Effects of irrigation on water balance, yield and WUE of winter wheat in NCP
污染水源带来的危害英语作文

污染水源带来的危害英语作文英文回答:Pollution of water sources can have serious consequences for both the environment and human health. One of the major hazards of contaminated water sources is the spread of waterborne diseases. When pollutants such as bacteria, viruses, and chemicals enter our water sources, they can cause illnesses such as diarrhea, cholera, and typhoid fever. These diseases can be particularly dangerous for children, the elderly, and people with weakened immune systems.In addition to the health risks, pollution of water sources can also harm aquatic ecosystems. For example, industrial runoff containing heavy metals can poison fish and other aquatic organisms, disrupting the balance of the ecosystem. This can have a ripple effect, impacting not only the aquatic life but also the animals and plants that depend on them for food and habitat.Furthermore, polluted water sources can also affect human activities such as agriculture and tourism. Contaminated water used for irrigation can lead to the accumulation of toxins in crops, posing a risk to human consumption. Similarly, polluted beaches and water bodies can deter tourists, leading to economic losses for communities that rely on tourism as a source of income.Overall, the hazards of polluted water sources are far-reaching and can have devastating effects on both the environment and human well-being. It is crucial that wetake steps to prevent water pollution and protect our water sources for future generations.中文回答:水源污染带来的危害不仅影响环境,也对人类健康造成严重威胁。
对水资源保护的看法英语作文
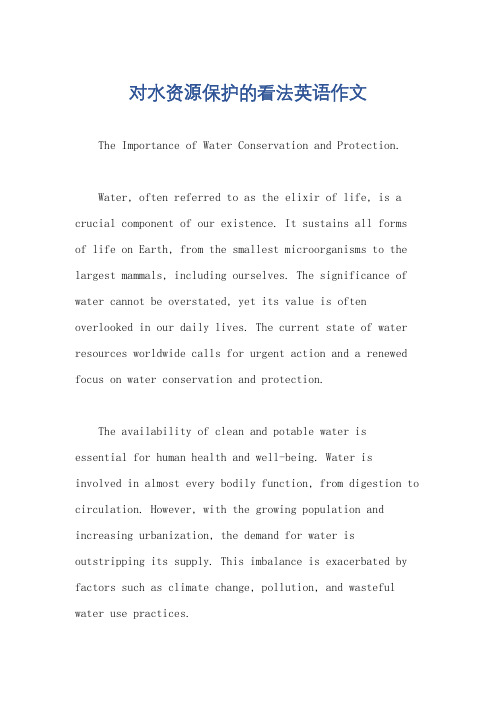
对水资源保护的看法英语作文The Importance of Water Conservation and Protection.Water, often referred to as the elixir of life, is a crucial component of our existence. It sustains all forms of life on Earth, from the smallest microorganisms to the largest mammals, including ourselves. The significance of water cannot be overstated, yet its value is often overlooked in our daily lives. The current state of water resources worldwide calls for urgent action and a renewed focus on water conservation and protection.The availability of clean and potable water isessential for human health and well-being. Water is involved in almost every bodily function, from digestion to circulation. However, with the growing population and increasing urbanization, the demand for water is outstripping its supply. This imbalance is exacerbated by factors such as climate change, pollution, and wasteful water use practices.Climate change, in particular, is having a profound impact on water resources. Rising temperatures lead to increased evaporation, drying up of rivers and lakes, and changes in precipitation patterns. These changes not only affect the quantity of water available but also its quality. Pollutants and contaminants, often carried by runoff from urban and agricultural areas, contaminate surface and groundwater sources, rendering them unsafe for human consumption.In addition to climate change, human activities also contribute significantly to water scarcity and pollution. Irrigation practices, industrial waste discharge, and sewage outflow are among the primary sources of water pollution. These activities release harmful chemicals,heavy metals, and nutrients into water bodies, causing eutrophication and toxic accumulation.To address these challenges, water conservation and protection measures are crucial. Water conservationinvolves the efficient use of water resources to minimizewaste and maximize benefits. Simple practices like fixing leaky faucets, using water-saving appliances, and harvesting rainwater can significantly reduce water consumption. Agricultural practices such as drip irrigation and conservation tillage can also help conserve water while improving crop yields.Water protection, on the other hand, focuses on preventing pollution and maintaining water quality. This involves the establishment of water treatment facilities, strict enforcement of environmental regulations, and the promotion of sustainable development practices. For instance, waste management practices that ensure proper disposal of solid and liquid waste can prevent pollution of water bodies. Similarly, promoting the use of renewable energy and reducing greenhouse gas emissions can mitigate the impact of climate change on water resources.Moreover, public awareness and education are essential in water conservation and protection efforts. People need to understand the value of water and their role in its conservation. Educational campaigns and community-basedinitiatives can raise awareness about water scarcity and pollution issues, encouraging individuals and communities to adopt water-saving practices.In conclusion, water conservation and protection are paramount for sustainable development and the well-being of future generations. The challenges we face in water management are complex and require a multifaceted approach. By combining efforts at the individual, community, and policy levels, we can ensure the availability of clean and potable water for ourselves and future generations.。
地下水专业英文文章

Current research in urban hydrogeology –A reviewMario Schirmer a ,⇑,Sebastian Leschik b ,1,Andreas Musolff caEawag,Swiss Federal Institute of Aquatic Science and Technology,Department Water Resources and Drinking Water,Ueberlandstr.133,8600Dübendorf,Switzerland bUFZ –Helmholtz Centre for Environmental Research,Department of Groundwater Remediation,Permoserstr.15,04318Leipzig,Germany cUFZ –Helmholtz Centre for Environmental Research,Department of Hydrogeology,Permoserstr.15,04318Leipzig,Germanya r t i c l e i n f o Article history:Available online 13July 2012Keywords:GroundwaterUrban hydrogeology PPCPContamination Monitoring Modellinga b s t r a c tUrban groundwater is a heritage at risk because urban land use practises puts enormous and highly com-plex pressure on this resource.In this article,we review urban groundwater studies in the context of urban water management,discuss advances in hydrogeological investigation,monitoring and modelling techniques for urban areas and highlight the challenges.We present how techniques on contaminant concentration measurements,water balancing and contaminant load estimation were applied and fur-ther developed for the special requirements in urban settings.To fully understand and quantify the com-plex urban water systems,we need to refine these methods and combine them with sophisticated modelling approaches.Only then we will be able to sustainably manage our water resources in and around our urban areas especially in light of growing cities and global climatic change.We believe that over the next few years much more effort will be devoted to research in urban hydrogeology.Ó2012Elsevier Ltd.All rights reserved.1.IntroductionUrbanisation is an emerging issue with ecological,economic and social implications.Currently half of the world’s and 70%of Europe’s population is living in urban areas.According to the Uni-ted Nations,by 2050these numbers are going to rise to 70%and 84%,respectively [1].In the year 2000urbanised areas made up 3.7%of Europe’s surface.Between the years 1990and 2000the an-nual land consumption by housing,services and recreation was 50,000ha which refers to half of the total land consumption (based on Corine land cover 1990and 2000for 23European countries,http://www.eea.europe.eu/).Of course,there are positive aspects of this development such as more efficient use of land resources and more effective public transport and centralised waste treat-ment,reducing per capita emissions of contaminants [2].Never-theless,urban land use leads to enormous pressure on the environment.Aside from drastic changes in the water balance,manifold and often diffuse and poorly regulated emissions have had a negative impact on the quality of air,soil and urban water resources [2].On one hand,this environmental stress is likely to in-crease with further urban growth at an unprecedented rate.On the other hand,the stress factors will change as urban areas undergo dramatic changes,like shrinking or large migration as seen in many cities in the former Eastern Bloc.Urban water usage as well as urban water quantity and quality problems are closely linked to the city’s development [3].The dif-ferent states of development can be seen in all major cities of the world [4].Over history,settlements often relied on groundwater from springs and shallow wells as a reliable source of clean potable water.With industrialisation and an accelerated urbanisation,water demand has increased.Due to the heavy abstraction,groundwater supplies beneath cities have been declining and as a consequence of unregulated waste management,groundwater quality has become more and more degraded [5].Cities have increasingly become importers of water from remote sources.Overexploitation of groundwater beneath urban areas,declining water levels,the resulting land subsidence and,for coastal cities,salt water intrusion,still are major concerns in many cities of the world [3,5,6].However,over recent decades,in the developed world,abstraction volumes have been reduced and groundwater levels are rising again.Consequently,pumping has to be increas-ingly employed to prevent flooding of underground structures [7].Maintaining the quality and quantity of urban water resources is recognised as a very complex task including different spatial and temporal scales.The key to understand the deterioration of ur-ban water resources is the knowledge of the tremendous impact of urbanisation on the entire water balance (Fig.1).The deterioration of the water balance can develop very differently in contrasting ur-ban areas and even within heterogeneous cities.Often,surface sealing in urban areas leads to an increase of surface runoff and thus to a reduction of water infiltrating into the subsoil.On the other hand,water is imported into the urban areas by water mains and transported after usage within the sewage system.Water can0309-1708/$-see front matter Ó2012Elsevier Ltd.All rights reserved./10.1016/j.advwatres.2012.06.015⇑Corresponding author.Tel.:+41587655382;fax:+41587655210.E-mail addresses:mario.schirmer@eawag.ch (M.Schirmer),andreas.musolff@ufz.de (A.Musolff).1New address:CDM Smith,Weißenfelser Str.65H,04229Leipzig,Germany.leak from these subsurface infrastructures as artificial groundwa-ter recharge,increasing the net recharge beneath urban areas[8]. Storm water runoff can also be transported in the sewage system as artificial interflow and mix with wastewater in combined sew-ers.When the water amount exceeds the capacity of the sewage system,this contaminated storm water can discharge into surface waters(combined sewer overflow,CSO).In urban settings