Review on sustainable thermal energy storage technologies -HEAT STORAGE MATERIALS AND TECHNIQUES
Renewable Energy Beyond Solar and Wind
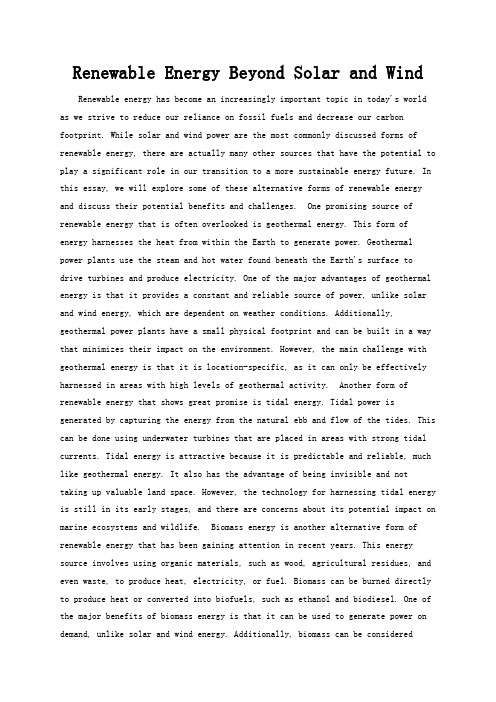
Renewable Energy Beyond Solar and Wind Renewable energy has become an increasingly important topic in today's world as we strive to reduce our reliance on fossil fuels and decrease our carbon footprint. While solar and wind power are the most commonly discussed forms of renewable energy, there are actually many other sources that have the potential to play a significant role in our transition to a more sustainable energy future. In this essay, we will explore some of these alternative forms of renewable energy and discuss their potential benefits and challenges. One promising source of renewable energy that is often overlooked is geothermal energy. This form of energy harnesses the heat from within the Earth to generate power. Geothermal power plants use the steam and hot water found beneath the Earth's surface to drive turbines and produce electricity. One of the major advantages of geothermal energy is that it provides a constant and reliable source of power, unlike solar and wind energy, which are dependent on weather conditions. Additionally, geothermal power plants have a small physical footprint and can be built in a way that minimizes their impact on the environment. However, the main challenge with geothermal energy is that it is location-specific, as it can only be effectively harnessed in areas with high levels of geothermal activity. Another form of renewable energy that shows great promise is tidal energy. Tidal power is generated by capturing the energy from the natural ebb and flow of the tides. This can be done using underwater turbines that are placed in areas with strong tidal currents. Tidal energy is attractive because it is predictable and reliable, much like geothermal energy. It also has the advantage of being invisible and not taking up valuable land space. However, the technology for harnessing tidal energy is still in its early stages, and there are concerns about its potential impact on marine ecosystems and wildlife. Biomass energy is another alternative form of renewable energy that has been gaining attention in recent years. This energy source involves using organic materials, such as wood, agricultural residues, and even waste, to produce heat, electricity, or fuel. Biomass can be burned directly to produce heat or converted into biofuels, such as ethanol and biodiesel. One of the major benefits of biomass energy is that it can be used to generate power on demand, unlike solar and wind energy. Additionally, biomass can be consideredcarbon-neutral, as the carbon dioxide released during its combustion is offset by the carbon dioxide absorbed by the plants during their growth. However, there are concerns about the sustainability of biomass energy, as large-scale productioncould lead to deforestation and competition with food crops for land and resources. Hydropower is a well-established form of renewable energy that has been in use for many years. It involves harnessing the energy from flowing water, typically by building dams and reservoirs to control the flow and generate electricity. Hydropower is a reliable and efficient source of energy, and it has the advantageof being able to store energy for later use. However, the construction of dams and reservoirs can have significant environmental and social impacts, includinghabitat destruction, displacement of communities, and changes to water quality and flow patterns. Additionally, the availability of suitable sites for hydropower development is limited, and there are concerns about the long-term sustainabilityof this energy source. Finally, there is a growing interest in ocean energy as a potential source of renewable power. This includes wave energy, which harnessesthe energy from ocean waves, and ocean thermal energy conversion, which uses the temperature difference between the warm surface waters and the cold deep waters to generate power. Ocean energy has the advantage of being abundant and predictable,as well as having a low environmental impact. However, the technology for harnessing ocean energy is still in the early stages of development, and there are challenges related to the harsh marine environment and the high costs ofinstallation and maintenance. In conclusion, while solar and wind energy havebeen at the forefront of the renewable energy revolution, it is important to recognize the potential of other alternative sources of renewable energy. Geothermal, tidal, biomass, hydropower, and ocean energy all offer unique advantages and challenges, and they have the potential to play a significant rolein our transition to a more sustainable energy future. By diversifying our sources of renewable energy, we can increase energy security, reduce greenhouse gas emissions, and minimize the environmental impact of our energy production. It is essential that we continue to invest in research and development to unlock thefull potential of these alternative forms of renewable energy and create a more diverse and resilient energy system for the future.。
二氧化钛的导热系数
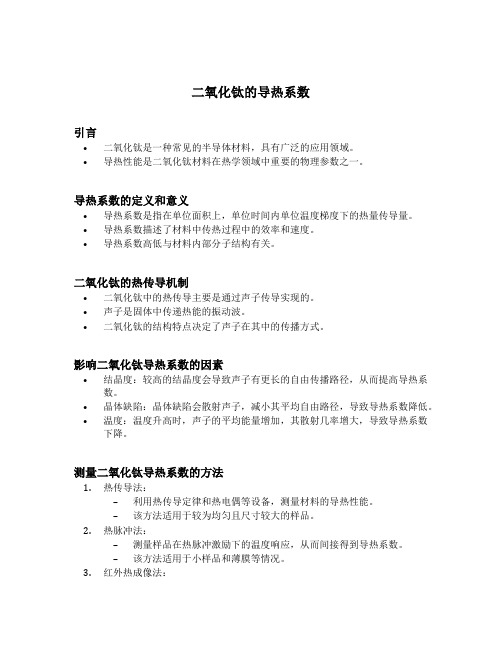
二氧化钛的导热系数引言•二氧化钛是一种常见的半导体材料,具有广泛的应用领域。
•导热性能是二氧化钛材料在热学领域中重要的物理参数之一。
导热系数的定义和意义•导热系数是指在单位面积上,单位时间内单位温度梯度下的热量传导量。
•导热系数描述了材料中传热过程中的效率和速度。
•导热系数高低与材料内部分子结构有关。
二氧化钛的热传导机制•二氧化钛中的热传导主要是通过声子传导实现的。
