Heavy Meson Production in Proton-Nucleus Reactions with Empirical Spectral Functions
古龙页岩油高温高压注CO2驱动用效果
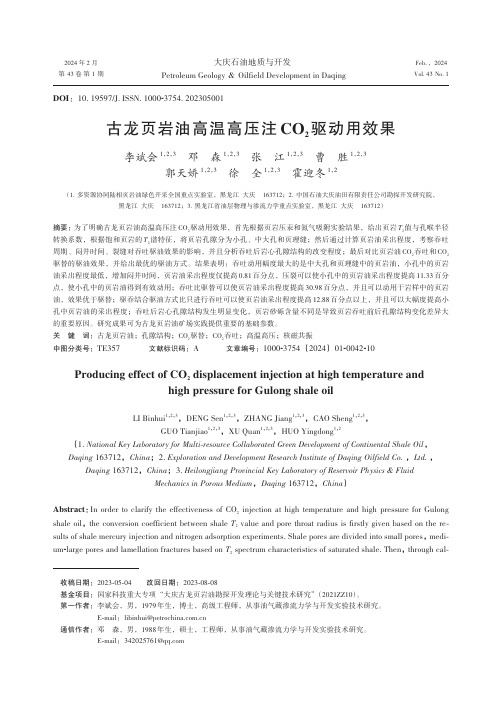
大庆石油地质与开发Petroleum Geology & Oilfield Development in Daqing2024 年 2 月第 43 卷 第 1 期Feb. ,2024Vol. 43 No. 1DOI :10.19597/J.ISSN.1000-3754.202305001古龙页岩油高温高压注CO 2驱动用效果李斌会1,2,3 邓森1,2,3 张江1,2,3 曹胜1,2,3郭天娇1,2,3 徐全1,2,3 霍迎冬1,2(1.多资源协同陆相页岩油绿色开采全国重点实验室,黑龙江 大庆163712;2.中国石油大庆油田有限责任公司勘探开发研究院,黑龙江 大庆163712;3.黑龙江省油层物理与渗流力学重点实验室,黑龙江 大庆163712)摘要: 为了明确古龙页岩油高温高压注CO 2驱动用效果,首先根据页岩压汞和氮气吸附实验结果,给出页岩T 2值与孔喉半径转换系数,根据饱和页岩的T 2谱特征,将页岩孔隙分为小孔、中大孔和页理缝;然后通过计算页岩油采出程度,考察吞吐周期、闷井时间、裂缝对吞吐驱油效果的影响,并且分析吞吐后岩心孔隙结构的改变程度;最后对比页岩油CO 2吞吐和CO 2驱替的驱油效果,并给出最优的驱油方式。
结果表明:吞吐动用幅度最大的是中大孔和页理缝中的页岩油,小孔中的页岩油采出程度最低,增加闷井时间,页岩油采出程度仅提高0.81百分点,压裂可以使小孔中的页岩油采出程度提高11.33百分点,使小孔中的页岩油得到有效动用;吞吐比驱替可以使页岩油采出程度提高30.98百分点,并且可以动用干岩样中的页岩油,效果优于驱替;驱吞结合驱油方式比只进行吞吐可以使页岩油采出程度提高12.88百分点以上,并且可以大幅度提高小孔中页岩油的采出程度;吞吐后岩心孔隙结构发生明显变化,页岩砂砾含量不同是导致页岩吞吐前后孔隙结构变化差异大的重要原因。
研究成果可为古龙页岩油矿场实践提供重要的基础参数。
关键词:古龙页岩油;孔隙结构;CO 2驱替;CO 2吞吐;高温高压;核磁共振中图分类号:TE357 文献标识码:A 文章编号:1000-3754(2024)01-0042-10Producing effect of CO 2 displacement injection at high temperature and high pressure for Gulong shale oilLI Binhui 1,2,3,DENG Sen 1,2,3,ZHANG Jiang 1,2,3,CAO Sheng 1,2,3,GUO Tianjiao 1,2,3,XU Quan 1,2,3,HUO Yingdong 1,2(1.National Key Laboratory for Multi⁃resource Collaborated Green Development of Continental Shale Oil ,Daqing 163712,China ;2.Exploration and Development Research Institute of Daqing Oilfield Co.,Ltd.,Daqing 163712,China ;3.Heilongjiang Provincial Key Laboratory of Reservoir Physics & FluidMechanics in Porous Medium ,Daqing 163712,China )Abstract :In order to clarify the effectiveness of CO 2 injection at high temperature and high pressure for Gulong shale oil , the conversion coefficient between shale T 2 value and pore throat radius is firstly given based on the re⁃sults of shale mercury injection and nitrogen adsorption experiments. Shale pores are divided into small pores , medi⁃um -large pores and lamellation fractures based on T 2 spectrum characteristics of saturated shale. Then , through cal⁃收稿日期:2023-05-04 改回日期:2023-08-08基金项目:国家科技重大专项“大庆古龙页岩油勘探开发理论与关键技术研究”(2021ZZ10)。
硫酸铅基底表面性质对铁矾异相成核生长的影响
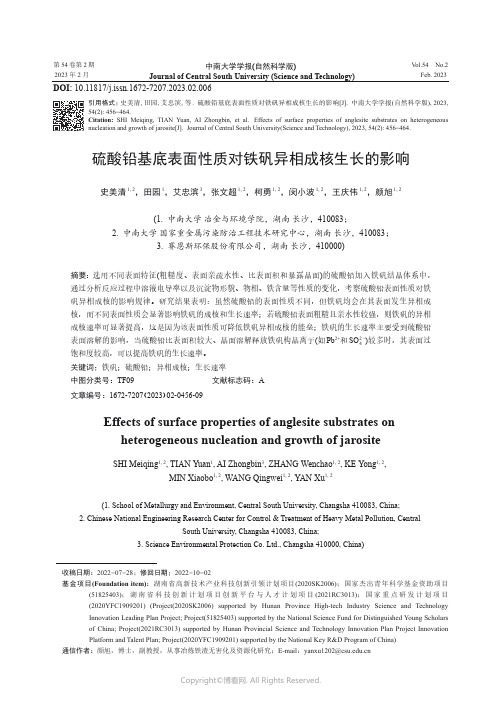
第 54 卷第 2 期2023 年 2 月中南大学学报(自然科学版)Journal of Central South University (Science and Technology)V ol.54 No.2Feb. 2023硫酸铅基底表面性质对铁矾异相成核生长的影响史美清1, 2,田园1,艾忠滨3,张文超1, 2,柯勇1, 2,闵小波1, 2,王庆伟1, 2,颜旭1, 2(1. 中南大学 冶金与环境学院,湖南 长沙,410083;2. 中南大学 国家重金属污染防治工程技术研究中心,湖南 长沙,410083;3. 赛恩斯环保股份有限公司,湖南 长沙,410000)摘要:选用不同表面特征(粗糙度、表面亲疏水性、比表面积和暴露晶面)的硫酸铅加入铁矾结晶体系中,通过分析反应过程中溶液电导率以及沉淀物形貌、物相、铁含量等性质的变化,考察硫酸铅表面性质对铁矾异相成核的影响规律。
研究结果表明:虽然硫酸铅的表面性质不同,但铁矾均会在其表面发生异相成核,而不同表面性质会显著影响铁矾的成核和生长速率;若硫酸铅表面粗糙且亲水性较强,则铁矾的异相成核速率可显著提高,这是因为该表面性质可降低铁矾异相成核的能垒;铁矾的生长速率主要受到硫酸铅表面溶解的影响,当硫酸铅比表面积较大、晶面溶解释放铁矾构晶离子(如Pb 2+和SO 2-4)较多时,其表面过饱和度较高,可以提高铁矾的生长速率。
关键词:铁矾;硫酸铅;异相成核;生长速率中图分类号:TF09 文献标志码:A 文章编号:1672-7207(2023)02-0456-09Effects of surface properties of anglesite substrates onheterogeneous nucleation and growth of jarositeSHI Meiqing 1, 2, TIAN Yuan 1, AI Zhongbin 3, ZHANG Wenchao 1, 2, KE Yong 1, 2,MIN Xiaobo 1, 2, WANG Qingwei 1, 2, YAN Xu 1, 2(1. School of Metallurgy and Environment, Central South University, Changsha 410083, China;2. Chinese National Engineering Research Center for Control & Treatment of Heavy Metal Pollution, CentralSouth University, Changsha 410083, China;3. Science Environmental Protection Co. Ltd., Changsha 410000, China)收稿日期: 2022 −07 −28; 修回日期: 2022 −10 −02基金项目(Foundation item):湖南省高新技术产业科技创新引领计划项目(2020SK2006);国家杰出青年科学基金资助项目(51825403);湖南省科技创新计划项目创新平台与人才计划项目(2021RC3013);国家重点研发计划项目(2020YFC1909201) (Project(2020SK2006) supported by Hunan Province High-tech Industry Science and Technology Innovation Leading Plan Project; Project(51825403) supported by the National Science Fund for Distinguished Young Scholars of China; Project(2021RC3013) supported by Hunan Provincial Science and Technology Innovation Plan Project Innovation Platform and Talent Plan; Project(2020YFC1909201) supported by the National Key R&D Program of China)通信作者:颜旭,博士,副教授,从事冶炼铁渣无害化及资源化研究;E-mail :*****************.cnDOI: 10.11817/j.issn.1672-7207.2023.02.006引用格式: 史美清, 田园, 艾忠滨, 等. 硫酸铅基底表面性质对铁矾异相成核生长的影响[J]. 中南大学学报(自然科学版), 2023, 54(2): 456−464.Citation: SHI Meiqing, TIAN Yuan, AI Zhongbin, et al. Effects of surface properties of anglesite substrates on heterogeneous nucleation and growth of jarosite[J]. Journal of Central South University(Science and Technology), 2023, 54(2): 456−464.第 2 期史美清,等:硫酸铅基底表面性质对铁矾异相成核生长的影响Abstract:Three kinds of anglesite with different surface properties (roughness, surface hydrophilicity, specific surface area and exposed crystal surface) were added to the iron-containing solution during the jarosite crystallization reaction. Through the analysis of conductivity of the solution and the morphology, phase, iron content of the precipitate obtained during the reaction process, the effect of surface properties of anglesite on heterogeneous nucleation and growth of jarosite was investigated. The results show that in spite of different surface properties of anglesite, all the jarosite is heterogeneously nucleated on anglesite, and the different surface properties can significantly affect the nucleation and growth rate of jarosite. When the surface of anglesite is rough and hydrophilic, the heterogeneous nucleation rate of jarosite can be significantly increased. Because the energy barrier for heterogeneous nucleation of jarosite can be reduced. The growth rate is mainly affected by the surface dissolution of anglesite. Anglesite has large specific surface area, and the crystal surface dissolves and releases more component of jarosite crystal (such as Pb2+and SO2-4), leading to a high local supersaturation, which can promote the growth rate of jarosite.Key words: jarosite; anglesite; heterogeneous nucleation; growth rate铁矾渣是湿法炼锌过程中铁矾法除铁所产生的沉淀渣,大多湿法炼锌企业采用焙烧—浸出—净化—电积工艺生产锌产品。