streams as major receivers for groundwater as well as for treated and un-treated wastewater are often degraded by a multitude of stressors [9].This degradation is summarised as the‘‘urban stream syn-drome’’and comprises amongst others the‘‘flashier’’hydrograph with shorter lag times to peakflow,changed baseflow magnitude and impaired channel morphology.These effects most likely influ-ence the magnitude and quality of groundwater-surface water interactions.In general the manifold interactions of the different urban water compartments are complex in time and space and still leaves many questions open[10–14].The disturbance of the natural water balance is closely con-nected with deteriorating quality,since new pathways for contam-inants are introduced.Probably most challenging is the variety of chemicals from human and industrial activities released via differ-ent wastewater sources.We live in a‘‘chemical society’’with thou-sands of chemical compounds available in the products of our daily life[15].Due to the concentrated accumulation,and the transport and treatment of wastewater in urban areas,urban water re-sources are at particular risk.The waste-water-borne contami-nants are often present in waters in low concentrations ranging from pg LÀ1to ng LÀ1and are therefore termed‘‘micropollutants’’[16].Examples of micropollutants are pharmaceuticals and per-sonal care products(PPCP)and endocrine disrupting chemicals (EDC)[17–19].These compounds are now frequently found in wastewater treatment plants and surface water bodies[20–23], and although in the last few years several research groups have begun to study these chemicals in urban groundwater(e.g., [17,24–29]),they are not usually the focus of groundwater investigations.Despite the fact that urban-source micropollutants are of con-cern,urban areas are also often associated with industrial activities which potentially introduce macro pollutants such as chlorinated solvents,polycyclic aromatic hydrocarbons(PAHs)and gasoline constituents.In addition,agricultural practises within cities and sewer leakages have contaminated urban aquifers with large amounts of nitrate and phosphate which are still of great concern (e.g.,[30]).The present and future tasks concerning the management of ur-ban water resources are not new.The urban population needs a reliable supply of clean drinking water on the one hand,and on the other hand,urban groundwater contamination and wastewater have to be treated and storm water has to be managed.This task has a substantial overlap with the concept of Integrated Urban Water Management(IUWM).In an IUWM approach water supply, drainage and the sewage systems are seen as parts of an integrated physical system[31].This approach is a logical consequence of the connection of the water compartments in the urban water balance. Nevertheless,groundwater is often not sufficiently integrated into IUWM concepts[32,33].This does not mean we only have to man-age the negative effects such as land subsidence,infiltration to the sewage system or building damage by high groundwater levels.Ur-ban groundwater is a heritage and deserves protection and sustain-able management in the same way as other water resources. Although being affected by urban land use and anthropogenic activity,a growing number of publications show the value and usability of urban groundwater resources as part of water re-sources management in urban areas(e.g.,[34]).Urban groundwater can be utilised for potable and non-potable water production(e.g.,[35]).Managed and cost-effective aquifer recharge and aquifer storage and recovery methods can be used to recycle storm water or treated sewage for non-potable and indi-rect potable reuse[36,37].Bankfiltration of surface water provides potable water for cities like Berlin(Germany)[38].Foster et al.[39] report the extensive and unregulated usage of shallow urban groundwater in many developing cities as a low-costalternative Simplified urban impact on the water balance.The red arrows represent waterflow which has been modified or newly introducedto the municipal water supply.Even if the groundwater is not used for water production,urban aquifers are a potential storage loca-tion for storm water,reducing surface runoff from sealed areas [40].Finally,urban groundwater is a valuable energy reservoir since subsurface temperatures are often higher below cities.This significant geothermal potential could be exploited by heat pump installations[41,42].Within the framework of a complex urban water balance,the management of urban groundwater has to be carried out very care-fully on a sound scientific basis.This includes urban water balanc-ing,the description of contaminant input,transport and fate and the integration into holistic modelling approaches.This review fo-cuses on the challenge to integrate the groundwater component into urban water management.More specifically,we will define the information needed to assess urban groundwater quality and quantity.Subsequently,we review the state-of-the-art of literature with a special focus on adapted methodologies for urban water balancing,the assessment of contaminant concentration distribu-tions in time and space and the estimation of contaminant loads and the implementation of holistic modelling approaches.Being an emerging issue in urban areas,we put special attention to the problem of micropollutants such as pharmaceuticals and personal care products(PPCPs).On this basis,we discuss state and future re-search needs concerning urban hydrogeology.2.Special settings in urban hydrogeologyUrban hydrogeology research has to deal with a high complex-ity of waterflow and contaminant transport for different reasons. In many cases,urban areas developed in geologically interesting and often complex environments,this means close to rivers,hills and other features(e.g.salt water springs and hot springs)which make an investigation a challenge.In addition,urban land use comprises a spatial highly heterogeneous pattern of surface sealing and vegetation which affects groundwater recharge processes.Fi-nally,the subsurface infrastructure,such as the water supply sys-tem and the sewage system introduces both spatial and temporal variability of water and contaminantflow.Especially for micropol-lutants,the temporal variability of wastewaterflow and quality is of crucial importance.Wastewaterflow often peaks in the morning and evening hours of a day as a result of higher water usage at these times.The highly dynamic waterflow is,however,only one side of the coin,there are also rapidlyfluctuating concentra-tions of the chemicals that areflushed down the sewer system. Wastewater has a dynamic composition of different packages from numerous users in the sewage catchment[43].A significant proportion of wastewater is lost to the subsurface by sewer leakages(5–20%of the wastewater dry weatherflow;[44]).At the small scale,these can be thought of as point sources. But in the case of many leaks,as wefind,for example,in older sew-age systems,these should be defined as urban line sources[45].On the catchment scale,they can be seen as diffuse contaminant sources.In summary,the temporally and spatially variable inputs from different wastewater sources combined with the variable transport pathways result in highly variable concentrations and load patterns in all receiving waters within urban environments [46].To assess and quantify such a heterogeneous and dynamic ur-ban water system,sophisticated andflexible new investigation and monitoring strategies need to be developed.To date,monitor-ing strategies for groundwater at highly contaminated sites and river basins have mainly relied on conventional monitoring wells. Due to the above-and belowground urban infrastructure and the characteristics of urban pollution sources,hydrogeological investi-gation techniques developed for macropollutants cannot be easily applied in urban settings[11,12].In the following,we give an over-view on urban groundwater quantity and quality assessments and show the challenges which occur in thefield of urban hydrogeology.3.Assessment approaches and investigation,monitoring and modelling techniques in urban hydrogeology3.1.OverviewFor assessing urban groundwater quantity and quality,several types of information as well as evaluation approaches are required. The following sets the scene for the major issues being discussed in this review.3.1.1.Water balancing and waterflowThe knowledge on the amount of waterflowing into,within and from the groundwater is crucial for urban hydrogeological issues. Therefore,a profound understanding of groundwater recharge via different pathways is needed.Moreover,the groundwater travel time and the residence time in reactive zones are important parameters for the description of contaminant turnover on the lar-ger scale.3.1.2.Contaminant concentration distributions in time and spaceAdequate risk analysis of urban contaminants demands a pro-found knowledge of the concentration distributions in time and space.These data are needed to derive exposure scenarios for (eco)toxicological risk assessment.For the temporal scale,we pay special attention to toxicological acute effects[47],acting fast, i.e.within minutes,for example in the form of wastewater outflow which is characterised by toxic contaminant concentrations,bio-logically active substances,faecal germs or pathogenic bacteria. To investigate these acute influences,contaminant concentrations, as well as their frequency are of interest.This task spans adapted field methods on the one hand and a sound statistical analysis and presentation of the concentrations in time and space on the other hand.3.1.3.Contaminant loadsThe loads of contaminantsflowing into,within and out of an ur-ban groundwater body combine the information of waterflow and contaminant concentrations.Load determination within the groundwater allows the estimation of attenuation,e.g.when con-sideringflow through sequential control planes.Moreover,quanti-fying the contaminant load into and from groundwater allows the weighting of the relevance of different contaminant pathways.For toxicological assessments,contaminant loads are needed to under-stand cumulative effects on ecosystems.Cumulative effects result from gradual changes in the water bodies such as accumulation of nutrients and toxicants in,and release from,sediment and occur only after the cumulative changes rise above a critical threshold le-vel.Short-term changes and small time scales are not important for cumulative effects.The main interests here are the loads and sub-stancefluxes over long time spans.3.1.4.Implementation of holistic modelling approachesSince only part of the groundwater system can be measured, models of water and contaminantflow and