•声子是固体中传递热能的振动波。
•二氧化钛的结构特点决定了声子在其中的传播方式。
影响二氧化钛导热系数的因素•结晶度:较高的结晶度会导致声子有更长的自由传播路径,从而提高导热系数。
•晶体缺陷:晶体缺陷会散射声子,减小其平均自由路径,导致导热系数降低。
•温度:温度升高时,声子的平均能量增加,其散射几率增大,导致导热系数下降。
测量二氧化钛导热系数的方法1.热传导法:–利用热传导定律和热电偶等设备,测量材料的导热性能。
–该方法适用于较为均匀且尺寸较大的样品。
2.热脉冲法:–测量样品在热脉冲激励下的温度响应,从而间接得到导热系数。
–该方法适用于小样品和薄膜等情况。
3.红外热成像法:–利用红外热像仪观测样品表面的温度分布,推导出导热系数。
–该方法适用于材料外观不规则或表面特殊的情况。
二氧化钛导热系数的应用1.光电器件:–二氧化钛具有光电转换性能,导热系数的高低会影响其热管理能力。
–在太阳能电池、光催化等领域中的应用得到了广泛研究。
2.热障涂层:–二氧化钛的导热系数可以影响其在涂层中的传热效果。
–在航空航天等高温环境下的应用中发挥了重要作用。
3.纳米材料研究:–通过调控二氧化钛的导热系数,可以实现对纳米材料的自组装和有序性控制。
–在纳米线、薄膜等领域的研究中具有潜在应用价值。
未来发展方向•现有的测量方法仍然存在一定的局限性,需要继续改进和发展新的测量技术。
•针对二氧化钛的导热性能优化,可通过合金化、掺杂等方式进行改变。
•进一步研究二氧化钛导热系数与结构、形貌等之间的关系,揭示其内在机制。
跨季节蓄热太阳能的英文文献

跨季节蓄热太阳能的英文文献Harnessing the Power of the Sun: A Seasonal Thermal Storage ApproachThe pursuit of sustainable energy solutions has become a global imperative, and one of the most promising avenues is the utilization of solar energy. As the world grapples with the pressing need to reduce greenhouse gas emissions and mitigate the effects of climate change, the exploration of innovative technologies that can harness the abundant and renewable power of the sun has become a focal point of research and development. One such approach is the concept of cross-seasonal thermal storage, which holds the potential to revolutionize the way we generate and utilize solar energy.The fundamental premise of cross-seasonal thermal storage is the ability to capture and store the sun's heat during periods of high solar irradiation, typically during the warmer months, and then release that stored energy during periods of lower solar availability, such as the colder seasons. This concept addresses a critical challenge faced by traditional solar energy systems, which often struggle to meet the energy demands of buildings and communities during periods of low sunlight.The process of cross-seasonal thermal storage involves a multi-step approach. First, solar thermal collectors, such as flat-plate or evacuated tube collectors, are used to absorb the sun's energy and convert it into thermal energy. This thermal energy is then stored in a suitable medium, such as water, rock, or phase-change materials, within an insulated storage system. The stored thermal energy can then be retrieved and utilized for heating, cooling, or other applications when the demand for energy is higher, effectively bridging the gap between the availability of solar energy and the energy needs of the end-users.One of the key advantages of cross-seasonal thermal storage is its ability to improve the overall efficiency and reliability of solar energy systems. By storing excess heat generated during the warmer months, the system can provide a consistent and reliable source of thermal energy during the colder seasons, reducing the reliance on fossil fuels or other energy sources for heating and cooling. This not only contributes to a reduction in greenhouse gas emissions but also enhances the energy security and self-sufficiency of communities and regions that adopt this technology.Moreover, cross-seasonal thermal storage can have a significant impact on the economics of solar energy systems. By providing a means to store and utilize solar energy throughout the year, thetechnology can help to mitigate the seasonal fluctuations in energy demand and supply, leading to a more stable and predictable energy market. This, in turn, can attract greater investment and support for the adoption of solar energy, further accelerating the transition towards a more sustainable energy future.The implementation of cross-seasonal thermal storage systems, however, is not without its challenges. One of the primary obstacles is the development of efficient and cost-effective thermal storage technologies that can maintain the integrity of the stored energy over extended periods. Additionally, the integration of these systems into existing energy infrastructure and building designs requires careful planning and coordination to ensure optimal performance and integration.Despite these challenges, the potential benefits of cross-seasonal thermal storage are substantial, and researchers and engineers around the world are actively exploring innovative solutions to overcome the barriers to widespread adoption. From the development of advanced insulation materials to the optimization of storage media and system designs, the field of cross-seasonal thermal storage is witnessing a surge of technological advancements that hold the promise of a more sustainable and resilient energy future.As the world continues to grapple with the urgent need to address climate change and energy security, the concept of cross-seasonal thermal storage stands as a shining example of the ingenuity and dedication of the scientific community. By harnessing the power of the sun and leveraging the principles of thermal energy storage, this technology has the potential to transform the way we generate, distribute, and consume energy, ultimately paving the way for a more sustainable and equitable energy landscape for generations to come.。
renewable and sustainable energy reviews投稿注意事项
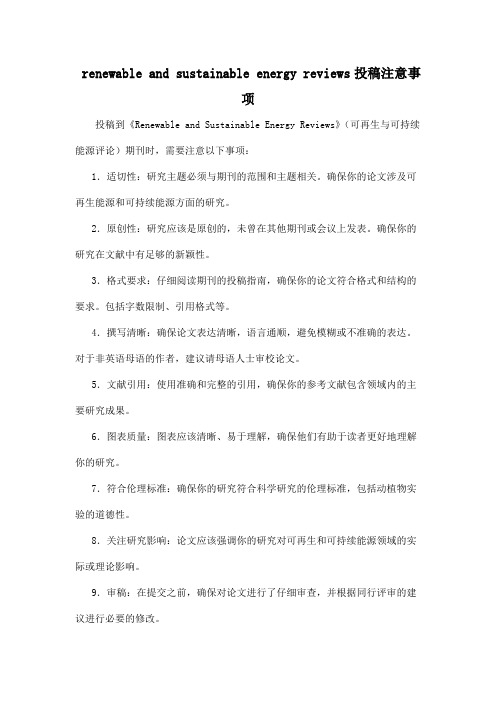
renewable and sustainable energy reviews投稿注意事
项
投稿到《Renewable and Sustainable Energy Reviews》(可再生与可持续能源评论)期刊时,需要注意以下事项:
1.适切性:研究主题必须与期刊的范围和主题相关。
确保你的论文涉及可再生能源和可持续能源方面的研究。
2.原创性:研究应该是原创的,未曾在其他期刊或会议上发表。
确保你的研究在文献中有足够的新颖性。
3.格式要求:仔细阅读期刊的投稿指南,确保你的论文符合格式和结构的要求。
