201004 International Journal of Minerals Metallurgy and Materials
基于Bayesian多分支岩石可钻性值估计
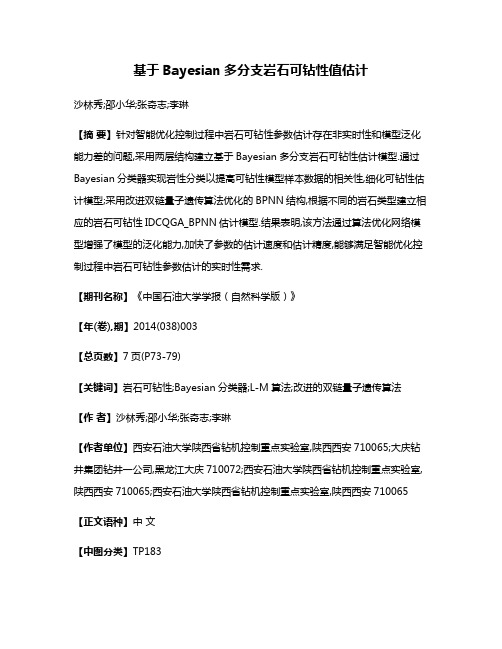
基于Bayesian多分支岩石可钻性值估计沙林秀;邵小华;张奇志;李琳【摘要】针对智能优化控制过程中岩石可钻性参数估计存在非实时性和模型泛化能力差的问题,采用两层结构建立基于Bayesian多分支岩石可钻性估计模型.通过Bayesian分类器实现岩性分类以提高可钻性模型样本数据的相关性,细化可钻性估计模型;采用改进双链量子遗传算法优化的BPNN结构,根据不同的岩石类型建立相应的岩石可钻性IDCQGA_BPNN估计模型.结果表明,该方法通过算法优化网络模型增强了模型的泛化能力,加快了参数的估计速度和估计精度,能够满足智能优化控制过程中岩石可钻性参数估计的实时性需求.【期刊名称】《中国石油大学学报(自然科学版)》【年(卷),期】2014(038)003【总页数】7页(P73-79)【关键词】岩石可钻性;Bayesian分类器;L-M算法;改进的双链量子遗传算法【作者】沙林秀;邵小华;张奇志;李琳【作者单位】西安石油大学陕西省钻机控制重点实验室,陕西西安710065;大庆钻井集团钻井一公司,黑龙江大庆710072;西安石油大学陕西省钻机控制重点实验室,陕西西安710065;西安石油大学陕西省钻机控制重点实验室,陕西西安710065【正文语种】中文【中图分类】TP183岩石可钻性是指在一定的外力和钻具条件下岩石抵抗钻头破坏的能力。
它表征岩石抗钻强度,是岩石物理性质在钻进时的综合表现。
岩石可钻性估计是钻头选型和钻进参数设计以及合理选择钻进方式、提高钻井效率、降低成本和减小钻头磨损等优化决策的前提。
因而在钻进过程中,如何实现岩石可钻性的实时估计,为钻井参数的动态优化和优化控制提供依据,是智能自动送钻技术的重要环节之一。
目前,岩石可钻性表示方法[1-3]主要有岩石物理力学性质表示法、微钻速度法、分形理论法[4-6]等。
然而,利用岩石物理力学性质分析可钻性受地层、钻头类型及钻进参数的影响,且条件性很强;微钻法滞后于实际钻进,不能随钻随测,周期长,费用高;分形法可避免传统方法的滞后性、周期长、费用高等不足。
历年公布最新JCR分区表

是否为TOP期刊刊名简称刊名全称ISSN大类名称复分大类分区1941-1405地学1YANNU REV MAR SCI Annual Review of Marin1680-7316地学1Y ATMOS CHEM PHYS ATMOSPHERIC CHEMISTRY0003-0007地学1YB AM METEOROL SOC BULLETIN OF THE AMERICCLIM DYNAM CLIMATE DYNAMICS0930-7575地学1Y CRYOSPHERE Cryosphere1994-0416地学1Y0012-821X地学1Y EARTH PLANET SC LETTEARTH AND PLANETARY SCEARTH-SCI REV EARTH-SCIENCE REVIEWS0012-8252地学1Y0016-7037地学1Y GEOCHIM COSMOCHIM ACGEOCHIMICA ET COSMOCHIGEOLOGY GEOLOGY0091-7613地学1Y GONDWANA RES GONDWANA RESEARCH1342-937X地学1YJ CLIMATE JOURNAL OF CLIMATE0894-8755地学1Y0263-4929地学1YJ METAMORPH GEOL JOURNAL OF METAMORPHICJ PETROL JOURNAL OF PETROLOGY0022-3530地学1YNAT GEOSCI Nature Geoscience1752-0894地学1Y PALEOCEANOGRAPHY PALEOCEANOGRAPHY0883-8305地学1Y PRECAMBRIAN RES PRECAMBRIAN RESEARCH0301-9268地学1Y0277-3791地学1Y QUATERNARY SCI REV QUATERNARY SCIENCE REVREV GEOPHYS REVIEWS OF GEOPHYSICS8755-1209地学1Y0002-9599地学2NAM J SCI AMERICAN JOURNAL OF SCAPPL CLAY SCI APPLIED CLAY SCIENCE0169-1317地学2N1867-1381地学2N ATMOS MEAS TECH Atmospheric Measuremen0258-8900地学2NB VOLCANOL BULLETIN OF VOLCANOLOGBASIN RES BASIN RESEARCH0950-091X地学2N BOREAS BOREAS0300-9483地学2N BOUND-LAY METEOROL BOUNDARY-LAYER METEORO0006-8314地学2N CHEM GEOL CHEMICAL GEOLOGY0009-2541地学2NCLIM PAST Climate of the Past 1814-9324地学2N CONTRIB MINERAL PETRCONTRIBUTIONS TO MINER0010-7999地学2N0967-0637地学2NDEEP-SEA RES PT I DEEP-SEA RESEARCH PART0377-0265地学2N DYNAM ATMOS OCEANS DYNAMICS OF ATMOSPHEREELEMENTS Elements1811-5209地学2N1525-2027地学2N GEOCHEM GEOPHY GEOSYGEOCHEMISTRY GEOPHYSIC0016-7606地学2NGEOL SOC AM BULL GEOLOGICAL SOCIETY OFGEOMORPHOLOGY GEOMORPHOLOGY0169-555X地学2N0956-540X地学2N GEOPHYS J INT GEOPHYSICAL JOURNAL IN1639-4488地学2N GEOSTAND GEOANAL RESGEOSTANDARDS AND GEOAN0266-1144地学2N GEOTEXT GEOMEMBRANESGEOTEXTILES AND GEOMEMHOLOCENE HOLOCENE0959-6836地学2N1027-5606地学2N HYDROL EARTH SYST SCHYDROLOGY AND EARTH SYINT J CLIMATOL INTERNATIONAL JOURNAL0899-8418地学2N1437-3254地学2NINT J EARTH SCI INTERNATIONAL JOURNALJ GEOL JOURNAL OF GEOLOGY0022-1376地学2NJ GEOL SOC LONDON JOURNAL OF THE GEOLOGI0016-7649地学2N1525-755X地学2NJ HYDROMETEOROL JOURNAL OF HYDROMETEOR0924-7963地学2NJ MARINE SYST JOURNAL OF MARINE SYST0921-2728地学2NJ PALEOLIMNOL JOURNAL OF PALEOLIMNOL0022-3670地学2NJ PHYS OCEANOGR JOURNAL OF PHYSICAL OC0267-8179地学2NJ QUATERNARY SCI JOURNAL OF QUATERNARY1477-2019地学2NJ SYST PALAEONTOL JOURNAL OF SYSTEMATICLITHOS LITHOS0024-4937地学2N MAR GEOL MARINE GEOLOGY0025-3227地学2N0377-8398地学2N MAR MICROPALEONTOL MARINE MICROPALEONTOLO0027-0644地学2N MON WEATHER REV MONTHLY WEATHER REVIEWOCEAN MODEL OCEAN MODELLING1463-5003地学2N ORE GEOL REV ORE GEOLOGY REVIEWS0169-1368地学2N ORG GEOCHEM ORGANIC GEOCHEMISTRY0146-6380地学2N PALAEOGEOGR PALAEOCLPALAEOGEOGRAPHY PALAEO0031-0182地学2N PALEOBIOLOGY PALEOBIOLOGY0094-8373地学2N0079-6611地学2N PROG OCEANOGR PROGRESS IN OCEANOGRAP0309-1333地学2N PROG PHYS GEOG PROGRESS IN PHYSICAL G0035-9009地学2N Q J ROY METEOR SOC QUARTERLY JOURNAL OF T1871-1014地学2N QUAT GEOCHRONOL Quaternary GeochronoloQUATERNARY RES QUATERNARY RESEARCH0033-5894地学2N1529-6466地学2N REV MINERAL GEOCHEM REVIEWS IN MINERALOGYSURV GEOPHYS SURVEYS IN GEOPHYSICS0169-3298地学2N TECTONICS TECTONICS0278-7407地学2N0280-6509地学2N TELLUS B TELLUS SERIES B-CHEMIC0094-8276地学2Y GEOPHYS RES LETT GEOPHYSICAL RESEARCH L0022-4928地学2Y J ATMOS SCI JOURNAL OF THE ATMOSPH0148-0227地学2Y J GEOPHYS RES JOURNAL OF GEOPHYSICALJ HYDROL JOURNAL OF HYDROLOGY0022-1694地学2Y LIMNOL OCEANOGR LIMNOLOGY AND OCEANOGR0024-3590地学2Y1000-9515地学3N ACTA GEOL SIN-ENGL ACTA GEOLOGICA SINICA-0567-7920地学3N ACTA PALAEONTOL POL ACTA PALAEONTOLOGICA PAM MINERAL AMERICAN MINERALOGIST0003-004X地学3N ANN GEOPHYS-GERMANY ANNALES GEOPHYSICAE0992-7689地学3N ANTARCT SCI ANTARCTIC SCIENCE0954-1020地学3N APPL GEOCHEM APPLIED GEOCHEMISTRY0883-2927地学3N ARCHAEOMETRY ARCHAEOMETRY0003-813X地学3N ARCT ANTARCT ALP RESARCTIC ANTARCTIC AND A1523-0430地学3N ATMOS RES ATMOSPHERIC RESEARCH0169-8095地学3N1530-261X地学3N ATMOS SCI LETT Atmospheric Science Le0812-0099地学3N AUST J EARTH SCI AUSTRALIAN JOURNAL OF0037-1106地学3N B SEISMOL SOC AM BULLETIN OF THE SEISMO0009-2819地学3N CHEM ERDE-GEOCHEM CHEMIE DER ERDE-GEOCHE0009-8604地学3N CLAY CLAY MINER CLAYS AND CLAY MINERALCLIM RES CLIMATE RESEARCH0936-577X地学3N0278-4343地学3N CONT SHELF RES CONTINENTAL SHELF RESE0967-0645地学3N DEEP-SEA RES PT II DEEP-SEA RESEARCH PARTEARTH INTERACT Earth Interactions 1087-3562地学3N0197-9337地学3N EARTH SURF PROC LANDEARTH SURFACE PROCESSEECON GEOL ECONOMIC GEOLOGY0361-0128地学3N EPISODES EPISODES0705-3797地学3N ESTUAR COAST SHELF SESTUARINE COASTAL AND0272-7714地学3N0935-1221地学3N EUR J MINERAL EUROPEAN JOURNAL OF MIFACIES FACIES0172-9179地学3N GEOARABIA GEOARABIA1025-6059地学3N1467-4866地学3N GEOCHEM T GEOCHEMICAL TRANSACTIOGEOFLUIDS GEOFLUIDS1468-8115地学3NGEOL ACTA GEOLOGICA ACTA 1695-6133地学3N GEOL J GEOLOGICAL JOURNAL0072-1050地学3N GEOL MAG GEOLOGICAL MAGAZINE0016-7568地学3N GEO-MAR LETT GEO-MARINE LETTERS0276-0460地学3N0016-8025地学3N GEOPHYS PROSPECT GEOPHYSICAL PROSPECTINGEOPHYSICS GEOPHYSICS0016-8033地学3N1991-959X地学3N GEOSCI MODEL DEV Geoscientific Model DeGEOSPHERE Geosphere1553-040X地学3N HELGOLAND MAR RES HELGOLAND MARINE RESEA1438-387X地学3N0020-6814地学3N INT GEOL REV INTERNATIONAL GEOLOGY0303-2434地学3N INT J APPL EARTH OBSInternational JournalINT J BIOMETEOROL INTERNATIONAL JOURNAL0020-7128地学3N1365-8816地学3N INT J GEOGR INF SCI INTERNATIONAL JOURNALINT J SPELEOL INTERNATIONAL JOURNAL0392-6672地学3N1558-8424地学3N J APPL METEOROL CLIMJournal of Applied Met0305-4403地学3N J ARCHAEOL SCI JOURNAL OF ARCHAEOLOGIJ ASIAN EARTH SCI JOURNAL OF ASIAN EARTH1367-9120地学3N0739-0572地学3N J ATMOS OCEAN TECH JOURNAL OF ATMOSPHERIC1364-6826地学3N J ATMOS SOL-TERR PHYJOURNAL OF ATMOSPHERIC0375-6742地学3N J GEOCHEM EXPLOR JOURNAL OF GEOCHEMICALJ GEODESY JOURNAL OF GEODESY0949-7714地学3N0264-3707地学3N J GEODYN JOURNAL OF GEODYNAMICSJ GLACIOL JOURNAL OF GLACIOLOGY0022-1430地学3N0022-2402地学3N J MAR RES JOURNAL OF MARINE RESE0895-9811地学3N J S AM EARTH SCI JOURNAL OF SOUTH AMERI1385-1101地学3N J SEA RES JOURNAL OF SEA RESEARC1527-1404地学3N J SEDIMENT RES JOURNAL OF SEDIMENTARY0191-8141地学3N J STRUCT GEOL JOURNAL OF STRUCTURAL0272-4634地学3N J VERTEBR PALEONTOL JOURNAL OF VERTEBRATEJ VOLCANOL GEOTH RESJOURNAL OF VOLCANOLOGY0377-0273地学3N JOKULL Jokull0449-0576地学3N LANDSLIDES Landslides 1612-510X地学3N LETHAIA LETHAIA0024-1164地学3N1541-5856地学3N LIMNOL OCEANOGR-METHLIMNOLOGY AND OCEANOGRLITHOSPHERE-US Lithosphere1941-8264地学3N0264-8172地学3N MAR PETROL GEOL MARINE AND PETROLEUM GMINER DEPOSITA MINERALIUM DEPOSITA0026-4598地学3N1561-8633地学3N NAT HAZARD EARTH SYSNATURAL HAZARDS AND EAOCEAN DYNAM OCEAN DYNAMICS1616-7341地学3N OCEANOGRAPHY OCEANOGRAPHY 1042-8275地学3N PALAEONTOLOGY PALAEONTOLOGY0031-0239地学3N PALAIOS PALAIOS0883-1351地学3N1045-6740地学3N PERMAFROST PERIGLAC PERMAFROST AND PERIGLA0099-1112地学3N PHOTOGRAMM ENG REM SPHOTOGRAMMETRIC ENGINEPHYS CHEM MINER PHYSICS AND CHEMISTRY0342-1791地学3N1040-6182地学3N QUATERN INT QUATERNARY INTERNATIONRADIOCARBON RADIOCARBON0033-8222地学3N REV PALAEOBOT PALYNOREVIEW OF PALAEOBOTANY0034-6667地学3N SEDIMENT GEOL SEDIMENTARY GEOLOGY0037-0738地学3N SEDIMENTOLOGY SEDIMENTOLOGY0037-0746地学3NSEISMOL RES LETT SEISMOLOGICAL RESEARCH0895-0695地学3N0038-6804地学3N SPEC PAP PALAEONTOL SPECIAL PAPERS IN PALASTRATIGRAPHY Stratigraphy 1547-139X地学3N1661-8726地学3N SWISS J GEOSCI Swiss Journal of GeoscTECTONOPHYSICS TECTONOPHYSICS0040-1951地学3N0280-6495地学3N TELLUS A TELLUS SERIES A-DYNAMITERRA NOVA TERRA NOVA0954-4879地学3N THEOR APPL CLIMATOL THEORETICAL AND APPLIE0177-798X地学3N0882-8156地学3N WEATHER FORECAST WEATHER AND FORECASTINACTA CARSOLOGICA ACTA CARSOLOGICA 0583-6050地学4N ACTA GEOD GEOPHYS HU Acta Geodaetica et Geo1217-8977地学4N1214-9705地学4N ACTA GEODYN GEOMATER Acta Geodynamica et Ge1581-6613地学4N ACTA GEOGR SLOV Acta Geographica SloveACTA GEOL POL ACTA GEOLOGICA POLONIC0001-5709地学4N ACTA GEOPHYS Acta Geophysica1895-6572地学4N1854-0171地学4N ACTA GEOTECH SLOV Acta Geotechnica Slove0894-0525地学4N ACTA METEOROL SIN Acta Meteorologica Sin1335-1788地学4N ACTA MONTAN SLOVACA Acta Montanistica Slov0253-505X地学4N ACTA OCEANOL SIN ACTA OCEANOLOGICA SINI0256-1530地学4N ADV ATMOS SCI ADVANCES IN ATMOSPHERI0065-2687地学4N ADV GEOPHYS ADVANCES IN GEOPHYSICSALCHERINGA ALCHERINGA0311-5518地学4N AMEGHINIANA AMEGHINIANA0002-7014地学4N ANDEAN GEOL Andean Geology0718-7092地学4N ANN GEOPHYS-ITALY ANNALS OF GEOPHYSICS1593-5213地学4N0003-4088地学4N ANN LIMNOL-INT J LIMANNALES DE LIMNOLOGIE-0753-3969地学4N ANN PALEONTOL ANNALES DE PALEONTOLOG0208-9068地学4N ANN SOC GEOL POL ANNALES SOCIETATIS GEOAPPL GEOPHYS Applied Geophysics1672-7975地学4N0141-1187地学4N APPL OCEAN RES APPLIED OCEAN RESEARCHAQUAT GEOCHEM AQUATIC GEOCHEMISTRY1380-6165地学4N1866-7511地学4N ARAB J GEOSCI Arabian Journal of GeoARCHAEOL PROSPECT Archaeological Prospec1075-2196地学4N ARCTIC ARCTIC0004-0843地学4N1976-7633地学4N ASIA-PAC J ATMOS SCIAsia-Pacific Journal oATMOS OCEAN ATMOSPHERE-OCEAN0705-5900地学4N ATMOSFERA ATMOSFERA0187-6236地学4N1836-716X地学4N AUST METEOROL OCEAN Australian Meteorologi0251-7493地学4N AUSTRIAN J EARTH SCIAustrian Journal of Ea0007-4802地学4N B CAN PETROL GEOL BULLETIN OF CANADIAN P1413-4853地学4N B CIENC GEOD Boletim de Ciencias Ge0006-6729地学4N B GEOFIS TEOR APPL Bollettino di Geofisic0011-6297地学4N B GEOL SOC DENMARK BULLETIN OF THE GEOLOG0367-5211地学4N B GEOL SOC FINLAND BULLETIN OF THE GEOLOG1214-1119地学4N B GEOSCI BULLETIN OF GEOSCIENCE0007-4977地学4N B MAR SCI BULLETIN OF MARINE SCI0037-9409地学4N B SOC GEOL FR BULLETIN