Adsorption of naphthalene derivatives on different macroporous polymeric adsorbents
化妆品禁用成分
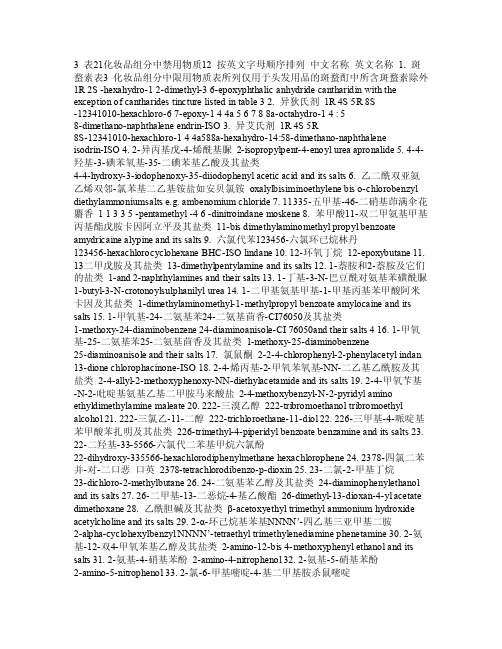
3 表21化妆品组分中禁用物质12 按英文字母顺序排列中文名称英文名称1. 斑蝥素表3 化妆品组分中限用物质表所列仅用于头发用品的斑蝥酊中所含斑蝥素除外1R 2S -hexahydro-1 2-dimethyl-3 6-epoxyphthalic anhydride cantharidin with the exception of cantharides tincture listed in table 3 2. 异狄氏剂1R 4S 5R 8S-12341010-hexachloro-6 7-epoxy-1 4 4a 5 6 7 8 8a-octahydro-1 4 : 58-dimethano-naphthalene endrin-ISO 3. 异艾氏剂1R 4S 5R8S-12341010-hexachloro-1 4 4a588a-hexahydro-14:58-dimethano-naphthaleneisodrin-ISO 4. 2-异丙基戊-4-烯酰基脲2-isopropylpent-4-enoyl urea apronalide 5. 4-4-羟基-3-碘苯氧基-35-二碘苯基乙酸及其盐类4-4-hydroxy-3-iodophenoxy-35-diiodophenyl acetic acid and its salts 6. 乙二酰双亚氨乙烯双邻-氯苯基二乙基铵盐如安贝氯铵oxalylbisiminoethylene bis o-chlorobenzyl diethylammoniumsalts e.g. ambenomium chloride 7. 11335-五甲基-46-二硝基茚满伞花麝香 1 1 3 3 5 -pentamethyl -4 6 -dinitroindane moskene 8. 苯甲酸11-双二甲氨基甲基丙基酯戊胺卡因阿立平及其盐类11-bis dimethylaminomethyl propyl benzoate amydricaine alypine and its salts 9. 六氯代苯123456-六氯环已烷林丹123456-hexachlorocyclohexane BHC-ISO lindane 10. 12-环氧丁烷12-epoxybutane 11. 13二甲戊胺及其盐类13-dimethylpentylamine and its salts 12. 1-萘胺和2-萘胺及它们的盐类1-and 2-naphthylamines and their salts 13. 1-丁基-3-N-巴豆酰对氨基苯磺酰脲1-butyl-3-N-crotonoylsulphanilyl urea 14. 1-二甲基氨基甲基-1-甲基丙基苯甲酸阿米卡因及其盐类1-dimethylaminomethyl-1-methylpropyl benzoate amylocaine and its salts 15. 1-甲氧基-24-二氨基苯24-二氨基茴香-CI76050及其盐类1-methoxy-24-diaminobenzene 24-diaminoanisole-CI 76050and their salts 4 16. 1-甲氧基-25-二氨基苯25-二氨基茴香及其盐类1-methoxy-25-diaminobenzene25-diaminoanisole and their salts 17. 氯鼠酮2-2-4-chlorophenyl-2-phenylacetyl indan 13-dione chlorophacinone-ISO 18. 2-4-烯丙基-2-甲氧苯氧基-NN-二乙基乙酰胺及其盐类2-4-allyl-2-methoxyphenoxy-NN-diethylacetamide and its salts 19. 2-4-甲氧苄基-N-2-吡啶基氨基乙基二甲胺马来酸盐2-4-methoxybenzyl-N-2-pyridyl amino ethyldimethylamine maleate 20. 222-三溴乙醇222-tribromoethanol tribromoethyl alcohol 21. 222-三氯乙-11-二醇222-trichloroethane-11-diol 22. 226-三甲基-4-哌啶基苯甲酸苯扎明及其盐类226-trimethyl-4-piperidyl benzoate benzamine and its salts 23. 22-二羟基-33-5566-六氯代二苯基甲烷六氯酚22-dihydroxy-335566-hexachlorodiphenylmethane hexachlorophene 24. 2378-四氯二苯并-对-二口恶口英2378-tetrachlorodibenzo-p-dioxin 25. 23-二氯-2-甲基丁烷23-dichloro-2-methylbutane 26. 24-二氨基苯乙醇及其盐类24-diaminophenylethanol and its salts 27. 26-二甲基-13-二恶烷-4-基乙酸酯26-dimethyl-13-dioxan-4-yl acetate dimethoxane 28. 乙酰胆碱及其盐类β-acetoxyethyl trimethyl ammonium hydroxide acetylcholine and its salts 29. 2-α-环己烷基苯基NNNN’-四乙基三亚甲基二胺2-alpha-cyclohexylbenzyl NNNN’-tetraethyl trimethylenediamine phenetamine 30. 2-氨基-12-双4-甲氧苯基乙醇及其盐类2-amino-12-bis 4-methoxyphenyl ethanol and its salts 31. 2-氨基-4-硝基苯酚2-amino-4-nitrophenol 32. 2-氨基-5-硝基苯酚2-amino-5-nitrophenol 33. 2-氯-6-甲基嘧啶-4-基二甲基胺杀鼠嘧啶2-chloro-6-methylpyrimidin-4-yldimethylamine crimidine-ISO 34. 3-羟基-4-苯基苯甲酸-2-二乙氨乙基酯及其盐类2-diethylaminoethyl 3-hydroxy-4-phenylbenzoate and its salts 35. 2-甲基庚胺及其盐类2-methylheptylamine and its salts 36. 2-甲基-间苯二胺2-methyl-m-phenylenediamine 5 37. 2-萘酚2-naphthol 38. 2-苯基茚满-13-二酮苯茚二酮2-phenylindan-13-dione phenindione 39. 3-1-萘基-4-羟基香豆素3-1-naphthyl-4-hydroxycoumarin 40. 33-二4-羟基苯基2-苯并C呋喃酮酚酞33-bis4-hydroxyphenyl phthalide phenolphthalein 41. 345-三溴水杨酰苯胺三溴沙仑345-tribromosalicylanilide tribromsalan 42. 345-三甲氧苯乙基胺及其盐类345-trimethoxyphenethylamine and its salts 43. 34-二羟基-2-甲氧基-2-甲基-4-苯基-2H5H吡咯32-c-1苯并吡喃-5-酮环香豆素34-dihydro-2-methoxy-2-methyl-4-phenyl-2H5Hpyrano 32-c-1benzopyran-5-one cyclocoumarol 44. 肉桂酸3二乙氨基丙酯3-diethylaminopropyl cinnamate 45. 3’-乙基-5678-四氢-5688-四甲基-2-乙酰萘乙酰乙基四甲萘满AETT3-ethyl-5678-tetrahydro-5688-tetramethyl-2-acetonaphthone acetyl ethyl tetramethyl tetralin AETT 46. 3-咪唑-4-基丙烯酸尿刊酸及其乙酯3-imidazol-4-ylacrylic acid and its ethyl ester urocanic acid 47. 44-二羟基-33-3-甲基硫代亚丙基双香豆素44-dihydroxy-33-3-methylthiopropylidene dicoumarin 48. 4—氨基-2-硝基酚4-amino-2-nitrophenol 49. 4-氨基水杨酸及其盐类4-aminosalicylic acid and its salts 50. 4-苄氧基苯酚4-甲氧基苯酚和4-乙氧基苯酚4-benzyloxyphenol4-methoxyphenol and 4-ethoxyphenol 51. 4-乙氧基-间-苯二胺及其盐类4-ethoxy-m-phenylenediamine and its salts 52. 4-甲基-间-苯二胺及其盐类4-methyl-m-phenylenediamine and its salts 53. 盐酸柠檬酸柯衣定盐4-phenylazophenylene-1 3-diamine citrate hydrochloride chrysoidine citrate hydrochloride 54. 4-苯基丁-3-烯-2-酮4-phenylbut-3-en-2-one 55. 4-叔丁基-3-甲氧基-26-二硝基甲苯葵子麝香4-tert-butyl-3-methoxy-26-dinitrotoluene musk ambrette 56. 4-叔丁基苯酚4-tert-butylphenol 57. 4-叔丁基邻苯二酚4-tert-butylpyrocatechol 58. 5-叔丁基-123-三甲基-46-二硝基苯西藏麝香5-tert -butyl -1 2 3 -trimethyl -4 6 –dinitrobenzene musk tibetene 6 59. 5-αβ-二溴苯乙基-5-甲基乙内酰脲5--dibromophenethyl-5-methylhydantoin 60. 二次亚碘酸55-二异丙基-22-二甲基联苯-44-二基酯55-di-isopropyl-22-dimethylbiphenyl-44-diyl dihypoiodite 61. 55-二苯基-4-咪唑酮55-diphenyl-4-imidazolidone 62. 6-哌嗪基-24-嘧啶二胺-3-氧化物米诺地尔及其盐和衍生物6-piperidinyl-24-pyrimidinediamine-3-oxide minoxidil and its salts and derivatives 63. 7-2-羟基-3-2-羟乙基-N-甲氨基丙基茶碱7-2-hydroxy-3-2-hydroxyethyl-N-methylamino propyl theophylline xanthinol 64. 11-α-羟基孕甾-4-烯-320-二酮及其酯类羟基孕甾烯醇酮11-alpha-hydroxypregn-4-ene-320-dione and its esters 65. 醋硝香豆素acenocoumarol 3-2-acetyl-1-p-nitrophenyl ethyl-4-hydroxycoumarin 66. 乙腈Acetonitrile 67. 乌头碱欧乌头主要生物碱及其盐类aconitine principal alkaloid of aconitum napellus L. andits salts 68. 欧乌头属叶子、根和草药制剂aconitum napellus L. leaves roots and galenical preparations 69. 侧金盏花及其制剂adonis vernalis L. and its preparations 70. 五氰亚硝酰基高铁酸碱金属盐alkali pentacyanonitrosylferrate 2- 71. 炔醇类以及它们的酯类、醚类、盐类alkyne alcohols their esters、ethers and salts 72. 阿洛拉胺及其盐类alloclamide and its salts 2-allyloxy-4-choro-N- 2-diethylaminoethyl benzamide 73. 烯丙基芥子油异硫氰酸烯丙酯allyl isothiocyanate 74. α-哌嗪-2-基苄基乙酸酯左旋的苏型左法哌酯及其盐类alpha-piperidin-2-yl benzyl acetate laevorotatory threoform levophacetoperane and its salts 75. α-山道年alpha-santonin3S5aR9bS-33a455a9b-hexahydro-35a9-trimethylnaphto 12-b furan-28-dione 76. 氨基已酸及其盐类aminocaproic acid 6-aminohexanoic acid and its salts 77. 阿米替林及其盐类amitriptyline 5- 3-dimethylaminopropylidene-10 11-dihydro-5H-dibenzo-ad cycloheptene and its salts 7 78. 大阿米芹及其草药制剂ammi majus and its galenical preparations 79. 4-二甲氨基苯甲酸戊酯混合的异构体帕地马酯amyl4-dimethylaminobenzoate mixed isomers padimate A INN 80. 亚硝酸戊酯类amyl nitrites 81. 印防己果实anamirta cocculus L. fruit 82. 苯胺及其盐类以及卤化、磺化的衍生物类aniline its salts and its halogenated and sulphonated derivatives 83. 蒽油anthracene oil 84. 带有甾族化合物结构的抗雄激素物质类固醇antiandrogens with steroid structure 85. 抗菌素类Antibiotics 86. 锑及锑化合物antimony and its compounds 87. 加拿大大麻夹竹桃麻大麻叶罗布麻及其制剂apocynum cannabinum L. and its preparations 88. 阿扑吗啡及其盐类apomorphine566a7-tetrahydro-6-methyl-4H-dibenzo deg-quinoline-1011-dihydric alcohol and its salts 89. 槟榔碱arecoline methyl 1256-tetrahydro-1-methylnicotinate 90. 马兜铃酸及其酯盐aristolochic acid and its salts 91. 砷及砷化合物arsenic and its compounds 92. 颠茄及其制剂atropa belladona L. and its preparations 93. 阿托品及其盐类和衍生物atropine its salts and derivatives 94. 阿扎环醇及其盐类azacyclonolαα-diphenyl-α-piperid-4-ylmethanol and its salts 95. 巴比妥酸盐类Barbiturates 96. 钡盐类除硫酸钡表3 中的硫化钡及表6中着色剂的不溶性钡盐色淀和颜料外barium salts with the exception of barium sulphate barium sulphide under the conditions laid down in table 3 and lakes salts and pigments prepared from the colouring agents listed with the reference3 in table 6 97. 贝美格及其盐类bemegrideethyl-3-methylglutarimideand its salts 98. 贝那替秦benactyzine 2-diethylaminoethyl benzilate 99. 苄氟噻嗪及其衍生物bendroflumethiazide3-benzyl-34dihydro-6-trifluoromethyl-2H-1 2 4-benzothiadiazine-7-sulfonamide11-dioxide and its derivatives 8 100. 苯扎托品及其盐类benzatropine tropine benzhydryl ether 3-diphenylmethoxytropane and its salts 101. 苯并吖庚因及苯并二吖庚因benzazepines and benzadiazepines 102. 苯Benzene 103. 联苯胺44-二氨基联苯benzidine 44-diaminobiphenyl 104. 苯咯溴铵benzilonium bromide11-diethyl-3-hydroxypyrrolidinium bromide benzilate 105. 苯并咪唑23H-酮benzimidazol-23H-one 106. 4-羟基-3-甲氧基肉桂醇的苯甲酸酯天然精油中的规定含量除外benzoates of 4-hydroxy-3-methoxycinnamyl alcohol except for normal content in natural essences used 107. 过氧化苯甲酰benzoyl peroxide 108. 铍及铍化合物beryllium and its compounds 109. 贝托卡因及其盐类betoxycaine2-2-diethylaminoethoxy ethyl 3-amino-4-butoxybenzoate and its salts 110. 比他维林bietamiverine 2-diethylaminoethyl α-phenyl-1-piperidineacetate 111. 硫氯酚bithionol 22-thiobis 46-dichlorophenol 112. 托西溴苄铵bretylium tosilate o-bromobenzyl ethyldimethylammonium p-toluenesulfonate 113. 溴元素状态bromine elemental 114.溴米索伐bromisoval 1-2-bromo-3-methylbutyryl urea 115. 溴苯那敏及其盐类brompheniramine 3-p-bromophenyl-NN-dimethyl-3-pyrid-2-ylpropylamine and its salts 116. 番木鳖碱Brucine 117. 布坦卡因及其盐类butanilicaine 2-butylamino-6-chloro-o-acetotoluidide and its salts 118. 布托哌啉及其盐类butopiprine2-butoxyethyl α-phenyl-1-piperidineacetate and its salts 119. 镉和镉的化合物cadmium and its compounds 120. 斑蝥表3 化妆品组分中限用物质表所列仅用于头发用品的斑蝥酊中所含斑蝥素除外cantharides cantharis vesicatoria with the exceptionof cantharides tincture listed in table 3 121. 卡普托胺captodiame2-p-butylmercaptobenzhydrylmercapto-NN-dimethylethylamine 9 122. 卡拉美芬及其盐类caramiphen 2-diethylaminoethyl ester of 1-phenylcyclopentanecarboxylic acidand its salts 123. 二硫化碳carbon disulphide 124. 四氯化碳carbon tetrachloride 125. 卡溴脲carbromal 1-2-bromo-2-ethylbutyryl urea 126. 氨磺丁脲carbutamide N- butylcarbamoyl sulfanilamide 1-butyl-3-sulfanilylurea 127. 卡立普多carisoprodol2-carbamyloxymethyl-2-isopropylcarbamyloxymethylpentane 128. 过氧化氢酶Catalase 129. 儿茶酚Catechol 130. 人的细胞、组织或其产品cells tissues or products of human origin 131. 吐根酚碱及其盐cephaeline and its salts 132. 土荆芥精油chenopodium ambrosioides essential oil 133. 氯Chlorine 134. 氮芥及其盐类chlormethine 22-dichloro-N-methyldiethylamine bis 2-chloroethyl methylamine and its salts 135. 氯美扎酮chloromezanone 136. 氯乙烷Chloroethane 137. 氯仿Chloroform 138. 氯苯沙明chlorphenoxamine2-1-p-chlorophenyl-1-phenylethoxy-NN-dimethylethylamine 139. 氯磺丙脲chlorpropamide 1-p-chlorophenylsulfonyl-3-propylurea 140. 氯普噻吨及其盐类chlorprothixene trans isomer of 3-2-chlorothioxanthen-9-ylidene-NN-dimethylpropylamine taractan and its salts 141. 氯噻酮chlortalidone2-chloro-5-1-hydroxy-3-oxo-1-isoindolinyl benzenesulfonamide 142. 氯唑沙宗chlorzoxazone 5-chloro-2-benzoxazolinone 143. 胆碱盐类及它们的酯类例如氯化胆碱choline salts and their esters e.g. choline chloride 2-hydroxyethyl-trimethylammonium chloride 10 144. 