Cloning and characterization of a soluble acid invertase-encoding gene from muskmelon
Cloning and characterization of a novel barley gene,HvORG4,induced by Fusarium graminearum infec
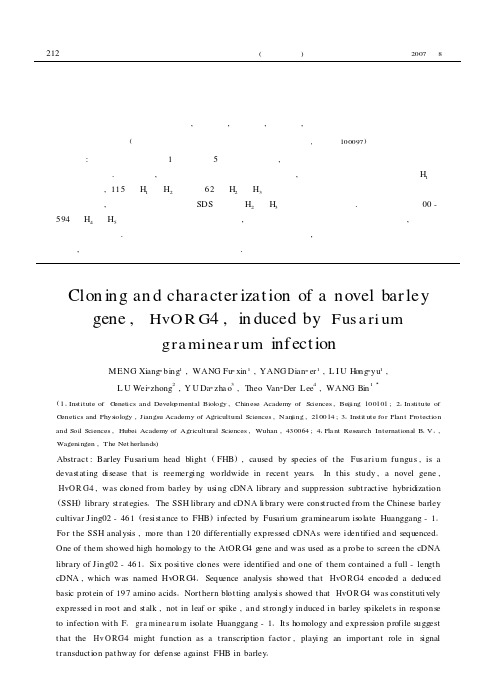
小麦花培品质育种各世代的品质参数分析张立平,苏 青,刘建平,单福华,赵昌平(北京市农林科学院北京杂交小麦工程技术研究中心,北京 100097)摘 要:通过对冬小麦花培1代至花培5代的品质测试,研究了花培品质育种的品质测试的世代程序和有效性.结果表明,品质性状在花培早代已趋于稳定,高分子量谷蛋白亚基组成从H 1起就稳定遗传,115对H 1与H 2材料和62对H 2与H 3材料的籽粒蛋白质含量、硬度和沉降值具有高度相关性,且籽粒蛋白质含量和SDS 沉降值H 2与H 3世代间差异不显著.对中选的京单00-594的H 4和H 5世代进一步品质测定结果表明,通过花培早代选出的优质材料是可靠的,其优质性状能稳定遗传.提出了花培品质育种各世代品质测试的程序,证明了花药培养结合早代品质测试,是一条快速、有效的小麦品质育种途径.Clon ing an d character izat ion of a novel bar leygene ,HvO R G 4,in duced by Fus a ri umgra minea r um inf ect ionMENG Xiang 2bing 1,WANG Fu 2xin 1,YANG Dian 2er 1,L I U H ong 2yu 1,L U Wei 2zhong 2,Y U Da 2zha o 3,Theo V an 2Der Lee 4,WANG Bin 13(11Institute of G enetics and Develo pmental Biology ,Chinese Academy of Sciences ,Beijing 100101;21Institute of G enetics and Phys iology ,Jiangsu Academy of Agricultural Sciences ,Nan jing ,210014;31Instit ute for Plant Protection and Soil Sciences ,Hubei Academy of Agricultural Sciences ,Wuhan ,430064;41Pla nt Research International B 1V 1,Wageningen ,The Net herlands)Abstract :Barley Fusari um head blight (FHB ),caused by species of t he Fus ari u m fungus ,is a devast at ing di sease t hat is reemergi ng worldwide in recent years 1In t his study ,a novel gene ,HvO R G 4,was cloned from barley by usi ng cDNA library and suppression subt ract ive hybridization (SSH )library st rat egies 1The SSH library and cDNA li brary were const ruct ed from t he Chinese barley cultivar Jing02-461(resi st ance to FHB )i nfected by Fusari um graminearum isolate Huanggang -11For t he SSH anal ysis ,more than 120differentially expressed cDNAs were i dentified and sequenced 1One of t hem showed high homology to t he AtOR G 4gene and was used as a probe to screen t he cDNA library of Jing02-4611Si x posi tive clones were identified and one of t hem cont ained a full -lengt h cDNA ,which was named HvOR G 41Sequence analysis showed t hat HvO RG4encoded a deduced basic prot ein of 197amino acids 1Nort hern blot ting analysi s showed t hat HvO R G 4was constit uti vely expressed i n root and st alk ,not in leaf or spike ,and st rongl y induced i n barley spikelet s in response f F 11I y x f O RG f f ,y y f f F B y 1212湖南农业大学学报(自然科学版)2007年8月to in ection wit h gra minearu m isolate Huanggang -1t s homolog and e pression pro ile suggest t hat the Hv 4might unction as a t ranscription actor pla i ng an import ant role in signal t ransduct ion pat hwa or de ense against H in barle。
UDP-糖基供体的生物合成途径分析

UDP-糖基供体的生物合成途径分析尹森;孔建强【期刊名称】《中国医药生物技术》【年(卷),期】2016(011)004【总页数】5页(P355-359)【作者】尹森;孔建强【作者单位】100050 北京,中国医学科学院北京协和医学院药物研究所天然药物活性物质与功能国家重点实验室/卫生部天然药物生物合成重点实验室;100050 北京,中国医学科学院北京协和医学院药物研究所天然药物活性物质与功能国家重点实验室/卫生部天然药物生物合成重点实验室【正文语种】中文糖基化是生物体中重要的生化反应,它可发生于大分子化合物,如蛋白质的糖基化[1];也可发生于小分子化合物,如各种天然产物苷元的糖基化[2]。
执行化合物糖基化反应的催化酶为糖基转移酶。
糖基转移酶的底物分为糖基受体和糖基供体。
糖基受体可以为生物大分子化合物如蛋白质、核酸,还可以为小分子化合物如各种植物或微生物的次生代谢产物[3-5]。
糖基受体通常含一些活性基团,如羟基、氨基、羧基、巯基等用于形成糖苷键,同时有少数糖基转移酶还可以催化 C-C 键的形成,生成碳苷类化合物[6-7]。
糖基供体为糖的活化形式,通常糖基供体中存在一个或多个高能磷酸键,这些高能磷酸键在糖基转移酶执行催化反应时能通过能量转移而形成化合物与糖的糖苷键。
常见糖基供体为尿苷二磷酸糖(UDP-糖)、胸腺苷二磷酸糖(TDP-糖)、鸟苷二磷酸糖(GDP-糖)、一磷酸糖等。
这些糖基供体之间可以通过不同的代谢酶进行相互转化(图 1)。
生物体中最常见的糖基供体形式为 UDP-糖。
常见的UDP-糖有两种类型,一种为六碳糖,包括 UDP-葡萄糖、UDP-葡萄糖醛酸、UDP-半乳糖、UDP-半乳糖醛酸和 UDP-鼠李糖等;一种为五碳糖,包括 UDP-木糖、UDP-阿拉伯糖和 UDP-芹菜糖等。
其中 UDP-葡萄糖是其他 UDP-单糖形成的起始物,而 UDP-葡萄糖醛酸是 UDP-单糖由六碳糖转变为五碳糖的关键底物。
CD90+和CD90-成纤维细胞亚群脂成纤维细胞转化的研究-论文
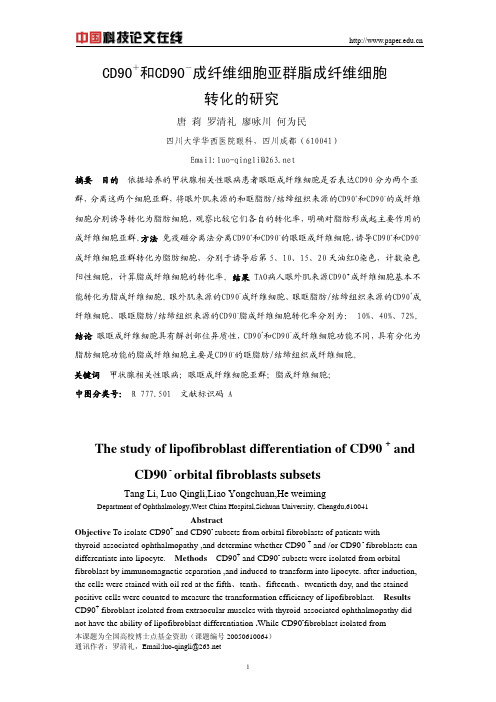
本课题为全国高校博士点基金资助(课题编号20050610064)通讯作者:罗清礼,Email:******************CD90+和CD90-成纤维细胞亚群脂成纤维细胞转化的研究唐 莉 罗清礼 廖咏川 何为民四川大学华西医院眼科,四川成都(610041) Email:******************摘要 目的 依据培养的甲状腺相关性眼病患者眼眶成纤维细胞是否表达CD90分为两个亚群,分离这两个细胞亚群,将眼外肌来源的和眶脂肪/结缔组织来源的CD90+和CD90-的成纤维细胞分别诱导转化为脂肪细胞,观察比较它们各自的转化率,明确对脂肪形成起主要作用的成纤维细胞亚群。
方法 免疫磁分离法分离CD90+和CD90-的眼眶成纤维细胞,诱导CD90+和CD90-成纤维细胞亚群转化为脂肪细胞,分别于诱导后第5、10、15、20天油红O染色,计数染色阳性细胞,计算脂成纤维细胞的转化率。
结果 TAO病人眼外肌来源CD90+成纤维细胞基本不能转化为脂成纤维细胞。
眼外肌来源的CD90-成纤维细胞、眼眶脂肪/结缔组织来源的CD90+成纤维细胞、眼眶脂肪/结缔组织来源的CD90-脂成纤维细胞转化率分别为: 10%、40%、72%。
结论 眼眶成纤维细胞具有解剖部位异质性,CD90+和CD90-成纤维细胞功能不同,具有分化为脂肪细胞功能的脂成纤维细胞主要是CD90-的眶脂肪/结缔组织成纤维细胞。
关键词 甲状腺相关性眼病;眼眶成纤维细胞亚群;脂成纤维细胞;中图分类号: R 777.501 文献标识码 AThe study of lipofibroblast differentiation of CD90 + andCD90 - orbital fibroblasts subsetsTang Li, Luo Qingli,Liao Yongchuan,He weimingDepartment of Ophthalmology,West China Hospital,Sichuan University, Chengdu,610041AbstractObjective To isolate CD90+ and CD90- subsets from orbital fibroblasts of patients withthyroid-associated ophthalmopathy ,and determine whether CD90 + and /or CD90 - fibroblasts can differentiate into lipocyte. Methods CD90+ and CD90- subsets were isolated from orbitalfibroblast by immunomagnetic separation ,and induced to transform into lipocyte. after induction, the cells were stained with oil red at the fifth 、tenth 、fifteenth 、twentieth day, and the stained positive cells were counted to measure the transformation efficiency of lipofibroblast. Results CD90+ fibroblast isolated from extraocular muscles with thyroid-associated ophthalmopathy did not have the ability of lipofibroblast differentiation .While CD90-fibroblast isolated fromextraocular muscles and CD90+ and CD90-fibroblast isolated from orbital connective tissue/fat can transform into lipofibroblas,their transformation efficiency were 10%、40% and 72%,respectively.Conclusion Orbital fibroblast have heterogeneity with respect to anatomic site. The function of CD90+ and CD90-fibroblast is different, the lipofibroblast that has an ability to differentiate towards lipocyte is main CD90-fibroblast of Orbital adipose/connective tissue. Keywords Thyroid associated ophthalmopathy; orbital fibroblasts subsets; Lipofibroblast;1. 