Eigenvalue bounds in the gaps of Schrodinger operators and Jacobi matrices
inequalities for graph eigenvalues
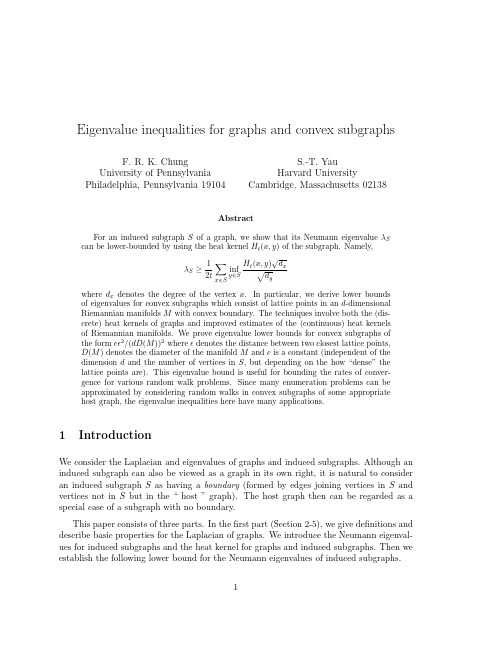
1
Introduction
We consider the Laplacian and eigenvalues of graphs and induced subgraphs. Although an induceas a graph in its own right, it is natural to consider an induced subgraph S as having a boundary (formed by edges joining vertices in S and vertices not in S but in the “ host ” graph). The host graph then can be regarded as a special case of a subgraph with no boundary. This paper consists of three parts. In the first part (Section 2-5), we give definitions and describe basic properties for the Laplacian of graphs. We introduce the Neumann eigenvalues for induced subgraphs and the heat kernel for graphs and induced subgraphs. Then we establish the following lower bound for the Neumann eigenvalues of induced subgraphs. 1
Abstract For an induced subgraph S of a graph, we show that its Neumann eigenvalue λS can be lower-bounded by using the heat kernel Ht (x, y ) of the subgraph. Namely, √ 1 Ht (x, y ) dx λS ≥ inf y ∈S 2t dy x ∈S where dx denotes the degree of the vertex x. In particular, we derive lower bounds of eigenvalues for convex subgraphs which consist of lattice points in an d-dimensional Riemannian manifolds M with convex boundary. The techniques involve both the (discrete) heat kernels of graphs and improved estimates of the (continuous) heat kernels of Riemannian manifolds. We prove eigenvalue lower bounds for convex subgraphs of the form cǫ2 /(dD(M ))2 where ǫ denotes the distance between two closest lattice points, D(M ) denotes the diameter of the manifold M and c is a constant (independent of the dimension d and the number of vertices in S , but depending on the how “dense” the lattice points are). This eigenvalue bound is useful for bounding the rates of convergence for various random walk problems. Since many enumeration problems can be approximated by considering random walks in convex subgraphs of some appropriate host graph, the eigenvalue inequalities here have many applications.
氢键的应用

how the standard perception of halogen substituents, which assumes an isotropic negative electron density around the halogen, was replaced by a description that takes the σ-hole into account. Halogen bonds have been found to occur in a multitude of inorganic, organic, and biological systems.4,5 In an early study from the 1950s, Hassel and Hvoslef solved the crystal structure of the equimolar Br2:dioxane adduct and found Br···O contacts featuring distances substantially below the sum of the van der Waals radii of both atoms, indicating a strong attractive interaction between both atoms.6,7 In 1984, a search of the Cambridge crystallographic data files for short iodine···N/O/S contacts revealed that these interactions are also formed in biologically relevant systems, being employed by nature for the molecular recognition of thyroid hormones at their target proteins such as transthyretin.8 In protein−ligand environments, halogen bonds can be formed between a halogenated ligand and any accessible Lewis base in the binding pocket.9 Probably because of its presence in every amino acid, the backbone carbonyl oxygen function is the most prominent Lewis base involved in halogen bonds in protein binding sites, as found from an analysis of the Protein Data Bank (PDB).10,11 Additionally, halogen bonds can be formed involving side chain groups, such as hydroxyls in serine, threonine, and tyrosine, carboxylate groups in aspartate and glutamate, sulfurs in cysteine and methionine, nitrogens in histidine, and the π surfaces of phenylalanine, tyrosine, histidine, and tryptophan. Several examples for these contacts are given in Figure 2.
energyS
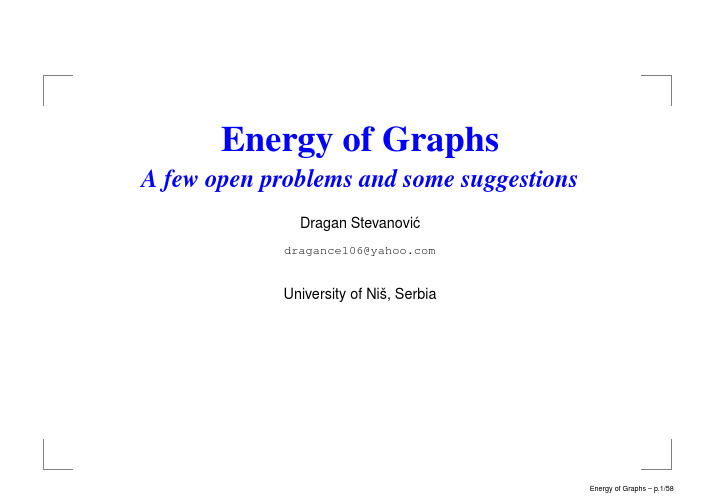
Interlacing: if H = G − u has eigenvalues µ1 ≥ µ2 ≥ . . . ≥ µn−1 , then
λ1 ≥ µ1 ≥ λ2 ≥ µ2 ≥ . . . ≥ λn−1 ≥ µn−1 ≥ λn .
