a proper isotherm model.In practice,several meth-ods are available.In this work,it is found that the dual-site Langmuir-Freundlich (DSLF)equation can be successful to fit this set of adsorption data.The dual-site Langmuir-Freundlich model can be expressed as followsq 5q m ;13b 1p 1=n 111b 1p 11q m ;23b 2p 1=n 211b 2p 2(6)Where p is the pressure of the bulk gas at equilibrium with the adsorbed phase (kPa),q m,1,q m,2are the saturation capaci-ties of sites 1and 2(mmol/g),b 1and b 2are the affinity coefficients of sites 1and 2(1/kPa),and n 1and n 2are the deviations from an ideal homogeneous surface.Figure 10shows a comparison of the model fits and the isotherm data.It is visible that the DSLF model can be applied favorably for fitting experimental data of CO 2and N 2adsorption.Table 2presents the fitting parameters ofFigure 7.a.Isotherms of N 2on the ZIF-8sample basedon unit surface area;b.isotherms of N 2on the ED-ZIF-8sample based on unit surface area.[Color figure can be viewed in the online issue,which is available at .]Figure 8.Recycle runs of CO 2adsorption-desorptionon the ED-ZIF-8at 298K and 25bar for adsorption and 1mbar for desorption.[Color figure can be viewed in the online issue,which is available at .]2200DOI 10.1002/aicPublished on behalf of the AIChE June 2013Vol.59,No.6AIChEJournalDSLF equation as well as the correlation coefficients (R 2).Examination of the data shows that this DSLF model is able to fit the adsorption data well since the correlation coeffi-cients R 2are up to 0.9997.In this work,the equilibrium adsorption data of single component CO 2as well as N 2are available,and the DSLF model can fit the experimental isotherms of CO 2and N 2adsorption very well.Therefore,the DSLF model can be combined with the ideal adsorbed solution theory (IAST)to predict the mixture adsorption isotherms and calculate the selectivities of the two samples for CO 2/N 2adsorption.Figure 11a and 11b present,respectively,the adsorption isotherms predicted by IAST for equimolar mixtures of CO 2/N 2in the samples ZIF-8and ED-ZIF-8as a function of total bulk pressure.It can be seen that CO 2is preferentially adsorbed over N 2on the two samples because of stronger interactions between CO 2and the ZIF-8sample,and the amount adsorbed of N 2is much lower in the mixtures than that in single-component adsorption because of competition adsorption from CO 2,which adsorbs more strongly.Figure 12shows the IAST-predicted selectivities of the two samples for equimolar CO 2and N 2mixtures at 298K as a function of total bulk pressure.It can be seen that the adsorption selectivity of the two samples for CO 2/N 2dropped with an increase in the pressure.More importantly,Figure 9.H 2O adsorption isotherms on the modifiedZIF-8samples at 298K.[Color figure can be viewed in the online issue,which is available at .]Table 2.The Fitting Parameters of the Dual-site Langmuir-Freundlich Equations for the Pure Isotherms of CO 2and N 2at 298KZIF-8ED-ZIF-8CO 2N 2CO 2N 2R 20.99970.99990.99970.9999q m,1(mmol/g)27.2527.8748.8828.32q m,2(mmol/g) 2.122 1.919 4.672 1.847b 1(atm 21)0.015330.0011700.012590.001388b 2(atm 21)0.0068950.026090.029480.02504n 1 1.6000.7875 1.4040.7704n 20.32440.96340.44300.8671Figure 10.DSLF fitting of the CO 2and N 2isotherms onZIF-8and ED-ZIF-8at 298K.[Color figure can be viewed in the online issue,which is available at .]Figure11.a.The IAST -predicted isotherm forequimolar CO 2/N 2mixtures of the ZIF-8sample at 298K as a function of total bulk pressure; b.the IAST -predicted isotherm for equimolar CO 2/N 2mixtures of the ED-ZIF-8sample at 298K as a function of total bulk pressure.