包括字数限制、引用格式等。
4.撰写清晰:确保论文表达清晰,语言通顺,避免模糊或不准确的表达。
对于非英语母语的作者,建议请母语人士审校论文。
5.文献引用:使用准确和完整的引用,确保你的参考文献包含领域内的主要研究成果。
6.图表质量:图表应该清晰、易于理解,确保他们有助于读者更好地理解你的研究。
7.符合伦理标准:确保你的研究符合科学研究的伦理标准,包括动植物实验的道德性。
8.关注研究影响:论文应该强调你的研究对可再生和可持续能源领域的实际或理论影响。
9.审稿:在提交之前,确保对论文进行了仔细审查,并根据同行评审的建议进行必要的修改。
10.开放获取政策:了解期刊的开放获取政策,并根据需要选择相应的出版选项。
在投稿前,最好直接查阅期刊的官方网站,详细了解其最新的投稿指南和要求,以确保论文符合期刊的标准。
无机水合盐相变储热材料的过冷性研究_曾翠华
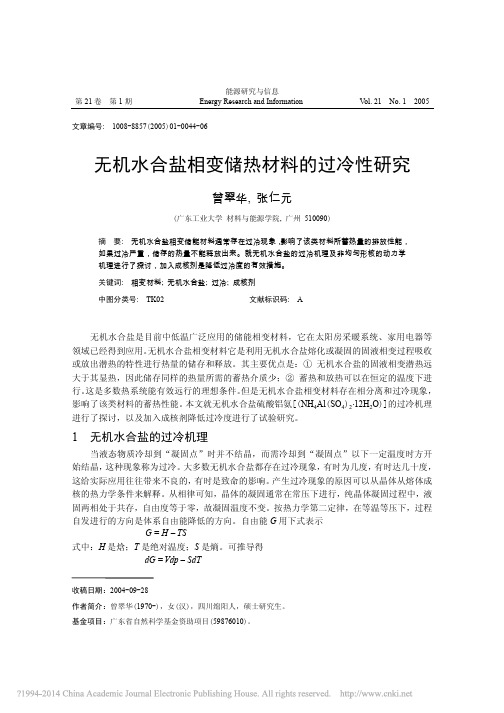
文章编号: 1008-8857(2005)01-0044-06
Vol. 21 No. 1 2005
无机水合盐相变储热材料的过冷性研究
曾翠华, 张仁元
(广东工业大学 材料与能源学院, 广州 510090)
摘 要: 无机水合盐相变储能材料通常存在过冷现象,影响了该类材料所蓄热量的排放性能, 如果过冷严重,储存的热量不能释放出来。就无机水合盐的过冷机理及非均匀形核的动力学 机理进行了探讨,加入成核剂是降低过冷度的有效措施。
式中:GS、GL 分别为固相和液相单位体积自由能。由 G = H − TS ,可得
∆Gv = (HS − HL) − T (SS − SL)
(1)
由于恒压下
∆HP = HS − HL = Lm
(2)
∆Sm = SL − SS = Lm
(3)
Tm
式中:Lm 为熔化热,表示固相转变为液相时体系向环境吸热,定义为正值;∆Sm 为固体的熔 化熵,它主要反映固体转变为液体时的组态熵的增加,可从熔化热与熔点的比值求得。
3 结论
(1) 依据非均匀成核机理,在无机水合盐相变储热材料中加入成核剂是降低无机水合盐 过冷度的有效也是较经济的措施。
48
能源研究与信息
2005 年 第 21 卷
(2) 成核剂对非均匀形核位置的促进作用取决于接触角θ。接触角θ 越小,成核剂对非均 匀形核的作用越大。
(3) 为了使θ 减小,应使 a -W 界面的比表明能σaW 尽可能降低,故要求成核剂与形核晶体 具有相近的结合键类型而且与晶核相接的彼此晶面具有相似的原子配置和小的点阵错配度 δ = | a − a1 | a ,其中 a 为晶核的相接晶面上的原子间距;a1 为成核剂相接面上的原子间距。 有些无机水合盐相变储热材料的成核剂与上述理论推断符合得较好[7~10]。但是,也有一些研究 结果表明,晶核与成核剂基底之间的点阵错配δ 并不象上述所强调的那样重要[6~9],因此在对 无机水合盐相变储热材料的成核剂的选取主要还是通过试验来确定有效的成核剂。
热水器技术支撑材料怎么写范文
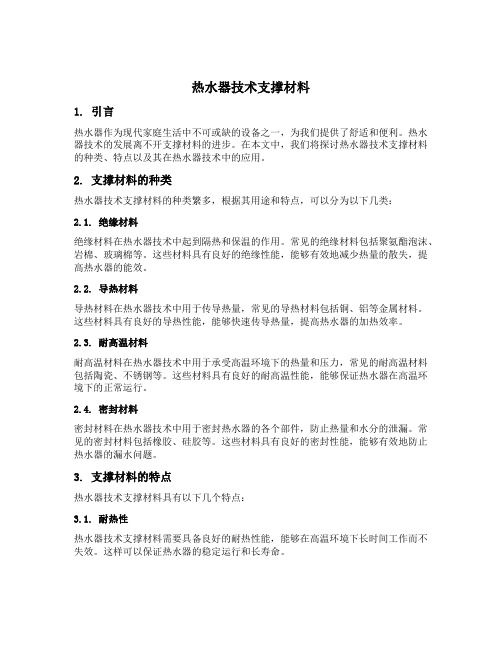
热水器技术支撑材料1. 引言热水器作为现代家庭生活中不可或缺的设备之一,为我们提供了舒适和便利。
热水器技术的发展离不开支撑材料的进步。
在本文中,我们将探讨热水器技术支撑材料的种类、特点以及其在热水器技术中的应用。
2. 支撑材料的种类热水器技术支撑材料的种类繁多,根据其用途和特点,可以分为以下几类:2.1. 绝缘材料绝缘材料在热水器技术中起到隔热和保温的作用。
常见的绝缘材料包括聚氨酯泡沫、岩棉、玻璃棉等。
这些材料具有良好的绝缘性能,能够有效地减少热量的散失,提高热水器的能效。
2.2. 导热材料导热材料在热水器技术中用于传导热量,常见的导热材料包括铜、铝等金属材料。
这些材料具有良好的导热性能,能够快速传导热量,提高热水器的加热效率。
2.3. 耐高温材料耐高温材料在热水器技术中用于承受高温环境下的热量和压力,常见的耐高温材料包括陶瓷、不锈钢等。
这些材料具有良好的耐高温性能,能够保证热水器在高温环境下的正常运行。
2.4. 密封材料密封材料在热水器技术中用于密封热水器的各个部件,防止热量和水分的泄漏。
常见的密封材料包括橡胶、硅胶等。
这些材料具有良好的密封性能,能够有效地防止热水器的漏水问题。
3. 支撑材料的特点热水器技术支撑材料具有以下几个特点:3.1. 耐热性热水器技术支撑材料需要具备良好的耐热性能,能够在高温环境下长时间工作而不失效。
这样可以保证热水器的稳定运行和长寿命。
3.2. 耐腐蚀性热水器技术支撑材料需要具备良好的耐腐蚀性能,能够抵御热水中的酸碱物质和氧化物的侵蚀。
这样可以延长热水器的使用寿命,并减少维修和更换的频率。
3.3. 导热性热水器技术支撑材料需要具备良好的导热性能,能够快速传导热量,提高热水器的加热效率。
这样可以减少能源消耗,提高热水器的能效。
3.4. 环保性热水器技术支撑材料需要具备良好的环保性能,不能对环境造成污染。
这样可以保护环境,减少对自然资源的消耗。
4. 支撑材料的应用热水器技术支撑材料在热水器的各个部件中起到重要的作用。
ieee transactions on sustainable energy投稿要求
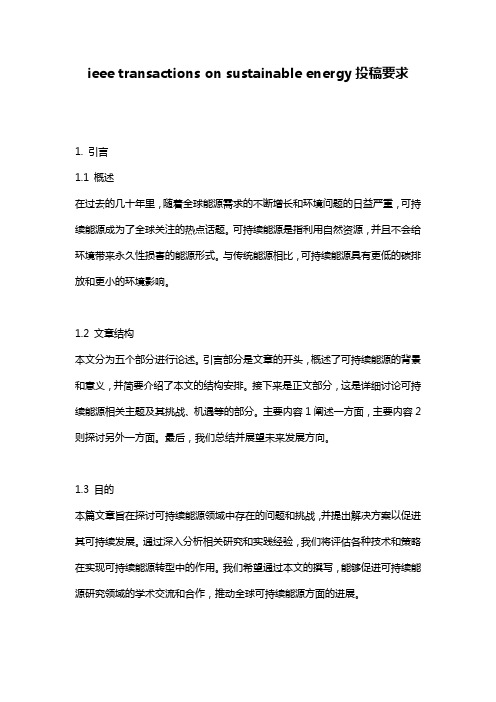
ieee transactions on sustainable energy投稿要求1. 引言1.1 概述在过去的几十年里,随着全球能源需求的不断增长和环境问题的日益严重,可持续能源成为了全球关注的热点话题。
可持续能源是指利用自然资源,并且不会给环境带来永久性损害的能源形式。
与传统能源相比,可持续能源具有更低的碳排放和更小的环境影响。
1.2 文章结构本文分为五个部分进行论述。
引言部分是文章的开头,概述了可持续能源的背景和意义,并简要介绍了本文的结构安排。
接下来是正文部分,这是详细讨论可持续能源相关主题及其挑战、机遇等的部分。
主要内容1阐述一方面,主要内容2则探讨另外一方面。
最后,我们总结并展望未来发展方向。
1.3 目的本篇文章旨在探讨可持续能源领域中存在的问题和挑战,并提出解决方案以促进其可持续发展。
通过深入分析相关研究和实践经验,我们将评估各种技术和策略在实现可持续能源转型中的作用。
我们希望通过本文的撰写,能够促进可持续能源研究领域的学术交流和合作,推动全球可持续能源方面的进展。
2. 正文在本篇文章的正文部分,我们将探讨以下几个方面:可持续能源发展的背景和意义、相关政策与国际合作的进展、可持续能源技术的研究和应用、以及相关领域的挑战和未来发展方向。
2.1 可持续能源发展的背景和意义可持续能源是指可以满足当前需求而不损害后代满足自身需求能力的能源形式。
随着人口增长和经济发展,传统化石燃料资源面临枯竭和环境污染等问题,寻找可替代能源成为当务之急。
因此,探索和推动可持续能源发展具有重要意义。
2.2 相关政策与国际合作的进展各国政府已开始制定并实施相关政策以鼓励可持续能源的发展。
例如,中国提出“碳中和”目标,欧盟制定了2030年气候与能源框架等一系列政策文件。
此外,各国也积极开展国际交流与合作,在技术研发、经验分享等方面取得了积极进展。
2.3 可持续能源技术的研究和应用可持续能源技术涵盖了多个领域,包括但不限于太阳能、风能、水能、生物质能等。
锂基于层次分析法和VIKOR法的低温相变材料优选

锂基于层次分析法和VIKOR法的低温相变材料优选张瑜;王毅;杨伟华;吉慧【摘要】In order to evaluate and filter the best low-temperature phase change materials for thermal energy storage, Analytic hierarchyprocess(AHP) and VlseKriterijumska Optimizacija I Kompromisno Resenjein Serbian (VIKOR) were introduced in the field of phase change material selection. The evaluation system of three levels and six different attributes was constructed and the optimization model based on AHP and VIKOR was established. Meanwhile, the evaluation method and steps were pointed out. Based on this, by the use of established model, ten kinds of low-temperature phase change materials were sorted according to their comprehensive performance. The results show that the established evaluation system and model based on AHP and VIKOR is effective and practical for selecting optimal PCMs. Sodiumacetate trihydrate and stearic acid are found to be the optimal inorganic and organic phase change materials, respectively.%为评价、筛选综合性能佳的低温相变储热材料,将层析分析法和多准则妥协解排序法引入相变材料多属性决策领域,构建了三层次六属性的低温相变材料评价体系,建立了基于层次分析法和多准则妥协解排序法的优选模型,指出了评价的方法和步骤。
- 1、下载文档前请自行甄别文档内容的完整性,平台不提供额外的编辑、内容补充、找答案等附加服务。
- 2、"仅部分预览"的文档,不可在线预览部分如存在完整性等问题,可反馈申请退款(可完整预览的文档不适用该条件!)。
- 3、如文档侵犯您的权益,请联系客服反馈,我们会尽快为您处理(人工客服工作时间:9:00-18:30)。
REVIEW ON SUSTAINABLE THERMAL ENERGY STORAGE TECHNOLOGIES,PART I:HEAT STORAGEMATERIALS AND TECHNIQUESS.M.HASNAINEnergy Research Institute,King Abdulaziz City for Science and Technology,P.O.Box 6086,Riyadh 11442,Saudi Arabia(Received 9July 1997)Abstract ÐThis paper reviews the development of available thermal energy storage (TES)technologies and their individual pros and cons for space and water heating applications.Traditionally,available heat has been stored in the form of sensible heat (typically by raising the temperature of water,rocks,etc.)for later use.In most of the low temperature applications,water is being used as a storage tent heat storage on the other hand,is a young and developing technology which has found considerable interest in recent times due to its operational advantages of smaller temperature swing,smaller size and lower weight per unit of storage capacity.It has been demonstrated that,for the devel-opment of a latent heat thermal energy storage system,the choice of the phase change material (PCM)plays an important role in addition to heat transfer mechanisms in the PCM.Attempts have also been made to utilize technical grade phase change materials as storage media and embedded heat exchange tubes/heat pipes with extended surfaces in order to enhance the heat transfer rate to/from the PCM.