Hydrogen adsorption in different carbon nanostructures
不同取代度的寡聚精氨酸壳聚糖的合成与鉴定_何文

将反应液装入透析袋( MW = 8 000 ~ 14 000) 中,浸没在去离子水中透析 48 h,然后冷冻干燥,得 到产物 HCS-R9-1。 2. 1. 2 高取代 HCS-R9 的制备 采用 2. 1. 1 中的
方法使 R9 与 HCS 以 40∶ 1 的摩尔比发生反应,经过 透析、冷冻干燥,得到产物 HCS-R9-2。 2. 1. 3 低取代 LCS-R9 的制备 采用 2. 1. 1 中的方 法使 R9 与 LCS 以 5 ∶ 1 的摩尔比发生反应,经过透 析、冷冻干燥,得到产物 LCS-R9-1。 2. 1. 4 高取代 LCS-R9 的制备 采用 2. 1. 1 中的 方法使 R9 与 LCS 以 35∶ 1 的摩尔比发生反应,经过 透析、冷冻干燥,得到产物 LCS-R9-2。 2. 2 CS-PR 的结构鉴定[12] 2. 2. 1 傅里叶红外光谱分析( FTIR) 分别取适量 的 CS、R9、HCS-R9-1、HCS-R9-2、LCS-R9-1、LCS-R92 冻干粉末,与干燥的 KBr 粉末以 1 ∶ 100 的质量比 混匀并溶 于 适 量 去 离 子 水,烘 干,用 玛 瑙 研 钵 研 成 细粉末,将 此 混 合 物 压 片,置 于 傅 氏 转 换 红 外 线 光 谱分 析 仪 ( FTIR) 内 扫 描,扫 描 范 围:4 000 ~ 400 cm - 1 ,记录其红外光谱。 2. 2. 2 1 H-核磁共振谱(1 H-NMR) 取适量的 CS、 R9、HCS-R9-1、HCS-R9-2、LCS-R9-1、LCS-R9-2,分别 溶于体 积 比 为 5 ∶ 95 的 CD3 COOD ∶ D2 O 中,在 150 MHz 频率照射,20℃ 下核磁共振仪记录图谱。 3 结果
常用分析化学专业英语词汇

常用分析化学专业英语词汇absorbance 吸光度absorbent 吸附剂absorption curve 吸收曲线absorption peak 吸收峰absorptivity 吸收系数accident error 偶然误差accuracy 准确度acid-base titration 酸碱滴定acidic effective coefficient 酸效应系数acidic effective curve 酸效应曲线acidity constant 酸度常数activity 活度activity coefficient 活度系数adsorption 吸附adsorption indicator 吸附指示剂affinity 亲和力aging 化amorphous precipitate 无定形沉淀amphiprotic solvent 两性溶剂amphoteric substance 两性物质amplification reaction 放大反响analytical balance 分析天平analytical chemistry 分析化学analytical concentration 分析浓度analytical reagent (AR) 分析试剂apparent formation constant 表观形成常数aqueous phase 水相argentimetry 银量法ashing 灰化atomic spectrum 原子光谱autoprotolysis constant 质子自递常数au*ochrome group 助色团back e*traction 反萃取band spectrum 带状光谱bandwidth 带宽bathochromic shift 红移blank 空白blocking of indicator 指示剂的封闭bromometry 溴量法buffer capacity 缓冲容量buffer solution 缓冲溶液burette 滴定管calconcarbo*ylic acid 钙指示剂calibrated curve 校准曲线calibration 校准catalyzed reaction 催化反响cerimetry 铈量法charge balance 电荷平衡chelate 螯合物chelate e*traction 螯合物萃取chemical analysis 化学分析chemical factor 化学因素chemically pure 化学纯chromatography 色谱法chromophoric group 发色团coefficient of variation 变异系数color reagent 显色剂color transition point 颜色转变点colorimeter 比色计colorimetry 比色法column chromatography 柱色谱plementary color 互补色ple* 络合物ple*ation 络合反响ple*ometry ple*ometrictitration 络合滴定法ple*one 氨羧络合剂concentration constant 浓度常数conditional e*traction constant 条件萃取常数conditional formation coefficient 条件形成常数conditional potential 条件电位conditional solubility product 条件溶度积confidence interval 置信区间confidence level 置信水平conjugate acid-base pair 共轭酸碱对constant weight 恒量contamination 沾污continuous e*traction 连续萃取continuous spectrum 连续光谱coprecipitation 共沉淀correction 校正correlation coefficient 相关系数crucible 坩埚crystalline precipitate 晶形沉淀cumulative constant 累积常数curdy precipitate 凝乳状沉淀degree of freedom 自由度demasking 解蔽derivative spectrum 导数光谱desiccant; drying agent 枯燥剂desiccator 保干器determinate error 可测误差deuterium lamp 氘灯deviation 偏差deviation average 平均偏差dibasic acid 二元酸dichloro fluorescein 二氯荧光黄dichromate titration 重铬酸钾法dielectric constant 介电常数differential spectrophotometry 示差光度法differentiating effect 区分效应dispersion 色散dissociation constant 离解常数distillation 蒸馏distribution coefficient 分配系数distribution diagram 分布图distribution ratio 分配比double beam spectrophotometer 双光束分光光度计dual-pan balance 双盘天平dual-wavelength spectrophotometry 双波长分光光度法electronic balance 电子天平electrophoresis 电泳eluent 淋洗剂end point 终点end point error 终点误差enrichment 富集eosin 曙红equilibrium concentration 平衡浓度equimolar series method 等摩尔系列法Erelenmeyer flask 锥形瓶eriochrome black T (EBT) 铬黑Terror 误差ethylenediaminetetraacetic acid (EDTA) 乙二胺四乙酸evaporation dish 蒸发皿e*change capacity 交换容量e*tent of crosslinking 交联度e*traction constant 萃取常数e*traction rate 萃取率e*traction spectrphotometric method 萃取光度法Fajans method 法斯法ferroin 邻二氮菲亚铁离子filter 漏斗filter 滤光片filter paper 滤纸filtration 过滤flue* 溶剂fluorescein 荧光黄flusion 熔融formation constant 形成常数frequency 频率frequency density 频率密度frequency distribution 频率分布gas chromatography (GC) 气相色谱grating 光栅gravimetric factor 重量因素gravimetry 重量分析guarantee reagent (GR) 保证试剂high performance liquid chromatography (HPLC) 高效液相色谱histogram 直方图homogeneous precipitation 均相沉淀hydrogen lamp 氢灯hypochromic shift 紫移ignition 灼烧indicator 指示剂induced reaction 诱导反响inert solvent 惰性溶剂instability constant 不稳定常数instrumental analysis 仪器分析intrinsic acidity 固有酸度intrinsic basicity 固有碱度intrinsic solubility 固有溶解度iodimetry 碘滴定法iodine-tungsten lamp 碘钨灯iodometry 滴定碘法ion association e*traction 离子缔合物萃取ion chromatography (IC) 离子色谱ion e*change 离子交换ion e*change resin 离子交换树脂ionic strength 离子强度isoabsorptive point 等吸收点Karl Fisher titration 卡尔•费歇尔法Kjeldahl determination 凯氏定氮法Lambert-Beer law 朗泊-比尔定律leveling effect 拉平效应ligand 配位体light source 光源line spectrum 线状光谱linear regression 线性回归liquid chromatography (LC) 液相色谱macro analysis 常量分析masking 掩蔽masking inde* 掩蔽指数mass balance 物料平衡matallochromic indicator 金属指示剂ma*imum absorption 最大吸收mean, average 平均值measured value 测量值measuring cylinder 量筒measuring pipette 吸量管median 中位数mercurimetry 汞量法mercury lamp 汞灯mesh [筛]目methyl orange (MO) 甲基橙methyl red (MR) 甲基红micro analysis 微量分析mi*ed constant 混合常数mi*ed crystal 混晶mi*ed indicator 混合指示剂mobile phase 流动相Mohr method 莫尔法molar absorptivity 摩尔吸收系数mole ratio method 摩尔比法molecular spectrum 分子光谱monoacid 一元酸monochromatic color 单色光monochromator 单色器neutral solvent 中性溶剂neutralization 中和non-aqueous titration 非水滴定normal distribution 正态分布occlusion 包藏organic phase 有机相ossification of indicator 指示剂的僵化outlier 离群值oven 烘箱paper chromatography(PC) 纸色谱parallel determination 平行测定path lenth 光程permanganate titration 高锰酸钾法phase ratio 相比phenolphthalein (PP) 酚酞photocell 光电池photoelectric colorimeter 光电比色计photometric titration 光度滴定法photomultiplier 光电倍增管phototube 光电管pipette 移液管polar solvent 极性溶剂polyprotic acid 多元酸population 总体postprecipitation 后沉淀precipitant 沉淀剂precipitation form 沉淀形precipitation titration 沉淀滴定法precision 精细度preconcentration 预富集predominance-area diagram 优势区域图primary standard 基准物质prism 棱镜probability 概率proton 质子proton condition 质子条件protonation 质子化protonation constant 质子化常数purity 纯度qualitative analysis 定性分析quantitative analysis 定量分析quartering 四分法random error 随机误差range 全距(极差)reagent blank 试剂空白Reagent bottle 试剂瓶recording spectrophotometer 自动记录式分光光度计recovery 回收率redo* indicator 氧化复原指示剂redo* titration 氧化复原滴定referee analysis 仲裁分析reference level 参考水平reference material (RM) 标准物质reference solution 参比溶液relative error 相对误差resolution 分辨力rider 游码routine analysis 常规分析sample 样本,样品sampling 取样self indicator 自身指示剂semimicro analysis 半微量分析separation 别离separation factor 别离因数side reaction coefficient 副反响系数significance test 显著性检验significant figure 有效数字simultaneous determination of multiponents 多组分同时测定single beam spectrophotometer 单光束分光光度计single-pan balance 单盘天平slit 狭缝sodium diphenylamine sulfonate 二苯胺磺酸钠solubility product 溶度积solvent e*traction 溶剂萃取species 型体(物种)specific e*tinction coefficient 比消光系数spectral analysis 光谱分析spectrophotometer 分光光度计spectrophotometry 分光光度法stability constant 稳定常数standard curve 标准曲线standard deviation 标准偏差standard potential 标准电位standard series method 标准系列法standard solution 标准溶液standardization 标定starch 淀粉stationary phase 固定相steam bath 蒸气浴stepwise stability constant 逐级稳定常数stoichiometric point 化学计量点structure analysis 构造分析supersaturation 过饱和systematic error 系统误差test solution 试液thermodynamic constant 热力学常数thin layer chromatography (TLC) 薄层色谱titrand 被滴物titrant 滴定剂titration 滴定titration constant 滴定常数titration curve 滴定曲线titration error 滴定误差titration inde* 滴定指数-titration jump 滴定突跃titrimetry 滴定分析trace analysis 痕量分析transition interval 变色间隔transmittance 透射比tri acid 三元酸true value 真值tungsten lamp 钨灯ultratrace analysis 超痕量分析UV-VIS spectrophotometry 紫外-可见分光光度法volatilization 挥发Volhard method 福尔哈德法volumetric flask 容量瓶volumetry 容量分析Wash bottle 洗瓶washings 洗液water bath 水浴weighing bottle 称量瓶weighting form 称量形weights 砝码working curve 工作曲线*ylenol orange (*O) 二甲酚橙zero level 零水平异步处理dispatch_async(dispatch_get_ global_queue(0, 0), ^{// 处理耗时操作的代码块... [self test1];//通知主线程刷新dispatch_async(dispatch_get_ main_queue(), ^{//回调或者说是通知主线程刷新,NSLog(............); });。
分子印迹聚合物对血液中野百合碱-血红蛋白加合物的分析

广东药科大学学报Journal of Guangdong Pharmaceutical University Mar.2024,40(2)分子印迹聚合物对血液中野百合碱-血红蛋白加合物的分析葛燕辉1,2,郑远茹1,郭景灿1,江芷惠1[1.广东药科大学药学院,广东广州510006;2.广东药科大学附属第一医院(临床医学院),广东广州510080]摘要:目的基于分子印迹技术,建立快速检测体内野百合碱和血红蛋白加合物的方法。
方法以制备的野百合碱-血红蛋白加合物为模板分子,经表面印迹聚合法制备野百合碱-血红蛋白分子印迹聚合物,用作固相萃取介质识别造模大鼠血液中的血红蛋白加合物。
结果理化性质表征结果显示聚合层成功接枝在碳纳米管表面,且聚合物具备良好的热稳定性;将聚合物作为固相萃取介质,对造模大鼠血液进行分析,结果显示聚合物血红蛋白加合物具有较强的吸附能力(吸附量达90.86mg/mg),该结果与肝脏病理检测结果呈正相关性。
结论所制备的聚合物可以快速分析体内血红蛋白加合物的含量,与肝脏病理结果相结合,为肝小静脉闭塞症的无创伤诊断提供新的研究思路。
关键词:野百合碱;血红蛋白加合物;分子印迹聚合物;肝小静脉闭塞症;无创诊断中图分类号:R917文献标识码:A文章编号:2096-3653(2024)02-0076-07DOI:10.16809/ki.2096-3653.2024011802Analysis of Monocrotaline-hemoglobin adducts in blood using molecularly imprinted polymersGE Yanhui1,2*,ZHENG Yuanru1,GUO Jingcan1,JIANG Zhihui1(1.School of Pharmacy,Guangdong Pharmaceutical University,Guangzhou510006,China;2.The First Affiliated Hospital, Guangdong Pharmaceutical University,Guangzhou510080,China)*Corresponding author Email:*****************.cnAbstract:Objective To establish a rapid analytical method for in vivo binding of monocrotaline and hemoglobin based on molecular imprinting technology.Methods The prepared monocrotaline hemoglobin adduct (Mct@BHb)was used as a template molecule to prepare molecularly imprinted polymers(Mct@BHb@MIPs)by using surface imprinting polymerization method,and then the Mct@BHb@MIPs were used as an extraction medium to adsorp toxic markers in rat blood.Results The results showed that the polymer layer was successfully grafted onto the surface of MWCNs,and the polymer exhibited good thermal stability after characterization.The Mct@BHb@MIPs were used as a solid-phase extraction medium to analyze the blood of model rats,and the results showed that the polymer hemoglobin adducts had good adsorption capacity(adsorption amount reached90.86mg/mg).The analysis results were consistent with liver pathological detection.Conclusio n The polymerprepared by this research can quickly analyze the content of hemoglobin conjugates in vivo,which provides new research ideas for non-invasive diagnosis of hepatic small vein occlusion,combined with pathological results.Key words:monocrotaline;hemoglobin adduct;molecularly imprinted polymers;hepatic veno-occlusive disease;non-invasive diagnosis含有吡咯双烷类生物碱(pyrrolizidine alkaloids,PAs)的中药在治疗疾病过程中易引发肝小静脉闭塞病(hepatic veno-occlusive disease,HVOD)[1],而目前我国有100多种含PAs的中药在临床上使用(约占《中药大辞典》收载量的1%),使得中药潜在的肝毒性问题不得不引起重视[2-3]。
Hydrogen desorption and ammonia adsorption on polycrystalline

’
Abstract We have studied the D, desorption and NH 3 adsorption on polycrystalline GaN surfaces using time-of-flight detection of recoiled H’ and D+ ions. Two surface deuterium states characterized by different thermal stability are identified. Rate analysis for isothermal D, desorption is performed near 25O”C, which we attribute to desorption from Ga sites. We assign the higher temperature D, desorption state decomposing near 500°C to desorption from N sites. Both clean and D-terminated GaN surfaces are quite reactive towards NH, adsorption. We observed that H/D exchange during NH, exposure occurs rapidly at room temperature.