Energy of Graphs – p.13/58
Trees are Ok! (3)
For example, the following special problems have been solved in this way: max and min E. for trees with perfect matchings; min E. for trees with a given maximum matching size; max E. for trees with n vertices and max degree ∆; min E. for trees with n vertices and max deg ∆ ≥ ⌈ n+1 3 ⌉; min E. for trees with n vertices and k pendant vertices; min E. for unicyclic graphs; max E. for bipartite unicyclic graphs; max and min E. for hexagonal chains; min E. for chains with polygons of 4n − 2 vertices.
The Coulson integral formula (1940):
1 E (G ) = π
微积分介值定理的英文

微积分介值定理的英文The Intermediate Value Theorem in CalculusCalculus, a branch of mathematics that has revolutionized the way we understand the world around us, is a vast and intricate subject that encompasses numerous theorems and principles. One such fundamental theorem is the Intermediate Value Theorem, which plays a crucial role in understanding the behavior of continuous functions.The Intermediate Value Theorem, also known as the Bolzano Theorem, states that if a continuous function takes on two different values, then it must also take on all values in between those two values. In other words, if a function is continuous on a closed interval and takes on two different values at the endpoints of that interval, then it must also take on every value in between those two endpoint values.To understand this theorem more clearly, let's consider a simple example. Imagine a function f(x) that represents the height of a mountain as a function of the distance x from the base. If the function f(x) is continuous and the mountain has a peak, then theIntermediate Value Theorem tells us that the function must take on every height value between the base and the peak.Mathematically, the Intermediate Value Theorem can be stated as follows: Let f(x) be a continuous function on a closed interval [a, b]. If f(a) and f(b) have opposite signs, then there exists a point c in the interval (a, b) such that f(c) = 0.The proof of the Intermediate Value Theorem is based on the properties of continuous functions and the completeness of the real number system. The key idea is that if a function changes sign on a closed interval, then it must pass through the value zero somewhere in that interval.One important application of the Intermediate Value Theorem is in the context of finding roots of equations. If a continuous function f(x) changes sign on a closed interval [a, b], then the Intermediate Value Theorem guarantees that there is at least one root (a value of x where f(x) = 0) within that interval. This is a powerful tool in numerical analysis and the study of nonlinear equations.Another application of the Intermediate Value Theorem is in the study of optimization problems. When maximizing or minimizing a continuous function on a closed interval, the Intermediate Value Theorem can be used to establish the existence of a maximum orminimum value within that interval.The Intermediate Value Theorem is also closely related to the concept of connectedness in topology. If a function is continuous on a closed interval, then the image of that interval under the function is a connected set. This means that the function "connects" the values at the endpoints of the interval, without any "gaps" in between.In addition to its theoretical importance, the Intermediate Value Theorem has practical applications in various fields, such as economics, biology, and physics. For example, in economics, the theorem can be used to show the existence of equilibrium prices in a market, where supply and demand curves intersect.In conclusion, the Intermediate Value Theorem is a fundamental result in calculus that has far-reaching implications in both theory and practice. Its ability to guarantee the existence of values between two extremes has made it an indispensable tool in the study of continuous functions and the analysis of complex systems. Understanding and applying this theorem is a crucial step in mastering the powerful concepts of calculus.。
The algebraic eigenvalue problem代数特征值问题

CLARENDON PRESS • OXFORD 1965
Contents
1. THEORETICAL BACKGROUND Page
Introduction Definitions Eigenvalues and eigenvectors of the transposed matrix Distinct eigenvalues Similarity transformations Multiple eigenvalues and canonical forms for general matrices Defective system of eigenvectors The Jordan (classical) canonical form The elementary divisors Companion matrix of the characteristic polynomial of A Non-derogatory matrices The Frobenius (rational) canonical form Relationship between the Jordan and Frobenius canonical forms Equivalence transformations Lambda matrices Elementary operations Smith's canonical form The highest common factor offc-rowedminors of a A-matrix Invariant factors of (A —XI) The triangular canonical form Hermitian and symmetric matrices Elementary properties of Hermitian matrices Complex symmetric matrices Reduction to triangular form by unitary transformations Quadratic forms Necessary and sufficient conditions for positive definiteness Differential equations with constant coefficients Solutions corresponding to non-linear elementary divisors Differential equations of higher order Second-order equations of special form Explicit solution of By = —Ay Equations of the form (AB— XI)x — 0 The minimum polynomial of a vector The minimum polynomial of a matrix Cayley-Hamilton theorem Relation between minimum polynomial and canonical forms Principal vectors Elementary similarity transformations Properties of elementary matrices Reduction to triangular canonical form by elementary similarity transformations Elementary unitary transformations Elementary unitary Hermitian matrices Reduction to triangular form by elementary unitary transformations Normal matrices Commuting matrices
拉格朗日乘子法
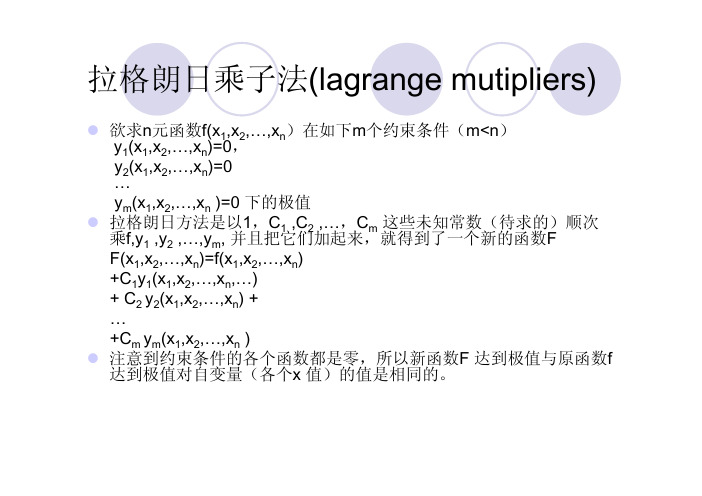
z 设长方体的三个棱长为x,y,z,则其体积f 为三个边长 的乘积:f(x,y,z)=xyz.要求表面积为a 的平方 于是长方体的6面的面积可以写成2xy+2xz+2yz=a2 即 2xy+2xz+2yz-a2 =0它也就是本问题中仅有一个约束条 件。根据前面介绍的拉格朗日方法制造一个新函数F,并 且放进一个未知的常数C ,于是有 F=xyz+C(2xy+2xz+2yz-a2 )
z 2. assume that the energy of the oscillators are quantized E = nhν = nhc / λ we see that at shortwavelength oscillators is very large. This energy is too large for the walls to supply it, so the shortwavelength oscillators remain unexcited.