引言甲状腺相关眼病(Thyroid associated ophthalmopathy, TAO)是眼科常见病,主要表现为眶周水肿、眼球突出、暴露性角膜炎、复视和压迫性视神经病变等临床改变,眼眶组织的病理研究证实病变主要累及眼外肌与眼眶脂肪/结缔组织,正是眼眶脂肪增多、眼外肌纤维化、亲水性细胞外基质的大量堆积导致了上述一系列的临床表现。
上海交通大学医学院举办第八届中国医院发展与管理学术大会

1467上海交通大学学报(医学版),2020,40 (11)presenting with hyperinsulinemic hypoglycemia and islet cell hyperplasia [J ]. J Clin Endocrinol Metab, 2005, 90(7): 4371-4375.[5] K ranz C, Sun LW, Eklund EA, et al. CDG-Id in two siblings with partiallydifferent phenotypes [J ]. Am J Med Genet, 2007, 143A(13): 1414-1420.[6] R imella-Le-huu A, Henry H, Kern I, et al. Congenital disorder of glycosylationtype Ⅰ d (CDG Ⅰd): phenotypic, biochemical and molecular characterization of a new patient [J ]. J Inherit Metab Dis, 2008, 31(Suppl 2): S381-S386.[7] S tibler H, Stephani U, Kutsch U. Carbohydrate-deficient glycoproteinsyndrome: a fourth subtype [J ]. Neuropediatrics, 1995, 26(5): 235-237.[8] R iess S, Reddihough DS, Howell KB, et al. ALG3-CDG (CDG-Ⅰ d ): clinical,biochemical and molecular findings in two siblings [J ]. Mol Genet Metab, 2013, 110(1/2): 170-175.[9] L epais L, Cheillan D, Frachon SC, et al. ALG3-CDG: Report of two siblingswith antenatal features carrying homozygous p.Gly96Arg mutation [J ]. Am J Med Genet A, 2015, 167(11): 2748-2754.[10] H aeuptle MA, Hennet T. Congenital disorders of glycosylation: an update ondefects affecting the biosynthesis of dolichol-linked oligosaccharides [J ]. Hum Mutat, 2009, 30(12): 1628-1641.[11] N g BG, Shiryaev SA, Rymen D, et al. ALG1-CDG: clinical and molecularcharacterization of 39 unreported patients [J ]. Hum Mutat, 2016, 37(7): 653-660.[12] F rank CG, Eyaid W, Berger EG, et al. Identification and functional analysisof a defect in the human ALG9 gene: definition of congenital disorder of glycosylation type IL [J ]. Am J Hum Genet, 2004, 75(1): 146-150.[13] Z ufferey R, Knauer R, Burda P, et al. STT3, a highly conserved protein requiredfor yeast oligosaccharyl transferase activity in vivo [J ]. EMBO J, 1995, 14(20): 4949-4960.[14] A ebi M, Gassenhuber J, Domdey H, et al. Cloning and characterization of the ALG3 gene of Saccharomyces cerevisiae [J ]. Glycobiology, 1996, 6(4): 439-444.[15] K orner C. Carbohydrate deficient glycoprotein syndrome type Ⅳ : deficiency ofdolichyl-P-Man: Man5GlcNAc 2-PP-dolichyl mannosyltransferase [J ]. EMBO J, 1999, 18(23): 6816-6822.[16] K ranz C, Basinger AA, Güçsava ş-Çalıko ğlu M, et al. Expanding spectrum ofcongenital disorder of glycosylation Ⅰ g (CDG- Ⅰg): sibs with a unique skeletal dysplasia, hypogammaglobulinemia, cardiomyopathy, genital malformations, and early lethality [J ]. Am J Med Genet A, 2007, 143(12): 1371-1378.[17] L i ST, Wang N, Xu S, et al. Quantitative study of yeast Alg1 β-1,4mannosyltransferase activity, a key enzyme involved in protein N-glycosylation [J ]. Biochim Biophys Acta Gen Subj, 2017, 1861(1): 2934-2941.[18] K efala G, Kwiatkowski W, Esquivies L, et al. Application of Mistic toimproving the expression and membrane integration of histidine kinase receptors from Escherichia coli [J ]. J Struct Funct Genomics, 2007, 8(4): 167-172.[19] 李盛陶. 体外重构N-糖基化途径中多萜醇寡糖前体的合成[D ]. 无锡: 江南大学, 2018.[20] L i ST, Lu TT, Xu XX, et al. Reconstitution of the lipid-linked oligosaccharidepathway for assembly of high-mannose N-glycans [J ]. Nat Commun, 2019, 10: 1813.[21] 李庆猛, 李盛陶, 王宁, 等. 酵母来源α-1,2甘露糖转移酶Alg11的异源表达、纯化和活性分析[J ]. 中国生物工程杂志, 2018, 38(6): 26-33.[22] H o SN, Hunt HD, Horton RM, et al. Site-directed mutagenesis by overlapextension using the polymerase chain reaction [J ]. Gene, 1989, 77(1): 51-59.[23] B urgos J, Hemming FW, Pennock JF, et al. Dolichol: a naturally-occurring c100isoprenoid alcohol [J ]. Biochem J, 1963, 88(3): 470-482.[收稿日期][本文编辑]2019-08-30瞿麟平罗冰洁,等 ALG3异常的先天性糖基化疾病相关突变蛋白活性的检测。
TGA 转录因子在植物氧化胁迫应答中的调控作用
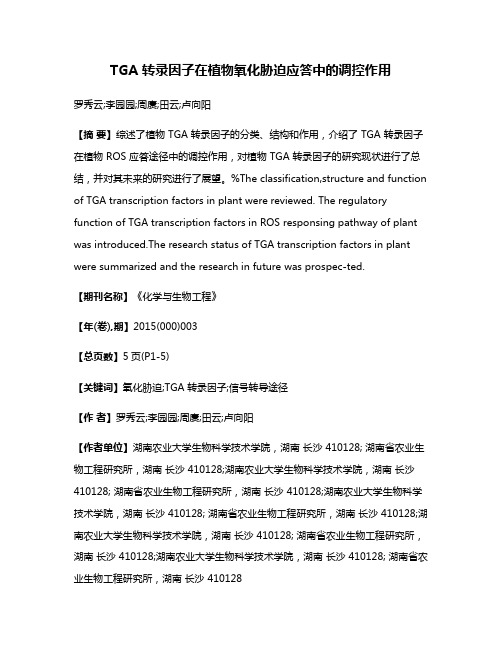
TGA 转录因子在植物氧化胁迫应答中的调控作用罗秀云;李园园;周赓;田云;卢向阳【摘要】综述了植物 TGA 转录因子的分类、结构和作用,介绍了 TGA 转录因子在植物 ROS 应答途径中的调控作用,对植物 TGA 转录因子的研究现状进行了总结,并对其未来的研究进行了展望。
%The classification,structure and function of TGA transcription factors in plant were reviewed. The regulatory function of TGA transcription factors in ROS responsing pathway of plant was introduced.The research status of TGA transcription factors in plant were summarized and the research in future was prospec-ted.【期刊名称】《化学与生物工程》【年(卷),期】2015(000)003【总页数】5页(P1-5)【关键词】氧化胁迫;TGA 转录因子;信号转导途径【作者】罗秀云;李园园;周赓;田云;卢向阳【作者单位】湖南农业大学生物科学技术学院,湖南长沙 410128; 湖南省农业生物工程研究所,湖南长沙 410128;湖南农业大学生物科学技术学院,湖南长沙410128; 湖南省农业生物工程研究所,湖南长沙 410128;湖南农业大学生物科学技术学院,湖南长沙 410128; 湖南省农业生物工程研究所,湖南长沙 410128;湖南农业大学生物科学技术学院,湖南长沙 410128; 湖南省农业生物工程研究所,湖南长沙 410128;湖南农业大学生物科学技术学院,湖南长沙 410128; 湖南省农业生物工程研究所,湖南长沙 410128【正文语种】中文【中图分类】Q78植物体内各组织器官(如根、茎、叶、花、果)时刻都在发生生理代谢,在代谢过程中会产生活性氧(ROS)。
