LIGHT WEIGHT MULTIFUNCTIONAL COMPOSITES
汽车轻量化的具体内容
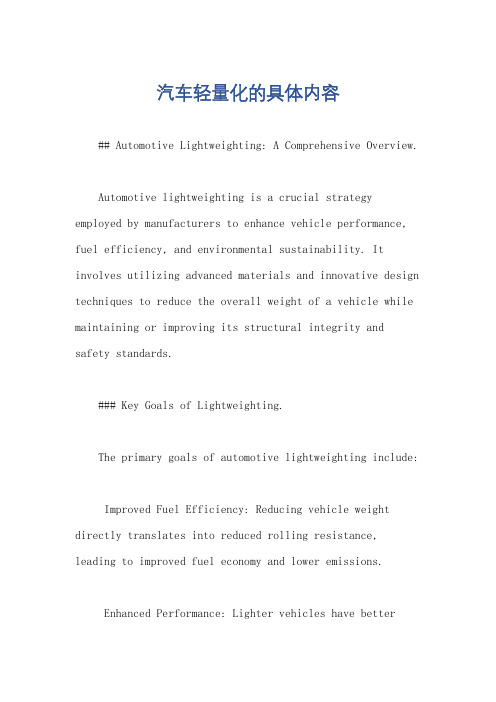
汽车轻量化的具体内容## Automotive Lightweighting: A Comprehensive Overview.Automotive lightweighting is a crucial strategy employed by manufacturers to enhance vehicle performance, fuel efficiency, and environmental sustainability. It involves utilizing advanced materials and innovative design techniques to reduce the overall weight of a vehicle while maintaining or improving its structural integrity and safety standards.### Key Goals of Lightweighting.The primary goals of automotive lightweighting include:Improved Fuel Efficiency: Reducing vehicle weight directly translates into reduced rolling resistance, leading to improved fuel economy and lower emissions.Enhanced Performance: Lighter vehicles have betteracceleration, braking, and handling capabilities, resulting in a more dynamic and engaging driving experience.Reduced Emissions: By reducing fuel consumption, lightweighting contributes to lower greenhouse gas emissions, supporting environmental sustainability.Increased Safety: Despite weight reduction, advanced lightweight materials often provide enhanced strength and crash protection, ensuring occupant safety.### Materials for Lightweighting.A variety of innovative materials are employed in automotive lightweighting, including:Advanced High-Strength Steel (AHSS): This high-strength steel offers exceptional strength-to-weight ratio, making it an ideal choice for structural components and crash zones.Aluminum Alloys: Aluminum is significantly lighterthan steel, and its alloys provide a good balance of strength, weight, corrosion resistance, and ease of formability.Composites: These materials combine lightweight fibers, such as carbon fiber or glass fiber, with a matrix material to create strong and durable structures with low weight.Magnesium Alloys: Magnesium is the lightest structural metal, offering excellent strength-to-weight ratio and vibration dampening properties.### Design Techniques for Lightweighting.In addition to material selection, lightweighting also involves optimizing vehicle design through techniques such as:Topology Optimization: This advanced computational technique analyzes load distribution within a structure and identifies areas where material can be removed without compromising strength.Multi-Material Design: Engineers combine different materials in a single component to optimize properties and reduce weight. For example, a steel frame can be reinforced with aluminum panels.Innovative Joining Techniques: Advanced adhesives, welding technologies, and composite bonding methods enable manufacturers to reduce the weight of joints and connections.Multifunctional Structures: Components are designed to serve multiple functions, such as a seat that also provides structural support, further reducing weight.### Benefits of Lightweighting.The benefits of automotive lightweighting are numerous and include:Reduced Fuel Consumption: Lightweight vehicles consume less fuel, resulting in lower operating costs andenvironmental benefits.Enhanced Vehicle Performance: Lighter vehicles have improved acceleration, braking, and handling, making them more fun and responsive to drive.Reduced Emissions: By consuming less fuel, lightweight vehicles emit fewer greenhouse gases, supporting environmental sustainability.Increased Safety: Advanced lightweight materials often provide enhanced strength and crash protection, ensuring occupant safety.Expanded Electric Vehicle Range: Lightweighting is particularly crucial for electric vehicles, as it extends their range on a single charge.### Challenges of Lightweighting.Despite its benefits, automotive lightweighting also faces challenges, including:Cost: Advanced lightweight materials and innovative design techniques can be more expensive than traditional materials and designs.Structural Integrity: It is essential to ensure that lightweight structures can withstand the same or higher loads as heavier components.Manufacturing Complexity: Lightweight materials often require specialized manufacturing processes and tooling, which can increase production costs.Material Durability: Some lightweight materials may be more susceptible to corrosion or damage than traditional materials.### Conclusion.Automotive lightweighting is an essential strategy to improve vehicle performance, fuel efficiency, environmental sustainability, and safety. By utilizing advanced materialsand innovative design techniques, manufacturers can significantly reduce vehicle weight while maintaining or enhancing structural integrity. While challenges remain, the benefits of lightweighting make it a key area of focus for the automotive industry as it strives towards a more sustainable and efficient future.## 汽车轻量化,全面概述。