DE LA SOCIETE0375-7633地学4N B SOC PALEONTOL ITALBOLLETTINO DELLA SOCIEBALTICA Baltica 0067-3064地学4N1679-8759地学4N BRAZ J OCEANOGR BRAZILIAN JOURNAL OF O0008-3674地学4N CAN GEOTECH J CANADIAN GEOTECHNICAL0008-4077地学4N CAN J EARTH SCI CANADIAN JOURNAL OF EACAN MINERAL CANADIAN MINERALOGIST0008-4476地学4N0891-2556地学4N CARBONATE EVAPORITE CARBONATES AND EVAPORI0254-4059地学4N CHIN J OCEANOL LIMN CHINESE JOURNAL OF OCE0001-5733地学4N CHINESE J GEOPHYS-CHCHINESE JOURNAL OF GEOCLAY MINER CLAY MINERALS0009-8558地学4N0098-3004地学4N COMPUT GEOSCI-UK COMPUTERS & GEOSCIENCECOMPUT GEOTECH COMPUTERS AND GEOTECHN0266-352X地学4N1420-0597地学4N COMPUTAT GEOSCI COMPUTATIONAL GEOSCIEN1631-0713地学4N CR GEOSCI COMPTES RENDUS GEOSCIE1631-0683地学4N CR PALEVOL COMPTES RENDUS PALEVOLCRETACEOUS RES CRETACEOUS RESEARCH0195-6671地学4N DISASTER ADV Disaster Advances 0974-262X地学4N DOKL EARTH SCI DOKLADY EARTH SCIENCES1028-334X地学4N1755-6910地学4N EARTH ENV SCI T R SOEarth and Environmenta0736-623X地学4N EARTH SCI HIST EARTH SCIENCES HISTORY1865-0473地学4N EARTH SCI INFORM Earth Science Informat1794-6190地学4N EARTH SCI RES J Earth Sciences ResearcENG GEOL ENGINEERING GEOLOGY0013-7952地学4N1078-7275地学4N ENVIRON ENG GEOSCI ENVIRONMENTAL & ENGINEERDE ERDE0013-9998地学4N ERDKUNDE Erdkunde0014-0015地学4N1736-4728地学4N EST J EARTH SCI Estonian Journal of Ea0367-0449地学4N ESTUD GEOL-MADRID ESTUDIOS GEOLOGICOS-MA0812-3985地学4N EXPLOR GEOPHYS Exploration Geophysics1863-9135地学4N FUND APPL LIMNOL Fundamental and ApplieGEMS GEMOL GEMS & GEMOLOGY0016-626X地学4N0883-6353地学4N GEOARCHAEOLOGY GEOARCHAEOLOGY-AN INTEGEOBIOS-LYON GEOBIOS0016-6995地学4N0016-7029地学4N GEOCHEM INT+GEOCHEMISTRY INTERNATIGEOCHEM J GEOCHEMICAL JOURNAL0016-7002地学4N1467-7873地学4N GEOCHEM-EXPLOR ENV AGEOCHEMISTRY-EXPLORATIGEOCHRONOMETRIA GEOCHRONOMETRIA1733-8387地学4N GEOD LIST Geodetski List0016-710X地学4N GEODIN ACTA GEODINAMICA ACTA0985-3111地学4N GEODIVERSITAS GEODIVERSITAS1280-9659地学4N GEOFIS INT Geofisica Internaciona0016-7169地学4N GEOFIZIKA Geofizika 0352-3659地学4N0435-3676地学4N GEOGR ANN A GEOGRAFISKA ANNALER SE0391-9838地学4N GEOGR FIS DIN QUAT Geografia Fisica e DinGEOINFORMATICA GEOINFORMATICA1384-6175地学4N GEOL BELG GEOLOGICA BELGICA1374-8505地学4N GEOL CARPATH GEOLOGICA CARPATHICA1335-0552地学4N GEOL ORE DEPOSIT+GEOLOGY OF ORE DEPOSIT1075-7015地学4N GEOL Q GEOLOGICAL QUARTERLY1641-7291地学4N1811-4598地学4N GEOL SURV DEN GREENLGEOLOGICAL SURVEY OF D1266-5304地学4N GEOMORPHOLOGIE Geomorphologie-ReliefGEOSCI CAN GEOSCIENCE CANADA0315-0941地学4N GEOSCI J GEOSCIENCES JOURNAL1226-4806地学4N1072-6349地学4N GEOSYNTH INT GEOSYNTHETICS INTERNATGEOTECTONICS+GEOTECTONICS0016-8521地学4N GFF GFF1103-5897地学4N1548-1603地学4N GISCI REMOTE SENS GIScience & Remote SenHIMAL GEOL HIMALAYAN GEOLOGY0971-8966地学4N HYDROGEOL J HYDROGEOLOGY JOURNAL1431-2174地学4N IDOJARAS Idojaras0324-6329地学4N1939-1404地学4N IEEE J-STARS IEEE Journal of Select0379-5136地学4N INDIAN J MAR SCI INDIAN JOURNAL OF MARI1753-8947地学4N INT J DIGIT EARTH International JournalISL ARC ISLAND ARC1038-4871地学4N ITAL J GEOSCI Italian Journal of Geo2038-1719地学4N0001-4338地学4N IZV ATMOS OCEAN PHY+IZVESTIYA ATMOSPHERIC1069-3513地学4N IZV-PHYS SOLID EART+IZVESTIYA-PHYSICS OF T1464-343X地学4N J AFR EARTH SCI JOURNAL OF AFRICAN EAR0926-9851地学4N J APPL GEOPHYS JOURNAL OF APPLIED GEO0167-7764地学4N J ATMOS CHEM JOURNAL OF ATMOSPHERIC1090-6924地学4N J CAVE KARST STUD JOURNAL OF CAVE AND KA0749-0208地学4N J COASTAL RES JOURNAL OF COASTAL RES1674-487X地学4N J EARTH SCI-CHINA Journal of Earth Scien0253-4126地学4N J EARTH SYST SCI Journal of Earth Syste1793-4311地学4N J EARTHQ TSUNAMI Journal of EarthquakeJ ENVIRON ENG GEOPH JOURNAL OF ENVIRONMENT1083-1363地学4N0096-1191地学4N J FORAMIN RES JOURNAL OF FORAMINIFER1009-637X地学4N J GEOGR SCI Journal of Geographica0016-7622地学4N J GEOL SOC INDIA JOURNAL OF THE GEOLOGI1802-6222地学4N J GEOSCI-CZECH Journal of Geosciences1698-6180地学4N J IBER GEOL JOURNAL OF IBERIAN GEOJ LIMNOL JOURNAL OF LIMNOLOGY1129-5767地学4N J MAPS Journal of Maps1744-5647地学4N0026-1165地学4N J METEOROL SOC JPN JOURNAL OF THE METEORO1345-6296地学4N J MINER PETROL SCI Journal of Mineralogic0916-8370地学4N J OCEANOGR JOURNAL OF OCEANOGRAPH1755-876X地学4N J OPER OCEANOGR Journal of OperationalJ PALEONTOL JOURNAL OF PALEONTOLOG0022-3360地学4N0141-6421地学4N J PETROL GEOL JOURNAL OF PETROLEUM G0963-0651地学4N J SEISM EXPLOR JOURNAL OF SEISMIC EXPJ SEISMOL JOURNAL OF SEISMOLOGY1383-4649地学4N0718-9508地学4N J SOIL SCI PLANT NUTJournal of Soil Scienc1449-8596地学4N J SPAT SCI Journal of Spatial Sci1006-8775地学4N J TROP METEOROL Journal of Tropical Me0742-0463地学4N J VOLCANOL SEISMOL+Journal of VolcanologyLIMNOLOGY LIMNOLOGY1439-8621地学4N0024-4902地学4N LITHOL MINER RESOUR+LITHOLOGY AND MINERALMAR GEOD MARINE GEODESY 0149-0419地学4N0025-3235地学4N MAR GEOPHYS RES MARINE GEOPHYSICAL RES1874-8961地学4N MATH GEOSCI Mathematical GeosciencMAUSAM Mausam 0252-9416地学4N1350-4827地学4N METEOROL APPL METEOROLOGICAL APPLICA0177-7971地学4N METEOROL ATMOS PHYS METEOROLOGY AND ATMOSPMETEOROL Z METEOROLOGISCHE ZEITSC0941-2948地学4N MICROPALEONTOLOGY MICROPALEONTOLOGY0026-2803地学4N0930-0708地学4N MINER PETROL MINERALOGY AND PETROLO0026-461X地学4N MINERAL MAG MINERALOGICAL MAGAZINE0276-4741地学4N MT RES DEV MOUNTAIN RESEARCH AND1569-4445地学4N NEAR SURF GEOPHYS Near Surface Geophysic0016-7746地学4N NETH J GEOSCI NETHERLANDS JOURNAL OF0077-7749地学4N NEUES JAHRB GEOL P-ANEUES JAHRBUCH FUR GEO0077-7757地学4N NEUES JB MINER ABH NEUES JAHRBUCH FUR MIN0028-8306地学4N NEW ZEAL J GEOL GEOPNEW ZEALAND JOURNAL OF0078-0421地学4N NEWSL STRATIGR NEWSLETTERS ON STRATIG1023-5809地学4N NONLINEAR PROC GEOPHNONLINEAR PROCESSES IN0029-196X地学4N NORW J GEOL NORWEGIAN JOURNAL OF GOCEAN SCI Ocean Science1812-0784地学4N1730-413X地学4N OCEANOL HYDROBIOL STOCEANOLOGICAL AND HYDROCEANOLOGIA OCEANOLOGIA0078-3234地学4N OCEANOLOGY+OCEANOLOGY0001-4370地学4N OFIOLITI OFIOLITI0391-2612地学4N P GEOLOGIST ASSOC PROCEEDINGS OF THE GEO0016-7878地学4N0044-0604地学4N P YORKS GEOL SOC PROCEEDINGS OF THE YOR0375-0442地学4N PALAEONTOGR ABT A PALAEONTOGRAPHICA ABTEPALAEONTOGR ABT B PALAEONTOGRAPHICA ABTE0375-0299地学4N1094-8074地学4N PALAEONTOL ELECTRON PALAEONTOLOGIA ELECTRO0031-0220地学4N PALAEONTOL Z Palaeontologische Zeit0031-0301地学4N PALEONTOL J+PALEONTOLOGICAL JOURNAPALYNOLOGY PALYNOLOGY0191-6122地学4N0369-8963地学4N PERIOD MINERAL Periodico di MineralogPETROL GEOSCI PETROLEUM GEOSCIENCE1354-0793地学4N PETROL SCI Petroleum Science1672-5107地学4N PETROLOGY+PETROLOGY0869-5911地学4N PETROPHYSICS Petrophysics 1529-9074地学4N1432-8364地学4N PHOTOGRAMM FERNERKUNPhotogrammetrie FernerPHOTOGRAMM REC PHOTOGRAMMETRIC RECORD0031-868X地学4N1474-7065地学4N PHYS CHEM EARTH PHYSICS AND CHEMISTRYPHYS GEOGR PHYSICAL GEOGRAPHY0272-3646地学4N POL POLAR RES POLISH POLAR RESEARCH0138-0338地学4N POLAR RES POLAR RESEARCH0800-0395地学4N0033-4553地学4N PURE APPL GEOPHYS PURE AND APPLIED GEOPH1470-9236地学4N Q J ENG GEOL HYDROGEQUARTERLY JOURNAL OF EQUATERNAIRE QUATERNAIRE1142-2904地学4N RESOUR GEOL RESOURCE GEOLOGY1344-1698地学4N1026-8774地学4N REV MEX CIENC GEOL REVISTA MEXICANA DE CI0035-6883地学4N RIV ITAL PALEONTOL SRIVISTA ITALIANA DI PA1129-8596地学4N RIV ITAL TELERILEVAMRivista Italiana di Te1068-7971地学4N RUSS GEOL GEOPHYS+Russian Geology and Ge1819-7140地学4N RUSS J PAC GEOL Russian Journal of Pac1068-3739地学4N RUSS METEOROL HYDRO+Russian Meteorology an1012-0750地学4N S AFR J GEOL SOUTH AFRICAN JOURNALSCI CHINA EARTH SCI Science China-Earth Sc1674-7313地学4N0036-9276地学4N SCOT J GEOL SCOTTISH JOURNAL OF GESOLA SOLA 1349-6476地学4N0869-5938地学4N STRATIGR GEO CORREL+STRATIGRAPHY AND GEOLO0039-3169地学4N STUD GEOPHYS GEOD STUDIA GEOPHYSICA ET GSURV REV SURVEY REVIEW0039-6265地学4N1017-0839地学4N TERR ATMOS OCEAN SCITERRESTRIAL ATMOSPHERI1300-0985地学4N TURK J EARTH SCI TURKISH JOURNAL OF EARWEATHER Weather0043-1656地学4N1860-1804地学4N Z DTSCH GES GEOWISS Zeitschrift der Deutsc0372-8854地学4N Z GEOMORPHOL ZEITSCHRIFT FUR GEOMORANNU REV ASTRON ASTRANNUAL REVIEW OF ASTRO0066-4146地学天文1Y0935-4956地学天文1Y ASTRON ASTROPHYS REVASTRONOMY AND ASTROPHY0067-0049地学天文1Y ASTROPHYS J SUPPL S ASTROPHYSICAL JOURNAL0084-6597地学天文2N ANNU REV EARTH PL SCANNUAL REVIEW OF EARTHASTRON J ASTRONOMICAL JOURNAL0004-6256地学天文2N2041-8205地学天文2N ASTROPHYS J LETT Astrophysical JournalJ COSMOL ASTROPART PJOURNAL OF COSMOLOGY A1475-7516地学天文2N0035-8711地学天文2N MON NOT R ASTRON SOCMONTHLY NOTICES OF THEASTROPHYS J ASTROPHYSICAL JOURNAL0004-637X地学天文2Y ASTRON ASTROPHYS ASTRONOMY & ASTROPHYSI0004-6361地学天文3N ASTROPART PHYS ASTROPARTICLE PHYSICS0927-6505地学天文3N0922-6435地学天文3N EXP ASTRON EXPERIMENTAL ASTRONOMY0921-8181地学天文3N GLOBAL PLANET CHANGEGLOBAL AND PLANETARY CICARUS ICARUS0019-1035地学天文3N1086-9379地学天文3N METEORIT PLANET SCI METEORITICS & PLANETAR1323-3580地学天文3N PUBL ASTRON SOC AUSTPUBLICATIONS OF THE AS0004-6264地学天文3N PUBL ASTRON SOC JPN PUBLICATIONS OF THE AS0004-6280地学天文3N PUBL ASTRON SOC PAC PUBLICATIONS OF THE ASSOL PHYS SOLAR PHYSICS0038-0938地学天文3N SPACE SCI REV SPACE SCIENCE REVIEWS0038-6308地学天文3N ACTA ASTRONOM ACTA ASTRONOMICA0001-5237地学天文4N0273-1177地学天文4N ADV SPACE RES ADVANCES IN SPACE RESE1366-8781地学天文4N ASTRON GEOPHYS ASTRONOMY & GEOPHYSICSASTRON LETT+ASTRONOMY LETTERS-A JO1063-7737地学天文4N0004-6337地学天文4N ASTRON NACHR ASTRONOMISCHE NACHRICHASTRON REP+ASTRONOMY REPORTS1063-7729地学天文4N ASTROPHYS BULL Astrophysical Bulletin1990-3413地学天文4N0004-640X地学天文4N ASTROPHYS SPACE SCI ASTROPHYSICS AND SPACEASTROPHYSICS+ASTROPHYSICS0571-7256地学天文4N0304-9523地学天文4N B ASTRON SOC INDIA Bulletin of the AstronBALT ASTRON BALTIC ASTRONOMY1392-0049地学天文4N CELEST MECH DYN ASTRCELESTIAL MECHANICS &0923-2958地学天文4N1335-1842地学天文4N CONTRIB ASTRON OBS S Contributions of the ACOSMIC RES+COSMIC RESEARCH0010-9525地学天文4N0167-9295地学天文4N EARTH MOON PLANETS EARTH MOON AND PLANETS1343-8832地学天文4N EARTH PLANETS SPACE EARTH PLANETS AND SPACGEOPHYS ASTRO FLUID GEOPHYSICAL AND ASTROP0309-1929地学天文4N0202-2893地学天文4N GRAVIT COSMOL-RUSSIAGravitation & Cosmolog1473-5504地学天文4N INT J ASTROBIOL International JournalINT J MOD PHYS D INTERNATIONAL JOURNAL0218-2718地学天文4N0250-6335地学天文4N J ASTROPHYS ASTRON JOURNAL OF ASTROPHYSICJ HIST ASTRON JOURNAL FOR THE HISTOR0021-8286地学天文4N1225-4614地学天文4N J KOREAN ASTRON SOC Journal of the Korean0884-5913地学天文4N KINEMAT PHYS CELEST+Kinematics and PhysicsNEW ASTRON NEW ASTRONOMY1384-1076地学天文4N NEW ASTRON REV NEW ASTRONOMY REVIEWS1387-6473地学天文4N OBSERVATORY OBSERVATORY0029-7704地学天文4N0031-9201地学天文4N PHYS EARTH PLANET INPHYSICS OF THE EARTH APLANET SPACE SCI PLANETARY AND SPACE SC0032-0633地学天文4N1674-4527地学天文4N