transport are indis-pensable.The urban groundwater compartment interacts closely with the unsaturated zone,sewage systems and surface water. Depending on the task,groundwater models often have to be cou-pled to the other compartments.This can be done by loose cou-pling,where output of one model,e.g.a model of wastewater flow and exfiltration,is taken as input for the groundwater model.282M.Schirmer et al./Advances in Water Resources51(2013)280–291This task can become complex if different compartments are inter-acting and influencing each other in both directions.Here,fully coupled codes are needed.Such integral models allow for reduced error propagation and better uncertainty analyses but also bear the potential of over-parameterisation and non-uniqueness.We will briefly explain some assessments using modelling in this section and will return to integrated modelling approaches in the section ‘‘Integrated modelling techniques in urban hydrogeology’’.To summarise the developments of urban groundwater quan-tity and quality assessments,we will review important research projects over the last several years which investigated at least some of the above-mentioned problems of urban waterfluxes,con-taminant concentrations and their interactions.These can be sub-divided into qualitative and quantitative investigation methods. Qualitative methods are targeted tofind concentration patterns in groundwater to detect the urban influence(e.g., [23,25,26,30,48]).Quantitative methods aim at the calculation of waterfluxes and contaminant loads.For load quantification,qual-itative methods and water/mass balances have to be investigated together.Several groups studied certain aspects of urban ground-waterflow,as for example groundwater recharge beneath urban areas(e.g.,[7,8])or the interactions between sewer systems and groundwater(e.g.,[49,50]).More recent work targets integrative approaches to describe urban effects using macropollutants,such as PAHs,nitrate,phosphate and boron(e.g.,[33,51,52])or micro-pollutants,such as PPCPs(e.g.,[28,33,53]).In the following,we will analyse the methodologies used to assess urban water quality and quantity of several of these studies.3.2.Water balancing and waterflowThe water balance of urban areas is crucial when dealing with urban groundwater problems.Urban groundwater recharge is a complex and multi-faceted process,involving gains and losses from different water compartments.Often,a significant amount of water is imported into urban areas,becoming part of the city’s water balance and increases groundwater recharge as well as sur-face waterflow by untreated and treated wastewater releases [8,54].There is a large body of scientific literature dealing with water balances at different spatial and temporal scales.In general, the approaches can be divided into methods based on waterflux balancing,empirical GIS methods and holistic combined ap-proaches,for example,on the base of groundwater modelling and solute balancing.Moreover,a growing number of studies deal with the quantification of sewer leakages.3.2.1.Water balanceZhang and Kennedy[55]balanced waterflow within the city of Beijing,China,to estimate sustainable yield of the city’s aquifer. Half of the city’s water supply is provided by the aquifer and faced severely falling water levels in the last century.The water balance is based on municipal information on precipitation,water usage, leakages from mains and the sewage system and assumptions on evapotranspiration.Sustainable yield was estimated for the year 1990and2000and projected for2010and2015.Monte Carlo anal-ysis was used to account for the component uncertainties.Since 30–60%of the water budget is provided by wastewater sources, there are serious concerns on the quality of the groundwater resource.3.2.2.Empirical GIS methodsWessolek et al.[56]proposed empirical hydro-pedotransfer functions to estimate annual groundwater recharge from data on soil type,vegetation cover,precipitation and evapotranspiration. This approach explicitly defines transfer functions for urban land-use too.Here,surface sealing is taken into account and the authors proposed an infiltration coefficient for partly sealed surfaces(infil-tration through seam materials andfissures).Estimation accuracy of actual evapotranspiration and thus groundwater recharge in ur-ban areas is stated to be about±50mm aÀ1.Haase[57]estimated the water balance of the city of Leipzig, Germany,for four different land use configurations over the last 130years.Surface runoff,actual evapotranspiration and ground-water recharge were estimated on the basis of empirical methods; imported water was not taken into account.Although results are not validated with data on the groundwater surface or runoff development,the author could clearly show how urban sprawl leads to a modified water balance,with an increase of surface run-off.In combination with a decrease of evapotranspiration as a con-sequence of surface sealing,net groundwater recharge was only slightly decreased in the study period.Thomas and Tellam[14]proposed a sophisticated GIS based model of urban recharge and non-point source pollution and ap-plied it to the city of Birmingham,UK.The model is able to quantify spatially distributed runoff,interception and evapotranspiration. The authors stress the potential relevance of groundwater recharge from paved areas due to the lack of evapotranspiration in compar-ison with less sealed areas.Results of the recharge estimations are not validated with measurements.GIS-based empirical models spatially combine the parameters that control groundwater recharge in urban areas.The case studies of Haase[57]and Thomas and Tellam[14]are sophisticated in terms of the used parameter but lack the validation by the use of measurements.The proposed methodology of Wessolek et al.[56]for longer-term recharge estimation is promising due to the validation with several case studies.3.2.3.Holistic approachesYang et al.[58]combined a spread sheet model for groundwater recharge with a numerical MODFLOW groundwaterflow and transport model for the city of Nottingham,UK.Groundwater re-charge estimation took precipitation and leakages from water mains and the sewage system in13stress periods from1850–1995into account.Recharge and groundwaterflow was connected to the input and transport of chloride,sulphate and total nitrogen. Historical and more recent concentration measurements enabled a calibration of the recharge recharge did not change much over the study period(with wide confidence intervals)but contribution from the different sources shifted.Leakages from water mains were found to be the major current contributor to ur-ban recharge while6–13mm aÀ1is recharge by sewer leakages. Trowsdale and Lerner[59]studied the age distribution of ground-water with depth in the same aquifer in more detail.The basic hypothesis is that water characteristics at any point of the aquifer can be related back to the time and origin of recharge.In a simpli-fied approach a multi-tracer non-reactive transport model was ap-plied to groundwater quality data from a multi-level well.The results clearly show that today’s urban groundwater is degraded by a long history of changing land use and pollutant inputs.Mohrlok et al.[60]stated the need for physically-based models of recharge in urban areas but also stressed the strong influence of soil heterogeneity and non-linearity of processes.The authors pro-posed a combination of a water balance model especially for im-ported water with1D models for areal infiltration sources and 3D models for point source infiltration for unsaturated waterflow and solute transport.The authors applied this approach to the city of Rastatt,Germany.Soil types,soil layering and depth to ground-water were classified in GIS and a1D model of unsaturated water flow was applied.The3D-approach was found to be too computa-tional demanding and only applied for limited cases.This approach is capable to model groundwater recharge on a physical basis and provides travel time distributions through the unsaturated zone.M.Schirmer et al./Advances in Water Resources51(2013)280–291283Musolff et al.[27]balanced the annual waterflow of an urban drainage catchment within the city of Leipzig,Germany,on the ba-sis of loosely coupled models.Runoff to the sewage system and infiltrating groundwater were directly derived from measured catchment wastewater volumes.Sewer exfiltration was estimated on the basis of carbamazepine and bisphenol A concentrations in wastewater and groundwater.Areal groundwater recharge was modelled for classified soil profiles and different land use types using Hydrus1D.Daily groundwater recharge was used as input for a numerical3D groundwaterflow model.The derived annual water balance indicated the relevance of imported water:in addi-tion to the663mm aÀ1of precipitation,247mm aÀ1water was im-ported and returned as treated wastewater.The net groundwater recharge by the sewage system was negative–more water was infiltrated to the sewers than lost(depending on the position of the sewers with respect to the groundwater table).Although over-all surface sealing was high(25%fully sealed),only11%of the pre-cipitation discharges as storm water to the combined sewage system.Most likely,sealed surfaces are not always connected to the sewage system and,moreover,infiltration through cracks and joints is possible.Vazquez-Sune et al.[61]identified urban recharge sources for the city of Barcelona,Spain,by an end member mixing model. Using a large hydrochemical database for more than20years,eight different recharge sources could be distinguished on the basis of12 and8solute species.The authors showed that50%of the water in the aquifer originates from the water supply and sewage network. Storm water had a variable but locally major impact on the water resources.Jeppesen et al.