高等植物重金属耐性与超积累特性及其分子机理研究

高等植物重金属耐性与超积累特性及其分子机理研究孙瑞莲2 周启星1*(1中国科学院沈阳应用生态研究所陆地生态过程重点实验室,沈阳 110016)(2中国科学院研究生院,北京 100039)摘 要 由于重金属污染日益严重,重金属在土壤_植物系统中的行为引起了人们的高度重视。
高等植物对重金属的耐性与积累性,已经成为污染生态学研究的热点。
近年来,由于分子生态学等学科的发展,有关植物对重金属的解毒和耐性机理、重金属离子富集机制的研究取得了较大进展。
高等植物对重金属的耐性和积累在种间和基因型之间存在很大差异。
根系是重金属等土壤污染物进入植物的门户。
根系分泌物改变重金属的生物有效性和毒性,并在植物吸收重金属的过程中发挥重要作用。
土壤中的大部分重金属离子都是通过金属转运蛋白进入根细胞,并在植物体内进一步转运至液泡贮存。
在重金属胁迫条件下植物螯合肽(PC)的合成是植物对胁迫的一种适应性反应。
耐性基因型合成较多的PC,谷胱甘肽(GS H)是合成PC 的前体,重金属与PC 螯合并转移至液泡中贮存,从而达到解毒效果。
金属硫蛋白(MTs)与PC 一样,可以与重金属离子螯合,从而降低重金属离子的毒性。
该文从分子水平上论述了根系分泌物、金属转运蛋白、MTs 、PC 、GS H 在重金属耐性及超积累性中的作用,评述了近10年来这方面的研究进展,并在此基础上提出存在的问题和今后研究的重点。
关键词 高等植物 重金属 耐性 超积累特性 植物修复HEAVY METAL TOLERA NCE AND HYPERACCUMULATION OF HIGHERPLANTS AND THEIR MOLEC ULAR MECHANISMS:A REVIEWSUN Rui_Lian 2and ZHOU Qi_Xing 1*(1Key L abo ratory o f Terrestrial Ec olo gical Proc ess ,Institute o f Applied Ecology ,Chine se Academy o f Sc ienc es ,Shenyang 110016,China)(2Graduate School o f Chine se Academy o f Sc ie nce s ,Bei j ing 100039,China)Abstract Owing to serious heavy metal pollution,much attention has been paid to its effects on soil_plant systems.The research of heavy metal tolerance and hyperaccumulation of higher plants has become a hot topic in the field of pollution ecology.With the development of molecular ecology,research on the mechanisms of heavy metal tolerance,detoxification and accumulation in higher plants has made progress in recent years.There are significant differences in the tolerance to and accumulation of heavy metals among higher plant species and genotypes.Root systems are the first entrance of heavy metal pollutants from the soil into plant.Root exudates reduce the availability and toxicity of metal pollutants and play an important role in ability for plants to absorb heavy metals.Almost all heavy metal ions enter root cells with the help of a metal transporter protein that are subsequently transported to the vacuole.The synthesis of PC in response to the stress caused by heavy metals is one of the adaptive responses com mon in higher plants.Heavy metal tolerant genotypes have higher le vels of PC than non_tolerant genotypes under heavy metal stress.GSH is the substrate that synthesizes PC,which chelates the heavy metals.Heavy metal_PC chelatins are subsequently transported from the cytosol to the vacuole and heavy metal detoxification is thus achieved.MTs play the same role and in the same wa y as PC under heavy metal stress.The article reviews recent advances in understanding the role of root exudates,metal transporter proteins (MTs,PC and GSH),molecular mechanisms of heavy metal tolerance and hyperac -cumulation in higher plants at the molecular level.Existing problems and major topics of future research were discussed.Key words Higher plant,Heavy metal,Tolerance,Hyperaccumulation,Phytoremediation 现代农业中各种农药和化肥的大量使用,汽车尾气的大量排放,城市污水及垃圾处理不当以及工收稿日期:2004-03-18 接受日期:2004-07-16基金项目:国家杰出青年科学基金(20225722)和国家自然科学基金重点项目(20337010) *通讯作者Author for correspondence E_mail:Zhouqi xing2003@E_mail:s unning527@植物生态学报 2005,29(3)497~504Acta Phytoecologica Sinica业生产所产生的 三废 的不合理排放,导致土壤中重金属含量急剧增加,土壤_植物系统中重金属污染问题日趋严重(周启星,1995;孙铁珩等,2001)。
乙酸蒸汽催化重整制氢的研究进展
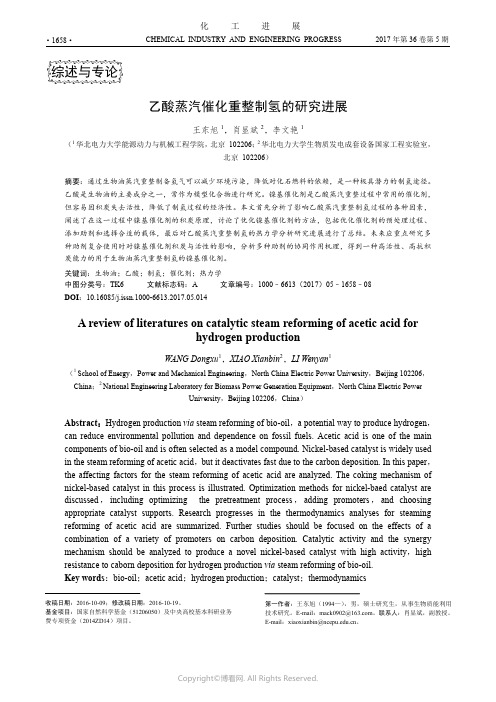
CHEMICAL INDUSTRY AND ENGINEERING PROGRESS 2017年第36卷第5期·1658·化 工 进展乙酸蒸汽催化重整制氢的研究进展王东旭1,肖显斌2,李文艳1(1华北电力大学能源动力与机械工程学院,北京 102206;2华北电力大学生物质发电成套设备国家工程实验室,北京 102206)摘要:通过生物油蒸汽重整制备氢气可以减少环境污染,降低对化石燃料的依赖,是一种极具潜力的制氢途径。
乙酸是生物油的主要成分之一,常作为模型化合物进行研究。
镍基催化剂是乙酸蒸汽重整过程中常用的催化剂,但容易因积炭失去活性,降低了制氢过程的经济性。
本文首先分析了影响乙酸蒸汽重整制氢过程的各种因素,阐述了在这一过程中镍基催化剂的积炭原理,讨论了优化镍基催化剂的方法,包括优化催化剂的预处理过程、添加助剂和选择合适的载体,最后对乙酸蒸汽重整制氢的热力学分析研究进展进行了总结。
未来应重点研究多种助剂复合使用时对镍基催化剂积炭与活性的影响,分析多种助剂的协同作用机理,得到一种高活性、高抗积炭能力的用于生物油蒸汽重整制氢的镍基催化剂。
关键词:生物油;乙酸;制氢;催化剂;热力学中图分类号:TK6 文献标志码:A 文章编号:1000–6613(2017)05–1658–08 DOI :10.16085/j.issn.1000-6613.2017.05.014A review of literatures on catalytic steam reforming of acetic acid forhydrogen productionWANG Dongxu 1,XIAO Xianbin 2,LI Wenyan 1(1 School of Energy ,Power and Mechanical Engineering ,North China Electric Power University ,Beijing 102206,China ;2 National Engineering Laboratory for Biomass Power Generation Equipment ,North China Electric PowerUniversity ,Beijing 102206,China )Abstract :Hydrogen production via steam reforming of bio-oil ,a potential way to produce hydrogen , can reduce environmental pollution and dependence on fossil fuels. Acetic acid is one of the main components of bio-oil and is often selected as a model compound. Nickel-based catalyst is widely used in the steam reforming of acetic acid ,but it deactivates fast due to the carbon deposition. In this paper ,the affecting factors for the steam reforming of acetic acid are analyzed. The coking mechanism of nickel-based catalyst in this process is illustrated. Optimization methods for nickel-baed catalyst are discussed ,including optimizing the pretreatment process ,adding promoters ,and choosing appropriate catalyst supports. Research progresses in the thermodynamics analyses for steaming reforming of acetic acid are summarized. Further studies should be focused on the effects of a combination of a variety of promoters on carbon deposition. Catalytic activity and the synergy mechanism should be analyzed to produce a novel nickel-based catalyst with high activity ,high resistance to caborn deposition for hydrogen production via steam reforming of bio-oil. Key words :bio-oil ;acetic acid ;hydrogen production ;catalyst ;thermodynamics第一作者:王东旭(1994—),男,硕士研究生,从事生物质能利用技术研究。
微生物生态学课件 PPT
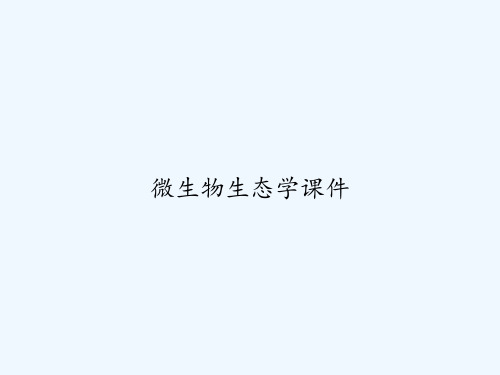
Mechanisms of resistence to heavy -metal
Oxidation
bonded
Reduce
Interactio n
Increase
Adsorption
Acidificati on
Reduce the absorption
the heavy metal content in the microbial cells at a low level is maintained without causing toxic effects on cells.