#1998Elsevier Science Ltd.All rights reservedSolar energy applications Thermal energy storage Sensible heat storagePhase changematerial Extended surfaces Heat pipeINTRODUCTIONStorage of thermal energy is very important in many engineering applications.For example,among the practical problems involved in solar energy systems is the need for an e ective means by which the excess heat collected during periods of bright sunshine can be stored,preserved and later released for utilization during the night or other periods.Similar problems arise for waste heat recovery systems where the waste heat availability and utilization periods are di er-ent.Heat storage can also be applied in most types of buildings where heating needs are signi®-cant and electricity rates allow heat storage to be competitive with other forms of heating.There are numerous types of commercially available systems,and many new developments in the technology are taking place.Therefore,the scope of this paper is to review sensible heat sto-rage and latent heat storage methods for space and water heating applications.In a second paper (Part II),a state-of-the-art on cool energy storage technologies as a Demand Side Management (DSM)as well as a Supply Side Management (SSM)tool for electric load manage-ment is discussed.The basic types of thermal energy storage techniques can be described as:Sensible heat sto-rage ,in which the temperature of the storage material varies with the amount of energy stored,and latent heat storage ,which makes use of the energy stored when a substance changes from one phase to another by melting (as from ice to water).Typical data of some relevant properties of heat storage materials used in thermal stores are given in Table 1for comparison.The last row in this table gives the relative volume occupied by each of the storage media for a heat sto-rage capacity of 106kJ and a temperature rise of 15K during heat storage [1].SENSIBLE HEAT STORAGESensible heat storage is e ected by raising the temperature of the storage medium.Thus,it is desirable for the storage medium to have high speci®c heat capacity,long term stability under thermal cycling,compatibility with its containment and,most importantly,low cost.Sensible heat storage may be classi®ed on the basis of the heat storage media as Liquid media storageEnergy Convers.Mgmt Vol.39,No.11,pp.1127±1138,1998#1998Elsevier Science Ltd.All rights reservedPrinted in Great Britain 0196-8904/98$19.00+0.00PII:S0196-8904(98)00025-91127(like water,oil based ¯uids,molten salts etc.)and Solid media storage (like rocks,metals and others).Liquid media storageHeat storage liquids are plentiful and economically competitive.The pros and cons of some of the selected media are given below.Storage in water.At low temperature water is one of the best storage media.It has higher speci®c heat than other materials,and it is cheap and widely available.However,due to its high vapor pressure,it requires costly insulation and pressure withstanding containment for high tem-perature applications.Water can be used over a wide range of temperature,say 25±908C.For a 608C temperature change,water will store 250kJ/kg or 2.5Â105kJ/m 3.Hot water is required for washing,bathing etc.,and it is commonly employed in radiators for space heating.Water can be used as a storage and as a transport medium of energy,for example,in a solar energy system.Consequently,it is the most widely used storage medium today for solar based warm water and space heating applications [2,3].Because of its simplicity,a large amount of pub-lished data are available on the design criteria for water storage media [1±4].Thermal strati®cation or thermocline in a solar water heat storage tank can be established due to the buoyancy forces,which ensure the highest temperature at the top and the lowest tem-perature at the bottom of the tank.Strati®cation is achieved through the elimination of mixing during storage,whereby a two-fold advantage is gained [2,3]:(i)the e ciency with which the energy can be used will be improved if it is supplied to the load at the temperature it was col-lected rather than at a lower mixed storage temperature and (ii)the amount of energy collected may be increased if the collector inlet ¯uid temperature is lower than the mixed storage tempera-ture.Water storage tanks are made from a wide variety of materials,like steel,aluminum,re-inforced concrete and ®ber glass.The tanks are insulated with glass wool,mineral wool or poly-urethane.The sizes of the tanks used vary from a few hundred liters to a few thousand cubic meters.For large scale storage applications,underground natural aquifers have been considered.Aquifers are geological formations containing ground water,o ering a potential way of storing heat for long periods of time.The storage medium in aquifers consists of water saturated gravel or rge storage volumes are available,as typical aquifer sizes range from hundreds of thousands to millions of cubic meters.For example,105m 3of aquifer material can store about 3MJ of heat for each 10K temperature di erence.This type of storage is well suited for