q = 25°C with atomic deuterium. D atoms were generated by flowing D, over a hot tungsten filament placed = 5 cm above the surface [7]. Without the hot filament, no D adsorbed on the surface, indicating that D, is not reactive with the GaN surface. A series of D+ and H+ recoiled ion spectra at various T, is displayed in the inset to Fig. 1. Each spectrum was signal averaged for 120 s. The H+ signals are attributed to the dissociation of residual H, in the vacuum chamber. Upon increasing the T,, spectrum (a) remains unchanged until = 250°C. At T, = 450°C lower coverage of surface D(H) is reached, as shown in spectrum (b). It is most likely that the recombinative desorption of D, (H,) from Ga sites is responsible for the decrease in D+ (H+) RI signals below 450°C. This assignment is based on bond energy (BE) arguments and on the literature concerning D, (H,) desorption from GaAs. Hydrogen is much more weakly bonded to Ga than to N [8] versus BE,_, = 92 (BE,,., = 66 kcal/mol kcal/mol [9]). On GaAs single crystal surfaces, recombinative desorption of hydrogen and deuterium bonded to surface gallium was previously reported to occur at 207-237°C by monitoring either the disappearance of the GaH species with high resolution electron energy loss spectroscopy (HREELS) [lo131, as well as infrared spectroscopy [ 14-161, or D, evolution in temperature programmed desorption (TPD) [17,18]. Hydrogen desorption from surfaces is typically classified as being either first or second order in surface H coverage (0,) [19]. By measuring 8, as a function of time (t), the desorption order can be determined. If the desorption is first order, a plot of [ - ln(&,/ 8:)] versus t is linear, while if the desorption is second order, a plot of [(et/f&) - 11 versus f is linear. 8, can be directly measured by RI signals as a function of time for isothermal D, desorption from Ga sites at T, = 250°C. In Fig. 2, the values of 8, are plotted according to a first-order rate law in the top panel and a second-order rate law in the bottom panel. Clearly, a good linear fit is reached in the second-order plot. Second-order desorption can be explained using a two-step reaction model.
从水中去除重金属离子和染料的农业固体废物吸附剂综述说明书
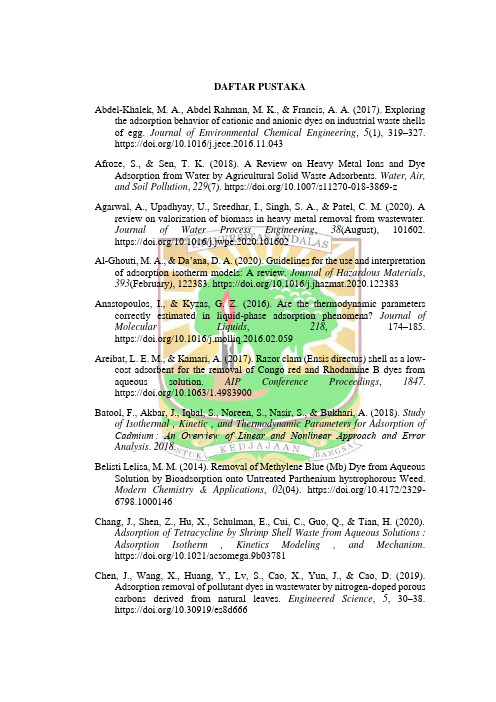
DAFTAR PUSTAKAAbdel-Khalek, M. A., Abdel Rahman, M. K., & Francis, A. A. (2017). Exploring the adsorption behavior of cationic and anionic dyes on industrial waste shells of egg. Journal of Environmental Chemical Engineering, 5(1), 319–327.https:///10.1016/j.jece.2016.11.043Afroze, S., & Sen, T. K. (2018). A Review on Heavy Metal Ions and Dye Adsorption from Water by Agricultural Solid Waste Adsorbents. Water, Air, and Soil Pollution, 229(7). https:///10.1007/s11270-018-3869-z Agarwal, A., Upadhyay, U., Sreedhar, I., Singh, S. A., & Patel, C. M. (2020). A review on valorization of biomass in heavy metal removal from wastewater.Journal of Water Process Engineering, 38(August), 101602.https:///10.1016/j.jwpe.2020.101602Al-Ghouti, M. A., & Da’ana, D. A. (2020). Guidelines for the use and interpretation of adsorption isotherm models: A review. Journal of Hazardous Materials, 393(February), 122383. https:///10.1016/j.jhazmat.2020.122383 Anastopoulos, I., & Kyzas, G. Z. (2016). Are the thermodynamic parameters correctly estimated in liquid-phase adsorption phenomena? Journal of Molecular Liquids, 218, 174–185.https:///10.1016/j.molliq.2016.02.059Areibat, L. E. M., & Kamari, A. (2017). Razor clam (Ensis directus) shell as a low-cost adsorbent for the removal of Congo red and Rhodamine B dyes from aqueous solution. AIP Conference Proceedings, 1847.https:///10.1063/1.4983900Batool, F., Akbar, J., Iqbal, S., Noreen, S., Nasir, S., & Bukhari, A. (2018). Study of Isothermal , Kinetic , and Thermodynamic Parameters for Adsorption of Cadmium : An Overview of Linear and Nonlinear Approach and Error Analysis. 2018.Belisti Lelisa, M. M. (2014). Removal of Methylene Blue (Mb) Dye from Aqueous Solution by Bioadsorption onto Untreated Parthenium hystrophorous Weed.Modern Chemistry & Applications, 02(04). https:///10.4172/2329-6798.1000146Chang, J., Shen, Z., Hu, X., Schulman, E., Cui, C., Guo, Q., & Tian, H. (2020).Adsorption of Tetracycline by Shrimp Shell Waste from Aqueous Solutions : Adsorption Isotherm , Kinetics Modeling , and Mechanism.https:///10.1021/acsomega.9b03781Chen, J., Wang, X., Huang, Y., Lv, S., Cao, X., Yun, J., & Cao, D. (2019).Adsorption removal of pollutant dyes in wastewater by nitrogen-doped porous carbons derived from natural leaves. Engineered Science, 5, 30–38.https:///10.30919/es8d666Dai, L., Zhu, W., He, L., Tan, F., Zhu, N., Zhou, Q., He, M., & Hu, G. (2018).Calcium-rich biochar from crab shell: An unexpected super adsorbent for dye removal. Bioresource Technology, 267(June), 510–516.https:///10.1016/j.biortech.2018.07.090d e Rezende, M. L. R., Coesta, P. T. G., de Oliveira, R. C., Salmeron, S., Sant’Ana,A. C. P., Damante, C. A., Greghi, S. L. A., & Consolaro, A. (2015). BoneDemineralization With Citric Acid Enhances Adhesion and Spreading of Preosteoblasts. Journal of Periodontology, 86(1), 146–154.https:///10.1902/jop.2014.130657Derakhshan, Z., Baghapour, M. A., Ranjbar, M., & Faramarzian, M. (2013).Adsorption of Methylene Blue Dye from Aqueous Solutions by Modified Pumice Stone: Kinetics and Equilibrium Studies. Health Scope, 2(3), 136–144. https:///10.17795/jhealthscope-12492Djelloul, C., & Hamdaoui, O. (2014). Removal of cationic dye from aqueous solution using melon peel as nonconventional low-cost sorbent. Desalination and Water Treatment, 52(40–42), 7701–7710.https:///10.1080/19443994.2013.833555Eletta, O. A. A., Adeniyi, A. G., Ighalo, J. O., Onifade, D. V., & Ayandele, F. O.(2020). Valorisation of Cocoa (Theobroma cacao) pod husk as precursors for the production of adsorbents for water treatment. Environmental Technology Reviews, 9(1), 20–36. https:///10.1080/21622515.2020.1730983Eljiedi, A. A. A., & Kamari, A. (2017). Removal of methyl orange and methylene blue dyes from aqueous solution using lala clam (Orbicularia orbiculata) shell.AIP Conference Proceedings, 1847. https:///10.1063/1.4983899Elwakeel, K. Z., Elgarahy, A. M., & Mohammad, S. H. (2017). Use of beach bivalve shells located at Port Said coast (Egypt) as a green approach for methylene blue removal. Journal of Environmental Chemical Engineering, 5(1), 578–587. https:///10.1016/j.jece.2016.12.032Faisal, A. A. H., Khalid, Z., Al-ansari, N., & Sharma, G. (2021). Chemosphere Precipitation of ( Mg / Fe-CTAB ) - Layered double hydroxide nanoparticles onto sewage sludge for producing novel sorbent to remove Congo red and methylene blue dyes from aqueous environment. Chemosphere, September, 132693. https:///10.1016/j.chemosphere.2021.132693Fajarwati, F. I., Ika Yandini, N., Anugrahwati, M., & Setyawati, A. (2020).Adsorption Study of Methylene Blue and Methyl Orange Using Green Shell (Perna Viridis). EKSAKTA: Journal of Sciences and Data Analysis, 1(1), 92–97. https:///10.20885/eksakta.vol1.iss1.art14Fosso-Kankeu, E., Webster, A., Ntwampe, I. O., & Waanders, F. B. (2017).Coagulation/Flocculation Potential of Polyaluminium Chloride and Bentonite Clay Tested in the Removal of Methyl Red and Crystal Violet. Arabian Journal for Science and Engineering, 42(4), 1389–1397.https:///10.1007/s13369-016-2244-xGhaedi, M., Hajjati, S., Mahmudi, Z., Tyagi, I., Agarwal, S., Maity, A., & Gupta, V. K. (2015). Modeling of competitive ultrasonic assisted removal of the dyes - Methylene blue and Safranin-O using Fe3O4 nanoparticles. Chemical Engineering Journal, 268, 28–37. https:///10.1016/j.cej.2014.12.090 Hevira, L., Rahmi, A., Zein, R., Zilfa, Z., & Rahmayeni, R. (2020). The fast and of low-cost-adsorbent to the removal of cationic and anionic dye using chicken eggshell with its membrane. Mediterranean Journal of Chemistry, 10(3), 294–301. https:///10.13171/mjc020********lhHevira, L., Zilfa, Rahmayeni, Ighalo, J. O., Aziz, H., & Zein, R. (2021). Terminalia catappa shell as low-cost biosorbent for the removal of methylene blue from aqueous solutions. Journal of Industrial and Engineering Chemistry, 97, 188–199. https:///10.1016/j.jiec.2021.01.028Hevira, L., Zilfa, Rahmayeni, Ighalo, J. O., & Zein, R. (2020). Biosorption of indigo carmine from aqueous solution by Terminalia Catappa shell. Journal of Environmental Chemical Engineering, 8(5), 104290.https:///10.1016/j.jece.2020.104290Hubbe, M. A., Azizian, S., & Douven, S. (2019). Implications of apparent pseudo-second-order adsorption kinetics onto cellulosic materials: A review.BioResources, 14(3), 7582–7626. https:///10.15376/biores.14.3.7582-7626Ighalo, J. O., & Adeniyi, A. G. (2020). A mini-review of the morphological properties of biosorbents derived from plant leaves. SN Applied Sciences, 2(3).https:///10.1007/s42452-020-2335-xIgwegbe, C. A., Mohmmadi, L., Ahmadi, S., Rahdar, A., Khadkhodaiy, D., Dehghani, R., & Rahdar, S. (2019). Modeling of adsorption of Methylene Blue dye on Ho-CaWO4 nanoparticles using Response Surface Methodology (RSM) and Artificial Neural Network (ANN) techniques. MethodsX, 6, 1779–1797. https:///10.1016/j.mex.2019.07.016Jafari, M., Vanoppen, M., van Agtmaal, J. M. C., Cornelissen, E. R., Vrouwenvelder, J. S., Verliefde, A., van Loosdrecht, M. C. M., & Picioreanu,C. (2021). Cost of fouling in full-scale reverse osmosis and nanofiltrationinstallations in the Netherlands. Desalination, 500(December 2020), 114865.https:///10.1016/j.desal.2020.114865Jawad, A. H., Rashid, R. A., Ishak, M. A. M., & Ismail, K. (2018). Adsorptive removal of methylene blue by chemically treated cellulosic waste banana ( Musa sapientum ) peels . Journal of Taibah University for Science, 12(6), 809–819. https:///10.1080/16583655.2018.1519893Jawad, A. H., Rashid, R. A., Ishak, M. A. M., & Wilson, L. D. (2016). Adsorption of methylene blue onto activated carbon developed from biomass waste by H2SO4 activation: kinetic, equilibrium and thermodynamic studies.Desalination and Water Treatment, 57(52), 25194–25206.https:///10.1080/19443994.2016.1144534Katneni, V. K., Shekhar, M. S., Jangam, A. K., Prabhudas, S. K., Krishnan, K., Kaikkolante, N., Paran, B. C., Baghel, D. S., Koyadan, V. K., Jena, J., & Mohapatra, T. (2020). Novel Isoform Sequencing Based Full-Length Transcriptome Resource for Indian White Shrimp, Penaeus indicus. Frontiers in Marine Science, 7(December), 1–4.https:///10.3389/fmars.2020.605098Kusumawardani, R., Rismawati, A., Retnowati, R., So, H., & Variabel, C. (2018).MODIFIKASI BIJI PEPAYA SEBAGAI BIOSORBEN ZAT WARNA TEKSTIL MORDANT BLACK 11 MODIFICATION OF PAPAYA SEEDS AS BIOSORBENT COLORS OF MORDANT BLACK 11 TEXTILES biosorben biji pepaya untuk mengadsorpsi zat warna tekstil mordant black 11 . Prinsip kerja dari mor. 1(2), 92–95.Li, W. (2011). Quantitative Analysis of the Reaction between Gliadin and Citric Acid under Weak Acidic and Weak Alkaline Conditions. Thesis.Liu, L., Fan, S., & Li, Y. (2018). Removal behavior of methylene blue from aqueous solution by tea waste: Kinetics, isotherms and mechanism. International Journal of Environmental Research and Public Health, 15(7).https:///10.3390/ijerph15071321Makeswari, M., Santhi, T., & Ezhilarasi, M. R. (2016). Adsorption of methylene blue dye by citric acid modified leaves of Ricinus communis from aqueous solutions. 