链格孢属真菌(Alternaria)属有丝分裂孢子真菌类群丝孢纲丝b...b-YNSTC
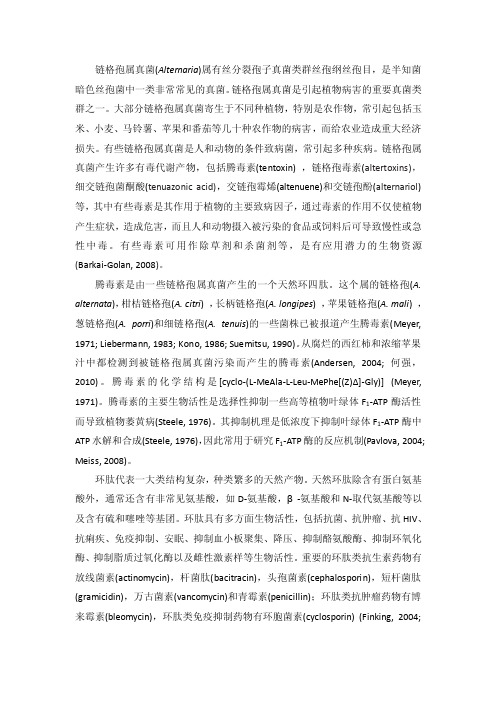
链格孢属真菌(Alternaria)属有丝分裂孢子真菌类群丝孢纲丝孢目,是半知菌暗色丝孢菌中一类非常常见的真菌。
链格孢属真菌是引起植物病害的重要真菌类群之一。
大部分链格孢属真菌寄生于不同种植物,特别是农作物,常引起包括玉米、小麦、马铃薯、苹果和番茄等几十种农作物的病害,而给农业造成重大经济损失。
有些链格孢属真菌是人和动物的条件致病菌,常引起多种疾病。
链格孢属真菌产生许多有毒代谢产物,包括腾毒素(tentoxin) ,链格孢毒素(altertoxins),细交链孢菌酮酸(tenuazonic acid),交链孢霉烯(altenuene)和交链孢酚(alternariol)等,其中有些毒素是其作用于植物的主要致病因子,通过毒素的作用不仅使植物产生症状,造成危害,而且人和动物摄入被污染的食品或饲料后可导致慢性或急性中毒。
有些毒素可用作除草剂和杀菌剂等,是有应用潜力的生物资源(Barkai-Golan, 2008)。
腾毒素是由一些链格孢属真菌产生的一个天然环四肽。
这个属的链格孢(A. alternata),柑桔链格孢(A. citri) ,长柄链格孢(A. longipes) ,苹果链格孢(A. mali) ,葱链格孢(A. porri)和细链格孢(A. tenuis)的一些菌株已被报道产生腾毒素(Meyer, 1971; Liebermann, 1983; Kono, 1986; Suemitsu, 1990)。
从腐烂的西红柿和浓缩苹果汁中都检测到被链格孢属真菌污染而产生的腾毒素(Andersen, 2004; 何强,2010)。
腾毒素的化学结构是[cyclo-(L-MeAla-L-Leu-MePhe*(Z)∆+-Gly)] (Meyer, 1971)。
腾毒素的主要生物活性是选择性抑制一些高等植物叶绿体F1-ATP酶活性而导致植物萎黄病(Steele, 1976)。
其抑制机理是低浓度下抑制叶绿体F1-ATP酶中ATP水解和合成(Steele, 1976),因此常用于研究F1-ATP酶的反应机制(Pavlova, 2004; Meiss, 2008)。
低温淀粉酶

目前国内已筛选到多种产低温淀粉酶的菌种,张刚等(2002)自黄、东海分离到野生青霉菌Penicillumsp. FS010441,其分泌的淀粉酶最适反应温度为40℃。范红霞等(2009)自南极土壤分离到的微球菌(Micrococcus antarcti⁃cus)所产α-淀粉酶最适作用温度为30℃。孙子羽等(2010)自渤海湾海泥中分离得到一株产低温生淀粉糖化酶能力较强的气单胞菌属菌株,所产低温生淀粉糖化酶最适反应温度为30℃[20]。师永生等(2011)克隆了蜡样芽孢杆菌(Bacillus cereus)GXBC-1的低温淀粉酶基因,重组蛋白最适反应温度为35℃[12]。齐兴娥等(2013)自天山1号冰川底部沉积层分离到产低温淀粉酶菌株Hymenobactersp.。从江布拉克冰川冻土中筛选到的高产低温淀粉酶菌株Pseudomonassp. D5-2,酶的最适作用温度为25℃。楚敏等(2014)获得低温菌株气单胞菌属LA77的低温淀粉酶基因及其生物学信息[21]。公颖卓等(2015)从秦皇岛海蟹样本获得一株高产低温α-淀粉酶的菌株,经鉴定为许旺酵母(Schwanniomyces),该酶的最适作用温度为35℃[2]。河北学者李青杨等人在东北地区也筛选到了能产低温淀粉酶的丁酸梭菌,该菌株所产的低温淀粉酶最适反应温度为35℃左右,属典型的低温淀粉酶。习彦花等(2016)前期筛选得到1株耐低温兼性厌氧淀粉酶产生菌Y89[22]。窦少华等(2017)从大连黄海海泥中分离出一株低温淀粉酶高产菌苏云金芽孢杆菌(Bacillus thuringiensis),发酵粗酶液最适作用温度为20℃,属于低温酶[23]。周新尚等(2017)从大连黄海海泥和海水中分离到菌株荧光假单胞菌(Pseudomonas fluorescens)ZXS-5,其所产淀粉酶的最适反应温度为25℃[24]。
波赛链霉菌dnrK基因中断突变株代谢产物鉴定
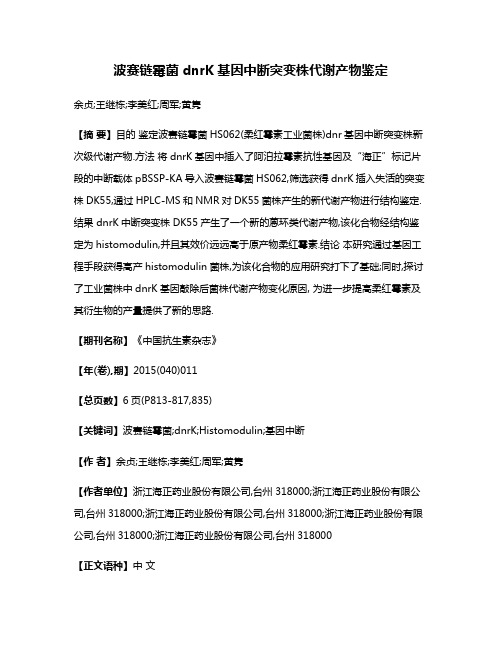
波赛链霉菌dnrK基因中断突变株代谢产物鉴定余贞;王继栋;李美红;周军;黄隽【摘要】目的鉴定波赛链霉菌HS062(柔红霉素工业菌株)dnr基因中断突变株新次级代谢产物.方法将dnrK基因中插入了阿泊拉霉素抗性基因及“海正”标记片段的中断载体pBSSP-KA导入波赛链霉菌HS062,筛选获得dnrK插入失活的突变株DK55,通过HPLC-MS和NMR对DK55菌株产生的新代谢产物进行结构鉴定.结果 dnrK中断突变株DK55产生了一个新的蒽环类代谢产物,该化合物经结构鉴定为histomodulin,并且其效价远远高于原产物柔红霉素.结论本研究通过基因工程手段获得高产histomodulin菌株,为该化合物的应用研究打下了基础;同时,探讨了工业菌株中dnrK基因敲除后菌株代谢产物变化原因, 为进一步提高柔红霉素及其衍生物的产量提供了新的思路.【期刊名称】《中国抗生素杂志》【年(卷),期】2015(040)011【总页数】6页(P813-817,835)【关键词】波赛链霉菌;dnrK;Histomodulin;基因中断【作者】余贞;王继栋;李美红;周军;黄隽【作者单位】浙江海正药业股份有限公司,台州318000;浙江海正药业股份有限公司,台州318000;浙江海正药业股份有限公司,台州318000;浙江海正药业股份有限公司,台州318000;浙江海正药业股份有限公司,台州318000【正文语种】中文【中图分类】R978.1自1963年意大利学者DiMarco等[1]从波赛链霉菌(Streptomyces peucetius)分离获得柔红霉素(daunorubicin,6)以来,国内外学者对波赛链霉菌进行了深入研究,先后分离出多柔比星(doxorubicin,7)[2]、表柔红霉素(epidaunorubicin)[3]、表阿霉素(epirubicin)[3]、洋红霉素(carminomycin,3)[4]、13-脱氧洋红霉素(13-deoxocarminomycin)[5]等一系列临床应用十分广泛的蒽环类抗肿瘤抗生素。
- 1、下载文档前请自行甄别文档内容的完整性,平台不提供额外的编辑、内容补充、找答案等附加服务。
- 2、"仅部分预览"的文档,不可在线预览部分如存在完整性等问题,可反馈申请退款(可完整预览的文档不适用该条件!)。
- 3、如文档侵犯您的权益,请联系客服反馈,我们会尽快为您处理(人工客服工作时间:9:00-18:30)。
Cloning and characterization of a soluble acid invertase-encoding gene from muskmelonHongmei Tian ÆQingguo Kong ÆYanqing Feng ÆXiyan YuReceived:12December 2007/Accepted:21February 2008/Published online:4March 2008ÓSpringer Science+Business Media B.V.2008Abstract Soluble acid invertase (S-AIV;EC 3.2.1.26)is thought to play a critical role in sucrose hydrolysis in muskmelon (Cucumis melo L.)fruit.A full-length cDNA clone encoding S-AIV was isolated from muskmelon by RT-PCR and RACE.The clone,designated as CmS-AIV1,contains 2178nucleotides with an open reading frame of 1908nucleotides.The deduced 636amino acid sequence showed high identities with other plant soluble acid in-vertases.Northern blot analysis indicated that CmS-AIV1was expressed in flowers and fruit,but was not detected in roots,stems or leaves.Moreover,the mRNA accumulation of CmS-AIV1showed its maximum level at 10days after pollination (DAP)and decreased gradually during fruit development until its minimum level at mature fruit.Interestingly,the sucrose content was very low in fruit before 20DAP but increased dramatically between 20and 30DAP during fruit development.In contrast to sucrose content,the activities of S-AIV was very high in fruit before 20DAP and decreased apparently between 20and 30DAP,suggesting that sucrose metabolism may be linked to the CmS-AIV1transcript level in muskmelon fruit.Keywords Cucumis melo ÁCmS-AIV1ÁGene expression ÁSucrose metabolismIntroductionSugars are the most important biochemical components for fruit quality.The kind and amount of sugars directly influence fruit flavor components,such as sweetness.As the first step toward the genetic improvement of the quality of muskmelon fruit,it is necessary to determine the sugar components accumulated in fruit,elucidate enzymes involved in sugar metabolism and clarify the relationship between the content of accumulated sugar and the activity of some related enzymes [1–3].At the middle stage of fruit development,muskmelon fruit undergoes a metabolic transition marked by both physical and compositional