[Color figure can be viewed in the online issue,which is available at .]AIChE Journal June 2013Vol.59,No.6Published on behalf of the AIChE DOI 10.1002/aic2201the adsorption selectivity of CO 2/N 2on the sample ED-ZIF-8is always higher than that on the sample ZIF-8,especially in the low-pressure region.For example,at 0.1and 0.5bar,the selectivity of the sample ED-ZIF-8for CO 2/N 2were up to 23and 13.9separately,which is almost twice of those of the sample ZIF-8.Figure 13a and 13b show,respectively,the IAST-pre-dicted selectivities of the samples ZIF-8and ED-ZIF-8for CO 2/N 2at different mixture compositions and different pres-sures.It is noticed that the selectivity increases rapidly as the gas-phase mole fraction of N 2approaches unity.For example,at yN 250.9,a typical feed composition of flue gas,high selectivities are obtained.Even at yN 250.5,the selectivity of the ED-ZIF-8for CO 2/N 2is in the range of 6–24,much higher than those on the ZIF-8sample and many other MOF samples such as ZIF-7030,ZIF-6830and MOF-508b.73This property is very important since some separa-tion processes could be operated at low pressures,such as vacuum swing adsorption (VSA),which could be extremely efficient by using the sample ED-ZIF-8because its selectiv-ity increases dramatically with decreasing pressure.Ideal adsorbed solution theory (IAST)selectivity of CO 2/N 2/H 2OThe major challenge of CO 2capture from power plant flue gas wastes is the separation of CO 2/N 2.In addition,competition adsorption of water molecule must be taken into account,because these flue gas wastes are usually saturated with certain amount of water (5–7%by volume)for the industrial postcombustion processes.Thus,for real industrial use of adsorbents,the effect of water on CO 2/N 2selectivity is another crucial factor that needs to be considered and evaluated.Here,the IAST was adopted to evaluate the ter-nary mixture CO 2/N 2/H 2O adsorption on the modified ZIF-8samples.First,the experimental isotherms of water on the modified ZIF-8samples at 298K were fitted using the DSLF model.Table 3presents the fitting parameters of DSLF equation as well as the correlation coefficients.It can be seen that theDSLF model fits the H 2O adsorption on both samples very well.Second,the DSLF model was combined with the ideal adsorbed solution theory (IAST)to predict the mixture adsorption isotherms,and then calculate the selectivities of the two samples for CO 2/N 2adsorption.Figure 14shows the predicted isotherms of ternary mix-ture CO 2/N 2/H 2O on the modified ZIF-8samples at 298K.It can be observed that in comparison with the ZIF-8,after modification,the CO 2adsorption capacity of the ED-ZIF-8in the ternary mixture obviously increased,and its N 2adsorption capacity somewhat increased,which made CO 2/N 2adsorption selectivity of the ED-ZIF-8increase.More importantly,its water adsorption capacity in the ternary mix-ture became lower compared to the ZIF-8,and it was also lower than the single component water uptake.It means the competition adsorption of H 2O in the ternary mixture was weakened on the surfaces of the ED-ZIF-8sample.Figure 12.The IAST -predicted selectivity for equimolarCO 2and N 2at 298K as a function of total bulk pressure.[Color figure can be viewed in the online issue,which is available at .]Figure 13.a.The IAST predicted selectivities atdifferent mixture compositions and different pressures for the ZIF-8sample at 298K;b.the IAST predicted selectivities at different mixture compositions and different pressures for the ED-ZIF-8sample at 298K.[Color figure can be viewed in the online issue,which is available at .]2202DOI 10.1002/aicPublished on behalf of the AIChE June 2013Vol.59,No.6AIChEJournal。