[62]used a modified MODFLOW groundwater model to describe the urban water cycle of the city of Copenhagen, Denmark.While groundwater recharge is modelled by a1D root zone model.Water supply andflow in the combined sewage sys-tem is quantified on a grid base,interaction of groundwater and the sewage system is accounted for by the MODFLOW sewer pack-age.Calibration was done in a step-wise approach using time series of hydraulic heads with different focus(groundwater extraction, leakages from water supply)andfinally wastewater and stream-flow ing an extensive dataset on land use,climate and water extraction history,the authors came up with an urban water balance spanning the years1850–2003.The authors showed an in-crease of groundwater recharge in Copenhagen due to an increase in precipitation,balancing the urbanisation effects.Water main leakages made up half of the total recharge in the1950s and were reduced to3%by intensive pipe renovation.Variable methods are used to quantify urban waterflow includ-ing groundwater as well as wastewater.Bottom up approaches on the basis of water quality measurements(see[61])as well as top down approaches(see[58,60,62])and even mixed approaches (see[27])are known.In the same way as for the GIS-based re-charge calculations,parameter uncertainty remains a problem and validation of the results is only partly realised.Some of the studies stressed the shift of groundwater recharge sources in rela-tion to the city’s development although net recharge may not change too much[58,62].As a result there may not be a visual se-vere effect such as a reduction in surface water baseflow but a strong shift in the resulting groundwater quality.The temporal shifts in recharge sources are not taken into account in the pre-sented top down studies tracing back groundwater quality to re-charge sources by the use of mixing models[61,27].Both studies assume the present groundwater quality to be directly linked to present recharge source concentrations which may be justified for the presented shallow and young groundwater systems.The application and integration of groundwater models(see [27,58,62])shows that in general numerical models are imple-mented in the same way as in rural studies.The most important difference is the definition of the spatially and temporally variable upper boundary conditions.The reviewed studies show how to combine various data sources to derive the proper forcing of the models.3.2.4.Sewer leakagesSewer leakages are an important source of urban groundwater, especially affecting groundwater quality.Rutsch et al.[44]gave a thorough review of methods and results of sewer exfiltration esti-mation at different spatial scales.Different methods also lead to different units for the exfiltration rate:Larger scale assessments of-ten define leakages as a percent of the wastewater dry weather flow or as mm aÀ1.At the scale of single sewer lines,L dÀ1mÀ1of the sewer is used while single leaks are characterised by L dÀ1cmÀ2of the leak’s area.The authors propose a stepwise assessment of sewer leakages to groundwater.At the larger scale, the overall impact of leakages can be evaluated on the basis of groundwater modelling or measurements of the groundwater quality.On the smaller scale,direct measurements of leakages should be used.Rieckermann et al.[63,64]showed the potential of artificial tracer tests in the sewage system to quantify leakages with a sophisticated uncertainty assessment.Chisala and Lerner[65]reviewed recent literature on sewer leakage quantification.Indirect methods on the basis of water bal-ancing and solute balancing are suitable methods for larger scale assessments but suffer from a significant uncertainty.On the other hand,direct methods such as pressure testing and tracer tests are less uncertain but cannot be easily extrapolated to the entire sew-age system.The authors proposed a likely range of exfiltration of 0.01–0.1L sÀ1kmÀ1.Furthermore,the authors estimated spatially distributed sewer leakages for the city of Nottingham,UK,on the basis of total leakages of10mm aÀ1.The methods used relations between sewer density,sewer age and defects,as well as hydraulic heads between sewers and groundwater to distribute the total leakages over the city.Huge diversity of rates stresses both:meth-odological problems and variability of parameters and boundary conditions(e.g.state of the system,hydraulic gradients,conductiv-ity of the surroundings).3.3.Contaminant concentration distributions in time and space3.3.1.Finding target substances in urban groundwater contaminationAs we can imagine from the statements above,there is an over-whelming number of substances present in the urban water cycle at the macro-and micropollutant levels.Therefore,it would make sense to prioritise especially the variety of organic micropollutants for target substances to effectively monitor urban water resources. There are several concepts for this prioritization.Strauch et al.[66] and Schirmer et al.[67]focus on the use of indicator substances. These substances represent classes of chemicals with comparable physicochemical properties or comparable input pathways.Both studies make use of micropollutant indicators,such as carbamaze-pine and galaxolideÒ,but broaden the range by the use of inorganic wastewater indicators such as boron and the isotopic composition of nitrate.This allows for the use of indicator substances represent-ing inputs by specific pathways which may not be of urgent toxico-logical concern[68,69].Recently,persistent artificial sweeteners, such as acesulfame,were found to be a good chemical marker for wastewater inputs to urban groundwater and surface water[70–73].To quantitatively use such markers for the estimation of,for example,sewer leakages,the concentration variability in the source wastewater and the difficulty to determine representative concentrations have to be kept in mind[43,46].Another way to prioritise substances is based on a simple com-bination of criteria such as consumption,persistence,potential for bioaccumulation or toxicity for each substance.A good overview is284M.Schirmer et al./Advances in Water Resources51(2013)280–291。
水污染的三个方面原因英语作文300字
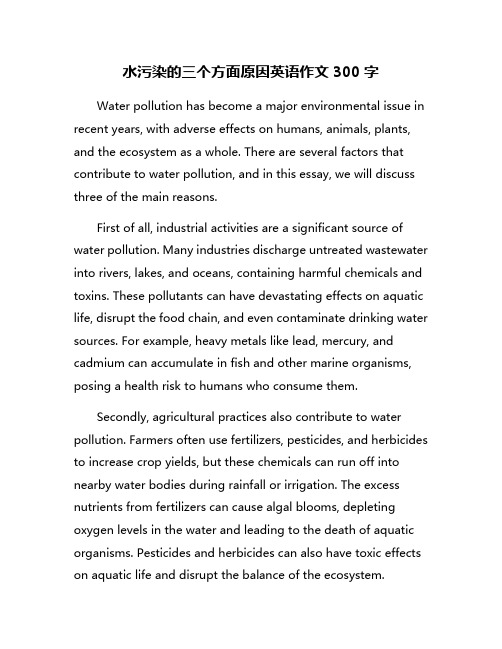
水污染的三个方面原因英语作文300字Water pollution has become a major environmental issue in recent years, with adverse effects on humans, animals, plants, and the ecosystem as a whole. There are several factors that contribute to water pollution, and in this essay, we will discuss three of the main reasons.First of all, industrial activities are a significant source of water pollution. Many industries discharge untreated wastewater into rivers, lakes, and oceans, containing harmful chemicals and toxins. These pollutants can have devastating effects on aquatic life, disrupt the food chain, and even contaminate drinking water sources. For example, heavy metals like lead, mercury, and cadmium can accumulate in fish and other marine organisms, posing a health risk to humans who consume them.Secondly, agricultural practices also contribute to water pollution. Farmers often use fertilizers, pesticides, and herbicides to increase crop yields, but these chemicals can run off into nearby water bodies during rainfall or irrigation. The excess nutrients from fertilizers can cause algal blooms, depleting oxygen levels in the water and leading to the death of aquatic organisms. Pesticides and herbicides can also have toxic effects on aquatic life and disrupt the balance of the ecosystem.Lastly, urbanization and population growth have led to increased levels of water pollution. Urban areas produce large amounts of sewage and solid waste, which can end up in water bodies if not properly treated or disposed of. The lack of adequate wastewater treatment facilities in many cities means that untreated sewage is often discharged directly into rivers and oceans, contaminating the water and posing a risk to public health. Additionally, urban runoff from roads, parking lots, and other impervious surfaces can carry pollutants like oil, heavy metals, and trash into storm drains, which eventually flow into waterways.In conclusion, water pollution is a complex issue with multiple causes, including industrial activities, agriculture, and urbanization. It is essential for governments, industries, and individuals to take proactive measures to prevent further contamination of our water resources. By implementing regulations, promoting sustainable practices, and raising awareness about the importance of clean water, we can work together to protect the environment and ensure a healthy future for generations to come.。
河流和湖泊的重要性英语作文
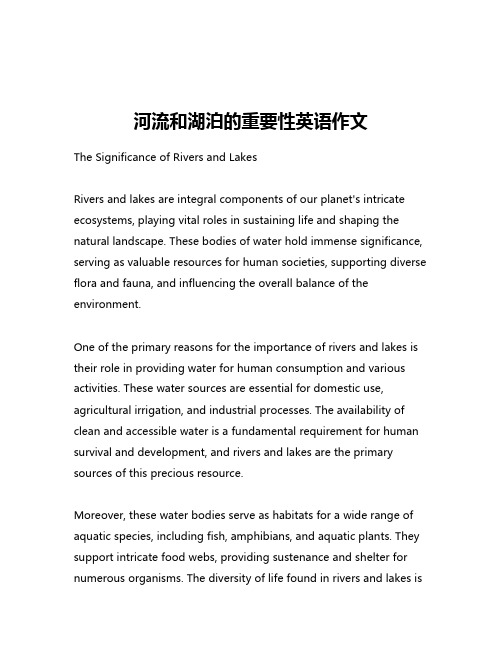
河流和湖泊的重要性英语作文The Significance of Rivers and LakesRivers and lakes are integral components of our planet's intricate ecosystems, playing vital roles in sustaining life and shaping the natural landscape. These bodies of water hold immense significance, serving as valuable resources for human societies, supporting diverse flora and fauna, and influencing the overall balance of the environment.One of the primary reasons for the importance of rivers and lakes is their role in providing water for human consumption and various activities. These water sources are essential for domestic use, agricultural irrigation, and industrial processes. The availability of clean and accessible water is a fundamental requirement for human survival and development, and rivers and lakes are the primary sources of this precious resource.Moreover, these water bodies serve as habitats for a wide range of aquatic species, including fish, amphibians, and aquatic plants. They support intricate food webs, providing sustenance and shelter for numerous organisms. The diversity of life found in rivers and lakes isa testament to the delicate balance of these ecosystems and their importance in maintaining the overall health of the natural world.Beyond their ecological significance, rivers and lakes hold immense cultural and recreational value. Many civilizations have historically settled along the banks of these water bodies, utilizing them for transportation, trade, and cultural festivities. Rivers and lakes have also become popular destinations for recreational activities such as fishing, boating, and swimming, allowing people to connect with nature and enjoy the serenity these water bodies provide.Furthermore, rivers and lakes play a crucial role in the water cycle, the continuous movement of water on, above, and below the Earth's surface. These water bodies act as reservoirs, storing and regulating the flow of water, which is essential for maintaining the balance of the hydrological cycle. This cycle, in turn, influences weather patterns, climate, and the overall health of the environment.However, the importance of rivers and lakes is not without its challenges. The increasing human demand for water, coupled with the effects of climate change and environmental degradation, pose significant threats to the integrity of these water bodies. Pollution, overexploitation, and the disruption of natural habitats can lead to the depletion and deterioration of rivers and lakes, with far-reaching consequences for both human and ecological communities.To address these challenges, it is crucial that we recognize the importance of rivers and lakes and take collective action to protect and preserve these vital resources. This may involve implementing sustainable water management practices, reducing pollution, and restoring degraded ecosystems. By prioritizing the conservation of rivers and lakes, we can ensure that these water bodies continue to provide essential services and maintain the delicate balance of the natural world.In conclusion, rivers and lakes are indispensable components of our planet, holding immense significance for human societies, ecological systems, and the overall well-being of the environment. Their importance cannot be overstated, and it is our responsibility to safeguard these precious resources for present and future generations. By recognizing the value of rivers and lakes and taking proactive steps to protect them, we can work towards a more sustainable and harmonious coexistence with the natural world.。