seaso-nal storage,i.e.transferring heat from the summer season to the heating season.Because of its bulk nature,aquifer storage is not feasible for small loads,e.g.individual houses.The usefulness of aquifer thermal energy storage has been widely acknowledged,and several experimental and theoretical studies on them have been performed [5,6].However,because of the multitude of di erent systems and physical parameters,a more detailed computer simulation study is needed to determine the optimum system con®guration.The attractiveness of aquifer storage is due to its low cost characteristics,its high input/output rates and its large capacity.Table parison of various heat storage media (stored energy =106kJ =300kWh;D T =15K)Heat Storage MaterialSensible heat storagePhase Change Materials PropertyRock Water Organic Inorganic Latent heat of fusion (kJ/kg)**190230Speci®c heat (kJ/kg) 1.0 4.2 2.0 2.0Density (kg/m 3)224010008001600Storage mass for storing 106kJ (kg)670001600053004350Relative mass **154 1.25 1.0Storage volume for storing 106kJ (m 3)3016 6.6 2.7Relative volume **1162.51.0*Latent heat of fusion is not of interest for sensible heat storage.**Relative mass and volume are based on latent heat storage in inorganic phase change materialsS.M.HASNAIN:REVIEW ON SUSTAINABLE THERMAL ENERGY1128S.M.HASNAIN:REVIEW ON SUSTAINABLE THERMAL ENERGY1129 Storage in salty water.Solar ponds o er a simple and economical method for collecting and storing large amounts of solar energy in the form of low temperature thermal energy(50±958C). They have potential in space heating and cooling applications,in supplying industrial process heat and in electric power generation.Solar ponds can be classi®ed according to four basic fac-tors:(a)convecting or non-convecting,(b)partitioned(multi-layered)or non-partitioned,(c) gelled or non-gelled and(d)separate collector and storage or in-pond storage.However,most of the research e orts are presently concentrated on the non-convecting salt gradient solar pond[7±9].In this type of solar pond,a density gradient is created by using water containing salt(or sea water),the concentration of which increases with depth from the surface.Sodium chloride(NaCl)and magnesium chloride(MgCl2)are the salts most commonly used in this type of pond.The salt gradient pond has a black or dark bottom where the solar radiation is absorbed,with a consequent increase in water temperature up to958C.Extraction of the ther-mal energy stored in the lower layers of the pond can easily be accomplished without disturbing the upper layers.The largest solar pond in the USA is a3355m2experimental facility in El Paso,Texas,which has operated reliably since its start-up in1986.The pond runs a70kWe or-ganic rankine-cycle turbine generator and a5000gallons per day desalting unit,while also pro-viding process heat to an adjacent food processing company.The pond has reached and sustained temperatures higher than908C in its heat storage zone,generated more than100kWe during peak power output and produced more than80,000gallons of potable water in a24h period[9].Storage in other¯uids.The most commonly proposed substitutes for water are petroleum based oils and molten salts.The heat capacities are25±40%of that of water on a weight basis. However,these substitutes have lower vapor pressure than water and are capable of operating at high temperatures exceeding3008C.The oils are limited to less than3508C due to stability and safety reasons and can be quite expensive.Some of the oil candidates that have been con-sidered are Therminol and Caloria-HT[2].A few molten mixtures of inorganic salts have been considered for high temperatures(3008C and above).One is sodium hydroxide which has a melting point of3208C and could be used for temperatures up to8008C[7].However,it is highly corrosive,and there is a di culty in containing it at high temperatures.Liquid metals are also mentioned as possible sensible heat storage media.While most of their properties are simi-lar to those of water,they,along with the petroleum based oils,have low speci®c heats and higher potential for reactivity with the container.They do,however,enjoy higher thermal con-ductivity.Type304stainless steel is the most common material used for containment of oils and liquid metals,with special attention usually given to the maintenance of an oxygen and oxide free environment in order to prevent corrosion[10].Solid media storageFor a low as well as high temperature thermal energy storage,solid materials such as rocks, metals,concrete,sand,bricks etc.,can be used.In this case,the energy can be stored at low or high temperatures,since these materials will not freeze or boil.The di culties of the high vapor pressure of water and the limitations of other liquids can be avoided by storing thermal energy as sensible heat in solids.Moreover,solids do not leak from their container.The highest pro-duct in the list of solid materials for sensible heat storage is cast iron,which exceeds the energy density level of water storage[11].However,cast iron is more expensive than stone or brick and,hence,the payback period is much longer.Pebble beds or rock piles are generally preferred as the storage material due to their low cost.Storage in rocks.The pebble bed or rock pile consists of a bed of loosely packed rock material through which the heat transport¯uid can¯ow.The thermal energy is stored in the packed bed by forcing