Advanced Materials and Processes
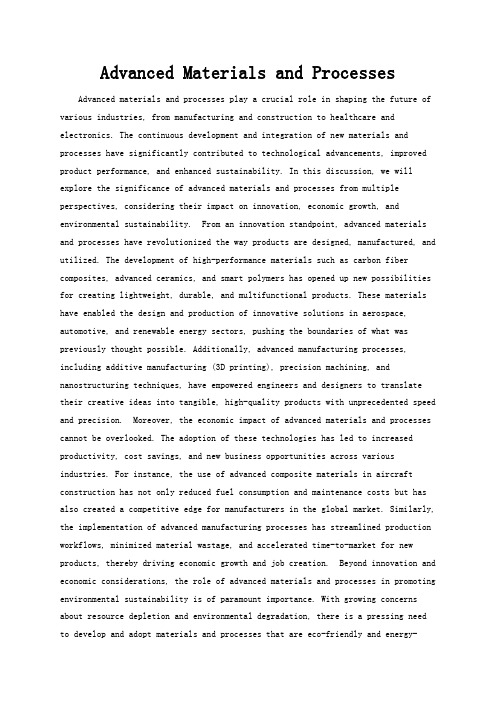
Advanced Materials and Processes Advanced materials and processes play a crucial role in shaping the future of various industries, from manufacturing and construction to healthcare and electronics. The continuous development and integration of new materials and processes have significantly contributed to technological advancements, improved product performance, and enhanced sustainability. In this discussion, we will explore the significance of advanced materials and processes from multiple perspectives, considering their impact on innovation, economic growth, and environmental sustainability. From an innovation standpoint, advanced materials and processes have revolutionized the way products are designed, manufactured, and utilized. The development of high-performance materials such as carbon fiber composites, advanced ceramics, and smart polymers has opened up new possibilities for creating lightweight, durable, and multifunctional products. These materials have enabled the design and production of innovative solutions in aerospace, automotive, and renewable energy sectors, pushing the boundaries of what was previously thought possible. Additionally, advanced manufacturing processes, including additive manufacturing (3D printing), precision machining, and nanostructuring techniques, have empowered engineers and designers to translate their creative ideas into tangible, high-quality products with unprecedented speed and precision. Moreover, the economic impact of advanced materials and processes cannot be overlooked. The adoption of these technologies has led to increased productivity, cost savings, and new business opportunities across various industries. For instance, the use of advanced composite materials in aircraft construction has not only reduced fuel consumption and maintenance costs but has also created a competitive edge for manufacturers in the global market. Similarly, the implementation of advanced manufacturing processes has streamlined production workflows, minimized material wastage, and accelerated time-to-market for new products, thereby driving economic growth and job creation. Beyond innovation and economic considerations, the role of advanced materials and processes in promoting environmental sustainability is of paramount importance. With growing concerns about resource depletion and environmental degradation, there is a pressing need to develop and adopt materials and processes that are eco-friendly and energy-efficient. In response to this challenge, researchers and industry experts are focusing on the development of sustainable materials, such as biodegradable polymers, recycled composites, and eco-friendly coatings, which minimize environmental impact throughout their lifecycle. Furthermore, advanced manufacturing processes are being optimized to reduce energy consumption, emissions, and waste generation, aligning with the principles of sustainable production and consumption. In conclusion, the significance of advanced materials and processes cannot be overstated, as they continue to drive innovation, economic prosperity, and environmental sustainability. By leveraging the capabilities of these technologies, industries can create value-added products, optimize production processes, and minimize their environmental footprint. As we look to the future, it is essential to prioritize research and investment in advanced materials and processes, recognizing their pivotal role in shaping a more resilient, efficient, and sustainable global economy.。
酱油曲中谷氨酰胺酶酶活测定条件优化
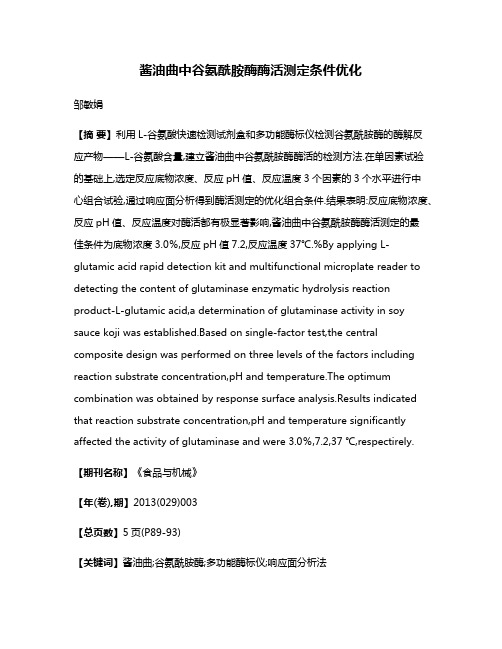
酱油曲中谷氨酰胺酶酶活测定条件优化邹敏娟【摘要】利用L-谷氨酸快速检测试剂盒和多功能酶标仪检测谷氨酰胺酶的酶解反应产物——L-谷氨酸含量,建立酱油曲中谷氨酰胺酶酶活的检测方法.在单因素试验的基础上,选定反应底物浓度、反应pH值、反应温度3个因素的3个水平进行中心组合试验,通过响应面分析得到酶活测定的优化组合条件.结果表明:反应底物浓度、反应pH值、反应温度对酶活都有极显著影响,酱油曲中谷氨酰胺酶酶活测定的最佳条件为底物浓度3.0%,反应pH值7.2,反应温度37℃.%By applying L-glutamic acid rapid detection kit and multifunctional microplate reader to detecting the content of glutaminase enzymatic hydrolysis reaction product-L-glutamic acid,a determination of glutaminase activity in soy sauce koji was established.Based on single-factor test,the central composite design was performed on three levels of the factors including reaction substrate concentration,pH and temperature.The optimum combination was obtained by response surface analysis.Results indicated that reaction substrate concentration,pH and temperature significantly affected the activity of glutaminase and were 3.0%,7.2,37 ℃,respectirely.【期刊名称】《食品与机械》【年(卷),期】2013(029)003【总页数】5页(P89-93)【关键词】酱油曲;谷氨酰胺酶;多功能酶标仪;响应面分析法【作者】邹敏娟【作者单位】佛山市海天调味食品股份有限公司,广东佛山 528000【正文语种】中文酿造酱油的主要原料大豆蛋白经微生物酶解之后形成各种氨基酸,是酱油呈鲜味的主要原因,而各种氨基酸中,谷氨酸对鲜味的贡献最为显著,故其含量更为重要[1,2]。