RES ASTRON ASTROPHYSResearch in Astronomy0185-1101地学天文4N REV MEX ASTRON ASTR REVISTA MEXICANA DE ASSOLAR SYST RES+SOLAR SYSTEM RESEARCH0038-0946地学天文4N1542-7390地学天文4N SPACE WEATHER SPACE WEATHER-THE INTEACM COMPUT SURV ACM COMPUTING SURVEYS0360-0300工程技术1Y0730-0301工程技术1Y ACM T GRAPHIC ACM TRANSACTIONS ON GRACS NANO ACS Nano 1936-0851工程技术1Y ACTA BIOMATER Acta Biomaterialia1742-7061工程技术1Y ACTA MATER ACTA MATERIALIA1359-6454工程技术1Y0065-2156工程技术1Y ADV APPL MECH ADVANCES IN APPLIED MEADV FUNCT MATER ADVANCED FUNCTIONAL MA1616-301X工程技术1Y ADV MATER ADVANCED MATERIALS0935-9648工程技术1Y1523-9829工程技术1Y ANNU REV BIOMED ENG ANNUAL REVIEW OF BIOMEANNU REV MATER RES ANNUAL REVIEW OF MATER1531-7331工程技术1Y0570-4928工程技术1Y APPL SPECTROSC REV APPLIED SPECTROSCOPY RBIOMATERIALS BIOMATERIALS0142-9612工程技术1Y BIORESOURCE TECHNOL BIORESOURCE TECHNOLOGY0960-8524工程技术1Y0956-5663工程技术1Y BIOSENS BIOELECTRON BIOSENSORS & BIOELECTR0734-9750工程技术1Y BIOTECHNOL ADV BIOTECHNOLOGY ADVANCES0006-3592工程技术1Y BIOTECHNOL BIOENG BIOTECHNOLOGY AND BIOE1754-6834工程技术1Y BIOTECHNOL BIOFUELS Biotechnology for BiofCARBON CARBON0008-6223工程技术1Y CHEM MATER CHEMISTRY OF MATERIALS0897-4756工程技术1Y0738-8551工程技术1Y CRIT REV BIOTECHNOL CRITICAL REVIEWS IN BI1040-8398工程技术1Y CRIT REV FOOD SCI CRITICAL REVIEWS IN FOCURR OPIN BIOTECH CURRENT OPINION IN BIO0958-1669工程技术1Y1359-0286工程技术1Y CURR OPIN SOLID ST MCURRENT OPINION IN SOL1388-2481工程技术1Y ELECTROCHEM COMMUN ELECTROCHEMISTRY COMMUELECTROCHIM ACTA ELECTROCHIMICA ACTA0013-4686工程技术1Y1301-8361工程技术1Y ENERGY EDUC SCI TECHEnergy Education Scien0737-0024工程技术1Y HUM-COMPUT INTERACT HUMAN-COMPUTER INTERAC0018-8646工程技术1Y IBM J RES DEV IBM JOURNAL OF RESEARC1553-877X工程技术1Y IEEE COMMUN SURV TUTIEEE Communications Su0733-8716工程技术1Y IEEE J SEL AREA COMMIEEE JOURNAL ON SELECTIEEE J SOLID-ST CIRCIEEE JOURNAL OF SOLID-0018-9200工程技术1Y1053-5888工程技术1Y IEEE SIGNAL PROC MAGIEEE SIGNAL PROCESSING1089-778X工程技术1Y IEEE T EVOLUT COMPUTIEEE TRANSACTIONS ON EIEEE T IND ELECTRON IEEE TRANSACTIONS ON I0278-0046工程技术1Y0162-8828工程技术1Y IEEE T PATTERN ANAL IEEE TRANSACTIONS ON P0920-5691工程技术1Y INT J COMPUT VISION INTERNATIONAL JOURNALINT J HYDROGEN ENERGINTERNATIONAL JOURNAL0360-3199工程技术1Y1565-1339工程技术1Y INT J NONLIN SCI NUMINTERNATIONAL JOURNALINT J PLASTICITY INTERNATIONAL JOURNAL0749-6419工程技术1Y0950-6608工程技术1Y INT MATER REV INTERNATIONAL MATERIALJ CATAL JOURNAL OF CATALYSIS0021-9517工程技术1Y J CIV ENG MANAG Journal of Civil Engin1392-3730工程技术1Y0304-3894工程技术1Y J HAZARD MATER JOURNAL OF HAZARDOUS M0959-9428工程技术1Y J MATER CHEM JOURNAL OF MATERIALS CJ MEMBRANE SCI JOURNAL OF MEMBRANE SC0376-7388工程技术1Y0378-7753工程技术1Y J POWER SOURCES JOURNAL OF POWER SOURCLAB CHIP LAB ON A CHIP1473-0197工程技术1Y MACROMOL RAPID COMM MACROMOLECULAR RAPID C1022-1336工程技术1Y MACROMOLECULES MACROMOLECULES0024-9297工程技术1Y0927-796X工程技术1Y MAT SCI ENG R MATERIALS SCIENCE & ENMATER TODAY Materials Today1369-7021工程技术1Y1361-8415工程技术1Y MED IMAGE ANAL MEDICAL IMAGE ANALYSISMETAB ENG METABOLIC ENGINEERING1096-7176工程技术1Y MICROB CELL FACT Microbial Cell Factori1475-2859工程技术1Y1613-4982工程技术1Y MICROFLUID NANOFLUIDMicrofluidics and NanoMIS QUART MIS QUARTERLY0276-7783工程技术1Y MOL NUTR FOOD RES MOLECULAR NUTRITION &1613-4125工程技术1Y MRS BULL MRS BULLETIN0883-7694工程技术1Y NANO LETT NANO LETTERS1530-6984工程技术1Y NANO TODAY Nano Today1748-0132工程技术1Y NANOMED-NANOTECHNOL Nanomedicine-Nanotechn1549-9634工程技术1Y NANOSCALE Nanoscale2040-3364工程技术1Y NANOTECHNOLOGY NANOTECHNOLOGY0957-4484工程技术1Y NAT BIOTECHNOL NATURE BIOTECHNOLOGY1087-0156工程技术1Y NAT MATER NATURE MATERIALS1476-1122工程技术1Y NAT NANOTECHNOL Nature Nanotechnology1748-3387工程技术1Y ORG ELECTRON ORGANIC ELECTRONICS1566-1199工程技术1Y0018-9219工程技术1Y P IEEE PROCEEDINGS OF THE IEEPLASMONICS Plasmonics 1557-1955工程技术1Y POLYM REV Polymer Reviews1558-3724工程技术1Y PROG CRYST GROWTH CHPROGRESS IN CRYSTAL GR0960-8974工程技术1Y0360-1285工程技术1Y PROG ENERG COMBUST PROGRESS IN ENERGY ANDPROG MATER SCI PROGRESS IN MATERIALS0079-6425工程技术1Y1062-7995工程技术1Y PROG PHOTOVOLTAICS PROGRESS IN PHOTOVOLTA0079-6727工程技术1Y PROG QUANT ELECTRON PROGRESS IN QUANTUM ELPROG SURF SCI PROGRESS IN SURFACE SC0079-6816工程技术1Y1364-0321工程技术1Y RENEW SUST ENERG REVRENEWABLE & SUSTAINABLSIAM J IMAGING SCI SIAM Journal on Imagin1936-4954工程技术1Y SMALL SMALL1613-6810工程技术1Y SOFT MATTER Soft Matter1744-683X工程技术1Y SOL ENERG MAT SOL C SOLAR ENERGY MATERIALS0927-0248工程技术1Y0167-7799工程技术1Y TRENDS BIOTECHNOL TRENDS IN BIOTECHNOLOG0924-2244工程技术1Y TRENDS FOOD SCI TECHTRENDS IN FOOD SCIENCEVLDB J VLDB JOURNAL1066-8888工程技术1Y0734-2071工程技术2N ACM T COMPUT SYST ACM TRANSACTIONS ON CO0098-3500工程技术2N ACM T MATH SOFTWARE ACM TRANSACTIONS ON MAACM T SENSOR NETWORK ACM Transactions on Se1550-4859工程技术2N1049-331X工程技术2N ACM T SOFTW ENG METHACM TRANSACTIONS ON SOACM T WEB ACM Transactions on th1559-1131工程技术2N1944-8244工程技术2N ACS APPL MATER INTERACS Applied Materials0724-6145工程技术2N ADV BIOCHEM ENG BIOTADVANCES IN BIOCHEMICAANN BIOMED ENG ANNALS OF BIOMEDICAL E0090-6964工程技术2N0066-4200工程技术2N ANNU REV INFORM SCI ANNUAL REVIEW OF INFORAPPL ENERG APPLIED ENERGY0306-2619工程技术2N APPL SOFT COMPUT APPLIED SOFT COMPUTING1568-4946工程技术2N1134-3060工程技术2N ARCH COMPUT METHOD EARCHIVES OF COMPUTATIO0004-3702工程技术2N ARTIF INTELL ARTIFICIAL INTELLIGENCAUST J GRAPE WINE R AUSTRALIAN JOURNAL OF1322-7130工程技术2N AUTOMATICA AUTOMATICA0005-1098工程技术2N1387-2532工程技术2N AUTON AGENT MULTI-AGAUTONOMOUS AGENTS AND1822-427X工程技术2N BALT J ROAD BRIDGE EBaltic Journal of Road1369-703X工程技术2N BIOCHEM ENG J BIOCHEMICAL ENGINEERINBIODEGRADATION BIODEGRADATION0923-9820工程技术2N BIOMASS BIOENERG BIOMASS & BIOENERGY0961-9534工程技术2N1617-7959工程技术2N BIOMECH MODEL MECHANBiomechanics and Model1387-2176工程技术2N BIOMED MICRODEVICES BIOMEDICAL MICRODEVICEBIOTECHNIQUES BIOTECHNIQUES0736-6205工程技术2N8756-7938工程技术2N BIOTECHNOL PROGR BIOTECHNOLOGY PROGRESSBMC BIOTECHNOL BMC BIOTECHNOLOGY1472-6750工程技术2N CELLULOSE CELLULOSE0969-0239工程技术2N CEMENT CONCRETE RES CEMENT AND CONCRETE RE0008-8846工程技术2N1385-8947工程技术2N CHEM ENG J CHEMICAL ENGINEERING J0169-7439工程技术2N CHEMOMETR INTELL LABCHEMOMETRICS AND INTELCOAST ENG COASTAL ENGINEERING0378-3839工程技术2N COMBUST FLAME COMBUSTION AND FLAME0010-2180工程技术2N0001-0782工程技术2N COMMUN ACM COMMUNICATIONS OF THE1359-835X工程技术2N COMPOS PART A-APPL SCOMPOSITES PART A-APPL0266-3538工程技术2N COMPOS SCI TECHNOL COMPOSITES SCIENCE AND1541-4337工程技术2N COMPR REV FOOD SCI FCOMPREHENSIVE REVIEWSCOMPUT EDUC COMPUTERS & EDUCATION0360-1315工程技术2N0824-7935工程技术2N COMPUT INTELL-US COMPUTATIONAL INTELLIG0891-2017工程技术2N COMPUT LINGUIST COMPUTATIONAL LINGUIST0045-7825工程技术2N COMPUT METHOD APPL MCOMPUTER METHODS IN AP1077-3142工程技术2N COMPUT VIS IMAGE UNDCOMPUTER VISION AND IM1093-9687工程技术2N COMPUT-AIDED CIV INFCOMPUTER-AIDED CIVIL ACOMPUTER COMPUTER0018-9162工程技术2N CORROS SCI CORROSION SCIENCE0010-938X工程技术2N CURR NANOSCI Current Nanoscience1573-4137工程技术2N1384-5810工程技术2N DATA MIN KNOWL DISC DATA MINING AND KNOWLEDECIS SUPPORT SYST DECISION SUPPORT SYSTE0167-9236工程技术2N DENT MATER DENTAL MATERIALS0109-5641工程技术2N0925-9635工程技术2N DIAM RELAT MATER DIAMOND AND RELATED MA1842-3582工程技术2N DIG J NANOMATER BIOSDigest Journal of NanoDYES PIGMENTS DYES AND PIGMENTS0143-7208工程技术2N EARTHQ SPECTRA EARTHQUAKE SPECTRA8755-2930工程技术2N ELECTROCHEM SOLID STELECTROCHEMICAL AND SO1099-0062工程技术2N0196-8904工程技术2N ENERG CONVERS MANAGEENERGY CONVERSION ANDENERG FUEL ENERGY & FUELS0887-0624工程技术2N。
阻抑动力学法测定北海地区海带中的痕量镍含量

阻抑动力学法测定北海地区海带中的痕量镍含量
马建强;陈实源;姚翰英;谢跃生
【期刊名称】《化工技术与开发》
【年(卷),期】2005(034)001
【摘要】发现了在pH=8~9的缓冲介质中,Ni2+对H2O2氧化溴甲酚紫的反应
有明显的阻抑作用.研究了Ni2+阻抑反应的最佳条件,建立了阻抑动力学光度法测
定镍的方法.方法检出限为0.0026 μg·mL-1,回收率为97 %.该方法可快速、简便、准确地用于海带中痕量镍的测定.
【总页数】4页(P27-29,35)
【作者】马建强;陈实源;姚翰英;谢跃生
【作者单位】广西师范学院化学系,广西,南宁,530001;广西师范学院化学系,广西,南宁,530001;广西师范学院化学系,广西,南宁,530001;广西师范学院化学系,广西,南宁,530001
【正文语种】中文
【中图分类】O657.3
【相关文献】
1.甲基蓝褪色阻抑动力学光度法测定痕量镍 [J], 王洪福;苏智先;张素兰;何黎明;刘
丽
2.以苄基三乙基氯化铵为增敏剂镍-溴酸钾-甲基紫体系阻抑动力学光度法测定痕量镍 [J], 王晓菊;高烨;孙凌晨;胡海龙
3.协同增敏Fe(Ⅱ)-邻二氮菲阻抑动力学光度法测定海带中的痕量碘 [J], 来守军;
张雪;关晓琳
4.协同增敏Fe(Ⅱ)-邻二氮菲阻抑动力学光度法测定海带中的痕量碘 [J], 来守军; 张雪; 关晓琳
5.阻抑动力学光度法测定白鼠肝脏中的超痕量镍(Ⅱ) [J], 汪燕芳;陈国树
因版权原因,仅展示原文概要,查看原文内容请购买。
塔里木板块东北部坡-镁铁质-超镁铁质层状侵入体岩石成因

塔里木板块东北部坡-镁铁质-超镁铁质层状侵入体岩石成因姜常义;郭娜欣;夏明哲;凌锦兰;郭芳放;邓小芹;姜寒冰;范亚洲【期刊名称】《岩石学报》【年(卷),期】2012(028)007【摘要】The Pobei rock body is located in northeastern Tarim Plate. As one of many small intrusions of Pobei body, the Poyi intrusion formed during the third stage of the magmatism and dated at 278 ?2Ma by the SHRIMP zircon U-Pb method. The Poyi layered intrusion, which is mainly composed of ultramafic rocks, develops cumulate texture and rhythmic layering. The magma differentiated thoroughly and formed many types of rocks, which range from dunite to quartz diorite. In the ultramafic rocks, all olivine and most orthopyroxene occur as cumulate phase, while small part of orthopyroxene, most clinopyroxene, brown hornblende and biotite are intercumulate. In the mafic rocks, all olivine and orthopyroxene are cumulate phase, while all brown hornblende, biotite and quartz are intercumulate. But the clinopyroxene and plagioclase may be cumulate or intercumulate. The ultramafic rocks are tholeiitic series and the mafic ones are calc-alkalic. Xenoliths occurred in the intrusion, geochemistry of trace elements and EM II evolutionary trend of Nd-Sr isotopic composition, fully demonstrate that the contamination increased as magma evolving. At the same time, contamination promoted magmatic differentiation and brought about the transformation of geochemical series. Geochemistry ofplatinum group and thiophile elements, as well as isotopic composition of sulfur, show that sulfur mainly came from mantle-derived magma and sulfide formed during magmatic stage. The sulfide segregation began in the late stage of the crystallization of peridotites and went on as the mngmfl evolving. The Fo value of olivine and FeO content of whole-rock show that the primary magma was picritic and formed by partial melting of axis plume. The main rocks of magmatic source should be garnet-pyroxenolite. The other Permian magmatite in northeastern Tarim Plate, which derived from asthenospheric or depleted continental lithospheric mantle, also should be part of the Tarim large igneous province (LIP).%坡一侵入体位于塔里木板块东北部坡北岩体内,是该岩体第三阶段岩浆活动形成的十几个小侵入体中的一个,锆石U-Pb年龄为278±2Ma.该侵入体属于以超镁铁质岩石为主的层状岩系,堆晶结构与韵律性堆晶层理非常发育.岩浆分异充分,形成了从纯橄岩到石英闪长岩的多种岩石类型.在超镁铁质岩石中,所有的橄榄石和大部分斜方辉石是堆晶相,少量斜方辉石是填隙相,大部分单斜辉石、褐色普通角闪石和黑云母是填隙相.在镁铁质岩石中,橄榄石和斜方辉石全部是堆晶相,单斜辉石与斜长石既可以是堆晶相,也可以是填隙相;褐色普通角闪石、黑云母和石英均为填隙相.超镁铁质岩石属拉斑玄武岩系列,镁铁质岩石属钙碱性系列.侵入体中大量存在的捕掳体、微量元素地球化学、Nd-Sr同位素组成的EMⅡ型演化趋势,充分证明了同化混染作用伴随岩浆演化过程而逐渐增强,并不断促进了岩浆的分异,而且导致了岩石化学系列的转化.PGE和亲硫元素地球化学以及硫同位素组成证明,硫主要来自于岩浆,硫化物形成于岩浆阶段,岩浆未经历过早期硫化物熔离作用,硫化物熔离起始于橄榄岩相结晶的晚期阶段,并伴随着此后的岩浆演化过程而继续熔离.硫化物熔离是岩浆自身演化和同化混染共同作用的结果.橄榄石Fo分子含量和全岩FeO含量显示,原生岩浆是苦橄质岩浆;源区物质应该是石榴石辉石岩;岩浆生成于地幔柱轴部.在塔里木板块东北部还存在分别来自于软流圈和亏损型大陆岩石圈地幔的二叠纪岩浆岩,它们都应该是塔里木大火成岩省的组成部分.【总页数】15页(P2209-2223)【作者】姜常义;郭娜欣;夏明哲;凌锦兰;郭芳放;邓小芹;姜寒冰;范亚洲【作者单位】长安大学地球科学与资源学院,西安710054;西部矿产资源与地质工程教育部重点实验室,西安710054;长安大学地球科学与资源学院,西安710054;西部矿产资源与地质工程教育部重点实验室,西安710054;长安大学地球科学与资源学院,西安710054;西部矿产资源与地质工程教育部重点实验室,西安710054;长安大学地球科学与资源学院,西安710054;西部矿产资源与地质工程教育部重点实验室,西安710054;长安大学地球科学与资源学院,西安710054;西部矿产资源与地质工程教育部重点实验室,西安710054;长安大学地球科学与资源学院,西安710054;西部矿产资源与地质工程教育部重点实验室,西安710054;西安地质矿产研究所,西安710054;长安大学地球科学与资源学院,西安710054;西部矿产资源与地质工程教育部重点实验室,西安710054【正文语种】中文【中图分类】P588.125;P597【相关文献】1.白马含矿层状镁铁-超镁铁质侵入体的岩石学研究 [J], 陈富文2.新疆北山地区漩涡岭镁铁质—超镁铁质层状岩体岩石学与矿物学研究 [J], 夏昭德;王壵;姜常义;凌锦兰;夏明哲;郭娜欣3.新疆北山地区罗东镁铁质-超镁铁质层状岩体岩石成因 [J], 凌锦兰;夏明哲;郭娜欣;汪帮耀;夏昭德;姜常义4.含硫化物镁铁质-超镁铁质侵入体中橄榄石Mg、Ni含量的制约:原理、模式及Voisey's Bay侵入体样品研究 [J],5.扬子克拉通北缘新元古代大陆岩石圈脱层作用信息——毕机沟镁铁质-超镁铁质侵入体同位素地球化学指示 [J], 凌文黎;张本仁;周炼;赵祖斌因版权原因,仅展示原文概要,查看原文内容请购买。