z 上面的n 个方程连同约束条件给的m 个方程式已经可以解出n+m 个未 知数。它们就是n 个x(即x1,x2,…,xn )和m 个C (即C1 ,C2 ,…, Cm )。于是我们就得到了这个函数达到极值时的各个自变量的值。可 以看到约束条件不同,得到的各个x 值也不同。这样就利用拉格朗日 方法解决了问题
11.2(b)
z Describe how a wavefunction determines the dynamical properties of a system and how those properties may be perdicted.
z 1. In quantum mechanics all dynamical properties of a physical system have associated with them a corresponding operator. The system itself is described by a wavefunction.
薛定谔—麦克斯韦尔方程径向解的存在性和多重性(英文)

In 1887, the German physicist Erwin Schrödinger proposed a radial solution to the Maxwell-Schrödinger equation. This equation describes the behavior of an electron in an atom and is used to calculate its energy levels. The radial solution was found to be valid for all values of angular momentum quantum number l, which means that it can describe any type of atomic orbital.The existence and multiplicity of this radial solution has been studied extensively since then. It has been shown that there are infinitely many solutions for each value of l, with each one corresponding to a different energy level. Furthermore, these solutions can be divided into two categories: bound states and scattering states. Bound states have negative energies and correspond to electrons that are trapped within the atom; scattering states have positive energies and correspond to electrons that escape from the atom after being excited by external radiation or collisions with other particles.The existence and multiplicity of these solutions is important because they provide insight into how atoms interact with their environment through electromagnetic radiation or collisions with other particles. They also help us understand why certain elements form molecules when combined together, as well as why some elements remain stable while others decay over time due to radioactive processes such as alpha decay or beta decay.。
How large can the first eigenvalue be on a surface of genus two
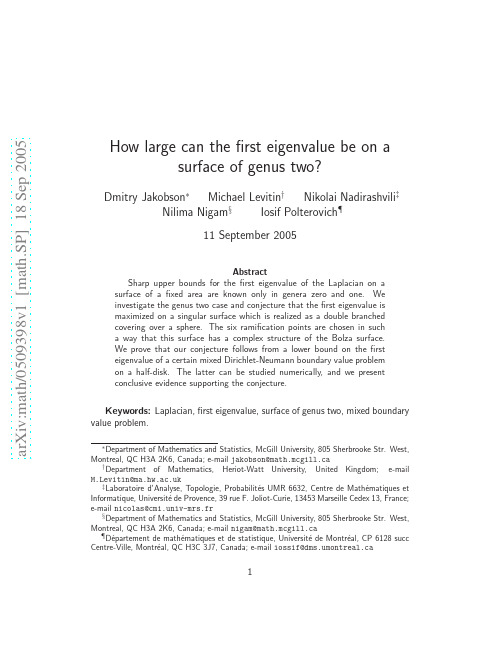
a rX iv:mat h /59398v1[mat h.SP]18Se p25How large can the first eigenvalue be on a surface of genus two?Dmitry Jakobson ∗Michael Levitin †Nikolai Nadirashvili ‡Nilima Nigam §Iosif Polterovich ¶11September 2005Abstract Sharp upper bounds for the first eigenvalue of the Laplacian on a surface of a fixed area are known only in genera zero and one.We investigate the genus two case and conjecture that the first eigenvalue is maximized on a singular surface which is realized as a double branched covering over a sphere.The six ramification points are chosen in such a way that this surface has a complex structure of the Bolza surface.We prove that our conjecture follows from a lower bound on the first eigenvalue of a certain mixed Dirichlet-Neumann boundary value problem on a half-disk.The latter can be studied numerically,and we present conclusive evidence supporting the conjecture.Keywords:Laplacian,first eigenvalue,surface of genus two,mixed boundary value problem.1Introduction and main results1.1Upper bounds on thefirst eigenvalueLet M be a closed surface of genusγand let g be the Riemannian metric on M.Denote by∆the Laplace-Beltrami operator on M,and byλ1the smallest positive eigenvalue of the Laplacian.Let the area Area(M)befixed.How large canλ1be on such a surface?Sharp bounds for thefirst eigenvalue are known only for the sphere([H],see also[SY]),the projective plane([LY]),the torus([Ber],[N]),and the Klein bottle ([JNP],[EGJ]).The present paper is concerned with the surface of genus2.Let M be orientable and letΠ:M→S2be a non-constant holomorphic map(or,conformal branched covering)of degree d.It was proved in[YY]thatλ1Area(M)≤8πd.(1.1.1) Any Riemann surface of genusγcan be represented as a branched cover over S2of degree d= γ+32 .(1.1.2) In general,(1.1.2)is not sharp,for example forγ=1([N]).Let M=P be a surface of genusγ=2.Then(1.1.2)impliesλ1Area(P)≤16π.