8(7), 452–462.Mohan, C. (2003). A guide for the preparation and use of buffers in biological systems.Naghizadeh, A., & Ghafouri, M. (2017). Synthesis and performance evaluation of chitosan prepared from Persian gulf shrimp shell in removal of reactive blue29 dye from aqueous solution (Isotherm, thermodynamic and kinetic study).Iranian Journal of Chemistry and Chemical Engineering, 36(3), 25–36. Pang, Y. L., Tan, J. H., Lim, S., & Chong, W. C. (2021). A state-of-the-art review on biowaste derived chitosan biomaterials for biosorption of organic dyes: Parameter studies, kinetics, isotherms and thermodynamics. Polymers, 13(17).https:///10.3390/polym13173009Pathak, P. D., Mandavgane, S. A., & Kulkarni, B. D. (2016). Characterizing fruit and vegetable peels as bioadsorbents. Current Science, 110(11), 2114–2123.https:///10.18520/cs/v110/i11/2114-2123Ponnusami, V., Vikram, S., & Srivastava, S. N. (2008). Guava (Psidium guajava) leaf powder: Novel adsorbent for removal of methylene blue from aqueous solutions. Journal of Hazardous Materials, 152(1), 276–286.https:///10.1016/j.jhazmat.2007.06.107Pratiwi, R. (2018). ASPEK BIOLOGI DAN ABLASI MATA PADA UDANG WINDU Penaeus monodon SUKU PENAEIDAE (DECAPODA: MALACOSTRACA). Oseana, 43(2), 34–47.https:///10.14203/oseana.2018.vol.43no.2.19Purnomo, J. S. (2022). PENINGKATAN KAPASITAS ADSORPSI METHYLENE BLUE SECARA BATCH MENGGUNAKAN AMPAS DAUN SERAI WANGI (Cymbopogon nardus L. Rendle) YANG DIMODIFIKASI DENGAN ASAM SITRAT. In Universitas Andalas (Issue8.5.2017).Qin, L., Zhou, Z., Dai, J., Ma, P., Zhao, H., He, J., Xie, A., Li, C., & Yan, Y. (2016).Novel N-doped hierarchically porous carbons derived from sustainable shrimp shell for high-performance removal of sulfamethazine and chloramphenicol.Journal of the Taiwan Institute of Chemical Engineers, 62, 228–238.https:///10.1016/j.jtice.2016.02.009Rafatullah, M., Sulaiman, O., Hashim, R., & Ahmad, A. (2010). Adsorption of methylene blue on low-cost adsorbents: A review. Journal of Hazardous Materials, 177(1–3), 70–80. https:///10.1016/j.jhazmat.2009.12.047 Rahmayeni, R., Oktavia, Y., Stiadi, Y., Arief, S., & Zulhadjri, Z. (2021). Spinel ferrite of MnFe2O4 synthesized in Piper betle Linn extract media and its application as photocatalysts and antibacterial. Journal of Dispersion Science and Technology, 42(3), 465–474.https:///10.1080/01932691.2020.1721011Rajumon, R., Anand, J. C., Ealias, A. M., Desai, D. S., George, G., & Saravanakumar, M. P. (2019). Adsorption of textile dyes with ultrasonic assistance using green reduced graphene oxide: An in-depth investigation on sonochemical factors. Journal of Environmental Chemical Engineering, 7(6), 103479. https:///10.1016/j.jece.2019.103479Ramadhani, P., Chaidir, Z., Zilfa, Tomi, Z. B., Rahmiarti, D., & Zein, R. (2020).Shrimp shell (Metapenaeus monoceros) waste as a low-cost adsorbent for metanil yellow dye removal in aqueous solution. Desalination and Water Treatment, 197, 413–423. https:///10.5004/dwt.2020.25963 Ramrakhiani, L., Ghosh, S., & Majumdar, S. (2016). Surface Modification of Naturally Available Biomass for Enhancement of Heavy Metal Removal Efficiency, Upscaling Prospects, and Management Aspects of Spent Biosorbents: A Review. Applied Biochemistry and Biotechnology, 180(1), 41–78. https:///10.1007/s12010-016-2083-yRápó, E., & Tonk, S. (2021). Factors affecting synthetic dye adsorption; desorption studies: A review of results from the last five years (2017–2021). Molecules, 26(17). https:///10.3390/molecules26175419Ravi, & Pandey, L. M. (2019). Enhanced adsorption capacity of designed bentonite and alginate beads for the effective removal of methylene blue. Applied Clay Science, 169(October 2018), 102–111.https:///10.1016/j.clay.2018.12.019Ribeiro, C., Scheufele, F. B., Espinoza-Quiñones, F. R., Módenes, A. N., da Silva,M. G. C., Vieira, M. G. A., & Borba, C. E. (2015). Characterization of Oreochromis niloticus fish scales and assessment of their potential on the adsorption of reactive blue 5G dye. Colloids and Surfaces A: Physicochemical and Engineering Aspects, 482, 693–701.https:///10.1016/j.colsurfa.2015.05.057Sadaf, S., & Bhatti, H. N. (2014). Batch and fixed bed column studies for the removal of Indosol Yellow BG dye by peanut husk. Journal of the Taiwan Institute of Chemical Engineers, 45(2), 541–553.https:///10.1016/j.jtice.2013.05.004Safa, Y., & Bhatti, H. N. (2011). Adsorptive removal of direct dyes by low cost rice husk : Effect of treatments and modifications. 10(16), 3128–3142.https:///10.5897/AJB10.1302Sandip, Q. (2015). Journal of Industrial and Engineering Chemistry Efficient adsorption and photocatalytic degradation of Rhodamine B dye over Bi 2 O 3 -bentonite nanocomposites : A kinetic study. 1–8.Sawasdee, S., & Watcharabundit, P. (2016). Effect of temperature on brilliant green adsorption by shrimp shell: Equilibrium and kinetics. Chiang Mai University Journal of Natural Sciences, 15(3), 221–236.https:///10.12982/cmujns.2016.0017Senthil Kumar, P., Janet Joshiba, G., Femina, C. C., Varshini, P., Priyadharshini, S., Arun Karthick, M. S., & Jothirani, R. (2019). A critical review on recent developments in the low-cost adsorption of dyes from wastewater.Desalination and Water Treatment, 172, 395–416.https:///10.5004/dwt.2019.24613Shakoor, S., & Nasar, A. (2016). Removal of methylene blue dye from artificially contaminated water using citrus limetta peel waste as a very low cost adsorbent. Journal of the Taiwan Institute of Chemical Engineers, 66, 154–163. https:///10.1016/j.jtice.2016.06.009Sun, C., Qiu, J., Zhang, Z., Marhaba, T. F., Zhang, Y., & Zhang, W. (2016).Characterization of Citric Acid-Modified Clam Shells and Application for Aqueous Lead (II) Removal. Water, Air, and Soil Pollution, 227(9).https:///10.1007/s11270-016-2975-zSuryawanshi, N., Jujjavarapu, S. E., & Ayothiraman, S. (2019). Marine shell industrial wastes–an abundant source of chitin and its derivatives: constituents, pretreatment, fermentation, and pleiotropic applications-a revisit.International Journal of Environmental Science and Technology, 16(7), 3877–3898. https:///10.1007/s13762-018-02204-3Taylor, P., Wang, P., Ma, Q., Hu, D., & Wang, L. (2015). Desalination and Water Treatment Adsorption of methylene blue by a low-cost biosorbent : citric acid modified peanut shell. May, 37–41.https:///10.1080/19443994.2015.1033651Taylor, P., Zhou, Y., Zhang, R., Gu, X., Lu, J., Zhou, Y., Zhang, R., Gu, X., & Lu, J. (2015). Separation Science and Technology Adsorption of Divalent Heavy Metal Ions from Aqueous Solution by Citric Acid Modified Pine Sawdust Adsorption of Divalent Heavy Metal Ions from Aqueous Solution by Citric Acid Modified Pine Sawdust. January, 37–41.https:///10.1080/01496395.2014.956223Thamaraiselvan, C., Lerman, S., Weinfeld-Cohen, K., & Dosoretz, C. G. (2018).Characterization of a support-free carbon nanotube-microporous membrane for water and wastewater filtration. Separation and Purification Technology, 202(March), 1–8. https:///10.1016/j.seppur.2018.03.038Xu, Y., Liu, Y., Liu, S., Tan, X., Zeng, G., Zeng, W., Ding, Y., Cao, W., & Zheng,B. (2016). Enhanced adsorption of methylene blue by citric acid modificationof biochar derived from water hyacinth (Eichornia crassipes). Environmental Science and Pollution Research, 23(23), 23606–23618.https:///10.1007/s11356-016-7572-6Zein, R. (2019). Pensi shell (Corbicula moltkiana)as a biosorbent for metanil yellow dyes removal: pH and equilibrium model evaluation. Jurnal Litbang Industri, 15–22.Zein, R., Chaidir, Z., Fauzia, S., & Ramadhani, P. (2022). Isotherm and Kinetic Studies on the Adsorption Behavior of Metanil Yellow Dyes onto Modified Shrimp Shell-Polyethylenimine ( SS-PEI ). 8(May), 10–22.https:///10.15408/jkv.v8i1.22566Zein, R., Purnomo, J. S., Ramadhani, P., Alif, M. F., & Safni, S. (2022).Lemongrass ( Cymbopogon nardus ) leaves biowaste as an effective and low-cost adsorbent for methylene blue dyes removal: isotherms, kinetics, and thermodynamics studies . Separation Science and Technology, 00(00), 1–17.https:///10.1080/01496395.2022.2058549Zein, R., Tomi, Z. B., Fauzia, S., & Zilfa, Z. (2020). Modification of rice husk silica with bovine serum albumin (BSA) for improvement in adsorption of metanil yellow dye. Journal of the Iranian Chemical Society, 17(10), 2599–2612.https:///10.1007/s13738-020-01955-6Zhou, Y., Ge, L., Fan, N., & Xia, M. (2018). Adsorption of Congo red from aqueous solution onto shrimp shell powder. Adsorption Science and Technology, 36(5–6), 1310–1330. https:///10.1177/0263617418768945。
吸附氢分子的振动态及熵的计算

吸附氢分子的振动态及熵的计算王小霞;刘鑫;张琼;陈宏善【摘要】The entropy and enthalpy changes upon absorption determine the equilibrium adsorption states,the adsorption/desorption kinetics,and the surface reaction rates.However,it is difficult to measure experimentally or calculate theoretically the entropy of adsorption state.Hydrogen is considered as the most promising candidate to solve the global energy problems,and the storage by adsorption on light porous solids constitutes a main avenue to research field.An ideal storage system should be able to operate under ambient conditions with high recycling capacity and suitable uptake-release kinetics.The entropy of adsorbed H2 molecules is of great significance for determining the optimum conditions for hydrogen storage and for designing the storage materials.To the best of our knowledge,however,the only report on the entropy of the adsorbed H2 molecules is that adsorbed on alkali-metal exchanged zeolites at temperatures around 100 K.Due to different assumptions of the entropy changes,the values of the optimum enthalpy AH reported in the publications cover a wide range.In this paper,the adsorptionstates,vibrational modes,and the entropies of H2 molecules adsorbed on (MgO)9 and (AlN)12 clusters are studied by using first principal method.The computation is performed by the second-order perturbation theory (MP2) with the triple zeta basis set including polarization functions 6-311G(d,p).The very-tight convergence criterion is used to obtain reliablevibration frequencies.Analysis shows that six vibrational modes of the adsorption complexes can be attributed to the vibration of H2 molecule.For these normal modes,the amplitudes of the displacements of cluster atoms are usually two orders smaller than those of the hydrogen atoms.As the vibrational frequency is inversely proportional to the square root of the mass,the zero-point energy has an important influence on the adsorption energy.The ZPE correction exceeds half of the adsorption energy,and the adsorption on the anions is not stable after including the correction.Under the harmonic approximation,the normal vibration modes are independent,so the entropy of adsorbed H2 molecules can be calculated by using the vibrational partition function based on the vibrational frequencies.The results indicate that the entropy values depend mainly on the two lowest in-phase vibrational frequencies and it is not directly related to the adsorption strength but determined by the shape of the potential energy surface.In a temperature range of 70-350 K and at a pressure of 0.1 MPa,there is a good linear correlation between the entropy of adsorbed H2 and the entropy of gas-phase.The entropy of H2 decreases about 10.2R after adsorption.%用第一性原理方法研究了H2在(MgO)9及(AlN)12团簇上的吸附态、振动模式及熵.分析表明,吸附体系的振动中有六个简正模式可归为氢分子的振动;由于氢分子质量很小,零点能修正对吸附能有重要影响.利用振动配分函数计算了吸附氢分子的熵,表明吸附态H2的熵主要决定于较低的同相振动的频率,并不完全与吸附强度相关;在标准大气压下70-350 K的温度范围内,吸附H2的熵与气态H2的熵之间存在很好的线性关系,吸附后H2的熵减小约10.2R.【期刊名称】《物理学报》【年(卷),期】2017(066)010【总页数】7页(P85-91)【关键词】团簇;H2吸附;振动态;吸附H2的熵【作者】王小霞;刘鑫;张琼;陈宏善【作者单位】西北师范大学物理与电子工程学院,甘肃省原子分子物理与功能材料重点实验室,兰州 730070;西北师范大学物理与电子工程学院,甘肃省原子分子物理与功能材料重点实验室,兰州 730070;西北师范大学物理与电子工程学院,甘肃省原子分子物理与功能材料重点实验室,兰州 730070;西北师范大学物理与电子工程学院,甘肃省原子分子物理与功能材料重点实验室,兰州 730070【正文语种】中文用第一性原理方法研究了H2在(MgO)9及(AlN)12团簇上的吸附态、振动模式及熵.分析表明,吸附体系的振动中有六个简正模式可归为氢分子的振动;由于氢分子质量很小,零点能修正对吸附能有重要影响.利用振动配分函数计算了吸附氢分子的熵,表明吸附态H2的熵主要决定于较低的同相振动的频率,并不完全与吸附强度相关;在标准大气压下70—350 K的温度范围内,吸附H2的熵与气态H2的熵之间存在很好的线性关系,吸附后H2的熵减小约10.2R.PACS∶36.40.—c,31.15.A—,68.43.Bc DOI∶10.7498/aps.66.103601气体或液体分子在固体表面的吸附涉及广泛的应用领域,在吸附态下分子的平动被限制,而分子具有振动或转动运动.吸附过程的焓变和熵变决定了吸附的平衡状态、吸脱附的动力学、表面反应的反应速率常数等重要物理量,但对吸附态的熵理论计算和实验测量都比较困难[1,2].氢气作为潜在的理想能源,吸附态的熵对于确定最佳的存储条件以及存储材料的选择都十分重要[3,4].Yang等[5]基于van’t Hof f方程分析了吸附熵变对于存储条件的影响,Tang等[6]测量了MSiH3(M=K,Rb,Cs)体系脱附过程的熵变并讨论了熵和焓的补偿作用.氢分子的物理吸附能很好地用朗缪尔模型描述,吸附平衡常数K=exp(∆S0/R−∆H/RT),∆S0为标准大气压下的吸附熵变[7];而对于氢分子物理吸附态的熵,仅有100 K左右H2吸附在分子筛中的实验研究报道[8].在有关讨论固体储氢的文献中,由于对吸附态的熵假定不同,给出的最佳储存条件差别很大.例如Bhatia和Myer[7]假定吸附的熵变为8R,给出室温下储氢的理想吸附能为15.1 kJ/mol;而Li等[9]和Garberoglio等[10]假定不同的熵变并给出了完全不同的理想吸附能.最近Campbell和Sellers[1]测量了多种气体吸附态的熵,但并未包含H2的吸附.本文利用第一性原理方法,研究了H2在(MgO)9及(AlN)12团簇上的吸附,分析了吸附H2的振动态并计算了吸附态H2的熵.氢分子的吸附结构优化及振动频率计算釆用二阶微扰理论(MP2)[11],基函数选取了包括极化函数的三ξ基组6-311 G(d,p)[12];为了得到可靠的振动频率,结构优化采用了verytight的收敛条件(原子上的最大力为0.000002 a.u.,最大位移0.000006 a.u.).计算用Gaussian09程序[13]完成.对吸附体系的振动进行分析,得到吸附H2的配分函数,利用熵与配分函数的关系[14]计算H2吸附态的熵,式中N为阿伏伽德罗常数,k为玻尔兹曼常数,β=1/(kT).3.1 吸附态H2的振动模式氢分子很小不易极化,吸附一般较弱.MgO和AlN都是典型的离子团簇,能够对H2形成较强的吸附[15,16].对于MgO团簇,我们选取了稳定的岩盐和管状(MgO)9[17,18],而对AlN团簇我们选择了笼状的(AlN)12幻数结构[19,20].