changes such as netting of the exocarp,mesocarp softening,and the onset of sucrose accumulation [4].Attempts to elucidate the changes in metabolism that lead to accumulation of sucrose have focused on sucrose metabolizing enzymes during fruit growth and development [5–8].It was reported that both acid invertase (EC 3.2.1.26)and sucrose phosphate syn-thase (EC 2.4.1.14)are determinants of sucrose accumulation in muskmelon fruit.Moreover,because sucrose accumulates in vacuoles,a reduction in acid invertase activity would be a prerequisite for muskmelon sucrose accumulation [9].We have cloned a sucrose phosphate synthase-encoding gene from muskmelon and it was shown to be expressed predominantly in mature fruit [10].Recently,acid invertase genes have been isolated from a number of higher plant species [11–16].In this report,we present the molecular cloning of CmS-AIV1from muskmelon.In addition,the differential expression of CmS-AIV1was determined in various tissues and fruit development stages of Cucumis melo .H.Tian ÁQ.Kong ÁY.Feng ÁX.Yu (&)State Key Laboratory of Crop Biology,College of Horticulture Science and Engineering,Shandong Agricultural University,Taian 271018,Chinae-mail:yuxiyan@Mol Biol Rep (2009)36:611–617DOI 10.1007/s11033-008-9219-2Materials and methodsPlant material and tissue samplingMuskmelon inbred line M01-3was grown in a greenhouse on the experimental farm of Shandong Agricultural Uni-versity in Tai’an,China March through June2007.Plant spacing was50cm between plants with120cm between rows.Average day/night temperatures were about30°C/ 20°C.Average daylight was about12h.Fertilizer was applied at two stages,a preplant broadcast application of 900kg ha-1of14N-14P-36K,followed by a sidedress application of150kg ha-1N atflowering stage.Irrigation by furrows was applied as needed.To identify fruit of known age freshly opened femaleflowers were tagged on the day of hand-pollination and one fruit per plant was allowed to develop.Different developing stage(5,10,15, 20and25DAP)and mature fruit were harvested;30DAP is considered commercial maturity.Five fruit from each stage were pooled to isolate total RNA.A portion of each fruit was diced into small pieces and used for S-AIV activity assay and sucrose determination.The S-AIV activity assays and sucrose determination were carried out on duplicate samples.The experiments were repeated three times.For each replicate,five fruit were used.Vegetative tissues,including leaves,stems,and roots were sampled along withflowers at bloom and harvested simultaneously fromfive7-to8-week-old plants.All tissues were quickly frozen in liquid nitrogen and stored at-80°C until late use. Extraction and purification of RNATotal RNA was isolated using the Guanidine Isothiocyanate-phenol-chloroform method as described by Sambrook et al.[17]with modification.Frozen tissue samples(5–10g)were ground to a powder in liquid N2using a mortar and pestle. Samples of the homogenized,powdered tissue were trans-ferred to50-ml screw-cap centrifuge tubes prechilled on the ice,followed by immediate addition of15ml solution D (4M Guanidine Isothiocyanate,0.025M trisodium citrate dehydrate,0.5%lauryl sarcosine)and of1.5ml of2M sodium acetate,pH4.0,15ml of phenol equilibrated with distilled water and3ml of49chloroform:1isopropyl alcohol(V/V).After vortexing and incubation for15min on the ice,the samples were processed according to Sam-brook’s method.The crude RNA preparations were then treated with DNase(TaKaRa Bio,Kyoto,Japan)to degrade genomic DNA,extracted with1phenol:1chloroform(V/V), and total RNA was precipitated by addition of1volume isopropyl alcohol plus0.1volume of3M sodium acetate, pH5.5.The precipitated RNA was pelleted by centrifuga-tion,washed with cold70%ethanol,and resuspended in diethyl pyrocarbonate-treated water.Quality of the extracted RNA was checked by agarose gel electrophoresis and RNA was quantified spectrophotomerically(UV2450,Shimadzu Corp.,Kyoto,Japan).Cloning of CmS-AIV1complete cDNATotal RNA isolated from5DAP fruit of C.melo M01-3 plants was used for the initial cloning of soluble acid invertase cDNA fragments,including products of30and50 RACE(rapid amplification of cDNA ends).As the initial step in cloning a C.melo soluble acid invertase gene, degenerate primers(P1:50-GACCCGACYACTGCTTGG-30,P2:50-CGATGTTATSACTGTTCT-30,Y=T+C, S=C+G)were designed based on the conserved domain of soluble acid invertase genes from other plants in Gen-Bank.RT-PCR was performed according to the protocol of RNA PCR Kit(AMV)Ver.3.0(TaKaRa Bio).Thefirst cDNA was synthesized by reverse transcription using reverse transcriptase with1l g RNA as template and with Oligo(dT)-Adaptor primer asfirst strand primer.The RT reaction program is42°C for30min,99°C for5min and 5°C for5min,1cycle.PCR was performed by running the following program:94°C pre-denature for5min;94°C for 1min,50°C for1min and72°C for1.5min,30cycles;at last,72°C for10min.The PCR product was separated by electrophoresis in1.0%agarose gel.The isolated fragment was cloned by using PMD18-T vector(TaKaRa Bio)for sequencing.A total of1l g RNA from