微波仿真理论基础
微波技术基础课件 (10)[49页]
![微波技术基础课件 (10)[49页]](https://img.taocdn.com/s3/m/fc9f5c04783e0912a3162a12.png)
2
1
1
1
1
1
4ZL
ZL
Z Z
0 0
2
4tg 2Z0ZL
ZL Z0 2
2
1
4 sec2 Z0Z
ZL Z0 2
L
2
1
4Z0
Z
L
1
1
ZL
Z0
2
sec2
2
ZL Z0 cos 2 Z0 ZL
2
>>1
12
λ/4阻抗变换器及幅频特性
m
m
2
m
l
T12
? 2 e j 3 e j T12
T12 T21 2 3 e2 j4
18
1 T12 T21 3 e j2 ...
1 #1
l T21
#2
T12
总的反射系数
3
1 T12 T21 3 e j2 ...
1 T12 T21 3 e j2 2n 3n e2 jn
ZL Z0 j2tg Z0ZL
11
λ/4阻抗变换器幅频特性
Zin Zin
Z0 Z0
Zm ZL Zm ZL
Z0 Z0
jtg jtg
Z
2 m
Z
2 m
Z0ZL Z0ZL
ZL Z0
ZL Z0
2 4tg 2Z0ZL
1 2
1
1
Z L Z L
Z0 Z0
2 2
4tg 2Z0ZL
Zn
Z n1
Zn Zn1 Zn1
16
Z1 1
Z1 1 1
单节变换器
Z2
Z3
Z3
1
Z2 Z2
微波技术基础实验报告
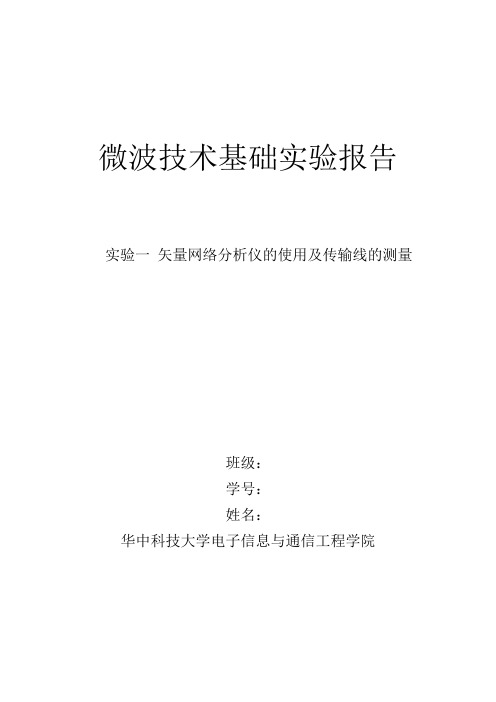
微波技术基础实验报告实验一矢量网络分析仪的使用及传输线的测量班级:学号:姓名:华中科技大学电子信息与通信工程学院一实验目的学习矢量网络分析仪的基本工作原理;初步掌握A V365380矢量网络分析仪的操作使用方法;掌握使用矢量网络分析仪测量微带传输线不同工作状态下的S参数;通过测量认知1/4波长传输线阻抗变换特性。
二实验内容矢量网络分析仪操作实验A.初步运用矢量网络分析仪A V36580,熟悉各按键功能和使用方法B.以RF带通滤波器模块为例,学会使用矢量网络分析仪A V36580测量微波电路的S参数。
微带传输线测量实验A.使用网络分析仪观察和测量微带传输线的特性参数。
B.测量1/4波长传输线在开路、短路、匹配负载情况下的频率、输入阻抗、驻波比、反射系数。
C.观察1/4波长传输线的阻抗变换特性。
三系统简图矢量网络分析仪操作实验通过使用矢量网络分析仪A V36580测试RF带通滤波器的散射参数(S11、S12、S21、S22)来熟悉矢量网络分析仪的基本操作。
微带传输线测量实验通过使用矢量网络分析仪A V36580测量微带传输线的端接不同负载时的S 参数来了解微波传输线的工作特性。
连接图如图1-10所示,将网络分析仪的1端口接到微带传输线模块的输入端口,另一端口在实验时将接不同的负载。
四实验步骤矢量网络分析仪操作实验步骤一调用误差校准后的系统状态步骤二选择测量频率与功率参数(起始频率600 MHz、终止频率1800 MHz、功率电平设置为-10dBm)步骤三连接待测件并测量其S参数步骤四设置显示方式步骤五设置光标的使用微带传输线测量实验步骤一调用误差校准后的系统状态步骤二选择测量频率与功率参数(起始频率100 MHz、终止频率400 MHz、功率电平设置为-25dBm)步骤三连接待测件并测量其S参数1.按照装置图将微带传输线模块连接到网络分析仪上;2.将传输线模块接开路负载(找老师要或另一端空载),此时,传输线终端呈开路。
微波技术基础 传输线理论1
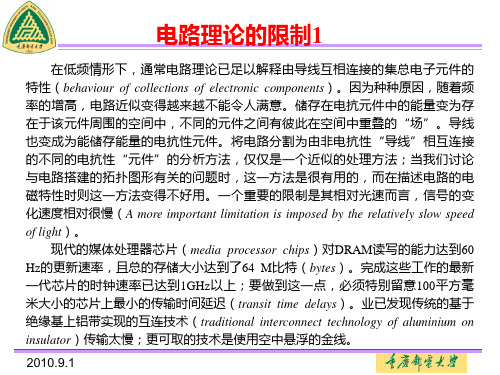
(2-2)
当典型Δz→0时,有
(2-3)
式(2-3)是均匀传输线方程或电报方程。
2010.9.1
如果我们着重研究时谐(正弦或余弦)的变化情况,有
u( z , t ) Re U ( z )e jt i( z , t ) Re I ( z )e jt
2010.9.1
(1-4)
一、低频传输线和微波传输线
r
r0 r0
图1-2 直线电流均匀分布
图1-3 微波集肤效应
损耗是传输线的重要指标,如果要将r0 R ,使损耗与直 流保持相同,易算出 1 R 3.03 m 2R0 也即直径是d=6.06 m。这种情况,已不能称为微波传输线,而 应称之为微波传输“柱”比较合适,其粗度超过人民大会堂的主 柱。2米高的实心微波传输铜柱约514吨重(铜比重是8.9T/m3),
同时考虑Ohm定律
V 1 Edl l R0 I Er02 r02 58 10 7 (2 10 3 )2 (1-1) . 137 10 3 / m .