Cyanobacteria
ቤተ መጻሕፍቲ ባይዱ
Fungi
• Small mold, single-celled yeast and fruiting body of large-scale fungus.
• Their cell walls contain consists largely of chitin and glucan.
Mode of resistance to heavy- metal
• Release of chelating agent and iron carrier.
• Acidification of the soil environment, • Change the REDOX potential. • Reduce the absorption. • Increase the discharge • Oxidation reduction
微生物生态学课件
Heavy –metal pollutants
Heavy metals being transferred to humans via the food chain would finally threaten human beings health.
高产抗菌脂肽Fengycin芽孢杆菌的诱变育种和发酵条件优化

陈尚里,于福田,沈圆圆,等. 高产抗菌脂肽Fengycin 芽孢杆菌的诱变育种和发酵条件优化[J]. 食品工业科技,2023,44(23):134−143. doi: 10.13386/j.issn1002-0306.2023020239CHEN Shangli, YU Futian, SHEN Yuanyuan, et al. Mutation Breeding and Optimization of Fermentation Conditions of Bacillus Highly Producing Antimicrobial Lipopeptide Fengycin[J]. Science and Technology of Food Industry, 2023, 44(23): 134−143. (in Chinese with English abstract). doi: 10.13386/j.issn1002-0306.2023020239· 生物工程 ·高产抗菌脂肽Fengycin 芽孢杆菌的诱变育种和发酵条件优化陈尚里,于福田,沈圆圆,刘小玲*(广西大学轻工与食品工程学院,广西南宁 530004)摘 要:为了提高Fengycin 产量,以芽孢杆菌YA-215为出发菌,通过复合诱变(紫外诱变、ARTP-LiCl 诱变)育种来获取高产Fengycin 突变体。
通过单因素实验和响应面试验等确定最佳发酵工艺优化。
结果表明,复合诱变选育获得一株高产Fengycin 突变株UA397,全基因组测序结合16S 进化样本分析显示为暹罗芽孢杆菌。
其最佳发酵工艺条件为:蔗糖25 g/L 、蛋白胨30 g/L 、发酵温度37.7 ℃、发酵时间37.8 h 、接种量5.01%。
在此发酵条件下,暹罗芽孢杆菌UA-397的Fengycin 产量为517.09 mg/L ,是野生型在未进行发酵条件优化时Fengycin 产量113.02 mg/L 的4.575倍。
铜冶炼脱硫石膏渣的环境稳定性与重金属释放机制

第 54 卷第 2 期2023 年 2 月中南大学学报(自然科学版)Journal of Central South University (Science and Technology)V ol.54 No.2Feb. 2023铜冶炼脱硫石膏渣的环境稳定性与重金属释放机制王云燕1, 2,何紫彤1,唐巾尧1, 3,孙竹梅1, 4,徐慧1,杜嘉丽1,张李敏1,柴立元1, 2(1. 中南大学 冶金与环境学院,湖南 长沙,410083;2. 国家重金属污染防治工程技术研究中心,湖南 长沙,410083;3. 湖南有色金属研究院有限责任公司,湖南 长沙,410100;4. 中北大学 环境与安全工程学院,山西 太原,030051)摘要:以铜冶炼脱硫石膏渣为对象,分析其矿物学性质,研究其中重金属的浸出毒性、赋存形态,评价其环境活性;并基于模拟堆存、静态侵蚀、半动态侵蚀等实验阐明脱硫石膏渣的环境稳定性、重金属释放特性、释放过程动力学及机制。
研究结果表明:脱硫石膏渣中As 、Cd 的浸出毒性分别为687.33 mg/L 和 3.8 mg/L ,As 、Cd 的酸可提取态分别占73.76%和96.06%,存在高浸出危险性,环境风险较大,不能直接堆存、填埋。
模拟堆存实验末期潜在生态风险指数较初期仅下降了5.92%,综合潜在生态风险等级仍为严重风险,因此在堆存过程中应切实做好防扬尘、防淋失等措施。
脱硫石膏渣中各金属元素在模拟填埋场环境中的浸出量明显高于其他模拟环境中的浸出量,需特别关注填埋过程的环境风险。
金属释放主要是通过表面吸附物质的解吸、溶解实现,主要风险元素As 、Cd 的释放均为两段式,其环境风险在堆存初期更为明显,应重点关注。
研究结果为铜冶炼脱硫石膏渣的环境污染防治及环境风险防控策略提供了理论支撑。
关键词:脱硫石膏渣;环境风险;模拟堆存;静态浸出;半动态浸出;重金属释放特性;释放机制中图分类号:X705 文献标志码:A 开放科学(资源服务)标识码(OSID)文章编号:1672-7207(2023)02-0562-15Long-term environmental stability and heavy metals release mechanism of desulfurized gypsum sludge from copper smelterWANG Yunyan 1, 2, HE Zitong 1, TANG Jinyao 1, 3, SUN Zhumei 1, 4, XU Hui 1,DU Jiali 1, ZHANG Limin 1, CHAI Liyuan 1, 2(1. School of Metallurgy and Environment, Central South University, Changsha 410083, China;收稿日期: 2022 −08 −04; 修回日期: 2022 −11 −22基金项目(Foundation item):国家重点研发计划项目(2018YFC1903301,2020YFC1909201);国家自然科学基金资助项目(51634010);湖南省自然科学基金资助项目(S2021JJZDXM0007) (Projects(2018YFC1903301, 2020YFC1909201) supported by the National Key Research and Development Program; Project(51634010) supported by the National Natural Science Foundation of China; Project(S2021JJZDXM0007) supported by the Natural Science Foundation of Hunan Province)通信作者:孙竹梅,博士,副教授,从事固废处理与资源化、环境电化学领域的研究;E-mail :*******************DOI: 10.11817/j.issn.1672-7207.2023.02.016引用格式: 王云燕, 何紫彤, 唐巾尧, 等. 铜冶炼脱硫石膏渣的环境稳定性与重金属释放机制[J]. 中南大学学报(自然科学版), 2023, 54(2): 562−576.Citation: W ANG Y unyan, HE Zitong, TANG Jinyao, et al. Long-term environmental stability and heavy metals release mechanism of desulfurized gypsum sludge from copper smelter[J]. Journal of Central South University(Science and Technology), 2023, 54(2): 562−576.第 2 期王云燕,等:铜冶炼脱硫石膏渣的环境稳定性与重金属释放机制2. Chinese National Engineering Research Center for Control & Treatment of Heavy Metal Pollution,Changsha 410083, China;3. Hunan Research Institute for Nonferrous Metals Co. Ltd., Changsha 410100, China;4. School of Environment and Safe Engineering, North University of China, Taiyuan 030051, China)Abstract:Based on simulated stockpiling, static erosion, and semi-dynamic erosion experiments, the environmental stability, heavy metal release characteristics, kinetics and mechanism of release process of desulfurization gypsum slag discharged from a copper smelter were elucidated. The results show that the leaching toxicity of As and Cd is 687.33 mg/L, 3.8 mg/L, and acid extractable state of As and Cd in desulfurization gypsum slag is 73.76%, 96.06%, respectively, with severe leaching hazard and environmental risk, which cannot be directly stored and landfilled. The PERI at the end of the simulated stockpiling experiment only decreases by5.92% compared to the beginning, and the overall potential ecological risk level remains high. Hence, theprevention of dust and leaching should be implemented during the stockpiling process. The amount of each metal element leached in desulfurization gypsum slag in the simulated landfill environment is much larger than that leached in other simulated environments, and the environmental risk of the landfill process should be given special consideration. The release of metals is mainly achieved by desorption and dissolution of surface adsorbed substances. The main risk elements, As and Cd, release in two stages, with the early stages of landfilling posing the greatest environmental dangers. This study can provide theoretical support for the prevention and control of copper smelting desulfurization gypsum slag pollution and risk to the environment.Key words: desulfurized gypsum sludge; environmental risk; simulated stockpiling; static erosion; semi-dynamic erosion; heavy metal release behavior; release mechanism中国作为铜的消费中心,铜的产量也居世界首位。
Adsorption of heavy metal ion from aqueous single metal solution by chemically
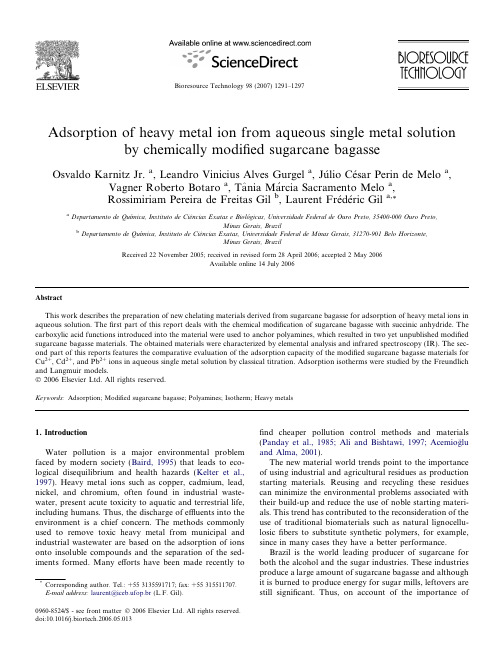
Adsorption of heavy metal ion from aqueous single metal solutionby chemically modified sugarcane bagasseOsvaldo Karnitz Jr.a ,Leandro Vinicius Alves Gurgel a ,Ju´lio Ce ´sar Perin de Melo a ,Vagner Roberto Botaro a ,Taˆnia Ma ´rcia Sacramento Melo a ,Rossimiriam Pereira de Freitas Gil b ,Laurent Fre´de ´ric Gil a,*aDepartamento de Quı´mica,Instituto de Cie ˆncias Exatas e Biolo ´gicas,Universidade Federal de Ouro Preto,35400-000Ouro Preto,Minas Gerais,BrazilbDepartamento de Quı´mica,Instituto de Cie ˆncias Exatas,Universidade Federal de Minas Gerais,31270-901Belo Horizonte,Minas Gerais,BrazilReceived 22November 2005;received in revised form 28April 2006;accepted 2May 2006Available online 14July 2006AbstractThis work describes the preparation of new chelating materials derived from sugarcane bagasse for adsorption of heavy metal ions in aqueous solution.The first part of this report deals with the chemical modification of sugarcane bagasse with succinic anhydride.The carboxylic acid functions introduced into the material were used to anchor polyamines,which resulted in two yet unpublished modified sugarcane bagasse materials.The obtained materials were characterized by elemental analysis and infrared spectroscopy (IR).The sec-ond part of this reports features the comparative evaluation of the adsorption capacity of the modified sugarcane bagasse materials for Cu 2+,Cd 2+,and Pb 2+ions in aqueous single metal solution by classical titration.Adsorption isotherms were studied by the Freundlich and Langmuir models.Ó2006Elsevier Ltd.All rights reserved.Keywords:Adsorption;Modified sugarcane bagasse;Polyamines;Isotherm;Heavy metals1.IntroductionWater pollution is a major environmental problem faced by modern society (Baird,1995)that leads to eco-logical disequilibrium and health hazards (Kelter et al.,1997).Heavy metal ions such as copper,cadmium,lead,nickel,and chromium,often found in industrial waste-water,present acute toxicity to aquatic and terrestrial life,including humans.Thus,the discharge of effluents into the environment is a chief concern.The methods commonly used to remove toxic heavy metal from municipal and industrial wastewater are based on the adsorption of ions onto insoluble compounds and the separation of the sed-iments formed.Many efforts have been made recently tofind cheaper pollution control methods and materials(Panday et al.,1985;Ali and Bishtawi,1997;Acemiog˘lu and Alma,2001).The new material world trends point to the importance of using industrial and agricultural residues as production starting materials.Reusing and recycling these residues can minimize the environmental problems associated with their build-up and reduce the use of noble starting materi-als.This trend has contributed to the reconsideration of the use of traditional biomaterials such as natural lignocellu-losic fibers to substitute synthetic polymers,for example,since in many cases they have a better performance.Brazil is the world leading producer of sugarcane for both the alcohol and the sugar industries.These industries produce a large amount of sugarcane bagasse and although it is burned to produce energy for sugar mills,leftovers are still significant.Thus,on account of the importance of0960-8524/$-see front matter Ó2006Elsevier Ltd.All rights reserved.doi:10.1016/j.biortech.2006.05.013*Corresponding