卡林型金精矿加压氧化—铁矾分解—非氰提金研究
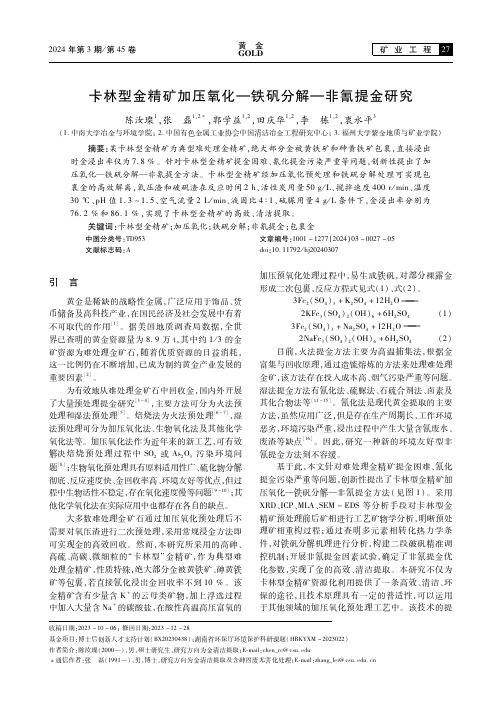
2024年第3期/第45卷黄 金GOLD矿业工程卡林型金精矿加压氧化—铁矾分解—非氰提金研究收稿日期:2023-10-06;修回日期:2023-12-28基金项目:博士后创新人才支持计划(BX20230438);湖南省环保厅环境保护科研课题(HBKYXM-2023022)作者简介:陈汝璨(2000—),男,硕士研究生,研究方向为金清洁提取;E mail:chen_rc@csu.edu通信作者:张 磊(1991—),男,博士,研究方向为金清洁提取及含砷固废无害化处理;E mail:zhang_lei@csu.edu.cn陈汝璨1,张 磊1,2,郭学益1,2,田庆华1,2,李 栋1,2,衷水平3(1.中南大学冶金与环境学院;2.中国有色金属工业协会中国清洁冶金工程研究中心;3.福州大学紫金地质与矿业学院)摘要:某卡林型金精矿为典型难处理金精矿,绝大部分金被黄铁矿和砷黄铁矿包裹,直接浸出时金浸出率仅为7.8%。
针对卡林型金精矿提金困难、氰化提金污染严重等问题,创新性提出了加压氧化—铁矾分解—非氰提金方法。
卡林型金精矿经加压氧化预处理和铁矾分解处理可实现包裹金的高效解离,氧压渣和破矾渣在反应时间2h、活性炭用量50g/L、搅拌速度400r/min、温度30℃、pH值1.3~1.5、空气流量2L/min、液固比4∶1、硫脲用量4g/L条件下,金浸出率分别为76.2%和86.1%,实现了卡林型金精矿的高效、清洁提取。
关键词:卡林型金精矿;加压氧化;铁矾分解;非氰提金;包裹金 中图分类号:TD953 文章编号:1001-1277(2024)03-0027-05文献标志码:Adoi:10.11792/hj20240307引 言黄金是稀缺的战略性金属,广泛应用于饰品、货币储备及高科技产业,在国民经济及社会发展中有着不可取代的作用[1]。
据美国地质调查局数据,全世界已查明的黄金资源量为8.9万t,其中约1/3的金矿资源为难处理金矿石,随着优质资源的日益消耗,这一比例仍在不断增加,已成为制约黄金产业发展的重要因素[2]。
玻璃的流变性与热力学性能
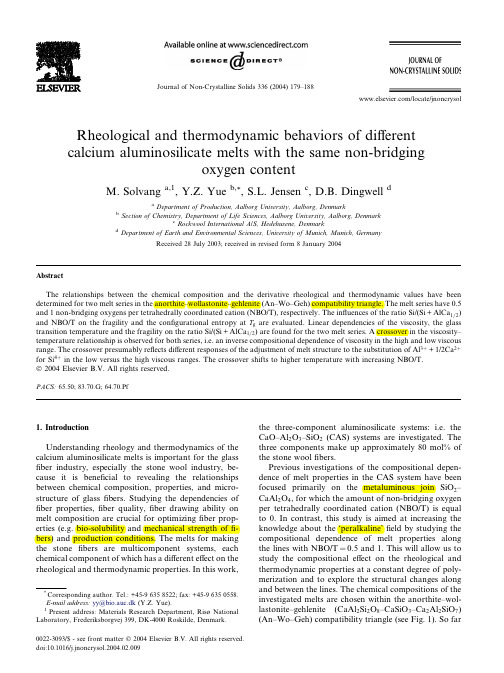
Rheological and thermodynamic behaviors of different calcium aluminosilicate melts with the same non-bridgingoxygen contentM.Solvanga,1,Y.Z.Yueb,*,S.L.Jensen c ,D.B.DingwelldaDepartment of Production,Aalborg University,Aalborg,DenmarkbSection of Chemistry,Department of Life Sciences,Aalborg University,Aalborg,DenmarkcRockwool International A/S,Hedehusene,DenmarkdDepartment of Earth and Environmental Sciences,University of Munich,Munich,GermanyReceived 28July 2003;received in revised form 8January 2004AbstractThe relationships between the chemical composition and the derivative rheological and thermodynamic values have been determined for two melt series in the anorthite–wollastonite–gehlenite (An–Wo–Geh)compatibility triangle.The melt series have 0.5and 1non-bridging oxygens per tetrahedrally coordinated cation (NBO/T),respectively.The influences of the ratio Si/(Si +AlCa 1=2)and NBO/T on the fragility and the configurational entropy at T g are evaluated.Linear dependencies of the viscosity,the glass transition temperature and the fragility on the ratio Si/(Si +AlCa 1=2)are found for the two melt series.A crossover in the viscosity–temperature relationship is observed for both series,i.e.an inverse compositional dependence of viscosity in the high and low viscous range.The crossover presumably reflects different responses of the adjustment of melt structure to the substitution of Al 3þ+1/2Ca 2þfor Si 4þin the low versus the high viscous ranges.The crossover shifts to higher temperature with increasing NBO/T.Ó2004Elsevier B.V.All rights reserved.PACS:65.50;83.70.G;64.70.Pf1.IntroductionUnderstanding rheology and thermodynamics of the calcium aluminosilicate melts is important for the glass fiber industry,especially the stone wool industry,be-cause it is beneficial to revealing the relationships between chemical composition,properties,and micro-structure of glass fibers.Studying the dependencies of fiber properties,fiber quality,fiber drawing ability on melt composition are crucial for optimizing fiber prop-erties (e.g.bio-solubility and mechanical strength of fi-bers)and production conditions.The melts for making the stone fibers are multicomponent systems,each chemical component of which has a different effect on the rheological and thermodynamic properties.In this work,the three-component aluminosilicate systems:i.e.the CaO–Al 2O 3–SiO 2(CAS)systems are investigated.The three components make up approximately 80mol%of the stone wool fibers.Previous investigations of the compositional depen-dence of melt properties in the CAS system have been focused primarily on the metaluminous join SiO 2–CaAl 2O 4,for which the amount of non-bridging oxygen per tetrahedrally coordinated cation (NBO/T)is equal to 0.In contrast,this study is aimed at increasing the knowledge about the ‘peralkaline’field by studying the compositional dependence of melt properties along the lines with NBO/T ¼0.5and 1.This will allow us to study the compositional effect on the rheological and thermodynamic properties at a constant degree of poly-merization and to explore the structural changes along and between the lines.The chemical compositions of the investigated melts are chosen within the anorthite–wol-lastonite–gehlenite (CaAl 2Si 2O 8–CaSiO 3–Ca 2Al 2SiO 7)(An–Wo–Geh)compatibility triangle (see Fig.1).So far*Corresponding author.Tel.:+45-96358522;fax:+45-96350558.E-mail address:yy@bio.auc.dk (Y.Z.Yue).1Present address:Materials Research Department,RisøNational Laboratory,Frederiksborgvej 399,DK-4000Roskilde,Denmark.0022-3093/$-see front matter Ó2004Elsevier B.V.All rights reserved.doi:10.1016/j.jnoncrysol.2004.02.009Journal of Non-Crystalline Solids 336(2004)179–188the rheological and thermodynamic aspects of the melts in this triangle have not been systematically studied. Hence,this work will contribute to broadening and deepening the knowledge about structure and properties of high aluminosilicate melts.The rheological and thermodynamic properties of aluminosilicate melts are determined by the arrange-ment of the tetrahedral structural units in the melt, which relates to the chemical bonding situation within a structural unit and between units.Aluminum differs from silicon,since tetrahedrally coordinated aluminum has to be charge-balanced by either two alkali cations or one earth alkaline cation[1].The charge-balancing ca-tions for the Al3þtetrahedra play a large role in the melt structure.The structural role of the alkali or earth alkaline cations depends on the melt composition,i.e. whether or not Al3þis present in the melt[2].The short range ordering in the aluminosilicate network depends on the composition and the charge-balancing or net-work modifying cations.For aluminosilicates it is as-sumed that an energetically favorably case is a random occurrence of the network forming linkages Si–O–Si,Si–O–Al and Al–O–Al.The principle of Al-avoidance[3] postulates that the Al–O–Si linkage is more favorable than the combination of Si–O–Si and Al–O–Al linkages. This postulate means that the short range ordering is not random(not totally disordered).A tendency towards Al-avoidance is inferred based on29Si MAS NMR line widths in aluminosilicate glasses[4].However the pres-ence of a small amount of Si–O–Si in glasses of anorthite compositions observed by triple quantum MAS NMR spectroscopy[5]suggests some Al–O–Al linkages[6].The aim of this work is to study the compositional dependencies of melt viscosity,glass transition temper-ature(T g),heat capacity(C p)as well as the derivative properties such as activation energy for viscousflow (D H g),fragility[7]and configurational entropy at T g (S cðT gÞ).The paper presents the results of systematic viscometric and calorimetric experiments on the melts covered by the An–Wo–Geh compatibility triangle,and discusses the difference in the structural arrangement in the high compared to that in the low viscous range. 2.Theoretical modelsViscosity is one of the melt properties,which has been studied intensively due to its sensitivity to compositional variation[8].At afixed temperature it varies by orders of magnitude as a function of composition.An increase in temperature decreases the viscosity,as the structural rearrangements in the melt become easier[8].The viscosity–temperature relationship has been de-scribed by various theories.Adam and Gibbs[9]found the correlation between the structural relaxation time of a glass forming melt and the configurational entropy (S c).In their analysis the relaxation time increases with 1=TS c.The S c decreases with decreasing temperature until it vanishes at the Kauzmann temperature.The Adam and Gibbs theory explains the temperature dependence of the relaxation in terms of the temperature dependence of the size of the cooperatively rearranging region[9].In this work the Adam–Gibbs equation(AG equa-tion)wasfitted to experimental viscosity data to derive S cðT gÞ.The AG equation is expressed aslog g¼log g1þBTS cðTÞ;ð1Þwhere g1is a pre-exponential constant and B is a con-stant containing a free-energy barrier which must be crossed by the rearranging group.The constant g1for the glass series studied in this work was obtained by fitting the viscosity data(in the range from g¼0:3to 1011Pa s)to Eq.(1),which equals2.88·10À4Pa s[10]. The viscosity range for the validity of Eq.(1)was dis-cussed in[11].S cðTÞcan be calculated from the equationS cðTÞ¼S cðT gÞþZ TT gD C pðTÞTd T;ð2Þwhere the D C pðTÞis the difference in heat capacity be-tween the liquid and the glassy state.For the silicate systems,the heat capacity of the liquid state,C p l,is only slightly temperature dependent and hence,can be approximately regarded as a constant.In this work,the C p value at T g is assigned as the heat capacity of the glassy state,C p g[10],Therefore,the D C pðTÞð¼C p lÀC p gÞcan also be treated as a constant D C p.Both C p l and C p g may be directly determined from the DSC measurement. Eq.(2)can thus be transformed into the formS cðTÞ¼S cðT gÞþD C p lnTT g:ð3Þ180M.Solvang et al./Journal of Non-Crystalline Solids336(2004)179–188Introducing Eq.(3)into Eq.