代入铜材料
58 107 .
2010.9.1
一、低频传输线和微波传输线
2. 微波传输线 当频率升高出现的第一个问题是导体的集肤效应 (Skin Effect)。导体的电流、电荷和场都集中在导体表面. [例2]研究 f=10GHz=1010Hz、l=3cm、r0=2mm导线的线耗R. 这种情况下, J 0 e a ( r0 r ) J 其中, J 0 是r r0 的表面电流密度, 是衰线常数。对于良导 体,由电磁场理论可知
(2-4)
电子科大HFSS(01)微波仿真技术与微波仿真软件
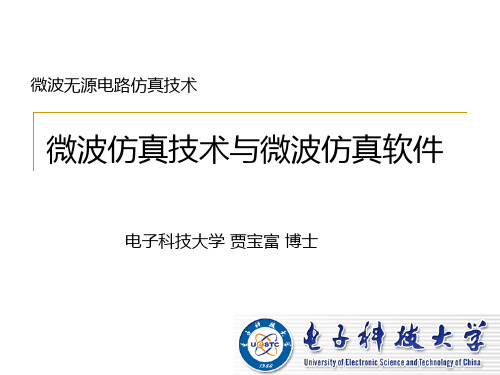
CST Design Studio界面
Ansoft Serenade
Ansoft Serenade 设计环境为现代的射频以及微波设计者们提供了一个强大 的电路、系统和电磁仿真的工具。简易的使用环境允许使用者们在仿真器和 其他的工具(如文字处理器)最大程度的产生数据资料的转移。简单的说, 它主要包括Harmonica电路仿真 和 Symphony系统仿真部分。 Symphony 可以在Serenade 文件夹(一种计算机标原理图获取、布局、和 仿真环境)下面运行。它是一个可以仿真有射频、微波和数字部分组成的通 信系统的软件工具。Symphony 添加了针对外围环境的高效的模拟、数字混 合方式(模拟和数字)和系统分析能力。使用者能够很快的构建一个系统包 括大量元件的库里的射频部分的模拟和数字信号处理。像信道编码,模拟和 数字信号处理滤波器,放大器,晶体震荡器衰减信道模型。这些都能使设计 者们迅速的组建有线的或者无线系统。由于系统自带了那么多模型,因此设 计者只需要对元器件键入很少的关键特征。它也能够对线性或非线性系统进 行彻底的操作。 它也能输出例如增益、噪声和在时域或者频域上的误码率。针对不同的设计 方法,在早期阶段的时候它就能够迅速的检查,以减少设计周期时间和避免 由于射频和数字信号处理系统之间的互相干扰而造成的高成本的重新设计。 一旦一个设计系统结构被确认了那么一个自上而下的设计流程就能被完成。 一个系统的误码率能够基于完整的系统分析而计算出来。对射频的描述,例 如阻抗不匹配,晶体震荡器的相位噪声和群延迟,在系统中(当然包括噪声, 输入功率,S参数和其他的输入信号扫描分析)把误码率的计算作为一个任何 参数的函数。
ADS主要应用
ADS功能非常强大,对整个现代通信系统及其子系统 的设计和仿真提供支持。主要应用有以下几个主要方 面:
微波技术基础_(PDF)

绪论0.1电磁波的频谱图 1 (J. D. Kraus: Electromagnetics)频段划分频率描述应用3-30kHz 超低频(Very low frequency,VLF)导航超长波大于10000米30-300kHz 低频(Low frequency, LF)导航台,导航设备长波:1000-10000米300-3000kHz 中频(Medium frequency, MF)调幅广播,海事无线,中波:100-1000米电,海岸巡逻通信,方向搜索3-30MHz 高频(High frequency,HF)电话,电报,传真,短波:10-100米短波国际广播,业余无线电,民用频段,船—岸和船—空通信30-300MHz 甚高频(Very high frequency, VHF)电视,调频广播,空米波:1-10米中交通控制,警用,出租车移动无线电300-3000MHz 超高频(Ultrahigh frequency UHF)电视,卫星通信,无分米波:1-10分米线电探空仪,监视雷达,导航设备3-30GHz 特高频(Superhigh frequency, SHF)机载雷达,微波传送,厘米波:1-10厘米卫星通信30-300GHz 极高频(Extreme high frequency, EHF)雷达毫米波:1-10毫米300GHz-3000GHz 太赫兹太赫兹技术0.2微波毫米波微波的频率范围不同的书有不同的说法,有将300MHz—30GHz、波长:1cm—1m特指微波;也有称300MHz—300GHz、波长:1mm—1m为微波;还有将300MHz—3000GHz、波长:0.1mm—1m统称为微波。