heated air into the bed and utilized again by recirculating ambient air into the heated bed.The energy stored in a packed bed storage system depends,apart from the thermophysical properties of the material,on several parameters,including rock size and shape,packing density, heat transfer¯uid etc.Solar energy can also be stored in rocks or pebbles(packed in insulated vessels),and it is con-venient for use in buildings.This type of storage is used very often for temperatures up to 1008C in conjunction with solar air heaters[12].Typically,the characteristic size of the pieces ofrock used varies from 1to 5cm.An approximate thumb rule followed for sizing is to use 300±500kg of rock per square meter of solar collector area for space heating applications.For a temperature change of 508C,rocks and concrete will store around 36kJ/kg or about 105kJ/m 3.Rock or pebble bed storage can also be used for much higher temperatures,up to 10008C.King and Burns [12]use a number of characteristics,typically particle size,void fraction,bed cross-sectional area and bed length,super®cial air velocity and Reynolds number,in order to describe the thermal and geometric properties of packed beds.Like rock beds,¯uidized beds can be uti-lized for low,intermediate and high temperature solar applications [13].In addition,the rate of heat exchange between the heat carrying ¯uid and the storage medium is much faster in ¯ui-dized beds than in rock beds,which can be an advantage in several applications.The ¯uidized bed thermal storage can also be used for waste heat recovery purposes.Storage in building fabric.Heat storage is applicable to both new and existing buildings and can be integrated with both air and water distribution systems.The most prevalent storage ma-terial is ceramic brick,consisting of olivine,magnesite,microtherm or feolite.Building mass and structural cement can also be used with active or passive storage designs.Improvements in insu-lation and storage materials continue to be made by manufacturers.The most common con-®guration using building mass for thermal storage is ¯oor warming.The ¯oor becomes a large low temperature radiating surface with the concrete acting as the heat storage medium.The ¯oor can be warmed with a heat transfer ¯uid,such as water,with direct electric resistance wir-ing or with air ducts.Water is the most common heat transfer medium.Many buildings with ¯oor warming do not utilize o peak electricity to charge the storage,although they could be retro®tted with a control system to utilize o peak heating.An advanced phase change material for ¯oor storage heating has been developed by a Swiss manufacturer,with applications in the UK,Spain,Korea and Japan [14].Two other categories of building mass storage are building inertia and hollow core construc-tion.Controls to minimize electricity costs for heating by using the overall mass of the building to decrease heating during on peak periods are being developed [15,16].Use of inertia in build-ing mass is a technique where no additional HVAC equipment is installed but special controls are required.Hollow core ventilation systems were developed in Sweden,in which the supply air is distributed through the ¯oor slabs,coupling it to the building mass [17].Storage in metals.Most of the materials proposed for high temperature (120±14008C)energy storage are either inorganic salts or metals [11,18,19].Among the metals,aluminium,mag-nesium and zinc have been mentioned as suitable examples.The use of metal media may be ad-vantageous where high thermal conductivity is required and where cost is of secondary importance.Solid industrial wastes like copper slag,iron slag,cast iron slag,aluminium slag and copper chips could be used as storage material for energy storage.LATENT HEAT STORAGELatent heat storage is a particularly attractive technique,since it provides a high energy sto-rage density and has the capacity to store heat as latent heat of fusion at a constant temperature corresponding to the phase transition temperature of the phase change materials (PCMs).For example,in the case of water,80times as much energy is required to melt 1kg of ice as to raise the temperature of 1kg of water by 18C.This means that a much smaller weight and volume of material is needed to store a certain amount of energy.PCMs undergo solid±solid,liquid±gas,and solid±liquid phase transformations.Relatively few solid±solid PCMs have been identi®ed that have heats of fusion and transition temperatures suitable for thermal storage applications.Liquid±gas PCMs usually have high heats of trans-formations,however,due to the large volume change during transformation,they are not usually considered for practical applications.Solid±liquid PCMs are useful because they store a relatively large quantity of heat over a narrow temperature range,without a corresponding large volume change [20].Typically,the PCM is placed in long thin tubes stacked in a container.During a heat storage cycle,the collected solar heat from the collector is circulated through narrow spaces between the tubes and melts the PCM by storing the heat as sensible heat as well as latent heat of fusion.During the heat recovery cycle,the circulation of low temperature airS.M.HASNAIN:REVIEW ON SUSTAINABLE THERMAL ENERGY1130S.M.HASNAIN:REVIEW ON SUSTAINABLE THERMAL ENERGY1131 would pick up the stored energy from the PCM and transport it to the heat