《食品添加剂》中的专业术语

《食品添加剂》中的专业术语一、食品增稠剂1.食品增稠剂:Food thickeners2.天然增稠剂:Natural thickeners3.合成增稠剂:Synthetic thickeners4.动物来源的增稠剂:Animal sources of thickeners5.植物来源的增稠剂:Plant sources of thickeners6.微生物来源的增稠剂:Microbe sources of thickeners 7.(非)离子性的增稠剂:(non-)Ion thickeners8.多肽类增稠剂:Peptide thickeners9.多糖类增稠剂:Polysaccharides thickeners10.(非)凝胶性增稠:(non-)Gel thickeners11.增稠:thickening 12.分散:dispersion13.稳定:stable 14.胶凝:gel15.澄清:clarification 16.成膜:film17.保鲜:fresh-keeping 18.持水:water retention19.起泡性:foaming 20.起霜:frosting21.结晶:crystal 22.黏性:adhesive23.凝胶性:gelatin properties24.协同性:coordination25.触变性:thixotropy 26.剪切力:shear force27.明胶:Gelatin 28酪蛋白酸钠:Sodium caseinate 29.壳聚糖:Chitosan 30.甲壳素:Chitin31.阿拉伯胶:Arabic gum 32.罗望子多糖胶:Tamarind gum 33.卡拉胶:Carrageenan 34.果胶:Pectin35.琼脂:Agar 36.海藻酸钠:Sodium alginate 37.变性淀粉:Modified starches 38.黄原胶:Corn sugar gum 39.结冷胶:Gellan gum 40.β-环状糊精: β-Cyclodextrine 41.凝胶多糖:Curdlan42.羧甲基纤维素钠:CMC-Na43.海藻酸丙二醇酯:Propylene glycol alginate44.羧甲基淀粉钠:CMS-Na45.羧甲基淀粉:Hydroxypropyl starch46.淀粉磷酸酯钠:Sodium starch phosphate二、食品防腐剂1.食品防腐剂:Food preservatives2.食品腐败:Food spoilage3.食品霉变:Food mildew4.食品发酵:Food fermentation5.食品保藏:Food preservation6.杀菌剂:Microbicides7.保存剂:Save agent8.有机化学防腐剂:Organic chemical preservatives9.无机化学防腐剂:Inorganic chemical preservatives10.酸型防腐剂:Acid preservatives11.酯型防腐剂:Ester preservatives12.无机防腐剂:Inorganic preservatives13.生物防腐剂:Biological preservatives14.合成防腐剂:Synthetic preservatives15.天然防腐剂:Natural preservatives16.微生物源天然食品防腐剂:Microbe sources of preservatives17.动物源天然食品防腐剂:Animal sources of preservatives18.植物源天然食品防腐剂:Plant sources of preservatives19.矿物提取物:Mineral extracts20.天然有机化合物:The natural organic compounds21.分配系数:Partition coefficient22.水分活度:Water activity23.苯甲酸:Benzoic acid 24.苯甲酸钠:Sodium benzoate 25.山梨酸:Sorbic acid 26.山梨酸钾:sorbic acid potassium salt 27.山梨酸钠:sodium sorbate 28.山梨酸钙:calcium sorbate29.对羟基苯甲酸酯类:Easters of P-hydroxybenzoic acid30.丙酸:Propionic acid 31.丙酸钠:Sodium propionate 32.丙酸钙:Calcium propionate 33.脱氢醋酸:Dehydroacetic acid 34.乳酸链球菌素:Nisin 35.聚赖氨酸Poly-lysine(PLL) 36.鱼精蛋白:Protemine 37.溶菌酶:Lysozme38.曲酸:Kojic acid 39.纳他霉素:Natamycin40.壳聚糖:Chitosan 41.蜂胶:Propolis42.贝壳提取物:Shells extract 43.防御素:Phylaxin44.昆虫抗菌肽:Insect antimicrobial peptides45.蚯蚓提取液:Earthworms extract46.芥菜提取物:Mustard extract47.胡椒提取物:Pepper extracts48.咖啡豆渣提取物:Coffee bean dregs extract49.过氧化氯:Chlorine dioxide 50.漂白粉:Bleaching powder 51.次氯酸:Hypochloric acid 52.次氯酸钠:Sodium Hypochlorite 53.塞苯咪唑:Thiabendazole 54.双乙酸钠:Sodium diaeetate55.过氧化氢:Hydrogen peroxide三、抗氧化剂1.抗氧化剂:Antioxidants2.油溶性抗氧化剂:Oil soluble antioxidants3.水溶性抗氧化剂:Water soluble antioxidants4.兼容性抗氧化剂:Also soluble antioxidants5.天然抗氧化剂:Natural antioxidants6.人工合成抗氧化剂:Synthetic antioxidants7.自由基吸收剂:Free radicals sorbents8.金属离子螯合剂:Metal ion chelating agent9.氧清除剂:Oxygen scavenger10.单线态氧淬灭剂:Singlet oxygen quencher11.甲基硅铜和甾醇抗氧化剂:Methyl silicone and sterol antioxidants12.过氧化物分解剂:Peroxide decomposition agent13.紫外线吸收剂:Ultraviolet ray absorber14.多功能抗氧化剂:Multifunctional antioxidants15.酶抗氧化剂:Enzymatic antioxidants16.香辛料:Spice17.中草药:Chinese herbal medicine18.增效剂:Synergistic agent19.油脂酸败:Rancidity oil20.醛式酸败:Aldehyde type rancidity21.酮式酸败:Ketone type rancidity22.丁基羟基茴香醚:Butyl hydroxy anisol(BHA)23.二丁基羟基甲苯: Bibutyl hydroxy toluene(BHT)24.没食子酸丙酯:Propyl Gallate(PG)25.特丁基对苯二酚:Tert-Butylhydrogquinone(TBHQ)26.维生素E:V e 27.愈创树脂:Guaiacum resin28.L-抗坏血酸:L-Ascorbic acid29.L-抗坏血酸钠:Sodium ascorbate 30.植酸:Phytic acid31.栎精:Quercetin 32.芦丁:Rutin33.抗坏血酸棕榈酸酯:Ascorbyl palmitate34.茶多酚:Tea polyphenols35.甘草抗氧化物:Antioxidants of glycyrrhiza36.植物单宁: Plant tannins 37.黄铜: Brass38.蜂胶: Propolis 39.姜黄素及其衍生物: Curcuminand its derivatives40.迷迭香提取物: Rosemary extract41.银杏叶提取物: Gingko leaf extrac 42.枸杞多糖: LBP43.木耳多糖: Agaric polysaccharide四、水分保持剂、面粉处理剂、被膜剂、胶姆糖基础剂1.水分保持剂:Water retention agent2.面粉处理剂:Flour treatment agent3.面粉漂白剂:Flour bleaching agent4.面粉增筋剂:Flour gluten agent5.面粉还原剂:Flour reducer6.面粉填充剂:Flour filler7.被膜剂:Coating agent8.胶姆糖:Chewing gum9.胶姆糖基础剂:Chewing gum base agent10.正磷酸盐: Is phosphate 11.焦磷酸盐: Focal phosphate12.聚磷酸盐: Gather phosphate 13.偏磷酸盐: Partial phosphate14.六偏磷酸钠:Six partial sodium15.过氧化苯甲酰:Benzoyl peroxide16.溴酸钾:Potassium bromate 17.偶氮甲酰胺:Azodicarbonamide18.紫胶:Shellac 19.