铬、铬酸及其盐类chromium chromic acid and its salts 145. 辛可卡因及其盐类cinchocaine 2-butoxy-N-2-diethylamincethyl cinchoninamide and its salts 146. 辛可芬及其盐类衍生物以及衍生物的盐类cinchophen 2-phenylcinchoninic acid its salts derivatives and salts of these derivatives 147. 麦角菌及其生物碱和草药制剂claviceps purpurea tul. its alkaloids and galenical preparations 148. 氯非那胺clofenamide 4-chloro-13-benzenedisulfon-amide 149. 滴滴涕clofenotane DDT ISO 150. 苯磺酸钴cobalt benzenesulphonate 151. 秋水仙碱及其盐类和衍生物colchicine its salts and derivatives 152. 秋水仙糖苷及其衍生物colchicoside and its derivatives 153. 秋水仙及其草药制剂colchicum autumnale L. and its galenical preparations 154. 着色剂CI 42555 着色剂CI 42555-1 着色剂CI 42555-2 colouring agent CI 42555 colouring agent CI 42555-1 colouring agent CI 42555-2 155. 着色剂CI 12075及其色淀、颜料及盐类colouring agent CI 12075 and its lakes pigments and salts 156. 着色剂CI 12140 colouring agent CI 12140 157. 着色剂CI 13065 colouring agent CI 13065 158. 着色剂CI 15585 colouring agent CI 15585 159. 着色剂CI 26105 colouring agent CI 26105 160. 着色剂CI 42535 colouring agent CI 42535 161.着色剂CI 42640 colouring agent CI 42640 162. 着色剂CI 45170 和CI 451701 colouring agent CI 45170 and CI 45170:1 163. 着色剂CI 61554 colouring agent CI 61554 164. 毒芹碱coniine 165. 毒参果实、粉末和草药制剂conium maculatum L. fruit powder galenical preparations 166. 铃兰毒甙convallatoxin 11 167. 库美香豆素coumetarol 33-2-methoxyethylidene bis 4-hydroxycoumarin 168. 巴豆巴豆油croton tiglium oil 169. 粗制和精制煤焦油crude and refined coal tars 170. 箭毒和箭毒碱curare and curarine 171. 环拉氨酯cyclarbamate 11-bis phenylcarbamoyloxymethyl cyclopentane 172. 赛克利嗪及其盐类cyclizine 1-benzhydryl-4-methylpiperazine and its salts 173. 环美酚及其盐类cyclomenol 2-cyclohexyl-35-xylenol2-cyclohexyl-35-dimethylphenol and its salts 174. 环磷酰胺及其盐类cyclophosphamide 2bis2-chloroethyl amino tetralydro-2H-1 3 2-oxazaphesphorine2-oxide and its salts 175. 曼陀罗及其草药制剂datura stramonium L. and its galenical preparations 176. 醋谷地阿诺deanol aceglumate 177. 癸亚甲基双三甲铵盐类。
从水中去除重金属离子和染料的农业固体废物吸附剂综述说明书
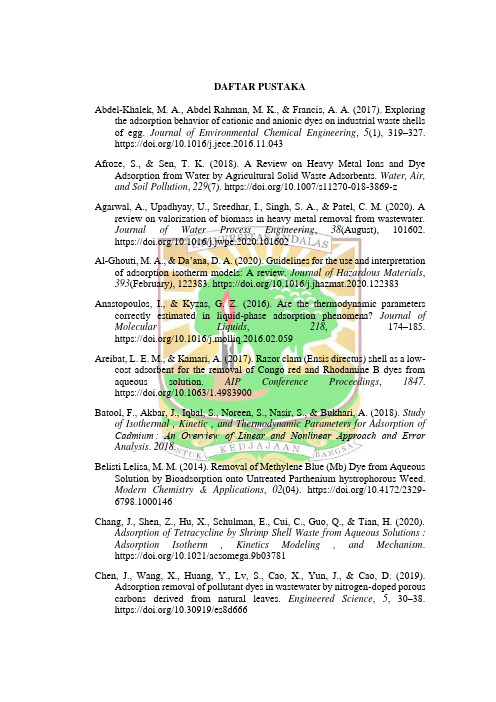
DAFTAR PUSTAKAAbdel-Khalek, M. A., Abdel Rahman, M. K., & Francis, A. A. (2017). Exploring the adsorption behavior of cationic and anionic dyes on industrial waste shells of egg. Journal of Environmental Chemical Engineering, 5(1), 319–327.https:///10.1016/j.jece.2016.11.043Afroze, S., & Sen, T. K. (2018). A Review on Heavy Metal Ions and Dye Adsorption from Water by Agricultural Solid Waste Adsorbents. Water, Air, and Soil Pollution, 229(7). https:///10.1007/s11270-018-3869-z Agarwal, A., Upadhyay, U., Sreedhar, I., Singh, S. A., & Patel, C. M. (2020). A review on valorization of biomass in heavy metal removal from wastewater.Journal of Water Process Engineering, 38(August), 101602.https:///10.1016/j.jwpe.2020.101602Al-Ghouti, M. A., & Da’ana, D. A. (2020). Guidelines for the use and interpretation of adsorption isotherm models: A review. Journal of Hazardous Materials, 393(February), 122383. https:///10.1016/j.jhazmat.2020.122383 Anastopoulos, I., & Kyzas, G. Z. (2016). Are the thermodynamic parameters correctly estimated in liquid-phase adsorption phenomena? Journal of Molecular Liquids, 218, 174–185.https:///10.1016/j.molliq.2016.02.059Areibat, L. E. M., & Kamari, A. (2017). Razor clam (Ensis directus) shell as a low-cost adsorbent for the removal of Congo red and Rhodamine B dyes from aqueous solution. AIP Conference Proceedings, 1847.https:///10.1063/1.4983900Batool, F., Akbar, J., Iqbal, S., Noreen, S., Nasir, S., & Bukhari, A. (2018). Study of Isothermal , Kinetic , and Thermodynamic Parameters for Adsorption of Cadmium : An Overview of Linear and Nonlinear Approach and Error Analysis. 2018.Belisti Lelisa, M. M. (2014). Removal of Methylene Blue (Mb) Dye from Aqueous Solution by Bioadsorption onto Untreated Parthenium hystrophorous Weed.Modern Chemistry & Applications, 02(04). https:///10.4172/2329-6798.1000146Chang, J., Shen, Z., Hu, X., Schulman, E., Cui, C., Guo, Q., & Tian, H. (2020).Adsorption of Tetracycline by Shrimp Shell Waste from Aqueous Solutions : Adsorption Isotherm , Kinetics Modeling , and Mechanism.https:///10.1021/acsomega.9b03781Chen, J., Wang, X., Huang, Y., Lv, S., Cao, X., Yun, J., & Cao, D. (2019).Adsorption removal of pollutant dyes in wastewater by nitrogen-doped porous carbons derived from natural leaves. Engineered Science, 5, 30–38.https:///10.30919/es8d666Dai, L., Zhu, W., He, L., Tan, F., Zhu, N., Zhou, Q., He, M., & Hu, G. (2018).Calcium-rich biochar from crab shell: An unexpected super adsorbent for dye removal. Bioresource Technology, 267(June), 510–516.https:///10.1016/j.biortech.2018.07.090d e Rezende, M. L. R., Coesta, P. T. G., de Oliveira, R. C., Salmeron, S., Sant’Ana,A. C. P., Damante, C. A., Greghi, S. L. A., & Consolaro, A. (2015). BoneDemineralization With Citric Acid Enhances Adhesion and Spreading of Preosteoblasts. Journal of Periodontology, 86(1), 146–154.https:///10.1902/jop.2014.130657Derakhshan, Z., Baghapour, M. A., Ranjbar, M., & Faramarzian, M. (2013).Adsorption of Methylene Blue Dye from Aqueous Solutions by Modified Pumice Stone: Kinetics and Equilibrium Studies. Health Scope, 2(3), 136–144. https:///10.17795/jhealthscope-12492Djelloul, C., & Hamdaoui, O. (2014). Removal of cationic dye from aqueous solution using melon peel as nonconventional low-cost sorbent. Desalination and Water Treatment, 52(40–42), 7701–7710.https:///10.1080/19443994.2013.833555Eletta, O. A. A., Adeniyi, A. G., Ighalo, J. O., Onifade, D. V., & Ayandele, F. O.(2020). Valorisation of Cocoa (Theobroma cacao) pod husk as precursors for the production of adsorbents for water treatment. Environmental Technology Reviews, 9(1), 20–36. https:///10.1080/21622515.2020.1730983Eljiedi, A. A. A., & Kamari, A. (2017). Removal of methyl orange and methylene blue dyes from aqueous solution using lala clam (Orbicularia orbiculata) shell.AIP Conference Proceedings, 1847. https:///10.1063/1.4983899Elwakeel, K. Z., Elgarahy, A. M., & Mohammad, S. H. (2017). Use of beach bivalve shells located at Port Said coast (Egypt) as a green approach for methylene blue removal. Journal of Environmental Chemical Engineering, 5(1), 578–587. https:///10.1016/j.jece.2016.12.032Faisal, A. A. H., Khalid, Z., Al-ansari, N., & Sharma, G. (2021). Chemosphere Precipitation of ( Mg / Fe-CTAB ) - Layered double hydroxide nanoparticles onto sewage sludge for producing novel sorbent to remove Congo red and methylene blue dyes from aqueous environment. Chemosphere, September, 132693. https:///10.1016/j.chemosphere.2021.132693Fajarwati, F. I., Ika Yandini, N., Anugrahwati, M., & Setyawati, A. (2020).Adsorption Study of Methylene Blue and Methyl Orange Using Green Shell (Perna Viridis). EKSAKTA: Journal of Sciences and Data Analysis, 1(1), 92–97. https:///10.20885/eksakta.vol1.iss1.art14Fosso-Kankeu, E., Webster, A., Ntwampe, I. O., & Waanders, F. B. (2017).Coagulation/Flocculation Potential of Polyaluminium Chloride and Bentonite Clay Tested in the Removal of Methyl Red and Crystal Violet. Arabian Journal for Science and Engineering, 42(4), 1389–1397.https:///10.1007/s13369-016-2244-xGhaedi, M., Hajjati, S., Mahmudi, Z., Tyagi, I., Agarwal, S., Maity, A., & Gupta, V. K. (2015). Modeling of competitive ultrasonic assisted removal of the dyes - Methylene blue and Safranin-O using Fe3O4 nanoparticles. Chemical Engineering Journal, 268, 28–37. https:///10.1016/j.cej.2014.12.090 Hevira, L., Rahmi, A., Zein, R., Zilfa, Z., & Rahmayeni, R. (2020). The fast and of low-cost-adsorbent to the removal of cationic and anionic dye using chicken eggshell with its membrane. Mediterranean Journal of Chemistry, 10(3), 294–301. https:///10.13171/mjc020********lhHevira, L., Zilfa, Rahmayeni, Ighalo, J. O., Aziz, H., & Zein, R. (2021). Terminalia catappa shell as low-cost biosorbent for the removal of methylene blue from aqueous solutions. Journal of Industrial and Engineering Chemistry, 97, 188–199. https:///10.1016/j.jiec.2021.01.028Hevira, L., Zilfa, Rahmayeni, Ighalo, J. O., & Zein, R. (2020). Biosorption of indigo carmine from aqueous solution by Terminalia Catappa shell. Journal of Environmental Chemical Engineering, 8(5), 104290.https:///10.1016/j.jece.2020.104290Hubbe, M. A., Azizian, S., & Douven, S. (2019). Implications of apparent pseudo-second-order adsorption kinetics onto cellulosic materials: A review.BioResources, 14(3), 7582–7626. https:///10.15376/biores.14.3.7582-7626Ighalo, J. O., & Adeniyi, A. G. (2020). A mini-review of the morphological properties of biosorbents derived from plant leaves. SN Applied Sciences, 2(3).https:///10.1007/s42452-020-2335-xIgwegbe, C. A., Mohmmadi, L., Ahmadi, S., Rahdar, A., Khadkhodaiy, D., Dehghani, R., & Rahdar, S. (2019). Modeling of adsorption of Methylene Blue dye on Ho-CaWO4 nanoparticles using Response Surface Methodology (RSM) and Artificial Neural Network (ANN) techniques. MethodsX, 6, 1779–1797. https:///10.1016/j.mex.2019.07.016Jafari, M., Vanoppen, M., van Agtmaal, J. M. C., Cornelissen, E. R., Vrouwenvelder, J. S., Verliefde, A., van Loosdrecht, M. C. M., & Picioreanu,C. (2021). Cost of fouling in full-scale reverse osmosis and nanofiltrationinstallations in the Netherlands. Desalination, 500(December 2020), 114865.https:///10.1016/j.desal.2020.114865Jawad, A. H., Rashid, R. A., Ishak, M. A. M., & Ismail, K. (2018). Adsorptive removal of methylene blue by chemically treated cellulosic waste banana ( Musa sapientum ) peels . Journal of Taibah University for Science, 12(6), 809–819. https:///10.1080/16583655.2018.1519893Jawad, A. H., Rashid, R. A., Ishak, M. A. M., & Wilson, L. D. (2016). Adsorption of methylene blue onto activated carbon developed from biomass waste by H2SO4 activation: kinetic, equilibrium and thermodynamic studies.Desalination and Water Treatment, 57(52), 25194–25206.https:///10.1080/19443994.2016.1144534Katneni, V. K., Shekhar, M. S., Jangam, A. K., Prabhudas, S. K., Krishnan, K., Kaikkolante, N., Paran, B. C., Baghel, D. S., Koyadan, V. K., Jena, J., & Mohapatra, T. (2020). Novel Isoform Sequencing Based Full-Length Transcriptome Resource for Indian White Shrimp, Penaeus indicus. Frontiers in Marine Science, 7(December), 1–4.https:///10.3389/fmars.2020.605098Kusumawardani, R., Rismawati, A., Retnowati, R., So, H., & Variabel, C. (2018).MODIFIKASI BIJI PEPAYA SEBAGAI BIOSORBEN ZAT WARNA TEKSTIL MORDANT BLACK 11 MODIFICATION OF PAPAYA SEEDS AS BIOSORBENT COLORS OF MORDANT BLACK 11 TEXTILES biosorben biji pepaya untuk mengadsorpsi zat warna tekstil mordant black 11 . Prinsip kerja dari mor. 1(2), 92–95.Li, W. (2011). Quantitative Analysis of the Reaction between Gliadin and Citric Acid under Weak Acidic and Weak Alkaline Conditions. Thesis.Liu, L., Fan, S., & Li, Y. (2018). Removal behavior of methylene blue from aqueous solution by tea waste: Kinetics, isotherms and mechanism. International Journal of Environmental Research and Public Health, 15(7).https:///10.3390/ijerph15071321Makeswari, M., Santhi, T., & Ezhilarasi, M. R. (2016). Adsorption of methylene blue dye by citric acid modified leaves of Ricinus communis from aqueous solutions. 