author.Tel.:+553135591717;fax:+55315511707.E-mail address:laurent@iceb.ufop.br (L.F.Gil).Bioresource Technology 98(2007)1291–1297bagasse sugar as an industrial waste,there is a great interest in developing chemical methods for recycling it.Sugarcane bagasse has around50%cellulose,27%polyoses,and23% lignin(Caraschi et al.,1996).These three biological poly-mers have many hydroxyl and/or phenolic functions that can be chemically reacted to produce materials with new properties(Xiao et al.,2001;Navarro et al.,1996).Despite the many studies of the chemical modification of cellulose published around the world in this area(Gurnani et al.,2003;Gellerested and Gatenholm,1999),only a few have investigated the modification of bagasse sugar(Krish-nan and Anirudhan,2002;Orlando et al.,2002).This work describes the preparation and the evaluation of new chelating materials from sugarcane bagasse to adsorb heavy metal ions in aqueous solution.In a prelimin-ary study,it has been chosen to study the adsorption of Cu2+,Cd2+,and Pb2+.Thefirst part of this work describes the modification of sugarcane bagasse with succinic an-hydride to introduce carboxylic functions to sugarcane bagasse and the chemical introduction of commercial linear polyamine via the formation of amide functions.It is well known that polyamines have powerful chelating properties, mainly towards ions such as Cu2+,Zn2+,and Pb2+(Bian-chi et al.,1991;Martell and Hancock,1996).The second part of this work evaluates the adsorption of Cu2+,Cd2+,and Pb2+onto three modified sugarcane bag-asses(MSBs)from aqueous single metal ion solutions by classical titration.The results were analyzed by the Lang-muir and Freundlich models(Ho et al.,2005).2.Methods2.1.MaterialsPolyamines ethylenediamine3and triethylenetetramine 4were used in this work.Succinic anhydride,1,3-diiso-propylcarbodiimide(DIC),and triethylenetetramine,from Aldrich,were used without purification.Ethylenediamine and dimethylformamide were distilled before use.Pyridine was refluxed with NaOH and distilled.2.2.Sugarcane bagasse preparationSugarcane bagasse was dried at100°C in an oven for approximately24h and nextfiber size was reduced to pow-der by milling with tungsten ring.The resulting material was sieved with a4-sieve system(10,30,45,and60mesh). Then,the material was washed with distilled water under stirring at65°C for1h and dried at100°C.Finally,it was washed anew in a sohxlet system with n-hexane/ ethanol(1:1)as solvent for4h.2.3.Synthesis of MSBs1and2Washed and dried sugarcane bagasse(5.02g)was trea-ted with succinic anhydride(12.56g)under pyridine reflux (120mL)for18h.The solid material wasfiltered,washed in sequence with1M solution of acetic acid in CH2Cl2, 0.1M solution of HCl,ethanol95%,distilled water,and finally with ethanol95%.After drying at100°C in an oven for30min and in a desiccator overnight,MSB1(7.699g) was obtained with a mass gain of53.4%.MSB2was obtained by treatment of1with saturated NaHCO3solu-tion for30min and afterwards byfiltering using sintered filter and washing with distilled water and ethanol.2.4.Synthesis of MSBs5and6The process used to introduce amine functions was the same as that used to prepare MSB5and6.MSB1was trea-ted with5equiv of1,3-diisopropylcarbodiimide(DIC)and 6equiv of polyamine in anhydrous DMF at room tempera-ture for22h under stirring.Afterfiltration,the materials were washed with DMF,a saturated solution of NaHCO3, distilled water,andfinally with ethanol.Next,they were dried at80°C in an oven for30min and in a desiccator overnight.2.5.Kinetic study of metal ion adsorption of MSBs2,5,and6Experiments with each material and metal ion were per-formed to determine the adsorption equilibrium time from 10to90min in10min intervals.The amount of100mg MSB was placed in a250-mL Erlenmeyer with100.0mL metal ion solution with concentration of200mg/L under stirring.The experiments were done at pHs5.8for Cu2+, 7.0for Cd2+,and6.2for Pb2+,optimal values to obtain the best adsorption.To adjust pH values,was added NaOH solution(0.01mol/L)into metal solutions with MSB.Afterfiltration,metal ion concentration was deter-mined by EDTA titration.2.6.pH study of metal ion adsorption of MSBs2,5,and6Experiments with each material and metal ion were per-formed to determine the effect of pH on ion adsorption.An amount of100mg MSB was placed into a250-mL Erlen-meyer with100.0mL of metal ion solution200mg/L under stirring.pH was calibrated with HCl or NaOH solutions (0.1–1.0mol/L).The reaction times used were30min (MSB2)or40min(MSB5and6)for Cu2+and Cd2+, and40min(MSB2)or50min(MSB5and6)for Pb2+. Metal ion concentration was determined afterfiltration by EDTA titration.No significative variation of pH was observed at the end of each experiment.2.7.Adsorption isotherms of MSBs2,5,and6Experiments were performed for each material and metal ion to determine adsorption isotherms.In each experiment,100mg of MSB was placed into a250-mL Erlenmeyer with100.0mL of metal ion solution in specific concentrations(between200mg/L and400mg/L)under stirring.Each experiment was performed at the pH of1292O.Karnitz Jr.et al./Bioresource Technology98(2007)1291–1297larger ion adsorption during the time necessary for equilib-rium (Tables 3and 4).After filtration,the metal ion con-centration was determined by EDTA titration.2.8.Characterization of the new obtained materials MSB 1,2,5,and 6were characterized by IR spectro-scopy in a Nicolet Impact 410equipment with KBr.Elemental analyses were accomplished in Analyzer 2400CHNS/O Perkin Elemer Series II.3.Results and discussion3.1.Synthesis of MSBs 1,2,5,and 6The synthesis route used to prepare MSBs 1,2,5,and 6are presented in Scheme 1.Prewashed sugarcane bagasse was succinylated for various periods of time.The degree of succinylation of the bagasse fibers was determined by measuring the quantity of acid function.The results are shown in Fig.1.The concentration of carboxylic functions per mg of bagasse was determined by retro titration.For this,MSB 1was initially treated with an excess solution of NaOH (0.01mol/L)for 30min.Soon afterwards the material was filtered and the obtained solution was titrated with an HCl solution (0.01mol/L).The highest degree of succinylation was reached after 18-h ing this reaction time,sugarcane bagasse was succinylated to pro-duce MSB 1,which presented a weight gain of 54%and a concentration of carboxylic acid function per mg of 3.83·10À6mol.Next,MSB 1was treated with a saturated NaHCO 3solution to produce MSB 2.Starting from MSB 1,two polyamines were introduced:ethylenediamine 3and triethylenetetramine 4.The method-ology used to introduce the polyamines was the same for the two MSBs 5and 6,as shown in Scheme 1.Concentra-tions of 2.4·10À6mol (5)and 2.6·10À6mol (6)of amine function per mg of material were determined by back titra-tion with excess HCl solution.The introduction of the amine functions was also verified by IR spectroscopy (Table 1)and elemental analysis (Table 2).3.2.Characterization of MSBs 1,5,and 6Characterization of carboxylated MSB 1was accom-plished by IR spectroscopy.The spectrum of unmodified sugarcane bagasse and MSB 1are presented in Fig.2.The spectrum of MSB 1displayed two strong bands at 1740and 1726cm À1in relation to that of unmodified sug-arcane bagasse.This demonstrated the presence of two types of carbonyl functions,one relative to carboxylic acid and another relative to the ester.The acid and ester IR bands indicate that succinic anhydride acylated theO.Karnitz Jr.et al./Bioresource Technology 98(2007)1291–12971293hydroxy group of bagasse to generate an ester bond with consequent release of a carboxylic acid functional group.The spectra of MSBs5and6(Figs.3and4,respectively) showed three new strong bands at1550–1650cmÀ1(see data in Table1)corresponding to the presence of amide and amine functions,and one band at1060cmÀ1 corresponding to C–N stretch.The bands at1635and 1650cmÀ1(Fig.3)correspond to the axial deformation of the carbonyl of the amide function and the angular deformation of the N–H bond of the amine function.The band at1575cmÀ1corresponds to the angular deformation of the N–H bond of the amide function.The band at 1159cmÀ1(Fig.4)corresponds to the asymmetric stretch of C–N–C bond.The main bands observed in all MSBs are presented in Table1.MSB elemental analysis data presented in Table2show a modification in the carbon and hydrogen composition of MSB1and a larger proportion of nitrogen as the number of amine functions in the used polyamine increases.3.3.Study of adsorption of Cu2+,Cd2+and Pb2+on MSBs2,5,and6The study of the MSB adsorption properties was accom-plished for each material and metal ion.A kinetic study and an adsorption study as a function of pH werefirst carried out.3.3.1.Effect of contact timeThe kinetic study of MSB2with Cu2+,Cd2+,and Pb2+ ions in aqueous solution is presented in Fig.5.Adsorption equilibrium was reached after20min for Cd2+ions.A time of30min was chosen for all studies of MSB2with Cd2+. The adsorption equilibrium times chosen for pH and con-centration dependent experiments are presented in Table3.Similar studies were accomplished for MSBs5and6for Cu2+,Cd2+,and Pb2+.The results are presented in Table3.3.3.2.pH EffectThe removal of metal ions from aqueous solutions by adsorption is dependent on solution pH as it affects adsor-Table1Main IR spectrum bands observed in MSBs1,5,and6MSB Main bands observed(cmÀ1)11740,172651745,1650,1635,1575,1423,1060 61738,1651,1635,1560,1400,1159,1060 Table2Elemental analysis of MSBs1,2,5,and6C(%)H(%)N(%) Sugarcane bagasse43.98 6.020.13MSB145.41 5.620.10MSB238.04 5.140.01MSB544.01 6.51 2.21MSB646.88 6.65 3.431294O.Karnitz Jr.et al./Bioresource Technology98(2007)1291–1297bent surface charge,the degree of ionization,and the species of adsorbates.The study of adsorption of Cd 2+,Cd 2+,and Pb 2+on MSB 2as a function of pH was accom-plished with the reaction times given in Table 3;the results are presented in Fig.6.The adsorption of the three metal ions increases with the increase in pH.Maximum removal of Cd 2+was observed above pH 6and in the case of Pb 2+and Cu 2,above pH 5and 5.5.Similar studies were accomplished for MSBs 5and 6and Cu 2+,Cd 2+and Pb 2+with similar results,as shown in Table 4.3.3.3.Adsorption isothermsThe Langmuir (Ho et al.,2005)(Eq.