对H2吸附结构的优化表明,稳定的吸附方式是H2吸附在单个原子上,其中H2在阳离子上以侧位方式吸附,在阴离子上以端位方式吸附.笼状(AlN)12中所有Al或N原子都等价,对于H2在 (MgO)9上的吸附,我们选择了在岩盐结构四配位Mg/O原子(Mg4c,O4c)和管状结构三配位Mg/O原子(Mg3c,O3c)上的吸附.吸附态的熵决定于氢分子的振动频率,而振动频率依赖于吸附强度,因此我们选取具有不同吸附强度的结构以便于考察吸附态的熵与吸附强度的关系.图1给出了六种吸附结构,也给出了各吸附结构的吸附能∶其中Ecluster,EH2为团簇与自由H2的能量,Ecluster@H2为吸附体系的能量. 对于一个N粒子体系,将其势能在平衡位置做级数展开,引入质量权重的位移坐标qi=则在简谐近似下忽略三次及以上的高次项,因选取平衡位置处Ue=0,则(3)式中仅剩二次项,是正定的实对称矩阵,可用正交矩阵L=[lij]将其对角化.对角化后有Nf(线性分子为3N−5,非线性分子为3N−6)个非负本征值ki,对应的本征矢为在上述变换下,体系振动的哈密顿量分离变量求解则得到一系列独立的简谐振动.在每个振动模式中所有原子都以相同的频率、相位振动,(5)式给出的Qi称为简正坐标.H2吸附在团簇上后,吸附体系比原团簇多出六个振动自由度,通过GaussView对振动模式逐一甄别,发现有六个简正振动可归为氢分子,图2为H2吸附在岩盐(MgO)9上的简正振动模式.对图1各吸附结构中H2的简正振动模式对应的简正坐标进行仔细核查,发现团簇原子的振幅比氢分子的振幅一般小两个数量级.在H2的六个振动模式中,沿着H2到吸附位方向的同相振动用FTz表示,与之垂直的同相振动中,频率较低的用FTx表示、频率较高的用FTy表示;H2以端位方式吸附在阴离子上时,与FTz相对应的异相振动即为H2内的伸缩振动ωH-H,而以侧位方式吸附在阳离子上时,ωH-H与FTy对应;另外两个异相振动相当于H2的转动,分别用F Rθ和FRϕ表示.表1列出了图1各吸附态H2的振动频率.除Al原子上的FTy外,其他FTx与FTy 的振动频率都在100 cm−1以下,表明势能面的底部较平.FTz的振动频率和吸附强度相关,H2吸附在阳离子上时FTz约为300 cm−1,在阴离子上时为100 cm−1多.H2吸附在阳离上时FRθ和FRϕ对应的频率差别较大,而在阴离子上时两个频率比较接近.用相同算法得到的自由氢分子的振动频率ωH-H为4533.23 cm−1,由于吸附后H2内的结合减弱,从表1中可看出ωH-H约降低100 cm−1.3.2 零点能对H2吸附能的影响(4)式给出的力常数与折合质量成反比,对应的振动频率与质量的平方根成反比;对于H2的六个简正模式,折合质量近似为H2的质量,所以其振动频率较高,零点能修正对吸附能有较大的影响.因频率计算比较费时,在许多研究储氢的文献中并没有考虑零点能的修正[21−26].表2列出了(2)式定义的吸附能∆E、零点能修正∆ZPE以及考虑零点能修正以后的吸附能Eb.对于零点能修正表2中列出了用两种方法计算的值,第一种是其中ZPEcluster,ZPEH2为团簇与自由H2的零点能,ZPEcluster@H2为吸附体系的零点能.第二种是利用表1的频率直接求得,鉴于吸附后H2内的振动频率明显降低,考虑了伸缩振动频率的变化ħ∆ωH-H/2∶从表2可以看出两种方法计算的零点能非常一致,再次验证了我们甄选氢分子简正模式的正确性.表2数据表明,在阳离子Mg3c,Al和Mg4c上零点能修正达到了∆E 的64%,88%和97%,因此修正后吸附能大幅减小;而在阴离子上零点能修正都已超过了∆E,因此在阴离子上的吸附不稳定.3.3 氢分子吸附态的熵在简谐近似下,N粒子体系的振动近似为Nf=3N−6个独立的简谐振动的迭加,其振动配分函数为其中θvj=ħωj/k为振动特征温度.由(1)式得到振动熵它们为各简正模式的熵的和.由于H2的振动和其他简正模式独立,根据表1中的振动频率便可得到吸附态H2的熵值Sads.Gaussian程序在进行频率计算时直接给出了体系在标准状态下的振动熵值,由于气态H2的振动频率很高,常温下处在振动基态对熵无贡献,将吸附体系与团簇的振动熵值相减也给出H2吸附态的熵.表3给出了利用(10)式计算的标准态下H2吸附态的熵Sads与∆S,两者也非常一致.图3为不同温度下吸附态H2的熵,温度较低时熵值增加较快,超过150 K时Sads 随温度近似做线性变化.(10)式表明振动熵对低频比较敏感,H2吸附态的熵值主要由两个同相的振动频率FTx和FTy决定;FTz与吸附强度相关,而FTx和FTy主要由势能面底部形状决定,因此吸附态的熵并不完全与吸附强度相关.如在Mg3c上的吸附最强,但在Al上吸附的熵值最小.为了研究H2吸附前后的熵变,我们进一步考察了H2吸附态的熵和气态熵Sgas的关系.气态H2的振动对熵无贡献,平动的熵容易由下式算出∶双原子分子的转动配分函数为其中θr=ħ2/(2Ik)(I为转动惯量).由于H2是同核双原子分子,仲氢(核自旋波函数为交换反对称的单重态)只能处在l为偶数的转动能级上,而正氢(核自旋波函数为交换对称的三重态)只能处在l为奇数的转动能级上.利用熵与配分函数的关系可得到仲氢和正氢的转动熵计算分别取了前四项,当温度低于350 K时上述两式能保证计算的精度高于10−6.由于自旋禁阻效应,常温下制备的H2当温度降低时仲氢和正氢并不相互转变而保持1∶3的比例,对仲氢和正氢的熵加权平均可得到气态H2的转动熵S(R).图4为仲氢、正氢、气态H2的转动熵以及气态H2的总熵S(T+R)(平动+转动)随温度的变化,当T高于150 K时转动熵及总的熵都随温度近似做线性变化.图5给出了标准大气压下温度从70 K到350 K时吸附态与气态H2熵的关系,可以看出二者具有较好的线性关系,对数据做线性拟合对于H2在Mg3c,Mg4c和Al上的吸附,a分别为0.98,1.05和0.95;S0分别为−81.6,−85.58和−85.95.即斜率接近1,而吸附后H2的熵减小约10.2R.因为H2的质量小,平动、转动及振动熵与温度的关系与较重的分子都有明显的差别,因此拟合结果相较Campbell给出的斜率0.7和截距−3.3R不同[1].但这一结果却偶然地与特鲁顿规则非常接近,即在沸点(饱和蒸气压为1 bar)时非极性液体的吸附熵与气相熵近似为线性关系,斜率为1时截距为−10.3R.选取了H2在岩盐和管状(MgO)9及笼状(AlN)12上的吸附,计算了振动频率,分析了吸附H2的振动,计算了吸附的熵变.对吸附体系振动模式的分析表明,氢分子的振动可以从其他简正模式中独立出来,属于H2的六个简正模式中团簇原子的振幅小两个数量级.由于振动频率与质量的平方根成反比,对氢分子的吸附,零点能修正超过了吸附能的一半,修正后阴离子上的吸附能为正.鉴于氢分子的振动与其他简正模式独立,可以利用氢分子的振动频率计算吸附H2的熵,因较低的两个同相振动频率主要由势能面底部形状决定,吸附态的熵并不完全与吸附强度相关.在标准大气压下温度从70 K到350 K变化时,H2吸附态的熵与气态熵之间存在很好的线性相关,吸附后H2的熵减小约10.2R.感谢国家超级计算机中心在广州和深圳提供的计算资源.The entropy and enthalpy changes upon absorption determine the equilibrium adsorption states,the adsorption/desorption kinetics,and the surface reaction rates.However,it is difficult to measure experimentally or calculate theoretically the entropy of adsorption state.Hydrogen is considered as the most promising candidate to solve the global energyproblems,and the storage by adsorption on light porous solids constitutes a main avenue to research field.An ideal storage system should be able to operate under ambient conditions with high recycling capacity and suitable uptake-release kinetics.The entropy of adsorbed H2molecules is of great significance for determining the optimum conditions for hydrogen storage and for designing the storage materials.To the best of our knowledge,however,the only report on the entropy of the adsorbedH2molecules is that adsorbed on alkali-metal exchanged zeolites at temperatures around 100 K.Due to different assumptions of the entropy changes,the values of the optimum enthalpy∆H reported in the publications cover a wide range.In this paper,the adsorptionstates,vibrational modes,and the entropies of H2 molecules adsorbedon(MgO)9and(AlN)12clusters are studied by usingfirst principal method.The computation is performed by the second-order perturbation theory(MP2)with the triple zeta basis set including polarization functions 6-311G(d,p).The very-tight convergence criterion is used to obtain reliable vibration frequencies.Analysis shows that six vibrational modes of the adsorption complexes can be attributed to the vibration of H2molecule.For these normal modes,the amplitudes of the displacements of cluster atoms are usually two orders smaller than those of the hydrogen atoms.As the vibrational frequency is inversely proportional to the square root of the mass,the zero-point energy has an important influence on the adsorption energy.The ZPE correction exceeds half of the adsorption energy,and the adsorption on the anions is not stable after including the correction.Underthe harmonic approximation,the normal vibration modes are independent,so the entropy of adsorbed H2molecules can be calculated by using the vibrational partition function based on the vibrational frequencies.The results indicate that the entropy values depend mainly on the two lowest in-phase vibrational frequencies and it is not directly related to the adsorption strength but determined by the shape of the potential energy surface.In a temperature range of 70–350 K and at a pressure of 0.1 MPa,there is a good linear correlation between the entropy of adsorbed H2and the entropy of gas-phase.The entropy of H2decreases about 10.2R after adsorption.【相关文献】[1]Campbell C T,Sellers J R 2012 J.Amer.Chem.Soc.134 18109[2]de Moor B A,Ghysels A,Reyniers M F,van S V,Waroquier M,Marin G B 2011J.Chem.Theory Comput.7 1090[3]Simon C M,Kim J,Lin L C,Martin R L,Haranczyk M,Smit B 2014 Phys.Chem.Chem.Phys.16 5499[4]Efremenko I,Sheintuch M 2005 Langmuir 21 6282[5]Yang J,Sudik A,Wolverton C,Siegel D J 2010 Chem.Soc.Rev.39 656[6]Tang W S,Chotard J N,Raybaud P,Janot R 2014 J.Phys.Chem.C 118 3409[7]Bhatia S K,Myers A L 2006 Langmuir 22 1688[8]Otero Areán C,Nachtigallová D,Nachtigall P,Garrone E,Rodríguez Delgado M 2007 Phys.Chem.Chem.Phys.9 1421[9]Li J,Furuta T,Goto H,Ohashi T,Fujiwara Y,Yip S 2003 J.Chem.Phys.119 2376[10]Garberoglio G,Skoulidas A I,Jognson J K 2005 J.Phys.Chem.B 109 13094[11]Møller C,Plesset M S 1934 Phys.Rev.46 618[12]Hehre W J,Pople J A 1972 J.Chem.Phys.56 4233[13]Frisch M J,Tracks G W,Schlegel H B,et al.2013 Gaussian09 Revision D.01 Wallingford CT:Gaussian,Inc.[14]Wang Z C 2008 Thermodynamics and Statistical Physics(Beijing:Higher Education Press)p192(in Chinese)[汪志成2008热力学统计物理(北京:高等教育出版社)第192页][15]Larese J Z,Arnold T,Frazier L,Hinde R J,Ramirez-Cuesta A J 2008 Phys.Rev.Lett.101 165302[16]Wang Q,Sun Q,Jena P,Kawazoe Y 2009 ACS Nano 3 621[17]Zhang Y,Chen H S,Yin Y H,Song Y 2014 J.Phys.B 47 025102[18]Dong R,Chen X S,Wang X F,Lu W 2008 J.Chem.Phys.129 044705[19]Liu Z F,Wang X Q,Liu G B,Zhou P,Sui J,Wang X F,Zhu H J,Hou Z 2013Phys.Chem.Chem.Phys.15 8186[20]Yong Y L,Song B,He P 2011 Phys.Chem.Chem.Phys.13 16182[21]Okamoto Y,Miyamoto Y 2001 J.Phys.Chem.B 105 3470[22]Sun Q,Wang Q,Jena P,Kawazoe Y 2005 J.Amer.Chem.Soc.127 14582[23]Yildirim T,Ciraci S 2005 Phys.Rev.Lett.94 175501[24]Zhao Y,Kim Y H,Dillon A C,Heben M J,Zhang S B 2005 Phys.Rev.Lett.94 155504[25]Sun Q,Jena P,Wang Q,Marquez M 2006 J.Amer.Chem.Soc.128 9741[26]Yoon M,Yang S,Hicke C,Wang E,Geohegan D,Zhang Z 2008 Phys.Rev.Lett.100 206806 PACS∶36.40.—c,31.15.A—,68.43.Bc DOI∶10.7498/aps.66.103601*Project supported by the National Natural Science Foundation of China(GrantNos.11164024,11164034).†Corresponding author.E-mail:***************.cn。
Computational Investigation of Adsorption of Molecular Hydrogen on Lithium-Doped Corannulene
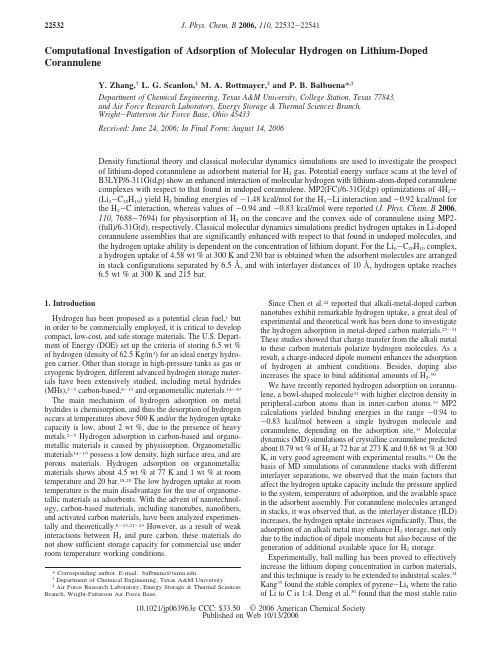
Computational Investigation of Adsorption of Molecular Hydrogen on Lithium-Doped CorannuleneY.Zhang,†L.G.Scanlon,‡M.A.Rottmayer,‡and P.B.Balbuena*,†Department of Chemical Engineering,Texas A&M Uni V ersity,College Station,Texas77843,and Air Force Research Laboratory,Energy Storage&Thermal Sciences Branch,Wright-Patterson Air Force Base,Ohio45433Recei V ed:June24,2006;In Final Form:August14,2006Density functional theory and classical molecular dynamics simulations are used to investigate the prospectof lithium-doped corannulene as adsorbent material for H2gas.Potential energy surface scans at the level ofB3LYP/6-311G(d,p)show an enhanced interaction of molecular hydrogen with lithium-atom-doped corannulenecomplexes with respect to that found in undoped corannulene.MP2(FC)/6-31G(d,p)optimizations of4H2-(Li2-C20H10)yield H2binding energies of-1.48kcal/mol for the H2-Li interaction and-0.92kcal/mol forthe H2-C interaction,whereas values of-0.94and-0.83kcal/mol were reported(J.Phys.Chem.B2006,110,7688-7694)for physisorption of H2on the concave and the convex side of corannulene using MP2-(full)/6-31G(d),respectively.Classical molecular dynamics simulations predict hydrogen uptakes in Li-dopedcorannulene assemblies that are significantly enhanced with respect to that found in undoped molecules,andthe hydrogen uptake ability is dependent on the concentration of lithium dopant.For the Li6-C20H10complex,a hydrogen uptake of4.58wt%at300K and230bar is obtained when the adsorbent molecules are arrangedin stack configurations separated by6.5Å,and with interlayer distances of10Å,hydrogen uptake reaches6.5wt%at300K and215bar.1.IntroductionHydrogen has been proposed as a potential clean fuel,1but in order to be commercially employed,it is critical to develop compact,low-cost,and safe storage materials.The U.S.Depart-ment of Energy(DOE)set up the criteria of storing6.5wt% of hydrogen(density of62.5Kg/m3)for an ideal energy hydro-gen carrier.Other than storage in high-pressure tanks as gas or cryogenic hydrogen,different advanced hydrogen storage mater-ials have been extensively studied,including metal hydrides (MHs),2-5carbon-based,6-13and organometallic materials.14-19 The main mechanism of hydrogen adsorption on metal hydrides is chemisorption,and thus the desorption of hydrogen occurs at temperatures above500K and/or the hydrogen uptake capacity is low,about2wt%,due to the presence of heavy metals.2-5Hydrogen adsorption in carbon-based and organo-metallic materials is caused by anometallic materials14-19possess a low density,high surface area,and are porous materials.Hydrogen adsorption on organometallic materials shows about4.5wt%at77K and1wt%at room temperature and20bar.18,20The low hydrogen uptake at room temperature is the main disadvantage for the use of organome-tallic materials as adsorbents.With the advent of nanotechnol-ogy,carbon-based materials,including nanotubes,nanofibers, and activated carbon materials,have been analyzed experimen-tally and theoretically.6-13,21-23However,as a result of weak interactions between H2and pure carbon,these materials do not show sufficient storage capacity for commercial use under room temperature working conditions.Since Chen et al.24reported that alkali-metal-doped carbon nanotubes exhibit remarkable hydrogen uptake,a great deal of experimental and theoretical work has been done to investigate the hydrogen adsorption in metal-doped carbon materials.25-31 These studies showed that charge transfer from the alkali metal to these carbon materials polarize hydrogen molecules.As a result,a charge-induced dipole moment enhances the adsorption of hydrogen at ambient conditions.Besides,doping also increases the space to bind additional amounts of H2.30We have recently reported hydrogen adsorption on corannu-lene,a bowl-shaped molecule32with higher electron density in peripheral-carbon atoms than in inner-carbon atoms.33MP2 calculations yielded binding energies in the range-0.94to -0.83kcal/mol between a single hydrogen molecule andcorannulene,depending on the adsorption site.33Molecular dynamics(MD)simulations of crystalline corannulene predicted about0.79wt%of H2at72bar at273K and0.68wt%at300 K,in very good agreement with experimental results.33On the basis of MD simulations of corannulene stacks with different interlayer separations,we observed that the main factors that affect the hydrogen uptake capacity include the pressure applied to the system,temperature of adsorption,and the available space in the adsorbent assembly.For corannulene molecules arranged in stacks,it was observed that,as the interlayer distance(ILD) increases,the hydrogen uptake increases significantly.Thus,the adsorption of an alkali metal may enhance H2storage,not only due to the induction of dipole moments but also because of the generation of additional available space for H2storage. Experimentally,ball milling has been proved to effectively increase the lithium doping concentration in carbon materials, and this technique is ready to be extended to industrial scales.34 Kang35found the stable complex of pyrene-Li4where the ratio of Li to C is1:4.Deng et al.30found that the most stable ratio*Corresponding author.E-mail:balbuena@.