细分:微波:300MHz—30GHz,波长:1cm—1m毫米波:30GHz—300GHz,波长:1mm—1cm亚毫米波:300GHz—3000GHz,波长:0.1mm—1mm微波频段划分频段标称波长旧波段新波段500-1000MHz VHF C1-2GHz 22cm L D2-3GHz 10cm S E3-4GHz S F4-6GHz 5cm C G6-8GHz C H8-10GHz 3cm X I10-12.4GHz X J12.4-18GHz 2cm Ku J18-20GHz 1.25cm K J20-26.5GHz K K26.5-40GHz 0.8cm Ka K40-60GHz 0.6cm U60-80GHz 0.4cm V80-100GHz 0.3cm W微波毫米波的特点低于1GHz的通信电路通常由集总参数电路元件构成,超过1GHz到100GHz,集总元件被传输线和波导元件取代。
微波技术基础-传输线理论(4)
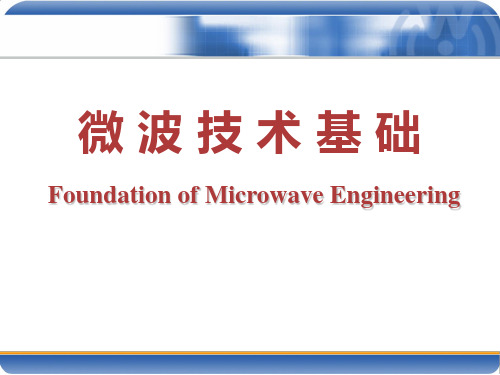
分界处波透射
A2 V0e j z T1 3 T2
9
四分之一波长变换器
➢ 多次反射观点
分量3:
Z0Z1分界处 V0e j z T1 3
分界处反射波 V0e j z T1 3 2
负载处入射波
V0e
j z
T132
e
负载处反射波 Z0Z1分界处
V0e
j
z
T1322
e
V0e
j z
T1322
0
Zin Zg*
Xin (Xin X g ) 0
Xin X g
——共轭匹配
源和负载失配
信号源与传输线的共轭匹配
设 Zg Rg jX g Zin Rin jX in
则
Rg Rin X g X in
➢可使信号源输出最大功率
源和负载失配
对于固定的源阻抗,可使最大的功率传向负载
P
1 2 Vg
s
1 (z) 1 (0) 1
——驻波比
源和负载失配
传送给负载的功率为:
p
1 2
Re{Vin Ii*n}
1 2
Vin
2
RRee{ZZ11i*nin}
2
1 2
Vg
2
Zin Zin Zg
RRe{ZZ11i*nin}
令
Zin Rin jX in
Z g Rg jX g
则得
p
1 2
Vg
2
( Rin
0
传到负载的功率为
p
1 2
Vg
2
Rg 4(Rg2 X g2 )
(2)
小于(1)给出的功率,可取Z0=Rg讨论
源和负载失配
分享一下我个人学习微波的过程,希望对初学微波者有用
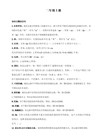
1、基础知识不仅要扎实,而且要经常复习。可能有些人会说这不是废话吗?基础不扎实怎么能学习的更深呢!但是仔细想想,人的记忆都符合遗忘规律,不经常接触的事物,一般都会遗忘(除牛人外),所以我经常复习基础知识,比如数学,它是所有学科的基础,微波行业更是如此,但是要用到哪学哪,因为你的学习时间太宝贵了了,不可能有太多的时间从头至尾学习,我经常复习高等数学和工程数学,高数贯穿于整个理论当中,工程数学也如此。既然学微波,微波技术基础必须学习,尤其第一章更要学习,它可是微波传输基础之基础,其他章节活学活用。本人推荐视频教程西电梁老的《微波技术基础》,讲的颇为精辟,不愧为国家精品课程!
4、理论与实践相结合。在学习理论知识的同时,应结合实际的工作过程强化理论,提高进一步的理解,就是在你做实际工作但中以理论为指导,自己掌握工作的完成度,在此过程当中要多思考,学会用理论分析实际问题的能力,经常请教高人,多交流,这样对自己的提升很快。
以上是我个人的学习微波的过程,希望对初学者有用,其中有错误之处请大家多多指点,也希望大家能多多分享自己的学习过程。
2、与此同时,电磁场与电磁波也要学习,微波传输是以电磁波为媒质,波技术的基础就是电磁波传输,学习的深度适个人情况而定,推荐书籍高等教育出版社,作者:谢处方 饶克谨的《电磁场与电磁波》,记得结合着数学啊!