muskmelon fruit was taken to convert mRNAs into cDNAs using a30RACE kit(TaKa-Ra)provided with AMV reverse transcriptase and a universal oligo(dT)containing adapter primer(50-CTGATCTAGAGGTACCGGATCCT17-30).The partial CmS-AIV1gene from the30end was then amplified by a pair of PCR primers:the gene specific forward primer1 (50-TACTCCGTGTTGACTCAGCTGCAG-30)and reverse primer(50-CTGATCTAGAGGTACCGGATCC-30)pro-vided with the kit.According to the manufacturer’s instructions,PCR was performed under the following conditions:cDNA was denatured at94°C for2min,fol-lowed by35cycles of amplification(94°C for30s,55°C for30s and72°C for1min)and7min at72°C.The PCR product was purified and cloned into pMD18-T vector for sequencing.Based on the sequence of the initial C.melo soluble acid invertase gene,the specific primers2,3,4(primer250-CGGGTTATCGGGTGTCCAT-30,primer350-CCCGGC CCGTTATATGATG-30,primer450-GCATGCAGCACT CCATCCA-30)were designed to amplify the50end of CmS-AIV1gene using50RACE system for rapid amplifi-cation of cDNA ends(version 2.0;Invitrogen Corp., Carlsbad,CA).The50RACE was performed according to the manufacturer’s instructions.The PCR product waspurified and cloned into pMD18-T vector for sequencing. DNAman 4.0(Lynnon Biosoft,Quebec,Canada),AN-THEPROT[version5.0;18],and NCBI web were used to analysis the DNA and protein sequences.Northern Blot analysisFor RNA gel-blot analysis,20l g of total RNA were sep-arated on 1.2%agarose gels containing formaldehyde. RNA gel blot analysis was performed according to the DIG Northern Starter Kit Manual(Roche,Penzberg,Germany). 30UTR fragment of CmS-AIV1cDNA(about300bp)was labeled with digoxygenin and used as the probe.RNA transfer andfixation were performed by capillary transfer with209SSC overnight and baking membrane at80°C for2h.Prehybridization was done at68°C in DIG Easy Hyb Buffer for30min with gentle agitation.Hybridization was carried out in the same buffer overnight at68°C.The blot was washed twice with29SSC and0.1%SDS at15–25°C under constant agitation for5min.Then the blot was washed twice in0.19SSC and0.1%SDS at68°C under constant agitation for15min.The hybridized probes are immunodetected with anti-digoxigenin-AP,Fab fragments and then visualized with the chemiluminescence substrate CDP-Star.Enzymatic dephosphorylation of CDP-Star by alkaline phosphatase leads to a light emission at a maxi-mum wavelength of465nm which is recorded on X-ray films.Exposure time was30min.Sucrose content measurementSucrose was extracted by grindingflesh tissues(10g fresh weight)in80%ethanol,adjusted to pH7.0with0.1N NaOH,and heated for5min at80°C.The extraction was repeated three times andfive fruit were used for each replicate.The extract corresponding to0.5g fresh weight was dried in vacuo and redissolved in water.An aliquot (10l l)of the solubilized material was analyzed by high performance liquid chromatography(Shimadzu Corp.)on a Sio2–NH2Lichrosorb column equipped with a RID–10A detector and a SCL-10AVP data acquisition system.Soluble acid invertase extraction and assayS-AIV extraction and assay were performed in a manner similar to that previously described by Lowell et al.[19] and Hubbard et al.[9]with some minor modifications. S-AIV was extracted from about1g of minced frozen muskmelon tissue by homogenizing for1min on ice in 200mM Hepes/NaOH(pH7.5),5mM MgCl2,1mM EDTA,0.5mg ml-1of BSA,0.05%Triton X-100 (Sigma-Aldrich Corp.,St.Louis,MO),1M NaCl,1% insoluble PVP,and5mM DTT.Homogenates were quickly desalted at4°C by centrifuging through a5-ml Sephadex G-50spin column[20].Desalted buffer con-tained20mM Hepes/NaOH(pH7.5),0.25mM MgCl2, 0.01%2-Mercaptoethanol,1mM EDTA,0.05%BSA, 0.2%glycerine.Desalted extract was assayed immediately.S-AIV was assayed in50mM Na-citrate buffer(pH5.0), using50mM sucrose as a substrate.Control was boiled for 10min immediately after the addition of the desalted enzyme extract.The reactions were incubated for30min at37°C and was terminated by addition of1ml3,5-dinitrosalicylic reagent containing0.01%(W/V)3,5-dinitrosalicylic acid, 1.6%(W/V)NaOH and30%(W/V)sodium potassium tart-arate[21].The reaction mixture was boiled for10min and the amounts of reducing sugars were measured with a microplate reader(3550-UV Bio-Rad Lab Inc.,Hercules,CA)at570nm and compared to glucose standards.ResultsCloning and characterization of CmS-AIV1To isolate S-AIV cDNA from muskmelon,wefirst designed a pair of degenerated primers according to the conserved domain of S-AIV amino acid sequences from other plant species.A1038bp cDNA fragment was amplified by RT-PCR.A900bp and a800bp cDNA fragments were pro-duced by50RACE and30RACE,respectively.After sequencing confirmation,a2178bp cDNA clone including a full-length coding region was isolated from muskmelon and named CmS-AIV1(GenBank accession EU260044).The deduced amino acid sequence of CmS-AIV1contains636 amino acid residues with a predicted signal sequence cleavage site between residue47and residue48.The protein domain includes the amino acid residues of b-fructosidase motif(NDPNG)and the catalytic domain conserved in vacuolar invertase(MWECVD).Therefore,based