load.Therefore,the latent heat storage units utilize the sensible heat in the solid and liquid phases and additionally the latent heat due to a phase change during melting or freezing of the storage medium.Any latent heat thermal energy storage system must possess at least the three following basic components[11,20]:(a)a heat storage substance that undergoes a solid-to-liquid phase tran-sition in the required operating temperature range and where the bulk of the heat added is stored as the latent heat of fusion,(b)a container for holding the storage substance and(c)a heat exchanging surface for transferring heat from the heat source to the PCM and from the lat-ter to the heat sink.The development of a latent heat thermal energy storage(LHTES)system, therefore,involves an understanding of two essentially diverse subjects:heat storage materials (or PCMs)and heat exchangers.These two main subjects will be discussed in the following two sections.Phase change materials(PCMs)A large number of latent heat storage materials have been reported in the literature for ther-mal storage applications in temperature ranges suitable for heating and cooling[20±38].Some of them according to their temperature range are listed in Table2.PCMs can be classi®ed into the following major categories:inorganic compounds,organic compounds and eutectics of inor-ganic and/or organic compounds.Inorganic compounds include salt hydrates,salts,metals and alloys,whereas organic compounds are comprised of para ns,non-para ns and polyalcohols. Salt hydrates.A number of salt hydrates,such as sodium sulfate decahydrate(Glauber's salt), calcium chloride hexahydrate,sodium thiosulphate pentahydrate,sodium acetate trihydrate and barium hydroxide octahydrate,were investigated largely because of their low cost[23,24,27,30,31,35±39].Some of the literature and measured thermophysical properties of important PCMs are listed in Tables3and4.The contents of these tables show a di erence in literature and measured thermophysical properties of salt hydrates.It is worth noting that most manufacturers do not perform any tests on thermophysical properties of technical grade ma-terials,but instead,they rely on the available data for analytical grade materials.Major problems with most of the salt hydrates are supercooling and phase segregation. Supercooling has been prevented in several salt hydrates by adding nucleating agents or by pro-moting nucleation by rough container walls or using the cold®nger technique[24,28,30,34].To prevent phase segregation,several techniques,such as use of thickening agents,rotating storage devices and direct contact heat transfer,have been used[34,38].The encapsulation of PCMs in metal and plastic matrices has been tried extensively.The macro-encapsulation of PCMs can(i) avoid large phase separations,(ii)increase the rate of heat transfer and(iii)provide a self sup-porting structure for the PCM.The most cost e ective containers are plastic bottles and tin pla-ted food cans/mild steel cans[31].However,corrosion could lead to disastrous consequences if internal and external lacquer®nishes are not applied properly to the mild steel metal cans.tent heat storage materials and their temperature rangesTemperature Range(8C)Material Transition Temperature(8C)Heat of Fusion(kJ/kg)0±100Water0335Para n20±60140±280Salt hydrate30±50170±270100±400AlC13192280LiNO3250370Na2O2360314400±80050LiOH/50LiF427512KC1O45271253LiH6992678800±1500LiF868932NaF993750MgF21271936Si14151654T a b l e 3.M e l t i n g t e m p e r a t u r e s a n d l a t e n t h e a t o f f u s i o n o f s e l e c t e d t e c h n i c a l g r a d e p h a s e c h a n g e m a t e r i a l s (P C M )u s i n g D S C -2i n s t r u m e n t [38]O r i g i n a l p h a s e c h a n g e m a t e r i a l s C h e m i c a l S u p p l i e r sM e l t i n g t e m p e r a t u r e T m (8C )D T m =T m e x p e r .ÀT m l i t r a t .(8C )H e a t o f f u s i o n D H f (J /g )d D H fD H f e x p e r X ÀD H f l i t r a t X Â100D H f l i t r a t XL i t r a t .E x p e r .O n s e tP e a k O n s e t P e a k L i t r a t .E x p e r .(%)P a r a n W a x B D H c h e m i c a l s 4944.1651.64À4.842.64210186À11.43C a l c i u m c h l o r i d e h e x a h y d r a t e B D H c h e m i c a l s 2927.4530.05À1.551.05170161.15À5.2D i s o d i u m h y d r o g e n p h o s p h a t e d o d e c a h y d r a t e J o h n s o n M a t h e y c h e m i c a l s 3638.6541.552.655.55280269.7À3.68S o d i u m t h i o s u l p h a t e p e n t a h y d r a t e B D H c h e m i c a l s4849.8551.351.853.35200217.28.6L i t r a t .=L i t e r a t u r e v a l u e s ;E x p e r .=E x p e r i m e n t a l v a l u e s ;B D H =B r i t i s h D r u g H o u s e sT a b l e 4.T h e r m o p h y s i c a l p r o p e r t i e s o f s a l t h y d r a t e s m a t e r i a l sM e l t i n g p o i n t r a n g e (8C )H e a t o f f u s i o n (k J /k g )M a t e r i a ll i t e r a t u r em e a s u r e d a v e r a g e l i t e r a t u r e m e a s u r e da v e r a g eD e n s i t y (k g /m 3)C a l c i u m c h l o r i d e h e x a h y d r a t e 29.7[36]28.5±30.2[36]29[36]171[36]140±180[36]160[36]1634(C a C 12Á6H 2O )29*27.45±30.05[38]170*161.15[38]1630*s 1500*l S o d i u m c a r b o n a t e d e c a h y d r a t e (N a 2C O 3Á10H 2O )32±36±±247±±1442D i s o d i u m p h o s p h a t e d o d e c a h y d r a t e 34±372651522*s (N a 2H P O 4Á12H 2O )36**38±41.5[38]280**269.7[38]1520**1442**S o d i u m s u l f a t e d e c a h y d r a t e (N a 2S O 4Á10H 2O )31±32±±251±±1534s S o d i u m t h i o s u l f a t e p e n t a h y d r a t e 4848.5±49[36]48.7[36]201190±200[36]195[36]1666s (N a 2S 2O 3Á5H 2O )48*49.8±51.3[38]200*217.2[38]1730*s 1660*l*B r i t i s h D r u g H o u s e s (B D H )C h e m i c a l s .