Radio Observations of Galaxy Clusters Connection to Cluster Mergers
科学中观察星空的方法的作文

科学中观察星空的方法的作文Observing the stars in the sky has been a fascination for humans since ancient times. 从古代开始,观察天空中的星星就一直是人类的迷恋。
Before the invention of telescopes, people relied on their naked eyes to observe and study the stars. 在望远镜发明之前,人们依靠赤裸的双眼来观察和研究星星。
The method of naked-eye observation still holds a special place in modern astronomy. 裸眼观测的方法在现代天文学中仍然占据着特殊的位置。
Apart from simply looking up at the sky without any aid, there are numerous tools and techniques that scientists use to observe the stars. 除了简单地凭空仰望,科学家使用了许多工具和技术来观察星星。
One of the most basic tools for observing stars is a pair of binoculars or a small telescope. 观察星星的最基本工具之一是一副双筒望远镜或小型望远镜。
These devices provide a closer and clearer view of the stars and celestial bodies. 这些设备可以更近距离、更清晰地观察星星和天体。
Astronomers can use binoculars or small telescopes to observe the moon, planets, and even some deep-sky objects such as star clustersand nebulae. 天文学家可以使用双筒望远镜或小型望远镜来观察月球、行星,甚至一些深空天体,如星团和星云。
VOA慢速英语听力:爱德文·哈勃改变人类对宇宙的认识
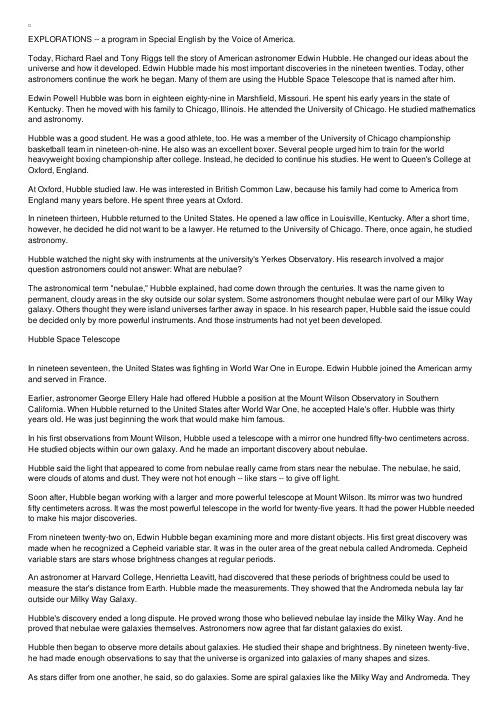
EXPLORATIONS -- a program in Special English by the Voice of America.Today, Richard Rael and Tony Riggs tell the story of American astronomer Edwin Hubble. He changed our ideas about the universe and how it developed. Edwin Hubble made his most important discoveries in the nineteen twenties. Today, other astronomers continue the work he began. Many of them are using the Hubble Space Telescope that is named after him. Edwin Powell Hubble was born in eighteen eighty-nine in Marshfield, Missouri. He spent his early years in the state of Kentucky. Then he moved with his family to Chicago, Illinois. He attended the University of Chicago. He studied mathematics and astronomy.Hubble was a good student. He was a good athlete, too. He was a member of the University of Chicago championship basketball team in nineteen-oh-nine. He also was an excellent boxer. Several people urged him to train for the world heavyweight boxing championship after college. Instead, he decided to continue his studies. He went to Queen's College at Oxford, England.At Oxford, Hubble studied law. He was interested in British Common Law, because his family had come to America from England many years before. He spent three years at Oxford.In nineteen thirteen, Hubble returned to the United States. He opened a law office in Louisville, Kentucky. After a short time, however, he decided he did not want to be a lawyer. He returned to the University of Chicago. There, once again, he studied astronomy.Hubble watched the night sky with instruments at the university's Yerkes Observatory. His research involved a major question astronomers could not answer: What are nebulae?The astronomical term "nebulae," Hubble explained, had come down through the centuries. It was the name given to permanent, cloudy areas in the sky outside our solar system. Some astronomers thought nebulae were part of our Milky Way galaxy. Others thought they were island universes farther away in space. In his research paper, Hubble said the issue could be decided only by more powerful instruments. And those instruments had not yet been developed.Hubble Space TelescopeIn nineteen seventeen, the United States was fighting in World War One in Europe. Edwin Hubble joined the American army and served in France.Earlier, astronomer George Ellery Hale had offered Hubble a position at the Mount Wilson Observatory in Southern California. When Hubble returned to the United States after World War One, he accepted Hale's offer. Hubble was thirty years old. He was just beginning the work that would make him famous.In his first observations from Mount Wilson, Hubble used a telescope with a mirror one hundred fifty-two centimeters across. He studied objects within our own galaxy. And he made an important discovery about nebulae.Hubble said the light that appeared to come from nebulae really came from stars near the nebulae. The nebulae, he said, were clouds of atoms and dust. They were not hot enough -- like stars -- to give off light.Soon after, Hubble began working with a larger and more powerful telescope at Mount Wilson. Its mirror was two hundred fifty centimeters across. It was the most powerful telescope in the world for twenty-five years. It had the power Hubble needed to make his major discoveries.From nineteen twenty-two on, Edwin Hubble began examining more and more distant objects. His first great discovery was made when he recognized a Cepheid variable star. It was in the outer area of the great nebula called Andromeda. Cepheid variable stars are stars whose brightness changes at regular periods.An astronomer at Harvard College, Henrietta Leavitt, had discovered that these periods of brightness could be used to measure the star's distance from Earth. Hubble made the measurements. They showed that the Andromeda nebula lay far outside our Milky Way Galaxy.Hubble's discovery ended a long dispute. He proved wrong those who believed nebulae lay inside the Milky Way. And he proved that nebulae were galaxies themselves. Astronomers now agree that far distant galaxies do exist.Hubble then began to observe more details about galaxies. He studied their shape and brightness. By nineteen twenty-five, he had made enough observations to say that the universe is organized into galaxies of many shapes and sizes.As stars differ from one another, he said, so do galaxies. Some are spiral galaxies like the Milky Way and Andromeda. Theyhave a center, and arms of matter that seem to circle the center like a pinwheel. Others are shaped like baseballs or eggs. A few have no special shape.Hubble proposed a system to describe galaxies by their shape. His system still is used today. He also showed that galaxies are similar in the kinds of bright objects they contain. All galaxies, he said, are related to each other, much as members of a family are related to each other.In the late nineteen twenties, Hubble studied the movement of galaxies through space. His investigation led to the most important astronomical discovery of the Twentieth century -- the expanding universe.Earlier observations about the movement of galaxies had been done by V. M. Silpher. He discovered that galaxies are moving away from Earth at speeds between three hundred kilometers a second and one thousand eight hundred kilometers a second.Hubble understood the importance of Silpher's findings. He developed a plan for measuring both the distance and speed of as many galaxies as possible. With his assistant at Mount Wilson, Milton Humason, Hubble measured the movement of galaxies. The two men did this by studying what Hubble called the "red shift." It also is known as the "Doppler effect."The Doppler effect explains changes in the length of light waves or sound waves as they move toward you or away from you. Light waves from an object speeding away from you will stretch into longer wavelengths. They appear red. Light waves from an object speeding toward you will have shorter wavelengths. They appear blue.Observations of forty-six galaxies showed Hubble that the galaxies were traveling away from Earth. The observations also showed that the speed was linked directly to the galaxies' distance from Earth. Hubble discovered that the farther away a galaxy is, the greater its speed. This scientific rule is called "Hubble's Law."Hubble's discovery meant a major change in our idea of the universe.The universe had not been quiet and unchanging since the beginning of time, as many people had thought. It was expanding. And that, Hubble said, meant it probably began with an explosion of unimaginable force. The explosion often is called "the big bang."Hubble's work did not end with this discovery. He continued to examine galaxies. He continued to gain new knowledge about them. Astronomers from all over the world went to study with him.Edwin Hubble looks through a guidescope of the 1.2 meter telescope at the Palomar Observatory in California.Hubble left the Mount Wilson Observatory during World War Two. He did research for the United States War Department. He returned after the war. Then, he spent much of his time planning a new, much larger telescope in Southern California. The telescope was completed in nineteen forty-nine. It had a mirror five hundred centimeters across. It was named after astronomer George Ellery Hale.Edwin Hubble was the first person to use the Hale Telescope. He died in nineteen fifty-three while preparing to spend four nights looking through the telescope at the sky.Hubble's work led to new research on the birth of the universe. One astronomer said scientists have been filling in the details ever since. And, he said, there is a long way to go.This Special English program was written by Marilyn Rice Christiano. Your narrators were Richard Rael and Tony Riggs. Listen again next week for another EXPLORATIONS program on the Voice of America.。