水污染英语高中作文译文
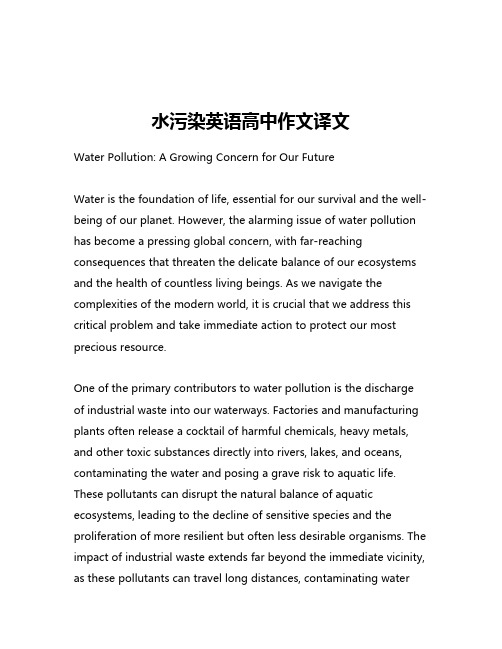
水污染英语高中作文译文Water Pollution: A Growing Concern for Our FutureWater is the foundation of life, essential for our survival and the well-being of our planet. However, the alarming issue of water pollution has become a pressing global concern, with far-reaching consequences that threaten the delicate balance of our ecosystems and the health of countless living beings. As we navigate the complexities of the modern world, it is crucial that we address this critical problem and take immediate action to protect our most precious resource.One of the primary contributors to water pollution is the discharge of industrial waste into our waterways. Factories and manufacturing plants often release a cocktail of harmful chemicals, heavy metals, and other toxic substances directly into rivers, lakes, and oceans, contaminating the water and posing a grave risk to aquatic life. These pollutants can disrupt the natural balance of aquatic ecosystems, leading to the decline of sensitive species and the proliferation of more resilient but often less desirable organisms. The impact of industrial waste extends far beyond the immediate vicinity, as these pollutants can travel long distances, contaminating watersources that serve entire communities.Another significant contributor to water pollution is the runoff of agricultural chemicals, such as pesticides and fertilizers, from farmlands. When these substances are applied in excessive amounts or during heavy rainfall, they are washed away, ultimately finding their way into nearby water bodies. The presence of these chemicals in our waterways can have devastating effects on aquatic life, leading to the disruption of food chains, the decline of sensitive species, and the contamination of water sources used for drinking, irrigation, and recreational activities.Urbanization and the rapid growth of human settlements have also played a significant role in exacerbating water pollution. The improper disposal of domestic waste, including sewage, plastics, and other household items, can lead to the contamination of nearby water sources. Additionally, the increased use of automobiles and the subsequent release of oil, grease, and other automotive fluids into storm drains and waterways further compound the problem.The consequences of water pollution are far-reaching and can have profound impacts on both human and environmental health. Contaminated water can lead to the spread of waterborne diseases, such as cholera, dysentery, and typhoid, which disproportionately affect vulnerable populations with limited access to clean water andsanitation. Moreover, the accumulation of pollutants in aquatic ecosystems can disrupt the delicate balance of food webs, leading to the decline of important species and the disruption of essential ecosystem services.In recent years, the global community has made strides in addressing the issue of water pollution, with the implementation of various policies, regulations, and technological solutions. However, the pace of progress has been slow, and much more needs to be done to effectively tackle this pressing environmental challenge.One of the key strategies in combating water pollution is the development and implementation of robust wastewater treatment systems. By investing in advanced water treatment technologies, we can effectively remove harmful contaminants from industrial and municipal effluents before they are discharged into our waterways. This not only protects the environment but also ensures the availability of clean water for various human and agricultural uses.Promoting sustainable agricultural practices is another crucial step in mitigating water pollution. Farmers and agricultural producers can adopt precision farming techniques, such as the targeted application of fertilizers and pesticides, to reduce the runoff of these substances into nearby water bodies. Additionally, the implementation of buffer zones and the preservation of natural wetlands can help to filter andtrap pollutants before they reach our water sources.Educating the public and raising awareness about the importance of water conservation and pollution prevention is also essential. By empowering individuals to make informed choices, such as reducing the use of single-use plastics, properly disposing of household waste, and advocating for stricter environmental regulations, we can collectively contribute to the solution and create a more sustainable future.Ultimately, addressing the issue of water pollution requires a multi-faceted approach that involves collaboration between governments, industries, environmental organizations, and individual citizens. By taking decisive action and investing in long-term solutions, we can protect our precious water resources, safeguard the health of our ecosystems, and ensure a sustainable future for generations to come.。
水源污染的坏处英语作文
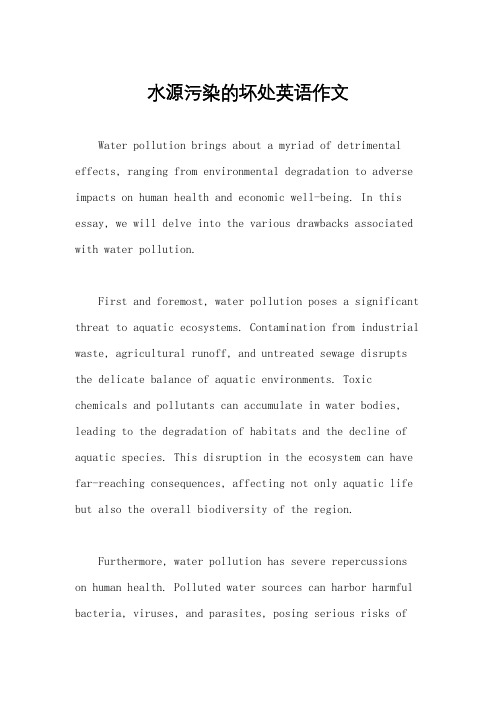
水源污染的坏处英语作文Water pollution brings about a myriad of detrimental effects, ranging from environmental degradation to adverse impacts on human health and economic well-being. In this essay, we will delve into the various drawbacks associated with water pollution.First and foremost, water pollution poses a significant threat to aquatic ecosystems. Contamination from industrial waste, agricultural runoff, and untreated sewage disrupts the delicate balance of aquatic environments. Toxic chemicals and pollutants can accumulate in water bodies, leading to the degradation of habitats and the decline of aquatic species. This disruption in the ecosystem can have far-reaching consequences, affecting not only aquatic life but also the overall biodiversity of the region.Furthermore, water pollution has severe repercussions on human health. Polluted water sources can harbor harmful bacteria, viruses, and parasites, posing serious risks ofwaterborne diseases such as cholera, typhoid, and dysentery. Contaminants like heavy metals and pesticides can also find their way into drinking water supplies, causing chronic health problems including cancer, neurological disorders, and reproductive issues. Access to clean and safe drinking water is essential for public health, and water pollution jeopardizes this fundamental necessity.Moreover, water pollution has detrimental effects on economies and livelihoods. Contaminated water sources can render agricultural lands infertile and unfit for cultivation. Crop yields may decline due to the presence of pollutants in irrigation water, leading to economic losses for farmers and food shortages for communities. Additionally, industries reliant on clean water for manufacturing processes may face increased operationalcosts due to the need for advanced water treatment technologies. Tourism, another vital sector of many economies, can suffer from water pollution as polluted beaches and water bodies deter visitors, leading to revenue losses for local businesses.In addition to these immediate impacts, water pollution also exacerbates broader environmental issues such as climate change. Polluted water bodies contribute to the emission of greenhouse gases through processes like eutrophication and anaerobic decomposition. Moreover, the degradation of aquatic ecosystems disrupts the natural carbon and nutrient cycles, further exacerbating climate change and its associated effects such as extreme weather events and rising sea levels.Addressing water pollution requires concerted efforts at local, national, and international levels. Implementing stringent regulations to control industrial discharges and agricultural runoff is crucial for mitigating pollution at its source. Investing in wastewater treatmentinfrastructure and promoting sustainable agricultural practices can help minimize contamination of water bodies. Additionally, raising public awareness about the importance of water conservation and pollution prevention is essential for fostering a culture of environmental stewardship.In conclusion, water pollution poses multifacetedchallenges with far-reaching implications for ecosystems, human health, and economies. Addressing this issue requires collaborative action and a commitment to sustainable practices to safeguard water resources for present and future generations.。
水资源短缺的英语作文
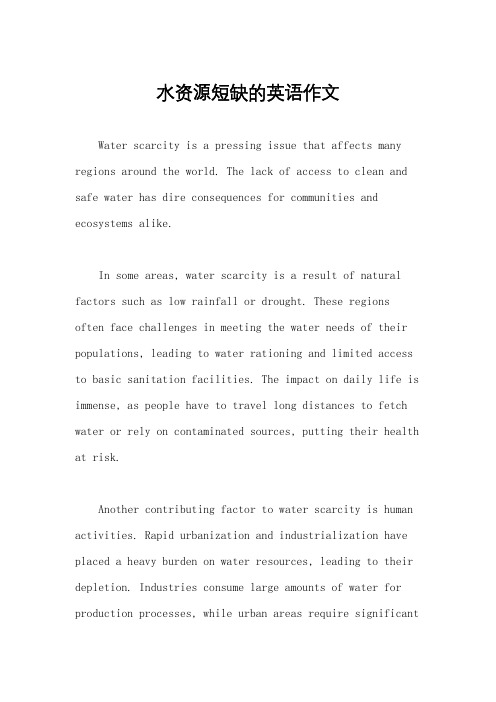
水资源短缺的英语作文Water scarcity is a pressing issue that affects many regions around the world. The lack of access to clean and safe water has dire consequences for communities and ecosystems alike.In some areas, water scarcity is a result of natural factors such as low rainfall or drought. These regions often face challenges in meeting the water needs of their populations, leading to water rationing and limited access to basic sanitation facilities. The impact on daily life is immense, as people have to travel long distances to fetch water or rely on contaminated sources, putting their health at risk.Another contributing factor to water scarcity is human activities. Rapid urbanization and industrialization have placed a heavy burden on water resources, leading to their depletion. Industries consume large amounts of water for production processes, while urban areas require significantamounts for domestic use. The overexploitation of water sources has led to their depletion, further exacerbating the problem.Furthermore, pollution is a significant concern when it comes to water scarcity. The discharge of untreated industrial waste and agricultural runoff into water bodies contaminates them, making the water unfit for human consumption and irrigation. This not only reduces the availability of clean water but also harms aquatic life and ecosystems, disrupting the delicate balance of nature.Climate change is also a contributing factor to water scarcity. Rising temperatures and changing precipitation patterns affect the availability of water resources. Some regions experience more frequent and intense droughts, while others face increased flooding events. Both scenarios have adverse effects on water availability and exacerbate the challenges faced by communities already struggling with water scarcity.The consequences of water scarcity are far-reaching.Beyond the immediate impact on human health and well-being, it also affects agriculture and food security. Without access to sufficient water for irrigation, crop yields decrease, leading to food shortages and higher prices. This, in turn, affects the livelihoods of farmers and exacerbates poverty and inequality.In conclusion, water scarcity is a complex issue with various causes and consequences. It is essential to address this problem through sustainable water management practices, including conservation, efficient use, and the developmentof alternative water sources. By taking immediate action,we can ensure the availability of clean and safe water for future generations.。