on the features of the deduced polypeptide,we conclude that CmS-AIV1encodes a vacuolar acid invertase.The alignment analysis showed that CmS-AIV1shared overall amino acid identities of99.53%,95.15%,85.71%,68.95%and61.50% to tomato(Lycoperscicon esculentum L.),potato(Solanum tuberosum L.)pepper(Capsicum annuum L.),sweet potato (Ipomoea batatas L.)and carrot(Daucus carota L.)soluble acid invertase,respectively(Fig.1).CmS-AIV1differentially expressed in different muskmelon tissuesTo determine the pattern of CmS-AIV1expression in dif-ferent muskmelon organs,a northern blot hybridization analysis was performed.The result showed that CmS-AIV1 transcripts were easily detected in theflowers and fruit,butcouldn’t be detected at all in roots,stems and leaves (Fig.2),suggesting that CmS-AIV1might play a role in flower and fruit development in muskmelon.Regulation of CmS-AIV1mRNA abundance during fruit development and ripeningTo further investigate the developmental regulation of CmS-AIV1in fruit mesocarp tissues,an RNA blot analysiswas carried out using different mesocarp tissues from 5DAP to ripening.The result showed that CmS-AIV1mRNA showed its maximum level at 10DAP,then decreased gradually during fruit development until its minimum level at mature fruit,indicating that CmS-AIV1may be involved in sucrose metabolism in muskmelon fruit (Fig.3).Sucrose content and S-AIV activity during fruit development and ripeningTo demonstrate the functions of CmS-AIV1in regulating fruit quality,sucrose content and S-AIV activitywereFig.1Alignment of predicted amino acid sequences of S-AIV genes from different plants.The GenBank accession numbers for the plant S-AIV sequences are:Cucumis melo ,(had been submitted);Lycoperscicon esculentum ,CAA78060;Solanum tuberosum ,ABF18956;Capsicum annuum ,AAB48484;Ipomoea batatas ,AAK71504;Daucus carota ,CAA53098.This alignment was produced using DNAman 4.0program.Conserved residues are shaded in black.Dark grey shading indicates similarresidues in five out of six of the sequences and clear grey shading indicates similarresidues in three out of six of the sequences.Amino acid residues of b -fructosidase motif (NDPNG)and the catalytic domain conserved in vacuolar invertase (MWECVD)are boxedMatureFruitRoot Stem Leaf FlowerCmS-AIV1rRNAFig.2RNA blot analysis of CmS-AIV1spatial expression in muskmelon plants.About 20l g of total RNA from root,stem,leaf,flower and mature fruit of muskmelon inbred line M01-3respectively,were separated on 1.2%agarose gels containing formaldehyde.Root,stem,leaf and flower tissues were harvested simultaneously from five 7-to 8-week-old plants and mature fruit were harvested as they mature from five 11-to 12-week-old plants.The blot was hybridized with a CmS-AIV1-specific probe.The ethidium bromide-stained rRNA band in the agarose gel is shown as a loading controlCmS-AIV1rRNA5DAP 10DAP 15DAP 20DAP 25DAP Mature fruitFig.3RNA blot analysis of CmS-AIV1expression in developmental muskmelon fruit.About 20l g of total RNA from muskmelon inbred line M01-3fruit of 5,10,15,20,25DAP and mature fruit respectively,were separated on 1.2%agarose gels containing formaldehyde.Five fruit of each stage were pooled to isolate RNA.The blot was hybridized with a CmS-AIV1-specific probe.The ethidium bromide-stained rRNA band in the agarose gel is shown as a loading controlanalyzed.The results showed that very low concentration of sucrose was detected in young and unripe muskmelons. However,a rapid accumulation of sucrose in fruit was observed between20and30DAP(Fig.4a).In contrast to sucrose content,S-AIV activity showed very high levels in fruit before20DAP,but a rapid decrease between20and 30DAP during fruit development(Fig.4b),indicating an essential role of S-AIV in sucrose metabolism in musk-melon fruit.DiscussionSoluble acid invertase(S-AIV)has important biological functions relating to sucrose import[22–24],and sugar signals[25–27]particularly during the expansion growth of diverse sink structures[22–24,26,28–30].These roles for S-AIV are additional to previously recognized functions including turgor regulation and the control of sugar balance in fruit tissues and mature tubers[23,26,31].The sucrose import and potential signaling influences of S-AIV arise from their role in the primary path of cleavage for sucrose entering growing/expanding sink tissues.The transfer of sucrose to vacuoles for initial metabolism has a dual advantage in,first,giving a two-for-one return on the cost of transporting osmotically active solutes into the vacuole for expansion and,second,adjusting organ expansion relative to carbohydrate supply.Such contributions by S-AIV depend,however,on continued capacity for cell wall expansion[32].Sturm et al.