外星人是否存在的英语作文

外星人是否存在的英语作文英文回答:The Existence of Extraterrestrial Life: A Scientific Inquiry.The question of whether extraterrestrial life exists has captivated humanity for centuries. From ancient myths and folklore to modern scientific inquiry, the search for intelligent beings beyond Earth continues to inspire awe and speculation.From a scientific perspective, the search for extraterrestrial life is guided by two fundamental principles: the Drake equation and the Fermi paradox. The Drake equation attempts to estimate the number of potentially habitable planets in the Milky Way galaxy based on factors such as the rate of star formation, the fraction of stars with planets, and the likelihood of those planets supporting life. While the estimates vary widely, theequation suggests that there could be a significant number of habitable planets in our galaxy.The Fermi paradox, on the other hand, poses the question: "If life is so common, why haven't we detected it yet?" Despite decades of radio telescope observations, space exploration missions, and SETI (Search for Extraterrestrial Intelligence) programs, we have yet to establish contact with extraterrestrial civilizations. This apparent contradiction has led to a range of hypotheses, including the Great Filter theory, which suggests that there are barriers that prevent most civilizations from advancing to a detectable level of intelligence.Recent astronomical discoveries have provided further impetus to the search for extraterrestrial life. The confirmation of exoplanets, planets outside our solar system, has shown that our Milky Way galaxy is teeming with planetary systems. Moreover, the discovery of potentially habitable exoplanets, such as TRAPPIST-1 and Proxima Centauri b, has raised the possibility that life may exist not only on Earth but also on nearby planets.中文回答:外星人是否存在,一个科学探究。
Deep HST Observations of Star Clusters in NGC 1275

a rXiv:as tr o-ph/98219v12Fe b1998Deep HST Observations of Star Clusters in NGC 12751Matthew N.Carlson 2,Jon A.Holtzman 2,Alan M.Watson 3,Carl J.Grillmair 4,Jeremy R.Mould 5,Gilda E.Ballester 6,Christopher J.Burrows 7,John T.Clarke 6,David Crisp 4,Robin W.Evans 4,John S.Gallagher III 8,Richard E.Griffiths 9,J.JeffHester 10,John G.Hoessel 8,Paul A.Scowen 10,Karl R.Stapelfeldt 4,John T.Trauger 4,and James A.Westphal 11ABSTRACT We present an analysis of compact star clusters in deep HST/WFPC2images of NGC 1275.B and R band photometry of roughly 3000clusters shows a bimodality in the B-R colors,suggesting that distinct old and young clusterpopulations are present.The small spread in the colors of the blue clusters isconsistent with the hypothesis that they are a single age population,with aninferred age of0.1to1Gyr.The luminosity function shows increasing numbersof blue clusters to the limit of our photometry,which reaches several magnitudespast the turnover predicted if the cluster population were identical to currentGalactic globulars seen at a younger age.The blue clusters have a spatialdistribution which is more centrally peaked than that of the red clusters.Theindividual clusters are slightly resolved,with core radii<∼0.75pc if they havemodified Hubble profiles.We estimate the specific frequencies of the old andyoung populations and discuss the uncertainties in these estimates.Wefind thatthe specific frequency of the young population in NGC1275is currently largerthan that of the old population and will remain so as the young populationevolves,even if the majority of the low mass clusters are eventually destroyed.If the young population formed during a previous merger,this suggests thatmergers can increase the specific frequency of globulars in a galaxy.However,the presently observed young population likely contains too few clusters to havea significant impact on the overall specific frequency as it will be observed inthe future.1.IntroductionEarly HST observations of NGC1275,the central galaxy in the Perseus cluster, revealed a population of about60blue(V-R∼0.3)star clusters surrounding the nucleus (Holtzman et al.1992).The color of these clusters suggests an age of roughly300million years based on the models of Charlot and Bruzual(1991).Spectra of the brightest object (Zepf et al.1995)also suggest an age of0.1to0.9Gyr,based on a comparison of the observed line widths to those predicted by the models of Bruzual and Charlot(1993).None of the clusters seem to have Hαemission,with the exception of one object found by Shields &Filippenko(1990),which appears to be a much younger object.The blue clusters in the original WFPC1images appear unresolved,suggesting sizes of less than15parsecs.The brightest object has V=18.9,which corresponds to M V=-15.8for H0=75km/s/Mpc and cz=5264km/s(Strauss et al.1992).The brightnesses of the blue clusters suggest masses of between2×104and1×108M⊙,depending on the assumed age and distance, if one assumes that the objects are star clusters with a Salpeter IMF.The observed sizes, luminosities,and the estimated masses suggest that these objects may be young analogues of globular clusters.In the past several years,massive young clusters have been observed in a variety of other galaxies.Lutz(1991)detected young globular cluster candidates in a ground-based study of the merger remnant NGC3597,and these have been confirmed to be compact by HST observations(Holtzman et al.1996).Candidate young globular clusters have also been found in other interacting systems,including NGC7252(Whitmore et al.1993), NGC4038/9(Whitmore&Schweizer1995),NGC3921(Schweizer et al.1996),among others.A few massive clusters are seen in the starburst galaxy NGC253(Watson et al.1996),while others have been detected in the ring galaxies NGC1097and NGC6951 (Barth et al.1995)and the dwarf galaxies NGC1569,NGC1705(O’Connell et al.1994), and He2-10(Conti&Vacca1994).While no generally accepted picture has emerged as to what conditions lead to the formation of young clusters,galaxy interactions appear to be an important component.It is particularly difficult to determine the precise mechanism responsible for the presence of these objects in NGC1275because of the myriad peculiarities of this galaxy, including the presence of a significant amount of dust,streamers of Hαemission,an active nucleus,and the location of the galaxy at the center of a coolingflow in the Perseus cluster. Two hypotheses have been offered for the origin of these clusters,namely,that they were formed from the substantial mass deposition of the Perseus cluster coolingflow(200M⊙/yr) or that the cluster formation was triggered by a galaxy-galaxy interaction.Holtzman et al.(1992)preferred the latter hypothesis based on the observed lack of spread in the WFPC1colors,which implies a common age for the objects,and the appearance of a ripple in the galaxy light which suggests that a previous interaction may have occurred.Richer et al.(1993)found larger color spreads in high resolution CFHT images and preferred the coolingflow hypothesis.However,Holtzman et al.