**J o h n s o n s ±M a t h e y C h e m i c a l s s =s o l i d ;l =l i q u i d .R e f e r e n c e s i n s q u a r e b r a c k e t sS.M.HASNAIN:REVIEW ON SUSTAINABLE THERMAL ENERGY1132S.M.HASNAIN:REVIEW ON SUSTAINABLE THERMAL ENERGY1133 Para ns.Para ns have been found to exhibit many desirable characteristics as PCMs for sto-rage applications,such as high heat of fusion,negligible supercooling,low vapor pressure in the melt,chemically inert and stable,self nucleating,no phase segregation and commercial avail-ability at reasonable cost[20,34,36±38].However,in spite of their many desirable properties, they have some undesirable properties,such as low thermal conductivity and large volume change during phase transition.Metallic®llers,metal matrix structures,®nned tube and alu-minium shavings were used to improve their thermal conductivity[38,40].To overcome the volume change on melting and freezing,plastic containers and di erent geometry of containers were used.Pure para n waxes are very expensive,therefore only technical grade para ns can be used for latent heat storage.Technical grade para ns are mixtures of many hydrocarbons and,therefore,have a melting temperature range rather than a sharp melting point.Some of the para ns investigated for heat storage applications include commercial waxes,n-eicosane and n-octadecane[37,38,40±42].Degradation of commercial grade para n wax was checked using Di erential Scanning Calorimetry(DSC)analysis after the completion of thermal cycling of wax in di erent types of tubes[38].Some of the results obtained from this study are reproduced in Table5.Quantitatively,the results of this study show that the addition of extended surfaces (aluminium honeycomb)in a tube reduces the melting temperature range by0.238C,but the as-sociated heat contents of the solid±liquid transition are increased by2.3%as compared to the original sample.The reason for this distortion could be the presence of impurities in the sample and,after extensive thermal cycling of the wax with metallic surfaces,might create hetero-geneous nucleation.Nevertheless,the(DSC)results with the technical grade para n wax did not show any indication that thermal cycling or contact with metal could degrade its thermal performance appreciably.The reported results showed[37,38]that one can not simply consider any available data on technical grade PCMs for designing an e ective heat storage device,as thermophysical properties vary from manufacturer to manufacturer,mainly due to di erent levels of impurities in technical grade PCMs.It is,therefore,recommended by Hasnain[38]to measure thermophysical properties of technical grade PCMs and not rely on published data unless at least the name of the supplier and purity level of the PCM are speci®ed.Non-para n organics.The non-para n organics include a wide variety of organic materials such as fatty acids,esters,alcohols and glycols.Hale et al.[22]reported about70non-para n organics that have melting points in the range of7to1878C and heats of fusion in the range of 42to250kJ/kg.Fatty acids have melting points suitable for heating applications and have heat of fusion values comparable to those of para ns and salt hydrates[43±45].They exhibit excel-lent melting/freezing characteristics without any supercooling.Their major drawback is their cost which is about three times greater than para ns.Feldman et al.[46,47],Galen and Brink[48],Hasan and Sayigh[49]and Hasan[50]performed investigations on a number of fatty acids by DSC measurement techniques.Some of the experimentally tested fatty acids are listed in Table6.This table reveals the di erent melting range of the same compound,probably due to di erent suppliers and di erent purity levels.The names of the suppliers and purity levels of the tested compounds are lacking in the literature.Fatty acid esters such as butyl stearate, vinyl stearate and methyl±12hydroxystearate,can be considered suitable for passive heat sto-rage.The compatibility of fatty acids with conventional materials of construction is another area which requires further investigation.Eutectics.Eutectics are mixtures of two or more salts which have de®nite melting/freezing points.Their behavior is analogous to congruent melting salt hydrates and have great potential for thermal energy storage applications.A large number of eutectics of inorganic and organic compounds have been reported in[7,34,39],and they can be classi®ed as inorganic eutectics,or-ganic eutectics and organic±inorganic eutectics.Some of the eutectics are listed in Table7. These eutectics can be used for cool and passive solar storage with low or medium temperature ¯at plate collectors,according to their melting point.Solid±solid PCMs,such as pentaerythritol(C5H12O4),pentaglycerine(CH3±C±(CH2±OH3), neopentyl glycol((CH3)2±C±(CH2OH)2)and their mixtures,were used as potential candidates for space heating and process heat applications[34].A highly crystalline polymer,such as high density polyethylene(HDPE)o ers de®nite advantages as a potential thermal energy storage material if it is rendered form stable by crosslinking.Salyer and Botham[51]demonstrated the。