(1))and Freundlich (Eq.(2))isotherms were evaluated by adsorption experi-ments as a function of the initial metal ion concentrations in aqueous solution under equilibrium time and pH condi-tions given in Tables 3and 4.The results of each material and metal ion are presented in Fig.7(Langmuir)and Fig.8(Freundlich)and Table 5.c q ¼1Q max Âb þc Q maxð1Þln q ¼ln k þ1nln cð2ÞTable 3Adsorption equilibrium times of MSBs 2,5and 6MSB Equilibrium time (min)Cu 2+Cd 2+Pb 2+230304054040506404050Table 4pH of largest adsorption of MSBs 2,5and 6MSB pH of largest adsorption Cu 2+Cd 2+Pb 2+2 5.5–6.0 6.5–7.5 5.0–6.05 5.5–6.0 6.5–7.5 5.0–6.065.5–6.06.5–7.55.0–6.0O.Karnitz Jr.et al./Bioresource Technology 98(2007)1291–12971295where q(mg/g)is the concentration of adsorbed metal ions per gram of adsorbent,c(mg/L)is the concentration of metal ion in aqueous solution at equilibrium,Q max and b are the Langmuir equation parameters and k and n are the Freundlich equation parameters.High correlation coefficients of linearized Langmuir and Freundlich equations indicate that these models can explain metal ion adsorption by the materials satisfactorily. Therefore,both models explained metal ion adsorption by MSBs2,5,and6as can be observed in Table5,with the exception of the Freundlich model for Pb2+adsorption by MSB2.The Langmuir isotherm parameter Q max indicates the maximum adsorption capacity of the material,in other words,the adsorption of metal ions at high concentrations. It can be observed in Table5that MSB5presents the larg-est Cu2+adsorption capacity while MSB6adsorbs Cd2+ and Pb2+the ngmuir parameter b indicates the bond energy of the complexation reaction of the material with the metal ion.It can be observed that MSB2presents the largest bond energy for Cu2+and Cd2+,while three materials do not differ significantly for Pb2.The Freundlich isotherm parameter k indicates the adsorption capacity when the concentration of the metal ion in equilibrium is unitary,in our case1mg/L.This parameter is useful in the evaluation of the adsorption capacity of metal ions in dilute solutions,a case closer to the characteristics of industrial effluents.The values of k of MSB2and5are much similar for Cu2+and Cd2+ and much higher than that for MSB6.This shows the superiority of both materials in the adsorption of these metal ions in low concentrations.MSB5has a higher k value for Pb2+when compared to those of the other materials.These results were compared with those of Vaughan et al.(2001)for a commercial macroreticular chelating resin with thiol functional groups,Duolite GT-73.The Q max of Duolite GT-73for Cu2+,Cd2+,and Pb2+were 62mg/g,106mg/g,and122mg/g,respectively.Duolite GT-73exhibited Q max lower than those of MSBs(Table5).4.ConclusionsThrough a fast,effective,and cheap methodology,it was possible to devise a strategy to introduce chelating func-tions(carboxylic acid and amine)to sugarcane bagasse. Modified sugarcane bagasses presented a good adsorption capacity for Cu2+,Cd2+,and Pb2+ions with maximum adsorption capacity observed for MSB6.It has been dem-onstrated that metal ion adsorption efficiency is propor-tional to the number of amine functions introduced into the material.MSB2,which contained only carboxylate functions,showed an efficiency similar to that of MSB5, a material of much more complex synthesis. AcknowledgementsWe thank FAPEMIG forfinancial support,CAPES and UFOP.Table5The Langmuir and Freundlich parameters for Cu2+,Cd2+and Pb2+ adsorptionMetalion MSB Langmuir FreundlichQ max (mg/g)b(L/mg)r2k(mg/g)n r2Cu2+21140.431191.623.90.919351390.1730.999898.315.80.906161330.0140.992722.8 3.640.9635Cd2+21960.1030.993459.4 4.160.977351640.0680.995762.8 5.490.983463130.0040.9528 5.15 1.630.9856Pb2+21890.1100.994566.0 4.660.757951890.1250.999914724.510.98163130.1210.9994121 5.210.8771296O.Karnitz Jr.et al./Bioresource Technology98(2007)1291–1297ReferencesAcemiog˘lu,B.,Alma,M.H.,2001.Equilibrium studies on adsorption of Cu(II)from aqueous solution onto cellulose.Journal of Colloid and Interface Science243,81–83.Ali,A.A.,Bishtawi,R.,1997.Removal of lead and nickel ions using zeolite tuff.Journal of Chemical Technology and Biotechnology69, 27–34.Baird,C.,1995.Environmental Chemistry.W.H.Freeman and Company, New York.Bianchi,A.,Micheloni,M.,Paoletti,P.,1991.Thermodynamic aspects of the polyazacycloalkane complexes with cations and anions.Coordi-nation Chemistry Reviews110,17–113.Caraschi,J.C.,Campana,S.P.,Curvelo, A.A.S.,1996.Preparac¸a˜o e Caracterizac¸a˜o de Polpas Obtidas a Partir de Bagac¸o de Cana de Ac¸u´car.Polı´meros:Cieˆncia e Tecnologia3,24–29.Gellerested,F.,Gatenholm,P.,1999.Surface properties of lignocellulosic fibers bearing carboxylic groups.Cellulose6,103–121.Gurnani,V.,Singh,A.K.,Venkataramani,B.,2003.2,3-Dihydroxypyri-dine-loaded cellulose:a new macromolecular chelator for metal enrichment prior to their determination by atomic absorption spectrometry.Analytical and Bioanalytical Chemistry377,1079–1086. Ho,Y.S.,Chiu,W.T.,Wang,C.C.,2005.Regression analysis for the sorption isotherms of basic dyes on sugarcane dust.Bioresource Technology96,1285–1291.Kelter,P.B.,Grundman,J.,Hage,D.S.,Carr,J.D.,Castro-Acun˜a,C.M., 1997.A discussion of water pollution in the United States and Mexico;with High School Laboratory Activities for the analysis of lead, atrazine,and nitrate.Journal of Chemical Education74,1413–1421. Krishnan,K.A.,Anirudhan,T.S.,2002.Removal of mercury(II)from aqueous solutions and chlor-alkali industry effluent by steam activated and sulphurised activated carbons prepared from bagasse pith:kinetics and equilibrium studies.Journal of Hazardous Materials92,161–183. Martell, A.E.,Hancock,R.D.,1996.Metal complexes in aqueous solutions.Plenum,New York.Navarro,R.R.,Sumi,K.,Fujii,N.,Matsumura,M.,1996.Mercury removal from wastewater using porous cellulose carrier modified with polyethyleneimine.Water Research30,2488–2494.Orlando,U.S.,Baes,A.U.,Nishijima,W.,Okada,M.,2002.Preparation of chelating agents from sugarcane bagasse by microwave radiation as an alternative ecologically benign procedure.Green Chemistry4,555–557.Panday,K.K.,Gur,P.,Singh,V.N.,1985.Copper(II)removal from aqueous solutions byfly ash.Water Research19,869–873. Vaughan,T.,Seo,C.W.,Marshall,W.E.,2001.Removal of selected metal ions from aqueous solution using modified corncobs.Bioresource Technology78,133–139.Xiao,B.,Sun,X.F.,Sun,R.,2001.The chemical modification of lignins with succinic anhydride in aqueous systems.Polymer Degradation and Stability71,223–231.O.Karnitz Jr.et al./Bioresource Technology98(2007)1291–12971297。
- 1、下载文档前请自行甄别文档内容的完整性,平台不提供额外的编辑、内容补充、找答案等附加服务。
- 2、"仅部分预览"的文档,不可在线预览部分如存在完整性等问题,可反馈申请退款(可完整预览的文档不适用该条件!)。
- 3、如文档侵犯您的权益,请联系客服反馈,我们会尽快为您处理(人工客服工作时间:9:00-18:30)。