[33]reported that S-AIV had two isoforms in carrot,sI and sII.The expression of the genes for sI and sII seemed to be under spatial and temporal control.The sI gene is expressed in primary root and leaves at the early growth stage,and sII is expressed prominently up to the middle stage of tap root enlargement,indicating their involvement in the partitioning,utilization and storage of sucrose.In this study,on the one hand,we demonstrated that CmS-AIV1was expressed inflower and fruit but not in root,stem and leaf tissues,implying that CmS-AIV1may be also involved in the partitioning,utilization and storage of sucrose in muskmelon.On the other hand,we showed that CmS-AIV1transcript level was high in the young fruit,then decrease gradually during fruit development,suggesting that CmS-AIV1may be linked to the cell division and dif-ferentiation during early fruit development.The availability of the complete plant genomes of rice and Arabidopsis allowed the identification of two genes encoding soluble acid invertase in rice and Arabidopsis[16].Two cDNAs of PsS-AIV1and PsS-AIV2isoforms were cloned from Japanese pear fruit[34].In this study,we cloned and characterized one S-AIV gene from muskmelon, CmS-AIV1.However,it is not clear how many S-AIV genes exist in muskmelon.We are constructing cDNA libraries from muskmelon fruit and trying to isolate other S-AIV gene family members involved in sucrose metabolism in muskmelon by screening the cDNA libraries.The inverse relationship between S-AIV activity and sucrose concentration has been reported in muskmelon and other fruit[9,28,35–37]as well as in other sink tissues such as carrot root[23]and tuber of potato[38].Recently, S-AIV-encoding genes have been isolated and character-ized from some higher plant species[12,35,39–41].To date,however,there have been only a few studies of S-AIV gene expression in relation to fruit tissues.Davies and Robinson[11]reported that transcripts of GIN1and GIN2, which are isogenes of S-AIV in grape berry,are expressed actively before the veraison stage and then decrease duringfruit maturation.In Japanese pear fruit(Cyrus pyrifolia Nakai cv.‘Hosui’),the levels of PsS-AIV1transcript had a maximum level at34days after full bloom and decreased rapidly during fruit development[34].Transgenic tomato containing the antisense gene of vacuolar invertase accu-mulates more sucrose than the control tomato during fruit maturation,implying that vacuolar invertase has an important role in determining sucrose content in fruit[28]. Our present results showed that the CmS-AIV1transcripts showed its maximum level at10DAP fruit,then decreased gradually during fruit development until its minimum level at mature fruit(Fig.3).This pattern of CmS-AIV1 expression in mesocarp tissues is in accordance with the alteration of S-AIV activity,but in contrast to the sucrose accumulation during fruit development of muskmelon (Fig.4).Taken together,these data led us to conclude that the sucrose content in plant sink organs may be regulated by the level of S-AIV expression.Therefore,decreasing S-AIV expression might be an important regulatory event of sweetening during melon fruit ripening.Currently we are suppressing CmS-AIV1expression by the antisense approach,which will help reveal the potential application of CmS-AIV1in controlling the quality of muskmelon fruit. Acknowledgments We thank professor Chengchao Zheng(state key laboratory of crop biology in Shandong agricultural university in china)for critical reading of the manuscript and for constructive criticism.This work was supported by the National Natural Sciences Foundation(No.30471191),Provincial Breeding Project of Shandong in China.References1.McCollum TG,Huber DJ,Cantliffe DJ(1988)Soluble sugaraccumulation and activity of related enzymes during muskmelon fruit development.J Amer Soc Hort Sci113:399–4032.Moriguchi T,Abe K,Sanada T(1992)Levels and role of sucrosesynthase,sucrose-phosphate synthase,and acid invertase in sucrose accumulation in fruit of Asian pear.J Amer Soc Hort Sci 117:274–2783.Sakalo VD,Kurchii VM(2004)Hormonal control of sucrosephosphate synthase and sucrose synthase in sugar beet.Russ J Plant Physiol51:183–1884.Lester GE,Dunlap JR(1985)Physiological changes duringdevelopment and ripening of‘Perlita’muskmelon fruits.Sci Hortic26:323–3315.Geromel C,Ferreira LP,Guerreiro SMC et al(2006)Biochemi-cal and genomic analysis of sucrose metabolism during coffee (Coffea arabica)fruit development.J Exp Bot57:3243–3258 6.Lingle SE,Dunlap JR(1987)Sucrose metabolism in nettedmuskmelon fruit during development.Plant Physiol84:386–389 7.Schaffer AA,Aloni B,Fogelman E(1987)Sucrose metabolismand accumulation in developing fruit of Cucumis.Phytochemistry 26:1883–18878.Winter H,Huber SC(2000)Regulation of sucrose metabolism inhigher plants:localization and regulation of activity of key enzymes.J Crit Rev Biochem Mol Biol35:253–2899.Hubbard NL,Huber SC,Pharr DM(1989)Sucrose phosphatesynthase and acid invertase as determinants of sucrose concen-tration in developing muskmelon(Cucumis melo L.)fruits.Plant Physiol91:1527–153410.Yu XY,Wang XF,Fan JD et al(2007)Cloning and character-ization of a sucrose phosphate synthase-encoding gene from muskmelon.J Amer Soc Hort Sci132:557–56211.Davies C,Robinson SP(1996)Sugar accumulation in grapeberries.Cloning of two putative vacuolar invertase cDNAs and their expression in grapevine tissues.Plant Physiol111: 275–28312.Elliott KJ,Buttler WO,Dickinson CD et al(1993)Isolation