离子掺杂对提高锐钛型TiO2高热稳定性的研究
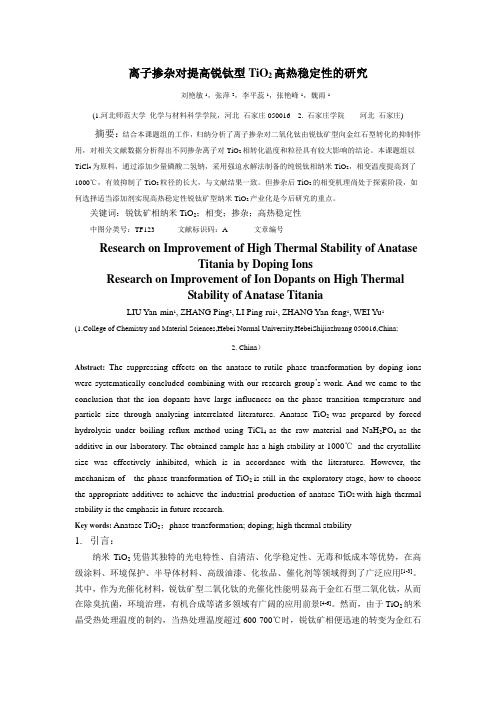
离子掺杂对提高锐钛型TiO2高热稳定性的研究刘艳敏1,张萍2,李平蕊1,张艳峰1,魏雨1(1.河北师范大学化学与材料科学学院,河北石家庄050016 2. 石家庄学院河北石家庄)摘要:结合本课题组的工作,归纳分析了离子掺杂对二氧化钛由锐钛矿型向金红石型转化的抑制作用,对相关文献数据分析得出不同掺杂离子对TiO2相转化温度和粒径具有较大影响的结论。
本课题组以TiCl4为原料,通过添加少量磷酸二氢钠,采用强迫水解法制备的纯锐钛相纳米TiO2,相变温度提高到了1000℃,有效抑制了TiO2粒径的长大,与文献结果一致。
但掺杂后TiO2的相变机理尚处于探索阶段,如何选择适当添加剂实现高热稳定性锐钛矿型纳米TiO2产业化是今后研究的重点。
关键词:锐钛矿相纳米TiO2;相变;掺杂;高热稳定性中图分类号:TF123 文献标识码:A 文章编号Research on Improvement of High Thermal Stability of AnataseTitania by Doping IonsResearch on Improvement of Ion Dopants on High ThermalStability of Anatase TitaniaLIU Yan-min1, ZHANG Ping2, LI Ping-rui1, ZHANG Yan-feng1, WEI Yu1(1.College of Chemistry and Material Sciences,Hebei Normal University,HebeiShijiazhuang 050016,China;2. China)Abstract:The suppressing effects on the anatase-to-rutile phase transformation by doping ions were systematically concluded combining with our research group’s work. And we came to the conclusion that the ion dopants have large influences on the phase transition temperature and particle size through analysing interrelated literatures. Anatase TiO2 was prepared by forced hydrolysis under boiling reflux method using TiCl4 as the raw material and NaH2PO4 as the additive in our laboratory. The obtained sample has a high stability at 1000℃and the crystallite size was effectively inhibited, which is in accordance with the literatures. However, the mechanism of the phase transformation of TiO2 is still in the exploratory stage, how to choose the appropriate additives to achieve the industrial production of anatase TiO2 with high thermal stability is the emphasis in future research.Key words:Anatase TiO2;phase transformation; doping; high thermal stability1.引言:纳米TiO2凭借其独特的光电特性、自清洁、化学稳定性、无毒和低成本等优势,在高级涂料、环境保护、半导体材料、高级油漆、化妆品、催化剂等领域得到了广泛应用[1-3]。
- 1、下载文档前请自行甄别文档内容的完整性,平台不提供额外的编辑、内容补充、找答案等附加服务。
- 2、"仅部分预览"的文档,不可在线预览部分如存在完整性等问题,可反馈申请退款(可完整预览的文档不适用该条件!)。
- 3、如文档侵犯您的权益,请联系客服反馈,我们会尽快为您处理(人工客服工作时间:9:00-18:30)。
Stability enhancement of ZnTPPS in acidic aqueous solutions by polymeric micelles wXin Wang,Lizhi Zhao,Rujiang Ma,Yingli An and Linqi Shi*Received 1st June 2010,Accepted 19th July 2010DOI:10.1039/c0cc01674hPoly(ethyleneglycol)-b -poly(4-vinylpyridine)(PEG-b -P4VP)and zinc meso -5,10,15,20-tetrakis-(4-sulfonatophenyl)porphyrin (ZnTPPS)form complex micelles based on electrostatic inter-actions.The complex micelles have a core-shell structure that can effectively prevent the demetallization and aggregation of ZnTPPS in acidic aqueous solutions (pH o 4.0).Metalloporphyrins have received much attention because of their important role as catalysts 1–4and sensitizers 5in a wide variety of biochemical and photochemical reactions.For example,Suda et al.reported that a high-valent metallo-porphyrin complex exhibited exceptional stability and efficiency for the control of regio-and stereochemistry of the rearrangement of epoxides to aldehydes.1Davis et al.studied the self-assembly of zinc metalloporphyrins on gold nanoparticles,which produced a novel anion-selective optical sensing system.2However,ZnTPPS,6MgTPPS,7CdTPPS,8etc.,are apt to be demetallized in acidic solutions due to hydrolysis.Besides,ZnTPPS is apt to form aggregates at a high concentration,which leads to a fluorescence self-quenching effect of the excited