吗啉脂肪酸盐:Morpholine fatty acid salt20.石蜡:Paraffin 21.白色油:Liquid paraffin22.海藻酸钠:Ammonlum algmate23.丁苯橡胶:Butadiene-styrene rubber24.丁基橡胶:Butyl rubber 25.糖胶树胶:Chicle26.聚乙酸乙烯酯:Polyvinyl acetate 27.萜烯树脂:Terpene resin五、膨松剂、凝固剂、抗结剂、消泡剂1.膨松剂:Leavening agent2.碱性膨松剂:Alkaline leavening agent3.酸性膨松剂:Acid leavening agent4.复合膨松剂:Composite leavening agent5.生物膨松剂:Biological leavening agent6.稳定和凝固剂:Stability and coagulate agent7.凝固剂:Coagulate agent8.果蔬硬化剂:Fruit and vegetable stiffening agent9.螯合剂:Chelating agent10.罐头除氧剂:Canned oxygen agent11.保湿剂:Moisturizing12.澄清剂:Clarify agent13.抗结剂:Anticaking agent14.消泡剂:Defoaming agent15.破泡剂:Foam breaken16.抑泡剂:Antifoaming agent17.碳酸氢钠:Sodium bicarbonate18.碳酸氢铵:Ammonium bicarbonate19.发酵粉:Baking powder 20.钾明矾:Potassium alum 21.氯化钙:Calcium chloride 22.硫酸钙:Calcium sulfate23.氯化镁:Magnesium chloride24.葡萄糖酸-δ-内酯:Glucono-δ-lactone25.亚铁氰化钾:Potassium ferrocyanide26.硅铝酸钠:Sodium aluminosilicate27.二氧化硅:Silicon dioxide28.微晶纤维素:Microcrystalline cellulose29.乳化硅油:Emulsifying silicon oil30.山梨糖醇:Sorbitol六、酶制剂1.食品酶制剂:Food enzyme preparations2.动物酶制剂:Animal sources of enzyme preparations3.植物酶制剂:Plant sources of enzyme preparations4.微生物酶制剂:Microbe sources of enzyme preparations5.糖类分解酶:Carbohydrate decomposing enzyme6.蛋白分解酶:Protein decomposing enzyme7.脂肪分解酶:Fat decomposing enzyme8.氧化还原酶:oxidoreductase9.粉状酶制剂:Powder decomposing enzyme preparations10.颗粒状酶制剂:Granular decomposing enzyme preparations11.液体状酶制剂:Liquid decomposing enzyme preparations12.精制酶制剂:Refined decomposing enzyme preparations13.结晶酶:Crystallization enzyme14.人工改性酶:Artificial modification enzyme15.固定化酶:Immobilized enzyme16.凝乳酶:Rennin 17.胃蛋白酶:Pepsin18.木瓜蛋白酶:Papain 19.菠萝蛋白酶:Bromelain20.液化型淀粉酶:α-amylase21.葡萄糖淀粉酶:Amyloglucosidase22.果胶酶:Pectinase 23.纤维素酶:Cellulase24.脂肪酶;Lipase七、营养强化剂1.食品营养强化剂:Food nutrition fortifiers2.维生素类营养强化剂:Vitamins, nutrition fortifiers3.氨基酸及其含氮化合物:Amino acid and its nitrogen-containing compounds4.氨基酸强化剂:Amino acid fortifiers5.矿物质及微量元素:Minerals and trace elements6.钙化剂:Calcification agent7.铁强化剂:Iron fortifiers8.锌强化剂:Zinc fortifiers9.硒强化剂:Selenium fortifiers10.镁强化剂:Magnesiun fortifiers11.铜强化剂:Copper fortifiers12.锰强化剂:Manganese fortifiers13.钾强化剂:Potassium fortifiers14.氟强化剂:Fluorine fortifiers15.不饱和脂肪酸类强化剂:The unsaturated acid kind fortifiers16.硝酸硫胺素:Thiamine mononitrate 17.烟酸:Nicotinic acid 18.烟酸胺:Nicotinamide 19.L-左旋肉碱:L-Carnitine20.L-赖氨酸盐:L-Lysine monohydrochloride21.L-赖氨酸-L-天门冬氨酸盐:L-Lysine-L-Aspartate21.牛磺酸:Taurine 22.碳酸钙:Calcium carbonate 23.葡萄糖酸钙:Calcium gluconate 24.乳酸钙:Calcium lactate 25.氯化铁:Ferric chloride 26.柠檬酸铁:Ferric citrate 27.硫酸锌:Zinc sulfate 28.乙酸锌:Zinc acetate 29.亚硒酸钠:Sodium selenite 30.硒蛋白:Selenoprotein。
山东美能达健身器材股份有限公司产品手册说明书
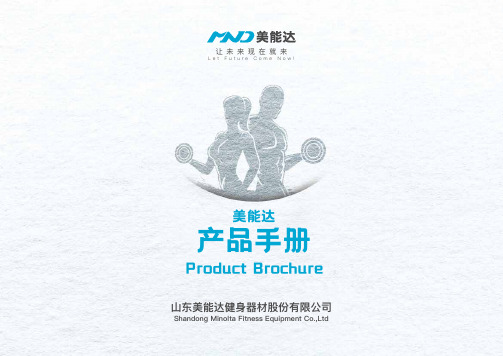
山东美能达健身器材股份有限公司让未来现在就来Shandong Minolta Fitness Equipment Co.,LtdL e t F u t u r e C o m e N o w !美能达Product Brochure产品手册资质证书Certification1目录Catalogue关于我们About us Cardio Strength有氧产品力量产品公司简介Company Profile Cardio SeriesFS SeriesFM SeriesF SeriesPL SeriesC SeriesH SeriesG SeriesHA Series360 SeriesCardio Series Product有氧系列FS系列FM系列更多有氧产品F系列PL系列C系列H系列G系列HA系列360系列003005039035033FB SeriesFF SeriesFH SeriesAN SeriesFB系列FF系列FH系列AN系列049053043061071084111091094097107010203Accessor Series配件系列1182公司简介CorporateProfile15万平方米150000 square meters factory site11 seriesmore than 500+ models11大系列500+规格山东美能达健身器材股份有限公司是一家专业从事研发、 设计、 生产、 销售、 服务为一体的综合健身器材生产厂家, 成立于2010年, 坐落于山东宁津银河开发区, 拥有自主兴建的15万平方米的厂房, 包括大型车间, 全方位一流的展示厅和质量检测实验室等。
我公司拥有一批优秀的设计师 工程师, 外贸和专业管理人才, 通过不断的研发和引进国外先进技术, 完善和改进工艺流程, 严抓严品质量, 我们公司的产品具有设计合理、 样式新颖、 美观耐用、 不退色、 抗老化等特点。
多功能聚吡咯
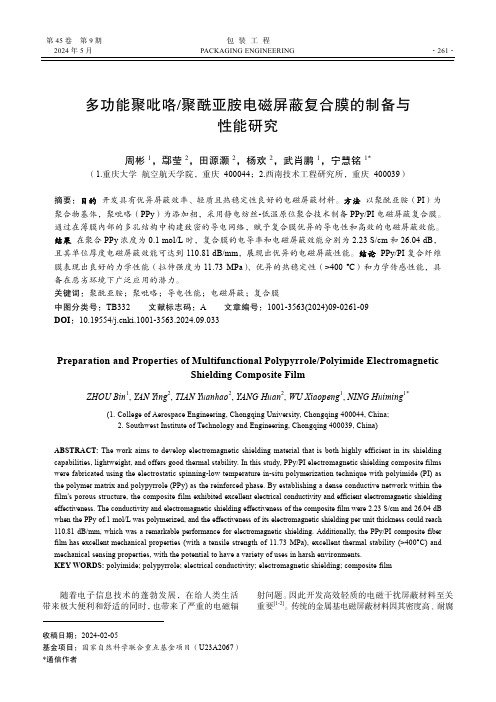
第45卷 第9期 包 装 工 程2024年5月PACKAGING ENGINEERING ·261·收稿日期:2024-02-05基金项目:国家自然科学联合重点基金项目(U23A2067) *通信作者多功能聚吡咯/聚酰亚胺电磁屏蔽复合膜的制备与性能研究周彬1,鄢莹2,田源灏2,杨欢2,武肖鹏1,宁慧铭1*(1.重庆大学 航空航天学院,重庆 400044;2.西南技术工程研究所,重庆 400039)摘要:目的 开发具有优异屏蔽效率、轻质且热稳定性良好的电磁屏蔽材料。
方法 以聚酰亚胺(PI )为聚合物基体,聚吡咯(PPy )为添加相,采用静电纺丝-低温原位聚合技术制备PPy/PI 电磁屏蔽复合膜。
通过在薄膜内部的多孔结构中构建致密的导电网络,赋予复合膜优异的导电性和高效的电磁屏蔽效能。
结果 在聚合PPy 浓度为0.1 mol/L 时,复合膜的电导率和电磁屏蔽效能分别为2.23 S/cm 和26.04 dB ,且其单位厚度电磁屏蔽效能可达到110.81 dB/mm ,展现出优异的电磁屏蔽性能。
结论 PPy/PI 复合纤维膜表现出良好的力学性能(拉伸强度为11.73 MPa )、优异的热稳定性(>400 ℃)和力学传感性能,具备在恶劣环境下广泛应用的潜力。