(1.1.3) The aim of this paper is to show,using a mixture of analytic and numerical tools, that(1.1.3)is sharp.Main results of this paper were announced(without proofs) in[JLNP,section4].1.2The Bolza surfaceLetΠ:P→S2be a branched covering of degree d=2.The Riemann-Hurwitz formula(see[GH])implies that this cover is ramified at6points.We choose these points to be the intersections of the round sphere S2centered at the origin with the coordinate axes in R3.The surface P can be realized as(z,w)∈C2:w2=F(z):=z(z−1)(z−i)This surface has the conformal structure of the Bolza surface.It has an octahe-dral group of holomorphic automorphisms and its symmetry group is the largest among surfaces of genus two[I,KW].Interestingly enough,the Bolza surface appears in some other extremal problems,in particular for systoles(see[KS]).To simplify calculations it is convenient to rotate the equatorial plane byπ/4. The equation of P becomesP:= (z,w)∈C2:w2=F(z):=z(z−eπi/4)(z−e3πi/4)u 2,where the scalar product ·,· and the norm · are taken in the space L2(P,g0). The Sobolev space H10(P,g0)of functions supported away from the singularities is obtained by the closure of C∞0(P,g0):={v∈C∞(P,g0)|Since(P,g0)is a double cover of the standard S2,we haveArea(P,g0)=2Area(S2)=8π.Therefore,in order to prove Conjecture1.3.1it suffices to show thatλ1(P,g0)=λ1(S2)=2.(1.3.2)Unfortunately,we are unable to prove(1.3.2),and therefore establish Con-jecture1.3.1.We can however reduce the conjecture to the following spectral problem on a quarter-sphere Q⊂S2that can be treated using numerical ly,let,in usual spherical coordinates(φ,θ),Q={(φ,θ):0<φ<π/2,0<θ<π}.We split the boundary∂Q into two parts:∂Q=Finally,we note that the spectral problem(1.3.3)easily reduces via the stere-ographic projection to the following mixed Dirichlet-Neumann problem on a half-disk D:={(r,ψ)∈R2:r<1,0<ψ<π}(here(r,ψ)are usual planar polar coordinates):−∆v=4Λ(1+r2)2v on D,v|∂2D=0,(∂v/∂n)|∂1D=0.(1.3.7)We refer to[JLNP]for a further discussion on Dirichlet-Neumann swap isospec-trality.Figure1:Geometry of boundary value problems(1.3.6)(left)and(1.3.7)(right). Here and further on,the solid red line denotes Dirichlet boundary condition and the dashed blue line—the Neumann one.Remark1.3.8.One can check that a surface with afinite number of conical singularities can be approximated by a sequence of smooth surfaces of the same genus and area in such a way that the corresponding sequence of thefirst non-zero eigenvalues converges toλ1on the original surface.Thus,Conjecture1.3.1 means that(1.1.3)is sharp in the class of smooth metrics,although the equality is not necessarily attained.For a general result about the convergence of the whole spectrum see[Ro].52Symmetries2.1Hyperelliptic involutionLet T:P→P,T2=Id be a map that intertwines the preimages of points of S2under a two-sheeted coveringΠ:P→S2.Clearly,the Laplace operator∆commutes with T.By the spectral theorem,we can consider separately the restrictions of the Laplacian onto the spaces of functions which are either even or odd with respect to T.The even functions on P can be identified with the functions on S2. Therefore,asλ1(S2)=2,we haveλ1(P)≤2,and the equality in(1.3.2)will be achieved if and only if thefirst eigenvalueλodd1of the Laplacian acting on the odd subspace satisfiesλodd1≥2.2.2Isometries of PConsider the following isometries of S2(as usual,we identify S2and C by stere-ographic projection):σ1:z→¯z or(χ,η,ξ)→(χ,−η,ξ),σ2:z→−¯z or(χ,η,ξ)→(−χ,η,ξ),(2.2.1)σ3:z→1/¯z or(χ,η,ξ)→(χ,η,−ξ).Here z=x+iy is a point in the equatorial plane upon which a point(χ,η,ξ)∈S2 is stereographically projected.The hyperelliptic involution T is given by T:(z,w)→(z,−w).For1≤j≤3,a symmetryσj of S2has two corresponding symmetries s j and T◦s j satisfyingΠ◦s j=Π◦T◦s j=σj◦Π.(2.2.2) Those symmetries,with account of(1.2.1)are given by the explicit formulaes1:(z,w)→(¯z,¯z/¯w),s2:(z,w)→(−¯z,i¯w),(2.2.3)s3:(z,w)→(1/¯z,¯w/¯z).As an illustration,we demonstrate how the last of these formulae is obtained:if w2=F(z),then by(1.2.1),F 1¯z(1/¯z−eπi/4)(1/¯z−e3πi/4)F(z)thus giving the expression for s3.It easily seen that all s j commute with T and satisfys2j=Id,j=1,2,3;(2.2.4)s1s3=s3s1,s2s3=s3s2;s2s1=T s1s2.Remark2.2.5.In the proof of Theorem1.3.5we will use only the symmetries s1and s3.Calculations for s2are presented for the sake of completeness(see Remark3.4.1).2.3Fixed point sets of isometriesLet Fix(S)denote afixed point set of a mapping S.As easily seen from(2.2.1), the sets Fix(σj),for j=1,2,3,lie in the union of the coordinate lines and a unit circle of C,and we introduce the following notation for future reference.The coordinate lines are divided into two rays each by the ramification point r0:=0, and we denotea1:={z=t,t>0},a2:={z=it,t>0},a3:={z=t,t<0},a4:={z=it,t<0}.The circle is divided into four arcs by the ramification points r1:=e−πi/4, r2:=eπi/4,r3:=e3πi/4,and r4:=e−3πi/4,and we denote the arcs bya k+4:={z=e tπi/4,t∈(2k−3,2k−1)},k=1,2,3,4,so that the arc a5goes from r1to r2,the arc a6goes from r2to r3,the arc a7 goes from r3to r4,andfinally a8goes from r4to r1.In this notation,thefixed point sets Fix(σj)are written asFix(σ1)=a1∪a3,Fix(σ2)=a2∪a4,Fix(σ3)=a5∪a6∪a7∪a8.