(1)leads to the equationlog g¼log g1þBT S cðT gÞþD C p ln Tg;ð4ÞS cðT gÞcan be obtained byfitting Eq.(4)to the viscosity–temperature data.Two contributions to the S cðTÞhave been involved:a chemical and a topological contribu-tion[12].The chemical contribution is independent of temperature,whereas the relative contribution from the topological arrangement increases with temperature. Finally,at high temperatures,the chemical contribution plays a minor role.The topological arrangement is re-flected by the increase of configurational entropy at T over that at T g,and it will increase more in a relative sense for compositions having a higher D C p.Avramov[13–15]proposed a three-parameter equa-tion that is able to predict the change in viscosity as a function of both temperature and composition.The model describes the kinetics of molecular motion in su-percooled melts[13]and the dependence of the viscosity on the entropy of the melt.According to Avramov,the structural units of the system jump with frequencies depending on the activation energy,which they have to overcome.The dependence of the activation energy on temperature is assumed to obey a power decay law [13–15].From this assumption,he proposed the equation describing the dependence of viscosity on temperature:log g¼log g1þe0T rTa;ð5Þwhere g1is a pre-exponent(in Pa s),i.e.the viscosity of a hypothetical liquid state for T!1,e0is the constantequal to log g Tr Àlog g1,a is a fragility index,and T r is areference temperature.In this work,T g is used as T r. Then,e0¼log g TgÀlog g1.The viscosity of silicate meltat T g is g Tg ¼1012Pa s.log g1and e0may be obtained byfitting the viscosity data to Eq.(5).For the melt series studied in this work,the optimal values of log g1and e0, which lead to bestfitting,are found to be)1.7and13.7,respectively,by using the relation log g Tg ¼log g1þe0¼12.Thus,the Avramov equation(AV equation)canbe rewritten aslog g¼À1:7þ13:7T gTa:ð6ÞThe two parameters,log g1and e0,are not sensitive tothe variation in chemical composition of the CAS sys-tems.However,the sensitivity of these parameters to thevariation in chemical composition has not been testedon other melt systems.The comparisons between the AG,the AV,and the VFT(Vogel–Fulcher–Tammann)equations[16–18]were made in[10]with respect to theirfitting qualities tothe viscosity data for the10CAS melts.Then,it wasfound that the order of thefitting quality isVFT<AG<AV,the average residual errors of whichwere0.116,0.113and0.063,respectively[19].3.ExperimentalTen melts covered by the CaAl2Si2O8–CaSiO3–CaAl2SiO7(anorthite–wollastonite–gehlenite)compati-bility triangle and placed along the two lines with NBO/T¼0.5and1,respectively,were synthesized(Fig.1).The melts were synthesized from analytical chemicalsSiO2,Al2O3and CaCO3in a platinum crucible at1898K for3h in a MoSi2box furnace.Subsequently the meltwas quenched on a metal plate.The chemical compo-sitions were analyzed using an X-rayfluorescence spec-trometer(XRF)(Philips1404).The compositions arelisted in Table1.The low viscosities(<104Pa s)were measured by means of a concentric cylinder viscometer in the tem-perature range from1316to1849K at ambient pressure.The viscometer consists of four parts:a furnace,a vis-cometer head,a spindle and a sample crucible.Thefurnace was a MoSi2box furnace(Deltech Inc.ModelDT-31-RS Mode EE)and the viscometer head was aBrookfield model RTVD with a full range torque of7.2·10À2N m,which both were controlled by aTable1The composition of the10CAS samplesSiO2(mol%)Al2O3(mol%)CaO(mol%)Si4þAl3þCa2þCAS149.48.342.30.460.150.39CAS245.610.543.90.410.190.40CAS341.312.746.10.370.220.41CAS437.314.747.90.330.260.42CAS533.016.450.50.280.280.43CAS649.815.135.10.430.260.30CAS744.117.438.50.380.300.33CAS839.519.940.50.330.330.34CAS934.822.542.70.280.370.35CAS1029.424.745.90.240.400.37The compositions are measured using XRF and given in mol%and the cation fraction.M.Solvang et al./Journal of Non-Crystalline Solids336(2004)179–188181computer.The spindle and the sample crucible were made of Pt80Rh20.The details about both method and equipment are described in[20].The viscometer was calibrated using the National Bureau of Standards (NBS)710standard glass.The high viscosities(107–1012Pa s)were measured using the micropenetration method.The measurements were done in a vertical push-rod dilatometer(B€AHR DIL802V)[21].The Deutsche Glasstechnische Gesell-schaft(DGG)Standard Glass I was used for calibration.A good agreement was achieved between the measured viscosity and standard viscosity values,with the least square R2¼0:996[10].In the intermediate viscous range from104to107Pa s,it was impossible to measure the viscosities,because the strong tendency for the melts to crystallize hindered the measurements.A differential scanning calorimeter(DSC)(Netzsch STA449C)was used to determine T g and C p.The glass samples for the DSC measurements were the ones that had already been measured using the concentric cylin-der viscometer.The samples were drilled out from the viscometer crucible wherein the glass melt was left after finishing the concentric cylinder viscometer measure-ments.The size of the samples for DSC measurements were4·4·1mm.For each composition a baseline was measured with two empty crucibles.Then a standard sapphire sample was measured andfinally the sample was measured in two runs.Thefirst run was made in order to give the sample a defined thermal history,i.e.a given cooling rate that equals to the heating rate.The second run was made for determination of T g and C p. All measurements were done under argon with the heating rate10K/min.The maximum temperature of each measurement was approximately100K above T g. T g and C p were determined from the second DSC up-scan curves.T g was defined as the onset temperature of the glass transition peak on the second DSC upscan curve.4.ResultsThe viscosity–temperature relationships measured by both the micropenetration and the concentric cylinder viscometer methods are shown in Fig.2and also listed in Table2.In Fig.2,a crossover is seen for both melt series with NBO/T¼0.5and1,respectively.The crossover means that all viscosity–temperature curves for each melt series pass through the same viscosity–temperature point.It also means that in the highly viscous range the viscosity increases and in the low viscous range decreases with decreasing Si/ (Si+AlCa1=2)ratio.In other words,the compositional dependence of the viscosity for a constant temperature is opposite in the high compared to the low viscosity range.The crossovers are located at T¼1280K(or 104=T¼7:81)for the line NBO/T¼0.5and at T¼1500K(or104=T¼6:67)for the line NBO/T¼1. The T g values obtained using the DSC are listed in Table3.Fig.3shows the influence of Si/(Si+AlCa1=2) on the two isokom temperatures,T log g¼12and T log g¼0, for the melt series NBO/T¼0.5and1,respectively. T log g¼12is the T g of each melt,since generally T g cor-responds to the viscosity g¼1012Pa s.The inverse slopes shown in Fig.3are just a consequence of the crossover.In classical rate theory,the activation energy may be thought of as a potential energy barrier,which is over-come by atoms when they move from one site to another in the melt[22].Melts with high activation energy show a larger response of viscosity to a temperature change than melts with low activation energy.In this work,the activation energies for viscousflow(D H g)were deter-mined both in the glass transition range and in the high temperature range from1750to1850K(see Table3).D H gðT gÞand D H gðHTÞrefer to the activation energy around T g and in the high temperature range,respec-182M.Solvang et al./Journal of Non-Crystalline Solids336(2004)179–188tively.The activation energies were obtained byfinding the slope of the ln g vs.1=T linear relationship over a narrow temperature range[10].Fig.4shows linear de-pendences of both D H gðT gÞand D H gðHTÞon the Si/ (Si+AlCa1=2)ratio.The inset of Fig.2shows the viscosity–temperature relationships scaled by T g.The fragility index a for each melt was found byfitting Eq.(6)to the log g vs.1=T data (see Table3).Fig.5shows that the fragility increases with decreasing Si/(Si+AlCa1=2)for both lines.In addition,it is found that a increases with increasing mol%CaO for both lines(Fig.6).The AG equation(Eq.(4))was used tofind the configurational entropy at T g ðS cðT gÞÞand the results are listed in Table3and shown in Fig.7,where it is seen that S cðT gÞdecreases with decreasing Si/(Si+AlCa1=2).Table2Viscosity as a function of temperature measured on the10CAS meltsCAS1CAS2CAS3CAS4CAS5T(K)log g(Pa s)T(K)log g(Pa s)T(K)log g(Pa s)T(K)log g(Pa s)T(K)log g(Pa s) 1052.711.441065.811.201091.510.561106.410.261106.510.61 1065.910.701066.811.121105.39.921119.19.751111.810.55 1095.69.531073.910.791116.19.401133.29.121124.69.95 1112.38.851094.39.981124.39.151143.48.761141.39.19 1132.68.141110.79.281133.18.721158.48.161153.18.701130.28.521144.78.341166.78.171316 3.221150.88.101340 2.851365 2.511389 2.211413 1.941437 1.751437 1.691461 1.511461 1.481486 1.311486 1.281510 1.141510 1.1015340.9815340.9315580.8215580.7815580.7315580.6915830.6915830.6815830.5815830.5416070.5616070.5116070.4516070.4016310.4416310.3416310.3316310.2816550.3216550.2916550.2316550.1616790.2216790.1916790.1316790.0517040.1217040.1017040.051704)0.0517280.0217280.021728)0.041728)0.141752)0.071752)0.061752)0.111752)0.221752)0.26 1776)0.151776)0.131776)0.181776)0.301776)0.34 1801)0.231801)0.201801)0.241801)0.371801)0.41 1825)0.311825)0.251825)0.311825)0.441825)0.48 1849)0.391849)0.311849)0.381849)0.501849)0.53CAS6CAS7CAS8CAS9CAS101099.210.301111.210.261107.110.621121.110.421129.410.43 1116.29.791123.49.831140.29.401139.99.721137.210.05 1133.09.151140.09.151150.38.931155.88.911144.49.81 1151.28.471153.98.661160.48.601168.18.581150.59.48 1164.57.981156.98.451169.98.251185.37.911166.28.931169.78.031173.88.591558 1.1215830.9616070.9116070.8016310.9016310.7716310.6616550.7716550.6416550.4916790.6516790.5316790.4217040.5417040.4217040.3017040.13 17280.4417280.3217280.2117280.02 17520.3417520.2317520.111752)0.011752)0.07 17760.2517760.1417760.031776)0.101776)0.16 18010.1618010.051801)0.061801)0.191801)0.24 18250.071825)0.031825)0.141825)0.271825)0.32 1849)0.021849)0.111849)0.221849)0.351849)0.39M.Solvang et al./Journal of Non-Crystalline