(1996)did not detect young clusters in three of a sample of four other coolingflow galaxies;the central cluster galaxy in Abell1795 may have a few clusters,but it also has a peculiar morphology which suggests a previous interaction.The merger hypothesis is particularly interesting in light of the theory that elliptical galaxies form through mergers and the observation that ellipticals have higher specific frequencies of globular clusters than spirals,where the specific frequency is a measure of the number of globular clusters per unit luminosity of the host galaxy.Ashman&Zepf (1992)investigate the proposition that clusters might form during mergers and suggest that,in such an event,cluster formation would occur in a brief burst,resulting in a set of newly formed clusters with a common age and color.This would lead to a cluster system with a bimodal distribution of cluster colors reflecting the difference in metallicity or age (or both)between the original and the newly formed clusters.They predict that the spatial distribution of the younger clusters would be more sharply peaked toward the center of thegalaxy than that of the old cluster system,because the old globular cluster populations of the two progenitor galaxies would remain spatially extended and probably be dynamically heated during the merger,while the new clusters would be formed out of gas which becomes more centrally concentrated during a merger.However,as Harris et al.(1995)note,an increase in specific frequency as a result of a merger requires not only that globular clusters form during such an event,but that they form preferentially over non-cluster stars as compared to the ratio of clusters to background stars in the progenitor galaxies.Few estimates of the specific frequency of these young cluster systems exist.Watson et al.(1996)suggest that the4young clusters in NGC253most likely have formed with a large specific frequency.In the merger remnant NGC3921Schweizer et al.(1996)find that the blue clusters will increase the overall number of clusters enough that the galaxy will come to have the specific frequency of an elliptical ler et al.(1997)find that the specific frequency of globular clusters in the merger remnant NGC7252will rise over the next15Gyr as the background population fades to resemble that of an elliptical. These results all suggest that interactions can increase the specific frequency in a galaxy.Luminosity functions of old globular cluster systems have been well studied and used as a part of the extragalactic distance scale because of their uniformity from galaxy to galaxy;they are roughly Gaussian in shape with a peak near M V=-7.3(Harris1996).The luminosity functions of most of the recently discovered young cluster systems are poorly determined because of either small number statistics(few young clusters in the galaxy)or incompleteness.A notable exception is the young cluster system in NGC4038/9,which shows no turnover to two magnitudes fainter than the turnover predicted for a typical old cluster system,even after allowing for the expected fading of the clusters based on stellar population models.Based on this,van den Bergh(1995)argues that this cluster system may be intrinsically different from old globular cluster systems.However,Mateo (1993)notes that,at least in the LMC,the observed increasing cluster luminosity function is well modelled by a combination of globular and open clusters.Also,several authors have recently suggested that substantial numbers of clusters may be destroyed over a Hubble time(Gnedin&Ostriker1997;Elmegreen&Efremov1997);mechanisms include evaporation and tidal disruption by galactic bulges and disks.We have obtained WFPC2images of NGC1275which go about4magnitudes deeper (in the red)than the WFPC1observations of Holtzman et al.(1992).These observations provide more accurate colors than previous observations and probe the cluster population to approximately2.5magnitudes fainter than the turnover expected if this population is identical to the Galactic globular cluster system seen at an younger age.Section2briefly summarizes the observations and the reductions.Section3discussesour analysis and potential sources of error.In section4we present our results including the photometry,luminosity function of the clusters,surface density distribution,an estimate of the specific frequency,and analysis of the sizes of the objects.2.Observations&Data ReductionObservations of NGC1275were made with the WFPC2on1995November16in the F450W and F702Wfilters.Exposure times in F450W were200,1000,and3×1300 seconds.In F702W the exposures were200,900,1000,and2×1300seconds.The F450W and F702Wfilters are roughly similar to broad band B and Rfilters.The data were reduced using the procedures discussed by Holtzman et al.(1995a). The individual exposures were all taken with a common pointing,and the frames in each color were combined.Cosmic rays were rejected in the averaging based on the expected variance from photon statistics and readout noise;an extra term was included in the expected variance which was proportional to the signal in the pixel to allow for small pointing differences between frames.3.AnalysisCombined color images of NGC1275in the PC and in the WFPC2fields are shown in Figures1a and1b,respectively.The nucleus is in the center of the PC,and the other bright point source in the PC is a foreground F star(Hughes&Robson1991).Almost all of the remaining point sources are candidate star clusters in NGC1275.To detect the compact clusters,we summed the two colors and subtracted a5×5 boxcar-smoothed version of this image.We divided this by the square root of the smoothed image to provide an image whose units were proportional to the local noise(assuming the noise is dominated by the background,as is the case here).We then used IRAF’s DAOFIND to detect objects on the resulting image,in order to provide a uniform detection threshold in signal-to-noise ratio across the image.The choice of detection threshold was determined by minimizing the number of spurious detections and maximizing the number of faint objects detected,judging from a visual inspection.Objects werefiltered by shape based on roundness and sharpness criteria computed by DAOFIND;we required-1< roundness<1and0.2<sharpness<1.Several areas of the frame were masked out, including the galaxy nucleus,the bright saturated star in the PC,and a few bright stars and galaxies in the WFs.Aperture photometry was performed using a1-pixel radius aperture in both the PC and the WFs.The small aperture was used to reduce background related errors,which are large in the central region of the galaxy.Sky values were determined by taking an estimate of the mode in a background annulus;in the PC,the annulus was2pixels wide with an inner radius of12pixels,while in each WF the corresponding annulus was3pixels wide with an inner radius of6pixels.We chose to use small sky annuli because the galaxy background is variable on small scales.Corrections from1-pixel apertureflux toflux within0.5′′were determined from the nine brightest sources.The average aperture corrections were1.03and1.15magnitudes in the PC frame for F450W and F702W,respectively,and0.63and0.71magnitudes in the WFs.These are slightly larger than the corrections for bright point sources measured by Holtzman et al.(1995a),but this is expected if these objects are slightly resolved.In the PC,measured aperture corrections varied on the order of0.05mag between different objects.Measured variations in the aperture corrections for the individual WFs were∼0.02 mag,so an average aperture correction was assumed for all three.If there is an intrinsic spread in cluster sizes,using a single aperture correction for all objects would lead to systematic errors in the derived magnitudes.Tofirst order,however,colors are unaffected by such a spread.The transformations from HST magnitudes(F450W,F702W)to Cousins magnitudes(B,R)were made using the transformations given by Holtzman et al.(1995b).Foreground Galactic extinction in the direction of NGC1275was taken to be A B= 0.70(Burstein&Heiles1984).We determined the extinction in the WFPC2passbands by numerically integrating the product of an A star spectrum,thefilter transmission,the system response,and an extinction curve from Cardelli et al.