3、微波电路时微波系统的组成的基础,微波电路的性能直接决定着微波系统的好与坏,因此对于微波电路的设计方法,应该掌握,比如放大器、滤波器、双工器、功分/合路器等,放大器的设计至关重要,应该重点掌握,像LNA、PA、Mixer、VCO等都是以放大器设计为基础,学习的同时结合EDA软件,通过仿真结果,来验证电路的功能与指标的符合性,为实际的电路设计作指导。推荐书籍电子工业出版,王子宇等翻译的《射频电路设计-理论与应用》,视频教程台湾中华大学的田庆成主讲的《射频放大器设计》,EDA软件推荐安捷伦科技的ADS,它不仅限与电路级仿真,在系统级仿真也是一个不错的软件。参考书籍《ADS应用详解-射频电路设计与仿真》。
微波工程-第1章电磁理论
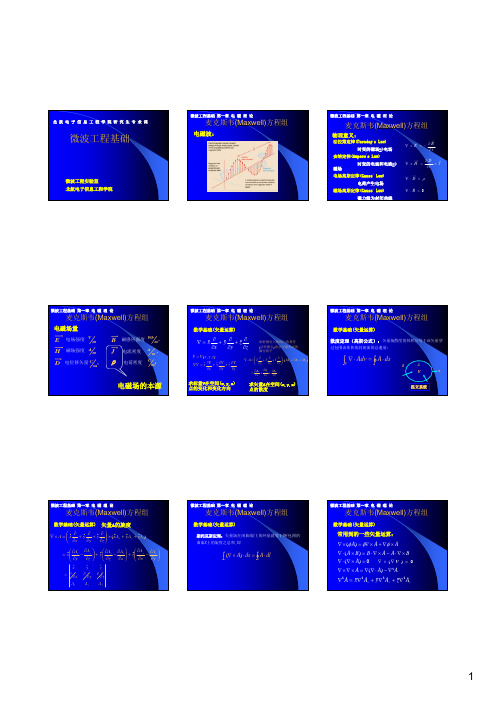
E H D
电场强度 V m 磁场强度 A m 电位移矢量 C
m2
B J
磁感应强度 Wb m 2 电流密度 电荷密度
A
C
ˆ x
ˆ ˆ y z x y z
哈密顿引入的倒三角算符 (读作德尔)表示矢量形式的 微分算子
m2
m3
V V ( x, y , z ) V V V y V x z x y z
xy yy zy
xz E x yz E y E z zz
xy yy zy
xz 1 yz 0 zz 0
0 1 0
0 0 I 1
界面源
任何一个方向的电场,如 E x可以产生三個不 同方向的电位移矢量,如以下三式
r 相对磁导率
7
r
相对介电常数
4 10
真空磁导率 真空介电常数
0 8.854 10 12
( H ) ( D ) J 0 t D J 0
t
B d s t D ( 2 ) s ( H ) d s c H d l s .d s s J d s t 利用散度定理: (1) ( E ) d s
P e 0 e E
Pe :
Polarization Vector(极化向量) 电极化强度 Susceptibility(电极化率)
媒质中的场和边界条件
电介质材料+电导率:
H j D J ; j E E
j [ ' ( j ''
- 1、下载文档前请自行甄别文档内容的完整性,平台不提供额外的编辑、内容补充、找答案等附加服务。
- 2、"仅部分预览"的文档,不可在线预览部分如存在完整性等问题,可反馈申请退款(可完整预览的文档不适用该条件!)。
- 3、如文档侵犯您的权益,请联系客服反馈,我们会尽快为您处理(人工客服工作时间:9:00-18:30)。
Basic BJT CircuitFigure 1 below shows the simplied ‘Pi’ model of a BJT.cVinVoutZinVoutBCΒ.ib ibFigure 1 Transistor symbol and simplified ‘Pi’ modelWe can see that output consists of a current source –gm.Vbe to get the output voltage we multiply by the load resistance Rce ie Vout = -gm.Vbe.Rce (the negative sign denotes signal inversion).The input resistance of the circuit is given by:e temperatur room at (23.5mV)0.0235V ely approximat is and voltage thermal the as known is V (mS)ctance Transcondu gm Kelvin in e Temperatur T 1.6022x10 charge Electron q 1.3807x10 constant Boltzmansk where q k.T V ; V I gm where gmβR T 19-123-T T CQ IN =========−CJKThe output resistance is given by:ge(V)EarlyVolta V Where I Vrce R A CQA OUT ===The voltage gain (Av) is given by:TA CQ A T CQ be be IN OUT V V VI V .V I rce . V rce .V . V V A ==−=−==gm gmThe current gain (Ai) is given by:β- i β.i - I I A bbIN OUT i ===The MOS TransistorThese devices are known as FET’s (Field effect transistors), which consist of three regions source, drain and gate. The resistance path between the drain and source is, controlled by applying a voltage to the gate. This varies the depletion layer under the gate and thusreduces or increases the conductance path. The FET input impedance (unlike the BJT which is a few K Ω) is very high (~M Ω’s) and as a result the gate current can be considered as zero.As per the BJT the FET is best described by it’s Output I-V DC characteristics (N-typeenhancement characteristics shown below), however things are complicated by the fact there are two types of FET depletion and enhancement that are both available as N-type or P-type devices. For low frequencies the enhancement devices is more commonly used (Depletion mode types will be described when discussing microwave devices).0 = 0V123Triode Region Or Linear RegionGST GS DS V V V −=(1) Cut-Off Region – In this region the gate voltage is less than the pinch-off voltage Vp and therefore very little current flows.(2) Triode Region – In this mode the device is operating below pinch-off and is effectively a variable resistor. R OUT is ~ linear but only over a small range of V DS .(3) Saturation Region – This is the main operating region for the device. The drain voltage has to be greater than the gate voltage less the pinch-off voltage – this sets the minimumsupply voltage. The curves in the saturation region can be extrapolated to a point 1/λ, where λ is known as the ‘Channel length modulation parameter, (units V -1), - this is analogous to the BJT Early voltage.Referring to the saturation region we can assume the response is approximately linear such that the:-resistance high e,conductanc small ie λ.I G λ1I λ1V I V 1if then0.01 to 0.001 region the in typically is λλ1V I G therefore 1I .G1- VDS then c mx - y form the of line straight a assume we If G e conductanc output Device curve of slope R resistance output Device curveof slope 1D O DDS D DS DS D O D OOO≈−−=⎟⎠⎞⎜⎝⎛−−>>⎟⎠⎞⎜⎝⎛−−=+⎟⎟⎠⎞⎜⎜⎝⎛=+===λλTo complete the model for the FET we need to add the term for the linear region which, is dependant on the device mobility and gate dimensions.I ()DS DS DS DS DS DSO DQ DQ D λ.V 1.I .V λ.I I .V G I I I +=++∆+=DS DS T GS OX O V .2-V -V .L .C .