8(7), 452–462.Mohan, C. (2003). A guide for the preparation and use of buffers in biological systems.Naghizadeh, A., & Ghafouri, M. (2017). Synthesis and performance evaluation of chitosan prepared from Persian gulf shrimp shell in removal of reactive blue29 dye from aqueous solution (Isotherm, thermodynamic and kinetic study).Iranian Journal of Chemistry and Chemical Engineering, 36(3), 25–36. Pang, Y. L., Tan, J. H., Lim, S., & Chong, W. C. (2021). A state-of-the-art review on biowaste derived chitosan biomaterials for biosorption of organic dyes: Parameter studies, kinetics, isotherms and thermodynamics. Polymers, 13(17).https:///10.3390/polym13173009Pathak, P. D., Mandavgane, S. A., & Kulkarni, B. D. (2016). Characterizing fruit and vegetable peels as bioadsorbents. Current Science, 110(11), 2114–2123.https:///10.18520/cs/v110/i11/2114-2123Ponnusami, V., Vikram, S., & Srivastava, S. N. (2008). Guava (Psidium guajava) leaf powder: Novel adsorbent for removal of methylene blue from aqueous solutions. Journal of Hazardous Materials, 152(1), 276–286.https:///10.1016/j.jhazmat.2007.06.107Pratiwi, R. (2018). ASPEK BIOLOGI DAN ABLASI MATA PADA UDANG WINDU Penaeus monodon SUKU PENAEIDAE (DECAPODA: MALACOSTRACA). Oseana, 43(2), 34–47.https:///10.14203/oseana.2018.vol.43no.2.19Purnomo, J. S. (2022). PENINGKATAN KAPASITAS ADSORPSI METHYLENE BLUE SECARA BATCH MENGGUNAKAN AMPAS DAUN SERAI WANGI (Cymbopogon nardus L. Rendle) YANG DIMODIFIKASI DENGAN ASAM SITRAT. In Universitas Andalas (Issue8.5.2017).Qin, L., Zhou, Z., Dai, J., Ma, P., Zhao, H., He, J., Xie, A., Li, C., & Yan, Y. (2016).Novel N-doped hierarchically porous carbons derived from sustainable shrimp shell for high-performance removal of sulfamethazine and chloramphenicol.Journal of the Taiwan Institute of Chemical Engineers, 62, 228–238.https:///10.1016/j.jtice.2016.02.009Rafatullah, M., Sulaiman, O., Hashim, R., & Ahmad, A. (2010). Adsorption of methylene blue on low-cost adsorbents: A review. Journal of Hazardous Materials, 177(1–3), 70–80. https:///10.1016/j.jhazmat.2009.12.047 Rahmayeni, R., Oktavia, Y., Stiadi, Y., Arief, S., & Zulhadjri, Z. (2021). Spinel ferrite of MnFe2O4 synthesized in Piper betle Linn extract media and its application as photocatalysts and antibacterial. Journal of Dispersion Science and Technology, 42(3), 465–474.https:///10.1080/01932691.2020.1721011Rajumon, R., Anand, J. C., Ealias, A. M., Desai, D. S., George, G., & Saravanakumar, M. P. (2019). Adsorption of textile dyes with ultrasonic assistance using green reduced graphene oxide: An in-depth investigation on sonochemical factors. Journal of Environmental Chemical Engineering, 7(6), 103479. https:///10.1016/j.jece.2019.103479Ramadhani, P., Chaidir, Z., Zilfa, Tomi, Z. B., Rahmiarti, D., & Zein, R. (2020).Shrimp shell (Metapenaeus monoceros) waste as a low-cost adsorbent for metanil yellow dye removal in aqueous solution. Desalination and Water Treatment, 197, 413–423. https:///10.5004/dwt.2020.25963 Ramrakhiani, L., Ghosh, S., & Majumdar, S. (2016). Surface Modification of Naturally Available Biomass for Enhancement of Heavy Metal Removal Efficiency, Upscaling Prospects, and Management Aspects of Spent Biosorbents: A Review. Applied Biochemistry and Biotechnology, 180(1), 41–78. https:///10.1007/s12010-016-2083-yRápó, E., & Tonk, S. (2021). Factors affecting synthetic dye adsorption; desorption studies: A review of results from the last five years (2017–2021). Molecules, 26(17). https:///10.3390/molecules26175419Ravi, & Pandey, L. M. (2019). Enhanced adsorption capacity of designed bentonite and alginate beads for the effective removal of methylene blue. Applied Clay Science, 169(October 2018), 102–111.https:///10.1016/j.clay.2018.12.019Ribeiro, C., Scheufele, F. B., Espinoza-Quiñones, F. R., Módenes, A. N., da Silva,M. G. C., Vieira, M. G. A., & Borba, C. E. (2015). Characterization of Oreochromis niloticus fish scales and assessment of their potential on the adsorption of reactive blue 5G dye. Colloids and Surfaces A: Physicochemical and Engineering Aspects, 482, 693–701.https:///10.1016/j.colsurfa.2015.05.057Sadaf, S., & Bhatti, H. N. (2014). Batch and fixed bed column studies for the removal of Indosol Yellow BG dye by peanut husk. Journal of the Taiwan Institute of Chemical Engineers, 45(2), 541–553.https:///10.1016/j.jtice.2013.05.004Safa, Y., & Bhatti, H. N. (2011). Adsorptive removal of direct dyes by low cost rice husk : Effect of treatments and modifications. 10(16), 3128–3142.https:///10.5897/AJB10.1302Sandip, Q. (2015). Journal of Industrial and Engineering Chemistry Efficient adsorption and photocatalytic degradation of Rhodamine B dye over Bi 2 O 3 -bentonite nanocomposites : A kinetic study. 1–8.Sawasdee, S., & Watcharabundit, P. (2016). Effect of temperature on brilliant green adsorption by shrimp shell: Equilibrium and kinetics. Chiang Mai University Journal of Natural Sciences, 15(3), 221–236.https:///10.12982/cmujns.2016.0017Senthil Kumar, P., Janet Joshiba, G., Femina, C. C., Varshini, P., Priyadharshini, S., Arun Karthick, M. S., & Jothirani, R. (2019). A critical review on recent developments in the low-cost adsorption of dyes from wastewater.Desalination and Water Treatment, 172, 395–416.https:///10.5004/dwt.2019.24613Shakoor, S., & Nasar, A. (2016). Removal of methylene blue dye from artificially contaminated water using citrus limetta peel waste as a very low cost adsorbent. Journal of the Taiwan Institute of Chemical Engineers, 66, 154–163. https:///10.1016/j.jtice.2016.06.009Sun, C., Qiu, J., Zhang, Z., Marhaba, T. F., Zhang, Y., & Zhang, W. (2016).Characterization of Citric Acid-Modified Clam Shells and Application for Aqueous Lead (II) Removal. Water, Air, and Soil Pollution, 227(9).https:///10.1007/s11270-016-2975-zSuryawanshi, N., Jujjavarapu, S. E., & Ayothiraman, S. (2019). Marine shell industrial wastes–an abundant source of chitin and its derivatives: constituents, pretreatment, fermentation, and pleiotropic applications-a revisit.International Journal of Environmental Science and Technology, 16(7), 3877–3898. https:///10.1007/s13762-018-02204-3Taylor, P., Wang, P., Ma, Q., Hu, D., & Wang, L. (2015). Desalination and Water Treatment Adsorption of methylene blue by a low-cost biosorbent : citric acid modified peanut shell. May, 37–41.https:///10.1080/19443994.2015.1033651Taylor, P., Zhou, Y., Zhang, R., Gu, X., Lu, J., Zhou, Y., Zhang, R., Gu, X., & Lu, J. (2015). Separation Science and Technology Adsorption of Divalent Heavy Metal Ions from Aqueous Solution by Citric Acid Modified Pine Sawdust Adsorption of Divalent Heavy Metal Ions from Aqueous Solution by Citric Acid Modified Pine Sawdust. January, 37–41.https:///10.1080/01496395.2014.956223Thamaraiselvan, C., Lerman, S., Weinfeld-Cohen, K., & Dosoretz, C. G. (2018).Characterization of a support-free carbon nanotube-microporous membrane for water and wastewater filtration. Separation and Purification Technology, 202(March), 1–8. https:///10.1016/j.seppur.2018.03.038Xu, Y., Liu, Y., Liu, S., Tan, X., Zeng, G., Zeng, W., Ding, Y., Cao, W., & Zheng,B. (2016). Enhanced adsorption of methylene blue by citric acid modificationof biochar derived from water hyacinth (Eichornia crassipes). Environmental Science and Pollution Research, 23(23), 23606–23618.https:///10.1007/s11356-016-7572-6Zein, R. (2019). Pensi shell (Corbicula moltkiana)as a biosorbent for metanil yellow dyes removal: pH and equilibrium model evaluation. Jurnal Litbang Industri, 15–22.Zein, R., Chaidir, Z., Fauzia, S., & Ramadhani, P. (2022). Isotherm and Kinetic Studies on the Adsorption Behavior of Metanil Yellow Dyes onto Modified Shrimp Shell-Polyethylenimine ( SS-PEI ). 8(May), 10–22.https:///10.15408/jkv.v8i1.22566Zein, R., Purnomo, J. S., Ramadhani, P., Alif, M. F., & Safni, S. (2022).Lemongrass ( Cymbopogon nardus ) leaves biowaste as an effective and low-cost adsorbent for methylene blue dyes removal: isotherms, kinetics, and thermodynamics studies . Separation Science and Technology, 00(00), 1–17.https:///10.1080/01496395.2022.2058549Zein, R., Tomi, Z. B., Fauzia, S., & Zilfa, Z. (2020). Modification of rice husk silica with bovine serum albumin (BSA) for improvement in adsorption of metanil yellow dye. Journal of the Iranian Chemical Society, 17(10), 2599–2612.https:///10.1007/s13738-020-01955-6Zhou, Y., Ge, L., Fan, N., & Xia, M. (2018). Adsorption of Congo red from aqueous solution onto shrimp shell powder. Adsorption Science and Technology, 36(5–6), 1310–1330. https:///10.1177/0263617418768945。
醌类物质结构分类为

醌类物质结构分类醌类物质是一类具有骨架中含有醌基团的有机化合物。
醌基团是由两个相连的酮基构成,通常被称为”1,4-二酮”。
根据醌基团的位置和物质的化学性质,可以将醌类物质分为不同的结构类型。
1. 单醌类物质单醌类物质是指只含有一个醌基团的化合物。
最常见的例子是苯醌,其分子式为C6H4O2。
苯醌的结构中,两个酮基位于苯环的相邻碳原子上。