(1989)derived assumingR V=3.1.An A star was used because of its similarity to the observed spectrum of the bright clusters.This gave A F450W=0.69and A F702W=0.40.These estimates do not include internal extinction,however,which may be important in NGC1275since patchy dust is evident;this is discussed further below.Potential photometry errors include intrinsic Poisson error in the signal,error in the aperture correction,and error in the background level determination.Typical errors were estimated from the simulated clusters from our completeness tests and are shown in Figure 2.The dashed lines in color and magnitude indicate input magnitudes for the artificial clusters.Completeness was estimated by generating simulated objects at each of9different input magnitudes(23.75to27.75in B).The simulated objects were given the same noise characteristics as real objects.In the PC,1000objects were placed randomly in each of3 separate annuli to better model the variation of completeness with distance from the centerof the galaxy.Each set of artificial clusters was given a spatial distribution which mimicked the distribution of the brightest(B<25)blue and red clusters.In the WFs,the1000 artificial objects were placed randomly in a uniform distribution.On each chip,a PSF of one of the isolated bright clusters was used to create the simulated objects(the use of other objects gave comparable results).Two sets of artificial objects were placed to estimate the difference in detection efficiency for objects of two different colors;the two sets of simulated clusters were given B-R=0.7and B-R=1.6,comparable to the colors of the observed cluster populations(next section).The derived average completeness(weighted by the spatial distribution of the observed clusters)as a function of B magnitude is shown in Figure3for the whole image as well as for the section which fell in the PC.We estimated the number of foreground stars based on the models of Bahcall and Soneira(1980)and expect<4objects with B<27in the PC.Since we expect such small numbers of foreground stars,we have neglected them.4.Results4.1.PhotometryWe present the photometry of all detected objects in NGC1275by plotting color against apparent magnitude in Figure4a.Extinction-corrected absolute magnitudes and colors are shown as well,using a distance of70.2Mpc determined by assuming pure Hubble flow with H0=75km/s/Mpc.Wefind1181blue objects(B-R<1.1)and1855red objects. Distinct red and blue populations are seen,although there is significant color scatter. Roughly50%of the blue objects are seen in the PC,as compared to about15%of the red objects.These objects cannot be single stars because they are marginally resolved and because they are too bright.With the exception of the Shields-Filippenko object,the objects lack Hαemission(Holtzman et al.1992),which precludes their being emission line objects. Consequently,they are probably star clusters,as supported by spectroscopy of the brightest object(Zepf et al.1995).They are brighter and more compact than open clusters in the Galaxy;the brightest object is250times brighter than the brightest Galactic open cluster. Their closest analog seems to be globular clusters,although the inferred ages are much smaller(see below).To test whether the observed color scatter is likely to be related to internal extinction within NGC1275and photometric errors from errors in background subtraction,we show in Figure4b only clusters in the southwestern half of the PC frame which have estimatedphotometric errors of less than0.15mag in both B and R.This region was chosen because it contains fewer obvious signatures of dust than the northeastern region.In fact,wefind that the spread in color among the blue objects in Figure4b is consistent with that associated with photometric errors(cf.Figure2),supporting the conclusion that the observed color spread within the two populations is likely to be caused by the variation of internal extinction in the environment of the galaxy,rather than differences in age.We conclude that the blue clusters are a single color,single age population,with the spread in colors coming from Poisson errors and background subtraction errors.Figure4b shows a distinct bimodality in the cluster population,consistent with the presence of two discrete populations.The blue clusters have a typical dereddened(B-R)0∼0.4.This is much bluer than typical old globular clusters,which have(B-R)0∼1.2(Harris1996).The uniformity of the colors suggests that the clusters were formed in a relatively short duration single burst rather than an ongoing or continuous process.Figure5shows the magnitude and color evolution of a single burst population for a variety of metallicities,assuming a Salpeter initial mass function from0.1to125M⊙and a total mass of1M⊙(Bruzual&Charlot 1993).If we compare the measured colors of the blue clusters in the PC to the models,we infer ages of107to109years,allowing for small possible systematic errors in the models and the data as well as the unknown metallicity of the objects.We note that the clusters are far too blue to be explained by a metallicity effect alone.Ages younger than107years are ruled out by the absence of Hαemission from the clusters.Spectra of the clusters(Zepf et al.1995)argue for a several hundred Myr old population dominated in the blue by A type stars.It is also simply less probable that we that we should happen to observe these clusters at a younger age.The LMC has a number of compact clusters with comparable colors(van den Bergh 1981).These objects are substantially fainter than the brightest objects in NGC1275, implying that they are less massive.Still,observations of blue clusters in the LMC,where we can directly measure ages using the main sequence turnoffin color-magnitude diagrams, give us a less model dependent idea of the spread in colors that could be expected from a spread in ages.Figure6shows the distribution of B-V versus age for LMC clusters;ages are from Elson&Fall(1988)and Hodge(1981,1983),and colors from van den Bergh(1981). Between108and109years,we see that the integrated colors of the clusters cover a range of 0.3in B-V.This argues against a large spread in the ages for the NGC1275clusters,which show no such spread in colors.While our observations were in B and R,not B and V,the color spread in B-R for the same age range would be0.8magnitudes,assuming a V-R vs B-R relation from the population synthesis models.Given ages,we can estimate masses for the clusters based on their luminosities.Age estimates of108and109years give mass estimates for the brightest object of2×107and 108M⊙,respectively.Blue clusters at our completeness limit of B=27have masses of2×104to105M⊙.These masses are very comparable with those of Milky Way globulars, which typically have masses between104and3×106M⊙,with a handful of clusters with M<104M⊙(Mandushev,Spassova,&Staneva1991,Pryor and Meylan1993).Our mass estimates depend on the assumption of a Salpeter IMF.Two of the three brightest objects in the southwest half of the PC(Figure4b)have much redder colors than the rest of the blue clusters(B-R of0.95and1.23).While these objects are close to the nucleus,they are bright enough that errors in determining the galaxy background are not a large source of error.Possible explanations are reddening by dust,a younger age(slightly less than107years old)when the integrated light is dominated by red supergiants(Leitherer&Heckman,1995),or the identification of these objects with late type foreground stars.For the redder object,the last interpretation is supported by our measurement of sizes(§4.5),since it appears to be unresolved while almost all other objects appear marginally resolved.We suggest that the objects with B-R∼1.6(∼1.3dereddened)are the old population of globular clusters around NGC1275.These are slightly redder than Galactic globular clusters which have an average(B-R)0∼1.2.The brightest(M B=-10.7)has a magnitude similar to the brightest globulars seen in other central cluster galaxies.4.2.Luminosity FunctionFigure7a shows the B luminosity function for the blue clusters(B-R<1.1),and Figure7b shows the B luminosity function for the red clusters(B-R>1.1).The dotted lines in each plot show the results after correction for incompleteness.The luminosity function of the blue clusters looks distinctly different from that expected for a typical old globular cluster population at a younger age.While the Galactic globular cluster luminosity