关键词:聚酰亚胺;聚吡咯;导电性能;电磁屏蔽;复合膜中图分类号:TB332 文献标志码:A 文章编号:1001-3563(2024)09-0261-09 DOI :10.19554/ki.1001-3563.2024.09.033Preparation and Properties of Multifunctional Polypyrrole/Polyimide ElectromagneticShielding Composite FilmZHOU Bin 1, YAN Ying 2, TIAN Yuanhao 2, YANG Huan 2, WU Xiaopeng 1, NING Huiming 1*(1. College of Aerospace Engineering, Chongqing University, Chongqing 400044, China; 2. Southwest Institute of Technology and Engineering, Chongqing 400039, China)ABSTRACT: The work aims to develop electromagnetic shielding material that is both highly efficient in its shielding capabilities, lightweight, and offers good thermal stability. In this study, PPy/PI electromagnetic shielding composite films were fabricated using the electrostatic spinning-low temperature in-situ polymerization technique with polyimide (PI) as the polymer matrix and polypyrrole (PPy) as the reinforced phase. By establishing a dense conductive network within the film's porous structure, the composite film exhibited excellent electrical conductivity and efficient electromagnetic shielding effectiveness. The conductivity and electromagnetic shielding effectiveness of the composite film were 2.23 S/cm and 26.04 dB when the PPy of.1 mol/L was polymerized, and the effectiveness of its electromagnetic shielding per unit thickness could reach 110.81 dB/mm, which was a remarkable performance for electromagnetic shielding. Additionally, the PPy/PI composite fiber film has excellent mechanical properties (with a tensile strength of 11.73 MPa), excellent thermal stability (>400°C) and mechanical sensing properties, with the potential to have a variety of uses in harsh environments.KEY WORDS: polyimide; polypyrrole; electrical conductivity; electromagnetic shielding; composite film随着电子信息技术的蓬勃发展,在给人类生活带来极大便利和舒适的同时,也带来了严重的电磁辐射问题。
玻璃纤维增强混凝土说明书

玻璃纖維增強混凝土Glass-fiber Reinforced Concrete (GRC)1. 材料簡介Introduction玻璃纖維增強混凝土(Glass fiber Reinforced Concrete)又名GRC,是以耐鹼玻璃纖維作增強材,硫鋁酸鹽低鹼度水泥為膠結材並摻入適宜集料構成基材,通過噴射、立模澆注、擠出、流漿等生產工藝而製成的輕質、高強高韌、多功能的新型無機複合材料。
Glass fiber Reinforced Concrete, also known as GRC, is made of alkali-resistant glass fiber as a reinforcing material. Sulphate-aluminate low-alkalinity cement is used as a cement material and is mixed with suitable aggregate to form a substrate. Lightweight, high-strength, high-tough, multi-functional new inorganic composite materials made by casting, extrusion, and slurry production.2. 材料性能及特徵PerformanceGRC採用特種低堿水泥與特種玻璃纖維複合材料經過多種工序精製而成,其具有高強度、抗老化、品質輕、成型多樣化、施工簡單、耐火、耐候化、耐酸堿等優點。
與混凝土同等性能及壽命,使其成為歐陸式建築及景觀等工程的新寵。
1.無限可塑性: GRC產品是將原料按一定配比攪拌,在模具內噴射成型,可生產出造型豐富,質感多樣的產品。
可根據客戶和設計師的不同需要,進行任意的藝術造型,完美實現設計師的設計夢想。
2.輕、強度高: GRC的體積密度約為1800-1900kg/m3,8mm厚標準GRC板重量僅為15kg,抗壓強度超過40Mpa,抗彎強度超過34Mpa,大大超過國際標準要求。
二氧化钛表面超强酸化光氧复合降解罗丹明B

第40卷第2期2021年3月Vol.40No.2Mar.2021大连工业大学学报JournalofDalianPolytechnicUniversityDOI:10.19670/ki.dlgydxxb.2021.0210二氧化钛表面超强酸化光氧复合降解罗丹明B温宇,杨大伟(大连工业大学轻工与化学工程学院,辽宁大连116034)摘要:采用共结晶方法制备了锌锆共掺杂的介孔二氧化钛,前驱体用硫酸处理使其具有超强酸性。
将制备的介孔二氧化钛用于降解废水模拟物罗丹明B,测试其光催化与氧催化降解能力。
通过紫外-可见分光光度计、X射线衍射、电镜扫描等对催化剂进行表征,实验结果表明,在强酸修饰二氧化钛前驱体的影响下,掺杂锌锆的介孔二氧化钛具有光催化与氧催化活性。
锌锆共掺杂介孔二氧化钛的光催化与氧催化效率分别达到了72%与25%o硫酸处理后在TiO2与掺杂原子表明形成酸性中心,在无光条件下氧化降解废水效率为30%,提高了降解效率。
关键词:二氧化钛;光催化;酸催化;罗丹明B中图分类号:X703.5文献标志码:A文章编号:1674-1404(2021)02-0136-04Composite degradation of rhodamine B using TiO2withphotocatalytic oxygen and super acidWEN Yu,YANG Dawei(SchoolofLightndustryandChemicalEngineering,DalianPolytechnicUniversity,Dalian116034,China) Abstract:The mesoporous titania doped with zinc oxide,zirconium dioxide,zinc and zirconium were prepared by the co-crystallization method and the precursor of mesoporous titania was pretreated with sulfuric acid to endowed it super acidic.The mesoporous titania was used for degradation of rhodamine B in simulated wastewater and its photocatalytic activity and oxygen catalytic ability was analyzed by UV-visible spectrophotometer,X ray diffraction,scanning electron microscopy.The results showed that the T1O2doped metal oxides and super acid exhibited excellent photocatalytic and oxygen catalytic ability.The degradation rate of rhodamine B photocatalyzed and oxygen catalyzed by the prepared catalysts were72%and25%,respectively.After treatment with sulfuric acid,the acidic centers were formed between the doped atoms and the surface of titanium dioxide,which improved the oxygen degrading efficiency of wastewater to30%.Keywords:TiO2;photocatalytic;acidic catalysis;rhodamine B0引言工业生产中生成的有机废水对环境造成严重污染,国家对废水排放标准执行越来越严格,如何降低或消除有机废水中大分子有机物成为研究的重点。
- 1、下载文档前请自行甄别文档内容的完整性,平台不提供额外的编辑、内容补充、找答案等附加服务。
- 2、"仅部分预览"的文档,不可在线预览部分如存在完整性等问题,可反馈申请退款(可完整预览的文档不适用该条件!)。
- 3、如文档侵犯您的权益,请联系客服反馈,我们会尽快为您处理(人工客服工作时间:9:00-18:30)。