†Department of Chemical Engineering,Texas A&M University.‡Air Force Research Laboratory,Energy Storage&Thermal SciencesBranch,Wright-Patterson Air Force Base.22532J.Phys.Chem.B2006,110,22532-2254110.1021/jp063963e CCC:$33.50©2006American Chemical SocietyPublished on Web10/13/2006of Li:C is1:6and1:8for Li-graphite intercalation compound (Li-GIC)at its equilibrium interlayer distance,while it is1:3 for Li-pillared graphene sheet(Li-PGS)for interlayer separa-tions greater than8Å.In this paper,we report ab initio and density functional theory(DFT)studies of the doping of lithium atoms to the corannulene molecule as well as on its interactions with H2.We also analyze the effect that doping of Li atoms to corannulene has on H2uptake capacity at finite temperatures and pressures using classical MD simulations.We investigate two types of structures,one with six lithium atoms doped on a single corannulene molecule and the other with five lithium atoms,which leads to Li to C ratios between1:3and1:4, consistent with results from other groups.30,35putational Methods2.1.Ab Initio and DFT Calculations.The Gaussian03 package36was used to undertake the molecular orbital theory calculations.Previous theoretical studies on corannulene indi-cated that a hybrid DFT method combined with double- plus polarization basis sets would well reproduce the structural parameters of corannulene37,38and protonation and lithiumcation binding on corannulene.32,39In this paper,we use B3LYP/ 6-31g(d,p)for geometry optimization of lithium-atom-doped corannulene complexes and B3LYP/6-311g(d,p)for potential energy surface scans.The optimized geometries are followed by frequency calculations to prove that the stationary points are local minima and find the zero point energy corrections. Single point calculations and full optimizations using second-order Mo¨ller-Plesset perturbation theory(MP2)with the6-31G-(d,p)basis set are performed to obtain more accurate binding energies accounting for weak van der Waals forces that are responsible for the H2/corannulene interaction based on phys-isorption.2.2.Classical MD Simulations.The derivation of the force fields for H2-corannulene interactions was reported previ-ously.33Lennard-Jones(LJ)parameters were derived using the LJ parameters for the Li atom,D Li-Li)0.025kcal/mol,and x Li-Li)2.451Å,40corresponding to the form:These parameters and eq1were used to generate a new set of parameters according to the equivalent formyielding LiLi)0.0275kcal/mol andσLiLi) 2.18Å.The Lorentz-Berthelot mixing rules were used to obtain the LJ cross parameters Li-H)0.0432kcal/mol andσLi-H)2.57Å.For the dipole-induced interaction,the dipole moment of the Li3-C20H10-Li2complex(3.856D)calculated at the level of B3LYP/6-311G(d,p)and the Li polarizability of2.43×10-23 cm3(ref41)were used to define an average pair interaction calculated as:The dipole-induced interaction between Li and H2(equation3) was added to eq2to generate new data using the nonbonded interaction parameters Li-H)0.0432kcal/mol andσLi-H) 2.57Å,and a new fitting of these data to the form of the LJ eq 2yielded Li-H)0.9kcal/mol andσLi-H)2Å,which are the parameters used in the MD simulations.The DL_POLY program,42version2.14,was used for all MD simulations.A cutoff value of10Åwas used for nonbonded interactions,and periodic boundary conditions in three dimen-sions were applied to the simulation cell.The simulations were run in the canonical NVT ensemble at temperatures of273K and300K and pressures up to250bar.Details of the assembled system are provided in the Results and Discussion Section.The total simulation time for each P and T is800ps,with300ps for the equilibration period and500ps for the production period. The evaluations of H2uptake are based on the500ps production period.3.Results and Discussion3.1.Doping of Li Atoms to Corannulene.Corannulene isa bowl-shape molecule with C5V symmetry.Figure1shows the structure of corannulene optimized at the level of B3LYP/6-311G(d,p).There are three types of carbon atoms:the outmost 10carbons bonded to one hydrogen each are named as“rim”carbons(C r),the innermost five carbons on the five-membered ring as“hub”carbons(C h),while the remaining five carbons connecting between the rim and hub carbon atoms are designated as“bridge”carbons(C b).The calculation shows that rim carbon atoms possess higher electron density than hub and bridge carbon atoms,thus the dipole vector would point toward the five-membered ring.The calculated dipole moment of corannulene is2.18D,which is close to the experimental data of2.07D.43The structural information and Mulliken charge distribution of corannulene are shown in Table1.Recent DFT studies35of Li-aromatic sandwich compounds, R-nLi-R,where R is benzene,naphthalene,or pyrene,reported that the Li atom was preferentially adsorbed over the six-membered ring instead of over individual or pairs of C atoms. For a single Li ion,it was found that the Li cation was bounded on the convex side over a six-membered ring of corannulene at the level of B3LYP/6-311G(d,p)//B3LYP/6-31G(d,p).32The data in Table2indicate that complexation of a Li ion at the convex side is more stable than at the concave side,which is in agreement with the results of Frash et al.,32whereas,for the Li atom,complexation at the concave side is more stable(by1.2 kcal/mol)than at the convex side.E vdw )DIJ{-2[x IJ x]6+[x IJ x]12}(1)E vdw )4 {[σIJr]12-[σIJ r]6}(2)Γh ij )-7759.74r6meV(3)Figure1.Optimized structure of corannulene at the level of B3LYP/6-311G(d,p).TABLE1:DFT(B3LYP/6-311G(d,p))Structural Parametersand Mulliken Charge Distribution in Corannulene aatom charge atom pair bond distance(Å)angle(deg)C h-0.04C h-C h 1.42C h-C h-C b122.88C b0.00C h-C b 1.38C h-C b-C r114.43C r-0.07C b-C r 1.45C b-C r-C r121.96H+0.09C r-C r 1.40C r-C b-C r129.78a The atomic charges are average values.Adsorption of Molecular H on Lithium-Doped Corannulene J.Phys.Chem.B,Vol.110,No.45,200622533The Mulliken charges on the various atoms (labels as in Figure 1)shown in Table 2suggest that the higher charge of the Li cation may be the reason for the stronger binding to the corannulene molecule.Multiple Li atoms can be adsorbed either at the concave or at the convex side.Figure 2shows attachment of five Li atoms on corannulene at the level of B3LYP/6-31G(d,p).Considering the effect of charge transfer from Li to C atoms and thus the repulsion between two positively charged lithium atoms,the initial geometries were arranged with Li atoms over the outer six-membered rings on either side.In the initial configuration,all five Li atoms were located at the same side (either convex or concave)of the corannulene molecule,and the optimized structure resulted always with the five Li atoms attached to the concave side,over the six-membered rings,as shown in Figure 2a.Thus,when the optimization was started locating the Li atoms over the convex side,there was an inversion of curvature of the corannulene molecule,in agreement with the tendency shown in Table 2for a single Li atom.Similarly,when the initial configuration contains two Li atoms on one side and three on the other side,the energetically favorable optimized conforma-tions contain more Li atoms on the concave side,that is,three Li atoms on the concave side and the other two Li atoms on the convex side (Figure 2b);this conformation has lower energy than that in Figure 2a by minimizing repulsion effects.Frequency calculations indicate that both complexes in Figure 2are local minima.Comparison of the respective energies is shown in Table 3,along with related structural information.The Li 5-C 20H 10and Li 3-C 20H 10-Li 2complexes have σV symmetry,as shown in Figure 2.Table 3illustrates that there is charge transfer from Li atoms to corannulene molecules,resulting in positive charges on Li atoms of Li 5-C 20H 10and Li 3-C 20H 10-Li 2.Overall,the total charge on Li atoms in Li 3-C 20H 10-Li 2is higher than those in Li 5-C 20H 10.Thus,C atoms of corannulene are more negatively charged in the first complex,resulting in higher electrostatic interaction and shorter distances from Li atoms to six-membered rings of corannulene in Li 3-C 20H 10-Li 2than in Li 5-C 20H 10.Each of the three Li atoms (1Li,4Li,and 5Li)on the concave side of Li 3-C 20H 10-Li 2locate close to the center of a six-membered ring,whereas the two attached to the convex side of Li 3-C 20H 10-Li 2are closer to a six-membered ring than those on the concave side of Li 5-C 20H 10.The calculated energies show that Li 3-C 20H 10-Li 2is about 4kcal/mol more stable than Li 5-C 20H 10;the binding energy per Li atom is -23.91kcal/mol-Li in Li 3-C 20H 10-Li 2and -23.11kcal/mol-Li in Li 5-C 20H 10.The doping of six Li atoms on corannulene is illustrated in Figure 3,which shows four different combinations tested on the basis of the results obtained for complexes with five Li atoms.Figure 3a displays the complex Li 6-C 20H 10,where the first five Li atoms are doped over six-membered rings on the concave side,and the sixth lithium atom is in the center over these five Li atoms.Alternatively,with the sixth Li atom doped at the convex side,we obtain the Li 5-C 20H 10-Li complex (Figure 3b).Both Li 6-C 20H 10and Li 5-C 20H 10-Li have σV symmetry,with the atoms labeled 1Li and 6Li located on theTABLE 2:Structural and Energetic Properties and Mulliken Charges in Complexes of Corannulene with a Lithium Cation/Atom Attached to a Six-Membered Ring,Optimized at the B3LYP/6-31G(d,p)Levelcharges on atoms (e)complex with corannulene Li -C distance(Å)electronic energy(hartrees)binding energy (kcal/mol)Li C r C b C h Li +on the concave side 2.28-2.31-775.52514-47.50.44-0.120.12-0.03Li +on the convex side 2.29-2.44-775.52700-48.70.46-0.130.15-0.06Li on the concave side 2.22-2.25-775.68264-16.80.33-0.150.11-0.05Li on the convex side2.14-2.38-775.68073-15.60.31-0.160.17-0.08Figure 2.Optimized (B3LYP/6-31G(d,p))conformations of corannulene complexed with five Li atoms doped at different positions.(a)Five Li atoms doped at the concave side over six-membered rings,Li 5-C 20H 10.