function is Gaussian in shape with peak at M B∼-6.6andσ=1.0mag(Harris1996),the luminosity function for the clusters in NGC1275is more closelyfit by a exponential.If we were to observe the current Galactic globular cluster system at an age of500million years and at the distance of NGC1275(and the same Galactic extinction),we would observe a turnover in the luminosity function at B∼24(H0=75km/s/Mpc).This prediction assumes4magnitudes(13Gyr)of fading as suggested by the models of Bruzual&Charlot (1993).Our photometry is complete at the50%level to3magnitudes fainter than this,yet there is no evidence of a turnover in the luminosity function of the clusters in NGC1275.The luminosity function of the blue clusters has the same trend as that of Galactic open clusters,namely,an increasing number of clusters at fainter magnitudes with no turnover (van den Bergh and LaFontaine1984).However,the most luminous clusters in NGC1275 are brighter than any observed Galactic open clusters,despite being older than the brightest of them.The compactness as inferred from the HST images is also different from that of open clusters(§4.5).Mateo(1993)finds that an increasing luminosity function for clusters in the LMC can be produced by a mixed population of true globulars and open clusters.In NGC1275,a similar explanation would require a large number of open clusters which are far more massive than any Galactic open clusters.Consequently,as a system,these clusters are different from any cluster system seen in Local Group galaxies.As individual objects, however,the physical properties appear most similar to globular clusters.In Figure8,we compare the luminosity function of clusters in NGC1275with the luminosity functions in several of the other galaxies where reasonable numbers of blue clusters are found,as well as with the luminosity functions of Milky Way clusters.Dashed lines show rough completeness limits for the different observations,and dotted lines give the location of the expected turnover given the various age estimates of the clusters.There is no strong evidence in any of the young cluster systems for an intrinsic turnover in the luminosity function.If these objects will evolve to look like the Galactic globular cluster system,some mechanism must preferentially destroy low luminosity,low mass clusters on timescalesof13Gyr.Two possibilities are tidal disruption and evaporation(Gnedin&Ostriker 1997;Elmegreen&Efremov1997).For tidal disruption to preferentially destroy low mass clusters,such clusters must have a lower mean density than more massive clusters. Evaporation through two-body relaxation can preferentially destroy lower mass clusters as their stars escape the cluster potential well and are captured by the gravitational potential well of the galaxy.Gnedin and Ostriker(1997)predict that from half to three quarters of the initial globular cluster population in a galaxy may be destroyed by a combination of these processes in a Hubble time.4.3.Surface Density ProfileFigure9shows the surface density distribution of red and blue clusters(open triangles andfilled triangles,respectively).Corrections have been made for incomplete annuli at large radii,for masked areas,and for differing completeness levels with distance from the bright galaxy center.Error bars are simple Poisson errors in counts of clusters.The central area,within18pixels(0.8arcsec)from the core of the galaxy,has been omitted from thesurface density profile due to the bright nucleus.It is clear that the blue clusters are more centrally concentrated than the red ones.Wefit the surface density distribution of the blue and red cluster systems from4′′to120′′with power laws.For the blue clusters,wefind that a power law slope of-1.3 provides a reasonablefit over the entire range.For the red clusters,we note aflattening in the inner portion of the distribution andfit the surface density with two power laws. Inside0′.5,we measure a slope of-0.6,while outside0′.5wefind a slope of-1.0.Flattening in the central part of the surface density distribution of a globular cluster system is also seen in other similar galaxies,such as M87(McLaughlin1995)and NGC5128(Harris et al.1984).Our measurements for the distribution of old clusters are in good agreement with those of Kaisler et al.(1996).However,they adopt a power law slope of-1.3,based on the observational relation between galaxy magnitude and power law slope of the surface density distribution of the globular cluster system(Harris et al.1986,1993).We note that our measured outer slope is sensitive to the choice of break point between the inner and outer regions;the data show a sign of steepening even more in the outermost parts. The spatial distribution of the clusters agrees with the galaxy merger model of Ashman and Zepf(1992),which predicts that the younger clusters will be peaked towards the center of the galaxy,while the older clusters will be moreflattened in their distribution.4.4.Specific FrequencyThe specific frequency of globular clusters in a galaxy is given byS N=N T100.4(15+M V)(Harris and van den Bergh1981)where N T is the total number of clusters and M V is the absolute V magnitude of the galaxy.This is of interest in light of the hypothesis that elliptical galaxies form via mergers of spirals and the observation that ellipticals havea higher specific frequency of globular clusters than spirals.Consequently,the merger hypothesis requires that globular clusters form during mergers with a higher specific frequency than that of the progenitor population.Also,it requires that clusters form in numbers comparable to those of the old cluster population(Zepf&Ashman1993).To address these questions,we calculate the specific frequency of the old population,the current specific frequency of the merger related population,and the predicted specific frequency of the merger related population in13Gyr.To calculate specific frequencies,we need to estimate the total number of clusters。
AGN-Galaxy Clustering with the Panoramic Deep Fields
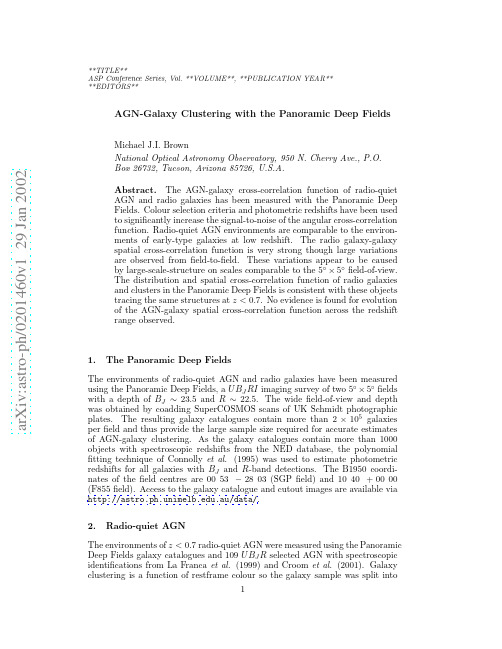
The environments of z < 0.7 radio-quiet AGN were measured using the Panoramic Deep Fields galaxy catalogues and 109 U BJ R selected AGN with spectroscopic identifications from La Franca et al. (1999) and Croom et al. (2001). Galaxy clustering is a function of restframe colour so the galaxy sample was split into
Michael J.I. Brown National Optical Astronomy Observatory, 950 N. Cherry Ave., P.O. Box 26732, Tucson, Arizona 85726, U.S.A.
Abstract. The AGN-galaxy cross-correlation function of radio-quiet AGN and radio galaxies has been measured with the Panoramic Deep Fields. Colour selection criteria and photometric redshifts have been used to significantly increase the signal-to-noise of the angular cross-correlation function. Radio-quiet AGN environments are comparable to the environments of early-type galaxies at low redshift. The radio galaxy-galaxy spatial cross-correlation function is very strong though large variations are observed from field-to-field. These variations appear to be caused by large-scale-structure on scales comparable to the 5◦ × 5◦ field-of-view. The distribution and spatial cross-correlation function of radio galaxies and clusters in the Panoramic Deep Fields is consistent with these objects tracing the same structures at z < 0.7. No evidence is found for evolution of the AGN-galaxy spatial cross-correlation function across the redshift range observed.
罕见的双星系统文献英语翻译

罕见"闪光灯"恒星实际可能是双星系统This Hubble image shows a a mysteriousprotostar, LRLL 54361, that behaves like a flashing light. The image wasreleased Feb. 7, 2013.CREDIT: NASA, ESA, J. Muzerolle (STScI)这幅哈勃望远镜图像显示了一个神秘原恒星LRLL 54361,其行为像一个闪光灯。
该图像发布于2013年2月7日。
来源:美国宇航局、欧空局、J·沐泽洛尔(太空望远镜科学研究所)An odd flashing star may actually be a pairof cosmic twins: two newly formed ba by stars that circle each other closely andflash like a strobe light, scientist s say.一颗古怪闪烁恒星实际上可能是一对宇宙双胞胎:两颗新形成幼年恒星彼此紧密环绕并且像一个闪光灯一样闪烁,科学家说。
Astronomers discovered the nascent starsystem, called LRLL 54361, with the infr ared Spitzer observatory and the HubbleSpace Telescope, and say the rare cosmic find could offer a chance to studystar formation and early evolution. It is on ly the third such "strobelight" object ever seen, researchers said.天文学家通过斯皮策红外观测站和哈勃太空望远镜发现了这个新生称为LRLL 54361恒星系统,并且表示这个罕见宇宙发现可能提供一种研究恒星形成和早期演化机会。