⎥⎦⎤⎢⎣⎟⎠⎜⎝µ()()parametermodulation length Channel voltagethreshold Device VT ratio aspect the as Known W/L length channel Effective L widthchannel Effective W oxidegate of area unit per e capacitanc tC device of mobility Surface Where onlyregion /linear saturation -non .V 1V .2V -V -V .L W .C . ID OXOX OX O DS DS DS T GS OX O ========+⎥⎦⎤⎢⎣⎡⎟⎠⎞⎜⎝⎛=λεµλµFor saturation region ie V DS > (V GS -V T )[]()[]()parameterctance transcondu Intrinsic the as Known .C µ K Where λ.V 1V -V 2L W .KI aswritten -re Sometimes parameter ctance transcondu the as Known 2L W .C µβ Where λ.V 1V -V β I OX O P DS 2T GS P D OX O DS 2T GS D =+⎦⎤⎢⎣⎡=⎥⎦⎤⎢⎣⎡=+=Usually λ.V DS << 1 so[]2T GS D V -V β I ≈The following page shows some typical values of the above parameters for use with a level 1 MOS model. The ADS version of this model is also shownTypical MOS Spice Parametersn-Well CMOS Level 1 SPICE Model parametersLevel 1 SPICE Parameter n-channelMOSFETp-channelMOSFETUnitsGate oxide thicknessTOX150 150 Angstrom TransconductanceParameter KP50 x 10-625 x 10-6Amp/V2 Threshold Voltage VT0 1.0 -1.0 VoltsChannel-length modulation parameter LAMBDA 0.1/LL in micron0.1/LL in micronV-1Bulk ThresholdParameter GAMMA0.6 0.6 V1/2Surface Potential PHI 0.8 0.8 VGate-drain overlapcapacitance CGDO5 x 10-10 5 x 10-10F/mGate-source overlapcapacitance CGSO5 x 10-10 5 x 10-10F/mZero-bias planar bulkdepeletioncapacitance CJ10-4 3 x 10-4F/m2Zero-bias sidewall bulkdepletion capacitanceCJSW5 x 10-10 3.5 x 10-10 F/mBulk junction potentialPB0.95 0.95 V Planar bulk junctiongrading coefficient MJ0.5 0.5 None Sidewall bulk junctiongrading coefficientMJSW0.33 0.33 NoneVAR VAR2LAMBDA=0.1/LL=0.5W=100MOSFET_NMOS MOSFET1Mode=nonlinearTemp=Region=Mult=Nrs=Nrd=Ps=Pd=As=Ad=Width=W um Length=L um Model=MOSFETM1LEVEL1_Model MOSFETM1AllParams=Imax=Ffe=Tt=N=Tnom=Rds=Rg=Fc=Af=Kf=Gdsnoi=1Nlev=Uo=Ld=Tpg=Nss=Nsub=Tox=150e-10Js=Mjsw=0.33Cjsw=5e-10Mj=0.5Cj=1e-4Rsh=Cgbo=Cgdo=5e-10Cgso=5e-10Pb=0.95Is=Cbs=Cbd=Rs=Rd=Lambda=LAMBDA Phi=0.8Gamma=0.6Kp=50e-6Vto=1PMOS=no NMOS=yesAs with the BJT it is possible to simulate a device under ADS to produce the device Output I-V curve trace for a typical N-type MOS 3.3V 0.25um process enhancement device. The Spice data for the MOSFET model is called up from the spice file ‘tsmc_’.spiceInclude SPICE1File="tsmc_"NetlistDebugMode=0VAR VAR1VDS=0VGS=0DC1SweepVar="VDS"Start=0Stop=3.3Step=.1Other=OutVar="MOSFET1.Gm"Sweep1SweepVar="VGS"SimInstanceName[1]="DC1"SimInstanceName[2]=SimInstanceName[3]=SimInstanceName[4]=SimInstanceName[5]=SimInstanceName[6]=Start=0Stop=2Step=0.2The resulting plot0.00.51.01.52.0 2.53.0 3.5-55 1015 20 25 30 35 40 45 VgsVDSIDS.i, mAThe device will also have a transconductance Curve ie V GS vs I DS . The ADS simulation below sweeps the gate voltage and measures the resulting drain current.DC1Other=OutVar="MOSFET1.Gm"Step=.1Stop=3Start=0SweepVar="VGS"VAR VAR1VDS=3.3VGS=0spiceInclude SPICE1File="tsmc_"NetlistDebugMode=0Resulting transconductance curve, slope is the G M of the device.0.00.20.40.60.81.0 1.2 1.4 1.6 1.82.0 2.2 2.4 2.62.83.00.0000.0050.0100.0150.0200.0250.0300.0350.0400.0450.0500.0550.0600.0650.0700.0750.0800.085VGSIDS.iSlope of curve = gmIDS (A)Pinch-off voltage = 0.6VAnd for the P-type deviceInput transconductance traceMOSFET_PMOS MOSFET1Mode=nonlinearTemp=Region=Mult=2Nrs=Nrd=Ps=Pd=As=Ad=Width=100 um Length=0.5 um Model=MODpch3_1DC1Other=OutVar="MOSFET1.Gm"Step=-.1Stop=-5Start=0SweepVar="VGS"VAR VAR1VDS=-3.3VGS=0spiceInclude SPICE1File="tsmc_"NetlistDebugMode=0Resulting trace-5-4-3-2-1-0.07-0.06-0.05-0.04-0.03-0.02-0.010.00VGSIDS.ispiceInclude