(2.3.1) Note that each of the rays a j(j=1,2,3,4)intersects an arc a j+4at a single point which we denote z j:z1=1,z2=i,z3=−1,z4=−i,see Figure2.7Figure2:Ramification points,rays,arcs and intersections 2.4Fixed point sets of s1,s2,s3Each of the points z j has exactly two pre-images p(m)j :=(w(m)j,z j)∈Π−1z j,m=1,2,where w(1,2)j are the solutions of the equation(w j)2=F(z j),with Fgiven in(1.2.1).These solutions are easily found from(1.2.1);we are of course at liberty to choose which of the two solutions is denoted w(1)j and which is8denoted w(2)j.For definiteness we setw(1)1=i,w(2)1=−i;w(1)2=1+i2,w(2)2=−1+i2; w(1)3=1,w(2)3=−1;w(1)4=1−i2,w(2)4=−1−i2.(2.4.1)For future use,we need to know the images of points p(m)j under the sym-metries s l,l=1,2,3.These are easily calculated from(2.2.3);it turns out thats l p(m)j =p(n)kwith some indices k∈{1,2,3,4},n∈{1,2}.The results of thecalculations are summarized in the following Table1.(j,m)(k,n)l=2(1,1)(1,1)(1,2)(1,2)(1,2)(1,1)(2,1)(2,2)(3,1)(3,2)(3,2)(3,2)(3,1)(3,1)(4,2)(4,1)the notation we postulate that b(m)k∋w(m)k′,k′=((k−1)mod4)+1,e.g. w(1)1∈b(1)1∩b(1)5,w(2)3∈b(2)3∩b(2)7,etc.We now have at our disposal all the information we need in order to obtain thefixed point sets of s1,s2,s3.We start with the following two simple Lemmas. Lemma2.4.2.ΠFix(s j)⊆Fix(σj).Proof.Let z∈ΠFix(s j).Then there exists p∈P such thatΠp=z and s j p= p.ThusΠs j p=z and by(2.2.2)σjΠp=σj z=z,so that z∈Fix(σj). Lemma2.4.3.Let a k⊆Fix(σj).Then,for m=1,2,either b(m)k⊆Fix(s j)or b(m)k⊆Fix(T◦s j).Proof.We haveΠb(m)k =a k,so thatσjΠb(m)k=σj a k=a k,and so by(2.2.2)Πs j b(m)k =a k=Πb(m)k=ΠT b(m)k.The result follows from the obvious observa-tion:ifΠα=Πβ,then eitherα=βorα=Tβ.The lemmas lead to the followingProposition2.4.4.Fix(s1)=b(1)1∪b(2)1,Fix(T s1)=b(1)3∪b(2)3,Fix(s2)=b(1)2∪b(2)2,Fix(T s2)=b(1)4∪b(2)4,Fix(s3)=b(1)6∪b(2)6∪b(1)8∪b(2)8,Fix(T s3)=b(1)5∪b(2)5∪b(1)7∪b(2)7.Proof.By Lemmas2.4.2and2.4.3,for any given j thefixed sets Fix(s j)and Fix(T s j)consist only of the pre-images of the components a k of the correspond-ingfixed sets Fix(σj)(given by(2.3.1)).However we still need to describe whichcomponent b(m)k,m=1,2,lies in Fix(s j)and which in Fix(T s j).As each com-ponent b k(m)is uniquely determined by the point w(m)kgiven by(2.4.1),it issufficient just to check in Table1whether s j w(m)k =w(m)kor T s j w(m)k=w(m)k.For example,tofind Fix(s2)we need only to inspect b(m)2and b(m)4.As,byTable1,s2w(m)2=w(m)2and T s2w(m)4=w(m)4,we have Fix(s2)=b(1)2∪b(2)2andFix(T s2)=b(1)4∪b(2)4.The rest of Proposition2.4.4is obtained in the same manner.103Proof of Theorem1.3.5We divide the proof of Theorem1.3.5into several steps.3.1Even eigenfunctions with respect to TConsider the subspace V+⊂L2(P)consisting of all even eigenfunctions with respect to T.Any such eigenfunction has a well-defined projection on S2.There-fore,if there exists afirst eigenfunction of P that belongs to V+,its projection is an eigenfunction on S2and hence the corresponding eigenvalue is greater or equal than two(recall thatλ1(S2)=2).Hence,in this case the Conjecture1.3.1 is verified.3.2Use of symmetries s1,s3.Denote by G13the subgroup of the automorphism group of P generated by the symmetries{T,s1,s3}.It follows from(2.2.4)that G13is commutative.Note also that all the elements of G13commute with the Laplacian on P.Therefore,we can choose a basis of L2(P)consisting of joint eigenfunctions of all s∈G13and∆.Given a joint eigenfunction f of all s∈G13,we denote byµ(f,s)the corresponding eigenvalue of s,i.e.f(sx)=µ(f,s)f(x).Since s2j=T2=Id for j=1,3,we see thatµ(f,s)=±1for all s∈G13.3.3Odd eigenfunctions with respect to TConsider now the space V−⊂L2(P)consisting of all eigenfunctions of the Laplacian which are odd with respect to T.Letφ1be a joint eigenfunction of {T,s1,s3,∆},corresponding to the smallest eigenvalue of∆ V−Now,sinceµ(φ1,T)=−1and s23T=T,we haveµ(φ1,s1)µ(φ1,s1T)=µ(φ1,T)=−1,and similarlyµ(φ1,s3)µ(φ1,s3T)=−1.Without loss of generality we may assume thatµ(φ1,s1)=−1.We recall from section2.3that thefixed point set Fix s1consists of the arcs b(1)1,b(2)1. Thusφ1must vanish on these arcs.11Consider now the symmetries s3,s3T.We must have one of the following two cases:i)µ(φ1,s3T)=−1,µ(φ1,s3)=1;ii)µ(φ1,s3)=−1,µ(φ1,s3T)=1.Considerfirst Case i).Proposition3.3.1.In Case i)the functionφ1vanishes on the arcsb(1)1,b(2)1,b(1)5,b(2)5,b(1)7,b(2)7,and its normal derivative∂nφ1vanishes