苯醌是一种黄色晶体,可用作染料、抗氧化剂和光敏材料等。
2. 双醌类物质双醌类物质是指含有两个相连的醌基团的化合物。
其中一个常见的例子是二氮杂苯并二恶唑二酮(简称NQ)。
NQ分子中,两个酮基团位于苯环和二恶唑环上。
NQ具有良好的电荷传输性能和光学性能,广泛应用于有机光电子器件中。
3. 多醌类物质多醌类物质是指含有多个醌基团的化合物。
这些化合物通常具有更复杂的分子结构和更丰富的化学性质。
其中一个重要的例子是萘醌,其分子式为C10H6O2。
萘醌分子中,两个酮基团位于萘环上。
萘醌可以通过氧化反应得到,是一种重要的合成中间体,在药物和染料工业中广泛应用。
4. 混合醌类物质混合醌类物质是指含有不同类型醌基团的化合物。
这些化合物具有更加复杂和多样的结构,因此在化学性质上也表现出更多样化的特性。
苯并三恶唑四酮(简称BTO)就是一种混合醌类物质。
BTO分子中,同时含有苯环和三恶唑环上的两个酮基团。
5. 衍生物除了以上几种基本类型的醌类物质外,还存在许多衍生物。
衍生物是通过在原始醌类分子中引入其他官能团而得到的化合物。
这些官能团可以改变醌类物质的化学性质和应用领域。
羟基苯醌和氨基苯醌就是苯醌的两种衍生物。
它们在染料工业和药物工业中具有广泛的应用。
总结起来,根据醌基团的位置和化学性质,醌类物质可以分为单醌类、双醌类、多醌类、混合醌类和衍生物等不同结构类型。
这些不同类型的醌类物质具有不同的化学性质和应用领域,对于进一步研究和开发新型有机材料具有重要意义。
参考文献: 1. Bredas, J.L. (2010). Organic semiconductors: A theoretical characterization of the basic parameters governing charge transport.Proceedings of the National Academy of Sciences, 107(9), 3904-3909. 2. Li, Y., et al. (2019). A general strategy for designing high-performance organic thermoelectric materials. Science Advances, 5(6), eaaw0911. 3. Liu, Y., et al. (2020). Recent advances in n-type organic semiconductors for organic field-effect transistors. Journal of Materials Chemistry C, 8(10), 3317-3336. 4. Wang, X., et al. (2019). High-performance organic field-effect transistors based on naphthalene diimide derivatives: Recent advances and perspectives. Advanced Materials Interfaces, 6(5), 1801895.。
氧化芳基偶联和Scholl反应的比较
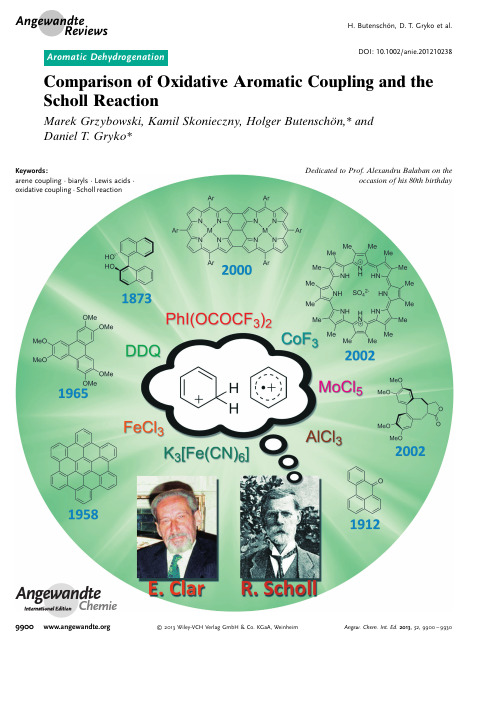
DOI:10.1002/anie.201210238Comparison of Oxidative Aromatic Coupling and the Scholl ReactionMarek Grzybowski,Kamil Skonieczny,Holger Butenschçn,*and Daniel T.Gryko*AngewandteChemieKeywords:arene coupling ·biaryls ·Lewis acids ·oxidative coupling ·Scholl reactionDedicated to Prof.Alexandru Balaban on theoccasion of his 80th birthday.Angewandte ReviewsH.Butenschçn,D.T.Gryko et al.99002013Wiley-VCH Verlag GmbH &Co.KGaA,WeinheimAngew.Chem.Int.Ed.2013,52,9900–99301.IntroductionThe first example of an oxidative dimerization of aromatic compounds was published in 1871,[1]and 39years later Roland Scholl reported that a similar effect can be achieved by heating certain aromatic compounds with AlCl 3.[2]For many years,these two reactions were distinguishable,and when Balaban and Nenitzescu published their fundamental review on the Scholl reaction,there was still a clear demar-cation between them.[3]Nowadays,however,there is a mix-up in the literature,and the oxidative coupling of electron-rich aromatic substances is often called the Scholl reaction.The aim of this Review is to summarize the development of both reactions,to discuss their mechanisms,and to show their current scope.We will present the historical origin of both of these processes in Section 2,followed by a discussion of their mechanisms in Section 3.In that section we will discuss the similarities and differences between reactions mediated by AlCl 3and reactions mediated by typical oxidants in terms of scope and electronic requirements.[4]In the following two sections we will present representative examples of the Scholl reaction (Section 4),intramolecular oxidative coupling,and intermolecular oxidative coupling reactions (Section 5).We will focus on the most important examples,with special emphasis given to the recent literature.Palladium-catalyzed oxidative aromatic cross-coupling and dehydrogenative cou-pling by C ÀH activation by organometallic catalysts are not included in this Review.[5]2.Historical Development2.1.Oxidative Aromatic CouplingThe first known example of the oxidative coupling of aromatic compounds,the formation of ellagic acid (2)from gallic acid (1),was published in 1868(Scheme 1).[1]The reaction was mediated by H 3AsO 4or Ag 2O;however,the yield was not reported.Other examples quickly followed,and in the 1870s it was shown that a variety of phenols and phenyl ethers can be coupled oxidatively using one-electron oxidants such as FeCl 3or K 3[Fe(CN)6].Seminal examples include the synthesis of 1,1’-bi-2-naphthol (binol,4)by the oxidation of 2-naphthol (3)with FeCl 3reported in 1873by Dianin (Scheme 2).[6b]Progress continued into the 20th century and accelerated after the discovery of the role of oxidative aromatic coupling in biogenesis.[7]The early literature has been summarized by[*]M.Grzybowski,K.Skonieczny,Prof.Dr.D.T.GrykoInstitute of Organic Chemistry,Polish Academy of Sciences Kasprzaka 44/52,Warsaw (Poland)E-mail:dtgryko@.pl Prof.Dr.H.ButenschçnInstitut für Organische Chemie Leibniz Universität,HannoverSchneiderberg 1B,30167Hannover (Germany)Prof.Dr.D.T.GrykoFaculty of Chemistry,Warsaw University of Technology 00-664Warsaw (Poland)D oes the dehydrogenative coupling of aromatic compounds mediatedby AlCl 3at high temperatures and also by FeCl 3,MoCl 5,PIFA,or K 3[Fe(CN)6]at room temperature proceed by the same mechanism in all cases?With the growing importance of the synthesis of aromatic compounds by double C ÀH activation to give various biaryl structures,this question becomes pressing.Since some of these reactions proceed only in the presence of non-oxidizing Lewis acids and some only in the presence of certain oxidants,the authors venture the hypothesis that,depending on the electronic structure of the substrates and the nature of the “catalyst”,two different mechanisms can operate.One involves the intermediacy of a radical cation and the other the formation of a sigma complex between the acid and the substrate.The goal of this Review is to encourage further mechanistic studies hopefully leading to an in-depth understanding of this phenomenon.From the Contents1.Introduction99012.Historical Development 99013.Mechanistic Considerations 99034.Scholl Reaction—Scope,Limitations,and Utilization 99065.Oxidative Aromatic Coupling 99076.Summary and Outlook9926Scheme 2.Scheme 1.Biaryl Synthesis9901Angew.Chem.Int.Ed.2013,52,9900–9930 2013Wiley-VCH Verlag GmbH &Co.KGaA,WeinheimHeuben,[8]and later examples have also been extensively reviewed.[9,10]2.2.Scholl ReactionThe Scholl reaction was first mentioned as early as 1910,when Scholl and Mansfeld reported the transformation of quinone 5to the p -extended quinone 6by treatment with an excess of neat anhydrous aluminum chloride for 45min at 140–1458C as a clean reaction,although no yield was given (Scheme 3).The authors mentioned that such a reaction had been observed earlier,for example in the formation of 1,1’-binaphthalene by heating naphthalene with aluminum chlo-ride,but they emphasized that the observation of the formation of quinone 6under comparatively mild reaction conditions was new.[2]In a subsequent publication,this reaction was applied to the synthesis of perylene (8)from 1,1’-binaphthalene (7;Scheme 4).Interestingly,Homer de-scribed the same reaction at the same time,but without the true formula of the product 8.[11]Perylene (8)was also obtained from naphthalene (9)without isolation of the intermediate 7;however,because of some decomposition,the yield of 8was poor.[12]Later,the reaction of 4,4’-dicyano-1,1’-binaphthalene was reported to give the corresponding coupling product in 72%yield.[13]The method was further elaborated and led to the synthesis of benzanthrone (11)from ketone 10and of compound 13from 12(no yield was given in the last case,Scheme 5).[14,15]Numerous other examples were published by Scholl and co-workers in the following years.[16,17]Possible mechanisms were discussed in some of the early reports on the dehydrogenative coupling of aromatic com-pounds by treatment with anhydrous aluminum chloride;these mechanisms were mainly based on similarities to Friedel Crafts reactions.[15]The original procedure by Scholl required baking the organic substrate with AlCl 3.This procedure was soon replaced by Kränzlein and Vollmann,who used an equimolar mixture of AlCl 3and NaCl,which is liquid above 1008C at a low vapor pressure,[18]and this procedure predominates in the later literature.Since the early 1920s,this reaction has been utilized in the industrial synthesis of many antraqui-none-derived dyes.It is difficult to overestimate the technical importance of the Scholl reaction.Indanthrene khaki 2G (15)Scheme 3.Scheme 4..Angewandte ReviewsH.Butenschçn,D.T.Gryko et al.2013Wiley-VCH Verlag GmbH &Co.KGaA,Weinheim Angew.Chem.Int.Ed.2013,52,9900–9930has been produced in millions of kilograms from 14(Scheme 6).[3,19]After World War II,the focus shifted and the Scholl reaction started to be used in the synthesis of extended aromatic hydrocarbons.This new direction sparked methodo-logical developments.In 1971,Wick revealed that the cyclization of di-(1-anthraquinonyl)amine into 1,2;7,8-di-phthaloylcarbazole (C.I.Vat Yellow 28)proceeds more efficiently in the presence of an AlCl 3/pyridine complex than in an AlCl 3/NaCl melt.[20]Other variants include the use of AlCl 3in high-boiling solvents such as dichlorobenzene and trichlorobenzene,[21]low-melting complex AlCl 3/SO 2,[22]or of ZrCl 4.[23]Needless to say,many protocols involve the addition of a certain amount of oxidant such as air,oxygen,nitro-benzene,or potassium m -nitrobenzene sulfonate.[24]In 1961,Kovacic and Kyriakis introduced new conditions for the oxidative polymerization of benzene (AlCl 3/CuCl 2/neat),[25a]which were later modified by Müllen and co-workers (AlCl 3/CuCl 2/CS 2[25b]and AlCl 3/Cu(OTf)2/CS 2),[25c]thus allowing the reaction temperature to be lowered to 258C.3.Mechanistic ConsiderationsNumerous examples of dehydrogenative coupling reac-tions of aromatic compounds in the presence of various Lewis acids have recently been published.[26]In most cases,they are assigned as Scholl reactions.