- 1、下载文档前请自行甄别文档内容的完整性,平台不提供额外的编辑、内容补充、找答案等附加服务。
- 2、"仅部分预览"的文档,不可在线预览部分如存在完整性等问题,可反馈申请退款(可完整预览的文档不适用该条件!)。
- 3、如文档侵犯您的权益,请联系客服反馈,我们会尽快为您处理(人工客服工作时间:9:00-18:30)。
Effects of irrigation on water balance,yield and WUE of winter wheat in the North China PlainHong-Yong Sun a,b,c,*,Chang-Ming Liu a,b,Xi-Ying Zhang a,Yan-Jun Shen a,Yong-Qiang Zhang aa Center for Agricultural Resources Research,Institute of Genetic and Developmental Biology,CAS,286Huaizhong Road,Shijiazhuang050021,Hebei Province,Chinab Institute of Geographical Sciences and Natural Resources Research of CAS,Beijing100101,Chinac Graduate School of Chinese Academy of sciences,Beijing100049,China1.IntroductionWinter wheat(Triticum aestivum L.)is a major crop in theNorth China Plain(NCP)and its food production accounts forabout71%of wheat production in China.However,water isthe most important limiting factor for wheat production.Inthe winter wheat season precipitation ranges from50mm indry years to150mm in wet years,with an average annualprecipitation of115.9mm.The total water consumption isabout453mm for winter wheat without water deficit,whichgreatly exceeds precipitation(Liu et al.,2002).To achievehigher grain yields(GY),farmers in this region pumpgroundwater to irrigate winter wheat to offset the ET deficit.More than80%of the water resources from surface runoffand groundwater have been exploited for irrigation.Theexcessive exploitation of groundwater resources fromshallow and deep aquifers in this region has caused thewater table to fall and created many other environmentalproblems within the plain(Liu and Wei,1989).The ground-water table is falling steadily at the rate of about1m per yearand the main factors leading to this fall are the expandingwheat area which is irrigated with groundwater and the lowwater-use efficiency(WUE)of crops(Hu et al.,2002).So it isnow very important to control or decrease the falling rate ofgroundwater table by reducing the amount of irrigation inkeeping with high GY.a g r i c u l t u r a l w a t e r m a n a g e m e n t85(2006)211–218a r t i c l e i n f oArticle history:Accepted30April2006Published on line15June2006Keywords:Triticum aestivum L.EvapotranspirationIrrigationWater-use efficiencyGrain yielda b s t r a c tLimited precipitation restricts yield of winter wheat grown in the North China Plain(NCP).Irrigation experiments were conducted during different growing stages of winter wheat(Triticum aestivum L.)at Luancheng agro-ecology systems station of the Chinese Academy ofSciences during1999/2000,2000/2001and2001/2002to identify suitable irrigation schedulesfor winter wheat.The aim was also to develop relationships between seasonal amounts ofirrigation and yield,water-use efficiency(WUE),irrigation water-use efficiency(WUEi),netwater-use efficiency(WUEet)and evapotranspiration(ET).A comparison of irrigationschedules for wheat suggested that for maximum yield in the NCP,300mm is an optimalamount of irrigation,corresponding to an ET value of426mm.Results showed that withincreasing ET,the irrigation requirements of winter wheat increase as do soil evaporationbut excessive amounts of irrigation can decrease grain yield,WUE,and WUEi.These resultsindicate that excessive irrigation might not produce greater yield or optimal economicbenefit,thus,suitable irrigation schedules must be established.#2006Elsevier B.V.All rights reserved.*Corresponding author.Tel.:+8631185814362;fax:+8631185815093.E-mail addresses:hysun@,sunhy.04b@(H.-Y.Sun).a v a i l ab l e a t w w w.s ci e nc e di r e ct.comj o u r n a l h o m e p a g e:w w w.e l s e v i e r.c o m/l o c a t e/a g w a t0378-3774/$–see front matter#2006Elsevier B.V.All rights reserved.doi:10.1016/j.agwat.2006.04.008The effects of irrigation on crop production are usually quantified using crop water production functions which relate crop yield to amounts of water applied(Yaron and Bresler, 1983;English,1990;English and Raja,1996).The rational irrigation can significantly increase the GY(Hagan et al.,1967; Gajri et al.,1997;Huang et al.,2004).Hagan et al.(1967)also asserted that excessive irrigation delays the maturity,harvest-ing and decreased GY.Jin et al.(1999)reported that excessive irrigation led to a decrease of crop WUE and that effective deficit irrigation may result in higher production and WUE.On the contrary,Olesen et al.(2000)showed that the effect of irrigation on wheat yield was almost solely due to increased transpiration,while WUE and harvest index remained unaffected.Kang et al.(2002)indicated that the responses of grain yield and WUE to irrigation varied considerably due to differences in soil water contents and irrigation schedules. Singh et al.(1991)concluded that the impact of limited irrigation and soil water deficit on crop yield or WUE depends on the particular growth stage of the crop.Many studies have shown that the relationship between wheat yield and seasonal ET is linear(Singh,1981;Mogenson et al.,1985;Steiner et al.,1985;Musick et al.,1994;Zhang and Oweis,1999;Zhang et al.,1999a,b).Though,Aggarwal et al. (1986)showed the curvilinear result,Kang et al.(2002)reported that relationships between seasonal ET and grain yield or WUE could be described by quadratic functions,While relationships between ET and GY have been widely used for water-saving purposes in water deficit areas as a guideline for irrigation, they cannot explain the effects of timing applications.So there has been an ongoing effort to reveal relationships between GY of winter wheat and soil water balance(especially irrigation) and water-use efficiency.In this paper we discuss the effects of irrigation at different growth stages on WUE,GY,ET and its components.On the basis of our results,guidelines are provided for farmers and irrigation agencies to achieve water-saving irrigation practice and efficient use of water resources for wheat production in the NCP.So objectives of this study are:(1)To investigate the significance of irrigation as a compo-nents of soil water balance.(2)To discover the effects of irrigation on ET,soil evaporation(E),E/ET and GY.(3)To interpret the impact of irrigation on irrigation water-useefficiency(WUEi),net water-use efficiency(WUEet)and WUE.2.Materials and methodsFrom1999to2002,experiments were performed at Luancheng experimental station(378530N,1148410E,with elevation at 50.1m),located in the high-yielding area of NCP and being one of the agricultural ecosystem stations of the Chinese Academy of Sciences.Local soil characteristics and parameters are shown in Table1,indicating fertile topsoil and plenty of organic matter in the loam soil.The climate is of the temperate semi-arid monsoon type,with a mean annual temperature of 12.28C,mean annual global radiation of5240MJ/m2,mean annual precipitation of475.4mm,and about75%of which occurs from late June to September.The distribution of rainfall in the three growing seasons is shown in Table2.The difference of total rainfall in the different growing seasons was larger,while the distribution also was uneven in the different growing stage and little rainfall occurred between December and March.There was about20mm rainfall before the harvest in2000–2001growing season.There are sixteen5mÂ10m plots divided by concrete walls which were built in1995forfive treatments of irrigation schedules.The concrete walls are24.5cm thick and extend 1.5m beneath the surface,in accordance with specifications set by the Food and Agricultural Organization.Winter wheat,Gaoyou503,was sown at the beginning of October and harvested in mid-June of the following year.The seed rate was150kg haÀ1,with a20cm row width.Before sowing,chemical fertilizers of N and P at rate of130kg haÀ1N and160kg haÀ1P2O5were applied and cultivation was done to mix the fertilizers with top soil manually.Each plot was also irrigated with about80mm of water to ensure better seedling establishment.The straw of previous crop(maize)was removed and there were not any straw or plant residues to mulch the plots.Other management practice was consistent with typicalfield conditions.Thefive irrigation schedules are shown in Table3and each treatment was replicated three times(treatment E had four replicates).a g r i c u l t u r a l w a t e r m a n a g e m e n t85(2006)211–2182121Soil depth0–20cm20–35cm35–65cm65–90cm90–145cm145–170cm170–190cm Texture Sandy loam Sandy loam Light loam Medium loam Light clay Light clay Sandy clay BD(g/cm3) 1.41 1.51 1.47 1.51 1.54 1.64 1.59FC(vol.%)36.434.933.334.334.439.038.1WP(vol.%)9.611.413.913.913.013.916.4BD:bulky density;FC:field capacity;WP:wilting point.2Seasons October November December January February March April May June Total rainfall 1999–200020.7 6.10.211.400.8 2.511.8053.5 2000–200153.78.408.1 6.90.523.320.218.2139.3 2001–20029.4100.4 1.20 5.530.145.7 6.3108.6Volumetric soil moisture was measured with a neutron probe(IH-II,Institute of Hydrology,UK)in access tubes located at the center of each of the16experimental plots down to 180cm at20cm intervals at about every5days.Precipitation was recorded at a standard weather station about100m from the plots.The irrigation application to each plot was measured using a water meter which was installed at the hydrant of a low-pressure tube water transportation system.GY of winter wheat was sampled from the3mÂ8m portion in the central area of each plot and the plant sampling measurements were performed in the surrounding area of each plots.Biomass was calculated by weighing air-dried harvest samples.Soil evaporation(E)beneath the winter wheat canopy was estimated by daily weighing of three micro-lysimeters(MLS), which were placed between two rows.MLS contain small isolated volumes of bare soil mountedflush with or slightly above the soil surface(Daamen et al.,1993)and these were weighed daily(or more frequently)to determine water loss using electronic balances with0.001kg precision.The MLS cylinders were150mm long,120mm diameter and with a 2mm thick wall.They were constructed of PVC with the bottom beingfixed using plastic adhesive tape.To keep soil moisture in the MLS in agreement with outside conditions,the original soil in the instruments was changed every2or3days, and after rain or irrigation it was changed immediately(Sun et al.,2002).ET was calculated using the soil water balance equation for the growing season and for individual growth periods as follows:ET¼SWDþPþIÀDþW gÀR(1)where ET is evapotranspiration(mm),SWD the soil water depletion in the measured soil depth during the growing stage, P rainfall(mm),I irrigation applications(mm),D soil water drainage(mm),R surface runoff(mm),W g water used by crop through capillary rise from groundwater(mm).When the groundwater table is lower than4m below the ground surface, W g is negligible(Liu and Wei,1989).There usually is no runoff in the area,so R was also ignored.Drainage was estimated as follows using a recharge coefficient(a)multiplied by the amount of irrigation or the effective rainfall(mm):D¼a I(2)The recharge coefficient(a)depends on soil texture and on the amount of irrigation.The coefficient ranges from0.1for clay soil to0.3for sandy soil,which was determined by monitoring the change of groundwater table after irrigation was applied in a large area(Ministry of Geology and Mineral Resources,1986).Values of a was taken to be0.1for irrigation amounts<90mm,0.15for irrigation between90and250mm, and0.2for irrigation250mm for the soils of this experimental condition.Water-use efficiency was calculated as(Hussain et al., 1995):WUE¼GYET(3)where WUE(kg mÀ3)is the water-use efficiency for the GY (kg mÀ2)and ET(m)is calculated as in Eq.