SPICE1File="tsmc_"NetlistDebugMode=0MM9_NMOS MOSFET1Mode=nonlinearMult=2Temp=Lg=Ls=Ab=Width=100 um Length=0.5 um Model=MODnch3_1ParamSweep Sweep1Step=1Stop=5Start=0SimInstanceName[6]=SimInstanceName[5]=SimInstanceName[4]=SimInstanceName[3]=SimInstanceName[2]=SimInstanceName[1]="DC1"SweepVar="VGS"DC1Other=OutVar="MOSFET1.Gm"Step=.1Stop=3Start=0SweepVar="VDS"VAR VAR1VDS=3.3VGS=0Output characteristic trace-5-4-3-2-1-0.07-0.06 -0.05 -0.04 -0.03 -0.02-0.01 0.00 0.01 VDSIDS.iBody EffectThe FET body or ‘Bulk’ is known either as the substrate, back gate or more commonly the Body. It is normally connected to the lowest voltage potential of the circuit (usually thesource). However if is left unconnected its effect on the DC characteristics of the device must be taken into account. If we include the bulk effect the value of the threshold voltage V T will increase with increasing bulk voltage.()source) the to connected bulk (ie 0 V for V V normally Therefore,(Volts)potential source Bulk V (Volts) potential level Fermi .F (Volts)parameter hold Bulk thres γ.F 2..F 2.V -γ V V BS T TO BS BS TO T ====Φ=Φ−Φ++=If the device was biased without the bulk node connected then a change in operating point could take the device out of its saturation region and significantly change the circuitperformance. The bulk voltage is thus a very important parameter in circuit applications and therefore it is best to connect the bulk to the device source connection.The circuit is drawn as follows:-The simulation below shows how varying the bulk voltage will vary the pinch-off voltage of the device.VAR VAR1VBS=0VGS=0Sweep1Step=0.1Stop=1Start=0SimInstanceName[6]=SimInstanceName[5]=SimInstanceName[4]=SimInstanceName[3]=SimInstanceName[2]=SimInstanceName[1]="DC1"SweepVar="VGS"DC1Other=OutVar="MOSFET1.Gm"Step=0.5Stop=3Start=0SweepVar="VBS"V_DC SRC4Vdc=VBSspiceInclude SPICE1File="tsmc_"NetlistDebugMode=0Resulting plot showing that the pinch-off voltage increases with increasing bulk voltage0.00.20.40.60.8 1.00.0000.0010.0020.0030.0040.0050.0060.0070.008IDS.iIncreasing pinch-off voltageFrom the last section we found that the drain current in the saturation region =[]2T GS D V -V β I ≈Transconductance[][][][][]D 5.0D 0.50.5-5.0D 1D DT GS 2T GS D T GS GST GS D GS 2T GS D GSD M I .β2 I .2β βI .2β βI 2βGM (1) into sub βIV -V then V -V β I rearrange we If(1) - V -V 2β GM V .V -V 2β I V wrt ate differenti therefore V -V β I curvetransfer of slope ie V IG =======∆=∆=∆∆=Output Conductance()()()()λ.I G V I V λ..I I (2)into sub V -V βI above From (2) - V λ..V -V β I V wrt ate Differenti λ.V 1V -V βI sticcharacteri output of slope ie ∆V ∆I G D O DSDDS D D 2T GS D DS 2T GS D S D DS 2T GS D DSDO ==∆∆=∆=∆=∆=∆+==Voltage Gain AL .W K .I 2.λ1 .I .L .2.W .K .λ2 I 1.2L .W K .λ2 2L .W K β and I β.λ2 A .λI .2.β .λ.I I .2.β λ.I β.I 2 G G e Conductanc Output ctance transconduA P D 0.5-D 0.5-0.5-0.50.5P 1-1D P P D10.5-D 0.511D 0.5D 0.5DD O M ==========−−−Common-Base/Gate CircuitsCommon-Base BJT circuitThe figure below shows the simplied ‘Pi’ model of a common-base BJT.r ceVinZinV I e wher gm 1r 1)(βi 1).r (βi I V R TCQ be b be b IN IN IN ===++==gm)r gm1(as V V I V .V I r r 1)r (βr .β 1)r (βi r .i .β V V A ce T A CQ A T CQ be ce be ce be b ce b IN OUT V ===≈+=+==()1 1ββ)1βi β.i I I A b b IN OUT i ≈+=+==r ceTo determine the Output impedance of the circuit we can connect a voltage source (V s + R s ) to the base and ground the input ie the emitter. We then have to resistances in parallel connected to the current source β.i b .