on the arcsb(1)3,b(2)3,b(1)6,b(2)6,b(1)8,b(2)8.Proof.By Proposition2.4.4,thefixed-point set of s3T consists of the arcs b15,b25,b17,b27.Accordingly,φ1vanishes on all those arcs,as well as on b11,b21. Moreover,φ1hasµ(φ1,s3)=µ(φ1,s1T)=1.It follows that the normal deriva-tive of∂nφ1vanishes on thefixed-point sets of those symmetries.It remains to apply once more Proposition2.4.4in order to complete the proof.Consider next Case ii).Proposition3.3.2.In Case ii)the functionφ1vanishes on the arcsb(1)3,b(2)3,b(1)6,b(2)6,b(1)8,b(2)8,and its normal derivative∂nφ1vanishes on the arcsb(1)1,b(2)1,b(1)5,b(2)5,b(1)7,b(2)7.Proposition3.3.2is proved in the same way as Proposition3.3.1.3.4Final step of the proofSinceφ1is an odd function with respect to the hyperelliptic involution T,its projection upon S2is not well-defined.However,the projection of|φ1|to S2is well-defined.Denote it byψ1.In Case i),the functionψ1can be chosen as a test function for the mixed Dirichlet-Neumann boundary value problem(1.3.3).Assume now Conjecture121.3.4is true and thefirst eigenvalue of(1.3.3)satisfiesΛ1≥2.Then the Rayleigh quotient ofψ1and hence ofφ1satisfies the same inequality.But this means thatψ1cannot be thefirst eigenfunction on P since we get a contradiction with(1.1.3).Therefore,thefirst eigenfunction of P is even with respect to T, and as was shown in section3.1this implies Conjecture1.3.1.Similarly,in Case ii),the functionψ1can be chosen as a test function for the mixed Dirichlet-Neumann boundary volume problem which is obtained from (1.3.3)by swapping the Dirichlet and the Neumann conditions.However,it was shown in[JLNP]that this problem is isospectral to(1.3.3).Therefore, repeating the same arguments as above we prove that Conjecture1.3.1holds. This completes the proof of Theorem1.3.5.Remark3.4.1.In the proof of Theorem1.3.5we have used only the symmetries s1and s3.Alternatively,we could have used s2and s3.One can check directly using Proposition2.4.4that applying s2one obtains a mixed Dirichlet-Neumann boundary value problem which is equivalent to(1.3.3)and hence no additional information about thefirst eigenfunction is obtained.3.5A family of extremal surfaces of genus twoThe purpose of this section is to prove the followingCorollary3.5.1.Conjecture1.3.4implies that there exists a continuous family P t of surfaces of genus2such thatλ1Area(P t)=16π.Proof.Consider the Riemann surface P t defined by the equation(z,w):w2=z z−e i(π/2−t) z−e i(π/2+t)(1+r2)2v on D,v|∂1(t)=0,(∂v/∂n)|∂2(t)=0.(3.5.2)and−∆v=4ΛHere∂1(t):={(r,0):r∈(0,1)}∪{(1,ψ):|ψ−π/2|<t}and∂(t)D:= {(r,π):r∈(0,1)}∪{(1,ψ):π/2>|ψ−π/2|>t}.We remark that for t=π/4these two problems are not ing Dirichlet-Neumann bracketing it is easy to see that(3.5.2)has a smallerfirst eigenvalue than(3.5.3)if t<π/4and a larger one if t>π/4.Denote the minimalfirst eigenvalue of the two problems byΛ1(t).According to Conjec-ture2and numerical calculations,Λ1(π/4)>2.Since thefirst eigenvalues of both problems depend continuously and monotonically on parameter t,and since Λ1(0)=Λ1(π/2)=0.75(see section1.3),there exist numbers t∗1∈(0,π/4) and t∗2∈(π/4,π/2)such thatΛ1(t∗1)=Λ1(t∗2)=2and soΛ1(t)≥2for t∈[t∗1,t∗2].Arguing is above,we deduce that for all surfaces P t corresponding to these values of t,estimate(1.1.3)is sharp.This completes the proof of the theorem.Corollary3.5.1implies that16πis a degenerate maximum forλ1Area(M) for surfaces of genus two.This is not the case for surfaces of lower genus on which the metric maximizing thefirst eigenvalue is unique.Note also that the extremal metrics in genera zero and one are analytic,while the surfaces P t have singular points.4Numerical investigations4.1Basics of the Finite Element MethodIn this section we describe the numerical experiments used to estimate thefirst eigenvalue of(1.3.7).We define the space H as the closure of{v∈C∞(D)|dD.