state.9For these reasons,application of metalloporphyrins as photosensitizers and photocatalysts is significantly limited.Therefore,it is desirable to enhance the stability of metallo-porphyrins against demetallization and aggregation.It is well-known that self-assembled block copolymer micelles can encapsulate substances in their core,which can effectively protect the encapsulated substances against invasion by molecules and ions in the solution.10,11Here we report a novel and effective method to enhance the stability of ZnTPPS in acidic aqueous solutions.Block copolymer PEG-b -P4VP and ZnTPPS form complex micelles in acidic solutions through electrostatic interaction between positively charged pyridyls on polymer and negatively charged sulfonte groups on ZnTPPS.The microenvironment provided by the micelles effectively reduces the chance of H +replacing Zn 2+,and thus prevents demetallization of the encapsulated ZnTPPS.Furthermore,fluorescence quenching is restrained to a certain extent due to segregation of ZnTPPS in the micelle core.ZnTPPS was synthesized according to a previously reported method.12The stock ZnTPPS solution was prepared by dis-solving ZnTPPS in neutral Milli-Q water and stored in the dark.The concentration of the solution was determinedspectrophotometrically using e 421=6.8Â105M À1cm À1at the Soret maximum.13In neutral aqueous solution,the absorption spectrum of ZnTPPS exhibits an intense Soret band at 421nm and two weak Q bands at 555and 595nm,13and their intensities keep constant against time.However,ZnTPPS is apt to be demetallized in aqueous solution when pH o 4.0.6Fig.1shows the evolution of UV-Vis absorptions of ZnTPPS solutions at pH 2.0with time.The intensities of the typical Soret band (421nm)and Q bands (555and 595nm)of ZnTPPS decrease progressively and the characteristic absorp-tions of the diacid form of TPPS (H 4TPPS 2À)at 434and 644nm 14can be observed immediately after the pH value of the ZnTPPS solution is changed to 2.0,indicating the fast hydrolysis of ZnTPPS.The demetallization of ZnTPPS is almost completed in 30min.This disadvantage limits the use of ZnTPPS in many aspects.The inset in Fig.1shows the changes of the fluorescence spectra of ZnTPPS (l ext =410nm)with time in aqueous solutions at pH 2.0.The bands at 607nm and 659nm are typical emissions of ZnTPPS in aqueous solution.9A new band appears at about 680nm,14which is attributed to the emission of H 4TPPS 2À,and its intensity increases with time at the expense of those at 607and 659nm.This further proves the demetallization of ZnTPPS at pH 2.0.The block copolymer PEG-b -P4VP was synthesized by atom transfer radical polymerization (ATRP),15and its composition was characterized through 1H NMR spectrum in CDCl 3using the PEG block as the inner standard (ESI,Fig.S1w ).The polydispersity index (M w /M n )of the block copolymer was found to be 1.23by gelpermeationFig.1Kinetic UV-Vis absorption spectra of ZnTPPS at various times in aqueous solutions at room temperature,where the concentra-tion of ZnTPPS was 8.0m mol L À1.The spectra were recorded at 0,10,20,and 30min after adjusting the pH from 7.0to 2.0.The inset shows the corresponding changes in the fluorescence emission spectra of ZnTPPS (l ext =410nm).Key Laboratory of Functional Polymer Materials,Ministry of Education,Institute of Polymer Chemistry,College of Chemistry,Nankai University,Tianjin,300071,P.R.China.E-mail:shilinqi@;Fax:0086-22-23503510;Tel:0086-22-23506103w Electronic supplementary information (ESI)available:Experimental details and