Solids336(2004)179–1881835.DiscussionThe appearance of the crossover(see Fig.2)and in-verse compositional dependence of D H gðT gÞand D H gðHTÞ(see Fig.4)suggest that the changes in struc-tural arrangement with chemical composition are dif-ferent in the high and low viscosity ranges.The change of viscosity of a silicate melt with temperature is directly associated with that of the frequency of the breaking and bridging of T0–O bonds,where T0stands for tetra-hedrally coordinated cations.With increasing tempera-ture,the breaking of T0–O bonds becomes more frequent,so that the structure units become more mobile and viscosity decreases.According to[23],the changes of viscosity of a melt is also associated with the exchange between different Q n units,where n refers to the number of the oxygens per SiO4or AlO4unit(Q),which are shared with another SiO4or AlO4unit[23].Such ex-change controls viscousflow of melts[23–25].The higher the exchange frequency is,the lower is the vis-cosity of the melt.The exchange frequency depends on the glass composition and hence fragility of a melt.In high temperature region a fragile melt has higher ex-change frequency than a strong one,whereas in low temperature region the former has lower exchange rate than the latter.Consequently,the ratio in the exchange rate between the low and the higher temperature in-creases with substitution of Al3þ+1/2Ca2þfor Si4þ.In the high viscous range,the melt structure is not per-Table3Rheological and thermodynamic quantities of the10CAS meltsT g a(K)D H gðT gÞb(kJ/mol)D H g(HT)b(kJ/mol)a c B d(kJ/mol)S cðT gÞd(J/(mol K)) CAS11043937208 4.18252 6.62CAS21057950170 4.36246 6.52CAS31065991172 4.40254 6.47CAS41075992189 4.61246 6.14CAS510841051175 4.62271 6.84CAS61066881231 3.833027.77CAS71075961219 4.052927.35CAS81082939217 4.252847.09CAS910931002217 4.402907.24CAS1011041036213 4.562847.08a Determined using the DSC,with the heating and cooling rates10K/min.b Estimated in the glass transition region as well as in the high temperature(HT)range1700–1850K.c Found by a least squarefit of the viscosity–temperature data using the Avramov equation[10,13].d Obtained byfitting the Adam–Gibbs equation to the viscosity data[9,10].184M.Solvang et al./Journal of Non-Crystalline Solids336(2004)179–188turbed in the same way as in the low viscous range where the structural units of the glass melt are mobile and, hence,the diffusion is faster.If temperature is scaled by T g,the crossover disappears as shown in the insets of Fig.2,but the curvatures of the log g vs.T g=T plots,that are quantified by the fragility index a,become different among the glasses.Thus the existence of a crossover implies the difference in the fragility between the differ-ent glasses.The dependence of viscosity on the temperature indicates a high and a low temperature structural arrangement in the melts.The diffusivity is dramatically lower in a high viscous range compared to that in a low viscous range.In the high viscous range(g¼108–1012 Pa s),both the charge-balancing ions and the network-modifying cations(Ca2þ)have the tendency to attract the neighboring tetrahedra of glass former.Recently,it has been experimentally confirmed that the Ca2þions contract the channels in the glass network as reported in [26].Therefore,the structural network becomes stronger with increasing substitution of Al3þ+1/2Ca2þfor Si4þbecause of the role of Ca2þions.In other words,the connection of tetrahedra becomes stronger,and hence the formation of larger clusters is favored,if Al3þ+1/ 2Ca2þsubstitutes for Si4þ.As a result,the viscosity in-creases with that substitution in the high viscous range. In this case,the topological and cooperative rearrange-ments of the melt structure are dominant in influencing the change in viscosity.In the high viscous range,the diffusion of the structural units(e.g.tetrahedral groups and Ca2þions)in the melt become more difficult with increasingly substitution of Al3þ+1/2Ca2þfor Si4þbe-cause of the strengthening of structural network by Ca2þ.That is why D H gðT gÞincreases with decreasing Si/ (Si+AlCa1=2)(see Fig.4).The strengthening of the melt structure is also reflected by the increase in T g with increasingly substitution of Al3þ+1/2Ca2þfor Si4þ(see Fig.3and Table3).This is because a high T g value corresponds to the high strength and/or the high degree of polymerization of the melt structure.In the low viscous range,the strength of the chemical bonds,including both the charge-balancing ions and the network-modifying cations lying in the interstice be-tween the Al-tetrahedrons becomes weaker and more unstable.The diffusion process of the structural units become faster and more dominant with substitution of Al3þ+1/2Ca2þfor Si4þand hence leads to a decrease in viscosity for both of the NBO/T lines(see Fig.3).The crossover can also be explained by the recent study on difference in structural relaxation time andM.Solvang et al./Journal of Non-Crystalline Solids336(2004)179–188185calcium mobility for calcium aluminosilicate melts.In [27],it has been found that in the high temperature range the characteristic time of the calcium mobility is the same as the structural relaxation.In contrast,in the low temperature range two kinds of relaxation processes exists.Firstly,a fast process,which corresponds to cal-cium mobility,and secondly,a slow one,which corre-sponds to structural relaxation of the aluminosilicate network.The crossover shifts to higher temperature with increasing NBO/T(see Fig.2).In other words,the temperature range above T g,in which a substitution of Al3þ+1/2Ca2þfor Si4þincreases the viscosity,becomes broader.This suggests that an increase in the concen-tration of Ca2þresults in broadening of the temperature range,in which Ca2þions strengthen the melt structure by attracting the tetrahedra and thus enhancing the grouping of these tetrahedra,i.e.the formation of the clusters.Such structure strengthening is reflected by the fact that the T g values of the melts with NBO/T¼1are higher than those of the melts with NBO/T¼0.5,as shown in Fig.3and Table3.The shift of the crossover also implies that the topological and cooperative rear-rangement of the melt structure in the high viscous range is more predominant and the formation of cluster is more favored for the aluminosilicate melt with more Ca2þcontent than for the melt with less Ca2þcontent, whereas the diffusion of the structural units become more hindered for the former than the latter.The fragility index a shows a linear dependence on both the Si/(Si+AlCa1=2)ratio(see Fig.5)and the CaO content(mol%)(see Fig.6)for both melt series with NBO/T¼0.5and1,respectively.This clearly indicates the sensitivity of fragility to the content of Ca2þin the manner that CaO greatly can increase the fragility of the studied glass melt system.From Fig.5,it can also be seen that the fragility changes more dramatically for melts with NBO/T¼0.5compared to that of melts with NBO/T¼1.In Fig.8,the configurational entropy S cðTÞis com-pared between different melts for a certain value of T=T g. At T=T g¼1:1,S cðTÞslightly increases with increasing Si/(Si+AlCa1=2)ratio,whereas at T=T g¼2,S cðTÞde-creases with increasing Si/(Si+AlCa1=2)ratio.This shows that the dependence of S cðTÞat T=T g¼1:1on the Si/(Si+AlCa1=2)ratio is inverse to that for T=T g¼2.In other words,the compositional dependencies of S cðTÞat T=T g¼1:1and at T=T g¼2are inverse.This indicates a relative change of the ratio between the chemical and topological contributions to the configurational en-tropy.With increasing temperature,the relative chemi-cal contribution to S cðTÞdecreases,while the topological contribution increases(see Eq.(4)).The relative increase in S cðTÞwith increasing temperature is largest for the melt with the lowest Si/(Si+AlCa1=2)ratio(see Fig.8), because it has the highest D C p(see Eq.(4))[10].This is related to the fact that the perturbation of the local structural environment becomes more intense with decreasing the Si/(Si+AlCa1=2)ratio.The increase in concentration of Al3þleads to an increase in degree of disorder of the melt structure.In the low temperature range,the chemical contribution controls the configu-rational entropy,whereas in the high temperature range the topological contribution controls the configurational entropy as also discussed in the work[12,28].Regarding each of the two compositional joins (NBO/T¼0.5and1)as part of a mixing series,a neg-ative deviation from linearity in T g values from the end members is anticipated.In an ideal mixing series,a minimum occurs when half of each end member is mixed.This is analogous to the phenomenon that is clearly seen when dealing with the mixed alkali effect [29].A minimum in T g vs.Si/(Si+AlCa1=2)for the lines NBO/T¼0.5and1is observed[10,30].The increasing amount of Al–O–Si bonds relative to that of Si–O–Si bonds results in the decrease in T g when looking in the direction of decreasing Si/(Si+AlCa1=2)until a mini-mum in T g is reached.The minimum is followed by an increase in T g when additionally decreasing the Si/ (Si+AlCa1=2)ratio.This behavior is attributed to the increasing possibility for the charge-balancing cations and the network modifying cations to attract tetrahe-drons.The formation of clusters becomes easier with decreasing the Si/(Si+AlCa1=2)ratio.The consequence is a strengthening of the melt structure.The occurrence of a minimum in T g for the lines NBO/T¼0.5and1can be understood as a consequence of the mixing effect in the manner that S cðT gÞdepends non-linearly on the Si/(Si–AlCa1=2)ratio,but with a maximum at a certain Si/(Si+AlCa1=2)ratio.The mix-ing effect can be regarded as similar to that of the mixed alkali effect[29].For both melt series with NBO/T¼0.5186M.Solvang et al./Journal of Non-Crystalline Solids336(2004)179–188。
2Stochastic averaging of quasi-generalized Hamiltonian systems

ABSTRACT
A stochastic averaging method for generalized Hamiltonian systems (GHS) subject to light dampings and weak stochastic excitations is proposed. First, the GHS are briefly reviewed and classified into five classes, i.e., non-integrable GHS, completely integrable and non-resonant GHS, completely integrable and resonant GHS, partially integrable and non-resonant GHS and partially integrable and resonant GHS. Then, the averaged Itoˆ and FPK equations and the drift and diffusion coefficients for the five classes of quasi-GHS are derived. Finally, the stochastic averaging for a nine-dimensional quasi-partially integrable GHS is given to illustrate the application of the proposed procedure, and the results are confirmed by using those from Monte Carlo simulation.