银河系漫游指南英文版pdf
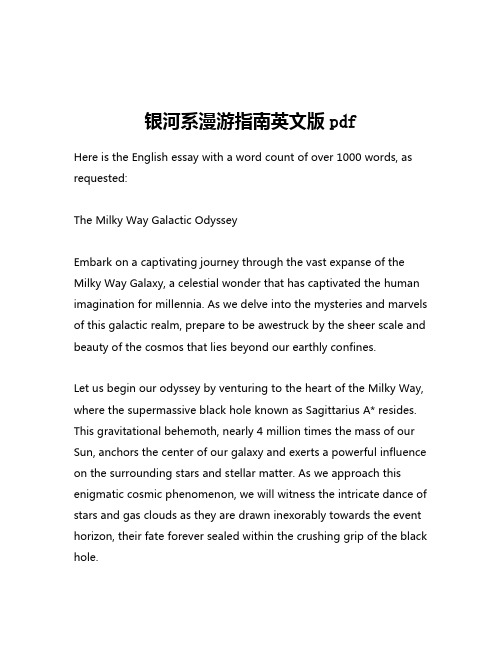
银河系漫游指南英文版pdfHere is the English essay with a word count of over 1000 words, as requested:The Milky Way Galactic OdysseyEmbark on a captivating journey through the vast expanse of the Milky Way Galaxy, a celestial wonder that has captivated the human imagination for millennia. As we delve into the mysteries and marvels of this galactic realm, prepare to be awestruck by the sheer scale and beauty of the cosmos that lies beyond our earthly confines.Let us begin our odyssey by venturing to the heart of the Milky Way, where the supermassive black hole known as Sagittarius A* resides. This gravitational behemoth, nearly 4 million times the mass of our Sun, anchors the center of our galaxy and exerts a powerful influence on the surrounding stars and stellar matter. As we approach this enigmatic cosmic phenomenon, we will witness the intricate dance of stars and gas clouds as they are drawn inexorably towards the event horizon, their fate forever sealed within the crushing grip of the black hole.Venturing outwards from the galactic center, we will encounter the diverse and vibrant neighborhoods that make up the Milky Way. Spiral arms, such as the Orion Arm in which our Solar System resides, are vast regions of star formation, with newborn stars and stellar nurseries dotting the landscape. We will marvel at the brilliant nebulae, glowing clouds of gas and dust that serve as the birthplaces of these young celestial bodies, their ethereal hues and intricate structures a testament to the dynamic processes that shape the galaxy.As we traverse the spiraling arms, we will come across the globular clusters – ancient, densely packed collections of stars that orbit the galactic center. These spherical assemblages, some of the oldest objects in the Milky Way, harbor valuable insights into the early history and evolution of our galaxy, their stars dating back to a time when the universe was a mere fraction of its current age.Amidst the stellar tapestry, we will discover the diverse array of stellar populations that call the Milky Way home. From the towering red giants, their brilliant crimson hues a testament to their advanced age and increased size, to the compact and enigmatic neutron stars, the collapsed remnants of once-mighty suns. Each type of star, with its unique properties and life cycle, contributes to the rich tapestry of the galactic landscape.But the Milky Way is not merely a collection of stars – it is a dynamic and ever-changing system, influenced by the complex interplay of gravity, stellar evolution, and the ever-present threat of cosmic catastrophes. We will explore the regions where massive stars meet their explosive demise, supernovae that briefly outshine entire galaxies and leave behind the dense, spinning neutron stars known as pulsars. These cataclysmic events not only shape the galactic environment but also provide the building blocks for new generations of stars and planets.As we venture deeper into the Milky Way, we will encounter the harrowing regions where the fabric of space-time is stretched and distorted by the intense gravitational fields of neutron stars and black holes. Here, we will witness the bizarre and mind-bending phenomena predicted by Einstein's theory of general relativity, from the warping of spacetime to the accretion disks that feed these cosmic monsters.Throughout our journey, we will be in awe of the sheer scale and majesty of the Milky Way. The galaxy, spanning nearly 100,000 light-years in diameter, is home to an estimated 200 to 400 billion stars, each one a unique and fascinating world unto itself. We will ponder the possibility of life elsewhere in this vast cosmic tapestry, wondering if intelligent civilizations have arisen on distant worlds and if they, too, gaze up at the night sky, marveling at the splendorof our shared galactic home.As our odyssey draws to a close, we will reflect on the profound impact that the study of the Milky Way has had on our understanding of the universe. From the groundbreaking work of pioneering astronomers to the cutting-edge research conducted with the most advanced observational tools, the Milky Way has been a constant source of fascination and discovery. And as we look to the future, we know that there are countless more secrets and mysteries waiting to be unveiled, beckoning us to continue our exploration of this awe-inspiring celestial realm.So let us embark on this Milky Way galactic odyssey, armed with a sense of wonder and a thirst for knowledge. For in unraveling the mysteries of our galactic home, we may just find the answers to some of the most profound questions that have puzzled humanity since the dawn of time.。
探索宇宙之谜:解码宇宙起源的秘密
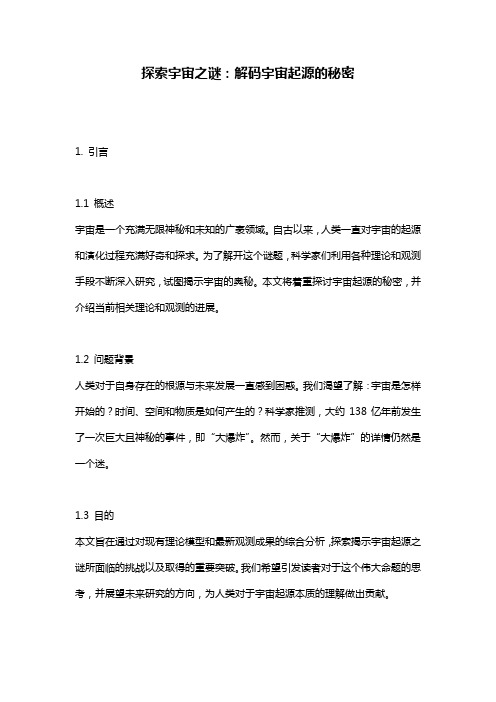
探索宇宙之谜:解码宇宙起源的秘密1. 引言1.1 概述宇宙是一个充满无限神秘和未知的广袤领域。
自古以来,人类一直对宇宙的起源和演化过程充满好奇和探求。
为了解开这个谜题,科学家们利用各种理论和观测手段不断深入研究,试图揭示宇宙的奥秘。
本文将着重探讨宇宙起源的秘密,并介绍当前相关理论和观测的进展。
1.2 问题背景人类对于自身存在的根源与未来发展一直感到困惑。
我们渴望了解:宇宙是怎样开始的?时间、空间和物质是如何产生的?科学家推测,大约138亿年前发生了一次巨大且神秘的事件,即“大爆炸”。
然而,关于“大爆炸”的详情仍然是一个迷。
1.3 目的本文旨在通过对现有理论模型和最新观测成果的综合分析,探索揭示宇宙起源之谜所面临的挑战以及取得的重要突破。
我们希望引发读者对于这个伟大命题的思考,并展望未来研究的方向,为人类对于宇宙起源本质的理解做出贡献。
在接下来的内容中,我们将首先探讨宇宙的起源理论,包括著名的大爆炸理论、平行宇宙假说和弦理论。
随后,我们将介绍观测宇宙的手段与进展,例如天文望远镜和射电望远镜等工具的运用。
然后,我们将深入探讨暗物质和暗能量这两个令科学家们困扰已久的谜题,并提出新思路和方法。
最后,在结语部分,我们将总结目前存在的难题,并展望未来研究的重点及前沿展望。
通过这篇文章,我们希望读者能够加深对于宇宙起源秘密的认识,并意识到解开这个谜题所带来的意义和价值。
随着科学技术不断发展,我们有信心逐渐揭示宇宙背后无尽奥秘,为人类提供更深刻、更全面地认识自身存在和命运的机会。
2. 宇宙的起源理论探讨2.1 大爆炸理论大爆炸理论是关于宇宙起源的一种主流理论。
根据这个理论,宇宙在约138亿年前发生了一次巨大的爆炸,从而形成了我们今天所看到的宇宙。
在大爆炸之前,整个宇宙存在于一个非常高温、非常致密的状态中。
随着时间的推移,物质在空间中扩散和冷却,形成了星系、行星和其他天体。
虽然大爆炸理论得到了广泛认可,并能够解释很多观测数据,但仍有一些问题需要解决。
- 1、下载文档前请自行甄别文档内容的完整性,平台不提供额外的编辑、内容补充、找答案等附加服务。
- 2、"仅部分预览"的文档,不可在线预览部分如存在完整性等问题,可反馈申请退款(可完整预览的文档不适用该条件!)。
- 3、如文档侵犯您的权益,请联系客服反馈,我们会尽快为您处理(人工客服工作时间:9:00-18:30)。
a r X i v :a s t r o -p h /0612185v 1 7 D e c 2006RADIO OBSER V ATIONS OF GALAXY CLUSTERS:CONNECTION TO CLUSTER MERGERS L.FERETTI Istituto di Radioastronomia INAF,Via P.Gobetti 10140129Bologna,Italy The main component of the intracluster medium (ICM)in clusters of galaxies is represented by the X-ray emitting thermal plasma.In addition,the presence of relativistic electrons and large-scale magnetic fields in a fraction of galaxy clusters is demonstrated by the detection of large-scale synchrotron radio sources,which have no optical counterpart and no obvious connection to the cluster galaxies.Observational results provide evidence that these phenomena are related to cluster merger activity,which supplies the energy for the reacceleration of the radio emitting particles.The investigation of the halo-merger connection is of great importance to the knowledge of the formation and evolution of clusters of galaxies.1IntroductionClusters are formed by hierarchical structure formation processes.In this scenario,smaller units formed first and merged to larger and larger units in the course of time.The merger activity appears to be continuing at the present time,and explains the relative abundance of substructures found from X-ray and optical studies,and the gas temperature gradients detected in rich clusters from X-ray observations.The ICM in merging clusters is likely to be in a violent or turbulent dynamical state.It is found that a fraction of clusters which have recently undergone a merger event show diffuse radio sources,thus leading to the idea that these sources are energized by turbulence and shocks in cluster mergers.In this paper,the link between diffuse radio sources and cluster merger processes is discussed,with particular emphasis on the observational aspect.2Radio halos and relicsThe Coma cluster is the first cluster where a radio halo (Coma C)and a relic (1253+275)have been detected (Willson 1970,Ballarati et al.1981).The radio halos are permeating the clusterFigure1:Examples of diffuse radio sources in clusters,overlaid onto the optical cluster image:the giant radio halo in A2163(Feretti et al.2001)is one of the most powerful known halos;the emission in A754(Bacchi et