andcharacterization of fruit vacuolar invertase genes from two tomato species and temporal differences in mRNA levels during fruit ripening.Plant Mol Biol21:515–52413.Klann E,Yelle S,Bennett AB(1992)Tomato fruit acid invertasecomplementary DNA.Plant Physiol99:351–35314.Haouazine-Takvorian N,Tymowska-Lalanne Z,Takvorian Aet al(1997)Characterization of two members of the Arabidopsis thaliana gene family,At b–fruct3and At b–fruct4,coding fro vacuolar invertases.Gene197:239–25115.Kim JY,Mahe A,Brangeon J et al(2000)A maize vacuolarinvertase,IVR2,is induced by water an/tissue speci-ficity and diurnal modulation of expression.Plant Physiol 124:71–8416.Ji XM,Ende WV,Laere AV et al(2005)Structure,evolution,and expression of the two invertase gene families of rice.J Mol Evol60:615–63417.Sambrook J,Fritsch EF,Maniatis T(1989)Molecular cloning:alaboratory manual.Cold Spring Harbor Laboratory Press, New York18.Deleage G,Combet C,Blanchet C et al(2001)ANTHEPROT:anintegrated protein sequence analysis software with client/server put Biol Med31:259–26719.Lowell CA,Tomlinson PT,Koch KE(1989)Sucrose metabo-lizing enzymes in transport tissues and adjacent sink structures in developing citrus fruit.Plant Physiol90:1394–140220.Helmerhorst E,Stokes GB(1980)Microcentrifuge desalting:arapid,quantitative method for desalting small amounts of protein.Anal Biochem104:130–13521.Fahrendorf T,Beck E(1990)Cytosolic and cell wall bound acidinvertase from leaves of Urtica dioica L.:a comparison.Planta 180:237–24422.Andersen MN,Asch F,Wu Y et al(2002)Soluble invertaseexpression is an early target of drought stress during the critical, abortion-sensitive phase of young ovary development in maize.Plant Physiol130:591–60423.Tang G-O,Lu¨scher M,Sturm A(1999)Antisense repression ofvacuolar and cell wall invertase in transgenic carrot alters early plant development and sucrose partitioning.Plant Cell11: 177–18924.Wachter R,Langhans M,Aloni R et al(2003)Vascularization,high-volume solutionflow,and localized roles for enzymes of sucrose metabolism during tumorigenesis by Agrobacterium tumefaciens.Plant Physiol133:1024–103725.Herbers K,Sonnewald U(1998)Altered gene expression broughtabout by inter-and intracellularly formed hexoses and its possible implications for plant–pathogen interactions.J Plant Res 111:323–32826.Koch KE,Zeng Y(2002)Molecular approaches to altered Cpartitioning:genes for sucrose metabolism.J Amer Soc Hort Sci 127:474–48327.Sonnewald U,Brauer M,Schaewen A von et al(1991)Transgenictobacco plants expressing yeast-derived invertase in either the cytosol,vacuole or apoplast:a powerful tool for studying sucrose metabolism and sink/source interactions.Plant J1:95–10628.Klann EM,Hall B,Bennett AB(1996)Antisense acid invertase(TIV1)gene alters soluble sugar composition and size in trans-genic tomato fruit.Plant Physiol112:1321–133029.Tymowska Z,Kreis M(1998)The plant invertases:physiology,biochemistry and molecular biology.Adv Bot Res28:71–117 30.Weschke W,Panitz R,Gubatz S et al(2003)The role of inver-tases and hexose transporters in controlling sugar ratios in maternal andfilial tissues of barley caryopses during early development.Plant J33:395–41131.Greiner S,Rausch T,Sonnewald U et al(1999)Ectopic expres-sion of a tobacco invertase inhibitor homolog prevents cold-induced sweetening of potato tubers Nat Biotechnol17:708–711 32.Koch K(2004)Sucrose metabolism:regulatory mechanisms andpivotal roles in sugar sensing and plant development.Curr Opin Plant Biol7:235–24633.Sturm A,Sebkove V,Lorenz K et al(1995)Development andorgan-specific expression of the genes for sucrose synthase and three isoenzymes of acid b-fructofuranosidase in carrot.Planta 195:601–61034.Yamada K,Kojima T,Bantog N et al(2007)Cloning of twoisoforms of soluble acid invertase of Japanese pear and their expression during fruit development.J Plant Physiol164: 746–75535.Hubbard NL,Pharr DM,Huber SC(1991)Sucrose phosphatesynthase and other sucrose metabolizing enzymes in fruits of various species.Physiol Plant82:191–19636.Miron D,Schaffer AA(1991)Sucrose phosphate synthase,sucrose synthase,and invertase activities in developing fruit of Lycopersicon esculentum Mill.and sucrose accumulating Lyco-persicon hirsutum Humb.and Bonpl.Plant Physiol95:623–627 37.Yamaki S,Moriguchi T(1989)Seasonalfluctuation of sorbitolrelated enzymes and invertase activities accompanying maturatio of Japanese pear(Pyrus serotina Rehder var.culta Rehder)fruit.J Ipn Soc Hortic Sci57:602–60738.Zrenner R,Schuler K,Sonnewald U(1996)Soluble acid inver-tase determines the hexose to sucrose ratio in cold stored potato tubers.Planta198:246–25239.Gonza´lez M-C,Cejudo FJ(2007)Gibberellin-regulated expres-sion of neutral and vacuolar invertase genes in petioles of sugar beet plants.Plant Sci172:839–84640.Gonza´lez M-C,Roitsch T,Cejudo FJ(2005)Circadian anddevelopmental regulation of vacuolar invertase expression in petioles of sugar beet plants.Planta22:386–39541.Nagaraj VJ,Galati V,Lu¨scher M et al(2005)Cloning andfunctional characterization of a cDNA encoding barley soluble acid invertase(HvINV1).Plant Sci168:249–258。