[27]Here,we come to the critical question of whether there is a difference between oxidative aromatic coupling and the Scholl reaction.The typical oxidative aromatic coupling relates to the reaction of electron-rich aromatic compounds such as phe-nols,alkyl aryl ethers and the like.The broadly accepted mechanism is shown in Scheme 7.It involves the formation ofa radical cation from one molecule of the substrate followed by substitution at the neutral second molecule and finally convergence to the biaryl product.This mechanism implies that:1)the substrate is reasonably electron rich and 2)the attack of the electrophilic radical cation occurs at the most electron-rich position of the second substrate molecule.Other mechanistic pathways have also been discussed for the coupling of phenols,such as oxidation to the radical followed by dimerization and radical substitution.[10]According to the definition proposed in very early reviews,the Scholl reaction is a dehydrogenation of aromatic nuclei under the influence of aluminum chloride that results in the formation of a condensed ring system.[28]Balaban and Nenitzescu reformulated it as “the elimination of two aryl-bound hydrogens accompanied by the formation of an aryl–aryl bond under the influence of Friedel–Crafts catalysts”.[3]Baddeley was the first to propose that the mechanism of the Scholl reaction involves the formation of a s complex between the Lewis acid with the aromatic compound followed by the formation of an arenium cation,an electrophilic attack,and finally a dehydrogenation.[29]This hypothesis was further reformulated by Nenitzescu and Balaban.[30]Kenner,on the other hand was the first to propose a radical cation mechanism for the Scholl reaction.[31]This concept was soon supported by Rooney and Pink [32]and later by Clover.[33]The arenium cation mechanism implies the protonation of the aryl species,for example,7,to form an electrophilic s complex 7’(shown with H +for simplicity,but this couldalsoScheme5.Scheme6.Scheme 7.Biaryl Synthesis9903Angew.Chem.Int.Ed.2013,52,9900–9930 2013Wiley-VCH Verlag GmbH &Co.KGaA,Weinheimbe a s complex with a Lewis acid;Scheme 8).The attack of the latter species at the other aromatic ring to form a new carbon–carbon bond (7’’)followed by hydrogen elimination regenerates the aromatic system,finally giving 8.Numerous experimental results supported this mecha-nism.Several research groups observed that dehydrogenation of certain aromatic compounds can occur not only in the presence of AlCl 3and similar Lewis acids,but also in media such as anhydrous HF [34,35]or PhSO 3H,[30]while radical cations cannot be formed under such conditions.Baddeley and Kenner observed that the presence of hydrogen chloride is essential for the synthesis of benzanthrone (11)to occur.[29]Electronic and steric effects in various positions play a con-siderable role in intramolecular cases.Remarkably,benzo-phenone does not yield fluorenone upon heating with AlCl 3at 180–2208C,[15]most likely because this would involve an electrophilic aromatic substitution at the ortho position relative to the carbonyl group.On the other hand,milder reaction conditions (1008C)allowed the synthesis of phenan-threnequinone from benzil.[14]The third step,that is,dehy-drogenation/aromatization,is the most controversial one,since studies have shown that only a substoichiometric amount of H 2was evolved when ketones were subjected to Scholl reaction conditions.To the best of our knowledge,no such study has so far been performed on hydrocarbons.There is an unquestionable positive influence on the yield of products from the Scholl reaction when hydrogen acceptors are added to AlCl 3.Various examples include O 2(conversion of 3,8-dibenzoylpyrene into pyranthrone,25%versus 80%;[36]conversion of 1,5-dibenzoylnaphthalene into 2,3;7,8-dibenzo-pyrene-1,6-quinone),[10]and nitrobenzene (intermolecular reaction of ethyl 1-naphthyl ether,0%versus 70%).[37]Importantly,the carbonyl group present in many Scholl reaction precursors can serve as a temporary oxidizing agent.In such cases,the corresponding secondary alcohol can be an intermediate,which is then reoxidized by O 2to a ketone.Balaban and Nenitzescu argue that this may be the reason for the high yields of the intramolecular Scholl reactions with these ketones,despite the electron-withdrawing (hence deactivating)character of the carbonyl group.[3]While discussing possible pathways of rearomatization,one has to remember that AlCl 3itself can catalyze the dehydrogenation of compounds such as 9,10-dihydroanthracene.[37]The second mechanism advocated by Kenner,Rooney,and Clover implies the formation of radical cations (Scheme 9).Although the mechanism has been studied only occasionally,the schism continued,and in recent years an intense discussion has evolved,prominently between the research groups of King and Rathore.The differentiationbetween the two mechanisms is clearly not a trivial issue.One of the key difficulties lies in the fact that most of the Lewis acids used in the Scholl reaction are also milder or stronger oxidants.Moreover,aromatic hydrocarbons can also form paramagnetic species in the presence of non-oxidizing AlCl 3.[32]The problem with the most commonly used oxidant,namely FeCl 3,is that this compound is both a Lewis acid and an oxidant capable of catalyzing or mediating a variety of reactions.For this reason,experiments with FeCl 3or MoCl 5cannot give the definitive answer to the key mechanistic question.One of the iron complexes broadly used in oxidative aromatic coupling,[9]which cannot be considered a Lewis acid,is K 3[Fe(CN)6],but its relatively low oxidation potential does not allow for direct comparative studies in many cases.King and co-workers published a series of reports that presented both computational and experimental evidence supporting the arenium ion mechanism.[38]Computational studies led to the conclusion that the mechanistic pathway involving the arenium cation is thermodynamically favored under both vacuum and solvated conditions,because of the transition states have lower energy than those found in the radical cation pathway.Additionally,the authors presented computational evidence showing that in the case of the oxidation of hexaphenylbenzene to hexa-peri -hexabenzocor-onene,the formation of the first C ÀC bond is the slowest.This explains the lack of observed intermediates in this process.One has to emphasize,however,that the interaction of the reagents with the organic substrate was not taken into account in these calculations.Given that this would probably bring about considerable energy differences,more advanced com-putational methods have to be applied to gain a more in-depth understanding of this process.Rathore and co-workers studied the reaction of electron-rich aromatic compounds in the presence of various oxidants in detail,focusing on DDQ-MeSO 3H.[39]The same system had been used previously by these authors to efficiently synthesize a number of triphenylenes and hexa-peri -hexabenzocoro-nenes under mild conditions.[40]They presented some impor-tant evidence suggesting that,for many o -terphenyls such as 19,the reaction indeed proceeds via radical cation inter-mediates such as 19 and 19 (Scheme 9).First theyshowedScheme8.Scheme 9..Angewandte ReviewsH.Butenschçn,D.T.Gryko et al.2013Wiley-VCH Verlag GmbH &Co.KGaA,WeinheimAngew.Chem.Int.Ed.2013,52,9900–9930that various Scholl precursors with oxidation potentials <1.7V versus the SCE readily undergo oxidative C ÀC bond formation with DDQ/H +as the oxidant,whereas those with oxidation potentials >1.7V versus the SCE do not react.Additionally,they noted that the reaction does not occur in mixtures of CH 2Cl 2and various acids.These authors also claimed that the necessity of using strong oxidants for this reaction to occur is inconsistent with the arenium ion mechanism,since oxidation of dihydro intermediates such as 19a (formed through the arenium ion mechanism,Scheme 9)can easily be accomplished even with molecular oxygen.They did not,however,extend their study further to include compounds with lower oxidation potentials and/or larger aromatic systems.In our opinion,one of the most important compounds with a behavior that may help to understand the difference between typical oxidative aromatic coupling and the Scholl reaction is 2,2’-dihydroxy-1,1’-binaphthyl (4).This compound is formed by the oxidative aromatic coupling of 2-naphthol (3,Scheme 2).Subjecting 4to further portions of FeCl 3is ineffective,regardless of the conditions.In contrast,as early as in 1922,Zinke and Dengg performed the reaction of both 2,2’-dihydroxy-1,1’-binaphthyl (4)and 2,2’-dimethoxy-1,1’-binaphthyl (21)with AlCl 3,which resulted in the formation of perylene-1,12-diol (22),thus involving a cleavage of the ether functions in the case of 21(Scheme 10).[41]The authors preferred to start from the dimethoxy derivative 21as thereaction was initially much more sluggish when starting from the respective diol 4,presumably because of side reactions of the hydroxy functions with AlCl 3.The reaction conditions are remarkable,because neat 4/21and a fourfold excess of AlCl 3are mixed and melted at 140–1508C for 1h,with no solvent being used.Phenol 22undergoes oxidation by basic solutions in air with the formation of the respective quinone 23.[42]Later,the authors reported the synthesis of crystalline 23by oxidation of the crude product of quinone 22with lead(IV)oxide,which was purified by crystallization.Subsequent reduction of 23with zinc dust or sodium dithionite affords 22in pure form as bright yellow leaves,which become green on standing in air over longer periods of time.