(b)Three lithium atoms doped at the concave side and two at the convex side over six-membered rings,Li 3-C 20H 10-Li 2.22534J.Phys.Chem.B,Vol.110,No.45,2006Zhang et al.symmetry plane.Another complex contains the first five Li atoms doped as in the complex Li 3-C 20H 10-Li 2,and the sixth Li atom is attached either to the concave or to the convex side over a six-membered ring,forming Li 4-C 20H 10-Li 2(Figure 3d)and Li 3-C 20H 10-Li 3(Figure 3c).These complexes are no longer symmetric after optimization.Frequency calculations on the optimized structures of these complexes also indicate they are local minima,except for Li 5-C 20H 10-Li,which is a saddle point.Table 4summarizes partial structural and energetic informa-tion.The most important difference is the negative charge over the Li atom on top of Li 6-C 20H 10,which may favor electrostatic Li -Li interactions,resulting in Li 6-C 20H 10being 9kcal/mol more stable than Li 3-C 20H 10-Li 3.The binding energies per mole of Li are -25.03and -23.53kcal/mol-Li for Li 6-C 20H 10and Li 3-C 20H 10-Li 3,respectively.The relatively large binding energies of the Li 6and Li 5complexes of corannulene might indicate stable Li adsorption on corannulene,with the ratio of Li:C between 1:4and 1:3.The lithium doping concentration is in agreement with results reported by other groups.30,353.2.Adsorption of H 2on Li-Doped Corannulene Com-plexes.3.2.1.Li 6-C 20H 10and Li 5-C 20H 10-Li.Adsorption of H 2is studied on the most stable complexes at each doping concentration,i.e.,on Li 6-C 20H 10and on Li 3-C 20H 10-Li 2.To obtain a qualitative comparison of the interaction strengths of H 2in corannulene and Li-doped corannulene,we computed a potential energy scan,as shown in Figure 4,which illustrates that no attractive interactions between hydrogen and the corannulene molecule are detected at this level of theory when a head-on H 2molecule approaches the corannulene moleculeTABLE 3:Li-Doped Corannulene Complexes:Structural Information and Electronic Energy (Ha)and Binding Energy (kcal/mol of Li)at the Level of B3LYP/6-31G(d,p)distance Li -C (Å)acomplex Li label (as shown in Figure 2)charge of Li(e)Li -C r Li -C bLi -C h Li 5-C 20H 1010.32 2.142.653.072,30.14 2.23,2.24 2.30,2.33 2.32,2.344,50.25 2.14,2.17 2.51,2.55 2.82,2.86Li 3-C 20H 10-Li 210.45 2.212.292.362,30.48 2.13,2.19 2.27,2.53 2.18,2.414,50.222.13,2.22 2.25,2.472.42,2.51electronic energy (Ha)zero point energy (Ha)binding energy (kcal/mol of Li)Li -7.49098C 20H 10-768.164830.23195Li 5-C 20H 10-805.803840.23366-23.11Li 3-C 20H 10-Li 2-805.810220.23260-23.91aWhen two values are shown,each one represents two pairs of the distance of Li -C in σv complexes.TABLE 4:Li -Corannulene Complexes:Structural Information,Electronic Energy (Ha),and Binding Energy Per Li Atom (kcal/mol-Li)at the Level of B3LYP/6-31G(d,p)adistance Li -C (Å)complex Li label (shown in Figure 3)Li charge Li -C r Li -C bLi -C h Li 6-C 20H 1010.22 2.16 2.673.162,30.31 2.19,2.20 2.27,2.31 2.34,2.364,50.26 2.14,2.18 2.55,2.64 2.97,3.026-0.23 5.08 4.76 4.55Li 5-C 20H 10-Li10.45 2.102.573.052,30.14 2.17,2.22 2.31,2.40 2.43,2.444,50.21 2.07,2.15 2.46,2.49 2.75,2.7960.51 2.152.422.25Li 4-C 20H 10-Li 210.26 2.22,2.28 2.33,2.45 2.44,2.5220.47 2.14,2.19 2.27,2.51 2.21,2.3330.45 2.12,2.13 2.36,2.43 2.24,2.3140.15 2.23,2.37 2.23,2.55 2.37,2.5150.05 2.18,2.18 2.51,2.52 2.81,2.8460.49 2.149,2.157 2.518,2.585 2.92,2.98Li 3-C 20H 10-Li 310.30 2.32,2.37 2.35,2.46 2.36,2.4220.45 2.17,2.22 2.31,2.50 2.21,2.3330.20 2.13,2.25 2.26,2.63 2.24,2.4640.15 2.17,2.28 2.29,2.54 2.50,2.6550.35 2.17,2.39 2.31,2.62 2.57,2.7560.392.17,2.17 2.40,2.412.29,2.32electronic energy(Ha)zero point energy(Ha)binding energy (kcal/mol-Li)Li -7.49098C 20H 10-768.164830.23195Li 6-C 20H 10-813.350080.23616-25.03Li 5-C 20H 10-Li -813.345990.23443-24.61Li 4-C 20H 10-Li 2-813.347630.23374-24.78Li 3-C 20H 10-Li 3-813.335680.23309-23.53aEach value in σv symmetry complexes,Li 6-C 20H 10and Li 5-C 20H 10-Li,represents two pairs of the distance of Li -C.Adsorption of Molecular H on Lithium-Doped Corannulene J.Phys.Chem.B,Vol.110,No.45,200622535along pathways a,b,c,and d (Figure 4).However,when H 2approaches Li 6-C 20H 10(pathway e)or Li 3-C 20H 10-Li 2(path-way f),a relatively strong attractive interaction between H 2and the Li atom -corannulene complex is observed.The strongest attraction appeared when the center of mass of the H 2molecule is at a distance of 2.47Åfrom the corresponding Li atom (Figure 5),yielding binding energies of -0.47and -2.06kcal/mol with Li 6-C 20H 10and Li 3-C 20H 10-Li 2,respectively.Figure 5shows the structures of H 2interacting with Li 6-C 20H 10and Li 3-C 20H 10-Li 2at the geometries corresponding to the minima on the potential surface curves of Figure 4.The separation of 2.47Å(Figure 5)corresponds to a distance of 2.10Å,shown in Figure 4,because that is the distance between the closest H atom and the corresponding Li atom;the difference between them is one-half of the calculated bond distance of H 2(0.74Å).To account for weak van der Waals forces that are responsible for the H 2/Li -corannulene complex interactions,we use second-order Moller -Plesset perturbation theory to determine the energy corresponding to such geometries using the 6-31G(d,p)basis set.The calculated MP2/6-31G(d,p)energies (single point calculations)are -1.83and -2.82kcal/mol for the H 2-Li 6-Figure 3.Optimized (B3LYP/6-31G(d,p))conformations of corannulene with six Li atoms.(a)Six Li atoms doped at the concave side,Li 6-C 20H 10.(b)Five Li atoms doped at the concave side and one at the convex side,Li 5-C 20H 10-Li.(c)Three Li atoms doped at the concave side and three at the convex side,Li 3-C 20H 10-Li 3.(d)Four Li atoms doped at the concave side and two at the convex side,Li 4-C 20H 10-Li 2.22536J.Phys.Chem.B,Vol.110,No.45,2006Zhang et al.C 20H 10system and H 2-Li 3-C 20H 10-Li 2,respectively.Recently,we reported binding energies of -0.94and -0.83kcal/mol for the physisorption of H 2on corannulene using MP2(full)/6-31G-(d)for H 2on the concave side and the convex side,respec-tively.33Thus,the H 2-Li interaction is stronger than that between H 2and C based on these binding energies.In the next section,we refine these estimates using optimizations with the MP2method.3.2.2.Li 2-C 20H 10.In this section,we analyze the most favorable hydrogen adsorption sites using Li 2-C 20H 10(Figure 6a),first with two hydrogen molecules on the convex side (Figure 6b)and then with two hydrogen molecules on the convex side and two hydrogen molecules on the concave side (a total of four H 2molecules,Figure 6c).The optimized configurations of these systems at the MP2(FC)/6-31G(d)level have a symmetry plane,and the two H 2molecules adsorbed on the convex side are located on that plane (Figure 6b and c),one molecule is adsorbed over the five-membered ring,and the other over a six-membered ring,and each of the lithium atoms is located over a six-membered ring.For the H 2molecule adsorbed over the five-membered ring,the distance between the closer H atom to hub carbons is in the range of 3.13-3.24Å,while the H 2molecule over the six-membered ring is closer to the pair of hub carbon atoms in the center,with a distance in the range of ∼3.18Å.Two additional H 2molecules adsorbed on the concave side (Figure 6c)adsorb on lithium atoms;the shortest H -Li distance is ∼2.12Å,significantly shorter than the distance between H 2molecules (on the convex side)and carbon atoms.The difference between adsorption distances on the various sites suggests that the Li atoms are the most favorable sites for hydrogen adsorption,which is confirmed by the calculated binding energies.For H 2adsorbed on the convex side (Figure 6b),a binding energy of -0.92kcal/mol-H 2is obtained.On the basis of the similar geometry of these two adsorbed H 2molecules in Figure 6b and c,we assume that the binding energy of these two H 2molecules are the same in the two cases,thus we infer that a binding energy of -1.48kcal/mol-H 2corresponds to the interaction between H 2(on the concave side)and a lithium atom.The energetic difference clearly shows that Li atoms are the favorable adsorption sites for H 2molecules,suggesting a mechanism of enhanced hydrogen adsorption on lithium-atom-doped corannulene systems.Another important feature in the Li-doped complexes of corannulene is the increase of the dipole moment.DFT predicts values of 5.39and 3.86D,respectively,for Li 6-C 20H 10and Li 3-C 20H 10-C 2,whereas the MP2calculation yields 3.47D for the Li 2-C 20H 10complex.The presence of this enhanced dipole in the molecule induces a dipole moment in the H 2molecules,which is taken into account to define the effective force field (Section 2.2)for molecular dynamics simulations discussed in the next section.3.3.Adsorption at Finite Temperatures and Pressures.3.3.1.Arrangement of Adsorbent Molecules.To investigate the available space and collective effects on H 2adsorption,we assume that lithium-doped corannulene molecules arrange in a stack configuration.In this case,we can adjust the interlayer and intermolecular distances (as defined below),performing analyses similar to those reported by other researchers using carbon nanotube bundles and graphite nanofibers.30,44-46Each MD simulation cell contains 16lithium-atom-doped corannulene molecules,with eight molecules in each layer located at the bottom of the cell.In the MD simulations,the dynamics of the adsorbent molecules is not included,thus the adsorbent mol-ecules are kept fixed in their initial positions and their distribution in each layer is determined by two parameters,the interlayer distance,ILD,and the intermolecular distance,IMD.The ILD is defined as the distance between two overlapped molecules and the IMD is the separation between the centers of two parallel molecules,as shown in Figure 7.The values of IMD and ILD were determined from DFT calculations discussed in the nextsection.Figure 4.Potential energy surface of H 2approaching corannulene and lithium-doped corannulene at the level of B3LYP/6-311G(d,p);in each curve,H 2approaches corannulene at:(a)the center five-membered ring at the concave side,(b)the center five-membered ring at the convex side,(c)the center of a six-membered ring at the concave side,(d)the center of a six-membered ring at the convex side,(e)the top Li atom in Li 6-C 20H 10(see Figure 3),(f)the Li atom on the convex side in Li 3-C 20H 10-Li 2(see Figure2).Figure 5.Structures corresponding to the minima in the potential energy scans:(a)Li 6-C 20H 10,(b)Li 3-C 20H 10-Li 2.Figure 6.Optimized configurations of H 2adsorption on Li 2-C 20H 10,with MP2(FC)/6-31G(d):(a)Li 2-C 20H 10;(b)2H 2-(Li 2-C 20H 10);(c)4H 2-(Li 2-C 20H 10).Figure 7.Definition of interlayer distance (ILD)and intermolecular distance (IMD).Adsorption of Molecular H on Lithium-Doped Corannulene J.Phys.Chem.B,Vol.110,No.45,2006225373.3.2.Estimation of the IMD and ILD Parameters.DFT partial optimizations at the level of B3LYP/6-311G(d,p)are performed for the characterization of the ILD and IMD distances in dimers of Li 6-C 20H 10,as shown in Figure 7.To determine the optimum ILD value,only the z coordinates of the dimer are allowed to change,whereas only the x coordinates of the dimer are allowed to change to investigate the optimum IMD value.The results are 11.4Åfor the IMD and 6.5Åfor the ILD.In our MD simulations,the IMD is fixed to 11.0Åfor all the adsorbent systems,and additional values of ILD of 8.0and 10.0Åare used for a simple stack of two different lithium-doped corannulene systems.Such a system is chosen to investigate the potential H 2uptake capacity,assuming thatsubstitution of H with bulky alkyl function groups to the rim carbons or bridging of lithium-atom-doped corannulene mol-ecules might be used to increase the ILD.3.3.3.Hydrogen Adsorption on Lithium-Doped Corannulene.Figure 8shows the predicted H 2uptake at 273and 300K and various ILD for Li 6C 20H 10as a function of H 2pressure.Overall,the MD simulations predict:(1)at all the conditions studied,the H 2uptake is higher than 2wt %;(2)the H 2uptake at a given ILD and temperature is almost linear with the increase of pressure;(3)adsorption decreases as temperature increases;(4)increasing ILD while keeping the same T and P significantly increases the H 2uptake,and the change is especially notorious increasing the ILD from 8.0to 10.0Å.At the estimated equilibrium separation ILD of 6.5Å,the predicted H 2uptake values are 2.75-5.08wt %at 70and 225bar at 273K,whereas at 300K,the values are reduced to 2.38-4.58wt %at 75and 230bar,respectively (Figure 8).Thus,according to the projection,the H 2uptake at an ILD of 6.5Åwould reach 6.5wt %at 300K and 315bar.Parts c -f of Figure 8illustrate that the rate of increase of H 2uptake (i.e.,the slope of the lines in Figure 8)increases as the interlayer distance increases at the same conditions of temper-ature and pressure,yielding significantly enhanced uptake at higher pressures for higher ILD separations.This could be attributed to the distribution of hydrogen molecules between adsorbent molecules,as seen from radial distribution functions (RDFs)between C and H 2,shown in Figure 9.At an ILD of 6.5Å,a relatively sharp peak is observed at a distance between 3.5and 4.5Å,with a shoulder appearing at about 5Å.This fairly sharp peak indicates that the majority of the H 2molecules located between adsorbent molecules are dis-tributed in a relatively thin layer,possibly interacting with the Li atom on top,whereas the shoulder may correspond to the molecules in a second layer located in the intermolecular space.As the ILD increases to 8Å,a broader peak (Figure 9)covers a distance between 4.0and 7Å,which reveals the larger space accessible to hydrogen molecules and the thickening of the adsorption layer.These features are clearly illustrated in Figure 10.At an ILD of 10Å,two broad (overlapping)peaks are evident in Figure 9,centered in ∼4and ∼7Årespectively,corresponding to the formation of two adsorption layers of hydrogen molecules between adsorbent molecules.The formation of two adsorption layers might explain the large increase in H 2uptake when the ILD increases from 8to 10Å.Similar H 2distribution has been found in single-wall and double-wall carbon nanotubes,and especially in GNFs as the VDW gaps increase in these systems.44According toourFigure 8.Calculated hydrogen uptake capacity of Li 6-C 20H 10molecules assembled in stacks.(a)ILD )6.5Å,273K;(b)ILD )6.5Å,300K;(c)ILD )8Å,273K;(d)ILD )8Å,300K;(e)ILD )10Å,273K;(f)ILD )10Å,300K.Figure 9.Radial distribution function of pairs C -H 2for the adsorption of H 2in stacks of the Li 6-C 20H 10complex at 273K:(a)ILD )6.5Å,P )119bar,and H 2uptake of 3.63wt %;(b)ILD )8Å,P )118bar,and H 2uptake of 3.95wt %;(c)ILD )10Å,P )114bar,and H 2uptake of 4.61wt%.Figure 10.Snapshots of Li 6-C 20H 10systems of adsorbent layers with hydrogen adsorbed at ILD )8Å,300K,and 124bar.22538J.Phys.Chem.B,Vol.110,No.45,2006Zhang et al.。