characterisation of materials.See DOI:10.1039/c0cc01674hD o w n l o a d e d b y N a n k a i U n i v e r s i t y o n 08 O c t o b e r 2010P u b l i s h e d o n 16 A u g u s t 2010 o n h t t p ://p u b s .r s c .o r g | d o i :10.1039/C 0C C 01674Hchromatography (GPC)using N ,N -dimethylformamide (DMF)as the eluent and narrowly distributed polystyrene as the calibration standard (ESI,Fig.S2).Complex micelliza-tion was carried out by adding the stock ZnTPPS solution dropwise into PEG-b -P4VP acid solutions with different concentrations under stirring.The obtained complex solu-tions at pH 2.0had the same concentration of 20m M for ZnTPPS and different molar ratios of 4VP units to ZnTPPS (R =4VP/ZnTPPS).Dynamic light scattering (DLS)analysis indicates that the complex micelles with R =10(Fig.2a)have a mean hydro-dynamic diameter of 105nm,larger than that of micelles of PEG-b -P4VP without ZnTPPS at pH 9.0(ESI,Fig.S3w ).The transmission electron microscopy (TEM)image (Fig.2b)shows spherical particles’distribution with diameters ranging from 70to 150nm.The size (DLS)of the complex micelles is independent of the variation of the 4VP/ZnTPPS ratios such as R =30,60,and 100.The complex micelles are stable against aggregation as indicated by the constant diameter observed over three weeks.The micelle solutions were prepared one day in advance for optical experiments to ensure complete interaction between ZnTPPS and the copolymer.In Fig.3a,however,the UV-Vis spectra of ZnTPPS in the presence of PEG-b -P4VP with R =100,60,30,10do not show the absorptions at about 493and 705nm,indicating that the copolymer micelles can effectively protect ZnTPPS from demetallization.Demetalli-zation of ZnTPPS can produce H 4TPPS 2Àwhich tends to form J -aggregates in the micelle core at pH 2.0with the characteristic UV-Vis absorptions at about 493and 705nm.14,16The intensities of UV-Vis absorption at 428nm,which is ascribed to the ZnTPPS monomer,decrease with the decline of polymer concentration,indicating partial aggregation of ZnTPPS.9Because a lower polymer concentration (lower R value)results in a higher local concentration in the micelle core for ZnTPPS it is apt to form aggregates.The lower the concentration of the copolymer,the more ZnTPPS aggregates form.As a result,the absorption of ZnTPPS monomer at 428nm decreases with the decreasing polymer concentration.The fluorescence emission intensities of ZnTPPS also decrease with decreasing R value as shown in Fig.3b,which is caused by increased fluorescence quenching due to the aggregation of ZnTPPS.9However,the UV-Vis absorptions at 493and 705nm appear at R =4,which indicates that ZnTPPS is partly demetallized and the resultant H 4TPPS 2Àforms J -aggregates.The greatly reduced intensities of UV-Vis absorption at about 428nm inFig.3a and fluorescence emissions in Fig.3b may be causedboth by partial demetallization and a higher degree of aggregation of ZnTPPS at R =4.It is worth noting that the UV-Vis absorption at about 428nm in Fig.3a becomes broader at R =4,which may be due to the contribution of the absorption of H 4TPPS 2Àat 434nm.14Fig.4a records the changes of UV-Vis absorption spectra of ZnTPPS with time in the presence of different amounts of copolymer.At R =4,stronger absorptions at 434and 493nm indicate the presence of H 4TPPS 2Àin the solution at different times,which is due to apparent demetallization of ZnTPPS when the concentration of polymer is very low.At R =10and 30,a slight decrease of the absorption at 428nm and a very weak absorption at 493nm suggest