a rXiv:n ucl-t h /96747v124Jul1996Heavy Meson Production in Proton-Nucleus Reactions with Empirical Spectral Functions ∗A.Sibirtsev,W.Cassing and U.Mosel Institut f¨u r Theoretische Physik,Universit¨a t Giessen D-35392Giessen,Germany February 7,2008PACS:24.10.-i;24.30.-v;24.50.+g;25.40.-h Abstract We study the production of K +,ρ,ωand φmesons in p +12C reactions on the basis of empirical spectral functions.The high momentum,high removal energy part of the spectral function is found to be negligible in all cases close to the absolute threshold.Furthermore,the two-step process (pN →πNN ;πN →N +K +,ρ,ω,φ)dominates the cross section at threshold energies in line with earlier calculations based on the folding model.1IntroductionThe production of heavy mesons in proton-nucleus reactions even below the free nucleon-nucleon threshold is of specific interest[1,2,3,4,5]as one hopes to learn about cooperative nuclear phenomena,high momentum components of the nuclear wavefunc-tion or in-medium modifications of the mesons themselves.Furthermore,a precise knowledge of the meson production channels in p+A collisions is a necessary step to-wards a microscopic understanding of meson production in nucleus-nucleus collisions, where in-medium modifications should be enhanced due to the higher baryon densities achieved[6,7].Especially the production channels for the vector mesons is of par-ticular interest because their abundancy can be controlled by dilepton spectroscopy independently.A possible’softening’of theρmeson at high baryon density[8]as possibly indicated by e+e−andµ+µ−data[9,10,11,12]should also show up in the ρ0production on nuclei and could be controlled by theπ+π−invariant spectrum.So far,the production ofηand K+mesons on nuclei has been addressed,both theoretically[13,14,15,16,17,18]and experimentally[19,20,21,22,23,24],whereas only a few model studies on the production of K−andρ,ω,φmesons are available[15, 25].Most of the former approaches-though partly using the experimental momentum distribution for the target of interest-employ a quasi-free dispersion relation for the struck target nucleon and thus come in conflict with the A-body energy-momentum conservation close to the absolute threshold because the struck target nucleon is off-shell.Experimental information about the nucleon momentum and energy distribution is available from(e,e′p)reactions[26].The data show in addition to the’mean-field’spectral function a high momentum,high energy component which might be attributed to nucleon-nucleon correlations with high relative but low center-of-mass momenta[27]. The influence of this’correlation’component on heavy meson production in proton-nucleus collisions is of particular interest in our present study as well as the role of primary and secondary(pion induced)reaction channels.Our paper thus is organized as follows:In Section2we describe our approach for meson production in proton induced reactions with spectral functions and discuss its ingredients,i.e.the spectral function itself,the elementary production cross sections in pN andπN collisions,the effective number of collisions as well as meson reabsorp-tion effects in Section3.By discarding possible in-medium effects we then present in Section4our results for p+12C reactions for K+,ρ,ωandφmesons as a function of the beam energy T0with their channel decompositions.A summary and discussion of open problems concludes this paper in Section5.2Reaction model for proton-nucleus collisionsIn terms of a multiple scattering theory the particle production in proton-nucleus col-lisions can be described as an incoherent sum of two-body interactions[28,29,30]. However,the individual interactions in nuclear matter might differ from those in free space due to the average interaction of the collision partners with the surrounding nuclear medium[31].The first term from the multiple scattering theory we denote as the ’direct’or ’one-step’production process.Furthermore,neglecting the medium effects on the re-action partners,this approach corresponds to the ’impulse approximation’which can be applied whenever the relevant energy scale is large compared to the nuclear binding energy.Within the single-scattering approximation the Lorentz-invariant differential cross section for meson production in p +A collisions then can be written asE M d 3σpA →MX A Ωd 3q dωS P (q ,ω)E ′M d 3σpN →MX (√d 3p ′M(1)with√A A j =1ωj =M A1We adopt the normalization d 3q dωS P (q,ω)=A ,where A denotes the target mass.As proposed in[37,38]the dominant contribution to subthreshold heavy meson production should arise from a two-step reaction mechanism with an intermediate pion.In accord with[13,17]the cross sections for K+,ρ,ωandφ-mesons from the secondary pion induced reactions are calculated asE M d3σpA→MXA Ωd3q dωd3p′πs)σtot×E′πd3σpN→πX(√d3p′π(5)where the double prime indices denote the system of the intermediate pion and a target nucleon,while the single prime indices are those for the beam and thefirst target nucleon.Moreover,Eπd3σpN→πX/d3pπstands for theπ-meson differential production cross section,which is calculated in line with(1),whileσtot is the total proton-nucleon cross section.We note that the on-shell elementaryπ-meson production e.g.can be described within the isobar model[39,40].The factor gπin Eq.(5)accounts for the probability that the pion interacts again with a target nucleon(cf.Section3.4).3Ingredients of the model3.1The spectral functionThe in-medium relation between the energyωand the momentum q of the target nucleon relevant to subthreshold particle production can be defined in analogy to Guet and Prakash[41],where the energy E prod(available for the meson production)is related to the incoming energy by total energy conservation asE∗0+M A=E prod+ M2A−1+q2 1/2+E exc,(6) where M A and M A−1are the masses of the initial and residual nuclei,respectively,q is the internal momentum and E exc stands for the excitation energy of thefinal nucleus.The excitation energy E exc now is related to the removal energy E R as[42]E exc=E R+M A−M A−1−m N,(7) where E R is the energy necessary to extract the nucleon from the nucleus.Thusω=m N−E R(8) with E R>0.The probability tofind a nucleon with momentum q and removal energy E R in a nucleus now is given by the nucleon spectral function S(q,E R).Accordingly, the function S P(q,ω)entering our calculations in(1),(5)can be evaluated from the spectral function S(q,E R)by substituting Eq.(8).The evaluation of the spectral function itself is quite an involved problem[43,44,45] and still a matter of discussion.In the present calculations we adopt S(q,E R)from Sick et al.[46]obtained within the orthogonal correlated basis approach as well as aphenomenological spectral function from Ciofidegli Atti and Simula[27],that both provide a good description of(e,e′p)reactions.The S(q,E R)for12C from ref.[46]is shown in Fig.1as a function of the nuclear nucleon momentum q and removal energy E R.In the upper part of Fig.2we display the spectral function up to E R≈60MeV on a larger scale in order to show the’mean-field’distribution dominated by the s1/2and p3/2single-particle levels at E R≈35MeVand E R≈20MeV,respectively.As a’background’under the horizontal shell structure one can notice a ridge roughly alongE R≃45MeV−q2.(10)2m NThis is close to the dispersion relation often used in folding model calculations.The full spectral function up to E R=500MeV is given in the lower part where the ’mean-field’contribution only shows up as tiny ridges,that extend up to q≃0.3GeV/c, i.e.up to the region of the Fermi momentum.Beyond this value the distribution is dominated by the correlations.The dashed line indicates the maximum of the spectral function for high momenta attributed to short-range and tensor correlations asq2E R=2ǫ+contributions from the uncorrelated part(dashed line)as well as the saturation of the momentum sum rule for the correlated part at E max=30MeV(dotted line)and 100MeV(dashed-dotted line).The uncorrelated part of the spectral function includes the ground and one-hole states of the residual nucleus.The short-range and tensor parts of the realistic NN interactions deplete the states below the Fermi level and partially occupy the states above thus generating the component of S(q,E R)with high momentum and high removal energy.The momentum distribution corresponding to the correlated part for E max=30MeV(dotted lines)is found to be small for all nuclei in comparison to the’mean-field’contribution.3.2Cross sections for pion induced reactionsFor our calculations we will need the elementary particle production channels over a wide kinematical regime and,in particular,down to the reaction threshold,where the experimental data often do not exist.In this section we,therefore,formulate a parametrization that allows us to perform an extrapolation of the cross section into the unmeasured region.The cross sections for meson production fromπ+N collisions can be separated into two parts.Thefirst one is an exclusive cross section related to binary processes, which are dominant at energies close to the reaction threshold,whereas the second part contains the inclusive production at higher energies.The cross section for the exclusive K+meson production fromπ+N collisions has been calculated by Tsushima et al.[47];we adopt their results for our present study.The cross section forρ,ωandφ-meson production from the reactionπ+N→R→M+N(13) is most conveniently described within a resonance model.Assuming that the squared matrix element is proportional to a Breit-Wigner function the cross section for the reaction(13)can be written asσ(π+N→M+N;s)=π2B in B outΓ2s−M R)2+Γ2/4×R2(s),(14)where J,B in and B out are the resonance spin and the branching ratios of the incoming and outgoing channels respectively,while the factor R2stands for the phase-space volume of thefinal particles,R2=πλ1/2(s,m N,m M)/s1/2,(15) with m N and m M denoting the masses of the nucleon and meson,respectively.In Eq.