(4.1.1)(1+r2)2We usefinite elements to approximate the eigenvalues and eigenfunctions of (4.1.1).The general procedure we follow is:1.Discretize the region D using a triangular mesh T h= N h i=1τi,with a sizeof an individual triangleτ∈T h parameterized by h>0.142.Introduce afinite-dimensional subspace V h of H,consisting offinite ele-ment basis functions{φi}N h i=1on T h;3.Denote(v h,λh)∈V h×R,with v h=(v1,v2,...,v N h)t,the solution ofthefinite-dimensional generalized eigenvalue problemA h v h=λhB h v h,(4.1.2)where(A h)ij:= D∇φi·∇φj dD,(B h)ij:= D4φiφjFigure3:Afinite element meshλh N h No.ofArnoldi iterates2.00434573363e-052882.36301118569625118.16135748742e-0846082.301112381849409112.79565739833e-1073728dD,(1+r2)2satisfied|Err|<5×10−10.The results are tabulated in Table2.Experiment2:This experiment was conducted using MATLAB’sfinite el-ement package PDEToolbox,and the eigenvalue solve was performed using ARPACK routines.A sequence of triangular meshes was created,starting from the coarsest mesh,and refining5times.The major difference between this and the previous experiment is in the manner in which the zero Dirichlet data is enforced.16λh No.ofTriangles772.4040011835691850410612.305829341498988064163372.28276090970583129024258881dD,(1+r2)2satisfied|Err|<5×10−10.The results are presented in Table4.In each of the experiments above,we found that the computed eigenvalues appeared to converge to a value greater than2.27.The associated eigenfunctions also appear to converge to a function whose contour lines are shown in Figure4.17λh N h No.ofArnoldi iterates -1.5494060025e-05288-7.89999667122e-071152-3.8455570927e-084608-1.87263030138e-0918432-8.78059287464e-1173728fellowship and Alfred P.Sloan Foundation fellowship.The research of N.Nig. and I.P.was partially supported by NSERC and FQRNT.References[ArDu]M.Armentano and R.Duran,Asymptotic lower bounds for eigenvalues by nonconformingfinite element methods,Electron.Trans.Numer.Anal.17 (2004),93–101.[Bab]I.Babuska and J.Osborn,Eigenvalue problems,in Handbook of Numerical Analysis Vol.II,Finite Element Methods(Part1).Edited by P.G.Ciarlet and J.L.Lions.1991,Elsevier.[Ber]M.Berger,Sur les premi`e res valeurs propres des vari´e t´e s riemanniennes, Compositio Math.26(1973),129–149.[Br]D.Braess,Finite elements,Cambridge University Press,1997.[EGJ]A.El Soufi,H.Giacomini,M.Jazar,Greatest least eigenvalue of the Laplacian on the Klein bottle,preprint math.MG/0506585(2005).[GovL]G.Golub,C.van Loan,Matrix Computations,3rd Ed.,John Hopkins University Press,Baltimore,1996.[GH]P.Griffiths,J.Harris,Principles of algebraic geometry,Wiley,N.Y.,1978.[Gun]R.Gunning,Lectures on Riemann surfaces,Jacobi varieties,Mathematical Notes,No.12.,Princeton Univ.Press,1972.[H]J.Hersch,Quatre propri´e t´e s isop´e rim´e triques de membranes sph´e rique ho-mog`e nes,C.R.Acad.Paris270(1970),1645–1648.[I]J.Igusa,Arithmetic varierty of moduli for genus two,Annals of Math.72(1960),612–649.[JLNP].D.Jakobson,M.Levitin,N.Nadirashvili,I.Polterovich,Spectral prob-lems with mixed Dirichlet-Neumann boundary conditions:isospectrality and beyond,to appear in p.Appl.Math.(2005).[JNP]D.Jakobson,N.Nadirashvili,I.Polterovich,Extremal metric for thefirst eigenvalue on the Klein bottle,to appear in Canadian J.Math.(2005).19[KW]H.Karcher,M.Weber,The geometry of Klein’s Riemann surface.The eightfold way,MSRI Publ.35(1999),9–49,Cambridge Univ.Press.[KS]M.Katz,S.Sabourau,An optimal systolic inequality for CAT(0)metrics in genus two,preprint math.DG/0501017(2005).[Ke]J.Keller,Singularities at the tip of a plane angular sector,J.Math.Phys.40(1999),no.2,1087–1092.[LY]P.Li,S.-T.Yau.A new conformal invariant and its applications to the Willmore conjecture and thefirst eigenvalue of compact surfaces,Invent.Math.69(1982),269–291.[N]N.Nadirashvili,Berger’s isoperimetric problem and minimal immersions of surfaces,GAFA6(1996),877–897.[Ro]J.Rowlett,Spectral convergence of the Laplacian on a compact manifold with degenerating metric,to appear as a part of the Stanford University Ph.D.thesis under the supervision of R.Mazzeo.[SY]R.Schoen and S.-T.Yau,Lectures on Differential Geometry,International Press,1994.[TrBa]L.N.Trefethen and D.Bau III,Numerical Linear Algebra,SIAM,1997.[YY]P.Yang,S.-T.Yau,Eigenvalues of the Laplacian of compact Riemann surfaces and minimal submanifolds,Ann.Scuola Norm.Sup.Pisa Cl.Sci.(4) 7(1980),no.1,55–63.20。
- 1、下载文档前请自行甄别文档内容的完整性,平台不提供额外的编辑、内容补充、找答案等附加服务。
- 2、"仅部分预览"的文档,不可在线预览部分如存在完整性等问题,可反馈申请退款(可完整预览的文档不适用该条件!)。
- 3、如文档侵犯您的权益,请联系客服反馈,我们会尽快为您处理(人工客服工作时间:9:00-18:30)。
2
D. HUNDERTMARK AND B. SIMON
for fixed λ, allows perturbations of either sign. The decoupling in steps we use does not work for the question raised by Deift–Hempel, which may be why it does not seem to be in the literature. We will present two applications: a Cwikel–Lieb–Rozenblum-type finiteness result [20, 34, 39] for suitable gaps in d ≥ 3 periodic Schr¨ odinger operators and a critical power estimate on eigenvalues in some one-dimensional almost periodic problems. To state our results precisely, we need some notation. For any selfadjoint operator C , EΩ (C ) will denote the spectral projections for C . We define #(C ∈ Ω) = dim(EΩ (C )) and #(C > α) = dim(E(α,∞) (C )) and similarly for #(C ≥ α), #(C < α), #(C ≤ α). We will write B = B+ − B− (1.2) (1.1)