()equationabove into sub R r R i i Also r .i r i .i V i VR sbe sT b be b ce b T OUT TOUTOUT +=++==βsbe be s s be s ce ce T OUT OUT be s ce sT ce s be s T TOUT R r r.R R r R r .r i V R r .R r R i r R r R i .i V ++++==++⎟⎟⎠⎞⎜⎜⎝⎛++=ββ()ce ce ce OUT s ceOUT s r .1 1r .r R then large R If r R then 0 R If +=++====ββCommon-Gate MOSFET Circuitgr dsVoutVinI INVoltage Gain A v()R r .R r gm A //R r gmVsg V Vsg V V VA L dgL dg V L dg O IN INOV ⎟⎟⎠⎞⎜⎜⎝⎛+====Input ResistanceλI gm1 gmVgs Vgs I VR D IN IN IN ====Output ResistanceAs the source is low impedance ie close to ground for R OUT – r ds appears to be connected across r ds to ground.ds dg OO OUT //r r I VR ==Current Gain A iA i = 1Common-Emitter/Source CircuitsCommon-emitter BJT circuitThe figure below shows the simplied ‘Pi’ model of a common-emitter BJT.VoutEBCΒ.ib ibVinVoutZin(mS)ctance Transcondu Kelvinin e Temperatur T 1.6022x10charge Electron q 1.3807x10 constant Boltzmans k whereq k.T V ; V I where R 19-123-T TCQ IN =========−gm C JK gm gm βge(V)EarlyVolta V Where I Vrce R A CQA OUT ===TA CQ A T CQ be be IN OUT V V VI V .V I rce . V rce .V . V V A ==−=−==gm gmββ- i .i - I I A b bIN OUT i ===Common-Source MOS FET CircuitdrainVoutsd IoVoltage Gain A v()R r .R r gm - A //R r gmV - V V VA L dsL ds L ds IN O INO V ⎟⎟⎠⎞⎜⎜⎝⎛+=== Input ResistanceR IN = ∞Output ResistanceL ds OOOUT //R r I VR ==Current Gain A iA i = ∞Common-Collector/Drain CircuitsCommon-Collector Drain BJT CircuitFigure 1 below shows the simplied ‘Pi’ model of a common-collector BJT.B Cβ.i bi bVinZinLLLLLLggoR1rce1'R1Rrcerce.R'R+=+=+='.R)1('RrII).1('RI.rIVVIVRLLbebbLbbeINRLbeINININβββ≈++=++=+==rceRthenrceRifRrcee.Rr'R1).i('R.1).i(IVROUTLLLLbLbOUTOUTOUT≈<<+==++==cββ1)(bybottom&topdivide)1('Rr)1('RI).1('RI.rI).1('RVVALbeLbLbbebLINOUTV++++=+++==βββββLLLLVbeLbeLVggo'R1abovefrom'R)1(.'RAgmr'R)1('RA+=++=∴=++=ββββgm1)1(.ggo1ggobybottom&topMultiplyggo1)1(.ggoggo1ALLLLLV+++=++++++=ββββgm gmδαδββα.11A gm g go let and 1Let V L +=+=+=()()βββ 1 i i .1I IA b b INOUT i ≈+=+==Common-Drain MOS FET CircuitdrainVoutVindVoltage Gain A v()()()()()()1A 1AAv Therefore, //R r gm Av //R r gm 1//R r gm Av by Vgsbottom & top divide //R r gmVgs Vgs //R r gmVgsAv //R r gmV Vo V V VV VAv L ds L ds L ds L ds L ds L ds gs Ogs OIN o ≈+==+=+==+==Input ResistanceR IN = ∞Output Resistancegm1I V R O O OUT ==Current Gain A iA i = ∞Common-Emitter Circuit with emitter degenerationThe figure below shows the simplied ‘Pi’ model of a common-emitter BJT with emitter de-generation.VinBCβ.ib ibVinVoutZinZoutLce L ce L EL E L E be L E b be b L b IN OUT V R r .R ris 'R Where R 'R - R 'R .- 1)R (βr 'β.R - 1)R (βi .r i 'R ..βi - V V A +=≈≈++=++==αE E be bEb be b IN IN IN 1)R (β 1)R (βr i 1)R (βi .r i I V R +≈++=++==ββ i .i I IA bbIN OUT i ===VoutTo determine the Output impedance of the circuit, we ground the base and the emitter. We then have two resistances in parallel connected to the current source β.i b .()()equation above into sub r //R i V Also V r gmV i Vi VR be E T be bece be T OUT TOUT OUT +=++==()()()()()()()()()()⎟⎟⎟⎟⎠⎞⎜⎜⎜⎜⎝⎛++==⎟⎟⎟⎟⎠⎞⎜⎜⎜⎜⎝⎛++=⎟⎟⎠⎞⎜⎜⎝⎛++=−++==++=++=1βgm.R R .gm 1r R βgm r 1 and 1r R R .gm 1r r by bottom and top divide r R r .R .gm 1r R ignored be can and small is term This r //R r //R r //R gm 1r i V R r //R i r //R gm 1.i r r //R i r r //R i .gm i V EE ce OUT be be E E ce be be E be E ce OUTbe E be E be E ce TOUTOUT be E T be E T ce be E T ce be E T T OUT)gmR (1r R gmR if E ce OUT E +=<<βGenerallyThe de-generated CE circuit provides DC regulation but the load andemitter resistors may have similar values and hence the voltage gain will be low. This however, can be overcome by RF bypassing RE, with a smaller RE, resulting in high voltage gain while maintaining the correct DC bias conditions.The circuits below illustrate the problem and solution.Say we require an amplifier with a voltage gain of 15, a C-E voltage of 5V with an Ic current of 5mA to give us best noise performance. If we equally apportion the voltage we get the following circuit:-V INThe voltage gain of this circuit will be ~ – 700/700 = ~ -1, but we can bypass the emitter resistor as shown below:-V INFor DC the capacitor is open circuit and we have the emitter resistor back to 700 Ω. However at high frequencies the capacitor shorts out the 650Ω so that R E is now effectively 46Ω.The gain is now – (700/46) = -15. (-ve