(14)k2is given byk2=λ(s,mπ,m N)(16) with the K¨a llen functionλ(z,x,y)= z−(x+y)2 z−(x−y)2 /4z.(17)The parameters M R and Γfrom Eq.(14)are the mass of the ’baryon resonance’and full width,respectively.We note that within the resonance description we neglect coherent contributions from the available baryons as well as possible interference terms since we do not have experimental information on their actual magnitude.Within our semiphenomenological approach we now fit the experimental data on ρ,ωand φ-meson production in pion induced reactions in order to extract the mass and width of the ’effective’resonance R .Our fits to the data [48]are shown in Figs.4,5,6with the parameters listed in Tab.1.For convenience,the data are plotted as a functionof √s th ,with s denoting the squared invariant energy of the colliding particles and√s in comparison to the experimental data from [48].The solid linesshow the fit to the LSM results according to Eq.(18)with the parameters from Tab.3,whereas in case of ωproduction the full dots stand for the LSM results.We additionally show the cross sections for exclusive meson production within the One-Boson Exchange Model (OBEM)[50]by the dashed lines in comparison to the respective data from [48](triangles).Whereas in case of K +,ρ,ωproduction the inclusive LSM results smoothly match with the OBEM calculations for the exclusive channels close to threshold,this is no longer the case for φ-meson production (cf.Fig.10)because the string fragmentation model requires the formation of two s ¯s -pairs,which shifts the threshold up to higherenergies.Since there are presently no experimental data available for √σpN −σMN d 2b (exp [−σMN T A (b )]−exp [−σpN T A (b )])(23)with T A (b )denoting the profile function of the target[52].In Eq.(23)the second term is related to the effective number of nucleons (of the target)participating in the interaction with the impinging proton while the first term accounts for the final state reabsorption.As shown in [17,38]N eff from Eq.(23)for σMN ≃0can adequately be parame-terized byN eff =A 0.75±0.01,(24)at bombarding energies above 0.8GeV,where A is again the target mass number.Thus the A -dependence of the direct production mechanism is proportional to (24)whenever the produced meson only weakly interacts with the residual nucleus.In case ofσpN≫σMN we can-assuming a uniform distribution of the nuclear density-reduce the absorption term from(23)to the simple expressionκ=exp[−σMNρ0R A)],(25)whereρ0=0.168fm−3and R A are the average density and the radius of the nucleus, respectively.We note that the factorκis frequently used to describe the reabsorption of the produced particles infinite nuclei in order to account for weak reabsorption effects.However,the application of(25)in case ofρandωproduction is not adequate because the reabsorption cross sectionσMN appearing in(20-25)is no longer small compared toσpN.In order to demonstrate the sensitivity of N eff to the various ap-proximation schemes(20),(23),(25)we show in Fig.11the effective collision number for12C as a function of the reabsorption cross section within the limits of Eq.(20) (solid line),Eq.(23)(dashed line)and Eq.(25)(dotted line)which deviate substan-tially already for absorption cross sections of about30mb.Consequently,we will use Eq.(20)in the following to describe the inclusive production and absorption of mesons in p+A reactions with the absorption cross sections described in Section3.5 We,furthermore,note that by Eq.(20)we only account for reabsorption and ne-glect elastic meson-nucleon rescattering.This is of no special interest when considering inclusive(integrated)cross sections only as in Section4,but will be essential when comparing the Lorentz invariant spectra with experimental data sets in a narrow kine-matic regime.For the latter purpose transport simulations as described in ref.[56] will provide a more adequate description.The calculation of N eff for the two-step reactions with an intermediate pion has been described by Vercellin et al.[54]within the Glauber formalism and is a more involved task.However,as shown in[38],a practicable approximation is to use N eff from Eq.(20)and to include the corrections from the two-step mechanism by a factor gπasgπ=1−1σpNρ0+1The cross sections forρ+N,ω+N andφ+N interactions in case of low relative momenta can be extracted from theπ+N reactions by assuming that this is the dominant absorption channel.Detailed balance provides the relationλ(s,m N,m M)σπ+N→M+N(2Iπ+1)(2I N+1)q2+m2N−ǫ(29) whereǫis again the average binding energy per nucleon.In spite of the quite different assumptions in both approaches,the results for the K+-meson cross sections for one-step and two-step reaction channels are remarkably close to each other.Whereas at energies above0.95GeV both results are practically the same,the folding model with the quasi-free dispersion relation(29)for the target nucleon over-estimates the energy available in the collision and thus leads to slightly higher cross sections than our spectral function approach for both reaction steps at lower bombard-ing energy.Thus the underestimation of the data below about T0=0.92GeV mightbe considered as an evidence for additional many-body effects or further production channels that become important close to the reaction threshold.On the other hand, the dominance of the two-step reaction mechanism is the same within both approaches and can be considered as model independent.Since in the spectral function from[46]we cannot separate the correlated and un-correlated parts,we adopt the spectral function from[27],that allows the separation of the two components.Within the latter parametrization we can study the relative contribution from the correlated part of S P(q,w)for meson production in p+A reac-tions.Our calculated results for K+-meson production in p+12C collisions are shown in Fig.13for the two-step mechanism(solid line)and primary reaction channel(dashed line)in comparison with the experimental data[19].In line with our arguments pre-sented above the contributions from the correlated part of the spectral function indeed are small compared to the data.For more pedagogical reason we also discuss the results for the K+-meson produc-tion cross section when employing the momentum distributionΦ(q),as obtained from integrating the spectral function from[27]over the removal energy,but using the free dispersion relationω=experimental data from[48]for the exclusive and inclusive reactions are described sufficiently well,however,our extrapolations for the various channels close to threshold should be controlled experimentally in the near future.Whereas in previous reaction models only the target momentum distribution has been employed using a quasi-free dispersion relation for the target nucleons,our present formulation is based on the full spectral function S(q,ω)where the nucleon may be far off-shell and high momentum,high removal energy components appear as well.This approach wasfirst used by Debowski,Grosse and Senger[23,24]for proton induced particle production.While those authors applied the method to their own measured differential spectra for K+-meson production on12C and208P b,we have tested our approach for K+production in comparison to the experimental data from Koptev et al.[19]using the spectral function for12C from ref.[46].What emerges from a detailed study of the spectral function is that one should use a quasifree dispersion relation(10)only for nuclear momenta below the Fermi level. For higher values of q a dispersion relation with(11)should be used for the origin of the high momentum component accounting for two-body correlations.It is clearly seen that the two-step mechanism with an intermediate pion gives by far the dominant contribution at subthreshold energies as in the folding model[13], but the actual cross section for K+-meson is underestimated below about920MeV indicating the need for further reaction channels or many-body effects.This result contrasts with the folding model where a better description of the data is achieved. We attribute this to the fact that the folding model-adopting a quasi-free dispersion relation for the target nucleon-overestimates the available energy in nucleon-nucleon collisions when approaching the absolute reaction threshold.On the other hand,when integrating the spectral function over the energy E R, we obtain a momentum distribution showing very high momentum components that can be attributed to short-range nucleon-nucleon or tensor correlations.However,these high momentum components on average are associated with high removal energies,too, such that they cannot be exploited for meson production close to the absolute reaction threshold.Thus,meson production close to threshold does not provide information on the high momentum components infinite nuclei or short-range two-body correlations.Within our approach we have also calculated the