(1).Net water-use efficiency(WUEet)and irrigation water-use efficiency(WUEi)can be written as follows,respectively(Bos, 1985):WUEet¼ðY iÀY dÞðET iÀET dÞ(4) WUEi¼ðGY iÀGY dÞI i(5)where GY i is the yield and ET i is the ET for irrigation level i, GY d is the grain yield and ET d is the ET for an equivalent dry land or rain-fed plot,and I i is the amount of irrigation applied for level i.In most cases,GY d would be zero or small in fully rain-fed conditions.In our experiment,treatment E is not irrigated after spring so this is regarded as a rain-fed treat-ment.WUEet can be regarded as net ET efficiency because it is based on the yield produced above the rain-fed yield divided by net ET difference for the irrigated crop.In our experiments all treatments were irrigated before winter dormancy so that the winter wheat could survive the cold winter.Analysis of variance(ANOVA)was used to test the difference in ET,I,SWD,E and WUE between different treatments.Mean comparisons were made by the LSD(the least significant difference)method with P<0.05.The ana-lyses were conducted using the SPSS program.3.Results and discussion3.1.Irrigation andfield water balanceIrrigation is one of the key factors affecting whether actual ET is close to the potential ponents of soil water balance as influenced by different irrigation schedules in the threea g r i c u l t u r a l w a t e r m a n a g e m e n t85(2006)211–2182133Treatments Winter dormancy Recovering Stem-elongation Heading Grain-fillingA 1.0–0.80.80.8B 1.00.8–0.80.8C 1.00.80.80.8–D 1.0 1.0 1.0 1.0 1.0E 1.0––––a‘‘–’’shows no irrigation applied;1.0and0.8means the ratio of u/uFC.crop seasons are shown in Table 4.ET of different treatments ranged from 200to 460mm in 1999/2000,240to 440mm in 2000/2001and 260to 445mm in 2001/2002.The most irrigated treatment D gave the maximum ET,and the least irrigated treatment E had the lowest ET.The results indicated ET of winter wheat was greatly affected by irrigation application.The SWD in Table 4showed the depletion of soil water storage during the whole growing season of winter wheat.It ranged from 16to 82.9mm.The SWD for the D treatment was the least among all the treatments,indicating irrigation could meet the needs of winter wheat.The SWD for the C treatment was the most,because it consumed the largest water amount during the grain-filling period.However,the SWD for the least irrigated treatment E was not the largest.The reason might be that under this treatment winter wheat was in serious water stress condition and both its canopy and its underground root system were restricted.The root might not be able to go deep to uptake soil water stored there that limited its soil water utilization.The SWD of treatments A and B was not significant difference (P =0.05).Percolation from the root zone was also one of the important components in soil water balance.The difference of drainage amount between treatments depended on the amount of total irrigation.Treatment D had the largestdrainage amount and treatment E had the least amount.The difference between irrigation and drainage would directly influenced the groundwater table declining rate in the region.Fig.1shows the relationships between irrigation and ET.They were linearly correlated with the increasing in irrigation,ET increased.ET was driven by meteorological factors,crop factors and soil factors and which is not only water consuming process but also an energy consuming process.In order to clarify the effects of irrigation on ET,regression analysis was carried out.Significant relationship (P =0.01)existed between irrigation water amount with ET and the relations were described with the following equation:ET ¼0:7361I þ178:88;R 2¼0:8893(7)where ET is evapotranspiration (mm)and I the total irrigation water applied in the whole growing period of winter wheat (mm).3.2.Impacts of irrigation on evaporation and transpirationET,the process by which water moves into the atmosphere through plants and soil,consists of soil evaporation (E )and plant transpiration (T ),is expressed by ET ¼E þT(8)Table 5shows the evaporation beneath the winter wheat canopy (Table 5).There were significant differences (P =0.05)in soil evaporation among the treatments.The evaporation in D treatments was the highest and in E treatment was the lowest.The reason might be that under larger irrigation amounts soil surface was wetter which promoted higher soil evaporation.The biggest differences between the highest and the lowest soil evaporation were 32mm in 1999/2000,34mm in 2000/2001and 42mm in 2001/2002.Generally with the increase in irrigation,E was increased.But the evaporation beneath winter wheat canopy among the different irrigationa g r i c u l t u r a l w a t e r m a n a g e m e n t 85(2006)211–218214419992002YearsTreatmentsRainfall (mm)Irrigation (mm)SWD (mm)Drainage (mm)ET (mm)1999–2000A 53.5284.565.7Æ15.2ab 20.5383.2Æ11.7bc B 323.961.1Æ5.8ab 17.1421.4Æ20.6b C 247.982.9Æ19.9a 10.8373.4Æ21.4c D 404.842.2Æ12.6bc 36.5464.0Æ35.1a E 80.066.5Æ18.5ab 8.0192.0Æ16.5d 2000–2001A 139.3272.716.0Æ8.8ns 11.0417.0Æ70.3a B 234.016.0Æ8.0ns 20.5368.8Æ11.2a C 230.033.3Æ17.3ns 17.1385.5Æ29.5a D 308.720.4Æ15.0ns 24.7443.7Æ70.8a E 80.030.0Æ7.6ns 8.0241.3Æ22.5b 2001–2002A 108.6249.358.5Æ10.3b 18.6397.9Æ36.4b B 247.571.1Æ20.9ab 20.7406.4Æ16.1ab C 250.782.4Æ5.9a 10.7431.0Æ11.8ab D 354.113.7Æ5.3c 31.4444.9Æ25.5a E80.079.1Æ11.7a8.0259.7Æ11.7cLetters indicate statistical significance at P =0.05level within the same column,and ‘‘a’’,‘‘b’’,‘‘c’’and so on show the statistical difference from the highest to the lowest.SWD represents soil profile depletion.aValues are means of three replicates (treatment E with fourreplicates).Fig.1–Relationships between evapotranspiration (ET)and irrigation in three crop seasons.level was similar between stem elongation and maturation for the plant factors(Sun et al.,2004).The ratios of E to ET were different and they ranged from 30%to56%in1999/2000,32%to45%in2000/2001and24%to 38%in2001/2002,respectively.E/ET of treatment E was the highest and the average ratio was about46%in three seasons. The average ratios of the other treatments ranged from31.3% to33.1%.High ratio of E/ET in treatment E was due to its smaller canopy coverage,and especially after rainfall E was quite bigger than that of other treatments.The above results indicated that E/ET for the whole growing period of winter wheat was more than30%with the highest ratio of E/ET occurring at the beginning of the growing season,after stem-elongation;it was getting less due to the canopy development.However,the absolute amount of evaporation was still large at the growing phases when plant transpiration consumed most of water(Liu et al.,2002).So how to decrease the soil evaporation and make it available for transpiration through the plant is an important way to save water.Some experiments conducted at the station showed that straw mulching was an effective way to reduce soil evapora-tion(Zhang et al.,1994,2005).But for winter crops the existing of straw on soil surface slowed down soil temperature rising after winter,which affected crop growth(Chen et al.,2005). Another method to reduce soil evaporation was to hoe the soil surface that cut down the soil moisture transfer to soil surface. According to Zhang et al.(1998),the best time to hoe soil was in spring when winter wheat begins to recover after winter dormancy.a g r i c u l t u r a l w a t e r m a n a g e m e n t85(2006)211–2182155Seasons Treatments Evapotranspiration(ET)(mm)Evaporation(E)(mm)Transpiration(T)(mm)E/ET(%)1999–2000A383.2129.9c253.333.9c B421.4134.9b286.532.0bC373.4128.9c244.534.5dD464.0140.4a323.630.3eE192.0108.0d84.056.3a2000–2001A417.0135.7b281.332.5d B368.8138.1b230.737.4bC385.5137.7b247.835.7cD443.7143.0a300.732.2eE241.3108.7c132.645.1a2001–2002A397.9121.9b276.030.6bc B406.4121.1b285.329.8cC431.0104.8c326.224.3dD444.9139.9a305.031.4bE259.797.9d161.937.7aLetters indicate statistical significance at P=0.05level within the same column,with‘‘a’’,‘‘b’’,‘‘c’’and so on showing the statistical difference from the highest to the lowest.a Values are means of three replicates(treatment E with four replicates).6Year Treatment Irrigation(mm)Grain yield(kg haÀ1)WUEi(kg mÀ3)WUEet(kg mÀ3)WUE(kg mÀ3)1999–2000A284.55467Æ179a0.67ab0.50b 1.43bc B323.95487Æ252a0.60b0.54ab 1.31cC247.95584Æ151a0.82a0.49c 1.50bD404.85306Æ64a0.43c0.59a 1.14dE80.03552Æ172b–– 1.83a2000–2001A272.74893Æ172a0.57ab0.42ns 1.19ab B234.04965Æ142a0.54ab0.35ns 1.33abC230.05177Æ276a0.80a0.37ns 1.34abD308.74972Æ96a0.53b0.46ns 1.12bE80.03328Æ80b–– 1.38a2001–2002A249.34299Æ174a0.31ab0.35ns 1.08b B247.54431Æ79a0.37a0.36ns 1.09bC250.74417Æ165a0.36a0.40ns 1.03bD354.14333Æ137a0.23b0.42ns0.97bcE80.03526Æ52b–– 1.36aLetters indicate statistical significance at P=0.05level within the same column,with‘‘a’’,‘‘b’’,‘‘c’’and so on showing the statistical difference from the highest to the lowest.a Values are means of three replicates(treatment E with four replicates).3.3.Irrigation and WUETable 6shows the WUEi,WUEet,and WUE of the different irrigation treatments in the three winter wheat seasons from 1999to 2002.WUEi ranged from 0.23to 0.82kg m À3and the trend was similar among the treatments for the three seasons and their difference was significant at P =0.05The WUEi of the most irrigated treatment D was the lowest and the moderately irrigated treatment C was the highest.For treatment C the water deficit occurred during grain-filling when winter wheat was not sensitive to water stress (Zhang et al.,1999a,b,2003).No significant difference was found between treatments A and B.This result was consistent with the findings of Li et al.