活性炭吸附甲苯动力学研究_李国文

∞ 0
ddCt dt =
C
( 12)
∫ ∫ _
′ 1
=
1 _0
∞ 0
ddCt t dt
=
1 Ci
ci
tdC
0
( 13)
∫ ∫ _ 2 =
1 _0
∞ 0
dC dt
(t
-
_′1 ) 2 dt =
1 Ci
ci
(t
-
_′1 ) 2 dC
0
( 14)
以上各式用于确定动力学参数及速度参数。 它们
为穿透曲线统计矩的函数 ,由这些函数及 Hermit 的时
P 大气压 , Pa
( 3) 内扩散系数 DC 及轴向分散系数 Dax
定义 Z=
_2 ( 2_′1 ) 2
,
将式
( 10)和
(
11)代入得:
Z=
Dax VX
+
W20
1
( 1+ W0 ) 2 X
R0 3K G
+
R20 1 5D C
( 22)
改变流速测定穿透曲线 ,由式 ( 13)、 ( 14)计算 _′1、_ 2 ,由
37
1. 13 0. 8900 0. 945
42
1. 69 0. 9832 0. 9916
间多项式 , 得出穿透曲线理论计算式:
∫ ∑ ∑ C(t) Ci
=
T
∞
ex p( - T2 ) an Hn ( T ) dt =
0
n= 0
∞
1 2
[ 1+
erf ( T ) ] - ( 2_ 2 ) 1 /2 an Hn- 1 ( T ) ex p( -
- 1、下载文档前请自行甄别文档内容的完整性,平台不提供额外的编辑、内容补充、找答案等附加服务。
- 2、"仅部分预览"的文档,不可在线预览部分如存在完整性等问题,可反馈申请退款(可完整预览的文档不适用该条件!)。
- 3、如文档侵犯您的权益,请联系客服反馈,我们会尽快为您处理(人工客服工作时间:9:00-18:30)。
Hydrogen adsorption in different carbon nanostructuresBarbara Panellaa,*,Michael Hirscher a ,Siegmar Rothba Max-Planck-Institut fu ¨r Metallforsc hung,Heisenbergstr.3,D-70569Stuttgart,Germany bMax-Planck-Institut fu ¨r Festko ¨rperforsc hung,Heisenbergstr.1,D-70569Stuttgart,GermanyReceived 23July 2004;accepted 29March 2005Available online 10May 2005AbstractHydrogen adsorption in different carbonaceous materials with optimized structure was investigated at room temperature and 77K.Activated carbon,amorphous carbon nanotubes,SWCNTs and porous carbon samples all show the same adsorption prop-erties.The fast kinetics and complete reversibility of the process indicate that the interaction between hydrogen molecules and the carbon nanostructure is due to physisorption.At 77K the adsorption isotherm of all samples can be explained with the Langmuir model,while at room temperature the storage capacity is a linear function of the pressure.The surface area and pore size of the carbon materials were characterized by N 2adsorption at 77K and correlated to their hydrogen storage capacity.A linear relation between hydrogen uptake and specific surface area (SSA)is obtained for all samples independent of the nature of the carbon mate-rial.The best material with a SSA of 2560m 2/g shows a storage capacity of 4.5wt%at 77K.Ó2005Elsevier Ltd.All rights reserved.Keywords:Activated carbon;Carbon nanotubes;adsorption;Adsorption properties;Surface area1.IntroductionHydrogen storage is the bottleneck for the break-through of hydrogen as energy carrier in automotive applications.In the last years different storage technolo-gies have been investigated [1,2]to develop a secure and cheap way to save hydrogen.Storage by gas compres-sion,hydrogen liquefaction,or in the form of metal hy-drides,possess severe disadvantages.These drawbacks have induced the study of new storage concepts as phys-ical adsorption on nanomaterials.Especially novel car-bon materials,such as carbon nanotubes,activated carbon and carbon nanofibers have attracted a lot of interest,however,the values for their hydrogen storage capacity scatter over several orders of magnitude [3].At present,the independently confirmed and repeatable storage capacities in carbon materials at room tempera-ture are less than 1wt%[4,5],however,higher values can be reached by lowering the temperature.The process responsible for hydrogen adsorption in carbon materials at moderate temperature is physisorption [6–10],which is based on weak van der Waals forces between adsor-bate and adsorbent.At room temperature the magni-tude of this interaction can be of the same order as the thermal motion energy of gas molecules on the surface,therefore only low storage capacities can be achieved [11,12].If the system is cooled down,the interaction be-tween the surface and the gas molecule becomes stronger than the thermal motion energy of the adsorbate,which is proportional to the temperature.In this work,different carbon nanostructures have been characterized and investigated in view of their hydrogen adsorption capacity up to high pressures both at room temperature and at 77K.These materials possess structures which have been optimized for the hydrogen uptake,e.g.,high specific surface area and micropore density.In order to understand the nature of the adsorption process,the hydrogen storage capacity0008-6223/$-see front matter Ó2005Elsevier Ltd.All rights reserved.doi:10.1016/j.carbon.2005.03.037*Corresponding author.Tel.:+497116891810;fax:+497116891952.E-mail address:bpanella@mf.mpg.de (B.Panella).Carbon 43(2005)2209–2214/locate/carbonis correlated to the structural properties of the carbon samples.2.Experimental2.1.Carbon nanostructuresVarious carbon materials have been obtained from different suppliers worldwide for this study.Firstly,dif-ferent types of carbon nanotubes (CNTs)with specific structures have been selected.Amorphous carbon nano-tubes have been produced by DC arc discharge in an atmosphere of hydrogen gas at temperatures above 300°C [13].These nanotubes are hollow pipes with diameter ranging from 10nm to 15nm,and the walls show no long-range ordered structure.Single-walled carbon nanotubes (SWCNTs),which have been pro-duced with the HiPco (High pressure CO conversion)method,have been purchased from CNI.This synthesis produces a high yield of well ordered SWCNTs with a narrow diameter distribution around 1nm,however,still containing metal catalyst particles.Therefore,this material has been purified by either selective oxidation and subsequent HCl treatment (sample I)[14]or stan-dard purification procedures giving 98wt%of SWCNTs (sample II).1Additionally,we received SWCNTs pre-pared by graphite arc discharge evaporation in helium atmosphere and collecting the soot from the cold walls of the reaction chamber [15].These samples contain typ-ically a high fraction of amorphous carbon and catalyst particles.Other samples have been produced by crack-ing of liquid hydrocarbons in a low voltage arc dis-charge or resistive heating.This method results in a mixture of MWCNTs,SWCNTs and polyhedral nano-particles of different compositions and are called Ros 1and Ros 3by the producer [16].Secondly,activated car-bon samples from different suppliers have been investi-gated.Activated carbon I is similar to AX-21[17],which is obtained from the reaction of coke with KOH,giving a product with a doubled specific surface area compared to regular grade porous carbon.Porous carbon samples 1and 2are carbon structures with or-dered pores of uniform size which have been prepared by the pyrolysis of sucrose embedded in mesoporous sil-ica [18].The surface area and the pore dimension of the sam-ples have been measured with quantachrome autosorb gas sorption system using nitrogen gas at 77K and applying the multipoint BET method for the surface area,and the MP-method which is an extension of the de Boer Õs t -method for the micropore volume.The differ-ent carbon nanostructures possess specific surface areas ranging from approximately 20m 2/g to 2560m 2/g,and high pore densities,see Table 1.The highest surface area is very close to the maximum theoretical surface of a double sided graphite sheet of 2630m 2/g.2.2.Hydrogen storage measurementThe hydrogen adsorption has been measured by a manually controlled Sieverts Õapparatus,which consists of two volumes connected through a valve,i.e.,sample holder and gas inlet chamber of constant volume.Owing to the design with small volumes,the apparatus can be used to investigate even small quantities of carbon mate-rial.A schematic diagram of the apparatus is shown in Fig.1.The apparatus was previously tested for leak ab-sence and for accuracy through calibration with the empty sample holder and with well known metal hy-drides.Ultra pure hydrogen gas has been used for the experiments.Typically the mass of the carbon samples used for hydrogen storage measurements is 100–300mg.Prior to measurement,the samples are degassed and heated at 200°C for approximately 2h until a pressure of 1·10À6mbar is reached.Then the desired hydrogen pressure is introduced in the thermostated chamber and after thermal equilibrium is reached,the gas is per-mitted to expand in the sample holder.The measured pressure drop is caused by the gas expansion in a bigger1Vajo J.Private communication.Table 1Carbon nanomaterials investigated for hydrogen storage and their structural properties Sample (country)BET SSA [m 2/g]Average porediameter [A ˚]Pore volume for pores withradius <6.5A ˚[cm 3/g]Helium density [g/cm 3]Activated carbon I (Canada)256411.750.752.0Porous carbon I (Mexico)164611.080.46 2.2Activated carbon II (Germany)106518.230.49 2.0Purified SWCNT I (USA/Germany)102415.370.39 2.0Porous carbon II (Mexico)94610.600.25 2.1Purified SWCNT II (USA)85417.090.36 2.1Amorphous CNT (China)36914.480.13 2.2SWCNTs (Russia)12415.520.05 1.7Ros 1(Cyprus)3411.460.01 2.1Ros 3(Cyprus)2211.320.00652.02210 B.Panella et al./Carbon 43(2005)2209–2214volume and hydrogen adsorption in the sample.After no more pressure change is observed,we wait for an additional10–15min to be sure that the thermal equilib-rium is reached.After hydrogen adsorption at room temperature,the sample holder is cooled in liquid nitro-gen until the new equilibrium condition is reached.In this case the decrease of the hydrogen pressure is due to the cooling of the gas and due to the enhancement of adsorption in the sample at low temperature.To eval-uate the storage capacity of the carbon materials the experiment is repeated under the same conditions with a non-adsorbing blind sample(Seasand)having the same volume as the carbon material.The volume of the sample is measured volumetrically with helium gas.Each adsorption cycle is repeated at different hydro-gen pressures from2bar to approximately60bar and after each adsorption the sample is heated and degassed. This means that each storage value is obtained indepen-dently from the previous step.The corrected ideal gas equation with a compressibility factor up to the second order is used to calculate the amount of adsorbed hydro-gen from the pressure drop.For some samples the hydrogen storage capacity in the pressure range from 0.2bar to0.9bar and at77K is measured gravimetri-cally with a Cahn microbalance.Here the mass change as a function of hydrogen pressure is recorded.The con-gruency of the values measured with the two different techniques is a further proof for the accuracy of our vol-umetric measuring system.3.Results and discussionSimilar adsorption isotherms have been obtained for all investigated carbon nanostructures.Furthermore, they show a complete reversibility of the