cross sections for the vector mesons ρ,ω,φin p+12C collisions from threshold up to a few GeV.We obtain estimates for their production in nuclei without incorporating any selfenergies for the mesons.These cross sections provide a baseline for experiments at COSY or CELSIUS that address the in-medium properties of the vector mesons as well as theirfinal state interaction with nuclei.Here,in context with chiral symmetry restoration,theρandω-mass at normal nuclear densityρ0is expected to be reduced by about18%[8],which will sizeably enhance their production cross section as compared to our calculations for free vector mesons presented above.A detailed study of their production cross section as a function of T0and target mass A might help to separate the effects from the real and imaginary part of the meson selfenergies.The authors acknowledge many fruitful discussions with M.B¨u scher,E.Grosse and B.Kamys.They like to thank,furthermore,I.Sick for providing the12C spectral function in numerical form.References[1]Grimm,P.,Grosse,E.:Prog.Part.Nucl.Phys.15,339(1985)[2]Braun-Munzinger,P.Stachel,J.:Ann.Rev.Nucl.Part.Sci.37,1(1987)[3]Mosel,U.:Ann.Rev.Nucl.Part.Sci.41,29(1991)[4]Jakobsson,B.:Phys.Scripta48,179(1993)[5]Shyam,R.,Knoll,J.:Nucl.Phys.A483,711(1988)[6]Bertsch,G.F.,Das Gupta,S.:Phys.Rep.160,189(1988)[7]Cassing,W.,Metag,V.,Mosel,U.,Niita,K.:Phys.Rep.188,363(1990)[8]Hatsuda,T,Lee,S.:Phys.Rev.C46,R34(1992)[9]Agakichiev,G.,Baur,R.,Breskin,A.,Chechnik,R.,Drees,A.,et al.,Phys.Rev.Lett.75,1272(1995)[10]Masera,M.,et al.:Nucl.Phys.A590,93c(1995)[11]Cassing,W.,Ehehalt,W.,Ko,C.M.:Phys.Lett.B363,35(1995);Cassing,W.,Ehehalt,W.,Kralik,I.:Phys.Lett.B377,5(1996)[12]Li,G.Q.,Ko,C.M.,Brown,G.E.:Phys.Rev.Lett.75,4007(1995)[13]Cassing,W.,Batko,G.,Vetter,T.,Wolf,G.:Z.Phys.A340,51(1991)[14]Cugnon,J.,Lombard,R.M.:Nucl.Phys.A422,635(1984)[15]Golubeva,E.S.,Ilinov,A.S.,Pshenichnov,I.A.:Nucl.Phys.A562,389(1993)[16]M¨u ller,H.,Sistemich,K.:Z.Phys.A344,197(1992)[17]Sibirtsev,A.,B¨u scher,M.:Z.Phys A347,191(1994)[18]Sibirtsev,A.A.:Sov.J.Nucl.Phys.55,729(1992)[19]Koptev,V.P.,Mikirtyhyants,S.M.,Nesterov,M.M.,Tarasov,N.A.,Scherbakov,G.V.,et al.:JETP94,1(1988)[20]Chiavassa,E.,Dellacassa,G.,De Marco,N.,Ferrero,F.,Gallio,M.,et al.:Z.Phys.A344,345(1993)[21]Chiavassa,E.,Dellacassa,G.,De Marco,N.,Ferrero,F.,Musso,M.,et al.:Z.Phys.A342,107(1992)[22]Chiavassa,E.,Dellacassa,G.,De Marco,N.,Ferrero,F.,Gallio,M.,et al.:Nucl.Phys.A538,121(1992)[23]Debowski,M.,Grosse,E.,Senger,P.:Preprint GSI10-95,3(1995)[24]Debowski,M.:Ph.D.Thesis(1995)[25]Sibirtsev,A.,B¨u scher,M.,M¨u ller,H.,Schneidereit,Ch.:Z.Phys.A351,333(1995)[26]Day,D.,McCarthy,J.S.,Meziani,Z.E.,Minehart,R.,Sealock,R.,et al.:Phys.Rev.C48,1849(1993)[27]Ciofidegli Atti,C.,Simula,S.:Phys.Rev.C53,1689(1996)[28]Kerman,A.K.,Manus,H.Mc,Thaler,R.M.:Annals of Phys.8,55(1959)[29]Newton,R.G.:Scattering Theory of Waves and Particles,New York,McCraw-Hill(1966).[30]Jackson,D.F.:Nuclear Reactions,London,Methuen(1970).[31]Satchler,G.R.:Introduction to Nuclear Reactions,Hong Kong,Macmillan Edu-cation LTD(1990)[32]Weber,K.,Bl¨a ttel,B.,Cassing,W.,D¨o nges,H.-C.,Koch,V.,Lang,A.,Mosel,U.:Nucl.Phys.A539,713(1992)[33]Cooper,E.D.:Phys.Rev.C36,2170(1987)[34]Hama,S.,Clark,B.C.,Cooper,E.D.,Sherif,H.S.,Mercer,R.L.:Phys.Rev.C41,2737(1990)[35]Welke,G.M.,Prakash,M.,Kuo,T.T.S.,Das Gupta,S.,Gale,C.:Phys.Rev.C38,2101(1988)[36]Sibirtsev,A.A.:Phys.Scr.RS21,167(1993)[37]Kilian,K.,Nann,H.:Meson production near threshold,ed.H.Nann and E.J.Stephenson,AIP Conf.Proc.221,185(1990)[38]Cassing,W.,Batko,G.,Mosel,U.,Niita,K.,Schult,O.,Wolf,Gy.:Phys.Lett.B238,25(1990)[39]Cugnon,J.,Lemaire,M.-C.:Nucl.Phys.A489,781(1988)[40]Sibirtsev,A.,Tsushima,K.,Faessler,A.:Z.Phys.A354,215(1996)[41]Guet,C.,Prakash,M.:Nucl.Phys.A428,119(1984)[42]Frullani,S.,Mougey,J.:Adv.Nucl.Phys.14,7(1984)[43]Benhar,O.,Fabrocini,A.,Fantoni,S.:Nucl.Phys.A505,267(1989)[44]Mahaux,C.,Sartor,R.:Nucl.Phys.A546,65(1992)[45]Ciofidegli Atti,C.,Pace,E.,Salme,G.:Phys.Rev.C21,805(1980)[46]Sick,I.,Fantoni,S.,Fabrocini,A.,Benhar,O.:Phys.Lett.B323,267(1994)andprivate communication.[47]Tsushima,K.,Huang,S.W.,Faessler,A.:Phys.Lett.B337,245(1994)[48]Landolt-B¨o rnstein,New Series,ed H.Schopper,I/12(1988)[49]Nilsson-Almqvist,B.,Stenlund,E.:m.43,387(1987)[50]Sibirtsev,A.A.:to be pub.in Nucl.Phys.A.[51]Margolis,B.:Phys.Lett.B26,524(1968)[52]Glauber,R.J.,Mathiae,J.:Nucl.Phys.,B21,135(1970)[53]Margolis,B.:Nucl.Phys.B4,433(1968)[54]Vercellin,E.,Chiavassa,E.,Dellacasa,G.,et al.:Nuovo Cimento A106,861(1993)[55]Knoll,J.,Randrup,J.:Nucl.Phys.A324,445(1979)[56]Rudy,Z.,Cassing,W.,Demski,T.,Jarczyk,L.,Kamys,B.,et al.:Z.Phys.A351,217(1995)[57]Sakurai,J.J.:Ann.Phys.(NY)11,1(1960)[58]Joos,H.:Phys.Lett.B24,103(1967)[59]Kajantie,K.,Trefil,J.S.:Phys.Lett.B24,106(1967)[60]Christillin,P.:Phys.Rep.190,64(1990)[61]Sibirtsev,A.,Dolgolenko,A.G.:to be pub.in Sov.J.Nucl.Phys.(1996)Table1:The effective mass and width of the baryonic resonance in Eq.(14),as well as B=B in×B out×(2J+1).MesonΓ(GeV)ρ00.991.809302φ0.99s th(GeV2)bρ0 3.6 1.25ω 4.8 1.26φ(1)0.09 2.1Table3:The parameters of Eq.(18)for proton induced reactions.Here the index(1) implies a shift x→x−1.3.Meson a(mb)c6.49 1.477.01 1.477.06 1.478.38 2.545.88 1.47Figure1:The spectral function S(q,E R)for12C from[46].The dashed line shows the relation(11).The contour lines decrease by a factor of1.5from line to line.Figure2:Saturation of the momentum sum rule for12C.The lines indicate the func-tion(12)integrated up to energy E max=30MeV(dashed),100MeV(dotted),200MeV (dash-dotted)and500MeV(solid line).The arrow indicates the Fermi momentum. Figure3:The momentum distribution for3He,12C,and208P b according to[27].The dashed lines result from the uncorrelated part of the spectral function.The dotted lines indicate(12)for the correlated spectral function integrated up to E max=30MeV and the dashed-dotted lines up to E max=100MeV.The solid lines display the sum of the uncorrelated and correlated parts for E max→∞.Figure4:Experimental cross sections for the exclusive reactionπ++p→ρ++p (triangles)and the inclusive reactionπ++p→ρo+X(full dots)from[48].The lines represent our parametrizations as discussed in the text.Figure5:Experimental cross sections for the exclusive reactionsπ++n→ω+p (squares)andπ−+p→ω+n(triangles)and the inclusive reactionπ++p→ω+X(full dots).Experimental data are taken from[48].The lines represent our parametrizations as discussed in the text.Figure6:Experimental cross sections for the exclusive reactionsπ++n→φ+p (squares)andπ−+p→φ+n(triangles)and inclusive reactionπ++p→φ+X(dots) from[48].The lines represent our parametrizations as discussed in the text.Figure7:Cross sections for the exclusive reaction p+p→K++Λ+p(triangles) and the inclusive reaction p+p→K++X(dots)from[48].The solid line shows the calculation within the LSM,while the dashed line refers to the OBEM.Figure8:Cross sections for the exclusive reaction p+p→ρo+p+p(triangles)and the inclusive reaction p+p→ρo+X(dots)from[48].The solid line shows the calculation within the LSM,while the dashed line refers to the OBEM.Figure9:Experimental cross sections for the exclusive reaction p+p→ω+p+p (triangles)from[48]in comparison to the OBEM calculation(dashed line).The full dots represent the results from LSM for the inclusive reaction p+p→ω+X,while the solid line shows ourfit with Eq.16.Figure10:Cross sections for the exclusive reaction p+p→φ+p+p(triangles)and the inclusive reaction p+p→φ+X(dots)from[48].The solid line shows the calculation within the LSM and the dashed line our results within the OBEM for the exclusive channel.Figure11:The effective collision number calculated with(20)-solid,(23)-dashed and with(25)-dotted line as a function of the meson absorption cross sectionσin mb.Figure12:Cross section of K+-meson production for p+12C as a function of the beam energy T0.The solid and dashed lines show the calculations with the total spectral function for the two-step and direct reaction mechanism,respectively,employing the spectral function from[46].The dotted and dashed-dotted lines show the calculations with mean-field approach(dashed curve in Fig.3)for the two-step and direct reaction mechanism,respectively,employing a quasifree dispersion relation.The dots show the experimental data from[19].The solid and the dotted lines were calculated with the same elementary cross sections.Figure13:Cross section of K+-meson production for p+12C as a function of the beam energy T0.The solid and dashed lines are results using only the correlated part of the spectral function from[27]for the two-step and direct reaction mechanism,respectively. The dotted and dashed-dotted lines show the on-shell calculations for the two-step and direct reaction mechanism,respectively.Experimental data are from[19].Figure14:Cross section forρ,ωandφ-meson production in p+12C reactions as a function of the beam energy T0.The solid and dashed lines show the calculations with the total spectral function[46]for the two-step and direct reaction mechanism, respectively.。