LIGHT WEIGHT MULTIFUNCTIONAL COMPOSITES WITH ENHANCEDMECHANICAL PROPERTIESDiego Pedrazzoli, G. W. Woodruff School of Mechanical Engineering - Georgia Institute of Technology, Atlanta, GA & Department of Industrial Engineering, University of Trento, Trento, Italy&Alessandro Pegoretti, Department of Industrial Engineering, University of Trento, Trento, ItalyKyriaki Kalaitzidou, G. W. Woodruff School of Mechanical Engineering & School of Materials Scienceand Engineering - Georgia Institute of Technology, Atlanta, GAAbstractIn this study, polypropylene (PP) composites reinforced with short glass fibers (GF) and expanded graphite nanoplatelets (xGnP) were produced by melt compounding and injection molding. Quasi-static tensile tests and morphological observations were carried out in order to investigate how the morphology and the mechanical properties of the composites were affected by the combined effect of two fillers of rather different size scales (i.e. micro- and nano- scale). The results indicate that it is possible to introduce the nano-materials at the GF-PP interphase and significantly improve the tensile modulus of the composites, leading to lighter and stronger composites, as part of the higher density GF can be replaced with a small amount of the nano-materials. In addition to decreasing the weight of the composite, the processability is significantly improved as the increase in polymer viscosity reduces with decreasing the GF content. In conclusion, the results lead to hybrid composites that combine the advantages of nano-materials and micro-size reinforcements.IntroductionFiber reinforced polymer composites have been widely used in structural applications such as aerospace, automotive, civil and marine structures, especially due to their high specific stiffness and strength, chemical and weather resistances, tailorable mechanical, thermal and electrical properties [1,2]. In most cases, high loadings of GF are required to achieve the desired performance; this leads to an undesirable increase in specific gravity, decreased melt flow, and increased brittleness [3]. Short-fiber reinforced composites have been recently applied in a growing number of applications in engineering and consumer products in order to improve or tailor certain thermo-mechanical properties of the matrix for specific applications or to reduce the cost of the article.In general, short-fiber reinforced composites are much less resistant to mechanical load and fatigue damage than the corresponding continuous-fiber-reinforced materials, mainly because the weak matrix has to sustain a greater proportion of the load [5]. On the other hand, polymer nanocomposites based upon nanofillers such as silica, expanded graphite and carbon nanotubes have recently attracted great interest due to the considerable enhancement in stiffness realized at very low filler loadings [6,7]. Moreover, substantial improvements in mechanical, thermal, and flammability properties have been reported, while maintaining similar density and optical properties to those of the neat polymer matrix [8,9]. Furthermore, there are some clear indications that nanoparticles could play a beneficial role on the interfacial properties of structural composites [10,11].Although polymer nanocomposites exhibit improved thermal and mechanical properties at very low filler contents, loadings of more than 10 wt% usually lead to poor dispersion and processing characteristics [11]. Nevertheless, glass fiber loadings of 30 to 50 wt% are quite common [12]. It is of great interest to explore whether combining two fillers of rather different size scales (i.e. micro- and nano- scale) would give the desired performance at low to intermediate filler loadings. Only few preliminary studies have been reported on the structure and properties of glass fiber reinforced polymer nanocomposites [13,14]. The aim of this study is to investigate how the morphology and the mechanical properties of short-glass fiber reinforced PP nanocomposites are affected by the combined effect of nanofiller and reinforcing fibers.Materials and Composite FabricationThe matrix of the composite samples used in this work was an isotactic homopolymer polypropylene (MFI of 6.9 g/10’ at 190 °C and 2.16 kg ; density=0.904 g·cm-3) produced by Polychim Industrie S.A.S. (LOON-PLAGE, France) and provided by Lati Industria Termoplastici S.p.A (Varese, Italy) with the commercial code PPH-B-10-FB. FUSABOND®P M-613-05 maleic anhydride modified polypropylene (PPgMA) (MFI at 190 °C and 2.16 kg = 106.8 g/10’, density=0.903 g·cm-3, with maleic anhydride content in the range of 0.35 - 0.70 wt%), was supplied by DuPont™ de Nemours (Geneva, Switzerland).Exfoliated graphite nanoplatelets xGnP®-M5 have been supplied from XG SCIENCE Inc. (East Lansing, USA). This filler is platelets with an average diameter of ~5 µm and thickness in the range of 10~20 nm.E-glass fibers, designed as RO99 P319, were