only a very small fraction of ZnTPPS is demetallized in 24days.In addition,the varia-tions of absorption intensities at 493nm demonstrate that the degree of demetallization of ZnTPPS is apparently different for systems with different R values.Thus,the change in absorption intensities at 493nm with time (Fig.4b)presents a kinetic profile for the demetallization of ZnTPPS in the presence of the polymer.For R =4,the absorptions at 493nm increase in the first 7days followed by a decrease later.This is because ZnTPPS is initially demetallized,which leads to an increase of J -aggregates of H 4TPPS 2Àin the solution,and then precipitation takes place after 7days as a result of large J -aggregates,which eventually results in a decrease of the absorption intensity.By contrast,the absorption intensity at 493nm increases slowly in 24days for R =10and 30,indicating that the polymer shows the enhancement effects for the stability of ZnTPPS.Besides,a higher concentration of the polymer displays more effective protective effects.At R =30,the increase of the absorption intensity at 493nm is slower than that of R =10in 24days,which implies that the higher the polymer concentration,the higher the stability of ZnTPPS against demetallization.Furthermore,the absorption at 493nm almost cannot be detected in 24days when R >30(R =60and 100).As discussed above,demetallization of ZnTPPS is almost completed in 30min at pH 2.0in the absence of polymer.The presence of PEG-b -P4VP defers or even avoids this phenomenon by adjusting the ratio of 4VP/ZnTPPS.The protecting effect of polymeric micelles on ZnTPPS is illustrated in Scheme 1and can be explained as follows.In acidic aqueous solution,ZnTPPS is easy to demetallize because of the replacement of Zn 2+by H +.TheresultantFig.2DLS profile (a)and TEM micrograph (b)of the complex micelles at pH 2.0,where R =10.The DLS measurements were performed at the scattering angle of 901at roomtemperature.Fig.3UV-Vis absorption spectra (a)and fluorescence emission spectra (l ext =410nm)(b)of ZnTPPS in complex micelles with different R (R =100,60,30,10,and 4)at pH 2.0and at room temperature.The concentration of ZnTPPS was 20m mol L À1in the micelle solutions.D o w n l o a d e d b y N a n k a i U n i v e r s i t y o n 08 O c t o b e r 2010P u b l i s h e d o n 16 A u g u s t 2010 o n h t t p ://p u b s .r s c .o r g | d o i :10.1039/C 0C C 01674Hdiacid TPPS (H 4TPPS 2À)is apt to form J -aggregates at pH 2.0as mentioned above.In the presence of PEG-b -P4VP,however,the electrostatic interaction between the cationic P4VP block and the anionic ZnTPPS that bears four negatively charged sulfonate groups leads to the formation of the com-plex micelles consisting of a complex P4VP/ZnTPPS core and a PEG shell.As a result,ZnTPPS is encapsulated in the micelle core which can protect ZnTPPS from the attack of protons to some extent,depending on the molar ratio of 4VP/ZnTPPS (R ).Variation of R values may result in different micelle micro-structures which have different effects on protecting ZnTPPS.At R =4,the quantity of 4VP is equal to that of sulfonte groups and,in principle,a neutral micelle core is formed.It is easy for protons to be close to and even diffuse into the micelle core,which leads to demetallization of ZnTPPS.When R >4,protonated 4VP units are excessive,and a positively charged surface of micelle core is expected.It is relatively difficult for protons to approach the encapsulated ZnTPPS due to the electrostatic repulsion between protons and the surface of the micelle