hydrogen up-take.After each adsorption cycle at a given pressure the hydrogen is removed from the sample and the mea-surement is repeated for a different pressure.The hydro-gen storage capacity is independent of the number of adsorption cycles which proves that hydrogen is stored reversibly in these carbon materials.If hydrogen gas was expanded in the sample holder,the equilibrium pressure is reached within a few seconds.During waiting for10–15min no further pressure change is observed, indicating that thermal equilibrium is reached.These fast kinetics are characteristic for physisorption.Fig.2shows the hydrogen adsorption isotherms of activated carbon I and of purified SWCNTs II at77K and at room temperature.Even though the two materi-als possess a completely different nanostructure,the shape of the isotherms is very similar.This shape ischaracteristic for all investigated carbon materials.At 298K the hydrogen uptake is a linear function of the pressure,which can be explained with HenryÕs law.At room temperature no saturation occurs in the investi-gated pressure range and the adsorbed hydrogen layer on the carbon surface is very diluted.At low temperature the isotherms can befitted with a Langmuir-type equation(type I isotherm)indicating that saturation takes place with a hydrogen monolayer formation,as usual for microporous surfaces.In fact, the pore dimensions limit the hydrogen adsorption to one molecular layer in structures with micropores [19,20].Additionally,an average value of the adsorption en-thalpy at77K can be estimated by applying the Lang-muir equation[21–23]:h¼11þexp eÀlkTÀÁ;ð1Þwhere e is the adsorption potential of the solid,k the Boltzmann constant,T the temperature,h the fractional coverage,and l the chemical potential of a hydrogen molecule in the gas.The chemical potential as a function of the hydrogen pressure is calculated using the tabu-lated values of the enthalpy and the entropy at77K [24].This evaluation yields an average adsorption en-thalpy ofÀ(56±10)meV for activated carbon I,which is with a storage capacity of about4.5wt%at77K,the best material analyzed.This adsorption potential has to be considered as an approximate value since any depen-dence between the adsorption potential and coverage has been neglected.Nevertheless,the low value clearly indicates that hydrogen is physically adsorbed in this carbonaceous nanoporous material.Chemisorption of hydrogen would require more than ten times higher en-thalpy of adsorption[25,26].The hydrogen uptake of the different carbon nano-structures at77K is correlated to their specific surface area(Fig.3).An almost linear relation between the stor-age capacity,evaluated from the saturation value of the Langmuirfit,and the specific surface area is obtained for all materials investigated.In contrast,the storage capacity seems to be independent of the nature of the carbon nanomaterial,as long-range order or curvature of the graphene sheets.In accordance,similarfindings by inelastic neutron scattering investigations have been reported in Refs.[8,9].An upper limit of the hydrogen adsorbed in a monolayer can be estimated by assuming the density of the liquid adsorbate[10].Accordingly,the maximum hydrogen storage capacity per specific surface area of carbon can be theoretically calculated to 2.28·10À3 mass%mÀ2g.The experimental results yield a slope of 1.91·10À3mass%mÀ2g,which is smaller than the theoretical value,because at77K the density of the monolayer is smaller than the density of liquid hydrogen.The mea-sured value corresponds to an occupancy of one hydro-gen molecule per17.5A˚2of carbon surface.Assuming a closely packed distribution of hydrogen molecules on the surface of the adsorbent,this occupancy corre-sponds to a reticular distance of4.5A˚between hydrogen molecules on the carbon surface.Further considering the area of a carbon hexagon of the graphene sheet (5.2A˚2),approximately six hydrogen molecules would occupy the area of20carbon hexagons.On the other hand,under the assumption that the closely packed hydrogen monolayer has an intermolecu-lar distance equal to that of liquid hydrogen(4.1A˚),the experimental value at77K corresponds to a surface coverage of84%.Similar results of a linear relation between hydrogen uptake and specific surface area of carbon materials have been obtained by Nijkamp et al.[27]with a slope of1.5·10À3mass%mÀ2g at a pressure of1bar and 77K.Theoretical calculations[28,12]indicate,that hydro-gen adsorption is favoured in small micropores.There-fore,the hydrogen adsorption capacity is correlated to the pore volume of pores possessing diameter less than 1.3nm(Fig.4).The linear relation between the pore vol-ume and the storage capacity demonstrates that carbon materials ideal for hydrogen storage should possess a high microporosity with a small pore dimension.Fig.5shows that at room temperature as well the storage capacity of the carbon samples at a pressure of 65bar depends almost linearly to their surface area.In this case the slope of the curve is only0.23·10À3mass%mÀ2g,which corresponds to a surface cov-erage of only10%assuming the density of the liquid adsorbate.Similar low hydrogen storage capacitiesatRT have been found by NMR investigations in SWCNTs [29–31].4.ConclusionDifferent carbon materials have been investigated with optimized structures for hydrogen adsorption.The fast kinetics and complete reversibility give clear evidence that the process responsible for hydrogen adsorption in carbon nanostructures is physisorption.The amount of hydrogen physisorbed depends almost linearly on the specific surface area,both at low temper-ature and at room temperature,and is independent of the type of carbon nanostructure.Therefore the hydro-gen adsorption process is based on the local interaction between the hydrogen molecule and the surface,how-ever,independent of long-range order,curvature or or-dered arrays of the graphene sheets.As a consequence promising materials for hydrogen adsorption are nanostructures with high surface area and micropore density.While at room temperature the highest measured storage value is less than 1wt%even at high hydrogen pressure,at 77K it is possible to store up to 4.5wt%of hydrogen.Among all investigated materials activated carbon with high grade of porosity is the best carbon nanostructure for hydrogen adsorption.Owing to the fast adsorption and desorption kinetics and the rever-sibility,physisorption is a very promising concept for hydrogen storage at moderate conditions.Future inves-tigations should concentrate on new materials with sur-face area,even higher than activated carbon,in order to achieve successful storage capacities not only at low temperature but also at ambient conditions.AcknowledgmentsThe authors are thankful to Richard Chahine fromthe Universite´du Quebec,Canada,for the synthesis of the activated carbon sample I,John Vajo from HRL Laboratories,California,for purifying the CNI SWCNTs and Channing Ahn from CalTech,California,for providing us with the samples.We are also grateful to Mauricio Terrones,Humberto Terrones,Eduardo Terres and Jose Manuel Dominguez from IPICyT,Mex-ico,for the synthesis of the porous carbon samples and Ursula Dettlaff-Wegliskowska from the Max-Planck-Institut fu ¨r Festko¨rperforschung in Stuttgart for the purification of the SWCNTs sample I.Our thank goes also to Alexander Okotrup and Lyubov Bulsheva from the Institute of Inorganic Chemistry SB RAS,Russia,for the SWCNTs sample,to W.Heschel from the TU Bergakademie Freiberg,Germany,for providing the activated Carbon II sample,to Maria Xenophontos-Ioannou from Rosseter Holdings Ltd,Cyprus,for the Ros1and Ros3samples,and to Yongning Liu from the Department of Material Science and Engineering at the Xi Õan Jiatong University,China for the amor-phous CNT sample.We are also thankful to Annette Fuchs for the N 2sorption measurements.References[1]Zu ¨ttel A.Materials for hydrogen storage.Mater Today 2003;6(9):24–33.[2]Schlapbach L,editor.Hydrogen storage special issue.MRS Bull 2002;27(2).[3]Hirscher M,Becher M.Hydrogen storage in carbon nanotubes.J Nanosci Nanotech 2003;3(1–2):3–17.[4]Ritschel M,Uhlemann M,Gutfleisch O,Leonhardt A,GraffA,Taeschner C,et al.Hydrogen storage in different carbon nano-structures.Appl Phys Lett2002;80(16):2985–7.[5]Chen X,Haluska M,Dettlaff-Wegliskowka U,Hirscher M,Becher M,Roth S.Mater Res Soc Symp Proc2002;706:Z9.11.1–6.[6]Haluska M,Hirscher M,Becher M,Dettlaff-Wegliskowka U,Chen X,Roth S.Interaction of hydrogen isotopes with carbon nanostructures.Mater Sci Eng B-Solid2004;108:130–3.[7]Williams KA,Pradhan BK,Eklund PC,Kostov MK,Cole MW.Raman spectroscopic investigation of H2,HD,D2physisorption on ropes of single-walled,carbon nanotubes.Phys Rev Lett 2002;88(16):165502-1–2-4.[8]Schimmel HG,Kearly GJ,Nijkamp MG,Visser CT,de Jong KP,Mulder FM.Hydrogen adsorption in carbon nanostructures: Comparison of nanotubes,fibers and coals.Chem Eur J2003;9(19):4764–70.[9]Schimmel HG,Nijkamp G,Kearly GJ,Rivera A,de Jong KP,Mulder FM.Hydrogen adsorption in carbon nanostructures compared.Mater Sci Eng B-Solid2004;108:124–9.[10]Zu¨ttel A,Sudan P,Mauron P,Wenger P.Model for the hydrogenadsorption on carbon nanostructures.Appl Phys A2004;78(7): 941–6.[11]Wang Q,Johanson JK.Optimization of carbon nanotubearrays for hydrogen adsorption.J Phys Chem B1999;103: 4809–13.[12]Rzepka M,Lamp P,de la Casa-Lillo MA.Physisorption ofhydrogen on microporous carbon and carbon nanotubes.J Phys Chem B1998;102(52):10894–8.[13]Liu Y,Xiaolong S,Tingkai Z,Jiewu Z,Hirscher M,Phillipp F.Amorphous carbon nanotubes produced by a temperature con-trolled DC arc discharge.Carbon2004;42:1852–5.[14]Dettlaff-Wegliskowska U,Roth S.Simple and efficient purifica-tion of carbon nanotubes.XV International Winterschool/Euro-conference Kirchberg.American Institute of Physics:Tirol Austria;2001.p.171–4.[15]Okotrub AV,Bulusheva LG,Tomanek D.X-Ray spectroscopicand quantum-chemical study of carbon tubes produced in arc-discharge.Chem Phys Lett1998;289:341–9.[16]Ryzhkov VA.Carbon nanotube production by a cracking ofliquid hydrocarbons.Physica B2002;323:324–6.[17]Chahine R,Bose TK.Low pressure adsorption storage ofhydrogen.Int J Hydrogen Energy1994;19(2):161–4.[18]Terres E,Panella B,Hayashi T,Kim YA,Endo M,DomingezJM,et al.Hydrogen storage in spherical nanoporous carbon.Chem Phys Lett2005;403:363–6.[19]Lowell S,Shields Powder JE.Surface area and porosity.3rdedition.Chapman&Hall;1991;14.[20]Kadono K,Kajiura H,Shiraishi M.Dense hydrogen adsorptionin carbon subnanopores at77K.Appl Phys Lett2003;83:3392–4.[21]Ye Y,Ahn CC,Witham C,Fultz B,Liu J,Rinzler AG,et al.Hydrogen adsorption and cohesive energy of single-walled carbon nanotubes.Appl Phys Lett1999;74(16):2307–9.[22]Shiraishi M,Takenobu T,Ata M.Gas-solid interactions in thehydrogen/single-walled carbon nanotube system.Chem Phys Lett 2003;367(5–6):633–6.[23]Shiraishi M,Takenobu T,Kataura H,Ata M.Hydrogenadsorption and desorption in carbon nanotube systems and its mechanisms.Appl Phys A2004;78(7):947–53.[24]McCarty RD.Hydrogen:Its technology and implications.Hydrogen Properties,Vol.III.Cleveland:CRC Press;1975.196–252.[25]Chan S,Chen G,Gong XG,Liu Z.Chemisorption of hydrogenmolecules on carbon nanotubes under high pressure.Phys Rev Lett2001;87(20):205502-1–2-4.[26]Atsumi H.Hydrogen retention in graphite and carbon materialsunder a fusion reactor environment.J Nucl Mater2003;313–316: 543–7.[27]Nijkamp MG,Raaymakers JEMJ,van Dillen AJ,de Jong KP.Hydrogen storage using physisorption-materials demands.Appl Phys A2001;72(5):619–23.[28]Wang Q,Johnson JK.Molecular simulation of hydrogenadsorption in single-walled carbon nanotubes and idealized carbon slit pores.J Chem Phys1999;110(1):577–84.[29]Kleinhammes A,Mao S-H,Yang X-J,Tang X-P,Shimoda H,LuJP,et al.Gas adsorption in single-walled carbon nanotubes studied by NMR.Phys Rev B2003;68:75418-1–6.[30]Shen K,PietraßT.1H and2H NMR of hydrogen adsorption oncarbon nanotubes.J Phys Chem B2004;108(28):9937–42. [31]Shen K,Xu H,Jiang Y,PietraßT.The role of carbon nanotubestructure in purification and hydrogen adsorption.Carbon 2004;42:2315–22.2214 B.Panella et al./Carbon43(2005)2209–2214。