supplied by Saint-GobainVetrotex (Chambèry Cedex, France) and were used as-received. These GF are indicated as treated with a silane based coupling agent specifically designed for polypropylene matrices. Chopped strand glass fibers (single fiber diameter of 15.3 ± 1.5 µm, and average length of 6.50 ± 0.44 mm) were obtained by chopping long glass fibers using a chopper gun CDA-08 provided by GlasCraft (Graco®, Bury, England).xGnP-coated glass fibers were prepared by sonication of xGnP-M5 in isopropanol with a filler concentration of 5 mg/ml. Sonication was carried out using a Misonix S-4000-010 (Farmingdale, NY) for 1 h (30% amplitude, 8 W power) equipped with a probe of 12.5 mm diameter. After adding the glass fibers to the solution, a second sonication was performed for ½h. Coated glass fibers were finally rinsed in isopropanol and left under hood overnight to let the solvent completely evaporate. The xGnP content added onto the fibers’ surfaces through sonication was measured around 0.1-0.2 wt%.Composite samples were produced by melt mixing and injection molding. A vertical, co-rotating, bench-top twin-screw micro-extruder (DSM Micro 15 cm3 Compounder) connected to a micro-injection molding unit (DSM) were used to obtain dogbone specimens. The compound was mixed for 3 min, at 190 °C and a screw speed of 250 rpm. After the polymer compound had melted and homogenized, short GF strands were added to the melt and further mixing for 2 min and final injection moulding were carried out. The temperature of the mold was 80 °C, while the injection molding pressure was about 800 KPa. Ternary nanocomposites were prepared by adding 5 wt% of PPgMA as a compatibilizer to the systems containing 5 wt% of nanofiller.Composites were designated indicating the matrix, the compatibilizer (if any) with its content, the kind of filler with its amount. For instance, the composite based on unfilled matrix loaded with 10 wt% GF was denoted as PP/GF-10. On the other hand, a sample filled with 5 wt% of PPgMA, 5 wt% of xGnP-M5 and 10 wt% GF was indicated as PP-PPgMA-5-xGnP-5/GF-10. Coated glass fibers were indicated as GFc.Experimental techniquesFracture surfaces of nanocomposite samples were observed at various magnifications by using a Phenom G2 Pro (Phenom-World BV., Eindhoven, The Netherlands) bench-top scanning electron microscope (SEM), at an acceleration voltage of 5 kV. Prior to the SEM observations, a thin gold coating was applied on the surface by plasma sputtering in order to minimize the charging effects.Uniaxial ramp tensile tests were performed according to ASTM D638 with an Instron model 33R 4466 (Norwood, USA) tensile tester equipped with a 500 N load cell, on samples consisting of at least five dogbone specimens. Tests were carried out at a crosshead speed of 5 mm/min. Axial strain was recorded by using a resistance extensometer Instron® model 2630-101 with a gauge length of 10 mm. The elastic modulus was measured as secant modulus between longitudinal deformation levels of 0.05 % and 0.25 %.Dynamic mechanical analyses (DMA) were carried out at a DMA Q800 (TA Instruments, New Castle, Delaware, USA) testing machine over a temperature range between -20 °C and 160 °C, imposing a heating rate of 5 °C/min and a frequency of 1 Hz. A preload of 0.2 MPa and a maximum strain of 0.05 % were imposed on rectangular samples 25 mm long, 3.30 mm wide and 3.27 mm thick. The most important viscoelastic functions (E’, E’’, tan(δ)) were recorded at different temperatures.Results and discussionAs the amount of xGnP increases both tensile modulus (E) and ultimate tensile strength (UTS) increase, as reported in Figure 1, while the elongation at ultimate tensile strength (εUTS) decreases. This trend is consistent with observations for adding fillers to a relatively brittle matrix. In addition, the higher yield strength exhibited by the hybrid samples with respect to the sample PP/GF-10, reflects the greater interfacial sheat strength between fiber and matrix which promotes a better stress transfer between matrix and fiber.Figure 1. Elastic modulus of hybrid PP compositesThe superiority of hybrid composites with respect to just GF composites (i.e. PP/GF-10 and PP/GF-30), see Figure 2, is more evident when the specific properties are taken into account (specific elastic modulus and the specific ultimate tensile strength, E* and UTS*, respectively). In particular, the values of UTS* exhibited by hybrid composites greatly overcome those of PP/GF-10 andPP/GF-30.Figure 2. Specific Elastic modulus of hybrid PPcompositesHybrid