al.2003) is quite irregular,including a radio halo and a radio relic;the complex emission in the cluster RXCJ1314.4−2515 (Feretti et al.2005)is dominated by a central halo and a western relic possibly connected to each other,plus a possible second relic located on the eastern side of the cluster.central regions,with a brightness distribution similar to that of the X-ray gas(Govoni et al. 2001)and a typical extent of>∼1Mpc.They are characterized by steep radio spectrum.Limits of a few percent have been derived for their polarized emission.Relic sources are similar to halos in their low surface brightness,large size and steep spectrum,but they are typically found in cluster peripheral regions.Unlike halos,relics are highly polarized(∼20−30%).The number of clusters with halos and relics is presently around50.Examples of radio images of cluster halos and relics are given in Fig. 1.Their properties have been recently reviewed by Giovannini&Feretti(2002,2004)and Feretti(2005).From radio data,under equipartition conditions,the minimum energy content in halos and relics is of about1060−1061 erg,with minimum non-thermal energy densities of10−14−10−13erg cm−3.The last values are about1000times lower than values of the energy density of the thermal X-ray gas.Thus, the non-thermal components are not contributing to a significant fraction of the mass or energy of a cluster.However,the presence of relativistic particles and large-scale magneticfields are important for a comprehensive physical description of the ICM in galaxy clusters.3Connection to cluster merging processesAll clusters hosting halos and relics are characterized by dynamical activity related to merging processes.These clusters indeed show:i)substructures and distortions in the X-ray brightness distribution(Schuecker et al.2001);ii)temperature gradients(Govoni et al.2004)and gas shocks(Markevitch et al.2003a);iii)absence of a strong coolingflow(Schuecker et al.2001); iv)values of spectroscopicβon average larger than1(Feretti2002);v)core radii significantly larger than those of clusters classified as single/primary(Feretti2002);vi)large distance from the nearest neighbours compared to clusters with similar X-ray luminosity(Schuecker&B¨o hringer 1999);the latter fact supports the idea that recent merger events lead to a depletion of the nearest neighbours.Buote(2001)derived a correlation between the radio power of halos and relics and the dipole power ratio of the cluster two-dimensional gravitational potential.Since power ratios are closely related to the dynamical state of a cluster,this correlation represents thefirst attempt to quantify the link between diffuse sources and cluster mergers.The link between cluster merger processes and the presence of radio halos and relics indicates a physical connection between the thermal and non-thermal ICM components.However,not all clusters showing merging processes are known to host halos and/or relics.This could be due to the limited sensitivity of current radio telescopes.Indeed it is found that the most powerful radioFigure2:Radio brightness of halos at1.4GHz versus the cluster bolometric X-ray luminosity.Empty circles represent halos of size smaller than1Mpc.Arrows indicate upper limits obtained for merging clusters where nodiffuse radio halo has been detected.halos and relics are detected in the most X-ray luminous clusters(see e.g.Feretti2005).For halos,this trend is reflected in the correlation of the radio brightness versus X-ray luminosity, presented in Fig. 2.The upper limits shown in the plot are consistent with the correlation suggesting that clusters of low X-ray luminosity might host faint diffuse sources.On the other hand,it is possible that giant halos are only present in the most X-ray luminous clusters,i.e. above a treshold of X-ray luminosity(see discussion in Bacchi et al.2003).This would be expected in the framework of electron reacceleration models(Cassano et al.2006).Future radio data with next generation instruments(LOFAR,LWA,SKA)will allow the detection of low brightness/low power large halos,in order to clarify if halos are present in all merging clusters or only in the most massive ones.4Spectral index distribution of radio halosA direct confirmation that the cluster merger supplies energy to the radio halo can be obtained from maps of the radio spectral index and their comparison to the X-ray properties.The radio spectral index is related to the energy and ageing of relativistic electrons,and to the strength of the magneticfield in which they emit.These parameters are both influenced by a cluster merger. Thus,by combining high resolution spectral information and X-ray images,it is possible to study the thermal-relativistic plasma connection both on small scales(e.g.spectral index variations vs.clumps in the ICM distribution)and on the large scale(e.g.radial spectral index trends).Feretti et al.(2004)obtained maps of the radio spectral index between0.3and1.4GHz in A665and A2163,and found that regions characterized byflatter radio spectra appear to trace the geometry of recent merger activity.A similar behaviour is derived in A2744(Orr´u et al.2006).Since regions offlatter spectra are indication of the presence of more energeticradiating particles,the above results support the idea that the merger process supplies energy to the radio emitting electrons.In particular,one can compute that in regions of identical volume and same brightness at0.3GHz,aflattening of the spectral index from1.3to0.8implies an amount of energy of the electron population larger by a factor of∼2.5.The difference in the spectral index can also be understood in terms of electron ageing,i.e.by considering that a flatter spectrum generally reflects a spectral cutoffoccurring at higher energies.The implication is that the electrons in theflat spectrum regions would have been reaccelerated more recently.The above results prove that the radio spectral index can be a powerful tracer of the current physical properties of clusters,and confirm the importance of cluster merger in the energetics of relativistic particles responsible for the halo radio emission.5Origin of radio halosThe relativistic particles radiating in halos are likely to originate from AGN activity(quasars, radio galaxies,etc.),or from star formation(supernovae,galactic winds,etc.),or from the thermal pool during violent processes connected to the cluster dynamical history.Because of radiation losses,the radiating particles have short lifetimes,of the order of0.1Gyr.This implies that they can travel a maximum distance of∼100kpc during their lifetime.Given the large size of the radio emitting regions,the relativistic particles need to be reaccelerated by some mechanism,acting with an efficiency comparable to the energy loss processes(Petrosian2001). It is found from observational results that a cluster merger plays a crucial role in the energetic of radio halos.Energy can be transferred from the ICM thermal component to the non-thermal component through two possible basic mechanisms:1)acceleration at shock waves(Keshet et al.2004);2)resonant or non-resonant interaction of electrons with MHD turbulence(Brunetti et al.2001,Fujita et al.2003).Shock acceleration is afirst-order Fermi process of great importance in radio astronomy,as it is recognized as the mechanism responsible for particle acceleration in the supernova remnants. The acceleration occurs diffusively,in that particles scatter back and forth across the shock, gaining at each crossing and recrossing an amount of energy proportional to the energy itself.The acceleration efficiency is mostly determined by the shock Mach number.Based on observational evidences,it seems difficult to associate giant halos to merger shocks,since the shocks are localized while the radio emission of halos is generally much more extended.In addition,Gabici &Blasi(2003)argue that only shocks of high Mach number(>∼3)are suitable for the electron reacceleration,whereas shocks detected so far with Chandra at the center of several clusters have inferred Mach numbers in the range of∼1-2.5(see e.g.Markevitch et al.2003a).Finally, the radio spectral index distribution in A665(Feretti et al.2004)shows no evidence of radio spectralflattening at the location of the hot shock detected by Chandra(Markevitch&Vikhlinin 2001).Therefore,although it cannot be excluded that shock acceleration may be efficient in some particular regions of a halo(e.g.in A520,Markevitch et al.2005),current observations globally favour the scenario that turbulence following a cluster shock,rather than a shock itself,might be the major mechanism responsible for the supply of energy to the electrons radiating in radio halos.Numerical simulations indicate that mergers can generate strongfluid turbulence on scales of0.1-1Mpc.The time during which the process is effective is of∼108years,so that the emission is expected to correlate with the most recent or ongoing merger event.The emerging scenario is that turbulence reacceleration is the likely mechanism to supply energy to the radio halos.Recent theoretical developments of this aspect can be found in Blasi2004,Brunetti et al.