because it is inverting).De-generated MOS FET CircuitThe de-generated MOSFET circuit is an extension of the common-drain circuit except in this case we have a load on the drain as well as the source as shown in Figure 1. The resulting gain will be a ratio of R S/R D and the output resistance will ~ R D. The resistance looking into VX will increase and therefore will make this circuit useful as a current sink especially on the differential amplifier tail device where high R improves the CMMR.dI SVXROUT’Figure 1 Schematic and small signal model of the de-generated MOSFET circuit. Output ResistanceFirstly consider in the increase in the output resistance due to R OUT prime.If we apply a current source on V OUT of value I T current will try to flow to ground via the device current source as source resistor. If we tried to force current to flow we would find the voltage would greatly increase as a result of the high resistance R OUT prime.Therefore, referring to figure 2 we can show this circuit.dAs the gate has been grounded then vg = 0,Therefore, Vgs = Vg - Vs = 0 - R S.I TVgs = Vs = -R S.I TThe current source in the device gives rise to a currentI T = gm.Vgs, so theVoltage Vs = -gm.Vgs.RsThe voltage drop across RS adds current to the output ie.The total current at the output will be I T and the current from the current source connected to Rs.()()()()gm.Rs Rds. R then small is Rs as )gmRs Rds(1Rs I )gmRs Rds(1Rs I I VR Now )gmRs Rds(1Rs I Vget to factorise R I .I Rds.gm.R Rds.I ie out multiply R I .I gm.R I Rds V 1equation in Sub R I Rds.Ids Vs Vds V (1)- gm.Rs.I I Ids OUT TT T T OUT T T S T T S T S T T S T T S T T T T ≈++=++==++=++++=+=+=+=This circuit exhibits negative feedback which gives rise to the increased resistance.Voltage Gain (Av)From the above equations we now easily calculate the voltage gain of the degenerated MOSFET circuit ie())gmRs Rds(1Rs I V V and .RsI Vs Vgs V work previous the from V VAv T T OUT T IN INOUT++======()()S IN OUT SIN OUT S T T T IN OUT T T OUT T IN R Rds V V to reduces this so Rds than is term Rds.gm the R Rds.gmRds 1V V R )gmRs Rds(1Rs give to cancel I .Rs I )gmRs Rds(1Rs I V V therefore)gmRs Rds(1Rs I V V and .RsI Vs Vgs V =<<++=∴++++=++=====Input resistanceThe input resistance of the circuit will be very, very high.The ADS simulation of figure 3 shows a simple common-source circuit. However, there is an ideal bias supply to the drain ie supplies only DC and is an open-circuit to AC/RF. There is also a DC decoupled S-parameter termination connected to Vout and set to a very highimpedance. This allows measurement of the resistance looking into the Vout port, setting the termination value very high ensures it doesn’t interfere with the already high impedance caused by Rd.Note for the first plot if figure 4 the value of RS (R3) was set at 0 ohms.The ADS simulation was modified but the value of RS was increased to 2500ohms (there needed to be a slight alteration to the gate bias to restore the drain current back to 10uA).The second simulation plot is shown in figure 5 and shows how the resistance has increased from 9.5M Ω to 13M Ω. S_Param SP1Stop=10 MHzStart=1 MHz S-PARAMETERSDCACW=10EqnVar V_DC SRC1Vdc=5 VFigure 3 ADS simulation set-up for a simple common-source amplifier. ROUT will effectively be defined by Rd of the FET. The S-parameter DC decoupled terminal and simulation box allow the measurement of R OUT .Sheet5 of 5freq, MHzreal(Z(1,1))Figure 4 Simulation of the circuit shown in figure 3 with the source resistor RS (R3) set to 0 ohms (ie the circuit is configured as common-source). Output resistance is 9.5M ohms.real(Z(1,1))freq, MHzFigure 5 Simulation of the circuit shown in figure 3 with the source resistor RS (R3) set to 2500 ohms (ie the circuit is configured common-source with de-generation). Output resistance has now 13M ohms. NOTE there was a slight alteration to the gate bias to restore the drain current back to 10uA.。