composites are thus lighter and stronger than GF composites. Interestingly, the composites of coated GF i.e. PP/GFc-10 and PP-xGnP-5/GFc-10 exhibit slightly higher E* and UTS* compared to PP/GF-10 and PP-xGnP-5/GF-10 composites respectively, where the graphite was added directly in the polymer instead of being introduced at the GF-polymer interface, probably indicating an enhanced adhesion between fiber and matrix. Improved interfacial properties can also be observed when the compatibilizer PPgMA is added to the matrix (i.e. PP-PPgMA-5-xGnP-5), as the UTS is significantly higher that of the PP-xGnP-5 composite that does not contain compatibilizer. Concurrently, the incorporation of xGnP in composites produces a substantial increase in glass transition temperature (T g), as evaluated at the tan(δ) peak during DMA experiments (Figure 3). Since changes in T g are related to the primary relaxation of polymer chains and the extent of the immobilized chains, the alteration of polymer chain mobility promoted by polymer-xGnP physical interactions might significantly contribute to the reinforcing mechanisms. Therefore xGnP reinforces PP not only because it is stiffer but also because it remarkably alters locally the physical properties of the polymer.In the same way, lower values of tanδrecorded upon nanofiller addition in hybrid composites can be attributed not only to the enhanced fiber-matrix adhesion, but also to polymer-xGnP physical interactions, resulting in lower loss modulus and enhanced elastic modulus of PP. Figure 3. Thermomechanical properties of the composites SEM micrographs of fracture surfaces for PP composites loaded with 10 wt% GF are shown in Figure 4a. The glass fibers are generally well dispersed in the PP matrix, and many fibers are pulled out from the matrix. The interfacial debonding appears to be the dominant failure mechanism, indicating low-adhesion condition. On the other hand, a different failure behavior is observed for hybrid PP composites that contain 5 wt% xGnP and 10 wt% glass fibers (Figure 4b) and 5 wt% PPgMA, 5 wt% xGnP and 10 wt% glass fibers (Figure 4c). In this case, a matrix crack occurred, showing a significantly better fiber-matrix adhesion. Furthermore, the substantial chemical affinity between fiber and matrix is evidenced by the presence of residual matrix on the fiber surface after the pull-out.(a)(b)(c)Figure 4. SEM micrographs of (a) PP/GF-10, (b) PP-xGnP-5/GF-10 and (c) PP-PPgMA-5-xGnP-5/GF-10.ConclusionsIn this study, the mechanical properties of the hybrid GF/PP composites are determined as a function of the graphite nanoplatelets content, and physical properties of PP including glass transition, as well as the dispersion of the nano-materials within the polymer, are investigated. Results indicated that hybrid composites can combine the advantages of nano-materials and micro-size reinforcements, resulting in an enhanced elastic modulus and ultimate tensile strength, concurrently with an improved visco-elastic behavior. Morphological observations confirmed that the higher UTS obtained in hybrid composites is a result of not only matrix reinforcement through nanofillers, but also of the strong interfacial interactions between matrix and glass fibers.References1. M.R. Piggot. Load bearing fibre composites. Oxford: Pergamon Press. 1980.2. J-K Kim, Y-M Mai. Engineered interfaces in fiber reinforced composites. Amsterdam, The Netherlands: Elsevier; 1998.3. A.G. Evans, F.W. Zok. Journal of Materials Science. 1994; 29:3857-3896.4. A. Pegoretti, T. Ricco. Composites Science and Technology. 1999; 59:1055-1062.5. D. Pedrazzoli, A. Dorigato, A. Pegoretti. Journal of Nanoscience and Nanotechnology. 2012; 12:4093-4102.6. M. Tait, A. Pegoretti, A. Dorigato, K. Kalaitzidou. Carbon. 2011; 49(13):4280-4290.7. F. Hussain. Journal of Composite Materials. 2006; 40(17):1511-1575.8. RF Gibson. Composite Structures. 2010; 92(12):2793-2810.9. D. Pedrazzoli, A. Pegoretti. Composites Science and Technology. 2013;76:77-83.10. M. Etcheverry M, M.L. Ferreira , N.J. Capiati, A Pegoretti, SE Barbosa. Composites Part A - Applied Science and Manufacturing. 2008; 39(12):1915-1923. 11. K. Kalaitzidou, H. Fukushima, L.T. Drzal. Carbon. 2007; 45(7):1446-1452.12. C.C Chamis. Journal of Reinforced Plastics and Composites. 2007; 26(10):987-1019.13. N.A. Isitman, H.O. Gunduz, C. Kaynak. Polymer Degradation and Stability. 2009; 94(12):2241-2250.14. D.P.N. Vlasveld, P.P. Parlevliet, H.E.N. Bersee, S.J. Picken. Composites Part A: Applied Science and Manufacturing. 2005; 36(1):1-11.。