core.Consequently,demetallization of ZnTPPS is restrained.The higher the R value,the more difficult it is for protons to approach the encapsulated ZnTPPS,and as a result,the more effective the protection of ZnTPPS against demetallization will be.When R >30,it is almost impossible for protons to diffuse into the micelle core and demetallization of ZnTPPS is not observed.It is worth noting that encapsulation of ZnTPPS in the complex micelle core can also restrain fluorescence self-quenching which is due to aggregation of ZnTPPS.It is considered that ZnTPPS is segregated in the micelle core and aggregation of ZnTPPS is restricted by the cross-linked network.At R =4,however,the local concentration of ZnTPPS in the micelle core is relatively high,which provides more opportunities for ZnTPPS to come into contact with each other and then to form aggregates.Increasing the R value leads to a decrease in the local concentration of ZnTPPS in the micelle core and consequently results in the reduced possibility of ZnTPPS aggregation.In conclusion,we have demonstrated that novel polymeric micelles formed by ZnTPPS and block copolymer PEG-b -P4VP can effectively protect ZnTPPS against demetallization in acidic aqueous media due to the microenvironment provided by micelles.Meanwhile,aggregation of ZnTPPS is also restricted with increasing copolymer concentration.More potential appli-cations of ZnTPPS with enhanced stability in acid aqueous media may be found in photocatalysis,photoredox systems,photochemical synthesis,solar cells and photodynamic therapy.This work was supported by the National Natural Science Foundation of China (No.50830103,20774051,50625310,and 20904025).Notes and references1K.Suda,T.Kikkawa,S.Nakajima and T.Takanami,J.Am.Chem.Soc.,2004,126,9554.2X.Q.Yu,J.S.Huang,W.Y.Yu and C.M.Che,J.Am.Chem.Soc.,2000,122,5337.3I.Tabushi,S.Kugimiya and T.Mizutani,J.Am.Chem.Soc.,1983,105,1658.4C.M.Che and J.S.Huang,mun.,2009,3996.5P.D.Beer,D.P.Cormode and J.J.Davis,mun.,2004,414.6A.Farajtabar,F.Gharib,P.Jamaat and N.Safari,J.Chem.Eng.Data ,2008,53,350.7X.Y.Zhang,K.Sasaki and Y.Kuroda,Bull.Chem.Soc.Jpn.,2007,80,536.8F.Gharib,F.Soleimani,A.Farajtabar and H.Aghaei,J.Chem.Eng.Data ,2009,54,2060.9S.C.Gandini,V.E.Yushmanov and T.Tabak,J.Inorg.Biochem.,2001,85,263.10M.Yokoyama,T.Okano,Y.Sakurai,S.Suwa and K.Kataoka,J.Controlled Release ,1996,39,351.11C.Allen,D.Maysinger and A.Eisenberg,Colloids Surf.,B ,1999,16,3.12Y.Saiki and Y.Amao,Biotechnol.Bioeng.,2003,82,710.13R.Purrello,E.Bellacchio,S.Gurrieri,uceri,A.Raudino,L.M.Scolaro and A.M.Santoro,J.Phys.Chem.B ,1998,102,8852.14N.C.Maiti,S.Mazumdar and N.Periasamy,J.Phys.Chem.B ,1998,102,1528.15K.Wu,L.Q.Shi,W.Q.Zhang,Y.L.An,X.Zhang,Z.Y.Li and X.X.Zhu,Langmuir ,2006,22,1474.16L.Z.Zhao,R.J.Ma,J.B.Li,Y.Li,Y.L.An and L.Q.Shi,Biomacromolecules ,2008,9,2601.Fig.4(a)Changes in UV-Vis absorption spectra of ZnTPPS in complex micelles (R =30,10,and 4)with time (A =0day,B =7days,and C =24days).(b)The kinetic profiles for the demetallization of ZnTPPS in the presence of polymer with different R values denoted by the absorption intensities of J -aggregates (493nm).The concentra-tions of ZnTPPS were 20m mol L À1in the micellesolutions.Scheme 1Illustration of the demetallization of ZnTPPS in the absence of polymer (a)and protection of ZnTPPS against demetalliza-tion and aggregation by the complex micelles (b).D o w n l o a d e d b y N a n k a i U n i v e r s i t y o n 08 O c t o b e r 2010P u b l i s h e d o n 16 A u g u s t 2010 o n h t t p ://p u b s .r s c .o r g | d o i :10.1039/C 0C C 01674H。