美国地质调查局确定2010年矿产资源外部研究计划(MRERP)资助项目
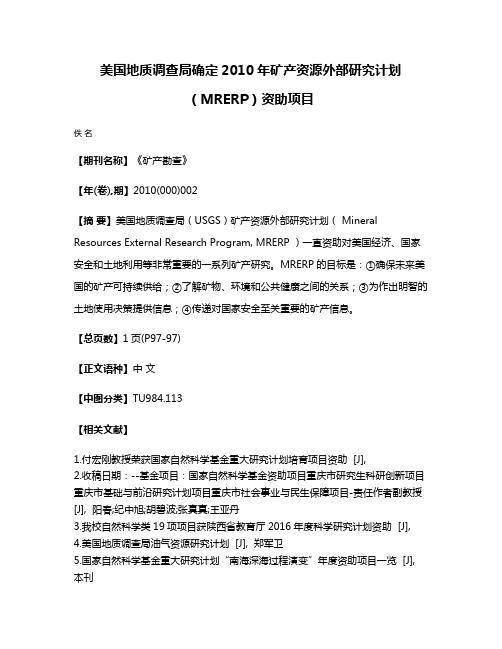
美国地质调查局确定2010年矿产资源外部研究计划
(MRERP)资助项目
佚名
【期刊名称】《矿产勘查》
【年(卷),期】2010(000)002
【摘要】美国地质调查局(USGS)矿产资源外部研究计划( Mineral Resources External Research Program, MRERP )一直资助对美国经济、国家安全和土地利用等非常重要的一系列矿产研究。
MRERP的目标是:①确保未来美国的矿产可持续供给;②了解矿物、环境和公共健康之间的关系;③为作出明智的土地使用决策提供信息;④传递对国家安全至关重要的矿产信息。
【总页数】1页(P97-97)
【正文语种】中文
【中图分类】TU984.113
【相关文献】
1.付宏刚教授荣获国家自然科学基金重大研究计划培育项目资助 [J],
2.收稿日期:--基金项目:国家自然科学基金资助项目重庆市研究生科研创新项目重庆市基础与前沿研究计划项目重庆市社会事业与民生保障项目-责任作者副教授[J], 阳春;纪中旭;胡碧波;张真真;王亚丹
3.我校自然科学类19项项目获陕西省教育厅2016年度科学研究计划资助 [J],
4.美国地质调查局油气资源研究计划 [J], 郑军卫
5.国家自然科学基金重大研究计划“南海深海过程演变”年度资助项目一览 [J], 本刊
因版权原因,仅展示原文概要,查看原文内容请购买。
- 1、下载文档前请自行甄别文档内容的完整性,平台不提供额外的编辑、内容补充、找答案等附加服务。
- 2、"仅部分预览"的文档,不可在线预览部分如存在完整性等问题,可反馈申请退款(可完整预览的文档不适用该条件!)。
- 3、如文档侵犯您的权益,请联系客服反馈,我们会尽快为您处理(人工客服工作时间:9:00-18:30)。
International Journal of Minerals, Metallurgy and Materials Volume 17, Number 4, August 2010, Page 500DOI:10.1007/s12613-010-0348-yCorresponding author: Zhuan Li E-mail: li_zhuan@© University of Science and Technology Beijing and Springer-Verlag Berlin Heidelberg 2010Preparation and properties of C/C-SiC brake compositesfabricated by warm compacted-in situ reactionZhuan Li, Peng Xiao, and Xiang XiongState Key Laboratory of Powder Metallurgy, Central South University, Changsha 410083, China(Received: 12 August 2009; revised: 28 August 2009; accepted: 2 September 2009)Abstract: Carbon fibre reinforced carbon and silicon carbide dual matrix composites (C/C-SiC) were fabricated by the warm compacted-in situ reaction. The microstructure, mechanical properties, tribological properties, and wear mechanism of C/C-SiC composites at different brake speeds were investigated. The results indicate that the composites are composed of 58wt% C, 37wt% SiC, and 5wt% Si. The density and open porosity are 2.0 g·cm–3 and 10%, respectively. The C/C-SiC brake composites exhibit good mechanical properties. The flexural strength can reach up to 160 MPa, and the impact strength can reach 2.5 kJ·m–2. The C/C-SiC brake composites show excellent tribological performances. The friction coefficient is between 0.57 and 0.67 at the brake speeds from 8 to 24 m·s−1. The brake is stable, and the wear rate is less than 2.02×10−6 cm3·J−1. These results show that the C/C-SiC brake composites are the promising candidates for advanced brake and clutch systems.Keywords: C/C-SiC; ceramic matrix composites; tribological properties; microstructure[This work was financially supported by the National High-Tech Research and Development Program of China (No.2006AA03Z560) and the Graduate Degree Thesis Innovation Foundation of Central South University (No.2008yb019).]1. IntroductionRecently, powder metallurgy (PM) and carbon/carbon (C/C) friction pads or linings are two kinds of materials used in the advanced brake system. The advantages of PM brakes are the maturity in material development and low cost, while the main disadvantages are the high density (7.8 g·cm–3) and poor performance at high temperatures [1-2]. C/C brakes are developed to overcome the disadvantages of PM brakes. Compared with PM brakes, C/C brakes have distinct advan-tages, such as excellent thermal and mechanical properties with a lower weight. However, C/C brakes suffer from the insufficient stability of friction coefficient caused by humid-ity. Moreover, C/C composites are prone to oxidation be-yond the temperature of 400°C and high cost, which prevent their wide use as brake composites in passenger cars and trains or emergency brakes of lifts and cranes [3-5].The C/C-SiC composite is a new type of high perform-ance brake material developed following the PM material and C/C composite material. In combination with lower density (about 2.0 g·cm–3), high thermal shock resistance, longer service life, especially lower sensibility to surround-ings and temperature for a silicon carbide share of at least 20wt%, C/C-SiC composites are promising candidates for the advanced friction material in the future [2, 6-7]. Several institutes and industries have been investigating C/C-SiC composites for their use as the friction material in brake pads and disks [8-10]. For example, the researchers of Stuttgart University and German Aerospace Center have applied C/C-SiC composites to the friction domain since the middle of 1990s and developed C/C-SiC brake linings ap-plied in 911 Turbo of Porsche and Audi A8 automobiles.At present, the main preparation methods of C/C-SiC composites are as follows: (1) a gas phase route, also re-ferred to as the chemical vapor infiltration (CVI); (2) a liq-uid phase route including the polymer impregna-Z. Li et al.,Preparation and properties of C/C-SiC brake composites fabricated by warm compacted-in situ reaction 501tion/pyrolysis (PIP) and the liquid silicon infiltration (LSI), which are also called (reactive) melt infiltration (RMI or MI) processes; (3) a ceramic route, i.e., a technique combining the impregnation of the reinforcement with a slurry and a sintering step at high temperatures and high pressures, also referred to as the high-pressure-sinter process (HP-Sinter). Each of the former routes displays both advantages and drawbacks. CVI and PIP with a long preparation process are mainly used to manufacture hot structural C/C-SiC compos-ites for spaceflight [11-13]; RMI has the best figuration and a short fabrication period, but the cost of preforms is high [14-15]. HP-Sinter does harm to carbon fibre and further impacts the composite’s performance.The aim of the current work was to develop an improved technique to reduce the processing and make the C/C-SiC brake composites practical for more industrial applications. The warm compacted-in situ reaction was proposed for fab-ricating the composites. The microstructure, mechanical property, and tribological characterization of the C/C-SiC brake composites were investigated.2. Experimental procedure2.1. Fabrication of the compositesThe short-cut carbon fibre (PAN, T700) with length be-tween 3 and 10 mm was employed, which was supplied by Toray, Japan. The carbon matrix in C/C-SiC composites was phenolic resin and graphite powder in origin. The di-ameter and purity of the silicon powder were 30-50 μm and 99.0%, respectively, according to the information supplied from the manufacturer of Da Zelin-silicon Co. Ltd, Beijing, China. The processing of warm compacted-in situ reaction consists of three main steps, as shown in Fig. 1. The first step involves the mix of C/C-Si green preforms at moderate temperature and pressure and followed by subsequent curing at 160 and 200°C. Curing was done under normal pressure to facilitate the escape of volatile matter by-products of cur-ing reactions and thereby generated a dense matrix with a minimal amount of closed porosity. The average amount of close porosity in the C/C-Si green body after curing was measured to be about 5vol%. The second step involves the pyrolysis of C/C-Si green preforms to convert phenolic resin into resin carbon as parts of carbon matrices in the tempera-ture range of 200-650°C. Consequently, the resin was slowly heated from 200 to 650°C during pyrolysis to allow volatile decomposition products to diffuse through the ma-trix without disrupting the integrity of the composites. Fi-nally, C/C-Si green preforms have the in-situ reaction be-tween 1500 and 1750°C during high temperature treatment (HTT). Thereby, Si reacted with the carbon matrices and then formed the SiC matrix. The density of the resulting C/C-SiC composites was about 2.0 g·cm–3, and the open porosity was about 10%. It is concluded that the warm compacted-in situ reaction exhibits many advantages in comparison to traditional routes, such as simple technology, the large range choice of raw materials, and low fabricationcost.Fig. 1. Schematic representation of the warm compacted-in situ reaction.2.2. Testing methodsThe density and the open porosity of the samples were measured by the Archimedes’ method. The flexural strength and flexural elastic modulus were measured using the three-point-bending method with the samples of 4 mm×10 mm×55 mm, and the loading rate was 0.5 mm·min–1. The compressive strength and compressive elastic modulus were measured with the samples of 10 mm×10 mm×10 mm, and the loading rate was 0.5 mm·min–1. All the tests were con-ducted on a CSS-44100 device at room temperature.The friction and wear properties of the C/C-SiC brake material were tested on a QDM150 friction testing machine with C/C-SiC composites as the static plate and corre-sponding steel disks (HRC 50, 30CrMoSiVA) as the mov-ing plate. The sizes of the test specimens and corresponding steel disks were 25 mm×25 mm×10 mm and φ300 mm, re-spectively. The friction testing required a dry condition, the brake pressure was 1.0 MPa, and the brake for 2000 circles (namely, 1884 m) was at the constant brake speeds of 8, 12, 16, 20, and 24 m·s–1, respectively.The brake speed, brake moment, and brake distance were recorded by computer, and the friction coefficient was502 Int. J. Miner. Metall. Mater ., Vol.17, No.4, Aug 2010transformed from the brake moment. The wear performance was evaluated with the volume wear rate. The volume wear rate was calculated with the weight difference of the sample before and after brake tests divided by the brake speed. 2.3. Microstructure observation and analysisThe microstructure of C/C-SiC brake composites, the morphology of worn surfaces, and wear debris were ana-lyzed by scanning electron microscopy (SEM, JSM- 6360LV). The phases as well as the SiC-polytypes were in-vestigated by X-ray diffraction (XRD, Rigaku-3014).3. Results and discussion3.1. Phases and microstructureThe XRD pattern of C/C-SiC composites is shown in Fig. 2. The phase analysis reveals that the C/C-SiC composites include residual silicon, carbon, and silicon carbide. The gravimetric analysis was employed to determine the content of carbon, residual silicon, and SiC in the composites. By etching the C/C-SiC specimen with the aqueous solution of 90vol% nitric acid and 10vol% hydrofluoric acid at room temperature for 48 h to remove the residual silicon, whereas the contents of carbon fibre and resin carbon matrix were measured by burning it off at 700°C for 10 h in air. There-fore, the content of each component could be calculated.The results indicated that the C/C-SiC composites wereFig. 