[43]It is clear that the highest electron density in compound 4is present at positions 3and 3’,and indeed electrophilic aromatic substi-tutions were performed at these positions.Oxidative aromatic coupling proceeding through the radical cation mechanism is very sensitive to the distribution of the electron density within the molecule.Numerous examples show that it proceeds:1)only if the overall electron density of the aromatic molecule is relatively high and 2)only at the position wherethe electron density is the highest.Consequently,the reaction of diol 4with FeCl 3,which tends to form radical cations,cannot proceed with carbon–carbon bond formation at positions 8and 8’(which possess rather moderate electron density).On the other hand,the reaction in the presence of AlCl 3is apparently less sensitive.It can proceed even when the overall electron density is significantly lower (the trans-formation of ketone 10into benzanthrone 11is a good example),and it can also proceed at positions which are not the most electron rich ones in the molecule (especially in an intramolecular fashion).The preparation of 1,12-dihydroxy-perylene (22)from (1,1’-binaphthalene)-2,2’-diol (4)through the action of AlCl 3was later patented by various authors.[44,45]The reactivity of naphthylisoquinolines is another exam-ple that emphasises this fundamental mechanistic difference stated above.We recently discovered the synthesis of 1-azaperylene (25)by anion radical coupling of two regioiso-meric naphthylisoquinolines,24and 26.[46]These substrates seemed to us to be perfect models to study the interrelation between AlCl 3-mediated reactions and FeCl 3-mediated reac-tions,since the oxidation potentials of naphthalene and isoquinoline are rather high.Indeed,both substrates are inert in the presence of stoichiometric or excess amounts of FeCl 3at 258C and 808C.[47]On the other hand,compound 24reacts in a AlCl 3/NaCl (5:1)melt at 1608C to afford 1-azaperylene (25)in 68%yield (Scheme 11).[47]In the case of 8-(naph-thalen-1-yl)isoquinoline (26),such a reaction would require an electrophilic attack at position 1of the isoquinoline moiety,which is very electron poor.Hence,submitting compound 26to Scholl reaction conditions does not result in the formation of 1-azaperylene (25).[47]An analogous example from our research group is the reaction of compound 27in the presence of AlCl 3/NaCl to afford p -extended coumarin 28in 30%yield (Scheme 12).[48]Again,the reaction of coumarin 27with FeCl 3does not proceeded,most likely because of the electronic effectsScheme 11.Scheme 12.Scheme 10.Biaryl Synthesis9905Angew.Chem.Int.Ed.2013,52,9900–99302013Wiley-VCH Verlag GmbH &Co.KGaA,Weinheimimparted by the OH group,which does not activate a suitable position for reaction to occur.In the context of the recent discussion concerning the mechanism of the dehydrogenation of aromatic compounds under various conditions,it seems that processes that occur at room temperature with a well-known one-electron oxidant (sometimes mild Lewis acids such as FeCl 3or MoCl 5)and processes which proceed at 120–1608C in the presence of AlCl 3(a strong Lewis acid)most probably undergo a different mechanism.We venture to say that the radical cation mechanism is operating in the first case and the arenium cation mechanism in the second case.The experiments by Nenitzescu and Balaban that show,in some cases,that dehydrogenation proceeds in the presence of a Brønsted acid (and nitrobenzene as the co-oxidant)supports this line of thought.[30]The reaction mediated by AlCl 3can generally proceed with substrates less electron rich than those for oxidative aromatic coupling.4.Scholl Reaction—Scope,Limitations,and UtilizationThe earliest examples of the Scholl reaction focused on the synthesis of large polyaromatic ketones and quinones.1-Benzoylpyrene is cyclized in an AlCl 3/NaCl melt to furnish dibenzo[def ,qr ]chrysene-8-one in approximately 40%yield.[36]The first example of multiple dehydrogenative coupling was reported by Scholl and Seer in 1913.The authors treated 4,4’-dibenzoyl-1,1’-binaphthyl (29)with AlCl 3for 8.5h at 95-1008C and obtained the nonacyclic dione “violanthrone”(30);however,again no yield was reported (Scheme 13).Oneof the largest systems ever synthesized by the Scholl reaction is quinone 32prepared from ketone 31(no yield given,Scheme 14).[49]The dehydrogenation of aromatic hydrocarbons can also take place upon melting in a vacuum (no yield given,Scheme 15).[50]Müllen and co-workers published a very interesting study showing that 3-(1-naphthyl)perylene (36)undergoes oxida-tive coupling to either 35or 37depending on the reaction conditions (Scheme 16).[51]Compound 36in the presence ofFeCl 3in dichloromethane forms 37in 46%yield,while an analogous reaction with AlCl 3in chlorobenzene gives rise to terrylene (35)in 43%yield.This result further emphasizes that the cyclization of aromatic hydrocarbons in the presence of oxidizing and non-oxidizing Lewis acids usually proceeds by different mechanisms.Cyclization of 12-(1-naphthyl)benz[a ]anthracene (39)in an AlCl 3/NaCl melt furnishes benzo[def ]naphtha[1,2-p ]chrys-ene (38;10%).However,if AlCl 3/SnCl 4is used,the main product is naphtho[1,2-a ]perylene (40;60%,Scheme 17).[52]These results further indicate that the nature of the reactive intermediates can vary quite significantly on changing the reaction conditions slightly.Scheme 13.Scheme 14.Scheme 15.Scheme 16.Scheme 17..Angewandte ReviewsH.Butenschçn,D.T.Gryko et al.2013Wiley-VCH Verlag GmbH &Co.KGaA,WeinheimAngew.Chem.Int.Ed.2013,52,9900–99302,3,4,5-Tetraphenylthiophene (41),when subjected to classical Scholl conditions (AlCl 3/NaCl,4:1),gives flavophen (42)in 26%yield (Scheme 18).[53]The reaction can be applied to heterocyclic systems,as has impressively been shown in an American patent disclosing the reaction of N -acylurea 43to afford pentacycle 44(no yield given,Scheme 19).[54]Weitzenbçck and Seer reported that dinaphtho[2,1-b :1’,2’-d ]furan undergoes cyclization to peryleno[1,12-bcd ]furan in the presence of AlCl 3,with the concomittent formation of perylen-1-ol.[13]Polyphosphoric acid has been used to induce the cyclization of precursor 45(Scheme 20).[55]Amino-substituted benzo[g ,h ,i ]perylene 46forms in excellent yield in a domino reaction involving an electrophilic sub-stitution followed by a Scholl reaction.5.Oxidative Aromatic Coupling5.1.Intermolecular Oxidative Aromatic CouplingAs stated above (see Section 3and Scheme 7),the reactions of reasonably electron-rich arenes with oxidants that lead to the formation of biaryls usually proceed through the radical cation mechanism.The term “oxidative coupling”will be used in this and the following sections for such reactions.The simplest variant of the oxidative coupling reaction is intermolecular oxidative homocoupling,in whichtwo molecules of one aromatic compound react to form a biaryl.The oxidative cross-coupling of aromatic compounds (the reaction between two different arenes)is much more difficult to achieve.This is due to the poor selectivity of the reaction,which strongly depends on steric factors as well as on the electron density of both aromatic molecules.The con-ditions must carefully be selected to avoid homocoupling.[56]Nonetheless,significant progress has been made in the field of intermolecular oxidative cross-coupling over recent years,and many interesting examples have been published.Some representative examples of intermolecular oxidative homo-and cross-coupling reactions of arenes from the recent literature are presented in the following sections.5.1.1.Homocoupling of Naphthalene DerivativesThe oxidative coupling of aromatic compounds often leads to the formation of axially chiral products.Structures of many widely used optically active catalysts are based on biaryl units.[57]Among them,binaphthyl and its derivatives are particularly important.1,1’-Bi-2-naphthol (4),whose first synthesis was described in Section 2.1,is a precursor of many important ligands used in asymmetric catalysis,with binap being the most prominent example.[58]The syntheses of binol (4)and other binaphthyls by the oxidative coupling of the corresponding naphthalenes were achieved under various conditions,and racemic products as well as pure enantiomers could be obtained.Many different catalytic systems,oxidants,and metal complexes were examined for this purpose.Besides the classical systems with FeCl 3,reasonable yields of binaphthyls were also achieved using reactants such as thallium(III)and mercury(II)trifluoroacetates,Pb(OAc)2,and CoF 3,[59]titanium(IV)chloride,[60]or CuCl 2in the presence of amines.[61]Particularly interesting are the cata-lyzed homocoupling reactions of naphthalenes to binaphthyls,for example,peroxidase-catalyzed oxidation with H 2O 2,[62]copper(I)-or copper(II)-catalyzed oxidation under oxygen or air,[63]as well as the oxidation catalyzed by methyltrioxo-rhenium.[64]The syntheses of various binaphthyls have been summarized in recent reviews.[65]Only a few recent examples are presented below.Shaw and co-workers employed a vanadium catalyst in the air oxidation of the chiral naphthol 47to binaphthol 48,a key intermediate in the synthesis of (À)-viriditoxin,which is a promising inhibitor of bacterial cell division.[66]The reaction in the presence of [VO(acac)2]as the catalyst gave the product 48in 67%yield with rather low diastereoselectivity (76:24).When [VO(acac)2]was replaced by the enantiopure chiral catalysts 49,which is a binol derivative,both the reaction yield and the diastereoselectivity substantially increased (Scheme 21).Many interesting copper complexes for the oxidative coupling of naphthols were developed by Kozlowski and co-workers,and and have successfully been applied in the total syntheses of numerous chiral,natural binaphthyl derivatives with high enantioselectivity.[65e,67]Wang and co-workers recently reported two new systems which are efficient in the oxidation of various 2-naphthols into the corresponding racemic binaphthols (Table 1).In thefirstScheme18.Scheme19.Scheme 20.Biaryl Synthesis9907Angew.Chem.Int.Ed.2013,52,9900–99302013Wiley-VCH Verlag GmbH &Co.KGaA,Weinheim。
溶剂效应对D-π-A-π-D型萘基衍生物光电性质的影响
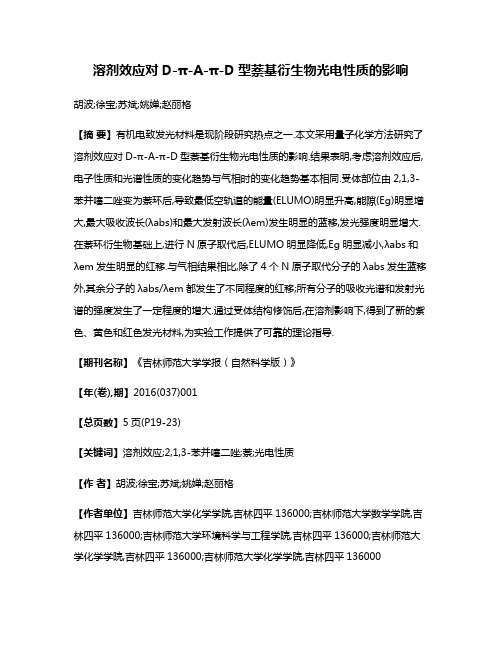