(1995)in the Loess Plateau,who reported that WUEi decreased with increasing in irrigation and because grain yield did not increase linearly with irrigation,excessive irrigation even decreased grain production.WUEet ranged from 0.35to 0.59kg m À3with treatment D at the highest.Increasing in the amounts of irrigation appeared not to increase grain yield.The results were similar in 2000/2001and 2001/2002seasons and there was not any significant difference among the treatments when seasonal rainfall was high.The results indicated that the amount of rainfall affected WUEet.WUE ranged from 0.97to 1.83kg m À3.It was similar to other values reported for winter wheat.Zhang et al.(1998)reported WUE values for winter wheat between 0.93and 1.51kg m À3and Wang et al.(2001)found that WUE was between 0.70and 1.30kg m À3in the NCP.The WUE of treatment E was the highest and the lowest WUE occurred in the treatment D.Although irrigation is an efficient measure,capable of decreasing water stress,both WUE and WUEet decreased with increasing in irrigation for the three seasons.This shows that higher irrigation decreased WUE and WUEet of crops,which is not consistent with the findings of Hedge (1987),who found that the irrigation significantly increased WUE of crops.GY ranged from 3328to 5583kg ha À1.The GY of treatment C was the highest while GY of treatment E was the lowest corresponding to the small amount of irrigation.There were significant differences between treatment E and other treat-ments at P =0.01.Differences in seasonal rainfall made no difference to the GY of E treatment,possibly because of rainfall distribution.The results of three seasons indicated thattreatment C was better for higher GY,WUEi,and WUE.The results were consistent with those of Yu (1995).3.4.Inter-relationships between GY,IR and ETThe responses of grain yield to the amount of irrigation and ET can be described using a quadratic equation and their relationships were significant at P =ing the equations,the calculated optimal amounts of irrigation for winter wheat at maximum yield were 304,303and 286mm for the three seasons,respectively,and averagely it was 298mm.The calculated optimal levels of ET were 395,427and 457mm for the 3years,respectively,and the average amount was 426mm.The average ET without water deficit for winter wheat was 453mm,reported by Liu et al.(2002)at the same site.The optimal ET for GY being lower than the potential ET indicated that it was not necessary to supply water sufficiently to achieve maximum GY.The relationships between GY and irrigation amounts,ET were related in a second order function for the different winter wheat seasons (Figs.2and 3).All this showed they had similar trends and the GY of winter wheat does not always increase with increasing amounts of irrigation and ET.When the amount of water reached a certain level,the grain yield would decrease.ET was controlled by the meteorological factors and plant factors and they were consistent in the three crop seasons.The reasons for the difference among the amounts of irrigation in the three seasons were soil water storage and rainfall.Thus,an irrigation strategy could be developed according to the rainfall and soil water storage.4.Conclusions and discussionIn this study ET was linearly related to the amount of irrigation.There was about 30mm difference in soil evapora-tion and 25mm difference in leakage among different treatments.Maximal yield was obtained when the optimal amount of irrigation was 298mm and ET was 426mm,averagely for the three seasons However,the seasonal irrigation application should vary with seasonal rainfall and the moisture condition before sowing,since the depletion to soil profile was considerable in the ET components.Excessive irrigation encouraged stem growth and delayeda g r i c u l t u r a l w a t e r m a n a g e m e n t 85(2006)211–218216Fig.2–Relationships between grain yield (GY)of winter wheat and irrigation in 1999/2000,2000/2001and 2001/2002.Fig.3–Relationships between grain yield (GY)andevapotranspiration (ET)in 1999/2000,2000/2001and 2001/2002.the development of winter wheat that decreased GY.In the NCP,the grain-filling period for winter wheat is shorter,lasting about1month from the beginning of May to the10th of June. Dry and hot wind in May and June accelerates the maturity that may reduce harvest index and seed weight.This might be one of the reasons that well-watered winter wheat did not produce the maximum yield.Another important reason is that winter wheat responses differently to water stress at its different growing stages.Zhang et al.(2003)founded that the most sensitive stage of winter wheat to water stress was from stem elongation to booting,followed by anthesis. Irrigation at recoverage reduced GY because it encouraged the growing of non-functional tillers that consumed nutrient reservation.The three seasons’results indicated that treatment C (without irrigation at grainfilling stage)was the best related to WUEi,WUE,and GY.Then irrigation at grain-filling should be withheld.The results showed that with the increase in irrigation ET increased and WUE decreased.Considering the serious water shortage situation in NCP,irrigation might be further reduced to prevent the rapidly falling groundwater level with less sacrifice in grain yield than that in ET. Furthermore,it is also useful to the other irrigated farming regions by the groundwater.Besides the irrigation scheduling to improve WUE of winter wheat,reducing soil evaporation is also an effective method. The results from this experiment showed that E/ET was around30%,considerable water was consumed in soil evaporation.How to improve straw mulching and no-till to winter wheat to reduce soil evaporation was being considered in this region(Huang et al.,2004;Chen et al.,2005).Some researchers reported that the effects of different tillage practices on conserving water(Radcliffe et al.,1988;Unger et al.,1991).Hoeing soil surface was effective to reduce soil evaporation,but it required labor input and it was not popular. Even under optimized irrigation scheduling and water-saving practices,winter wheat still requires large amount of irriga-tion.From a long point of view,reducing winter wheat cropping area might be an option.Yang and Zehnder(2001) proposed to reduce irrigated area to deal with water scarcity in NCP through virtual water import.Policies dealing with water scarcity should be taken into account.AcknowledgmentsProject supported by the National Natural Science Foundation of China(No:40371024);the project of Hebei science and technology department(No:06220112D),China.r e f e r e n c e sAggarwal,P.K.,Singh,A.K.,Chaturvedei,G.S.,Sinha,S.K.,1986.Performance of wheat and triticale cultivars in a variablesoil-water environment.II.Evapotranspiration,water useefficiency,harvest index and grain yield.Field Crops Res.13, 301–315.Bos,M.G.,1985.Summary of ICID definitions of irrigation efficiency.ICID Bull.34,28–31.Chen,S.,Zhang,X.,Pei,D.,Sun,H.,2005.Effects of corn straw mulching on soil temperature and soil evaporation of winter wheatfield.Transact.CSAE21(10),171–173(in Chinese). Daamen,C.C.,Simmonds,L.P.,Wallace,J.S.,Laryea,K.B.,1993.Use micro-lysimeters to measure evaporation from sandy soils.Agric.For.Mete.96,159–173.English,M.,1990.Deficit irrigation.I.Analytical framework.J.Irrig.Drainage ASCE116,99–412.English,M.,Raja,S.N.,1996.Perspectives on deficit irrigation.Agric.Water Manage.32,1–14.Gajri,P.R.,Gill,K.S.,Chaudhary,M.R.,1997.Irrigation of sunflower(Helianthus annuus)in relation to tillage andmulching.Agric.Water Manage.34,149–160.Hagan,R.M.,Howard,R.H.,Talcoh,W.E.,1967.Irrigation of agricultural lands.Am.Soc.Agron.U.S.A.680–681. Hedge,D.M.,1987.The effect of soil water potential method of irrigation,canopy temperature,yield and water use ofradish.Hortic.Sci.62(4),507–511.Hu,C.S.,Zhang,X.Y.,Cheng,Y.S.,Pei,D.,2002.An analysis on dynamics of water table and overdraft in the piedmont of pr.Stud.Agric.18(2),89–91(in Chinese).Huang,Y.,Chen,L.,Fu,B.,Huang,Z.,Gong,J.,2004.The wheat yields and water-use efficiency in the Loess Plateau:straw mulch and irrigation effects.Agric.Water Manage. Hussain,G.,Al-Jaloud,A.A.,Al-Shammary,S.F.,Karimulla,S., 1995.Effect of saline irrigation on the biomass yield and the protein,nitrogen,phosphorus and potassium composition of alfalfa in a pot experiment.J.Pl.Nutr.18,2389–2408. Jin,M.G.,Zhang,R.Q.,Gao,Y.F.,1999.Temporal and spatial soil water management:a case study in the Heiloonggangregion,PR China.Agric.Water Manage.42,173–187. Kang,S.Z.,Zhang,L.,Liang,Y.L.,Hu,X.T.,Cai,H.J.,Gu,B.J.,2002.Effects of limited irrigation on yield and water use efficiency of winter wheat in the Loess Plateau of China.Agric.Water Manage.55,203–216.Li,F.M.,Zhao,S.L.,Duan,S.S.,1995.The strategy for limited irrigation of spring wheat in semiarid Loess Plateau,China.Chin.J.Appl.Ecol.6(3),259–264(in Chinese).Liu,C.,Wei,Z.,1989.Agricultural Hydrology and Water Resources in the North China Plain.Chinese Scientific Press, Beijing,236pp.(in Chinese).Liu,C.M.,Zhang,X.Y.,Zhang,Y.Q.,2002.Determination of daily evaporation and evapotranspiration of winter wheat and maize by large-scale weighing lysimeter and micro-lysimeter.Agric.For.Mete.111,109–120.Ministry of Geology and Mineral Resources,1986.Report on groundwater resources assessment.Beijing,China. Mogenson,V.O.,Jeensen,H.E.,Rab,M.A.,1985.Grain yield,yield components,drought sensitive,and water use efficiency of spring wheat subjected to water stress at various growth stages.Irrig.Sci.6,131–140.Musick,J.T.,Jones,O.R.,Stemart,B.A.,Dusek,D.A.,1994.Water–yield relationships for irrigated and dry land wheat in the US southern plains.Agron.J.86,980–986.Olesen,J.E.,Mortensen,J.V.,Jorgensen,L.N.,Andersen,M.N., 2000.Irrigation strategy,nitrogen application and fungicide control in winter wheat on a sandy soil.I.Yield,yieldcomponents and nitrogen uptake.J.Agric.Sci.134,1–11. Radcliffe,D.E.,Tollner,E.W.,Hargrove,W.L.,Clark,R.L.,Golabi, M.H.,1988.Effect of tillage practices on infiltration and soil strength of a Typic Hapludult soil after10years.Soil Sci.Soc.Am.J.52,798–804.Singh,S.D.,1981.Moisture-sensitive growth stages of dwarf wheat and optimal sequencing of evapotranspirationdeficits.Agron.J.73,387–391.Singh,P.K.,Mishra,A.K.,Imtiyaz,M.,1991.Moisture stress and the water use efficiency of mustard.Agric.Water Manage.20,245–253.a g r i c u l t u r a l w a t e r m a n a g e m e n t85(2006)211–218217。