2. XRD pattern of the C/C-SiC composite.composed of 58wt% C, 37wt% SiC, and 5wt% Si.The microstructures of C/C-SiC composites are shown in Fig. 3. It can be seen that the carbon fibre and matrix dis-tribute alternately in the parallel direction, which is perpen-dicular to the direction of warm compaction (as shown in Fig. 3 (a)). The decomposed products of phenolic resin dif-fuse through the matrix, so there are some microcracks and holes in the matrix during carbonization. With the help of X-ray energy dispersive analysis (EDAX), it is obvious that the round and filiform components are short carbon fibres. The bright white matters are SiC and residual Si. The gray regions are carbon matrices including graphite and resincarbon.Fig. 3. Microstructures of C/C-SiC composites: (a) cross section; (b) optical micrograph.3.2. Mechanical propertiesThe C/C-SiC brake composites fabricated by the warm compressed-in situ reaction show good mechanical proper-ties. The values of flexural strength and impact strength can reach 160 MPa and 2.5 kJ·m –2, respectively, and the values of vertical compression and parallel compression can reach 112 and 84 MPa, respectively.The load-displacement curves and SEM micrographs of fracture surfaces after compressive failure of C/C-SiC com-posites are shown in Figs. 4 and 5, respectively. The vertical compressive failure behavior shows ‘toughness’ and a higher amount of fibre pulled-out on the fracture surface (Fig. 5 (a)), which suggests a weak bonding between the fi-bre and the matrix. The parallel compressive failure behav-ior exhibits elastic response at the initial stage, followed by inelastic behavior as the stress increases. After the stress in-creases to its maximum value, it then decreases rapidly. This is responsible for brittle failure within the specimens. A fracture surface with the matrix sheared is observed after compressive failure (Fig. 5 (b)).Z. Li et al., Preparation and properties of C/C-SiC brake composites fabricated by warm compacted-in situ reaction 503Fig. 4. Typical load-displacement curves for C/C-SiC underthe compressive load.Fig. 5. Typical SEM micrographs of the samples after the compressive test: (a) vertical compression; (b) parallel compression.3.3. Tribological propertiesThe relationships among brake speed, friction coefficient, and wear rate are shown in Fig. 6. Apparently, the friction coefficient is 0.57 at 8 m/s and reaches the maximum value of 0.67 at 16 m/s with the increase of brake speed, which afterward decreases as far as to 0.61 at 24 m/s. The wear rate increases at first and then decreases with the increase of brake speed, but the maximum value of 2.02×10−6 cm 3·J –1 appears at 20 m/s. The tribological phenomena will be dis-cussed later.Fig. 6. Relationship between friction properties and brake speed of C/C-SiC composites.The typical brake curves of C/C-SiC composites at dif-ferent brake speeds are shown in Fig. 7. It can be seen that the shape of the curves is basically similar, and it becomes steadier with the increase of brake speed. At the prior stage of braking, the friction coefficient rapidly rises with a slight ‘prepeak’ in the curve. After the ‘prepeak’, it slowly de-creases and tends to be relatively smooth when the brakeFig. 7. Brake curves of C/C-SiC composites at different brake speeds.504 Int. J. Miner. Metall. Mater ., Vol.17, No.4, Aug 2010condition is stabilized. The cause will be discussed in the following section of worn surface and worn debris analyses. Braking is a mutual affection between two relative mo-tional worn surfaces, so the tribological characteristics are usually determined by brake conditions for certain compos-ites. After the brake test at each speed, the C/C-SiC compos-ites samples showed different friction surfaces. The typical friction surfaces are shown in Fig. 8.The C/C-SiC composites have amounts of micro-peaks on the surfaces of the brake samples, including the hard SiC phase, resin carbon, and the second hard phase Si. These micro-peaks mesh with each other, leading to deformation, shearing, and breaking, which result in the friction coeffi-cient rising at the early stage of each brake and shows the abrasive wear mechanism. At the same time, a large amount of abrasive particles are generated as a result of broken mi-cro-peak ploughing on the friction surfaces (as shown in Fig.8(a)). The higher the brake speed, the more the abrasive par-ticles. Therefore, the friction coefficient and the wear rate at 12 m/s are higher than those at 8 m/s. As shown in Figs. 8(a) and (b), some grooves are left behind on the surfaces of the C/C-SiC brake samples because of the debris ploughing. As the brake speed increases, the brake energy enhances accordingly and reaches the value that makes the wear de-bris deformed, and it fills the micro-valleys in the surfaces of the C/C-SiC brake samples to form friction films covered on the friction surface under the brake pressure. Therefore, when the brake speed reaches 16 and 20 m/s, there are fric-tion films covering the worn surfaces (as shown in Figs. 8(c) and (d)), and there are more at the speed of 20 m/s. The fric-tion films lead to the true friction surface between the C/C-SiC composite samples and the corresponding steel disk enhancing, and the friction coefficient and wear rateincreasing accordingly.Fig. 8. SEM images of the worn surfaces of C/C-SiC brake composites at different brake speeds: (a) 8 m/s; (b) 12 m/s; (c) 16 m/s; (d) 20 m/s; (e) 24 m/s; (f) 24 m/s.Z. Li et al.,Preparation and properties of C/C-SiC brake composites fabricated by warm compacted-in situ reaction 505When the brake speed reaches 24 m/s, the friction film on the friction surface becomes more continuous (as shown in Fig. 8(e)). At the same time, the temperatures of the fric-tional surface of C/C-SiC composites rise fast with the in-crease of brake speed. The friction heat can cause to an un-stable temperature field in the C/C-SiC composites surface for the different coefficients of thermal expansion of various components. This phenomenon causes the thermal expan-sion rates between the frictional surface and the subsurface to become different, as well as the different region of the frictional surface. Meanwhile, the brake pressure leads to an unstable pressure field for the concavo-convex frictional surface of the C/C-SiC composite sample. There are many microcracks on the friction surface because of the unstable temperature and pressure fields (as shown in Fig. 8(f)), they are rubbed repeatedly with the corresponding steel disk and easily fall off, which become flake wear debris.4. Conclusions(1) The warm compacted-in situ reaction offers a poten-tial method for manufacturing C/C-SiC brake composites. The C/C-SiC composites are composed of 58wt% C, 37wt% SiC, and 5wt% Si.(2) The C/C-SiC brake composites exhibit good me-chanical properties, especially excellent toughness, to avoid the catastrophic brittle fracture.(3) The C/C-SiC brake composites show excellent tri-bological performances at different brake speeds, including a good stability of brake and higher friction coefficients between 0.57 and 0.67, in combination with the wear rate less than 2.02×10−6 cm–3·J–1.(4) With a low density of 2.0 g·cm–3, good mechanical properties, outstanding frictional performances, and good abrasive resistance, it is concluded that C/C-SiC brake composites are the promising candidates for advanced brake and clutch systems.References[1] P.P. Yao, H.C. Sheng, X. Xiong, and B.Y. Huang, Worn sur-face characteristics of Cu-based powder metallurgy bake ma-terials for aircraft, Trans. Nonferrous Met. Soc. China,17(2007), No.1, p.99.[2] W. Krenkel, B. Heidenreich, and R. Renz, C/C-SiC compos-ites for advanced friction systems, Adv. Eng. Mater., 4(2002), No.7, p.427.[3] C. Blanco, J. Bermejo, H. Marsh, and R. Menendez, Chemi-cal and physical properties of carbon as related to brake per-formance, Wear, 213(1997), p.1.[4] J.D. Chen, J.H.C. Chern, and C.P. Ju, Effect of humidity onthe tribological behavior of carbon-carbon composites, Wear, 193(1996), p.38.[5] B.K. Yen, Influence of water vapor and oxygen on the tri-bology of carbon materials with sp2 valence configuration, Wear, 192(1996), p.208.[6] S. Vaidyaraman, M. Purdy, T. Walker, and S. Horst, C/SiCmaterial evaluation for aircraft brake applications, [in] The 4th International Conference on High Temperature Ceramic Matrix Composites (HT-CMC4) Proceedings, Munich, 2001, p.802.[7] W.R. Jones and M.J. Jansen, Tribology for space applications,Proc. Inst. Mech. Eng. Part J, 222(2008), No.8, p.997.[8] R. Gadow, Current status and future prospects of CMC brakecomposites and their manufacturing technologies, Ceram.Eng. Sci. Proc., 21(2000), No.3, p.15.[9] Z. Stadler, K. Krnel, and T. Kosmac, Friction behavior of sin-tered metallic brake pads on a C/C-SiC composite brake disc, J. Eur. Ceram. Soc., 27(2007), No.2-3, p.1411.[10] Z. Li, P. Xiao, X. Xiong, and S.H. Zhu, Tribological charac-teristics of C/C-SiC braking composites under dry and wet conditions, Trans. Nonferrous Met. Soc. China, 18(2008), No.5, p.1071.[11] R. Naslain, CVI composites, [in] Ceramic Matrix Composite,Chapman and Hall, London, 1992, p.199.[12] X.H. He, B. Ye, X.H. Qu, C.R. Zhang, and X.G. Zhou, Proc-essing and characterization of C f/SiC composites, J. Univ. Sci.Technol. Beijing, 12(2005), No.5, p.460.[13] Y.W. Kim, J.S. Song, S.W. Park, and J.G. Lee, Nicalon fiberreinforced silicon carbide composites via polymer solution infiltration and chemical vapor infiltration, J. Mater. Sci., 28(1993), p.3866.[14] W. Krenkel, Cost effective processing of CMC compositesby melt infiltration (LSI-Process), Ceram. Eng. Sci. Proc., 22(2001), No.3, p.443.[15] W.B. Hillig, Making ceramic composites by melt infiltration,Am. Ceram. Soc. Bull., 73(1994), No.4, p.56.。