溶剂效应对D-π-A-π-D型萘基衍生物光电性质的影响胡波;徐宝;苏斌;姚婵;赵丽格【摘要】有机电致发光材料是现阶段研究热点之一.本文采用量子化学方法研究了溶剂效应对D-π-A-π-D型萘基衍生物光电性质的影响.结果表明,考虑溶剂效应后,电子性质和光谱性质的变化趋势与气相时的变化趋势基本相同.受体部位由2,1,3-苯并噻二唑变为萘环后,导致最低空轨道的能量(ELUMO)明显升高,能隙(Eg)明显增大,最大吸收波长(λabs)和最大发射波长(λem)发生明显的蓝移,发光强度明显增大.在萘环衍生物基础上,进行N原子取代后,ELUMO明显降低,Eg明显减小,λabs和λem发生明显的红移.与气相结果相比,除了4个N原子取代分子的λabs发生蓝移外,其余分子的λabs/λem都发生了不同程度的红移;所有分子的吸收光谱和发射光谱的强度发生了一定程度的增大.通过受体结构修饰后,在溶剂影响下,得到了新的紫色、黄色和红色发光材料,为实验工作提供了可靠的理论指导.【期刊名称】《吉林师范大学学报(自然科学版)》【年(卷),期】2016(037)001【总页数】5页(P19-23)【关键词】溶剂效应;2,1,3-苯并噻二唑;萘;光电性质【作者】胡波;徐宝;苏斌;姚婵;赵丽格【作者单位】吉林师范大学化学学院,吉林四平136000;吉林师范大学数学学院,吉林四平136000;吉林师范大学环境科学与工程学院,吉林四平136000;吉林师范大学化学学院,吉林四平136000;吉林师范大学化学学院,吉林四平136000【正文语种】中文【中图分类】O640有机电致发光材料应用范围广泛,已经成为现阶段研究热点之一.量子化学方法作为材料科学中不可或缺的一种重要研究手段,通过研究有机电致发光材料的结构与性能间的关系,可以从理论上评价分子的结构与性能,为新型有机电致发光材料的设计提供理论依据[1-5].在前期工作[6-11]中已经知道,对给体-π桥-受体-π桥-给体(D-π-A-π-D)型分子中给体或受体结构进行修饰后,母体分子的光电性质会得到调节,从而得到新的发光材料.因此,在前期工作基础上,本文主要研究溶剂(二氯甲烷)效应对D-π-A-π-D型萘基衍生物光电性质的影响.在前期工作基础上[6-11],本文主要研究溶剂效应对D-π-A-π-D型萘基衍生物光电性质的影响.文中研究分子的结构示于图1中.采用ab initio Hartree-Fock(HF)[12]方法进行基态(S0)分子结构优化.在已优化的S0结构基础上,S0的电子性质采用密度泛函理论 (DFT)[13]PBE0[14]方法计算.采用单组态相互作用(CIS)[15]方法进行激发态(S1)分子结构优化.在已优化的S0和S1的结构基础上,吸收和发射光谱运用含时DFT[16](TD-DFT) PBE0方法分别计算.计算中使用的基组是6-31G(d)[17].采用自洽反应场 (SCRF) 方法中的极化连续模型(PCM)[18]计算了考虑溶剂相(二氯甲烷)时分子的电子性质、吸收和发射光谱.计算均采用Gaussian03W[19]中的程序进行.为了更好地分析计算数据,在表1中同时列出了研究分子的溶剂相和气相两种情况的基态和激发态的最高占据轨道能量 (EHOMO),最低空轨道能量(ELUMO) 以及能隙(Eg).图2中给出了溶剂相HOMO和LUMO的能级图.从图2和表1中可以看出,考虑溶剂效应后,基态和激发态时EHOMO、ELUMO 和Eg的变化趋势与气相时的变化趋势基本相同.基态时,与母体分子OMC相比(-5.19 eV,-2.05 eV,3.14 eV),受体部分由2,1,3-苯并噻二唑(BTD)变成萘环后,BMC的EHOMO少量降低(-5.23 eV),ELUMO明显升高(-0.96 eV),导致Eg明显增大(4.27 eV).激发态时,与OMC相比(-4.84 eV,-2.42 eV,2.42 eV),BMC的EHOMO少量降低(-4.85 eV),ELUMO明显升高(-1.58 eV),Eg明显增大(3.27 eV).在BMC基础上进行两个N原子取代.基态时,与BMC相比,BMC-2N的EHOMO少量升高(-5.19 eV),ELUMO明显降低(-1.71 eV),导致Eg明显减小(3.48 eV).激发态时,与BMC相比,BMC—2N的EHOMO少量降低(-4.87 eV),ELUMO明显降低(-2.12 eV),Eg明显减小(2.75 eV).在BMC基础上进行四个N原子取代.基态时,与BMC相比,BMC—4N的EHOMO少量降低(-5.26 eV),ELUMO明显降低(-2.29 eV),导致Eg明显减小(2.97 eV).激发态时,与BMC相比,BMC—4N的EHOMO少量降低(-4.99 eV),ELUMO明显降低(-2.67 eV),Eg明显减小(2.32 eV).显然,与BMC相比,N取代数越多,EHOMO和ELUMO降低的程度越大,Eg越小.与气相相比较,考虑溶剂相后,EHOMO和ELUMO少量降低,Eg变化不大.体系的光电性质与前线分子轨道电子密度分布密切相关.OMC、BMC、BMC-2N 和BMC-4N的溶剂相和气相的基态和激发态HOMO和LUMO电子密度分布图示于图3中.考虑溶剂效应后,在基态,如图3a所示,OMC、BMC、BMC—2N和BMC—4N的LUMO、HOMO具有相似的电子密度分布.LUMO中,电子密度大部分分布在受体部位上,分别为BTD环、萘环、萘的氮取代衍生物;HOMO中,电子密度主要分布在三芳胺(TPA)上.在激发态,如图3b所示,OMC、BMC、BMC—2N和BMC—4N的LUMO中,和基态相同,电子密度大部分分布在受体部位上,分别为BTD环、萘环、萘的氮取代衍生物.但是激发态的HOMO中,电子密度分布与基态不同,电子密度主要分布在BTD/萘/萘的氮取代衍生物的苯环和TPA的π-桥苯环上.产生这种现象的原因是激发态与基态结构的不同.从基态到激发态,分子结构中,BTD/萘/萘的氮取代衍生物的苯环和TPA的π-桥苯环之间的二面角明显减小,增加了BTD/萘/萘的氮取代衍生物与TPA之间的共轭程度,从而使激发态HOMO中的电子密度分布与基态不同.由图3a、3b和3c、3d的比较可以看出,考虑溶剂效应后,HOMO和LUMO电子密度分布与气相情况相似,没有发生明显的变化,说明溶剂对HOMO和LUMO电子密度分布的影响极小.为了更好地分析计算数据,在表2中同时列出了研究分子OMC、BMC、BMC—2N和BMC—4N的溶剂相和气相两种情况的的垂直激发能(Ev),最大吸收/发射波长(λabs/λem),以及振子强度(f).为了更清楚地了解吸收和发射光谱的变化情况,图4中给出了吸收和发射光谱图.表2显示,考虑溶剂效应后,与OMC相比(483.1 nm,651.4 nm),BMC的λabs和λem发生明显的蓝移(340.3 nm,461.1 nm),蓝移的程度为142.8 nm 和190.3 nm.吸收和发射光谱发生蓝移与Eg的明显增大相对应,可以把λabs/λem的变化归因于Eg的变化,因为吸收/发射过程中对应的电子跃迁主要是HOMO→LUMO/LUMO→HOMO.在BMC基础上,进行N原子取代后,BMC—2N的λabs和λem为426.9和568.0 nm,BMC—4N的λabs和λem为513.4 和684.5 nm.显然,与BMC 相比,BMC—2N和BMC—4N的λabs和λem发生明显的红移,红移的程度分别为86.6、106.9 nm和173.1、223.4 nm,明显看出,N取代数越多,λabs和λem红移的程度越大.N原子取代后,吸收和发射光谱发生红移与Eg的明显减小相对应,也可以把λabs/λem的变化归因于Eg的变化,因为吸收/发射过程中对应的电子跃迁主要是HOMO→LUMO/LUMO→HOMO.由振子强度的比较可以看出,与OMC相比(0.47,0.90),BMC的吸收和发射光谱的强度明显增大(0.70,1.48),说明受体由BTD环变为萘环后,有利于光谱发光强度的增大.因为BMC、BMC—2N和BMC—4N 的λem分别处于紫色、黄色和红色光谱范围,说明通过结构修饰后,得到了新的发光材料.从HOMO和LUMO的电子密度分布可以确定吸收/发射跃迁具有电荷转移特征(见图3a和3b).溶剂相与气相结果相比,除了BMC—4N的λabs发生蓝移(蓝移的程度为7.3 nm)外,其余分子的λabs/λem都发生了不同程度的红移(红移的程度为1.1~34.3 nm).从振子强度可以看出,在溶剂相中,吸收光谱和发射光谱的强度都发生了一定程度的增大.采用量子化学方法研究了溶剂效应对D-π-A-π-D型萘基衍生物光电性质的影响.结果表明,考虑溶剂效应后,电子性质和光谱性质的变化趋势与气相时的变化趋势基本相同.受体部位由BTD环变为萘环后,导致ELUMO明显升高,Eg明显增大,λabs和λem发生明显的蓝移,发光强度明显增大;在萘环衍生物基础上进行N原子取代后,ELUMO明显降低,Eg明显减小,λabs和λem发生明显的红移.与气相结果相比,除了BMC—4N的λabs发生蓝移外,其余分子的λabs/λem都发生了不同程度的红移;所有分子的吸收光谱和发射光谱的强度都发生了一定程度的增大.通过受体结构修饰后,得到了新的紫色、黄色和红色发光材料,为实验工作提供了可靠的理论指导.【相关文献】[1]Han D,Zhang G,Li T,Li H G,Cai H X,Zhang X H,Zhao L H.Theoretical study on the electronic structures and phosphorescence properties of five osmium(II) complexes with different P^P ancillary ligands[J].Chemical Physics Letters,2013,573:29~34.[2]Han D,Li C Y,Zhang L H,Sun X P,Zhang G.Theoretical investigation on the electronic structures and phosphorescent properties of seven iridium(III) complexes with the different substituted 2-phenylpyridinato ancillary ligand[J].Chemical PhysicsLetters,2014,595-596:260~265.[3]Zhu Y L,Ma K R,Yin Q F,Zhong X D,Cao L.A new D-A derivative with a 1,3-dithiol-2-ylidene unit:Structural,spectroscopic and theoretical studies[J].Polyhedron,2013,63:36~40.[4]Sun F Y,Jin R F.Optical and charge transport properties of N-butyl-1,8-naphthalimide derivatives as organic light-emitting materials:A theoretical study[J].Journal of Luminescence,2014,149:125~132.[5]Saranya G,Navamani K,Senthilkumar K.A theoretical study on optical and charge transport properties of anthra-[1,2-b:4,3-b′:5,6-b″:8,7-b‴]tetrathiophenemolecules[J].Chemical Physics,2014,433:48~59.[6]胡波,董明明.给、吸电子取代基对2,1,3-苯并噻二唑衍生物的电子、光谱、电荷传输性质以及稳定性影响的理论研究[J].吉林师范大学学报(自然科学版) 2011,32(3):24~27.[7]胡波,刘腊梅,张浩.“CH”/N取代对BTD衍生物光电性质影响的理论研究[J].分子科学学报2012,28:79~83.[8]胡波,齐晓影.基于2,1,3-苯并噻二唑衍生物的有机发光材料的设计与性质的理论研究[J].化学研究与应用 2012,24:168~174.[9]胡波,周凌云,靳瑞发.F原子取代对喹喔啉 (quinoxaline) 衍生物光电性质影响的理论研究[J].吉林师范大学学报(自然科学版) 2012,33(2):12~14.[10]胡波,王悦.环戊二噻吩中的硫原子被CH2、SiH2取代对化合物光电性质影响的理论研究[J].化学通报 2012,75:63~68.[11]Hu B,Yao C,Wang Q W,Zhang H,Yu J K.Electronic,optical and charge transport properties of D-π-A-π-D type naphthalene-based derivatives[J].Acta Phys.-Chim.Sin.,2012,28:1651~1657.[12](a) Roothaan C C J.New developments in molecular orbitaltheory[J].Rev.Mod.Phys.,1951,23:69-89.(b) Pople J A,Nesbet R K.Self-consistent orbitals for radicals[J].J.Chem.Phys.,1954,22:571-578.(c) McWeeny R,Diercksen G.Self-consistent perturbation theory.II.Extension to open shells[J].J.Chem.Phys.,1968,49:4852~4856. [13]Parr R G,Yang W.Density functional theory of atoms and molecules[M].Oxford University.Press:Oxford,1989.[14](a) Ernzerhof M,Scuseria G E.Assessment of the Perdew-Burke-Ernzerhof exchange- correlation functional[J].J.Chem.Phys.,1999,110:5029-5036.(b) Adamo C,Barone V.Toward reliable density functional methods without adjustable parameters:the PBE0model[J].J.Chem.Phys.,1999,110:6158~6170.[15]Foresman J B,Head-Gordon M,Pople J A,et al.Toward a systematic molecular orbital theory for excited states[J].J.Phys.Chem.,1992,96:135~149.[16](a) Stratmann R E,Scuseria G E,Frisch M J.An efficient implementation of time-dependent density- functional theory for the calculation of excitation energies of large molecules[J].J.Chem.Phys.,1998,109:8218-8224.(b) Bauernschmitt R,Ahlrichs R.Treatment of electronic excitations within the adiabatic approximation of time dependent density functional theory[J].Chem.Phys.Lett.,1996,256:454-464.(c) Casida M E,Jamorski C,Casida K C,et al.Molecular excitation energies to high-lying bound states from time-dependent density-functional response theory:Characterization and correction of the time- dependent local density approximation ionizationthreshold[J].J.Chem.Phys.,1998,108:4439~4449.[17](a) Hariharan P C,Pople J A.Accuracy of ah equilibrium geometries by single determinant molecular-orbital theory[J].Mol.Phys.,1974,27:209-214.(b) Gordon M S.The isomers of silacyclopropane[J].Chem.Phys.Lett.,1980,76:163-168.(c) Frisch M J,Pople J A,Binkley J S.Self-consistent molecular orbital methods 25.Supplementary functions for Gaussian basis sets[J].J.Chem.Phys.,1984,80:3265~3269.[18](a) Miertuš S,Scrocco E,Tomasi J.Electrostatic interaction of a solute with a continuum.A direct utilizaion of AB initio molecular potentials for the prevision of solvent effects[J].Chem.Phys.,1981,55:117-129.(b) Miertuš S,Tomasi J.Approximate evaluations of the electrostatic free energy and internal energy changes in solutionprocesses[J].Chem.Phys.,1982,65:239-245.(c) Cossi M,Barone V,Cammi R,et al.Ab initio study of solvated molecules:a new implementation of the polarizable continuummodel[J].Chem.Phys.Lett.,1996,255:327~335.[19]Frisch M J,Trucks G W,Schlegel H B,et al.Gaussian 03,revisionB.03;Gaussian,Inc.,Pittsburgh,PA,2003.。
吸附热力学的英文
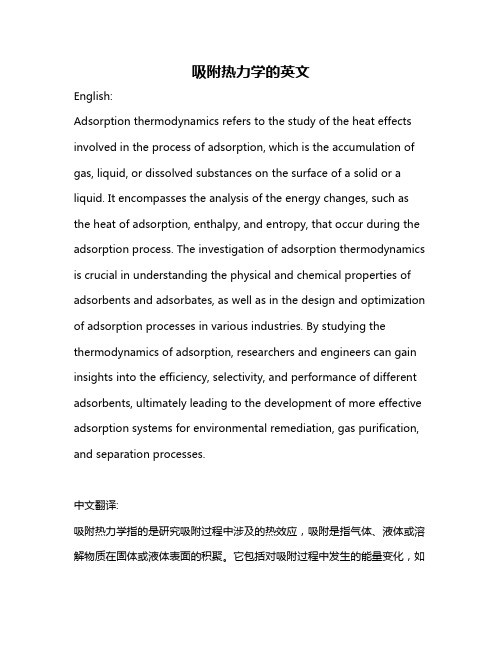
吸附热力学的英文English:Adsorption thermodynamics refers to the study of the heat effects involved in the process of adsorption, which is the accumulation of gas, liquid, or dissolved substances on the surface of a solid or a liquid. It encompasses the analysis of the energy changes, such as the heat of adsorption, enthalpy, and entropy, that occur during the adsorption process. The investigation of adsorption thermodynamics is crucial in understanding the physical and chemical properties of adsorbents and adsorbates, as well as in the design and optimization of adsorption processes in various industries. By studying the thermodynamics of adsorption, researchers and engineers can gain insights into the efficiency, selectivity, and performance of different adsorbents, ultimately leading to the development of more effective adsorption systems for environmental remediation, gas purification, and separation processes.中文翻译:吸附热力学指的是研究吸附过程中涉及的热效应,吸附是指气体、液体或溶解物质在固体或液体表面的积聚。
电化学吸附英文
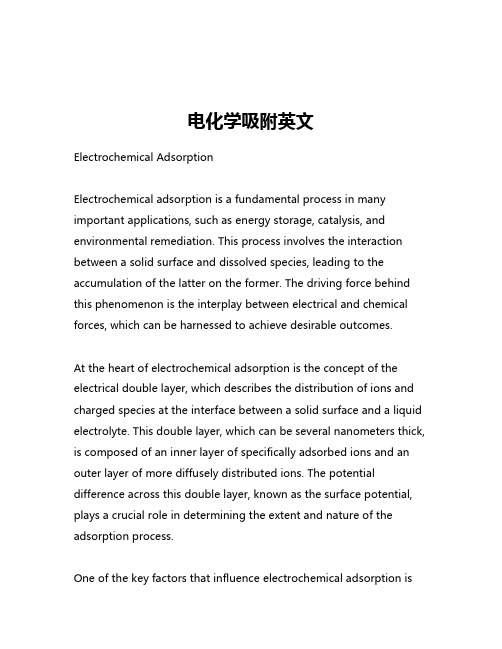