(2004),Cassano&Brunetti(2005).Figure3:Results of hydrodynamical simulations of a symmetric off-center merger by Ricker&Sarazin(2001). The colors show the temperature,while the contours represent the X-ray surface brightness.A shocked region is initially at the cluster center,then shocks propagate to the outer part of the cluster.6Origin of radio relicsCurrent theoretical models predict that relativistic particles radiating in radio relics are powered by energy dissipated in shock waves produced in the ICM during the cluster formation history. This picture is supported by numerical simulations on cluster mergers(Ricker&Sarazin2001, Ryu et al.2003),which predict that shocks forming at the cluster center at the early stages of a cluster merger further propagate to the cluster periphery(Fig.3).Two models have been proposed for the origin of the relic radio emission.It could result from Fermi-I diffusive shock acceleration which produces relativistic electrons from the ICM electrons,or it could be due to fossil radio bubbles,related to former active radio galaxies,that are compressed by the passing shock wave and thus induced to emit observable synchrotron emission again(Ensslin&Gopal-Krishna2001,Ensslin&Br¨u ggen2002,Hoeft et al.2004).In both case,because of the electron short radiative lifetimes,radio emission is produced close to the location of the shock waves.This is consistent with the relic elongated structure,almost perpendicular to the merger axis.The detection of shocks in the cluster outskirts is presently very difficult because of the very low X-ray brightness in these regions.The X-ray data for radio relics are indeed very scarce. The Chandra data of A754(Markevitch et al.2003b)indicate that the easternmost boundary of the relic(see Fig.1)coincides with a region of hotter gas.From XMM data of the same cluster, Henry et al.(2004)show that the diffuse radio sources(halo+relic)appear to be associated with high pressure regions.A recent study of the region of the radio relic1253+275in the Coma cluster has been performed by Feretti&Neumann(2006)using XMM-Newton data.X-ray emission is detected at the location of the radio relic(Fig.4)and is found to be of thermal origin,connected to the sub-group around NGC4839.The best-fit gas temperature in the region of the relic and in its vicinity is in the range2.8−4.0keV,comparable to the temperature of the NGC4839Figure4:Color-scale X-ray emission of the NGC4839group obtained from the XMM-Newton data(Feretti &Neumann2006).Dark blue areas are regions with no X-ray data.The green contours represent the X-ray brightness at2,3,and5σlevel over the background,respectively.The radio emission from the Coma cluster relic1253+275at0.3GHz is shown by the black contours.sub-group.No gas of higher temperature,resulting from a possible shock in the region of the Coma relic,is detected.Therefore,the connection between relics and cluster shocks seems to be disproved by these data on the Coma cluster.The authors suggest that turbulence may be the major mechanism responsible for the energy of particles.In particular,during its infall onto the Coma cluster,the sub-group around NGC4839encounters a region of relativistic particles and magneticfields.The interaction between the ionized moving plasma and the magneticfield would imply energy transfer from the ICM to the relativistic particles.Data on more objects will be crucial to test the models and fully understand the relic phenomenon.7ConclusionsMassive clusters of galaxies showing strong dynamical activity and merger processes can host diffuse radio emission,which demonstrates the existence of relativistic particles and magnetic fields in the ICM.From the comparison between radio and X-ray emission there is evidence that recent merger phenomena would provide the energy for the relativistic electron reacceleration, thus allowing the production of a detectable diffuse radio emission.A question which is still unanswered is whether all merging clusters have cluster-wide radio halos.This will be answered by systematic deep studies with future instruments.Spectral index maps of the halos in A665,A2163and A2744show that spectra areflatter in regions more directly influenced by the merger activity,confirming that electrons are likely gaining energy from the merger process.Observational results favour a scenario where cluster turbulence might be the major responsible for the electron reacceleration in radio halos.Theoretical models predicting a connection between cluster shocks and radio relics need to be tested through X-ray observations of cluster peripheral regions.In the Coma cluster,no clear shock is detected at the location of the radio relic.More data on a significant number of clusters,however,are needed to drawfirm conclusions.AcknowledgmentsI wish to thank the organizers for the very interesting meeting.This work was partially supported by INAF under PRIN-2005.References1.Bacchi M.,Feretti L.,Giovannini G.,Govoni F.,A&A400,465(2003)2.Ballarati B.,Feretti L.,Ficarra A.,Giovannini G.,Nanni M.,Olori C.,Gavazzi G.,A&A100,323(1981)3.Blasi P.,Jour.Kor.Astr.Soc.37,483(2004)4.Brunetti G.,Setti G.,Feretti L.,Giovannini G.,MNRAS320,365(2001)5.Brunetti G.,Blasi P.,Cassano R.,Gabici S.,MNRAS350,1174(2004)6.Buote D.A.,ApJ553,L15(2001)7.Cassano R.,Brunetti G.,MNRAS357,1313(2005)8.Cassano R.,Brunetti G.,Setti G.,MNRAS369,1577(2006)9.Enßlin T.A.,Gopal-Krishna,A&A366,26(2001)10.Enßlin T.A.,Br¨u ggen M.,MNRAS331,1011(2002)11.Feretti L.,Fusco-Femiano R.,Giovannini G.,Govoni F.,A&A373,106(2001)12.L.Feretti,in:The Universe at low radio frequencies,IAU Symp.199,ASP ConferenceSeries,Vol.199,p.133(2002)13.Feretti L.,Orr´u E.,Brunetti G.,Giovannini G.,Kassim N.,Setti G.,A&A423,111(2004)14.Feretti L.,AdSpR36,729(2005)15.Feretti L.,Schuecker P.,B¨o hringer H.,Govoni F.,Giovannini G.,A&A444,157(2005)16.Feretti L.,Neumann D.M.,A&A450,L21(2006)17.Fujita Y.,Takizawa M.,Sarazin C.L.,ApJ584,190(2003)18.Gabici S.,Blasi P.,ApJ583,695(2003)19.Giovannini G.,Feretti L.,in:Merging Processes of Galaxy Clusters,ASSL,Kluwer Ac.Publish.,p.197(2002)20.Giovannini G.,Feretti L.,Jour.Kor.Astr.Soc.37,323(2004)oni F.,Enßlin T.A.,Feretti L.,Giovannini G.,A&A369,441(2001)oni F.,Markevitch M.,Vikhlinin A.,VanSpeybroeck L.,Feretti L.,Giovannini G.,ApJ605,695(2004)23.Henry P.J.,Finoguenov A.,Briel U.G.,ApJ615,181(2004)24.Hoeft M.,Br¨u ggen M.,Yepes G.,MNRAS347,389(2004)25.Keshet U.,Waxman E.,Loeb A.,ApJ617,281(2004)26.Markevitch M.,Vikhlinin A.,ApJ563,95(2001)27.Markevitch M.,Vikhlinin A.,Forman W.R.,in:Matter and energy in clusters of galaxies,ASP Conference Series Vol.301,p.37(2003a)28.Markevitch M.,Mazzotta P.,Vikhlinin A.,ApJ586,L19(2003b)29.Markevitch M.,Govoni F.,Brunetti G.,Jerius D.,ApJ627,733(2005)30.Orr´u E.,Feretti L.,Govoni F.,Murgia M.,Giovannini G.,Brunetti G.,Setti G.,AN327,565(2006)31.Petrosian V.,ApJ557,560(2001)32.Ricker P.M.,Sarazin C.L.,ApJ561,621(2001)33.Ryu D.,Kang H.,Hallman E.,Jones T.W.,ApJ593,599(2003)34.Schuecker P.,B¨o hringer H.,in:Diffuse thermal and relativistic plasma in galaxy clusters,MPE Report271,p.43(1999)35.Schuecker P.,B¨o hringer H.,Reiprich T.H.,Feretti L.,A&A378,408(2001)36.Willson M.A.G,MNRAS151,1(1970)。