(1.3)
with B± ≥ 0. While often we will take B± = max(±B, 0), we do not require B+ B− = 0 or [B+ , B ] = 0. Our main technical result, which we will prove in Section 2, is Theorem 1.1. Let A be a selfadjoint operator and x, y ∈ R so (x, y ) ∩ σ (A) = ∅. Let B be given by (1.3) with B+ , B− both compact. Let 1 C = A + B . Let x < e0 < e1 = 2 (x + y ), then
k k
(1.5)
We say y is a nondegenerate gap edge if and only if min εℓ+1(k ) > y
k
(1.6)
EIGENVALUE BOUNDS
3
and εℓ (k ) = y at a finite number of points {kj }N j =1 in the unit cell so that for some C and all k in the unit cell, εℓ (k ) − y ≥ C min|k − kj |2 (1.7)
+1 If E = ∪ℓ j =1 Ej is a finite union of bounded closed disjoint intervals, there is an isospectral torus TE associated to E of almost periodic J ’s with σ (J ) = E (see [3, 4, 18, 19, 24, 37, 46, 47]). We conjecture the following: d/2
arXiv:0705.3646v1 [math.SP] 24 May 2007
EIGENVALUE BOUNDS IN THE GAPS OF ¨ SCHRODINGER OPERATORS AND JACOBI MATRICES
DIRK HUNDERTMARK1 AND BARRY SIMON2 Abstract. We consider C = A + B where A is selfadjoint with a gap (a, b) in its spectrum and B is (relatively) compact. We prove a general result allowing B of indefinite sign and apply it to obtain a (δV )d/2 bound for perturbations of suitable periodic Schr¨ odinger operhirring bound for perturbations of algebro-geometric almost periodic Jacobi matrices.
1 #(C ∈ (e0 , e1 )) ≤ #(B+ (e0 − A)−1 B+ ≥ 1) + #(B− ≥ 2 (y − x)) (1.4) 1/2 1/2
In Section 3, we discuss an analog when A is unbounded but bounded below and B± are only relatively compact. If V is a periodic locally Ld/2 function on Rd (d ≥ 3), then A = −∆ + V can be written as a direct integral of operators, A(k ), with compact resolvent, with the integral over the fundamental cell of a dual lattice (see [38]). If ε1 (k ) ≤ ε2 (k ) ≤ . . . are the eigenvalues of A(k ), then (x, y ) is a gap in σ (A) (i.e., connected component of R \ σ (A)) if and only if there is ℓ with max εℓ−1 (k ) = x < y = min εℓ (k )
There is a similar definition at the bottom edge if x > −∞. It is a general theorem [31] that the bottom edge is always nondegenerate. In Section 4, we will prove Theorem 1.2. Let d ≥ 3. Let V ∈ Lloc (Rd ) be periodic and let W ∈ Ld/2 (Rd ). Let (x, y ) be a gap in the spectrum A = −∆ + V which is nondegenerate at both ends, and let N(x,y) (W ) = #(−∆ + V + W ∈ (x, y )). Then N(x,y) (W ) < ∞. This will be a simple extension of the result of Birman [11] who proved this if W has a fixed sign. Note we have not stated a bound by d/2 W d/2 . This is discussed further in Section 4. In the final section, Section 5, we will consider certain two-sided Jacobi matrices, J , on ℓ2 (Z) with bk k=ℓ a ℓ=k+1 k Jkℓ = (1.8) ak−1 ℓ = k − 1 0 |ℓ − k | ≥ 2
Date : May 18, 2007. 2000 Mathematics Subject Classification. 47B36, 81Q10, 35P15. Key words and phrases. eigenvalue bounds, Jacobi matrices, Schr¨ odinger operators. 1 School of Mathematics, Watson Hall, University of Birmingham, Birmingham, B15 2TT, UK. On leave from Department of Mathematics, Altgeld Hall, University of Illinois at Urbana-Champaign, Urbana, IL 61801, USA. Email: hundertd@. Supported in part by NSF grant DMS-0400940. 2 Mathematics 253-37, California Institute of Technology, Pasadena, CA 91125, USA. E-mail: bsimon@. Supported in part by NSF grant DMS-0140592 and U.S.–Israel Binational Science Foundation (BSF) Grant No. 2002068.
1. Introduction The study of the eigenvalues of Schr¨ odinger operators below the essential spectrum goes back over fifty years to Bargmann [5], Birman [6], and Schwinger [43], and of power bounds on the eigenvalues to Lieb–Thirring [35, 36]. There has been considerably less work on eigenvalues in gaps—much of what has been studied followed up on seminal work by Deift and Hempel [23]; see [1, 2, 25, 26, 27, 28, 29, 32, 33, 40, 41, 42] and especially work by Birman and collaborators [7, 8, 9, 10, 11, 12, 13, 14, 15, 16, 17]. Following Deift–Hempel, this work has mainly focused on the set of λ’s so that some given fixed e in a gap of σ (A) is an eigenvalue of A + λB and the growth of the number of eigenvalues as λ → ∞ most often for closed intervals strictly inside the gap. Most, but not all, of this work has focused on B ’s of a definite sign. Our goal in this note is to make an elementary observation that, as regards behavior at an edge