电化学吸附英文Electrochemical AdsorptionElectrochemical adsorption is a fundamental process in many important applications, such as energy storage, catalysis, and environmental remediation. This process involves the interaction between a solid surface and dissolved species, leading to the accumulation of the latter on the former. The driving force behind this phenomenon is the interplay between electrical and chemical forces, which can be harnessed to achieve desirable outcomes.At the heart of electrochemical adsorption is the concept of the electrical double layer, which describes the distribution of ions and charged species at the interface between a solid surface and a liquid electrolyte. This double layer, which can be several nanometers thick, is composed of an inner layer of specifically adsorbed ions and an outer layer of more diffusely distributed ions. The potential difference across this double layer, known as the surface potential, plays a crucial role in determining the extent and nature of the adsorption process.One of the key factors that influence electrochemical adsorption isthe surface charge of the solid material. Depending on the pH of the solution and the point of zero charge (PZC) of the solid, the surface can be positively or negatively charged. This surface charge, in turn, affects the adsorption of ions and molecules from the solution. For example, if the surface is positively charged, it will preferentially adsorb anions from the solution, while a negatively charged surface will attract cations.The strength of the adsorption interaction is also influenced by the chemical nature of the adsorbate and the adsorbent. Specific interactions, such as hydrogen bonding, ion-dipole interactions, and van der Waals forces, can all contribute to the overall adsorption energy. Additionally, the morphology and surface area of the adsorbent material can play a significant role in the adsorption capacity and kinetics.One of the key applications of electrochemical adsorption is in the field of energy storage. In electrochemical capacitors, also known as supercapacitors, the storage of energy is achieved through the reversible adsorption and desorption of ions at the electrode-electrolyte interface. The high surface area of the electrode materials, combined with the rapid kinetics of the adsorption process, allows for the development of high-power energy storage devices with long cycle life.Another important application of electrochemical adsorption is in the area of catalysis. Many catalytic processes, such as fuel cell reactions and electrochemical water splitting, involve the adsorption of reactants and intermediates on the catalyst surface. The controlled adsorption of these species can enhance the catalytic activity and selectivity, leading to improved efficiency and performance.Environmental remediation is yet another field where electrochemical adsorption plays a crucial role. The removal of heavy metals, organic pollutants, and other contaminants from water and wastewater can be achieved through the adsorption of these species onto electrode materials. The ability to tune the surface properties of the adsorbent, as well as the application of an external electric field, can enhance the selectivity and efficiency of the adsorption process.In addition to these well-established applications, electrochemical adsorption is also being explored in emerging fields, such as electrochemical sensors, energy harvesting, and biomedical applications. The versatility and tunability of this process make it a valuable tool in the development of innovative technologies.To further advance the understanding and application of electrochemical adsorption, ongoing research is focused on several key areas. These include the development of novel adsorbent materials with tailored surface properties, the investigation of thefundamental mechanisms governing the adsorption process, and the optimization of the operating conditions and system design for various applications.In conclusion, electrochemical adsorption is a complex and multifaceted phenomenon that underpins a wide range of important technologies. By harnessing the interplay between electrical and chemical forces, researchers and engineers can harness the power of this process to address pressing challenges in energy, environment, and beyond. As our understanding of electrochemical adsorption continues to deepen, we can expect to see even more innovative applications emerge in the years to come.。
视网膜静脉阻塞的药物治疗进展

综述视网膜静脉阻塞的药物治疗进展安〒u,杨相颖I2,张福燕\秦波*"*(1.贵州医科大学临床医学院,贵州贵阳550004; 2.暨南大学附属深圳爱尔眼科医院眼底病眼外伤科,广东深圳518032)摘要:视网膜静脉阻塞(retinal vein occlusion, RVO)是临床上严重危及视功能的视网膜血管疾病,病情易迁延反复,治疗 药物有限。
本文总结了抗血管内皮生长因子(vascular endothelial growth factor, VEGF)类经典药物和最新的临床研究,及其 在RVO黄斑水肿中的应用,归纳了糖皮质激素类药物对RVO的治疗及其不良反应;展望了正处于临床前研究及临床研究的 新兴药物,包括干细胞疗法、酪氨酸激酶受体抑制剂、醛固酮受体拮抗剂、萘衍生物、烟酸等,以期为RVO提供新的治疗 思路,并为其临床研究提供指导和依据。
关键词:视网膜静脉阻塞•,抗VEGF药物;糖皮质激素;药物治疗中图分类号:R774 文献标志码:A文章编号:1672-9188(2021)04-0314-07DOI:10.13683/j.wph.2021.04.015Progress in medication treatment of retinal vein occlusionAN Ning1'2, YANG Xiangying1,2, ZHANG Fuyan1, QIN Bo2*(7. Department o f c linical medicine, Guizhou Medical University, Guiyang 550004, Guizhou Province, China; 2. Department of o cular f undus andtrauma disease, Shenzhen Aier Eye Hospital Affiliated to Jinan University, Shenzhen 518032, Guangdong Province, China) Abstract:Retinal vein occlusion (RVO), a common retinal vascular disorder, is characterized by severe vision reduction and repeated attack. This article reviewed the classic and recent clinical studies on anti-vascular endothelial growth factor (VEGF) agents, and its application in RVO macular edema, and summarized the therapeutic effects and adverse reactions of glucocorticoids on RVO. The emerging drugs in pre-clinical and clinical research is also prospected, including stem cell therapy, tyrosine kinase receptor inhibitors, aldosterone receptor antagonists, naphthalene derivatives, niacin and so on. It is hoped that this paper can serve as a reference for clinical research to provide new ideas for RVO treatment.Key words: retinal vein occlusiony; anti-VEGF drug; glucocorticoid; drug therap视网膜静脉阻塞(retinal vein occlusion,RVO)按阻塞部位可分为视网膜中央静脉阻塞(central retinal vein occlusion,CRVO)、视网膜分支静脉阻塞(branch retinal vein occlusion,BRVO)和半侧分支 静脉阻塞(hemiretina vein occlusion,HRVO)。
- 1、下载文档前请自行甄别文档内容的完整性,平台不提供额外的编辑、内容补充、找答案等附加服务。
- 2、"仅部分预览"的文档,不可在线预览部分如存在完整性等问题,可反馈申请退款(可完整预览的文档不适用该条件!)。
- 3、如文档侵犯您的权益,请联系客服反馈,我们会尽快为您处理(人工客服工作时间:9:00-18:30)。
2271
0
/
0
I
100
I
200
I
300
I
4cc
I
500
I 600
I 700
/ 8W
I 900
Figure 1 Equilibrium adsorption isotherms at 30 C for 1-naphtbol on XAD-series polymeric adsorbents:( x )XAD-2; (*)XAD-4; (A )XAD-7; (O)XAD-8
The initial concentration C, and residual concentration (CJ were measured using a 7520 model UV spectrophotometer (Shanghai Analytical Instruments Factory) at the maximum wavelength (L&l of the adsorbence. These wavelength were determined for each compounds according to reference [6].
(Receivai in Gemmy 27 April 1997; amepted 30 May 1997)
ABSTRACT
The adsorption of I-naphthol, 2- naphthol, I-naphthylamine and 2- naphthylamine fi-om aqueous solutions on macroporous polymeric adsorbents was studied. The equilibrium data were fitted to Freundlich-type isotherms and the equilibrium constauts K, and n were obtained. The values of the adsorption capacity depend on the surface area of adsorbents, the chemical properties of naphthalene derivatives, as well as the polarity of adsorbents. 91997 Elsevier Science Ltd KEY WORDS: macroporous polymeric adsorbents, naphthalene derivatives, adsorption
derivatives, one group of PAHs, often occur as a result of effluents fi-om dye in~ediate However, the effectiveness of biological processes against naphthalene
EXPERIMENTAL Materiels
The polymeric adsorbents used in this study were Amberlite XAD-2, XAD-4, XAD-7 and XAD-8 obtained from Rohm-haas Company. Adsorbents were reflux extracted with absolute alcohol for two hours and washed with 4% aqueous HCl and NaOH solution alternatively twice, then washed with distilled water and dried at 100 C until the constant weight was reached. The naphthalene derivatives studied in the adsorption experiment were 1-naphthol, 2-naphthol, naphthylamine and 2-naphthylamine. All the compounds were of A.R. grade. l-
Methods
The adsorption of naphthalene derivatives was performed as follows: 0.25g polymeric adsorbent was introduced in a flask bottle. Then, 200 cm3 of aqueous solution of naphthalene derivatives with known concentration was added to each flask. The flasks were then shaked (1OOrpm) in a G25 model incubator shaker (New Brunswick Scientific Co. Inc.) at 30C for 48 hours. The initial concentrations(C,,) of solutions were 200,400,600,800 and 1OOOmg/L respectively.
2270 and 2- naphthylamine from aqueous solution with high concentration. Research was focused on the effect of surface area and polarity of the adsorbents and the characteristics of the adsorbates on the adsorptive properties, as well as the adsorption isotherms were determined.
on adsorption of naphthalene derivatives from aqueous solution with high concentration, as the actual situation in induskal ef%ents tnzitment may be, with these polymeric adsorbents have been reported. In this paper, four commonIy used macroporous adsorbents Amberlite XAD-2, XAD4, XAD-7 and XAD8 were used to adsorb a series of naphthalene derivatives such as I -naphthol, 2-naphthol, I-naphthylamine 2269
RESULTS AND DISCUSSION Adsorption Isotherms
Equilibrium adsorption isotherms at 3O’ for the four compounds on the four XAD polymeric adsorbents C are shown in Figure l-4 respectively. It is well known that the Freundlich equation has the general form[7] q,=KF. C c l’ n (1)
INTRODUCTION Many of the poIynuckar enviromnental aromatic hydmcarbons(PAH), probably the most widespread of all chemical Naphthalene and its
contankan ts, are known to be carcinogenic or cocarcinogenic.
production.
derivatives removal is not
satisfactory because they can not be degraded through biological treatment[ I]. Adsorption is considered a prominent method for treatment of effluents containing organic substances. Compared with classical adsorbents such as the silica gels, aluminas and the activated carbons, the macroporous polymers is a more attractive alternative because of the wide range of pore structures and surface chamcteristics[2]. The use of these poIymeric adsorbents for the treatment of efkluents containing benzoid compounds has been widely studied[3]. There are also example-s of adsorbing trace naphthalene compounds with polymeric adsorbents for drinking water preparation[4,5]. However, few systematic studies
where KP and l/n are characteristic constants. Equation (1) linearizes in logarithmic form log q, = log KF+l/n log C, (2)